- 1School of Pharmacy, Changchun University of Chinese Medicine, Changchun, Jilin, China
- 2School of Traditional Chinese Medicine, Changchun University of Chinese Medicine, Changchun, Jilin, China
- 3School of Electrical Engineering, Dalian University of Technology, Dalian, Liaoning, China
- 4Jiangxi University of Traditional Chinese Medicine, Nanchang, China
Pulsed Electric Fields (PEF) technology is an emerging non-thermal food processing technology that is widely used because of its low damage to food quality and its ability to kill harmful microorganisms in food. The oxidative stress caused by PEF results in the synthesis and accumulation of nutrients, and plant foods stimulated by PEF produce large amounts of Reactive Oxygen Species (ROS), which activate the metabolite synthesis pathway and eventually synthesize proteins, polyphenols, thioglucosides and carotenoids; ROS accumulate in the cell membrane of microorganisms and cause protein expression. The accumulation of ROS in the cell membrane of microorganisms can cause abnormal protein expression, damage the lipid layer and Deoxyribonucleic Acid (DNA), and eventually lead to microbial inactivation. PEF technology in food processing are multifaceted, enhancing both the nutritional value of food through the augmented presence of health-beneficial compounds and ensuring food safety by effectively inactivating harmful microorganisms. This innovative technology stands at the forefront of food processing solutions, promising to fulfill the growing consumer demand for foods that are not only safe and of high quality but also rich in nutritional value. PEF’s role as a cornerstone technology in the food industry heralds a new era of food processing, where nutrition and safety converge to meet the sophisticated needs of today’s consumers.
1 Introduction
Traditional food processing techniques, while essential for nutrient extraction, food storage, and drying, often negatively impact the nutritional value and safety of food. These techniques, including large-scale industrial processes like heat treatment, can lead to nutrient loss and the formation of hazardous substances, ultimately posing risks to human health (Vorobiev and Lebovka, 2006; Aghamirzaei et al., 2015; Pan et al., 2017). The primary drawback of these traditional methods is their tendency to compromise the nutritional quality and flavor of food in pursuit of processing efficiency (Golshan Tafti et al., 2013; Tafti et al., 2013). Specifically, thermal processing methods can irreversibly damage thermally unstable nutrients or those present in trace amounts, thereby hindering nutrient accumulation in foods (Lee et al., 2011).
Furthermore, the reduction in nutrient levels is exacerbated by the excessive use of chemical additives in some traditional processing techniques, raising concerns about their long-term implications for food quality and safety (Sanchez-Moreno et al., 2009). This context highlights the urgent need for innovative food processing technologies. Such technologies aim to not only minimize nutrient loss but also enhance the biosynthesis of vital compounds like phenols, carotenoids, and proteins through the stimulation of metabolic pathways (Cisneros-Zevallos, 2003; Jacobo-Velazquez and Cisneros-Zevallos, 2012; Jacobo-Velazquez and Cisneros-Zevallos, 2013). Emerging techniques, including high static pressure, pulsed electric fields (PEF), ultrasound, and ultraviolet light, represent promising alternatives to traditional methods by offering the potential to improve both the nutritional quality and safety of food products (Chen et al., 2010; Kumar and Han, 2012; Xu et al., 2017; Asaithambi et al., 2021; Chen et al., 2022; Liu et al., 2023).
With advantages such as requiring low temperatures, rapid processing time, and being environmentally friendly, PEF is rapidly gaining attention as a promising food processing technology (Manzoor et al., 2019a,b; Rahaman et al., 2020; Lal et al., 2021). PEF causes negligible damage to food nutrients compared to traditional food processing techniques and can eliminate some hazardous microorganisms to extend the preservation time of food (Soliva-Fortuny et al., 2009; Napotnik et al., 2016; Peng et al., 2016; Liu C. Y. et al., 2021; Nabilah et al., 2022; Preetha et al., 2023). Most research in PEF has focused on active substance extraction, pasteurization, and the drying and thawing of food products, and there is relatively little research on the influence of PEF on biometabolic pathways that induce the synthesis of nutrients in food products (Boussetta et al., 2011, 2012; Rahaman et al., 2019; Ahmed et al., 2020; Preetha et al., 2021).
PEF causes serious stimulation and damage to plants, resulting in the production of reactive oxygen species (ROS), which can further damage plant cells. Plants secrete many metabolites to protect themselves by reducing this damage, and some of these metabolites are also essential nutrients for the human body (Kris-Etherton et al., 2002; Liu J. H. et al., 2021). These nutrients include primary and secondary metabolites, such as polyphenolic compounds and proteins, which have a strong protective effect on human cells and reduce the damage caused by oxidative stress in the human body (Kris-Etherton et al., 2002; Alegret, 2007). In response to external stimuli, plant cells undergo an immediate response, releasing stress signaling factors to activate signaling networks, which then participate in the synthesis of transcription factors and eventually generate metabolites. In the stress physiology of plant foods after treatment with PEF (Pereira et al., 2009; Vallverdu-Queralt et al., 2012; Banks et al., 2015; Kasprzycka et al., 2021), this process causes an increase in nutrients (Vallverdu-Queralt et al., 2013). PEF increases cell membrane permeability and even causes cell membrane rupture, which triggers a stress response in cells, similar to injury stress (Bussler et al., 2015). The cells then secrete several substances to protect themselves from damage. When PEFs are applied to microorganisms, they also produce large amounts of ROS, which will eventually cause structural damage to DNA and proteins, lipid peroxidation, and even cell membrane rupture, leading to the death of microorganisms and achieving sterilization (Sierra and McComb, 2008; Takatsuji et al., 2017; Wang et al., 2022).
This paper reviews the synthesis of plant nutrients through the oxidative stress pathway in PEF to enhance the extraction rate of active ingredients. The mechanism of microbial killing by PEF through the oxidative stress pathway is also summarized to provide future research directions for the effects of PEF technology on food quality via the oxidative stress pathway.
2 PEF induces nutrient synthesis in plant foods
Previous studies have shown that PEF stimulates cells and thus, greatly increases intracellular ROS generation (Gabriel and Teissie, 1994; Pereira et al., 2009). It has also been shown that the level of ROS is closely related to the health status of cells and is usually a common indicator of plant cell response to stress owing to damage caused by external stimuli. Specifically, when plant cell membrane is damaged or ruptured, ATP released from the damaged cell binds to its cell membrane receptor resulting in ROS production (Vallverdu-Queralt et al., 2012, 2013). Thus, when plants are exposed to external stimuli, their stress response is triggered. It has also been shown that ethylene production involves the conversion of methionine, generation of aminoethoxyvinylglycine, and release of ethylene. Further, the synthesis of jasmonic acid primarily involves the lipid metabolism pathway, wherein lipid molecules, such as phospholipids undergo oxidation and degradation to produce precursors of jasmonic acid after which enzymes, such as lipoxygenases, play a pivotal role in the synthesis of jasmonic acid from these precursors by catalyzing lipid oxidation reactions (Vallverdu-Queralt et al., 2012). These secondary stress signaling molecules diffuse through the cell membrane and initiate signal transduction networks leading to the activation of transcription factors that promote nutrient synthesis in plants (Jacobo-Velazquez et al., 2017). The ROS signaling pathway typically involves the activation of specific transcription factors, such as those belonging to the MYB (Myeloblastosis) and bHLH (basic Helix–Loop–Helix) families. These factors play crucial regulatory roles in controlling the synthesis of phenolic compounds. Further, activated transcription factors stimulate the expression of relevant genes that encode the key enzymes involved in the biosynthesis pathways of phenolic compounds, such as phenoloxidases, peroxidases, and polyphenoloxidases. Under induction by ROS and ethylene, plants initiate the synthesis and accumulation of various phenolic compounds, including flavonoids, anthocyanins, phenolic acids, and polyphenols. These compounds then exhibit diverse biological activities, such as antioxidant activity, resist pathogen infection, and act as a defense against oxidative stress (Jacobo-Velazquez et al., 2015). Conversely however, ethylene and jasmonic acid induce the synthesis of thioglucosidic substances (Villarreal-Garcia et al., 2016). ROS also induce the synthesis of compounds, such as carotenoids (Bouvier et al., 1998; Figure 1).
PEF technology is lauded for its ability to enhance the synthesis of secondary metabolites in plant cells through significant stimulation, yet it also poses potential collateral damage to plant cells, potentially impacting plant metabolism and overall quality (Attri et al., 2022). To mitigate these adverse effects, optimizing PEF parameters—such as intensity, duration, and frequency—is crucial to minimize cellular damage while maximizing the production of desired metabolites. By fine-tuning the PEF process parameters, we can ensure that they contribute positively to food quality and safety, aligning with the overarching goal of improving the healthfulness and sustainability of our food systems (Pipliya et al., 2023; Trusinska et al., 2023; Negi et al., 2024).
2.1 Phenols and carotenoids
Galindo et al. (2009) treated potatoes with PEF (100–400 kv/cm, 1 ms) to produce tissue trauma and then observed a series of metabolic reactions. First, a significant amount of ROS was generated, peaking at 30–60 min (Bolwell, 1996). Second, crosslinking of hydroxyproline-rich glycoproteins in the cell wall of potatoes occurred within 1 min after treatment. Over time, biosynthesis of suberin occurred at the cut surface (Bernards et al., 1999). This was accompanied by a significant increase in chlorogenic acid, which improves the protection of plant tissues against oxidative stress (Matsuda et al., 2003). Levels of cell membrane-associated metabolites, such as sterols and galactosylceramide, were also significantly increased after injury (Piironen et al., 2000). After 24 h, the potatoes synthesized peptides to increase protein content in response to oxidative stress damage (von Wiren et al., 1999).
Leong et al. investigated the effect of PEF treatment on the protective responses in different carrot species to resist H2O2-induced oxidative damage (Table 1). Purple Haze and Nutri Red under 303 kJ/kg treatment improved the resistance of Caco-2 cells to oxidative damage by restoring cell viability and inhibiting NO production (Leong et al., 2016a). The total carotenoid content in purée was higher than that of the untreated variety under treatment conditions of 0.8 kV/cm. Total carotenoids increased significantly when the electric field intensity was increased from 0.3 kV/cm to 0.8 kV/cm. The total phenolic content increased significantly when the energy input was increased from 35 to 303 kJ/kg compared to the untreated control. The greatest increase in total phenolic content was observed after the PEF treatment at 303 kJ/kg compared to that after PEF treatment under different conditions. These findings suggest that greater recovery of total phenols from carrots may require more intense treatment, either by increasing the electric field intensity to induce higher cell damage or by supplying more energy to the cells to make cell damage last longer and to damage more cells. The Yellow Solar purée extract obtained at 0.8 kV/cm significantly increased Caco-2 cell viability and inhibited lactate dehydrogenase leakage and NO production. PEF-treated carrot extracts exhibited a good protective effect on cell viability and were able to restore the number of viable cells and the percentage of NO production in oxidatively stressed (H2O2-containing) cells to levels similar to those in non-stressed (H2O2-free) cells. Furthermore, the cytoprotective capacity of PEF extracts was linearly related to total carotenoid content and total anthocyanin content. For Yellow Solar carrots, which had a low total carotenoid content, PEF significantly increased the carotenoid content (Leong et al., 2016a; Rezaeinezhad et al., 2022). Leong et al. (2016b) also found that PEF treatment shortened the immersion time and improved the protection against H2O2 oxidative stress in Vitis Vinifera. Leong et al. also evaluated the effect of PEFs on the release of anthocyanins and the health effects of Pinot noir grape juice; Pinot noir grapes treated with PEF were able to produce substances with stronger antioxidant activity and exhibited greater protection against oxidative stress (Leong et al., 2016c; Chen et al., 2023).
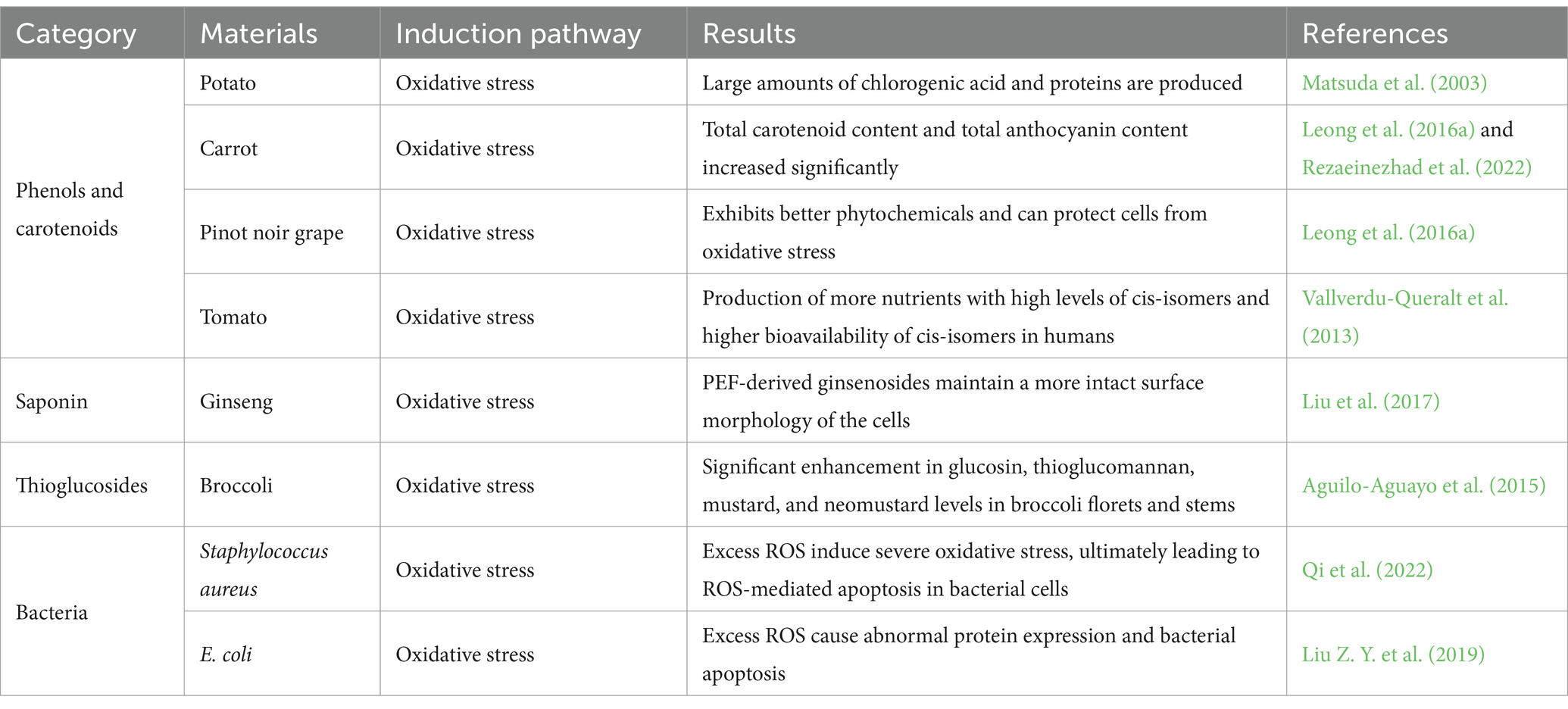
Table 1. Effect of PEF on the synthesis of bioactive substances and bacterial apoptosis through the oxidative stress pathway.
PEF-processed tomatoes contained much higher concentrations of lutein, carotene, trans-lycopene, and cis-lycopene than heat-processed tomatoes. This was not only because the low-temperature treatment reduced nutrient decomposition but also because tomato cells damaged by PEF released ATP. This ATP diffused between cellular spaces as a trauma signal, thereby stimulating a stress response, enhancing the metabolic activity of tomatoes, and synthesizing a large number of nutrients (Basu et al., 2001; Perveen et al., 2015; Desmarchelier et al., 2018; Ali et al., 2021). Application of PEF treatment leads to the production of more nutrients with high levels of cis-isomers and higher bioavailability of cis-isomeric nutrients in the human body. Vallverdú-Queralt et al. applied medium intensity (1 kV/cm, 16 monopolar pulses of 4 μs, 0.1 Hz) PEF to raw tomatoes, which increased the content of 15-cis lycopene in tomato juice by 63%–65% (Vallverdu-Queralt et al., 2013). Martinez et al. (2020) used PEF (15 kV/cm, 6.8 μs pulse width) to aid the extraction of carotenoids from dried Rhodotorula glutinis yeast and improved the extraction rate of carotenoids through PEF by optimizing the experimental conditions. The extraction rate was 20% without PEF treatment but increased to 80% after PEF treatment. Kokkali et al. (2020) found that the antioxidant capacity of PEF-treated microalgae bio extracts was significantly enhanced. PEF can increase the number of nutrients synthesized in plant-based foods, which can be utilized to customize extraction parameters for low levels of active substances and synthesize more of the nutrients required by humans (Leong et al., 2016a; Rezaeinezhad et al., 2022).
2.2 Thioglucosides
Thioglucoside is widely used in the pharmaceutical and food industries for its antibacterial, neuroprotective, and anticancer effects (Watson et al., 2013; Choudhury et al., 2022; Hoffmann et al., 2022; Marcinkowska and Jelen, 2022; Youseif et al., 2022; Zhou et al., 2022). Thioglucoside is water-soluble and heat-sensitive; therefore, heating causes a large loss of thioglucosides, and heat treatment should be avoided when extracting these components (Hoffmann et al., 2022; Marcinkowska and Jelen, 2022). Thioglucoside maintains stable chemical properties and biological activity in the subcellular space of plant tissues. When cells rupture, black mustard enzymes come into contact with and hydrolyze thioglucoside, and the main product, isothiocyanate, is responsible for the strong taste of mustard, radish, and broccoli sprouts. The loss of thioglucoside is also closely related to the inactivation of black mustard enzymes (Hanschen and Schreiner, 2017). Whether PEF can inactivate black mustard enzymes for the purpose of retaining thioglucosides has been a focus of research (Yen and Wei, 1993; Ghawi et al., 2012; Cebeci et al., 2022).
PEF injury triggers the production of ROS, which activates plant primary and secondary metabolism (Jacobo-Velazquez et al., 2015). In addition, other signaling molecules, such as ethylene and jasmonic acid, are produced after injury and play a key role as regulators of ROS levels and the expression of secondary metabolic genes, triggering the accumulation of specific secondary metabolites (Torres-Contreras et al., 2014a,b). In the case of broccoli, wounding triggers the biosynthesis and accumulation of indolic glucosinolates (Torres-Contreras et al., 2014b). The black mustard enzyme in broccoli catalyzes the enzymatic reaction of thioglucosinolates to produce other bioactive products, the content of which is the main factor affecting the quality of broccoli products. Frandsen et al. found that PEF treatment at 35 kv/cm inactivated the black mustard enzyme and that most thioglucosins were degraded prior to PEF treatment. If PEF can successfully extract thioglucoside, then the inactivation of the black mustard enzyme by high pressure treatment is required (Frandsen et al., 2014).
Extraction of thioglucosides from broccoli flower stems using PEF technology was performed by Aguilo-Aguayo et al. (2015). The samples were subjected to an electric field intensity of 1–4 kV/cm at 5 Hz and a treatment time of 50 to 1,000 μs. The results of the experiments revealed that glucosinolates, thioglucosinolates, mustardolates, and neo mustardolates increased by approximately two-fold in broccoli florets after PEF pretreatment, while black mustard enzymes were found to remain active. This experiment found that PEF can increase the extraction efficiency of thioglucosides without enzyme inactivation, which may become a new research direction.
3 PEF induces bacterial apoptosis
PEF technologies are potentially the most important cold pasteurization and sterilization food preservation technique to replace or partially substitute thermal processes. During the PEF process, lysis of microorganisms is caused by irreversible structural changes in the membranes, leading to pore formation and destruction of the semipermeable barrier of the membrane (Qin et al., 1996; Lasekan et al., 2017; Pimentel et al., 2023; Wei et al., 2023; Wu et al., 2023; Zhu et al., 2023; Wang et al., 2024; Figure 2).
Qi et al. (2022) analyzed the mechanism of PEF action on Staphylococcus aureus from the perspective of ROS-mediated oxidative stress. After PEF treatment, the level of ROS in S. aureus increased, and the excess ROS induced severe oxidative stress, leading to lipid peroxidation, DNA oxidation, cytosolic fatty acid damage, structural damage to proteins and DNA, and significant abnormalities in the expression of oxidative stress-related genes, ultimately leading to ROS-mediated apoptosis in bacterial cells (Qi et al., 2022), as shown in Figure 2. Liu Z. Y. et al. (2019) compared the changes in the protein expression of E. coli before and after PEF treatment using mass spectrometry. Excessive accumulation of ROS has been shown to significantly reduce AcnB protein levels in superoxide dismutase-deficient Salmonella enterica (Thorgersen and Downs, 2009). Another upregulated enzyme was the bifunctional proline utilization enzyme A (PutA; A0A0G3K113), the deletion of which in E. coli has been shown to increase cellular susceptibility to oxidative damage (Zhang et al., 2015). Membrane permeability increases after applying PEF to E. coli, which not only increases the entry of harmful substances into E. coli cells but also causes osmotic imbalance (Aronsson et al., 2005). Particularly strong electric fields can cause irreparable perforations in the cell membrane, leading to irreversible cell damage, destruction, and eventual death (Locke et al., 2006). To ensure the inactivation of microorganisms, an electric field with a field strength of 25 kV/cm or more is required in food processing (Toepfl et al., 2007).
4 Conclusions future research
As an emerging non-thermal processing technology for food, PEF has become a hot new research topic due to its ability to increase nutrient content and kill microorganisms through oxidative stress pathways (Zulueta et al., 2013; Jacobo-Velazquez et al., 2017; Andreou et al., 2022). PEF stimulates plants to secrete metabolites by causing damage to plant cells, thereby increasing the accumulation of nutrients (Ye et al., 2004; Liu Z. B. et al., 2019). To elucidate the conditions for the optimal accumulation of nutrients in food caused by PEF, future research should be based on “omics” approaches (genomics, proteomics, and metabolomics) to elucidate the mechanisms in terms of genetic material and proteins, allowing the design of optimized biological processes to obtain the highest accumulation of bioactive molecules in plant foods.
PEF technology has a high extraction efficiency compared to traditional extraction techniques and can extract nutrients more efficiently (Minamitani et al., 2015; Qi et al., 2022). The nutrients extracted from PEF have a more pronounced health effect on the human body (Wang et al., 2013; Zandalinas and Mittler, 2018; Jin et al., 2021). The cis-isomeric metabolites produced by PEF stimulation are better absorbed by the body, and PEF extracts significantly increase cell viability (During and Harrison, 2004; Unlu et al., 2007). We can use this theory to customize extraction methods for nutrients that have strong health effects but are low in content.
In addition to increasing nutrient accumulation, oxidative stress damage after PEF stimulation can also kill microorganisms. In addition to membrane damage, which is the main cause of microbial inactivation, PEF affects the metabolism of microorganisms through the oxidative stress pathway and kills microorganisms by affecting their protein expression and damaging their genetic material. The process of killing microorganisms does not cause a thermal effect, does not affect the taste of food, and even increases the accumulation of nutrients (Morales-de la Pena et al., 2011; Khan et al., 2017). Thus, PEF treatment results in foods with increased nutrient content and low microbial content, with no adverse effects on food quality parameters such as taste and color, meaning the produced foods can be marketed as commodities or raw materials for further processing (Ortega-Rivas and Salmeron-Ochoa, 2014; Misra et al., 2017; Skowron and Wantuch, 2020).
5 Future research
In the field of food processing, the PEF extraction technique only allows the treatment of a limited volume of food owing to the dual limitations of field strength and electrode spacing, which are particularly prominent in industrial-scale applications. Under such conditions, a small flux makes industrial scale-up challenging. Further, even though PEF shows potential for highly efficient, safe, and environmental friendly food processing, the small volume of its treatment chamber and the lack of flexibility limit its industrial scale application. Therefore, strategies for obtaining a sufficiently large treatment chamber volume for large-scale industrialized food processing are necessary.
Finally, owing to its high maintenance cost, the PEF extraction technique is more intensive than other extraction technologies. Therefore, the design of a food processing unit that is suitable for large-scale industrialized applications is key for advancing this field. Until these technical and cost-related challenges are overcome, maximizing the potential of this extraction technology in the food processing field will remain challenging.
Author contributions
WS: Conceptualization, Data curation, Formal analysis, Investigation, Methodology, Project administration, Resources, Software, Supervision, Validation, Visualization, Writing – original draft, Writing – review & editing. QW: Investigation, Project administration, Writing – original draft, Writing – review & editing. JL: Resources, Writing – review & editing. ZQ: Funding acquisition, Writing – review & editing, Resources, Supervision, Visualization. YQ: Funding acquisition, Writing – review & editing.
Funding
The author(s) declare that financial support was received for the research, authorship, and/or publication of this article. This research was supported financially by the Education Department of Jilin Province (JJKH20210994KJ).
Conflict of interest
The authors declare that the research was conducted in the absence of any commercial or financial relationships that could be construed as a potential conflict of interest.
Publisher’s note
All claims expressed in this article are solely those of the authors and do not necessarily represent those of their affiliated organizations, or those of the publisher, the editors and the reviewers. Any product that may be evaluated in this article, or claim that may be made by its manufacturer, is not guaranteed or endorsed by the publisher.
References
Aghamirzaei, M., Peighambardoust, S. H., Azadmard-Damirchi, S., and Majzoobi, M. (2015). Effects of grape seed powder as a functional ingredient on flour physicochemical characteristics and dough rheological properties. J. Agric. Sci. Technol. 17, 365–373.
Aguilo-Aguayo, I., Suarez, M., Plaza, L., Hossain, M. B., Brunton, N., Lyng, J. G., et al. (2015). Optimization of pulsed electric field pre-treatments to enhance health-promoting glucosinolates in broccoli flowers and stalk. J. Sci. Food Agric. 95, 1868–1875. doi: 10.1002/jsfa.6891
Ahmed, Z., Manzoor, M. F., Ahmad, N., Zeng, X. A., Din, Z. U., Roobab, U., et al. (2020). Impact of pulsed electric field treatments on the growth parameters of wheat seeds and nutritional properties of their wheat plantlets juice. Food Sci. Nutr. 8, 2490–2500. doi: 10.1002/fsn3.1540
Alegret, M. (2007). Concentrated red grape juice exerts antioxidant, hypolipidemic, and antiinflammatory effects in both hemodialysis patients and healthy subjects. Clin E Invest Arterioscler 19, 265–266. doi: 10.1016/S0214-9168(07)74209-2
Ali, M. Y., Sina, A. I., Khandker, S. S., Neesa, L., Tanvir, E. M., Kabir, A., et al. (2021). Nutritional composition and bioactive compounds in tomatoes and their impact on human health and disease: a review. Food Secur. 10:45. doi: 10.3390/foods10010045
Andreou, V., Kourmbeti, E., Dimopoulos, G., Psarianos, M., Katsaros, G., and Taoukis, P. (2022). Optimization of virgin olive oil yield and quality applying nonthermal processing. Food Bioproc. Tech. 15, 891–903. doi: 10.1007/s11947-022-02788-2
Aronsson, K., Ronner, U., and Borch, E. (2005). Inactivation of Escherichia coli, listeria innocua and Saccharomyces cerevisiae in relation to membrane permeabilization and subsequent leakage of intracellular compounds due to pulsed electric field processing. Int. J. Food Microbiol. 99, 19–32. doi: 10.1016/j.ijfoodmicro.2004.07.012
Asaithambi, N., Singh, S. K., and Singha, P. (2021). Current status of non-thermal processing of probiotic foods: a review. J. Food Eng. 303:110567. doi: 10.1016/j.jfoodeng.2021.110567
Attri, P., Okumura, T., Koga, K., Shiratani, M., Wang, D. Y., Takahashi, K., et al. (2022). Outcomes of pulsed electric fields and nonthermal plasma treatments on seed germination and protein functions. Agronomy 12:482. doi: 10.3390/agronomy12020482
Banks, T. A., Luckman, P. S. B., Frith, J. E., and Cooper-White, J. J. (2015). Effects of electric fields on human mesenchymal stem cell behaviour and morphology using a novel multichannel device. Integr. Biol. 7, 693–712. doi: 10.1039/c4ib00297k
Basu, H. N., Del Vecchio, A. J., Flider, F., and Orthoefer, F. T. (2001). Nutritional and potential disease prevention properties of carotenoids. J. Am. Oil Chem. Soc. 78, 665–675. doi: 10.1007/s11746-001-0324-x
Bernards, M. A., Fleming, W. D., Llewellyn, D. B., Priefer, R., Yang, X. L., Sabatino, A., et al. (1999). Biochemical characterization of the suberization-associated anionic peroxidase of potato. Plant Physiol. 121, 135–146. doi: 10.1104/pp.121.1.135
Bolwell, G. P. (1996). The origin of the oxidative burst in plants. Biochem. Soc. Trans. 24, 438–442. doi: 10.1042/bst0240438
Boussetta, N., Vorobiev, E., Deloison, V., Pochez, F., Falcimaigne-Cordin, A., and Lanoiselle, J. L. (2011). Valorisation of grape pomace by the extraction of phenolic antioxidants: application of high voltage electrical discharges. Food Chem. 128, 364–370. doi: 10.1016/j.foodchem.2011.03.035
Boussetta, N., Vorobiev, E., Le, L. H., Cordin-Falcimaigne, A., and Lanoiselle, J. L. (2012). Application of electrical treatments in alcoholic solvent for polyphenols extraction from grape seeds. LWT-Food Sci Technol 46, 127–134. doi: 10.1016/j.lwt.2011.10.016
Bouvier, F., Backhaus, R. A., and Camara, B. (1998). Induction and control of chromoplast-specific carotenoid genes by oxidative stress. J. Biol. Chem. 273, 30651–30659. doi: 10.1074/jbc.273.46.30651
Bussler, S., Herppich, W. B., Neugart, S., Schreiner, M., Ehlbeck, J., Rohn, S., et al. (2015). Impact of cold atmospheric pressure plasma on physiology and flavonol glycoside profile of peas (Pisum sativum 'Salamanca'). Food Res. Int. 76, 132–141. doi: 10.1016/j.foodres.2015.03.045
Cebeci, F., Mayer, M. J., Rossiter, J. T., Mithen, R., and Narbad, A. (2022). Molecular cloning, expression and characterisation of a bacterial Myrosinase from Citrobacter Wye1. Protein J. 41, 131–140. doi: 10.1007/s10930-021-10034-5
Chen, Y., Belwal, T., Xu, Y., Ma, Q., Li, D., Li, L., et al. (2023). Updated insights into anthocyanin stability behavior from bases to cases: why and why not anthocyanins lose during food processing. Crit. Rev. Food Sci. Nutr. 63, 8639–8671. doi: 10.1080/10408398.2022.2063250
Chen, J. H., Chen, X., Zhou, G. H., and Xu, X. L. (2022). Ultrasound: a reliable method for regulating food component interactions in protein-based food matrices. Trends Food Sci. Technol. 128, 316–330. doi: 10.1016/j.tifs.2022.08.014
Chen, P., Deng, S., Cheng, Y., Lin, X., Metzger, L., and Ruan, R. (2010). “Non-thermal food pasteurization processes: an introduction” in Case studies in novel food processing technologies: Innovations in processing, Packaging, and Predictive Modelling. eds. C. J. Doona, K. Kustin, and F. E. Feeherry, 1–18.
Choudhury, S., Rao, M. H., Kashyap, A., Ahmaed, S., Prasad, L., Singh, N., et al. (2022). Jasmonate mediated inducible accumulation of indole glucosinolates confers resistance against Alternaria blight disease in cruciferous wild species Diplotaxis erucoides. Physiol. Mol. Plant Pathol. 122:101904. doi: 10.1016/j.pmpp.2022.101904
Cisneros-Zevallos, L. (2003). The use of controlled postharvest abiotic stresses as a tool for enhancing the nutraceutical content and adding-value of fresh fruits and vegetables. J. Food Sci. 68, 1560–1565. doi: 10.1111/j.1365-2621.2003.tb12291.x
Desmarchelier, C., Landrier, J. F., and Borel, P. (2018). Genetic factors involved in the bioavailability of tomato carotenoids. Curr. Opin. Clin. Nutr. Metab. Care 21, 489–497. doi: 10.1097/MCO.0000000000000515
During, A., and Harrison, E. H. (2004). Intestinal absorption and metabolism of carotenoids: insights from cell culture. Arch. Biochem. Biophys. 430, 77–88. doi: 10.1016/j.abb.2004.03.024
Frandsen, H. B., Markedal, K. E., Martin-Belloso, O., Sanchez-Vega, R., Soliva-Fortuny, R., Sorensen, H., et al. (2014). Effects of novel processing techniques on Glucosinolates and membrane associated Myrosinases in broccoli. Polish J Food Nutr Sci 64, 17–25. doi: 10.2478/pjfns-2013-0005
Gabriel, B., and Teissie, J. (1994). Generation of reactive-oxygen species induced by ELECTROPERMEABILIZATION of CHINESE-HAMSTER ovary cells and their consequence on cell viability. Eur. J. Biochem. 223, 25–33. doi: 10.1111/j.1432-1033.1994.tb18962.x
Galindo, F. G., Dejmek, P., Lundgren, K., Rasmusson, A. G., Vicente, A., and Moritz, T. (2009). Metabolomic evaluation of pulsed electric field-induced stress on potato tissue. Planta 230, 469–479. doi: 10.1007/s00425-009-0950-2
Ghawi, S. K., Methven, L., Rastall, R. A., and Niranjan, K. (2012). Thermal and high hydrostatic pressure inactivation of myrosinase from green cabbage: a kinetic study. Food Chem. 131, 1240–1247. doi: 10.1016/j.foodchem.2011.09.111
Golshan Tafti, A., Peighambardoust, S. H., Behnam, F., Bahrami, A., Aghagholizadeh, R., Ghamari, M., et al. (2013). Effects of spray-dried sourdough on flour characteristics and rheological properties of dough. Czech J. Food Sci. 31, 361–367. doi: 10.17221/183/2012-CJFS
Hanschen, F. S., and Schreiner, M. (2017). Isothiocyanates, nitriles, and Epithionitriles from Glucosinolates are affected by genotype and developmental stage in Brassica oleracea varieties. Front. Plant Sci. 8:1095. doi: 10.3389/fpls.2017.01095
Hoffmann, H., Andernach, L., Kanzler, C., and Hanschen, F. S. (2022). Novel transformation products from glucosinolate-derived thioglucose and isothiocyanates formed during cooking. Food Res. Int. 157:111237. doi: 10.1016/j.foodres.2022.111237
Jacobo-Velazquez, D. A., and Cisneros-Zevallos, L. (2012). An alternative use of horticultural crops: stressed plants as biofactories of bioactive phenolic compounds. Agriculture 2, 259–271. doi: 10.3390/agriculture2030259
Jacobo-Velazquez, D. A., and Cisneros-Zevallos, L. (2013). An alternative use of horticultural crops: stressed plants as biofactories of bioactive phenolic compounds (vol 2, pg 259, 2012). Agriculture 3, 596–598. doi: 10.3390/agriculture3030596
Jacobo-Velazquez, D. A., Cuellar-Villarreal, M. D., Welti-Chanes, J., Cisneros-Zevallos, L., Ramos-Parra, P. A., and Hernandez-Brenes, C. (2017). Nonthermal processing technologies as elicitors to induce the biosynthesis and accumulation of nutraceuticals in plant foods. Trends Food Sci. Technol. 60, 80–87. doi: 10.1016/j.tifs.2016.10.021
Jacobo-Velazquez, D. A., Gonzalez-Aguero, M., and Cisneros-Zevallos, L. (2015). Cross-talk between signaling pathways: the link between plant secondary metabolite production and wounding stress response. Sci. Rep. 5:8608. doi: 10.1038/srep08608
Jin, T. L., Wang, X., Deng, Z. Y., Liu, X. F., and Liang, D. C. (2021). ROS-induced dramatic lipid changes in Arabidopsis. Redox Rep. 26, 190–196. doi: 10.1080/13510002.2021.2002001
Kasprzycka, W., Trebinska-Stryjewska, A., Lewandowski, R. B., Stepinska, M., Osuchowska, P. N., Dobrzynska, M., et al. (2021). Nanosecond pulsed electric field only transiently affects the cellular and molecular processes of Leydig cells. Int. J. Mol. Sci. 22:1236. doi: 10.3390/ijms222011236
Khan, I., Tango, C. N., Miskeen, S., Lee, B. H., and Oh, D. H. (2017). Hurdle technology: a novel approach for enhanced food quality and safety—a review. Food Control 73, 1426–1444. doi: 10.1016/j.foodcont.2016.11.010
Kokkali, M., Marti-Quijal, F. J., Taroncher, M., Ruiz, M. J., Kousoulaki, K., and Barba, F. J. (2020). Improved extraction efficiency of antioxidant bioactive compounds from Tetraselmis chuiiand Phaedoactylum tricornutum using pulsed electric fields. Molecules 25:3921. doi: 10.3390/molecules25173921
Kris-Etherton, P. M., Hecker, K. D., Bonanome, A., Coval, S. M., Binkoski, A. E., Hilpert, K. F., et al. (2002). Bioactive compounds in foods: their role in the prevention of cardiovascular disease and cancer. Am. J. Med. 113, 71–88. doi: 10.1016/S0002-9343(01)00995-0
Kumar, P., and Han, J. H. (2012). “Packaging materials for non-thermal processing of food and beverages” in Emerging food packaging technologies: Principles and practice. eds. K. L. Yam and D. S. Lee, 323–334.
Lal, A. M. N., Prince, M. V., Kothakota, A., Pandiselvam, R., Thirumdas, R., Mahanti, N. K., et al. (2021). Pulsed electric field combined with microwave-assisted extraction of pectin polysaccharide from jackfruit waste. Innov. Food Sci. Emerg. Technol. 74:102844. doi: 10.1016/j.ifset.2021.102844
Lasekan, O., Ng, S., Azeez, S., Shittu, R., Teoh, L., and Gholivand, S. (2017). EFFECT OF PULSED ELECTRIC FIELD PROCESSING ON FLAVOR AND COLOR OF LIQUID FOODS. J Food Process Preserv 41:940. doi: 10.1111/jfpp.12940
Lee, H. S., Lee, H. J., Yu, H. J., Ju, D. W., Kim, Y., Kim, C. T., et al. (2011). A comparison between high hydrostatic pressure extraction and heat extraction of ginsenosides from ginseng (Panax ginseng CA Meyer). J. Sci. Food Agric. 91, 1466–1473. doi: 10.1002/jsfa.4334
Leong, S. Y., Burritt, D. J., and Oey, I. (2016b). Effect of combining pulsed electric fields with maceration time on merlot grapes in protecting Caco-2 cells from oxidative stress. Food Bioproc. Tech. 9, 147–160. doi: 10.1007/s11947-015-1604-y
Leong, S. Y., Burritt, D. J., and Oey, I. (2016c). Evaluation of the anthocyanin release and health-promoting properties of pinot noir grape juices after pulsed electric fields. Food Chem. 196, 833–841. doi: 10.1016/j.foodchem.2015.10.025
Leong, S. Y., Oey, I., and Burritt, D. J. (2016a). Pulsed electric field improves the bioprotective capacity of purees for different coloured carrot cultivars against H2O2−induced oxidative damage. Food Chem. 196, 654–664. doi: 10.1016/j.foodchem.2015.09.104
Liu, Z. B., Esveld, E., Vincken, J. P., and Bruins, M. E. (2019). Pulsed electric field as an alternative pre-treatment for drying to enhance polyphenol extraction from fresh tea leaves. Food Bioproc. Tech. 12, 183–192. doi: 10.1007/s11947-018-2199-x
Liu, J. H., Fu, C. C., Li, G. J., Khan, M. N., and Wu, H. H. (2021). ROS homeostasis and plant salt tolerance: plant Nanobiotechnology updates. Sustain. For. 13:552. doi: 10.3390/su13063552
Liu, C. Y., Grimi, N., Bals, O., Lebovka, N., and Vorobiev, E. (2021). Effects of pulsed electric fields and preliminary vacuum drying on freezing assisted processes in potato tissue. Food Bioprod. Process. 125, 126–133. doi: 10.1016/j.fbp.2020.11.002
Liu, D., Zhang, T., Chen, Z. F., Wang, Y., Ma, S., Liu, J. Y., et al. (2017). The beneficial effect of ginsenosides extracted by pulsed electric field against hydrogen peroxide-induced oxidative stress in HEK-293 cells. J. Ginseng Res. 41, 169–179. doi: 10.1016/j.jgr.2016.02.007
Liu, W. C., Zhang, M., Mujumdar, A. S., and Chen, J. J. (2023). Role of dehydration technologies in processing for advanced ready-to-eat foods: a comprehensive review. Crit. Rev. Food Sci. Nutr. 63, 5506–5520. doi: 10.1080/10408398.2021.2021136
Liu, Z. Y., Zhao, L. Y., Zhang, Q., Huo, N., Shi, X. J., Li, L. W., et al. (2019). Proteomics-based mechanistic investigation of Escherichia coli inactivation by pulsed electric field. Front. Microbiol. 10:2644. doi: 10.3389/fmicb.2019.02644
Locke, B. R., Sato, M., Sunka, P., Hoffmann, M. R., and Chang, J. S. (2006). Electrohydraulic discharge and nonthermal plasma for water treatment. Ind. Eng. Chem. Res. 45, 882–905. doi: 10.1021/ie050981u
Manzoor, M. F., Ahmad, N., Aadil, R. M., Rahaman, A., Ahmed, Z., Rehman, A., et al. (2019b). Impact of pulsed electric field on rheological, structural, and physicochemical properties of almond milk. J. Food Process Eng. 42:13299. doi: 10.1111/jfpe.13299
Manzoor, M. F., Zeng, X. A., Rahaman, A., Siddeeg, A., Aadil, R. M., Ahmed, Z., et al. (2019a). Combined impact of pulsed electric field and ultrasound on bioactive compounds and FT-IR analysis of almond extract. J Food Sci Technol 56, 2355–2364. doi: 10.1007/s13197-019-03627-7
Marcinkowska, M. A., and Jelen, H. H. (2022). Role of sulfur compounds in vegetable and mushroom aroma. Molecules 27:116. doi: 10.3390/molecules27186116
Martinez, J. M., Schottroff, F., Haas, K., Fauster, T., Sajfrtova, M., Alvarez, I., et al. (2020). Evaluation of pulsed electric fields technology for the improvement of subsequent carotenoid extraction from dried Rhodotorula glutinis yeast. Food Chem. 323:126824. doi: 10.1016/j.foodchem.2020.126824
Matsuda, F., Morino, K., Miyashita, M., and Miyagawa, H. (2003). Metabolic flux analysis of the phenylpropanoid pathway in wound-healing potato tuber tissue using stable isotope-labeled tracer and LC-MS spectroscopy. Plant Cell Physiol. 44, 510–517. doi: 10.1093/pcp/pcg063
Minamitani, Y., Kobayashi, Y., and Kageyama, R., (2015). Investigation of difference of origin of ROS generated in a cell by frequency components of pulsed electric field, In: 2015 IEEE Pulsed power conference (PPC), Austin, TX.
Misra, N. N., Koubaa, M., Roohinejad, S., Juliano, P., Alpas, H., Inacio, R. S., et al. (2017). Landmarks in the historical development of twenty first century food processing technologies. Food Res. Int. 97, 318–339. doi: 10.1016/j.foodres.2017.05.001
Morales-de la Pena, M., Elez-Martinez, P., and Martin-Belloso, O. (2011). Food preservation by pulsed electric fields: an engineering perspective. Food Eng. Rev. 3, 94–107. doi: 10.1007/s12393-011-9035-7
Nabilah, U. U., Sitanggang, A. B., Dewanti-Hariyadi, R., Sugiarto, A. T., and Purnomo, E. H. (2022). Meta-analysis: microbial inactivation in milk using pulsed electric field. Int. J. Food Sci. Technol. 57, 5750–5763. doi: 10.1111/ijfs.15942
Napotnik, T. B., Rebersek, M., Vernier, P. T., Mali, B., and Miklavcic, D. (2016). Effects of high voltage nanosecond electric pulses on eukaryotic cells (in vitro): a systematic review. Bioelectrochemistry 110, 1–12. doi: 10.1016/j.bioelechem.2016.02.011
Negi, A., Nimbkar, S., Thirukumaran, R., Moses, J. A., and Sinija, V. R. (2024). Impact of thermal and nonthermal process intensification techniques on yield and quality of virgin coconut oil. Food Chem. 434:137415. doi: 10.1016/j.foodchem.2023.137415
Ortega-Rivas, E., and Salmeron-Ochoa, I. (2014). Nonthermal food processing alternatives and their effects on taste and flavor compounds of beverages. Crit. Rev. Food Sci. Nutr. 54, 190–207. doi: 10.1080/10408398.2011.579362
Pan, Y. Y., Sun, D. W., and Han, Z. (2017). Applications of electromagnetic fields for nonthermal inactivation of microorganisms in foods: an overview. Trends Food Sci. Technol. 64, 13–22. doi: 10.1016/j.tifs.2017.02.014
Peng, Y., Liu, T., Gong, H. F., and Zhang, X. M. (2016). Review of the dynamics of coalescence and Demulsification by high-voltage pulsed electric fields. Int. J. Chem. Eng. 2016, 1–8. doi: 10.1155/2016/2492453
Pereira, R. N., Galindo, F. G., Vicente, A. A., and Dejmek, P. (2009). Effects of pulsed electric field on the viscoelastic properties of potato tissue. Food Biophys 4, 229–239. doi: 10.1007/s11483-009-9120-0
Perveen, R., Suleria, H. A. R., Anjum, F. M., Butt, M. S., Pasha, I., and Ahmad, S. (2015). Tomato (Solanum lycopersicum) carotenoids and Lycopenes chemistry; metabolism, absorption, nutrition, and allied health claims-a comprehensive review. Crit. Rev. Food Sci. Nutr. 55, 919–929. doi: 10.1080/10408398.2012.657809
Piironen, V., Lindsay, D. G., Miettinen, T. A., Toivo, J., and Lampi, A. M. (2000). Plant sterols: biosynthesis, biological function and their importance to human nutrition. J. Sci. Food Agric. 80, 939–966. doi: 10.1002/(SICI)1097-0010(20000515)80:7<939::AID-JSFA644>3.0.CO;2-C
Pimentel, T. C., Cruz, A. G., Pereira, E., da Costa, W. K. A., Rocha, R. D., Pedrosa, G. T. D., et al. (2023). Postbiotics: an overview of concepts, inactivation technologies, health effects, and driver trends. Trends Food Sci. Technol. 138, 199–214. doi: 10.1016/j.tifs.2023.06.009
Pipliya, S., Kumar, S., and Srivastav, P. P. (2023). Effect of dielectric barrier discharge nonthermal plasma treatment on physicochemical, nutritional, and phytochemical quality attributes of pineapple [Ananas comosus (L.)] juice. J. Food Sci. 88, 4403–4423. doi: 10.1111/1750-3841.16767
Preetha, P., Pandiselvam, R., Varadharaju, N., Kennedy, Z. J., Balakrishnan, M., and Kothakota, A. (2021). Effect of pulsed light treatment on inactivation kinetics of Escherichia coli (MTCC 433) in fruit juices. Food Control 121:107547. doi: 10.1016/j.foodcont.2020.107547
Preetha, P., Varadharaju, N., Jeevarathinam, G., Deepa, J., Kumar, A. P. M., Balakrishnan, M., et al. (2023). Optimization of continuous flow pulsed light system process parameters for microbial inactivation in tender coconut water, pineapple and orange juice. J. Food Process Eng. 46:14254. doi: 10.1111/jfpe.14254
Qi, M. Y., Liu, Y., Shi, S. S., Xian, Y. H., Liu, Q. Y., Yan, H. Y., et al. (2022). Inhibition mechanism of high voltage prick electrostatic field (HVPEF) on Staphylococcus aureus through ROS-mediated oxidative stress. LWT-Food Sci Technol 155:112990. doi: 10.1016/j.lwt.2021.112990
Qin, B. L., Pothakamury, U. R., BarbosaCanovas, G. V., and Swanson, B. G. (1996). Nonthermal pasteurization of liquid foods using high-intensity pulsed electric fields. Crit. Rev. Food Sci. Nutr. 36, 603–627. doi: 10.1080/10408399609527741
Rahaman, A., Siddeeg, A., Manzoor, M. F., Zeng, X. A., Ali, S., Baloch, Z., et al. (2019). Impact of pulsed electric field treatment on drying kinetics, mass transfer, colour parameters and microstructure of plum. J Food Sci Technol 56, 2670–2678. doi: 10.1007/s13197-019-03755-0
Rahaman, A., Zeng, X. A., Farooq, M. A., Kumari, A., Murtaza, M. A., Ahmad, N., et al. (2020). Effect of pulsed electric fields processing on physiochemical properties and bioactive compounds of apricot juice. J. Food Process Eng. 43:13449. doi: 10.1111/jfpe.13449
Rezaeinezhad, A., Eslami, P., Afrasiabpour, G., Mirmiranpour, H., and Ghomi, H. (2022). Effect of pulsed electric field on diabetes-induced glycated enzyme, oxidative stress, and inflammatory markers in vitro and in vivo. J. Phys. D Appl. Phys. 55:015401. doi: 10.1088/1361-6463/ac2530
Sanchez-Moreno, C., De Ancos, B., Plaza, L., Elez-Martinez, P., and Cano, M. P. (2009). Nutritional approaches and health-related properties of plant foods processed by high pressure and pulsed electric fields. Crit. Rev. Food Sci. Nutr. 49, 552–576. doi: 10.1080/10408390802145526
Sierra, S., and McComb, P. E. (2008). Hysterosalpingography in the investigation of women requesting reversal of sterilization—should it play a role? J. Reprod. Med. 53, 20–24.
Skowron, M., and Wantuch, A. (2020). New food preservation technologies—model research. Przeglad Elektrotechniczny 96, 125–128.
Soliva-Fortuny, R., Balasa, A., Knorr, D., and Martin-Belloso, O. (2009). Effects of pulsed electric fields on bioactive compounds in foods: a review. Trends Food Sci. Technol. 20, 544–556. doi: 10.1016/j.tifs.2009.07.003
Tafti, A. G., Peighambardoust, S. H., Hesari, J., Bahrami, A., and Bonab, E. S. (2013). Physico-chemical and functional properties of spray-dried sourdough in breadmaking. Food Sci. Technol. Int. 19, 271–278. doi: 10.1177/1082013212452415
Takatsuji, Y., Ishikawa, S., and Haruyama, T. (2017). Efficient sterilization using reactive oxygen species generated by a radical vapor reactor. Process Biochem. 54, 140–143. doi: 10.1016/j.procbio.2017.01.002
Thorgersen, M. P., and Downs, D. M. (2009). Oxidative stress and disruption of labile iron generate specific auxotrophic requirements in Salmonella enterica. Microbiology 155, 295–304. doi: 10.1099/mic.0.020727-0
Toepfl, S., Heinz, V., and Knorr, D. (2007). High intensity pulsed electric fields applied for food preservation. Chem Engineer Process Process Intensif 46, 537–546. doi: 10.1016/j.cep.2006.07.011
Torres-Contreras, A. M., Nair, V., Cisneros-Zevallos, L., and Jacobo-Velazquez, D. A. (2014a). Plants as biofactories: stress-induced production of chlorogenic acid isomers in potato tubers as affected by wounding intensity and storage time. Ind. Crop Prod. 62, 61–66. doi: 10.1016/j.indcrop.2014.08.018
Torres-Contreras, A. M., Nair, V., Cisneros-Zevallos, L., and Jacobo-Velazquez, D. A. (2014b). Effect of exogenous Amylolytic enzymes on the accumulation of Chlorogenic acid isomers in wounded potato tubers. J. Agric. Food Chem. 62, 7671–7675. doi: 10.1021/jf5026983
Trusinska, M., Drudi, F., Rybak, K., Tylewicz, U., and Nowacka, M. (2023). Effect of the pulsed electric field treatment on physical, chemical and structural changes of vacuum impregnated apple tissue in Aloe Vera juices. Food Secur. 12:3957. doi: 10.3390/foods12213957
Unlu, N. Z., Bohn, T., Francis, D., Clinton, S. K., and Schwartz, S. J. (2007). Carotenoid absorption in humans consuming tomato sauces obtained from tangerine or high-beta-carotene varieties of tomatoes. J. Agric. Food Chem. 55, 1597–1603. doi: 10.1021/jf062337b
Vallverdu-Queralt, A., Odriozola-Serrano, I., Oms-Oliu, G., Lamuela-Raventos, R. M., Elez-Martinez, P., and Martin-Belloso, O. (2013). Impact of high-intensity pulsed electric fields on carotenoids profile of tomato juice made of moderate-intensity pulsed electric field-treated tomatoes. Food Chem. 141, 3131–3138. doi: 10.1016/j.foodchem.2013.05.150
Vallverdu-Queralt, A., Oms-Oliu, G., Odriozola-Serrano, I., Lamuela-Raventos, R. M., Martin-Belloso, O., and Elez-Martinez, P. (2012). Effects of pulsed electric fields on the bioactive compound content and antioxidant capacity of tomato fruit. J. Agric. Food Chem. 60, 3126–3134. doi: 10.1021/jf205216m
Villarreal-Garcia, D., Nair, V., Cisneros-Zevallos, L., and Jacobo-Velazquez, D. A. (2016). Plants as biofactories: postharvest stress-induced accumulation of phenolic compounds and Glucosinolates in broccoli subjected to wounding stress and exogenous Phytohormones. Front. Plant Sci. 7:45. doi: 10.3389/fpls.2016.00045
von Wiren, N., Klair, S., Bansal, S., Briat, J. F., Khodr, H., Shioiri, T., et al. (1999). Nicotianamine chelates both Fe-III and Fe-II. Implications for metal transport in plants. Plant Physiol. 119, 1107–1114. doi: 10.1104/pp.119.3.1107
Vorobiev, E., and Lebovka, N. I. (2006). “Extraction of intercellular components by pulsed electric fields” in Pulsed electric fields Technology for the Food Industry: Fundamentals and applications. eds. J. Raso and V. Heinz, 153–193.
Wang, X. H., Fang, H. Q., Huang, Z. L., Shang, W., Hou, T. T., Cheng, A. W., et al. (2013). Imaging ROS signaling in cells and animals. J. Mol. Med. 91, 917–927. doi: 10.1007/s00109-013-1067-4
Wang, J., Wang, Y., Zhang, D., Ren, W. Y., and Yang, Z. X. (2022). Discovering the direct evidence of photocatalytic sterilization mechanism on bimetallic sulfides heterostructures. J. Colloid Interface Sci. 623, 182–195. doi: 10.1016/j.jcis.2022.05.019
Wang, Q., Yang, Y. Q., Li, Z., Jin, H. B., Shu, D. W., Jin, Y. G., et al. (2024). Research advances on the effects of thermal and non-thermal processing techniques on the physicochemical properties and microbiological control of liquid eggs. Food Control 155:110106. doi: 10.1016/j.foodcont.2023.110106
Watson, G. W., Beaver, L. M., Williams, D. E., Dashwood, R. H., and Ho, E. (2013). Phytochemicals from cruciferous vegetables, epigenetics, and prostate Cancer prevention. AAPS J. 15, 951–961. doi: 10.1208/s12248-013-9504-4
Wei, S., Chen, T., Hou, H. W., and Xu, Y. H. (2023). Recent advances in electrochemical sterilization. J. Electroanal. Chem. 937:117419. doi: 10.1016/j.jelechem.2023.117419
Wu, Y. C., Qin, S., Zang, Y. F., Zhou, M. Q., Chen, S., and Huang, S. H. (2023). Numerical study of the effects of pulsed electric field on β-casein. Innov. Food Sci. Emerg. Technol. 89:103484. doi: 10.1016/j.ifset.2023.103484
Xu, J. C., Zhang, M., Mujumdar, A. S., and Adhikari, B. (2017). Recent developments in smart freezing technology applied to fresh foods. Crit. Rev. Food Sci. Nutr. 57, 2835–2843. doi: 10.1080/10408398.2015.1074158
Ye, H., Huang, L. L., Chen, S. D., and Zhong, J. J. (2004). Pulsed electric field stimulates plant secondary metabolism in suspension cultures of Taxus chinensis. Biotechnol. Bioeng. 88, 788–795. doi: 10.1002/bit.20266
Yen, G. C., and Wei, Q. K. (1993). MYROSINASE activity and total GLUCOSINOLATE content of cruciferous vegetables, and some properties of cabbage MYROSINASE in TAIWAN. J. Sci. Food Agric. 61, 471–475. doi: 10.1002/jsfa.2740610415
Youseif, S. H., Abdel-Fatah, H. M. K., and Khalil, M. S. (2022). A new source of bacterial myrosinase isolated from endophytic Bacillus sp. NGB-B10, and its relevance in biological control activity. World J. Microbiol. Biotechnol. 38:215. doi: 10.1007/s11274-022-03385-3
Zandalinas, S. I., and Mittler, R. (2018). ROS-induced ROS release in plant and animal cells. Free Radic. Biol. Med. 122, 21–27. doi: 10.1016/j.freeradbiomed.2017.11.028
Zhang, L., Alfano, J. R., and Becker, D. F. (2015). Proline metabolism increases katG expression and oxidative stress resistance in Escherichia coli. J. Bacteriol. 197, 431–440. doi: 10.1128/JB.02282-14
Zhou, B., Huang, W., Feng, X., Liu, Q., Ibrahim, S. A., and Liu, Y. (2022). Identification and quantification of intact glucosinolates at different vegetative growth periods in Chinese cabbage cultivars by UHPLC-Q-TOF-MS. Food Chem. 393:133414. doi: 10.1016/j.foodchem.2022.133414
Zhu, B., Su, H., Fang, Z. H., Wu, G. Y., and Wei, X. L. (2023). Development of a high-voltage pulsed electric field sterilization power supply using a new topology circuit. Energies 16:2741. doi: 10.3390/en16062741
Zulueta, A., Barba, F. J., Esteve, M. J., and Frigola, A. (2013). Changes in quality and nutritional parameters During refrigerated storage of an Orange juice-Milk beverage treated by equivalent thermal and non-thermal processes for mild pasteurization. Food Bioproc. Tech. 6, 2018–2030. doi: 10.1007/s11947-012-0858-x
Keywords: non-thermal processing, nutrient enhancement, reactive oxygen species (ROS) generation, nutritional bioenhancement, microbial safety, non-thermal pasteurization alternatives
Citation: Su W, Wang Q, Li J, Qiu Z and Qiu Y (2024) Effects of pulsed electric field technology on the nutritional value and biological function of plant food. Front. Sustain. Food Syst. 8:1385533. doi: 10.3389/fsufs.2024.1385533
Edited by:
Qianwang Zheng, South China Agricultural University, ChinaReviewed by:
R. Pandiselvam, Central Plantation Crops Research Institute (ICAR), IndiaYanqun Xu, Zhejiang University, China
Copyright © 2024 Su, Wang, Li, Qiu and Qiu. This is an open-access article distributed under the terms of the Creative Commons Attribution License (CC BY). The use, distribution or reproduction in other forums is permitted, provided the original author(s) and the copyright owner(s) are credited and that the original publication in this journal is cited, in accordance with accepted academic practice. No use, distribution or reproduction is permitted which does not comply with these terms.
*Correspondence: Qianxinhong Wang, a2512607690@163.com; Jie Li, lijie@dlut.edu.cn; Zhidong Qiu, qzd_ccucm@163.com; Ye Qiu, ccyeqiu@163.com