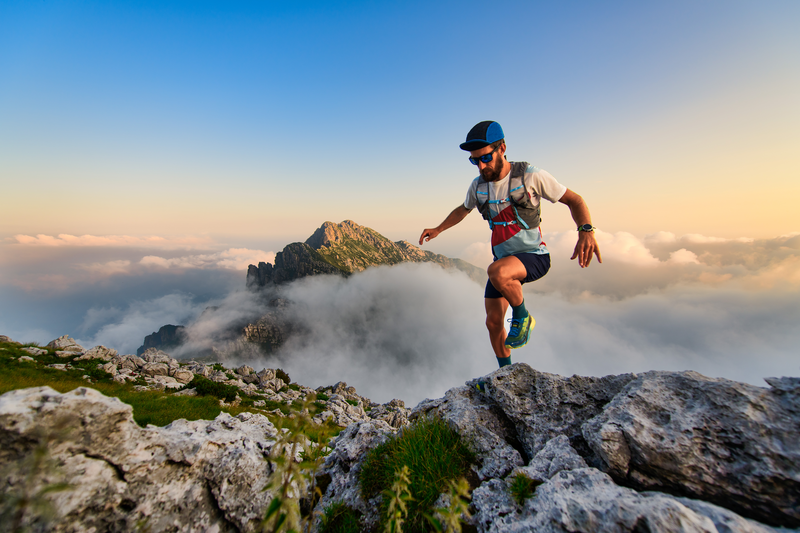
94% of researchers rate our articles as excellent or good
Learn more about the work of our research integrity team to safeguard the quality of each article we publish.
Find out more
REVIEW article
Front. Sustain. Food Syst. , 03 June 2024
Sec. Nutrition and Sustainable Diets
Volume 8 - 2024 | https://doi.org/10.3389/fsufs.2024.1385081
This article is part of the Research Topic Pushing The Frontiers Of Nutritional Life Cycle Assessment (nLCA) To Identify Globally Equitable And Sustainable Agri-Food Systems View all 15 articles
This review explores the significance of consuming edible insects, as well as their use in the food industry, agro-industry for animal husbandry, agricultural fertilizers and bio-pesticides, and pharmaceuticals. It emphasizes the increasing interest and relevance of this practice. The study starts by investigating the earliest evidence of anthropoentomophagy, which is the consumption of insects by humans, in the region. The review offers an overview of the consumption and utilization of insects in specific regions of the world, emphasizing their significance in various cultures and geographic areas. It also identifies the types of edible insects commonly consumed in Latin American countries, such as Mexico, and explains their preparation and consumption. Furthermore, the review assesses the nutritional value of edible insects, emphasizing their potential as a valuable source of protein, vitamins, and minerals. It also explores the various promising applications of insects, including their role in the food industry, animal husbandry, production of agricultural fertilizers and bioprotectants, and even their potential in the pharmaceutical sector. Finally, the article highlights the significance of entomophagy in Latin America by exploring its historical origins, nutritional benefits, and potential applications in various industries.
The demand for animal-based food and feed products is expected to increase significantly due to the projected global population growth, estimated to reach 9–11 billion by 2050. This will result in a significant increase of up to 70% in the demand for animal-based food protein to meet the dietary needs of the growing population (Varelas, 2019; Thornton et al., 2023). As the population continues to grow, there is an increasing need to explore sustainable and nutritious food sources (Hazarika and Kalita, 2023; Papastavropoulou et al., 2023). Insects play a critical role in meeting this demand as a sustainable and efficient food source. Compared to traditional protein sources, insects require fewer natural resources, such as water and land, for production. They also have a high feed conversion rate, efficiently converting consumed food into body mass, making them ideal for large-scale food production (Oonincx et al., 2015; Lange and Nakamura, 2023). Insects have long been a common part of the diet in many Asian, African, and Latin American cultures due to their nutritional content, providing a rich source of high-quality protein, essential amino acids, fiber, monounsaturated and polyunsaturated fats, as well as such as vitamins and minerals. Surprisingly, they offer a nutritional profile comparable to traditional animal protein sources such as beef or chicken (Dobermann et al., 2017; Orkusz, 2021; Khalifah et al., 2023). Insect consumption can also help address issues of malnutrition and nutritional deficiencies, especially in regions with limited food availability (Imathiu, 2020). There are several reasons why many people do not include insects in their usual diet, such as cultural factors, aversion to their appearance, association with pests (Sogari et al., 2022, 2023), unfamiliarity, stigmas and superstitions, and mainly ignorance about the advantages of its consumption (Yen, 2009; Tan et al., 2015; Hlongwane et al., 2020; Alhujaili et al., 2023). Nevertheless, there is a growing interest in consuming insects due to their potential as a sustainable food source. As awareness of their nutritional value and environmental benefits spreads, attitudes towards insects as a viable food option are gradually changing (Grabowski et al., 2022). Latin American countries have a rich cultural tradition of incorporating insects into their diet. This arises from their culinary heritage, where traditional techniques have been developed to prepare insects, considered a delicacy. The availability of a wide variety of insect species in both rural and urban areas contributes to their consumption, often including them in festivals and celebrations (Bermúdez-Serrano, 2020; Guiné et al., 2023; Tzompa-Sosa et al., 2023). The objective of this work is to highlight the usefulness and potential that insects represent, given their environmental, social, and health benefits.
Academic publications are increasing at an accelerating rate. As a result, it is becoming increasingly challenging to keep pace with and comprehend the current state of specific fields. Several scholars argue that literature reviews are essential for synthesizing the current state of specific fields. A structured bibliographic review is a traditional approach to analyzing and assessing the published scientific literature. This type of review provides an in-depth analysis of the literature content, as demonstrated by Rousseau (2012), Wang et al. (2019), and Ghadimi et al. (2019). A review of pertinent research articles was conducted by searching prominent academic databases, including SCOPUS, Web of Science (WOS), MDPI, and PubMed, among others. To ensure an unbiased search, synonyms for the consumption of the specified insects were included. These synonyms included “edible insects,” “entomophagy,” and “anthropo-entomophagy,” as well as the terms “protein sources” and “Latin America.” In SCOPUS, the search query was “edible insect” AND (“consumption” OR “meal”). In Web of Science (WOS), the search queries included all fields, titles, abstracts, and author keywords using the phrase “insect consumption.” The same search strategy was applied to all the databases utilized. Tables have been created to present information about the primary categories of edible insects in Mexico, the proximate nutrient composition, a comparison of proximate nutrient content within species of the same category, the fatty acid composition of specific edible insects, mineral content, a comparison of proximate nutrient content at different stages of development, and the antinutrient content of insect-based foods. Each table includes data on the distribution of species in the Americas and the Caribbean, supported by the Global Biodiversity Information System (GBIF, https://www.gbif.org/es/). Database searches were last conducted and reviewed for relevant literature on December 22, 2023.
Entomophagy, defined as the consumption of insects by humans, falls under the term anthropoentomophagy when insects are consumed as food or in products like honey and propolis (Costa-Neto and Ramos-Elorduy, 2006; Ramos-Elorduy, 2009; Dagevos and Taufik, 2023). Although early human entomophagy has received limited research attention due to preservation challenges, various studies employing tools, residues, DNA, coprolites, dental wear, stable isotopes, osteology, and cave paintings contribute valuable insights (McGrew, 2014). Evidence suggests that early hominids engaged in the search for and consumption of termites for nearly a million years during the Plio-Pleistocene period. Wear patterns on bone tools used by Paranthropus robustus to extract termites from mounds support this hypothesis (Backwell and d’Errico, 2001). Coprolite analysis in the United States indicates that 4,500 years ago, humans collected and consumed Melanoplus sanguinipes grasshoppers (Madsen and Kirkman, 1988). Chitinous insect exoskeletons have been found in coprolites of prehistoric humans in the United States, Mexico, and Peru (Reinhard and Bryant, 1992; Brothwell and Brothwell, 1998). Dental plaque studies on a 1.2 million-year-old hominid in northern Spain revealed microfossils of insect fragments (Hardy et al., 2016). Fossil studies in South Africa propose insect consumption as a potential explanation for high strontium/calcium levels in the dental enamel of the Australopithecus genus, existing 2 to 4 million years ago (Sponheimer et al., 2005). Insect consumption during periods of fruit scarcity may have influenced hominid intelligence evolution, providing minerals like iron and omega-3 fatty acids (Kyriacou, 2014; Melin et al., 2014).
Human insect consumption dates back to prehistoric times, evident in archaeological and anthropological findings across diverse cultures worldwide. In Latin America, countries like Mexico, Peru, Colombia, Venezuela, and Ecuador have a notable history of insect consumption, contributing to food security for local communities. Depending on the species and development stage, these insects are rich sources of proteins, fats, carbohydrates, and minerals (Costa-Neto, 2015). Insects, with their substantial biomass, have a historical association with human consumption, even being mentioned in sacred texts like the Bible and the Quran (Ramos-Elorduy and Viejo-Montesinos, 2007). Certain insect species, including cochineal insects, ants, and wasps, were cultivated long before the arrival of the Spanish to the American continent (Costa-Neto, 2015). In Aztec culture, insects were used as a tribute to emperors, and pre-Hispanic delicacies like escamoles (ant larvae) continue to be consumed as exotic dishes in Mexico. The maguey worm holds a prestigious place in Mexican gastronomy, featuring various dishes incorporating roasted, fried, or stewed insects with aromatic herbs (Ramos-Elorduy, 2004). Onore (1997) documented 83 edible species in Ecuador, and Costa-Neto and Ramos-Elorduy et al. (2006) noted 95 species used by 39 ethnic groups in Brazil (Costa-Neto, 2015). In Colombia and Venezuela, palm worms are prominently consumed, while in Peru, there is a tradition of consuming large black crickets in the Ayacucho region (Ramos-Elorduy and Viejo-Montesinos, 2007). Entomophagy in Brazil dates back to the 16th century, with indigenous peoples already consuming various insects during early European colonization. This practice has become ingrained in Brazilian culinary traditions (Costa-Neto and Ramos-Elorduy, 2006).
Insects can be consumed directly at different developmental stages or indirectly through insect-derived products like propolis, honey, pollen, wax, and royal jelly. Throughout history, non-stinging bee products, such as those used by the Mayan and Aztec civilizations, played a significant role in socioeconomic and religious activities. The Aztecs even used honey for trade with Spanish colonizers in the 16th century. Similarly, native communities in Brazil, Paraguay, Uganda, Madagascar, the Himalayas, and Australia have incorporated bee products into their traditions and cultures over time (Gupta et al., 2014; Cumo, 2015; Grüter, 2023). Grüter (2023) highlights the medicinal use of Lepidotrigona arcifera honey by Nepalese individuals in India and the therapeutic applications among Ugandan pygmies, who utilize it as a remedy for constipation. Calderón-Fallas et al. (2021) emphasize the sacred significance of bees, particularly the Mayan bee (Melipona beecheii), in spiritual, cosmological, and mythological contexts. Costa-Neto (2015) and Medeiros (2014) present an overview of edible insects in Latin America, with Mexico leading at 415 species (56.46% of the total) and Brazil following closely with 122 species (16.6% of the total). The diverse culinary traditions and entomophagy practices across Latin American countries contribute to a rich tapestry of cuisine, totaling 735 edible insects.
The modernization of bee-derived product marketing has led to meliponiculture, involving the breeding and care of bees from the Meliponini tribe (Álvarez, 2016; Cortes-Martínez et al., 2021). This practice, primarily carried out by indigenous cultures and producers in the Neotropics, focuses on species such as M. beecheii, M. eburnea, M. quadrifasciata, M. scutellaris, and Tetragonisca angustula (Jaffé et al., 2015; Quezada-Euán et al., 2018; Quezada-Euán and Alves, 2020). Meliponiculture, a valuable biocultural heritage, has been consistently practiced for approximately 2000 years, particularly with M. beecheii in Mesoamerica (Nates-Parra and Rosso-Londoño, 2013; Grüter, 2023). In traditional medicine, products derived from stingless bees, particularly T. angustula, are employed for treating skin and eye diseases. These products have also shown effectiveness in addressing respiratory and digestive ailments, attributed to the antibiotic properties of hydrogen peroxide and gluconic acid present in honey. Additionally, honey is recognized as a natural food source that may help prevent certain types of cancer associated with oxidative stress on physiological cells in humans (Kumul et al., 2015). Stingless bee honey, along with honey from Apis mellifera, plays a role in the preparation of alcoholic beverages. Pollen derived from these bees is occasionally used as a protein supplement in food. Moreover, in Mexico, Brazil, Ecuador, and Paraguay, bee larvae and pupae are consumed as sources of protein and vitamins (Grüter, 2023). Apicultural products and alcoholic beverages made from honey have gained popularity in Latin American markets, valued as artisanal products that offer natural and healthy nourishment.
Latin America holds the second-highest market value for edible insects globally, reaching $92.2 million, with expectations of nearly tripling to $250.6 million by 2030. This projection, close to the estimated European market value of $261.5 million, highlights the region’s attractiveness to both local and international traders, with Mexico particularly standing out. Mexico’s market value was reported at $26 million in 2018, with an 18% annual growth rate, projected to reach $59 million by 2023. North America, especially the United States, is also experiencing growth, making it an intriguing market for Hispanic entrepreneurs (Research and Markets, 2018; Bermúdez-Serrano, 2020; Guiné et al., 2021). Insects offer a wide range of benefits in various areas, including food, medicine, spiritual and religious rituals, cosmology, mythology, art, economics, and culture. These diverse uses have contributed to the continued use and consumption of insects by indigenous and local communities over the years (Costa-Neto, 2015; Van Huis et al., 2022). In addition, certain insects used as aphrodisiacs have influenced people from various cultures (Costa-Neto and Ramos-Elorduy, 2006). Omuse et al. (2024) compiled a comprehensive list of 2,205 identified species of edible insects. Beetles are the largest category of edible insects, comprising 468 species. Hymenoptera ranks second with 351 species, followed by Orthoptera with 267 species and Lepidoptera with 253 species (Costa-Neto and Ramos-Elorduy, 2006). According to Jongema (2017), the majority of these edible insect species are concentrated in tropical countries. These edible insects can be categorized as follows: beetles (31%), caterpillars (17%), ants, bees, and wasps (15%), grasshoppers (13%), bugs (11%), dragonflies (3%), termites (3%), cockroaches (2%), spiders (1%), and other unspecified species (2%).
In Latin American countries, the consumption of insects is influenced by both the accessibility of these food sources and their cultural significance. Insects are commonly prepared using various methods such as frying, roasting, or as ingredients in traditional dishes. Beyond their nutritional benefits, entomophagy may hold cultural and symbolic importance within specific communities (Ong’Or et al., 2024). For example, when considering experiences in other parts of the world, such as Africa, a wide variety of insects are consumed, including termites, caterpillars, grasshoppers, and crickets. These insects are collected from the wild or reared on a small scale for consumption (Womeni et al., 2009; Pal and Roy, 2014; Kipkoech et al., 2023). In Asia, especially in countries like Thailand, Cambodia, and Laos, edible insects are considered a culinary delicacy. Some popular insect species include silkworms, beetles, bees, and ants. In addition to being part of the local diet, insects have also become tourist attractions, as visitors can sample various dishes prepared with insects (Hanboonsong et al., 2013; Durst and Hanboonsong, 2015; Krongdang et al., 2023). In Europe and North America, although the consumption of insects is not yet widespread, it has gained popularity in recent years. Insect-based products can be found in specialty stores, such as cricket flour for making bread or energy bars containing beetle larvae (Reverberi, 2021; Skotnicka et al., 2021). Currently, edible insects serve as a nutrient-rich food source in many parts of the world, and their consumption is gaining acceptance and popularity due to their sustainability and nutritional value.
In Mexico, 415 species of insects have been documented as being consumed by various ethnic groups throughout the country (Ramos-Elorduy et al., 2003; Ramos-Elorduy and Pino, 2005). Of the total, 83% of these insects are terrestrial, while 17% come from continental aquatic ecosystems. Furthermore, it has been observed that 55.8% of these species are consumed in their immature stages, such as eggs, larvae, pupae, and nymphs, while 44.2% are consumed in their adult state. It is important to note that certain species are consumed at any stage of their development (Costa-Neto and Ramos-Elorduy, 2006). There are species with esteemed reputations and flavors that are highly valued in national and international markets. However, their exploitation is unregulated, which can have environmental consequences (Ramos-Elorduy et al., 2003 and Table 1). Currently, the consumption of insects has evolved from a local or regional practice to a significant commercial and agro-industrial phenomenon (Montalbán et al., 2022). For example, Black soldier fly (BSF) and mealworm larvae are commercially available for feeding ornamental fish in the market (Thrastardottir et al., 2021).
Table 1. Main groups of edible insects in Mexico and their distribution in Latin America and the Caribbean.
In Latin America, the potential uses of edible insects represent a unique opportunity to address several pressing issues, such as poverty eradication, food sovereignty, and sustainable development (Dossey et al., 2016). By embracing this innovative and culturally relevant food source, the region can create a competitive chain that not only improves livelihoods but also contributes to a more resilient and equitable food system. Establishing a competitive edible insect supply chain can create income opportunities, particularly in rural areas where poverty rates are high (Bermúdez-Serrano, 2020). Small-scale insect farming can be relatively inexpensive to start and maintain, offering a source of income for marginalized communities. However, this requires the support of local governments, which have a crucial role to play in promoting sustainable production and consumption of edible insects through supportive policies and regulations. This includes incentivizing insect farmers, investing in research and infrastructure, and raising awareness of the nutritional and environmental benefits of insect-based diets (Stull and Patz, 2020). Investing in research and innovation related to edible insects can lead to the development of new products and technologies, thereby enhancing the competitiveness of the insect value chain. This includes exploring alternative uses such as animal feed, pharmaceuticals, and sustainable packaging materials (Melgar-Lalanne et al., 2019). As global interest in sustainable and alternative protein sources grows, Latin America has the opportunity to position itself as a leader in the edible insects market. By capitalizing on its biodiversity and rich culinary traditions, the region can attract both domestic and international consumers.
From a nutritional standpoint, edible insects are a significant source of protein, fat, minerals, and fiber. However, the nutritional value of insects can vary depending on their habitat, the insect’s diet, the edible stage of development (egg, larva, nymph, or adult), sex, and the type of processing they undergo, such as being consumed whole (dehydrated, boiled, roasted, fried, etc.). In addition, the storage of edible insects directly affects the content and availability of nutrients due to potential changes in the physicochemical properties of proteins and lipids (Cruz, 2017; Kulma et al., 2019; Cerisuelo, 2021). Not only is the quantity of proteins present in edible insects important, but also the quality of these proteins, depends on the amount of amino acids they contain. Edible insects can offer a range of essential amino acids, serving as a crucial supplement to address amino acid deficiencies in local staple foods. The orders Lepidoptera, Orthoptera, Coleoptera, and Diptera are characterized by high levels of glutamic and aspartic acid, phenylalanine, and alanine (Avendaño et al., 2020). On the other hand, the suborder Heteroptera (Hemiptera) is characterized by its high levels of proline, leucine, tyrosine, alanine, valine, and methionine. The percentage of protein in insects is expressed on a dry weight basis. Accordingly, the percentage of Coleoptera ranges from 20 to 71%, Diptera from 35 to 70%, Ephemeroptera from 37 to 68%, Hymenoptera from 10 to 81%, Lepidoptera from 13 to 78%, the suborders Sternorrhyncha and Archaeorrhyncha (Hemiptera) from 33 to 72%, Heteroptera from 36 to 71%, and Orthoptera from 27 to 77% (Ramos-Elorduy, 2004; Avendaño et al., 2020). Conventional foods have a lower protein content compared to insects. For example, eggs from birds, chicken, and pork typically contain protein amounts ranging from 68.9 to 75% of dry weight, with beef and fish being exceptions with a higher range (Ramos-Elorduy, 2004; Lizhang et al., 2008). On the other hand, insects also contain significant amounts of healthy unsaturated fats and essential fatty acids, which provide the necessary energy for protein assimilation (Ramos-Elorduy, 2004; Glover and Sexton, 2015).
In general, the fat content of insects ranges from 10 to 40% of dry weight, reaching 50% in Coleoptera and 77% in Lepidoptera (Lizhang et al., 2008; Van-Huis et al., 2021). According to Lizhang et al. (2008), in certain insect orders, the protein content tends to be higher than the fat content, being approximately twice as high. Insects with high protein content include Coleoptera, Lepidoptera, and Heteroptera (Hemiptera), followed by Sternorrhyncha and Archaeorrhyncha (Hemiptera), Hymenoptera, Diptera, and Orthoptera. Notably, there is a negative correlation between protein and fat content (Lizhang et al., 2008). Insects typically contain significant amounts of essential micronutrients, including copper, iron, magnesium, manganese, phosphorus, selenium, and zinc. They also provide smaller amounts of potassium and calcium. Some insects are a valuable source of specific vitamins, including A, C, D, E, K, and the B-complex (B1, B2, B3, B5, B6, B12, H) (DeFoliart, 1989; Ramos-Elorduy, 2004; Lizhang et al., 2008; Van-Huis, 2013; Van-Huis et al., 2021). However, despite the enormous potential of insects as a nutritious food (Kowalski et al., 2022), some people may experience allergic reactions to insect proteins. Allergic sensitivity can develop from prolonged exposure to insects and has been documented by entomologists. It is believed that individuals with pre-existing shellfish allergies may also experience cross-reactivity with insects, as crickets and shrimp are relatively close relatives. However, it is important to note that cross-reactivity is not inevitable (Glover and Sexton, 2015). On the other hand, it has been suggested that childhood exposure to chitin, the primary substance that forms the exoskeleton of insects, may enhance the immune system’s response to intestinal parasitic infections and reduce certain allergic conditions (Van-Huis, 2013). It is important to note that there is considerable variation in the nutritional composition of insect species depending on factors such as harvest location, processing methods, insect life stage, rearing techniques, and insect feed. Based on the available data (on a dry weight basis), it is suggested that specific treatments can enhance the nutritional content, aroma, appearance, and taste of edible insects. However, it is important to consider additional factors that may affect the content and composition of insects. The factors responsible for the nutritional content and quality of edible insects are not well understood. These factors include the chemical composition of insects, their handling and storage practices, microbial contamination, insect diet, feeding time, host plants, and phytonutrient content (Imathiu, 2020; Stull and Weir, 2023).
The following tables present comprehensive information on the nutrient composition of various insect orders, as documented in a study by Meyer-Rochow et al. (2021). Table 2 from Meyer-Rochow et al. illustrates the proximate nutrient composition of edible insects per 100 grams of dry matter. In Table 3, you will find a comparative analysis of the nutrient content among different species within the same genus, also presented per 100 grams of dry matter. Table 4 presents the amino acid composition of various species within the same genus. The fatty acid composition of various edible insects is presented in Table 5, while Table 6 displays the mineral content of selected edible insects, measured in milligrams per 100 grams. Table 7 presents a comparative overview of the nutrient content at various developmental stages of edible insects, per 100 grams of dry matter. Finally, Table 8 presents information about the anti-nutrient content of insect-based foods, expressed in milligrams per 100 grams. Table 9 shows the elemental composition of insect excrements and organic fertilizers. The distribution in Latin America, the Americas, and the Caribbean are given in all tables.
Table 2. Proximate nutrient composition of edible insects (g/100 g dry matter basis) and their distribution in Latin America and the Caribbean.
Table 3. A comparative account of the proximate nutrient content of different species belonging to the same genus (g/100 g dry matter basis) and their distribution in Latin America and the Caribbean.
Table 4. Amino acid composition of different species belonging to the same genus and their distribution in Latin America and the Caribbean.
Table 5. Fatty acid composition of selected edible insects and their distribution in Latin America and the Caribbean.
Table 6. Minerals content of selected edible insects (mg/100 g) and their distribution in Latin America and the Caribbean.
Table 7. A comparative account of the proximate nutrient content of different developmental stages of edible insects (g/100 g dry matter basis).
Table 8. Antinutrient content in Insect-based Foods (mg/100 g) and their distribution in Latin America and the Caribbean.
Traditionally consumed in various Latin American countries, edible insects face potential barriers in Western countries, where they may be perceived as unsafe and unappetizing (Baiano, 2020; Kim et al., 2021; Van-Huis et al., 2021). Overcoming such biases is crucial for promoting insect-based economies in Latin America, emphasizing the significance of insect processing technologies (de Castro et al., 2018; Kim et al., 2021; Van-Huis et al., 2021). Given the rising global demand for protein, which is projected to grow by 9.1% from 2020 to 2027, and the necessity for sustainable protein sources in contrast to traditional livestock-based supply chains, insect processing technologies are anticipated to have a dominant role in the future (da Costa-Rocha et al., 2021; Van-Huis et al., 2021; Munialo et al., 2022). The global market for insect-based products is expected to grow significantly, from $406 million in 2018 to an estimated $1.18 billion in 2023 (Gkinali et al., 2022; Munialo et al., 2022). This trend represents a significant opportunity for Latin American countries to participate in the growing global market (Kouřimská and Adámková, 2016). In particular, several commercial brands such as Gricha®, Griyum®, In Insect Nutri-tion®, and CrickEx® offer a variety of insect-based food products produced in Mexican insect farms. These products are currently available online to Latin American consumers (Cordoba-Aguilar et al., 2023).
Recent research in the field of edible insects has embraced a biorefinery approach, aiming to maximize the value of the three main fractions obtained from insects: proteins, lipids, and chitin, as well as other valuable products derived from insect biomass within the same processing chain (Caligliani et al., 2018; da Costa-Rocha et al., 2021). New methodologies and techniques are essential for achieving optimal yields, quality, and functional properties of chemical compounds from insect biomass. The selection of techniques and processing steps directly impacts the quality, content, functional properties, palatability, and biosafety of insect extracts (de Castro et al., 2018; Ojha et al., 2021; Queiroz et al., 2023; Rahman et al., 2023). Various methods have been explored to achieve these goals, including nitrogen freeze-drying, vacuum drying, supercritical CO2 extraction, ultrasound, electric pulse field, high hydrostatic pressure, and ohmic heating (Queiroz et al., 2023; Rahman et al., 2023). The development of new technologies for processing insect biomass is crucial for enhancing the technological and functional properties of insect proteins. These technologies aim to optimize solubility, water and oil retention capacity, emulsifying and foaming ability, and gelling capacity, while ensuring the safety and nutritional value of the products (Van-Huis et al., 2021). While the initial investment in new technologies is substantial, they have demonstrated their value in addressing the challenges of processing industrial insect biomass. These technologies have demonstrated the ability to preserve the essential bioactive properties of insect-derived molecules, reduce the allergenicity of insect proteins, and increase the stability of reaction products (Mintah et al., 2019; Ojha et al., 2021).
In agro-industrial applications, the black soldier fly (BSF) Hermetia illucens and other insect species, such as the house fly and Tenebrio molitor (TM), are widely used as valuable sources of meal for animal feed due to their high protein content (Hall et al., 2018; Sánchez et al., 2021). Tenebrio molitor larvae have also been utilized as animal feed because of their high protein and essential amino acid content. These larvae are rich in saturated, polyunsaturated, and monounsaturated fatty acids, as well as minerals, iron, and zinc making them a viable option for poultry feed. They have high nutrient availability for chickens and exhibit angiotensin-converting enzyme inhibitory activity, effectively stabilizing blood pressure (Dalmoro et al., 2021; Nascimento-Filho et al., 2021). Dietary treatments with BSF larvae and TM were found to beneficially reduce total blood cholesterol levels while increasing phosphorus levels in turkeys fed this protein source (Kozłowski et al., 2021). The meal derived from house fly larvae, with a protein content of 54% and a lipid content of 22%, is suitable for human consumption due to its favorable microbiological activity. It is rich in essential amino acids and unsaturated fatty acids, making it a promising source of protein for the diet of broiler chickens (Hall et al., 2018; Sánchez et al., 2021). Another case is the larval biomass of BSF contains 40% protein and 30% fat, making it suitable as a highly nutritious fish feed and a potential substitute for soy and maize in poultry diets (Park, 2016). Studies have shown that quail and broiler chickens fed BSF larvae have increased concentrations of amino acids and fatty acids in their meat composition (Cullere et al., 2016, 2018). BSF larvae are globally recognized as high-quality animal feed and have been deemed safe for human and animal consumption by the Food and Agriculture Organization of the United Nations (FAO) [Wang and Shelomi, 2017; Association of American Feed Control Officials (AAFCO), 2023].
In the global marketplace, BSF larvae have become a popular choice for various animals in the agricultural industry. They are available in a variety of forms and packaging options. Dehydrated BSF larvae are tightly sealed in high-density polyethylene packaging specifically designed for poultry and ornamental fish. In addition, fat-free cakes made from BSF larvae, packaged in the same sealed polyethylene, are designed for smaller animals such as pigs and rabbits. Live or dehydrated black soldier fly pupae are also available (Wanjiku, 2018; Cullere et al., 2019).
BSF larval cakes have a protein profile similar to soy, with elevated levels of essential amino acids, making them an excellent source of protein for high-protein food markets (Patterson et al., 2021). Furthermore, black soldier fly (BSF) larvae can be processed into a high-quality, protein-rich meal that can serve as a substitute for concentrated feed in poultry and ornamental fish. The flour is also used to make treats for exotic pets, wildlife rehabilitators, and urban farmers (Bußler et al., 2016; Queiroz et al., 2021). Due to the nutritional value of the larval protein, it is possible to replace up to 25% of fish meal and 38% of fish oil in balanced animal diets with BSF larvae, providing a sustainable alternative (Xiao et al., 2018). In addition to being globally accessible, these BSF larvae products are specifically designed for urban and rural communities involved in poultry and ornamental fish farming. Insect farming and promoting environmental education contribute to converting organic materials into valuable resources. During this process, larval or pre-pupal insect biomass is generated on a small to medium scale for direct consumption or processing into feed for poultry, fish, and pig farming. This approach promotes the adoption of sustainable agro-industrial production methods and encourages ecological innovation and the use of technological tools (Wu et al., 2022).
Insect farming residues, such as frass (a mixture of insect excreta, exuvia, and undigested residues) and cadavers, can play a crucial role in developing a circular economy management strategy for both the food industry and agro-industrial applications. By utilizing these residues in sustainable agriculture, particularly as alternatives to chemical fertilizers and pesticides, additional income can be generated (Fielding et al., 2013; Chavez and Uchanski, 2021; Poveda, 2021). This approach holds particular significance for Latin American countries where agricultural practices often align with subsistence agriculture because the use of residual biomass from insect farming can reduce economic costs associated with acquiring chemical fertilizers. The research on using insect farming byproducts as organic fertilizers are still limited (Khan et al., 2016; Poveda et al., 2019; Beesigamukama et al., 2022; Wantulla et al., 2023), existing evidence indicates the potential impact of insect frass and cadaver deposition on soil nutrient cycling processes. Ecological studies have demonstrated that frass from certain herbivorous insects, rich in nitrogen and labile carbon, promotes microbial growth, accelerates organic matter decomposition, and affects carbon and nitrogen mineralization and immobilization. The nitrogen content in insect frass may vary among different species, emphasizing the need to evaluate frass quality across different insect species (Kagata and Ohgushi, 2012b).
Recent research has focused on assessing the elemental composition of insect-produced frass, including nitrogen (N), phosphorus (P), potassium (K), sulfur (S), calcium (Ca), magnesium (Mg), manganese (Mn), zinc (Zn), and other elements. Comparative analyses reveal that frass from various insect species contains concentrations of essential macronutrients (N, P, K), secondary macronutrients (Mg, Ca, S), and micronutrients (Mn, Fe, Cu, Zn, B) comparable or even higher than those found in commonly used organic fertilizers in agriculture, such as manures, composts, and agricultural by-products. However, the elemental composition of frass from various insect species needs further exploration due to the wide range of variations in nutritional quality (Frost and Hunter, 2008; Hillstrom et al., 2010; Kagata and Ohgushi, 2011, 2012a,b; Fielding et al., 2013). The elemental composition of frass from various edible insects shows a balanced ratio of primary macronutrients (N:P:K) at 2:1:2. Different groups of insects, such as coleopterans and termites, exhibit nitrogen-enriched ratios (5,1:2), while orthopterans and dipterans display potassium-enriched ratios (6,1,15 and 1:1:3, respectively). Coleopterans and Lepidopterans exhibit nitrogen-to-potassium enriched ratios of 14:7 and 10:8, respectively. This variation in elemental composition is closely related to the diet of insects (Fielding et al., 2013; Zhang et al., 2014; Poveda et al., 2019). By managing the nutritional quality of the food given to insects in agricultural practices, it is possible to adjust the proportions of macronutrients in insect waste (frass) to meet specific requirements for fertilizer production (Poveda et al., 2019). While altering insect diets to enhance the quality of their excrement (frass) is a viable strategy, additional research is necessary to evaluate its feasibility. In addition, given that the nitrogen and phosphorus content of insect bodies is nearly ten times higher than that of insect frass, utilizing carcasses produced during insect farming offers another opportunity to achieve the desired adjustments in frass composition (Elser et al., 2000).
Insects like the black soldier fly larvae are commonly utilized for organic waste decomposition, as they serve as efficient decomposers and biological controllers of other fly species during their larval stage. Black soldier fly larvae can reduce organic waste by approximately 65 to 78%, producing a valuable material for composting and agricultural fertilization. This approach is proving to be more efficient than traditional composting and vermiculture, which require longer processing times. The resulting humus is of exceptional quality and serves as an environmentally friendly fertilizer for a variety of indoor and outdoor crops, such as those found in gardens, parks, golf courses, and sports fields (Erickson et al., 2004). Controlled trials on crops such as lettuce, Swiss chard, basil, tomato, onion, barley, and corn using black soldier fly (BSF) and mealworm wastes as fertilizers have shown positive effects on plant characteristics, including increased fresh and dry weight, height, basal stem width, and leaf number (Buenrostro et al., 2000; Singh and Kumari, 2019; Chavez and Uchanski, 2021).
In addition to serving as an organic fertilizer, insect frass also demonstrates bioprotective and biostimulant properties in agriculture. These properties are likely due to the microorganisms present in the frass, which stimulate beneficial soil microorganisms that enhance various plant responses. These responses include enhanced growth, increased tolerance to abiotic stresses, and activation of systemic defense mechanisms against natural pests. The microorganisms, referred to as plant growth-promoting microorganisms, play a crucial role in improving plant growth and productivity through various activities such as synthesizing hormones, solubilizing phosphate and potassium, fixing nitrogen, and producing enzymes like glucanases, chitinases, and ACC (1-aminocyclopropane-1-carboxylate) deaminases, among others (Poveda et al., 2019; Barragán-Fonseca et al., 2022).
Tenebrio molitor frass was found to contain a diverse microbial community, including over 4,700 bacterial and 1,200 fungal strains, many of which were identified as plant growth-promoting microbes. The removal of these microbial strains from TM frass resulted in reduced plant growth and yield in fertilization experiments (Poveda et al., 2019). Studies have shown that using insect frass in fertilization experiments can activate plant defenses against pathogens and pests, leading to improved overall plant health. Root-colonizing microbes, such as beneficial rhizobacteria like Bacillus, Pseudomonas, and Serratia, can trigger systemic resistance in plants and bolster defense mechanisms against potential pathogen or insect attacks (Pineda et al., 2013; Ray et al., 2015, 2016; Chavez and Uchanski, 2021).
The bioprotective effect of insect frass on plants is attributed to specific chemical compounds, known as effectors or elicitors, present in the frass. Chitin and chitosan, derived from the exoskeletons of insects, are considered potent elicitors that mimic compounds to which plants respond when attacked by pathogens containing chitin. These compounds elicit various plant responses, including the expression of defense-related genes, activation of jasmonate hormones, production of phytoalexins, phenolics, terpenes, and reactive oxygen species, and cellular changes such as cytoplasmic acidification, deposition of callose and lignin, and cell death (Sharp, 2013; Barragán-Fonseca et al., 2022). The positive effects of using frass on plant health emphasize its potential for controlling plant pathogens and pests. However, further studies are needed to determine the minimum effective dose of insect frass to stimulate plant defense responses and whether these responses vary among frass from different insect species (Poveda, 2021; Barragán-Fonseca et al., 2022; Lopes et al., 2022; Wantulla et al., 2023).
Insects are valuable sources of chemical compounds with significant pharmaceutical applications. Alloferons, which are peptides extracted from bacteria-infected Calliphora vicina fly maggots, such as alloferon-1, have been found to stimulate natural killer cell activity and interferon synthesis. They also exhibit antitumor and antiviral properties. Alloferon-1 has been implicated in regulating acute and chronic inflammatory responses in various diseases, such as skin and corneal epithelial cells, rheumatoid arthritis, and asthma (Ryu et al., 2008; Zhang et al., 2014; Jo et al., 2022; Lee et al., 2023).
Cantharidin, a toxic compound extracted from blister beetles such as Mylabris phalerata and M. cichorii, has demonstrated promising antitumor effects by inhibiting the proteins phosphatase 1 (PP1) and phosphatase 2A (PP2A). It has the potential to treat various cancers, including bladder, colon, pancreatic, liver, breast, oral, and leukemia (Naz et al., 2020). Melittin, a peptide extracted from bee venom, has been shown to possess antitumor properties. Comprising 26 amino acids, melittin induces the creation of pores in lipid membranes, resulting in cell disruption and potential antitumor effects. However, its clinical application is limited due to significant hemolytic activity (Wang et al., 2022). Sericin, produced by silkworm larvae, offers several health benefits due to its composition of 18 amino acids, including eight that are essential for human metabolic processes. It has therapeutic properties such as accelerating wound healing, reducing blood pressure, protecting the nervous system, exhibiting anti-tumor activity, controlling blood sugar, reducing wrinkles, providing anti-aging effects, and possessing antioxidant capacity (Kunz et al., 2016; Suryawanshi et al., 2020).
Insects significantly contribute to our understanding of bioactive compounds. Philanthotoxins from digger wasps are helping researchers understand ligand-gated ion channels. Solenopsin from fire ants inspires the synthesis of insecticidal compounds. Bee venom components, such as apamin and melittin, have specific effects on potassium channels and act as membrane-active peptides. The saliva toxins of assassin bugs interact with the voltage-gated calcium channels in their prey. Some beetles produce diamphotoxin and leptinotarsin, which are hemolytic peptide toxins traditionally used as arrow venom. Wasp venom contains mastoparan, a potent peptide toxin (Kachel et al., 2018; Biondi et al., 2022; Ye et al., 2023). The investigation of insects as sources of bioactive compounds is a continuing area of research.
Latin America’s adoption of insect consumption not only surpasses that of the European market but also demonstrates a promising growth trajectory. Insects are deeply rooted in the region’s culinary heritage, holding a unique position as a traditional and significant food source. Their popularity stems not only from their culinary appeal but also from their substantial nutritional content, making them a valuable asset in addressing food security challenges prevalent in many Latin American communities (Halloran et al., 2018). Despite the acknowledged benefits, widespread hesitancy persists, driven by aesthetic concerns regarding insect appearance. However, as global challenges such as population growth, limited agricultural space, and environmental degradation intensify, the need to explore alternative food sources becomes urgent (Klaus and Nakamura, 2021). Insects offer a sustainable solution, providing an opportunity to address these challenges while also supporting cultural preservation and economic development. Insects provide a rich source of essential nutrients, offering a promising avenue to combat malnutrition, especially in regions with limited access to diverse and nutrient-dense foods. To ensure the safety and quality of insect-derived products, it is imperative to prioritize the development of regulated insect farming practices over consuming wild-caught specimens, which may pose health risks (Van Huis, 2016; Imathiu, 2020; Aguilar-Toalá et al., 2022). The establishment of legislation is crucial to standardize production methods and uphold consumer confidence in insect-based foods.
The growing acceptance of consuming edible insects in Latin America presents a multifaceted opportunity that includes economic prosperity, cultural preservation, and geopolitical influence. Economically, the cultivation and utilization of insects offer the potential to create new industries and job opportunities, contributing to the region’s socio-economic development. The low production costs and high nutritional value of insects position them as a lucrative commodity in both domestic and international markets, fostering economic growth and trade expansion. Integrating insects into Latin American culinary traditions not only preserves cultural heritage but also fosters a sense of identity and pride within communities. By embracing insect consumption, Latin America reaffirms its cultural richness and diversity while addressing pressing global challenges sustainably. From a geopolitical standpoint, the region’s leadership in insect production and consumption grants it a strategic advantage, elevating its prominence in the global food trade arena. Effective utilization of this valuable resource has the potential to propel Latin America to the forefront of the emerging insect-based food industry, solidifying its position as a key player in shaping the future of sustainable food systems worldwide. Through strategic investment, innovation, and collaboration, Latin America can harness the full potential of edible insects, paving the way for a more resilient, fair, and sustainable food future.
For these reasons, it is necessary to carry out activities aimed at the proper conservation and use of this privileged resource, such as
• Implementing educational programs to dispel myths and misconceptions about insect consumption, working with communities at the local level to raise awareness of the nutritional benefits and cultural importance of edible insects. This could include workshops, cooking demonstrations, and information campaigns tailored to different demographic groups.
• We can also encourage culinary professionals to incorporate edible insects into traditional and contemporary dishes by supporting initiatives that showcase the versatility and deliciousness of insect-based cuisine through food festivals, cooking competitions, and culinary events. This can help increase consumer acceptance and demand.
• In addition, there is a need to collaborate with government authorities to establish clear rules and regulations for the production, processing, and sale of edible insects. This should focus on food safety regulations to ensure the quality and integrity of insect products.
• Provide resources for research projects aimed at improving the nutritional profile, taste, and texture of edible insects. Encourage collaboration between academia, industry, and agricultural stakeholders to drive innovation in insect-rearing techniques and product development.
• In addition, there is a need to facilitate access to resources, training, and infrastructure required to establish community insect farms. Emphasizing the socio-economic benefits of insect farming, such as income generation and food security, can encourage community participation.
• Facilitate access to insect-derived products by expanding distribution networks and increasing market visibility. Explore innovative packaging methods and marketing strategies that cater to various consumer preferences and food trends.
• Direct efforts should be made to foster collaboration with international partners, agencies, research institutions, and industry stakeholders to leverage expertise and resources. It is important to learn from success stories and adapt proven strategies to the unique context of Latin America.
CG-E: Conceptualization, Formal analysis, Investigation, Methodology, Project administration, Resources, Supervision, Validation, Visualization, Writing – original draft, Writing – review & editing. AV-L: Writing – review & editing, Validation, Supervision, Software, Resources, Methodology, Investigation, Funding acquisition, Data curation. NC-C: Investigation, Methodology, Resources, Supervision, Validation, Writing – review & editing. HG-O: Funding acquisition, Investigation, Methodology, Project administration, Resources, Software, Supervision, Writing – review & editing. CG-R: Investigation, Methodology, Project administration, Resources, Software, Supervision, Writing – review & editing. JR-V: Funding acquisition, Investigation, Methodology, Project administration, Resources, Software, Supervision, Writing – review & editing. MS-C: Funding acquisition, Investigation, Methodology, Project administration, Resources, Software, Supervision, Writing – review & editing. LS-C: Funding acquisition, Investigation, Methodology, Project administration, Resources, Software, Supervision, Writing – review & editing. BR-V: Writing – review & editing, Supervision, Software, Resources, Project administration, Methodology, Investigation, Funding acquisition. FA-B: Funding acquisition, Investigation, Methodology, Project administration, Resources, Software, Supervision, Writing – review & editing. PA-H: Writing – review & editing, Supervision, Software, Resources, Project administration, Methodology, Investigation, Funding acquisition.
The author(s) declare that no financial support was received for the research, authorship, and/or publication of this article.
We would like to express our gratitude to the Instituto Politécnico Nacional CIIDIR Oaxaca. We would like to thank the National Council of Humanities, Sciences, and Technologies (CONAHCYT-Mexico) for the “Researchers for Mexico” program. We would also like to express our gratitude to Guadalupe Cab-Paat and Viviana Chan, who were appointed by the Government Program “Young People Building the Future-JCF 2022–2023” to provide support to the Laboratory of Applied Entomology at CEDESU-UACAM.
The authors declare that the research was conducted in the absence of any commercial or financial relationships that could be construed as a potential conflict of interest.
All claims expressed in this article are solely those of the authors and do not necessarily represent those of their affiliated organizations, or those of the publisher, the editors and the reviewers. Any product that may be evaluated in this article, or claim that may be made by its manufacturer, is not guaranteed or endorsed by the publisher.
Adámková, A., Mlcˇek, J., Kourˇimska, L., Borkovcová, M., Bušina, T., Adámek, M., et al. (2017). Nutritional potential of selected insect species reared on the island of Sumatra. Int. J. Environ. Res. Public Health 14:521. doi: 10.3390/ijerph14050521
Adeduntan, S. A. (2005). Nutritional and antinutritional characteristics of some insects foraging in Akure forest reserve Ondo state. Nigeria. J. Food Technol. 3, 563–567.
Ademolu, K. O., Idowu, A. B., and Olatunde, G. O. (2010). Nutritional value assessment of variegated grasshopper, Zonocerus variegatus (L.) (Acridoidea: Pyrgomorphidae) during post- embryonic development. Afr. Entomol. 18, 360–364. doi: 10.4001/003.018.0201
Aguilar-Toalá, J. E., Cruz-Monterrosa, R. G., and Liceaga, A. M. (2022). Beyond human nutrition of edible insects: health benefits and safety aspects. Insects 13:1007. doi: 10.3390/insects13111007
Akullo, J., Agea, J. G., Obaa, B. B., Okwee-Acai, J., and Nokimbugwe, D. (2018). Nutritional composition of commonly consumed edible insects in the Lango sub-region of northern Uganda. Int. Food Res. J. 25, 159–166.
Alhujaili, A., Nocella, G., and Macready, A. (2023). Insects as food: consumers’ acceptance and marketing. Food Secur. 12, 886–895. doi: 10.3390/foods12040886
Álvarez, L. J. (2016). Diversidad de las abejas nativas de la tribu Meliponini (Hymenoptera, Apidae) en Argentina. Doctoral Dissertation, Universidad de la Plata, España. 288
Ashiru, M. O. (1989). The food value of the larvae of Anaphe venata Butler (Lepidoptera: Notodontidae). Ecol. Food Nutr. 22, 313–320. doi: 10.1080/03670244.1989.9991080
Association of American Feed Control Officials (AAFCO). (2023). Available at: https://www.aafco.org/Portals/0/SiteContent/Meetings/Midyear/2018/2018_AAFCO_agenda_book.pdf (Accessed on 04 January 2023).
Avendaño, C., Sánchez, M., and Valenzuela, C. (2020). Insectos: Son realmente una alternativa para la alimentación de animales y humanos. RCHNUT 47, 1029–1037. doi: 10.4067/S0717-75182020000601029
Backwell, L. R., and d’Errico, F. (2001). Evidence of termite foraging by Swartkrans early hominids. PNAS 98, 1358–1363. doi: 10.1073/pnas.98.4.1358
Baiano, A. (2020). Edible insects: an overview on nutritional characteristics, safety, farming, production technologies, regulatory framework, and socio-economic and ethical implications. Trends Food Sci. Technol. 100, 35–50. doi: 10.1016/j.tifs.2020.03.040
Baigts-Allende, D., Doost, A. S., Ramirez-Rodrigues, M., Dewettinck, K., Van der Meeren, P., De Meulenaer, B., et al. (2021). Insect protein concentrates from Mexican edible insects: structural and functional characterization. LWT 152:112267. doi: 10.1016/j.lwt.2021.112267
Banjo, A. D., Lawal, O. A., and Songonuga, E. A. (2006). The nutritional value of fourteen species of edible insects in southwestern Nigeria. Afr. J. Biotechnol. 5, 298–301. doi: 10.5897/AJB05.250
Barragán-Fonseca, K. Y., Nurfikari, A., Van De Zande, E. M., Wantulla, M., Van Loon, J. J., De Boer, W., et al. (2022). Insect frass and exuviae to promote plant growth and health. Trends Plant Sci. 6:e7. doi: 10.1016/j.tplants.2022.01.007
Bbosa, T., Ndagire, C. T., Mukisa, I. M., Fiaboe, K. K. M., and Nakimbugwe, D. (2019). Nutritional characteristics of selected insects in Uganda for use as alternative protein sources in food and feed. J. Insect Sci. 19:23. doi: 10.1093/jisesa/iez124
Beesigamukama, D., Subramanian, S., and Tanga, C. M. (2022). Nutrient quality and maturity status of frass fertilizer from nine edible insects. Sci. Rep. 12:7182. doi: 10.1038/s41598-022-11336-z
Bermúdez-Serrano, I. M. (2020). Challenges and opportunities for the development of an edible insect food industry in Latin America. J. Insects Food Feed 6, 537–556. doi: 10.3920/JIFF2020.0009
Biondi, M., D’Alessandro, P., Cerasoli, F., De Simone, W., and Iannella, M. (2022). Taxonomy, habitat preference, and niche overlap of two arrow-poison flea beetle species of the genus Polyclada in sub-Saharan Africa (Coleoptera, Chrysomelidae). Insects 13:668. doi: 10.3390/insects13080668
Brothwell, D., and Brothwell, P. (1998). Food in antiquity: A survey of the diet of early people. Baltimore, MD, USA: John Hopkins University Press, 283.
Buenrostro, O., Cram, S., Bernache, G., and Bocco, G. (2000). La digestión anaerobia como alternativa de tratamiento a los residuos sólidos orgánicos generados en los mercados municipales. Rev. Int. de Contam. Ambient. 16.
Bukkens, S. G. F. (1997). The nutritional value of edible insects. Ecol. Food Nutr. 36, 287–319. doi: 10.1080/03670244.1997.9991521
Bußler, S., Rumpold, B. A., Jander, E., Rawel, H. M., and Schlüter, O. K. (2016). Recovery and techno-functionality of flours and proteins from two edible insect species: meal worm (Tenebrio molitor) and black soldier fly (Hermetia illucens) larvae. Heliyon 2:e00218. doi: 10.1016/j.heliyon.2016.e00218
Calderón-Fallas, R. Á., Sánchez-Chávez, L. A., and Aguilar-Monge, I. (2021). XII Congreso Mesoamericano de Abejas Nativas: Desafíos y oportunidades para la conservación de las abejas nativas, 20 y 21 de noviembre
Caligliani, A., Marseglia, A., Leni, G., Baldassarre, S., Maistrello, L., Dossena, A., et al. (2018). Composition of black soldier fly prepupae and systematic approaches for extraction and fractionation of proteins, lipids and chitin. Food Res. Int. 105, 812–820. doi: 10.1016/j.foodres.2017.12.012
Chakravorty, J., Ghosh, S., Jung, C., and Meyer-Rochow, V. B. (2014). Nutritional composition of Chondacris rosea and Brachytrupes orientalis: two common insects used as food by tribes of Arunachal Pradesh, India. J. Asia Pac. Entomol. 17, 407–415. doi: 10.1016/j.aspen.2014.03.007
Chakravorty, J., Ghosh, S., Megu, K., Jung, C., and Meyer-Rochow, V. B. (2016). Nutritional and anti-nutritional composition of Oecophylla smaragdina (Hymenoptera: Formicidae) and Odontotermes sp. (Isoptera: Termitidae): two preferred edible insects of Arunachal Pradesh, India. J. Asia Pac. Entomol. 19, 711–720. doi: 10.1016/j.aspen.2016.07.001
Chakravorty, J., Ghosh, S., and Meyer-Rochow, V. B. (2011). Chemical composition of Aspongopus nepalensis Westwood 1837 (Hemiptera; Pentatomidae), a common food insect of tribal people in Arunachal Pradesh (India). Int. J. Vitam. Nutr. Res. 81, 49–56. doi: 10.1024/0300-9831/a000050
Chavez, M., and Uchanski, M. (2021). Insect left-over substrate as plant fertiliser. J. Insects Food Feed 7, 683–694. doi: 10.3920/JIFF2020.0063
Chen, Y. A., and Forschler, B. T. (2016). Elemental concentrations in the frass of saproxylic insects suggest a role in micronutrient cycling. Ecosphere 7:e01300. doi: 10.1002/ecs2.1300
Chinweuba, A. J., Otuokere, I. E., Opara, M. O., and Okafor, G. U. (2011). Nutritional potentials of Rhynchophorus phoenicis (Rahia palm weevil): implications for food security. Asian J. Res. Chem. 4, 452–454.
Cordoba-Aguilar, A., Ensaldo-Cárdenas, A. S., Sánchez-Rivero, X. J., Martínez-Castaneira, M. X., Valencia-López, L. A., Vargas Abasolo, R., et al. (2023). Consejos prácticos ante la extinción masiva de insectos. Boletín de la Sociedad Científica Mexicana de Ecología 3, 50–85.
Cortes-Martínez, D. E., Olarte Blandón, O. J., and Valdés Cortes, L. M. (2021). Captura de enjambres de abejas nativas sin aguijón con dispositivos - trampa en el campus universitario del CEAD Acacias–meta. Agricolae Habitat 4, 54–64. doi: 10.22490/26653176.4289
Costa-Neto, E. M. (2015). Anthropo-entomophagy in Latin America: an overview of the importance of edible insects to local communities. J. Insects Food Feed. 1, 1723. doi: 10.3920/JIFF2014.0015
Costa-Neto, E., and Ramos-Elorduy, J. (2006). Los insectos comestibles de Brasil: etnicidad, diversidad e importancia en la alimentación. Bol. Soc. Entomol. Aragonesa 38, 423–442.
Cruz, F. D. (2017). La contribución de los insectos a la seguridad alimentaria. In: Congreso Sociedad Colombiana de Entomología, Memorias y Resúmenes. R. D. F. Rincón, R. Y. P. Ardila, and D. Acosta (Comp.), 44 Congreso SOCOLEN. Bogotá, D.C., 5, 6 y 7 de julio de 2017, 64–75
Cullere, M., Schiavone, A., Dabbou, S., Gasco, L., and Dalle Zotte, A. (2019). Meat quality and sensory traits of finisher broiler chickens fed with black soldier fly (Hermetia illucens L.) larvae fat as alternative fat source. Animals 9:140. doi: 10.3390/ani9040140
Cullere, M., Tasoniero, G., Giaccone, V., Acuti, G., Marangon, A., and Dalle-Zotte, A. (2018). Black soldier fly as dietary protein source for broiler quails: meat proximate composition, fatty acid and amino acid profile, oxidative status and sensory traits. Animals 12, 640–647. doi: 10.1017/S1751731117001860
Cullere, M., Tasoniero, G., Giaccone, V., Miotti-Scapin, R., Claeys, E., De Smet, S., et al. (2016). Black soldier fly as dietary protein source for broiler quails: apparent digestibility, excreta microbial load, feed choice, performance, carcass and meat traits. Animals 10, 1923–1930. doi: 10.1017/S1751731116001270
Cumo, C. (2015). The ongoing Columbian exchange: Stories of biological and economic transfer in world history: Stories of biological and economic transfer in world history : ABC-CLIO, 396.
da Costa-Rocha, A. C., de Andrade, C. J., and de Oliveira, D. (2021). Perspective on integrated biorefinery for valorization of biomass from the edible insect Tenebrio molitor. Trends Food Sci. Technol. 116, 480–491. doi: 10.1016/j.tifs.2021.07.012
Dagevos, H., and Taufik, D. (2023). Eating full circle: exploring consumers’ sympathy for circularity in entomophagy acceptance. Food Qual. Prefer. 105:104760. doi: 10.1016/j.foodqual.2022.104760
Dalmoro, Y. K., Adams, C. B., Haetinger, V. S., Bairros, L., Yacoubi, N., and Stefanello, C. (2021). Energy values of Tenebrio molitor larvae meal and tilapia byproduct meal for broiler chickens determined using the regression method. Anim. Feed Sci. Technol. 272:114784. doi: 10.1016/j.anifeedsci.2020.114784
de Castro, R. J. S., Ohara, A., dos Santos Aguilar, J. G., and Domingues, M. A. F. (2018). Nutritional, functional and biological properties of insect proteins: processes for obtaining, consumption and future challenges. Trends Food Sci. Technol. 76, 82–89. doi: 10.1016/j.tifs.2018.04.006
DeFoliart, G. R. (1989). The human use of insects as food and as animal feed. Bull. Entomol. Soc. Am. 35, 22–35. doi: 10.1093/besa/35.1.22
Dobermann, D., Swift, J. A., and Field, L. M. (2017). Opportunities and hurdles of edible insects for food and feed. Nut. Bull. 42, 293–308. doi: 10.1111/nbu.12291
Dossey, A. T., Morales-Ramos, J. A., and Rojas, M. G. (Eds.) (2016). Insects as sustainable food ingredients: Production, processing and food applications. Amsterdam, Netherlands: Elsevier.
Durst, P. B., and Hanboonsong, Y. (2015). Small-scale production of edible insects for enhanced food security and rural livelihoods: experience from Thailand and Lao People’s Democratic Republic. J. Insects Food Feed 1, 25–31. doi: 10.3920/JIFF2014.0019
Ekop, K. E., Onigbinde, A. O., and Asia, I. O. (2009). Pharmaceutical potentials of the oils of some popular insects consumed in Southeast Nigeria. Afr. J. Pharm. Pharmacol 3, 51–57. doi: 10.5897/AJPP.9000216
Ekop, E. A., Udoh, A. I., and Akpan, P. E. (2010). Proximate and anti-nutrient composition of four edible insects in Akwa Ibom state. Nigeria. World. J. Appl. Sci. Technol. 2, 224–231.
Elser, J. J., Fagan, W. F., Denno, R. F., Dobberfuhl, D. R., Folarin, A., Huberty, A., et al. (2000). Nutritional constraints in terrestrial and freshwater food webs. Nature 408, 578–580. doi: 10.1038/35046058
Erickson, M. C., Islam, M., Sheppard, C., Liao, J., and Doyle, M. P. (2004). Reduction of Escherichia coli O157:H7 and Salmonella enterica serovar enteritidis in chicken manure by larvae of the black soldier fly. JFP 67, 685–690. doi: 10.4315/0362-028x-67.4.685
Fielding, D. J., Trainor, E., and Zhang, M. (2013). Diet influences rates of carbon and nitrogen mineralization from decomposing grasshopper frass and cadavers. Biol. Fertil. Soils 49, 537–544. doi: 10.1007/s00374-012-0702-5
Finke, M. D. (2002). Complete nutrient composition of commercially raised invertebrates used as food for insectivores. Zoo Biol. 21, 269–285. doi: 10.1002/zoo.10031
Frost, C. J., and Hunter, M. D. (2008). Insect herbivores and their frass affect Quercus rubra leaf quality and initial stages of subsequent litter decomposition. Oikos 117, 13–22. doi: 10.1111/j.2007.0030-1299.16165.x
Ganguly, A., Chakravorty, R., Das, M., Gupta, M., Mandal, D. K., Haldar, P., et al. (2013). A preliminary study on the nutrients and antinutrients in Oedaleus abruptus (Thunberg) (Orthoptera: Acrididae). Int. J. Nutr. Metab. 5, 60–65. doi: 10.5897/IJNAM12.022
Ghadimi, P., Wang, C., and Lim, M. K. (2019). Sustainable supply chain modeling and analysis: past debate, present problems, and future challenges. Resour. Conserv. Recycl. 140, 72–84. doi: 10.1016/j.resconrec.2018.09.005
Ghosh, S., Choi, K. C., Kim, S., and Jung, C. (2017). Body compositional changes of fatty acid and amino acid from the queen bumblebee, Bombus terrestris during overwintering. J. Apic. 32, 11–18. doi: 10.17519/apiculture.2017.04.32.1.11
Ghosh, S., Chuttong, B., Burgett, M., Meyer-Rochow, V. B., and Jung, C. (2020). Nutritional value of brood and adult workers of the Asia honeybee species Apis cerana and Apis dorsata. African edible insects as alternative source of food, oil, protein and bioactive components, A. A. Mariod., Ed.; Springer: Cham, Switzerland, 265–273.
Ghosh, S., Haldar, P., and Mandal, D. K. (2016). Evaluation of nutrient quality of a short-horned grasshopper, Oxya hyla hyla Serville (Orthoptera: Acrididae), in search of new protein source. J. Entomol. Zool. Stud. 4, 193–197.
Ghosh, S., Jung, C., Chuttong, B., and Burgett, M. (2020) in Nutritional aspects of the dwarf honeybee (Apis florea F.) for human consumption. In the future role of dwarf honeybees in natural and agricultural systems. ed. D. P. Abrol (FL, USA: CRC Press, Boca Raton), 137–145.
Ghosh, S., Jung, C., and Meyer-Rochow, V. B. (2016). Nutritional value and chemical composition of larvae, pupae and adults of worker honey bee, Apis mellifera ligustica as a sustainable food source. J. Asia Pac. Entomol. 19, 487–495. doi: 10.1016/j.aspen.2016.03.008
Ghosh, S., Lee, S. M., Jung, C., and Meyer-Rochow, V. B. (2017). Nutritional composition of five commercial edible insects in South Korea. J. Asia Pac. Entomol. 20, 686–694. doi: 10.1016/j.aspen.2017.04.003
Ghosh, S., Namin, S. M., Meyer-Rochow, V. B., and Jung, C. (2021). Chemical composition and nutritional value of different species of Vespa hornets. Food Secur. 10:418. doi: 10.3390/foods10020418
Gkinali, A. A., Matsakidou, A., Vasileiou, E., and Paraskevopoulou, A. (2022). Potentiality of Tenebrio molitor larva-based ingredients for the food industry: a review. Trends Food Sci. Technol. 119, 495–507. doi: 10.1016/j.tifs.2021.11.024
Glover, D., and Sexton, A. (2015). Edible insects and the future of food: A foresight scenario exercise on entomophagy and global food security report. IDS Evidence report (149) : IDS-Institute of Development Studies, 57.
Grabowski, N. T., Abdulmawjood, A., Acheuk, F., Barragán-Fonseca, K., Chhay, T., Costa-Neto, E. M., et al. (2022). Insects—a source of safe and sustainable food?—“Jein”(yes and no). Front. Sustain. Food Syst. 5:701797. doi: 10.3389/fsufs.2021.701797
Green, B. W. (2022). Fertilizers in aquaculture. Feed and Feeding Practices in Aquaculture Woodhead Publishing. 29–63
Grüter, C. (2023). Stingless bees. Available at: www.springer.com/series/15408 (Accessed on 02 March 2023).
Guiné, R. P., Correia, P., Coelho, C., and Costa, C. A. (2021). The role of edible insects to mitigate challenges for sustainability. Open Agric. 6, 24–36. doi: 10.1515/opag-2020-0206
Guiné, R. P., Klava, D., Straumite, E., Kruma, Z., Florença, S. G., Anjos, O., et al., (2023). Consumer perception about edible insects’ relation with environment and sustainability. 16th Baltic conference on food science and technology FOODBALT, 119
Gupta, R. K., Khan, M. S., Srivastava, R. M., and Goswami, V. (2014). History of beekeeping in developing world in Beekeeping for poverty alleviation and livelihood security. Dordrecht: Springer, 3–62.
Hall, H. N., O’Neill, H. V. M., Scholey, D., Burton, E., Dickinson, M., and Fitches, E. C. (2018). Amino acid digestibility of larval meal (Musca domestica) for broiler chickens. Poult. Sci. 97, 1290–1297. doi: 10.3382/ps/pex433
Halloran, A., Flore, R., Vantomme, P., and Roos, N. (2018). Edible insects in sustainable food systems. Switzerland: Springer International Publishing, 479.
Hanboonsong, Y., Jamjanya, T., and Durst, P. B. (2013). Six-legged livestock: edible insect farming, collection and marketing in Thailand. RAP publication 3, 62–70.
Hardy, K., Radini, A., Buckley, S., Blasco, R., Copeland, L., Burjachs, F., et al. (2016). Diet and environment 1.2 million years ago revealed through analysis of dental calculus from Europe’s oldest hominin at Sima del Elefante, Spain. Sci. Nat. 104:2. doi: 10.1007/s00114-016-1420-x
Hazarika, A. K., and Kalita, U. (2023). Human consumption of insects. Sci 379, 140–141. doi: 10.1126/science.abp8819
Hillstrom, M., Meehan, T. D., Kelly, K., and Lindroth, R. L. (2010). Soil carbon and nitrogen mineralization following deposition of insect frass and greenfall from forests under elevated CO 2 and O 3. Plant Soil 336, 75–85. doi: 10.1007/s11104-010-0449-4
Hlongwane, Z. T., Slotow, R., and Munyai, T. C. (2020). Indigenous knowledge about consumption of edible insects in South Africa. Insects 12, 22–30. doi: 10.3390/insects12010022
Igwe, C. U., Ujowundu, C. O., Nwaogu, L. A., and Okwu, G. N. (2011). Chemical analysis of an edible African termite, Macrotermes nigeriensis, a potential antidote to food security problem. Biochem. Anal. Biochem. 1:1000105. doi: 10.4172/2161-1009.1000105
Imathiu, S. (2020). Benefits and food safety concerns associated with consumption of edible insects. NFS J. 18, 1–11. doi: 10.1016/j.nfs.2019.11.002
Islam, M., Munmun, M. I., Islam, M. A., and Mondal, S. (2018). Effect of different sources of organic nutrients in combination with fertilizers on the production of cabbage. Eco-Friendly Agril. 11, 13–20. doi: 10.13140/RG.2.2.12985.47208
Jaffé, R., Pope, N., Carvalho, A. T., Maia, U. M., Blochtein, B., and de Carvalho, C. (2015). Bees for development: Brazilian survey reveals how to optimize stingless beekeeping. PLoS One 10:e0121157. doi: 10.1371/journal.pone.0121157
Jo, H., Lee, D., Go, C., Jang, Y., Bae, S., Agura, T., et al. (2022). Alloferon affects the Chemosensitivity of pancreatic Cancer by regulating the expression of SLC6A14. Biomedicines 10:1113. doi: 10.3390/biomedicines10051113
Jongema, Y. (2013). Worldwide list of recorded edible insects. Available at: https://www.wur.nl/en/Research-Results/Chair-groups/Plant-Sciences/Laboratory-of-Entomology/Edible-insects/Worldwide-species-list.htm (Accessed on 02 March 2023).
Jongema, Y. (2017). List of edible insects of the world. Available at: https://www.wur.nl/en/research-results/chair-groups/plant-sciences/laboratory-of-entomology/edible-insects/worldwide-species-list.htm, university fund Wageningen (Accessed on 02 March 2023)
Kachel, H. S., Buckingham, S. D., and Sattelle, D. B. (2018). Insect toxins—selective pharmacological tools and drug/chemical leads. Curr. Opin. Insect Sci. 30, 93–98. doi: 10.1016/j.cois.2018.10.001
Kagata, H., and Ohgushi, T. (2011). Ingestion and excretion of nitrogen by larvae of a cabbage armyworm: the effects of fertilizer application. Agric. For. Entomol. 13, 143–148. doi: 10.1111/j.1461-9563.2010.00502.x
Kagata, H., and Ohgushi, T. (2012a). Non-additive effects of leaf litter and insect frass mixture on decomposition processes. Ecol. Res. 27, 69–75. doi: 10.1007/s11284-011-0868-6
Kagata, H., and Ohgushi, T. (2012b). Positive and negative impacts of insect frass quality on soil nitrogen availability and plant growth. Popul. Ecol. 54, 75–82. doi: 10.1007/s10144-011-0281-6
Khalifah, A., Abdalla, S., Rageb, M., Maruccio, L., Ciani, F., and El-Sabrout, K. (2023). Could insect products provide a safe and sustainable feed alternative for the poultry industry? A comprehensive review. Animals 13:1534. doi: 10.3390/ani13091534
Khan, D., Sahito, Z. A., Dawar, S., and Zaki, M. J. (2016). Frass of saproxylic-cerambycid larvae from dead twigs of Acacia stenophylla a. Cunn. EX. Benth. And its effects on germination and seedling growth of Lactuca sativa L. var. grand rapids. Int. J. Biol. Biotechnol. 13, 461–470.
Kim, T. K., Yong, H. I., Kim, Y. B., Jung, S., Kim, H. W., and Choi, Y. S. (2021). Effects of organic solvent on functional properties of defatted proteins extracted from Protaetia brevitarsis larvae. Food Chem. 336:127679. doi: 10.1016/j.foodchem.2020.127679
Kinyuru, J. N., Konyole, S. O., Roos, N., Onyango, C. A., Owino, V. O., Owuor, B. O., et al. (2013). Nutrient composition of four species of winged termites consumed in western Kenya. J. Food Compos. Anal. 30, 120–124. doi: 10.1016/j.jfca.2013.02.008
Kipkoech, C., Jaster-Keller, J., Gottschalk, C., Wesonga, J. M., and Maul, R. (2023). African traditional use of edible insects and challenges towards the future trends of food and feed. J. Insect Food Feed 1–24:e76. doi: 10.3920/JIFF2022.0076
Klaus, L. W., and Nakamura, Y. (2021). Edible insects as future food: chances and challenges. J. Future Foods 1, 38–46. doi: 10.1016/j.jfutfo.2021.10.001
Kouřimská, L., and Adámková, A. (2016). Nutritional and sensory quality of edible insects. NFS J. 4, 22–26. doi: 10.1016/j.nfs.2016.07.001
Kowalski, S., Mikulec, A., Mickowska, B., Skotnicka, M., and Mazurek, A. (2022). Wheat bread supplementation with various edible insect flours. Influence of chemical composition on nutritional and technological aspects. LWT 159:113220. doi: 10.1016/j.lwt.2022.113220
Kozłowski, K., Ognik, K., Stępniowska, A., Juśkiewicz, J., Zduńczyk, Z., Kierończyk, B., et al. (2021). Growth performance, immune status and intestinal fermentative processes of young turkeys fed diet with additive of full fat meals from Tenebrio molitor and Hermetia illucens. Anim. Feed Sci. Technol. 278:114994. doi: 10.1016/j.anifeedsci.2021.114994
Krongdang, S., Phokasem, P., Venkatachalam, K., and Charoenphun, N. (2023). Edible insects in Thailand: an overview of status, properties, processing, and utilization in the food industry. Food Secur. 12, 21–62. doi: 10.3390/foods12112162
Kulma, M., Kouřimská, L., Plachý, V., Božik, M., Adámková, A., and Vrabec, V. (2019). Effect of sex on the nutritional value of house cricket, Acheta domestica L. Food Chem. 272, 267–272. doi: 10.1016/j.foodchem.2018.08.049
Kumul, R. C., Ruiz, J. C. R., Vázquez, E. O., and Campos, M. R. S. (2015). Antioxidant potential of Melipona beecheii honey and its relationship to health: a review. Nutr. Hosp. 32, 1432–1442. doi: 10.3305/nh.2015.32.4.9312
Kunz, R. I., Brancalhão, R. M. C., Ribeiro, L. D. F. C., and Natali, M. R. M. (2016). Silkworm sericin: Properties and biomedical applications. Bio Med. Res. Int. 16:175701. doi: 10.1155/2016/8175701
Kyriacou, K. (2014). Coastal resources and nutrition among middle stone age hunter-gatherers in the southwestern cape, PhD Thesis : University of Cape Town, Cape Town, South Africa.
Lange, K. W., and Nakamura, Y. (2023). Potential contribution of edible insects to sustainable consumption and production. Front. Sustain. 4:1112950. doi: 10.3389/frsus.2023.1112950
Lara-Juárez, P., Aguirre-Rivera, J. R., Castillo-Lara, P., and Reyes-Agüero, J. A. (2015). Biología y aprovechamiento de la hormiga de escamoles, Liometopum apiculatum Mayr (Hymenoptera: Formicidae). Act. Zoo. Mex. 31, 251–264.
Lee, D., Jo, H., Jang, Y., Bae, S., Agura, T., Kang, D., et al. (2023). Alloferon and Zanamivir show effective antiviral activity against influenza a virus (H1N1) infection in vitro and in vivo. Int. J. Mol. Sci. 24:678. doi: 10.3390/ijms24010678
Lizhang, W., Viejo, M. J. L., and Dinghong, Y. (2008). Insectos como fuente de alimento: Análisis del contenido en proteína y grasa de 100 especies. Bol. Mus. Munic. Funchal 14, 55–70.
Lopes, I. G., Yong, J. W., and Lalander, C. (2022). Frass derived from black soldier fly larvae treatment of biodegradable wastes. A critical review and future perspectives. Waste Mant. 142, 65–76. doi: 10.1016/j.wasman.2022.02.007
Mariod, A. A., Abdel-Wahab, S. I., and Ain, N. M. (2011). Proximate amino acid, fatty acid and mineral composition of two Sudanese edible pentatomid insects. Int. J. Trop. Insect Sci. 31, 145–153. doi: 10.1017/S1742758411000282
McGrew, W. C. (2014). The ‘other faunivory’ revisited: insectivory in human and non-human primates and the evolution of human diet. J. Human Evol. 71, 4–11. doi: 10.1016/j.jhevol.2013.07.016
Medeiros, E. (2014). Anthropo-entomophagy in Latin America: an overview of the importance of edible insects to local communities. J. Insects Food Feed 1, 17–23. doi: 10.3920/JIFF2014.0015
Melgar-Lalanne, G., Hernández-Álvarez, A. J., and Salinas-Castro, A. (2019). Edible insects processing: traditional and innovative technologies. Compr. Rev. Food Sci. Food Saf. 18, 1166–1191. doi: 10.1111/1541-4337.12463
Melin, A. D., Young, H. C., Mosdossy, K. N., and Fedigan, L. M. (2014). Seasonality, extractive foraging and the evolution of primate sensorimotor intelligence. J. Human Evol. 71, 77–86. doi: 10.1016/j.jhevol.2014.02.009
Meyer-Rochow, V. B., Gahukar, R. T., Ghosh, S., and Jung, C. (2021). Chemical composition, nutrient quality and acceptability of edible insects are affected by species, developmental stage, gender, diet, and processing method. Food Secur. 10:1036. doi: 10.3390/foods10051036
Mintah, B. K., He, R., Dabbour, M., Golly, M. K., Agyekum, A. A., and Ma, H. (2019). Effect of sonication pretreatment parameters and their optimization on the antioxidant activity of Hermetia illucens larvae meal protein hydrolysates. J. Food Process. Preserv. 43:e14093. doi: 10.1111/jfpp.14093
Montalbán, A., Sánchez, C. J., Hernández, F., Schiavone, A., Madrid, J., and Martínez-Miró, S. (2022). Effects of agro-industrial byproduct-based diets on the growth performance, digestibility, nutritional and microbiota composition of mealworm (Tenebrio molitor L.). Insects 13:323. doi: 10.3390/insects13040323
Munialo, C. D., Stewart, D., Campbell, L., and Euston, S. R. (2022). Extraction, characterisation and functional applications of sustainable alternative protein sources for future foods: a review. Future Foods 2022:100152. doi: 10.1016/j.fufo.2022.100152
Nascimento-Filho, M. A., Pereira, R. T., Oliveira, A. B. S. D., Suckeveris, D., Junior, A. B., Soares, C. A. P., et al. (2021). Nutritional value of Tenebrio molitor larvae meal for broiler chickens: metabolizable energy and standardized ileal amino acid digestibility. J. Appl. Poul. Res. 30:100102. doi: 10.1016/j.japr.2020.10.001
Nates-Parra, G., and Rosso-Londoño, J. M. (2013). Diversity of stingless bees (Hymenoptera: Meliponini) used in meliponiculture in Colombia. Colomb: Act. Biol, 18.
Naz, F., Wu, Y., Zhang, N., Yang, Z., and Yu, C. (2020). Anticancer attributes of Cantharidin: involved molecular mechanisms and pathways. Molecules 25:3279. doi: 10.3390/molecules25143279
Nyakeri, E. M., Ogola, H. J., Ayieko, M. A., and Amimo, F. A. (2017). An open system for farming black soldier fly larvae as a source of proteins for small scale poultry and fish production. J. Insects Food Feed 3, 51–56. doi: 10.3920/JIFF2016.0030
Ojha, S., Bußler, S., Psarianos, M., Rossi, G., and Schlüter, O. K. (2021). Edible insect processing pathways and implementation of emerging technologies. J. Insects Food Feed 7, 877–900. doi: 10.3920/JIFF2020.0121
Omotoso, O. T. (2015). Nutrition composition, mineral analysis and antinutrient factors of Oryctes rhinoceros L. (Scarabaeidae: Coleoptea) and winged termites, Macrotermes nigeriensis Sjostedt (Termitidae: Isoptera). Br. J. Appl. Sci. Technol. 8, 97–106. doi: 10.9734/BJAST/2015/15344
Omotoso, O. T. (2018). The nutrient profile of the development stages of palm beetle, Oryctes rhinoceros. Br. J. Environ. Sci. 6, 1–11.
Omotoso, O. T., and Adedire, C. O. (2007). Nutrient composition, mineral content and the solubility of the proteins of palm weevil, Rhynchophorus phoenicis (Coleoptera: Curculionidae). J Zhejiang Univ Sci B 8, 318–322. doi: 10.1631/jzus.2007.B0318
Omuse, E. R., Tonnang, H. E., Yusuf, A. A., Machekano, H., Egonyu, J. P., Kimathi, E., et al. (2024). The global atlas of edible insects: analysis of diversity and commonality contributing to food systems and sustainability. Sci. Rep. 14:5045. doi: 10.1038/s41598-024-55603-7
Ong’Or, D. O., Orinda, M. A., and Abel, B. O. (2024). Farmers’ knowledge and perceptions in the production of coconut Rhinoceros beetle (Oryctes rhinoceros) for enhanced food security in Kenya. EAJAB. 7, 13–25. doi: 10.37284/eajab.7.1.1683
Onore, G. (1997). A brief note on edible insects in Ecuador. Ecol. Food Nutr. 36, 277–285. doi: 10.1080/03670244.1997.9991520
Onyeike, E. N., Ayalogu, E. O., and Okaraonye, C. C. (2005). Nutritive value of the larvae of raphia palm beetle (Orytes rhinoceros) and weevil (Rhynchophorus pheonicis). J. Sci. Food Agric. 85, 1822–1828. doi: 10.1002/jsfa.2054
Oonincx, D. G., Van-Broekhoven, S., Van-Huis, A., and Van-Loon, J. J. (2015). Feed conversion, survival and development, and composition of four insect species on diets composed of food by-products. PLoS One 10:e0144601. doi: 10.1371/journal.pone.0144601
Opara, M. N., Sanyigha, F. T., Ogbuewu, I. P., and Okoli, I. C. (2012). Studies on the production trend and quality characteristics of palm grubs in the tropical rainforest zone of Nigeria. Int. J. Agric. Technol. 8, 851–860.
Orkusz, A. (2021). Edible insects versus meat—nutritional comparison: knowledge of their composition is the key to good health. Nutrients 13:1207. doi: 10.3390/nu13041207
Pal, P., and Roy, S. (2014). Edible insects: future of human food–a review. ILNS 21–30:e26. doi: 10.18052/www.scipress.com/ILNS.26.1
Papastavropoulou, K., Xiao, J., and Proestos, C. (2023). Edible insects: tendency or necessity (a review). eFood 4:e58. doi: 10.1002/efd2.58
Patterson, P. H., Acar, N., Ferguson, A. D., Trimble, L. D., Sciubba, H. B., and Koutsos, E. A. (2021). The impact of dietary black soldier Fly larvae oil and meal on laying hen performance and egg quality. Poult. Sci. 100:101272. doi: 10.1016/j.psj.2021.101272
Pérez-Ramírez, R., Torres-Castillo, J. A., Barrientos-Lazano, L., Almaguee-Sierra, P., and Torres-Acosta, R. I. (2019). Schistocerca piceifrons piceifrons as a source of compounds of biotechnological and nutritional interest. J. Insect Sci. 19:10. doi: 10.1093/jisesa/iez088
Pineda, A., Dicke, M., Pieterse, C. M., and Pozo, M. J. (2013). Beneficial microbes in a changing environment: are they always helping plants to deal with insects? Funct. Ecol. 27, 574–586. doi: 10.1111/1365-2435.12050
Pino, J. M., and Ramos-Elorduy, J. (2021). Taxonomic analysis of some edible insects from the state of Michoacán. Mexico. Front. Vet. Sci. 8:629194. doi: 10.3389/fvets.2021.629194
Poveda, J. (2021). Insect frass in the development of sustainable agriculture. Agro. for Sust. Devel. 41:5. doi: 10.1007/s13593-020-00656-x
Poveda, J., Jiménez-Gómez, A., Saati-Santamaría, Z., Usategui-Martín, R., Rivas, R., and García-Fraile, P. (2019). Mealworm frass as a potential biofertilizer and abiotic stress tolerance-inductor in plants. Appl. Soil Eco. 142, 110–122. doi: 10.1016/j.apsoil.2019.04.016
Queiroz, L. S., Regnard, M., Jessen, F., Mohammadifar, M. A., Sloth, J. J., Petersen, H. O., et al. (2021). Physico-chemical and colloidal properties of protein extracted from black soldier fly (Hermetia illucens) larvae. Int. J. Biol. Macrom. 186, 714–723. doi: 10.1016/j.ijbiomac.2021.07.081
Queiroz, L. S., Silva, N. F. N., Fernandes de Carvalho, A., and Casanova, F. (2023). Impact of emerging technologies on colloidal properties of insect proteins. Curr. Opin. Food Sci. 16:100958. doi: 10.1016/j.cofs.2022.100958
Quezada-Euán, J. J. G., and Alves, D. A. (2020). Meliponiculture. Encyclopedia of social insects. C. Starr Cham: Springer. 55–61.
Quezada-Euán, J. J. G., Nates-Parra, G., Maués, M. M., Roubik, D. W., and Imperatriz-Fonseca, V. L. (2018). The economic and cultural values of stingless bees (Hymenoptera: Meliponini) among ethnic groups of tropical America. Sociobiology 65, 534–557. doi: 10.13102/sociobiology.v65i4.3447
Rahman, M. M., Byanju, B., and Lamsal, B. P. (2023). Protein, lipid, and chitin fractions from insects: method of extraction, functional properties, and potential applications. Crit. Rev. Food Sci. Nutr. 2023, 1–17. doi: 10.1080/10408398.2023.2168620
Ramos-Elorduy, J. (2004). La etnoentomología en la alimentación, la medicina y el reciclaje. Biodiversidad, taxonomía y biogeografía de artrópodos de México: hacia una síntesis de su conocimiento. J. B. Llorente, O. Morrone, and I. F. Vargas (Eds.) México: UNAM, 329–413
Ramos-Elorduy, J. (2009). Anthropo-entomophagy: cultures, evolution and sustainability. Entomol. Res. 39, 271–288. doi: 10.1111/j.1748-5967.2009.00238.x
Ramos-Elorduy, J., González, E. A., Hernández, A. R., and Pino, J. M. (2002). Use of Tenebrio molitor (Coleoptera: Tenebrionidae) to recycle organic wastes and as feed for broiler chickens. J. Econ. Entomol. 95, 214–220. doi: 10.1603/0022-0493-95.1.214
Ramos-Elorduy, B. J., Moreno, J. M. P., and Camacho, V. H. M. (2012). Could grasshoppers be a nutritive meal? Food Nutr. Sci. 3, 164–175. doi: 10.4236/fns.2012.32025
Ramos-Elorduy, J., Moreno, J. M. P., and Correa, S. C. (1998). Edible insects of the state of Mexico and determination of their nutritive values. An. Inst. Biol. Univ. Nac. Auton. Mex. Ser. Zool. 69, 65–104.
Ramos-Elorduy, J., Moreno, J. M. P., Prado, E. E., Perez, M. A., Otero, J. L., and de Guevara, O. L. (1997). Nutritional value of edible insects from the state of Oaxaca. Mexico. J. Food Compos. Anal. 10, 142–157. doi: 10.1006/jfca.1997.0530
Ramos-Elorduy, J., and Pino, M. (2005). “Base de datos de insectos Comestibles de México” in Unibio (Unidad de Biodiversidad del IBUNAM), 113.
Ramos-Elorduy, J., Pino, J. M., and Conconi, M. A. (2003). Necesidad de una legislación para la explotación y venta de insectos comestibles en México. Congreso Méxicano De Etnobiología, 5., Chipango, Resúmenes. Chipango: Universidad Autónoma de Chipango, (2003), 59
Ramos-Elorduy, J. R., Pino, J. M., and Conconi, M. (2006). Ausencia de una reglamentación y normalización de la explotación y comercialización de insectos comestibles en México. Folia Entomol. Mex. 45, 291–318.
Ramos-Elorduy, J., and Viejo-Montesinos, J. L. (2007). Los insectos como alimento humano: Breve ensayo sobre la entomofagia, con especial referencia a México. Bol. Soc. Esp. Hist. Nat. 102, 61–84.
Ray, S., Alves, P. C., Ahmad, I., Gaffoor, I., Acevedo, F. E., Peiffer, M., et al. (2016). Turnabout is fair play: herbivory-induced plant chitinases excreted in fall armyworm frass suppress herbivore defenses in maize. Plant Physiol. 171, 694–706. doi: 10.1104/pp.15.01854
Ray, S., Gaffor, I., Acevedo, F. E., Helms, A., Chuang, W. P., Tooker, J., et al. (2015). Maize plants recognize herbivore-associated cues from caterpillar frass. J. Chem. Ecol. 41, 781–792. doi: 10.1007/s10886-015-0619-1
Reinhard, K. J., and Bryant, V. M. (1992). Coprolite analysis: a biological perspective on archaeology. Archaeological method and theory, M. Schiffer (Ed.), University of Arizona Press, Tucson, AZ, USA, 245–288.
Research and Markets. (2018). Mexico edible insect and insect protein market. Available on: https://tinyurl.com/ydbc 49no (Accessed on 02 March 2023).
Reverberi, M. (2021). The new packaged food products containing insects as an ingredient. J. Insects Food Feed 7, 901–908. doi: 10.3920/JIFF2020.0111
Rousseau, D. M. (2012). The Oxford handbook of evidence-based management. Oxford, New York: Oxford University Press, 460.
Rumpold, B. A., Fröhling, A., Reineke, K., Knorr, D., Boguslawski, S., Ehlbeck, J., et al. (2014). Comparison of volumetric and surface decontamination techniques for innovative processing of mealworm larvae (Tenebrio molitor). Innov. Food Sci. Emerg. Technol. 26, 232–241. doi: 10.1016/j.ifset.2014.09.002
Rumpold, B. A., and Schlüter, O. K. (2013). Nutritional composition and safety aspects of edible insects. Mol. Nutr. Food Res. 57, 802–823. doi: 10.1002/mnfr.201200735
Ryu, M. J., Anikin, V., Hong, S. H., Jeon, H., Yu, Y. G., Yu, M. H., et al. (2008). Activation of NF-κB by alloferon through down-regulation of antioxidant proteins and IκBα. Mol. Cell. Biochem. 313, 91–102. doi: 10.1007/s11010-008-9746-0
Sánchez, M., Gómez, C., Avendaño, C., Harmsen, I., Ortiz, D., Ceballos, R., et al. (2021). House fly (Musca domestica) larvae meal as an ingredient with high nutritional value: microencapsulation and improvement of organoleptic characteristics. Food Res. Int. 145, 25–32. doi: 10.1016/j.foodres.2021.110423
Santos, O. J. F., Passos de Carvalho, J., Bruno de Sousa, R. F. X., and Madalena, S. M. (1976). The nutritional value of four species of insects consumed in Angola. Ecol. Food Nutr. 5, 91–97. doi: 10.1080/03670244.1976.9990450
Shantibala, T., Lokeshwari, R. K., and Debaraj, H. (2014). Nutritional and anti-nutritional composition of the five species of aquatic edible insects consumed in Manipur. India. J. Insect Sci. 14:14. doi: 10.1093/jis/14.1.14
Sharp, R. G. (2013). A review of the applications of chitin and its derivatives in agriculture to modify plant-microbial interactions and improve crop yields. Agron. 3, 757–793. doi: 10.3390/agronomy3040757
Singh, A., and Kumari, K. (2019). An inclusive approach for organic waste treatment and valorisation using black soldier Fly larvae: a review. J. Env. Man. 251:13. doi: 10.1016/j.jenvman.2019.109569
Skotnicka, M., Karwowska, K., Kłobukowski, F., Borkowska, A., and Pieszko, M. (2021). Possibilities of the development of edible insect-based foods in Europe. Food Secur. 10:766. doi: 10.3390/foods10040766
Sogari, G., Dagevos, H., Amato, M., Taufik, D., Scaffardi, L., and Formici, G. (2022). Consumer perceptions and acceptance of insects as feed and food: current findings and future outlook. L. Scaffardi and G. Formici (eds), Novel foods and edible insects in the European Union: An interdisciplinary analysis. Springer International Publishing, Cham, 147–169.
Sogari, G., Riccioli, F., Moruzzo, R., Menozzi, D., Sosa, D. A. T., Li, J., et al. (2023). Engaging in entomophagy: the role of food neophobia and disgust between insect and non-insect eaters. Food Qual. Prefer. 104:104764. doi: 10.1016/j.foodqual.2022.104764
Sponheimer, M., De-Ruiter, D., Lee-Thorp, J., and Späth, A. (2005). Sr/ca and early hominin diets revisited: new data from modern and fossil tooth enamel. J. Human Evol. 48, 147–156. doi: 10.1016/j.jhevol.2004.09.003
Stull, V., and Patz, J. (2020). Research and policy priorities for edible insects. Sustain. Sci. 15, 633–645. doi: 10.1007/s11625-019-00709-5
Stull, V. J., and Weir, T. L. (2023). Chitin and omega-3 fatty acids in edible insects have underexplored benefits for the gut microbiome and human health. Nat. Food 7, 1–5. doi: 10.1038/s43016-023-00728-7
Suryawanshi, R., Kanoujia, J., Parashar, P., and Saraf, S. A. (2020). Sericin: a versatile protein biopolymer with therapeutic significance. Curr. Pharm. Des. 26, 5414–5429. doi: 10.2174/1381612826666200612165253
Tan, H. S. G., Fischer, A. R., Tinchan, P., Stieger, M., Steenbekkers, L. P. A., and Van-Trijp, H. C. (2015). Insects as food: exploring cultural exposure and individual experience as determinants of acceptance. Food Qual. Pref. 42, 78–89. doi: 10.1016/j.foodqual.2015.01.013
Thornton, P., Gurney-Smith, H., and Wollenberg, E. (2023). Alternative sources of protein for food and feed. COSUST 62:101277. doi: 10.1016/j.cosust.2023.101277
Thrastardottir, R., Olafsdottir, H. T., and Thorarinsdottir, R. I. (2021). Yellow mealworm and black soldier fly larvae for feed and food production in europe, with emphasis on Iceland. Food Secur. 10:2744. doi: 10.3390/foods10112744
Tzompa-Sosa, D. A., Moruzzo, R., Mancini, S., Schouteten, J. J., Liu, A., Li, J., et al. (2023). Consumers’ acceptance toward whole and processed mealworms: a cross-country study in Belgium, China, Italy, Mexico, and the US. PLoS One 18:e0279530. doi: 10.1371/journal.pone.0279530
Ukhun, M. E., and Osasona, M. A. (1985). Aspects of the nutritional chemistry of Macrotermes bellicosus. Nutr. Rep. Int. 32, 1121–1130.
Van Huis, A., Halloran, A., Van Itterbeeck, J., Klunder, H., and Vantomme, P. (2022). How many people on our planet eat insects: 2 billion? J. Insects Food Feed 8, 1–4. doi: 10.3920/JIFF2021.x010
Van-Huis, A. (2013). Potential of insects as food and feed in assuring food security. Annu. Rev. Entomol. 58, 563–583. doi: 10.1146/annurev-ento-120811-153704
Van-Huis, A., Rumpold, B., Maya, C., and Roos, N. (2021). Nutritional qualities and enhancement of edible insects. Annu. Rev. Nut. 41, 551–576. doi: 10.1146/annurev-nutr-041520-010856
Varelas, V. (2019). Food wastes as a potential new source for edible insect mass production for food and feed: a review. Ferment. 5, 81–87. doi: 10.3390/fermentation5030081
Wang, C., Ghadimi, P., Lim, M. K., and Tseng, M.-L. (2019). A literature review of sustainable consumption and production: a comparative analysis in developed and developing economies. J. Clean. Prod. 206, 741–754. doi: 10.1016/j.jclepro.2018.09.17
Wang, Y.-S., and Shelomi, M. (2017). Review of black soldier Fly (Hermetia illucens) as animal feed and human food. Food Secur. 6:e91. doi: 10.3390/foods6100091
Wang, A., Zheng, Y., Zhu, W., Yang, L., and Yang, Y. (2022). Melittin-based nano-delivery systems for cancer therapy. Biomol. Ther. 12, 118–132. doi: 10.3390/biom12010118
Wanjiku, E. K. (2018). Physicochemical and microbiological stability of semi-processed edible crickets (Acheta domesticus) and black soldier fly larvae (Hermetia illucens) during storage. Doctoral dissertion : JKUAT-Agriculture.
Wantulla, M., van Zadelhoff, K., van Loon, J. J., and Dicke, M. (2023). The potential of soil amendment with insect exuviae and frass to control the cabbage root fly. J. Appl. Entomol. 147, 181–191. doi: 10.1111/jen.13097
Womeni, H. M., Linder, M., Tiencheu, B., Mbiapo, F. T., Villeneuve, P., Fanni, J., et al. (2009). Oils of Oryctes owariensis and Homorocoryphus nitidulus consumed in Cameroon: sources of linoleic acid. J. Food Technol. 7, 54–58.
Wu, N., Wang, X., Mao, Z., Liang, J., Liu, X., and Xu, X. (2022). Bioconversion of chicken meat and bone meal by black soldier fly larvae: effects of straw addition on the quality and microbial profile of larval frass. J. Env. Man. 307:114579. doi: 10.1016/j.jenvman.2022.114579
Xiao, X., Jin, P., Zheng, L., Cai, M., Yu, Z., Yu, J., et al. (2018). Effects of black soldier fly (Hermetia illucens) larvae meal protein as a fishmeal replacement on the growth and immune index of yellow catfish (Pelteobagrus fulvidraco). Aquaculture Res. 49, 1569–1577. doi: 10.1111/are.13611
Ye, X., Liu, X., Luo, X., Sun, F., Qin, C., Ding, L., et al. (2023). Characterization of the molecular diversity and degranulation activity of mastoparan family peptides from wasp venoms. Toxins 15:331. doi: 10.3390/toxins15050331
Yen, A. L. (2009). Edible insects: traditional knowledge or western phobia? Entomol. Res. 39, 289–298. doi: 10.1111/j.1748-5967.2009.00239.x
Ying, F., Xiaoming, C., Long, S., and Zhiyong, C. (2010). Common edible wasps in Yunnan Province, China and their nutritional value. Forest insects as food: Human bite Back. P. B. Durst, D. V. Johnson, R. N. Leslie, and K. Shono (Eds.), Food and Agriculture Organization of the United Nations Regional Office for Asia and the Pacific: Bangkok, Thailand. 93–98
Keywords: food security, nutrition, Caribbean, list, distribution
Citation: Granados-Echegoyen C, Vásquez-López A, Calderón-Cortés N, Gallego-Ocampo HL, Gómez-Rodríguez CH, Rodríguez-Vélez JM, Sarmiento-Cordero MA, Salamanca-Canizales LJ, Rodríguez-Vélez B, Arroyo-Balán F and Andrade-Hoyos P (2024) Brief overview of edible insects: exploring consumption and promising sustainable uses in Latin America. Front. Sustain. Food Syst. 8:1385081. doi: 10.3389/fsufs.2024.1385081
Received: 11 February 2024; Accepted: 15 May 2024;
Published: 03 June 2024.
Edited by:
Rakesh Bhardwaj, Indian Council of Agricultural Research (ICAR), IndiaReviewed by:
Lenka Kourimska, Czech University of Life Sciences Prague, CzechiaCopyright © 2024 Granados-Echegoyen, Vásquez-López, Calderón-Cortés, Gallego-Ocampo, Gómez-Rodríguez, Rodríguez-Vélez, Sarmiento-Cordero, Salamanca-Canizales, Rodríguez-Vélez, Arroyo-Balán and Andrade-Hoyos. This is an open-access article distributed under the terms of the Creative Commons Attribution License (CC BY). The use, distribution or reproduction in other forums is permitted, provided the original author(s) and the copyright owner(s) are credited and that the original publication in this journal is cited, in accordance with accepted academic practice. No use, distribution or reproduction is permitted which does not comply with these terms.
*Correspondence: Carlos Granados-Echegoyen, Z3JhbmFkb3MuZWNoZWdveWVuQHlhaG9vLmNvbQ==; Alfonso Vásquez-López, YXZhc3F1ZXpAaXBuLm14
Disclaimer: All claims expressed in this article are solely those of the authors and do not necessarily represent those of their affiliated organizations, or those of the publisher, the editors and the reviewers. Any product that may be evaluated in this article or claim that may be made by its manufacturer is not guaranteed or endorsed by the publisher.
Research integrity at Frontiers
Learn more about the work of our research integrity team to safeguard the quality of each article we publish.