- 1Engineering Research Center of Grassland Industry, Ministry of Education, College of Pastoral Agriculture Science and Technology, Lanzhou University, Lanzhou, China
- 2Qinghai University, Xining, China
Plant and soil C:N:P stoichiometry reflects the element content and energy flow, which are important for biogeochemical cycling in ecosystems. Although plantation age has been verified to affect leaf C:N:P stoichiometry in alfalfa plants, its effect on plant and soil C:N:P stoichiometry in grass remains poorly documented. A 10-year field experiment of Kentucky bluegrass (Poa pratensis) was used to test how plantation age affect plant and soil C:N:P stoichiometry in a perennial rhizomatous grass pasture. This study demonstrated that leaf C:N, C:P, and N:P ratios exhibited a rapid increasing trend from 2 to 6 years of age, whereas leaf C:N showed a slight decreasing trend, and leaf C:P and N:P maintained stability from 6 to 9 years of age. Stem C:N and N:P were not different among plantation ages, while stem C:P increased from 2 to 4 years of plantation age and then maintained stability from 4 to 9 years of plantation age. Root N:P showed an increasing trend from 2 to 6 years of plantation age and relative stability from 6 to 9 years of plantation age, whereas root C:N and C:P showed decreasing trends from 2 to 9 years of plantation age. Although soil C:P did not differ among nine plantation ages, soil C:N and N:P remained relatively stable from 2 to 6 years of plantation age. However, soil C:N showed a decreasing trend, while soil N:P showed an increasing trend after 6 years of plantation age. The results from an ecological stoichiometric homeostasis analysis further showed that N in the leaf, stem, and root and P in the stem had strict homeostasis, whereas P in the leaf and root showed plastic and weakly homeostatic status, respectively. These results present a pattern concerning the plantation age in relation to plant and soil C:N:P stoichiometry in a perennial grass and provide useful information for N and P management in Kentucky bluegrass pastures.
1 Introduction
Carbon (C), nitrogen (N), and phosphorus (P) are three vital elements for plants, regulating plant growth and development (Zhang et al., 2021). Soil C:N:P stoichiometry is considered to provide insight into the potential contribution of plant organics to soil fertility, as well as soil nitrogen and phosphorus availability (Pang et al., 2021). The C:N:P stoichiometries between plants and soil are suggested as a major indicator to understand their ecological functions and processes (Cao and Chen, 2017), in which plant productivity is one of the main functions of pastures (Lindsey et al., 2020).
Most previous studies have focused on the spatial variability of C:N:P stoichiometry in terrestrial ecosystems (Dijkstra et al., 2012; He and Dijkstra, 2015; Hu et al., 2019) or the responses of plant and soil C:N:P stoichiometry to biotic and abiotic disturbances (Pathak et al., 2017; Kleyer et al., 2019). Only a few studies have addressed the temporal variability of leaf C:N:P stoichiometry in shrubs (Zhang et al., 2016; Zeng et al., 2017; Yu et al., 2021) and legumes (Wang et al., 2015) to examine the relationship between leaf C:N:P stoichiometries and plantation ages, and the previous studies found that leaf N:P in Haloxylon ammodendron shows a rapid increase from 2 to 5 years of plantation age, but leaf C:N exhibits a decline, and they are stable after the 5-year plantation age (Zhang et al., 2016). Leaf C:N, C:P, and N:P ratios in Caragana korshinskii decreased with an increase in plantation ages from 10 to 30 years (Zeng et al., 2017); those ratios in Zanthoxylum planispinum showed no significant difference in 5- , 10- , and 20-year plantation ages, respectively (Yu et al., 2021); and those ratios in alfalfa proved to be the highest at the 8-year plantation age (Wang et al., 2015). However, how plantation age simultaneously affects the temporal variability of the plant (leaf, stem, and root), and soil C:N:P stoichiometry receives less attention. Therefore, examining the effects of plantation ages on plant and soil C:N:P stoichiometry is one of the most important approaches to understanding the effect of plantation ages on the ecological functions and processes of pastures.
In agricultural practices, perennial grasses are promising candidates for the establishment of artificial pastures with good quality and higher productivity for grazing livestock (Jones et al., 2015) or for the regeneration of vegetation populations in degraded grasslands with their high reproductivity. Many previous studies have found that the aboveground biomass of perennial grass pastures often differs during different plantation ages (Folck et al., 2023), and this difference is related to soil nitrogen availability and supplies because grass is nitrophile (Mazzucato et al., 1996). Kentucky bluegrass (Poa pratensis), a perennial rhizomatous grass with high tolerance to environments, has become increasingly important (Pi et al., 2015; Wu et al., 2023) because of its ability to be productive in cool temperatures and high soil moisture conditions (DeKeyser et al., 2015; Zhu et al., 2023). This grass has been widely grown in Eurasia (Cui et al., 2020), Asia (Wang C. et al., 2023), and North America (Folck et al., 2023; Toledo et al., 2023) due to its unique ecological advantage: the relatively longer time intervals between tilling, which reduce the risk of soil erosion compared to annual grass pastures (Lindsey et al., 2020).
Kentucky bluegrass is recognized as the most preferred choice for the establishment of pastures or the management of degraded grassland in the Qinghai-Tibetan plateau (Pi et al., 2015). At present, pasture areas of Kentucky bluegrass cover over 4.33 million ha in the Qinghai-Tibetan plateau. Previous studies were focused on the growth features of Kentucky bluegrass in relation to seeding time (Jing et al., 2018), the role of Kentucky bluegrass in vegetation rebuilding of “black soil type” in degraded grassland (Wen et al., 2006), the responses of reproductive phenology and vegetative growth of Kentucky bluegrass to climate change (Wei et al., 2022), and high-yield and high-quality cultivation techniques for Kentucky bluegrass (Jing et al., 2019). Whether the plantation ages affect plant and soil C:N:P stoichiometry in Kentucky bluegrass pasture remains poorly documented.
This study uses Kentucky bluegrass as a focal grass to investigate the effect of plantation ages on plant and soil C:N:P stoichiometry in a perennial rhizomatous grass pasture through an 8-year field experiment. The hypotheses are that (1) C:N, C:P, and N:P in the leaf, stem, and root of Kentucky bluegrass increase and then decrease as plantation age increases; (2) C:N, N:P, and C:P in the soil decrease with the increase in plantation ages, which can present a general pattern concerning the effect of plantation ages on plant and soil C:N:P stoichiometry in a crop and provide a reference for the nitrogen and phosphorus management of Kentucky bluegrass pastures in the Qinghai-Tibetan plateau.
2 Materials and methods
2.1 Experimental site description
The experiment site is located at the Haibei experiment station (36°59.36′N, 100°52.848′E, elevation 3,156 m) of Qinghai University in Qinghai Province, China. This station is on the eastern edge of the Qinghai-Tibetan plateau. This experiment site experiences a cold plateau continental climate without an absolute frost-free period. The average annual precipitation at this experiment station is 369.1 mm, most of which occurs from June to August of each year, corresponding with the growing season, while the mean annual evaporation is ~1,400 mm. The average annual temperature at this experiment station is 0.5°C, with a maximum in August (19°C) and a minimum in January (−4°C; Figure 1). The soil at the experiment site is chestnut soil (Wang Y. et al., 2023), similar to Entisol in FAO classification, and this soil is the major type for crop production in this area. The soil chemical properties measured at the 0–40 cm layer are as follows: pH (8.43), soil organic carbon (6.95 g kg−1), total N (0.6886 g kg−1), total P (0.52 g kg−1), and total K (9.84 g kg−1).
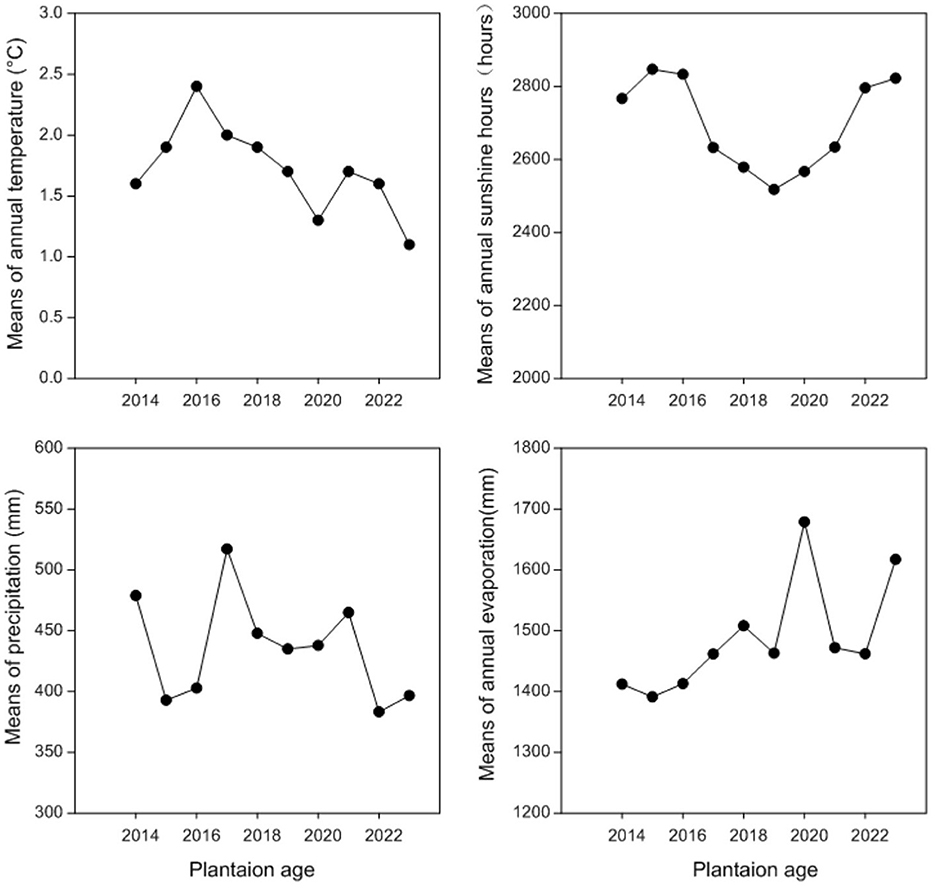
Figure 1. Means of annual temperature (°C), annual sunshine hours (h), annual precipitation (mm), and annual evaporation (mm) at Haibei Station during 2015–2022.
2.2 Establish pastures of Kentucky bluegrass
Three field plots were established in 2014 to monitor the relationships between plant productivity and plantation ages. The plot size was 30 m × 30 m. The distance between the two plots was 200 m, which ensured that precipitation and air temperature were the same among the three plots, avoiding the biases introduced by precipitation and air temperature on plant productivity.
To quantify the relationship between aboveground biomass and stoichiometry, phosphorus is not applied to the experiment plots because a high amount could promote the rapid growth of Kentucky bluegrass roots. Therefore, 75 kg ha−1 of urea (N 46%) was applied to each experiment plot in 2014. Kentucky bluegrass seeds with an 88% germination rate were broadcasted in each plot on 5 April 2014. The seeding density was 3 g m−2. During the experimental period of 2015–2022, the Kentucky bluegrass pastures were generally rainfed without external irrigation or further fertilizer application. The plots were fenced during the experiment period.
2.3 Plant and soil samplings
In the process of plant and soil sampling, three plots were considered replicates. Sampling was conducted at the flowering stages of Kentucky bluegrass in July from 2015 to 2022 (Supplementary Table S1). In each plot, five subplots with a size of 0.5 m × 0.5 m were randomly selected to sample plants (leaves, stems, and roots) and soils, and these samples were completely mixed as a composite sample to measure C, N, and P concentrations. First, at each subplot, shoots were harvested by shear and then divided into leaves and stems, in which flowers were classified as stems. Second, the root auger with a 10-cm inner diameter was used to collect a soil column with a 20-cm layer at this subplot, and this soil column was screened out of garbage and stones and then divided into root samples and soil samples using a 2-mm sieve (Duan et al., 2024). The leaves, stems, and roots were placed into envelopes, and the soil samples were collected in fabric sample bags and carried back to the laboratory. These composite samples of leaves, stems, and roots were used to measure total C, total N, and total P concentrations, and soil composite samples were used to measure organic C, total N, and total P concentrations.
2.4 Measurement of C, N, and P
All composite samples of leaves, stems, and roots were dried at 65°C until no further mass loss could be found. Soil composite samples were air-dried. The total C and total N in leaves, stems, and roots and the total N in soils were analyzed with the Dumas dry combustion method using a Flash-II EA112 Elemental Analyzer (Thermo Fisher Scientific, Waltham, MA, USA). The total P in leaves, stems, roots, and soils was determined using the molybdenum blue colorimetric method. Soil organic C was determined using dichromate heating and oxidation method.
2.5 Estimation of ecological stoichiometric homeostasis
C:N, C:P, and N:P refer to the ratio between the total concentrations of C, N, and P in Kentucky bluegrass or in soil organics, respectively.
A regulation index (1/H) proposed by Hood and Sterner (2010) was used to quantify the degree of stoichiometric homeostasis in an organism. This index was calculated by the following equation: ln(y) = lnc + 1/H × ln(x), where x represents the soil nutrient stoichiometry (e.g., N or P) and y represents the nutrient stoichiometry of leaf, stem, and root. Regressions were used to estimate the relationship between soil and plant (leaf stem and root). The regression coefficient was used to produce new datasets, and these new datasets were used to calculate the regulation index (1/H). Based on a previous study (Persson et al., 2010), the regulation index was interpreted as follows: 0 < 1/H < 0.25, “homeostatic”; 0.25 < 1/H < 0.5, “weakly homeostatic”; 0.5 < 1/H < 0.75, “weakly plastic”; 1/H > 0.75, “plastic”; and 1/H ≥ 1, “not homeostatic.”
2.6 Data analysis
The data were transformed when necessary to meet normality and homogeneity. Repeated measures analysis of variance (ANOVA) using least significant difference (LSD) was used to determine whether the plantation age affected the total C, total N, and total P of the plant, the total N, total P, and organic C of the soil, and the plant and soil C:N:P stoichiometry. A linear model (LM) was used to examine the regression between soil and plant (leaf, stem and root) in 95% confidence intervals. All analyses were carried out in R 4.3.2, R Foundation for Statistical Computing, Vienna, Austria.
3 Results
3.1 Effect of plantation ages on leaf C:N, C:P, and N:P
Total C concentration in leaves increased and then decreased as the plantation age increased, reaching its peak when plantation ages ranged from 4 to 6 years (Figure 2A), whereas total N and P concentrations in leaves first decreased and then maintained a relatively stable level with the increase in plantation ages, and there were no significant differences observed when plantation ages increased from 5 to 9 years (Figures 2B, C).
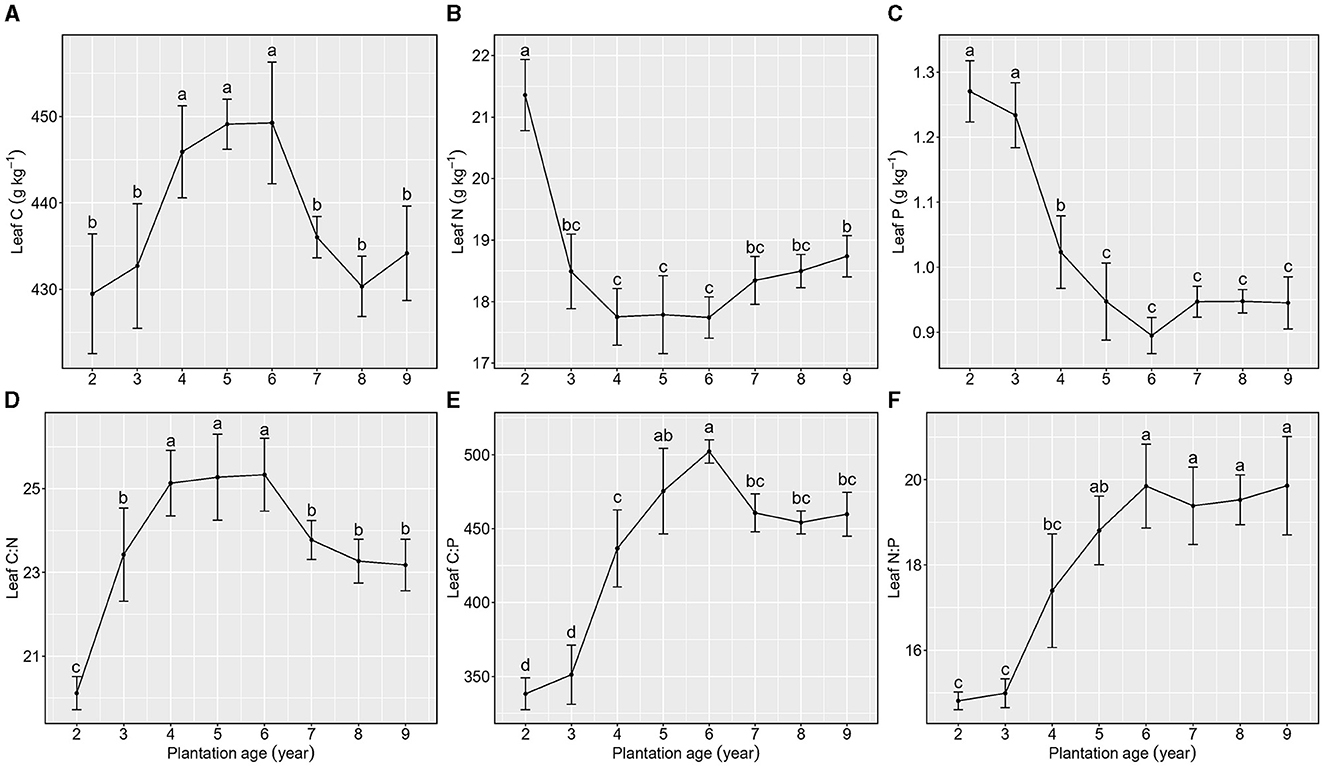
Figure 2. Stoichiometric characteristics of leaf C, N, and P of Kentucky bluegrass at plantation age sequences (mean ± SE; different letters denote significant differences at the α = 0.05 level). (A) Leaf C, (B) Leaf N, (C) Leaf P, (D) Leaf C:N, (E) Leaf C:P, and (F) Leaf N:P. The error bars represent the standard deviation.
Leaf C:N, C:P, and N:P exhibited various patterns as the plantation age increased (Figures 2D–F). Leaf C:N increased from 2 to 4 years of plantation age, maintained a relatively stable level from 5 to 6 years of plantation age, and then decreased from 7 to 9 years of plantation age. Leaf C:P increased from 2 to 6 years of plantation age, decreased from 6 to 7 years of plantation age, and maintained a stable level from 7 to 9 years of plantation age. Leaf N:P increased from 2 to 5 years of plantation age and then maintained stability from 6 to 9 years of plantation age.
3.2 Effect of plantation ages on stem C:N, C:P, and N:P
Total C and N concentrations in stems were not different among different plantation ages (Figures 3A, B), whereas total P concentration in stems showed a decreasing trend with the increase in plantation age (Figure 3C).
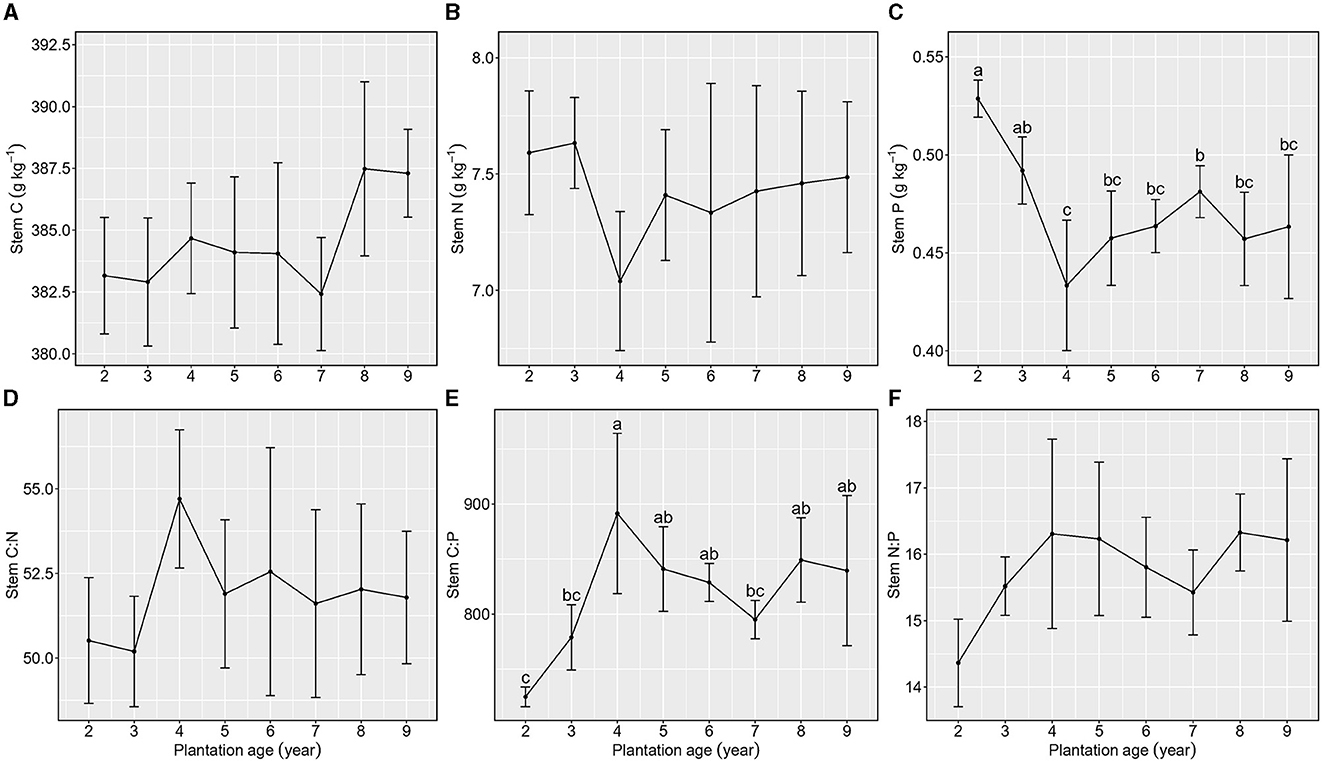
Figure 3. Stoichiometric characteristics of stem C, N, and P of Kentucky bluegrass at plantation age sequences (mean ± SE; different letters denote significant differences at the α = 0.05 level). (A) Stem C, (B) Stem N, (C) Stem P, (D) Stem C:N, (E) Stem C:P, and (F) Stem N:P. The error bars represent the standard deviation.
Stem C:N and N:P were not different among plantation ages, and their averages were 51.91 ± 2.33 and 15.78 ± 0.95, respectively (Figures 3D, E), whereas stem C:P increased from 2 to 4 years of plantation age and then maintained a stable level from 4 to 9 years of plantation age (Figure 3F).
3.3 Effect of plantation ages on root C:N, C:P, and N:P
With the increase in plantation age, total C, N, and P concentrations in roots exhibited a similar unimodal distribution, approximately peaking from 4 to 5 years of plantation age.
Root C:N exhibited a reduction from 2 to 3 years of plantation age, an increase from 3 to 6 years of plantation age, and relative stability from 6 to 9 years of plantation age, whereas root C:P showed a decreasing trend but with a stable phase. Root N:P showed a reduction from 2 to 3 years of plantation age, an increasing trend from 3 to 6 years of plantation age, and relative stability from 7 to 9 years of plantation age (Figure 4).
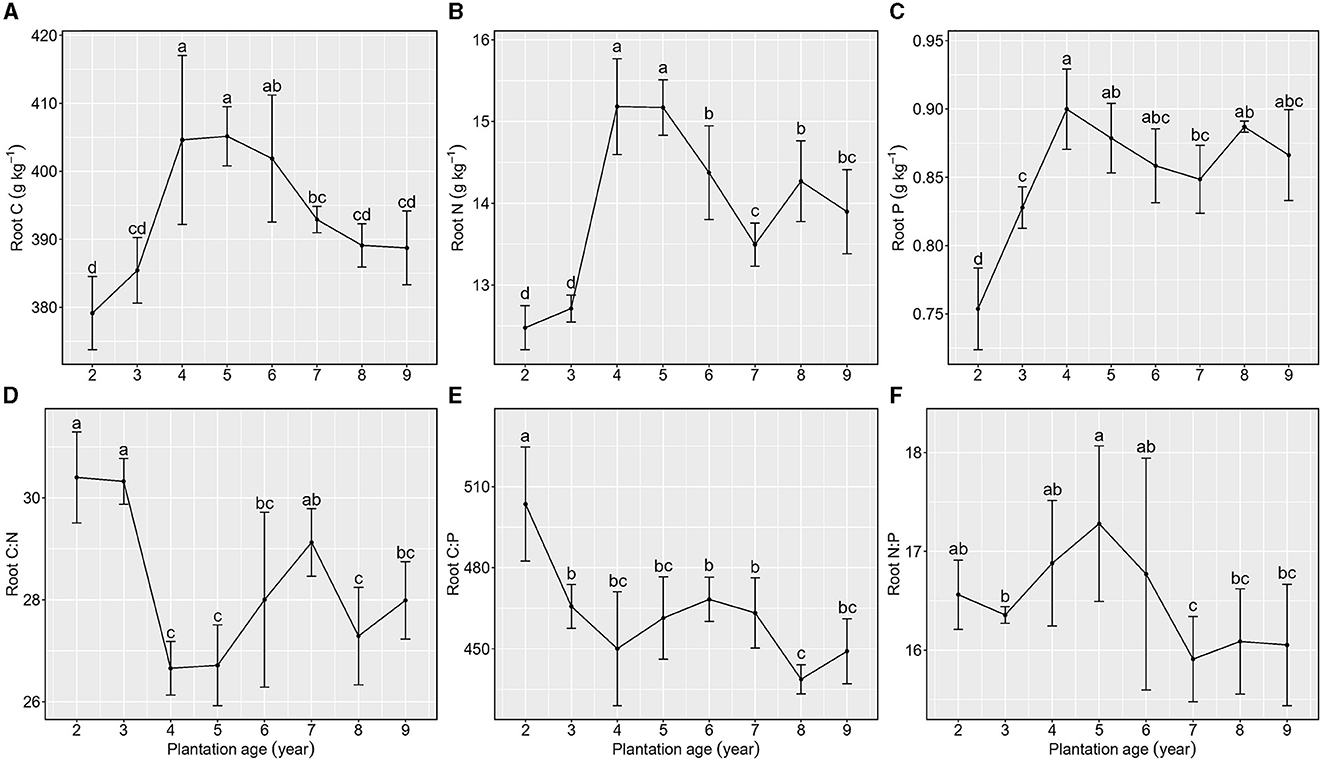
Figure 4. Stoichiometric characteristics of root C, N, and P of Kentucky bluegrass at plantation age sequences (mean ± SE; different letters denote significant differences at the α = 0.05 level). (A) Root C, (B) Root N, (C) Root P, (D) Root C:N, (E) Root C:P, and (F) Root N:P. The error bars represent the standard deviation.
3.4 Effect of plantation ages on soil C:N, C:P, and N:P
Soil organic C, total N, and total P initially showed increasing trends from 2 to 5 years of plantation age, and then, they were relatively stable after 6 or 7 years of plantation age.
Soil C:P was not different among the nine plantation ages. In the increasing process of plantation age, soil C:N showed a slight decreasing trend and then maintained a relatively stable level, whereas soil N:P showed a slight increasing trend and then maintained a relatively stable level, in which the 7-year plantation age of Kentucky bluegrass was the inflection point for soil C:N and N:P (Figure 5).
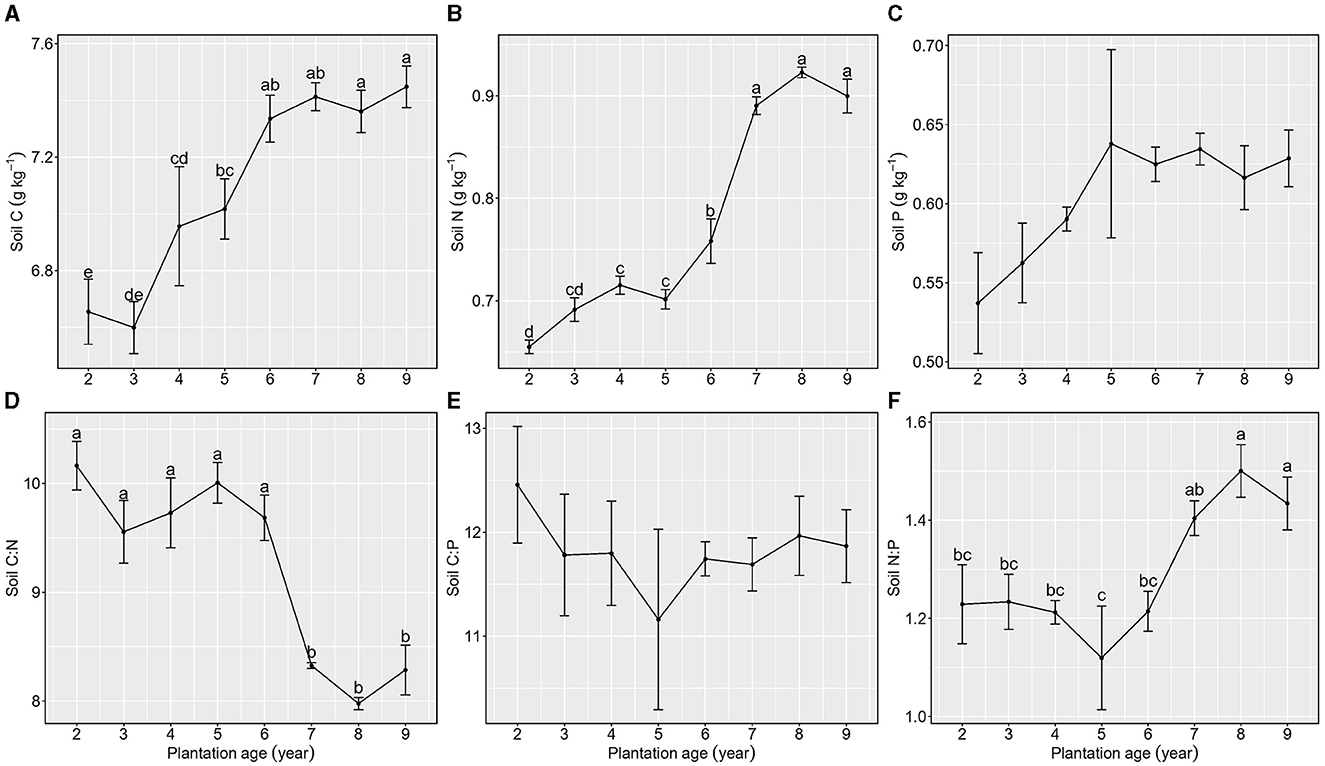
Figure 5. Stoichiometric characteristics of Soil C, N, and P of Kentucky bluegrass at plantation age sequences (mean ± SE; different letters denote significant differences at the α = 0.05 level). (A) Soil C, (B) Soil N, (C) Soil P, (D) Soil C:N, (E) Soil C:P, and (F) Soil N:P. The error bars represent the standard deviation.
3.5 Ecological stoichiometric homeostasis
N concentration and P concentration in plants and soil were found to differ in ecological stoichiometric homeostasis. No significant correlation was found between plant N (leaf, stem, and root) and soil N. The 1/H values of leaf, stem, and root N were 0.114, 0.001, and 0.106, respectively, indicating that N in the leaf, stem, and root had strict homeostasis (Figure 6).
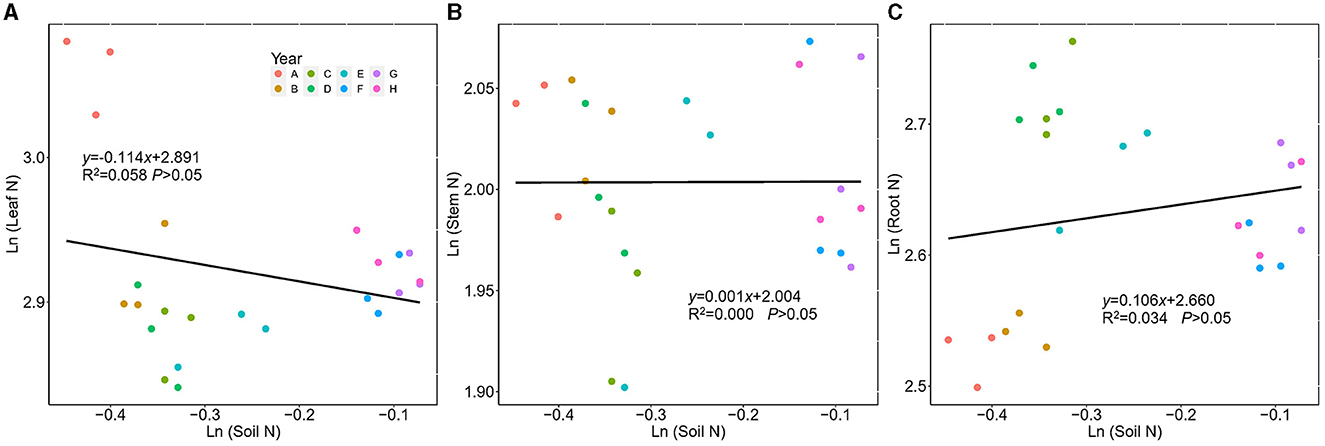
Figure 6. Regressions between ln-transformed N concentration in Kentucky bluegrass leaf, stem, root, and soil from 2 to 9 years of plantation age. ln(y) = 1/H × ln(x) + lnc; 1/H indicates the degree of stoichiometric homeostasis. (A) Leaf, (B) Stem, and (C) Root.
The leaf, stem, and root P showed different stoichiometric homeostasis (Figure 7). The stem P exhibited homeostasis with a 1/H value of 0.208, the leaf P showed plastic status with 1/H of 0.790, whereas the root P exhibited weak homeostasis with a 1/H value of 0.401.
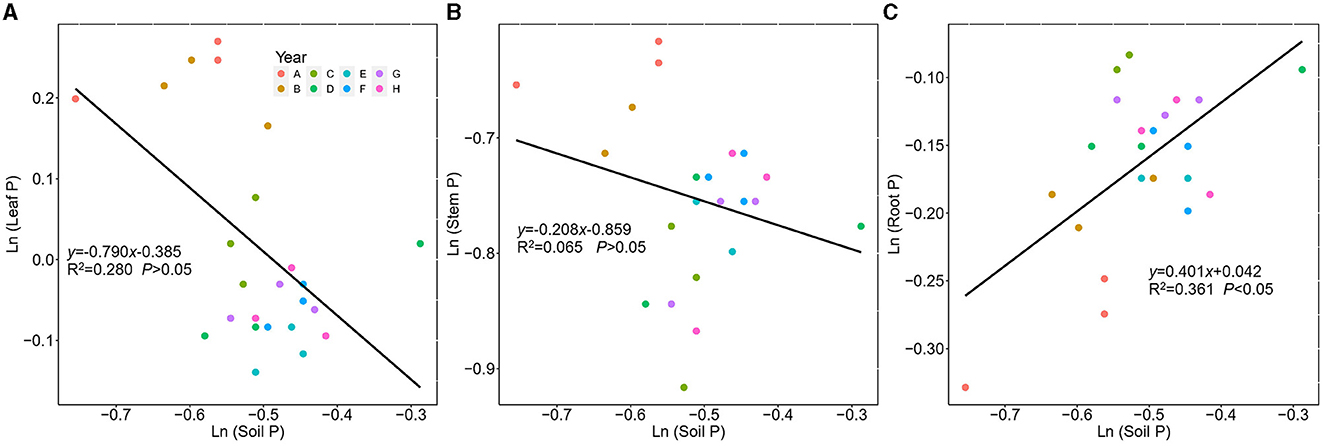
Figure 7. Regressions between ln-transformed P concentration in Kentucky bluegrass leaf, stem, root, and soil from 2 to 9 years of plantation age. ln(y) = 1/H × ln(x) + lnc; 1/H indicates the degree of stoichiometric homeostasis. (A) Leaf, (B) Stem, and (C) Root.
4 Discussion
Pastures with a single crop are verified to be usually degraded as the plantation age increases (Wang et al., 2015; Zhang et al., 2016). Previous studies have examined the relationship between leaf C:N:P stoichiometry (in alfalfa of legumes or in H. ammodendron of Chenopodiaceae) and plantation ages or stand ages. This study uses Kentucky bluegrass as a focal crop to examine the effects of plantation ages on C:N:P stoichiometry in leaves, stems, roots, and soils in perennial rhizomatous grass pastures, which can present a pattern of pasture degradation with the increase in plantation age from a N or P limitation perspective.
This study indicates that the C:N ratio in leaves increases from 2 to 6 years of plantation age and decreases from 6 to 9 years of plantation age; the C:N ratio in roots decreases from 2 to 5 years of plantation age, increases from 5 to 7 years of plantation age, and decreases after 7 years of plantation age; whereas the C:N ratio in stems does not differ in plantation ages. These aspects contradict the first hypothesis in this study.
The C:N ratio in leaves in the increasing process of plantation ages is dependent on the changes in leaf C and N concentration from 2 to 9 years of plantation age. The change in C concentrations in leaves may be ascribed to the photosynthetic capacity of Kentucky bluegrass among different plantation ages (Wang C. et al., 2023). The higher plant density in the early stages of plantation often limits the spatial distribution of light (Aerts, 1996; Xu et al., 2023), wherein lower-height plants may not receive sufficient light for photosynthesis and carbon sequestration (Poorter et al., 2012), resulting in the lower leaf C in 2–3 years of plantation age. When the plantation age ranges from 4 to 6 years, plant density often decreases because of self-thinning (Walter et al., 2023), which enables plants to receive more light, resulting in higher photosynthesis with a high C concentration in leaves. When plantation ages are over 6 years, biological processes may decline for perennial plants (Constable and Bange, 2015), leading to a weakened growth potential for Kentucky bluegrass (Yang et al., 2019), negatively contributing to leaf C concentration. In addition, Kentucky bluegrass uptakes soil nitrogen to supply plant growth, and the soil nitrogen can be gradually consumed with the increase in plantation age (Han et al., 2019). The decrease in soil nitrogen concentration is insufficient to meet plant requirements, leading to a decrease in leaf nitrogen concentration (Shi et al., 2015; Wang C. et al., 2023) when plantation age is below 6 years. However, when the plantation age is over 6 years, the accumulation of plant residues gradually increases soil nitrogen, and this added nitrogen can be released into the soil again (Hu et al., 2019; Zhang et al., 2021), which can provide relatively sufficient soil nitrogen for plant uptake, leading to an increase in leaf N when plantation age ranges from 6 to 9 years. With a simultaneous change in leaf C and leaf N in the increasing process of plantation age, leaf C:N increases from 2 to 6 years of plantation age and decreases from 6 to 9 years of plantation age. However, root C:N decreases from 4 to 9 years of plantation age, and this is because N is stored in roots during the period of well-developed roots (Yan et al., 2016) because perennial grass can develop well-rooted over a long plantation age (Robinson et al., 2010; Liu et al., 2018). In general, root C:N is considered an important indicator of the plant growth rate, in which a higher C:N ratio leads to a lower growth rate of perennial plants (Xiao et al., 2021; Wu et al., 2023). Therefore, these results indicated that leaves contribute to more N accumulation for Kentucky bluegrass plants during 2 to 3 years of plantation age, while roots contribute to more N accumulation for Kentucky bluegrass from 4 to 9 years of plantation age. A lower C:N ratio after 4 years of plantation age indicates that litter decomposition and nutrient return become fast (Ning et al., 2021; Wang C. et al., 2023), and this would be conducive to greater nutrient transfer to the soil from 4 to 9 years of plantation age.
Leaf and root C:P show different changeable trends with the increase in plantation age, in which leaf C:P increases and root C:P decreases as plantation age increases, and these are mainly regulated by C and P concentrations in leaf and root. The C concentration in leaves and roots was discussed in the earlier paragraph. The leaf P showed a gradually decreasing trend with the increase in plantation ages, which was mainly caused by soil phosphorus consumption and plant nutrient allocation (Zhang et al., 2021). In the early plantation stages, perennial plants may prioritize the allocation of nutrients to leaves to enhance photosynthesis (Robinson et al., 2010; Poorter et al., 2012), resulting in a higher phosphorus concentration in leaves. In contrast to early plantation stages, Kentucky bluegrass with long-term plantation ages may allocate more nutrients for roots and rhizomes (Jing et al., 2018), resulting in a further decrease in leaf P and an increase in root P.
Leaf N:P ranges from 14.873 to 15.032 between 2 and 3 years of plantation age and exceeds 16 from 4 to 9 years of plantation age, indicating that Kentucky bluegrass is co-limited by nitrogen and phosphorus (Wu et al., 2023) in 2–3 years of plantation age and becomes limited by phosphorus from 4 to 9 years of plantation age. These aspects imply that P is very important to the management of Kentucky bluegrass pasture when the plantation age is considered in practice.
This study also finds that soil C:N increases as the plantation age of Kentucky bluegrass increases. Kentucky bluegrass can sequester CO2 from the atmosphere, and then, the fixed C can be stored in soil by plant litter (Cotrufo et al., 2013; Jackson et al., 2017) because plant tissues are the principal sources of soil C in terrestrial ecosystems (Yuan et al., 2021). In this case, soil carbon (C) content demonstrates a positive correlation with the age of Kentucky bluegrass plantations, attributed to increased plant biomass. Especially, a significant increase in soil C concentration is observed at the 4-year plantation age, explained by variations in litter quantity and quality (De Deyn et al., 2008; Cotrufo et al., 2013). Reduced litter quantity between 2 and 3 years of plantation age corresponds to diminished organic matter input, thereby decreasing soil organic carbon levels. Moreover, poorer quality litter, characterized by higher C:N ratios during the 2- and 3-year plantation age periods, tends to retard organic matter decomposition (Yuan et al., 2021), in contrast to later plantation stages (from 4 to 9 years of plantation age), fostering a low organic matter content with accelerated decomposition rates during early plantation phases. However, leaf C:N is lower at 2–3 years of plantation age than that during 4–9 years of plantation age, and this contradicts evidence for lower quality plant litter alleviating the decomposition rate of organic matter. In fact, plant roots generate a greater contribution to soil C formation than aboveground litter because roots can immediately interact with the surrounding soil minerals, microbes, and aggregates, which usually form soil C (Sokol and Bradford, 2019; Sokol et al., 2019). Soil N increases greatly from 6 to 9 years of plantation age and the increase in soil nitrogen caused by plant litter can compensate for the soil nitrogen loss due to plant uptake. Although Kentucky bluegrass litter can replenish soil N pools, it can absorb more N from soils because Kentucky bluegrass is nitrophile, and this process often delays soil N accumulation. Thus, soil N storage by plants shows a hysteresis effect compared to soil C accumulation. In this study, soil C and N concentrations exhibit an increase from 2 to 6 years of plantation age, which leads soil C:N to maintain relative stability. When the plantation age is over 7 years, the soil N concentration shows a great increase and soil C concentration shows relative stability, which causes soil C:N to show a great decrease when the plantation age is over 7 years.
Soil N:P increases with the increase in plantation ages. An increase in soil N:P can be explained by the gradual increase in soil N because soil total P is not changeable among nine plantation ages (Figure 4). However, soil N:P is < 10 among nine plantation ages, indicating that the growth of Kentucky bluegrass may be limited more by N than by P (Bui and Henderson, 2013). However, soil N:P becomes higher when the plantation age is over 7 years, suggesting that soil N limitation for plant growth may be alleviated due to higher soil N and its availability with lower soil C:N (Bengtsson et al., 2003). These results demonstrate that Kentucky bluegrass pasture can improve the C sequestration potential and provide a good habitat for plant growth when the plantation age is over 7 years.
This study further finds that N in the leaf, stem, and root and P in the stem show a “homeostatic” status, and P in the root shows a “weakly homeostatic” status, whereas P in the leaf shows a “plastic” status, demonstrating that Kentucky bluegrass may maintain leaf P nutrient stability by modulating the P nutrient in the stems (Xiao et al., 2021). These results imply that soil P is more important in regulating the health growth of Kentucky bluegrass than soil N, which provides precise information for N and P management in perennial rhizomatous grass pastures.
5 Conclusion
This study used Kentucky bluegrass as a focal crop to investigate the plant (root, stem, and leaf) and soil C:N:P stoichiometry to understand the stoichiometric homeostasis as plantation age increases. This study found that plantation age had significant effects on plant (root, stem, and leaf) and soil C:N:P stoichiometry. Leaf C:N, C:P, and N:P increased from 2 to 6 years of plantation age, whereas leaf C:N decreased and leaf C:P and N:P maintained stability from 6 to 9 years of plantation age. Stem C:P increased and then maintained stability. Root N:P increased from 2 to 6 years of plantation age and maintained stability from 6 to 9 years of plantation age, whereas root C:N and C:P decreased from 2 to 9 years of plantation age. Soil C:N and N:P were found to maintain stability from 2 to 6 years of plantation age, and soil C:N was found to decrease and soil N:P was found to increase after 6 years of plantation age. The N in the leaf, stem, and root and the P in the stem had the “homeostatic” status, and P in the root had the “weakly homeostatic” status, whereas the P in the leaf had the “plastic” status. These results present a pattern concerning the effect of plantation age on plant (leaf, stem, and root) and soil C:N:P stoichiometry in a crop and suggest that Kentucky bluegrass pastures with plantation age over 6 years can improve soil quality and nutrient availability for plant growth.
Data availability statement
The raw data supporting the conclusions of this article will be made available by the authors, without undue reservation.
Author contributions
XW: Conceptualization, Data curation, Formal analysis, Funding acquisition, Investigation, Methodology, Resources, Writing—original draft. KH: Data curation, Investigation, Methodology, Writing—original draft, Visualization. QW: Data curation, Formal analysis, Visualization, Writing—original draft. WL: Methodology, Project administration, Resources, Writing—original draft. XP: Data curation, Formal analysis, Visualization, Writing—original draft. ZG: Conceptualization, Methodology, Supervision, Writing—original draft, Resources.
Funding
The author(s) declare financial support was received for the research, authorship, and/or publication of this article. This study was jointly funded by the Natural Science Foundation of Qinghai Province (2023-ZJ-942J) and the Western Light Talent Culture Project (2021-XBZG-12).
Acknowledgments
The authors thank the Key Laboratory of Superior Forage Germplasm in the Qinghai-Tibetan Plateau for providing a platform for this research, the team teachers for their suggestions on this article, and the team students for their help in sample collection, data analysis, etc.
Conflict of interest
The authors declare that the research was conducted in the absence of any commercial or financial relationships that could be construed as a potential conflict of interest.
Publisher's note
All claims expressed in this article are solely those of the authors and do not necessarily represent those of their affiliated organizations, or those of the publisher, the editors and the reviewers. Any product that may be evaluated in this article, or claim that may be made by its manufacturer, is not guaranteed or endorsed by the publisher.
Supplementary material
The Supplementary Material for this article can be found online at: https://www.frontiersin.org/articles/10.3389/fsufs.2024.1368418/full#supplementary-material
References
Aerts, R. (1996). Nutrient resorption from senescing leaves of perennials are there general patterns. J. Ecol. 84, 597–608. doi: 10.2307/2261481
Bengtsson, G., Bengtson, P., and Månsson, K. F. (2003). Gross nitrogen mineralization-, immobilization-, and nitrification rates as a function of soil C/N ratio and microbial activity. Soil Biol. Biochem. 35, 143–154. doi: 10.1016/S0038-0717(02)00248-1
Bui, E. N., and Henderson, B. L. (2013). C: N: P stoichiometry in Australian soils with respect to vegetation and environmental factors. Plant Soil 373, 553–568. doi: 10.1007/s11104-013-1823-9
Cao, Y., and Chen, Y. M. (2017). Coupling of plant and soil C:N:P stoichiometry in black locust (Robinia pseudoacacia) plantations on the Loess Plateau, China. Trees 31, 1559–1570. doi: 10.1007/s00468-017-1569-8
Constable, G. A., and Bange, M. P. (2015). The yield potential of cotton (Gossypium hirsutum L.). Field Crops Res. 182, 98–106. doi: 10.1016/j.fcr.2015.07.017
Cotrufo, M. F., Wallenstein, M. D., Boot, C. M., Denef, K., and Paul, E. (2013). The Microbial Efficiency-Matrix Stabilization (MEMS) framework integrates plant litter decomposition with soil organic matter stabilization: do labile plant inputs form stable soil organic matter? Glob. Change Biol. 19, 988–995. doi: 10.1111/gcb.12113
Cui, J., Shah, S., Fahad, S., and Chen, Y. (2020). A review on kentucky bluegrass responses and tolerance to drought stress. Abiotic Stress Plants 1, 1–10. doi: 10.5772/intechopen.93812
De Deyn, G. B., Cornelissen, J. H., and Bardgett, R. D. (2008). Plant functional traits and soil carbon sequestration in contrasting biomes. Ecol Lett. 11, 516–531. doi: 10.1111/j.1461-0248.2008.01164.x
DeKeyser, E., Dennhardt, L., and Hendrickson, J. (2015). Kentucky bluegrass (Poa pratensis) invasion in the northern great plains: a story of rapid dominance in an endangered ecosystem. Invasive Plant Sci. Manage. 8, 255–261. doi: 10.1614/IPSM-D-14-00069.1
Dijkstra, F. A., Pendall, E., Morgan, J. A., Blumenthal, D. M., Carrillo, Y., Lecain, D. R., et al. (2012). Climate change alters stoichiometry of phosphorus and nitrogen in a semiarid grassland. New Phytol. 196, 807–815. doi: 10.1111/j.1469-8137.2012.04349.x
Duan, Y. Y., Zhang, J., Jiang, Z., Wei, X. X., Guo, Z. G., and Liu, H. X. (2024). Partial root-zone drying (PRD) leads to lower carbon retention in the soil-plant systems of alfalfa. Irrig Sci. 42, 45–56. doi: 10.1007/s00271-023-00902-6
Folck, A. J., Bigelow C, A., Jiang, Y., and Patton, A. (2023). Growth analysis of Kentucky bluegrass cultivars from six classification groups. Agrosyst. Geosci. Environ. 6:e20444. doi: 10.1002/agg2.20444
Han, X. Y., Gao, G. Y., Li, Z. S., Chang, R., Jiao, Y., and Fu, L. (2019). Effects of plantation age and precipitation gradient on soil carbon and nitrogen changes following afforestation in the Chinese Loess Plateau. Land Degrad. Dev. 30, 2298–2310. doi: 10.1002/ldr.3422
He, M. Z., and Dijkstra, F. A. (2015). Phosphorus addition enhances loss of nitrogen in a phosphorus-poor soil. Soil Biol Biochem. 82, 99–106. doi: 10.1016/j.soilbio.2014.12.015
Hood, J. M., and Sterner, R. W. (2010). Diet mixing: do animals integrate growth or resources across temporal heterogeneity? Am. Soc Nat. 176, 651–663. doi: 10.1086/656489
Hu, C. C., Lei, Y. B., Tan, Y. H., Sun, X. C., Xu, H., Liu, C. Q., et al. (2019). Plant nitrogen and phosphorus utilization under invasive pressure in a montane ecosystem of tropical China. J Ecol. 107, 372–386. doi: 10.1111/1365-2745.13008
Jackson, R. B., Lajtha, K., Crow, S. E., Hugelius, G., Kramer, M. G., and Piñeiro, G. (2017). The ecology of soil carbon: pools, vulnerabilities, and biotic and abiotic controls. Annu. Rev. Ecol. Evol. Syst. 48, 419–445. doi: 10.1146/annurev-ecolsys-112414-054234
Jing, M. L., Wang, Y. L., Ma, Y. S., and Li, S. X. (2019). Optimus conditions of Poa pratensis L. cv. Qinghai cultivation in Qilian mountain. Acta Agr. Bor. Occ. Sin. 28, 31–40. doi: 10.7606/j.issn.1004-1389.2019.01.005
Jing, M. L., Wang, Y. L., Ma, Y. S., Li, S. X., and Yang, H. Z. (2018). Effects of sowing dates on growth of Poa pratensis L. cv. Qinghai. Acta Agr. Bor. Occ. Sin. 27, 1754−1762. doi: 10.7606/j.issn.1004-1389.2018.12.007
Jones, M. B., Finnan, J., and Hodkinson, T. R. (2015). Morphological and physiological traits for higher biomass production in perennial rhizomatous grasses grown on marginal land. Glob. Change Bio. 7, 375–385. doi: 10.1111/gcbb.12203
Kleyer, M., Trinogga, J., Cebrián-Piqueras, M. A., Trenkamp, A., Fløjgaard, C., Ejrnæs, R., et al. (2019). Trait correlation network analysis identifies biomass allocation traits and stem specific length as hub traits in herbaceous perennial plants. J. Ecol. 107, 829–842. doi: 10.1111/1365-2745.13066
Lindsey, A. J., Thoms, A. W., and Christians, N. (2020). Kentucky bluegrass and bermudagrass rooting response to humic fertilizers during greenhouse establishment. Agron. J. 112, 3396–3401. doi: 10.1002/agj2.20199
Liu, Z. Y., Taogetao, B. Y., Sun, J., Hugjiltu, M., and Li, X. L. (2018). Plant sizes mediate mowing-induced changes in nutrient stoichiometry and allocation of a perennial grass in semi-arid grassland. Ecol. Evol. 8, 3109–3118. doi: 10.1002/ece3.3866
Mazzucato, A., Falcinelli, M., and Veronesi, F. (1996). Evolution and adaptedness in a facultatively apomictic grass, Poa pratensis L. Euphytica 92, 13–19. doi: 10.1007/BF00022823
Ning, Z. Y., Zhao, X. Y., Li, Y. L., Wang, L. L., Lian, J., Yang, H. L., et al. (2021). Plant community C: N: P stoichiometry is mediated by soil nutrients and plant functional groups during grassland desertification. Ecol. Eng. 162:106179. doi: 10.1016/j.ecoleng.2021.106179
Pang, X. P., Yang, H., Wei, X. X., and Guo, Z. G. (2021). Effect of plateau pika (Ochotona curzoniae) bioturbation on soil C-N-P stoichiometry in alpine meadows. Geoderma 397:115098. doi: 10.1016/j.geoderma.2021.115098
Pathak, K., Nath, A. J., Sileshi, G. W., Lal, R., and Das, A. K. (2017). Annual burning enhances biomass production and nutrient cycling in degraded imperata grasslands. Land Degrad. Dev. 28, 1763–1771. doi: 10.1002/ldr.2707
Persson, J., Fink, P., Goto, A., Hood, J. M., Jonas, J., and Kato, S. (2010). To be or not to be what you eat: regulation of stoichiometric homeostasis among autotrophs and heterotrophs. Oikos 119, 741–751. doi: 10.1111/j.1600-0706.2009.18545.x
Pi, E. R., Qu, L. Q., Tang, X., Peng, T. T., Jiang, B., Guo, J. F., et al. (2015). Application of genetic algorithm to predict optimal sowing region and timing for kentucky bluegrass in China. PLoS ONE 10:e0131489. doi: 10.1371/journal.pone.0131489
Poorter, H., Niklas, K. J., Reich, P. B., Oleksyn, J., Poot, P., Mommeret, L., et al. (2012). Biomass allocation to leaves, stems and roots: meta-analyses of interspecific variation and environmental control. New Phytol. 193, 30–50. doi: 10.1111/j.1469-8137.2011.03952.x
Robinson, D., Davidson, H., Trinder, C., and Brooker, R. (2010). Root-shoot growth responses during interspecific competition quantified using allometric modelling. Ann. Bot. 106, 921–926. doi: 10.1093/aob/mcq186
Shi, Z. M., Tang, J. C., Cheng, R. M., Luo, D., and Liu, S. R. (2015). A review of nitrogen allocation in leaves and factors in its effects. Acta Ecol. Sin. 35, 5909–5919. doi: 10.5846/stxb201401240184
Sokol, N. W., and Bradford, M. A. (2019). Microbial formation of stable soil carbon is more efficient from belowground than aboveground input. Nat. Geosci. 12, 46–53. doi: 10.1038/s41561-018-0258-6
Sokol, N. W., Kuebbing, S. E., Karlsen-Ayala, E., and Bradford, M. A. (2019). Evidence for the primacy of living root inputs, not root or shoot litter, in forming soil organic carbon. New Phytol. 221, 233–246. doi: 10.1111/nph.15361
Toledo, D., Swanson, K., Meehan, M., Dahlen, C., Christensen, R., Asplin, D., et al. (2023). Agronomic and forage nutritive responses of Kentucky bluegrass dominated pastures in the northern Great Plains. Grass Forage Sci. 78, 268–274. doi: 10.1111/gfs.12610
Walter, G. M., Clark, J., Terranova, D., Cozzolino, S., Cristaudo, A., Hiscock, S. J., et al. (2023). Hidden genetic variation in plasticity provides the potential for rapid adaptation to novel environments. New Phytol. 239, 374–387. doi: 10.1111/nph.18744
Wang, C., Wang, S., Fu, B. J., Li, Z. S., and Lü, Y. H. (2023). Plantation understorey legume functional groups enhance soil organic carbon sequestration by promoting species richness. Land Degrad Dev. 34, 2177–2188. doi: 10.1002/ldr.4598
Wang, Y., Niu, D., Yuan, X., Guo, D., Fu, H., Elser, J. J., et al. (2023). Dominant plant species alter stoichiometric imbalances between soil microbes and their resources in an alpine grassland: Implications for soil microbial respiration. Geoderma 431:116336. doi: 10.1016/j.geoderma.2023.116336
Wang, Z. N., Lu, J. Y., Yang, M., and Zhang, Q. (2015). Stoichiometric characteristics of carbon, nitrogen, and phosphorus in leaves of differently aged lucerne (Medicago sativa) stands. Front. Plant Sci. 6:1062. doi: 10.3389/fpls.2015.01062
Wei, Y., Ma, Z. Y., Zhou, J. Y., and Zhang, Z. H. (2022). Experimental warming changed reproductive phenology and height of alpine plants on the Qingzang Plateau. Chin. J. Plant Ecol. 46, 995–1004. doi: 10.17521/cjpe.2021.0450
Wen, J. H., Ma, Y. S., Shi, J. J., Dong, Q. M., and Wang, Y. L. (2006). Study on vegetation rebuilding of “black soil type” degraded grassland with Poa pratensis in the riparian areas of rivers. Grassland Turf 2, 41–44. doi: 10.13817/j.cnki.cyycp
Wu, G., Gao, J., Li, H., Ren, F., Liang, D., and Li, X. (2023). Shifts in plant and soil C, N, and P concentrations and C: N: P stoichiometry associated with environmental factors in alpine marshy wetlands in West China. Catena 221:106801. doi: 10.1016/j.catena.2022.106801
Xiao, L., Bi, Y., Du, S., Wang, Y., Guo, C., and Christie, P. (2021). Response of ecological stoichiometry and stoichiometric homeostasis in the plant-litter-soil system to re-vegetation type in arid mining subsidence areas. J. Arid Environ. 184:104298. doi: 10.1016/j.jaridenv.2020.104298
Xu, X. P., Liu, Y. J., and Wang, J. J. (2023). Density management diagrams for Larix gmelinii plantations in Daxing'an mountains. Natural Protected Areas 3, 79–88. doi: 10.12335/2096-8981.2023070601
Yan, B. G., Ji, Z. H., Fan, B., Wang, X., He, G., Shi, L., et al. (2016). Plants adapted to nutrient limitation allocate less biomass into stems in an arid-hot grassland. New Phytol. 211, 1232–1240. doi: 10.1111/nph.13970
Yang, D., Song, L., and Jin, G. (2019). The soil C: N: P stoichiometry is more sensitive than the leaf C: N: P stoichiometry to nitrogen addition: a four-year nitrogen addition experiment in a Pinus koraiensis plantation. Plant Soil 442, 183–198. doi: 10.1007/s11104-019-04165-z
Yu, Y. H., Zheng, W., Zhong, X. P., and Ying, B. (2021). Stoichiometric characteristics in Zanthoxylum planispinum var. dintanensis plantation of different ages. Agron. J. 113, 685–695. doi: 10.1002/agj2.20388
Yuan, X., Chen, Y., Qin, W., Xu, T., Mao, Y., Wang, Q., et al. (2021). Plant and microbial regulations of soil carbon dynamics under warming in two alpine swamp meadow ecosystems on the Tibetan Plateau. Sci. Total Environ. 790:148072. doi: 10.1016/j.scitotenv.2021.148072
Zeng, Q., Lal, R., Chen, Y., and An, S. (2017). Soil, leaf and root ecological stoichiometry of Caragana korshinskii on the Loess Plateau of China in relation to plantation age. PLoS ONE 12:e0168890. doi: 10.1371/journal.pone.0168890
Zhang, J., Wang, Q., Pang, X. P., Xu, H. P., Wang, J., Zhang, W. N., et al. (2021). Effect of partial root-zone drying irrigation (PRDI) on the biomass, water productivity and carbon, nitrogen and phosphorus allocations in different organs of alfalfa. Agr. Water Manage. 243:106525. doi: 10.1016/j.agwat.2020.106525
Zhang, K., Su, Y. Z., Liu, T. N., and Wang, T. (2016). Leaf C:N:P stoichiometrical and morphological traits of Haloxylon ammodendron over plantation age sequences in an oasis-desert ecotone in North China. Ecol. Res. 7, 185−191. doi: 10.1007/s11284-016-1353-z
Keywords: Kentucky bluegrass, ecologic stoichiometry, plantation age, leaf, stem, root, soil
Citation: Wei X, He K, Wang Q, Liu W, Pang X and Guo Z (2024) Effect of plantation age on plant and soil C:N:P stoichiometry in Kentucky bluegrass pastures. Front. Sustain. Food Syst. 8:1368418. doi: 10.3389/fsufs.2024.1368418
Received: 10 January 2024; Accepted: 15 February 2024;
Published: 05 March 2024.
Edited by:
Zhou Li, Guizhou University, ChinaReviewed by:
Xiaojun Yu, Gansu Agricultural University, ChinaGang Fu, Chinese Academy of Sciences (CAS), China
Copyright © 2024 Wei, He, Wang, Liu, Pang and Guo. This is an open-access article distributed under the terms of the Creative Commons Attribution License (CC BY). The use, distribution or reproduction in other forums is permitted, provided the original author(s) and the copyright owner(s) are credited and that the original publication in this journal is cited, in accordance with accepted academic practice. No use, distribution or reproduction is permitted which does not comply with these terms.
*Correspondence: Zhenggang Guo, guozhg@lzu.edu.cn