- 1Agricultural Innovation and Technology Transfer Center (AITTC), College of Agriculture and Environmental Sciences (CAES), University Mohammed VI Polytechnic (UM6P), Ben Guerir, Morocco
- 2Research Institute of Organic Agriculture (FIBL), Frick, Switzerland
- 3Centre Régional de la Recherche Agronomique de Settat (CRRA-Settat), Institut National de la Recherche Agronomique, Settat, Morocco
- 4Département de Production, Protection et Biotechnologie Végétales, Institut Agronomique et Vétérinaire Hassan II (IAV Hassan II), Rabat, Morocco
- 5Centre Régional de la Recherche Agronomique de Rabat (CRRA-Rabat), Institut National de la Recherche Agronomique, Rabat, Morocco
- 6Société L’Ouest Marocain, Meknes, Morocco
Conservation Agriculture is a farming system based on no mechanical soil disturbance, permanent soil cover, and crop diversification. A study was carried out in an on-farm field trial set up in Meknes (Morocco) under a long-term no-till (NT) system to evaluate the residual effect of one-time occasional tillage (OT) on crop performance, soil water, and water-use efficiency (WUE) one and two years after OT implementation. Shallow and deep options of OT were compared with common NT practices (with crop residue retention and with crop residue removal) for two consecutive seasons of 2021–2022 (year 1) and 2022–2023 (year 2). The four tillage practices were implemented in November 2020. Three crops were studied each year: durum wheat (Triticum durum), faba bean (Vicia faba minor), and chickpea (Cicer arietinum) all grown under NT in both the years and arranged in four crop rotations. Our findings show that grain yield of wheat and chickpea was negatively affected by OT for all years considered. In wheat, there was a grain yield loss of 18 and 20% for shallow and deep OT, respectively compared to NT with crop residue retention. In chickpea, the grain yield loss was as high as 47 and 49% for shallow and deep OT, respectively. Average soil water storage measured at 0–60 cm at sowing was also lower in deep OT (133 mm) compared to NT with crop residue retention (151 mm) for all years and rotations considered. Yet, in wheat year 1, deep OT slightly improved soil water content at 30 cm depth compared to NT treatments. The comparison of WUE between treatments showed that, under NT with crop residue retention, the crops produced more grain and aboveground biomass per mm of water. Wheat/faba bean rotation had a greater grain yield and WUE (all years considered) and overall greater soil water content (year 1), compared to the wheat/chickpea rotation. The results suggest that the effects of OT on crop performance and water productivity in the short term can be adverse. On the other hand, grain yield of wheat can be improved by a judicious choice of legume to be used as a preceding crop.
1 Introduction
Conservation Agriculture’s (CA) three principles of no mechanical soil disturbance, permanent soil cover, and crop diversification are increasingly promoted in Africa (Kassam et al., 2022). NT is a major component of CA and often the only CA principle that is consistently applied. Extensive stubble grazing and monoculture grain production annihilate the chances of stubble retention and diverse rotations in North Africa. Regardless, CA is still a crucial climate change adoption strategy for the Mediterranean region particularly due to its advantages in soil water use efficiency resulting from greater water capture and storage (Mrabet, 2011). In response, the Moroccan government initiated the Green Generation strategy (2020–2030) where one million hectares of cropland is projected to be converted to CA by 2030 (Devkota et al., 2022). Morocco has a rich experience in NT farming since the introduction of this technology in the 1980s (Diop et al., 2022).
Despite the increased frequency of droughts in North Africa during the last decades causing yield losses (Karrou and Oweis, 2014), the adoption of CA is still marginal in North Africa (Cicek et al., 2023). Long-term NT systems may present several constraints such as weed proliferation and the development of herbicide-resistant weed species, an increased incidence of soil- and crop residues-borne diseases, subsoil compaction, and nutrient and soil organic matter (SOM) stratification in the topsoil (Dang et al., 2015a,b). Occasional tillage (OT) in NT systems, known also as strategic tillage (Dang et al., 2020), is intended to address these different constraints. Single-tillage-based OT is suggested as an adaptation strategy within CA systems to maintain the advantages of continuous NT and lessen its negative impacts (Crawford et al., 2018; Liu et al., 2020).
There are limited studies regarding the effect of OT on crop performance in the short and long terms (Stavi et al., 2011; Crawford et al., 2018). The effect of OT on crop performance varies according to soil type, tillage implements (depth and frequency) used and climatic conditions (Liu et al., 2016). Depending on soil type and the nature of NT constraints to be overcome, OT might be shallow or deep cultivation (Hall et al., 2020). Most studies investigating the effects of OT used chisel (depth ≤ 40 cm), plow/harrow (depth ≤ 30 cm) and subsoiler (depth > 40 cm) (Peixoto et al., 2020).
In Mediterranean rainfed cropping systems, crop performance highly depends on the rainfall received during the growing season but also on the soil’s capacity to retain water (Plaza-Bonilla et al., 2017). Although there are studies conducted in France (e.g., Cordeau et al., 2020), Spain (e.g., López-Garrido et al., 2011) and Türkiye (e.g., Çelik et al., 2019) on OT, to our knowledge, no researcher investigated the effect of OT on crop productivity and soil quality including water dynamics in North Africa. In drylands, a number of mechanisms, including high evaporation, high runoff, poor infiltration, and low SOM, limit soil water availability to crops (Liniger et al., 2011). This could lower the production of biomass and grain. Hence, it is important to evaluate how OT affects soil water content and WUE. In water-limited areas, OT could lower yields if it decreases the amount of soil water available to plants through increased evaporation (Crawford et al., 2015). Blanco-Canqui and Wortmann (2020) reported that OT does not generally decrease soil water content. Water loss due to OT will obviously depend on the amount of water stored in the soil during the OT implementation. In dryland cropping systems, crop residues management is crucial in managing water capture and reducing water evaporation (Mrabet, 2008). However, grazing of crop residues is considered a major issue in North Africa where stubble is consumed by sheep and goats after harvest (Pala et al., 2000).
Furthermore, OT can lead to increased yield through an improvement of soil physical properties, including the alleviation of soil compaction, reduction of bulk density and increased total porosity (Díaz-Zorita et al., 2004; Liu et al., 2020). Not only does grazing crop residues limit soil cover in NT systems, it can also cause soil compaction, especially when carried out at high stocking densities and on wet soil (Rakkar and Blanco-Canqui, 2018). In the Mediterranean context, the risk of soil compaction caused by grazing may be low when grazing is carried out during the summer (the dry season, from end of June to end of September) but can be significant when it is carried out after the summer storms or the first rains (October–November) before sowing, especially when these rains are fairly heavy, due to wet soils. Hence, OT could be a relevant practice and area of research in North African areas affected by soil compaction problems due to grazing in NT conditions. Through the mixing and redistribution of soil nutrients within the root zone and increased mineralization of crop residues, OT can also improve nutrient availability and uptake, hence increasing yields (Crawford et al., 2015; Blanco-Canqui and Wortmann, 2020).
Beyond the lack of studies on the effects of OT on crop performance in North Africa, few studies have dealt with the residual effects of OT on soil water status in the short term (1–2 years after OT implementation) in long-term NT systems worldwide. In addition, there are research gaps worldwide regarding the effects of OT on WUE after OT implementation. The present study aims to investigate the residual effects of OT on crop performance, soil water storage, and WUE in a long-term (10 years) NT system. We hypothesized that OT would improve soil water storage and result in better crop yield and WUE compared to NT practices. The results of this study will provide the first evidence on OT’s short-term residual effect on crop productivity and soil water status in North Africa and help farmers to make informed decisions on the use of OT under challenging circumstances.
2 Materials and methods
2.1 Study area
The study area was located in the region of Meknes, North-east of Morocco situated at 33°72 N, 5°69 W, and 702 m altitude. The OT was done in November 2020 and crop and soil water monitoring were performed during two consecutive crop growing seasons (from November/December to June/July): 2021–2022 (year 1) and 2022–2023 (year 2). The experimental site has a semi-arid and Mediterranean climate with wet winters and hot- and dry- summers. The trial was conducted on a leveled flat field and soil was clayey in nature classified as a luvisol according to the World Reference Base for Soil Resources (IUSS Working Group WRB, 2022). Data regarding soil characterization at the trial implementation, including the content of clay, silt, and sand, pH, EC, SOM, and the levels of nitrogen (N), phosphorus (P), potassium (K), magnesium (Mg), and calcium (Ca) is presented in Table 1. The meteorological data (monthly temperature and rainfall) of the trial site during these two growing seasons are presented in Figure 1. Total rainfall received from October to July during the 1st and 2nd years were 327.5 mm and 316.5 mm, respectively.
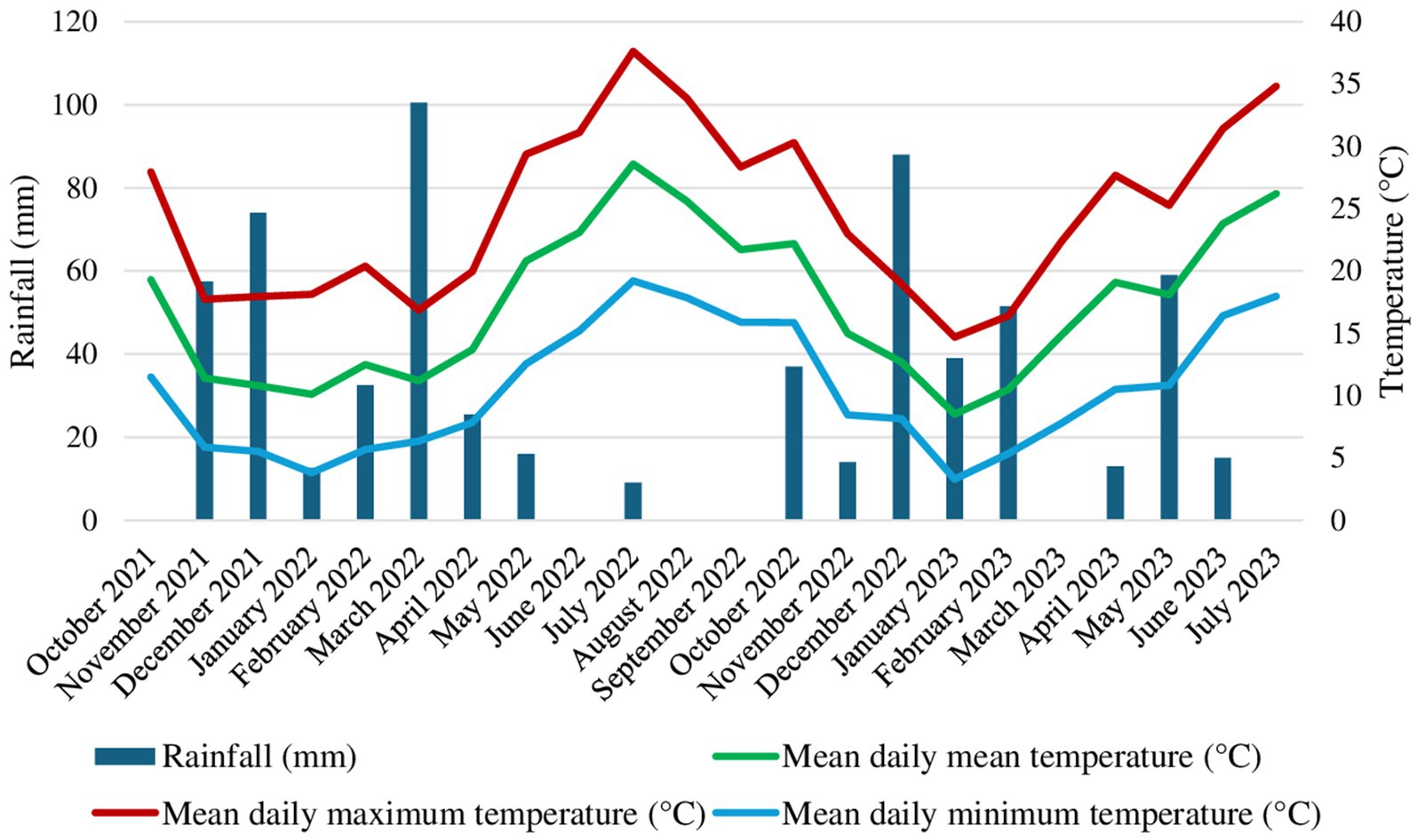
Figure 1. Meteorological data (rainfall and temperature) of the study area in the growing seasons 2021–2022 (year 1) and 2022–2023 (year 2).
2.2 Experimental details
The trial included four tillage treatments applied once in November 2020 in a 10-year continuous no-till field: continuous NT with crop residues initially (during trial set up) maintained (NT + residue); continuous NT with crop residues initially not maintained (NT-residue); shallow inversion tillage (1st OT option, depth: 10 cm) with an offset disk harrow (shallow OT); deep non-inversion tillage (2nd OT option, depth: 25 cm) with a chisel (deep OT). The names of treatments NT + residues, NT-residues, shallow OT, and deep OT refer to the tillage and residue management practices involved when the treatments are implemented in November 2020. Shallow and deep OT were free of crop residues during their implementation. All tillage treatments were monitored in 2021–2022 (year 1) and 2022–2023 (year 2) growing seasons. Three crops were investigated: durum wheat (the main crop of interest in this study), faba bean and chickpea all grown under NT and through four rotations in both the years, i.e., wheat grown after faba bean (wheat/faba bean), wheat sown after chickpea (wheat/chickpea), faba bean sown after wheat (faba bean/wheat) and chickpea sown after wheat (chickpea/wheat). The experiment was conducted in a split-plot design with crop rotations in the main plots and tillage methods in the subplots, with 3 replications. The dimensions of the experimental units (plots) of the trial were: 15 m × 36 m.
Historically, the field on which the trial is set up has been managed under NT since 2010 with biennial cereal-legume rotations. Prior to 2010, it was conducted in conventional tillage. At the time of the installation of the trial, the land was homogeneous for all the crops and treatments. The implementation of OT treatments and the residue management in continuous NT treatments (NT + residue and NT-residue) were carried out two days before sowing in the 2020–2021 crop growing season. The crop grown in the field trial in 2019–2020 season was faba bean and its residues after harvest were used in treatment NT + residue (1.5 t ha−1). Even though tillage treatments were applied only once in November 2020, their effects were monitored during 2021–2022 (year 1) and 2022–2023 (year 2) growing seasons to test the assumption of their residual effect on crop performance, soil water, and WUE. Each year, after harvest, 80% of the harvested crop residues were exported and the remaining 20% were left in the field to imitate the stubble grazing practices common across the region (i.e., all tillage treatments were similar for both tillage and residues management practices in year 1 and year 2) within each crop. Details on crop management in year 1 and year 2 can be found in Supplementary Table 1. The dates of appearance of the phenological stages of the three crops studied during the two years of study are summarized in Supplementary Table 2.
2.3 Measurements
2.3.1 Soil water
Soil water was assessed by two methods: the gravimetric method and by capacitive probes (Delta-T probes type PR2/4) method which measures volumetric soil water content (SWC). Gravimetric SWC (% w/w), in different treatments, was measured for four soil layers: 0–15, 15–30, 30–45, and 45–60 cm each year at sowing and at crop harvest. Soil water storage (SWS, in mm) of each soil layer, was calculated using Equation (1) (Ye et al., 2022):
Bulk density was determined by the core method (Blake and Hartge, 1986). Soil water storage at 0–60 cm was calculated by summing SWS in 0–15, 15–30, 30–45, and 45–60 cm soil layers. Soil water storage at 0–60 cm was used to evaluate SWS at sowing and harvest for both year 1 and year 2.
For measurements of volumetric SWC by Delta-T probes, they were performed in year 1 in wheat plots (wheat/faba bean and wheat/chickpea), at 10, 20, 30, and 40 cm depths and five selected dates, i.e., 38, 54, 69, and 98 DAS. On the other hand, in year 2, we monitored SWC in faba bean (faba bean/wheat) in time intervals of about a week. The choice of faba bean for SWC measurement in year 2 is justified by the willingness to have soil moisture data for at least one of the legumes studied. To measure SWC (% v/v), Delta-T probes were placed inside access tubes placed approximately in the center of each plot. The objective of these SWC measurements throughout the growing season was to evaluate the different tillage treatments in terms of water content in the soil, especially the crop root zone.
2.3.2 Crop performance
Crop performance was evaluated through yield and yield components in year 1 and year 2. For all crops, grain yield (GY), total (aboveground) biomass yield (TBY), and thousand grain weight (TGW) were evaluated at crop harvest, from three quadrats of 1 m2 in each plot. For wheat, we also determined the number of spikes (NSpk) m−2. Straw yield (SY) was calculated as the difference between TBY and GY and harvest index (HI, in %) was calculated using Equation (2).
2.3.3 Water-use and water-use efficiency
Water-use efficiency for grain (GWUE) and total biomass (TBWUE) was calculated during both the years. Water-use efficiency was expressed in kg ha−1 mm−1 and calculated using GY and TBY data and crop evapotranspiration (water-use) through the following formulas:
Water-use (WU) was calculated from the soil water balance formula evaluated during the growing season (from sowing to harvest):
Where GSR is the growing season rainfall (mm); SWSS is the soil water storage up to 60 cm at sowing (mm); WU is water-use (mm); SWSH is the soil water storage up to 60 cm at harvest (mm); R is runoff (mm); D is drainage (mm); CR is the capillarity rise (mm). CR and D were taken to be zero because the experimental site had a relatively flat and deep soil layer, as assumed by Devkota et al. (2022). Furthermore, R was considered negligible due to the flatness of the study site. Then, Equation (5) can be simplified as:
2.4 Statistical analysis of data
All variables under study were subject to analysis of variance (ANOVA), and means were compared by Sidak’s test. All statistical analysis was done using R software (R version 4.2.1.) (R. Core Team, 2017). The lme (linear mixed effect) function of the package nlme was used to determine the effects of tillage, crop rotation, and year on (i) yield and yield components of wheat for the rotations wheat/faba bean and wheat/chickpea and (ii) SWS (mm) at sowing and harvest, WU, and WUE for all four rotations studied. The same function was used to determine the effect of tillage and rotation on SWC (%, v/v) measured at different dates in wheat (wheat/faba bean and wheat/chickpea) in year 1. In addition, lm (linear model) function was used to study the effect of tillage and year on the yield and yield components of faba bean (faba bean/wheat) and chickpea (chickpea/wheat). The significance level of all statistical tests was set at 0.05. To explain the (significant) variability of GY between tillage treatments and/or rotations, a linear regression analysis was done between GY and SWS for each crop and all years.
3 Results
3.1 Soil water at sowing and harvest as affected by tillage, crop rotation, and year
The effects of tillage, rotation, and year on SWSS and SWSH are presented in Figures 2, 3, and the corresponding ANOVA results are summarized in Supplementary Table 3. Tillage had a significant effect on SWSS measured at 30–45 and 0–60 cm soil depth (Figure 2A), and onSWSH measured at 15–30, 45–60, and 0–60 cm depth (Figure 3A). Crop rotation significantly affected SWSS measured on all soil layers studied (Figure 2B), whereas for SWSH, only at 45–60 cm was not significantly affected by rotation (Figure 3B). Year had a significant effect on SWSS measured on all soil layers studied except 45–60 cm (Figure 2C), while it had no significant effect on SWSH (Figure 3C).
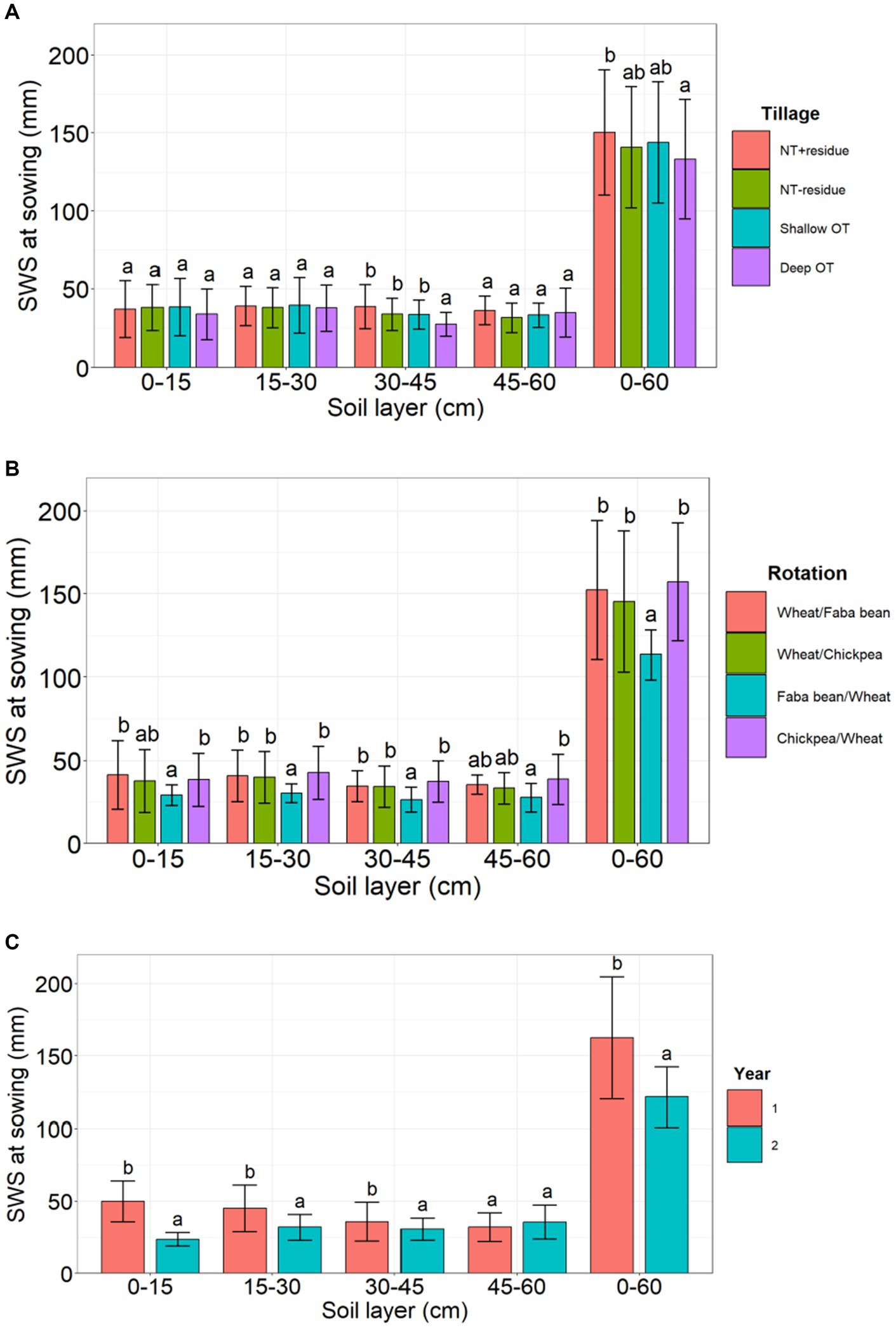
Figure 2. Soil water storage (SWS) at sowing as affected by tillage (A), rotation (B), and year (C) in wheat, faba bean and chickpea. Within the same subgraph (A–C), lower-case letters indicate if means are significantly different (different letters) or similar (at least one letter in common) according to Sidak’s test. Error bars represent standard deviation.
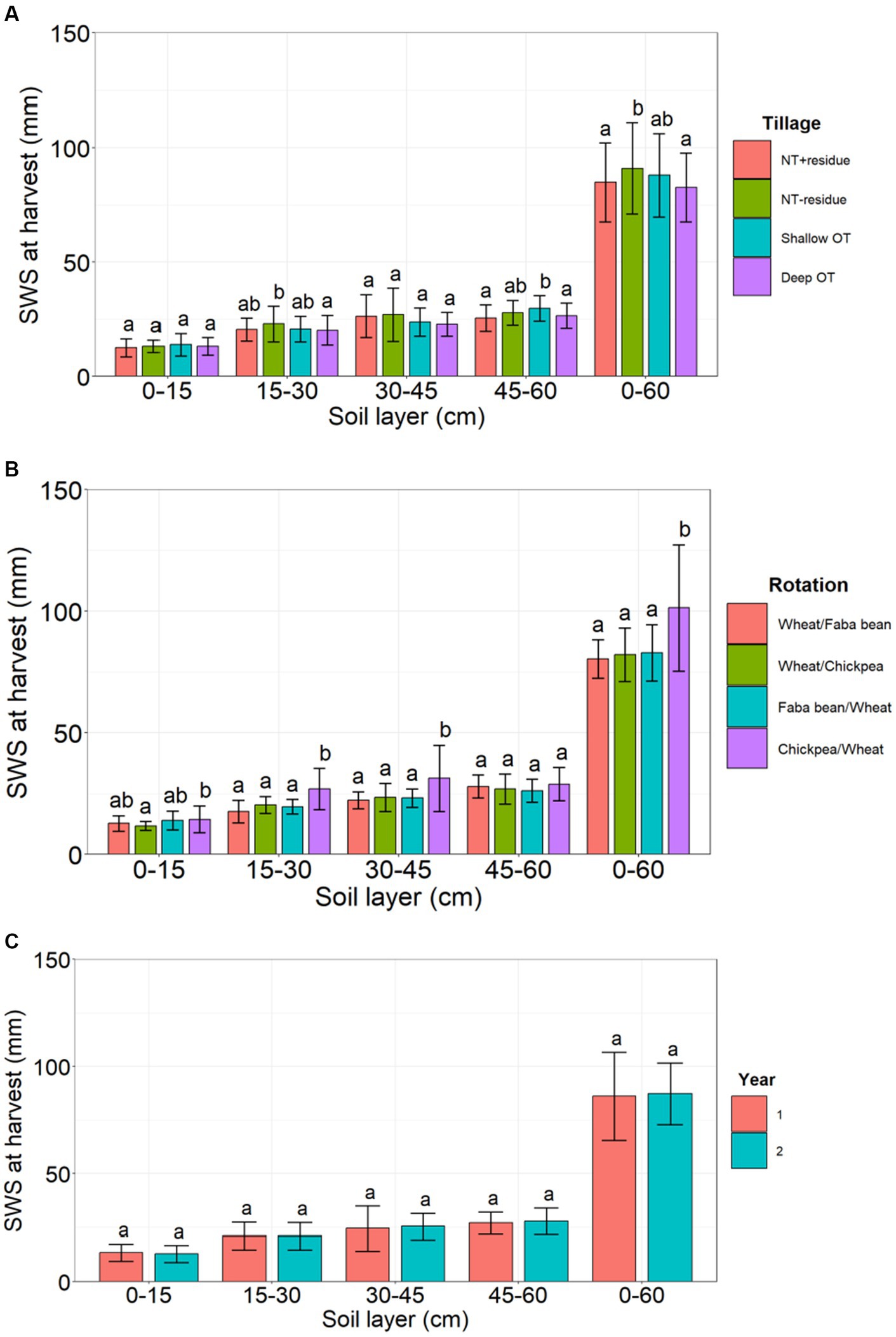
Figure 3. Soil water storage (SWS) at harvest as affected by tillage (A), rotation (B), and year (C) in wheat, faba bean and chickpea. Within the same subgraph (A–C) and soil layer, lower-case letters indicate if means are significantly different (different letters) or similar (at least one letter in common) according to Sidak’s test. Error bars represent standard deviation.
At 30–45 cm soil depth, SWSS was significantly lower under deep OT (27.3 mm) compared with NT + residue (38.5 mm), NT-residue (33.7 mm), and shallow OT (33.4 mm) (Figure 2A). At 0–60 cm depth, deep OT (133.3 mm) had a significantly lower SWSS value than NT + residue (150.5 mm), while shallow OT and NT-residue had intermediate values between those of NT + residue and shallow OT (Figure 2A). Regarding the effect of rotation, faba bean/wheat generally had lower SWSS values than wheat/faba bean, wheat/chickpea and chickpea/wheat in most of the soil layers studied (Figure 2B). Finally, SWSS values measured at 0–15, 15–30, 30–45, and 0–60 cm were higher in year 1 than in year 2 (Figure 2C).
Soil water storage at harvest was significantly higher under NT-residue compared to NT + residue and deep OT at 0–60 cm soil depth compared to deep OT at the 45–60 cm depth (Figure 3A). At 45–60 cm, shallow OT recorded a higher SWSH than NT + residue and deep OT (Figure 3A). On the other hand, SWSH was significantly higher under chickpea/wheat rotation compared to the other rotations (Figure 3B).
3.2 Soil water content during the growing season in wheat in year 1 as affected by tillage and crop rotation
Details on the dates of moisture readings in wheat in year 1, including the positioning of these dates in relation to rainfall received are provided in Supplementary Table 4. The 1st reading date (Date 1: 38 DAS) follows a rainfall event (date: 36 DAS, rainfall received: 7.6 mm). The 2nd reading date (Date 2: 54 DAS) follows a relatively long period characterized by the absence of a rainfall event (from 37 to 53 DAS). The 3rd reading date (Date 3: 69 DAS) also follows a period of no rainfall (from 58 to 68 DAS) and a low-volume rainfall event (date: 57 DAS, volume: 0.2 mm). Cumulative rainfall hardly varied between Dates 1, 2 and 3. As for the last reading date (Date 4: 98 DAS), although it is close to a rainfall event (96 DAS), the volume of rain received during this event is very low (0.6 mm). However, the cumulative rainfall between Dates 3 and 4 is 40.6 mm.
In wheat in year 1, the tillage methods as well as the rotation modalities (wheat/faba bean vs. wheat/chickpea) were not significantly different in terms of SWC at 10, 20 and 40 cm soil depths for all the measurement dates (Supplementary Table 5). Figures 4, 5 respectively show the effects of tillage and rotation on SWC (% v/v) measured at different dates in wheat in year 1. At 30 cm, the highest SWC values were generally obtained with deep OT, whereas the lowest values were generally obtained with NT-residue. At 38 DAS, no significant differences were recorded between tillage types in terms of SWC measured at 30 cm. At 54 DAS, the SWC at 30 cm was significantly higher under deep OT (29% v/v) compared with NT practices (NT + residue: 18% v/v and NT-residue: 16% v/v). At 69 and 98 DAS, deep OT had a significantly higher SWC value at 30 cm depth (25 and 24% respectively) than NT-residue (12.5 and 12.3% respectively) but statistically similar to NT + residue (14.7 and 15.2% respectively). Similarly, at 98 DAS and 30 cm depth, shallow OT (22.1%) had a significantly higher SWC value than NT-residue but statistically similar to NT + residue.
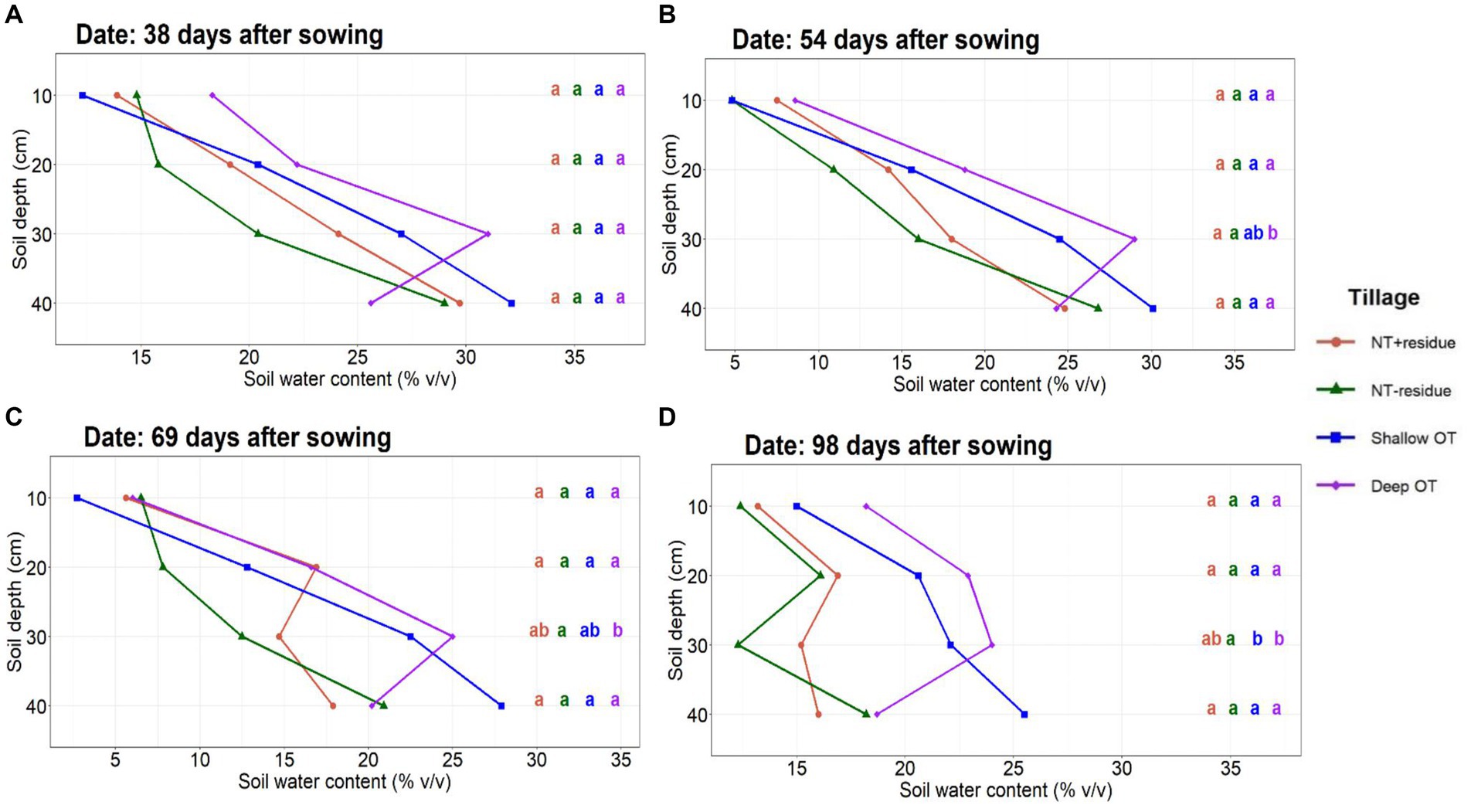
Figure 4. Effects of tillage on soil water content (% v/v) measured at different dates (A) 38 DAS, (B) 54 DAS, (C) 69 DAS, and (D) (98 DAS) in wheat in year 1. Within the same subgraph (i)–(iv) and soil depth, lower-case letters indicate if means are significantly different (different letters) or similar (at least one letter in common) according to Sidak’s test.
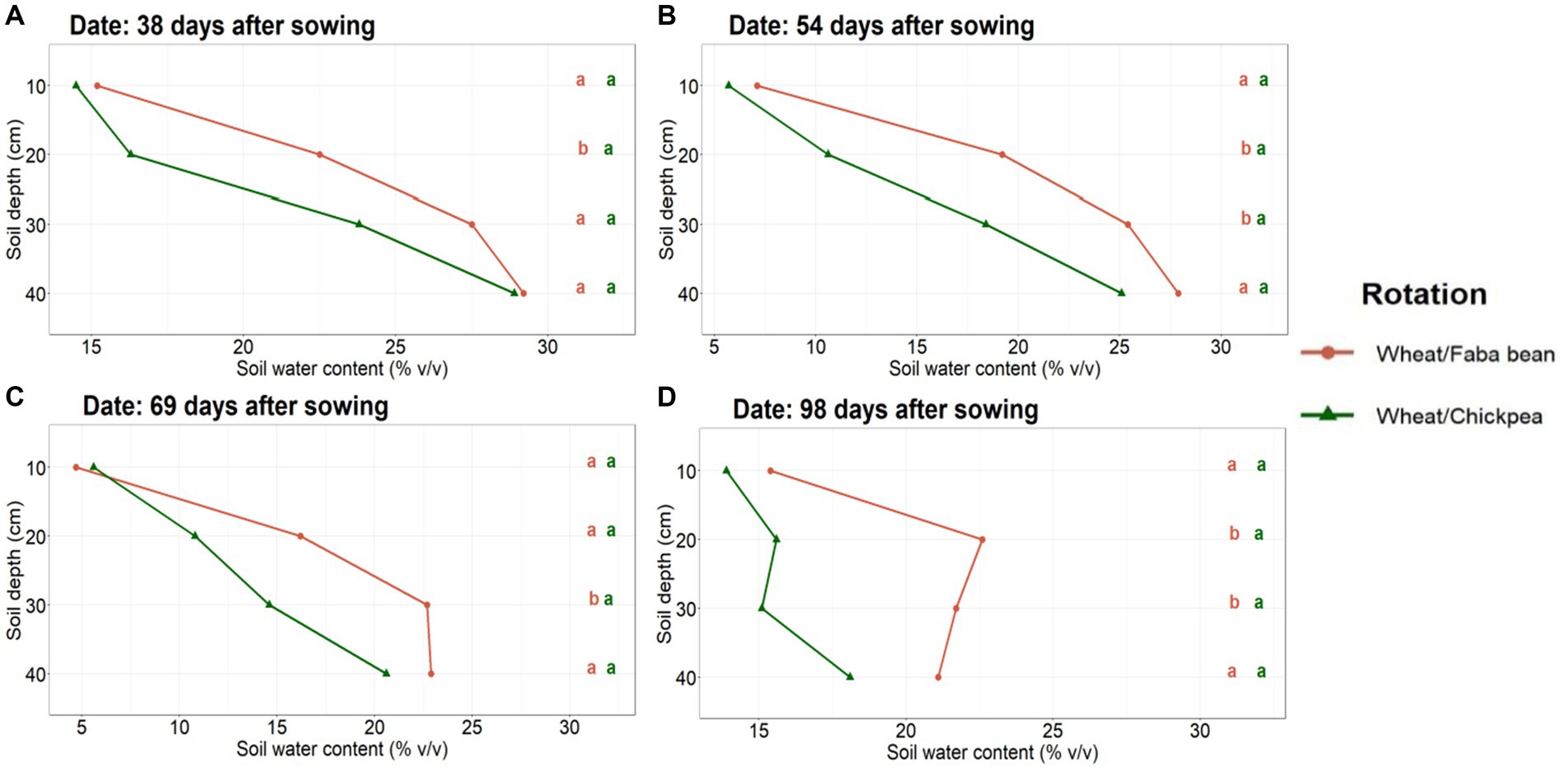
Figure 5. Effects of rotation on soil water content (% v/v) measured at different dates (A) 38 DAS, (B) 54 DAS, (C) 69 DAS, and (D) (98 DAS) in wheat in year 1. Within the same subgraph and soil depth (A–D), lower-case letters indicate if means are significantly different (different letters) or similar (at least one letter in common) according to Sidak’s test.
The effect of crop rotation on SWC measured during the growing season was not significant at 10 and 40 cm depth (Figure 5). At 38, 54, and 98 DAS and 20 cm soil depth, and at 54, 69, and 98 DAS and 30 cm soil depth, SWC was significantly lower with wheat/chickpea bean than with wheat/faba bean.
3.3 Dynamics of soil water during the growing season in the different tillage treatments in faba bean year during 2
The temporal dynamics of SWC at different depths in faba bean during year 2 show a high variability in terms of SWC between tillage methods over the growing season (Figure 6). At 10 cm soil depth (Figure 6A), the highest SWC values were generally obtained with NT + residue (especially at the beginning of the growing season, at 45, 52, 59, 67, and 73 DAS, then for the rest of the season at 87, 199 and 207 DAS) and shallow OT (at 110, 115, 130, 136, 187, and 192 DAS). At 20 cm soil depth (Figure 6), NT-residue had lower SWC values than the other tillage treatments during the first SWC measurements (45, 52, 59, 73, 80, and 87 DAS). In addition, at 20 cm depth, NT + residue had higher SWC values than the other tillage methods at 115, 122, and 130 DAS (the period covering flowering in faba bean, which took place at 121 DAS) but also at 87 and 178 DAS. Between flowering (121 DAS) and maturity (143 DAS) of faba bean, soil moisture at 20 cm was generally higher with NT + residue or NT-residue.
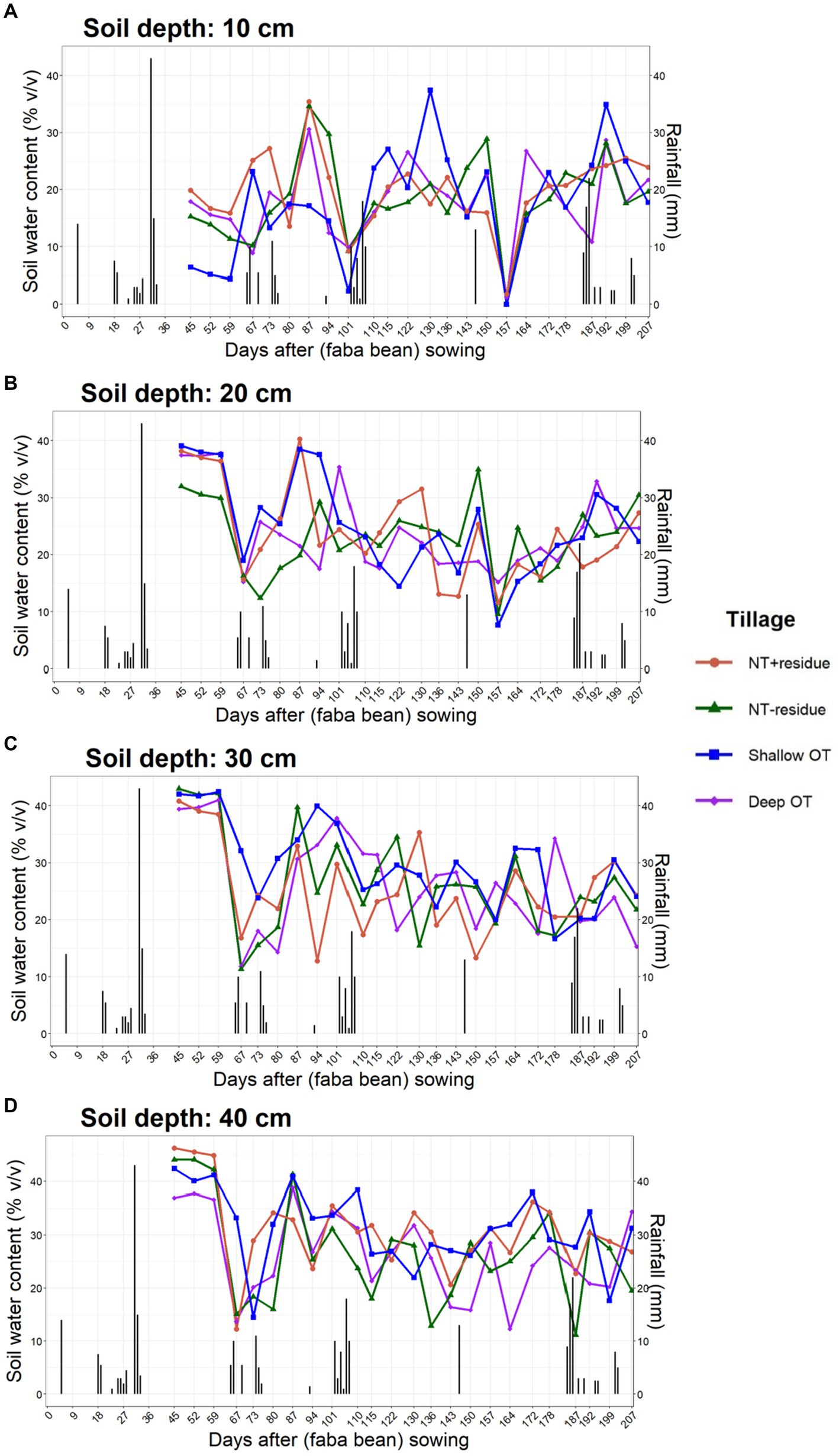
Figure 6. Dynamics of soil water content (% v/v) in different tillage modes in faba bean in year 2 (A) at 10 cm soil depth, (B) at 20 cm soil depth, (C) at 30 cm soil depth, and (D) at 10 cm soil depth. Vertical bars represent daily rainfall.
At 30 cm soil depth (Figure 6C), NT + residue had the lowest SWC values at 94, 101, 110, 115, 136, 143, and 150 DAS. At the same depth, deep OT had the lowest SWC values at 45, 80, 87, 172, 199, and 207 DAS. At 40 cm soil depth (Figure 6D), the lowest SWC values were generally noted under shallow OT (at 45, 52, 59, 143, 150, 164, 172, 178, and 192 DAS) and NT-residue (at 80, 101, 110, 115, 136, 187, and 207 DAS).
3.4 Effects of one-time occasional tillage on crop performance
3.4.1 Yield and yield components of wheat as affected by tillage, crop rotation, and year
In wheat, GY and HI were the only crop performance parameters significantly (p ≤ 0.05) affected by tillage methods (Table 2). Both of these variables were significantly higher under NT + residue compared with OT practices (shallow and deep OT). Crop rotation significantly affected yield and yield components except for the NSpk and HI. Wheat sown after faba bean recorded higher GY, TGW, TBY, and SY than wheat sown after chickpea. Finally, except for HI, the yield and yield components were significantly higher in year 1 compared with year 2. GY reduction in year 2 compared to year 1 was 42%.
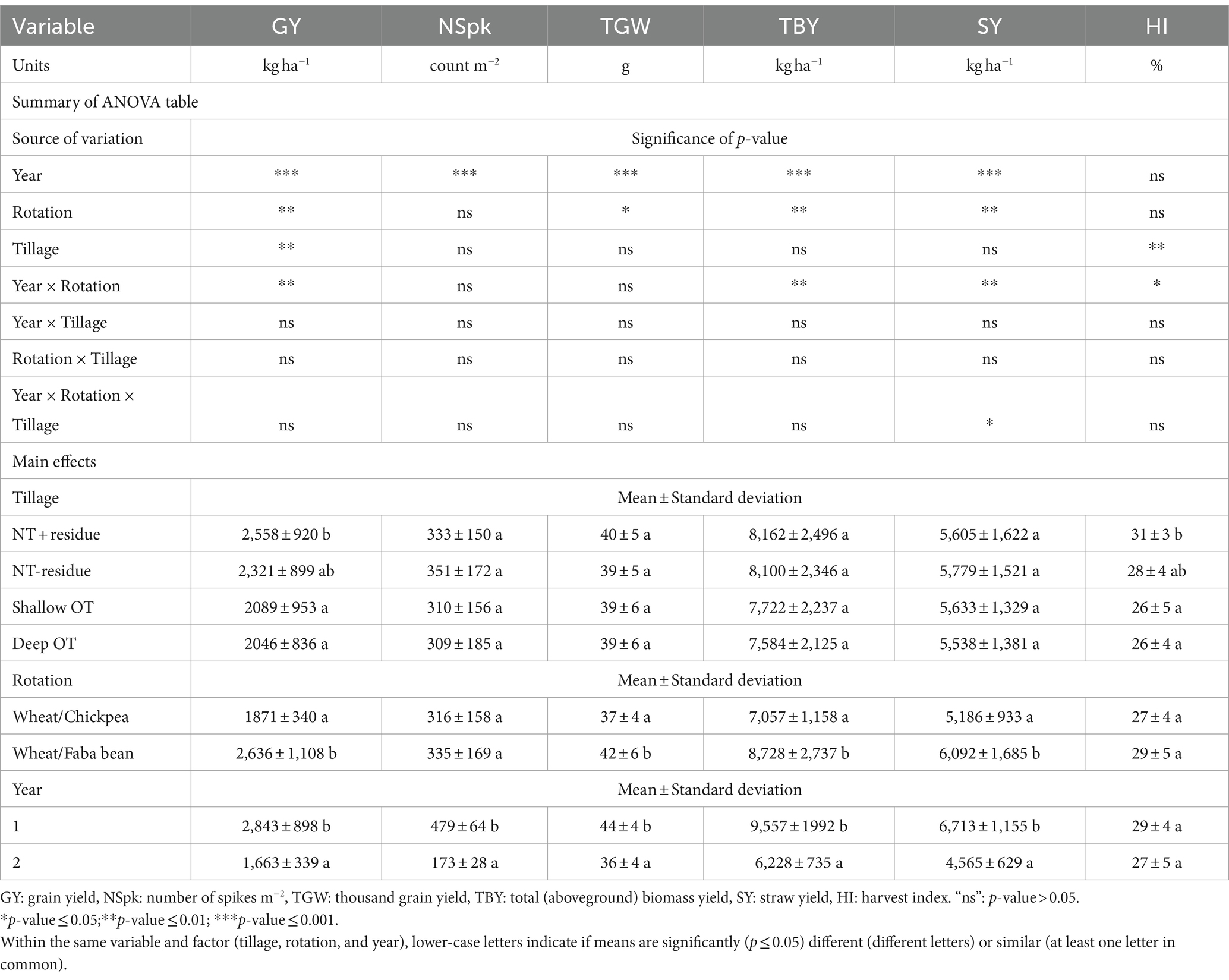
Table 2. Significance levels from ANOVA test and means for yield and yield components of wheat as function of tillage, rotation, and year.
3.4.2 Yield and yield components of faba bean and chickpea as affected by tillage and year
Yield and yield components of faba bean (faba bean/wheat) and chickpea (chickpea/wheat) in the different tillage modes are presented in Table 3. In faba bean, tillage had no significant effect on yield and yield components. However, NT + residue recorded slightly higher values of GY, TBY, SY, and HI compared with NT-residue, shallow and deep OT. On the other hand, GY and TBY values were slightly higher under the NT-residue treatment compared to shallow and deep OT. Finally, all yield and yield components were significantly higher in year 1 compared to year 2. GY of faba bean in year 2 was drastically reduced by 77% compared to year 1.
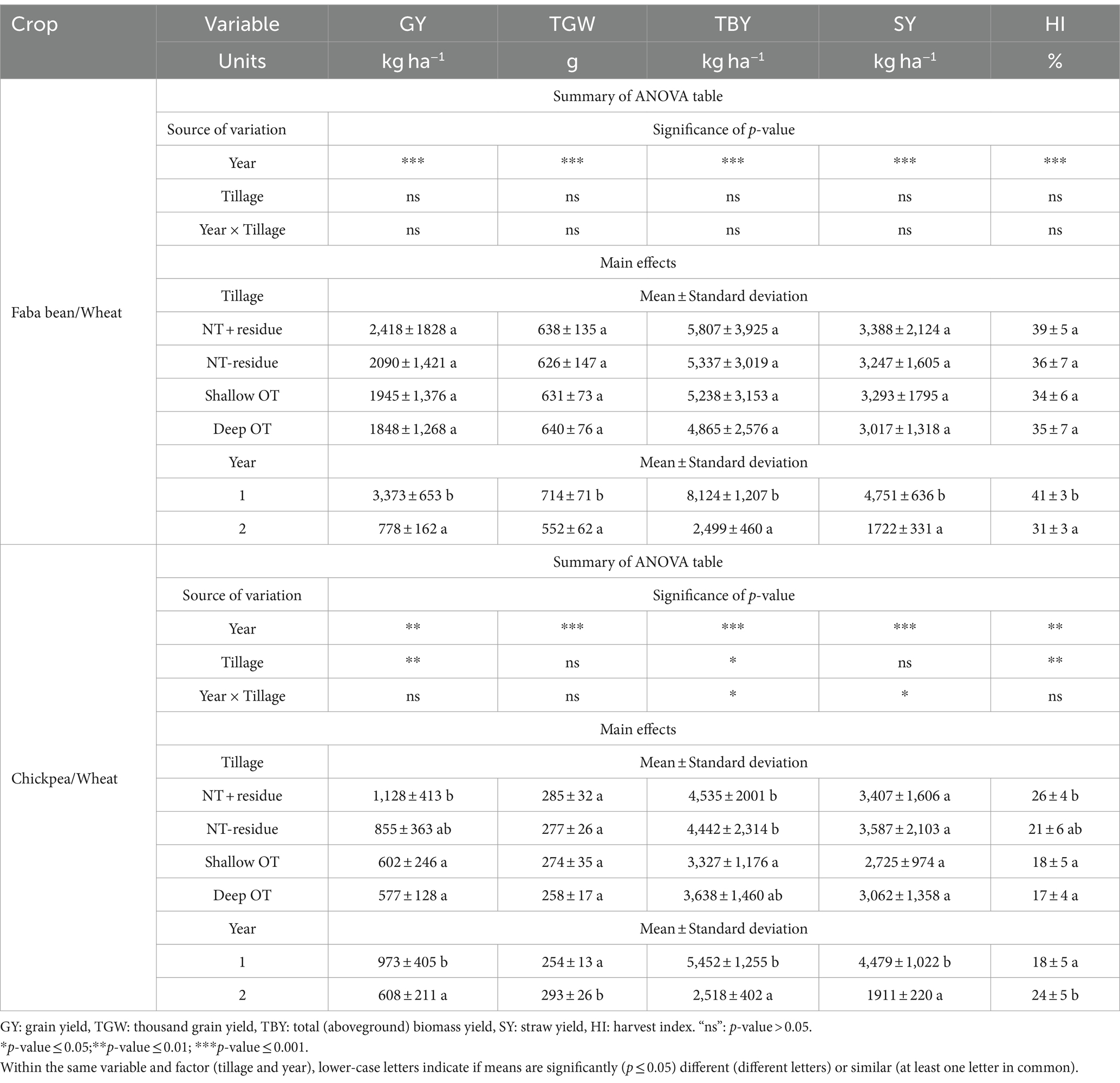
Table 3. Significance levels from ANOVA test and means for yield and yield components of faba bean and chickpea wheat as function of tillage and year.
In chickpea, yield and yield components were generally higher under the NT modalities (NT + residue and NT-residue) compared to the OT methods. In particular, NT + residue had significantly higher GY, TBY and HI values than shallow OT and deep OT. Regarding the effect of year, GY, TBY, and SY were significantly higher in year 1 compared with year 2, while TGW and HI were higher in year 2 than in year 1. Compared with year 1, in year 2, GY of chickpea was reduced by 38%.
3.5 Water-use and water-use efficiency as affected by tillage, crop rotation, and year
Water-use (WU) was not significantly affected by tillage, despite slightly higher means for shallow OT and deep OT (Figure 7A). Regarding the effect of crop rotation, WU was significantly lower in faba bean/wheat compared to wheat/faba bean, wheat/chickpea, and chickpea/wheat rotations (Figure 7B). In addition, WU was significantly higher in year 1 (337.4 mm) compared to year 2 (243.5 mm) (data not shown). Water-use efficiency for grain (GWUE) and total biomass (TBWUE) were significantly affected by tillage, crop rotation, and year (Supplementary Table 6). Regarding the effect of tillage on WUE, NT + residue had significantly higher GWUE and TBWUE values than shallow and deep OT (Figure 8A). The chickpea/wheat rotation had significantly lower GWUE and TBWUE values than the other rotations (Figure 8B). In wheat, wheat/faba bean produced more grain and biomass per mm of water than wheat/chickpea (Figure 8B). Finally, GWUE and TBWUE were significantly higher in year 1 (8 and 25 kg ha−1 mm−1, respectively) compared to year 2 (5 and 18 kg ha−1 mm−1, respectively) (data not shown).
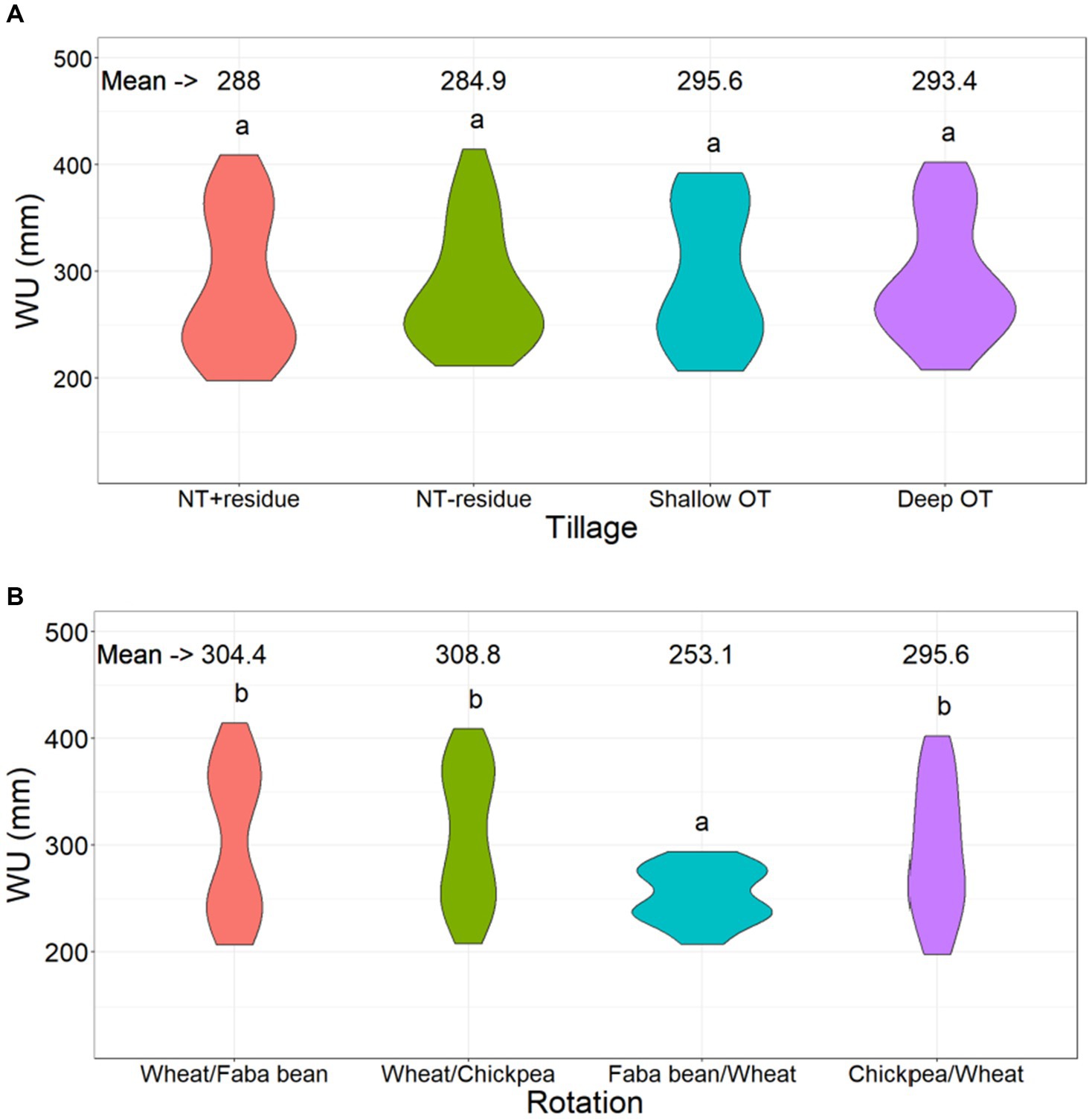
Figure 7. Water use (WU) as affected by tillage (A) and rotation (B) in wheat, faba bean and chickpea. Within the same subgraph (A,B), lower-case letters indicate if means are significantly different (different letters) or similar (at least one letter in common) according to Sidak’s test.
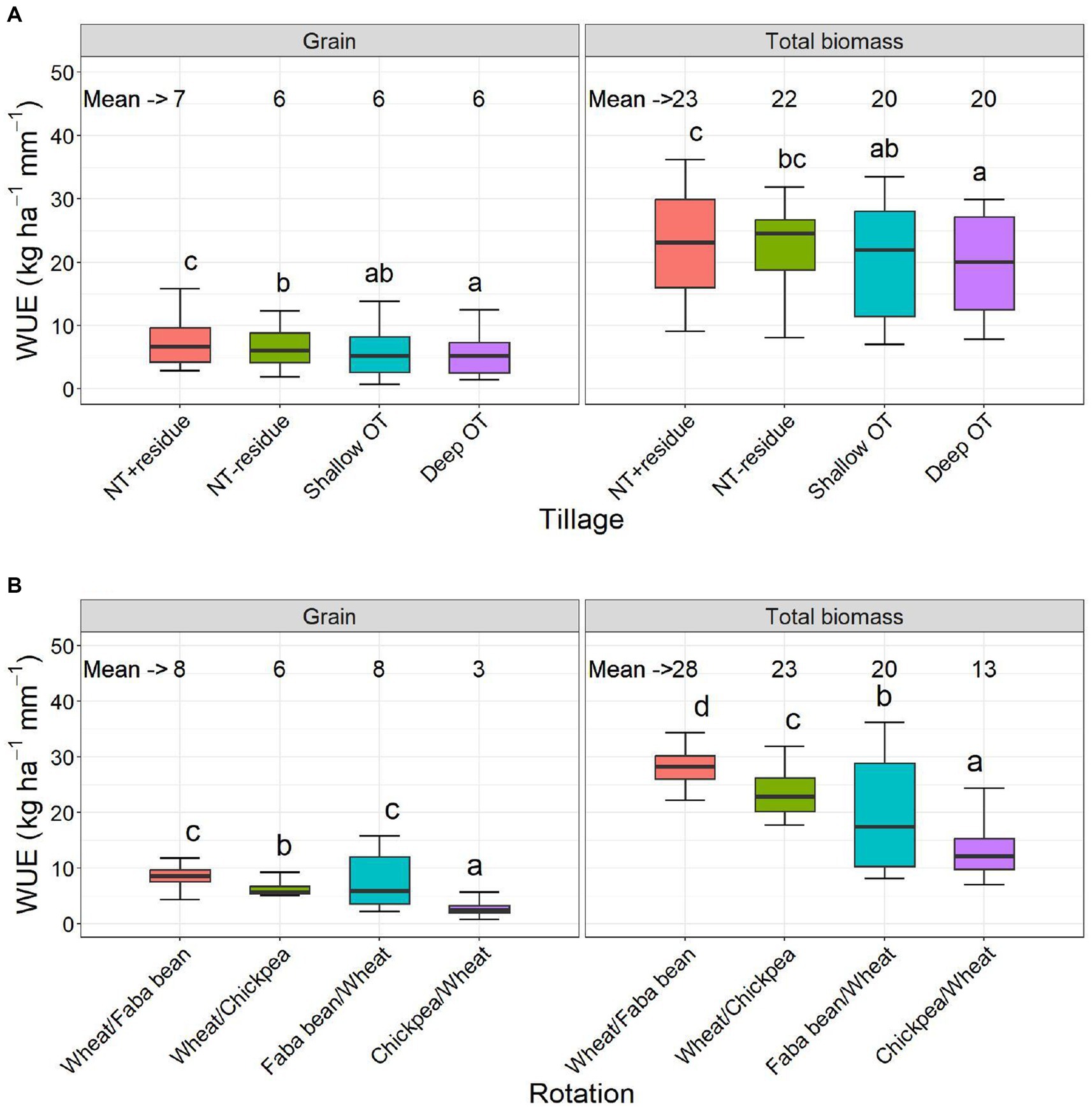
Figure 8. Water use efficiency (WUE) of grain and total (aboveground) biomass as affected by tillage (A) and rotation (B) in wheat, faba bean and chickpea. Within the same subgraph (A,B) and plant component (grain or total biomass), lower-case letters indicate if means are significantly different (different letters) or similar (at least one letter in common) according to Sidak’s test.
4 Discussion
The higher SWSS in year 1 compared to year 2 (Figure 2C) can be explained by the fact that the cumulative rainfall recorded from October (start of the first rains in general) until the time of sowing was markedly higher in year 1 (57.5 mm) than in year 2 (37 mm), despite no rain in October in year 1 and earlier rain in year 2 compared to year 1 (Figure 1). The highest SWSS (all rotations and years considered) in the soil profile (0–60 cm depth) was achieved by NT + residue while NT-residue recorded the highest SWSH (Figures 2A, 3A). A greater SWSS at 0–60 cm in NT + residue compared to deep OT could be attributed to a greater SWSS at 30–45 cm in NT + residue given that the two tillage treatments were not significantly different for SWSS at 0–15 and 15–30 cm. High SWS in NT compared to tillage can be the result of an increased infiltration which itself could be attributable to a more stable structure resulting from a more continuous pore network (Giambalvo et al., 2012). Soil’s ability to store water depends on soil pore distribution and continuity, aggregate stability and initial soil water content, which all are affected by tillage (Azooz and Arshad, 1998; Zhang et al., 2017). As observed by Lampurlanés et al. (2001) in a study under Mediterranean conditions (Spain), NT contributes to a higher and deeper water storage in the soil profile. Despite that tillage generally increases soil porosity, it destroys pore continuity, which can lead to a lower infiltration of water compared to NT (Azooz and Arshad, 1998). Tillage increases soil macroporosity over a short duration while disrupting the continuity of macro- and micropores (Shukla et al., 2003). Occasional tillage is reported to cause soil disturbance and alter surface-connected macropores (Blanco-Canqui and Wortmann, 2020).
Volumetric SWC measured by probes at 10, 20, 30, and 40 cm soil depth do not necessarily reflect the full amount of soil water available to the crop, but it allows a comparison of tillage practices at specific dates. Regarding volumetric SWC in wheat (wheat/faba bean and wheat/chickpea) in year 1, which were generally slightly higher in deep OT at 30 cm as compared to continuous NT treatments (Figure 4), treatment deep OT may have broken the pre-existing compacted soil layer and increased soil macroporosity, which improved the infiltration rate of rainwater. This may in turn contribute to soil moisture increase at 30 cm depth under deep OT. Occasional tillage can increase water infiltration through increased macroporosity (>24 mm pore radii) of the tilled zone (Dang et al., 2015a; Blanco-Canqui and Wortmann, 2020). It also contributes to breaking soil hardness (Dang et al., 2020). Our results on the performance of deep OT in terms of SWC (% v/v) at 30 cm depth in wheat year 1 are consistent with those found by Crawford et al. (2014) who found at Biloela in Australia that soil moisture recorded was significantly (p ≤ 0.05) increased in the 10–20 cm depth between the 3- and 12-month period for the chisel treatments. Although in our study the SWC measured at 30 cm in wheat in year 1 shows the superiority of deep OT over both NT + residue and NT-residue at 54 DAS and over NT-residue at 69 and 98 DAS, the SWC at 30 cm depth measured at these dates may have had a low impact on crop performance. In addition, we can hardly say that deep OT allowed a better soil water conservation in the short term when we consider the dynamics of SWC in faba bean in year 2 with a greater number of measurement dates (Figure 6). Despite the great variability between tillage types in terms of SWC in faba bean in year 2, the treatment that gave overall higher soil moisture at 10 and 20 cm, respectively at the start of crop growth and during the flowering-maturing period (critical phases for water availability) was NT + residue.
Our overall results for the three crops studied indicate a yield loss in OT treatments (shallow and deep OT) compared with NT + residue (Tables 2, 3). A review performed by Blanco-Canqui and Wortmann (2020) on the global effects of OT revealed that crop yields increased in 15% of the cases, decreased in 5% of the cases, and remained the same in 80% of situations. Dang et al. (2015a) reported that OT contributes to increasing crop yields in NT systems in the short term and our results are different from their results. However, our findings are consistent with those of Çelik et al. (2019) who found that under Mediterranean climate (Türkiye) yield of rainfed winter wheat with one-time moldboard-based OT was lower compared to NT. In a field experiment in the United States, Díaz-Zorita et al. (2004) found that OT improved winter wheat yields, mostly under low-yielding conditions, but it resulted in lower subsequent summer crop yields (soybean and maize) compared to continuous NT. They attributed the differences in maize yields between NT and OT to a higher water supply in NT soil through the maintenance of a higher number of mesopores and a great hydraulic conductivity.
Higher overall yield in NT methods in our study could be attributed to the absence of soil disturbance in NT, which maintains soil structure and improves the water conservation. The performance of NT + residue in improving yield, which was marked in wheat and chickpea could be attributed to higher SWSS at 0–60 cm in this treatment (Figure 2A). The linear regression between GY (y) and SWSS (x) was significantly (p ≤ 0.05) positive in wheat (y = 93 + 15x, r = 0.68, both rotations wheat/faba bean and wheat/chickpea considered), and chickpea (y = 0.12 + 5x, r = 0.49). In rainfed agriculture, SWSS is a key parameter that can significantly affect crop productivity. Maximizing SWSS is a strategy for managing water availability for crops (Aboudrare et al., 2006) and buffers the long drought period, giving the crop more chance to survive and catch the next rainfall event (El Mejahed, 1993).
In addition, wheat, faba bean and chickpea yields in NT + residue was slightly higher than yield obtained in NT-residue (Tables 2, 3). NT + residue may have benefited from the positive effect of the residues of the previous crop maintained on the soil surface at the trial establishment. The faba bean residues kept on the soil surface in treatment NT + residue at the beginning of the trial may have played a role in improving soil N fertility and crop nutrient uptake (mainly in year 1) through the decomposition of the faba bean residues which have a low C/N ratio (Truong and Marschner, 2020). Etemadi et al. (2018) have observed that NT with faba bean residues maintained on soil surface recorded higher corn ear yield as compared to NT without faba bean residues. Beyond providing plant nutrients, maintaining crop residues on the soil surface has many other benefits for soil quality, especially in the topsoil. It contributes to enhancing SOM (i.e., improvement of soil structure), water retention, and aggregate stability and protecting the soil against raindrop impact and erosion (Mulumba and Lal, 2008). In semiarid north-central Morocco, Mrabet (2002) found that NT with residue cover outperformed bare NT in terms of average GY.
The absence of a significant effect of OT on faba bean yield (Table 3) is consistent with the results obtained by Crawford et al. (2018) who found that OT, including chisel, offset disc and prickle/disc chain, had an insignificant effect on yield (barley, chickpea, sorghum, and wheat) in the Northern Grains Region in Australia. Under Mediterranean climate (Spain), López-Garrido et al. (2011) found no significant difference between long-term NT and OT using moldboard + disk harrowing in terms of wheat GY, TGW and HI. Furthermore, in the United States, Schlegel et al. (2020) found no significant effect between a single OT with a sweep plow and continuous NT on crop yield and yield components. In Brazil, Fidalski et al. (2015) found that OT with plowing and harrowing had an insignificant effect on GY of maize, soybean, and black oats.
Not only NT + residue recorded overall a better crop performance but also had higher GWUE and TBWUE compared to OT treatments in all crop rotations (Figure 8A). However, WU was slightly higher in OT practices (Figure 7A). This may be due to higher soil water loss through evaporation in OT compared to NT treatments. High WUE in NT is generally the result of decreased soil evaporation and increased infiltration, which is mainly favored by the maintenance of crop residues on the soil surface (Bahri et al., 2019). Other factors linked to soil quality may explain the variability in WUE between tillage treatments in our study. Improved soil structure can contribute to a high crop WUE in NT (Radford and Thornton, 2011). A high soil aggregation, which is common in NT systems (Mrabet, 2002), is frequently associated with an increase in root growth, soil water infiltration, and WUE (Paye et al., 2023). In the short term, tillage can cause a decline in soil structure, soil surface crusting, and soil macroporosity, consequently amplifying water loss through evaporation, and low crop productivity (Mrabet, 2008). A higher root length density under NT can lead to a better WUE through enhancement of water uptake by the crop (Cantero-Martínez et al., 2007). Lampurlanés et al. (2001) observed a greater root growth in NT compared to tillage practices, not only on the soil surface but also in the lower layers. This could be due to increased soil moisture or to a higher soil strength that limits the elongation of root main axes while stimulating branching. In NT conditions, crop roots can grow into biopores created by root channels of previous crops or earthworms (López and Arrúe, 1997). This can hardly be performed in tilled soil given that tillage contributes to breaking preexisting biopores.
Regarding the impact of crop rotation, the preceding crop had a significant impact on crop yield and its components in wheat. The better performance of wheat cultivated after faba bean (wheat/faba bean) compared to wheat grown after chickpea (wheat/chickpea) (Table 2) may be due to higher weed pressure in wheat/chickpea than in wheat/faba bean, which may have favored a higher weed evapotranspiration in wheat/chickpea compared to wheat/faba bean. This may explain the overall lower SWC observed in wheat/chickpea compared to wheat/faba bean in year 1 (Figure 5). In Mediterranean conditions, it has been found that faba bean has a higher competitive ability against weeds than chickpea, which may be attributable to the plant’s higher height and more vigorous early growth, which contribute to a superior shading capacity and, as a result, weed suppression (Frenda et al., 2013). The higher performance of wheat/faba bean compared to wheat/chickpea could also be attributed to the effect of the preceding crop on soil fertility, specifically nitrogen fixation, and soil physical properties, such as soil structuration and infiltration. Faba bean has been reported to have a higher dependence on N2 fixation for growth, fix more N, and substantially use less soil N than chickpea under the same soil N supply (Turpin et al., 2002). The higher GY in wheat/faba bean compared to wheat/chickpea is compatible with the results obtained by López-Bellido and López-Bellido (2001) who found under Mediterranean conditions (Spain) that the wheat/faba bean rotation was more effective than the wheat/chickpea rotation (as well as the other rotations tested) in improving wheat GY. In our study, higher GWUE and TBWUE in wheat/faba bean compared to wheat/chickpea (Figure 8B) could be explained by higher GY and TBY in wheat/faba bean compared to wheat/chickpea given that WU was not significantly different between these two rotations (Figure 7B).
As for higher yield for wheat, faba bean and chickpea in year 1 compared to year 2, it could be mainly attributed to a higher amount and better distribution through the growing season of rainfall in year 1 (Figure 1). The drastic reduction of faba bean grain yield in year 2 compared to year 1 (Table 3) is mainly the effect of drought (no rainfall) in March 2023 (Figure 1), which corresponds to the flowering and grain maturing periods of faba bean in year 2. In year 1 (2021–2022), no month recorded zero rainfall during the growing season (November–June). The development of seed kernels is directly impacted by drought stress during the reproductive processes with the shortening of the grain-filling and ripening periods (Dietz et al., 2021). Faba bean is reported to be more sensitive to drought than chickpea and other grain legumes like common bean and pea (Khan et al., 2007).
The relatively high temperatures in April 2023 (mean daily maximum temperature of 27.7°C) were identified as another possible cause of the severe yield decline in faba bean in year 2 as compared to year 1. Both chickpea and faba bean are cool-season legumes whose grain yield can be significantly affected by heat stress, especially during the reproductive phase (flowering and seed set) (Saxena et al., 1988). Seed filling of legumes is negatively impacted by heat stress (Sita et al., 2017). However, faba bean is reported to be highly sensitive to heat stress, and significant yield loss is observed when daily temperatures >25°C (Alharbi and Adhikari, 2020). Faba bean is less heat-tolerant than chickpea, whose seed yields are drastically reduced when it is exposed to the critical temperature of 35°C and above at flowering and podding (Gaur et al., 2014).
In summary, the present study demonstrated the performance of continuous NT in terms of SWS and crop yield compared to OT in two years of monitoring. The positive effects of OT on crop performance reported in the literature were not found in our study. This raises the uncertainty regarding the adoption of OT in long-term NT in Morocco, which becomes more challenging given the few studies conducted on OT in Mediterranean conditions and the lack of studies on OT in similar environments in North Africa. However, in Morocco, many studies have evaluated crop performance and WUE in NT as compared to tillage with disk harrow and chisel. For instance, in semiarid north-central Morocco, Mrabet (2000) found a yield increase in NT as compared to offset-disk harrowing while yields under chisel tillage were not significantly different from NT. In Morocco, Bouzza (1990) found that WUE of wheat grain was increased by 13% in NT as compared to chisel tillage (Mrabet, 2011). In the North-East of Morocco, in a newly established two-year field experiment, Wafae et al. (2023) found a higher NSpk, TGW, GY GWUE for wheat in NT as compared to chisel tillage in the second year of experiment.
5 Conclusion and perspectives
Our study shows that deep (non-inversion) OT overall caused a GY loss among the three crops compared to NT + residue. Deep OT, slightly improved soil moisture at 30 cm in wheat in year 1 compared to continuous NT practices but NT + residue had higher SWSS at 0–60 cm depth, which translated in higher GY in NT + resdue for wheat and chickpea. Both shallow (inversion) and deep (non-inversion) OT recorded lower GWUE and TBWUE compared to NT + residue. Furthermore, in wheat, the wheat/faba bean rotation contributed to higher crop performance, GWUE and TBWUE, an overall higher SWC in year 1 than the wheat/chickpea rotation.
Our results indicate that in drylands where water is the main factor limiting crop performance, the effects of OT on GY and soil WUE can be detrimental. This means that the application of OT in NT systems must be guided not only by the identification of the NT constraints that justify the use of OT, but also by the availability of water (i.e., the climatic context). This raises the question of whether OT might not be better suited to NT systems in relatively more well-watered regions.
Data availability statement
The raw data supporting the conclusions of this article will be made available by the authors, without undue reservation.
Author contributions
MD: Methodology, Software, Visualization, Writing – original draft, Writing – review & editing, Investigation. ABe: Investigation, Methodology, Resources, Supervision, Writing – review & editing, Validation. HC: Conceptualization, Funding acquisition, Investigation, Methodology, Project administration, Resources, Supervision, Writing – review & editing, Validation. HO: Conceptualization, Investigation, Methodology, Resources, Supervision, Writing – review & editing, Validation. ABa: Conceptualization, Investigation, Methodology, Supervision, Writing – review & editing, Resources, Validation. OE: Writing – review & editing, Conceptualization, Investigation, Methodology, Resources, Supervision. RD: Writing – review & editing, Conceptualization, Investigation, Methodology, Resources, Supervision. AZ: Writing – review & editing, Conceptualization, Investigation, Methodology, Resources. ME: Writing – review & editing, Funding acquisition, Resources, Supervision. KE: Writing – review & editing, Funding acquisition, Methodology, Resources, Supervision, Validation.
Funding
The author(s) declare that financial support was received for the research, authorship, and/or publication of this article. This research was funded by the Agricultural Innovation and Technology Transfer Center (AITTC), University Mohammed VI Polytechnic (UM6P) and the ConServeTerra project (a Research and Innovation project financed by PRIMA (prima-med.org) under Grant No 1913).
Acknowledgments
The authors would like to acknowledge the “Société L’Ouest Marocain,” the “AGENDA” (Agriculture, Environment and Development for the Avenir) research team and the AITTC researchers and staff.
Conflict of interest
The authors declare that the research was conducted in the absence of any commercial or financial relationships that could be construed as a potential conflict of interest.
Publisher's note
All claims expressed in this article are solely those of the authors and do not necessarily represent those of their affiliated organizations, or those of the publisher, the editors and the reviewers. Any product that may be evaluated in this article, or claim that may be made by its manufacturer, is not guaranteed or endorsed by the publisher.
Supplementary material
The Supplementary material for this article can be found online at: https://www.frontiersin.org/articles/10.3389/fsufs.2024.1375666/full#supplementary-material
References
Aboudrare, A., Debaeke, P., Bouaziz, A., and Chekli, H. (2006). Effects of soil tillage and fallow management on soil water storage and sunflower production in a semi-arid Mediterranean climate. Agric. Water Manag. 83, 183–196. doi: 10.1016/j.agwat.2005.12.001
Alharbi, N. H., and Adhikari, K. N. (2020). Factors of yield determination in faba bean (Vicia faba). Crop Pasture Sci. 71, 305–321. doi: 10.1071/CP19103
Azooz, R. H., and Arshad, M. A. (1998). Effect of tillage and residue management on barley and canola growth and water use efficiency. Can. J. Soil Sci. 78, 649–656. doi: 10.4141/S97-098
Bahri, H., Annabi, M., Cheikh M’Hamed, H., and Frija, A. (2019). Assessing the long-term impact of conservation agriculture on wheat-based systems in Tunisia using APSIM simulations under a climate change context. Sci. Total Environ. 692, 1223–1233. doi: 10.1016/j.scitotenv.2019.07.307
Blake, G. R., and Hartge, K. H. (1986). “Bulk density” in Methods of soil analysis. Part I: Physical and mineralogical method. ed. A. Klute (Madison: American Society of Agronomy, Inc. Soil Science Society of America, Inc).
Blanco-Canqui, H., and Wortmann, C. S. (2020). Does occasional tillage undo the ecosystem services gained with no-till? A review. Soil Tillage Res. 198, 104534–104512. doi: 10.1016/j.still.2019.104534
Bouzza, A. (1990). Water conservation in wheat rotations under several management and tillage systems in semiarid areas. Lincoln, Nebraska, USA: University of Nebraska.
Cantero-Martínez, C., Angás, P., and Lampurlanés, J. (2007). Long-term yield and water use efficiency under various tillage systems in Mediterranean rainfed conditions. Ann. Appl. Biol. 150, 293–305. doi: 10.1111/j.1744-7348.2007.00142.x
Çelik, İ., Günal, H., Acar, M., Acir, N., Bereket Barut, Z., and Budak, M. (2019). Strategic tillage may sustain the benefits of long-term no-till in a vertisol under Mediterranean climate. Soil Tillage Res. 185, 17–28. doi: 10.1016/j.still.2018.08.015
Cicek, H., Topp, E., Plieninger, T., Blanco-Moreno, J. M., Gultekin, I., Mohamed, H. C., et al. (2023). A critical assessment of conservation agriculture among smallholders in the Mediterranean region: adoption pathways inspired by agroecological principles. Agron. Sustain. Dev. 43, 1–17. doi: 10.1007/s13593-023-00926-4
Cordeau, S., Baudron, A., and Adeux, G. (2020). Is tillage a suitable option for weed management in conservation agriculture? Agronomy 10, 1–14. doi: 10.3390/agronomy10111746
Crawford, M. H., Bell, K., Kodur, S., and Dang, Y. (2018). The influence of tillage frequency on crop productivity in sub-tropical to semi-arid climates. J. Crop. Sci. Biotechnol. 21, 13–22. doi: 10.1007/s12892-017-0044-0
Crawford, M., Dang, Y., Balzer, A., Rincon-Florez, V., and Carvalhais, L. (2014). “Strategic tillage in conservation farming systems; its impact on soil health and productivity” in Soil change matters, international workshop 24–27 march 2014, Bendigo, Victoria, Australia (East Melbourne, Australia: Department of Environment and Primary Industries), 33–38.
Crawford, M. H., Rincon-Florez, V., Balzer, A., Dang, Y. P., Carvalhais, L. C., Liu, H., et al. (2015). Changes in the soil quality attributes of continuous no-till farming systems following a strategic tillage. Soil Res. 53, 263–273. doi: 10.1071/SR14216
Dang, Y. P., Dalal, R. C., and Menzies, N. W. (2020). No-till farming Systems for Sustainable Agriculture: Challenges and opportunities. Cham, Switzerland: Springer.
Dang, Y. P., Moody, P. W., Bell, M. J., Seymour, N. P., Dalal, R. C., Freebairn, D. M., et al. (2015a). Strategic tillage in no-till farming systems in Australia’s northern grains-growing regions: II. Implications for agronomy, soil and environment. Soil Tillage Res. 152, 115–123. doi: 10.1016/j.still.2014.12.013
Dang, Y. P., Seymour, N. P., Walker, S. R., Bell, M. J., and Freebairn, D. M. (2015b). Strategic tillage in no-till farming systems in Australia’s northern grains-growing regions: I. Drivers and implementation. Soil Tillage Res. 152, 104–114. doi: 10.1016/j.still.2015.03.009
Devkota, M., Devkota, K. P., and Kumar, S. (2022). Conservation agriculture improves agronomic, economic, and soil fertility indicators for a clay soil in a rainfed Mediterranean climate in Morocco. Agric. Syst. 201, 103470–103414. doi: 10.1016/j.agsy.2022.103470
Díaz-Zorita, M., Grove, J. H., Murdock, L., Herbeck, J., and Perfect, E. (2004). Soil structural disturbance effects on crop yields and soil properties in a no-till production system. Agron. J. 96, 1651–1659. doi: 10.2134/agronj2004.1651
Dietz, K. J., Zörb, C., and Geilfus, C. M. (2021). Drought and crop yield. Plant Biol. 23, 881–893. doi: 10.1111/plb.13304
Diop, M., Chirinda, N., Beniaich, A., El Gharous, M., and El Mejahed, K. (2022). Soil and water conservation in Africa: state of play and potential role in tackling soil degradation and building soil health in agricultural lands. Sustain. For. 14, 1–29. doi: 10.3390/su142013425
El Mejahed, K. (1993). Effect of N on yield, N uptake and water use efficiency of wheat in rotation systems under semiarid conditions of Morocco. Lincoln, Nebraska, USA: University of Nebraska.
Etemadi, F., Hashemi, M., Zandvakili, O., Dolatabadian, A., and Sadeghpour, A. (2018). Nitrogen contribution from winter-killed faba bean cover crop to spring-sown sweet corn in conventional and no-till systems. Agron. J. 110, 455–462. doi: 10.2134/agronj2017.08.0501
Fidalski, J., Yagi, R., and Tormena, C. A. (2015). Occasional soil turnover and liming in a clayey oxisol under a consolidated no-tillage system. Rev. Bras. Cienc. do Solo 39, 1483–1489. doi: 10.1590/01000683rbcs20140428
Frenda, A. S., Ruisi, P., Saia, S., Frangipane, B., Di Miceli, G., Amato, G., et al. (2013). The critical period of weed control in Faba bean and chickpea in Mediterranean areas. Weed Sci. 61, 452–459. doi: 10.1614/ws-d-12-00137.1
Gaur, P. M., Jukanti, A. K., Samineni, S., Chaturvedi, S. K., Basu, P. S., Babbar, A., et al. (2014). Climate change and heat stress tolerance in chickpea. Weinheim, Germany: Wiley-VCH Verlag Gmb H & Co. KGaA, 837–855.
Giambalvo, D., Ruisi, P., Saia, S., Di Miceli, G., Frenda, A. S., and Amato, G. (2012). Faba bean grain yield, N2 fixation, and weed infestation in a long-term tillage experiment under rainfed Mediterranean conditions. Plant Soil 360, 215–227. doi: 10.1007/s11104-012-1224-5
Hall, D. J. M., Davies, S. L., Bell, R. W., and Edwards, T. J. (2020). Soil management systems to overcome multiple constraints for dryland crops on deep sands in a water limited environment on the south coast of Western Australia. Agronomy 10, 1–21. doi: 10.3390/agronomy10121881
IUSS Working Group WRB (2022). World Reference Base for soil resources. International soil classification system for naming soils and creating legends for soil maps. 4th Edn. Vienna, Austria: International Union of Soil Sciences (IUSS).
Karrou, M., and Oweis, T. (2014). Assessment of the severity and impact of drought spells on rainfed cereals in Morocco. African J. Agric. Res. 9, 3519–3530. doi: 10.5897/2014.9130
Kassam, A., Friedrich, T., and Derpsch, R. (2022). Successful experiences and lessons from conservation agriculture worldwide. Agronomy 12, 1–19. doi: 10.3390/agronomy12040769
Khan, H. U. R., Link, W., Hocking, T. J., and Stoddard, F. L. (2007). Evaluation of physiological traits for improving drought tolerance in faba bean (Vicia faba L.). Plant Soil 292, 205–217. doi: 10.1007/s11104-007-9217-5
Lampurlanés, J., Angás, P., and Cantero-Martínez, C. (2001). Root growth, soil water content and yield of barley under different tillage systems on two soils in semiarid conditions. F. Crop. Res. 69, 27–40. doi: 10.1016/S0378-4290(00)00130-1
Liniger, H., Mekdaschi Studer, R., Hauert, C., and Gurtner, M. (2011). “Sustainable land Management in Practice: guidelines and best practices for sub-Saharan Africa” in Terr Africa, world overview of conservation approaches and technologies (WOCAT) and food and agriculture Organization of the United Nations (FAO) (Rome, Italy: FAO).
Liu, H., Crawford, M., Carvalhais, L. C., Dang, Y. P., Dennis, P. G., and Schenk, P. M. (2016). Strategic tillage on a Grey Vertosol after fifteen years of no-till management had no short-term impact on soil properties and agronomic productivity. Geoderma 267, 146–155. doi: 10.1016/j.geoderma.2016.01.002
Liu, Q., Kan, Z., He, C., and Zhang, H. (2020). Effects of strategic tillage on soil physicochemical properties and grain yield in the North China plain. Agronomy 10, 1–14. doi: 10.3390/agronomy10081167
López, M. V., and Arrúe, J. L. (1997). Growth, yield and water use efficiency of winter barley in response to conservation tillage in a semi-arid region of Spain. Soil Tillage Res. 44, 35–54. doi: 10.1016/S0167-1987(97)00030-5
López-Bellido, R. J., and López-Bellido, L. (2001). Effects of crop rotation and nitrogen fertilization on soil nitrate and wheat yield under rainfed Mediterranean conditions. Agronomie 21, 509–516. doi: 10.1051/agro:2001140
López-Garrido, R., Madejón, E., Murillo, J. M., and Moreno, F. (2011). Soil quality alteration by mouldboard ploughing in a commercial farm devoted to no-tillage under Mediterranean conditions. Agric. Ecosyst. Environ. 140, 182–190. doi: 10.1016/j.agee.2010.12.001
Mrabet, R. (2000). Differential response of wheat to tillage management systems in a semiarid area of Morocco. F. Crop. Res. 66, 165–174. doi: 10.1016/S0378-4290(00)00074-5
Mrabet, R. (2002). Wheat yield and water use efficiency under contrasting residue and tillage management systems in a semiarid area of Morocco. Exp. Agric. 38, 237–248. doi: 10.1017/S0014479702000285
Mrabet, R. (2008). No-tillage systems for sustainable dryland agriculture in Morocco. Rabat, Morocco: INRA.
Mrabet, R. (2011). “No-tillage agriculture in West Asia and North Africa” in Rainfed farming systems. eds. P. G. Tow, I. M. Cooper, I. Partridge, and C. J. Birch (Berlin/Heidelberg, Germany: Springer Science+Business Media B.V), 1015–1042.
Mulumba, L. N., and Lal, R. (2008). Mulching effects on selected soil physical properties. Soil Tillage Res. 98, 106–111. doi: 10.1016/j.still.2007.10.011
Pala, M., Harris, H. C., Ryan, J., Makboul, R., and Dozom, S. (2000). Tillage systems and stubble management in a Mediterranean-type environment in relation to crop yield and soil moisture. Exp. Agric. 36, 223–242. doi: 10.1017/S0014479700002052
Paye, W. S., Thapa, V. R., and Ghimire, R. (2023). Limited impacts of occasional tillage on dry aggregate size distribution and soil carbon and nitrogen fractions in semi-arid drylands. Int. soil water Conserv. Res. doi: 10.1016/j.iswcr.2023.04.005
Peixoto, D. S., Silva, L. C. M., Melo, L. B. B., Azevedo, R. P., Araújo, B. C. L., Carvalho, T. S., et al. (2020). Occasional tillage in no-tillage systems: a global meta-analysis. Sci. Total Environ. 745:140887. doi: 10.1016/j.scitotenv.2020.140887
Plaza-Bonilla, D., Cantero-Martínez, C., Bareche, J., Arrúe, J. L., Lampurlanés, J., and Álvaro-Fuentes, J. (2017). Do no-till and pig slurry application improve barley yield and water and nitrogen use efficiencies in rainfed Mediterranean conditions? F. Crop. Res. 203, 74–85. doi: 10.1016/j.fcr.2016.12.008
R. Core Team (2017). R: A language and environment for statistical computing. Available at: https://www.r-project.org/ (Accessed May 10, 2023).
Radford, B. J., and Thornton, C. M. (2011). Effects of 27 years of reduced tillage practices on soil properties and crop performance in the semi-arid subtropics of Australia. IJEEE 19, 1–22,
Rakkar, M. K., and Blanco-Canqui, H. (2018). Grazing of crop residues: impacts on soils and crop production. Agric. Ecosyst. Environ. 258, 71–90. doi: 10.1016/j.agee.2017.11.018
Saxena, M. C., Saxena, N. P., and Mohamed, A. K. (1988). “High temperature stress” in World crops: Cool season food legumes. ed. R. J. Summerfield (Dordrecht, The Netherlands: Kluwer Academic Publishers).
Schlegel, A., Holman, J. D., and Assefa, Y. (2020). A single tillage in a long-term no-till system on dryland crop performance. Agron. J. 112, 3174–3187. doi: 10.1002/agj2.20284
Shukla, M. K., Lal, R., and Ebinger, M. (2003). Tillage effects on physical and hydrological properties of a typic argiaquoll in Central Ohio. Soil Sci. 168, 802–811. doi: 10.1097/01.ss.0000100470.96182.4a
Sita, K., Sehgal, A., Hanumantharao, B., Nair, R. M., Vara Prasad, P. V., Kumar, S., et al. (2017). Food legumes and rising temperatures: effects, adaptive functional mechanisms specific to reproductive growth stage and strategies to improve heat tolerance. Front. Plant Sci. 8, 1–30. doi: 10.3389/fpls.2017.01658
Stavi, I., Lal, R., and Owens, L. B. (2011). On-farm effects of no-till versus occasional tillage on soil quality and crop yields in eastern Ohio. Agron. Sustain. Dev. 31, 475–482. doi: 10.1007/s13593-011-0006-4
Truong, T. H. H., and Marschner, P. (2020). Plant residues differing in C/N ratio in mulch and soil — the effect of the mulch on nutrient availability and microbial biomass is more pronounced with higher leaching amount. Soil Ecol. Lett. 2, 317–326. doi: 10.1007/s42832-020-0036-4
Turpin, J. E., Herridge, D. F., and Robertson, M. J. (2002). Nitrogen fixation and soil nitrate interactions in field-grown chickpea (Cicer arietinum) and fababean (Vicia faba). Aust. J. Agric. Res. 53, 599–608. doi: 10.1071/AR01136
Wafae, S., Bendidi, A., Daoui, K., Abdelghani, N., Bouichou, E. H., Khalfi, C. D., et al. (2023). Tillage systems effect on wheat yield in the Saïs region of Morocco. Univers. J. Agric. Res. 11, 22–31. doi: 10.13189/ujar.2023.110103
Ye, T., Ma, J., Zhang, P., Shan, S., Liu, L., Tang, L., et al. (2022). Interaction effects of irrigation and nitrogen on the coordination between crop water productivity and nitrogen use efficiency in wheat production on the North China plain. Agric. Water Manag. 271:107787. doi: 10.1016/j.agwat.2022.107787
Keywords: conservation agriculture, crop rotation, crop yield, drylands, durum wheat, strategic tillage, water productivity, water storage at sowing
Citation: Diop M, Beniaich A, Cicek H, Ouabbou H, Bamouh A, El Gharras O, Dahan R, Zine El Abidine A, El Gharous M and El Mejahed K (2024) Short-term residual effects of occasional tillage on crop performance, soil water, and water-use efficiency in a 10-year no-till system under a dry Mediterranean climate. Front. Sustain. Food Syst. 8:1375666. doi: 10.3389/fsufs.2024.1375666
Edited by:
Rishi Raj, Indian Agricultural Research Institute (ICAR), IndiaReviewed by:
Mohammad Hashim, Indian Institute of Pulses Research (ICAR), IndiaAnchal Dass, Indian Agricultural Research Institute (ICAR), India
Copyright © 2024 Diop, Beniaich, Cicek, Ouabbou, Bamouh, El Gharras, Dahan, Zine El Abidine, El Gharous and El Mejahed. This is an open-access article distributed under the terms of the Creative Commons Attribution License (CC BY). The use, distribution or reproduction in other forums is permitted, provided the original author(s) and the copyright owner(s) are credited and that the original publication in this journal is cited, in accordance with accepted academic practice. No use, distribution or reproduction is permitted which does not comply with these terms.
*Correspondence: Harun Cicek, harun.cicek@fibl.org