- 1Department of Floriculture and Landscaping, Faculty of Horticulture, Bidhan Chandra Krishi Viswavidylaya, Mohanpur, West Bengal, India
- 2College of Horticulture, Central Agricultural University, Bermiok, Sikkim, India
- 3College of Community Science, Central Agricultural University, Tura, Meghalaya, India
- 4Department of Plant Production, College of Food and Agriculture Sciences, King Saud University, Riyadh, Saudi Arabia
- 5Department of Agricultural Biotechnology, Faculty of Agriculture, Bidhan Chandra Krishi Viswavidylaya, Mohanpur, West Bengal, India
- 6Department of Horticulture, M. S. Swaminathan School of Agriculture, Centurion University of Technology and Management, Gajapati, Odisha, India
- 7Department of Agronomy, Dr. Rajendra Prasad Central Agricultural University, Pusa, Bihar, India
- 8Biology Department, College of Science and Humanity Studies, Prince Sattam Bin Abdulaziz University, Riyadh, Saudi Arabia
- 9Department of Biosystems and Agricultural Engineering, College of Agriculture and Natural Resources, Michigan State University, East Lansing, MI, United States
- 10Department of Crop Sciences, University of Illinois Urbana-Champaign, Urbana, IL, United States
This study uses inflorescence stalk node as explants to establish an efficient and quick Phalaenopsis orchids cloning procedure for the most significant monopodial orchid in floriculture, without callus formation. The current study aimed to develop a rapid and easy regeneration process utilizing flower stalk nodes as explants, while also evaluating the clonal fidelity of the in vitro micropropagated plants through the analysis of RAPD markers. The tissue-cultured plantlets were grown on a solidified half-strength Murashig and Skoog (MS) base medium enriched with 15% coconut water (CW), 150 mg L−1 activated charcoal, and a mixture of 6-benzylaminopurine, BAP (cytokinins) and α-napthalene acetic acid, NAA and indole 3-butyric acid, IBA (auxins). After 14 weeks of growth, the early production of shoot bud was reported in ½ MS medium enriched with 2.5 mg L−1 BAP alone. Maximum shoot bud multiplication was observed in ½ MS fortified with BAP (2.5 mg L−1) + NAA (1.0 mg L−1), while the lowest was observed in 1.5 mg L−1 BAP + 0.5 mg L−1 IBA after 4 months of culturing. In this investigation, roots emerged simultaneously with shoot elongation from the axil, indicating the absence of a distinct rooting stage. The largest number of roots (3.25) was produced by BAP (2.5 mg L−1) + IBA (1.0 mg L−1) compared to NAA. Control, on the other hand, displayed no signs of root growth. Tissue cultured plantlets with well developed root systems while planted in a potting mixture of brick and charcoal (1: 1) resulted in a 70% survival rate during hardening. The clonal faithfulness of in vitro regenerated crop plantlets to the mother plant was demonstrated by the DNA extraction method with ten micropropagated plants’ young leaves as well as the mother plant using random amplification of a polymorphic DNA marker.
1 Introduction
The world’s most exquisite flowers are orchids. It belongs to the Orchidaceae family, which has 25,000–35,000 species worldwide and 600–800 genera (Ninawe and Swapna, 2017). Orchids can be found in any ecological circumstance and kind of habitat (Zhang et al., 2022; Xue et al., 2023). Orchids are said to contribute a substantial proportion of the global floriculture trade in both potted plants and cut flowers. De et al. (2014) reported that the worldwide orchid business is valued at US$ 504 million, with over 40 nations exporting and 60 importing orchids. Owing to ease in cultural operations, variation in size and form, bloom color, availability throughout the year, delicateness, and extended shelf life, Phalaenopsis orchids is considered as 2nd most favorite flower in both potted plant and cut flower. Phalaenopsis orchids is a monopodial orchid species, meaning that it neither branches nor produces new shoots. Phalaenopsis orchids is a plant that grows in a monopodial manner on a single stem which grows vertically and produces flowers on lateral branches. Phalaenopsis orchids have a 2–3 years growth cycle. Traditionally, Phalaenopsis orchids are propagated by cutting or division of offshoots. However, these techniques have a limited rate of multiplication and hinder the growth of the mother plant, making them unsuitable for large-scale production. Their vegetative propagation is so challenging, and the features of their seedlings vary. One of the main issues with commercial Phalaenopsis orchid production is that it takes at least three years for it to flower in a greenhouse. Therefore, it is of utmost importance to propagate through tissue culture.
Even though a vast majority of orchids are frequently multiplied using tissue culture, Phalaenopsis orchids are one of the few economically significant genera that is reticent and causes difficulties for rapid clonal propagation (Singh et al., 2007; David et al., 2022). In addition to meristem culture, flower stalks, internodal segments of flower stalks, flower stalks with axillary bud, root tip (Park et al., 2002), and leaf segments (Park et al., 1996), numerous tissue culture procedures have been established for Phalaenopsis orchids. Despite these challenges, the mentioned approaches have limitations in vegetative proliferation. While many protocorms were produced by some of these techniques, most of these structures matured slowly or poorly into vital plants. The appropriate concentration and combination of the hormones 6-benzylamino purine (BAP) and α-napthalenacetic acid (NAA) in the culture medium is thought to be crucial for the commercial micropropagation of Phalaenopsis orchids (Tokuhara and Mii, 1993). Kosir et al. (2004) reported that direct regeneration without callus formation reduces somaclonal variability occurrence and shortens the regeneration time. The genetic preservation of the mother plant is an advantage of this propagation technique, and it’s essential that the parent plant itself not be destroyed during the tissue harvesting procedure (Holmes et al., 2021).
Bhatia et al. (2011) stated that synthetic plant growth regulators occasionally cause alterations to the micropropagated plants’ genome that could result in DNA methylation, point mutations, and rearrangements. In the context of micropropagation, maintaining genetic homogeneity is crucial for ensuring the uniformity of the propagated plants. To ensure genetic homogeneity, it is of the utmost importance to check the genetic fidelity of in vitro micropropagated plants. Chromosome counts and polymerase chain reaction (PCR)-based molecular markers such as inter simplified sequence repeats (ISSR) and random amplified polymorphic DNA (RAPD) can be used to evaluate clonal stability (Li et al., 2019). Molecular techniques are a more reliable and useful tool than other methods to check the genetic stability of in vitro micropropagated plants because they are not affected by environmental conditions (Xiang et al., 2021; Huang et al., 2022). As it is very easy to use, quick, profitable, highly discriminative, and authentic, random amplified polymorphic DNA (RAPD) is a commonly used molecular marker for determining the genetic fidelity of in vitro micropropagated plantlets (Razaq et al., 2013; Bhattacharyya et al., 2014; Dey et al., 2021). RAPD and ISSR markers are incredibly easy to use, quick, affordable, highly discriminating, and reliable. To design the primer, they only need a minimal amount of DNA material and no prior sequencing information. Since they do not use radioactive probes like restriction fragment length polymorphism (RFLP) does (Lakshmanan et al., 2007), they can be used to evaluate the genetic integrity of clones that were produced in vitro.
In a distinctive approach, this study not only focuses on the successful regeneration but also delved into the clonal faithfulness of the in vitro micropropagated plants. The objective of the current work was to create an efficient regeneration process using flower stalk nodes as explants. In order to assess the effectiveness of the approach, clonal faithfulness of the in vitro micropropagated plants was also investigated using RAPD markers. Consequently, DNA fingerprinting aids in verifying, through RAPD results, that the micropropagated clones generated in vitro retained their integrity. The primary advantage of using this propagation technique is the genetic preservation of the mother plant. This study provides a crucial means to verify and ensure the integrity of the in vitro micropropagated clones, contributing to the advancement of reliable and efficient micropropagation methods.
2 Materials and methods
2.1 Plant material and processing of explants
For experimentation inflorescence stalks as explants were taken from Phalaenopsis orchids (variety: P21-L-34-1033 having white color flower, suitable for both as potted plant and cut flower) which was presented at the orchidarium, AICRP on floriculture, at Mondouri Farm, Bidhan Chandra Krishi Viswavidyalaya, West Bengal. It is one of the most popular commercial potted orchid in West Bengal because of its long-lasting flower, delicate texture and durability. The orchidarium has vast collection of different genera of orchid under All India Coordinated Research Project on Floriculture. When the first flower bud appeared during the first bloom stage, inflorescence stalk explants were gathered. Cotton dipped in 70% alcohol was used to swab the surface of fresh, vigorous, healthy flower stalks. The explant was split into pieces of 2 cm long, each with single node. The segments were then cleaned in distilled water and teepol water. After the removal of the bracts from the buds, fungicidal and antibiotic treatments were applied to the sections. The items were dried with sterile blotting paper, cleaned with cotton dipped in 70% alcohol, and placed in the sterile room. The node was disinfected for 5 min with a 0.1% (v/v) sodium hypochlorite solution, and then for 10 min with a mixture of bavistin (0.5%) and streptomycin (0.1%) as a sterilant (Long et al., 2022).
2.2 Shoot bud induction
In order to initiate the shoot bud, the inflorescence stalk node was placed on ½ MS medium that was supplemented with 30 g sucrose, 150 mg L−1 activated charcoal, 15% coconut water (CW), and BAP (1.0, 1.5, 2.0, 2.5, and 3.0 mg L−1) alone or with auxin, i.e., NAA, at a concentration of 0.5–1.0 mg L−1. Even though many plant species grown in tissue culture can withstand a broad pH range of 4.0–7.2, slightly acidic media, typically around pH 5.8 usually give the best growth result. Thus, the pH of the entire medium was brought to 5.6–5.8 using 0.1 N NaOH. For 17 min, all media were autoclaved at 0.1 MPa and 121°C. The cultures were maintained at 26 ± 2°C in a growth chamber with a 16-h light or 8-h dark photoperiod (Piątczak et al., 2015). Every experiment was conducted under the same incubation conditions. The shoot bud induction response was seen after a 14-week culture period.
2.3 Proliferation of shoot buds and root formation
For shoot proliferation and elongation, well-responding shoot buds (2 cm in length) from nodal explants were grown on ½ MS media supplemented with 1.5–2.5 mg L−1 BAP and 0.5–1.0 mg L−1 NAA and IBA (For specifics, refer Table 1). In this investigation, with the elongation of shoot, roots were found to develop simultaneously from the axil. The number of shoots, leaves, root per explants was recorded after 20 weeks of culture.
2.4 Hardening
After 40 weeks of development, shoots firm, round and silvery white air roots which is called as velamen were taken away from the culture medium and cleaned with sterile water to get removal of agar. Plantlets were then transplanted to a plastic pot with holes in it that held potting medium of uniformly autoclaved bricks and charcoal pieces (1.1), because during the early stages of plant growth, brick and charcoal offer improved moisture and nutrient retention capacity. This process allowed for in vitro hardening.
2.5 Genetic fidelity study of the in vitro micropropagated plantlets with their parents
Ten micropropagetd plants’ young leaves, as well as the mother plant, were used to obtain total genomic DNA. Murray and Thomson (1980) DNA extraction method was followed for standardization of the crop in laboratory condition. A UV spectrophotometer was used to quantify and examine the amount and purity of the extracted DNA at 260 nm. A comparison was made between the absorbance ratios at two wavelengths (A260 and A280) and the standard ratio of pure DNA. It was found that the extracted DNA amounts were optimal for further PCR amplification.
Each 0.2 mL microfuge tube (Dialabs) had 40 ng template of DNA, 2 μM of each of the four dNTPs, 1× PCR buffer (10 mM Tris pH 9.0, 50 mM KCl), 1 U of Taq polymerase (Bangalore Genei, India), 2.5 mM of MgCl2, and 20 pmol of primer. These were used for all PCR processes. In a Veritti Thermal Cycler (Applied Biosystems, USA), the RAPD reaction program was set to 94°C for 3 min. This was followed by 45 cycles of 94°C for 45 s, 36°C for 30 s, 36°C for 45 s, and 72°C for 5 min. After the amplified product was mixed with 2.5 μL of 10 × blue dyes, it was tested on a 1.5% agarose gel in 1× TAE buffer at 65–70 V for 3–4 h. DNA fragments were visible under UV light, and photos were obtained with the Gel Documentation System.
2.6 Data analysis
Each treatment was conducted three times, with the mean ± standard error of the data replicated four times. Version 7.5 of the SPSS (Statistical Package for Social Science) was used to statistically analyze quantitative data at the 5% level. Banding profiles were created based on whether bands were present (1) or absent (0). When scoring the data, only distinct and repeatable amplified bands were taken into account. Non clear, weak and smeared were discarded. Prevost and Wilkison (1999) determined that a primer’s resolving power was determined by its capacity to differentiate between individuals.
3 Results
3.1 Axillary shoot bud initiation
For initiating shoot bud, nodal explants were cultured on half strength Murashig and Skoog (MS) basal media supplemented with BAP alone and in combination with NAA and IBA (Table 2). Among all the treatments BAP 2.5 mg L−1 showed earliest swelling of node, bud development (26 days) (Figures 1A,B) and multiple bud development (35 days) (Figure 1C). On the same treatment, the earliest observations of leaf initiation (84 days) and shoot induction (60 days) (Figure 1D) were recorded (Figure 1E). Nodal explants cultured on 2.0 mg L−1 BAP + 1.0 mg L−1 IBA delayed shoot induction (89.33 days) and bud development (78.66 days) as well as leaf initiation (107 days) after 14 weeks of culture (Table 2).
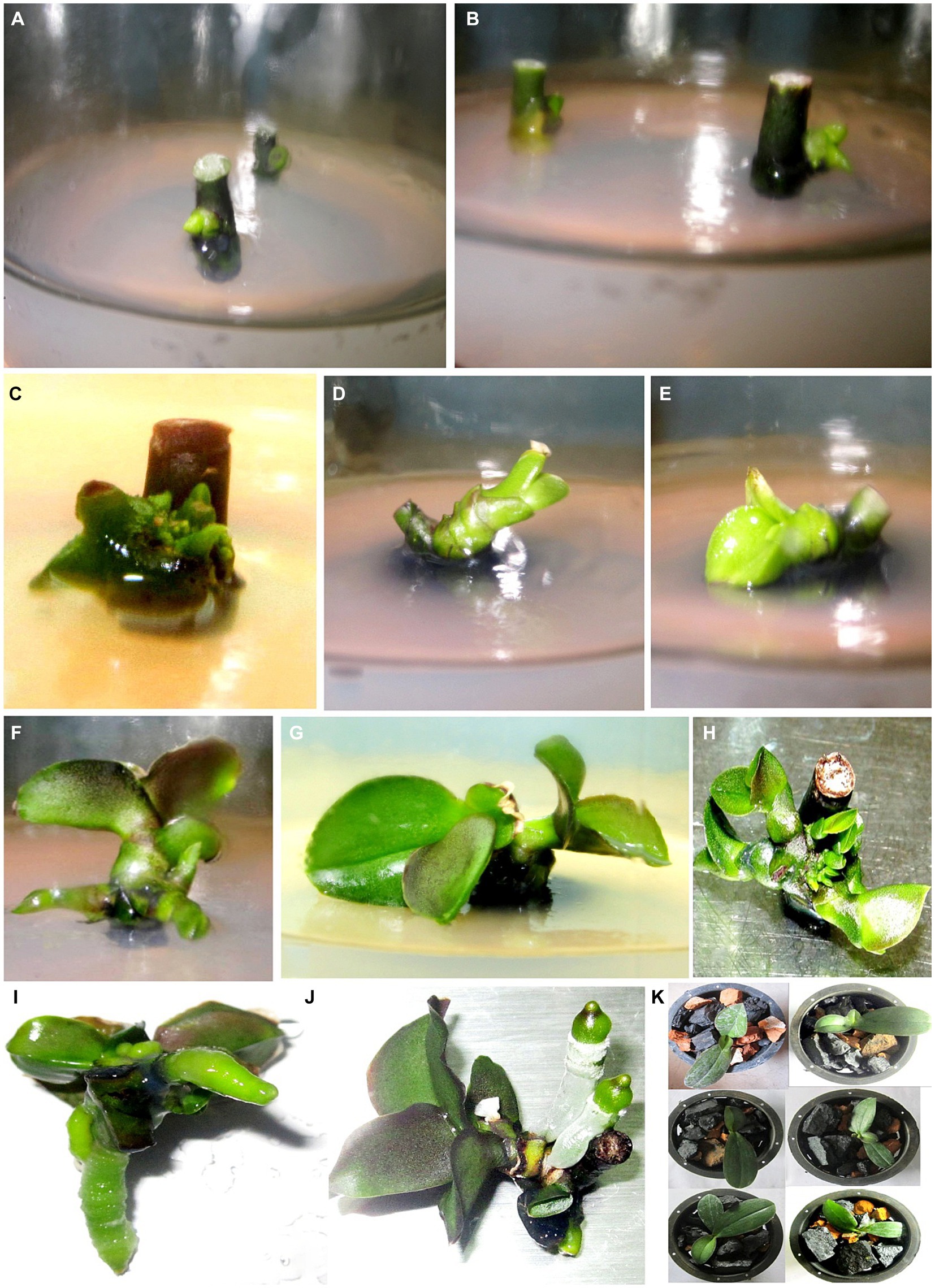
Figure 1. Effect of BAP (2.5 mg L−1), sucrose (30 g L−1) and + NAA 1.0 mg L−1 on shoot bud induction from nodal explants (A) Node swelling at advanced stage, (B) Bud development, (C) Multiple bud development, (D) Shoot induction, (E) Leaf initiation, (F) Shoot induction, (G) Shoot development, (H) Multiple shoots induction, (I) At initiation stage, (J) At development stage, and (K) Hardening of plantlets.
3.2 Proliferation of axillary shoot buds
The shoot buds that were extracted from the nodal segments were placed in half-strength MS medium that was enhanced with BAP along with NAA and IBA (Table 1). The maximum number of shoots (4.15) (Figure 1F) and leaves (7.00) per explant (Figures 1G,H) were produced by shoot buds cultured on 2.5 mg L−1 BAP + 1.0 mg L−1 NAA. Up to a certain point, the number of shoots and leaves increased with increasing concentrations of BAP (1.5–2.5 mg L−1) and NAA (0.5–1.0 mg L−1). At higher concentrations, BAP (3.0 mg L−1) and NAA (1.5 mg L−1) were seen to have an inhibitory response on the number of shoots and leaves.
3.3 Rooting and acclimatization
The current study did not find a distinct rooting stage; instead, roots were found to form concurrently from the axil during the initiation stage (Figure 1I) and development stage (Figure 1J), along with the elongation of a normal shoot. Every examined culture medium reacted favorably to the growth of roots. After 20 weeks of culture, the highest (3.25) and lowest (2.85) number of roots were found on 2.5 mg L−1 BAP + 1.0 mg L−1 IBA and 2.5 mg L−1 BAP + 1.0 mg L−1 NAA, respectively (Table 1).
A method of acclimatization was developed to increase the survival rate. Individually separated and healthy rooted plants were removed from the media before being placed in the acclimatization chamber. The three to four primary leaves of the marginally acclimated plantlets were moved to the greenhouse. After 2 months of hardening of plantlets using potting medium of brick and charcoal bits (1:1), resulted in more than 70% of success while transplanted (Figure 1K).
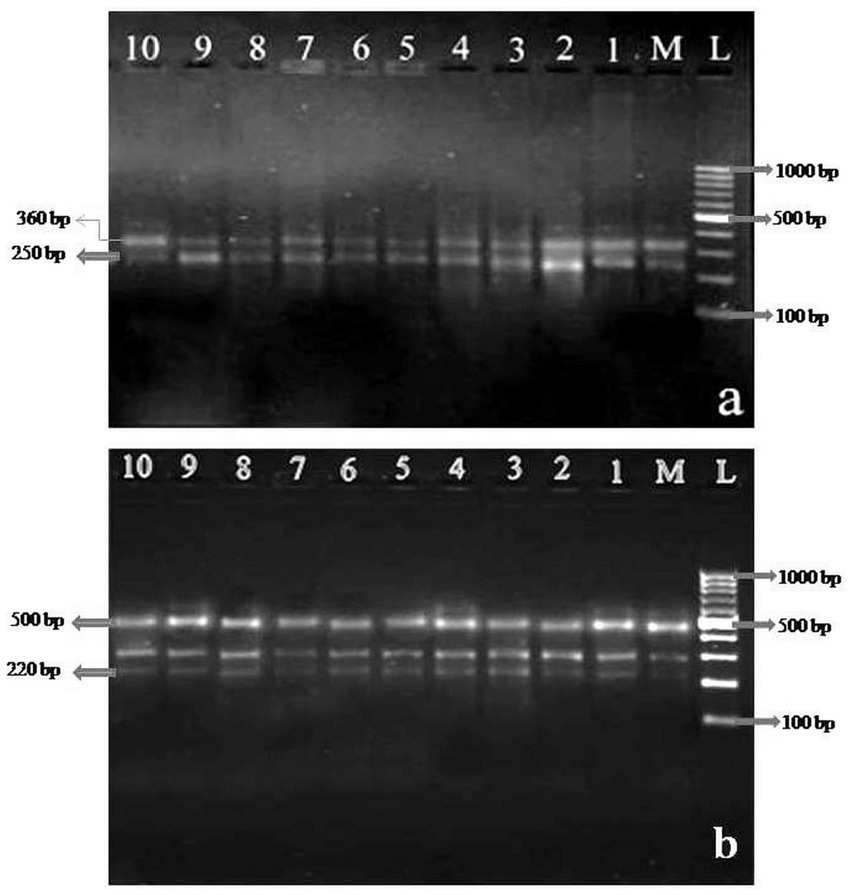
Figure 2. Polymerase chain reaction (PCR) amplification products obtained with random amplified polymorphic DNA (RAPD) (A) Primer (OPU-13) (B) Primer (OPU-16). Lane L represents100-bp ladder, lane M represents the mother plant and lanes 1–10 represent in vitro raised clones derived from nodal explant.
3.4 Genetic fidelity of in vitro micropropagated plantlets to their parents
The mother plant from which the in vitro micropropagated plantlets initially developed showed no differences in morphogenetic and phenotypic expression when compared to the source from which they were derived. Their morphology was true to type, indicating their genetic stability. Afterwards, they underwent molecular analysis using DNA fingerprinting in order to verify genetic fidelity.
3.5 Assay for RAPD markers
OPU 09, 10, 11, and 12 were the only three of the ten RAPD primers that did not amplify when tested in a PCR using Phalaenopsis orchids DNA. While OPU 13 and OPU 16 demonstrated positive reproducible bands, the primers OPU 14, OPU 15, P 14 and P 16 exhibited a positive reaction in PCR but were unable to replicate any substantial scorable bands (Table 3). In vitro micropropagated plantlets exhibited 55 repeatable monomorphic amplicons, including their mother. Distinctive amplicons were assessed in this study between 220 and 500 base pairs. The same banding patterns displayed by all the primers (Figure 2) suggest that the in vitro regenerated clones’ purity was preserved.
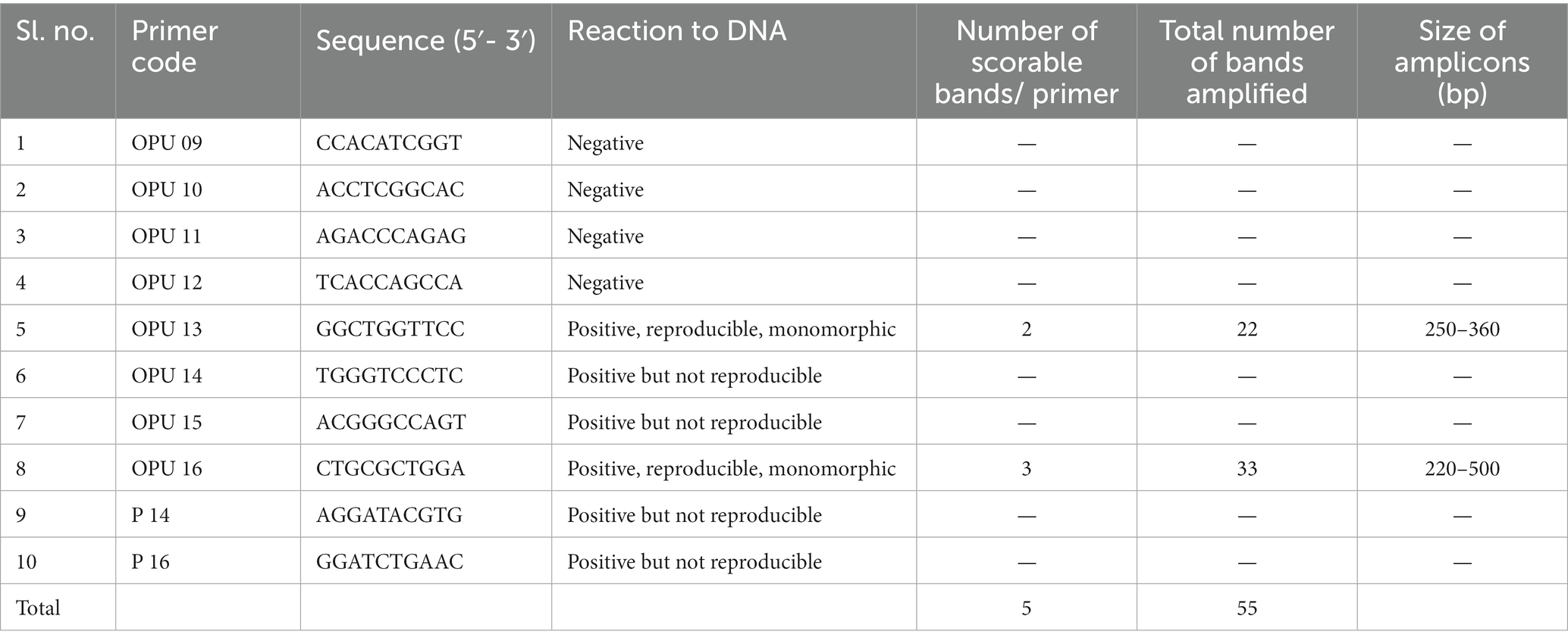
Table 3. List of primer code, sequences, reaction to DNA, number, and size of the amplified fragments generated by random amplified polymorphic DNA (RAPD) primers.
4 Discussion
Phalaenopsis orchid is ranked 2nd most popular potted plant and cut flower in global market due to its variation in flower color, shape, and size, durability. Variety: P21-L-34-1033 is one of the highly demand potted flower in West Bengal because of its durability. The current study has contributed to the development of an improved nodal explant cloning protocol for Phalaenopsis orchid. In this experiment, after 14 weeks of culture on ½ MS medium supplemented with BAP alone and in combination with NAA and IAA, shoot bud initiation happened straight from the nodal explants. No protocrom-like bodies (PLB) production or intervening callus was seen during the bud development process. Initially, the nodes began to enlarge and developed green buds that later transformed into shoots. 2.5 mg L−1 caused the commencement of early shoot buds. Since BAP is a major cytokinin that stimulates organ development and cell division, this may be the cause. Moreover, Kumari et al. (2013) found that early bud break was promoted in Dendrobium Sonia “Earsakul” by culture medium of half-strength MS media supplemented with 4 mg L−1 BAP.
According to Asghar et al. (2010), adding cytokinin to the culture media promotes the growth of multiple shoots. The combination that was shown to be most beneficial for shoot reproduction was BAP 2.5 mg L−1 + NAA 1.0 mg L−1. While the control group did not respond to the increased concentration of BAP in terms of shoot bud proliferation, the number of shoots, leaves, and roots per explant increased up to a certain point when the concentration of BAP was increased. Shoot growth is inhibited by the external application of cytokinins at levels over the optimum threshold (Roy and Banerjee, 2003; Bhattacharyya et al., 2023). Due to its potent properties, it decreases shoot length by promoting the proliferation of axillary buds. Poor growth with yellow and necrotic shoots was the result of higher concentrations of BAP and KIN (Asghar et al., 2010). Higher ratios of cytokinins are in responsible for enhancing the ethylene synthesis leading to plant tissue senescence (Iqbal et al., 2017). Neither PLB development nor callusing was observed for any combination of treatments. BAP 2.5 mg L−1 + NAA 1.0 mg L−1 showed the largest number of shoots and leaves per explant after 20 weeks of culture. According to Yakimova et al. (2000) and Ron'zhina (2003), it may be explained by the fact that BAP stimulates rapid cell division, which leads to shoot multiplication. Comparable results were found by in Dendrobium primulinum Lindl. (Pant and Thapa, 2012), Aerides odorata (Devi et al., 2013), Dendrobium orchid (Talukdar et al., 2003), and Cymbidium aloifolium (Rajkarnikar, 2011). After 60 days of growth, Suntibala and Rajkumar (2009) counted 7.5 shoots from the nodal part of in vitro Dendrobium tranparens L. seedlings grown in half strength MS media supplemented with 2.0 mg L−1 BAP and 1.0 mg L−1 NAA.
When it came to the rooting stage, 2.5 mg L−1 BAP + 1.0 mg L−1 IBA produced more roots than NAA out of all the treatments. After receiving combinations of 1.5–2.0 mg L−1 BAP and 0.5–1.5 mg L−1 IBA, the roots showed the greatest number of long, dark green, strong, and healthy roots which are the attributes of physiologically sound plant. By increasing the amount of endogenous enzymes, auxins—an effective plant growth regulator increase the root initiation process. Auxins have a significant influence on the processes of cell division, elongation, and differentiation (He et al., 2023). The processes of root induction and development are accelerated by auxins, which are considered to be effective plant growth regulators that differentiate vascular bundles. They promote rooting by changing the plant biochemical system (Henrique et al., 2006). According to Han et al. (2009), auxin causes shoot buds to sprout, which in turn promotes the growth substances present in roots allowing them to expand and elongate (Sherif et al., 2020). Through repeated cell divisions, auxin administration causes the complex processes of lateral root development (Liu et al., 2002). According to George et al. (2008), they are so significant that they are considered to be necessary for the polarity of plants and their organs to be established and maintained (vascular system). Because it functions as a precursor to endogenous IAA, IBA is biologically a more active auxin for root initiation and produces a greater number of roots than NAA and IAA (Liu et al., 2002; Oliya et al., 2020). IAA and IBA developed more roots than NAA in Dendrobium primulinum (Stephin et al., 2020; Pradhan et al., 2023). Compared to IBA, NAA produced fewer roots, most likely because it accumulates and cannot be quickly catabolized, especially at greater levels than IBA (Vuylsteker et al., 1997). IBA is recognized to promote rooting more effectively than other auxins because of its low toxicity and increased stability for root induction (Han et al., 2009). It is very effective at raising endogenous auxin amounts and demonstrating increased stability against catabolism and inactivation through conjugation with growth inhibitors, it produced good root number results (George et al., 2008). Orchid potting media should have good drainage capacity, good water holding capacity and aeration for better growth. Over 70% of the transplanted seedlings survived through the 60-day hardening period in a potting mixer filled with brick and charcoal pieces (1:1). The observation that Dendrobium Sonia “Earsakul” survived 66.67% of its transplantation in a greenhouse with charcoal and brick pieces (1:1) media suggests that the reason for this could be appropriate drainage and aeration of the medium, which is primodial importance in orchid culture. Kumari et al. (2013) corroborates this conclusion. Being an epiphyte, in the natural environment, their exposed roots take up moisture from dew and wet environments. As a result, when growing in artificial conditions, the subsrate’s capacity to hold nutrients, water, capillary action, and aeration should all be taken into account. It’s also necessary to take into account the medium components’ weight and stability, ease of availability, prices, and consistency. Conversely, under greenhouse conditions, a 90% survival rate of Dendrobium tranparens L. was demonstrated by a 2:1 potting mixture of brick and charcoal (Vilcherrez-Atoche et al., 2023).
One of the most crucial prerequisites for crop species micropropagation is true-to-type clonal fidelity. The broader utility of the micropropagation method may be severely limited by the incidence of cryptic genetic defects resulting via somaclonal variation in the regenerates (Salvi et al., 2001). Therefore, in order to verify the quality of the plantlets for their commercial utility, it is essential to achieve genetic uniformity of micropropagated plants. The RAPD markers in this study exhibit a consistent banding pattern with no variation. All of the amplified bands on the mother and its tissue culture-raised offspring were monomorphic in nature. Several in vitro micropropagated plants viz. bananas by Alizadeh and Singh (2009), gerberas by Bhatia et al. (2011), Dendrobium densiflorum by Mohanty and Das (2013), Spilanthes calva by Razaq et al. (2013), and Rauvolfia hookeri by Ranjush and Gangaprasad (2014) cleared the genetic fidelity test using RAPD primers. But when clonality tests were performed on the mother and in vitro micropropagated seedlings, two of the ten selected RAPD primers showed clear monomorphic bands (Joshi et al., 2023). It was determined that genetic clonality was preserved by taking into consideration the monomorphic banding pattern that the mother and the in vitro cloned plant both displayed.
5 Conclusion
Phalaenopsis orchids are commonly known as moth orchid greatly appreciated for its attractive foliar venation which resemblance to moths but is still underexplored for its ornamental potential. The resplendent allure of these orchids lies in their exquisite and intricate floral design, characterized by graceful arching stems adorned with vibrant, delicate blossoms. Beyond their visual appeal, Phalaenopsis orchids exhibit a captivating fragrance, filling the surroundings with a delightful scent. Considering the rare and threatened status of this orchid, a protocol for in vitro regeneration of Phalaenopsis orchids has been successfully established. It avoids the production of callus and can be readily used for commercial micropropagation. In a short amount of time, this approach effectively produced a high frequency of plantlets. It has been found that growing seven well-hardened plants from a single nodal segment would require around 186 days, or nearly 27 weeks (from bud initiation to complete the acclimatization of plantlets). This study aims to underscore the significance of the micropropagation protocol in Phalaenopsis, demonstrating its efficacy in expediting plantlet development without the occurrence of callus formation. This protocol has diverse applications and commercial implications, ranging from meeting market demand and ensuring consistent quality to exploring new genetic possibilities and international trade opportunities. Furthermore, this study not only contribute to the specific understanding of Phalaenopsis orchid biology but also serve as a valuable model for advancing conservation strategies for endangered orchid biodiversity. The application of these findings to broader conservation efforts underscores the potential of in vitro techniques in preserving and restoring threatened orchid species on a global scale.
Data availability statement
The datasets presented in this study can be found in online repositories. The names of the repository/repositories and accession number(s) can be found at: https://krishikosh.egranth.ac.in/communities/b2086dee-88ee-4546-90b1-753bcfee495b?spc.page=1&spc.sf=dc.date.accessioned&spc.sd=DESC&scope=b2086dee-88ee-4546-90b1-753bcfee495b.
Author contributions
DS: Conceptualization, Data curation, Formal analysis, Investigation, Methodology, Writing – original draft, Writing – review & editing. PM: Data curation, Formal analysis, Writing – original draft, Writing – review & editing. MS: Data curation, Resources, Supervision, Writing – review & editing. TM: Conceptualization, Formal analysis, Methodology, Project administration, Resources, Supervision, Writing – review & editing. NM: Conceptualization, Methodology, Project administration, Resources, Supervision, Writing – review & editing. KP: Writing – review & editing. CJ: Writing – review & editing. SS: Data curation, Writing – review & editing. BA: Data curation, Resources, Formal Analysis, Writing – review & editing. NA: Writing – review & editing. SR: Writing – review & editing. DW: Writing – review & editing.
Funding
The author(s) declare financial support was received for the research, authorship, and/or publication of this article. The authors extend their appreciation to Prince Sattam bin Abdulaziz University for funding this research work through project number (PSAU/2023/01/8920).
Conflict of interest
The authors declare that the research was conducted in the absence of any commercial or financial relationships that could be construed as a potential conflict of interest.
Publisher’s note
All claims expressed in this article are solely those of the authors and do not necessarily represent those of their affiliated organizations, or those of the publisher, the editors and the reviewers. Any product that may be evaluated in this article, or claim that may be made by its manufacturer, is not guaranteed or endorsed by the publisher.
References
Alizadeh, M., and Singh, S. K. (2009). Molecular assessment of clonal fidelity in micropropagated grape (Vitis spp) rootstock genotypes using RAPD and ISSR markers. Iran. J. Biotech. 7, 36–44.
Asghar, S., Ahmed, T., Ahmed, H. I., and Yaseen, M. (2010). In vitro propagation of orchid (Dendrobium nobile) var Emma white. Afr. J. Biotechnol. 10, 3097–3103. doi: 10.5897/AJB10.401
Bhatia, R., Singh, K. P., Sharma, T. R., and Jhang, T. (2011). Evaluation of the genetic fidelity of in vitro- propagated gerbera (Gerbera jamesonii bolus) using DNA-based markers. Plant Cell Tissue Organ Cult. 104, 131–135. doi: 10.1007/s11240-010-9806-5
Bhattacharyya, P., Kumar, S., Lalthafamkimi, L., Sharma, R., Kumar, D., Singh, D., et al. (2023). Molecular and phytomedicinal stability of long term micropropagated Malaxis acuminata: an endangered terrestrial orchid of biopharmaceutical importance. S. Afr. J. Bot. 155, 372–382. doi: 10.1016/j.sajb.2023.01.051
Bhattacharyya, P., Kumaria, S., Diengdoh, R., and Tandon, P. (2014). Genetic stability and phytochemical analysis of the in vitro regenerated plants of Dendrobium nobile Lindl, an endangered medicinal orchid. Meta Gene 2, 489–504. doi: 10.1016/j.mgene.2014.06.003
David, D., Rusdi, N. A., Mohd Mokhtar, R. A., Mohd Faik, A. A., and Gansau, J. A. (2022). Establishment of in vitro regeneration protocol for Sabah’s Jewel orchid, Macodes limii J.J. Wood & A.L. lamb. Horticulture 8:155. doi: 10.3390/horticulturae8020155
De, L. C., Khan, A. M., Kumar, R., and Medhi, R. P. (2014). Orchid farming- a remunerative approach for farmers livelihood. Int. J. Sci. Res. 3, 468–471. doi: 10.36106/ijsr
Devi, H. S., Devi, S. I., and Singh, T. D. (2013). High frequency plant regeneration system of Aerides odorata Lour through foliar and shoot tip culture. Not. Bot. Hortic. Argob. 41, 169–176. doi: 10.15835/nbha4119007
Dey, A., Nongdam, P., Nandy, S., Mukherjee, S., Mukherjee, A., Tikendra, L., et al. (2021). Polyamine elicited aristolochic acid production in in vitro clonally fidel Aristolochia indica L.: an ISSR and RAPD markers and HPTLC based study. S. Afr. J. Bot. 140, 326–335. doi: 10.1016/j.sajb.2020.06.018
George, E. F., Hall, M. A., and Deklerk, G. J. (2008). Plant propagation by tissue culture. Springer 1, 206–217. doi: 10.1007/978-1-4020-5005-3
Han, H., Zhang, S., and Sun, X. (2009). A review of molecular mechanism of plant rooting modulated by auxin. Afr. J. Biotechnol. 8, 348–353.
He, M., Ren, T., Jin, Z. D., Deng, L., Liu, H., Cheng, Y. Y., et al. (2023). Precise analysis of potassium isotopic composition in plant materials by multi-collector inductively coupled plasma mass spectrometry Spectrochim. Acta Part B At. Spectrosc. 209:106781. doi: 10.1016/j.sab.2023.106781
Henrique, A., Campinhos, E. N., Ono, E. O., and Pinho, S. Z. D. (2006). Effect of plant growth regulators in the rooting of Pinus cuttings. Braz. Arch. Biol. Technol. 49, 189–196. doi: 10.1590/S1516-89132006000300002
Holmes, J. E., Lung, S., Collyer, D., and Punja, Z. K. (2021). Variables affecting shoot growth and plantlet recovery in tissue cultures of drug-type Cannabis sativa L. Front. Plant Sci. 12:732344. doi: 10.3389/fpls.2021.732344
Huang, H., Wu, N., Liang, Y., Peng, X., and Shu, J. (2022). SLNL: a novel method for gene selection and phenotype classification. Int. J. Intell. Syst. 37, 6283–6304. doi: 10.1002/int.22844
Iqbal, N., Khan, N. A., Ferrante, A., Trivellini, A., Francini, A., and Khan, M. I. R. (2017). Ethylene role in plant growth, development and senescence: interaction with other Phytohormones. Front. Plant Sci. 8:475. doi: 10.3389/fpls.2017.00475
Joshi, P. R., Pandey, S., Maharjan, L., and Pant, B. (2023). Micropropagation and assessment of genetic stability of Dendrobium transparens wall. Ex Lindl. Using RAPD and ISSR markers. Fcosc 3:1083933. doi: 10.3389/fcosc.2022.1083933
Kosir, P., Skof, F., and Luthar, Z. (2004). Direct shoot regeneration from nodes of Phalaenopsis orchids Acta agric Slov. Acta Agriculturae Slovenica 83, 233–242.
Kumari, P., George, S. T., and Rajmohan, K. (2013). Influence of plant growth regulators on in vitro clonal propagation of dendrobium sonia ‘earsakul’. J. Biol. Innov. 2, 51–58.
Lakshmanan, V., Venkataramareddy, S. R., and Neelwarne, B. (2007). Molecular analysis of genetic stability in long-term micropropagated shoots of banana using RAPD and ISSR markers. Electron. J. Biotechnol. 10, 01–8. doi: 10.2225/vol10-issue1-fulltext-12
Li, F., Li, D., Liu, H., Cao, B., Jiang, F., Chen, D., et al. (2019). RNF216 regulates the migration of immortalized GnRH neurons by suppressing Beclin1-mediated autophagy. Front. Endocrinol. 10:12. doi: 10.3389/fendo.2019.00012
Liu, C., Zhu, J., Liu, Z., Li, L., Pan, R., and Jin, L. (2002). Exogenous auxin effects on growth and phenotype of normal and hairy roots of Pueraria lobata (wild)Ohwi. Plant Growth Regul. 38, 37–43. doi: 10.1023/A:1020904528045
Long, Y., Yang, Y., Pan, G., and Shen, Y. (2022). New insights into tissue culture plant-regeneration mechanisms. Front. Plant Sci. 13:926752. doi: 10.3389/fpls.2022.926752
Mohanty, P., and Das, J. (2013). Synthetic seed technology for short term conservation of medicinal orchid Dendrobium densiflorum LindlEx Wall and assessment of genetic fidelity of regenerants. Plant Growth Regul. 70, 297–303. doi: 10.1007/s10725-013-9801-z
Murray, M. G., and Thomson, W. F. (1980). Rapid isolation of high molecular weigh plant DNA. Nucleic. Acid Res. 8, 4321–4325.
Ninawe, A. S., and Swapna, T. S. (2017). Orchid diversity of Northeast India – traditional knowledge and strategic plan for conservation. J. Orchid Soc. India 31, 41–56.
Oliya, B. K., Chand, K., Thakuri, L. S., Baniya, M. K., Sah, A. K., and Pant, B. (2020). Assessment of genetic stability of micropropagated plants of Rhynchostylis retusa (L.) using RAPD markers. Sci. Hortic. 281:110008. doi: 10.1016/J.SCIENTA.2021.110008
Pant, B., and Thapa, D. (2012). In vitro mass propagation of an epiphytic orchid, Dendrobium primulinum Lindl through shoot tip culture. Afr. J. Biotechnol. 11, 9970–9974. doi: 10.5897/AJB11.3106
Park, S. Y., Kakuta, S., Kano, A., and Okabe, M. (1996). Efficient propagation of protocorm- like–bodies of Phalaenopsis in liquid medium. Plant Cell Tissue Organ Cult. 45, 79–85. doi: 10.1007/BF00043432
Park, S. Y., Murthy, H. N., and Paek, K. Y. (2002). Rapid propagation of Phalaenopsis from floral stalk derived leaves in vitro cell. Dev. Biol. Plant 38, 168–172. doi: 10.1079/IVP2001274
Piątczak, E., Kuźma, Ł., Sitarek, P., Sitarek, P., and Wysokińska, H. (2015). Shoot organogenesis, molecular analysis and secondary metabolite production of micropropagated Rehmannia glutinosa Libosch. Plant Cell Tissue Organ Cult. 120, 539–549. doi: 10.1007/s11240-014-0620-3
Pradhan, S., Paudel, Y. P., Qin, W., and Pant, B. (2023). Genetic fidelity assessment of wild and tissue cultured regenerants of a threatened orchid, Cymbidium aloifolium using molecular markers. Plant Gene 34:100418. doi: 10.1016/j.plgene.2023.100418
Prevost, A., and Wilkison, M. J. (1999). A new system of comparing PCR primers applied to IISR fingerprinting of potato cultivars. Theor. Appl. Genet. 98, 107–112. doi: 10.1007/s001220051046
Rajkarnikar, K. M. (2011). Propagation of Cymbidium aloifolium (L) Sw in vitro by seed. Bull. Dept. Pl. Res. 33, 27–30.
Ranjush, A. P., and Gangaprasad, A. (2014). An efficient micropropagation protocol for Rauvolfia hookeri Srinivas and Chithra and assessment of clonalfidelity by RAPD analysis. Int. J. Agric. Environ. Biotechnol. 7, 205–212.
Razaq, M., Heikrujam, M., Chetri, S. K., and Agrawal, V. (2013). In vitro clonal propagation and genetic fidelity of the regenerants of Spilanthes calva DC using RAPD and ISSR marker. Physiol. Mol. Biol. Plants 19, 251–260. doi: 10.1007/s12298-012-0152-4
Ron'zhina, E. S. (2003). Effect of 6-benzyleaminopurine on the structure of photosynthetic apparatus of Faba bean (Vicia faba L.) Appl. Biochem. Microbiol. 39, 411–417. doi: 10.1023/A:1024580804275
Roy, J., and Banerjee, N. (2003). Induction of callus and plant regeneration from shoot tip explants of Dendrobium fimbriatum Lindl. Var. Oculatum Hk. f. Sci. Hortic. 97, 333–340. doi: 10.1016/S0304-4238(02)00156-5
Salvi, N. D., George, L., and Eapen, S. (2001). Plant regeneration from leaf base callus of turmeric and random amplified polymorphic DNA analysis of regenerated plants. Plant Cell Tissue Organ Cult. 66, 113–119. doi: 10.1023/A:1010638209377
Sherif, N. A., Kumar, T. S., and Rao, M. V. (2020). DNA barcoding and genetic fidelity assessment of micropropagated Aenhenrya rotundifolia (blatt.) C.S. Kumar and F.N. Rasm.: a critically endangered jewel orchid. Physiol. Mol. Biol. Plants 26, 2391–2405. doi: 10.1007/s12298-020-00917-9
Singh, M. K., Sherpa, A. R., Hallan, V., and Zaidi, A. A. (2007). A potyvirus in Cymbidium spp. in northern India. Aust Plant Dis Notes 2, 11–13. doi: 10.1071/DN07005
Stephin, S., Gangaprasad, A., Mathew, S. P., and Muthukrishnan, S. (2020). Enhanced in vitro shoot multiplication of Piper sarmentosum by suppression of apical dominance. Proc. Natl. Acad. Sci. India Sect. B-Biol. Sci. 90, 87–94. doi: 10.1007/s40011-019-01086-w
Suntibala, H., and Rajkumar, K. (2009). Micropropagation of Dendrobium trnaparens L. from axenic pseudobulb segments. Indian J. Biotechnol. 8, 448–452.
Talukdar, S. K., Nasiruddin, K. M., Yasmin, S., Hasan, L., and Begum, R. (2003). Shoot proliferation of Dendrobium orchid with BAP and NAA. J. Biol. Sci. 3, 1058–1062. doi: 10.3923/jbs.2003.1058.1062
Tokuhara, K., and Mii, M. (1993). Micropropagation of Phalaenopsis and Doritaenopsis by culturing shoot tips of flower stalk buds. Plant Cell Rep. 13, 7–11. doi: 10.1007/BF00232306
Vilcherrez-Atoche, J. A., Silva, J. C., Clarindo, W. R., Mondin, M., and Cardoso, J. C. (2023). In vitro Polyploidization of Brassolaeliocattleya hybrid orchid. Plan. Theory 12:281. doi: 10.3390/plants12020281
Vuylsteker, C., Leleu, O., and Rambour, S. (1997). Influence of BAP and NAA on the expression of nitrate reductase in excised chicory roots. J. Exp. Bot. 48, 1079–1085. doi: 10.1093/jxb/48.5.1079
Xiang, Q., Cao, Y., Xu, H., Yang, Z., Tang, L., Xiang, J., et al. (2021). Novel MFSD8 variants in a Chinese family with nonsyndromic macular dystrophy. J. Ophthalmol. 2021:6684045. doi: 10.1155/2021/6684045
Xue, Y., Bai, X., Zhao, C., Tan, Q., Li, Y., Luo, G., et al. (2023). Spring photosynthetic phenology of Chinese vegetation in response to climate change and its impact on net primary productivity. Agric. For. Meteorol. 342:109734. doi: 10.1016/j.agrformet.2023.109734
Yakimova, E., Toteva, V. K., Groshkoff, I., and Ivanova, G. (2000). Effect of BA and CPPU on protease and α-amylase activity of in vitro cultured explants of Rosa hybrid L. Bulg. J. Plant Physiol. 26, 39–47.
Keywords: 6-benzylaminopurine, indole 3-butyric acid, inflorescence stalk node, Murashig and Skoog medium, Phalaenopsis, α-Napthalene acetic acid
Citation: Sarmah D, Mohapatra PP, Seleiman MF, Mandal T, Mandal N, Pramanik K, Jena C, Sow S, Alhammad BA, Ali N, Ranjan S and Wasonga DO (2024) Efficient regeneration of in vitro derived plants and genetic fidelity assessment of Phalaenopsis orchid. Front. Sustain. Food Syst. 8:1359486. doi: 10.3389/fsufs.2024.1359486
Edited by:
Muhammad Saqlain Zaheer, Khwaja Fareed University of Engineering and Information Technology (KFUEIT), PakistanReviewed by:
Muhammad Sajid Iqbal, Chinese Academy of Agricultural Sciences, ChinaMarc Janssens, University of Bonn, Germany
Copyright © 2024 Sarmah, Mohapatra, Seleiman, Mandal, Mandal, Pramanik, Jena, Sow, Alhammad, Ali, Ranjan and Wasonga. This is an open-access article distributed under the terms of the Creative Commons Attribution License (CC BY). The use, distribution or reproduction in other forums is permitted, provided the original author(s) and the copyright owner(s) are credited and that the original publication in this journal is cited, in accordance with accepted academic practice. No use, distribution or reproduction is permitted which does not comply with these terms.
*Correspondence: Dipika Sarmah, ZHNkaXBpa2FzYXJtYWhAZ21haWwuY29t; Mahmoud F. Seleiman, bXNlbGVpbWFuQGtzdS5lZHUuc2E=