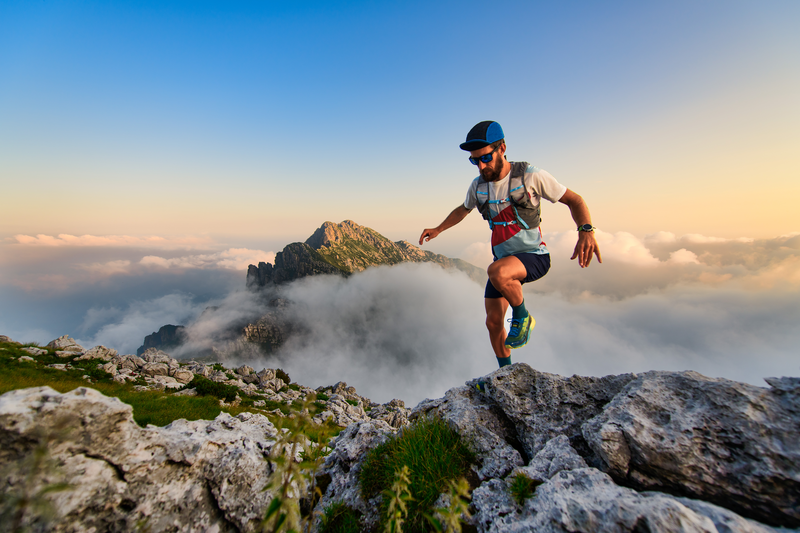
95% of researchers rate our articles as excellent or good
Learn more about the work of our research integrity team to safeguard the quality of each article we publish.
Find out more
ORIGINAL RESEARCH article
Front. Sustain. Food Syst. , 27 May 2024
Sec. Waste Management in Agroecosystems
Volume 8 - 2024 | https://doi.org/10.3389/fsufs.2024.1392445
This article is part of the Research Topic RAMIRAN 2023: Managing Organic Resources in a Changing Environment View all 15 articles
The accumulation of excess manure and the energy costs of the buildings housing the animals are the most important problems that chicken farms have to face nowadays. The combustion of chicken manure to obtain thermal energy for animal holdings has been demonstrated through the research project AVIENERGY, in Spain. To ensure the circularity of the process, the combustion by-product, bottom ash, needs to be recycled and used. This work studies the agricultural use of ash from chicken manure combustion as a fertiliser product and determines its capacity for mineral fertiliser substitution. Ashes from co-combustion of chicken (broiler) manure with wood at different proportions were chemically analysed to determine their nutrient concentrations and availabilities. Then, one ash sample was used as a fertiliser for lettuce cultivation in a pot experiment. Different application rates were tested according to the phosphorus (P) content of the ash, being equivalent to 50, 65, 80 and 100% of the standard inorganic P fertilisation. The results indicate that the ash from combustion of chicken manure meets the requirements for inclusion in the fertiliser component material category ‘CMC 13’, and can be used for the preparation of fertiliser ‘PFC 1(C)(I)(a)(ii): Compound Solid Inorganic Macronutrient Fertiliser (multi-nutrients)’, and also possesses characteristics of “PFC 2: Liming Material” according to the EU legislation for fertiliser products. Although plant production was slightly reduced with ash fertilisation, the ashes reached a fertiliser capacity able to substitute 80% of the mineral P fertiliser, with a liming capacity of 30%. Hence, the ash from chicken manure combustion could be applied to soil, especially if acidic, in partial substitution of mineral fertilisers, to supply P.
The poultry sector is the largest meat producer in the world, with more than 127 mT/y, followed by pork with more than 120 mT/y (FaoStat, 2021). However, in Europe the largest meat production is of pork (30.8 mT/y), followed by poultry (21.5 mT/y), which has experienced the greatest growth in recent years, due to the increase in demand for chicken and turkey meat as well as eggs (FaoStat, 2021). This has triggered the problems associated with the accumulation of animal manure in farms, which entails a serious environmental and economic challenge for the farms. As a consequence, poultry farmers are increasingly forced to optimise manure treatment practices based on circular economy criteria. Poor manure management practices can trigger harmful effects on the environment (contamination of soils and aquifers, and gas emissions; Burton and Turner, 2003). Furthermore, feed production is considered the main factor responsible for greenhouse gas (GHG) emissions in the poultry meat sector, together with changes in land use, energy consumption and manure management and storage (Zisis et al., 2023). Manure management, therefore, represents one of the most relevant problems in the poultry sector nowadays. Energy use is also an important source of GHG emissions in poultry production, both for eggs and meat (Zisis et al., 2023), as it is required for ventilation, feeding, lighting, egg collection, sorting, heating and operation of the mechanical equipment.
The direct application to agricultural soils is the most frequent use of animal manure, which, when well managed, is a correct way to recycle organic matter and nutrients in the soil–plant system. The use of organic wastes, such as manure, as a source of organic matter for soils acts on the soil–plant system, both directly stimulating plant development and improving plant nutrition. It also indirectly increases the water retention capacity and decreases the risk of erosion of the soil. However, without adequate management, pollution and environmental impact problems may occur (e.g., salinity, nutrient imbalances, dispersion of pathogens, odour and gas emissions, etc.; Burton and Turner, 2003). Animal manure is not considered an equilibrated fertilising material in terms of nutrient composition, and excess P can be applied to the soil when poultry manure is applied based on crop N requirements (Bolan et al., 2010). To address all these issues, the Spanish Operational Group AVIENERGY aimed to enable an efficient use of manure generated in poultry farming, through the implementation of a thermochemical combustion process for the production of thermal energy on farms. The objective was to improve the competitiveness and reduce the environmental impact of the poultry sector in areas with high livestock loads, where manure production exceeds greatly the capacity of the agricultural soil for its use. To ensure the circularity of the process, the combustion by-product, bottom ash, needs to be recycled and used.
According to the European List of Wastes (European Commission, 2000, 2014), bottom ash generated from biomass combustion is identified as a solid waste (code 100101). The biomass ash can be used in the construction sector as a cement replacement in mortars (Modolo et al., 2017), and for soil stabilisation, as a structural filler and additive in synthetic aggregates (Gomez-Barea et al., 2009). Also, ash can be used in agriculture as a nutrient source or liming material (James et al., 2012; Silva et al., 2019), or even as a buffer for control of pH in anaerobic digestion (Novais et al., 2018). The application of ash to agricultural soils allows the recycling of essential nutrients in the soil–plant system (Schiemenz and Eichler-Löbermann, 2010; Omil et al., 2011). In addition, the direct application of ashes to soil can be an easy form to supply nutrients, such as P from ash, instead of using more complicated methods such as their extraction with acid solutions (Zeng et al., 2021). However, several concerns have been highlighted regarding the application of combustion ash to agricultural soils, such as the presence of metals, organic contaminants, water soluble salts (that can cause a potential increase in soil salinity) and potentially toxic elements such as chloride (Demeyer et al., 2001; Vassilev et al., 2013; Huygens et al., 2019; Silva et al., 2019).
The European legislation for fertiliser products (EU Regulation, 2009) has recently added the materials from thermal oxidation to the list for component material category CMC 13 for EU fertiliser products (EU Commission, 2021), with specific quality requirements. Ash materials obtained through thermochemical conversion under non-oxygen-limiting conditions from Category 2 of animal by-products, such as animal manure (EU Regulation, 2009), can be included in EU fertilising products. However, the characteristics of the combustion ashes are not homogeneous and depend on the input material and the combustion conditions (Demirbas, 2007).
In the present study, the starting hypothesis was that the ash material remaining after combustion of chicken manure can be a valuable fertiliser material for farmers, to substitute for mineral fertilisers. Then, the objective of this work was to determine the characteristics and the capacity for mineral fertiliser substitution of the bottom ash obtained from the combustion of chicken manure at the farm level, in order to establish its possible use as a fertiliser product.
Ash samples from co-combustion of chicken (broiler) manure with wood chips at different proportions were chemically analysed to determine their nutrient concentrations. Ash samples were obtained directly from the combustion system built in a chicken farm to obtain thermal energy. The burner (50 kW) was adapted to the starting material with a fixed grill, but with a mobile cleaning system to avoid the accumulation of the combustion material at the entry area and to facilitate the correct distribution of the aeration throughout the grill. Mixtures with different proportions of chicken manure and wood chips were burnt in the farm system. The proportions were (by weight): manure at 100%, manure:wood at 50:50 and 60:40 and wood at 100%. The resulting bottom ashes were sampled. An initial ash sample of the 50:50 manure:wood mixture was obtained during the optimisation of the burning system, while the rest of the ash samples were obtained after equipment optimisation. The ash samples were fractionated into three particle sizes: > 4 mm, 2–4 mm and < 2 mm. The >4 mm fraction was discarded due to the presence of impurities, mainly from unburned material. The 2–4 mm fraction was ground to <2 mm and incorporated into the smaller fraction, to obtain a homogeneous sample.
The phytotoxicity of the ashes was determined by a germination test with seeds of Lepidium sativum, using 1:10 (w:v) water extracts and dilutions with distilled water to obtain extract concentrations of 12.5, 25, 50 and 75%, in addition to the undiluted extracts (100%). Ten seeds were placed in a Petri dish (11 cm in diameter) containing filter paper with 2 mL of the corresponding extract or distilled water (control); ten Petri dishes were used for each extract concentration and the control. After 48 h of incubation in the dark at 25°C, the germinated seeds were counted and the root length of each germinated seed measured. The germination index was calculated by multiplying the percentages of root length and germination, both with respect to the control (Zucconi et al., 1981).
Ashes from the 50:50 manure/wood mixtures were used in a pot experiment as a P fertiliser. The selection was based on the difficulties found for the combustion of 100% manure associated to the low bulk density, making the mixture with wood more feasible from a practical point of view. Pots (14.5 cm in diameter) were filled with 1.5 kg of an agricultural soil. The soil was a sandy loam of pH 7.5 with 7.8% CaCO3, electrical conductivity of 0.230 dS m−1, 6.7% organic matter, 3.9 g kg−1 total organic carbon (TOC), 2.03 g kg−1 total-N and 15.8 mg kg−1 available-P. Six treatments were run: non-fertilised control soil, mineral fertiliser and four different application rates of ash, equivalent to 50, 65, 80 and 100% of the phosphorus (P) supplied in the mineral fertiliser treatment; these doses were equivalent to 262, 350, 437 and 525 kg of ash per ha, respectively. The mineral fertiliser treatment comprised a 15:15:15 N:P:K fertiliser and KNO3, equivalent to 130 kg N/ha, 40 kg P2O5/ha and 205 kg K2O/ha (García-Serrano et al., 2010). The ash treatments were complemented with NH4NO3 and KNO3, to achieve the same N and K fertilisation as the mineral fertiliser treatment. Four pots per treatment were prepared and in each pot three plants of lettuce were grown: plantlets of variety Almadraba baby (1 month-old), acquired commercially, were transplanted from germination trays. The experiment was run for 60 days in a growth chamber under controlled conditions of temperature, humidity and light (12 h photoperiod, 25/18°C day/night and 60/70% humidity day/night). The soils were watered at least twice per week using tap water. The above-ground parts of the plants were harvested, weighed fresh, washed with distilled water and dried at 60°C to determine the dry weight. The plant material was then ground to 0.5 mm for chemical analysis. Soil samples were taken from each pot at harvesting, air-dried and sieved to 2 mm for analysis.
From the results for the nutrient concentrations in the plants and their production (plant biomass as dry weight) in each treatment and in the unfertilised control, the efficiency in the use of the nutrients was determined. The apparent recovery efficiency (RE) of P was calculated as Fixen et al. (2015):
Where Uptaketreatment is the plant uptake of P in the treatment ([P] × dry weight), Uptakecontrol is the plant uptake of P in the unfertilised control and Papplied is the amount of P applied (all in g per pot).
The equivalent fertiliser efficiency (EFE) of the ash at the different application rates was calculated as the percentage of the apparent recovery efficiency of the mineral fertiliser.
Where REash is the apparent recovery efficiency of the ash treatment and REfert the apparent recovery efficiency of the mineral fertiliser treatment.
The ash and soil samples were analysed for pH (Crison Basic 20 pH meter) in a water saturated paste for soil and in a 1:5 water extract (w:v) for ash, electrical conductivity (EC) (Crison GLP 31 Conductimeter) in a 1:5 water extract (w:v) and TOC and total nitrogen (also for plants) using an automatic elemental microanalyser (EuroVector elemental analyser, Milan, Italy). The total concentrations of macronutrients, micronutrients and heavy metals were determined by inductively coupled plasma-optical emission spectrometry (ICP-OES; ICAP 6500DUO ONE FAST, Thermo Scientific, Waltham, MA, United States) after microwave (Ethos-1, Milestone, Sorisole, Italy) acid digestion using HNO3 + HCl for ash samples and H2O2 + HNO3 for plant samples. The soluble cations (K, Na, Ca and Mg) and anions (chloride, nitrite, nitrate, phosphates and sulphates) in ash were determined in water extracts (1:10 w:v), by ICP-OES and ion chromatography (IC), respectively. In soils, nitrate was determined using a selective electrode in 1:5 water extracts (USEPA, 2007), available-P was measured colorimetrically in 0.5 M NaHCO3 extracts (Watanabe and Olsen, 1965) and available-K was measured by flame photometry after extraction with 1 N ammonium acetate at pH 7 (Kudsen et al., 1985). Available micronutrients and heavy metals in the soil were analysed by ICP-OES after extraction with 0.005 M DTPA + 0.01 M CaCl2 + 0.1 M triethanolamine (pH 7.3; 1:5 w/v; 1 h) (Lindsay and Norvell, 1978). The availability of P and K in the ash samples was analysed both in water extracts (room temperature and 65°C) and in ammonium citrate extracts (UNE EN 15957). The neutralising capacity of the ashes was determined by titration according to UNE-EN 12945. All results for soil and ash refer to dry matter (105°C, 24 h).
The results were evaluated by one-way ANOVA and the differences between means were determined by the post-hoc Tukey’s test, at a level of significance of p < 0.05. The data were tested for normality using the Shapiro–Wilk test. The statistical software IBM SPSS Statistics version 26 for Windows was used for the statistical analysis.
The ash samples showed high pH and EC values (Table 1), with the initial sample (the one taken during the stabilisation of the combustion system) having the highest EC. The concentrations of TOC and TN were low in all samples, which indicates the loss of organic material during the combustion process; the highest values were again observed in the initial sample, while there were no significant differences among the rest of the samples. Contrastingly, the concentration of nitrate (NO3-N), which is directly available to plants, was lower in the initial sample than in the rest of the ashes. A different situation occurred with the concentration of total P, which was highest in the initial sample. Despite the ash from 100% manure was the second richest in total-P, it showed the highest concentration of soluble phosphates (> 330 mg kg−1), which was unexpected and possibly a consequence of the peculiar characteristics of that particular manure sample. Generally, P showed rather low solubility in water (below 1.5% of total P), whereas the concentration of available-P (extracted in ammonium citrate) reached 4.15% of total-P in the sample from 100% manure, and less than 1% in the rest of the samples (data not shown).
The main plant nutrients that could be provided by the ashes were P (as indicated) and K (Table 1). In contrast to P, almost 50% of total K was water-soluble (at room temperature) and directly available to plants. Potassium solubility was higher in both hot water and ammonium citrate extracts, with values reaching 80.1 > 73.8 > 64.4 ≥ 63.9% of total-K in hot water extracts for ashes from 100% manure, 100% wood and the manure:wood 60:40 and 50:50 mixtures, respectively, and 94.9 > 87.7 > 84.3 > 74.8% of total-K, respectively, in ammonium citrate extracts. The high soluble K and Na concentrations were mainly responsible for the high EC of the ashes. Sodium can be considered a potentially toxic element when it reaches high levels, causing toxicity in plants. However, the concentration of total-K was 7.4 times higher (on average) than that of Na, which may prevent Na toxicity in plants. Furthermore, there is a predominance of soluble K over soluble Na (Table 1), so the risk of Na toxicity is very low, although the high EC may cause phytotoxicity problems due to osmotic stress in plants.
Other main elements present in the ashes include Ca and Mg, with very similar values for all samples, the ranges being 126–146 g kg−1 for Ca and 36–38 g kg−1 for Mg. These elements can contribute significantly to the high pH values of the ashes, and may also be responsible for their high neutralising capacity, mainly regarding those derived from mixtures of chicken manure and wood chips (Figure 1). These data indicate that combustion ash has a CaO substitution capacity of 34% by weight, which can be very relevant for the use of ash in acidic soils, partially replacing the lime required for pH correction.
Figure 1. Neutralising value of the combustion ash from chicken manure (M) and wood (W) mixtures (n = 2). Bars with different letters indicate significant differences between treatments according to the Tukey test at p < 0.05.
The ash materials were rich in essential plant micronutrients (Fe, Cu, Mn and Zn). Small differences in the concentrations of these elements were observed between samples (Table 2); the greatest differences occurred for Zn, with the initial M + W 50:50 sample having the highest value, followed by ash from 100% manure. This may indicate the possible transfer of this element from the metallic part of the burner to ash prepared of the system, but could be associated also with the presence of Zn in the chicken manure. Potentially toxic elements, such as heavy metals and metalloids (As, Cd, Cr, Ni and Pb), were all low, with the only exception of Pb in the initial sample, that reached 133 mg kg−1, again probably due to an early contamination with this element, coming from the burner system during its optimisation period. In agreement with this, the concentration of Pb in the rest of the samples was low. The ash from chicken manure combustion had the lowest concentrations of potentially toxic elements such as Cr, Tl and V (Table 2).
Table 2. Concentrations of micronutrients and potentially toxic elements in the combustion ash from chicken manure (M) and wood (W) mixtures.
The most relevant issue regarding the agricultural use of the combustion ash samples analysed was their high salt content, as shown by their very high EC values. The potential toxicity associated with the salinity of the ashes was determined in a germination test (Figure 2). Water extracts of the ashes, whether pure (100%) or partially diluted (75%), were highly phytotoxic and completely inhibited seed germination (germination index (GI) value of zero). Greater dilution of the extracts (50%) allowed the germination of some seeds, but the GI was still very low (<4%). Further dilution was necessary to obtain an adequate number of germinated seeds and the GI increased as the concentration of the extract decreased: 81 ± 7.8% for a dilution of the extract to 25% and 102 ± 6.9% for a dilution to 12.5%. Then, it was necessary to dilute the extract to 25% to obtain adequate germination without symptoms of toxicity (> 80%). The 12.5% extract may even have been beneficial for germination, probably due to the supply of nutrients from the ashes.
Figure 2. Phytotoxicity tested by the elongation index (EI) and germination index (GI) for the 1:10 water extract of the ash samples and respective dilutions. Average values, standard errors are within the symbol size.
The plant growth was adequate with all ash treatments; it was lowest in the control without fertilisation and highest with the mineral fertiliser, followed by the ash that supplied 100% P (Figure 3). The treatments with ash at 50 to 80% P produced similar plant yields, greater than the control but lower than for the mineral fertiliser treatment. The concentration of N in the plants was highest in the mineral fertiliser and ash at 100% P treatments, being lowest for the control soil without fertilisation; the rest of the treatments with ash gave a N concentration in the plants that was significantly lower than for the mineral fertiliser (Table 3). However, the P concentration in the plants was not significantly different among the different fertilising treatments (with mineral fertiliser or with ash), but all showed higher values than the unfertilised control. These results indicate that the ash can supply enough P to plants for their growth.
Figure 3. Lettuce production as fresh weight of the plants grown per pot (n = 4). Bars with different letters indicate significant differences between treatments according to the Tukey test at p < 0.05.
Table 3. Composition of the plants regarding the main macro- and micro-nutrients and sodium under the different fertiliser treatments.
The concentration of K was highest in the plants receiving the mineral fertiliser and lowest in those grown in the control soil. Ash treated plants showed intermediate K concentrations, with no significant differences among them (Table 3). Contrastingly, Na levels in the plants were not affected by the addition of ash, in agreement with the fact that the total and soluble concentrations of this element were lower than those of K in the ashes (Table 1). The concentrations of the different micronutrients in the plants were all very similar for all treatments—Fe and Zn showed no statistically significant differences, while small differences were observed for Cu and Mn between treatments (Table 3) – and all can be considered normal for plant growth. No accumulation of heavy metals was observed in the plants after the use of combustion ash as a fertiliser substitute (data not shown).
The most relevant changes in the characteristics of the soil were found for the pH values at plant harvest, with a concomitant increase as the ash rate increased, from 7.7 in the control to 8.0 in the treatment with the highest ash rate (Table 4). This effect clearly indicates the usefulness of ash to regulate the pH in acidic soils, as it would decrease the amount of lime required for soil pH adjustment.
Despite the high EC of the ash, this parameter did not increase significantly in the soil (Table 4). Parameters such as the concentrations of TOC and total N (and also nitrate) in the soil showed only very small differences among the treatments, which could be associated with their low concentrations in the ash, and also to the fact that N was supplied at the same rate to all treatments (except the control). The concentration of available P in the soil was increased significantly in the treatment with the highest ash rate, followed by the treatment with the lowest rate of ash and the mineral fertiliser. These results might be showing that the P potentially provided by the ash in excess of the plant requirements was fixed in the soil, due to its calcareous character and the low solubility of this element in the ash, as previously discussed. Furthermore, the concentration of available K in the soil was significantly lower in all the ash treatments than for the mineral fertiliser and the unfertilised control (Table 4), which suggests that the K provided by the ashes and not assimilated by the plants (if any) did not remain in the soil in plant available forms.
Despite the presence of certain heavy metals in the ashes, their available concentrations (extractable with DTPA) in the soil were all quite low (the highest being 2 mg kg−1, for Pb), which indicates a scarce risk of metal accumulation in the soil or plant toxicity. In fact, the total amount of heavy metals added to the soil with the ash at the highest application rate was (mg kg−1): 1.12 Zn, 0.28 Cu and < 0.1 for the rest of heavy metals (Cd, Co, Cr, Ni, Pb), which support the assessment of scarce risk of metal accumulation. These values are far below the limit values for amounts of heavy metals which may be added annually to agricultural land, based on a 10-year average (Cd 0.15, Cu 12, Ni 3, Pb 15 and Zn 30 mg kg−1) according to the European legislation (Council Directive, 1986).
Using the values of the concentration of P in the plants, the plant biomass (the dry weight of the aerial part) and the amount of P added in each treatment, the use of the P applied through fertilisation was calculated as its apparent RE. The apparent RE of P from the mineral fertiliser was 72.8% of the applied P, greater than for the ash treatment at 100% P (61%). The values diminished as the application rate of ash increased, following the order 125 > 69 > 64 ≈ 61% in the ash treatments at 50, 65, 80 and 100% P, respectively. The high P efficiency at the lowest ash rate indicates that the amount of P added was not enough for the plant requirements, and the plants had to take up part of the P already available in the soil.
The fertilisation efficiency of the ash treatments in comparison with the mineral fertiliser indicates the equivalent fertiliser efficiency and therefore the fertiliser replacement value (Figure 2). The ash treatments were able to replace 79% of the mineral P fertiliser when the ash was applied at the same P rate as the mineral fertiliser (ash treatment 100% P), with greater values for 80 and 65% P application by ash (82 and 90% of the mineral P fertiliser, respectively). All this indicates that the ash P fertilising capacity is capable of providing 80% of the P supplied by the mineral fertiliser for the P nutrition of the plants.
The characteristics of combustion ashes depend both on the biomass properties and on the combustion system used (Demirbas, 2007). The chemical reactions that take place during biomass combustion mostly oxidise organic carbon to carbon dioxide and hydrogen to water, while nitrogen is transformed to N2 and emitted to the atmosphere. However, N2O can be formed as a result of the partial oxidation of the NH3 present in animal manures (such as chicken manure), from the partial reduction of NOx by NH3, or by the decomposition of NH4NO3 (Billen et al., 2015). Most of the inorganic components of the biomass will remain in the resulting ash, mainly in the fly ash (Knapp and Insam, 2011). The different characteristics of the initial ash sample taken during the optimisation of the burning system, such as the greater TOC, TN and EC values, as well as the high soluble NO3-N concentrations, clearly indicate a deficiency in the combustion process (Knapp and Insam, 2011). The homogeneous values found for the rest of the samples demonstrate the effective optimisation of the combustion system. The P and K concentrations in the ashes were lower than the average values found in ashes derived from other poultry manures (Lanzerstorfer, 2017; Huygens et al., 2019), but in general were higher than the values obtained for wood. Once the combustion system was optimised, the ash from 100% manure had the highest concentration of P, related to the high concentration of this element in chicken manure (Alvarenga et al., 2020). In addition, the conditions of the combustion system could also affect the concentration of soluble-P in the ash. Combustion of manure with wood could increase the flame temperature due the greater higher heating value (HHV) of wood (19.59 MJ kg−1) than poultry manure (16.18 MJ kg−1) and the increase in the amount of volatile matter in mixtures with wood (Turzyński et al., 2022). These could affect the degree of crystallinity of the ash and P availability, as the lower the fraction of crystalline P-phases was, the higher the bioavailability of the material (Nordin et al., 2020). According to Nordin et al. (2020) the chemical compounds found in bottom ash during combustion depends on the fuel and affected by temperature reached; for instance, hydroxyapatite (Ca5(PO4)3OH) was the more common compound found in bottom ash from woody-type biomass, while AlPO4 survives the combustion of sewage sludge and, on co-combustion with wood, it breaks and reacts with Ca and Mg with the formation of new compounds (Ca and Mg phosphates). These changes in phosphate composition can alter P plant availability in bottom ash and may account, at least in part, for the high soluble phosphate concentrations found in the 100% manure sample ashes. However, P was generally poorly soluble in water, the highest proportion of soluble-P occurred in ash from 100% manure, with 1.38% of total P as water soluble PO43−-P. Other authors have also found a low proportion of water-soluble P in combustion ash (Choudhury et al., 2020). However, the solubility of P from the ashes in ammonium citrate, indicating the plant available P concentration (Bougnom et al., 2011; Omil et al., 2011; Schiemenz et al., 2011), was greater, in agreement with the results obtained for soil available-P in the pot experiment. The abundant basic cations (Ca, Mg and K) present in the ashes were likely responsible for their alkaline pH and their high neutralising capacity (Omil et al., 2011). These elements are transformed into oxides at high temperature, and then hydrated and carbonated during the combustion process (Demeyer et al., 2001). The values found for chicken manure ashes are within the range for wood ash (20–90% CaCO3, equivalent to 11–50.4% CaO) according to Bougnom et al. (2011), which is mainly due to their content of hydroxides and carbonates of calcium, magnesium and K. For this reason, the use of combustion ash has traditionally been considered to be useful in acid and forest soils (Bougnom et al., 2011; Omil et al., 2011).
Although the utilisation of waste materials as fuels can be considered as a positive environmental approach for the production of renewable energy, thereby decreasing the use of fossil fuels, the presence of certain elements can cause problems in the combustion system and may also result in environmental risks after soil application of the ash. Chlorine plays a major role in ash formation as it facilitates the mobility of many inorganic compounds (Demirbas, 2007). Sodium and K lower the melting point of ash and, hence, can increase ash deposition and fouling. In addition, the content of heavy metals and soluble salts in the combustion ash can lead to negative effects in the soil after repeated ash applications. In fact, the high phytotoxicity of the ash was associated with its high salinity (high EC). Consideration should be given to this before its potential agricultural use; its use with young plants or non-germinated seeds should be avoided, and its application to the soil well before seeding or transplanting is recommended.
The EU fertiliser legislation includes, in the component materials category CMC 13, the “thermal oxidation materials and their derivatives” (EU Commission, 2021). However, to protect plants and soils, the regulation limits the total concentrations of potentially toxic elements in combustion ash to Cr < 400, Tl < 2, V < 600 (all in mg kg−1) and Cl− < 30 g kg−1. The ash from chicken manure studied here did not exceed these limit values (Table 2), guaranteeing the safe use of the bottom ash generated from chicken manure as a fertiliser material or as a component material of fertilisers. The characteristics of the ash samples can be compared with the requirements for fertiliser products according to the European legislation (EU Regulation, 2019). These materials can be included in some product functions categories (PFCs) such as “Solid Inorganic Macronutrient Fertiliser” (multi-nutrients) (PFC 1), “Liming Material” (PFC 2) and “Inorganic Soil Improvers” (PFC3) (EU Regulation, 2019). The multi-nutrients option ‘PFC 1(C)(I)(a)(ii): Compound Solid Inorganic Macronutrient Fertiliser (multi-nutrients)’ requires a minimum content of 3% P2O5 plus one of the other considered plant macronutrients, at a concentration above 3% for K2O and above 1.5% for CaO, MgO, SO3, or Na2O. The bottom ash from chicken manure, alone or mixed with wood, of the present experiment, obtained after the optimisation of the combustion system, complied with these nutrient requirements, with average values of (% ± SE): P2O5 = 3.1 ± 0.76; K2O = 8.78 ± 0.63; CaO = 19.83 ± 0.69; MgO = 6.16 ± 0.08; SO3 = 2.11 ± 0.16 and Na2O = 1.66 ± 0.22 (below the maximum value of 40%). The P2O5 concentration of the ash was very close to the limit required; then, the addition of other source of P2O5 may be required to obtain a multi-nutrients option ‘PFC 1(C)(I)(a)(ii). The sum of the nutrients is above the minimum (18%) established in this European legislation (EU Regulation, 2019). The neutralising value of the ash was above the 15% CaO equivalence required for a liming material (PFC 2). In addition, the concentrations of all inorganic contaminants in the ash were below the maximum limit values for PFC 1 (with the exception of the sample taken during system optimisation), but values higher than the limit for PFC 2 and PFC 3 could occur for Cu and Zn. Thus, special attention should be paid to the presence of Cu and Zn in chicken manure destined for combustion, in order to obtain ash useful as a liming material or an inorganic soil improver in line with the European legislation.
The results of the pot experiment indicate that the amount of nutrients supplied to the plants when using ash was enough for their growth. In fact, the application of ash at a rate equivalent to 100% of the P supplied by the mineral fertiliser gave a plant production and P concentration in the plants that were very close to those in the fertiliser treatment. Although the apparent RE for P was slightly lower for ash than for mineral fertiliser, a fertiliser replacement value of 80% can be estimated for the ash used (i.e., the combustion ash can replace 80% of the inorganic P fertiliser). This is highly relevant in terms of reducing the use of P extracted from rock phosphate, which can be considered a strategic resource; in fact, the reserves of P are expected to have fallen by 25% in 2100 based on current demand (Malingreau et al., 2012). The use of ash as a fertiliser material has a clear advantage over that of fresh manure, since (as the N has been removed) it can be applied based on crop P requirements, which would reduce the potential P over-application, P run-off and soil P accumulation that could result from the application of the manure on a N requirement basis for the crop (Choudhury et al., 2020). In spite of the low solubility of the ash P in water, this material is an effective P fertiliser for crops, as indicated by the enhanced extractability of P in ammonium citrate. In addition, this low percentage of water-soluble P could prevent any loss by run-off after soil application (Codling, 2006; Choudhury et al., 2020).
From the results of the present work, the following conclusions can be drawn:
• The ash materials derived from combined chicken manure and wood chips combustion are rich in essential nutrients, mainly P and K. When chicken manure was burnt alone (without wood biomass) the concentration of P in the ashes was highest. Phosphorus was generally poorly soluble in water, while K was highly soluble in warm (65°C) water.
• The combustion ash can replace 80% of the inorganic P fertiliser and its low percentage of water-soluble P could prevent any P-loss by run-off after soil application.
• The high pH value and the elevated concentrations of Ca and Mg give chicken manure ashes high neutralising values of up to 30% of CaO eq., which can be relevant regarding their use as amendments in acid soils in partial substitution of the usual liming materials.
• The high salinity of the ash, with a much greater abundance of K relative to Na, should not cause problems in the development of the crop or in the soil, if applied at agronomic rates based on the crop P requirement. However, phytotoxicity due to salinity may occur, which implies that agricultural use is not recommended for non-germinated seeds or young plants; ash should be applied to soils at low application rates and well before sowing.
• The ash from combustion of chicken manure satisfies the requirements for inclusion in the fertiliser component material category CMC 13, with total nutrient concentration close or above the limits established for PFC 1(C)(I)(a)(ii): Compound Solid Inorganic Macronutrient Fertiliser (multi-nutrients), and also has characteristics that allow its inclusion in PFC 2: liming material.
• However, the combustion process must be efficient enough to reduce the TOC concentration in ash to below 3%, while the Cu and Zn in the input manure need to be controlled so that their concentrations in the ash do not exceed the limits for liming materials and soil improvers.
The original contributions presented in the study are included in the article/supplementary material, further inquiries can be directed to the corresponding author.
MPB: Conceptualization, Data curation, Funding acquisition, Investigation, Methodology, Supervision, Visualization, Writing – original draft, Writing – review & editing. MÁ-R: Data curation, Formal analysis, Investigation, Methodology, Writing – original draft. PB-M: Data curation, Formal analysis, Investigation, Methodology, Writing – original draft. RC: Conceptualization, Data curation, Funding acquisition, Investigation, Methodology, Supervision, Writing – review & editing.
The author(s) declare financial support was received for the research, authorship, and/or publication of this article. The Project AVIENERGY has been co-financed by the European Agricultural Fund for Rural Development (EAFRD, FEADER) of the European Union (80%) and by the Spanish Ministry of Agriculture, Fisheries and Food (20%), within the framework of the National Rural Development Program 2014–2020 (ref. O00000226e2000044442). Budget: €582,960.04. Total grant: €565,160.04. The General Directorate for Rural Development, Innovation and Agri-Food Training (DGDRIFA) is the authority for applying the grants.
The authors declare that the research was conducted in the absence of any commercial or financial relationships that could be construed as a potential conflict of interest.
The author(s) declared that they were an editorial board member of Frontiers, at the time of submission. This had no impact on the peer review process and the final decision.
All claims expressed in this article are solely those of the authors and do not necessarily represent those of their affiliated organizations, or those of the publisher, the editors and the reviewers. Any product that may be evaluated in this article, or claim that may be made by its manufacturer, is not guaranteed or endorsed by the publisher.
Alvarenga, P., Carneiro, J. P., Fangueiro, D., Cordovil, C. M. S., and Bernal, M. P. (2020). “Managing organic amendments in agroecosystems to enhance soil carbon storage and mitigate climate change” in Climate change and soil interactions (Ed. M.N.V. Prasad: Elsevier), 89–141. doi: 10.1016/B978-0-12-818032-7.00005-9
Billen, P., Costa, J., van der Aa, L., van Caneghem, J., and Vandecasteele, C. (2015). Electricity from poultry manure: a cleaner alternative to direct land application. J. Clean. Prod. 96, 467–475. doi: 10.1016/j.jclepro.2014.04.016
Bolan, N. S., Szogi, A. A., Chuasavathi, T., Seshadri, B., Rothrock, M. J. Jr., and Panneerselvam, P. (2010). Uses and management of poultry litter. World's Poultry Sci. J. 66, 673–698. doi: 10.1017/S0043933910000656
Bougnom, B. P., Knapp, B. A., Etoa, F. X., and Insam, H. (2011). “Possible use of wood ash and compost for improving acid tropical soils” in Recycling of biomass ashes. eds. H. Insam and B. A. Knapp (Berlin Heidelberg: Springer-Verlag), 87–106.
Burton, C. H., and Turner, C. (2003). Manure Management. Bedford: Treatment Strategies for Sustainable Agriculture, Silsoe Research Institute.
Choudhury, A., Felton, G., Moyle, J., and Lansing, S. (2020). Fluidized bed combustion of poultry litter at farm-scale: environmental impacts using a life cycle approach. J. Clean. Prod. 276:124231. doi: 10.1016/j.jclepro.2020.124231
Codling, E. E. (2006). Laboratory characterization of extractable phosphorus in poultry litter and poultry litter ash. Soil Sci. 171, 858–864. doi: 10.1097/01.ss.0000228059.38581.97
Council Directive (1986). Council directive of 12 June 1986 on the protection of the environment, and in particular of the soil, when sewage sludge is used in agriculture (86/278/EEC). Of. J. Eur. Comm. No L 181, 6–12,
Demeyer, A., Voundi Nkana, J. C., and Verloo, M. G. (2001). Characteristics of wood ash and influence on soil properties and nutrient uptake: an overview. Bioresour. Technol. 77, 287–295. doi: 10.1016/S0960-8524(00)00043-2
Demirbas, A. (2007). Combustion of biomass. Energy Sources A: Recovery Util. Environ. Eff. 29, 549–561. doi: 10.1080/009083190957694
EU Commission. (2021). Commission delegated regulation amending annexes II, III and IV to regulation (EU) 2019/1009 of the European Parliament and of the council for the purpose of adding thermal oxidation materials and derivates as a component material category in EU fertilising products. Brussels, 6.7.2021. C(2021) 4751 final.
European Commission (2000). Commission decision (2000/532/EC) of 3 may 2000 replacing decision 94/3/EC establishing a list of wastes pursuant to article 1(a) of council directive 75/442/EEC on waste and council decision 94/904/EC establishing a list of hazardous waste pursuant to article 1(4) of council directive 91/689/EEC on hazardous waste. Off. J. Eur. Communities 226, 3–6.
European Commission (2014). Commission Decision of 18 December 2014 Amending decision 2000/532/EC on the list of waste pursuant to directive 2008/98/EC of the European Parliament and of the council. Offic. J. Europ. Union L 370, 44–86,
FaoStat. (2021). Available at: https://www.fao.org/statistics/es (Accessed May 15, 2024).
Fixen, P., Brentrup, F., Bruulsema, T. W., Garcia, F., Norton, R., and Zingore, S. (2015). “Nutrient/fertilizer use efficiency: measurement, current situation and trends” in Managing water and fertilizer for sustainable agricultural intensification. eds. P. Drechsel, P. Heffer, H. Magen, R. Mikkelsen, and D. Wichelns. First ed (Paris, France: International fertilizer industry association (IFA), international water management institute (IWMI), International Plant Nutrition Institute (IPNI), and international potash institute (IPI)), 8–38.
García-Serrano, P., Lucena Marotta, J. J., Ruano Criado, S., and Nogales García, M. (2010). Guía práctica de la fertilización racional de los cultivos en España, part. I. Edn. Madrid: Ministerio de Medio Ambiente y Medio Rural y Marino. Secretaría General Técnica. Centro de Publicaciones.
Gomez-Barea, A., Vilches, L., Campoy, M., and Fernandez-Pereira, C. (2009). Plant optimization and ash recycling in fluidised waste gasification. Chem. Eng. J. 146, 227–236. doi: 10.1016/j.cej.2008.05.039
Huygens, D., Saveyn, H. G. M., Tonini, D., Eder, P., and Delgado, S. L. (2019). Technical proposals for selected new fertilising materials under the Fertilising products regulation (regulation (EU) 2019/1009)—process and quality criteria, and assessment of environmental and market impacts for precipitated phosphate salts & derivates, thermal oxidation materials & derivates and pyrolysis & gasification materials, EUR 29841 EN. Luxembourg: Publications Office of the European Union. doi: 10.2760/186684
James, A. K., Thring, R. W., Helle, S., and Ghuman, H. S. (2012). Ash management review—applications of biomass bottom ash. Energies 5, 3856–3873. doi: 10.3390/en5103856
Knapp, B. A., and Insam, H. (2011). “Recycling of biomass ashes: current technologies and future research needs” in Recycling of biomass ashes. eds. H. Insam and B. A. Knapp (Berlin Heidelberg: Springer-Verlag), 1–16.
Kudsen, D., Peterson, G. A., and Pratt, P. F. (1985). “Lithium, sodium, and potassium” in Methods of Soil Analysis. Part 2 Chemical and Microbiological Properties, 2nd Ed. Agronomy series. eds. A. L. Page, R. H. Miller, and D. R. Keeney (Madison, Wisconsin: American Society of Agronomy, Inc., Soil Science Society of America, Inc.), 225–246.
Lanzerstorfer, C. (2017). Chemical composition and properties of ashes from combustion plants using Miscanthus as fuel. J. Environ. Sci. 54, 178–183. doi: 10.1016/j.jes.2016.03.032
Lindsay, W. L., and Norvell, W. A. (1978). Development of a DTPA soil test for zinc, iron, manganese, and copper. Soil Sci. Soc. Am. J. 42, 421–428. doi: 10.2136/sssaj1978.03615995004200030009x
Malingreau, J. P., Eva, H., and Maggio, A. M. (2012). NPK: Will there be enough plant nutrients to feed a world of 9 billion in 2050? European Commission: Joint Research Centre, Publications Office.
Modolo, R. C. E., Senff, L., Ferreira, V. M., Tarelho, L. A. C., and Moraes, C. A. M. (2017). Fly ash from biomass combustion as replacement raw material and its influence on the mortars durability. J. Mater. Cycles Waste Manag. 20, 1006–1015. doi: 10.1007/s10163-017-0662-9
Nordin, A., Strandberg, A., Elbashir, S., Åmand, L. E., Skoglund, N., and Pettersson, A. (2020). Co-combustion of municipal sewage sludge and biomass in a grate fired boiler for phosphorus recovery in bottom ash. Energies 13:1708. doi: 10.3390/en13071708
Novais, R. M., Gameiro, T., Carvalheiras, J., Seabra, M. P., Tarelho, L. A. C., Labrincha, J. A., et al. (2018). High pH buffer capacity biomass fly ash-based geopolymer spheres to boost methane yield in anaerobic digestion. J. Clean. Prod. 178, 258–267. doi: 10.1016/j.jclepro.2018.01.033
Omil, B., Sánchez-Rodríguez, F., and Merino, A. (2011). “Effects of ash applications on soil status, nutrition, and growth of Pinus radiata D. Don plantations” in Recycling of biomass ashes. eds. H. Insam and B. A. Knapp (Berlin Heidelberg: Springer-Verlag), 69–86.
EC Regulation. (2009). Laying down health rules as regards animal by-products and derived products not intended for human consumption and repealing regulation (EC) no 1774/2002 (animal by-products regulation). Regulation (EC) No 1069/2009 of the European Parliament and of the Council of 21 October 2009. Regulation (EC) No 1069/2009 of the European Parliament and of the Council of 21 October 2009. (EC) No 1069/2009 and (EC) No 1107/2009 and repealing Regulation (EC) No 2003/2003. Offic. J. Europ. Union, 300, 1–33.
EU Regulation. (2019). Of the European Parliament and of the council of 5 June 2019 laying down rules on the making available on the market of EU fertilising products and amending regulations. (EC) No 1069/2009 and (EC) No 1107/2009 and repealing Regulation (EC) No 2003/2003. Off. J. Eur. Union, 170, 1–114.
Schiemenz, K., and Eichler-Löbermann, B. (2010). Biomass ashes and their phosphorus fertilizing effect on different crops. Nutrient Cycl. Agroecosyst. 87, 471–482. doi: 10.1007/s10705-010-9353-9
Schiemenz, K., Kern, J., Paulsen, H. M., Bachmann, S., and Eichler-Löbermann, B. (2011). “Phosphorus fertilizing effects of biomass ashes” in Recycling of biomass ashes. eds. H. Insam and B. A. Knapp (Berlin Heidelberg: Springer-Verlag), 17–32.
Silva, F. C., Cruz, N. C., Tarelho, L. A. C., and Rodrigues, S. M. (2019). Use of biomass ash-based materials as soil fertilisers: critical review of the existing regulatory framework. J. Clean. Prod. 214, 112–124. doi: 10.1016/j.jclepro.2018.12.268
Turzyński, T., Kluska, J., and Kardaś, D. (2022). Study on chicken manure combustion and heat production in terms of thermal self-sufficiency of a poultry farm. Renew. Ener. 191, 84–91. doi: 10.1016/j.renene.2022.04.034
USEPA (2007). Method 9210A. Potentiometric determination of nitrate in aqueous samples with an ion-selective electrode. Available at: https://www.epa.gov/hw-sw846/sw-846-test-method-9210a-potentiometric-determination-nitrate-aqueous-samples-ion-selective).
Vassilev, S. V., Baxter, D., Andersen, L. K., and Vassileva, C. G. (2013). An overview of the composition and application of biomass ash: Part 1. Phase-mineral and chemical composition and classification. Fuel 105, 40–76. doi: 10.1016/j.fuel.2012.09.041
Watanabe, F. S., and Olsen, S. R. (1965). Test of an ascorbic acid method for determining phosphorus in water and NaHCO3 extracts from soil. Soil Sci. Soc. Am. Proc. 29, 677–678. doi: 10.2136/sssaj1965.03615995002900060025x
Zeng, W., Dehn Wang, D., Wu, Z., He, L., Luo, Z., and Yang, J. (2021). Recovery of nitrogen and phosphorus fertilizer from pig farm biogas slurry and incinerated chicken manure fly ash. Sci. Total Environ. 782:146856. doi: 10.1016/j.scitotenv.2021.146856
Zisis, F., Giamouri, E., Mitsiopoulou, C., Christodoulou, C., Kamilaris, C., Mavrommatis, A., et al. (2023). An overview of poultry greenhouse gas emissions in the Mediterranean area. Sustain. For. 15:1941. doi: 10.3390/su15031941
Keywords: ash nutrients, fertiliser efficiency, mineral fertiliser substitution, neutralising capacity, phosphorus, plant nutrition, recovery efficiency
Citation: Bernal MP, Álvarez-Robles MJ, Bernal-Molina P and Clemente R (2024) Bottom ash from combustion of chicken manure as a fertiliser material. Front. Sustain. Food Syst. 8:1392445. doi: 10.3389/fsufs.2024.1392445
Received: 27 February 2024; Accepted: 10 May 2024;
Published: 27 May 2024.
Edited by:
David Robert Chadwick, Bangor University, United KingdomReviewed by:
Arturo F. Chica, University of Cordoba, SpainCopyright © 2024 Bernal, Álvarez-Robles, Bernal-Molina and Clemente. This is an open-access article distributed under the terms of the Creative Commons Attribution License (CC BY). The use, distribution or reproduction in other forums is permitted, provided the original author(s) and the copyright owner(s) are credited and that the original publication in this journal is cited, in accordance with accepted academic practice. No use, distribution or reproduction is permitted which does not comply with these terms.
*Correspondence: María Pilar Bernal, cGJlcm5hbEBjZWJhcy5jc2ljLmVz
Disclaimer: All claims expressed in this article are solely those of the authors and do not necessarily represent those of their affiliated organizations, or those of the publisher, the editors and the reviewers. Any product that may be evaluated in this article or claim that may be made by its manufacturer is not guaranteed or endorsed by the publisher.
Research integrity at Frontiers
Learn more about the work of our research integrity team to safeguard the quality of each article we publish.