- 1Laboratorio Para Investigaciones Biomédicas, Facultad de Ciencias de la Vida, Escuela Superior Politécnica del Litoral, ESPOL, Guayaquil, Ecuador
- 2Epigenetic Signaling PPES Lab, Department of Biomedical Sciences, University of Antwerp, Antwerp, Belgium
- 3Universidad Regional Amazónica Ikiam, Research Group of Tropical Ecosystems and Global Change (EcoTroCG), Tena, Ecuador
Purpose: Traditional fermented beverages are a valuable cultural, economic, and social heritage of Latin American societies that deserve to be preserved and strengthened with sustainable development and respect for diversity. Therefore, this article will demonstrate, through scientific literature, the importance for indigenous societies and the nutritional contributions of the most traditional beverages of North and South America.
Methods: This systematic review followed the PRISMA (Preferred Reporting Items for Systematic Reviews and Meta-Analysis) verification protocol. The study focused on exploring the nutritional information of South and Central American fermented beverages. The search was conducted in the Biomed, PubMed, ScienceDirect, Scopus, Scielo, and Google Scholar databases to identify publications published within the past 15 years.
Results: However, the presence of toxic compounds such as biogenic amines and phenols that affect the quality of the product and human health has been detected. In addition, it was found that macro-and micronutrients, vitamins, and microorganisms differ according to the type of raw material used. It was concluded that corn is the most widely used raw material to produce fermented beverages in Latin America. At the same time, Mexico has conducted more research on this product, and most analyses are being carried out on the use of corn in the production of fermented beverages. In addition, several studies have shown that using validated and standardized methodologies to detect biogenic weapons is crucial to ensure accurate identification, effective response, and compliance with legal and security requirements in this critical area.
1 Introduction
1.1 General considerations
The production of fermented foods and beverages is one of the oldest food processing technologies known to mankind. Fermentation is probably one of the oldest preservation methods practiced by humans (Caplice and Fitzgerald, 1999), dating back to 10,000 and 15,000 years ago when the way of life changed from food gatherers to food producers (Bintsis and Papademas, 2022). Thousands of fermented foods and beverages, many predating histories, remain unknown outside their areas of origin (Fernandes, 2018). This diversity is due to the heterogeneity of traditions, cultural preferences, geographical areas, and commodities or by-products used for fermentation (Maicas, 2020). Initially, the methods of elaborating these fermented foods probably arose by chance and were transmitted through cultural traditions over time (Elizaquível et al., 2015).
Traditional or indigenous fermented foods are popular products that have been an integral part of the region’s diet since ancient times (El-Gendy, 1983). These foods can be prepared at home and in cottage industries using simple techniques and equipment (Aidoo et al., 2006). These kinds of fermented products are deeply rooted in local culture and customs. Their preparation has been passed down through generations, adapting to each community’s resources and culinary practices (Krause and Fleischer, 2010). The preparation of these products generally does not require complex machinery or infrastructure. On the contrary, they are usually made using artisanal methods, using basic utensils and tools that allow taking advantage of the natural fermentation processes to obtain products with distinctive sensory characteristics and nutritional properties (Jimenez et al., 2022). These techniques of using, preserving, and processing food for medicinal purposes have been an essential pillar in the eating habits and daily life of these communities (Pérez-Armendáriz and Cardoso-Ugarte, 2020), which have been developed and transmitted from generation to generation (Castilla Corzo et al., 2019), which explains that traditional fermented beverages entangle more than just food needs, they are identitarian features, moreover, main plant ingredients are inscribed in mythological stories of the beginning of the times (Jolly, 2007); so, traditional fermented beverage fabrication and consumption embrace symbolic, political and social aspects of daily life and festive events of Latin American societies (Jolly, 2007).
1.2 Fermentation types
In the context of food microbiology at the industrial level, the term “fermentation” encompasses both anaerobic and aerobic processes carried out by microorganisms, which serve the chemical purpose of transforming primary nutrients into secondary substances of commercial interest (Siddiqui et al., 2023). Based on these biochemical transformations, we can distinguish four main types of fermentation: acid, alkaline, alcoholic, and lactic fermentation. Each of these variants involves specific reactions that give rise to end products with distinct characteristics and applications (Wang and Fung, 1996; Navarrete-Bolaños, 2012).
Acetic acid bacteria (AAB) are a group of Gram-negative microorganisms that have the ability to oxidize ethanol and transform it into acetic acid (Gomes, 2018). These bacteria play a fundamental role in acetic fermentation processes, converting alcohol into acetic acid and producing products such as vinegar and kombucha (Yamada et al., 2012). Acetic acid bacteria are characterized as obligate aerobic bacteria, i.e., they require oxygen to carry out their metabolic processes. In addition, their classification as Gram-negative indicates specific characteristics in terms of cell wall structure and composition (Trček and Barja, 2015). Thanks to the oxidative activity of acetic acid bacteria, various fermented products with a distinctive acid profile can be obtained, which have diverse applications in the food and beverage industry (Andrés-Barrao et al., 2013).
Unlike acetic fermentation, the alkaline fermentation process is driven by mixed bacterial cultures, which are mainly dominated by the species Bacillus subtilis (Wang and Fung, 1996; Giuffrè and Giuffrè, 2024). This bacterium, which is classified as Gram-positive, bacillary, and aerobic (Wang and Fung, 1996), plays a fundamental role in the transformation of the nitrogenous compounds present in the initial substrates used for fermentation (Giuffrè and Giuffrè, 2024). Through the metabolic activity of these bacterial consortia, ammonia is released, which leads to an increase in the pH of the system (Ye et al., 2020).
Beyond the acetic and alkaline fermentation processes, there is another fundamental type of fermentation: alcoholic fermentation. This fermentative variant is driven by the activity of yeasts, with the Saccharomyces genus being the predominant one in this type of metabolic transformation (Albergaria and Arneborg, 2016; Zhang et al., 2023). Yeasts of the genus Saccharomyces are eukaryotic microorganisms that can carry out alcoholic fermentation (Albergaria et al., 2010; Walker and Stewart, 2016). Through their metabolic pathways, these yeasts can metabolize sugars present in various substrates, such as grape must or cereals, and convert them into ethanol and carbon dioxide (Fiechter and Seghezzi, 1992). This fermentative process driven by Saccharomyces yeasts is of fundamental importance in producing alcoholic beverages such as beer and wine, where alcoholic fermentation is responsible for forming characteristic bubbles and spongy texture (Albergaria et al., 2010).
In addition, Gram-positive microorganisms belonging to the lactic acid bacteria (LAB) group have been identified that promote BA biosynthesis. These usually occur in raw materials or are included as starter cultures for fermentation (Visciano and Schirone, 2022). The genera Lactococcus, putrescine producers; Enterococcus (Pérez-Martín et al., 2014), putrescine and tyramine producers; Lactobacillus, histamine, putrescine, and tyramine producers; Oenococcus and Pediococcus (Elizaquível et al., 2015), histamine producers; and Streptococcus, tyramine, and histamine producers. Histamine-, putrescine-, cadaverine-, and tyramine-producing bacteria have been identified within the genus Staphylococcus (Martín et al., 2006).
1.3 Traditional fermented beverages in North and South America
With all the above context, the production of these traditional fermented beverages in North and South America in countries such as Ecuador, Argentina, Peru, and Mexico (Elizaquível et al., 2015; Guerra et al., 2022), are generally made from raw materials such as cassava, corn, coconut stalk, pineapple, sugar cane, agave and sweet potato, among others. Some popular examples are pulque, tepache, atole agrio, tuba and chicha (Robalino Vallejo et al., 2020). Natural fermentation of foods has been practiced historically by various cultures. This fermentation process varies according to regional particularities and local customs but generally involves a process that includes soaking, cooking, and fermentation (Elizaquível et al., 2015). Several other traditional beverages, such as Masato, derived from the fermentation of cassava roots, and Cauim, are beverages indigenous Brazilians produce (Chacón Mayorga et al., 2020). In addition, each region presents variations in the recipe, using local ingredients and adapting the process to specific customs. The production of chicha by indigenous societies in Latin America is of utmost importance due to its central role in preserving cultural identity, community cohesion, and expressing ancestral traditions. Chicha, produced mainly by spontaneous fermentation of foods made from maize, was the identity of several ancestral indigenous societies over the centuries before the arrival of European colonizers (Jolly and Philippe, 2007; Emperaire, 2023).
Traditional fermented beverages are characterized by a fermentation process, either natural - spontaneous or induced, involving bacteria, yeasts, and, in some cases, fungi. The microorganisms responsible for this fermentation can come from different sources, such as human saliva, water added to the mixture, the surrounding environment, the utensils used, and the containers used, which could harbor microbial remnants or traces of previous fermentations. Therefore, it is not only a beverage but a culinary art and a tangible manifestation of the cultural identity of Indigenous societies.
According to several authors, many fermented foods result from a prolonged evolutionary process. They consist of raw materials that are naturally inoculated by harmless microorganisms, whose enzymes, such as amylases, proteases, and lipases, break down the polysaccharides, proteins, and lipids, respectively, degrade the complex compounds present; this process results in products with pleasant and attractive flavors, aromas, and textures for human consumption. The lactic acid bacteria responsible for generating the acidification and pH reduction in these products (Narvhus and Axelsson, 2003), within the microbial spectrum, the Lactobacillus genus stands out as the predominant species. Among the most common strains are Lactobacillus Plantarum, Lactobacillus Brevis, Lactobacillus Fermentums, Lactobacillus casei, Lactobacillus Acidophilus, Lactobacillus reuteri, together with Leuconostoc Mesenteroides, Weissella confusa and Weissella. cibaria, all recognized for their ability to provide the typical acidic characteristics (Muñoz and Moreno-Arribas, 2011; Freire et al., 2016; Mendoza et al., 2017).
Research has shown that Lactobacillus plantarum and other microorganisms, such as lactic acid bacteria and yeasts, are mainly responsible for the fermentation of cassava (Ben et al., 2000; Lacerda et al., 2005; Albergaria and Arneborg, 2016; Panda and Ray, 2016). In Brazil, the liquid extracted from the stalk of sugarcane (Saccharum officinarum) is used as a raw material to produce cachaça, a distilled alcoholic beverage with a long tradition in the country. The production process of this aged beverage is based mainly on the spontaneous fermentation of the sugars present in the sugarcane juice, a process in which the yeast Saccharomyces cerevisiae is the predominant microbial species (Badotti et al., 2010).
1.4 Presence of phenolic and biogenic amines compounds
However, many fermented foods contain compounds like phenolic and biogenic amines, which can impact food quality and safety in addition to being refreshing and delicious. One of these groups of compounds is phenolic compounds, which may contain a variety of bioactive compounds that may have health benefits. The presence of phenolic compounds in fruit peels and cereal, mostly in chicha (Grijalva-Vallejos et al., 2021). These are molecules with one or more hydroxyl groups bound to an aromatic ring, where they are classified into two groups: flavonoid and non-flavonoid phenolic compounds. Non-flavonoid compounds range from simple chemical structures such as simple phenols (C6) to more complex structures such as tannins (C6) n (Navarrete-Bolaños, 2012). Fruits, vegetables, roots, and cereals are all sources of phenolic compounds, which are important dietary antioxidants. However, these compounds can also have antinutritional effects if consumed in very high and chronic amounts. This is because they can interact with some aspects of the diet, such as inhibiting iron absorption and potentially causing anemia (Brune et al., 1989).
Phenolic compounds have been the subject of increasing interest due to their potential impact on human health. Numerous studies have suggested they may have antioxidant, anti-inflammatory, and anticarcinogenic properties, among others (Lopez-Martinez et al., 2009; Adebo and Medina-Meza, 2020). These beneficial effects are due to the ability of phenolic compounds to interact with different molecules and biological processes in our body. For example, it has been suggested that phenolic compounds may act as antioxidants, protecting cells from oxidative stress and preventing cell damage (Lao et al., 2017; Muñoz-Bernal et al., 2020). It has also been proposed that these compounds may modulate gene expression and enzyme activity, influencing different metabolic pathways (Cano and Arnao, 2004). Despite the potential health benefits, the possibility of risks associated with excessive consumption of phenolic compounds in traditional beverages has also been raised. Some studies have suggested that high concentrations of phenolic compounds may be toxic to cells and interfere with the absorption of certain nutrients (Lao et al., 2017; Mashau et al., 2021).
Likewise, considerable concentrations of biogenic amines (BA) have been detected in fermented products due to the presence of lactic acid bacteria during this process (Alvarez and Moreno-Arribas, 2014). Biogenic amines are low molecular weight nitrogenous compounds with biological activity formed by the decarboxylation of specific amino acids, and a small amount is synthesized by animation and transamination reactions of aldehydes and ketones (Tsafack and Tsopmo, 2022). These are classified according to their chemical structure and volatility; according to their structure, there are aromatic amines, such as tyramine, histamine, tryptamine, and fe-phenylethylamine, while aliphatic amines are monoamines, such as methylamine; and polyamines include putrescine, cadaverine, agmatine, spermine, and spermidine. However, the significant heterogeneity of amines according to their volatility is classified as volatile and non-volatile. These are diethylamine, isopropylamine, phenylethylamine, histamine, tyramine, spermine, spermidine, and agmatine (Önal, 2007).
In Table 1, the authors highlight the primary biogenic amines, precursor, and precursor enzymes (Moy and Todd, 2014).
Biogenic amines (BA) (Munir et al., 2020) are metabolized in the liver. This action is mediated by several enzymes such as monoamine oxidase (MAO), diamine oxidase (DAO), and polyamine oxidase (PAO), mitochondrial enzymes that catalyze the oxidative deamination of monoamines, diamines, and polyamines. The main function of the detoxification system is the metabolism of various biogenic amines involved in human metabolic functions, such as the aforementioned amines, histamine, and tyramine (Durak-Dados et al., 2020) which play an effective role in the detoxification system (Figure 1). Therefore, this decarboxylation process depends on three main factors, such as the level of activity of the decarboxylases, the availability of amino acids as substrates and the presence or absence of specific bacterial strains (Naila et al., 2010). With the importance of the above-mentioned, the alteration of this enzymatic mechanism in the elimination of nitrogenous compounds is caused by several factors, such as genetic conditions, gastric pathologies, excessive consumption of foods containing amines, and self-medication that inhibit the enzymatic activity, leading to an accumulation of this amine in the system (Håkanson et al., 1967; Mastrangelo et al., 2023).
The elaboration of these traditional beverages through the fermentation process of the ingredients depends on the microorganisms present in the raw material, on natural conditions, and, in some cases, on human saliva. A specific example is the traditional production of chicha, which is distinguished by using a unique method that takes advantage of the properties of human saliva. The process begins with cooking the cereal grains, which gelatinize the starch present. The cooked grains are then ground and undergo a chewing process. This step is fundamental since saliva contains an enzyme called amylase, which has the ability to break down starch into simpler, fermentable sugars. Finally, spit it into a container, where these sugars are naturally fermented, producing alcohol, where the bacteria are in the oral cavity (Freire et al., 2016).
Gram-positive microorganisms (LAB) usually appear in the elaboration of fermented beverages, thus promoting the biosynthesis of Amine biogenic (BA). Once the beverage is ingested, these compounds are metabolized by enzymes in the gastrointestinal tract, playing an essential role in the circulatory system and contributing to the organism’s hydro-homeostasis. Currently, Gram-positive microorganisms belonging to the lactic acid bacteria group that favors amine biogenic biosynthesis have been identified to produce fermented beverages. These usually appear in raw materials or are included as starter cultures for fermentation (Alvarez and Moreno-Arribas, 2014).
During food and beverage fermentation processes, the ability to produce biogenic amines is a property that varies according to the microbial strain involved in these processes (Ma et al., 2024). This is because the formation of biogenic amines depends on the presence of specific decarboxylase enzymes in the fermenting microorganisms (Barbieri et al., 2019). For example, some strains may contain enzymes capable of converting the amino acid histidine to histamine or tyrosine to tyramine. In contrast, other strains lack these decarboxylase enzymes and will not produce biogenic amines during fermentation (Cachaldora et al., 2013; Kahraman-Ilıkkan, 2024).
All living organisms contain biogenic amines, which serve crucial metabolic functions. Nonetheless, some microorganisms can alter the enzymatic pathways involved in amine metabolism, leading to high concentrations of these compounds in food. The enzymatic pathways involved in the metabolism of biogenic amines in fermented foods can be altered in several ways: By the activity of microorganisms present in the food, which produce decarboxylase enzymes (Peregrín-Alvarez et al., 2009) that convert amino acids into biogenic amines, and due to factors such as temperature, pH (Costa et al., 2015), oxygen availability, carbon sources, coenzymes (Erdag et al., 2019) and the presence of free amino acids and fermentable carbohydrates, which influence the formation of biogenic amines (Cossu et al., 2023). By reactions of biogenic amines with nitrite and nitrogen acids, forming carcinogenic (Bott et al., 2019; Tumor, 2019) nitrosamines, or by transformations from one type of biogenic amine to another. Finally, due to the alteration in the processes of absorption and transport of free amino acids, the displacement of the cellular redox potential affects the anabolic and catabolic reactions of amino acid (Lee and Finkel, 2013) metabolism. As this study (Geamanu et al., 2016) shows the toxic action of tumor breakdown products that deplete amino acids such as cysteine, lysine, and glutathione, this study also identified alterations in amino acid metabolic pathways in patients with sarcoidosis, including changes in alanine, aspartate, glutamate metabolism, synthesis and degradation of ketone bodies. Imbalances in amino acids such as isoleucine and glutamine were observed, suggesting a disturbance in these patients’ amino acid and energy metabolism (Figure 1).
This poses a risk to consumer health, as depicted in Figure 2. Consuming fermented foods can cause various health issues such as gastrointestinal problems (nausea, vomiting, diarrhea, abdominal pain), hemodynamic issues (hypotension, hypertension), and skin reactions (itching, urticaria, edema, inflammation) due to the presence of biogenic amines. Parameters and concentrations have been established for these amines in fermented foods to avoid such issues. The parameters to consider are whether they occur naturally, due to microbiological contamination, or during fermentation. Fresh foods should have a sum of different biogenic amine concentrations (such as tyramine, histamine, putrescine, and cadaverine) less than 5 mg/kg for optimal freshness (Gargantilla et al., 2016). Additionally, the concentration of spermidine and spermine should be less than 0.5 to indicate freshness and exceeding 0.7 indicates that the food is in the process of putrefaction (Sirocchi et al., 2013; Sentellas et al., 2016).
When enzymatic pathways are changed, diamine oxides can become harmful substances in the body. One example is histamine, which is produced when food is broken down. In the intestines, these substances can cause health problems like nausea, vomiting, diarrhea, and damage to internal organs (Figure 3).
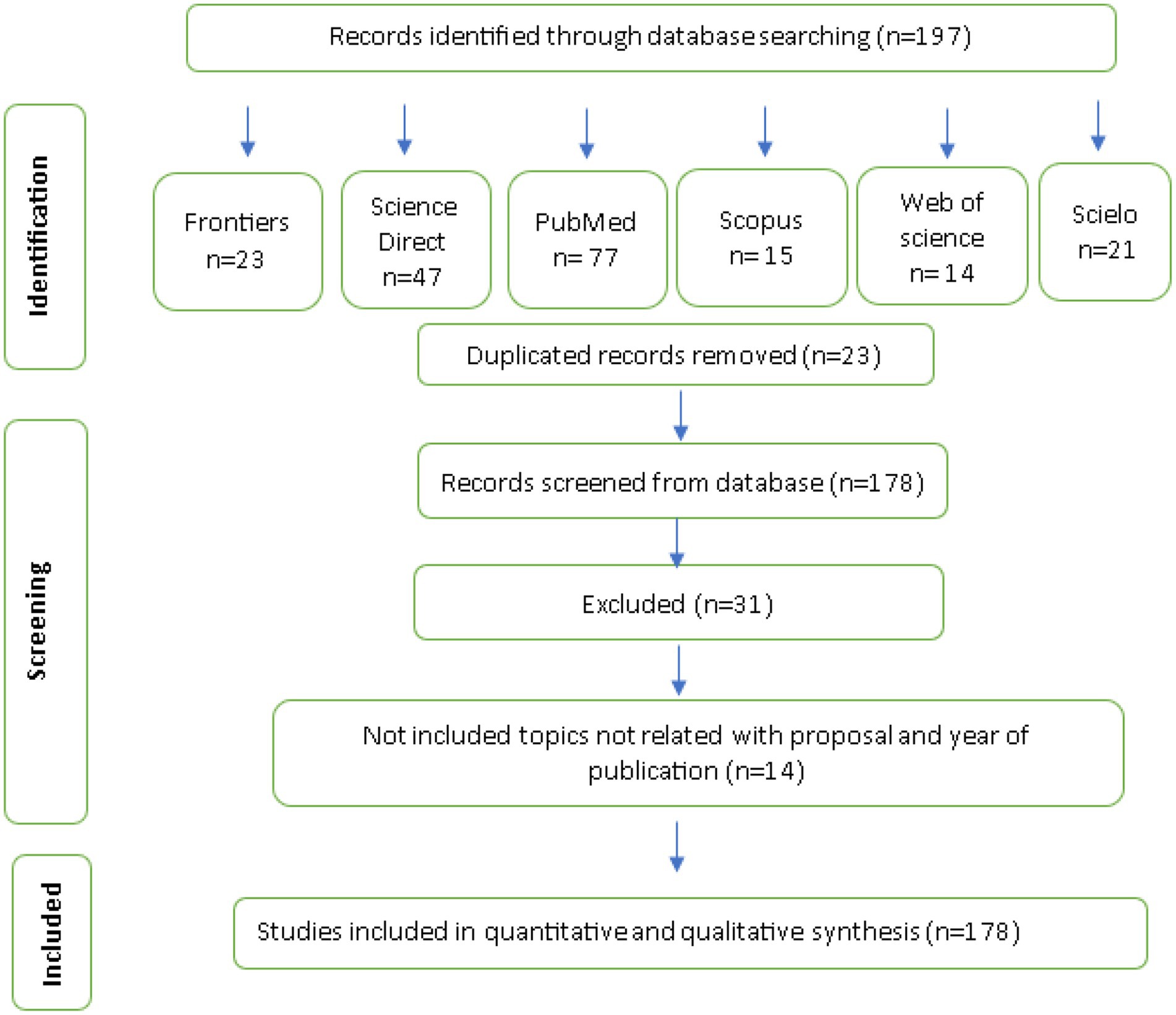
Figure 3. Flow diagram of literature search and selection criteria for fermented beverages among indigenous Latin American societies from PRISMA.
Concerning the problems described in the previous paragraphs, various analytical techniques have been implemented to detect bacterial strains forming these nitrogenous compounds, making it possible to evaluate the safety and innocuousness of fermented products. For techniques, liquid chromatography (LC), ultra-performance liquid chromatography (UPLC), and high-performance liquid chromatography (HPLC) (Marcobal et al., 2006).
The two compounds (phenolic and biogenic amines) in fermented drinks can harm human health by causing nausea, headaches, gastrointestinal discomfort, and intoxication. Unfortunately, there is limited information on measuring the amount of these compounds in beverages despite their prevalence in ancestral cultures. However, the primary biogenic amines (histamine, tyramine, putrescine, and cadaverine) in foods and beverages do not illustrate the concentrations of fermented beverages from ancestral foods (Ordóñez et al., 2016). An important issue is the safety of traditional fermented foods, which casts doubt on their nutraceutical benefits. Biogenic amines and phenolic compounds are linked to food safety and quality in most fermented foods.
Fermented beverages like apple juice, wine, and green tea are frequently consumed and are rich in phenolic compounds. These compounds are absorbed and metabolized in the body, which increases the antioxidant capacity of plasma and helps prevent chronic-degenerative diseases. People who want to improve their digestive system and overall health find these beneficial and widely accepted. This systematic review aims to explore the nutritional information of South and Central American fermented beverages.
2 Materials and methods
This systematic review follows the PRISMA (Preferred Reporting Items for Systematic Reviews and Meta-Analysis) verification protocol (Moher et al., 2009). The study focused on exploring the nutritional information of South and Central American fermented beverages by searching for articles or reviews specifically in the Biomed, PubMed, ScienceDirect, Scopus, Scielo and Google Scholar databases. The following studies were excluded: review articles published more than 15 years ago, not found in PubMed or the databases, or not meeting the search criteria.
The study selection process was carried out in two stages. In the first, three authors independently reviewed the titles and abstracts of the studies based on the inclusion and exclusion criteria. They identified studies that met the inclusion criteria and excluded those that did not. In the second stage, two authors thoroughly reviewed all full articles and excluded studies that did not meet the inclusion criteria. Customized search strategies were developed for the PubMed bibliographic database. The search was performed directly and included all articles published in the last 15 years without language restrictions. In addition, the reference lists of the selected articles were thoroughly examined to identify any relevant research that might have been overlooked during the electronic database search. Three authors collected the necessary data from the selected reports. For all incorporated studies, the following details were recorded: author (s), year of publication, traditional fermented beverages, nutritional benefits and health impact, and the presence of biogenic amines during fermentation in South and Central America. The authors independently reviewed all full articles.
2.1 Eligibility criteria
2.1.1 Inclusion and exclusion criteria
Studies were included if they met the following criteria: (1) included reviews of traditional fermented foods in Spanish-speaking countries; (2) fermentation of foods by LAB; (3) systematic reviews of biogenic amines and phenolic compounds during the manufacture of fermented beverages; (4) nutritional benefits of ancestral fermented beverages (5) examined articles about food safety during the fermentation process (6) Countries of South and Central-Latin America. Studies that were purely theoretical, books, and theses and did not meet the research objectives and over 15 years were excluded.
2.2 Search strategies
To refine the search, specific keywords such as “fermented beverages” “chicha de chonta” “nutrition” “chicha de yuca” “ancestral communities in Latin America” “biogenic amines” “food safety” “bioactive compounds” “nutritional benefits of ancestral fermented beverages” were used in conjunction with the use of search operators (“traditional fermented beverages*” OR “ancestral beverages*”) AND (“chicha de chonta*” OR “chicha de chontaduro” OR “peach palm” OR “Bactris gasipaes”) AND (“chicha de yuca*” OR “chicha de cassava” OR “Manihot esculenta”) AND (“ancestral communities in Latin America” OR “indigenous societies in Latin America” OR “indigenous population in Latin America”) AND [“food safety” OR “food safety” OR “good manufacturing practices (GMP)”] AND (“nutritional benefits of ancestral fermented beverages”) AND [“food safety” OR “food safety” OR “good manufacturing practices (GMP)”] AND (“nutritional benefits of fermented beverages*” OR “nutritional properties* of fermented beverages” OR “nutritional value* of fermented beverages” OR “nutrient content of fermented beverages”) AND (“nutritional benefits of fermented beverages*” OR “nutritional value* of fermented beverages” OR “nutrient content of fermented beverages”).
2.3 Identification of relevant studies
An exhaustive analysis of the fermented beverages made by the native populations of Latin America has been carried out. Furthermore, this has been carried out using a bibliographic search following the verification protocol with the PRISMA format and using the following MeSH (Medical Subject Headings) terms, with keywords in the search strategy such as: “fermentation,” “chicha,” “Biogenic Amines,” “food safety,” “raw material” and “nutritional benefits” of ancestral fermented beverages along with the use of AND, OR, NOT search operators. The explore databases, including Biomed, Pubmed, ScienceDirect, Scopus, Scielo, Google Scholar, to identify publications published within the past 15 years. The final database search was completed on April 29, 2024.
3 Results
3.1 Study selection
In the first phase of study selection, 52 citations were found in the PubMed electronic database. After thoroughly reviewing the abstracts, we excluded 31 articles with 23 duplicates. We then identified 25 additional articles through PubMed and reviewed their full text. Thus, this study included 178 references.
3.2 Study characteristics
The following table summarizes the key characteristics of the studies analyzed in this research. These studies were conducted in different countries and published between 2015 and 2023, both in English and Spanish. All the selected studies focused on traditional fermented beverages from South and Central-Latin American countries, indicating the origin of the country where their population traditionally consumes them, the main components, the raw material from which the beverage is made, and their potential functional benefits. The studies have produced significant findings on traditional beverages are consumed mainly for nutritional, gustatory and shared pleasure needs, the former during festivities and the presence of antioxidant compounds, short-chain fatty acids, amino acids, vitamins, minerals and beneficial microorganisms that enrich the nutritional profile of the beverage.
3.3 Data extraction
For each study in the review, the following information was extracted: beverage, origin, composition, raw material, benefits, and its corresponding reference.
Table 2 shows the nutritional composition, origin, raw material, benefits, and sources of the reviewed traditional fermented beverages. Certain drinks contain macro-and micronutrients and vitamins that differ based on the type of raw material used by Indigenous people.
The following table highlights that Mexico contributes most significantly to research on fermented beverages, primarily consumed in ceremonies (Rocha-Arriaga et al., 2020).
3.4 Pulque o poliuhqui octli beverage
This article (Pérez-Armendáriz and Cardoso-Ugarte, 2020) mentions that in Mexico, an essential collection of traditional fermented beverages has been preserved through generations, such as pozole, atole agrio, choose, tepache, tuba, taberna, tesgüino, colonche, and axolotl, among others. These food products are produced artisanally through spontaneous fermentations carried out by various species of microorganisms, including yeasts, bacteria, and filamentous fungi. In this way, ancestral recipes and techniques developed by indigenous peoples are perpetuated. The metabolic action of one or more of these microorganisms on the substrate confers to the fermented food its characteristic sensory and qualitative attributes and specific functionalities to the final product. In addition to the microbial influence, the quality of these homemade products is subject to extrinsic factors such as temperature, rainfall, and humidity, which are uncontrollable for producers and lead to differences in flavor, aroma, color, texture, and quality of the batches.
Pulque is a traditional Mexican alcoholic beverage made from the fermented sap of the agave plant, while Poliuhqui Octli is a similar beverage made from wild agave varieties (Pérez-Armendáriz and Cardoso-Ugarte, 2020). Fermentation involves extracting, collecting, and transporting the mead to the tanks. Once in the tanks, a microbial inoculum called “seed” is added, obtained from a previous pulque preparation. It takes about 36 h after inoculation for fermentation to be complete, and the pulque is ready for consumption (Valdivieso Solís et al., 2021).
The relevance of the fermentation process lies in preservation. It helps preserve the highly perishable agave sap, increasing the product’s shelf life and allowing it to be stored for longer periods. Moreover, the fermentation process breaks down sugar complexes and releases vitamins and minerals, increasing the nutritional value of the beverage. This could lead to health benefits, making it (Gutiérrez-Uribe et al., 2017).
3.5 Pineapple tepache beverage
The pineapple tepache is a traditional Mexican beverage enjoyed for centuries and is an integral part of the country’s cultural heritage (de la Fuente-Salcido et al., 2015). This beverage is made from pineapple, piloncillo (unrefined cane sugar), and spices such as cinnamon and cloves. The composition of pineapple tepache can vary depending on the recipe and fermentation process used. The fermentation was made from ripe pineapple peels (unwashed) and a previously pasteurized solution of 13% panela (brown cane sugar) in a 1: 2 ratio pineapple/panela solution (Gutiérrez-Sarmiento et al., 2022). The container was covered and sealed with self-adhesive plastic to simulate semi-aerobic conditions. The nutritional composition of pineapple tepache can also vary depending on the amount of sugar used and the fermentation time. However, it is generally considered a source of carbohydrates and some vitamins and minerals. It also contains probiotics, which can benefit gut health and the immune system (De La Fuente-Salcido et al., 2015).
3.6 Sour atole beverage
Sour atole is a traditional Mexican beverage typically consumed as a breakfast drink (Väkeväinen et al., 2018). The composition of sour atole can vary depending on the recipe and preparation method. For the fermentation, the grains of the ears of corn were cut with a knife, crushed, and mixed with water until a white mass was obtained (Teller et al., 2012). Then, the dough was manually moulded to ferment the sour atole in a solid state. The balls were left to ferment for 12 h at 34°C; Water (1 L) and hand-homogenized corn water slurry were added (Tchekessi et al., 2021).
The fermentation times were selected based on the traditional elaboration of the sour atole process. The fermentation process of sour atole is significant in nutrition as it lowers glycemic indexes, making it a potentially beneficial beverage for people with diabetes or those seeking to manage their blood sugar levels. Moreover, the lactic acid bacteria generated during fermentation aids in the digestion of corn masa and other ingredients in sour atole (Cuamatzin-garcía et al., 2022).
3.7 Tuba beverage
The tuba is an alcoholic beverage made from the sap of various palm trees, such as coconut, nipa, or kaong (Gregorio, 2023). The exact composition of the tuba can vary depending on the type of palm tree used and the fermentation stage. The fermentation process begins by bending the axis of the coconut stem so that the sap flows freely from the incision or cut made. However, this bending must be done carefully to avoid breaking the floral cluster. The inflorescence is between 45 and 75 cm long (Gibbons et al., 2015), and the process is slow, taking 3-4 days. The color of the tuba can change due to the action of microorganisms, and fermentation can begin 5 h after the tuba harvest (Chacón Mayorga et al., 2020).
The fermentation of tuba can have several relevant effects on health (Pandiselvam et al., 2023). Firstly, during the fermentation process, the carbohydrates in the coconut sap are converted into organic acids, reducing the beverage’s sugar content and glycemic index. This makes it a healthier option for persons with diabetes or those looking to control their blood sugar levels. Secondly, probiotic bacteria in tuba are strain-dependent properties that can promote gut health and improve digestion (Flores-Gallegos et al., 2019). Lastly, tuba contains several micronutrients, such as vitamins B and C, amino acids, and minerals like potassium, calcium, and magnesium, which can benefit overall health and well-being (Flores-Gallegos et al., 2019).
3.8 Ecuador, Perú and Argentina Chicha corn or maize (Zea mays)
Chicha is a traditional fermented beverage from South and Central America. It receives different names as masato, cahuana, among others, including Ecuador. There have been several studies on the chemical composition of Ecuadorian chicha, which can vary depending on the recipe and the region where it is produced (Aidoo et al., 2006). In most native populations, fermented foods and beverages are produced by spontaneous fermentation.
The fermentation process of these beverages starts from the grain of either cassava (Manihot esculenta) or corn (Zea mays) or, depending on the raw materials mentioned above, they are then malted (germinated and dried). First, the grains are left in water for a day. Although ethanol in fermented beverages is mainly attributed to yeasts coexisting with lactic acid bacteria (LAB), some of these bacteria have the heterofermentative capacity to generate ethanol. However, the analysis of these bacteria is beyond the scope of this review (Carboni et al., 2023). This step is necessary to achieve optimum grain moisture for germination. Subsequently, the water is drained, and the corn is placed in straw baskets to germinate in 13 days. Once germinated, the corn is placed on straw mats or plastic tarpaulins under the sun for 2 days to dry completely, stopping the grain’s enzymatic activity. After drying, the beans are ground, and the flour obtained is used to prepare these beverages. For this, the Jora or maize flour is added to cold water, and the mixture is transferred to containers with hot water and boiled for approximately 20 min. After boiling, the mixture is strained and placed in a container to ferment. Usually, these fermentation containers are open. In the past, clay pots were used for boiling and fermenting. However, they have been replaced by various aluminum and/or plastic containers. Finally, after 2 days of spontaneous fermentation by indigenous microorganisms, the beverage is ready for consumption (Chaves-López et al., 2020; Carboni et al., 2023).
The exact chemical composition of Peruvian Chicha can vary depending on the recipe and fermentation process used, but several studies have reported some common characteristics. Peruvian Chicha typically has a pH ranging from 4 to 4.5 and an alcohol content ranging from 2 to 12% (Chacón Mayorga et al., 2020). The fermentation process also involves the conversion of starches to sugars, including glucose and fructose, which contribute to its sweet taste. In addition to organic acids and sugars, Peruvian Chicha contains several amino acids, including proline, glutamic acid, and arginine. These amino acids are essential for the body and can contribute to the beverage’s nutritional value (Salvador-Reyes and Clerici, 2020). Peruvian Chicha is also a good source of vitamins B and C essential for energy metabolism and immune function. It also contains minerals such as calcium, magnesium, and phosphorus, essential for bone health and other physiological functions (Ramos Guerrero et al., 2021).
It should be noted that in Argentina (Giovannetti, 2021), chicha made from corn is consumed and is commonly known as chicha de jora. For chicha (Maimará production), the fermentation process began with criollo corn flour (old regional variety) was soaked with warm water (about 40°C, approximately 1:1, w/v) and mixed thoroughly until a semi-solid mass was obtained. After the mixture had cooled, flat buns were shaped by hand and baked on a tray in a rudimentary clay oven for 1.5 h. To produce tumaya, cornmeal toasted in a clay oven was used before being added to water. Roasting or baking allows the Maillard reaction for chicha’s typical colour and brownish flavor (Hiroshi et al., 2020).
The layers were then separated: an upper liquid layer (supernatant), a middle gelatinous layer, and a lower residual layer (discarded). In the elaboration of Maimará (chicha M), the intermediate layer was pressed, filtered with a cloth, and cooked over low heat to caramelize (arrope). Then, the supernatant was mixed with the separates, added with more water, and left to ferment for 2–4 days at 20°C. When the bubbling stopped, it was transferred to narrow-mouthed pots and was ready for consumption (Jimenez et al., 2022).
3.9 Caxiri
The caxiri is prepared mainly in the eastern Amazon and produced by the Juruna or Yudjá Indians in Brazil. Each woman uses different starch-rich plants called in Portuguese “temperos do caxiri,” an individual knowledge of recipes and fermentation processes. In the intercultural region of Rio Negro (Capredon, 2018), some of the ingredients are manioc, sweet potatoes, macoari (Heliconia Hirsuta), long potatoes (Maranta arundinacea L.), sugar cane, cucura (Pourouma cecropiifolia), cotton beans (Inga edulis) or plantains. Among the Wayapi of French Guiana, many caxiri recipes are prepared with bitter cassava (Jolly and Philippe, 2007). Caxiri is obtained by fermenting the purple cassava roots for 2 days in running water to soften the bark. This procedure allows the degradation of cyanogenic compounds and the formation of aromatic substances; consequently, the roots are softened by the action of lactic bacteria (Chacón Mayorga et al., 2020). The cassava is peeled, cut into small pieces, pressed, grated, and roasted. The resulting flour is mixed with water and placed in barrels to initiate the fermentation process, which usually lasts up to 120 h after its preparation and varies its alcohol content (Schwan et al., 2007); all preparations involve a detoxification procedure. According to mythical accounts, once the yucca is without poisonous, it is chewed and mixed with sweet potatoes and honey from stingless Melipona bees. These examples show the diversity in preparing the beers of the name caxiri (Jolly, 2007; Emperaire, 2023).
3.10 Cauim
Cauim is a beverage traditionally from the Amazon rainforest of Brazil (Schwan et al., 2017; Lima et al., 2022). This fermented beverage is integral to indigenous culture and has been consumed for centuries in rituals and celebrations. Brazil is a highly diverse country regarding indigenous peoples, and many indigenous societies have traditions related to Cauim in different regions. Some of the indigenous societies that have been historically involved in the elaboration of Cauim in Brazil include, Guarani-Kaiowá (Heurich, 2015) from the Jaguapiru village, the Tapirapé indigenous people (Schwan et al., 2007), Tikuna and Tukano peoples, and the Tikuna and Tukano peoples. The primary raw materials used in the elaboration of Cauim are cassava, known as manioc or cassava, and corn are ground and then chewed to release enzymes that break down the starches into sugars, giving way to fermentation for a few days (Naupert, 2019). This drink is linked to the connection with nature and the spiritual world and is a deeply rooted part of the community (Resende et al., 2018; Chacón Mayorga et al., 2020).
3.11 Chicha Yuca or cassava
In South American Lowlands, traditional fermented beverages are mainly prepared from cassava (Manihot esculenta). M. esculenta contains different levels of cyanide, which is differentiated between bitter and sweet manioc (Castilla Corzo et al., 2019; Maicas, 2020). To avoid poisoning, the first needs a laborious transformation process, which supposes a stage of putrefaction of the tubers, fungus used also for the fermentation process. The consumption of sweet manioc requires boiling the tubers, and women mainly masticate the cooked paste to ferment and prepare the traditional fermented beverages. Sometimes, to accelerate the fermentation process with both cassava varieties, yams (Diosocrea sp.) or sweet potato (Ipomoea batata) are added (Mendoza et al., 2017; Castilla Corzo et al., 2019; Maicas, 2020).
3.12 Peach palm or Chicha Chonta alcoholic beverage
Peach palm, Chontaduro (Bactris gasipaes) is distributed from Central America to Bolivia. It is a symbolic plant among Indigenous groups associated with the forest abundance period and for social reproduction (González-Jaramillo et al., 2022). During the stationary ripening period, people consume peach palm daily as a soft drink and an alcoholic beverage. The drink preparation is simple: cooked, peeled, and mashed; after a few days, the drink is naturally fermented (Komesu et al., 2017). Recent research has identified the core microbiome, consisting of six main genera belonging to lactic acid bacteria (LAB) and acetic acid (AAB). The predominance of the genus Lactobacillus was evidenced (more than 50% of the identified sequences belonged to this taxon), followed by Weissella, Leuconostoc, Lactococcus, and Streptococcus. In addition, they showed that the main metabolic pathways involved in the fermentative processes that occur during the elaboration of chicha involve the catabolism of carbohydrates and amino acids, as well as the mechanisms of coenzyme transport. These metabolic pathways are represented by the predominant functional categories within the KEGG (Kyoto Encyclopedia of Genes and Genomes) database associated with the microbiome of this traditional beverage (Bassi et al., 2020).
Chonta chicha is a highly appreciated drink because of its high vitamin content. Traditionally, people used to prepare and bury the chicha dough to preserve it for several months. The process consisted of placing 5-10 cooked chonta seeds in the dough, wrapping everything in banana leaves tied with ropes, burying it in a 50 cm deep hole with sticks or stones at the bottom, then covering it with more banana leaves. After 3-4 months, the buried chicha could be consumed, especially in times of scarcity (Pazmiño et al., 2014).
3.13 Food safety of fermented beverages
According to the Food and Agriculture Organization of the United Nations (FAO), since the 1996 World Food Summit (WFS), food security “at the individual, household, national and global levels, is achieved when all people, always, have physical and economic access to sufficient, safe, and nutritious food to meet their dietary needs and food preferences for an active and healthy life” (Food and Agriculture Organization of the United Nations, 1996). According to this, food safety is one of the main concerns in developed countries as it directly affects the health of all citizens. This concern has increased in recent years due to serious incidents that have made food safety a significant social issue. Additionally, there is a lack of quality assurance, a negative impact on the growth and development of microorganisms, and the belief of small-scale producers that every stage in producing artisanal fermented beverages should be under a similar condition. Therefore, these factors interfere with the product’s nutritional quality, organoleptic characteristics, and safety modifications (McGuire et al., 2015).
Research indicates the presence of microbial agents and various types of toxic chemical compounds that are metabolic products of microbial origin throughout the fermentation process, causing harmful health risks for consumers (Fayyaz et al., 2022). Some microbial agents produce chemical compounds such as biogenic amines, such as Bacillus cereus, Escherichia coli, and Staphylococcus aureus (El-Sayed et al., 2022). Therefore, the relevance of food safety in investigating components that harm humans is highlighted, and two types are currently being studied: biogenic and phenolic compounds (Insfran-Rivarola et al., 2020). The microbial load found in fermentation generates organoleptic characteristics and toxic substances, which researchers are currently more interested in studying.
Some indications that must be taken into consideration for the elaboration and storage of safe and harmless non-alcoholic fermented beverages and to prevent microbial growth are as follows: (1) control of the primary product that is in physicochemical conditions suitable for consumption, (2) establishment of hygiene protocols throughout the production phases, (3) implementation of equipment that prevents contamination-proliferation of microorganisms, (4) inspecting factors such as temperature, pH, substrate concentration, among others. These factors will reduce the risk to human health (Gómez-Rave et al., 2022).
Biogenic amines in these foods have vasoactive properties, including histamine and tyramine. These components do not have maximum limits; however, exceeding 8-20 mg/L for histamine (Shimoji et al., 2020) and 25-40 mg/L for tyramine in wine is not recommended (Mukherjee et al., 2022). Symptoms of toxicity include discomfort, nausea, respiratory alterations, migraines, itching eyes, and intestinal problems (Wójcik et al., 2021). Histamine is present in fermented products and fish, and its main action is vasoactive and psychoactive due to the alarming symptoms caused by this chemical compound. A study was conducted in Peru using HPLC to analyze chicha de Jora. It was found that histamine was present at 37.53 mg/L and tyramine at 77.96 mg/L, which is concerning because the levels are close to the permitted limits (Periche Pérez, 2018).
According to these studies, international organizations seek to implement biotechnologies to preserve and conserve fermented products from foods such as tubers (Mukherjee et al., 2022). This ensures the safety of products intended for human consumption, which would lead to increased trade, reduced production costs, prevention of loss of raw materials, and prevention of alteration of ingredients that could affect the product’s nutritional value. Currently, the FAO states that during 2020-2021, countries in Latin America and the Caribbean (LAC) have reported severe food insecurity, affecting 40.6% of the region’s population (Regional Overview of Food Security and Nutrition - Latin America and the Caribbean 2022, 2023).
3.14 Nutritional benefits of fermented beverages
Humans have consumed fermented beverages for thousands of years, not only for their sensory properties but also for their nutritional and health benefits. These benefits derive from the bioactive compounds produced through the fermentation process and the probiotic microorganisms themselves. During fermentation, nutrients already present may become more bioavailable. Additionally, vitamins, minerals, bioactive peptides, enzymes, and phenolic compounds are presented. These compounds have antioxidant, anti-inflammatory, and antimicrobial properties and are often found in fermented drinks. And in the microbiota within fermented beverages may also positively impact digestive and immune health. Regular consumption has been linked with reduced risks of cardiovascular disease and hypertension (Liburdi et al., 2020).
Chicha de chonta is a traditional fermented beverage made from the palm tree Bactris gasipaes (Guerra et al., 2022). Native societies in Latin America have used chicha de chonta for centuries due to its rich nutritional properties and cultural significance. Recent investigations into traditional fermented foods have brought renewed interest toward this ancestral drink (Thierry et al., 2023). Chonta palm contains an array of essential nutrients. Its carotene-rich palm hearts are an excellent source of vitamin A, and vitamin E (González-Jaramillo et al., 2022).
Chontaduro or peach palm, the starch-storing inner pith, provides abundant carbohydrates and some protein. These inherent nutrients carry over into the fermented chicha. However, the fermentation process induced by naturally occurring yeasts and bacteria further enhances the nutritional quality in various ways. Thus, fermentation augments the vitamin profile, rendering chicha de chonta a nutritious functional drink (Costa et al., 2022; Pinheiro et al., 2022).
Fermenting also increases protein digestibility and bioavailability of certain minerals through phytase enzymatic activity. Microorganisms degrade complex phytic acid polymers, liberating bound minerals. This effect combined with vitamin C present vastly improves iron absorption. Alpha-amylase fungal enzymes additionally hydrolyze starch into simpler sugars. Other metabolites generated like organic acids, amino acids and antioxidants further contribute to the nutritional value. Lactic, acetic, and succinic acid concentrations increase. All affect acidity, flavor, and preservation. Amino acids like glutamate accumulate, enhancing taste. Phenolic compounds and carotenoids with antioxidant potential are also amplified, increasing health benefits (de Cássia et al., 2022).
Chicha de yuca is a traditional Latin American beverage that ferments boiled cassava roots. Women in indigenous communities have prepared it for generations using ancestral techniques that enhance its nutritional quality. Recent scientific analysis is now shedding more light on this nutritional enrichment process. Fresh bitter yuca roots contain toxic cyanogenic glycosides, rendering them inedible without proper processing. However, they are rich in carbohydrates and provide vitamins A and C and minerals. Traditional elaboration involves germinating, soaking, boiling, and masticating the roots to reduce toxicity. This liberates starch and nutrients, facilitating microbial fermentation (Emperaire, 2023).
The resulting chicha becomes more nutritious and digestible through biochemical transformations induced by wild yeast and lactic acid bacteria. Protein digestibility and mineral availability also increase during fermentation. Fungal amylases help hydrolyze starch, while phytases liberate protein and minerals like iron and zinc bound to phytic acids. This improves nutritional quality and absorption. Antioxidant phenolic content increases due to galacto-oligosaccharides and folates emerging via enzymatic glycosylation. Carotenoid levels are also boosted, conferring attractive colors. Overall, traditional chicha making enhances the nutritional utilization of a staple like bitter yuca from initially toxic roots. Fermenting with natural microflora reduces toxins and increases safety for consumption, but also effectively turns this simple carbohydrate and water drink into a probiotic functional beverage rich in vitamins, bioactive phenolics, antioxidants and a composition of compounds beneficial for health. This ancient process is an exemplary model of early food biotechnology (Aro, 2010; Ana Lúcia Barretto Penna, 2016; Mohidin et al., 2023).
The caxiri fermentation process introduces many beneficial microorganisms, including lactic acid bacteria, yeasts, and bacilli (Miguel et al., 2015). These microorganisms bioconvert the compounds in the cassava, generating bioactive compounds that improve the beverage’s nutritional profile and health effects. Specifically, antioxidants such as phenols and flavonoids that prevent oxidative damage from free radicals are generated. Lactic acid bacteria synthesize B vitamins such as riboflavin, folic acid, and cyanocobalamin (Capozzi et al., 2012). Organic acids such as lactic, acetic, and propionic acids provide prebiotic effects (Punia Bangar et al., 2022). Peptides and essential amino acids are released to improve protein digestibility (Amigo and Hernández-Ledesma, 2020). Thus, through fermentation, caxiri becomes a valuable source of functional and bioactive nutrients that greatly benefit the health of traditional communities (Jimenez et al., 2022).
Several authors have investigated the isolation and evaluation of the probiotic potential of lactic acid bacteria (LAB) from meat and fermented pulque (Escalante et al., 2016). The objective is to use these LABs to formulate new non-dairy functional foods. LAB strains such as Lactobacillus acidophilus (Villarreal-Morales et al., 2018) and Lactobacillus plantarum have been identified and appear to play an essential role in pulque due to their antimicrobial activities. In addition, the natural resistance of these LAB to the acid pH (Ramírez-Guzmán et al., 2019; Villarreal-Morales et al., 2019) and alcohol content of pulque, as well as the traditional use of pulque to treat gastrointestinal diseases, suggest that LAB present in pulque are good candidates to be investigated as probiotics (Gutiérrez-Uribe et al., 2017). In summary, these studies propose that particular LAB from the pulque fermentation process could be used to develop new non-dairy functional foods with probiotic properties (Villarreal-Morales et al., 2019). Continuing with the previous idea, it has been demonstrated that fructooligosaccharides derived from agave have a prebiotic function. Several studies have shown that these compounds promote the in vitro growth of different species of beneficial Lactobacillus and bifidobacteria, including probiotic strains known as Bifidobacterium animalis, Bifidobacterium bifidum; Bifidobacterium longum, Bifidobacterium infantis and Bifidobacterium adolescentis (Olveira and González-Molero, 2016). Some prebiotic bacteria are considered predominant and favorable in the human gut microbiota. In summary, previous research suggests that agave fructooligosaccharides exert a prebiotic effect by selectively stimulating the development of gut health-promoting bacteria (Lee et al., 2023).
Aguamiel is the sweet sap extracted from different species of the Agave genus and is considered a beverage with high nutritional content and bioactive compounds (Villarreal-Morales et al., 2019). Therefore, aguamiel could be commercially positioned as a healthy and functional drink. It also contains fructooligosaccharides, which give it prebiotic properties, and saponins that have demonstrated various biological activities. It has been shown that aguamiel exhibits antioxidant activity, which is attributed to its phenolic compounds (Castañeda-Ovando et al., 2023). In addition, it has been suggested that its consumption could help meet the recommended daily requirements of nutrients such as iron and zinc (Castañeda-Ovando et al., 2023). Another relevant characteristic is that aguamiel has phytase activity, which could improve the bioavailability of minerals in foods containing phytates.
Lopez and Urias-Silvas, in a research study, demonstrated that aguamiel fructans have a prebiotic effect, promoting the growth of bifidobacteria and lactobacilli (Lopez et al., 2007). They also found differences in the prebiotic effect between fructans from the same agave species but different geographic areas. While, Gutiérrez-Uribe and Serna-Saldivar (Serna-Saldívar et al., 2013) found that concentrated aguamiel stored for at least 8 weeks inhibited the in vitro growth of colon cancer Caco-2 (84.8%) and liver cancer HepG2 (67.9%) cell lines (Figueroa et al., 2017). Leal-Díaz evidenced in mice that concentrated aguamiel improves glucose tolerance, prevents increased LDL-cholesterol, and modulates gut microbiota (Leal-Díaz et al., 2015).
4 Discussion and perspectives
For centuries, fermented beverages have played an important role among the Indigenous people of Latin America, providing both nutrition and cultural and social significance. Recently, these traditional drinks have gained renewed interest due to their potential health benefits, which include probiotics, antioxidants, and other helpful compounds. An example of such a beverage is Andean chicha, made from fermented corn and still widely consumed in Peru, Ecuador, and Bolivia. Recent studies have revealed that Andean chicha contains beneficial bacteria such as Lactobacillus and Bifidobacterium, which can improve gut health and strengthen the immune system (Guerra et al., 2022).
Similarly, traditional Mexican beverages such as pulque, tepache, and pozol have also gained attention for their potential health benefits. Pulque, a fermented drink made from the sap of the agave plant, has been found to contain antioxidants and antimicrobial compounds. In contrast, tepache, made from fermented pineapple, has been shown to have anti-inflammatory properties (Liburdi et al., 2020). These traditional fermented beverages are not only beneficial for health but also serve as an essential cultural and social practice in indigenous societies. They are often consumed during religious and festive events and are considered a symbol of identity and tradition. Following the development of science, especially microbiology, yeasts, bacteria, and fungi are identified as the main actors in fermentation.
The development of science, particularly microbiology, has led to identifying yeasts, bacteria, and fungi as the key organisms responsible for fermentation. This article provides an overview of the production of fermented beverages, emphasizing both their geographical distribution worldwide and the content of bioactive compounds derived from the microbial activity that gives them special properties (Liburdi et al., 2020).
The food industry has shown increasing interest in producing fermented foods derived from various sources, such as dairy products, cereals, legumes, and fermented meat. A popular example is tempeh, derived from fermented legumes and produced using fungi from the Rhizopus genus. During fermentation, various bacteria such as L. acidophilus, L. delbrueckii, L. plantarum, L. sakei, and S. thermophilus are produced, which are also commonly used in the dairy industry to produce yogurt. These fermented foods have functional properties, such as improving intestinal transit and reducing muscle pain after intense physical activity (Chacón Mayorga et al., 2020).
Today’s fermentation of cereals in beverages still needs to be researched since it depends a lot on the geographical area, the culture, and the procedure of preparing each drink. However, there is no product in the food industry, and even the studies of these products are very few. However, the trend of using sourdough dough is revolutionizing the bakery market because a product is obtained that has various benefits for humans, such as improvement of symptoms of irritable bowel syndrome and gluten intolerance (Guerra et al., 2022). The recent focus on the health advantages of fermented drinks in amont Indigenous groups emphasizes the significance of preserving their traditional practices and knowledge while promoting the potential health benefits (Grijalva-Vallejos et al., 2021). In addition, the levels of certain compounds, such as biogenic amines, are regulated by concentration limits established by agencies such as the FDA (U.S. Food and Drug Administration) and EFSA (European Food Safety Authority) (Florez Duque et al., 2023).
Several studies analyzed bacterial community composition in a traditional yucca-based fermented alcoholic beverage produced by the indigenous Shuar people in Ecuador. They found that the genus Lactobacillus dominated all samples, accounting for more than 70% of the sequences. This indicates that lactic acid bacteria are the main microorganisms in spontaneous fermentation (Colehour et al., 2014). In addition, two Peruvian fermented beverages were analyzed in this study: Masato de Yuca, typical of Amazonian communities, made from cassava (Manihot esculenta); and Chicha de Siete Semillas, prepared with cereal, pseudocereal and legume flours. Lactic acid bacteria were isolated after obtaining permits from local authorities under the Nagoya protocol. From 33 initial isolates, 16 strains were obtained with different genetic profiles according to RAPD-and REP-PCR. In Chicha all were Lactiplantibacillus plantarum, while in Masato, Limosilactobacillus fermentum, Pediococcus acidilactici and Weissella confusa were also identified. Analyses evaluating their carbohydrate fermentation patterns and enzyme profiles allowed grouping the Lactobacillus separately from other genera. The 16 strains were subjected to in vitro static digestion simulating gastrointestinal transit. In addition, their ability to adhere to HT29 human intestinal cells was evaluated. After both procedures, the best probiotic candidate was Lactiplantibacillus plantarum Ch13, a strain able to withstand gastrointestinal conditions and with higher epithelial adhesion capacity than the commercial probiotic strain 299v. This Ch13 strain will be studied in depth in future work to characterize its benefit to human health (Rebaza-Cardenas et al., 2021). This work investigated by next-generation sequencing the bacterial biodiversity of 27 chicha samples from 14 different chicha producers in 7 provinces of Peru. A large variation in the microbial composition of chicha was found due to ingredients, manufacturing processes, and geographical influences. The core microbiome was represented by six main lactic acid and acetic acid bacteria. Lactobacillus was predominant in more than 50% of the sequences, followed by Weissella, Leuconostoc, Lactococcus, and Streptococcus. Acetobacter was the only genus of acetic acid bacteria identified. Sequences associated with spoilage and pathogenic bacteria such as Bacillus, Clostridium and Enterobacteriaceae were observed only in some samples, validating the safety of the beverage. Predictive functional annotation revealed that carbohydrate and amino acid metabolism are the main KEGG categories associated with fermentation pathways. Each producer’s recipes and traditional processing help maintain native microorganisms with technological potential and health benefits (Bassi et al., 2020).
This study conducted a literature review on the benefits of pulque consumption, its chemical and nutritional properties, including the health benefits associated with various lactic acid bacteria with probiotic potential isolated from the beverage. Thus, aguamiel, sap obtained from agave, contains highly digestible carbohydrates, minerals such as iron and zinc, essential amino acids, and bioactive compounds such as GABA, fructans, and fructooligosaccharides that have prebiotic properties, improving the absorption of calcium and iron, preventing colon cancer and promoting the growth of beneficial bacteria. Finally, lactic acid bacteria have been isolated from mead and pulque, mainly Lactobacillus acidophilus and L. plantarum, which have probiotic potential due to their antimicrobial activities and abundance at the end of fermentation. The isolation of these probiotic bacteria from non-dairy sources is a trend to develop new health-promoting functional foods (Escalante et al., 2016).
Studies have shown that the composition of Agave varies by species but contains mostly water; sugars such as fructose, glucose, and sucrose; minerals such as calcium, phosphorus, magnesium, and potassium; proteins; fiber; ascorbic acid; and antioxidants (Silos-Espino et al., 2007; Ortiz-Basurto et al., 2008; Narváez-Zapata and Sánchez-Teyer, 2009; Santos-Zea et al., 2012; Gutiérrez-Uribe et al., 2017). It also has fructooligosaccharides with prebiotic properties and saponins with diverse biological activities and antioxidant activity due to phenolic compounds, and its consumption can satisfy daily requirements of nutrients such as iron and zinc. It has phytase activity, improving mineral bioavailability in foods with phytates.
Nowadays, detecting amines in foods is crucial due to their potential toxicity and role as markers of food quality. This study (Önal, 2007) shows different methods of analysis of these substances, present in a variety of products such as meat, cheese, and fish, can cause adverse reactions in humans, such as hypertensive crises and migraines. Therefore, monitoring the levels of biogenic amines in foods is essential to ensure food safety and public health. In detecting biogenic amines in foods, analytical methods such as high-performance liquid chromatography and capillary electrophoresis are used, with UV, fluorescence, and conductimetry detection. These methods allow the precise identification and quantification of various amines in foods such as meats, cheeses, and beverages, which is crucial for evaluating food safety and product quality. These analyses reveal the presence of biogenic amines in variable concentrations, which may indicate inappropriate food storage or processing conditions, as well as the possible formation of toxic compounds such as nitrosamines. These findings highlight the importance of closely controlling and monitoring the levels of biogenic amines in foods to prevent public health risks and ensure the quality of consumed products. Furthermore, the detection of amines in foods can also reveal the presence of polyamines, compounds with critical physiological functions in the growth and development of fruits and other agricultural products. Identifying polyamines in foods using specialized analytical techniques provides valuable information on the quality and freshness of products and their potential nutritional value. The food industry can use these results to improve production and storage processes, guaranteeing the safety and quality of the food that reaches the final consumer. Likewise, some biogenic amines present in foods have the ability to react with nitrites to form nitrosamines, compounds known to be carcinogenic. This interaction may occur especially in meat products that contain nitrite and nitrate salts as curing agents. The formation of nitrosamines represents a significant health risk since these compounds have been associated with the development of cancer in humans. Other important findings to highlight are the toxic effects associated with these compounds, such as the induction of hypertensive crises and migraines in specific sensitive individuals. These adverse reactions can occur after consumption of foods with high levels of biogenic amines, highlighting the importance of controlling their presence in the diet. In particular, histamine has been identified as a causal agent in food poisoning outbreaks, which underlines the relevance of monitoring and regulating the levels of this amine in food products to prevent public health risks and ensure the safety of the foods consumed.
Another similar study (Marcobal et al., 2006) used thin-layer chromatography (TLC) and high-performance liquid chromatography (HPLC) to analyze biogenic amines in foods such as fish, dairy products, and meats, revealing the presence of histamine, tyramine, putrescine, and phenylethylamine. This method allowed a clear separation and precise identification of these amines in the bacterial cultures studied. The specific enzymatic methods for histamine-producing bacteria are based on the production of hydrogen peroxide by the action of an oxidase enzyme on histamine. These methods allow the precise and specific detection of bacteria capable of producing histamine in foods such as fish, dairy products and meat, essential to guarantee food safety by identifying potentially dangerous microorganisms. In this study (Landete, 2007), molecular methods like PCR were used to detect hdc gene products from gram-positive bacteria. It was observed that the primers JV16HC/JV17HC, CL1/CL2 and CL1/JV17HC were effective in the amplification of internal fragments of the hdc gene, with the PCR with JV16HC/JV17HC being able to detect all histamine-producing LAB. These molecular methods offer a valuable alternative for the early detection of biogenic amine-producing bacteria in foods, especially in detecting histamine in products such as raw fish, where histidine decarboxylase plays a crucial role.
Likewise, this study (Munir et al., 2021) developed an HPLC validation method for detecting histamine in mackerel samples and their products. Derivatization with FMOC-Cl improved the method’s sensitivity, allowing an efficient chromatographic separation in 15 min. The validation of the method, based on Decision 657/2002/EC, demonstrated a linear range of 0.16 – 5 μg·mL-1, detection and quantification limits of 0.10 and 0.30 μg·mL-1 respectively, and a recovery of 103 % in real samples. These results confirm the effectiveness and applicability of the method to guarantee the quality and safety of mackerel products intended for human consumption. Continuing along the same lines, this study (Munir et al., 2023) focused on detecting histamine in fish using an electrochemical sensor approach with molecularly modified screen-printing electrodes (SPE/MIP). Electrochemical techniques such as cyclic voltammetry (CV) and electrochemical impedance spectroscopy (EIS) were used to analyze histamine in fish samples. The results demonstrated the effectiveness of the electrochemical method for detecting histamine, with high selectivity and sensitivity compared to other biogenic amines, thus developing an effective and rapid method for detecting histamine in fish samples using SPE modified with MIP. The validation of the technique and the results obtained demonstrated the feasibility of this approach for the accurate detection of histamine in foods. These findings are fundamental to guaranteeing food safety and the prevention of histamine poisoning in fish products.
Now, it has been shown that fermented foods contain higher concentrations of biogenic amines due to the presence of Gram-negative and positive bacteria. For example, in fermented sausages, concentrates exceeding 500 mg/kg of biogenic amines have been found, mainly histamine, tyramine, putrescine, and cadaverine. Now, to prevent the foods produced from containing limits higher than recommended, several organizations have defined legal limits for these compounds in foods, but they depend on the country and the food (Florez Duque et al., 2023). The FDA governing food safety in the United States indicates that for histamine, the maximum limit is 50 mg/kg (Debeer et al., 2021). Meanwhile, the European Union establishes a maximum limit range between 100 mg/kg – 400 mg/kg depending on these products. Other biogenic amines have no legal limits, but there is a recommended intake to avoid toxic and harmful effects on health (Florez Duque et al., 2023; Gao et al., 2023). On the other hand, there are still no established legal limits for alcoholic beverages. However, in the case of wine, it is recommended that the concentrations of histamine and tyramine do not exceed the ranges of 8 – 20 mg/L and 25-40 mg/L, respectively, because they are the biogenic amines with the greatest toxic effects derived from them. of the vasoactive properties they possess. The recommended ranges must be considered when preparing any fermented drink (Kashyap et al., 2023).
5 Limitations
Importantly, these fermented beverages are still alcoholic beverages. The ethanol during fermentation further reduces the possible final nutrients; therefore, when looking up the nutritional benefits of the different beverages, they are very scarce. There are not enough articles that provide more detailed information on these products’ nutritional composition and tables.
This research article provides updated information to encourage the elaboration of articles involving the nutritional information of traditional fermented beverages.
One of the possible strategies is the implementation of future investigations that could focus on further characterizing the biological and cultural diversity associated with fermented beverages. Some potential approaches would include investigating the diversity of microorganisms present in different types of traditional fermented beverages, documenting the practices, recipes, and traditional knowledge associated with their production, analyzing how sociocultural factors are intertwined with their production and consumption, assessing the potential of these beverages as reservoirs of biological and cultural diversity, and exploring their role in the construction of identities and the transmission of intangible cultural heritage. In addition, future research will focus on ensuring strict control of the parameters involved in fermentation processes, the development of protocols and good manufacturing practices adapted to artisanal production, the design of tools and training to transfer this knowledge to traditional producers, and comparative studies between artisanal and industrial fermented beverages to ensure the effective elimination of any pathogenic microbial load, in order to obtain fermented products that are safe for human consumption. Finally, future research focused on the detailed characterization of the nutritional composition of traditional fermented beverages (exhaustive chemical analysis to determine the profile of nutrients, vitamins, minerals, and bioactive compounds). Another aspect to explore would be the effects of traditional fermented beverages on health and the impact of regular consumption of these products on aspects such as digestive function, the immune system, the lipid profile, and the regulation of blood glucose.
By rigorously addressing these aspects, future research could help ensure that artisanal fermented beverages, with all their cultural and biological value, are also completely safe for human consumption, making it possible to sustainably preserve and promote these age-old traditions. So, this review provides an overview of the production of fermented beverages with particular attention to their geographical distribution and the content of microbial bioactive compounds.
6 Conclusion
This review discovered that corn is Latin America’s most used raw material in traditional fermented beverages. The review also delves into the different fermentation processes used to create these beverages, each based on unique traditions and accessible raw materials. One of the defining characteristics of these drinks is that they are made in their natural surroundings, allowing for a natural fermentation process thanks to fermentative bacteria in the environment. Scientific research has primarily supported the nutritional benefits of fermented beverages from Mexico. However, this review sheds light on the need for more nutritional information on other traditional fermented beverages, which could be a starting point for further research. Lastly, it is important to note that chicha is the primary ancestral drink that uses corn as its primary raw material and is prepared in various ways.
To ensure the safety of fermented beverages, researchers are studying the microbiological risks associated with food insecurity and determining the maximum allowed levels of biogenic amines. It’s important to incorporate new technologies to maintain the safety and suitability of these popular drinks, commonly consumed by Latin American ancestral societies. Establishing safety protocols during the preparation of non-alcoholic fermented products is crucial to guarantee the safety of consumers.
Author contributions
CL: Visualization, Writing – review & editing, Investigation, Writing – original draft. FG: Investigation, Writing – original draft, Writing – review & editing. WV: Investigation, Visualization, Writing – review & editing, Funding acquisition, Software, Supervision. MG: Funding acquisition, Supervision, Writing – review & editing. AO-M: Supervision, Writing – review & editing, Conceptualization, Methodology, Project administration, Visualization.
Funding
The author(s) declare financial support was received for the research, authorship, and/or publication of this article. This research was funded by the Presidency of the Council of Ministers of the Republic of Italy through the project “Mikuna Kawsana - Comer es vivir”, allocated to the Entidad Nacional Giuseppini De Murialdo (ENGIM).
Acknowledgments
The author thanks ESPOL-University, VLIR-UOS funding, and Universidad Regional Amazónica Ikiam University’s support.
Conflict of interest
The authors declare that the research was conducted in the absence of any commercial or financial relationships that could be construed as a potential conflict of interest.
Publisher’s note
All claims expressed in this article are solely those of the authors and do not necessarily represent those of their affiliated organizations, or those of the publisher, the editors and the reviewers. Any product that may be evaluated in this article, or claim that may be made by its manufacturer, is not guaranteed or endorsed by the publisher.
Abbreviations
BA, Biogenic amines; MAO, Monoamine oxidase; DAO, Diamine oxidase; PAO, Polyamine oxidase; LAB, Lactic acid bacteria; AAB, Acetic acid bacteria; LC, Liquid chromatography; UPLC, Ultra-high performance liquid chromatography; HPLC, High-Performance Liquid Chromatography; FAO, Food and Agriculture Organization; WFS, World Food Summit; LAC, Latin America, and the Caribbean.
References
Adebo, O. A., and Medina-Meza, I. G. (2020). Impact of fermentation on the phenolic compounds and antioxidant activity of whole cereal grains: a Mini review. Molecules 25:927. doi: 10.3390/molecules25040927
Aidoo, K. E., Rob Nout, M. J., and Sarkar, P. K. (2006). Occurrence and function of yeasts in Asian indigenous fermented foods. FEMS Yeast Res. 6, 30–39. doi: 10.1111/j.1567-1364.2005.00015.x
Albergaria, H., and Arneborg, N. (2016). Dominance of Saccharomyces cerevisiae in alcoholic fermentation processes: role of physiological fitness and microbial interactions. Appl. Microbiol. Biotechnol. 100, 2035–2046. doi: 10.1007/s00253-015-7255-0
Albergaria, H., Francisco, D., Gori, K., Arneborg, N., and Gírio, F. (2010). Saccharomyces cerevisiae CCMI 885 secretes peptides that inhibit the growth of some non-Saccharomyces wine-related strains. Appl. Microbiol. Biotechnol. 86, 965–972. doi: 10.1007/s00253-009-2409-6
Alvarez, M. A., and Moreno-Arribas, M. V. (2014). The problem of biogenic amines in fermented foods and the use of potential biogenic amine-degrading microorganisms as a solution. Trends Food Sci. Technol. 39, 146–155. doi: 10.1016/j.tifs.2014.07.007
Amigo, L., and Hernández-Ledesma, B. (2020). Current evidence on the bioavailability of food bioactive peptides. Molecules 25:4479. doi: 10.3390/molecules25194479
Ana Lúcia Barretto Penna, LAN. (2016). Fermented foods and beverages from cassava (Manihot esculenta Crantz) in South America. Available at: https://www.researchgate.net/publication/314827193_Fermented_Foods_and_Beverages_from_Cassava_Manihot_esculenta_Crantz_in_South_America
Andrés-Barrao, C., Benagli, C., Chappuis, M., Ortega Pérez, R., Tonolla, M., and Barja, F. (2013). Rapid identification of acetic acid bacteria using MALDI-TOF mass spectrometry fingerprinting. Syst. Appl. Microbiol. 36, 75–81. doi: 10.1016/j.syapm.2012.09.002
Aro, S. (2010). Improvement in the nutritive quality of cassava and its by-products through microbial fermentation. Afr. J. Biotechnol. 7, 4789–4797.
Badotti, F., Belloch, C., Rosa, C. A., Barrio, E., and Querol, A. (2010). Physiological and molecular characterisation of Saccharomyces cerevisiae cachaça strains isolated from different geographic regions in Brazil. World J. Microbiol. Biotechnol. 26, 579–587. doi: 10.1007/s11274-009-0206-0
Barbieri, F., Montanari, C., Gardini, F., and Tabanelli, G. (2019). Biogenic amine production by lactic acid Bacteria: a review. Food Secur. 8:17. doi: 10.3390/foods8010017
Bassi, D., Orrù, L., Cabanillas Vasquez, J., Cocconcelli, P. S., and Fontana, C. (2020). Peruvian chicha: a focus on the microbial populations of this ancient maize-based fermented beverage. Microorganisms 8:93. doi: 10.3390/microorganisms8010093
Ben, O. N., Ampe, F., Raimbault, M., Guyot, J. P., and Tailliez, P. (2000). Molecular diversity of lactic acid bacteria from cassava sour starch (Colombia). Syst. Appl. Microbiol. 23, 285–291. doi: 10.1016/S0723-2020(00)80016-8
Bintsis, T., and Papademas, P. (2022). The evolution of fermented milks, from artisanal to industrial products: a critical review. Fermentation 8:679. doi: 10.3390/fermentation8120679
Bott, A., Maimouni, S., and Zong, W. X. (2019). The pleiotropic effects of glutamine metabolism in Cancer. Cancers (Basel) 11:770. doi: 10.3390/cancers11060770
Brune, M., Rossander, L., and Hallberg, L. (1989). Iron absorption and phenolic compounds: importance of different phenolic structures. Eur. J. Clin. Nutr. 43, 547–557.
Cachaldora, A., Fonseca, S., Franco, I., and Carballo, J. (2013). Technological and safety characteristics of Staphylococcaceae isolated from Spanish traditional dry-cured sausages. Food Microbiol. 33, 61–68. doi: 10.1016/j.fm.2012.08.013
Cano, A., and Arnao, M. B. (2004). Actividad antioxidante hidrofílica y lipofílica y contenido en vitamina C de zumos de naranja comerciales: relación con sus características organolépticas. Ciencia y Tecnología Alimentaria 4, 185–189. doi: 10.1080/11358120409487759
Caplice, E., and Fitzgerald, G. F. (1999). Food fermentations: role of microorganisms in food production and preservation. Int. J. Food Microbiol. 50, 131–149.
Capozzi, V., Russo, P., Dueñas, M. T., López, P., and Spano, G. (2012). Lactic acid bacteria producing B-group vitamins: a great potential for functional cereals products. Appl. Microbiol. Biotechnol. 96, 1383–1394. doi: 10.1007/s00253-012-4440-2
Capredon, É. (2018). El encuentro de Iglesias evangélicas indígenas con Iglesias «nacionales» y sus repercusiones político-religiosas en el Alto Río Negro (Amazonas, Brasil). Bulletin de l’Institut français d’études andines 47, 227–246.
Carboni, A. D., Martins, G. N., Gómez-Zavaglia, A., and Castilho, P. C. (2023). Lactic acid Bacteria in the production of traditional fermented foods and beverages of Latin America. Fermentation 9:315. doi: 10.3390/fermentation9040315
Castañeda-Ovando, A., Moreno-Vilet, L., Jaimez-Ordaz, J., Ramírez-Godínez, J., Pérez-Escalante, E., Cruz-Guerrero, A. E., et al. (2023). Aguamiel syrup as a technological diversification product: composition, bioactivity and present panorama. Future Foods 8:100249. doi: 10.1016/j.fufo.2023.100249
Castilla Corzo, F, Burbano Argoti, CA, and Salazar, Duque D. (2019). La chicha, producto gastronómico y ritual: caso Chorro de Quevedo (Colombia) y Otavalo (Ecuador). Turismo y Sociedad. 26, 205–224. doi: 10.18601/01207555.n26.09
Chacón Mayorga, G. A., Arias Palma, G. B., Sandoval-Cañas, G. J., and Ordoñez-Araque, R. H. (2020). Ancestral fermented indigenous beverages from South America made from cassava (Manihot esculenta). Food Sci. Technol. 41, 360–367. doi: 10.1590/fst.15220
Chaves-López, C., Rossi, C., Maggio, F., Paparella, A., and Serio, A. (2020). Changes occurring in spontaneous maize fermentation: an overview. Fermentation 6:36. doi: 10.3390/fermentation6010036
Colehour, A. M., Meadow, J. F., Liebert, M. A., Cepon-Robins, T. J., Gildner, T. E., Urlacher, S. S., et al. (2014). Local domestication of lactic acid bacteria via cassava beer fermentation. PeerJ 2014:e479. doi: 10.7717/peerj.479
Corzo, F. C., Argoti, C. A. B., and Duque, D. A. S. (2019). La chicha, producto gastronómico y ritual: caso Chorro de Quevedo (Colombia) y Otavalo (Ecuador). Turismo y Sociedad 26, 205–224. doi: 10.18601/01207555.n26.09
Cossu, M., Pintus, R., Zaffanello, M., Mussap, M., Serra, F., Marcialis, M. A., et al. (2023). Metabolomic studies in inborn errors of metabolism: last years and future perspectives. Meta 13:447. doi: 10.3390/metabo13030447
Costa, M. P., Balthazar, C. F., Rodrigues, B. L., Lazaro, C. A., Silva, A. C. O., Cruz, A. G., et al. (2015). Determination of biogenic amines by high-performance liquid chromatography (HPLC - DAD) in probiotic cow’s and goat’s fermented milks and acceptance. Food Sci. Nutr. 3, 172–178. doi: 10.1002/fsn3.200
Costa, R. D. S. d, Rodrigues, A. M. d. C., and Silva, L. H. M. d. (2022). The fruit of peach palm (Bactris gasipaes) and its technological potential: an overview. Food Sci. Technol. 42:e82721. doi: 10.1590/fst.82721
Cuamatzin-garcía, L., Rodríguez-rugarcía, P., El-kassis, E. G., Galicia, G., Meza-jiménez, M.d. L., Baños-lara, M. D. R., et al. (2022). Traditional fermented foods and beverages from around the world and their health benefits. Microorganisms 10:1151. doi: 10.3390/microorganisms10061151
de Cássia, S. K., Corrêa, R. C. G., Uber, T. M., Barros, L., Ferreira, I. C. F. R., Peralta, R. A., et al. (2022). Full exploitation of peach palm (Bactris gasipaes Kunth): state of the art and perspectives. Plants 11:3175. doi: 10.3390/plants11223175
de la Fuente-Salcido, N. M., Castañeda-Ramírez, J. C., García-Almendárez, B. E., Bideshi, D. K., Salcedo-Hernández, R., and Barboza-Corona, J. E. (2015). Isolation and characterization of bacteriocinogenic lactic bacteria from M-tuba and tepache, two traditional fermented beverages in México. Food Sci. Nutr. 3, 434–442. doi: 10.1002/fsn3.236
Debeer, J., Bell, J. W., Nolte, F., Arcieri, J., and Correa, G. (2021). Histamine limits by country: a survey and review. J. Food Prot. 84, 1610–1628. doi: 10.4315/JFP-21-129
Durak-Dados, A, Michalski, M., and Osek, J. (2020). Histamine and other biogenic amines in food. J. Vet. Res. 64, 281–288. doi: 10.2478/jvetres-2020-0029
El-Gendy, S. M. (1983). Fermented foods of Egypt and the Middle East. J. Food Prot. 46, 358–367. doi: 10.4315/0362-028X-46.4.358
Elizaquível, P., Pérez-Cataluña, A., Yépez, A., Aristimuño, C., Jiménez, E., Cocconcelli, P. S., et al. (2015). Pyrosequencing vs. culture-dependent approaches to analyze lactic acid bacteria associated to chicha, a traditional maize-based fermented beverage from northwestern Argentina. Int. J. Food Microbiol. 198, 9–18. doi: 10.1016/j.ijfoodmicro.2014.12.027
El-Sayed, A. S., Ibrahim, H., and Farag, M. A. (2022). Detection of potential microbial contaminants and their toxins in fermented dairy products: a comprehensive review. Food Anal. Methods 15, 1880–1898. doi: 10.1007/s12161-022-02253-y
Emperaire, L. (2023). Les maniocs et les autres: éléments pour une histoire souterraine des plantes cultivées en Amazonie du nord-ouest. Revue d’Ethnoécologie 23:35. doi: 10.4000/ethnoecologie.10153
Erdag, D., Merhan, O., and Yildiz, B. (2019). Biochemical and pharmacological properties of biogenic amines. Biogenic Amines. IntechOpen. doi: 10.5772/intechopen.81569
Escalante, A., López Soto, D. R., Velázquez Gutiérrez, J. E., Giles-Gómez, M., Bolívar, F., and López-Munguía, A. (2016). Pulque, a traditional Mexican alcoholic fermented beverage: historical, microbiological, and technical aspects. Front. Microbiol. 7:204241. doi: 10.3389/fmicb.2016.01026
Fayyaz, K., Nawaz, A., Olaimat, A. N., Akram, K., Farooq, U., Fatima, M., et al. (2022). Microbial toxins in fermented foods: health implications and analytical techniques for detection. J. Food Drug Anal. 30:523. doi: 10.38212/2224-6614.3431
Fernandes, T. (2018). Fermented food guidelines for children. J. Pediatr. Pediatr. Med. 2, 1–4. doi: 10.29245/2578-2940/2018/1.1111
Fiechter, A., and Seghezzi, W. (1992). Regulation of glucose metabolism in growing yeast cells. J. Biotechnol. 27, 27–45. doi: 10.1016/0168-1656(92)90028-8
Figueroa, L. M., Santos-Zea, L., Escalante, A., and Gutiérrez‐Uribe, J. A. (2017). Mass spectrometry-based metabolomics of agave sap (agave salmiana) after its inoculation with microorganisms isolated from agave sap concentrate selected to enhance anticancer activity. Sustainability 9:2095. doi: 10.3390/su9112095
Flores-Gallegos, A. C., Vázquez-Vuelvas, O. F., López-López, L. L., Sainz-Galindo, A., Ascacio-Valdes, J. A., Aguilar, C. N., et al. (2019). “Tuba, a fermented and refreshing beverage from coconut palm sap,” in Non-alcoholic beverages. Woodhead Publishing, 163–184.
Florez Duque, A. V., Moreno Arango, M. A., and Franco Tobón, Y. N. (2023). Aminas Biógenas encontradas en carnes, pescado y productos cárnicos. Hechos Microbiológicos. 14, 26–44. doi: 10.17533/udea.hm.v14n1a04
Food and Agriculture Organization of the United Nations. (1996). Rome declaration on world food security and world food summit plan of action: World food summit, 13-17 November 1996. Rome, Italy. 43. Available at: https://digitallibrary.un.org/record/195568
Freire, A. L., Zapata, S., Mosquera, J., Mejia, M. L., and Trueba, G. (2016). Bacteria associated with human saliva are major microbial components of Ecuadorian indigenous beers (chicha). PeerJ 2016:e1962
Gao, X., Li, C., He, R., Zhang, Y., Wang, B., Zhang, Z. H., et al. (2023). Research advances on biogenic amines in traditional fermented foods: emphasis on formation mechanism, detection and control methods. Food Chem. 405:134911. doi: 10.1016/j.foodchem.2022.134911
Gargantilla, P., Arroyo, N., Montero, J., and Montero, G. (2016). Escombroidosis: causa frecuente de intoxicación alimentaria. SEMERGEN - Medicina de Familia. 42:353. doi: 10.1016/j.semerg.2015.05.003
Geamanu, A., Gupta, S. V., Bauerfeld, C., and Samavati, L. (2016). Metabolomics connects aberrant bioenergetic, transmethylation, and gut microbiota in sarcoidosis. Metabolomics 12:35. doi: 10.1007/s11306-015-0932-2
Gibbons, K. L., Conn, B. J., and Henwood, M. J. (2015). Status of names of mitrasacme species occurring outside australia. Telopea 18, 495–502. doi: 10.7751/telopea9036
Giovannetti, M. A. (2021). Chicha and food for the inka feasts: their materiality in state production contexts in southern tawantinsuyu. J. Anthropol. Archaeol. 62:101279. doi: 10.1016/j.jaa.2021.101279
Giuffrè, D., and Giuffrè, A. M. (2024). Fermentation technology and functional foods. Front. Biosci. Elite 16:8. doi: 10.31083/j.fbe1601008
Gomes, R. J. (2018). Acetic acid Bacteria in the food industry: systematics, characteristics and applications. Food Technol. Biotechnol. 56:139. doi: 10.17113/ftb.56.02.18.5593
Gómez-Rave, N. A., Gil Uribe, M. A., Luján-Roldán, B. D., López-Cadena, Y. S., Marín-Pareja, N., and Valencia-García, F. E. (2022). Peligros microbiológicos relacionados con alimentos fermentados de elaboración artesanal comercializados en tunja, nariño y cauca. Revista Colombiana De Biotecnología 24, 4–15. doi: 10.15446/rev.colomb.biote.v24n2.97013
González-Jaramillo, N., Bailon-Moscoso, N., Duarte-Casar, R., and Romero-Benavides, J. C. (2022). Peach palm (Bactris gasipaes Kunth.): ancestral tropical staple with future potential. Plan. Theory 11:3134. doi: 10.3390/plants11223134
Gregorio, CGC. (2023). Philippine traditional alcoholic beverages: a germinal study. 1–30. doi: 10.1007/978-3-031-04195-2_188-1
Grijalva-Vallejos, N., Krogerus, K., Nikulin, J., Magalhães, F., Aranda, A., Matallana, E., et al. (2021). Potential application of yeasts from Ecuadorian chichas in controlled beer and chicha production. Food Microbiol. 98:103644. doi: 10.1016/j.fm.2020.103644
Guerra, L. S., Cevallos-Cevallos, J. M., Weckx, S., and Ruales, J. (2022). Traditional fermented foods from Ecuador: a review with a focus on microbial diversity. Food Secur. 11:1854. doi: 10.3390/foods11131854
Gutiérrez-Sarmiento, W., Peña-Ocaña, B. A., Lam-Gutiérrez, A., Guzmán-Albores, J. M., Jasso-Chávez, R., and Ruíz-Valdiviezo, V. M. (2022). Microbial community structure, physicochemical characteristics and predictive functionalities of the Mexican tepache fermented beverage. Microbiol. Res. 260:127045. doi: 10.1016/j.micres.2022.127045
Gutiérrez-Uribe, J. A., Figueroa, L. M., Martín-del-Campo, S. T., and Escalante, A. (2017). Pulque. Ferment. Foods Health Dis. Prevent. 543–556. doi: 10.1016/B978-0-12-802309-9.00023-6
Håkanson, R., Lilja, B., Owman, C., and Thunell, S. (1967). Changes in gastric secretion induced by certain amines, amine precursors and related enzyme inhibitors. Eur. J. Pharmacol. 1, 425–433. doi: 10.1016/0014-2999(67)90105-7
Heurich, G. O. (2015). Outras Alegrias: Cachaça E Cauim Na Embriaguez Mbyá-Guarani. Mana 21, 527–552. doi: 10.1590/0104-93132015v21n3p300
Hiroshi, S. H., Guemra, S., Bosso, A., de Pádua, A. É., and Rodrigo Ito, M. L. (2020). Reducción de proteínas y glucosa por reacción de Maillard en leche con lactosa hidrolisada. Revista chilena de nutrición. 47, 14–21. doi: 10.4067/S0717-75182020000100014
Insfran-Rivarola, A., Tlapa, D., Limon-Romero, J., Baez-Lopez, Y., Miranda-Ackerman, M., Arredondo-Soto, K., et al. (2020). A systematic review and Meta-analysis of the effects of food safety and hygiene training on food handlers. Foods 9:1169. doi: 10.3390/foods9091169
Jimenez, M. E., O’Donovan, C. M., de Ullivarri, M. F., and Cotter, P. D. (2022). Microorganisms present in artisanal fermented food from South America. Front. Microbiol. 13:941866. doi: 10.3389/fmicb.2022.941866
Jolly, É. (2007). Erikson Philippe (éd.), La pirogue ivre. Bières traditionnelles en Amazonie, Musée français de la Brasserie, Saint-Nicolas de Port, 2004, 140 p., ill.(ouvrage édité et publié avec le concours de l’université de Paris X-Nanterre, de l’UMR 7535 et de l’UPR 324; 2e édition: 2006). Journal de la société des américanistes, 92. doi: 10.4000/jsa.7403
Kahraman-Ilıkkan, Ö. (2024). Comparative genomics of four lactic acid bacteria identified with Vitek MS (MALDI-TOF) and whole-genome sequencing. Mol. Gen. Genomics. 299:31. doi: 10.1007/s00438-024-02129-2
Kashyap, S., Tehri, N., Verma, N., Gahlaut, A., and Hooda, V. (2023). Recent advances in development of electrochemical biosensors for the detection of biogenic amines. 3 Biotech 13:2. doi: 10.1007/s13205-022-03414-w
Komesu, A., Oliveira, J. A. R. d., Martins, L. H. d. S., Maciel, M. R. W., and Filho, R. M. (2017). Lactic acid production to purification: a review. BioResources 12, 4364–4383. doi: 10.15376/biores.12.2.4364-4383
Krause, J., and Fleischer, O. (eds.). (2010). Industrial fermentation: Food processes, nutrient sources and production strategies. Nova Science Publishers, 217-247. Available at: https://www.researchgate.net/publication/264524557_Fermentation_Strategies_Nutritional_Requirement
Lacerda, I. C. A., Miranda, R. L., Borelli, B. M., Nunes, Á. C., Nardi, R. M. D., Lachance, M. A., et al. (2005). Lactic acid bacteria and yeasts associated with spontaneous fermentations during the production of sour cassava starch in Brazil. Int. J. Food Microbiol. 105, 213–219.
Landete, J. M. (2007). Molecular methods for the detection of biogenic amine-producing bacteria on foods. Int. J. Food Microbiol. 117, 258–269. doi: 10.1016/j.ijfoodmicro.2007.05.001 de las Rivas B, Marcobal A, Muñoz R
Lao, F., Sigurdson, G. T., and Giusti, M. M. (2017). Health benefits of purple corn (Zea mays L.) phenolic compounds. Compr. Rev. Food Sci. Food Saf. 16, 234–246. doi: 10.1111/1541-4337.12249
Leal-Díaz, A. M., Santos-Zea, L., Martínez-Escobedo, H. C., Guajardo-Flores, D., Gutiérrez-Uribe, J. A., and Serna-Saldivar, S. O. (2015). Effect of Agave Americana and Agave salmiana ripeness on Saponin content from Aguamiel (Agave sap). J. Agric. Food Chem. 63, 3924–3930. doi: 10.1021/acs.jafc.5b00883
Lee, I. H., and Finkel, T. (2013). Metabolic regulation of the cell cycle. Curr. Opin. Cell Biol. 25, 724–729. doi: 10.1016/j.ceb.2013.07.002
Lee, J., Ji, Y. R., Cho, Y., and Choi, M. J. (2023). Effects of lyoprotectant and encapsulated Lactobacillus acidophilus KBL409 on freeze-drying and storage stability. LWT 182:114846. doi: 10.1016/j.lwt.2023.114846
Liburdi, K, Bernini, R, and Esti, M. (2020). Fermented beverages: geographical distribution and bioactive compounds with health benefits. New and Future Developments in Microbial Biotechnology and Bioengineering. 131–151.
Lima, T. T. M., Hosken, B. O., Venturim, B. C., Lopes, I. L., and JGP, M. (2022). Traditional Brazilian fermented foods: cultural and technological aspects. J. Ethnic Foods 9, 1–15. doi: 10.1186/s42779-022-00153-4
Lopez, M., Peralta-Videa, J., Castillo-Michel, H., Martinez-Martinez, A., Duarte-Gardea, M., and Gardea-Torresdey, J. (2007). Lead toxicity in alfalfa plants exposed to PHYTOHORMONES and ethylenediaminetetraacetic acid monitored by peroxidase, catalase, and amylase activities. Environ. Toxicol. Chem. 26, 2717–2723. doi: 10.1897/07-302.1
Lopez-Martinez, L. X., Oliart-Ros, R. M., Valerio-Alfaro, G., Lee, C. H., Parkin, K. L., and Garcia, H. S. (2009). Antioxidant activity, phenolic compounds and anthocyanins content of eighteen strains of Mexican maize. LWT Food Sci. Technol. 42, 1187–1192. doi: 10.1016/j.lwt.2008.10.010
Ma, X., Wang, Y., Liu, Y., Li, X., Wang, F., Huang, Y., et al. (2024). Mechanisms and factors influencing the ability of lactic acid bacteria on reducing biogenic amines in fermented food: a mini review. LWT 197:115890. doi: 10.1016/j.lwt.2024.115890
Maicas, S. (2020). The role of yeasts in fermentation processes. Microorganisms 8, 1–8. doi: 10.3390/microorganisms8081142
Marcobal, A., de las Rivas, B., and Muñoz, R. (2006). Methods for the detection of Bacteria producing biogenic amines on foods: a survey. J. Verbr. Lebensm. 1, 187–196. doi: 10.1007/s00003-006-0035-0
Martín, B., Garriga, M., Hugas, M., Bover-Cid, S., Veciana-Nogués, M. T., and Aymerich, T. (2006). Molecular, technological and safety characterization of gram-positive catalase-positive cocci from slightly fermented sausages. Int. J. Food Microbiol. 107, 148–158. doi: 10.1016/j.ijfoodmicro.2005.08.024
Mashau, M. E., Maliwichi, L. L., and Jideani, A. I. O. (2021). Non-alcoholic fermentation of maize (Zea mays) in sub-Saharan Africa. Fermentation 7:158. doi: 10.3390/fermentation7030158
Mastrangelo, M., Tolve, M., Artiola, C., Bove, R., Carducci, C., Carducci, C., et al. (2023). Phenotypes and genotypes of inherited disorders of biogenic amine neurotransmitter metabolism. Genes 14:263. doi: 10.3390/genes14020263
McGuire, S.FAOIFADWFP (2015). The state of food insecurity in the world 2015: meeting the 2015 international hunger targets: taking stock of uneven Progress. Rome: FAO, 2015. Adv. Nutr. 6:623. doi: 10.3945/an.115.009936
Mendoza, L. M., Neef, A., Vignolo, G., and Belloch, C. (2017). Yeast diversity during the fermentation of Andean chicha: a comparison of high-throughput sequencing and culture-dependent approaches. Food Microbiol. 67, 1–10. doi: 10.1016/j.fm.2017.05.007
Miguel, M. G. C. P., Collela, C. F., de Almeida, E. G., Dias, D. R., and Schwan, R. F. (2015). Physicochemical and microbiological description of Caxiri – a cassava and corn alcoholic beverage. Int. J. Food Sci. Technol. 50, 2537–2544. doi: 10.1111/ijfs.12921
Moher, D., Liberati, A., Tetzlaff, J., and Altman, D. G. (2009). Preferred reporting items for systematic reviews and meta-analyses: the PRISMA statement. BMJ 339, 332–336. doi: 10.1371/journal.pmed.1000097
Mohidin, S. R. N. S. P., Moshawih, S., Hermansyah, A., Asmuni, M. I., Shafqat, N., and Ming, L. C. (2023). Cassava (Manihot esculenta Crantz): a systematic review for the pharmacological activities, traditional uses, nutritional values, and Phytochemistry. J. Evid. Based Integr. Med. 28. doi: 10.1177/2515690X231206227
Moy, G. G., and Todd, E. C. D. (2014). Foodborne diseases: overview of chemical, physical, and other significant hazards. Encyclopedia of Food Safety 243–252. doi: 10.1016/b978-0-12-378612-8.00418-2
Mukherjee, A., Gómez-Sala, B., O’Connor, E. M., Kenny, J. G., and Cotter, P. D. (2022). Global regulatory frameworks for fermented foods: a review. Front. Nutr. 9:902642. doi: 10.3389/fnut.2022.902642
Munir, M. A., Badri, K. H., Heng, L. Y., and Ibrahim, S. (2020). Biogenic amines detection by chromatography and sensor methods: a comparative review. Sci. Technol. Indonesia 5, 90–110. doi: 10.26554/sti.2020.5.4.90-110
Munir, M. A., Badri, K. H., Heng, L. Y., Mackeen, M. M. M., and Sage, E. E. (2021). Histamine detection in mackerel (Scomberomorus Sp.) and its products Derivatized with 9-Flourenilmethylchloroformate. Pak. J. Anal. Environ. Chem. 22, 243–251. doi: 10.21743/pjaec/2021.12.03
Munir, M. A., Jamal, J. A., Said, M. M., Ibrahim, S., and Ahmad, M. S. (2023). Polyurethane application to transform screen-printed electrode for rapid identification of histamine isolated from fish. Scientifica 2023, 1–10. doi: 10.1155/2023/5444256
Muñoz, R., and Moreno-Arribas, M. V. (2011). Lactic acid Bacteria. Molecular Wine Microbiol. 191–226. doi: 10.1016/B978-0-12-375021-1.10008-6
Muñoz-Bernal, O. A., Coria-Oliveros, A. J., Vazquez-Flores, A. A., de la Rosa, L. A., Núñez-Gastélum, J. A., Rodrigo-García, J., et al. (2020). Evolution of phenolic content, antioxidant capacity and phenolic profile during cold pre-fermentative maceration and subsequent fermentation of cabernet sauvignon red wine. South Afri. J. Enol. Viticul. 41, 72–82. doi: 10.21548/41-1-3778
Naila, A., Flint, S., Fletcher, G., Bremer, P., and Meerdink, G. (2010). Control of biogenic amines in food—existing and emerging approaches. J. Food Sci. 75, R139–R150. doi: 10.1111/j.1750-3841.2010.01774.x
Narváez-Zapata, J. A., and Sánchez-Teyer, L. F. (2009). Agaves as a raw material: recent technologies and applications. Recent Pat. Biotechnol. 3, 185–191. doi: 10.2174/187220809789389144
Narvhus, J. A., and Axelsson, L. (2003). Lactic Acid Bacteria. Encycl. Food Sci. Nutr., 3465–3472. doi: 10.1016/B0-12-227055-X/00673-8
Naupert, C. (2019). Misión y traducción en el Brasil colonial: los misioneros jesuitas como mediadores lingüísticos y culturales. CLINA 5:63. doi: 10.14201/clina2019516378
Navarrete-Bolaños, J. L. (2012). Improving traditional fermented beverages: how to evolve from spontaneous to directed fermentation. Eng Life Sci 12, 410–418. doi: 10.1002/elsc.201100128
Olveira, G., and González-Molero, I. (2016). Actualización de probióticos, prebióticos y simbióticos en nutrición clínica. Endocrinol. Nutr. 63, 482–494. doi: 10.1016/j.endonu.2016.07.006
Önal, A. (2007). A review: current analytical methods for the determination of biogenic amines in foods. Food Chem. 103, 1475–1486. doi: 10.1016/j.foodchem.2006.08.028
Ordóñez, J. L., Troncoso, A. M., García-Parrilla, M. D. C., and Callejón, R. M. (2016). Recent trends in the determination of biogenic amines in fermented beverages - a review. Anal. Chim. Acta. 939, 10–25. doi: 10.1016/j.aca.2016.07.045
Ortiz-Basurto, R. I., Pourcelly, G., Doco, T., Williams, P., Dormer, M., and Belleville, M. P. (2008). Analysis of the Main components of the Aguamiel produced by the maguey-Pulquero (Agave mapisaga) throughout the harvest period. J. Agric. Food Chem. 56, 3682–3687. doi: 10.1021/jf072767h
Panda, S. K., and Ray, R. C. (2016). Fermented foods and beverages from tropical roots and tubers. Tropical roots and tubers: production. Proces. Technol. 225–252. doi: 10.1007/978-3-319-19018-1_11
Pandiselvam, R., Akshay, A. T., Manikantan, M. R., Ramesh, S. V., Patil, H., and Gopal, M. (2023). Synergetic effect of fermented coconut inflorescence sap for the production of virgin coconut oil. J. Food Biochem. 2023, 1–12. doi: 10.1155/2023/7125810
Pazmiño, D., Escudero, M., and Grijalva, N. (2014). Diversidad microbiana asociada a la chicha de arroz: una bebida tradicional de Bolívar - Ecuador. Enfoque UTE. 5, 1–14. doi: 10.29019/enfoqueute.v5n3.40
Peregrín-Alvarez, J. M., Sanford, C., and Parkinson, J. (2009). The conservation and evolutionary modularity of metabolism. Genome Biol. 10:R63. doi: 10.1186/gb-2009-10-6-r63
Pérez-Armendáriz, B., and Cardoso-Ugarte, G. A. (2020). Traditional fermented beverages in Mexico: biotechnological, nutritional, and functional approaches. Food Res. Int. 136:109307. doi: 10.1016/j.foodres.2020.109307
Pérez-Martín, F., Seseña, S., Izquierdo, P. M., and Palop, M. L. (2014). Are Enterococcus populations present during malolactic fermentation of red wine safe? Food Microbiol. 42, 95–101. doi: 10.1016/j.fm.2014.03.001
Periche Pérez, J. (2018). Contenido de aminas biógenas (histamina y tiramina) y su relación con características fisicoquímicas en muestras de chicha de jora elaboradas en la provincia de Abancay, Apurímac. Industrial Data. 21, 35–45.
Pinheiro, R. C., Ballesteros, L. F., Cerqueira, M. A., Rodrigues, A. M. C., Teixeira, J. A., and Silva, L. H. M. (2022). Peach palm (Bactris gasipaes Kunth) and mammee apple (Mammea americana L.) seeds: properties and potential of application in industry. LWT 170:114089. doi: 10.1016/j.lwt.2022.114089
Punia Bangar, S., Suri, S., Trif, M., and Ozogul, F. (2022). Organic acids production from lactic acid bacteria: a preservation approach. Food Biosci. 46:101615. doi: 10.1016/j.fbio.2022.101615
Ramírez-Guzmán, K. N., Torres-León, C., Martinez-Medina, G. A., de la Rosa, O., Hernández-Almanza, A., Alvarez-Perez, O. B., et al. (2019). “Traditional fermented beverages in Mexico” in Fermented Beverages (Elsevier), 605–635.
Ramos Guerrero, F. G., López Flores, B. C., Ramos Gorbeña, J. C., and Silva Jaimes, M. I. (2021). Factors that affect the microbiological stability of Chicha Morada during its production on an industrial scale: a review. J. Food Prot. 84, 2151–2158. doi: 10.4315/JFP-21-190
Rebaza-Cardenas, T. D., Silva-Cajaleón, K., Sabater, C., Delgado, S., Montes-Villanueva, N. D., and Ruas-Madiedo, P. (2021). “Masato de Yuca” and “Chicha de Siete Semillas” two traditional vegetable fermented beverages from Peru as source for the isolation of potential probiotic Bacteria. Probiotics Antimicrob. Proteins 15, 300–311. doi: 10.1007/s12602-021-09836-x
Regional Overview of Food Security and Nutrition - Latin America and the Caribbean 2022 [Internet]. (2023). PAHO/WHO | Pan American Health Organization. Available from: https://www.paho.org/en/documents/regional-overview-food-security-and-nutrition-latin-america-and-caribbean-2022
Resende, L. V., Pinheiro, L. K., Miguel, M. G. C. P., Ramos, C. L., Vilela, D. M., and Schwan, R. F. (2018). Microbial community and physicochemical dynamics during the production of ‘Chicha’, a traditional beverage of indigenous people of Brazil. World J. Microbiol. Biotechnol. 34, 1–11. doi: 10.1007/s11274-018-2429-4
Robalino Vallejo, J. A., Moreno Guerra, A. M., Zurita Gallegos, R. M., and Peralta Saá, L. O. (2020). Memorias culinarias y representaciones tradicionales caracterizadas en la cultura ecuatoriana. Horizontes Revista de Investigación en Ciencias de la Educación. 4, 613–633. doi: 10.33996/revistahorizontes.v4i16.141
Rocha-Arriaga, C., Espinal-Centeno, A., Martinez-Sánchez, S., Caballero-Pérez, J., Alcaraz, L. D., and Cruz-Ramírez, A. (2020). Deep microbial community profiling along the fermentation process of pulque, a biocultural resource of Mexico. Microbiol. Res. 241:126593. doi: 10.1016/j.micres.2020.126593
Salvador-Reyes, R., and Clerici, M. T. P. S. (2020). Peruvian Andean maize: general characteristics, nutritional properties, bioactive compounds, and culinary uses. Food Res. Int. 130:108934. doi: 10.1016/j.foodres.2019.108934
Santos-Zea, L., Leal-Diaz, A., Cortes-Ceballos, E., and Gutierrez-Uribe, J. (2012). Agave (Agave spp.) and its traditional products as a source of bioactive compounds. Curr Bioact Compd. 8, 218–231. doi: 10.2174/157340712802762410
Schwan, R. F., Almeida, E. G., Souza-Dias, M. A. G., and Jespersen, L. (2007). Yeast diversity in riceâ cassava fermentations produced by the indigenous Tapirapé people of Brazil. FEMS Yeast Res. 7, 966–972. doi: 10.1111/j.1567-1364.2007.00241.x
Schwan, RF, Ramos, CL, de Almeida, EG, Alves, VF, and De Martinis, ECP. (2017). Brazilian Indigenous Fermented Food: Abstract. Fermented Foods of Latin America. 224–236. Available at: https://www.taylorfrancis.com/chapters/edit/10.1201/9781315369433-14/brazilian-indigenous-fermented-food-rosane-freitas-schwan-cintia-lacerda-ramos-euziclei-gonzaga-de-almeida-virg%C3%ADnia-farias-alves-elaine-cristina-pereira-de-martinis
Sentellas, S., Núñez, Ó., and Saurina, J. (2016). Recent advances in the determination of biogenic amines in food samples by (U) HPLC. J. Agric. Food Chem. 64, 7667–7678. doi: 10.1021/acs.jafc.6b02789
Serna-Saldívar, S. O., Gutiérrez-Uribe, J. A., Mora-Rochin, S., and García-Lara, S. (2013). Nutraceutical potential of native maize and changes during traditional and extrusion processing. Revista fitotecnia mexicana 36, 295–304. doi: 10.35196/rfm.2013.3-S3-A.295
Shimoji, K., Isono, E., and Bakke, M. (2020). Modified enzymatic assays for the determination of histamine in fermented foods. J. Food Prot. 83, 1430–1437. doi: 10.4315/JFP-20-082
Siddiqui, S. A., Erol, Z., Rugji, J., Taşçı, F., Kahraman, H. A., Toppi, V, et al. (2023). An overview of fermentation in the food industry-looking back from a new perspective. Bioresour. bioprocess 10:85. doi: 10.1186/s40643-023-00702-y
Silos-Espino, H., González-Cortés, N., Carrillo-López, A., Guevara-Lara, F., Valverde-González, M. E., and Paredes-López, O. (2007). Chemical composition and in vitro propagation of Agave salmiana ‘gentry’. J. Hortic. Sci. Biotechnol. 82, 355–359. doi: 10.1080/14620316.2007.11512242
Sirocchi, V., Caprioli, G., Cecchini, C., Coman, M. M., Cresci, A., Maggi, F., et al. (2013). Biogenic amines as freshness index of meat wrapped in a new active packaging system formulated with essential oils of Rosmarinus officinalis. Int. J. Food Sci. Nutr. 64, 921–928. doi: 10.3109/09637486.2013.809706
Tchekessi, C. C. K., Choucounou, O. I., Matha, D. R., Gandeho, G. J., Sachi, S. A. P., Banon, S. J., et al. (2021). Technological and socio-economic study of akandji, a neglected traditional foodstuff made from corn (Zea mays L.) in Benin. Int. J. Biol. Chem. Sci. 15, 1629–1647. doi: 10.4314/ijbcs.v15i4.26
Teller, R. S., Schmidt, R. J., Whitlow, L. W., and Kung, L. (2012). Effect of physical damage to ears of corn before harvest and treatment with various additives on the concentration of mycotoxins, silage fermentation, and aerobic stability of corn silage. J. Dairy Sci. 95, 1428–1436. doi: 10.3168/jds.2011-4610
Thierry, A., Baty, C., Marché, L., Chuat, V., Picard, O., Lortal, S., et al. (2023). Lactofermentation of vegetables: an ancient method of preservation matching new trends. Trends Food Sci. Technol. 139:104112. doi: 10.1016/j.tifs.2023.07.009
Trček, J., and Barja, F. (2015). Updates on quick identification of acetic acid bacteria with a focus on the 16S–23S rRNA gene internal transcribed spacer and the analysis of cell proteins by MALDI-TOF mass spectrometry. Int. J. Food Microbiol. 196, 137–144. doi: 10.1016/j.ijfoodmicro.2014.12.003
Tsafack, P. B., and Tsopmo, A. (2022). Effects of bioactive molecules on the concentration of biogenic amines in foods and biological systems. Heliyon 8:e10456. doi: 10.1016/j.heliyon.2022.e10456
Tumor, N. L. (2019). The formation of amino acid imbalances and metabolic processes in the background of malignant growth: the levels and nature of the interaction. Open Access J. Oncol. and Med. 3. doi: 10.32474/OAJOM.2019.03.000161
Väkeväinen, K., Valderrama, A., Espinosa, J., Centurión, D., Rizo, J., Reyes-Duarte, D., et al. (2018). Characterization of lactic acid bacteria recovered from atole agrio, a traditional Mexican fermented beverage. LWT 1, 109–118. doi: 10.1016/j.lwt.2017.10.004
Valdivieso Solís, D. G., Vargas Escamilla, C. A., Mondragón Contreras, N., Galván Valle, G. A., Gilés-Gómez, M., Bolívar, F., et al. (2021). Sustainable production of Pulque and maguey in Mexico: current situation and perspectives. Front. Sustain. Food Syst. 5:678168. doi: 10.3389/fsufs.2021.678168
Villarreal-Morales, S. L., Montañez-Saenz, J. C., Aguilar-González, C. N., and Rodriguez-Herrera, R. (2018). “Metagenomics of traditional beverages” in Advances in biotechnology for food industry. eds. A. M. Holban and A. M. Grumezescu (Elsevier), 301–326.
Villarreal-Morales, S. L., Muñiz-Márquez, D. B., Michel-Michel, M., González-Montemayor, Á. M., Escobedo-García, S., Salas-Tovar, J. A., et al. (2019). “Aguamiel a fresh beverage from Agave spp. sap with functional properties” in Natural Beverages. eds. A. M. Holban and A. M. Grumezescu (Elsevier), 179–208. doi: 10.1016/B978-0-12-816689-5.00007-9
Visciano, P., and Schirone, M. (2022). Update on biogenic amines in fermented and non-fermented beverages. Food Secur. 11:353. doi: 10.3390/foods11030353
Walker, G. M., and Stewart, G. G. (2016). Saccharomyces cerevisiae in the production of fermented beverages. Beverages 2:30. doi: 10.3390/beverages2040030
Wang, J., and Fung, D. Y. C. (1996). Alkaline-fermented foods: a review with emphasis on Pidan fermentation. Crit. Rev. Microbiol. 22, 101–138. doi: 10.3109/10408419609106457
Wójcik, W., Łukasiewicz, M., and Puppel, K. (2021). Biogenic amines: formation, action and toxicity - a review. J. Sci. Food Agric. 101, 2634–2640. doi: 10.1002/jsfa.10928
Yamada, Y., Yukphan, P., Vu, H. T. L., Muramatsu, Y., Ochaikul, D., and Nakagawa, Y. (2012). Subdivision of the genus Gluconacetobacter Yamada, Hoshino and Ishikawa 1998: the proposal of Komagatabacter gen. Nov., for strains accommodated to the Gluconacetobacter xylinus group in the α-Proteobacteria. Ann. Microbiol. 62, 849–859. doi: 10.1007/s13213-011-0288-4
Ye, M., Luo, J., Zhang, S., Yang, H., Li, Y. Y., and Liu, J. (2020). In-situ ammonia stripping with alkaline fermentation of waste activated sludge to improve short-chain fatty acids production and carbon source availability. Bioresour. Technol. 301:122782. doi: 10.1016/j.biortech.2020.122782
Keywords: fermented bevarages, South and Central America, food safety, nutritional value, native societies
Citation: Lasso García C, German Bermúdez FA, Vanden Berghe W, Gabriela Zurita-Benavides M and Orellana-Manzano A (2024) Fermented beverages among indigenous Latin American societies. Front. Sustain. Food Syst. 8:1390162. doi: 10.3389/fsufs.2024.1390162
Edited by:
Rakesh Bhardwaj, Indian Council of Agricultural Research (ICAR), IndiaReviewed by:
Simardeep Kaur, The ICAR Research Complex for North Eastern Hill Region, IndiaMuhammad Abdurrahman Munir, University of Malaysia Sarawak, Malaysia
Copyright © 2024 Lasso García, German Bermúdez, Vanden Berghe, Gabriela Zurita-Benavides and Orellana-Manzano. This is an open-access article distributed under the terms of the Creative Commons Attribution License (CC BY). The use, distribution or reproduction in other forums is permitted, provided the original author(s) and the copyright owner(s) are credited and that the original publication in this journal is cited, in accordance with accepted academic practice. No use, distribution or reproduction is permitted which does not comply with these terms.
*Correspondence: Andrea Orellana-Manzano, ZW1haWxAdW5pLmVkdQ==; YWtvcmVsbGFAZXNwb2wuZWR1LmVj