- 1School of Food Science and Chemical Engineering, Hubei University of Arts and Science, Xiangyang, China
- 2Analysis and Testing Center, School of Food Science and Chemical Engineering, Hubei University of Arts and Science, Xiangyang, China
Introduction: The contamination of farmland soil with the heavy metal cadmium (Cd) has consistently been a globally recognized environmental issue, especially with the rapid development of the central part of the Hanjiang River basin. The Cd contamination has become increasingly serious, and the accumulation and translocation of Cd in the soil-crop system warrant further research.
Methods: In this study, representative yellowish-brown soil and limestone soil from Hubei Province in China, as well as five varieties of potato (Solanum tuberosum L), were selected to investigate Cd accumulation and transfer regulation from soil to plants using pot tests at varying Cd concentration levels.
Results and discussion: For Eshu-12, Favorita, Huashu-6, Mira, and Zhongshu-5, the accumulation capacity of Cd in different parts exhibits a consistent pattern: Cdroot > Cdstem > Cdleaf > Cdtuber. The potato varieties exhibited distinct differences in Cd concentration, as follows: CdFavorita > CdMira > CdEshu-12 > CdHuashu-6 > CdZhongshu-5. The yellowish-brown soil demonstrated a higher accumulation and lower transport capacity to the plants of Cd, while limestone soil exhibited the opposite trend. The bio-concentration factor and transfer coefficients in yellowish-brown soil revealed significant (p < 0.05) accumulation and transformation of Cd in potatoes.
Conclusion and recommendations: This significant accumulation of Cd posed a higher risk of transfer through the food chain. Therefore, in the Hanjiang River basin, where Cd contamination is a concern, the cultivation of potatoes in limestone soil is more advisable than yellowish-brown soil to mitigate food safety risks.
Introduction
In the soil environment, heavy metals have long been recognized as serious pollutants due to their persistence, toxicity, and ability to bioaccumulate through the food chain (Ahmed et al., 2021). Therefore, heavy metal pollution is the most universal and influential problem in the world (Fang et al., 2019). Under the influence of human activities, cadmium (Cd) is one of the most widely toxic trace elements in agricultural soil and water (Rizwan et al., 2017). Globally, high-quality freshwater is a scarce natural resource in many river basins. The Hanjiang River, the largest tributary of the Yangtze River, extends over a distance of 1,577 km, traversing Shaanxi and Hubei Provinces and ultimately converging with the Yangtze River in Wuhan city. This river covers an area of 159,000 km2 (Li et al., 2023). The Xiangyang section of the Hanjiang River basin is in the middle reaches of Northwest Hubei Province, approximately 270 km long, and this area has wide river channels and abundant water, making it a developed area for industrial and agricultural cultivation. Therefore, Cd contamination risk exists in farmland soil in the central part of the Hanjiang River basin. The mean geochemical background value of Cd in the central Hanjiang River basin (0.27 mg kg−1) is 2.78 times that of the mean geochemical background values for China (0.097 mg kg−1) (Bi et al., 2005). In farmland soil within Northwest Hubei Province, Cd concentrations have been observed to range from 0.018 to 7.64 mg kg−1 (Li et al., 2006). The accumulation of Cd poses a significant environmental concern and presents a considerable risk to agricultural production.
The primary impact of Cd on plants is the disruption of physiological metabolism in the roots, resulting in inhibited root growth and reduced water and nutrient absorption, and this imbalance manifests as abnormalities in plant height, taproot length, leaf area, and various other physiological features, ultimately leading to nutritional deficiency (Jócsák et al., 2010; Wang et al., 2018). Additionally, Cd diminishes the activity of plant enzymes and disrupts the integrity of the cell membrane system, thereby exerting an influence on various physiological and biochemical processes in plants, ultimately reducing crop yields and quality (Ali et al., 2018). Cd can imbalance plant nutrients and reduce the quality of crop food. It will hinder the absorption of potassium, resulting in softening of the fruit and poor food flavor, which will affect the sales value of the food (Lai et al., 2020). The soil of rice, vegetables, and other foods contains too much Cd, which will cause chronic poisoning of the human body and endanger human health (Liu et al., 2021).
Apart from the concentration of total Cd in the soil, soil pH is a crucial factor that impacts the absorption of Cd by plants (Li et al., 2022). Other factors, such as the availability of Cd in the soil, plant varieties, and the presence of organic matter in the soil, should also be considered. Soil pH significantly affects the availability and morphological distribution of Cd in the soil (Oliveira et al., 2016), and pH is the most significant soil environmental factor influencing plant absorption of Cd (Scanlan et al., 2015). When the redox or alkaline acid balance changes, the high percentage of phyto-available Cd can reach up to 58%; the pH of the soil is a crucial factor influencing the soil Cd fraction, and carbonate-bound Cd can be significantly influenced by both pH and organic matter in the soil (Cheng et al., 2018; Ullah et al., 2019). In soil contaminated with heavy metals, Cd is primarily found in exchangeable and reducible fractions (Zygmunt and Kulikowska, 2016). A significant correlation exists between the Cd concentration in plants and the available Cd in the soil, and the available Cd in the soil has a greater impact than the total Cd concentration (Luo et al., 2019). The effect of organic matter on Cd activity was related to the variety of plants and the growing environment. The decomposition of organic substances in the soil environment can lead to the formation of stable complexes between Cd and organic acids, humic acids, and other small molecules, thereby reducing the activity of Cd (Hu et al., 2007; Xu et al., 2010). Ligands present in organic acids also form chelates with Cd ions in the soil solution, thereby enhancing the solubility and bioavailability of Cd in the soil (Zeng et al., 2011). The competition between cations such as calcium and magnesium present in the soil can hinder the absorption of Cd by plant roots, particularly Ca2+, which exhibits a higher affinity for clay minerals, oxides, and cation exchange adsorption sites within the soil (Kim et al., 2023).
Potato (Solanum tuberosum L) is a crucial staple food in numerous countries, ranking fourth after rice, wheat, and corn. Globally, more than 2.1 × 108 ha are dedicated to potato cultivation (Volkogon et al., 2021; Wang et al., 2021). China, the world’s largest potato producer, is followed by India and Russia. In China, potatoes are grown on approximately 4.61 × 106 ha per year, accounting for over 4.0% of the food crop area and yielding 1.83 × 107 tons of potatoes. The potato is a significant industrial and food crop in Hubei Province, with an annual planting area of 2.53 × 105 ha. Potato has been reported to have moderate tolerance to Cd (Ye et al., 2019), and in comparison to other Solanaceae vegetables such as black nightshade (Solanum nigrum L.) and aubergine (Solanum melongena), potato tubers are less prone to Cd accumulation. Otherwise, the potato roots exhibit an enhanced capacity for Cd accumulation, which is disadvantageous for Cd transfer into tubers (Mamun et al., 2017; Mengist et al., 2021). The yellowish-brown soil is prevalent in Hubei Province, spanning an area of 7.68 × 105 ha—accounting for 14.5% of the province’s total arable land (5.3 × 106 ha). Additionally, the limestone soil in Hubei covers an area of 3.63 × 105 ha, representing 6.85% of the province’s total arable area. Moreover, the Hanjiang River basin exhibits significant ecological vulnerability and faces elevated risks of Cd pollution. Therefore, it is imperative to investigate Cd adsorption in potato plants grown in yellowish-brown soil and limestone soil within the central Hanjiang River basin.
In this study, typical yellowish-brown soils and limestone soils were selected from the central region of the Hanjiang River basin in Hubei Province for pot experiments. Different concentrations of Cd were applied to various potato varieties to investigate the enrichment and transfer patterns of Cd within the soil–potato system and to provide valuable insights for ensuring safe potato production and the rational utilization of soil at risk of Cd contamination.
Materials and methods
Experimental plants and soils
Five potato varieties that are widely used in Hubei Province and have been cultivated by the Xiangyang Academy of Agricultural Sciences have been selected for this study: Eshu-12, Favorita, Huashu-6, Mira, and Zhongshu-5. Seedlings of these varieties were cultivated in accordance with the potato breeding matrix. (patent license CN 202111647906.9). Seed potatoes were placed in a wet matrix indoors (18–20°C). When the buds grew to approximately 1.0 cm, the seed potatoes were cut into pieces with a ceramic knife soaked in 0.3% potassium permanganate solution and dried in a ventilated place for 24 h. Healthy and growth-stable seedlings were selected and transplanted into the pots.
Acid yellowish-brown soil (burozem) and alkaline limestone soil (calcareous) (based on standard classification World Reference Base 2022) (WRB IWG, 2022) collected from two farmland areas (112°2′56.255″ E, 32°1′14.202″ N; 112°11′20.219″ E, 31°53′42.175″ N) in Xiangcheng District, Xiangyang City, Hubei Provence. This region is a subtropical monsoon area in the central part of the Hanjiang River basin; the climate is mild and humid, and the water is abundant, with an annual precipitation of 1,100 mm. Randomly collected surface soil (0–20 cm) was mixed using wooden spades and stored in cotton cloth bags. The soil samples were air-dried, and after removing any plant residues, they were sieved through a four-mesh nylon sieve.
Pot experiment design
The yellowish-brown soil and limestone soil were prepared with different Cd concentrations to simulate various Cd stress levels. The low Cd concentration soils were designed to have Cd levels below the risk screening standard for soil environmental pollution quality in agricultural land (pH ≤ 7.5, C = 0.3 mg kg−1; pH > 7.5, C = 0.6 mg kg−1) specified in National Standard (GB15618-2018, China) (Luo et al., 2020). The medium and high Cd concentration soils were set to exceed these standards. To achieve the desired Cd concentrations, exogenous Cd (CdCl2·2.5H2O) was added to the soils, resulting in a total Cd concentration of 0.6 mg kg−1 for the medium Cd soil and 6.0 mg kg−1 for the high Cd soil. The medium and high Cd soils represented the medium and high concentration levels of Cd stress treatment. The physicochemical properties of the soils used in this study are summarized in Table 1.
The pot experiments were conducted in a glasshouse at the School of Food Science and Technology, Hubei University of Arts and Science, Xiangyang (112°2′29.354″E, 32°0′6.752″ N) for 60 days. Plastic pots (26.0 cm high × 25.6 cm diameter) were used, and each pot contained 9.0 kg oven-dry base soil after the bottom of the pot was filled with gauze. To create Cd stress treatments, cadmium chloride (analytically pure) was dissolved in ultrapure water and evenly sprayed into the soil using a plastic watering can. The Cd concentration in the soil was mixed well to ensure homogeneity. Each treatment was repeated three times. After filling the pots with soil, the moisture content was maintained at approximately 70% of the field water holding capacity using the weight method for 336 h to allow the Cd concentration in the soil to stabilize. Potato seeds were provided by the Xiangyang Academy of Agricultural Sciences. When the potato sprouts grew to approximately 1.0 cm, well-grown seedlings were selected for transplanting, with one plant per pot. Throughout the growth stage, a solid compound fertilizer (the ratio of nitrogen, phosphorus, and potassium is 15:15:15) was applied to the soil in an amount of 9.0 g per pot. Weeding was performed every 4 weeks, and irrigation used the weighing method to maintain the soil moisture content at 70% of the field water holding capacity. Watering (purified water) was provided every 1–2 days until harvest. Trays were placed at the bottom of each pot to prevent soil loss during irrigation.
Sample collection
According to the different growth stages of potatoes, they were divided into the seedling stage at the sixth–eighth leaf flattening after transplanting, flowering stage, tuber formation stage, starch accumulation stage, and maturity stage. Potatoes were harvested after the maturity stage. The entire potato plants were carefully removed from the pots and transported back to the laboratory. They were then thoroughly rinsed with pure water to remove any adhering soil. The plants were divided into their respective organs: root, stem, leaf, and tuber (the edible portion). The tuber biomass was weighed to determine the fresh weight (g). All samples were then placed in paper bags and deactivated at 105°C in an oven for 5 min to inactivate enzymes. They were then oven-dried at 75°C until they reached a constant weight. The dry weight of each sample was recorded, and they were ground into a powder using a grinder. The powder was sieved through a 60-mesh nylon screen for further processing or analysis.
The plant samples collected were matched with the soil samples taken from corresponding layers. Soil samples were taken from the top to bottom layers, mixed thoroughly, and placed in clean sample bags. The bags were then stored in a cool, well-ventilated place to air dry. Using the four-part method, a portion of the soil samples was selected for grinding. Plant residues and crushed gravels were also included in the sample selection. All the samples were first filtered through a 20-mesh nylon screen. Once the sifted samples were well-mixed, a portion of them was removed for further grinding, passed through a 100-mesh nylon screen, and stored for future use.
Plant and soil sample analysis
Plant sample
Accurately weighed 0.200 g plant samples in a microwave digestion tank, 6.0 mL of HNO3 (guarantee reagent) was added, then 2.0 mL of H2O2 (guarantee reagent) was added after 2 h and let stand for 8 h in the high-pressure microwave digestion system (CEM Mars 6) at 180°C for 45 min. After cooling the acid to 1.0 mL, the solution was diluted to a constant volume of 20 mL using 3% dilute HNO3 (guarantee reagent). Finally, the Cd concentrations in the solutions were determined using an ICP-MS (Thermo Fisher Scientific X2) (Mamun et al., 2017; Dong et al., 2022).
Soil physicochemical properties
The soil pH was determined using a potentiometric method. The organic matter content in the soil was measured using the volumetric potassium dichromate method. The clay content in the soil was analyzed using a laser particle size analyzer (Malvern Panalytical Mastersizer 3000). Total nitrogen (N), available phosphorus (P), and potassium (K) were measured according to the Kjeldahl method (GB 7173-1987) (Madagoudra et al., 2021), combined extraction and colorimetry (NY/T 1848-2010) (Militaru et al., 2019), and combined extraction and colorimetry (NY/T 1849-2010) (Zhai et al., 2013), respectively.
Soil total cadmium
A measure of 0.100 g soil samples with a 100-mesh sieve was weighed and placed into the polytetrafluoroethylene inner tanks of the digestion kettle. A measure of 3.0 mL of HNO3 (guarantee reagent) and 3.0 mL of HF (guarantee reagent) was added and the mixture was allowed to sit for 8 h. Subsequently, 2.0 mL of HClO4 (guarantee reagent) was added, and the entire mixture was transferred to the metal outer tank of the digestion kettle. The kettle was then placed in the oven and heated to 180°C for 12 h to complete the digestion process. After the digestion was complete, the inner polytetrafluoroethylene tanks were placed on an electric hot plate and heated until the acid was drained to dryness, ensuring that no HF residue remained. Once dry, 1.0 mL of HNO3 (guarantee reagent) was added to dissolve any remaining solids. The volume was then diluted to 50 mL using 3% dilute HNO3 (guarantee reagent), and the Cd concentrations in the solutions were determined by ICP-MS (Thermo Fisher Scientific X2) (Zheng et al., 2021; Yuan et al., 2022).
Soil available cadmium
Accurately weighed 2.000 g soil samples with a 20-mesh sieve into a 50-mL centrifuge tube, mixed with 0.01 mol L−1 of CaCl2 solution (guarantee reagent), and shocked for 2 h to ensure thorough mixing, centrifuged at 3,500 rpm for 5 min and filtered. The Cd concentrations in the filtrate were then determined using ICP-MS (Thermo Fisher Scientific X2) (Guo et al., 2022).
Heavy metal evaluation criteria
The capacity for Cd enrichment and transfer in the soil–potato system was comprehensively analyzed. The bio-concentration factor (BCF) and transfer coefficients (TCs) were used as indicators of Cd′s enrichment and transfer capacity (Vystavna et al., 2015; Tudi et al., 2020). The BCF, defined as the ratio of heavy metal concentrations in potato tubers to those in the soil, served as a measure of the potato’s ability to accumulate heavy metals relative to the soil. The TC1, which compared heavy metal concentrations in the stem to those in the root, indicated the transfer capacity of heavy metals within the potato from root to stem. The TC2, which compared heavy metal concentrations in leaves to those in roots, highlighted the transfer capacity between root and leaf. Finally, the TC3, which compared heavy metal concentrations in tubers to those in roots, characterized the transfer capacity between root and tuber.
The potato tubers were rated based on the national food safety standard (GB 2762-2022, China), which limited Cd content to 0.1 mg kg−1 (Tian et al., 2022). To assess the overall soil Cd rating, the soil environmental quality standard was used to control soil pollution risks from farmland (Table 2). This standard defines a screening value for farmland soil pollution risk, referring to contaminants in soil from farmland that are equal to or less than the value, ensuring quality and safety. The risks to agricultural products, plant growth, and the soil’s ecological environment are considered low and can usually be ignored. However, if the value exceeds the threshold for the quality and safety of agricultural products, plant growth, and the soil ecological environment, it poses a risk that requires strengthened monitoring of the soil environment. Collaborative surveillance of agricultural products generally requires safety measures. The basis for assessment is the control of pollution in farmland, referring to values exceeding the pollutants’ standards in farmland. The risk of pollution is high, necessitating strict control measures in principle (Table 2) (Yang et al., 2019).

Table 2. Risk screening value and control value of soil Cd pollution in agricultural land (mg kg−1).
Statistical analyses
The inter-group comparison was conducted using a multi-factor, complete randomized analysis of variance. Factor 1 encompassed the soil’s pH, with yellowish-brown soil and limestone soil as categories. Factor 2 involved the potato varieties, including Eshu-12, Favorita, Huashu-6, Mira, and Zhongshu-5. Factor 3 encompassed the soil’s Cd concentration levels: low, medium, and high (Table 3). Blind controls and the national standard reference material, GSV-2, were included in every batch of samples to ensure quality control. Descriptive statistical analysis and data visualization were performed using Origin Pro 2021 (OriginLab Corp., Northampton) and Excel 2016 (Microsoft Corp., Waltham). Each value represents the mean of four repetitions ± standard deviation (SD). The correlation analysis was conducted using IBM SPSS Statistics Version 22.0 for Windows (IBM, Armonk). Statistical significance levels are denoted as * (p < 0.05) and ** (p < 0.01).
Results
Analysis of cadmium concentration in potato tubers
The Cd concentration (fresh weight concentration) in potato tubers based on their moisture content is shown in Figure 1. Notably, the Cd concentration in Eshu-12 was the lowest for limestone soil treatment (B1), at only 0.0077 mg kg−1. In contrast, the highest Cd concentration of 0.165 mg kg−1 was observed in yellowish-brown soil with Favorita (A6). When compared to the limit concentration of Cd in potato tuber (0.1 mg kg−1) specified by the National Food Safety Standard (GB2762-2022, China) (National Health Commission of the People’s Republic of China, 2022), seven treatments exceeded this limit: B12 (0.102 mg kg−1), B6 (0.110 mg kg−1), A15 (0.133 mg kg−1), A9 (0.136 mg kg−1), A3 (0.140 mg kg−1), A12 (0.150 mg kg−1), and A6 (0.165 mg kg−1). These concentrations exceeded the standard by 1.02–1.65 times, representing a rate of 23.33%.
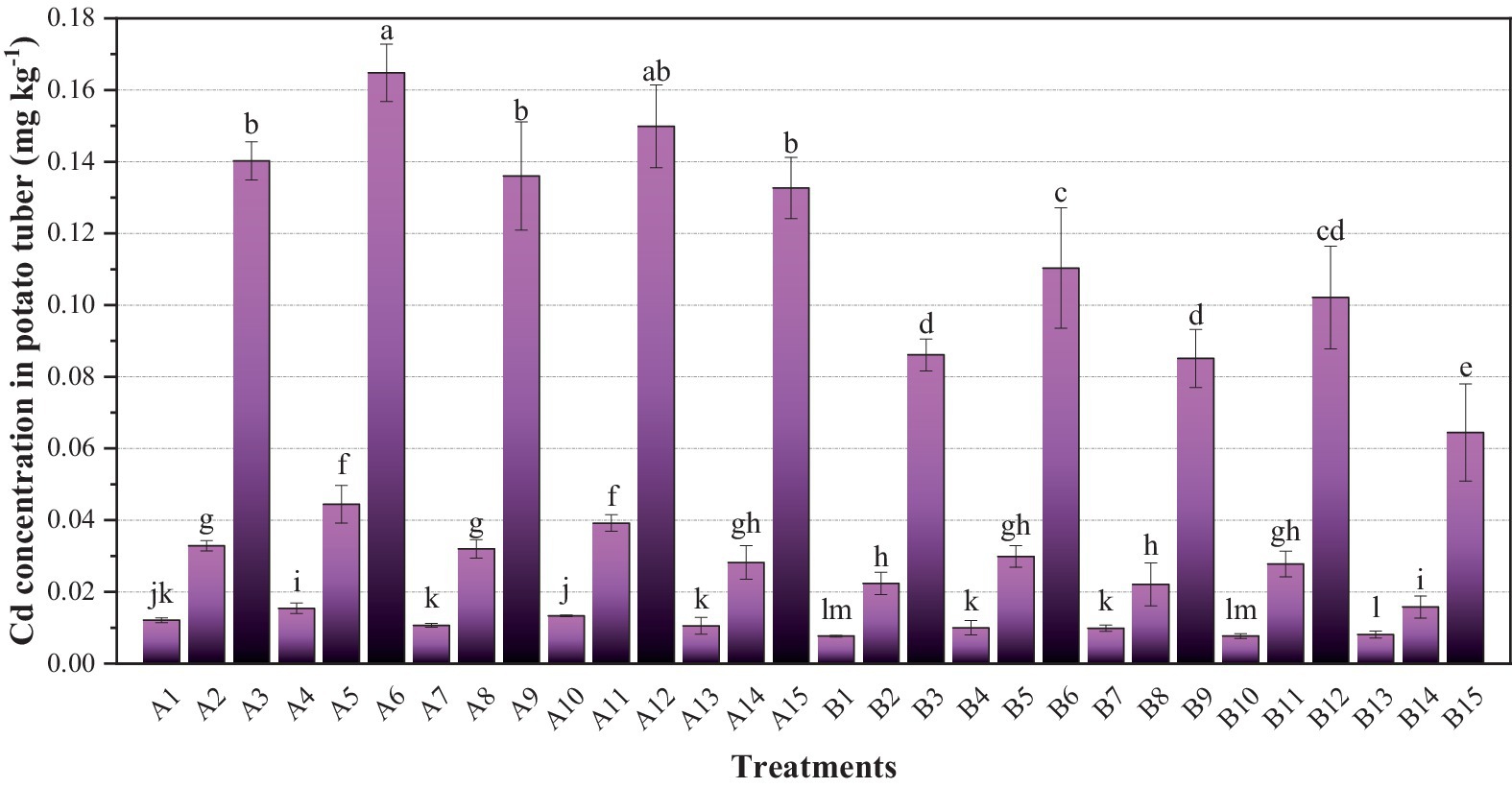
Figure 1. Cd concentrations in potato tubers (fresh weight). A1–B15 represent different potato varieties and soil types of potting treatments. Each value is the mean of three replicates ± standard derivation (SD). Different letters in the columns denote a significant difference at a p value of <0.05 between treatments.
To understand the relationship between Cd concentration in potato tubers and soil factors, as well as the effects on Cd tuber enrichment, Pearson correlation analysis was conducted on Cd in potato tuber, total Cd in soil, available Cd in soil, and soil pH (Table 4). The Pearson correlation coefficients between Cd concentration in potato tuber and total Cd in soil, as well as available Cd in soil, were 0.926** and 0.870**, respectively, indicating a very significant positive correlation. Similarly, there was a very significant positive correlation between total Cd and available Cd in soil (0.681**).
Enrichment of cadmium in different potato varieties
The yellowish-brown soil–potato system exhibited the Cd concentration in each part of the five potato varieties in Figure 2 (Cd concentrations were the dry weight concentrations, the same as below). Notably, there were no significant differences (p < 0.05) among the five potato varieties at low, medium, and high Cd levels. The various soil Cd concentrations of potato were classified as high Cd > medium Cd > low Cd; additionally, the Cd concentrations in the potato parts were arranged in the order of root > stem > leaf > tuber.
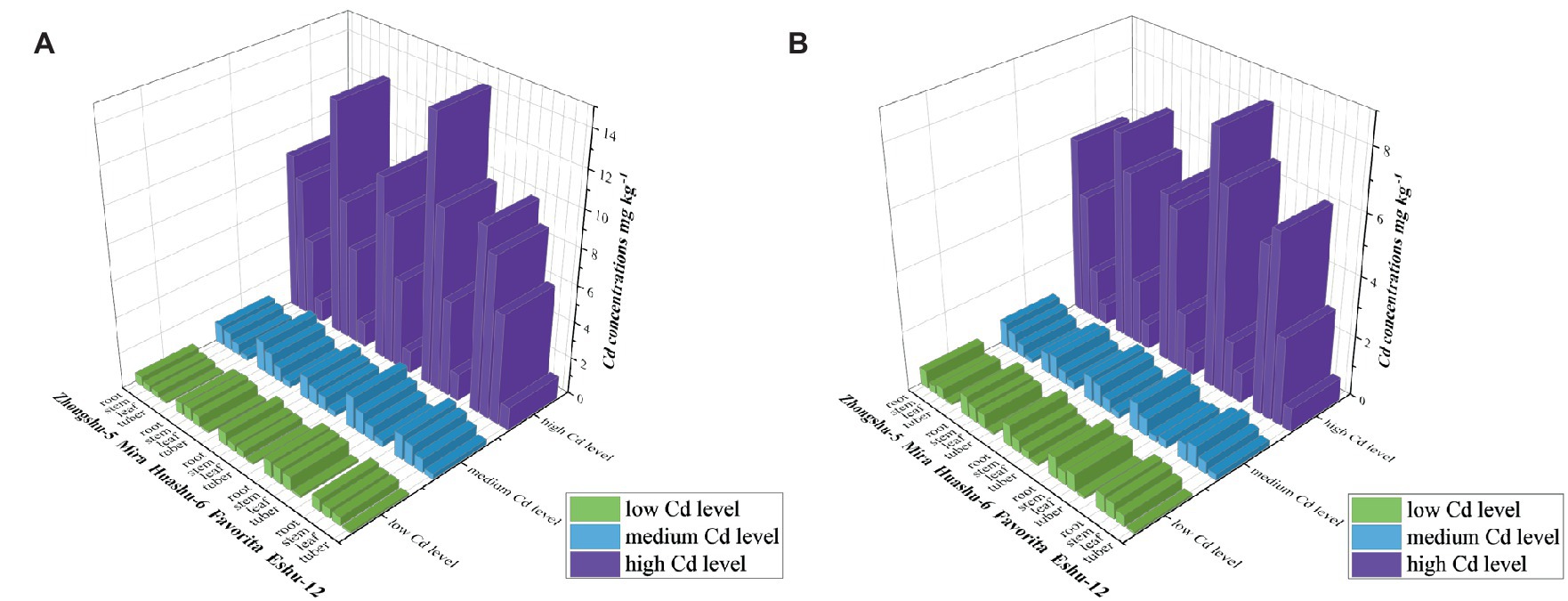
Figure 2. Cd concentration in different varieties of potato in soils (dry weight). (A) In yellowish-brown soil and (B) in limestone soil.
The Cd concentration in each part of the five potato varieties in the limestone soil–potato system is shown in Figure 2. Notably, there were no significant differences (p < 0.05) among the five potato varieties at low, medium, and high Cd levels. In various soil Cd concentrations, the Cd concentrations in potatoes were ranked as follows: high Cd > medium Cd > low Cd. Moreover, the Cd concentrations in the potato parts were arranged in the order of root > stem > leaf > tuber.
In the five potato varieties, the accumulation of Cd in the root was ranked as follows: Favorita > Mira > Huashu-6 > Eshu-12 > Zhongshu-5; for the stem and leaf, the accumulation order was Eshu-12 > Mira > Favorita > Huashu-6 > Zhongshu-5.
Enrichment and transfer of cadmium with potatoes in different soil types
The Cd concentrations of potatoes with low, medium, and high Cd levels in the yellowish-brown soil–potato system were in the range of 0.095–0.868, 0.254–1.766, and 1.194–14.481 mg kg−1, respectively. However, in the limestone soil–potato system, the Cd concentration in potatoes with low, medium, and high Cd levels was 0.069–0.715, 0.142–0.906, and 0.670–8.349 mg kg−1 (Figure 2). As the Cd concentration in the yellowish-brown and limestone soils increased, so did the Cd concentration in potatoes.
The Cd concentration in potato plants is not only influenced by the total Cd content in the soil but also by the available Cd. In the pot experiment, three levels of soil total Cd concentrations were established: low, medium, and high (Figure 3). The Cd concentration in the parts of potato plants grown in the yellowish-brown soil system exhibited different values. In contrast, in the limestone–soil–potato system, there was no significant difference between the medium and low values.
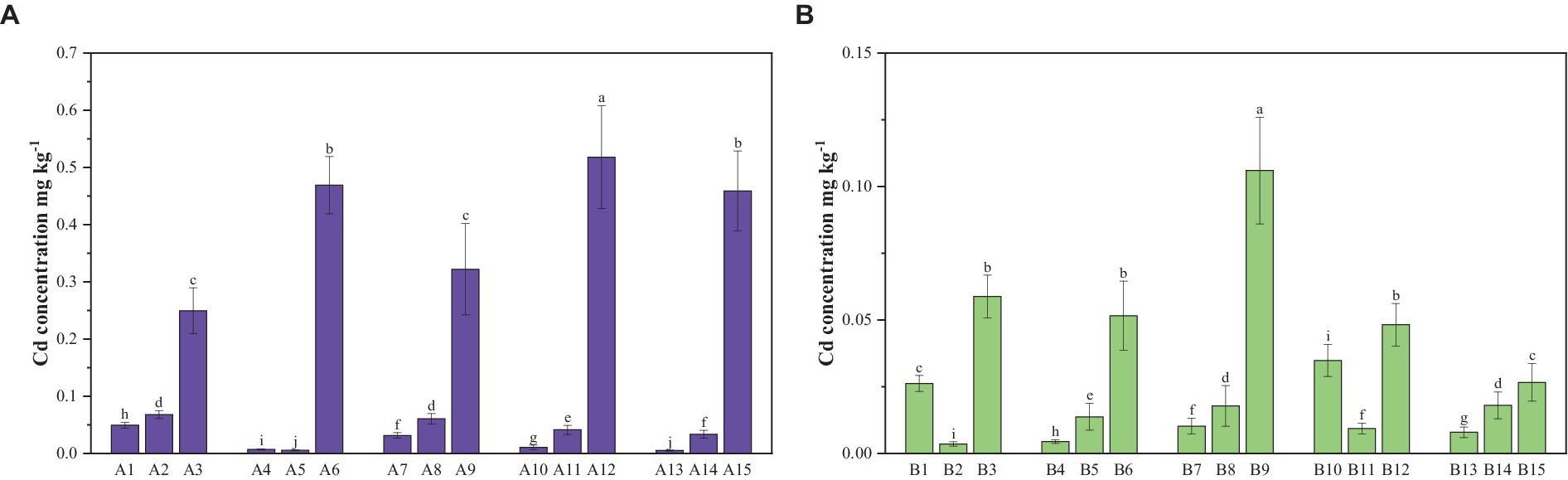
Figure 3. Available Cd concentrations in potting soils (dry weight). (A) In yellowish-brown soil with low, medium, and high Cd levels. (B) In limestone soil with low, medium, and high Cd levels. Each value is the mean of three replicates ± standard derivation (SD). Different letters in the columns denote a significant difference at a p value of <0.05 between treatments.
Cadmium transfer regularity in soil–potato system
The Cd concentration with the low Cd level in yellowish-brown soil was lower than that in limestone soil (0.14 and 0.18 mg kg−1), while the medium Cd level in two types of soil (0.62 and 0.61 mg kg−1) were consistent with those in the high Cd level (6.03 and 6.08 mg kg−1).
The BCF and TCs (TC1, TC2, and TC3) of Cd are shown in Figure 4. In the yellowish-brown soil–potato system, the BCFs of potato tuber at low, medium, and high Cd concentrations were 0.810, 0.541, and 0.226, while the TC1–TC3 values ranged from 0.769 to 0.816, 0.473 to 0.743, and 0.118 to 0.164, respectively. In the limestone soil–potato system, the BCFs of potatoes at low, medium, and high Cd concentrations were 0.451, 0.367, and 0.139, while the TC1–TC3 values ranged from 0.662 to 0.930, 0.340 to 0.618, and 0.119 to 0.287, respectively.
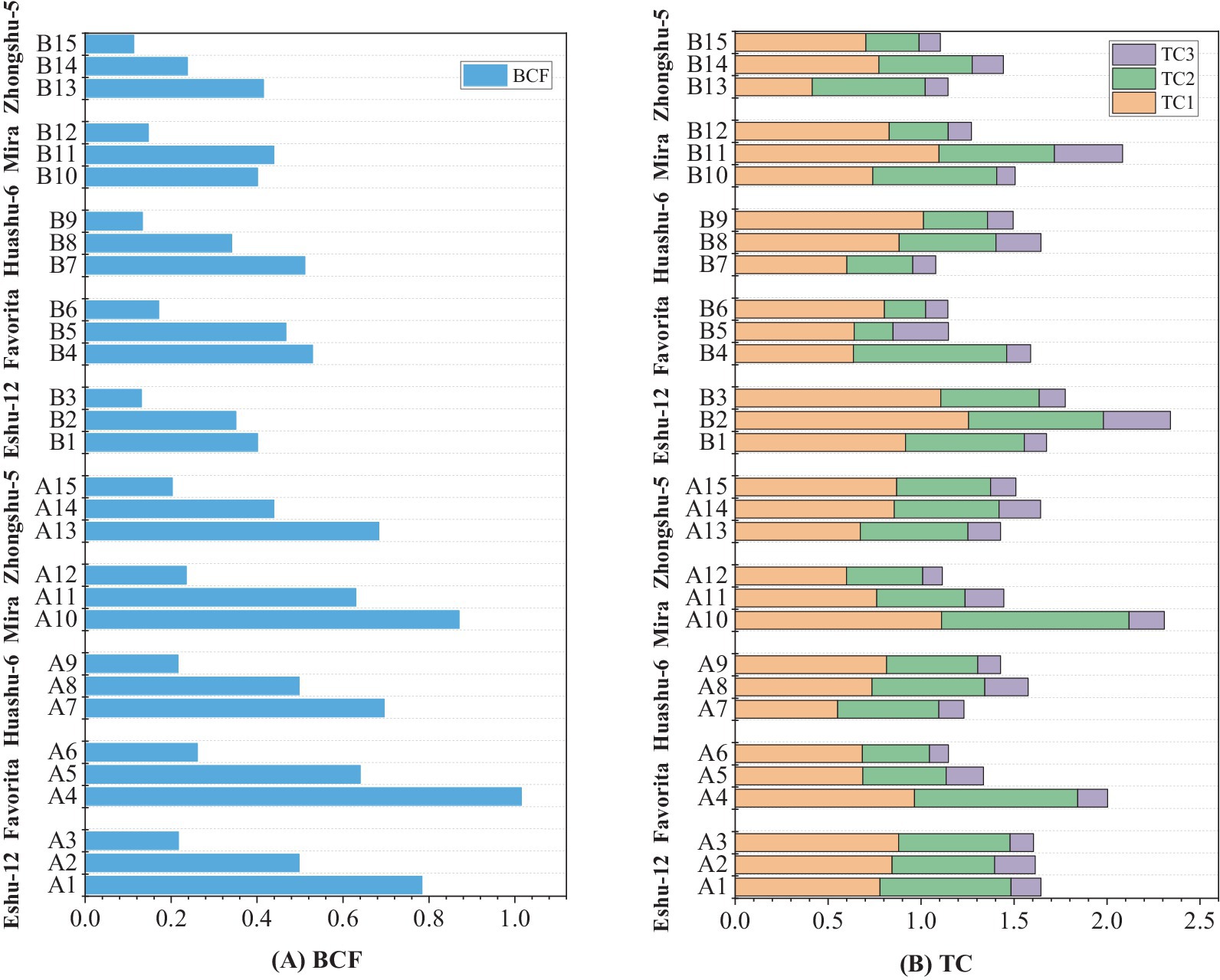
Figure 4. Bio-concentration factor (BCF) and transfer coefficient (TC) of potato in soils with different Cd concentration levels. (A) BCF of five varieties of potato, (B) TCs of five varieties of potato.
In yellowish-brown soil with a low level of Cd, the BCFs and TCs of potatoes were higher, ranging from 1.20 to 1.79 times those of the limestone soil (Figure 5). However, in limestone soil with medium and high Cd levels, the TCs of potatoes were 0.974–1.322 and 0.719–1.158 times, respectively, those in the yellowish-brown soil, while the BCFs were 0.614–0.679 times. It showed a higher enrichment and a lower transfer of Cd in yellowish-brown soil, while in limestone soil, potatoes showed a lower enrichment and a higher transfer of Cd.
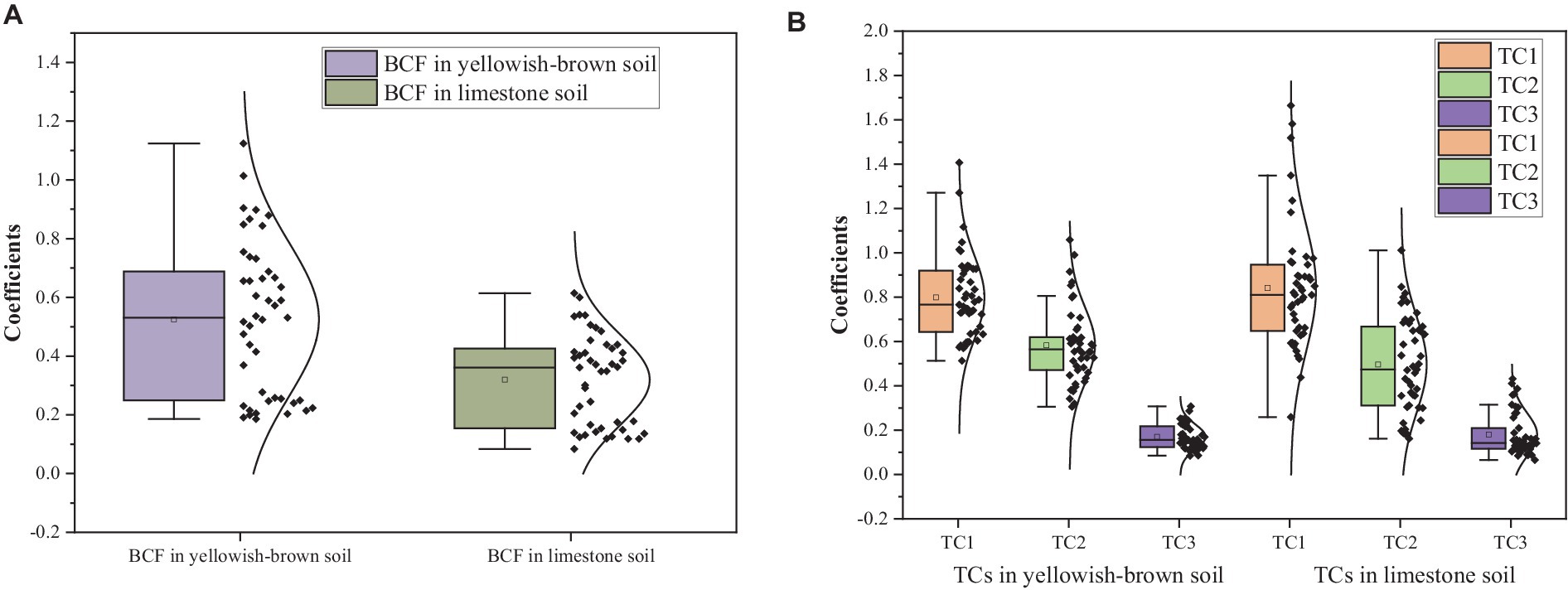
Figure 5. Box of bio-concentration factor (BCF) and transfer coefficient (TC) in soils. (A) BCF of potato in two types of soil and (B) TCs of potato in two types of soil.
Discussion
The accumulation of Cd causes serious environmental problems and poses great risks to crop and food safety. In this study, Cd concentrations were varied across potato varieties to explore Cd enrichment and transfer patterns within the soil–potato system and to provide valuable insights for ensuring potato food safety and the rational utilization of soil at risk of Cd contamination. In the highest Cd concentration of yellowish-brown soil, the tubers of all five varieties of potato exceeded the standard, but the standards were not exceeded in the low Cd and medium Cd concentrations. Except for the high Cd concentration treatments of Favorita and Mira, other tubers’ Cd concentrations in the lime soil did not exceed the standard. There was no significant difference (p < 0.05) in the Cd concentration of the tubers among the five varieties of potato. The concentration of Cd in potato tubers was positively correlated with the concentration of total Cd and available Cd in the soil. It suggests that as the total Cd in the soil increases, the Cd concentration in potato tubers also increases. At the same time, the total Cd in soil was positively correlated with the available Cd, indicating that with the increase of the available Cd in soil, the total Cd in soil also increased. It was similar to the research of some scholars (Carla et al., 2007; Sanderson et al., 2019), which has shown that the availability of Cd in soil plays a crucial role in its enrichment in potato tubers. Moreover, the correlation coefficients between soil pH and Cd in potato tubers, as well as available Cd, were − 0.233* and − 0.429*, respectively, indicating a significant negative correlation, indicating that as the soil pH increases, the concentration of available Cd in soil decreases (Hu et al., 2022), leading to a corresponding decrease in Cd concentration in potato tubers.
The distribution regularities of Cd concentration in the different parts of the five potato varieties were consistent, with the concentration of Cd highest in the potato root, followed by the stem and leaf, and lowest in the tuber, which was in line with the research of Mengist (Mengist et al., 2021). In most plants, the extracellular channels of the root have the highest Cd content, which is primarily concentrated in vacuoles and nuclei, while the intracellular Cd concentration is very low (Xin et al., 2013). When plants are exposed to Cd stress in their roots, Cd2+ is blocked from spreading to the central cortex by the Casparian band in the apoplast pathway, while when Cd2+ reaches the normally developed endodermis, the Casparian band in the cell wall prevents it from spreading further (Khan et al., 2019; Chen et al., 2022). In consequence, the Casparian band acts as a natural barrier, preventing the free diffusion of Cd2+ within plants. Cd2+ is transported through the vascular column, from envelope cells to the xylem, and can then be transported to the aerial part of the xylem and other plant parts (Xue et al., 2014). The allocation pattern of Cd in potato plants changes under Cd stress, resulting in a higher concentration of Cd in the root system compared to other organs, and this is probably the reason for the low Cd concentration in potato tubers. Mira and Huashu-6 showed no significant differences in stability compared to Favorita and Eshu-12 varieties in terms of Cd accumulation and translocation. However, Zhongshu-5 exhibited significant differences in stability from the other four varieties (p < 0.05). It suggests that the root of Favorita has a stronger ability to absorb Cd from the soil but a weaker ability to transport it upward, while the root of Eshu-12 has a lower ability to absorb Cd from the ground but a stronger ability to carry it upward. Satoru (Ishikawa et al., 2011) studied the Cd dynamic curves of Japonica rice varieties with low Cd content and Indica rice varieties with high Cd content and found that the root of Japonica rice varieties with low Cd content had a higher capacity for Cd absorption. Similar results were observed in studies comparing Cd accumulation among varieties of potato (Gonçalves et al., 2009; Moghadam and Shahmoradi, 2021; Pinheiro-Araújo et al., 2021).
In principle, the Cd concentration was significantly higher in each part of the potato grown in both yellowish-brown soil and limestone soil. However, there were notable differences in the low and medium Cd concentrations between the two soil types. When compared to potatoes grown in yellowish-brown soil, the medium concentration treatment had a higher Cd concentration than the low concentration treatment, and most of these differences were statistically significant (p < 0.05). In contrast, there was no significant difference in Cd concentration between the low and medium treatments in potatoes grown in limestone soil.
The concentration of Cd in different parts of potatoes planted in yellowish-brown soil was higher than that in limestone soil. This could potentially be attributed to the disparity in pH levels among the two soil types. The acid–base environment plays a crucial role in determining the surface charge of soil particles and the form of Cd2+, which dictates its transfer capacity (Luo et al., 2020). As the pH of the soil increases, Cd2+ exhibits a lower transfer capacity and is less likely to be absorbed by the vegetation (Stephan et al., 2013). The addition of clay to soil can mitigate the negative impact of Cd by adsorbing the Cd2+ onto the clay particles and stabilizing soil enzymes (Shahriari and Tamura, 2010). The clay content of yellowish-brown soil was lower than that of limestone soil, which might also be the reason for the higher Cd accumulation. Furthermore, the higher concentration of Ca2+ in limestone soil can inhibit the absorption of Cd2+ by potato plants. Previous studies have shown that Ca2+ competes with Cd2+ for absorption sites at the roots (Castaldi et al., 2009; Lahori et al., 2017). At concentrations of 1.0–2.0 mmol L−1, Ca2+ can lower the Cd concentration in crop roots and shoots, enhance the absorption and accumulation of essential elements, and mitigate oxidative damage caused by Cd stress in crop seedlings (Zhang et al., 2012). The lack of Ca2+ would intensify the vulnerability to Cd2+ stress in wheat, but a surge in Ca2+ could significantly mitigate the toxic impact of Cd2+ stress. When Ca2+ levels were elevated, the Cd concentration in wheat was noticeably reduced (Plasencia et al., 2021).
The Cd concentration with the low Cd level in yellowish-brown soil was lower than that in limestone soil. It suggests that potatoes grown in yellowish-brown soil are more prone to Cd enrichment compared to limestone soil, posing a higher safety risk for Cd stress. It is likely due to the dissimilar pH levels and other physicochemical properties between the two types of soil, as these properties significantly influence the vegetative ability to accumulate Cd (Zhang et al., 2018; Zheng et al., 2021). In the yellowish-brown soil–potato system, the BCF was the highest at 1.12, which indicates that the tubers of the five potato varieties could easily accumulate Cd from the soil. However, the mean TC1, TC2, and TC3 were all less than 1, indicating that the Cd transportability from the root to the stem and from the stem to the tuber was restricted after it was absorbed into the root system. Moreover, the transport of Cd from the root to the tuber was the weakest. The BCFs of five potato varieties were less than 1 in the limestone soil–potato system, indicating that the enrichment of soil Cd by the tuber in limestone soil was less. Additionally, TC3 was less than 1, while 6.67% of TC2 and 26.7% of TC1 were greater than 1. It suggested that the Cd transportability from the root to tuber of potato was more limited, while that from the root, stem, and leaf was less limited comparatively.
Two distinct types of soil were utilized in this study, each possessing unique physicochemical properties that ultimately influenced the Cd absorption and transportation properties of potatoes. At identical concentrations of Cd, the accumulation of Cd in grains can vary significantly, and it is possible for grains with a lower overall Cd content to exhibit a higher concentration of Cd than those grown in soils with higher Cd levels (Huang et al., 2017). In addition to the physicochemical properties of the soil, the Cd concentrations in the soil also play a significant role in determining the absorption and transportation of Cd (Cui et al., 2021). Semane et al. (2010) discovered that at lower Cd concentrations in the rhizosphere, the absorbed Cd2+ was primarily transported to the aboveground parts of the plant. As the Cd concentration rose, the Cd2+ in the plant root system increased rapidly until it reached balance with the environment (Katz and Chamovitz, 2017). This study observed that the concentration of available Cd in limestone soil was significantly lower than that in yellowish-brown soil, leading to a relatively low Cd level. In the limestone soil–potato system, the root system accumulated more Cd and transported Cd2+ to stem and leaf parts with lower Cd levels, which generally resulted in less Cd accumulation and more transfer.
Conclusion
The accumulation capacity of Cd in various parts of potato plants was ranked as follows: Cdroot > Cdstem > Cdleaf > Cdtuber, and the Cd accumulation among five potato varieties was as follows: Favorita > Mira > Eshu-12 > Huashu-6 > Zhongshu-5. The results indicate that it is safe to grow potatoes in medium and low Cd risk soil, with the Huashu-6 and Zhongshu-5 varieties accumulating less Cd and posing a lower risk.
In the soil–potato system, the higher pH, clay content, and calcium content of limestone soil, along with the lower available Cd concentration, might explain why different varieties of potatoes in yellowish-brown soil accumulate higher levels of Cd. Correlation analysis further indicated a significant relationship between the Cd concentration in potato plants and the levels of total and available Cd in the soil.
The BCF and TCs revealed that the five potato varieties were different in two types of soil. The enrichment and transfer abilities of Cd in potatoes were more significant in yellowish-brown soil (p < 0.05) and posed a higher risk of transfer through the food chain. It is suggested that choosing limestone soil as potato planting soil is more beneficial to reduce food safety risks than yellowish-brown soil in the Hanjiang River basin with Cd contamination risk.
Data availability statement
The original contributions presented in the study are included in the article/supplementary material, further inquiries can be directed to the corresponding author.
Author contributions
XQ: Investigation, Methodology, Software, Writing – original draft. KL: Data curation, Funding acquisition, Project administration, Writing – original draft, Writing – review & editing. YL: Supervision, Writing – review & editing. YZ: Formal analysis, Investigation, Methodology, Writing – review & editing. YF: Data curation, Investigation, Writing – original draft. MY: Formal analysis, Investigation, Writing – original draft.
Funding
The author(s) declare that financial support was received for the research, authorship, and/or publication of this article. This research was financially supported by the National Natural Science Foundation of China (grant number 42207525), the Xiangyang Science and Technology Project (grant number 2021ABA003624), the Science and Technology Support Action Plan of the Hubei Provincial Department of Education (grant number BXLBX0693), and the Hubei Natural Science Foundation Innovation and Development Joint Fund Project (grant number 2022CFD099).
Conflict of interest
The authors declare that the research was conducted in the absence of any commercial or financial relationships that could be construed as a potential conflict of interest.
Publisher’s note
All claims expressed in this article are solely those of the authors and do not necessarily represent those of their affiliated organizations, or those of the publisher, the editors and the reviewers. Any product that may be evaluated in this article, or claim that may be made by its manufacturer, is not guaranteed or endorsed by the publisher.
References
Ahmed, S. F., Kumar, P. S., Rozbu, M. R., Chowdhury, A. T., Nuzhat, S., Rafa, N., et al. (2021). Heavy metal toxicity, sources, and remediation techniques for contaminated water and soil. Environ. Technol. Innov. 25:102114. doi: 10.1016/j.eti.2021.102114
Ali, E., Hussain, N., Shamsi, I. H., Jabeen, Z., and Jiang, L. X. (2018). Role of jasmonic acid in improving tolerance of rapeseed (Brassica napus L.) to cd toxicity. J Zhejiang Univ Sci B 19, 130–146. doi: 10.1631/jzus.B1700191
Bi, X. Y., Ma, Z. D., Ren, L. M., Gong, M., and Jin, Z. S. (2005). Distribution of trace elements and cadmium forms in sediments from the middle reaches of Yangtze river, Hubei Province. Environ. Chem. 33, 19–24. doi: 10.1007/s10971-005-6694-y
Carla, O., Carlo, V., and Erik, S. (2007). Elevated cadmium concentrations in potato tubers due to irrigation with river water contaminated by Mining in Potosí, Bolivia. J. Environ. Qual. 36, 1181–1186. doi: 10.2134/jeq2006.0401
Castaldi, P., Melis, P., Silvetti, M., Deiana, P., and Garau, G. (2009). Influence of pea and wheat growth on Pb, Cd, and Zn mobility and soil biological status in a polluted amended soil. Geoderma 151, 241–248. doi: 10.1016/j.geoderma.2009.04.009
Chen, F., Bashir, A., Rehman, M. Z. U., Adrees, M., Qayyum, M. F., Ma, J., et al. (2022). Combined effects of green manure and zinc oxide nanoparticles on cadmium uptake by wheat (Triticum aestivum L.). Chemosphere 298:134348. doi: 10.1016/j.chemosphere.2022.134348
Cheng, S., Liu, G., Zhou, C., and Sun, R. (2018). Chemical speciation and risk assessment of cadmium in soils around a typical coal mining area of China. Ecotoxicol. Environ. Saf. 160, 67–74. doi: 10.1016/j.ecoenv.2018.05.022
Cui, S., Gao, S., Zhang, F., Fu, Q., Wang, M., Liu, D., et al. (2021). Heavy metal contamination and ecological risk in sediment from typical suburban rivers. River Res. Appl. 37, 1080–1088. doi: 10.1002/rra.3701
Dong, Q., Xiao, C., Cheng, W., Yu, H., Shi, J., Yin, Y., et al. (2022). Cadmium isotope analysis of environmental reference materials via microwave digestion-resin purification-double-spike MC-ICP-MS. At. Spectrosc. 43, 145–153. doi: 10.46770/AS.2021.1109
Fang, T., Yang, K., Lu, W., Cui, K., and Li, H. (2019). An overview of heavy metal pollution in Chaohu Lake, China: enrichment, distribution, speciation, and associated risk under natural and anthropogenic changes. Environ. Sci. Pollut. Res. 26, 29585–29596. doi: 10.1007/s11356-019-06210-x
Gonçalves, J. F., Antes, F. G., Maldaner, J., Pereira, L. B., Tabaldi, L. A., Rauber, R., et al. (2009). Cadmium and mineral nutrient accumulation in potato plantlets grown under cadmium stress in two different experimental culture conditions. Plant Physiol. Biochem. 47, 814–821. doi: 10.1016/j.plaphy.2009.04.002
Guo, J., Chang, X., Chen, L., Liu, X., Jia, S., Chen, Y., et al. (2022). Dynamic changes in the intestinal microbial community of two time-aged soils under combined cadmium and ciprofloxacin contaminated conditions. Sci. Total Environ. 806:150558. doi: 10.1016/j.scitotenv.2021.150558
Hu, X., Li, F., Pan, Y., Li, Z., Lu, J., Li, Y., et al. (2022). A novel BC-HMRPGPB-plant system for remediation of low concentration cd in soil: the process of metal migration and microbial community evolution. J. Clean. Prod. 360, 132164–132113. doi: 10.1016/j.jclepro.2022.132164
Hu, H.-Q., Liu, H.-L., He, J.-Z., and Huang, Q.-Y. (2007). Effect of selected organic acids on cadmium sorption by variable-and permanent-charge soils. Pedosphere 17, 117–123. doi: 10.1016/S1002-0160(07)60016-2
Huang, G., Ding, C., Guo, F., Li, X., Zhou, Z., Zhang, T., et al. (2017). The role of node restriction on cd accumulation in the brown rice of twelve Chinese rice (Oryza sativa L.) cultivars. Journal of agricultural. Food Chem. 65, 10157–10164. doi: 10.1021/acs.jafc.7b03333
Ishikawa, S., Suzui, N., Ito-Tanabata, S., Ishii, S., and Fujimaki, S. (2011). Real-time imaging and analysis of differences in cadmium dynamics in rice cultivars (Oryza sativa) using positron-emitting107Cd tracer. BMC Plant Biol. 11, 172–183. doi: 10.1186/1471-2229-11-172
Jócsák, I., Droppa, M., Horváth, G., Bóka, K., and Vozáry, E. (2010). Cadmium-and flood-induced anoxia stress in pea roots measured by electrical impedance. C J. Biosci. 65, 95–102. doi: 10.1515/znc-2010-1-216
Katz, E., and Chamovitz, D. A. (2017). Wounding of Arabidopsis leaves induces indole-3-carbinol-dependent autophagy in roots of Arabidopsis thaliana. Plant J. Cell Mol. Biol. 91, 779–787. doi: 10.1111/tpj.13610
Khan, Z. S., Rizwan, M., Hafeez, M., Ali, S., Javed, M. R., and Adrees, M. (2019). The accumulation of cadmium in wheat (Triticum aestivum) as influenced by zinc oxide nanoparticles and soil moisture conditions. Environ. Sci. Pollut. Res. 26, 19859–19870. doi: 10.1007/s11356-019-05333-5
Kim, S., An, H., Kim, C., and Yang, K. (2023). Mineralogy, geochemistry, and sources of clay minerals in sediments of the Ulleung Basin, East Sea (sea of Japan). Geosci. J. 27, 385–397. doi: 10.1007/s12303-023-0011-z
Lahori, A. H., Zhang, Z., Guo, Z., Mahar, A., Li, R., Sial, T. A., et al. (2017). Potential use of lime combined with additives on (im)mobilization and phytoavailability of heavy metals from Pb/Zn smelter contaminated soils. Ecotoxicol. Environ. Saf. 145, 313–323. doi: 10.1016/j.ecoenv.2017.07.049
Lai, J. L., Liu, Z. W., Chen, L. I., and Luo, X. G. (2020). Analysis of accumulation and phytotoxicity mechanism of uranium and cadmium in two sweet potato cultivars. J. Hazard. Mater. 409:124997. doi: 10.1016/j.jhazmat.2020.124997
Li, Y., Wang, J., Lin, C., Lian, M., Wang, A., He, M., et al. (2023). Riverine antibiotic occurrence and potential ecological risks in a low-urbanized and rural basin of the middle Yangtze River: socioeconomic, land use, and seasonal effects. Environ. Res. 228:115827. doi: 10.1016/j.envres.2023.115827
Li, W. L. I., Zhang, J., and Ma, Z. (2006). Environmental geochemistry of the cadmium contaminated soil in Luoqiao area, Hubei Province. Geol. Res. 3, 1–6. doi: 10.3969/j.issn.1671-1947.2006.03.011
Li, C., Zhang, C., Yu, T., Liu, X., Yang, Y., Hou, Q., et al. (2022). Use of artificial neural network to evaluate cadmium contamination in farmland soils in a karst area with naturally high background values. Environ. Pollut. 304, 119234–119210. doi: 10.1016/j.envpol.2022.119234
Liu, K., Liu, H., Zhou, X., Chen, Z., and Wang, X. (2021). Cadmium uptake and translocation by potato in acid and calcareous soils. Bull. Environ. Contam. Toxicol. 107, 1149–1154. doi: 10.1007/s00128-021-03377-3
Luo, K., Liu, H., Yu, E., Tu, Y., and Xu, M. (2020). Distribution and release mechanism of heavy metals in sediments of Yelang Lake by DGT. Stoch. Env. Res. Risk A. 34, 793–805. doi: 10.1007/s00477-020-01799-9
Luo, L. Y., Xie, L. L., Jin, D. C., Mi, B. B., Wang, D. H., Li, X. F., et al. (2019). Bacterial community response to cadmium contamination of agricultural paddy soil. Appl. Soil Ecol. 139, 100–106. doi: 10.1016/j.apsoil.2019.03.022
Madagoudra, Y. B., Chaithanya, J., Terin, P., and Lokesh, R. (2021). Effect of rate and time of nitrogen application on nutrient content and uptake in summer maize (Zea mays L.) and soil nutrient status after harvest. Int. J. Econ. Plants 8, 57–61. doi: 10.23910/2/2021.0410a
Mamun, S. A., Lehto, N. J., Cavanagh, J., Mcdowell, R., and Robinson, B. H. (2017). Effects of lime and organic amendments derived from varied source materials on cadmium uptake by potato. J. Environ. Qual. 46, 836–844. doi: 10.2134/jeq2017.02.0059
Mengist, M. F., Byrne, S. L., Griffin, D., and Milbourne, D. (2021). A note on the early transcriptional response in leaves and root of potato plants to cadmium exposure. Irish J. Agric. Food Res. 60, 27–32. doi: 10.15212/ijafr-2020-0124
Militaru, B. A., Pode, R., Manea, F., and Linul, P. A. (2019). Simple and combined acidic extraction of phosphorus from sewage sludge ash. Rev. Chim. 70, 133–139. doi: 10.37358/RC.19.1.6867
Moghadam, A. D., and Shahmoradi, A. (2021). Heavy metals accumulation and health risk consumption in some vegetables, Isfahan, Iran. Annal. Milit. Health Sci. Res. 19:e101311. doi: 10.5812/amh.101311
National Health Commission of the People’s Republic of China (2022). National standards for food safety—limits of contaminants in food.
Oliveira, V. H. D., Melo, L. C. A., Abreu, C. A., and Coscione, A. R. (2016). Influences of soil pH on cadmium toxicity to eight plant species. Ecotoxicol. Environ. Contam. 11, 45–52. doi: 10.5132/eec.2016.01.07
Pinheiro-Araújo, L., Camargos De-Oliveira, R., Quintao-Lana, R. M., Queiroz-Luz, J. M., Apolinário-Guimares, J. P., and De Oliveira-Alves, E. (2021). Accumulation of macronutrients and productivity of potato with foliar application of biofertilizer. Int. J. Agric. Nat. Res. 48, 70–82. doi: 10.7764/ijanr.v48i2.2269
Plasencia, F. A., Fortes, Y. E., Flores, F., and Egea, I. (2021). The ca(2+) sensor Calcineurin B-like protein 10 in plants: emerging new crucial roles for plant abiotic stress tolerance. Front. Plant Sci. 11:2155. doi: 10.3389/fpls.2020.599944
Rizwan, M., Ali, S., Hussain, A., Ali, Q., Shakoor, M. B., Zia-Ur-Rehman, M., et al. (2017). Effect of zinc-lysine on growth, yield and cadmium uptake in wheat (Triticum aestivum L.) and health risk assessment. Chemosphere 187, 35–42. doi: 10.1016/j.chemosphere.2017.08.071
Sanderson, D.-V., Voutchkov, M., and Benkeblia, N. (2019). Bioaccumulation of cadmium in potato tuber grown on naturally high levels cadmium soils in Jamaica. Sci. Total Environ. 649, 909–915. doi: 10.1016/j.scitotenv.2018.08.220
Scanlan, C., Brennan, R., and Sarre, G. A. (2015). Effect of soil pH and crop sequence on the response of wheat (Triticum aestivum) to phosphorus fertiliser. Crop Past. Sci. 66, 23–31. doi: 10.1071/CP14192
Semane, B., Cuypers, A., Smeets, K., Belleghem, F. V., Horemans, N., Schat, H., et al. (2010). Cadmium responses in Arabidopsis thaliana: glutathione metabolism and antioxidative defence system. Physiol. Plant. 129, 519–528. doi: 10.1111/j.1399-3054.2006.00822.x
Shahriari, F., and Tamura, K. (2010). Effects of clay addition on soil protease activities in andosols in the presence of cadmium. Soil Sci. Plant Nutr. 56, 560–569. doi: 10.1111/j.1747-0765.2010.00491.x
Stephan, C., Mark, G. M. A., Sébastien, T., and Nathalie, V. (2013). Plant science: the key to preventing slow cadmium poisoning. Trends Plant Sci. 18, 92–99. doi: 10.1016/j.tplants.2012.08.003
Tian, W., Huang, Y., Li, D., Meng, L., He, T., and He, G. (2022). Identification of StAP2/ERF genes of potato (Solanum tuberosum) and their multiple functions in detoxification and accumulation of cadmium in yest: implication for genetic-based phytoremediation. Sci. Total Environ. 810:152322. doi: 10.1016/j.scitotenv.2021.152322
Tudi, M., Ruan, H. D., Yu, Y., Wang, L., and Yang, L. S. (2020). Bioaccumulation and translocation of trace elements in soil-irrigation water-wheat in arid agricultural areas of Xin Jiang, China. Ecotoxicology 30, 1290–1302. doi: 10.1007/s10646-020-02267-4
Ullah, M., Nazir, R., Khan, M., Khan, W., and Zada, A. (2019). The effective removal of heavy metals from water by activated carbon adsorbents of Albizia lebbeck and Melia azedarach seed shells. Soil Water Res. 15, 30–37. doi: 10.17221/212/2018-SWR
Volkogon, V. V., Dimova, S. B., Volkogon, K. I., Sidorenko, V. P., and Volkogon, M. V. (2021). Biological nitrogen fixation and denitrification in rhizosphere of potato plants in response to the fertilization and inoculation. Front. Sustain. Food Syst. 5:606379. doi: 10.3389/fsufs.2021.606379
Vystavna, Y., Rätsep, R., Klymenko, N., Drozd, O., Pidlisnyuk, V., and Klymenko, M. (2015). Comparison of soil-to-root transfer and translocation coefficients of trace elements in vines of chardonnay and Muscat white grown in the same vineyard. Sci. Hortic. 192, 89–96. doi: 10.1016/j.scienta.2015.05.019
Wang, Y., Wang, C., Liu, Y., Yu, K., and Zhou, Y. (2018). GmHMA3 sequesters cd to the root endoplasmic reticulum to limit translocation to the stems in soybean. Plant Sci. 270, 23–29. doi: 10.1016/j.plantsci.2018.02.007
Wang, L., Zhang, J., and Liu, C. (2021). Antioxidant activity of potato seedlings at different storage temperatures. Int. J. Chem. Eng. 2021, 1–11. doi: 10.1155/2021/5573644
WRB IWG (2022). World Reference Base for soil resources, international soil classification system for naming soils and creating legends for soil maps 4th edition, 2022. World Soil Resources Reports No. 106. FAO, Rome, International Union of Soil Sciences.
Xin, J., Huang, B., Yang, Z., Yuan, J., and Zhang, Y. (2013). Comparison of cadmium subcellular distribution in different organs of two water spinach (Ipomoea aquatica Forsk.) cultivars. Plant Soil 372, 431–444. doi: 10.1007/s11104-013-1729-6
Xu, W., Li, Y., He, J., Ma, Q., Zhang, X., Chen, G., et al. (2010). Cd uptake in rice cultivars treated with organic acids and EDTA. J. Environ. Sci. 22, 441–447. doi: 10.1016/S1001-0742(09)60127-3
Xue, M., Zhou, Y., Yang, Z., Lin, B., Yuan, J., and Shanshan, W. U. (2014). Comparisons in subcellular and biochemical behaviors of cadmium between low-cd and high-cd accumulation cultivars of pakchoi (Brassica chinensis L.). Front. Environ. Sci. Eng. 8, 226–238. doi: 10.1007/s11783-013-0582-4
Yang, Y. U., Wenqing, L., Renji, X. U., Linlin, Z., Cong, S., and Fusheng, W. (2019). Investigation of agricultural land pollution in typical areas and discussion on risk control standards. Environ. Monitor. Chin. 35, 1–7. doi: 10.3969/j.issn.1002-6002.2019.35.007
Ye, Y., Dong, W., Luo, Y., Fan, T., and Hu, X. (2019). Cultivar diversity and organ differences of cadmium accumulation in potato (Solanum tuberosum L.) allow the potential for cd-safe staple food production on contaminated soils. Sci. Total Environ. 711:134534. doi: 10.1016/j.scitotenv.2019.134534
Yuan, B., Huang, L., Liu, X., Bai, L., Liu, H., Jiang, H., et al. (2022). Application of mixotrophic acidophiles for the bioremediation of cadmium-contaminated soils elevates cadmium removal, soil nutrient availability, and rice growth. Ecotoxicol. Environ. Saf. 236:113499. doi: 10.1016/j.ecoenv.2022.113499
Zeng, F., Ali, S., Zhang, H., Ouyang, Y., Qiu, B., Wu, F., et al. (2011). The influence of pH and organic matter content in paddy soil on heavy metal availability and their uptake by rice plants. Environ. Pollut. 159, 84–91. doi: 10.1016/j.envpol.2010.09.019
Zhai, Y., Cui, L., Zhou, X., Gao, Y., Fei, T., and Gao, W. (2013). Estimation of nitrogen, phosphorus, and potassium contents in the leaves of different plants using laboratory-based visible and near-infrared reflectance spectroscopy: comparison of partial least-square regression and support vector machine regression meth. Int. J. Remote Sens. 34, 2502–2518. doi: 10.1080/01431161.2012.746484
Zhang, L., Chen, Z., and Zhu, C. (2012). Endogenous nitric oxide mediates alleviation of cadmium toxicity induced by calcium in rice seedlings. J. Environ. Sci. 24, 940–948. doi: 10.1016/S1001-0742(11)60978-9
Zhang, H., Huo, S., Yeager, K. M., Xi, B., Zhang, J., He, Z., et al. (2018). Accumulation of arsenic, mercury and heavy metals in lacustrine sediment in relation to eutrophication: impacts of sources and climate change. Ecol. Indicat. 93, 771–780. doi: 10.1016/j.ecolind.2018.05.059
Zheng, N., Yue, W., Wu, J., Hou, K., Wu, L., Guo, M., et al. (2021). Responses of soil cadmium desorption under different saline environments and its controlling factors. Agronomy 11, 2175–2183. doi: 10.3390/agronomy11112175
Keywords: bio-concentration factor and transfer coefficient, cadmium, Hanjiang River basin, potato, yellowish-brown and limestone soils
Citation: Qi X, Luo K, Li Y, Zhang Y, Fang Y and Yang M (2024) Cadmium accumulation and transfer in yellowish-brown and limestone soils of five potato varieties based on a pot experiment in the central Hanjiang River basin. Front. Sustain. Food Syst. 8:1364643. doi: 10.3389/fsufs.2024.1364643
Edited by:
Xian Xia, Hubei Normal University, ChinaReviewed by:
Junia Jean-Gilles Beaubrun, United States Department of Agriculture (USDA), United StatesShijuan Wu, Wuhan University, China
Copyright © 2024 Qi, Luo, Li, Zhang, Fang and Yang. This is an open-access article distributed under the terms of the Creative Commons Attribution License (CC BY). The use, distribution or reproduction in other forums is permitted, provided the original author(s) and the copyright owner(s) are credited and that the original publication in this journal is cited, in accordance with accepted academic practice. No use, distribution or reproduction is permitted which does not comply with these terms.
*Correspondence: Kai Luo, a29va2F5OEBnbWFpbC5jb20=