- 1Institute of Agricultural Sciences, ETH Zürich, Lindau, Switzerland
- 2Department of Plant and Environmental Sciences, University of Copenhagen, Copenhagen, Denmark
- 3Department of Agricultural, Forest and Food Sciences, University of Turin, Turin, Italy
- 4Department of Agroecology, Aarhus University, Aarhus, Denmark
- 5Department of Plant Sciences, Wageningen University, Wageningen, Netherlands
- 6Department of Green Chemistry and Technology, Ghent University, Ghent, Belgium
- 7Instituto Superior de Agronomia, University of Lisbon, Lisbon, Portugal
- 8School of Environmental and Natural Sciences, Bangor University, Bangor, United Kingdom
- 9National Research Institute for Agriculture, Food and the Environment (INRAE), Paris, France
To meet global food demands sustainably, it is necessary to safeguard finite natural resources and reduce harmful emissions to the environment. Nutrients in biowastes are often not managed appropriately. Instead, they can be recovered, recycled into bio-based fertilizers (BBFs) and reincorporated into food production systems. This review addresses three critical issues for developing and adopting new BBFs, focusing on the European context: (1) BBFs should match the agronomic efficiency of mineral fertilizers. We propose that the agronomic efficiency of BBFs can be increased through pre-treating the inputs in biowaste transformation processes (e.g., anaerobic digestion), chemical treatments of existing BBFs, organo-mineral combinations, and soil placement strategies. (2) Production and use of new BBFs is not free of environmental impacts, and these are influenced by regional conditions. (3) Public perception and end-user preferences play a significant role in the adoption of BBFs. Therefore, it is vital to address the requirements of end-users of BBFs. Our findings indicate that for widespread adoption, BBFs need sufficient and reliable nutrient amounts and crop-adequate ratios, as well as competitive pricing. A key advantage of BBFs over mineral fertilizers is their ability to improve soil fertility. However, farmers also require fertilizers that can be handled and applied with existing machinery and offer the practicality of commercial products. Another important aspect is the willingness of consumers to buy products fertilized with BBFs. Designing and promoting BBFs requires a careful assessment of environmental impacts and regional conditions, as the sustainability of BBFs depends on factors like energy sources and biowaste transport distances. Ultimately, the goal is to promote a circular economy and not just to substitute mineral fertilizers with new products. This review aims to guide researchers, policymakers, and stakeholders by highlighting key innovations and addressing critical barriers.
1 Introduction
An increase in agricultural productivity is necessary to properly feed a growing human population. However, improving the sustainability of global food production is also a priority. Agriculture is responsible for 17 % of global greenhouse gas (GHG) emissions (FAO, 2021). Additionally, current agriculture significantly contributes to the degradation of ecosystems and causes the depletion of freshwater and nutrient reserves (Foley et al., 2011; Poore and Nemecek, 2018). Making agriculture more sustainable can be achieved through recovering and recycling nutrients into bio-based fertilizers (BBFs), which can be reincorporated into food production systems.
The concept of planetary boundaries (Rockström et al., 2009) defines the limits of nine key systems crucial for maintaining Earth’s safety and habitability. One of these key systems is the biogeochemical flow of phosphorus (P) and nitrogen (N). Currently, 22.6 Tg of P and 190 Tg of N are emitted yearly, doubling and tripling, respectively, the planetary boundaries for each nutrient (Richardson et al., 2023). The main driver of exceeding the system’s boundaries is the continuous and excessive P and N inputs in agriculture (Sandström et al., 2023). Correcting this issue requires recycling, improving retention, and utilizing nutrients more efficiently (Carpenter and Bennett, 2011; Sandström et al., 2023).
Fertilizer nutrient use occurs mostly within an inefficient linear economy: a take-make-dispose approach (Chojnacka et al., 2020; Donner et al., 2020). Take refers to mineral fertilizers, which are derived, taken, from phosphate rock deposits or synthesized through energy-intensive industrial processes, as in the case of Haber-Bosch N. Then, to make food and grow crops, mineral fertilizers are applied to agricultural land. As consumption of agricultural products is centralized in urban areas, nutrients concentrate in urban wastewater and end up being lost to the atmosphere, aquatic environments or disposed in landfills (Witek-Krowiak et al., 2022). This perpetuates the inefficient system as new mineral fertilizers need to be continually produced to maintain agricultural outputs.
On a global scale, P fertilizer use efficiency is between 9 and 12% for cereal crops (Yu et al., 2021), while N fertilizer use efficiency averages between 48 and 78% in croplands (You et al., 2023). In Europe, P fertilizer use efficiency is 57% (Schoumans et al., 2015). Despite improvements in recent decades, significant P and N surpluses persist, leading to eutrophication and other forms of ecosystem damage (Bouwman et al., 2013; Reid et al., 2018; Ural-Janssen et al., 2023; Muntwyler et al., 2024). For example, extracting mineral P generates hazardous mine tailings (Silva et al., 2022). Production of synthetic Haber-Bosch N is responsible for ca. 2% of carbon emissions globally and relies on non-renewable natural gas (Osorio-Tejada et al., 2022). These problems highlight an urgent need to shift to circular nutrient economies (Foley et al., 2011).
A circular economy is a system where materials are kept inside, instead of disposed, thus minimizing external resource usage and energy requirements (Ritzén and Sandström, 2017). Achieving this involves reducing nutrient losses during food production and consumption and recovering and reutilizing nutrients (Harder et al., 2021). Sourcing fertilizers from P and N that otherwise would be wasted, significantly contributes to shaping circular nutrient economies, as opposed to mined P or synthetic N.
The concept of Doughnut Economics (Raworth, 2017) emphasizes that circular economies should exist within the boundaries of meeting human economic and social needs, while safeguarding the planet’s ecosystems. In consequence, the production and use of fertilizers with recovered nutrients must develop in conjunction with the agricultural, economic, and social needs of the specific context in which it takes place, involving stakeholders in the decision-making process (Schengel and Goehlich, 2024). Furthermore, it is essential to consider the available P and N sources, the existing infrastructure, and the regulatory and market conditions (Moshkin et al., 2023; Garmendia-Lemus et al., 2024).
In the European Union (EU), approximately 1 Tg of mineral P fertilizers and 10 Tg of synthetic N fertilizers are applied annually (Eurostats, 2023). Meanwhile, considerable sources of P and N, such as sewage sludge, are underutilized compared to the United States and Australia (Marchuk et al., 2023). Therefore, the EU is attempting to transition to a circular economy and maximize the recovery of nutrients (Sporchia and Caro, 2023). At the forefront of the EU’s efforts is the European Green Deal, a set of policy initiatives with the goal of making the EU climate neutral by 2050 (European Commission, 2019). The food system and agricultural core of the European Green Deal is the Farm to Fork Strategy, which aims to overhaul policy and regulation to create a more circular European food system. From an agricultural perspective, one of the central aims of the Farm to Fork Strategy is to reduce nutrient losses by at least 50% (Heyl et al., 2023).
The required changes are gradually being incorporated into policy and legislation, reflected in the Common Agricultural Policy reforms, which now promote a precision approach to plant nutrition to improve nutrient use efficiency (Heyl et al., 2023). The Circular Economy Action Plan (European Commission, 2020) has underlined the need to foster nutrient recycling and facilitate the reincorporation of waste streams into agriculture. Reincorporation and upcycling/recycling of nutrient-rich wastes into new circular fertilizer products replacing synthetic fertilizers has been supported by the recent updates in the Fertilizing Product Regulation (Regulation (EU) 2019/2009). These policy and regulation changes have caused a renewed interest in approaches for incorporating waste-derived nutrients into fertilizers.
In this context, the EU has committed significant research efforts through initiatives such as Horizon 2020 and Horizon Europe. Among these initiatives is the EU-funded FertiCycle project, a Marie Skłodowska-Curie Actions Innovative Training Network, in which all authors of this review paper were actively involved. The FertiCycle project aimed at proposing solutions for nutrient recovery and recycling within the European framework, addressing agronomical, environmental, and economic challenges associated with the development and adoption of improved and new bio-based fertilizers, and thus contributing to a more circular nutrient economy in the EU. The aim of this review is to present the contributions of the FertiCycle project within the context of critical challenges and current state-of-the-art literature on BBFs in the EU, guiding researchers, policymakers, and stakeholders by highlighting key innovations and addressing critical barriers for BBF adoption. Figure 1 provides an overview of the agronomic challenges, improvements, and pending challenges presented and discussed in this review.
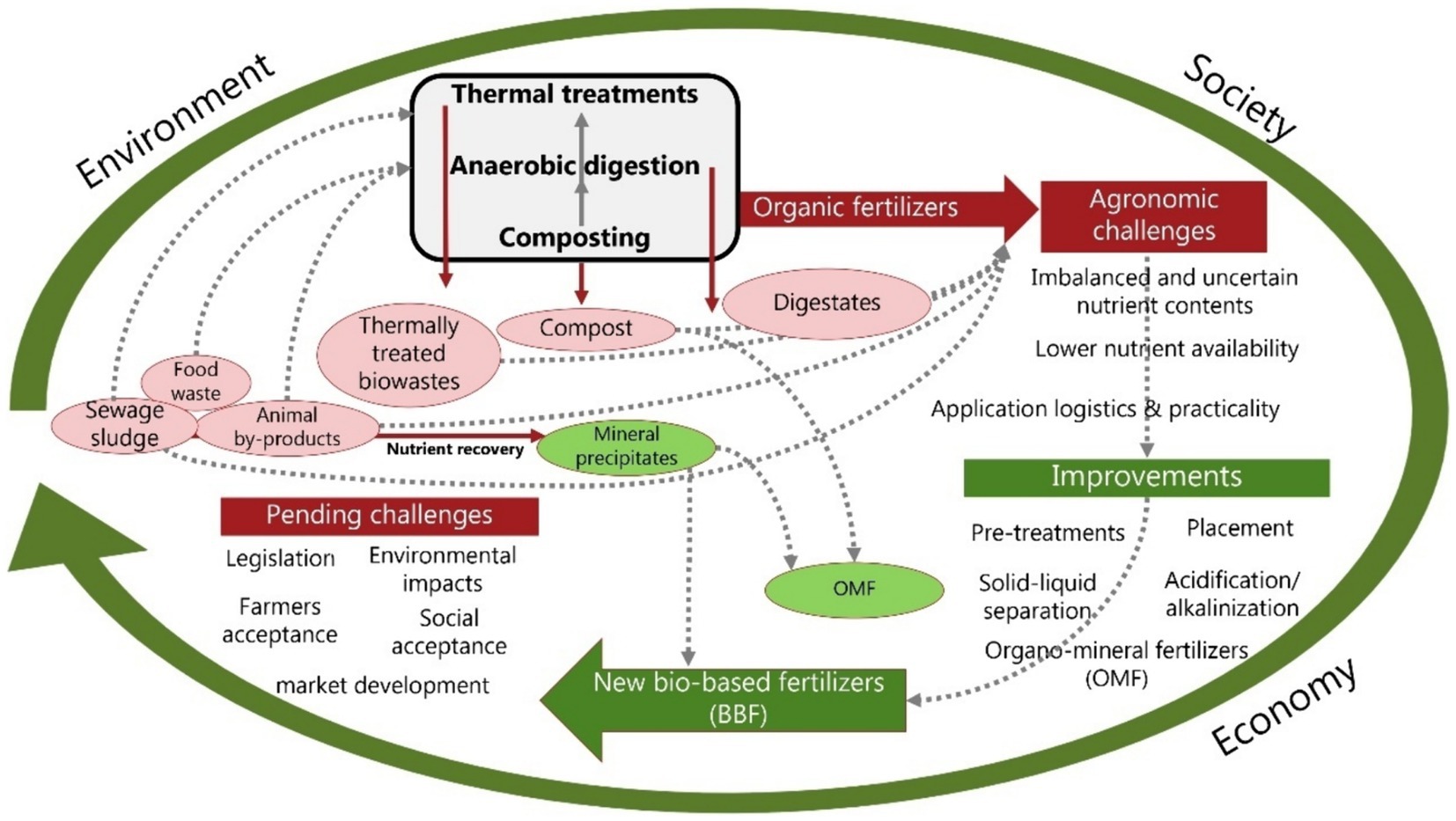
Figure 1. Overview of different types of input materials, biowaste transformation methods, agronomic challenges, improvements, and pending challenges for a successful widespread adoption of bio-based fertilizers, in the context of circular nutrient economy that considers its environmental, social, and economic boundaries.
2 What is a bio-based fertilizer?
The use of the term bio-based fertilizer or BBF has increased lately in academic publications for describing a broad category of materials of biological origin used to supply nutrients to crops (Chojnacka et al., 2020; Wester-Larsen et al., 2022; Rodríguez-Alegre et al., 2023; Kurniawati et al., 2023a). However, distinguishing BBFs from organic fertilizers and biowastes presents a challenge.
The term “biowaste” encompasses materials of biological origin discarded as wastes, including byproducts from agriculture, food production, animal husbandry, and human life. Nutrient-rich biowastes, when applied to soils to provide nutrients for plants, are included under the category of organic fertilizers (Pain and Menzi, 2011). In everyday language, a distinction is made between a general biowaste and an organic fertilizer, only based on their actual usage. Manure, defined by the Oxford English Dictionary as “Dung, excrement or compost, esp. as spread over or mixed with soil to fertilize it” is a prevalent example.
The term “animal manure” describes a mixture of animal feces, urine and bedding material that is utilized as a fertilizer (Shober and Maguire, 2018). Animal manures often undergo some degree of processing, such as anaerobic storage, addition of water or homogenization to facilitate its application to the field. However, the term BBF is employed in literature advocating alternatives to mineral fertilizers or describing materials of enhanced fertilizer value, implying a more technologically advanced material than a basic, raw organic fertilizer such as an animal manure (Luo et al., 2022; Egas et al., 2023; Moshkin et al., 2023; Garmendia-Lemus et al., 2024). Therefore, it is arguable that an animal manure is not a BBF, since it had no treatment specifically to improve its fertilizing qualities.
The core value of a BBF lies in its circularity (Chojnacka et al., 2020). Bio-based fertilizers are intended to allow the reincorporation of nutrients that otherwise would be wasted, have an improved nutrient use efficiency compared to a less processed organic fertilizer, or decrease possible negative environmental impacts compared to its organic or mineral fertilizer counterparts. Furthermore, organic fertilizers can be associated with containing organic matter and being capable of, e.g., improving soil structure or being a source of organic carbon. However, a BBF can also include nutrients derived from biomass, in the absence of organic matter, and resemble mineral fertilizers in their chemical and physical forms, as long as the nutrients are from biological origin.
The concept of biological origin is one of the main points of discussion for recognizing a fertilizer as bio-based according to the European Sustainable Phosphorus Platform (ESPP, 2023). In the context of bioplastics, the European Standard EN 16575 (August 2014) recognizes the biological origin, when the material is wholly or partly derived from biomass renewable during human lifespans, i.e., excluding materials fossilized and embedded in geological formations.
The European Union updated Fertilizing Product Regulation (FPR, regulation EU 2019/1009) has not yet established a precise definition for BBFs. However, it distinguishes between materials of inorganic and organic origin and introduces categories for nutrients of biological origin. The FPR incorporates so-called product function categories (PFC) into legislation, which categorize different types of fertilizing products based on their primary purpose. Products within these categories need to be composed of materials recognized within specific component material categories (CMCs). Therefore, for a bio-based product to be recognized as a fertilizer, or be included as a part of one, its components need to fit within a CMC. Relevant PFCs and CMCs are summarized in Table 1. Main novelties include CMC 12, which regulates the use of recovered phosphate from, e.g., wastewater treatment plants. This category sets limits of tolerance for chemical impurities and pathogens, and allows materials of less purity than those classified under CMC 15. Animal by-products are still outside the scope of the FPR (EC, 2022), and regulated under the Animal By-products Regulation EC 1069/2009 (EC, 2022). However, this will change once the European Commission establishes endpoints in the processing chain for individual by-products. Currently, there is a draft proposal for the inclusion of animal by-products in the FPR.1
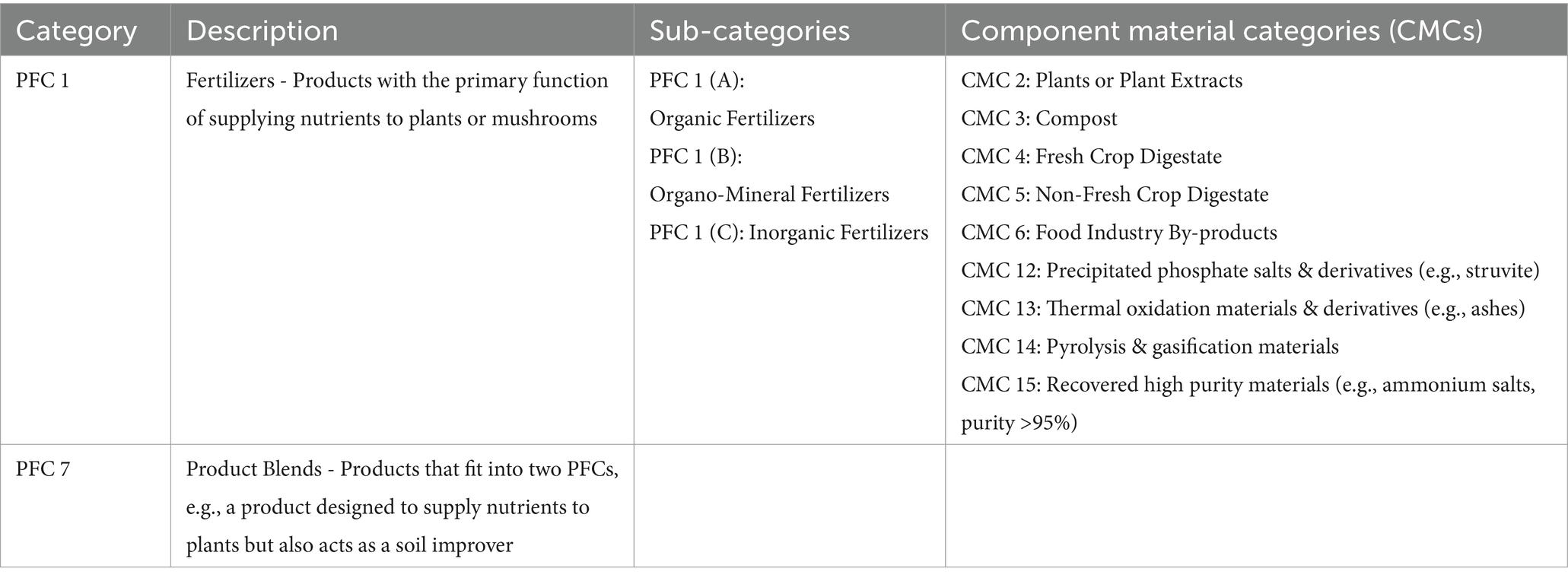
Table 1. Product function categories (PFC) and sub-categories from the updated fertilizing product regulation (Regulation (EU) 2019/1009) pertinent to new bio-based fertilizers.
The clear definition of the term BBF is challenging and still debatable. One definition that has been proposed is that BBFs are materials or products derived from materials of biological origin, with a content of bioavailable nutrients high enough to be used as a fertilizer (Wester-Larsen et al., 2022). On the other hand, Rodríguez-Alegre et al. (2023) proposed that waste streams, i.e., manure, sewage sludge and food processing wastes, which can be valorized, through processing, into a fertilizer product should be termed BBFs. Both definitions indicate that some degree of processing is necessary for a biowaste or an organic fertilizer to acquire BBF status. Moreover, literature also has assigned bio-based status to fertilizers where the nutrients are recovered (e.g., via ammonia stripping or P precipitation) from biowastes, or when they are contained in new fertilizing products combining synthetic nutrients with those of biological origin.
It is not our goal to replace the term “organic fertilizer” with BBF. It is rather to provide an overview of the challenges and opportunities for reincorporating nutrients from biowastes into the food system and improving the agronomic efficiency of existing organic and bio-based fertilizers. Therefore, creating new, improved BBFs. For the purpose of this review, we will consider a BBF, any material of biological origin (e.g., a biowaste), that has been treated with the purpose of enhancing its fertilizing properties (e.g., an acidified slurry); as well as fertilizing products, where a significant proportion of its nutrients is of biological origin, for example. an organo-mineral fertilizer containing a combination of mined rock phosphate and ammonium nitrate gained through ammonia stripping of manure (Wester-Larsen et al., 2022).
3 From biowastes to new bio-based fertilizers
The importance of BBFs in a more sustainable agriculture is underscored by the abundance of waste materials such as agricultural and food waste (e.g., olive pomace), sewage sludge and animal excreta (Kummu et al., 2012; Mateo-Sagasta et al., 2015; Shober and Maguire, 2018). These biowastes contain carbon (C), P and N, making them valuable but potentially harmful for the environment if improperly handled (Chojnacka et al., 2020).
One of the main challenges in utilizing biowastes and organic fertilizers as nutrient sources is maximizing the availability of the contained P and N, which are often only partly or slowly available (Chojnacka et al., 2020). Raw biowastes also pose challenges such as nutrient imbalances relative to crop demand, pathogen contents and high volumes, requiring chemical and physical modifications (Overmeyer et al., 2023; Zireeni et al., 2023; Kopp et al., 2023b). Biowaste transformations (e.g., composting, anaerobic digestion, pyrolysis) designed to tackle logistical or environmental challenges can negatively impact the nutrient availability (Jameson et al., 2016).
Biowastes undergo several treatments depending on legal, economic, or environmental requirements. For example, cattle excreta are composted, or subjected to ammonia stripping; sewage sludge can be anaerobically digested, composted, dewatered, or incinerated (Kanteraki et al., 2022). An overview of available input materials for new BBFs is given in Table 2.
3.1 Phosphorus and nitrogen in raw materials for new bio-based fertilizers
3.1.1 Phosphorus
Increasing the P fertilizer value in biowastes is challenging, due to the diverse chemical forms of inorganic P (Pi) and organic P (Po), influenced by the biowaste source and processing (Meyer et al., 2018). Phosphorus is plant available only in the form of dissolved inorganic orthophosphates (Tate, 1984).
Most of P in manure and feces is water-soluble Pi, whereas Po varies based on the feed and animal and type (Barnett, 1994; Poulsen and Kristensen, 1998). Dairy cattle manures contain between 6 g·kg-1 and 16 g·kg-1 of total P, mostly Pi (Barnett, 1994). Poultry manures contain between 13 g·kg-1 and 23 g·kg-1 of total P, have variable proportions of Pi and Po, and are rich in calcium-bound Pi forms (Barnett, 1994; Neijat et al., 2011). One of the most important challenges regarding manures are their imbalanced P to N ratios, that may result in excessive P application when fertilization aims to meet plant N demand (Sharpley, 1996). High concentrations of water-soluble Pi in and organic compounds have been linked to high P leaching in soils treated with manure (Glæsner et al., 2011). The transformation of manure with composting significantly concentrates the contained P due to dry matter loss, and reduces P solubility (Zhang et al., 2023).
Phosphorus in composts is predominantly inorganic (Frossard et al., 2002; Zhang et al., 2023). In an evaluation of 16 samples of composted Swiss solid urban biowastes and woody wastes, Frossard et al. (2002) measured P concentrations between 2 g·kg-1 and 7 g·kg-1, mostly in the form of Pi. Phosphorus in compost included both water-soluble Pi as well as relatively insoluble calcium phosphates like apatites or octacalcium phosphates. Sewage sludges contain Po and Pi forms of low solubility [e.g., associated to iron (Fe), aluminum (Al) or calcium (Ca)] influenced by the diversity of P removal techniques (e.g., flocculation with iron or aluminum salts or biological P removal) used in wastewater treatment plants (Frossard et al., 1997; Wang et al., 2022). Anaerobic digestion (AD) of sludges can increase the crystallinity of Pi forms and leave behind only recalcitrant Po (Wang et al., 2022). Alkalinization by liming, done for sanitization purposes (Anderson et al., 2015; Malinowska, 2017), produces the formation of calcium phosphates that can become plant available in acidic soil conditions (Lindsay, 1972; Meyer et al., 2018). Solubility of P in sewage sludge is strongly decreased during thermal treatments for stabilization or volume reduction (e.g., pyrolysis or incineration), causing sewage sludge ashes to contain little available Pi due to the formation of stable P mineral forms (Nanzer et al., 2014; Lemming et al., 2017). Sewage sludge ashes and meat and bone meal have low mineral P fertilizer equivalencies, of 30 and 40%, respectively; untreated sewage sludge typically ranges from 60 to 90%, depending on the method used for P removal (Möller et al., 2018). The P in meat and bone meal is mainly in the form of hydroxyapatite (Jeng et al., 2007).
3.1.2 Nitrogen
The challenges associated with the N fertilizer value from biowastes include the diversity of N forms in biowastes and the losses through collection, treatment, storage, and soil application (Lassaletta et al., 2014; Fangueiro et al., 2015; Nigussie et al., 2017). In biowastes, N is usually contained in organic forms (urea and other amides, amino acids, undigested feed and food protein residues, nucleic acids) with variable amounts of inorganic plant available forms like ammonium (NH4+) and nitrate (NO3) (Langmeier et al., 2002; de Guardia et al., 2010; Bosshard et al., 2011). Human and animal urine contain most N in the form of urea, which is enzymatically transformed shortly after excretion into NH4+. Feces contain more complex N forms, for example, bacterial or endogenous debris for which availability will depend on mineralization processes (Dion et al., 2020).
Anaerobic digestion of manures and sewage sludges increases the inorganic N content (Hafner and Bisogni, 2009; Jiang et al., 2022). During AD, mineralization of N forms takes place (Möller and Müller, 2012). Nitrogen losses during the digestion process are significantly smaller compared to composting or thermal treatments (Chojnacka et al., 2020). Anaerobic digestion leads to decreased C to N ratios in the digestate effluent (Möller and Müller, 2012) as C is converted to biomethane gas (mixture of CO2 and CH4), while N is conserved in the digestate. Mechanical separation of solid–liquid phases from the anaerobic digestate concentrates directly available inorganic N (as dissolved ions) in the liquid fraction and slowly available organic N in the solid fraction (Zabaleta and Rodic, 2015; Chojnacka et al., 2020).
Composting of household waste, pig slaughterhouse sludge, and green algae resulted in losses of 36 - 66% of total N to the atmosphere (de Guardia et al., 2010), predominantly caused by ammonia (NH3) volatilization (Chowdhury et al., 2014). Volatility and speciation of ammoniacal-N in biowastes is influenced by the pH (Moraes et al., 2017). Acidification of animal manures prevents N emissions during processing, storage and land spreading and reduces NH3 volatilization by shifting the equilibrium toward the non-volatile NH4+ (Fangueiro et al., 2015). Similarly, during storage of biowastes or composting, acidification and pH management minimize N losses (Chowdhury et al., 2014; Cao et al., 2020; Kupper et al., 2020). Delayed applications of N-rich materials during composting can also reduce N losses (Nigussie et al., 2017).
Much of the existing waste management infrastructure prioritizes sanitation and emission reduction rather than recycling nutrients (Magid et al., 2006). For instance, wastewater treatment plants emit substantial amounts of N into the atmosphere through nitrification–denitrification processes aiming to reduce wastewater N (Marchuk et al., 2023). The goal of current and future waste management is to transform these facilities into nutrient recovery centers (Marchuk et al., 2023). The application of chemical and physical treatments to the outputs of waste management infrastructure, coupled with enhancements in existing processes are a step in that direction. Table 3 includes an overview of P and N forms in biowastes, as well as related agronomical challenges.
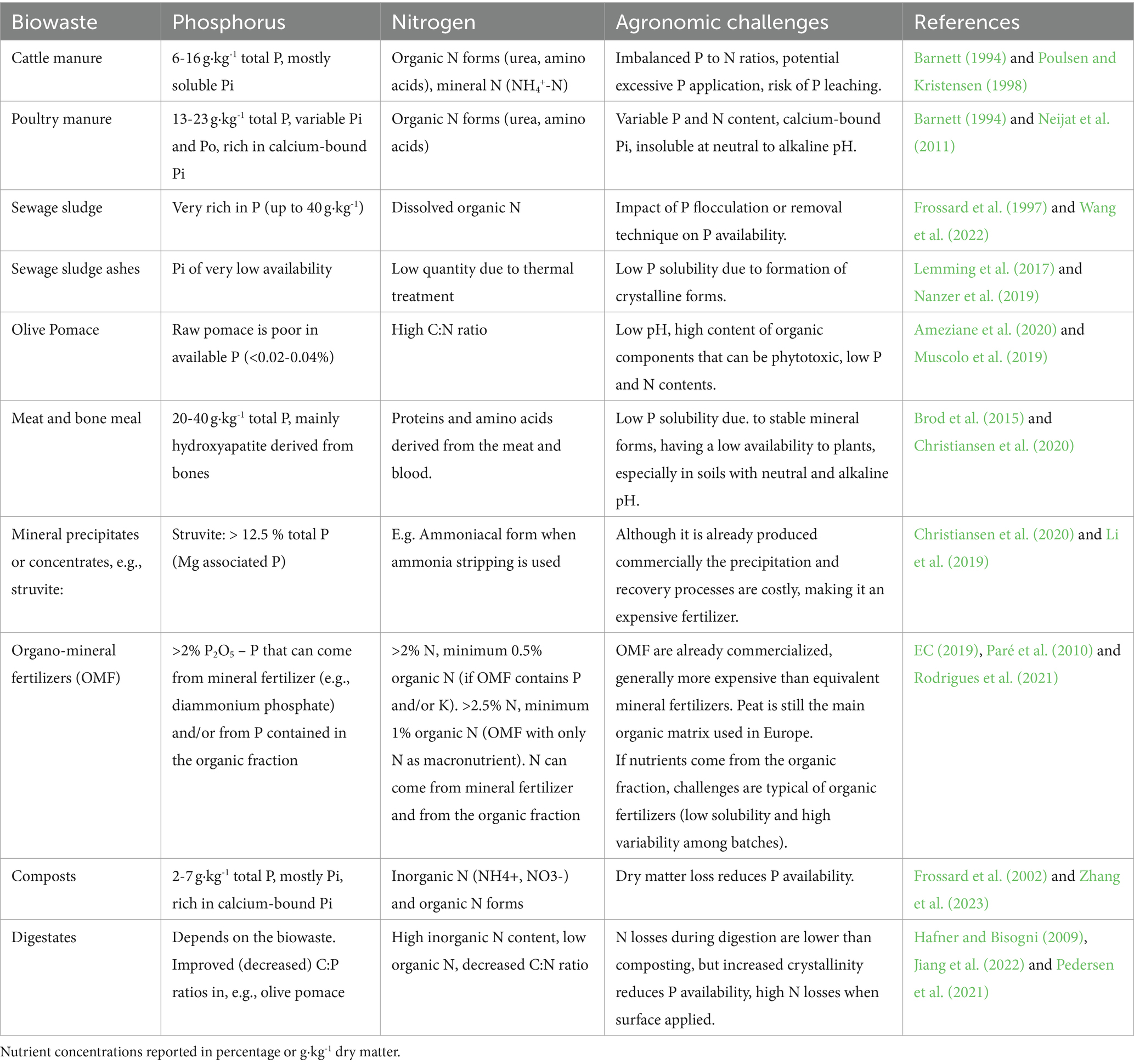
Table 3. Aspects regarding the form and contents of phosphorus and nitrogen and agronomic challenges of biowastes and potential input materials for new bio-based fertilizers.
3.2 Treatments to enhance the P and N fertilizer value of bio-based fertilizers
3.2.1 Pre-treatment of inputs in anaerobic digestion
Anaerobic digestion improves the N fertilizer value of digestates compared to untreated inputs. Anerobic digestions mineralize decomposable organic matter in slurries, reduce the C to N by converting C to biogas, and increase the NH4–N to total N ratio through organic N mineralization (Sørensen and Møller, 2009). However, with an increasing shift toward using high solid co-substrates such as lignocellulosic agricultural wastes (e.g., straw and other crop residues) with short hydraulic retention times, a smaller proportion of the organic matter (40–60 %) is degraded into biogas and the rest remains in digestats (Romio et al., 2021). Resulting digestates have reduced fertilizer values due to their high dry matter contents, higher C to N ratios and lower infiltration rates enhancing the risk of NH3 loss (Møller et al., 2022; Pedersen and Hafner, 2023). Biogas plants prioritize biogas yields over digestate quality (Logan and Visvanathan, 2019). This is driven by the higher value of biogas compared to digestate and incentives such as renewable energy targets.
Optimization of AD for enhanced biogas production and also nutrient availability can be achieved through biomass pre-treatments. Biomass pre-treatment techniques, classified as physical, chemical, biological, or a combination of these, have variable effects on biomass utilization in the AD process, dependent on the pre-treatment mechanism and feedstock characteristics. Biomass containing lignin or bacterial cells are the most affected during pre-treatment for enhanced AD process (Carlsson et al., 2012).
Nyang’au et al. (2023a) investigated the effects of electrokinetic and ultrasonication pre-treatments of biowastes in a two-step AD process on nitrogen fertilizer replacement value of digestates obtained from two biogas plants. The electrokinetic pre-treatment step significantly increased the ratio of ammonium-N to total N in the digestates before the second AD step. However, the effect leveled off after the secondary digestion step. The study demonstrated how integrating pre-treatment technologies into biogas plants could improve the fertilizing properties of the digestates.
Another study by Nyang'au et al. (2023b) highlighted the use of ensiling as a biological pre-treatment method to enhance biogas yield and improve the fertilizer value of the digestates. Ensiling significantly impacted physico-chemical properties of straw, increased methane yield by 4 to 14 %, and increased net inorganic N and S release in the soil compared to non-ensiled straw. They attributed the positive effect to enhanced substrate biodegradation during the ensiling, which increased biochemical accessibility and nutrient solubilization during AD.
Pre-treatments can be effective measures to increase the fertilizer value of digestates. However, the selection of a pre-treatment technique should consider net effects, including cost and energy consumption (Meegoda et al., 2018). Many studies solely evaluate pre-treatment benefits by comparing extra energy output against energy consumption, overlooking other potential advantages such as increased digestate fertilizing value.
3.2.2 Acidification of slurries
Emissions derived from barns and slurry storage represent 80% of agricultural NH3 emissions, which can be mitigated by acidification to a pH in the range of 4.5-6.8 (Fangueiro et al., 2015). Moreover, utilization of untreated animal slurry in horticulture poses potential risks in terms of food safety. Slurry acidification can be employed to address both NH3 emissions and food safety risks (Fangueiro et al., 2015; J. Rodrigues et al., 2021).
Utilization of sulfuric acid for slurry acidification represents an addition of available sulfur that increases the fertilizer value of the slurry (Zireeni et al., 2023). Acidification increased the concentration of water-soluble P in 20-65% compared to raw slurry (Regueiro et al., 2020). Moreover, there are benefits associated with the soil application of acidified slurry. In the study conducted by Schreiber et al. (2023) where acidified slurry (pH 5.5) was applied to a Haplic Cambisol (pH 7), a significant increase of 38% in N use efficiency was observed in plant biomass. Zireeni et al. (2023) found that the application of acidified slurry (pH 5.5) to a Cambisol (pH 6.8) transitorily reduced soil pH by at least 0.4 units for up to two months, before the pH went back to its baseline. A transitory acidification of soil can be beneficial in soil conditions where P is a limiting factor.
For slurry acidification, sulfuric acid, and to a lesser extent, nitric and hydrochloric acids are utilized (Fangueiro et al., 2015). However, other additives and processes for bio-acidification involving agro-industrial by-products were tested by Chrysanthopoulos et al. (2024) for acidifying pig slurries. It was shown that bio-acidification of pig slurries through fermentation is possible when the fermentation substrate contains sufficient organic C (Chrysanthopoulos et al., 2024). Moreover, slurry bio-acidification using rice bran, a biowaste rich in N, as a fermentation substrate significantly increased the total N content compared to untreated slurry (Prado et al., 2020). Conclusively, acidification of slurries can be used beyond addressing hygienic concerns and mitigating NH3 emissions. Acidification can be a way to utilize agro-industrial by products and increase the fertilizer value of slurries.
3.2.3 Acidification and alkalinization of sewage sludge, sewage sludge ashes and meat and bone meal
Sica et al. (2023) evaluated the impact of acidification and alkalinization pre-treatments on the P solubility of sewage sludge, sewage sludge ashes and meat and bone meal. For acidification, a 2 to 1 biowaste to solution ratio was utilized, with sulfuric acid concentrations ranging from 0.25 M to 10 M. Alkalinization was carried out using sodium hydroxide concentrations ranging from 1 M to 2.5 M. For alkalinization with lime [Ca(OH)2], lime quantities equivalent to 10 to 40% of the fresh weight of the biowaste were added. Acidification resulted in a decrease in the pH of the biowastes to a range between 1 and 4, while alkalinization treatments raised the pH of the biowastes between 8 and 12. It was observed that acidification significantly increased the solubility of P, leading to a greater release of P into the soil and an increase in soil water-extractable P. Water-extractable P in the sewage sludge ashes increased up to 60 times. In meat and bone meal, water-extractable P rose from 4% to more than 80% of the total P when pH dropped below 4. Alkalinization with sodium hydroxide was found effective in increasing soil P availability in sewage sludge, and potentially providing similar sanitation effects to lime. However, plant trials were not conducted by Sica et al. and they highlighted that the higher costs of sodium hydroxide compared to lime may limit the large-scale application of this pretreatment.
Similarly, Keskinen et al. (2023) used residual organic acids from cellulose extraction to acidify sewage sludge to both pH 7 and 4.5. They observed a significant increase in P solubility at pH 4.5, but it did not impact P uptake by ryegrass. It is important to mention that their results demonstrated that the acidification of sewage sludge solubilizes other elements, including harmful metals, which may harm plant growth (Imadi et al., 2016).
3.2.4 Chemical modifications of thermally treated biowastes to enhance P availability
In the Netherlands, Switzerland, and Belgium, mono-incineration has emerged as the predominant practice (Mininni et al., 2015). Recent legislative changes in Sweden, the Czech Republic, and Denmark have made possible utilizing sewage sludge biochar for agricultural purposes.
Both incineration and pyrolysis offer advantages such as volume reduction, increased P concentration, pathogen elimination or reduction, and potential energy recovery. Additionally, pyrolysis forms recalcitrant C and contributes to soil C sequestration and climate change mitigation (Smith, 2016). However, as previously discussed, thermal treatment decreases the P availability.
The use of additives before pyrolysis can enhance P availability in biochar. The addition of magnesium hydroxide alters the resulting P forms after pyrolysis, mainly by avoiding the formation of crystalline Ca-P minerals in favor of amorphous and more soluble Mg-P forms (e.g., MgNH4PO4 and Mg3(PO4)2), which has resulted in a 20% increase in the P availability of poultry litter biochar (Zwetsloot et al., 2015; Leite et al., 2023). The addition of calcium oxide (CaO) in thermal treatment may offer similar benefits. Liu et al. (2019) found that addition of 10% CaO converted non-apatite inorganic P forms into apatitic forms, (e.g., Ca3(PO4)2 and Ca3Mg3(PO4)4). This increased the sewage sludge biochar P availability and promoted plant growth in acidic soils.
Another alternative is offered by pH modification treatments after pyrolysis or incineration. The effect of acidification with sulfuric acid was assessed in three ashes (sewage sludge, poultry litter, digestate solids) and four biochars (digestate solids, sewage sludge, meat and bone meal, insect frass) (Kopp et al., 2023b). While the P availability from untreated ashes and biochars was very low, the acidification significantly increased the total plant P uptake from all materials from 2 to 35 times. Acidification solubilized mainly Ca-P and did not increase heavy metal uptake nor strongly affected soil pH which remained at 6.4. A synthesis of the effectiveness of alkalinization and acidification treatments for P solubilization is provided in Figure 2.
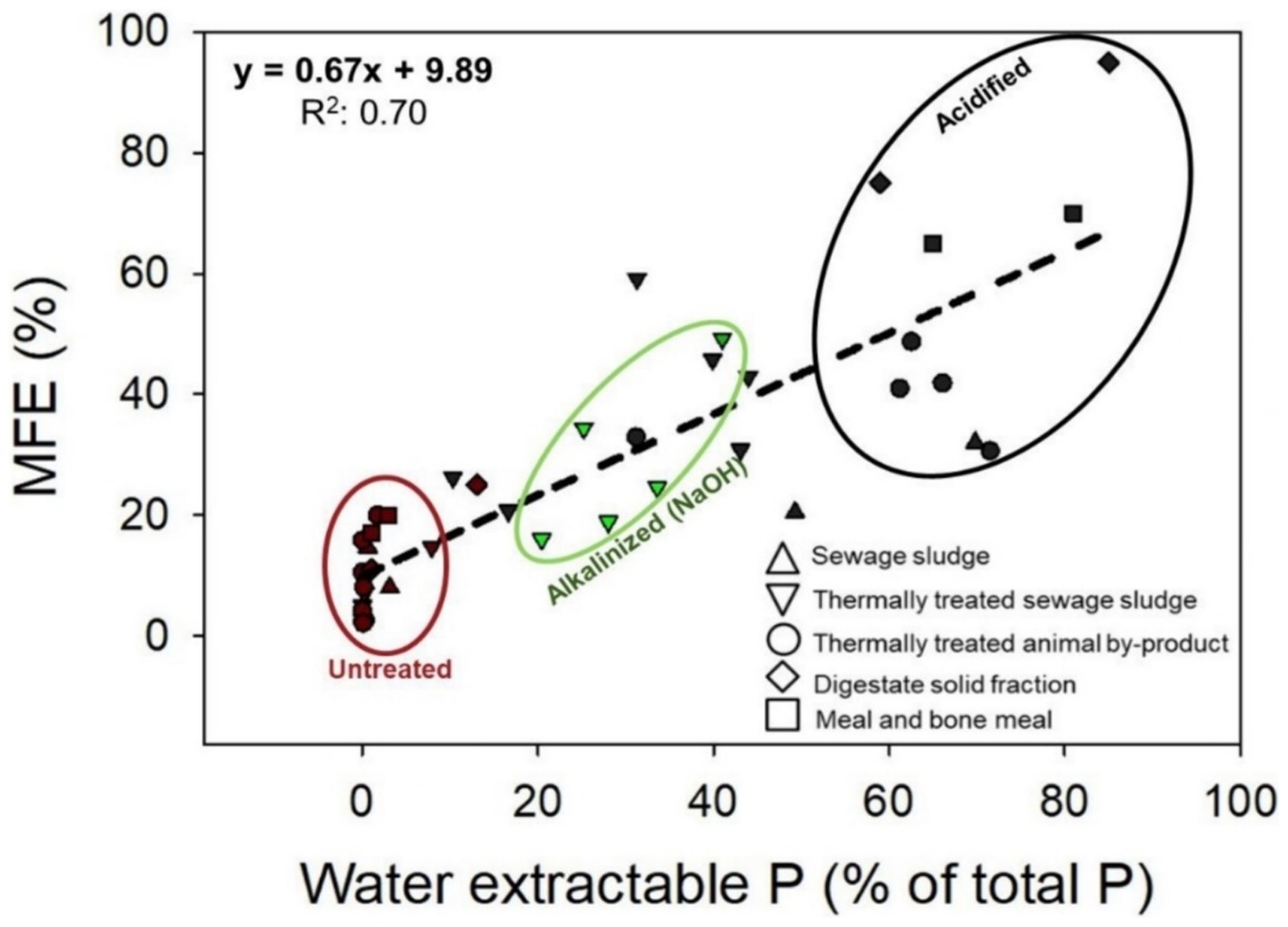
Figure 2. Correlation between the P solubility (water extractable P) and the mineral fertilizer equivalent of untreated, alkalinized, and acidified biowastes (Sica et al., 2023; Kopp et al., 2023a,b). The figure shows that biowastes with initially low water-soluble P (<5% of total P content) significantly increased this proportion after acidification, while thermally treated sewage sludge also did so after alkalinization. Furthermore, a correlation (R2 = 0.7) between the water-extractable P content of these materials and their mineral fertilizer equivalent values was observed.
3.2.5 Combined treatments
Integrated approaches throughout manure management chains elevate the fertilizer value and nutrient concentration and mitigate trade-offs between NH3 and GHG emissions (Aguirre-Villegas et al., 2019). One such effective integrated approach involves coupling anaerobic digestion of biomass with solid–liquid separation of the resulting digestate. In this strategy, the solid fraction is re-digested to enhance further biogas and nutrient recovery. The liquid fraction is then used as a fertilizer, since it is enriched with a higher proportion of NH4+ and overall higher N. Alternatively, the solid fraction, rich in P, may be utilized to fertilize P-deficient soil or transformed into value-added products like biochar, contributing to soil C retention (Fangueiro et al., 2015), or used as livestock bedding material.
Other integrated approaches that could improve the fertilizer value of digestates include AD followed by acidification of the digestates, source segregation of manure followed by anaerobic digestion and AD followed by plasma treatment of digestates. Plasma treatment of digestates fixes reactive N from the atmosphere to the slurries in nitrite and nitrate forms (Graves et al., 2019). The nitrite and nitrate fixation forms nitric acid (HNO3) and nitrous acid (HNO2), which lowers the slurry pH and produces the previously mentioned benefits (Winter and Chen, 2021). Plasma treatment of slurry has shown a potential to replace more mineral fertilizer and increase yields compared to untreated slurry (Cottis et al., 2023). Moreover, this treatment reduces both methane (CH4) and NH3 emissions during storage and field applications, although the risk of increased losses via nitrous oxide (N2O) emissions and nitrate leaching needs to be assessed (Cottis et al., 2023). Integrated approaches offer a comprehensive solution for optimizing fertilizer values and contribute to sustainable agricultural practices by minimizing environmental impacts.
3.3 Organo-mineral combinations
Organo-mineral fertilizers (OMFs) are a mixture of materials of biological origin with one or more mineral fertilizers (Smith et al., 2020). This allows the use of BBFs rich in organic C as the organic matrix, such as compost. The use of OMF has been reported to have some advantages over mineral fertilizers alone, as addition of organic C can reduce N losses and increase N use efficiency (Richards et al., 1993; Antille et al., 2014; Florio et al., 2016). Similarly, OMFs increase plant P use efficiency by causing a prolonged release of plant available P in soil (Antille et al., 2013).
The enhanced nutrient use efficiency can be linked to various processes. For instance, there is an electrostatic attraction of phosphates to organic materials, reducing the P mobility in soil that could be otherwise fixed to clay minerals (Gwenzi et al., 2018; Luo et al., 2021). The chemical interactions between the organic matrix and mineral fertilizer can result in the formation of insoluble compounds that precipitate out of the soil solution (Mazeika et al., 2016; Carneiro et al., 2021; Luo et al., 2021). Additionally, the organic material acts as a physical barrier between the mineral fertilizer granule and the soil solution, thereby decreasing the solubility of the fertilizer granule (Limwikran et al., 2018). Lastly, the stimulation of soil microbial activity leads to the immobilization of nutrients into the microbial biomass and a subsequent gradual N release that reduces leaching (Richards et al., 1993; Mandal et al., 2007).
The FPR establishes that at least 3% of the total mass of an OMF must be composed of organic C if it is a liquid OMF, and at least 7.5% on a solid OMF, while the sum of macronutrients needs to be 6% or 8%, respectively (EC, 2019). This broad definition allows the production of OMFs with varying characteristics.
One type of OMF is where the organic matrix is used as the main source of one or more nutrients and mineral fertilizer is added to produce a specific nutrient ratio that will depend on specific soil and plant nutrient requirements (Rady, 2012; Antille et al., 2013; Anetor and Omueti, 2014). This type of solid OMF, in addition to supplying macro and micronutrients, can be used as a soil amendment to improve physical properties due to its large organic C content (Babalola et al., 2007). On the flip side, OMFs rich in organic C require the application of large quantities to the soil - in the order of tons per hectare- to adequately fulfill crop nutrient requirements (Antille et al., 2013; Mazeika et al., 2016). Such large required application volumes could result impractical.
A second group of solid OMFs has a small proportion of organic C from a material of biological origin. This material acts mainly as a protecting matrix for the mineral nutrients and not as a relevant nutrient source. Low organic C OMFs are designed to produce a slow release of mineral nutrients into the soil solution increasing the nutrient use efficiency (Richards et al., 1993). However, although the use of low organic C OMFs has the advantage of increasing the efficiency of the mineral fertilizer – and therefore reducing its application volume – they have the disadvantage of negligibly contributing with organic C to the soil.
There is a significant tradeoff between OMFs with high and low organic C. In those with low organic C, biowastes are not a significant source of nutrients. Therefore, their potential contribution to biowaste recycling is limited. If the mineral nutrients in the OMF are not from biological origin, it is advisable not to consider them a bio-based fertilizer (ESPP, 2023). Despite this limitation, biowastes in low organic C OMF play a crucial role in enhancing the nutrient use efficiency of mineral fertilizers, which is a significant benefit.
3.4 Application methods and strategies to improve the fertilizer value of bio-based fertilizers
Various studies highlight the importance of fertilizer P placement for enhancing the fertilizer value (Quinn et al., 2020; Freiling et al., 2022). Applying mineral P fertilizers by placement close to the seeds maximizes crop P uptake in the early growth stages (Grant et al., 2001; Grant and Flaten, 2019). Another strategy is subsurface band application (10 cm to 20 cm depth). This is mainly adopted in tropical countries with highly sorbing P soils that are susceptible to long drought periods. Subsurface band application creates P-rich bands with high available P contents, that are less susceptible to the soil surface drying (Meyer et al., 2023). However, the placement of P-rich fertilizers, as would be the case with some BBFs, is not as effective as the placement of mineral P fertilizers. Two possible explanations are the lower P solubility in BBFs compared to mineral fertilizers; and nutrient imbalances, leading to the over application of elements that may be toxic to the plant (Lemming et al., 2016).
Placement of sewage sludge increased root proliferation in the placement zone but did not enhance plant growth and total P uptake (Lemming et al., 2016). It significantly reduced P uptake from the soil compared to a treatment where the fertilizer was completely mixed with the soil. Regarding sewage sludge ash, Lemming et al. (2016) observed that placement did not attract root growth to the placement zone and significantly reduced both P uptake and plant growth. Building up on that, Sica et al. (2023) suggested that biowastes may have sufficient soluble P to attract roots to the placement zone, however, available P may not be sufficient to sustain plant growth for longer periods. Therefore, treatments to increase the P solubility of bio-based fertilizers are needed, along with placement strategies.
Fertilization with organic fertilizers may lead to the overapplication of organic N in the placement zone. Accumulated organic N is mineralized over time, releasing high amounts of NH3 into the soil (Chaves et al., 2004). Plants can suffer NH3 toxicity symptoms, such as inhibiting root growth at concentrations above 100 mg·kg-1 (Nkebiwe et al., 2016). To reduce NH3 toxicity effects, placement should be done more than 10 cm from the seeds, and/or days before sowing, allowing NH3 to be nitrified thus lowering its concentration over time, while the plant is starting to develop (Delin et al., 2018; Baral et al., 2021). Acidification may also reduce the negative effects of NH3 toxicity (Pedersen et al., 2017).
4 Environmental impacts of bio-based fertilizers
4.1 Environmental benefits of bio-based fertilizers production and use
Recycling P reduces phosphate rock mining, thereby avoiding the associated environmental impacts such as landscape degradation, contamination of water bodies, and emission of GHGs associated with transport (Higgins, 2001; Fayiga and Nwoke, 2016). Moreover, using phosphate rock and derivatives for fertilization can result in the contamination of water bodies and soils with heavy metals and hazardous elements like cadmium, uranium and arsenic (Fayiga and Nwoke, 2016). Similarly, reducing the reliance on synthetic N contributes to lowering net GHG emissions and energy consumption, as Haber-Bosch synthesis of NH3 is one of the major fossil energy consuming processes worldwide (Osorio-Tejada et al., 2022; Gao and Cabrera Serrenho, 2023).
Beyond the substitution of mineral fertilizers, bio-based fertilizers offer additional environmental benefits. For example, by offering a slower release of nutrients compared to mineral fertilizers, nutrient leaching is reduced (Mandal et al., 2007). Bio-based fertilizers containing organic C enhance soil structure by increasing the organic matter content which also may be beneficial for microbial communities (Mayer et al., 2022). In soils in a Danish long-term field experiment, soils treated with compost significantly increased their organic C content compared to the mineral NPK treatment by up to 3% Moreover, they significantly reduced their bulk density from 1.6 kg·l-1 down to 1.2 kg·l-1, and improved their soil structure, thus requiring less energy consumption for tillage (Peltre et al., 2015).
4.2 Negative environmental impacts associated with bio-based fertilizer production and use, and mitigation strategies
Due to non-optimized nutrient ratios relative to crop demand in many BBFs compared to mineral fertilizers, fertilization with BBF and organic fertilizers could lead to overapplication of nutrients. An overview of environmental impacts associated with BBFs is available in Table 4. For instance, fertilization with manures can lead to overapplication of P when aiming to meet crop N demand (Sharpley, 1996). Similar problems arise when using digestates (Kadam et al., 2022), sewage sludge and other biowastes (Deeks et al., 2013; Lemming et al., 2019). Mitigation strategies encompass minimizing N losses during production, storage, and application of the BBF, thus maximizing the N content and its use efficiency, for which Ndegwa et al. (2008) and Pedersen and Hafner (2023) offer extensive reviews. The N to P ratio of some BBFs such as those derived from animal manures can be optimized by separating N from P through solid–liquid separation, or through segregation at-source of feces and urine before any processing step (Hjorth et al., 2009; Vu et al., 2016). Another strategy is using an organo-mineral combination, that provides the desired N to P ratio and corrects for the nutrient imbalance (Deeks et al., 2013).
The presence of contaminants could be a problem for some BBFs. Sewage sludges can contain cadmium, lead, and other heavy metals that may accumulate in soils after fertilization (Lu et al., 2012). In a study in Chile, significant amounts of microplastics accumulated in the soil after a decade of fertilization with sewage sludge (Corradini et al., 2019). The presence of persistent organic compounds like polychlorinated biphenyls, polycyclic aromatic hydrocarbons, traces of pharmaceutical compounds and hormones in sewage sludges, is discussed by Kanteraki et al. (2022). An overview of pollutant removal techniques for sewage sludge is provided by Geng et al. (2020). Olive pomaces contain phytotoxic phenolic compounds, lipids, and organic acids. Composting pomaces can help mitigate their toxicity by degrading some of the harmful compounds and increasing their fertilizer value (Muscolo et al., 2019; Ameziane et al., 2020).
Heavy metals are of potential concern in digestates and composts depending on the local input materials (Kupper et al., 2014; Kadam et al., 2022). Therefore, there is a pressing need for thorough monitoring and management of these biowastes. A breakthrough for the simplification of monitoring on a local scale was the obtention of conversion factors to predict the concentration of heavy metals in Belgian manure-derived digestates (de Castro et al., 2023). These conversion factors were based on process parameters of the AD processes, allowing the prediction of the concentrations of aluminum, chromium, cupper, iron, manganese and zinc based on the dry matter and the biodegradable fraction content of digestates.
Emissions of GHGs can result from both the production of BBFs and their soil application. In the production stage, mineral precipitates and thermally treated biowastes specifically raise concern as they require energy-intensive treatments. Thermal treatments and separation processes such as ammonia stripping, membrane electrodialysis, ion-exchange and struvite precipitation may emit less C to the atmosphere compared to traditional mineral fertilizer synthesis. However, their operational processes also demand substantial energy consumptions (Meneses-Quelal and Velázquez-Martí, 2020; Kar et al., 2023). Therefore, the source of the utilized energy will influence the environmental impacts of such treatments.
During soil application, NH3 emissions from manure digestates are often higher than from untreated manure (Holly et al., 2017; Emmerling et al., 2020). Furthermore, using ammonium sulphate to target sulfur fertilization has been linked with increased and overlooked NH3 emissions in neutral to alkaline soils (Powlson and Dawson, 2022). This not only emits NH3, contributing to undesired N deposition in natural ecosystems, but also results in losses of recovered N and the energy invested in its recovery. Likewise, fertilization with sewage sludge causes emissions of N2O and CH4 (Alvarez-Gaitan et al., 2016).
Implementing mitigation strategies is essential to minimize emissions from BBFs. These strategies include selecting appropriate application timing and methods, managing soils to maximize C retention, and using stabilizing additives like nitrification inhibitors (Severin et al., 2016; Tariq et al., 2022). For instance, injecting digestates into the soil reduces NH3 emissions compared to surface applications (Hou et al., 2015). Similarly, applying slurry to grasslands during spring rather than autumn is linked to less N2O emissions (Maris et al., 2021). Soil management practices such as minimizing or eliminating tillage and implementing crop rotations and leys, maximize the amount of C retained in agricultural soils (Jarecki et al., 2003). Acidifying digestates with, e.g., sulfuric acid can significantly reduce NH3 emissions (Pedersen and Nyord, 2023).
4.3 Assessment of environmental impacts from bio-based fertilizers
Single mitigation strategies can potentially result in pollution swapping. Therefore, understanding the interaction of all processes involved and the impact of specific local conditions is necessary. A comprehensive and integrated approach such as a life cycle assessment (LCA) is optimal to assess the potential environmental impacts of fertilization with BBFs (Jensen et al., 2020; Egas et al., 2023).
For example, Styles et al. (2018) used a LCA to assess BBF production from the liquid fraction of digestate of food waste in Sweden, applying NH3 stripping and struvite precipitation. The BBF production and field application was compared to the conventional management of digestate’s liquid fraction, including storage and field application. They concluded that producing BBF from liquid digestate results in significant environmental benefits due to the avoidance of CH4, N2O and NH3 emissions compared to the conventional management of liquid digestate. Moreover, application of that BBF enhanced the substitution of synthetic fertilizer due to the targeted use of nutrients. Another LCA on digestate utilization compared four alternative BBF production scenarios in relation to mineral fertilizer production (Alengebawy et al., 2022). This study included two technologies for nutrient extraction from the solid fraction and two more for the liquid fraction. Results showed that in all scenarios, BBFs constituted environmentally beneficial alternatives compared to mineral fertilizers, provided the digestate was pretreated to remove pollutants and pathogens.
Several studies have applied LCA to assess different aspects of fertilization with sewage sludge (Yoshida et al., 2018; Ding et al., 2021). For example, Yoshida et al. (2018) assessed the long-term impacts after field application of sewage sludge by using emission factors calculated by Bruun et al. (2016). Emission factors for sewage sludges were calculated considering sewage sludges with different properties, applied to three soil types, using three precipitation regimes and varying application amounts. This approach enabled the use of region-specific emission factors in the LCA (Yoshida et al., 2018). Normalizing the LCA results to yearly per capita emissions showed that human toxicity and ecotoxicity impacts were of greatest concern, largely due to the zinc and copper content in sewage sludge (Yoshida et al., 2018).
Ultimately, regional and local conditions may determine the sustainability and feasibility of using one BBF over another. Walling and Vaneeckhaute (2020) reviewed emission factors on organic and inorganic fertilizer production and use. This study recommended that emission factors should be estimated based on case-specific data due to the high variation in emissions depending on the composition of the fertilizer and the impact of local conditions like soil type and climate. In the LCA study of Beyers et al. (2022), the environmental impact of pig slurry acidification was assessed for the climatic, agronomic, and legislative conditions of Denmark, Spain, and the Netherlands. Slurry acidification reduced the environmental impacts related to emissions of GHG and NH3. However, the acquisition of energy and materials for the acidification process led to increased off-farm impacts in some categories, including fossil resource depletion and human toxicity. Furthermore, the effectiveness of acidification to reduce environmental impacts varied between countries due to differences in legislative requirements and energy sources. Thus, specific regional conditions (soil, climate, legislation, and farming practices) are crucial for the overall environmental sustainability of fertilization with specific BBFs. The PLCI 2.0 model has been developed to account for regional differences in LCAs of P-containing BBFs applied in European regions (Rydgård et al., 2024). This model incorporates factors such as regional soil P concentrations, soil erosion rates and distribution of crop types. Furthermore, it enables the modeling of the impact of different fertilization practices on P losses, harvesting of P in crops and the substitution of mineral P fertilizer.
5 Bio-based fertilizer market developments – social acceptance and economic drivers
Concerns regarding biosafety and environmental impact raised in recent decades, particularly for sewage sludge, have cast a negative perception on fertilization with some biowastes (Ekane et al., 2021). There is still a significant gap between the EU and other regions in the West in biosolids (treated sewage sludge) adoption. For instance, on average, only 35% of produced sewage sludge is reincorporated into agriculture in the EU (Hušek et al., 2022), compared to 55% in the United States and Canada, and more than 70% in Australia (Marchuk et al., 2023). However, the European average varies significantly by nation; for example, it is 0% in the Netherlands and Slovakia, but as high as 80% in Ireland (Hudcová et al., 2019). A comprehensive understanding of regulations, as well as preferences and needs of end-users and major stakeholders is necessary for a better acceptance and adoption of BBFs (Goldstein and Beecher, 2007; Ekane et al., 2021).
5.1 Stakeholders in the BBF market
In the BBF market, primary stakeholders include livestock farmers with a surplus of manure for export/processing, and crop farmers who are potential end-users of BBFs (Jensen et al., 2017; Kurniawati, et al., 2023a). Other important players are food and pharmaceutical industries, waste management companies, recycling fertilizer companies and farmers with crop residues on the supplier side, as well as garden owners and horticultural producers on the end-user’s side (Jensen et al., 2017; Venegas et al., 2021). Governments, public institutions (local/national/EU), civil society, non-governmental organizations (NGO), the food industry, investors, media, and scientists are also stakeholders in the European BBF market (Nedelciu et al., 2019).
The influence of primary stakeholders (farmers) on the supply and demand of BBFs varies. While livestock farmers are incentivized to utilize surplus manure; crop farmers exert more power in accepting or declining recycled products based on their perceptions and preferences (Case et al., 2017). Thus, defining and addressing stakeholder requirements and preferences is crucial for BBF adoption.
5.2 Attributes of bio-based fertilizers that influence acceptance and perception
The acceptance of BBFs among farmers in Europe is mainly influenced by four attributes: known nutrient contents, organic matter contents, cost and ease of application (Egan et al., 2022). Negative perceptions often arise from uncertainty regarding the N, P and K contents in BBFs (Tur-Cardona et al., 2018; Egan et al., 2022), contrasting with the precision offered by mineral fertilizers. Farmers are aware of the uncertainties in nutrient contents in organic fertilizers, and they prefer BBFs with nutrient ratios that fit crop demands (Egan et al., 2022). Therefore, reliable and known amounts and ratios of nutrients are essential for facilitating adoption.
A strength of BBFs is their organic matter content, and their perceived capacity to enhance soil structure, improve soil productivity, and increase water retention capacity of soils (Case et al., 2017; Gwara et al., 2021). These benefits are well-recognized by farmers (Egan et al., 2022). However, these advantages alone are not enough to completely substitute mineral fertilizers with BBFs and need to be accompanied by the other three attributes.
The cost of BBFs and their fertilizing properties relative to mineral fertilizers play a pivotal role in social acceptance. Another advantage of BBFs is their perceived low-cost and high nutrient content (Case et al., 2017). Farmers are more likely to adopt BBFs if they are competitively priced (Egan et al., 2022). Logistical costs and perceived higher overall expenses may deter adoption, necessitating a cost at least half that of mineral fertilizers for widespread acceptance (Tur-Cardona et al., 2018). Despite potential cost differences, the ecological co-benefits of BBFs mentioned before, could sway farmers toward their adoption with proper awareness (Egan et al., 2022). We discuss such potential in the next section.
The ease of application and the practicality of BBFs also play a major role. The form of the BBF (solid, semi-solid, liquid, or granulated) significantly influences farmer preferences and acceptance (Tur-Cardona et al., 2018; Egan et al., 2022). Solid and semi-solid forms are favored over liquids due to ease of application with existing farm machinery and improved forms, such as pellets, can enhance acceptance and willingness to pay (Hills et al., 2021). Thus, farmers prefer BBFs to be granular like mineral fertilizers.
Farmers may be reluctant to utilize BBFs due to the potential negative perception from clients and consumers of their products (Simha et al., 2017). In a Polish survey, an important source of negative perception stemmed from concerns about potential health risks associated with fertilization using nutrient-rich biowastes (Smol, 2021). Consumer acceptance of products fertilized with BBFs is greater for ornamental plants than for horticultural crops for consumption (Segrè Cohen et al., 2020). This indicates that final consumers of produce fertilized with BBFs may hold negative perceptions. Media and NGOs also influence consumer opinion and their perceptions of BBF products (Jensen et al., 2017) Even if farmers are willing to use recycled nutrients, the reluctance of final consumers and the food industry to consume products fertilized with BBFs may prevent them from doing so (Barquet et al., 2020; McConville et al., 2023). Therefore, it is important to raise awareness among produce consumers and inform them about the environmental advantages of BBFs.
5.3 Current bio-based fertilizer market
Although precise estimations of the European BBF market size are not available, it is evident that the BBF market is smaller compared to the conventional mineral fertilizer market. Approximately half of the EU’s fertilizer inputs for P come from mineral P, and about two-thirds of N fertilizers are produced through the Haber-Bosch process (Schoumans et al., 2015; Einarsson et al., 2021). Both studies recognize animal manures as the most significant alternative sources of P and N aside from mineral fertilizers. Therefore, the BBF market is still in early stages, requiring substantial changes in product availability, quality, legislation, competition dynamics, and stakeholder perceptions (Kvakkestad et al., 2023).
A favorable landscape is currently emerging for the development of the BBF market. Legislative developments, as outlined in section 2, have established a legal framework for BBF products in the European market, providing a conducive environment for market growth. However, a crucial question arises: where should the focus be directed? In the previous section, we outlined five criteria important for BBF adoption. Additionally, literature has emphasized that BBFs should resemble mineral fertilizers in consistent supply, allowing for a seamless transition for farmers accustomed to using commercially available mineral fertilizers (Gregson et al., 2015; Case et al., 2017; Buysse and Cardona, 2020; Kvakkestad et al., 2023).
Buysse and Cardona (2020) suggested that rising prices of mineral fertilizers would create an opportunity for the BBF industry to develop, given the higher production costs of BBFs. The fertilizer market has experienced significant volatility (Figure 3) during the period from 2021 to 2023, particularly due to rising geopolitical tensions and the war in Ukraine, leading to several increases in mineral fertilizer prices (AGRIDATA, 2023). The price increase in late 2021 and 2022 created a new reality for BBF producers, making more nutrient recovery technologies economically feasible (Hermann and Hermann, 2021). Since early 2023, fertilizer prices have been decreasing and stabilizing. At the time of writing this article, mineral fertilizer prices have returned at the levels of mid-2021. However, despite the prices being lower than in 2022, they remain higher than previous pre-2021 levels. Given the ongoing geopolitical pressures on the European Union, it is unlikely that the prices will decrease further in the foreseeable future (Alexander et al., 2022; Brownlie et al., 2023; Rabbi et al., 2023).
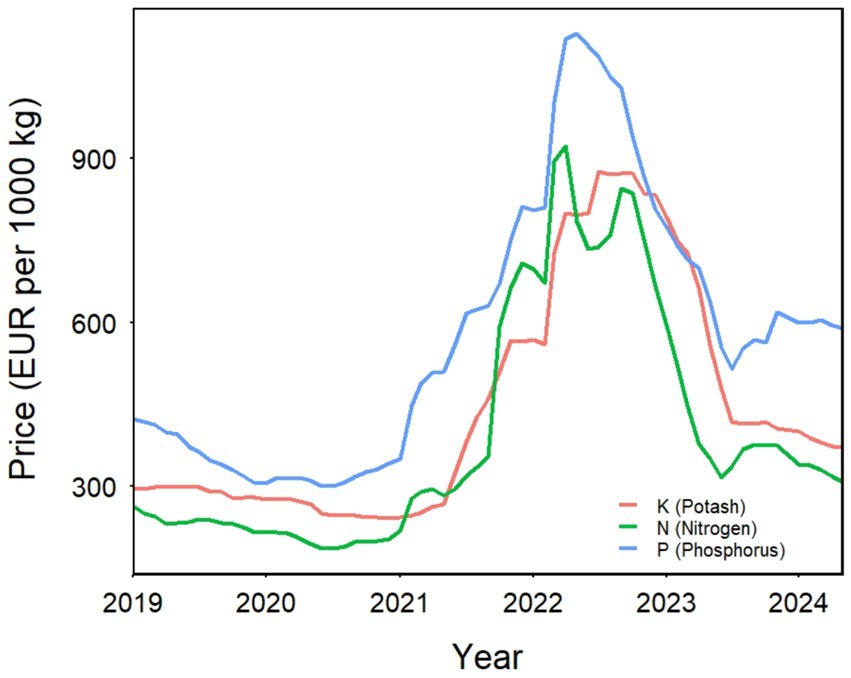
Figure 3. Fertilizer price evolution in EU, elaborated with data from AGRIDATA (2023).
The pricing and competitive position of BBF compared to mineral fertilizers are influenced by many factors: demand, availability of adequate BBFs, logistics, local legislation, and regional fertilization practices (Case et al., 2017; Kvakkestad et al., 2023). Previous studies recommended pricing of BBF significantly lower than mineral fertilizers (Case et al., 2017; Tur-Cardona et al., 2018). However, Moshkin et al. (2023) considered the willingness-to-pay and perceptions of potential users in an EU farmer survey and suggested that BBFs should be priced equivalently to mineral fertilizers. While willingness-to-pay may still be lower for BBFs compared to mineral fertilizers, marketing strategies could help justify higher prices and contribute to the development of the BBF industry. Moreover, branding of BBFs plays an important role in their adoption. For example, using the term “biosolids” instead of “treated sewage sludge” positively impacts consumer attitudes and acceptance (Lu et al., 2012).
6 Discussion
The EU’s initiatives like the Farm to Fork strategy and the new EU Fertilizer Regulation signal a shift toward a more circular nutrient economy, with BBFs playing a crucial role. However, agronomic and environmental challenges persist for BBFs. The main challenge identified is the uncertainty surrounding the quantity and availability of nutrients, as well as the lack of adequate nutrient ratios in biowastes (Egan et al., 2022).
Many biowastes have deficiencies in their nutrient compositions when used as organic fertilizers, and these deficiencies can be exacerbated by transformation treatments applied to biowastes. However, deficiencies can be corrected through chemical treatments or pre-treatments that enhance the nutritional properties of the transformation outputs (e.g., digestate). Despite these improvements, many BBFs still have limitations compared to the practicality of more concentrated and stable mineral fertilizers. To ensure adequate nutrient quantities and ratios, organo-mineral combinations are a promising alternative (Deeks et al., 2013; Sitzmann et al., 2024), as well as improved processes of nutrient recovery in waste management facilities (Marchuk et al., 2023). To maximize the agricultural efficiency of BBFs, including organo-mineral combinations, it is essential to use them alongside appropriate placement strategies. This approach ensures that the benefits of both organic and mineral components are fully realized, promoting sustainable and effective fertilizations.
The current economic conditions, marked by high prices of mineral fertilizers and changes to the EU Fertilizer Regulation, present an unique opportunity for the growth of the BBF market. However, barriers such as negative perceptions of BBF and logistical issues hinder their adoption by farmers. Improving perception could be achieved by offering BBF products that match mineral fertilizers in both agronomic efficiency and physical attributes. To improve perception and acceptance of BBFs, it is imperative to work with stakeholders and end-users of BBFs and include them in research and development processes to address their logistical and agricultural needs (Nedelciu et al., 2019; Venegas et al., 2021). While agricultural efficiency is crucial, it is also important for BBFs to provide additional benefits to the soil, like addition of organic matter (Case et al., 2017). Practicality is another key element, along with customer and societal acceptance of products fertilized with BBFs. It is one of the most crucial factors to develop to unlock the BBF market. Literature has evidenced a demand for BBFs that offer the same convenience, meaning they are always available, can be applied without major logistical complications, and are designed for the needs of a specific crop, i.e., commercial products.
Based on the literature review, we propose that to enhance BBF acceptance among farmers and growers, it is important to demonstrate that: (1) BBFs have sufficient and reliable nutrient amounts, as well as crop-adequate nutrient ratios: (2) BBF can maintain or improve soil fertility by being a supply of organic matter to soils; (3) BBFs need to be priced competitively with mineral fertilizers, but not significantly cheaper than mineral fertilizers. The close pricing ensures that BBFs remain an attractive alternative while covering costs of production and innovation.; (4) Handling and application of BBF with existing machinery is possible; (5) There is willingness among consumers of the farmer’s products to buy products fertilized with BBFs.
Increasing refinement of bio-based fertilizing products will be associated with higher energy and material consumption, and environmental impacts. For instance, pollution swapping caused by some treatment techniques may result in trade-offs between the agronomic and environmental benefits of BBFs. To overcome this, integrated measures that consider local conditions are crucial. Multiple LCAs have shown that the sustainability of BBFs is strongly influenced by local factors such as available biowastes, electricity sources, local legislation, soil and climatic conditions, which ultimately determine the agronomic value and environmental impacts (Jensen et al., 2020; Beyers et al., 2022). Thus, local, and small to middle-scale production of BBFs utilizing regional biowastes may be a more optimal solution that centralized, large-scale national facilities. Similarly, transforming waste management facilities like wastewater treatment plants into nutrient recovery centers, is an important aspect of locally sourcing BBFs.
Strategies of fertilization with BBFs should also consider adequate timing and placement to maximize nutrient efficiency and minimize nutrient losses to the environment (Lemming et al., 2016; Maris et al., 2021). Therefore, effective agricultural extension services are needed to assist farmers in transitioning to BBFs and ensure that they are used and placed appropriately to maximize their fertilizer value.
7 Conclusion
Maximizing the potential of BBFs in the European context and overcoming existing barriers requires a comprehensive assessment. We acknowledge that the agricultural use of BBFs at present could be demanding for farmers, given the regulatory framework, the scarcity of new, innovative BBFs, the planning required to ensure proper nutrient supply to crops, and the potential reluctance of consumers to buy products that have been fertilized with BBFs. It is crucial to carefully consider legislative, logistical, economic, soil fertility, and climatic requirements, with a primary focus on the crop’s nutrient needs to develop BBFs that can be adopted by farmers. Therefore, substantial research and product development efforts are still required to overcome these barriers and provide viable alternatives. However, the goal is to achieve a circular nutrient economy and not only substitute mineral fertilizers with BBFs. Therefore, it is important for all stakeholders to recognize the value of producing and consuming products fertilized with recycled nutrients, understanding how their purchase contributes to a more circular economy and sustainable development in general.
Author contributions
MÁ: Writing – review & editing, Writing – original draft, Visualization, Project administration, Methodology, Investigation, Formal analysis, Data curation, Conceptualization. PS: Writing – review & editing, Writing – original draft, Visualization, Formal analysis, Data curation, Conceptualization. TS: Writing – review & editing, Writing – original draft, Formal analysis, Data curation, Conceptualization. MR: Writing – review & editing, Writing – original draft, Conceptualization. JN: Writing – review & editing, Writing – original draft, Methodology, Formal Analysis, Conceptualization. EM: Writing – review & editing, Writing – original draft, Visualization, Conceptualization. JM: Writing – review & editing, Writing – original draft, Validation. CK: Writing – review & editing, Writing – original draft. HC: Writing – review & editing, Writing – original draft. AU-J: Writing – review & editing, Writing – original draft. SC: Writing – review & editing, Writing – original draft. FL: Writing – review & editing, Writing – original draft. KW: Writing – review & editing, Writing – original draft. YZ: Writing – review & editing, Writing – original draft. SH: Writing – review & editing, Writing – original draft. DC: Writing – review & editing, Writing – original draft, Supervision. LJ: Writing – review & editing, Writing – original draft, Supervision, Conceptualization.
Funding
The author(s) declare that financial support was received for the research, authorship, and/or publication of this article. This project has received funding from the European Union’s Horizon 2020 research and innovation program under the Marie Sklodowska-Curie grant agreement no. 860127. Open access funding by ETH Zürich.
Acknowledgments
We would like to thank Astrid Oberson from ETH Zürich for her feedback on the later version of this manuscript and valuable discussions.
Conflict of interest
The authors declare that this review was conducted in the absence of any commercial or financial relationships that could be regarded as a potential conflict of interest.
The author(s) declared that they were an editorial board member of Frontiers, at the time of submission. This had no impact on the peer review process and the final decision.
Publisher’s note
All claims expressed in this article are solely those of the authors and do not necessarily represent those of their affiliated organizations, or those of the publisher, the editors and the reviewers. Any product that may be evaluated in this article, or claim that may be made by its manufacturer, is not guaranteed or endorsed by the publisher.
Footnotes
1. ^The latest version of the draft amendment of the FPR was elaborated on October 2, 2023, and can be consulted under the document name Ares(2023)6629522.
References
AGRIDATA (2023). Fertiliser prices. Directorate-General for Agriculture and Rural Development: European Commission. Available at: https://agridata.ec.europa.eu/extensions/DataPortal/fertiliser.html.
Aguirre-Villegas, H. A., Larson, R. A., and Sharara, M. A. (2019). Anaerobic digestion, solid-liquid separation, and drying of dairy manure: measuring constituents and modeling emission. Sci. Total Environ. 696:134059. doi: 10.1016/J.SCITOTENV.2019.134059
Alengebawy, A., Mohamed, B. A., Jin, K., Liu, T., Ghimire, N., Samer, M., et al. (2022). A comparative life cycle assessment of biofertilizer production towards sustainable utilization of anaerobic digestate. Sustainable Prod. Consump. 33, 875–889. doi: 10.1016/J.SPC.2022.08.016
Alexander, P., Arneth, A., Henry, R., Maire, J., Rabin, S., and Rounsevell, M. D. A. (2022). High energy and fertilizer prices are more damaging than food export curtailment from Ukraine and Russia for food prices, health and the environment. Nature Food 4, 84–95. doi: 10.1038/s43016-022-00659-9
Alvarez-Gaitan, J. P., Short, M. D., Lundie, S., and Stuetz, R. (2016). Towards a comprehensive greenhouse gas emissions inventory for biosolids. Water Res. 96, 299–307. doi: 10.1016/J.WATRES.2016.03.059
Ameziane, H., Nounah, A., Khamar, M., and Zouahri, A. (2020). Composting olive pomace: evolution of organic matter and compost quality. Agron. Res. 18, 5–17. doi: 10.15159/AR.20.004
Anderson, C., Malambo, D. H., Perez, M. E. G., Nobela, H. N., de Pooter, L., Spit, J., et al. (2015). Lactic acid fermentation, urea and lime addition: promising Faecal sludge sanitizing methods for emergency sanitation. Int. J. Environ. Res. Public Health 12, 13871–13885. doi: 10.3390/IJERPH121113871
Anetor, M. O., and Omueti, J. A. I. (2014). Organo-mineral fertilizer effects in some phosphorus-unresponsive soils of southwestern Nigeria: 1. Effects on maize growth response and soil properties. Agric. Biol. J. N. Am. 5, 265–280. doi: 10.5251/abjna.2014.5.6.265.280
Antille, D. L., Sakrabani, R., and Godwin, R. J. (2014). Nitrogen release characteristics from biosolids-derived Organomineral fertilizers. Commun. Soil Sci. Plant Anal. 45, 1687–1698. doi: 10.1080/00103624.2014.907915
Antille, D. L., Sakrabani, R., Tyrrel, S. F., Le, M. S., and Godwin, R. J. (2013). Development of organomineral fertilisers derived from nutrient-enriched biosolids granules: product specification. Amer. Soci. Agricul. Biol. Eng. Ann. Int. Meet. 2013 5, 4152–4170. doi: 10.13031/aim.20131620153
Babalola, O., Oshunsanya, S. O., and Are, K. (2007). Effects of vetiver grass (Vetiveria nigritana) strips, vetiver grass mulch and an organomineral fertilizer on soil, water and nutrient losses and maize (Zea mays, L) yields. Soil Tillage Res. 96, 6–18. doi: 10.1016/j.still.2007.02.008
Baral, K. R., Pedersen, I. F., Rubæk, G. H., and Sørensen, P. (2021). Placement depth and distribution of cattle slurry influence initial maize growth and phosphorus and nitrogen uptake. J. Plant Nutr. Soil Sci. 184, 461–470. doi: 10.1002/jpln.202000492
Barnett, G. M. (1994). Phosphorus forms in animal manure. Bioresour. Technol. 49, 139–147. doi: 10.1016/0960-8524(94)90077-9
Barquet, K., Järnberg, L., Rosemarin, A., and Macura, B. (2020). Identifying barriers and opportunities for a circular phosphorus economy in the Baltic Sea region. Water Res. 171:115433. doi: 10.1016/J.WATRES.2019.115433
Beyers, M., Duan, Y. F., Jensen, L. S., and Bruun, S. (2022). Effect of natural and regulatory conditions on the environmental impacts of pig slurry acidification across different regions in Europe: a life cycle assessment. J. Clean. Prod. 368:133072. doi: 10.1016/J.JCLEPRO.2022.133072
Bosshard, C., Oberson, A., Leinweber, P., Jandl, G., Knicker, H., Wettstein, H. R., et al. (2011). Characterization of fecal nitrogen forms produced by a sheep fed with 15N labeled ryegrass. Nutr. Cycl. Agroecosyst. 90, 355–368. doi: 10.1007/S10705-011-9437-1
Bouwman, L., Goldewijk, K. K., Van Der Hoek, K. W., Beusen, A. H. W., Van Vuuren, D. P., Willems, J., et al. (2013). Exploring global changes in nitrogen and phosphorus cycles in agriculture induced by livestock production over the 1900-2050 period. Proc. Natl. Acad. Sci. USA 110, 20882–20887.
Brod, E., Øgaard, A. F., Hansen, E., Wragg, D., Haraldsen, T. K., and Krogstad, T. (2015). Waste products as alternative phosphorus fertilisers part I: inorganic P species affect fertilisation effects depending on soil pH. Nutr. Cycl. Agroecosyst. 103, 167–185. doi: 10.1007/s10705-015-9734-1
Brownlie, W. J., Sutton, M. A., Cordell, D., Reay, D. S., Heal, K. V., Withers, P. J. A., et al. (2023). Phosphorus price spikes: a wake-up call for phosphorus resilience. Front. Sustainable Food Syst. 7:1088776. doi: 10.3389/FSUFS.2023.1088776
Bruun, S., Yoshida, H., Nielsen, M. P., Jensen, L. S., Christensen, T. H., and Scheutz, C. (2016). Estimation of long-term environmental inventory factors associated with land application of sewage sludge. J. Clean. Prod. 126, 440–450. doi: 10.1016/J.JCLEPRO.2016.03.081
Buysse, J., and Cardona, J. T. (2020). “Economics of biobased products and their mineral counterparts”, in Biorefinery of Inorganics. eds. E. Meers, G. Velthof, E. Michels, and R. Rietra. John Wiley & Sons, Ltd. 311–328.
Cao, Y., Wang, X., Liu, L., Velthof, G. L., Misselbrook, T., Bai, Z., et al. (2020). Acidification of manure reduces gaseous emissions and nutrient losses from subsequent composting process. J. Environ. Manag. 264:110454. doi: 10.1016/J.JENVMAN.2020.110454
Carlsson, M., Lagerkvist, A., and Morgan-Sagastume, F. (2012). The effects of substrate pre-treatment on anaerobic digestion systems: a review. Waste Manag. 32, 1634–1650. doi: 10.1016/J.WASMAN.2012.04.016
Carneiro, J. S. d. S., Ribeiro, I. C. A., Nardis, B. O., Barbosa, C. F., Lustosa Filho, J. F., and Melo, L. C. A. (2021). Long-term effect of biochar-based fertilizers application in tropical soil: agronomic efficiency and phosphorus availability. Sci. Total Environ. 760:143955. doi: 10.1016/J.SCITOTENV.2020.143955
Carpenter, S. R., and Bennett, E. M. (2011). Reconsideration of the planetary boundary for phosphorus. Environ. Res. Lett. 6:014009. doi: 10.1088/1748-9326/6/1/014009
Case, S. D. C., Oelofse, M., Hou, Y., Oenema, O., and Jensen, L. S. (2017). Farmer perceptions and use of organic waste products as fertilisers – a survey study of potential benefits and barriers. Agric. Syst. 151, 84–95. doi: 10.1016/J.AGSY.2016.11.012
Chaves, C., Canet, R., Albiach, R., Marín, J., and Pomares, F. (2004). Meat and bone meal: fertilizing value and rates of nitrogen mineralization. Sustainable Organic Waste Management for Environmental Protection and Food Safety. ed. M. P. Bernal FAO and CSIC. 177–180.
Chojnacka, K., Moustakas, K., and Witek-Krowiak, A. (2020). Bio-based fertilizers: a practical approach towards circular economy. Bioresour. Technol. 295:122223. doi: 10.1016/J.BIORTECH.2019.122223
Chowdhury, M. A., de Neergaard, A., and Jensen, L. S. (2014). Composting of solids separated from anaerobically digested animal manure: effect of different bulking agents and mixing ratios on emissions of greenhouse gases and ammonia. Biosyst. Eng. 124, 63–77. doi: 10.1016/j.biosystemseng.2014.06.003
Christiansen, N. H., Sørensen, P., Labouriau, R., Christensen, B. T., and Rubæk, G. H. (2020). Characterizing phosphorus availability in waste products by chemical extractions and plant uptake. J. Plant Nutr. Soil Sci. 183, 416–428. doi: 10.1002/JPLN.202000015
Chrysanthopoulos, S., Coutinho, J., Silva, A. C., Brito, L., and Fangueiro, D. (2024). Agro-industrial by-products as alternative additives for the agronomic valorization of pig slurry through pH modification. J. Clean. Prod. 434:140052. doi: 10.1016/J.JCLEPRO.2023.140052
Corradini, F., Meza, P., Eguiluz, R., Casado, F., Huerta-Lwanga, E., and Geissen, V. (2019). Evidence of microplastic accumulation in agricultural soils from sewage sludge disposal. Sci. Total Environ. 671, 411–420. doi: 10.1016/J.SCITOTENV.2019.03.368
Cottis, T., Mousavi, H., and Solberg, S. Ø. (2023). Plasma treated cattle slurry moderately increases cereal yields. Agronomy 13:1549. doi: 10.3390/AGRONOMY13061549
Crossman, J., Hurley, R. R., Futter, M., and Nizzetto, L. (2020). Transfer and transport of microplastics from biosolids to agricultural soils and the wider environment. Sci. Total Environ. 724:138334. doi: 10.1016/J.SCITOTENV.2020.138334
de Castro, H., Sigurnjak, I., Robles-Aguilar, A., Adriaens, A., and Meers, E. (2023). Development of conversion factors to estimate the concentrations of heavy metals in manure-derived digestates. Waste Manag. 168, 334–343. doi: 10.1016/j.wasman.2023.06.008
de Guardia, A., Mallard, P., Teglia, C., Marin, A., Le Pape, C., Launay, M., et al. (2010). Comparison of five organic wastes regarding their behaviour during composting: part 2, nitrogen dynamic. Waste Manag. 30, 415–425. doi: 10.1016/J.WASMAN.2009.10.018
Deeks, L., Chaney, K., Murray, C., Sakrabani, R., Gedara, S., Le, M., et al. (2013). A new sludge-derived organo-mineral fertilizer gives similar crop yields as conventional fertilizers. Agronom. sustain. Dev. 33. doi: 10.1007/s13593-013-0135-zï
Delin, S., Engström, L., and Lundkvist, A. (2018). Optimal placement of meat bone meal pellets to spring oats. Front. Sustain. Food Syst. 2:27. doi: 10.3389/FSUFS.2018.00027
Ding, A., Zhang, R., Ngo, H. H., He, X., Ma, J., Nan, J., et al. (2021). Life cycle assessment of sewage sludge treatment and disposal based on nutrient and energy recovery: a review. Sci. Total Environ. 769:144451. doi: 10.1016/J.SCITOTENV.2020.144451
Dion, P. P., Jeanne, T., Thériault, M., Hogue, R., Pepin, S., and Dorais, M. (2020). Nitrogen release from five organic fertilizers commonly used in greenhouse organic horticulture with contrasting effects on bacterial communities. Can. J. Soil Sci. 100, 120–135.
Donner, M., Gohier, R., and de Vries, H. (2020). A new circular business model typology for creating value from agro-waste. Sci. Total Environ. 716:137065. doi: 10.1016/J.SCITOTENV.2020.137065
EC (2019). Regulation (EU) 2019/1009 of the European Parliament and of the Council of 5 June 2019 laying down rules on the making available on the market of EU fertilising products and amending regulation (EC) no 1069/2009 and (EC) no 1107/2009 and repealing Regulat. Off. J. Eur. Union 2019, 1–114.
EC (2022). “Frequently asked questions” in European Comission: Regulation (EU) 2019/1009 – The Fertilising products regulation.
Egan, A., Saju, A., Sigurnjak, I., Meers, E., and Power, N. (2022). What are the desired properties of recycling-derived fertilisers from an end-user perspective? Clean Respons Consum. 5. doi: 10.1016/j.clrc.2022.100057
Egas, D., Azarkamand, S., Casals, C., Ponsá, S., Llenas, L., and Colón, J. (2023). Life cycle assessment of bio-based fertilizers production systems: where are we and where should we be heading? Int. J. Life Cycle Assess. 28, 626–650. doi: 10.1007/s11367-023-02168-8
Einarsson, R., Sanz-Cobena, A., Aguilera, E., Billen, G., Garnier, J., van Grinsven, H. J. M., et al. (2021). Crop production and nitrogen use in European cropland and grassland 1961–2019. Scientific Data 8, 1–29. doi: 10.1038/s41597-021-01061-z
Ekane, N., Barquet, K., and Rosemarin, A. (2021). Resources and risks: perceptions on the application of sewage sludge on agricultural land in Sweden, a Case study. Frontiers in Sustainable Food Systems 5:94. doi: 10.3389/fsufs.2021.647780
Emmerling, C., Krein, A., and Junk, J. (2020). Meta-analysis of strategies to reduce NH3 emissions from slurries in European agriculture and consequences for greenhouse gas emissions. Agronomy 10:1633. doi: 10.3390/AGRONOMY10111633
ESPP . (2023). Proposed ESPP position paper on the definition of “Bio-Based Fertiliser.” Available at: https://phosphorusplatform.eu/images/download/ProposedESPPpositionBio-Based%20Fertilisersv8_2_23.pdf
European Commission . (2019). Communication from the Commission to the European Parliament, the European Council, the Council, the European economic and social Committee and the Committee of the Regions. In The European green Deal. COM.
European Commission . (2020). Communication from the commission to the European parliament, the council, the European economic and social committee and the committee of the regions a new circular economy action plan for a cleaner and more competitive Europe. COM/2020/98 final. Available at: https://eur-lex.europa.eu/legal-content/EN/TXT/?uri=COM%3A2020%3A98%3AFIN
Eurostats . (2023). Agri-environmental indicator - mineral fertiliser consumption. Available at: https://ec.europa.eu/eurostat/statistics-explained/index.php?title=Agri-environmental_indicator_-_mineral_fertiliser_consumption
Fangueiro, D., Hjorth, M., and Gioelli, F. (2015). Acidification of animal slurry– a review. J. Environ. Manag. 149, 46–56. doi: 10.1016/J.JENVMAN.2014.10.001
Fangueiro, D., Snauwaert, E., Provolo, G., Hidalgo, D., Adani, F., Kabbe, C., et al. (2017). Mini-paper–available technologies for nutrients recovery from animal manure and digestates. EIP-AGRI Focus Group-Nutr. Recycling.
FAO . (2021). FAOSTAT analytical brief series no 18. Emissions due to agriculture. Global, regional and country trends 2000-2018.
Fayiga, A. O., and Nwoke, O. C. (2016). Phosphate rock: origin, importance, environmental impacts, and future roles. In. Environ. Rev. 24, 403–415. doi: 10.1139/er-2016-0003
Florio, A., Felici, B., Migliore, M., Dell’Abate, M. T., and Benedetti, A. (2016). Nitrogen losses, uptake and abundance of ammonia oxidizers in soil under mineral and organo-mineral fertilization regimes. J. Sci. Food Agric. 96, 2440–2450. doi: 10.1002/jsfa.7364
Foley, J. A., Ramankutty, N., Brauman, K. A., Cassidy, E. S., Gerber, J. S., Johnston, M., et al. (2011). Solutions for a cultivated planet. Nature 478, 337–342. doi: 10.1038/nature10452
Freiling, M., von Tucher, S., and Schmidhalter, U. (2022). Factors influencing phosphorus placement and effects on yield and yield parameters: a meta-analysis. Soil Tillage Res. 216:105257. doi: 10.1016/j.still.2021.105257
Frossard, E., Bauer, J. P., and Lothe, F. (1997). Evidence of vivianite in FeSO4 - flocculated sludges. Water Res. 31, 2449–2454. doi: 10.1016/S0043-1354(97)00101-2
Frossard, E., Skrabal, P., Sinaj, S., Bangerter, F., and Traore, O. (2002). Forms and exchangeability of inorganic phosphate in composted solid organic wastes. Nutr. Cycl. Agroecosyst. 62, 103–113. doi: 10.1023/A:1015596526088
Gao, Y., and Cabrera Serrenho, A. (2023). Greenhouse gas emissions from nitrogen fertilizers could be reduced by up to one-fifth of current levels by 2050 with combined interventions. Nature Food 4, 170–178. doi: 10.1038/s43016-023-00698-w
Garmendia-Lemus, S., Moshkin, E., Hung, Y., Tack, J., and Buysse, J. (2024). European farmers’ perceptions and intentions to use bio-based fertilisers: insights from the theory of planned behaviour and perceived utility. J. Clean. Prod. 434:139755. doi: 10.1016/J.JCLEPRO.2023.139755
Geng, H., Xu, Y., Zheng, L., Gong, H., Dai, L., and Dai, X. (2020). An overview of removing heavy metals from sewage sludge: achievements and perspectives. Environ. Pollut. 266:115375. doi: 10.1016/J.ENVPOL.2020.115375
Gianico, A., Braguglia, C. M., Gallipoli, A., Montecchio, D., and Mininni, G. (2021). Land application of biosolids in Europe: Possibilities, con-Straints and future perspectives. Water. 13. doi: 10.3390/w13010103
Glæsner, N., Kjaergaard, C., Rubæk, G. H., and Magid, J. (2011). Interactions between soil texture and placement of dairy slurry application: I. Flow characteristics and leaching of nonreactive components. J. Environ. Qual. 40, 337–343. doi: 10.2134/JEQ2010.0317
Goldstein, N., and Beecher, N. (2007). Invest in the social aspects of biosolids management. Water Practice Technol. 2. doi: 10.2166/WPT.2007.082
Grant, C. A., and Flaten, D. N. (2019). 4R Management of Phosphorus Fertilizer in the northern Great Plains. J. Environ. Qual. 48, 1356–1369. doi: 10.2134/jeq2019.02.0061
Grant, C. A., Flaten, D. N., Tomasiewicz, D. J., and Sheppard, S. C. (2001). The importance of early season phosphorus nutrition. Can. J. Plant Sci. 81, 211–224. doi: 10.4141/P00-093
Graves, D. B., Bakken, L. B., Jensen, M. B., and Ingels, R. (2019). Plasma activated organic fertilizer. Plasma Chem. Plasma Process. 39, 1–19. doi: 10.1007/s11090-018-9944-9
Gregson, N., Crang, M., Fuller, S., and Holmes, H. (2015). Interrogating the circular economy: the moral economy of resource recovery in the EU. Econ. Soc. 44, 218–243. doi: 10.1080/03085147.2015.1013353
Gwara, S., Wale, E., Odindo, A., and Buckley, C. (2021). Attitudes and perceptions on the agricultural use of human excreta and human excreta derived materials: a scoping review. Agriculture 11:153. doi: 10.3390/AGRICULTURE11020153
Gwenzi, W., Nyambishi, T. J., Chaukura, N., and Mapope, N. (2018). Synthesis and nutrient release patterns of a biochar-based N–P–K slow-release fertilizer. Int. J. Environ. Sci. Technol. 15, 405–414. doi: 10.1007/S13762-017-1399-7
Hafner, S. D., and Bisogni, J. J. (2009). Modeling of ammonia speciation in anaerobic digesters. Water Res. 43, 4105–4114. doi: 10.1016/J.WATRES.2009.05.044
Harder, R., Giampietro, M., and Smukler, S. (2021). Towards a circular nutrient economy. A novel way to analyze the circularity of nutrient flows in food systems. Resour. Conserv. Recycl. 172:105693. doi: 10.1016/J.RESCONREC.2021.105693
Hermann, L., and Hermann, R. (2021). Business Case evaluation report. Available at: https://systemicproject.eu/wp-content/uploads/D2.2-Business-Case-Evaluation-Report-vfin3_201203_post-eva_rev_201204.pdf
Heyl, K., Ekardt, F., Roos, P., and Garske, B. (2023). Achieving the nutrient reduction objective of the farm to fork strategy. An assessment of CAP subsidies for precision fertilization and sustainable agricultural practices in Germany. Front. Sustain. Food Syst. 7:1088640. doi: 10.3389/FSUFS.2023.1088640/BIBTEX
Higgins, J. (2001). Environmental aspects of phosphate and potash mining. In J. Aloisi Larderelde and M. Prud’homme (Eds.), Na : Vol. NA (Issu. United Nations Environment Programme, International Fertilizer Industry Association. Available at: www.uneptie.org
Hills, K., Yorgey, G., and Cook, J. (2021). Demand for bio-based fertilizers from dairy manure in Washington state: a small-scale discrete choice experiment. Renew. Agricul. Food Syst. 36, 207–214. doi: 10.1017/S174217052000023X
Hjorth, M., Christensen, K. V., Christensen, M. L., and Sommer, S. G. (2009). Solid-liquid separation of animal slurry in theory and practice. Sustain. Agricult. 2, 953–986. doi: 10.1007/978-94-007-0394-0_43
Holly, M. A., Larson, R. A., Powell, J. M., Ruark, M. D., and Aguirre-Villegas, H. (2017). Greenhouse gas and ammonia emissions from digested and separated dairy manure during storage and after land application. Agric. Ecosyst. Environ. 239, 410–419. doi: 10.1016/J.AGEE.2017.02.007
Hou, Y., Velthof, G. L., and Oenema, O. (2015). Mitigation of ammonia, nitrous oxide and methane emissions from manure management chains: a meta-analysis and integrated assessment. Glob. Chang. Biol. 21, 1293–1312. doi: 10.1111/GCB.12767
Hudcová, H., Vymazal, J., and Rozkošný, M. (2019). Present restrictions of sewage sludge application in agriculture within the European Union. Soil Water Res 14, 104–120. doi: 10.17221/36/2018-SWR
Hušek, M., Moško, J., and Pohořelý, M. (2022). Sewage sludge treatment methods and P-recovery possibilities: current state-of-the-art. J. Environ. Manag. 315:115090. doi: 10.1016/J.JENVMAN.2022.115090
Imadi, S. R., Waseem, S., Kazi, A. G., Azooz, M. M., and Ahmad, P. (2016). “Aluminum toxicity in plants” in Plant metal interaction (Elsevier), 1–20.
Jameson, M., White, J. G., Osmond, D. L., and Aziz, T. (2016). Determination of biosolids phosphorus solubility and its relationship to wastewater treatment. Water Environ. Res. 88, 602–610. doi: 10.2175/106143016x14609975746406
Jarecki, M. K., Lal, R., Stewart, B. A., and Texas, W. (2003). Crop Management for Soil Carbon Sequestration. Crit. Rev. Plant Sci. 22, 471–502. doi: 10.1080/713608318
Jeng, A. S., Haraldsen, T. K., Grønlund, A., and Pedersen, P. A. (2007). “Meat and bone meal as nitrogen and phosphorus fertilizer to cereals and rye grass” in Advances in integrated soil fertility management in sub-Saharan Africa: Challenges and opportunities. eds. A. Bationo, B. Waswa, J. Kihara, and J. Kimetu (Springer), 245–253.
Jensen, L. S., Oelofse, M., ten Hoeve, M., and Bruun, S. (2020). Environmental impact assessment on the production and use of biobased fertilizers. Biorefinery Inorganics, 329–362. doi: 10.1002/9781118921487.CH7-1
Jensen, L. S., Thornton, C., Forrestal, P., Brandsma, J., Külvet, A., Riiko, K., et al., (2017). Mini-paper-end-user requirements for recycled and bio-based fertiliser products. European Commission EIP-AGRI Focus Groups-Nutrient Recycling. Available at: https://ec.europa.eu/eip/agriculture/sites/default/files/fg19_minipaper_6_end_user_requirements_en.pdf
Jiang, L., Dang, Q., Zhao, X., Zhang, C., Tan, X., and Yan, Q. (2022). Mechanism of microbial involvement in nitrogen conversion affecting methane production in dry anaerobic digestion. J. Clean. Prod. 369:133324. doi: 10.1016/J.JCLEPRO.2022.133324
Kadam, R., Khanthong, K., Jang, H., Lee, J., and Park, J. (2022). Occurrence, fate, and implications of heavy metals during anaerobic digestion: a review. Energies 15:8618. doi: 10.3390/EN15228618/S1
Kanteraki, A. E., Isari, E. A., Svarnas, P., and Kalavrouziotis, I. K. (2022). Biosolids: the Trojan horse or the beautiful Helen for soil fertilization? Sci. Total Environ. 839:156270. doi: 10.1016/j.scitotenv.2022.156270
Kar, S., Singh, R., Gurian, P. L., Hendricks, A., Kohl, P., McKelvey, S., et al. (2023). Life cycle assessment and techno-economic analysis of nitrogen recovery by ammonia air-stripping from wastewater treatment. Sci. Total Environ. 857:159499. doi: 10.1016/J.SCITOTENV.2022.159499
Keskinen, R., Hyväluoma, J., Nikama, J., Sainio, T., and Ylivainio, K. (2023). Improving plant availability of recycled phosphorus with organic acids. Environ. Technol. Innovation 32:103401. doi: 10.1016/j.eti.2023.103401
Kopp, C., Sica, P., Eising, A. G., Madsen, D. E., Magid, J., and Müller-Stöver, D. S. (2023a). Enhancing phosphorus fertilizer value of sewage sludge ashes and biochars through chemical pre-treatments and application methods. Waste Biomass Valorization 1, 1–17. doi: 10.1007/S12649-023-02351-W
Kopp, C., Sica, P., Lu, C., Tobler, D., Jensen, L. S., and Müller-Stöver, D. (2023b). Increasing phosphorus plant availability from P-rich ashes and biochars by acidification with sulfuric acid. J. Environ. Chem. Eng. 111489. doi: 10.1016/J.JECE.2023.111489
Kummu, M., de Moel, H., Porkka, M., Siebert, S., Varis, O., and Ward, P. J. (2012). Lost food, wasted resources: global food supply chain losses and their impacts on freshwater, cropland, and fertiliser use. Sci. Total Environ. 438, 477–489. doi: 10.1016/J.SCITOTENV.2012.08.092
Kupper, T., Bürge, D., Bachmann, H. J., Güsewell, S., and Mayer, J. (2014). Heavy metals in source-separated compost and digestates. Waste Manag. 34, 867–874. doi: 10.1016/J.WASMAN.2014.02.007
Kupper, T., Häni, C., Neftel, A., Kincaid, C., Bühler, M., Amon, B., et al. (2020). Ammonia and greenhouse gas emissions from slurry storage-a review. Ecosystems Environ. 300:106963. doi: 10.1016/j.agee.2020.106963
Kurniawati, A., Stankovics, P., Hilmi, Y. S., Toth, G., Smol, M., and Toth, Z. (2023a). Understanding the future of bio-based fertilisers: the EU’s policy and implementation. Sustain. Chem. Climate Action 3:100033.
Kurniawati, A., Toth, G., Ylivainio, K., and Toth, Z. (2023b). Opportunities and challenges of bio-based fertilizers utilization for improving soil health. Organic Agricul. 13, 335–350. doi: 10.1007/s13165-023-00432-7
Kvakkestad, V., Brod, E., Flø, B. E., Hanserud, O., and Helgesen, H. (2023). Circulation of nutrients through bio-based fertilizer products: perspectives from farmers, suppliers, and civil society. Front. Sustain. Food Syst. 7:1239353. doi: 10.3389/FSUFS.2023.1239353/BIBTEX
Langmeier, M., Frossard, E., Kreuzer, M., Mäder, P., Dubois, D., and Oberson, A. (2002). Nitrogen fertilizer value of cattle manure applied on soils originating from organic and conventional farming systems. Agronomie 22, 789–800. doi: 10.1051/AGRO:2002044
Lassaletta, L., Billen, G., Grizzetti, B., Anglade, J., and Garnier, J. (2014). 50 year trends in nitrogen use efficiency of world cropping systems: the relationship between yield and nitrogen input to cropland. Environ. Res. Lett. 9:105011. doi: 10.1088/1748-9326/9/10/105011
Leite, A., Melo, L. C. A., Hurtarte, L. C. C., Zuin, L., Piccolla, C. D., Werder, D., et al. (2023). Magnesium-enriched poultry manure enhances phosphorus bioavailability in biochars. Chemosphere 331:138759. doi: 10.1016/j.chemosphere.2023.138759
Lemming, C., Bruun, S., Jensen, L. S., and Magid, J. (2017). Plant availability of phosphorus from dewatered sewage sludge, untreated incineration ashes, and other products recovered from a wastewater treatment system. J. Plant Nutr. Soil Sci. 180, 779–787. doi: 10.1002/jpln.201700206
Lemming, C., Oberson, A., Hund, A., Jensen, L. S., and Magid, J. (2016). Opportunity costs for maize associated with localised application of sewage sludge derived fertilisers, as indicated by early root and phosphorus uptake responses. Plant Soil 406, 201–217. doi: 10.1007/S11104-016-2865-6
Lemming, C., Oberson, A., Magid, J., Bruun, S., Scheutz, C., Frossard, E., et al. (2019). Residual phosphorus availability after long-term soil application of organic waste. Agric. Ecosyst. Environ. 270–271, 65–75. doi: 10.1016/j.agee.2018.10.009
Li, B., Boiarkina, I., Yu, W., Huang, H. M., Munir, T., Wang, G. Q., et al. (2019). Phosphorous recovery through struvite crystallization: challenges for future design. Sci. Total Environ. 648, 1244–1256. doi: 10.1016/J.SCITOTENV.2018.07.166
Limwikran, T., Kheoruenromne, I., Suddhiprakarn, A., Prakongkep, N., and Gilkes, R. J. (2018). Dissolution of K, ca, and P from biochar grains in tropical soils. Geoderma 312, 139–150. doi: 10.1016/J.GEODERMA.2017.10.022
Lindsay, W. L. (1972). Inorganic phase equilibria of micronutrients in soils. In: Micronutrients in Agriculture. Soil Sci. Soc. Am. eds. J. J. Mortvedt, P. M. Giordano, and W. L. Lindsay (Wisconsin: Madison), 41–57.
Liu, Q., Fang, Z., Liu, Y., Liu, Y., Xu, Y., Ruan, X., et al. (2019). Phosphorus speciation and bioavailability of sewage sludge derived biochar amended with CaO. Waste Manag. 87, 71–77. doi: 10.1016/j.wasman.2019.01.045
Logan, M., and Visvanathan, C. (2019). Management strategies for anaerobic digestate of organic fraction of municipal solid waste: current status and future prospects. Waste Manag. Res. 37, 27–39.
Lu, Q., He, Z. L., and Stoffella, P. J. (2012). Land application of biosolids in the USA: a review. Appl. Environ. Soil Sci. 2012:11. doi: 10.1155/2012/201462
Luo, H., Dewitte, K., Landschoot, S., Sigurnjak, I., Robles-Aguilar, A. A., Michels, E., et al. (2022). Benefits of biobased fertilizers as substitutes for synthetic nitrogen fertilizers: field assessment combining minirhizotron and UAV-based spectrum sensing technologies. Front. Environ. Sci. 10:988932. doi: 10.3389/FENVS.2022.988932/BIBTEX
Luo, W., Qian, L., Liu, W., Zhang, X., Wang, Q., Jiang, H., et al. (2021). A potential mg-enriched biochar fertilizer: excellent slow-release performance and release mechanism of nutrients. Sci. Total Environ. 768:144454. doi: 10.1016/J.SCITOTENV.2020.144454
Magid, J., Luxhi, J., Jensen, L. S., Mller, J., and Bruun, S. (2006). “Establishment of a long-term field trial with urban fertilizers - is recycling of nutrients from urban areas to peri-urban organic farms feasible?” in Long-term field experiments in organic farming. eds. J. Raupp, C. Pekrun, M. Oltmanns, and U. Köpke. Verlag Dr. Köster 59–78.
Malinowska, E. (2017). The effect of liming and sewage sludge application on heavy metal speciation in soil. Bull. Environ. Contam. Toxicol. 98, 105–112. doi: 10.1007/S00128-016-1984-3
Mandal, A., Patra, A. K., Singh, D., Swarup, A., and Ebhin Masto, R. (2007). Effect of long-term application of manure and fertilizer on biological and biochemical activities in soil during crop development stages. Bioresour. Technol. 98, 3585–3592. doi: 10.1016/J.BIORTECH.2006.11.027
Marchuk, S., Tait, S., Sinha, P., Harris, P., Antille, D. L., and McCabe, B. K. (2023). Biosolids-derived fertilisers: a review of challenges and opportunities. Sci. Total Environ. 875:162555. doi: 10.1016/J.SCITOTENV.2023.162555
Maris, S. C., Abalos, D., Capra, F., Moscatelli, G., Scaglia, F., Cely Reyes, G. E., et al. (2021). Strong potential of slurry application timing and method to reduce N losses in a permanent grassland. Agric. Ecosyst. Environ. 311:107329. doi: 10.1016/J.AGEE.2021.107329
Mateo-Sagasta, J., Raschid-Sally, L., and Thebo, A. (2015). Global wastewater and sludge production, treatment and use. Wastewater, 15–38. doi: 10.1007/978-94-017-9545-6_2
Mayer, M., Krause, H.-M., Fliessbach, A., Mäder, P., and Steffens, M. (2022). Fertilizer quality and labile soil organic matter fractions are vital for organic carbon sequestration in temperate arable soils within a long-term trial in Switzerland. Geoderma 426:116080. doi: 10.1016/j.geoderma.2022.116080
Mazeika, R., Dambrauskas, T., Baltakys, K., Mikolajunas, M., Staugaitis, G., Virzonis, D., et al. (2016). Molecular and morphological structure of poultry manure derived Organo-mineral fertilizers (OMFs). ACS Sustain. Chem. Eng. 4, 4788–4796.
McConville, J. R., Metson, G. S., and Persson, H. (2023). Acceptance of human excreta derived fertilizers in Swedish grocery stores. City Environ. Interactions 17:100096. doi: 10.1016/J.CACINT.2022.100096
Meegoda, J. N., Li, B., Patel, K., and Wang, L. B. (2018). A review of the processes, parameters, and optimization of anaerobic digestion. Int. J. Environ. Res. Public Health 15. doi: 10.3390/IJERPH15102224
Meneses-Quelal, O., and Velázquez-Martí, B. (2020). Pretreatment of animal manure biomass to improve biogas production: a review. Energies 13:3573. doi: 10.3390/EN13143573
Meyer, G., Bell, M. J., Kopittke, P. M., Lombi, E., Doolette, C. L., Brunetti, G., et al. (2023). Mobility and lability of phosphorus from highly concentrated fertiliser bands. Geoderma 429. doi: 10.1016/J.GEODERMA.2022.116248
Meyer, G., Frossard, E., Mäder, P., Nanzer, S., Randall, D. G., Udert, K. M., et al. (2018). Water soluble phosphate fertilizers for crops grown in calcareous soils – an outdated paradigm for recycled phosphorus fertilizers? Plant Soil 424, 367–388. doi: 10.1007/s11104-017-3545-x
Mininni, G., Blanch, A. R., Lucena, F., and Berselli, S. (2015). EU policy on sewage sludge utilization and perspectives on new approaches of sludge management. Environ. Sci. Pollut. Res. 22, 7361–7374. doi: 10.1007/s11356-014-3132-0
Möller, K., and Müller, T. (2012). Effects of anaerobic digestion on digestate nutrient availability and crop growth: a review. Eng. Life Sci. 12, 242–257. doi: 10.1002/ELSC.201100085
Möller, K., Oberson, A., Bünemann, E. K., Cooper, J., Friedel, J. K., Glæsner, N., et al. (2018). Improved phosphorus recycling in organic farming: navigating between constraints. Adv. Agron. 147, 159–237. doi: 10.1016/BS.AGRON.2017.10.004
Møller, H. B., Sørensen, P., Olesen, J. E., Petersen, S. O., Nyord, T., and Sommer, S. G. (2022). Agricultural biogas production—climate and environmental impacts. Sustainability 14:1849. doi: 10.3390/SU14031849/S1
Moraes, L. E., Burgos, S. A., DePeters, E. J., Zhang, R., and Fadel, J. G. (2017). Short communication: urea hydrolysis in dairy cattle manure under different temperature, urea, and pH conditions. J. Dairy Sci. 100, 2388–2394. doi: 10.3168/JDS.2016-11927
Moshkin, E., Garmendia Lemus, S., Bamelis, L., and Buysse, J. (2023). Assessment of willingness-to-pay for bio-based fertilisers among farmers and agricultural advisors in the EU. J. Clean. Prod. 414:137548. doi: 10.1016/J.JCLEPRO.2023.137548
Muntwyler, A., Panagos, P., Pfister, S., and Lugato, E. (2024). Assessing the phosphorus cycle in European agricultural soils: looking beyond current national phosphorus budgets. Sci. Total Environ. 906:167143. doi: 10.1016/J.SCITOTENV.2023.167143
Muscolo, A., Papalia, T., Settineri, G., Romeo, F., and Mallamaci, C. (2019). Three different methods for turning olive pomace in resource: benefits of the end products for agricultural purpose. Sci. Total Environ. 662, 1–7. doi: 10.1016/J.SCITOTENV.2019.01.210
Nanzer, S., Oberson, A., Berger, L., Berset, E., Hermann, L., and Frossard, E. (2014). The plant availability of phosphorus from thermo-chemically treated sewage sludge ashes as studied by 33P labeling techniques. Plant Soil 377, 439–456. doi: 10.1007/s11104-013-1968-6
Nanzer, S., Oberson, A., Eggenberger, U., and Frossard, E. (2019). Predicting phosphate release from sewage sludge ash using an ion sink assay. J. Environ. Qual. 48, 746–754. doi: 10.2134/JEQ2018.11.0394
Ndegwa, P. M., Hristov, A. N., Arogo, J., and Sheffield, R. E. (2008). A review of ammonia emission mitigation techniques for concentrated animal feeding operations. Biosyst. Eng. 100, 453–469. doi: 10.1016/J.BIOSYSTEMSENG.2008.05.010
Nedelciu, C. E., Ragnarsdóttir, K. V., and Stjernquist, I. (2019). From waste to resource: a systems dynamics and stakeholder analysis of phosphorus recycling from municipal wastewater in Europe. Ambio 48, 741–751. doi: 10.1007/S13280-018-1097-9
Neijat, M., House, J. D., Guenter, W., and Kebreab, E. (2011). Calcium and phosphorus dynamics in commercial laying hens housed in conventional or enriched cage systems. Poult. Sci. 90, 2383–2396. doi: 10.3382/PS.2011-01401
Nigussie, A., Bruun, S., Kuyper, T. W., and de Neergaard, A. (2017). Delayed addition of nitrogen-rich substrates during composting of municipal waste: effects on nitrogen loss, greenhouse gas emissions and compost stability. Chemosphere 166, 352–362. doi: 10.1016/J.CHEMOSPHERE.2016.09.123
Nkebiwe, P. M., Weinmann, M., Bar-Tal, A., and Müller, T. (2016). “Fertilizer placement to improve crop nutrient acquisition and yield: a review and meta-analysis” in Field crops research, vol. 196 (Elsevier B.V), 389–401.
Nyang’au, J. O., Møller, H. B., Larsen, S. U., and Sørensen, P. (2023a). Brown juice assisted ensiling of straw and press cake for enhanced biogas production and nutrient availability in digestates. Environ. Technol. Innov. 32:103248. doi: 10.1016/j.eti.2023.103248
Nyang'au, J. O., Møller, H. B., and Sørensen, P. (2023b). Effects of electrokinetic and ultrasonication pre-treatment and two-step anaerobic digestion of biowastes on the nitrogen fertiliser value by injection or surface banding to cereal crops. J. Environ. Manag. 326:116699. doi: 10.1016/J.JENVMAN.2022.116699
Osorio-Tejada, J., Tran, N. N., and Hessel, V. (2022). Techno-environmental assessment of small-scale Haber-Bosch and plasma-assisted ammonia supply chains. Sci Total Environ. 826. doi: 10.1016/j.scitotenv.2022.154162
Overmeyer, V., Trimborn, M., Clemens, J., Hölscher, R., and Büscher, W. (2023). Acidification of slurry to reduce ammonia and methane emissions: deployment of a retrofittable system in fattening pig barns. J. Environ. Manag. 331:117263. doi: 10.1016/j.jenvman.2023.117263
Pain, B., and Menzi, H. (2011). Glossary of terms on livestock manure management. RAMIRAN. Available at: http://ramiran.net/doc11/RAMIRAN Glossary_2011.pdf
Paré, M. C., Allaire, S. E., Parent, L. É., and Khiari, L. (2010). Variation in the physical properties of organo-mineral fertilisers with proportion of solid pig slurry compost. Biosyst. Eng. 106, 243–249. doi: 10.1016/J.BIOSYSTEMSENG.2010.02.009
Pedersen, J., Andersson, K., Feilberg, A., Delin, S., Hafner, S., and Nyord, T. (2021). Effect of exposed surface area on ammonia emissions from untreated, separated, and digested cattle manure. Biosyst. Eng. 202, 66–78. doi: 10.1016/J.BIOSYSTEMSENG.2020.12.005
Pedersen, J., and Hafner, S. D. (2023). Ammonia emissions after field application of anaerobically digested animal slurry: literature review and perspectives. Agric. Ecosyst. Environ. 357:108697. doi: 10.1016/J.AGEE.2023.108697
Pedersen, J., and Nyord, T. (2023). Effect of low-dose acidification of slurry digestate on ammonia emissions after field application (Short communication). Atmos. Environ. 17:100205. doi: 10.1016/J.AEAOA.2023.100205
Pedersen, I. F., Rubaek, G. H., and Sørensen, P. (2017). Cattle slurry acidification and application method can improve initial phosphorus availability for maize. Plant Soil 414, 143–158. doi: 10.1007/s11104-016-3124-6
Peltre, C., Nyord, T., Bruun, S., Jensen, L. S., and Magid, J. (2015). Repeated soil application of organic waste amendments reduces draught force and fuel consumption for soil tillage. Agric. Ecosyst. Environ. 211, 94–101. doi: 10.1016/J.AGEE.2015.06.004
Poore, J., and Nemecek, T. (2018). Reducing food’s environmental impacts through producers and consumers. Science 360, 987–992. doi: 10.1126/SCIENCE.AAQ0216
Poulsen, H., and Kristensen, V. (1998). DIAS report No.7 Animal Husbandry: Standard Values for Farm Manure.
Powlson, D. S., and Dawson, C. J. (2022). Use of ammonium sulphate as a Sulphur fertilizer: implications for ammonia volatilization. Soil Use Manag. 38, 622–634. doi: 10.1111/SUM.12733
Prado, J., Chieppe, J., Raymundo, A., and Fangueiro, D. (2020). Bio-acidification and enhanced crusting as an alternative to sulphuric acid addition to slurry to mitigate ammonia and greenhouse gases emissions during short term storage. J. Clean. Prod. 263:121443. doi: 10.1016/j.jclepro.2020.121443
Quinn, D. J., Lee, C. D., and Poffenbarger, H. J. (2020). Corn yield response to sub-surface banded starter fertilizer in the U.S.: a meta-analysis. Field Crop Res. 254:107834. doi: 10.1016/j.fcr.2020.107834
Rabbi, M. F., Ben Hassen, T., El Bilali, H., Raheem, D., and Raposo, A. (2023). Food security challenges in Europe in the context of the prolonged Russian–Ukrainian conflict. Sustain. For. 15:4745. doi: 10.3390/SU15064745
Rady, M. M. (2012). A novel organo-mineral fertilizer can mitigate salinity stress effects for tomato production on reclaimed saline soil. S. Afr. J. Bot. 81, 8–14. doi: 10.1016/j.sajb.2012.03.013
Raworth, K. (2017). A doughnut for the Anthropocene: humanity’s compass in the 21st century. Lancet Planet. Health 1, e48–e49. doi: 10.1016/S2542-5196(17)30028-1
Regueiro, I., Siebert, P., Liu, J., Müller-Stöver, D., and Jensen, L. S. (2020). Acidified animal manure products combined with a nitrification inhibitor can serve as a starter fertilizer for maize. Agronomy 2020. 10:1941. doi: 10.3390/AGRONOMY10121941
Reid, K., Schneider, K., and McConkey, B. (2018). Components of phosphorus loss from agricultural landscapes, and how to incorporate them into risk assessment tools. Front. Earth Sci. 6:356053. doi: 10.3389/FEART.2018.00135
Richards, J. E., Daigle, J.-Y., LeBlanc, P., Paulin, R., and Ghanem, I. (1993). Nitrogen availability and nitrate leaching from organo-mineral fertilizers. Can. J. Soil Sci. 73, 197–208. doi: 10.4141/cjss93-022
Richardson, K., Steffen, W., Lucht, W., Bendtsen, J., Cornell, S. E., Donges, J. F., et al. (2023). Earth beyond six of nine planetary boundaries. Science. Advances 9. doi: 10.1126/SCIADV.ADH2458
Ritzén, S., and Sandström, G. Ö. (2017). Barriers to the circular economy – integration of perspectives and domains. Procedia CIRP 64, 7–12. doi: 10.1016/J.PROCIR.2017.03.005
Rockström, J., Steffen, W., Noone, K., Persson, Å., Chapin, F. S. I. I. I., Lambin, E., et al. (2009). Planetary boundaries: exploring the safe operating space for humanity. Ecol. Soc. 14:32. doi: 10.5751/ES-03180-140232
Rodrigues, J., Alvarenga, P., Silva, A., Brito, L., Tavares, J., Fangueiro, D., et al. (2021). Animal slurry sanitization through pH adjustment: process optimization and impact on slurry characteristics. Agronomy 11:517. doi: 10.3390/AGRONOMY11030517
Rodrigues, M. M., Viana, D. G., Oliveira, F. C., Alves, M. C., and Regitano, J. B. (2021). Sewage sludge as organic matrix in the manufacture of organomineral fertilizers: physical forms, environmental risks, and nutrients recycling. J. Clean. Prod. 313. doi: 10.1016/j.jclepro.2021.127774
Rodríguez-Alegre, R., Zapata-Jiménez, J., You, X., Pérez-Moya, M., Sanchis, S., and García-Montaño, J. (2023). Nutrient recovery and valorisation from pig slurry liquid fraction with membrane technologies. Sci. Total Environ. 874:162548. doi: 10.1016/j.scitotenv.2023.162548
Romio, C., Vedel, M., Kofoed, W., Møller, H. B., Lohani, P., and Siciliano, A. (2021). Digestate post-treatment strategies for additional biogas recovery: a review. Sustain. For. 13:9295. doi: 10.3390/SU13169295
Rydgård, M., Jensen, L. S., Kroeze, C., Strokal, M., Möller, K., and Bruun, S. (2024). Regionalised modelling of recycled fertiliser P in agricultural fields: development of the life cycle inventory model PLCI 2.0. J. Clean. Prod. 443:141088. doi: 10.1016/J.JCLEPRO.2024.141088
Sandström, V., Kaseva, J., Porkka, M., Kuisma, M., Sakieh, Y., and Kahiluoto, H. (2023). Disparate history of transgressing planetary boundaries for nutrients. Glob. Environ. Chang. 78:102628. doi: 10.1016/J.GLOENVCHA.2022.102628
Schengel, L., and Goehlich, V. (2024). Adaptation of the doughnut economics model to a rural Community in Germany. Palgrave Stud. Cross-Discip. Busi. Res. Assoc. EuroMed Acad. Busi. Part F1799, 261–284. doi: 10.1007/978-3-031-37365-7_12/FIGURES/3
Schoumans, O. F., Bouraoui, F., Kabbe, C., Oenema, O., and van Dijk, K. C. (2015). Phosphorus management in Europe in a changing world. Ambio 44, 180–192. doi: 10.1007/s13280-014-0613-9
Schreiber, M., Bazaios, E., Ströbel, B., Wolf, B., Ostler, U., Gasche, R., et al. (2023). Impacts of slurry acidification and injection on fertilizer nitrogen fates in grassland. Nutr. Cycl. Agroecosyst. 125, 171–186. doi: 10.1007/S10705-022-10239-9
Segrè Cohen, A., Love, N. G., Nace, K. K., and Árvai, J. (2020). Consumers’ acceptance of agricultural fertilizers derived from diverted and recycled human urine. Environ. Sci. Technol. 54, 5297–5305. doi: 10.1021/acs.est.0c00576
Sena, M., Seib, M., Noguera, D. R., and Hicks, A. (2021). Environmental impacts of phosphorus recovery through struvite precipitation in wastewater treatment. J. Clean. Prod. 280:124222. doi: 10.1016/j.jclepro.2020.124222
Severin, M., Fuß, R., Well, R., Hähndel, R., and Van den Weghe, H. (2016). Greenhouse gas emissions after application of digestate: short-term effects of nitrification inhibitor and application technique effects. Arch. Agron. Soil Sci. 62, 1007–1020. doi: 10.1080/03650340.2015.1110575
Sharpley, A. N. (1996). Availability of residual phosphorus in manured soils. Soil Sci. Soc. Am. J. 60, 1459–1466. doi: 10.2136/SSSAJ1996.03615995006000050025X
Shober, A. L., and Maguire, R. O. (2018). Manure Management. Refer. Mod. Earth Syst. Environ. Sci. doi: 10.1016/B978-0-12-409548-9.09115-6
Sica, P., Kopp, C., Müller-Stöver, D. S., and Magid, J. (2023). Acidification and alkalinization pretreatments of biowastes and their effect on P solubility and dynamics when placed in soil. J. Environ. Manag. 333. doi: 10.1016/J.JENVMAN.2023.117447
Silva, L. F. O., Oliveira, M. L. S., Crissien, T. J., Santosh, M., Bolivar, J., Shao, L., et al. (2022). A review on the environmental impact of phosphogypsum and potential health impacts through the release of nanoparticles. Chemosphere 286:131513. doi: 10.1016/J.CHEMOSPHERE.2021.131513
Simha, P., Lalander, C., Vinnerås, B., and Ganesapillai, M. (2017). Farmer attitudes and perceptions to the re-use of fertiliser products from resource-oriented sanitation systems - the case of Vellore, South India. Sci. Total Environ. 581–582, 885–896. doi: 10.1016/J.SCITOTENV.2017.01.044
Sitzmann, T. J., Sica, P., Grignani, C., and Magid, J. (2024). Testing biowaste materials as peat replacement in organo-mineral fertilizers. Front. Sustain. Food Syst. 8:1330843. doi: 10.3389/FSUFS.2024.1330843
Smith, P. (2016). Soil carbon sequestration and biochar as negative emission technologies. Glob. Chang. Biol. 22, 1315–1324. doi: 10.1111/gcb.13178
Smith, W. B., Wilson, M., and Pagliari, P. (2020). “Organomineral fertilizers and their application to field crops” in Animal manure: Production, characteristics, environmental concerns, and management (John Wiley & Sons, Ltd.), 229–244. doi: 10.2134/asaspecpub67.c18
Smol, M. (2021). Transition to circular economy in the fertilizer sector—analysis of recommended directions and end-users’ perception of waste-based products in Poland. Energies 14:4312. doi: 10.3390/EN14144312
Sørensen, P., and Møller, H. B. (2009). “Fate of nitrogen in pig and cattle slurries applied to the soil-crop system” in Anaerobic digestion: Opportunities for agriculture and environment. eds. F. Adani, A. Schievano, and G. Boccasile. 27–37.
Sorensen, J. N., and Thorup-Kristensen, K. (2011). Plant-based fertilizers for organic vegetable production. J. Plant Nutr. Soil Sci. 174, 321–332. doi: 10.1002/jpln.200900321
Sporchia, F., and Caro, D. (2023). Exploring the potential of circular solutions to replace inorganic fertilizers in the European Union. Sci. Total Environ. 892:164636. doi: 10.1016/J.SCITOTENV.2023.164636
Styles, D., Adams, P., Thelin, G., Vaneeckhaute, C., Chadwick, D., and Withers, P. J. A. (2018). Life Cycle Assessment of Biofertilizer Production and Use Compared with Conventional Liquid Digestate Management. Environ. Sci. Technol. 52, 7468–7476. doi: 10.1021/acs.est.8b01619
Tariq, A., Larsen, K. S., Hansen, L. V., Jensen, L. S., and Bruun, S. (2022). Effect of nitrification inhibitor (DMPP) on nitrous oxide emissions from agricultural fields: automated and manual measurements. Sci. Total Environ. 847:157650. doi: 10.1016/J.SCITOTENV.2022.157650
Tate, K. R. (1984). The biological transformation of P in soil. Biological Processes Soil Fertility 76, 245–256. doi: 10.1007/978-94-009-6101-2_22
Tur-Cardona, J., Bonnichsen, O., Speelman, S., Verspecht, A., Carpentier, L., Marchand, F., et al., (2018). Farmers’ reasons to accept bio-based fertilizers: a choice experiment in seven different European countries. J. Clean. Produc. 197, 406–416. doi: 10.1016/j.jclepro.2018.06.172
Ural-Janssen, A., Kroeze, C., Lesschen, J. P., Meers, E., Van Puijenbroek, P. J. T. M., and Strokal, M. (2023). Hotspots of nutrient losses to air and water: an integrated modeling approach for European River basins. Front. Agricul. Sci. Eng. 10, 579–592. doi: 10.15302/j-fase-2023526
Venegas, C., Sánchez-Alfonso, A. C., Celis, C., Vesga, F. J., and Mendez, M. G. (2021). Management strategies and stakeholders analysis to strengthen the management and use of biosolids in a Colombian municipality. Sustain. For. 13:12180. doi: 10.3390/SU132112180
Verordnung Über Umweltgefahrdende Stoffe (StoV, Swiss: Ordinance on Environmentally Hazardous Substances) (2002).
Vu, P. T., Melse, R. W., Zeeman, G., and Groot Koerkamp, P. W. G. (2016). Composition and biogas yield of a novel source segregation system for pig excreta. Biosyst. Eng. 145, 29–38. doi: 10.1016/J.BIOSYSTEMSENG.2016.02.005
Walling, E., and Vaneeckhaute, C. (2020). Greenhouse gas emissions from inorganic and organic fertilizer production and use: a review of emission factors and their variability. J. Environ. Manag. 276:111211. doi: 10.1016/J.JENVMAN.2020.111211
Wang, Q., Raju, C. S., Almind-Jørgensen, N., Laustrup, M., Reitzel, K., and Nielsen, U. G. (2022). Variation in phosphorus speciation of sewage sludge throughout three wastewater treatment plants: determined by sequential extraction combined with microscopy, NMR spectroscopy, and powder X-ray diffraction. Environ. Sci. Technol. 56, 8975–8983.
Wester-Larsen, L., Müller-Stöver, D. S., Salo, T., and Jensen, L. S. (2022). Potential ammonia volatilization from 39 different novel biobased fertilizers on the European market – a laboratory study using 5 European soils. J. Environ. Manag. 323:116249. doi: 10.1016/J.JENVMAN.2022.116249
Winter, L. R., and Chen, J. G. (2021). N2 fixation by plasma-activated processes. Joule 5, 300–315. doi: 10.1016/J.JOULE.2020.11.009
Witek-Krowiak, A., Gorazda, K., Szopa, D., Trzaska, K., Moustakas, K., and Chojnacka, K. (2022). Phosphorus recovery from wastewater and bio-based waste: an overview. Bioengineered 13:13474. doi: 10.1080/21655979.2022.2077894
Yoshida, H., ten Hoeve, M., Christensen, T. H., Bruun, S., Jensen, L. S., and Scheutz, C. (2018). Life cycle assessment of sewage sludge management options including long-term impacts after land application. J. Clean. Prod. 174, 538–547. doi: 10.1016/J.JCLEPRO.2017.10.175
You, L., Ros, G. H., Chen, Y., Shao, Q., Young, M. D., Zhang, F., et al. (2023). Global mean nitrogen recovery efficiency in croplands can be enhanced by optimal nutrient, crop and soil management practices. Nat. Commun. 14, 1–12. doi: 10.1038/s41467-023-41504-2
Yu, X., Keitel, C., and Dijkstra, F. A. (2021). Global analysis of phosphorus fertilizer use efficiency in cereal crops. Glob. Food Sec. 29:100545. doi: 10.1016/j.gfs.2021.100545
Zabaleta, I., and Rodic, L. (2015). Recovery of essential nutrients from municipal solid waste – impact of waste management infrastructure and governance aspects. Waste Manag. 44, 178–187. doi: 10.1016/J.WASMAN.2015.07.033
Zhang, J., Wu, Z., Huang, Y., Zhan, X., Zhang, Y., and Cai, C. (2023). Industrial-scale composting of swine manure with a novel additive-yellow phosphorus slag: variation in maturity indicators, compost quality and phosphorus speciation. Bioresour. Technol. 384:129356. doi: 10.1016/J.BIORTECH.2023.129356
Zireeni, Y., Jones, D., and Chadwick, D. (2023). Influence of slurry acidification with H2SO4 on soil pH, N, P, S, and C dynamics: incubation experiment. Environ. Adv. 14:100447. doi: 10.1016/j.envadv.2023.100447
Keywords: sewage sludge, bio-based fertilizer, compost, circular economy, fertilizer
Citation: Álvarez Salas M, Sica P, Rydgård M, Sitzmann TJ, Nyang’au JO, El Mahdi J, Moshkin E, de Castro e Silva HL, Chrysanthopoulos S, Kopp C, Wali K, Zireeni Y, Ural-Janssen A, El Hajj Hassan S, Kebalo LF, Chadwick D and Jensen LS (2024) Current challenges on the widespread adoption of new bio-based fertilizers: insights to move forward toward more circular food systems. Front. Sustain. Food Syst. 8:1386680. doi: 10.3389/fsufs.2024.1386680
Edited by:
José Luis Garcia-Morales, University of Cádiz, SpainReviewed by:
Stefan Hörtenhuber, University of Natural Resources and Life Sciences Vienna, AustriaJosé Manuel Rato Nunes, Polytechnic Institute of Portalegre, Portugal
Copyright © 2024 Álvarez Salas, Sica, Rydgård, Sitzmann, Nyang’au, El Mahdi, Moshkin, de Castro e Silva, Chrysanthopoulos, Kopp, Wali, Zireeni, Ural-Janssen, El Hajj Hassan, Kebalo, Chadwick and Jensen. This is an open-access article distributed under the terms of the Creative Commons Attribution License (CC BY). The use, distribution or reproduction in other forums is permitted, provided the original author(s) and the copyright owner(s) are credited and that the original publication in this journal is cited, in accordance with accepted academic practice. No use, distribution or reproduction is permitted which does not comply with these terms.
*Correspondence: Mario Álvarez Salas, bWFyaW8uYWx2YXJlekB1c3lzLmV0aHouY2g=