- 1Department of Agricultural, Forest and Food Sciences (DISAFA), University of Turin, Turin, Italy
- 2Department of Veterinary Sciences (DSV), University of Turin, Turin, Italy
Organo-mineral fertilizers (OMFs) with low organic carbon (Corg) content have been associated with higher mineral fraction nutrient use efficiency. However, the extraction of peat, which is typically used in these OMFs, from endangered ecosystems causes long-time stored Corg to mineralize and to be released back into the atmosphere as carbon dioxide (CO2). This study analyzes the replacement of peat in OMFs with biowaste materials. These materials, considered organic byproducts that microorganisms and other living things can decompose through composting and aerobic or anaerobic digestion, offer a viable opportunity. This study investigated three stabilized biowastes—green compost (GC) from pruning residues, municipal solid waste compost (MSWC), and manure-based vermicompost (VC)—as the organic matrices for granular OMFs. These matrices were impregnated with dissolved ammonium sulfate and urea and used to coat diammonium phosphate granules. Each biowaste OMF contained 7.5% Corg, 20% mineral N, and 10% mineral P2O5 (OMF20 − 10). Fertilizers with high nutrient concentrations have the advantage of requiring low application volumes, facilitating their application in the field. Biowaste OMFs were compared with peat OMFs with the same Corg-N-P2O5 concentration. Peat and MSWC were also used to create OMFs containing 7.5% Corg, 10% mineral N, and 5% mineral P2O5 (OMF10 − 5). A 75-day tunnel trial was conducted under semi-controlled conditions using tomato plants (Solanum lycopersicum L.) fertilized to an equivalent of 81 mg N kg−1 soil and 18 mg P kg−1 soil. Controls included no fertilization (N0P0) and mineral N and P fertilization (MFNP). The Soil Plant Analysis Development (SPAD) chlorophyll meter and the BBCH (from German Biologische Bundesanstalt, Bundessortenamt und CHemische Industrie) scale as well as the number of shoots were measured over time, as berry and total aboveground yield, N and P uptakes, and N and P use efficiencies (NUE and PUE, respectively) were calculated at harvest. All treatments outperformed the control N0P0 in most indicators. Peat20 − 10 did not have more berry yield than other OMF20 − 10; however, the higher number of shoots indicated a higher potential yield in the event of prolonging the experiment. At the end of 75 days, VC20 − 10 and MSWC20 − 10 showed similar PUE to peat, suggesting that those materials can be used as replacements. In the case of OMF10 − 5, MSWC10 − 5 had yield and N and P uptakes like peat OMFs, confirming the potential use of MSWC as peat replacement even at different nutrient concentrations. This research provides reassuring evidence of the effectiveness of biowaste OMFs, offering a positive outlook for sustainable agriculture. However, their use is not recommendable for short growing seasons.
1 Introduction
Organo-mineral fertilizers (OMFs) refers to a combination of an organic material with one or more mineral fertilizers (Smith et al., 2020). The mixture is made to correct the nutrient ratio of an organic material (Rady, 2012; Antille et al., 2013b; Anetor and Omueti, 2014) or to use the organic material as a matrix for the mineral nutrients (Florio et al., 2016). Generally, this second type of OMF adopts low quantities of organic carbon (Corg), which in Europe can be as low as 3 or 7.5% for liquid or solid OMFs, respectively (EC, 2019), and their use has been reported to reduce N losses, improve P availability, and enhance N and P use efficiency (Richards et al., 1993; Antille et al., 2013b, 2014; Florio et al., 2016).
The proportion of the organic matrix in the OMF chemically and physically influences the mineral fraction in close contact with it. In OMFs with different organic material fractions, the lower organic material fractions in the OMF decrease the fertilizer density and increase the abrasion fragility and crushing strength (Pare et al., 2010); physical differences in fertilizer granules can create discrepancies in P release as they interact differently with the soil. Moreover, it has been shown that a higher Corg content in close contact with mineral nutrients increases the immobilization of P (Sitzmann et al., 2024b), probably due to Ca-P bonds and a pH effect.
In low-Corg OMFs, the organic matrix proportion can be considered fixed, but the proportion of the mineral fertilizer can be adjusted to adopt a desirable nutrient concentration (Sitzmann et al., 2023). However, reaching a specific nutrient concentration in OMFs will require using a mixture of mineral fertilizers that can cause one type of mineral fertilizer preference over another, e.g., urea for a high N concentration over ammonium sulfate. Differences in concentration in OMFs—and therefore in mineral fertilizers—will not only cause different interactions in soil and plant nutrient uptake but could also interact differently based on the organic fraction of the OMF.
Low-Corg OMFs can be produced from organic geogenic materials such as peat, leonardite, or lignite (EC, 2019). Traditionally, peat has been preferred over other materials because of its high humic carbon (C) content (Alianiello et al., 1999; Florio et al., 2016). This fraction has been linked to promoting crop growth by influencing microbial and physicochemical soil properties (Li et al., 2019), stimulating plant metabolism (Asli and Neumann, 2010; Vujinović et al., 2020), and helping the remediation of soil contaminants due to an increase in sorption capacity (Piccolo, 2002; Conte et al., 2005). However, peatlands—which account for 55,000 Mt C, corresponding to 27% of the soil C stock in the world (Parish et al., 2008)—should be protected from mining to avoid the volatilization of long-term stored C as carbon dioxide (CO2) and the emissions of other greenhouse gases (Saarikoski et al., 2019; Humpenöder et al., 2020). Therefore, replacing organic geogenic materials with degraded and stabilized renewable biowaste materials that are produced locally has been proposed for improved circularity of carbon and other nutrients (Schmilewski, 2008; Pare et al., 2010; Taparia et al., 2021).
Replacing a raw material with another in an industrial process involves a series of challenges. The search process can be long and expensive, and companies may face reluctance to change from the market and the established industry (Alexander et al., 2008), as well as establish a new production chain of quality-certified products. For OMF manufacturing, any potential raw organic material needs to be stable over time, homogeneous in larger volumes, and interact foreseeably with mineral fertilizers (Sakurada et al., 2016; Bouhia et al., 2022). Materials with high and easy availability on the market are desirable for OMF production to provide them with circularity through reutilization (Taparia et al., 2021). Low cost and certified technological quality are also fundamental when selecting a suitable organic material.
In a previous study from this research group, composted and digested dry materials similar to peat with a particle size below 5 mm, Corg content between 17 and 25%, and a humification degree >50% were proposed as peat replacements for preparing granular and pelletized low-Corg OMFs (Sitzmann et al., 2023). The results identified three potential candidates: green compost (GC) from pruning residues, municipal solid waste compost (MSWC), and manure-based vermicompost (VC). GC and VC can be used as organic fertilizers or soil amendments; on the other hand, municipal solid waste is not recognized as a fertilizer by the European Union (EU) regulation unless the organic fraction is separated from any other residue (EC, 2019), which can pose technical and economic challenges. However, when other potential organic fractions, such as manure, are unavailable, MSWC can serve as an alternative to traditional fertilizers in the future (Ros et al., 2006). MSWC used in the research by Sitzmann et al. (2023) is a physically separated organic source of municipal waste, which is on the threshold of being considered an organic fertilizer. The use of MSWC has been considered because its contribution to heavy metals can be almost negligible in OMFs with 7.5% Corg. The inclusion of MSWC among potential peat replacements can be a reference for future revisions of the current fertilizer regulations.
In a previous small pot trial with OMFs using GC, MSWC, VC, and peat, with Corg, N, and P2O5 concentrations of 7.5, 10, and 5%, respectively, peat and mineral control led to higher maize plant P uptake and growth yield after 4 weeks compared to biowaste OMFs (Sitzmann et al., 2024a). The reduced uptake in biowaste treatments was attributed to a slow release of nutrients, bonds between metals in the organic material and the phosphates from the mineral fertilizer, or the shift of phosphates to unavailable forms caused by the high pH of the organic material, which reduced the nutrient use efficiency of GC, MSWC, and VC OMFs. Microbial immobilization in soil caused by the organic matrix of OMFs was discarded in low-Corg OMFs due to the limited Corg addition compared to mineral controls (Sitzmann et al., 2024a). Considering the 1st days of plant growth, an initial lower nutrient use efficiency based on the ratio of absorbed nutrients to total applied nutrients was frequently observed in OMFs due to slow chemical release (Bouhia et al., 2023; de Morais et al., 2023) or initial nutrient immobilization (Mandal et al., 2007; Mazeika et al., 2016; Carneiro et al., 2021; Llovet et al., 2023), and both processes could increase nutrient availability in more prolonged periods. While a previous study on GC, MSWC, and VC granular OMFs focused on their performance over a few weeks, further study is needed to determine if the nutrient immobilization is temporary and if these OMFs will increase their efficiency after 1 month; alternatively, it must be determined if the immobilization is permanent and if the addition of these organic materials to mineral fertilizers is counterproductive.
This study aimed to evaluate the N and P use efficiencies (NUE and PUE, respectively) of three new granulated biowaste-based OMFs characterized by low-Corg content. They were compared with peat OMFs and a mineral control in a pot experiment where tomato plants (Solanum lycopersicum), as a medium-term growing crop, were managed for 75 days. Additionally, it sought to evaluate if the ratio between organic fraction and mineral nutrient can affect the nutrient use efficiency and physiological development of the plants; therefore, the MSWC and peat materials were used with two different concentrations of mineral nutrients but with the same Corg concentration.
We hypothesized the following:
1) differences in growing parameters would emerge in the initial and medium growth phases of tomato plants due to differences in N and P release of OMFs compared to the mineral control;
2) granular OMFs made with GC, MSWC, and VC would have a similar nutrient use efficiency compared to peat OMF and the mineral control after 75 days; and
3) a lower concentration of minerals N and P in the OMF, which results in higher Corg/N and Corg/P, would result in a higher immobilization effect.
Compared to the study conducted by Sitzmann et al. (2024a), this study contributes to a deeper understanding of the nutritional dynamics derived from biowastes and peat-based OMFs over a mid-term period that is more appropriate for a broader range of commercial crops such as tomatoes. Additionally, this study evaluates the significance of mineral nutrient concentration within the OMF granules, serving as an indicator for fertilizer application per unit soil area.
2 Materials and methods
This study was conducted through a plant growth trial with tomato plants (Solanum lycopersicum L.). It involved two sets of comparisons: (i) four OMFs made from three different biowastes or peat and (ii) four OMFs made from one biowaste or peat with two different concentrations of the mineral nutrient. In the first trial, four OMFs with a Corg-N-P2O5 content of 7.5-20-10 (4.2% of total P) were created using peat (hereafter mentioned Peat20 − 10), green compost (hereafter mentioned GC20 − 10), municipal solid waste compost (hereafter mentioned MSWC20 − 10), and vermicompost (hereafter mentioned VC20 − 10). Two control treatments were also included: (1) no fertilization (N0P0) and (2) mineral fertilization (MFNP), consisting of a blend of ammonium sulfate, urea, and triple superphosphate (TSP) at an N:P ratio of 10-2.1. For the second comparison, two additional OMFs were created using a Corg-N-P2O5 content of 7.5-10-5 (2.1% of total P) using peat (Peat10 − 5) and municipal solid waste compost (MSWC10 − 5). These treatments were compared to Peat20 − 10 and MSWC20 − 10.
Therefore, for the given amount of N and P fertilization per kg of soil, the 7.5-10-5 OMFs will provide twice the amount of Corg of the 7.5-20-10 OMFs. Treatment details and amounts of fertilization are described in Table 1.
2.1 Fertilizer production
Biowaste materials and peat for OMFs were collected from industries across Italy. Biowaste materials were selected based on their similarities to peat Corg content, Corg stability based on the alkyl C/O alkyl C ratio and the humification degree, low heavy metal contents, and processing facilities. The GC was produced by combining vegetable waste with pruning residues composted for 6 months and was manufactured by Roffino S.R.L. The VC was produced from bovine manure that was composted for 6 months, which then undergoes a vermicomposting process, and was manufactured by Conitalo S.p.A. ACEA Pinorelese Industriale S.p.A. manufactured the MSWC by mechanically separating the organic fraction of municipal household waste and stabilizing it through integrated anaerobic digestion and aerobic composting (Mainero, 2008). Details regarding MSWC production can be found in the study conducted by Moretti et al. (2020). Organic materials were fully characterized in the study of Sitzmann et al. (2023).
The OMFs were manufactured by SCAM S.p.A. (Modena, Italy). The OMF manufacturing process involved mixing the organic material with ammonium sulfate, urea, diammonium phosphate, and water in a granulator. The water dissolves the mineral N, whereby impregnating the organic material with the dissolved fertilizer, forming a cohesive dough. This cohesive mass acts as a coating for the diammonium phosphate that acts as the granule nucleus. After creating the OMF granules, they are dried at 60°C until they reach ~4% moisture content. The mineral N fertilizer is added slightly over the target concentration of OMF N, accounting for volatilization of a partial N concentration during the OMF drying. Finally, the granules are sieved, resulting in OMFs with a diameter of 2–5 mm (60% <3 mm). For more details, see Sitzmann et al. (2024a,b).
2.2 Experimental setup
A pot experiment was conducted in the summer of 2022 on the campus of the Department of Agricultural, Forest, and Food Sciences of the University of Turin in Grugliasco (NW, Italy) under semi-controlled conditions in an open tunnel greenhouse with a North-South orientation. The greenhouse was covered with a transparent cloth to guarantee proper illumination for plant development while preventing rainwater from entering. During the growing period, the minimum and maximum average temperatures recorded were 18 and 33°C, respectively. A tomato (Solanum lycopersicum L.) cultivar H1301, suitable for industrial processing, was used as a test plant. Twenty-day-old tomato plants grown in a nursery were transplanted, one per pot, into pre-fertilized pots on 25th May.
The experiment was a randomized block design separated by benches with four replicates for each treatment (the total number of pots was 36). However, one block was discarded due to pest development during the 2nd month of the tomato growth cycle. Each pot was 20 cm in diameter at the top and slightly thinner at the bottom, and it was placed on a plate that was used to provide sub-irrigation. Each pot was filled with 12 kg of air-dried soil sieved to 5 mm at a density of 1.40 g cm−3, resulting in a soil depth of 30 cm. The soil used in the experiment has a loam texture, a neutral pH in water (7.2), 8.4 g kg−1 organic C, and 1.1 g kg−1 total N. The total carbonate content was 4.4 g kg−1. The exchangeable K was low (0.1 meq g−1), while the exchangeable Ca and Mg were 7.1 and 0.9 meq g−1, respectively, giving a low cation exchange capacity of 110. The Olsen P-value was 13.5 mg kg−1.
To simulate fertilizer incorporation, fertilization was carried out by removing soil of ~2 cm depth, then dispersing the fertilizers homogeneously, and later covering again with the previously removed soil. Except for N0P0, each treatment received 81 mg N kg−1 soil (equivalent to 170 kg N ha−1 for 15 cm soil depth) and 18 mg P kg−1 soil in pre-planting (Table 1). OMFs with a Corg-N-P2O5 concentration of 7.5-20-10 and 7.5-10-5 kg 100 kg−1 OMF received ~4.9 and 9.8 g OMF pot−1, respectively. For mineral control, MFNP, urea, and ammonium sulfate were combined so that 75% of the N was supplied in the form of ammonium and 25% in the form of urea to emulate the N forms of OMF. Supplemental fertilization was carried out using with potassium chloride (KCl 60%), adding 79 mg K kg−1 soil to all pots to avoid potential K deficiencies.
During the 1st month, pots were watered daily with 1 L of water and then with 2 L daily until harvesting. Water was added to the bottom plate to avoid nutrient leaching.
Tomato plants received lambda-cyhalothrin (KarateZeon®, Seneffe, Belgium; 17th June), deltamethrin (Decis® Evo, Villefranche, France; 1st July), and copper oxide (Fenicrit®, Bologna, Italy; 6th June and 4th July) as insecticides and fungicides.
2.3 Plant analyses on nutritional status and yield production
During the 2nd and 3rd months of tomato growth, phenology development, the number of stems, and leaf chlorophyll content were measured weekly. Phenological stage measurements were performed using the BBCH scale, focusing on the growth stage coding system for Solanaceae, provided by the Federal Biological Research Center for Agriculture and Forestry (Meier, 2001). The BBCH index was measured by considering the emergence of inflorescences, flowering, and fruit development, while the number of stems and fruits was counted manually. All shoots and branches with at least one visible node and a first genuine well-extended leaf were considered lateral stems. The measurements were taken on the same dates as the phenological stage survey. Leaf chlorophyll content was measured on a 6-mm2 area using the SPAD-502 Portable Chlorophyll Meter (Konica Minolta Camera Co. Ltd., Tokyo, Japan). The tomato was harvested on 8th August, 75 days after the transplant. Tomato fruits were collected manually, and shoot mass was harvested at the soil level. The soil contained in the pot was divided into two sections (top and bottom) of 15 cm in height each, and from each section, roots were manually collected and hand-washed with the help of sieves. Dry matter (DM) biomass was measured separately for fruits, shoots, and top and bottom roots after drying samples at 60°C for 72 h. The total epigean biomass (fruit and shoot mass) was ground to 0.5 mm. Total N concentration in tissue was measured in an elemental analyzer (Thermo Scientific FlashEA 1112, Thermoquest). Similarly, total P concentration in tissue samples was analyzed through digestion with sulfuric and perchloric acid, followed by a spectrophotometer analysis using green malachite (Ohno and Zibilske, 1991). The epigean biomass N and P uptake was calculated as the product between the N and P concentration and the total epigean biomass.
The N use efficiency (NUE) and the P use efficiency (PUE) were used as indicators for the fertilizer's efficiency. The nutrient use efficiency was calculated as follows:
2.4 Statistical analyses
Data analysis was performed using R statistical software (version 4.2.2). For each crop, two sets of comparisons were made: (a) treatments N0P0, MFNP, GC20 − 10, MSWC20 − 10, Peat20 − 10, and VC20 − 10 were analyzed using treatments and block as independent values to determine differences between controls and organic materials in OMFs and (b) treatments MSWC20 − 10, MSWC10 − 5, Peat20 − 10, and Peat10 − 5 were analyzed using Corg to mineral fertilizer ratios and block as independent variables. Values were tested for normality and homogeneity and transformed into a log or square scale when needed. A general linear model (GLM) with two or three factors was performed to compare treatments. The SPAD index and the number of stems were analyzed, including the day after transplanting, as independent variables and also independently for each sampling date. In contrast, biomass production, nutrient uptake, and efficiency were analyzed only once.
3 Results and discussion
3.1 Plant growth parameters
The chlorophyll content in leaves was analyzed based on the SPAD index, which is associated with the N content in tomato leaves (Wood et al., 1993) and fruits (Jiang et al., 2017). The SPAD index decreased by an average of 8% between 23rd June and 4th August (Figure 1A), indicating a lower chlorophyll concentration over time. Fertilized treatments had a significantly higher SPAD index overall than the control N0P0. However, when the data were analyzed for each day separately, there were no significant differences between treatments.
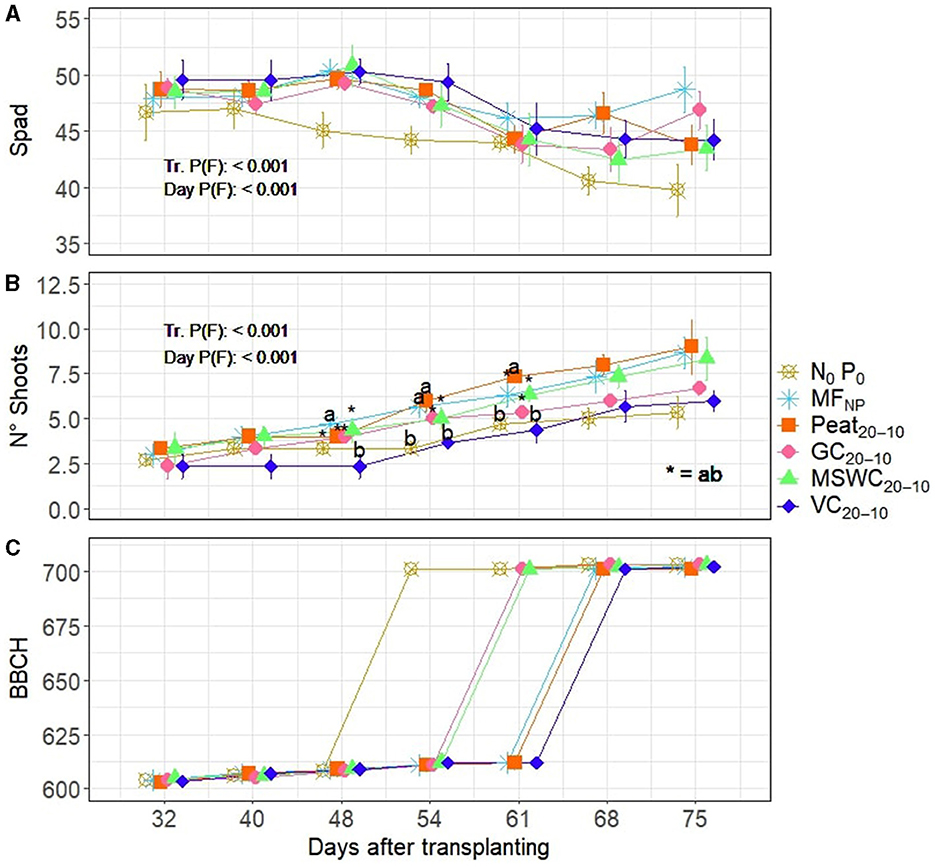
Figure 1. Growing parameters of tomatoes between weeks 5 and 11 for OMF20 − 10, mineral control (MFNP), and unfertilized control (N0P0). The parameters are average and standard error SPAD index (A), number of shoots (B), and median BBCH (C). Differences between treatment (Tr) and day are calculated as the average values of the multiple sampling events. The letters indicate differences between treatments during each specific sampling day. No differences were calculated for BBCH because the values are expressed on a scale with disjoint intervals.
The chlorophyll content in leaves indicates no differences in N availability in soil between OMFs and mineral control, contradicting other reports that observed a slower N release into the N solution (Richards et al., 1993; Antille et al., 2013a, 2014; Florio et al., 2016). However, in previous reports from our study, we did not find significant differences in N release or NUE between OMFs and mineral controls in short-term ryegrass or maize pot experiments (Sitzmann et al., 2024a,b), where the interactions of the OMF organic fraction were mostly with P.
Morphological parameters such as the number of stems, or branch number, in tomatoes can be used as a reference for potential yield, as more stems can lead to increased branching and potentially more fruit-bearing sites, influencing overall yield and plant health (Ece and Darakci, 2009). In general, the number of shoots was significantly higher in Peat20 − 10, the mineral control MFNP, and MSWC20 − 10 than in the N0P0 control and VC20 − 10, with intermediate values for GC20 − 10 (Figure 1B). Differences in the number of shoots occurred between the third, fourth, and fifth sampling dates, and the trend followed was higher values for Peat20 − 10 and MFNP and lower values for N0P0 and VC.
The earlier entrance into the reproductive stage on week 7 for N0P0 was expected compared to the OMFs and the mineral control MFNP (Figure 1C), as an earlier entrance to a reproductive phenological stage can indicate a nutritional deficiency in plants (Gaj et al., 2020). Peat20 − 10, MSWC20 − 10, and VC20 − 10 had the latest entrance to the reproductive stage (week 9). The results for VC20 − 10 are opposite to the number of shoots; however, as with Peat20 − 10 and MSWC20 − 10, those treatments are correlated to a higher P uptake. In the formerly mentioned study, Sitzmann et al. (2024a) found that peat had the same initial P availability as the mineral control. At the same time, a vermicompost OMF—as well as the other biowaste materials—immobilized a fraction of the mineral P. This initial lower P availability could explain why VC20 − 10 initially had fewer stems than Peat20 − 10, limiting their counting for the rest of the experiment.
The hypothesis of differences in growing parameters between OMFs and mineral control has not been confirmed. However, the results show that peat OMFs tend to outperform biowaste OMFs in terms of early growth, but no significant differences were observed after 75 days.
3.2 Nutrient use efficiency of OMFs
Adding mineral fertilizers alone or through OMFs increased the dry matter production in the shoots and top roots of the tomato plants compared to the control N0P0 (Table 2). Differences between treatments were observed only in the first 15 cm of the soil, which had, on average, six times higher root dry matter than the bottom 15 cm; the root DM was significantly higher in fertilized treatments than in the control N0P0. The shoot DM was significantly higher in fertilized treatments than in the N0P0 control; among the fertilized treatments, GC20 − 10 had a considerably lower DM than Peat20 − 10, with other fertilized treatments being between these two. The highest total—berries, shoots, and roots—DM production was found in Peat20 − 10 and the mineral control, while GC20 − 10, MSWC20 − 10, and VC20 − 10 had intermediate values between the mineral control and N0P0.
Total plant N uptake at the end of the growing season was correlated to the SPAD index measured on the harvest day by a Pearson value of r = 0.5 (p-value <0.01), as all fertilized treatments were significantly higher than the control N0P0 (Table 3). OMFs did not present significant differences in NUE compared to the mineral control MFNP.
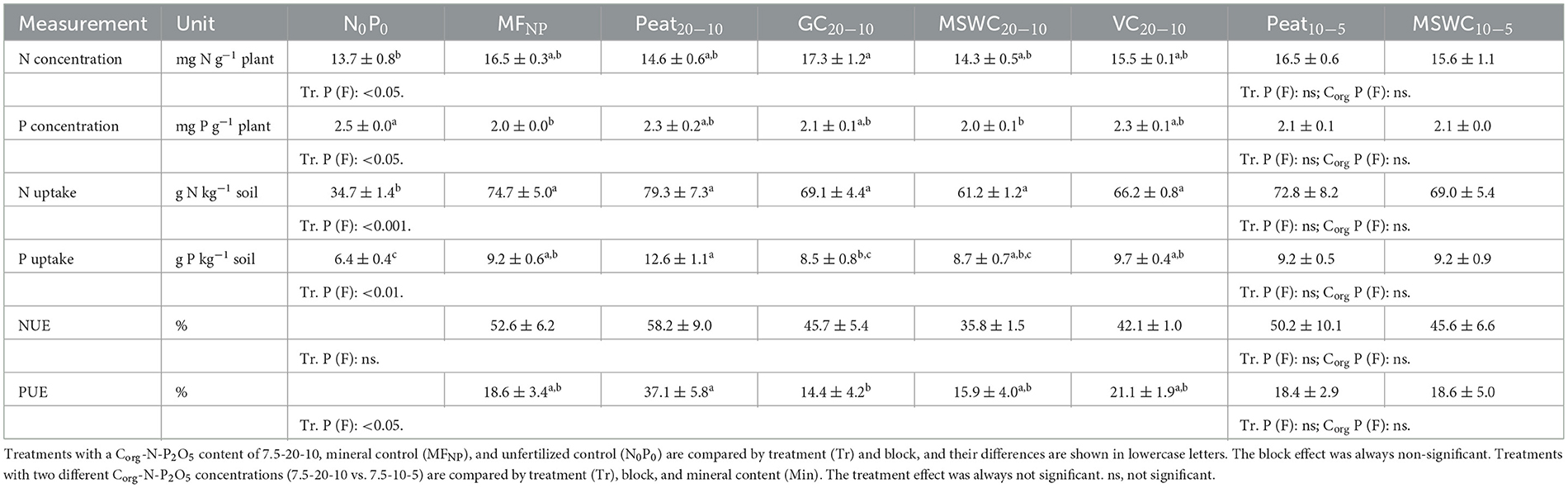
Table 3. Shoot plus fruit N and P plant concentration, uptake, and efficiencies (NUE and PUE, respectively).
As mentioned above, biowaste OMFs made with the same materials as the one used in this experiment had a lower content of plant-available P in the soil after 10 days of incubation than a peat OMF and mineral control, which caused a lower P uptake in maize plants after 30 days (Sitzmann et al., 2024a). The lower P availability caused by the biowaste OMF partially remained after 75 days, especially for GC20 − 10, which demonstrated a lower P uptake and PUE than Peat20 − 10 at harvest (Table 3). Other biowaste materials mitigated the immobilization effects, showing intermediate values for P uptake, and did not differ from Peat20 − 10. The organic material influence on the P availability of low-Corg OMFs has been associated with chemical interactions between both fractions rather than microbial immobilization (Sitzmann et al., 2024a). The Ca-P bonds in the OMF and the organic matrix pH have a higher influence on reducing P availability. However, as OMFs contained large fractions of ammonium sulfate and urea, it was expected that the nitrification process would reduce the pH in the fertilizer hotspot (Bouman et al., 1995; Dal Molin et al., 2020), and therefore, it could increase the P availability. However, this effect was not observed. The OMF granules were not concentrated in one unique hotspot, and hence, the buffer capacity of the soil, one with a high pH, could have readily adsorbed the H ions released during the nitrification, thereby reducing the impact of potential soil acidification on P availability.
Our second hypothesis considered that, despite differences during plant growth, after 75 days, tomato plants would have the same yield and nutrient use efficiency across OMF treatments. The second hypothesis has been confirmed for MSWC20 − 10 and VC20 − 10 but not for GC20 − 10.
3.3 Effects of Corg and mineral fertilizer ratios in OMFs
Differences in the mineral composition of the OMFs were expected to cause differences in N uptake between treatments. However, no differences were observed between OMF20 − 10 and OMF10 − 5 during the growth (Figure 2) and final yield (Table 2) of tomatoes. To fertilize at a ratio of 81 mg N kg−1, OMF10 − 5 treatments used twice the amount of organic material. During the fertilization process, adding supplementary organic material will result in an increase of 4% N and 6% P for MSWC10 − 5 compared to MSWC20 − 10. Furthermore, there will be an increase of 2% N and 2% P for Peat10 − 5 compared to Peat20 − 10 (Table 1). In addition, as urea is a richer source of N than ammonium sulfate, it will be in higher proportion in OMFs with 20% N (OMF20 − 10). It can be considered that the OMF10 − 5 treatment, which contains a higher content of ammonium sulfate, received an additional S fertilization because the soil used in the experiment did not receive a supplementary S fertilization. A synergism between S and N was reported as S fertilization increases the NUE by promoting a higher N uptake (Jackson, 2000; Salvagiotti et al., 2009; Rietra et al., 2017).
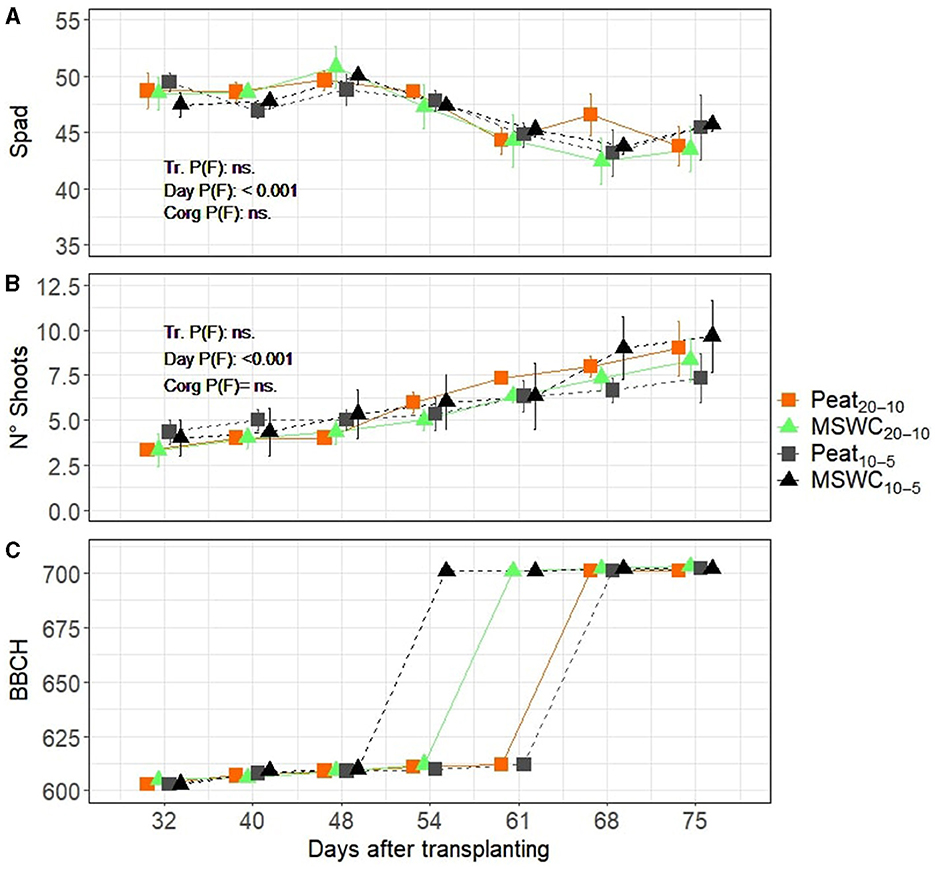
Figure 2. Growing parameters of tomatoes between weeks 5 and 11 for OMF10 − 5 and OMF20 − 10. Treatments Peat20 − 10 and MSWC20 − 10 are the same as those shown in Figure 1. Parameters are SPAD index (A), number of shoots (B), and BBCH (C). Differences between treatment (Tr) and day are calculated as the average values of the multiple sampling events. The letters above indicate differences between treatments during each specific sampling day. No differences were calculated for BBCH because the values are expressed on a scale with disjoint intervals.
However, a higher ratio of Corg to mineral nutrients could have promoted a higher degree of immobilization in soil, which would reduce the benefits of the additional organic N, humic C, and mineral S fertilization. Based on the characterization of the organic fraction discussed in the study conducted by Sitzmann et al. (2023), the immobilization would be more relevant for P than for N since pH and Ca values of MSWC (9.0, 47 mg g−1 DM) and peat (8.0, 41 mg g−1 DM) can enhance Ca-P bonds between the organic fraction and the mineral fertilizer and between the mineral fertilizer and the surrounding soil (Sica et al., 2023). The low soil P-values at the beginning of the experiment, associated with a lower release in OMF10 − 5 treatments, can be the reason for the lack of differences between the treatments, as P resulted in a limiting nutrient for a higher N and S uptake (Robertson and Vitousek, 2009; Vitousek et al., 2010).
Although the results showed no differences between using different concentrations of mineral fertilizers in OMFs, the results were inconclusive. Therefore, it is not possible to accept or reject the original hypothesis.
4 Conclusion
This study investigated the influence of organic materials in the impact of OMFs on nutrient availability and the performance of tomato plants. Materials such as vermicompost (VC20 − 10) and municipal solid waste compost (MSWC20 − 10) had a similar final PUE compared to that of a peat OMF (Peat20 − 10) and a mineral control (MFNP), suggesting that these materials could be used to replace peat in OMFs designed to be used for crops with medium to long growing cycles. Green compost (GC20 − 10) had a lower PUE and overall growing parameters compared to Peat20 − 10, indicating that not all organic materials are suitable to replace peat, even when the OMFs are used in crops that grow over several months.
The lack of differences between treatments with different mineral concentration implies that, in low-Corg OMFs, the organic fraction has a limited effect on performance, allowing flexibility in adjusting the mineral fraction based on specific market requirements. Although the OMF used in this study, with its low-Corg content, supplies low amounts of organic C to the soil through fertilization, lower concentrations of the mineral component imply that more significant amounts of the organic materials are distributed for the same fertilization target. Therefore, this type of OMF could contribute to recycling biowaste materials.
Data availability statement
The raw data supporting the conclusions of this article will be made available by the authors without undue reservation.
Author contributions
TS: Conceptualization, Data curation, Formal analysis, Investigation, Methodology, Writing – original draft, Writing – review & editing. AA: Investigation, Methodology, Writing – review & editing. CL: Methodology, Writing – review & editing. BM: Conceptualization, Investigation, Methodology, Writing – review & editing. LZ: Conceptualization, Investigation, Methodology, Supervision, Writing – review & editing. CG: Funding acquisition, Supervision, Writing – review & editing.
Funding
The author(s) declare financial support was received for the research, authorship, and/or publication of this article. This project has received funding from the European Union's Horizon 2020 Research and Innovation Program under the Marie Sklodowska-Curie Grant (Agreement No. 860127).
Acknowledgments
We appreciate the support of Stefano Tagliavini from SCAM S.p.A. for manufacturing the OMFs used in this experiment.
Conflict of interest
The authors declare that the research was conducted in the absence of any commercial or financial relationships that could be construed as a potential conflict of interest.
The handling editor RT declared a past co-authorship with the author LZ.
Publisher's note
All claims expressed in this article are solely those of the authors and do not necessarily represent those of their affiliated organizations, or those of the publisher, the editors and the reviewers. Any product that may be evaluated in this article, or claim that may be made by its manufacturer, is not guaranteed or endorsed by the publisher.
References
Alexander, P., Bragg, N., Meade, R., Padelopoulos, G., and Watts, O. (2008). Peat in horticulture and conservation: the UK response to a changing world. Mires Peat 3, 1–11.
Alianiello, F., Dell'Orco, S., Benedetti, A., and Sequi, P. (1999). Identification of primary substrates in organo-mineral fertilizers by means of isoelectric focusing. Commun. Soil Sci. Plant Anal. 30, 2169–2181. doi: 10.1080/00103629909370363
Anetor, M. O., and Omueti, J. A. I. (2014). Organo-mineral fertilizer effects in some phosphorus-unresponsive soils of southwestern Nigeria: 1. Effects on maize growth response and soil properties. Agricult. Biol. J. North Am. 5, 265–280. doi: 10.5251/abjna.2014.5.6.265.280
Antille, D. L., Sakrabani, R., and Godwin, R. J. (2014). Nitrogen release characteristics from biosolids-derived organomineral fertilizers. Commun. Soil Sci. Plant Anal. 45, 1687–1698. doi: 10.1080/00103624.2014.907915
Antille, D. L., Sakrabani, R., Tyrrel, S. F., Le, M. S., and Godwin, R. J. (2013a). Characterisation of organomineral fertilisers derived from nutrient-enriched biosolids granules. Appl. Environ. Soil Sci. 2013:11. doi: 10.1155/2013/694597
Antille, D. L., Sakrabani, R., Tyrrel, S. F., Le, M. S., and Godwin, R. J. (2013b). Development of organomineral fertilisers derived from nutrient-enriched biosolids granules: product specification. Am. Soc. Agricult. Biol. Eng. Ann. Int. Meet. 5, 4152–4170. doi: 10.13031/aim.20131620153
Asli, S., and Neumann, P. M. (2010). Rhizosphere humic acid interacts with root cell walls to reduce hydraulic conductivity and plant development. Plant Soil 336, 313–322. doi: 10.1007/s11104-010-0483-2
Bouhia, Y., Hafidi, M., Ouhdouch, Y., Boukhari, M. E. M., El Mphatso, C., Zeroual, Y., et al. (2022). Conversion of waste into organo-mineral fertilizers: current technological trends and prospects. Rev. Environ. Sci. Biotechnol. 21, 425–446. doi: 10.1007/s11157-022-09619-y
Bouhia, Y., Hafidi, M., Ouhdouch, Y., Zeroual, Y., and Lyamlouli, K. (2023). Organo-mineral fertilization based on olive waste sludge compost and various phosphate sources improves phosphorus agronomic efficiency, zea mays agro-physiological traits, and water availability. Agronomy 13, 1–22. doi: 10.3390/agronomy13010249
Bouman, O. T., Curtin, D., Campbell, C. A., Biederbeck, V. O., and Ukrainetz, H. (1995). Soil acidification from long-term use of anhydrous ammonia and urea. Soil Sci. Soc. A. J. 59, 1488–1494. doi: 10.2136/sssaj1995.03615995005900050039x
Carneiro, J. S. da. S., Ribeiro, I. C. A., Nardis, B. O., Barbosa, C. F., Lustosa Filho, J. F., and Melo, L. C. A. (2021). Long-term effect of biochar-based fertilizers application in tropical soil: agronomic efficiency and phosphorus availability. Sci. Tot. Environ. 760:143955. doi: 10.1016/j.scitotenv.2020.143955
Conte, P., Agretto, A., Spaccini, R., and Piccolo, A. (2005). Soil remediation: humic acids as natural surfactants in the washings of highly contaminated soils. Environ. Pollut. 135, 515–522. doi: 10.1016/j.envpol.2004.10.006
Dal Molin, S. J., Ernani, P. R., and Gerber, J. M. (2020). Soil acidification and nitrogen release following application of nitrogen fertilizers. Commun. Soil Sci. Plant Anal. 51, 2551–2558. doi: 10.1080/00103624.2020.1845347
de Morais, E. G., Silva, C. A., Maluf, H. J. G. M., Paiva, I. de. O., and de Paula, L. H. D. (2023). Effects of compost-based organomineral fertilizers on the kinetics of NPK release and maize growth in contrasting oxisols. Waste Biomass Valorizat. 14, 2299–2321. doi: 10.1007/s12649-022-02019-x
EC (2019). Regulation (EU) 2019/1009 of the European Parliament and of the Council of 5 June 2019 laying down rules on the making available on the market of EU fertilising products and amending Regulation (EC) No 1069/2009 and (EC) No 1107/2009 and repealing Regulat. Off. J. Eur. Un. 2019, 1–114.
Ece, A., and Darakci, N. (2009). Effect of number of different stems on some fruit quality characteristics and yield in tomatoes (Lycopersicon lycopersicum L.). J. Appl. Biol. Sci. 3, 160–163.
Florio, A., Felici, B., Migliore, M., Dell'Abate, M. T., and Benedetti, A. (2016). Nitrogen losses, uptake and abundance of ammonia oxidizers in soil under mineral and organo-mineral fertilization regimes. J. Sci. Food Agricult. 96, 2440–2450. doi: 10.1002/jsfa.7364
Gaj, R., Szulc, P., Siatkowski, I., and Waligóra, H. (2020). Assessment of the effect of the mineral fertilization system on the nutritional status of maize plants and grain yield prediction. Agriculture 10, 1–14. doi: 10.3390/agriculture10090404
Humpenöder, F., Karstens, K., Lotze-Campen, H., Leifeld, J., Menichetti, L., Barthelmes, A., et al. (2020). Peatland protection and restoration are key for climate change mitigation. Environ. Res. Lett. 15:104093. doi: 10.1088/1748-9326/abae2a
Jackson, G. D. (2000). Effects of nitrogen and sulfur on canola yield and nutrient uptake. Agron. J. 92, 644–649. doi: 10.2134/agronj2000.924644x
Jiang, C., Johkan, M., Hohjo, M., Tsukagoshi, S., and Maturo, T. (2017). A correlation analysis on chlorophyll content and SPAD value in tomato leaves. HortResearch 71, 1–6. doi: 10.20776/S18808824-71-P37
Li, Y., Fang, F., Wei, J., Wu, X., Cui, R., Li, G., et al. (2019). Humic acid fertilizer improved soil properties and soil microbial diversity of continuous cropping peanut: a three-year experiment. Sci. Rep. 9, 1–9. doi: 10.1038/s41598-019-48620-4
Llovet, A., Vidal-Durà, A., Alcañiz, J. M., Ribas, A., and Domene, X. (2023). Biochar addition to organo-mineral fertilisers delays nutrient leaching and enhances barley nutrient content. Archiv. Agron. Soil Sci. 69, 2537–2551. doi: 10.1080/03650340.2022.2161092
Mainero, D. (2008). “An advanced example of anaerobic-aerobic integrated system. Venice 2008,” in Second International Symposium on Energy from Biomass and Waste, 17–20. Available online at: www.compost.it (accessed February 15, 2024).
Mandal, A., Patra, A. K., Singh, D., Swarup, A., and Ebhin Masto, R. (2007). Effect of long-term application of manure and fertilizer on biological and biochemical activities in soil during crop development stages. Bioresour. Technol. 98, 3585–3592. doi: 10.1016/j.biortech.2006.11.027
Mazeika, R., Dambrauskas, T., Baltakys, K., Mikolajunas, M., Staugaitis, G., Virzonis, D., et al. (2016). Molecular and morphological structure of poultry manure derived organo-mineral fertilizers (OMFs). ACS Sustain. Chem. Eng. 4, 4788–4796. doi: 10.1021/acssuschemeng.6b00984
Meier, U. (2001). Growth stages of mono- and dicotyledonous plants. Berlin, Germany German Federal Biological Research Centre for Agriculture and Forestry, BBCH-Monograph, Blackwell Science. Available online at: http://pub.jki.bund.de/index.php/BBCH/article/view/515/464
Moretti, B., Bertora, C., Grignani, C., Lerda, C., Celi, L., and Sacco, D. (2020). Conversion from mineral fertilisation to MSW compost use: nitrogen fertiliser value in continuous maize and test on crop rotation. Sci. Tot. Environ. 705:135308. doi: 10.1016/j.scitotenv.2019.135308
Ohno, T., and Zibilske, L. M. (1991). Determination of low concentrations of phosphorus in soil extracts using malachite green. Soil Sci. Soc. A. J. 55, 892–895. doi: 10.2136/sssaj1991.03615995005500030046x
Pare, M. C., Allaire, S. E., Khiari, L., and Parent, L. E. (2010). Improving physical properties of organo-mineral fertilizers: substitution of peat by pig slurry composts. Appl. Eng. Agricult. 26, 447–454. doi: 10.13031/2013.29945
Parish, F., Sirin, A., Charman, D., Joosten, H., Minayeva, T., Silvius, M., et al. (2008). Assessment on Peatlands, Biodiversity and Climate Change: Main Report. Global Environment Centre, Kuala Lumpur; Wetlands International, Wageningen.
Piccolo, A. (2002). The supramolecular structure of humic substances: a novel understanding of humus chemistry and implications in soil science. Adv. Agron. 75, 57–134. doi: 10.1016/S0065-2113(02)75003-7
Rady, M. M. (2012). A novel organo-mineral fertilizer can mitigate salinity stress effects for tomato production on reclaimed saline soil. South Afri. J. Bot. 81, 8–14. doi: 10.1016/j.sajb.2012.03.013
Richards, J. E., Daigle, J.-Y., LeBlanc, P., Paulin, R., and Ghanem, I. (1993). Nitrogen availability and nitrate leaching from organo-mineral fertilizers. Can. J. Soil Sci. 73, 197–208. doi: 10.4141/cjss93-022
Rietra, R. P. J. J., Heinen, M., Dimkpa, C. O., and Bindraban, P. S. (2017). Effects of nutrient antagonism and synergism on yield and fertilizer use efficiency. Commun. Soil Sci. Plant Anal. 48, 1895–1920. doi: 10.1080/00103624.2017.1407429
Robertson, G. P., and Vitousek, P. M. (2009). Nitrogen in agriculture: balancing the cost of an essential resource. Ann. Rev. Environ. Resour. 34, 97–125. doi: 10.1146/annurev.environ.032108.105046
Ros, M., Klammer, S., Knapp, B., Aichberger, K., and Insam, H. (2006). Long-term effects of compost amendment of soil on functional and structural diversity and microbial activity. Soil Use Manag. 22, 209–218. doi: 10.1111/j.1475-2743.2006.00027.x
Saarikoski, H., Mustajoki, J., Hjerppe, T., and Aapala, K. (2019). Participatory multi-criteria decision analysis in valuing peatland ecosystem services—trade-offs related to peat extraction vs. pristine peatlands in Southern Finland. Ecol. Econ. 162, 17–28. doi: 10.1016/j.ecolecon.2019.04.010
Sakurada, R., Batista, L. M. A., Inoue, T. T., Muniz, A. S., and Pagliar, P. H. (2016). Organomineral phosphate fertilizers: agronomic efficiency and residual effect on initial corn development. Agron. J. 108, 2050–2059. doi: 10.2134/agronj2015.0543
Salvagiotti, F., Castellarín, J. M., Miralles, D. J., and Pedrol, H. M. (2009). Sulfur fertilization improves nitrogen use efficiency in wheat by increasing nitrogen uptake. Field Crops Res. 113, 170–177. doi: 10.1016/j.fcr.2009.05.003
Schmilewski, G. (2008). The role of peat in assuring the quality of growing media. Mires Peat 3, 1–8.
Sica, P., Kopp, C., Müller-Stöver, D. S., and Magid, J. (2023). Acidification and alkalinization pretreatments of biowastes and their effect on P solubility and dynamics when placed in soil. J. Environ. Manag. 333:117447. doi: 10.1016/j.jenvman.2023.117447
Sitzmann, T. J., Sica, P., Grignani, C., and Magid, J. (2024a). Testing biowaste materials as peat replacement in organo-mineral fertilizers. Front. Sustain. Food Syst. 8:1330843. doi: 10.3389/fsufs.2024.1330843
Sitzmann, T. J., Sica, P., Zavattaro, L., Moretti, B., Grignani, C., and Oberson, A. (2024b). An isotope study on nitrogen and phosphorus use efficiency and movement in soil in a mimicked vermicompost-based organo-mineral fertilizer. Agrosyst. Geosci. Environ. 7:e20473. doi: 10.1002/agg2.20473
Sitzmann, T. J., Zavattaro, L., Tagliavini, S., Celi, L., Padoan, E., Moretti, B., et al. (2023). Suitability of recycled organic materials in organo-mineral fertilizers manufacturing: a discussion about organic geogenic material replacement. SSRN 2023:4653759. doi: 10.2139/ssrn.4653759
Smith, W. B., Wilson, M., and Pagliari, P. (2020). “Organomineral fertilizers and their application to field crops,” in Animal Manure: Production, Characteristics, Environmental Concerns, and Management (Hoboken, NJ: John Wiley & Sons, Ltd), 229–244.
Taparia, T., Hendrix, E., Nijhuis, E., de Boer, W., and van der Wolf, J. (2021). Circular alternatives to peat in growing media: a microbiome perspective. J. Clean. Product. 327:129375. doi: 10.1016/j.jclepro.2021.129375
Vitousek, P. M., Porder, S., Houlton, B. Z., and Chadwick, O. A. (2010). Terrestrial phosphorus limitation: mechanisms, implications, and nitrogen-phosphorus interactions. Ecol. Appl. 20, 5–15. doi: 10.1890/08-0127.1
Vujinović, T., Zanin, L., Venuti, S., Contin, M., Ceccon, P., Tomasi, N., et al. (2020). Biostimulant action of dissolved humic substances from a conventionally and an organically managed soil on nitrate acquisition in maize plants. Front. Plant Sci. 10:1652. doi: 10.3389/fpls.2019.01652
Wood, C. W., Reeves, D. W., and Himelrick, D. G. (1993). “Relationships between chlorophyll meter readings and leaf chlorophyll concentration, N status, and crop yield: a review,” in Proceedings of the Agronomy Society of New Zealand, Vol. 23, 1–9. Available online at: https://scholar.google.com/scholar?cluster=3291803579416522208&hl=en&oi=scholarr#0
Keywords: biowaste, municipal solid waste, peat replacement, pruning compost, vermicompost
Citation: Sitzmann TJ, Alpigiano A, Lerda C, Moretti B, Zavattaro L and Grignani C (2024) Response of tomato to innovative organo-mineral fertilizers. Front. Sustain. Food Syst. 8:1385828. doi: 10.3389/fsufs.2024.1385828
Received: 13 February 2024; Accepted: 19 June 2024;
Published: 15 July 2024.
Edited by:
Rachel E. Thorman, Agricultural Development Advisory Service, United KingdomReviewed by:
Maria Pilar Bernal, Spanish National Research Council (CSIC), SpainDiogenes Antille, Commonwealth Scientific and Industrial Research Organisation (CSIRO), Australia
Copyright © 2024 Sitzmann, Alpigiano, Lerda, Moretti, Zavattaro and Grignani. This is an open-access article distributed under the terms of the Creative Commons Attribution License (CC BY). The use, distribution or reproduction in other forums is permitted, provided the original author(s) and the copyright owner(s) are credited and that the original publication in this journal is cited, in accordance with accepted academic practice. No use, distribution or reproduction is permitted which does not comply with these terms.
*Correspondence: Laura Zavattaro, laura.zavattaro@unito.it