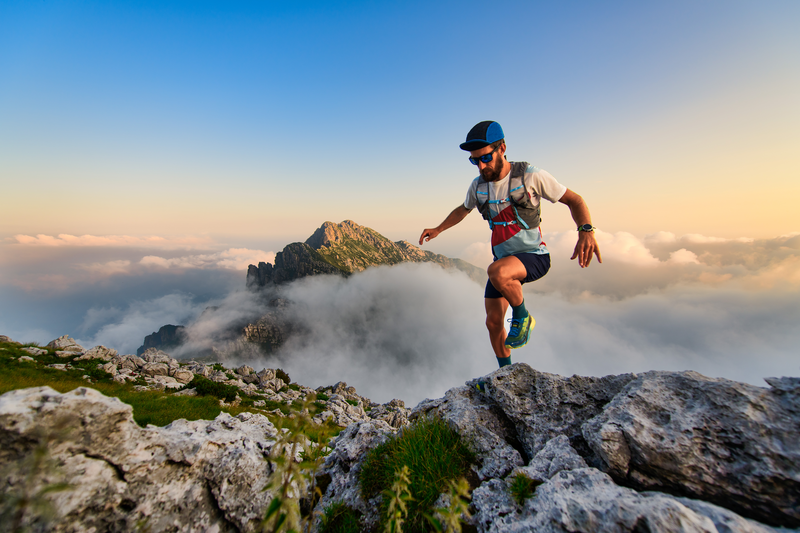
95% of researchers rate our articles as excellent or good
Learn more about the work of our research integrity team to safeguard the quality of each article we publish.
Find out more
ORIGINAL RESEARCH article
Front. Sustain. Food Syst. , 23 July 2024
Sec. Sustainable Food Processing
Volume 8 - 2024 | https://doi.org/10.3389/fsufs.2024.1386519
This article is part of the Research Topic Fermented-Based Foods As Sustainable Alternative Sources To Meet Future Demand For Protein View all 8 articles
Cheese whey is an industrial by-product that is generated in excess during the cheese production process in the dairy industry. Despite the potential utility of whey, it continues to pose environmental threats in the industry. This study comprehensively evaluates the utilization of two fermentation techniques (solid-state fermentation and submerged fermentation) for producing fungal biomass from cheese whey powder, employing Aspergillus oryzae, Rhizopus oryzae, and Neurospora intermedia for sustainable food production. It has been observed that submerged fermentation is more effective in increasing the protein content of whey powder compared to solid-state fermentation. The highest biomass yield was achieved with A. oryzae (5.29 g/L, 0.176 g biomass/g substrate), followed by N. intermedia (3.63 g/L, 0.121 g biomass/g substrate), and R. oryzae (1.9 g/L, 0.063 g biomass/g substrate). In the bubble column reactor, the protein content of the substrate (78.65 g/kg) increased by 165.54 and 176.69% with A. oryzae (208.85 g/kg) and N. intermedia (217.62 g/kg), respectively. This study has demonstrated that whey powder can be converted into protein-rich biomass through fungal bioconversion. The obtained biomass has the potential to be developed as an alternative food and feed source, contributing to waste management and sustainable food production.
The increasing food demand in parallel with the human population growth will pose growing challenges in accessing a diet that contains sufficient nutrients in the future. Moreover, the availability of current plant and animal-based food sources is climate-dependent and can have long-term adverse effects on the environment. The anticipated challenges to be faced in the future have accelerated efforts to explore alternative sources for transitioning towards a more sustainable diet (Gastaldello et al., 2022). The Food and Agriculture Organization (FAO) defines a sustainable diet as one that has a minimal environmental footprint while promoting food and nutrition security and ensuring the well-being of both current and future generations (Atta-Delgado et al., 2023). In recent years, researchers have focused towards investigating the biomass obtained from filamentous fungi due to their significant nutritional content for global food sustainability (Derbyshire et al., 2023).
In cheese making, whey is the greenish-yellow liquid that separates from the curd when milk, cream, and/or skimmed milk coagulate with the addition of acid or rennin enzyme (Karagül-Yüceer et al., 2003; Şen and Yüceer, 2019). Typically, 9 kg of whey is generated during the production of 1 kg of cheese (Valdez Castillo et al., 2021). While whey is edible, it becomes the primary pollutant in the dairy industry if left untreated due to its rich composition (Zotta et al., 2020; Sar et al., 2022a). The untreated release of cheese whey, characterized by high organic and inorganic loads, poses environmental risks such as water body eutrophication, increased biochemical oxygen demand (BOD), soil structure accumulation, and elevated salt levels in environmental matrices (Meng et al., 2020; Valdez Castillo et al., 2021). Despite these challenges, whey finds extensive application in advancing food and feed product development, with ongoing research endeavors exploring its potential across diverse domains. Presently, 50% of whey is utilized in its liquid form, 30% is converted into powder, 15% is used for lactose and by-products, and the remaining portion is processed into protein concentrates (Barba, 2021). The powdered form of whey offers various advantages, such as ease of transportation, convenient transfer to production facilities, and the ability to be stored for extended periods under room conditions (Domínguez-Niño et al., 2018). In addition, cheese whey, owing to its lactose content, remains a substrate with high potential for transformation into value-added products through microbial fermentation (Meng et al., 2020). Recent published studies tested cheese whey as a substrate for microorganisms to synthesize various microbial compounds such as biomass, single-cell oil (microbial oil), lactate, ethanol, microbial biosurfactants, and organic acids (Sar et al., 2022a).
Filamentous fungi, composed of thread-like branched structures known as hyphae, can break down monomers in complex substrates and convert them into valuable products such as biomass (high in protein), organic acid, pigment, enzymes, alkaloids, and antibiotics (Amara and El-Baky, 2023; Niego et al., 2023). Various genera of filamentous fungi, including Aspergillus, Trichoderma, Fusarium, Mortierella, Mucor, Umbelopsis and Rhizopus, have been used in biotechnology applications (Dzurendová et al., 2021; Wikandari et al., 2023). The traditional use of filamentous fungi in food production dates back 3,000 years (Barzee et al., 2021). For instance, starter cultures, A. oryzae and A. sojae, employ their enzymes to break down soybeans, facilitating the formation of specific aroma compounds in koji and soy sauce. Additionally, N. intermedia is used in the production of Oncom, a soybean-based product, in the Indonesian island of Java (Mahboubi et al., 2017a,b). It has been reported that the fungal biomass produced can serve both as a human dietary supplement and as animal feed (Uwineza et al., 2021). Moreover, researchers have conducted numerous studies on the production of valuable products, including protein sources derived from the biological conversion of filamentous fungi using food waste and/or by-products as substrates (Mahboubi et al., 2017a; Souza Filho et al., 2018; Aruna, 2019; Hashempour-Baltork et al., 2020; Svensson et al., 2021; Borujeni et al., 2022; Yang et al., 2022).
The potential applications of whey for producing bioethanol, enzymes, and various organic acids have been extensively investigated (Sar et al., 2017; Karim and Aider, 2022; Sar et al., 2022a). Additionally, microbial oil has been extracted from filamentous fungal biomass produced from whey (Vamvakaki et al., 2010; Carota et al., 2018; Chan et al., 2018). However, although research on protein production from whey remains limited, obtaining fungal biomass and protein from whey is promising (Mahboubi et al., 2017a). Hashemi et al. (2022) also stated that fungal biomass can be produced by using co-substrate of whey and vinasse. Therefore, this study aims to investigate the effects of cheese whey powder on fungal protein-rich biomass production. In fungal cultivation, biomass and protein yields vary depending on factors such as the type of fermentation [solid-state fermentation (SSF) and submerged fermentation (SmF)], the fungal species, incubation period, and the pH conditions (Souza Filho et al., 2017; Gmoser et al., 2020; Sar et al., 2022b). The research focused on a comparative examination of A. oryzae, N. intermedia, and R. oryzae for biomass production from cheese whey powder, involving a comprehensive analysis of two fermentation techniques (SSF and SmF), to efficiently utilize the substrate. Cheese whey powder, both UV-sterilized and non-sterilized, was incubated at varying moisture levels (40, 50, and 60%) and incubation times (4, 7, and 10 days) to compare the growth of tested fungal strains in SSF. In the cultivation of fungal strains in SmF, three different fungal species were comparatively examined under two different pH conditions (non-controlled pH, and controlled pH 5.0).Then, the fungal cultivation was scaled up to 4.5 L capacity buble-column bioreactor. The biomass yield and protein content of the obtained fungal biomass were determined for their potential utilization as sustainable food. This research aims to contribute to the assessment of feedstocks for resource recovery and protein production by exploring microbial conversion strategies applied to cheese by-products generated within the dairy industry.
Aspergillus oryzae var. oryzae CBS 819.72 (Centraalnureau Voor Schimmelcultures, Utrecht, The Netherlands), Rhizopus oryzae CCUG 61.147 (Culture Collection, University of Gothenburg, Sweden), and Neurospora intermedia CBS 131.92 (Centraalbureau voor Schimmelcultures, The Netherlands) were selected to carry out the fermentation. The fungal strains were maintained on the plate of PDA (Potato Dextrose Agar). The medium contained 4 g/L potato extract (Sigma Aldrich, Buchs, Switzerland), 20 g/L glucose (Fisher Scientific, Loughborough, UK) and 15 g/L agar (Sigma, Buchs, Switzerland). To prepare the fungal inoculation, the pre-grown PDA plates were flooded with 20 mL of sterile distilled water, carefully scraped the spores using an L-shape sterile disposable plastic spreader, and the spore suspension was collected in a sterile falcon tube. Then each newly prepared PDA plate is inoculated with 100 μL spore suspension and spread using an L-shape sterile disposable plastic spreader. The fungal PDA plates were incubated at 30°C for 3 days and then stored at 4°C. The spore suspension was added to the substrate to reach a concentration ranging from 2 × 105 to 5 × 106 spores/mL (Sar et al., 2022b).
Cheese whey powder collected from a cheese manufacturing (ENKA, Konya, Türkiye) was used throughout the study. The whey powder used in the study contains 86.32% lactose (ENKA, Konya, Türkiye). The characterization of cheese whey powder is also presented in Table 1.
Solid-state fermentation of each fungal strain was carried out on UV-sterilized and non-sterilized cheese whey powder, with three different substrate moisture contents (40, 50 and 60%). UV sterilization was achieved by spreading 10 grams of cheese whey powder into a petri dish as a thin film and then exposing it to UV for 20 min. The substrate in each plate was then aseptically inoculated with 2 mL of spore suspension and then incubated in a climate cabinet (Memmert, Germany) at 35°C for 10 days (90% RH, 35% Light). All plates were photographed on days 4, 7 and 10 of the fermentation. The biomass on plates was dried using an oven at 50°C for 24 h to obtain dry biomass. The dried biomass samples were powdered with a grinder (Bosch Coffee Grinder, Germany) and the powdered samples were stored for use in protein analysis.
Fungal cultivation under SmF was conducted in 250 mL cotton plugged shake flasks containing 100 mL of 3% (w/v) cheese whey powder prepared with distilled water. The prepared substrates were sterilized before fungal inoculation in an autoclave at 121°C for 20 min (VX-95, Systec, Linden, Germany). In submerged fermentation, fungal growth was assessed at two distinct pH levels, namely initial pH (5.70, non-controlled pH) and controlled pH (5.0), for each microorganism. Each flask was then inoculated with a 2 mL fungal spore suspension, and cultivation was performed at 35°C in a water bath shaker (Grant Instruments Ltd., Cambridge, UK) at 125 rpm. After the 6, 24, 30, and 48 h of incubation, the substrate pH was adjusted to 5.0 with 2 M H2SO4. The wet fungal biomass was harvested by sieving through a screen after 48 h of cultivation. The harvested biomass was washed with water, and dried in a freeze dryer (Labconco, United States).
Cultivation of two fungal strains (A. oryzae and N. intermedia) was carried out in 4.5 L bench-scale bubble column reactors (Belach Bioteknik, Stockholm, Sweden). The bioreactors were filled with 3 L of substrate that contained 3% (w/v) of cheese whey powder (initial pH adjusted to 5.0 with 2 M H2SO4 [Sigma-Aldrich, Darmstadt, Germany)] and were sterilized at 121°C for 20 min. Then, the media were inoculated with 20 mL/L of fungal spore suspension, and the cultivation was set at 35°C with an aeration rate of 1.0 vvm (volume of air per volume of medium per minute). The pH was continuously adjusted by pumping either 2 M H2SO4 or 2 M NaOH solutions using peristaltic pumps. The biomass was harvested after 48 h and subsequently subjected to freeze-drying at 0.05 bar and − 50°C prior to analysis.
The levels of total solids, volatile solids and ash of cheese whey powder (CWP) were determined according to Braho et al. (2024). The protein content was analyzed by Kjeldahl method and a factor of 6.25 was used to determine the crude protein content (Sar et al., 2022b).
Data were presented as means ± standard error of the mean based on duplicate parallel experiments. The statistical significances were analyzed by one-way analysis of variance (ANOVA) (p < 0.05) using SPSS 23.0 (SPSS Inc., Chicago, IL, United States). The differences between the samples, and the pairwise comparisons were made using Duncan’s multiple range test.
In this study, fungal biomass production from cheese whey powder was evaluated using both solid-state fermentation and submerged fermentation through A. oryzae, R. oryzae and N. intermedia. The study investigated the effects of cheese whey powder moisture content and incubation duration on fungal growth under SSF. In SmF, the influence of varying pH conditions on fungal growth was examined in shake flasks. Then, fungal biomass yields, and their protein contents were comparatively analyzed. Subsequently, fungal biomass production was scaled up to the level of 4.5-liter capacity bubble column bioreactor and potential applications of the produced biomass were evaluated.
The characterization of cheese whey powder is presented in Table 1. Previous studies have reported that the pH range of cheese whey can vary within the range of 3 to 9 (Prazeres et al., 2012). It was determined that the pH of the cheese whey powder (6.45) used in this study was within the range. While the protein content in a typical cheese whey composition is reported to be a minimum of 12% (Chegini and Taheri, 2013), the analyzed cheese whey powder in this study was determined to have a protein content of 7.8%. The quantity of cheese whey proteins varies depending on the source of cheese whey (cheese type, type of milk), the cheese production process, and the processes and conditions employed during cheese whey powder production (Pires et al., 2021).
Initially, three fungal strains were cultivated in both UV-sterilized and non-UV-sterilized whey powder, and varying moisture contents, for 10 days,. Photographs of all petri plates were taken throughout the incubation period (days 4, 7, and 10; Figure 1). No visual growth differences were observed between UV-sterilized and non-UV-sterilized petri plates for all fungal species. Optimum growth for N. intermedia occurred with whey powder substrate containing 40% moisture, while the ideal moisture level for A. oryzae and R. oryzae was 60%. Visually, an increase in the incubation period corresponded to enhanced microbial growth for all fungal strains. The growth period of R. oryzae was 7 days, and that of A. oryzae and N. intermedia was 10 days. However, it was determined that all these factors did not increase the substrate’s protein content (Table 2). Gmoser et al. (2020) reported that the observed increase in protein content after SSF was attributed to the metabolic activity of the fungi during growth and the decomposition of the substrate into H2O and CO2. The lack of an increase in protein content in the current study may be attributed to fungus’ insufficient access to nutrients in the substrate for its growth. To solve this problem, blending whey powder with porous products, such as bread, could be more suitable for SSF (Gmoser et al., 2020). Moreover, according to the results of this study, growth was observed on the petri-dish surface for all tested fungal species. Therefore, whey powder has the potential to serve as an alternative to commercial culture media used for fungi, such as potato dextrose agar and malt extract agar. Similarly, Macwan et al. (2018) also reported that the use of cheese whey as a culture medium for biomass production by lactic acid bacteria offers a cost-effective alternative compared to commercial media.
Figure 1. UV-sterilized whey powder cultivated through solid state fermentation using fungal strains (Ao, A. oryzae; Ro, R. oryzae; Ni, N. intermedia) with different incubation times (day 4, 7 and 10) and varying moisture contents. (A) 40%, (B) 50%, and (C) 60%.
Table 2. The protein content of whey powder cultivated through solid-state fermentation using A. oryzae, R. oryzae and N. intermedia.
During submerged fungal cultivation, the growth at two parameters, namely non-controlled pH (pH 5.7) and controlled pH (pH 5.0) was evaluated for A. oryzae, R. oryzae, and N. intermedia. After 48 h of cultivation under non-controlled pH conditions, the lowest pH (6.73) was observed with N. intermedia (Figure 2A). For A. oryzae (8.02) and R. oryzae (7.97), the final pH of the medium was similar at the end of the cultivation. Similarly, under controlled pH conditions, the lowest pH at the end of cultivation was measured in N. intermedia (5.49), while the final pH values for A. oryzae and R. oryzae were 7.19 and 6.77, respectively (Figure 2B). The low pH observed at the 48 h of cultivation in N. intermedia might be associated with the formation of organic acids such as pyruvic acid and acetic acid, which contribute to the biosynthesis of various compounds, including bioethanol (Restiawaty et al., 2023).
Figure 2. Profiles of pH during 48 h cultivation of fungal strains in 3% cheese whey powder under non-controlled pH (A) and controlled pH at 5.0 (B) conditions. Error bars indicate sample standard deviations, which were determined individually for each average.
The biomass production at both pH parameters exhibited statistical differences among the fungal species (Figure 3A). The highest fungal biomass amount (based on dry weight) after the cultivation was obtained under controlled pH conditions for all test fungal species. The highest amount of biomass was observed for A. oryzae under controlled pH (5.29 g/L, p < 0.05) and non-controlled pH (3.25 g/L, p < 0.05) conditions.
Figure 3. The levels of biomass mass concentration (A) and protein contents (B) of fungal strains (A. oryzae, R. oryzae, and N. intermedia) in 3% cheese whey powder under non-controlled pH and controlled pH at 5.0. Different letters among columns of the same color indicate significant differences (p < 0.05). Error bars indicate sample standard deviations, which were determined individually for each average.
In the current study, the high biomass yield harvested from the controlled pH substrate of Aspergillus oryzae can be correlated with high enzyme activity. In fungal biomass cultivation with A. oryzae, pH varies depending on the substrate used. For example, pH 5.2 in olive oil mill wastewater (OOMW) (Sar et al., 2020a) and pH 5.0 in pomegranate peel (Braho et al., 2024), while pH 6.5 in fish processing wastewater (Sar et al., 2021) and vinasse (Karimi et al., 2019) increased biomass yield. Nair et al. (2016) observed that pH is a significant factor in the pellet formation of the N. intermedia strain and determined that the biomass yield increased at the optimum pH of 5.5. It was also observed that when the R. delemar was grown on potato protein liquor, higher amounts of fungal biomass were obtained at acidic pH values, with higher protein yield at pH levels of 4.0–4.5 (Sar et al., 2022b). It can be stated that slightly acidic conditions (pH 5.0), as obtained in this study, can be considered ideal for fungal biomass production for cheese whey. Although A. oryzae and N. intermedia are both members of the Ascomycetes, they exhibited differences in biomass yield under the same conditions. A similar trend was also observed in OOMW with 8.02 g/L biomass obtained by cultivating A. oryzae, while 2.1 g/L biomass was obtained with N. intermedia (Sar et al., 2020a). Differences were also observed between A. oryzae and N. intermedia grown on pomegranate juice and peel (Braho et al., 2024) and dairy by-products (Mahboubi et al., 2017a). In a study investigating the growth of different filamentous fungal strains in pure olive oil, A. oryzae showed superior performance compared to N. intermedia and R. oryzae in terms of biomass growth (Nazir et al., 2022). In another study, 19 g/L and 14 g/L biomass were obtained from A. oryzae and N. intermedia grown in thin stillage, respectively (Ferreira et al., 2014). Although N. intermedia yields a lower amount of biomass, it contributes to the development of bioprocesses with high protein yield and ethanol production (Ferreira et al., 2014; Parchami et al., 2021).
The hydrolysis of lactose into glucose and galactose in fungi is associated with galactosidase activity (Tonelotto et al., 2014; Kamran et al., 2017). Mahboubi et al. (2017a) proposed that galactosidase activity may vary within the pH range among Ascomycetes strains. According to their study, galactosidase produced by A. oryzae demonstrated higher expression/activity at pH <5, while the galactosidase produced by N. intermedia exhibited similar expression/activity within the pH range of 4–6. Various studies also reported the galactosidase activity of R. oryzae (Battaglia et al., 2011; Gajdhane et al., 2016). However, in the study conducted by Seyis and Aksoz (2004), it was determined that the galactosidase activity of Rhizopus sp. was low (less than 10 U/mL) during submerged fermentation on a substrate containing lactose. The low R. oryzae biomass obtained in this study may be related to the low galactosidase enzyme activity of the strain. Another factor affecting biomass production is pH changes, especially pH increases, during the cultivation. Souza Filho et al. (2017) reported that high ammonia concentration ammonia resulting from the final pH being alkaline (7.0–8.0) stopped the growth of R. oryzae. In this study, pH reached 6.97 after 24 h and 7.80 after 48 h of incubation for R. oryzae in whey substrate (Figure 2A). Although lower pH increases were observed in R. oryzae under controlled pH conditions, pH exceeded 6.50 after 48 h (Figure 2B). Additionally, Ibarruri and Hernández (2019) explained the decrease in biomass yield despite increasing whey concentration by proposing that the high concentration of lactoferrin and derived peptides in whey (> 1 g/L of whey) could potentially lead to the inhibition of R. oryzae.
In this study, the protein contents of fungal biomasses derived from A. oryzae, R. oryzae, and N. intermedia were investigated under varying pH conditions. Notable differences in protein levels among the strains were revealed by finding results. Under controlled pH conditions, the protein content of A. oryzae biomass was found to be 299.20 g/kg, while R. oryzae exhibited a slightly lower content at 265.31 g/kg. In contrast, a significantly higher protein content was displayed by N. intermedia under the same conditions. Further analysis indicated that biomass with higher protein levels was consistently produced by N. intermedia compared to the other strains, regardless of pH conditions. Specifically, both controlled pH (379.26 g/L) and non-controlled pH (385.23 g/L) conditions resulted in elevated protein levels for N. intermedia (p < 0.05). However, no significant difference in protein levels between controlled and non-controlled pH conditions was observed for A. oryzae and R. oryzae. Regarding biomass production, the highest yield was demonstrated by A. oryzae at 5.29 g/L, while N. intermedia showed the most significant increase in protein yield, reaching a 4.89-fold increase compared to initial levels. Overall, the potential of utilizing cheese whey powder through SmF to enhance protein content in fungal biomass is highlighted by our study. This increase is attributed to the production of protein-rich fungal biomass and efficient nutrient utilization from the substrate (Kumitch et al., 2020).
Among three different filamentous fungi, the highest biomass and highest protein levels in shake-flasks were obtained by A. oryzae and N. intermedia, respectively (Figure 3). Therefore, the fungal biomass and crude protein content of A. oryzae and N. intermedia were compared under controlled pH conditions in both Erlenmeyer flasks (250 mL capacity) and bubble column reactors (4.5 Liter capacities) (Figures 4, 5). Furthermore, pH changes were examined throughout the cultivation period of both fungal species in both flasks and bioreactor systems (Figure 6). Throughout the cultivation period, pH measured lower in the bioreactor scale than in Erlenmeyer-scale for fungal species. However, the final pH for Neurospora increased to 5.49 in the Erlenmeyer-scale, while it decreased to 4.63 in the bioreactor scale (Figure 6B).
Figure 4. The representation of the morphology of the wet and dry A. oryzae biomass obtained from (A) shake flasks and (B) bubble column bioreactor.
Figure 5. The representation of the morphology of the wet and dry N. intermedia biomass obtained from (A) shake flasks and (B) bubble column bioreactor.
Figure 6. Profiles of pH during 48 h cultivation of A. oryzae (A) and N. intermedia (B) in shake flasks and bubble column bioreactor. Error bars indicate sample standard deviations, which were determined individually for each average.
The biomass yield harvested from the Erlenmeyer flasks for N. intermedia, which was 3.63 g/L, was increased to 6.39 g/L at the reactor scale. For A. oryzae, the biomass level increased from 5.29 g/L to 7.05 g/L (Figure 7A). In a study conducted by Mahboubi et al. (2017a), 0.27 g biomass/g substrate by Aspergillus oryzae and 0.12 g biomass /g substrate by N. intermedia were obtained from cheese whey. In this study, the biomass yield of A. oryzae obtained from cheese whey powder (0.23 g biomass/g substrate) was in accordance with that reported by Mahboubi et al. (2017a), while a higher biomass yield of N. intermedia (0.21 g biomass/g substrate) was obtained compared to same study. In the present study, fermentation was conducted at pH 5, whereas Mahboubi et al. (2017a) studied within the pH range of 4–7. Therefore, the difference in N. intermedia biomass yield efficiency may be attributed to the fermentation pH. It was determined that the protein values of the biomass obtained from bioreactor-scale were lower (30.19% for A. oryzae, 43.50% for N. intermedia) than those obtained from Erlenmeyer-scale (Figure 7B). This phenomenon can be attributed to the rapid fungal growth leading to decrease in crude protein levels under aeration conditions (Sar et al., 2020b, 2022a). It is also suggested that the aeration rate could play a significant role in yields of biomass and protein (Ferreira et al., 2015).
Figure 7. Comparison of the growth performance of A. oryzae and N. intermedia considering the mass concentration (A) and protein content (B) of fungal biomass obtained after 48 h cultivation in shake flasks and bioreactor. Different letters between shake flasks and bubble column bioreactor indicate significant differences (p < 0.05). Error bars indicate sample standard deviations, which were determined individually for each average.
Waste management is consistently regarded as a costly process in terms of investment and/or operational expenses. However, the addition of a new product to the process will yield a positive economic impact for facilities (Mahboubi et al., 2017a). Cheese whey, particularly arising in dairy operations, is a product with high potential (Domínguez-Niño et al., 2018). Cheese whey serves as a suitable substrate for fungal bioconversion due to its nutritional content. Considering the results obtained in this study, it is believed that transforming it into a product with enhanced nutritional value through fermentation will yield greater advantages. Worldwide, the annual cheese production is estimated to be 20.5 million tons, resulting in approximately 185 million tons of whey production (Domínguez-Niño et al., 2018). Accordingly, only 55.5 million tons of whey are converted into cheese whey powder each year. According to the analysis results, this value is equivalent to an annual biomass production up to 1 million tons for A. oryzae and N. intermedia (Figure 8). From a protein perspective, it can be estimated that through bioconversion, 215 thousand tons of protein can be obtained annually with A. oryzae, and 200 thousand tons of protein with N. intermedia (Figure 8).
Figure 8. Sustainable food production from dairy industry by-products through submerged fermentation by A. oryzae and N. intermedia.
Upon evaluating fungal biomass cultivation in cheese whey powder, it is observed that the protein content of A. oryzae (20.86 ± 0.71%) and N. intermedia (21.76 ± 2.08%) is inferior to beef but similar to chicken meat (20.96–21.82%, Taşkıran et al., 2020). Nevertheless, the biomass produced in the study exhibits a higher protein content compared to that of cereals (8–15%). Moreover, the protein content of the studied N. intermedia biomass was similar to the protein content of traditionally produced red Oncom (24.16%, Andayani et al., 2020) using the same strain and visually exhibited a similar color. This could be an alternative to Oncom, which is produced from whey produced by N. intermedia.
Hence, evaluated in terms of biomass yield and protein content, the fermentation of cheese whey powder by A. oryzae and N. intermedia may provide advantages in waste management and sustainable food production.
Filamentous fungi are promising producers of protein-rich, high-quality biomass suitable for both animal/fish feed or human consumption. Among the three fungal strains tested, submerged fermentation was determined to be the most suitable fermentation to convert whey powder into protein-rich fungal biomass. Up to 0.235 g of biomass and 0.046 g of protein per gram of substrate were obtained when growing A. oryzae and N. intermedia on whey powder in the bubble-column reactor. Whey powder, a by-product of the dairy industry, could serve as a potential substrate for biotransformation to increase its nutritional value and transform it into an alternative food source.
The original contributions presented in the study are included in the article, further inquiries can be directed to the corresponding author.
BK: Formal analysis, Investigation, Methodology, Visualization, Writing – original draft, Writing – review & editing. EW: Formal analysis, Writing – review & editing. YY: Writing – review & editing, Resources, Supervision. SA: Supervision, Writing – review & editing. MT: Resources, Supervision, Writing – review & editing. TS: Conceptualization, Data curation, Investigation, Project administration, Supervision, Writing – review & editing.
The author(s) declare that financial support was received for the research, authorship, and/or publication of this article. The open access was provided by University of Borås, Sweden. BK would like to acknowledge the direct support by COST (European Cooperation in Science and Technology; www.cost.eu), in the framework of COST Action CA20128 (Promoting Innovation of fermented foods; https://fermentedfoods.eu/).
The authors declare that the research was conducted in the absence of any commercial or financial relationships that could be construed as a potential conflict of interest.
All claims expressed in this article are solely those of the authors and do not necessarily represent those of their affiliated organizations, or those of the publisher, the editors and the reviewers. Any product that may be evaluated in this article, or claim that may be made by its manufacturer, is not guaranteed or endorsed by the publisher.
Amara, A. A., and El-Baky, N. A. (2023). Fungi as a source of edible proteins and animal feed. J. Fungi. 9:73. doi: 10.3390/jof901007
Andayani, S. N., Lioe, H. N., Wijaya, C. H., and Ogawa, M. (2020). Umami fractions obtained from water-soluble extracts of red oncom and black Oncom - Indonesian fermented soybean and peanut products. J. Food Sci. 85, 657–665. doi: 10.1111/1750-3841.14942
Aruna, T. E. (2019). Production of value-added product from pineapple peels using solid state fermentation. Innov. Food Sci. Emerg. Technol. 57:102193. doi: 10.1016/j.ifset.2019.102193
Atta-Delgado, M. X., Lozano, S. P. G., and Torres, J. A. (2023). A survey on the prevalence of sustainable diets and the eating experience satisfaction. Innov. Food Sci. Emerg. Technol. 84:103305. doi: 10.1016/j.ifset.2023.103305
Barba, F. J. (2021). An integrated approach for the valorization of cheese whey. Food Secur. 10:564. doi: 10.3390/foods10030564
Barzee, T. J., Cao, L., Pan, Z., and Zhang, R. (2021). Fungi for future foods. J. Future Foods 1, 25–37. doi: 10.1016/j.jfutfo.2021.09.002
Battaglia, E., Benoit, I., van den Brink, J., Wiebenga, A., Coutinho, P. M., Henrissat, B., et al. (2011). Carbohydrate-active enzymes from the zygomycete fungus Rhizopus oryzae: a highly specialized approach to carbohydrate degradation depicted at genome level. BMC Genom. 12, 1–12. doi: 10.1186/1471-2164-12-38
Borujeni, N. E., Karimi, K., Denayer, J. F., and Kumar, R. (2022). Apple pomace biorefinery for ethanol, mycoprotein, and value-added biochemicals production by Mucor indicus. Energy 240:122469. doi: 10.1016/j.energy.2021.122469
Braho, V., Sar, T., and Taherzadeh, M. J. (2024). Cultivation of edible filamentous fungi on pomegranate by-products as feedstocks to produce mycoprotein. Syst. Microbiol. Biomanufact. 4, 675–686. doi: 10.1007/s43393-023-00212-0
Carota, E., Crognale, S., D’Annibale, A., and Petruccioli, M. (2018). Bioconversion of agro-industrial waste into microbial oils by filamentous fungi. Process Saf. Environ. 117, 143–151. doi: 10.1016/j.psep.2018.04.022
Chan, L. G., Cohen, J. L., Ozturk, G., Hennebelle, M., Taha, A. Y., de Moura Bell, L. N., et al. (2018). Bioconversion of cheese whey permeate into fungal oil by Mucor circinelloides. J. Biol. Eng. 12, 1–14. doi: 10.1186/s13036-018-0116-5
Chegini, G., and Taheri, M. (2013). Whey powder: process technology and physical properties: a review. Middle East J. Sci. Res. 13, 1377–1387. doi: 10.5829/idosi.mejsr.2013.13.10.1239
Derbyshire, E. J., Theobald, H., Wall, B. T., and Stephens, F. (2023). Food for our future: the nutritional science behind the sustainable fungal protein–mycoprotein. A symposium review. J. Nutr. Sci. 12, 1–6. doi: 10.1017/jns.2023.29
Domínguez-Niño, A., Cantú-Lozano, D., Ragazzo-Sanchez, J. A., Andrade-González, I., and Luna-Solano, G. (2018). Energy requirements and production cost of the spray drying process of cheese whey. Dry. Technol. 36, 597–608. doi: 10.1080/07373937.2017.1350863
Dzurendová, S., Shapaval, V., Tafintseva, V., Kohler, A., Byrtusová, D., Szotkowski, M., et al. (2021). Assessment of biotechnologically important filamentous fungal biomass by Fourier transform Raman spectroscopy. Int. J. Mol. Sci. 22:6710. doi: 10.3390/ijms22136710
Ferreira, J. A., Lennartsson, P. R., and Taherzadeh, M. J. (2014). Production of ethanol and biomass from thin stillage using food-grade Zygomycetes and Ascomycetes filamentous fungi. Energies 7, 3872–3885. doi: 10.3390/en7063872
Ferreira, J. A., Lennartsson, P. R., and Taherzadeh, M. J. (2015). Production of ethanol and biomass from thin stillage by Neurospora intermedia: a pilot study for process diversification. Eng. Life Sci. 15, 751–759. doi: 10.1002/elsc.201400213
Gajdhane, S. B., Bhagwat, P. K., and Dandge, P. B. (2016). Statistical media optimization for enhanced production of α-galactosidase by a novel Rhizopus oryzae strain SUK. Biocatal. Agric. Biotechnol. 8, 301–309. doi: 10.1016/j.bcab.2016.08.016
Gastaldello, A., Giampieri, F., De Giuseppe, R., Grosso, G., Baroni, L., and Battino, M. (2022). The rise of processed meat alternatives: a narrative review of the manufacturing, composition, nutritional profile and health effects of newer sources of protein, and their place in healthier diets. Trends Food Sci. Technol. 127, 263–271. doi: 10.1016/j.tifs.2022.07.005
Gmoser, R., Fristedt, R., Larsson, K., Undeland, I., Taherzadeh, M. J., and Lennartsson, P. R. (2020). From stale bread and brewers spent grain to a new food source using edible filamentous fungi. Bioengineered 11, 582–598. doi: 10.1080/21655979.2020.1768694
Hashemi, S. S., Karimi, K., and Taherzadeh, M. J. (2022). Valorization of vinasse and whey to protein and biogas through an environmental fungi-based biorefinery. J. Environ. Manag. 303:114138. doi: 10.1016/j.jenvman.2021.114138
Hashempour-Baltork, F., Hosseini, S. M., Assarehzadegan, M. A., Khosravi-Darani, K., and Hosseini, H. (2020). Safety assays and nutritional values of mycoprotein produced by Fusarium venenatum IR372C from date waste as substrate. J. Sci. Food Agric. 100, 4433–4441. doi: 10.1002/jsfa.10483
Ibarruri, J., and Hernández, I. (2019). Valorization of cheese whey and orange molasses for fungal biomass production by submerged fermentation with Rhizopus sp. Bioprocess Biosyst. Eng. 42, 1285–1300. doi: 10.1007/s00449-019-02127-4
Kamran, A., Bibi, Z., Aman, A., and Qader, S. A. U. (2017). Hyper production of β-galactosidase from newly isolated strain of Aspergillus nidulans. J. Food Process Eng. 40:e12452. doi: 10.1111/jfpe.12452
Karagül-Yüceer, Y., Drake, M. A., and Cadwallader, K. R. (2003). Aroma-active components of liquid cheddar whey. J. Food Sci. 68, 1215–1219. doi: 10.1111/j.1365-2621.2003.tb09627.x
Karim, A., and Aider, M. (2022). Bioconversion of electro-activated lactose, whey and whey permeate to produce single cell protein, ethanol, aroma volatiles, organic acids and fat by Kluyveromyces marxianus. Int. Dairy J. 129:105334. doi: 10.1016/j.idairyj.2022.105334
Karimi, S., Mahboobi Soofiani, N., Lundh, T., Mahboubi, A., Kiessling, A., and Taherzadeh, M. J. (2019). Evaluation of filamentous fungal biomass cultivated on vinasse as an alternative nutrient source of fish feed: protein, lipid, and mineral composition. Fermentation 5:99. doi: 10.3390/fermentation5040099
Kumitch, H. M., Stone, A. K., Nickerson, M. T., Korber, D. R., and Tanaka, T. (2020). Effect of fermentation time on the physicochemical and functional properties of pea protein-enriched flour fermented by Aspergillus oryzae and Aspergillus Niger. Cereal Chem. 97, 416–428. doi: 10.1002/cche.10257
Macwan, S. R., Dabhi, B. K., Parmar, S. C., Hati, S., Prajapati, J. B., and Aparnathi, K. D. (2018). Development of cheddar cheese whey based growth medium for Lactobacillus helveticus MTCC 5463. Int. J. Fermented Foods 7, 73–83. doi: 10.30954/2321-712X.12.2018.2
Mahboubi, A., Ferreira, J. A., Taherzadeh, M. J., and Lennartsson, P. R. (2017a). Value-added products from dairy waste using edible fungi. Waste Manag. 59, 518–525. doi: 10.1016/j.wasman.2016.11.017
Mahboubi, A., Ferreira, J. A., Taherzadeh, M. J., and Lennartsson, P. R. (2017b). Production of fungal biomass for feed, fatty acids, and glycerol by Aspergillus oryzae from fat-rich dairy substrates. Fermentation 3:48. doi: 10.3390/fermentation3040048
Meng, W., Zhang, Y., Cao, M., Zhang, W., Lü, C., Yang, C., et al. (2020). Efficient 2, 3-butanediol production from whey powder using metabolically engineered Klebsiella oxytoca. Microb. Cell Factories 19, 1–10. doi: 10.1186/s12934-020-01420-2
Nair, R. B., Lennartsson, P. R., and Taherzadeh, M. J. (2016). Mycelial pellet formation by edible ascomycete filamentous fungi Neurospora intermedia. Amb Express. 6, 31–10. doi: 10.1186/s13568-016-0203-2
Nazir, M. T., Soufiani, A. M., Ferreira, J. A., Sar, T., and Taherzadeh, M. J. (2022). Production of filamentous fungal biomass with increased oil content using olive oil as a carbon source. J. Chem. Tech. Biotech. 97, 2626–2635. doi: 10.1002/jctb.7135
Niego, A. G. T., Lambert, C., Mortimer, P., Thongklang, N., Rapior, S., Grosse, M., et al. (2023). The contribution of fungi to the global economy. Fungal Divers 121, 95–137. doi: 10.1007/s13225-023-00520-9
Parchami, M., Ferreira, J. A., and Taherzadeh, M. J. (2021). Starch and protein recovery from brewer’s spent grain using hydrothermal pretreatment and their conversion to edible filamentous fungi–a brewery biorefinery concept. Bioresour. Technol. 337:125409. doi: 10.1016/j.biortech.2021.125409
Pires, A. F., Marnotes, N. G., Rubio, O. D., Garcia, A. C., and Pereira, C. D. (2021). Dairy by-products: a review on the valorization of whey and second cheese whey. Food Secur. 10:1067. doi: 10.3390/foods10051067
Prazeres, A. R., Carvalho, F., and Rivas, J. (2012). Cheese whey management: a review. J. Environ. Manag. 110, 48–68. doi: 10.1016/j.jenvman.2012.05.018
Restiawaty, E., Dewi, A., Wibisono, T. A. S. E., and Budhi, Y. W. (2023). Lignocellulosic bioethanol production using Neurospora intermedia in consolidated bioprocessing (CBP) system. Biofuels 14, 343–352. doi: 10.1080/17597269.2022.2137948
Sar, T., Ferreira, J. A., and Taherzadeh, M. J. (2020b). Bioprocessing strategies to increase the protein fraction of Rhizopus oryzae biomass using fish industry sidestreams. Waste Manag. 113, 261–269. doi: 10.1016/j.wasman.2020.06.005
Sar, T., Ferreira, J. A., and Taherzadeh, M. J. (2021). Conversion of fish processing wastewater into fish feed ingredients through submerged cultivation of Aspergillus oryzae. Syst. Microbiol. Biomanufact. 1, 100–110. doi: 10.1007/s43393-020-00009-5
Sar, T., Harirchi, S., Ramezani, M., Bulkan, G., Akbas, M. Y., Pandey, A., et al. (2022a). Potential utilization of dairy industries by-products and wastes through microbial processes: a critical review. Sci. Total Environ. 810:152253. doi: 10.1016/j.scitotenv.2021.152253
Sar, T., Larsson, K., Fristedt, R., Undeland, I., and Taherzadeh, M. J. (2022b). Demo-scale production of protein-rich fungal biomass from potato protein liquor for use as innovative food and feed products. Food Biosci. 47:101637. doi: 10.1016/j.fbio.2022.101637
Sar, T., Ozturk, M., Taherzadeh, M. J., and Ferreira, J. A. (2020a). New insights on protein recovery from olive oil mill wastewater through bioconversion with edible filamentous fungi. PRO 8:1210. doi: 10.3390/pr8101210
Sar, T., Stark, B. C., and Yesilcimen Akbas, M. (2017). Effective ethanol production from whey powder through immobilized E. coli expressing Vitreoscilla hemoglobin. Bioengineered 8, 171–181. doi: 10.1080/21655979.2016.1218581
Şen, İ., and Yüceer, Y. K. (2019). Production and characterization of whey beverage by using grain or lyophilized kefir cultures. Akademik Gıda 17, 362–370. doi: 10.24323/akademik-gida.647719
Seyis, I., and Aksoz, N. (2004). Production of lactase by Trichoderma sp. Food Technol. Biotechnol. 42, 121–124.
Souza Filho, P. F., Nair, R. B., Andersson, D., Lennartsson, P. R., and Taherzadeh, M. J. (2018). Vegan-mycoprotein concentrate from pea-processing industry byproduct using edible filamentous fungi. Fungal Biol. Biotechnol. 5, 5–10. doi: 10.1186/s40694-018-0050-9
Souza Filho, P. F., Zamani, A., and Taherzadeh, M. J. (2017). Production of edible fungi from potato protein liquor (PPL) in airlift bioreactor. Fermentation 3:12. doi: 10.3390/fermentation3010012
Svensson, S. E., Bucuricova, L., Ferreira, J. A., Souza Filho, P. F., Taherzadeh, M. J., and Zamani, A. (2021). Valorization of bread waste to a fiber-and protein-rich fungal biomass. Fermentation 7:91. doi: 10.3390/fermentation7020091
Taşkıran, M., Olum, E., and Candoğan, K. (2020). Changes in chicken meat proteins during microwave and electric oven cooking. J. Food Process. Preserv. 44:e14324. doi: 10.1111/jfpp.14324
Tonelotto, M., Pirota, R. D. P. B., Delabona, P. D. S., Barros, G. D. O. F., and Farinas, C. S. (2014). Isolation and characterization of β-galactosidase from a new Amazon forest strain of Aspergillus niger as a potential accessory enzyme for biomass conversion. Biocatal. Biotransform. 32, 13–22. doi: 10.3109/10242422.2013.801018
Uwineza, C., Mahboubi, A., Atmowidjojo, A., Ramadhani, A., Wainaina, S., Millati, R., et al. (2021). Cultivation of edible filamentous fungus Aspergillus oryzae on volatile fatty acids derived from anaerobic digestion of food waste and cow manure. Bioresour. Technol. 337:125410. doi: 10.1016/j.biortech.2021.125410
Valdez Castillo, M., Tahmasbi, H., Pachapur, V. L., Brar, S. K., Vuckovic, D., Sitnikov, D., et al. (2021). Production of aroma and flavor-rich fusel alcohols by cheese whey fermentation using the Kluyveromyces marxianus and Debaryomyces hansenii yeasts in monoculture and co-culture modes. J. Chem. Technol. Biotechnol. 96, 2354–2367. doi: 10.1002/jctb.6763
Vamvakaki, A. N., Kandarakis, I., Kaminarides, S., Komaitis, M., and Papanikolaou, S. (2010). Cheese whey as a renewable substrate for microbial lipid and biomass production by Zygomycetes. Eng. Life Sci. 10, 348–360. doi: 10.1002/elsc.201000063
Wikandari, R., Tanugraha, D. R., Yastanto, A. J., Gmoser, R., and Teixeira, J. A. (2023). Development of meat substitutes from filamentous fungi cultivated on residual water of tempeh factories. Molecules 28:997. doi: 10.3390/molecules28030997
Yang, Y., Zhong, H., Yang, N., Xu, S., and Yang, T. (2022). Quality improvement of sweet rice wine fermented with Rhizopus delemar on key aroma compounds content, phenolic composition, and antioxidant capacity compared to Rhizopus oryzae. J. Food Sci. Technol. 59, 2339–2350. doi: 10.1007/s13197-021-05250-x
Keywords: dairy by-products, mycoprotein, bioconversion, waste management, sustainability, alternative food
Citation: Kaya B, Wijayarathna ERKB, Yüceer YK, Agnihotri S, Taherzadeh MJ and Sar T (2024) The use of cheese whey powder in the cultivation of protein-rich filamentous fungal biomass for sustainable food production. Front. Sustain. Food Syst. 8:1386519. doi: 10.3389/fsufs.2024.1386519
Received: 15 February 2024; Accepted: 01 July 2024;
Published: 23 July 2024.
Edited by:
Dimas Rahadian Aji Muhammad, Sebelas Maret University, IndonesiaReviewed by:
Karina Cruz-Aldaco, Universidad Autónoma de Querétaro, MexicoCopyright © 2024 Kaya, Wijayarathna, Yüceer, Agnihotri, Taherzadeh and Sar. This is an open-access article distributed under the terms of the Creative Commons Attribution License (CC BY). The use, distribution or reproduction in other forums is permitted, provided the original author(s) and the copyright owner(s) are credited and that the original publication in this journal is cited, in accordance with accepted academic practice. No use, distribution or reproduction is permitted which does not comply with these terms.
*Correspondence: Taner Sar, dGFuZXIuc2FyQGhiLnNl;; c3QudGFuZXJAZ21haWwuY29t Burcu Kaya, YnVyY3Uua2F5YUBjb211LmVkdS50cg==
Disclaimer: All claims expressed in this article are solely those of the authors and do not necessarily represent those of their affiliated organizations, or those of the publisher, the editors and the reviewers. Any product that may be evaluated in this article or claim that may be made by its manufacturer is not guaranteed or endorsed by the publisher.
Research integrity at Frontiers
Learn more about the work of our research integrity team to safeguard the quality of each article we publish.