- 1Department of Horticulture, Heilongjiang Bayi Agricultural University, Daqing, China
- 2Facility Agriculture Research Institute, Daqing, China
Introduction: Trichoderma spp. is a recognized bio-control agent that promotes plant growth and enhances resistance against soil-borne diseases, especially Fusarium wilt. It is frequently suggested that there is a relationship between resistance to melon wilt and changes in soil microbiome structures in the rhizosphere with plant metabolites. However, the exact mechanism remains unclear.
Method: This study aims to investigate the effects of Trichoderma application on the metabolic pathway of oriental melon roots in response to Fusarium oxysporum f. sp. melonis in a pot experiment. The experiment consisted of three treatments, namely water-treated (CK), FOM-inoculated (KW), and Trichoderma-applied (MM) treatments, that lasted for 25 days. Ultra-performance liquid chromatography-electron spray ionization-mass spectrometry (UPLC-ESI-MS) was used to analyze the compounds in melon roots.
Results: The results show that Trichoderma harzianum application resulted in a reduction in the severity of oriental melon Fusarium wilt. A total of 416 distinct metabolites, categorized into four groups, were detected among the 886 metabolites analyzed. Additionally, seven differential metabolites were identified as key compounds being accumulated after inoculation with Fusarium oxysporum f. sp. melonis (FOM) and Trichoderma. The mechanism by which Trichoderma enhanced melon's resistance to Fusarium wilt was primarily associated with glycolysis/gluconeogenesis, phenylpropanoid biosynthesis, flavone and flavonol biosynthesis, and the biosynthesis of cofactors pathway. In comparison with the treatments of CK and MM, the KW treatment increased the metabolites of flavone and flavonol biosynthesis, suggesting that oriental melon defended against pathogen infection by increasing flavonol biosynthesis in the KW treatment, whereas the application of Trichoderma harzianum decreased pathogen infection while also increasing the biosynthesis of glycolysis/gluconeogenesis and biosynthesis of cofactors pathway, which were related to growth. This study also aims to enhance our understanding of how melon responds to FOM infection and the mechanisms by which Trichoderma harzianum treatment improves melon resistance at the metabolic level.
1 Introduction
Melon (Cucumis melo L.) is highly regarded and valued globally due to its abundant nutritional contents, including ascorbic acid, carotene, folic acid, potassium, and various other bioactive compounds (Thakur et al., 2019). In intensive and large-scale melon production systems, it tends to be monocropped due to its high economic value and the limited availability of land. Consequently, soil sickness is a serious issue that can cause growth retardation, yield loss, poor quality, and increased pathogen colonization. Fusarium wilt is the primary cause of soil sickness in China, which limits melon yield and marketability. Therefore, the pressing requirement in melon production is to research how to manage agriculture better in terms of both quality and quantity.
Fusarium wilt, a widespread soil-borne fungus that colonizes and blocks the vascular systems of host plants (Gordon, 2017), caused by Fusarium oxysporum f. sp. melonis (FOM), is living in the soil and is hardly eliminated from continuously cropped melon soil. It is one of the most destructive diseases affecting the production and quality of melon widely, which is the bottleneck problem of melon in production. The utilization of microbial strategies, specifically the manipulation of the microbiome, has become a prominent area of focus in both research and production (Carrion et al., 2019; Pascale et al., 2019; Li et al., 2020a; Snelders et al., 2020). Biocontrol is considered a desirable approach for managing disease through beneficial microorganisms in host plants and soil-borne pathogens, such as Trichoderma, Bacillus, and Pseudomonas (Shi et al., 2019; Wen et al., 2020). Trichoderma, as bio-control agents, can alleviate the incidence of soil-borne disease; meanwhile, accumulating evidence indicates that microbiota in the rhizosphere directly or indirectly participate in host plant metabolic processes by regulating the secondary metabolisms of host plants (Chen et al., 2018; Huang et al., 2018; Pang et al., 2021). However, it is unclear whether the exogenous application of Trichoderma could change the melon metabolites related to resistance.
It recognizes that beneficial microorganisms can cause an immune response in the entire plant when they come together in the soil around a plant's roots (Qin et al., 2022). This defense mechanism includes releasing antibiotics, changing the composition of substances in the roots, and modifying the soil's microbial community. Together, these measures prevent the spread of dangerous pathogens (Wang et al., 2023). Numerous studies have shown that certain proteins released from the roots of plants act as signals when they deal with the beneficial fungus Trichoderma, such as SSCPs (small, secreted, cysteine-rich proteins), which act as negative effectors, reducing the defense level (Amdan et al., 2015). The plant's defense system makes use of these signals (Kan et al., 2023; Zhang T. et al., 2023). It has been demonstrated that Trichoderma application reduces the possibility of diseases, particularly those that affect the soil. This decrease is closely associated with modifications in the root's metabolic compounds, particularly indole-3-acetic acid, during the Trichoderma interaction (Bader et al., 2020; Li et al., 2023). Meanwhile, melon secreted different kinds of metabolic compounds (including hormones and phytoalexins) and improved the growth indirectly when Trichoderma colonized in the rhizosphere (López-Bucio et al., 2015; Malmierca et al., 2015).
Previously, we found that the application of Trichoderma promoted the growth of orient melon, induced resistance against FOM, and alleviated the incidence of Fusarium wilt. However, it remains uncertain whether the increased resistance was related to changes in metabolic compounds. Therefore, we hypothesized that the application of Trichoderma improved the resistance of orient melon by changing its root metabolism. To verify the hypothesis, the pot experiments were conducted with the following specific objectives: (1) to determine if the application of Trichoderma enhanced the resistance of orient melon against FOM, thereby reducing the occurrence of Fusarium wilt; (2) to analyze the changes in metabolites using UPLC-Q-TOF technology; and (3) to clarify the altered metabolic pathway in orient melon treated with Trichoderma in response to the pathogen, which will provide a technological foundation for future applications of Trichoderma in production.
2 Method and materials
2.1 Materials
The “YangJiaoMi” melon seeds, a self-pollinating line that is susceptible to Fusarium oxysporum f. sp. melonis (FOM), were provided by the Department of Horticulture, Heilongjiang Bayi Agricultural University. The soil used was collected from a greenhouse located in Daqing, China (46°58′N, 125°03′E), where orient melon has been cultivated exclusively for multiple consecutive years. The soil had a composition of 3.64% organic matter, 139 mg kg−1 available N, 271 mg kg−1 Olsen P, and 223 mg kg−1 available K. The electrical conductivity (EC) of the solution was measured to be 0.68 mS cm−1 (1:2.5, w/v), while the pH was determined to be 6.91 (1:2.5, w/v). The soil was used repeatedly to cultivate melon over several years, leading to a notable prevalence of melon wilt and the subsequent accumulation of pathogens, particularly Fusarium spp. Fusarium oxysporum f. sp. Melonis was isolated from diseased orient melon plants in a continuously monocropped melon field (Datong Farm in Daqing, China) cultured on potato dextrose agar (PDA) media. The Trichoderma harzianum (Xue et al., 2021) was provided by the Department of Horticulture, Heilongjiang Bayi Agricultural University, Daqing, Heilongjiang province, China.
2.2 Experiments design
The pot experiment was conducted in a greenhouse from 20 May to 15 June 2021, located in Heilongjiang Bayi Agriculture University, Daqing, Heilongjiang province, under a 16/8-h light/dark regime with a day temperature of 26°C and a night temperature of 16°C, the light intensity was ~8,000–10,000 lux. Orient melon seeds were sterilized with 2.5% sodium hypochlorite solution for 10 min, rinsed with sterilized water three times, and potted in pots (12 cm × 15 cm) filled with the soil from monocropping with orient melon. When they had two fully expanded leaves, the inoculations with FOM and Trichoderma harzianum were carried out using the root irrigation method, and three treatments were arranged: (1) treated with water (CK); (2) treated with FOM (105 spores/g soil); (3) treated with Trichoderma harzianum (105 spores/g soil). These pots were transferred to a plant growth chamber with a 16-h light/8-h dark photoperiod and a temperature cycle of 28°C (light) and 18°C (dark). The humidity level was consistently maintained at 70%. After 15 days, the incidence of Fusarium wilt was investigated, and the roots of orient melon were harvested. In total, 15 orient melon roots were considered one replicate sample, and each treatment contained three treatments. Furthermore, the roots were washed with water and wrapped in tinfoil, immediately frozen in liquid nitrogen, and stored at −80°C, which was used for the analysis of different metabolic compounds with UPLC-ESI-MS/MS.
2.3 Sample preparation and extraction
The orient melon roots were freeze-dried by a vacuum freeze-dryer (Scientz-100F) and then ground using a grinding mill (MM 400; Retsch, Haan, Germany) for 1.5 min at a frequency of 30 Hz. Then, 100 mg of powder was weighed and subjected to an extraction process at 4°C with 1.2 ml of 70% aqueous methanol. The extraction process lasted for a duration of 24 h. After centrifugation at 12,000 g for 10 min to remove undissolved residues, the extracts were filtered using SCAA-104 with a 0.22 μm pore size (ANPEL, Shanghai, China), followed by LC-MS analysis.
2.4 High-performance liquid chromatography conditions
The extracts above were analyzed using an LC-ESI-MS/MS system (UPLC Shimadzu CBM30A system, https://www.shimadzu.com.cn/; MS, 4500 Q TRAP; Applied Biosystems, https://www.thermofisher.cn/cn/zh/home/brands/applied-biosystems.html). The analytical conditions were as follows: the chromatographic column was Waters Acquity UPLC: Agilent SB-C18 (2.1 mm*100 mm, particle size 1.8 μm) and the mobile phase consisted of solvent A (pure water with a 0.1% formic acid solution) and solvent B (acetonitrile with a 0.1% formic acid solution). Sample measurements were performed with a binary gradient program that employed the starting conditions of 95% A and 5% B. Within 9 min, a linear gradient of 5% A and 95% B was programmed, and a composition of 5% A and 95% B were kept for 1 min. Subsequently, a composition of 95% A and 5.0% B was adjusted within 1.1 min and maintained for 12.9 min. The flow velocity was set at 0.35 ml min−1; the column oven was set to 40°C; and the sample injection volume was 4 μl. The effluent was alternatively connected to an ESI-triple quadrupole-linear ion trap (QTRAP)-MS (Chen et al., 2013; Peng et al., 2017; Zhu et al., 2018).
2.5 ESI-Q trap-MS/MS
A system equipped with linear ion trap (LIT) and triple quadrupole (QQQ) scans was utilized to detect metabolites. The system was equipped with an ESI turbo-ion spray interface that operated in positive and negative ion monitoring modes. The interface was controlled using the Analyst v1.6.3 software, developed by AB Sciex, Foster City, CA, United States. The operation parameter of the ESI source was an ion source utilizing turbo spray technology, operating at a temperature of 550°C and a voltage of 5,500 V. The pressure settings for gas I, gas II, and curtain gas were 55, 60, and 25 psi, respectively. The collision gas was adjusted to a high level. For the instrument tuning and mass calibration, we utilized polypropylene glycol solutions with concentrations of 10 and 100 μmol/L in the QQQ and LIT modes, respectively. The QQQ scans were acquired during the multiple reaction monitoring (MRM) experiments using nitrogen as collision gas at a pressure of 5 psi. Optimization was conducted to determine the de-clustering potential (DP) and collision energy (CE) for each MRM transition. The monitoring process focused on a specific set of MRM transitions, which were determined based on the metabolites that were eluted during each period (Chen et al., 2013; Peng et al., 2017; Zhu et al., 2018).
2.6 Qualitative and quantitative metabolite analyses
Analyst v1.6.3 software was used to analyze the MS data. Based on the metabolic database (Sigma-Aldrich, St. Louis, MO, United States) and self-compiled MWDB database (MetWare Biological Science and Technology Co., Ltd., Wuhan, China), we compared the accurate precursor ions (Q1), product ion (Q3) values, retention times, and fragmentation patterns with standards to analyze the primary and secondary MS data.
The quantitative analysis of each metabolite was carried out in the MRM mode. The characteristic ions of metabolites were screened by QQQ MS to get the signal strengths. MultiaQuant v3.0.2 (AB Sciex, Concord, Ontario, Canada) was used for the integration and correction of chromatographic peaks. The peak area of each chromatographic peak represented the relative content of the corresponding substance compared to standards. In the absence of standards, we utilized a self-compiled database called MWDB, as well as publicly accessible metabolite databases, to conduct data analysis.
2.7 Multivariate statistical analysis
Multivariate statistical analysis was used to make high-dimensional data more simplified and was reduced by retaining the original information to the greatest extent, and reliable mathematical models were established to summarize the characteristics of the metabolic data of the objects. Metabolite data were log2-transformed for statistical analysis to improve normality and were normalized. Metabolites from nine samples were used for principal component analysis (PCA), orthogonal partial least squares discriminant analysis (OPLS-DA), and hierarchical clustering analysis (HCA) using R software to study metabolite accession-specific accumulation. The p-values and fold change values were set to 0.05 and 2.0, respectively. The number of differential metabolites in three comparison pairs was expressed by Venn diagrams. Identified metabolites were annotated using the KEGG COMPOUND database (http://www.kegg.jp/kegg/compound/), and then, the annotated metabolites were mapped to the KEGG Pathway database (http://www.kegg.jp/kegg/pathway.html). Pathways with significantly regulated metabolites mapped were then fed into metabolite sets enrichment analysis (MSEA), and their significance was determined by the hypergeometric test's p-values. All data were graphed using Origin12.0.
3 Results
3.1 The incidence and index of melon Fusarium wilt
The incidence and index of melon Fusarium wilt are shown in Figure 1. After 15 days of inoculation with FOM and Trichoderma, the incidence of melon Fusarium wilt in the FOM-inoculated (KW) treatment showed a significant increase compared to the control (CK) treatment, while the Trichoderma-application (MM) treatments resulted in a significant decrease in the incidence of melon Fusarium wilt. However, the index of melon Fusarium wilt was at a comparable level to the incidence of melon Fusarium wilt.
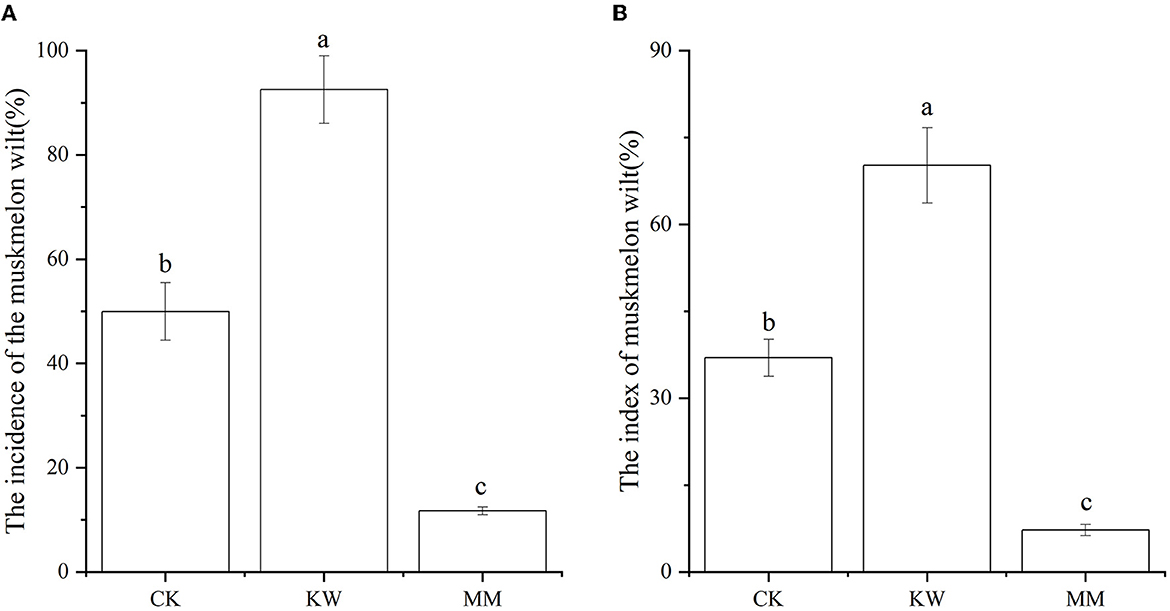
Figure 1. The incidence (A) and index (B) of melon Fusarium wilt (CK: control; KW: inoculated with FOM; MM: inoculated with Trichoderma). Columns labeled with different letters are significantly different at the 0.05 probability level based on Tukey's HSD.
3.2 Metabolic profiling
A total of 886 metabolic compounds were found in the three treatment samples (Supplementary material), which were divided into 10 classes, including 396 primary metabolites, 398 secondary metabolites, and 92 others. The primary metabolites included amino acids and derivatives of 84 types, organic acids of 85 types, lipids of 168 types, nucleotide and derivatives of 59 types. In addition, secondary metabolites included flavonoids of 100 types, phenolic acids of 176 types, terpenoids of 40 types, alkaloids of 54 types, lignins and coumarins of 28 types, and others of 92 types (Figure 2A). To ensure the repeated ability and reliability of the data, hierarchical cluster analysis and analysis of the mass spectrometry results of different quality control (QC) samples are shown in Figure 2B and Supplementary Figure 1, respectively. These results indicated that the curves of the QC samples exhibited a substantial degree of overlap in the total ion current (TIC), suggesting that the test results were reliable.
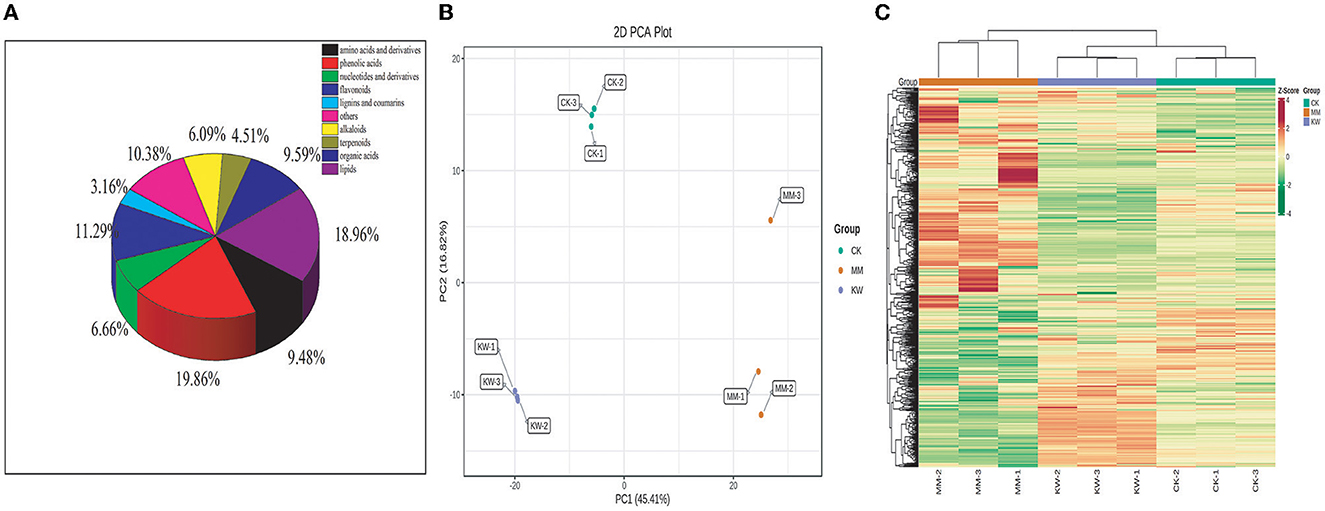
Figure 2. (A) Classification of the 774 metabolites of melon samples; (B) hierarchical cluster analysis (HCA); (C) principal component analysis (PCA).
Hierarchical cluster analysis (HCA) was used to analyze the differences in the accumulation patterns of metabolites among CK, KW, and MM treatments (Figure 2B). The analysis revealed a significant disparity in the accumulation of compounds among the treatments, with these metabolites being categorized into four clusters. The metabolite contents in Cluster I exhibited the highest level in MM, an intermediate level in KW, and the lowest in CK. The metabolite contents in Cluster II exhibited the highest level in MM, an intermediate level in CK, and the lowest level in KW. The metabolite contents in Cluster III exhibited the highest level in CK, an intermediate level in KW, and the lowest level in MM. The metabolite contents in Cluster IV exhibited the highest level in KW, an intermediate level in CK, and the lowest level in MM. According to principal component analysis (PCA) results, the orient melon samples under different treatments were separated significantly, indicating that the metabolites of different treatments were significantly different after inoculation with FOM and Trichoderma, corresponding to the observation of the incidence and index of melon wilt. The first principal component (PC1) can explain 45.41% of the characteristics of the original dataset. Different treatments were separated by the principal components, while the inoculated orient melon plants and non-inoculated orient melon plants were separated by the second principal component (PC2), which explained 16.82% of the characteristics of the original dataset (Figure 2C).
3.3 Analysis of differential metabolites
Orthogonal partial least squares discriminant analysis (OPLS-DA) was used to maximize population differentiation to identify distinct metabolites, which is a multivariate statistical analysis method. Comparison pairwise was analyzed using the optimization OPLS-DA model, and the score plots are shown in Figure 3. In the optimization model, R2X and R2Y were used to represent the interpretation rate for the X and Y matrices, respectively, and Q2 represented the prediction ability. Comparison pairwise results showed that both R2Y and Q2 scores were higher than 0.9, indicating that the model was suitable. In addition, the effectiveness of the OPLS-DA model was confirmed through 200 alignment experiments, with the results demonstrating its efficacy (Supplementary Figure 2).
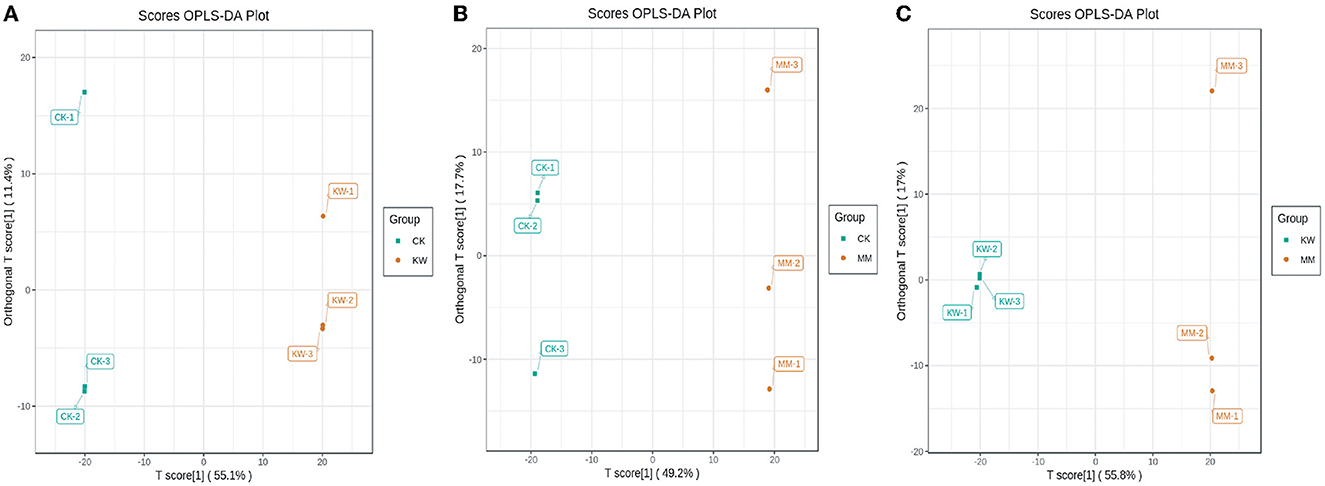
Figure 3. The score plots of OPLS-DA pairwise comparisons of differential metabolites. (A) CK vs. KW; (B) CK vs. MM; and (C) KW vs. MM.
The metabolites that showed differential expression were identified by considering both the fold change value and the VIP value. Specifically, metabolites with a fold change of ≥2, a fold change of ≤ 0.5, and a VIP ≥ 1 were considered and selected as differential metabolites. As shown in Figure 4A, in the three comparison pairs (CK vs. KW, CK vs. MM, and KW vs. MM), the number of upregulated metabolites was comparable to the number of downregulated metabolites. In the comparison of CK and KW, a total of 70 differential metabolites were identified, consisting of 33 metabolites that were upregulated and 37 metabolites that were downregulated. A total of 128 metabolites exhibited differential expression in the comparison pairs of CK and MM, with 67 metabolites being upregulated and 61 metabolites being downregulated. In the comparison of KW and MM, a total of 218 differential metabolites were identified, consisting of 111 upregulated metabolites and 107 downregulated metabolites. Figure 4B illustrates the division of different metabolites into 10 categories: four primary metabolites (nucleotides, organic acids, amino acids and derivatives, and lipids) and five secondary metabolites (alkaloids, flavonoids, lignins and coumarins, phenolic acids, and terpenoids). The analysis revealed that orient melon plants treated with Trichoderma harzianum had a higher presence of upregulated flavonoids and derivatives compared to those treated with KW. This suggested that the Trichoderma harzianum treatment might stimulate the synthesis of these metabolites in melon plants, protecting against the Fusarium pathogens. The downregulated metabolites between comparison pairs were all concentrated in amino acids and derivatives and lipids, while the upregulated metabolites between the three comparison pairs were all concentrated in flavonoids and phenolic acids, indicating that these differential metabolites were accumulated more in orient melon after FOM and Triochderma infection, indicating that orient melon plants had a defense response to pathogens.
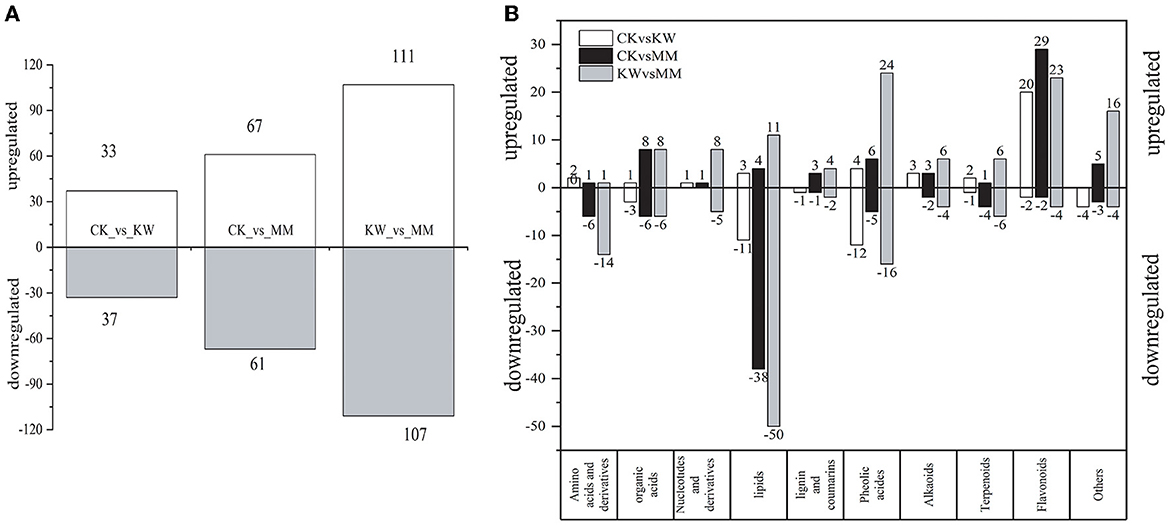
Figure 4. (A) The number of differentially expressed metabolites of each pairwise comparison of melons; (B) classification of differentially expressed metabolites of three pairwise comparisons.
3.4 KEGG functional annotation and enrichment analysis of differential metabolites
According to the KEGG annotation and enrichment results (Figure 5), the differential metabolites between CK and KW were mainly annotated and enriched in the following pathways: flavonoid biosynthesis, flavone and flavonol biosynthesis, phenylpropanoid biosynthesis, linoleic acid metabolism, biosynthesis of unsaturated fatty acids, and arachidonic acid metabolism, which may be related to the resistance of plants (Figure 5A).
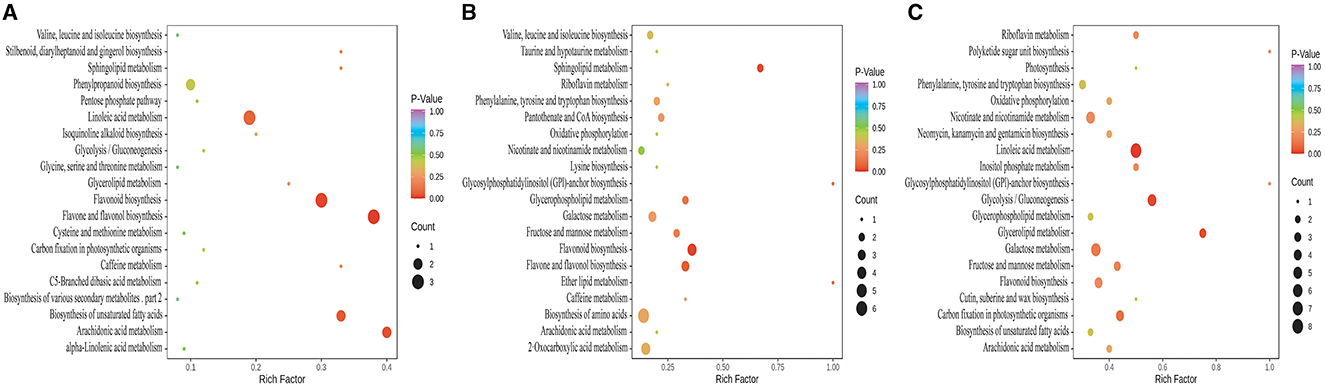
Figure 5. KEGG annotations and enrichment of differentially expressed metabolites of each pairwise comparison of melon. (A) CK vs. KW; (B) CK vs. MM; and (C) KW vs. MM.
In the comparison between CK and MM, the incidence and index of Fusarusim wilt were higher in KW, and the differential metabolites were primarily annotated and enriched in the following pathways: flavonoid biosynthesis, flavone and flavonol biosynthesis, amino acid biosynthesis, 2-oxocarboxylic acid metabolism, galactose metabolism, fructose and mannose metabolism, glycerophospholipid metabolism, nicotinate and nicotinamide metabolism, phenylalanine, tyrosine and tryptophan biosynthesis, sphingolipid metabolism and valine, and leucine and isoleucine biosynthesis (Figure 5B). Otherwise, the differential metabolites between KW and MM were primarily annotated and enriched in the subsequent pathways: linoleic acid metabolism, galactose metabolism, glycolysis/gluconeogenesis, nicotinate and nicotinamide metabolism, carbon fixation in photosynthetic organisms, phenylalanine, tyrosine and tryptophan biosynthesis, fructose and mannose metabolism, and flavonoid biosynthesis (Figure 5C).
In these comparison pairs, the overlapped metabolic pathways included flavone and flavonol biosynthesis, flavonoid biosynthesis, and phenylpropanoid biosynthesis. The remarkable thing was that the metabolic pathways mentioned above all pertain to resistance against pathogen infection.
3.5 Key metabolites and pathways related to orient melon resistance to pathogens
An analysis was conducted to identify the key metabolites related to the incidence of Fusarium wilt in response to pathogen infection. Figure 6 depicts a Venn diagram, illustrating that the overlapping and distinct metabolites were shared among CK vs. KW, CK vs. MM, and KW vs. MM. According to Figure 6, we identified seven key metabolites in three pairwise comparisons. These metabolites were found to be significant in response to pathogen infection and could be divided into four distinct classes. The details of these metabolites are provided in Table 1.
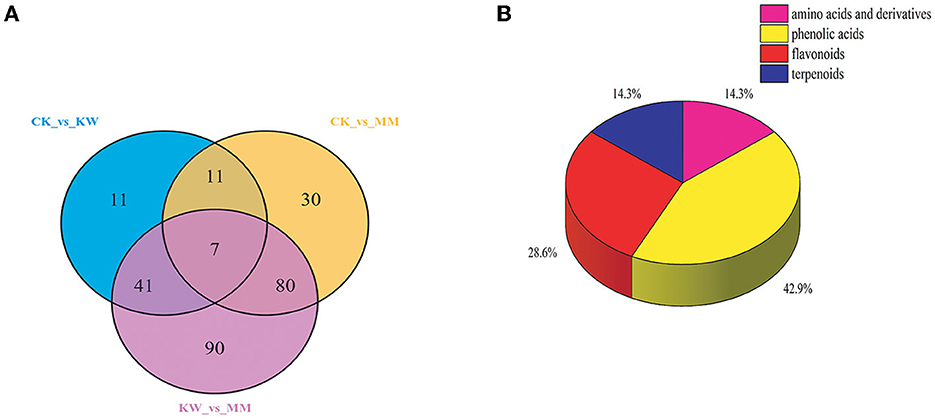
Figure 6. A Venn diagram between CK, KW, and MM (A) and the classification of the seven key metabolites (B).
3.6 Analysis of the pathway of common differential metabolites
With the CK vs. KW pairwise comparison, the metabolites of coniferyl alcohol and chlorogenic acid showed significant enrichment and downregulation in the phenylpropanoid biosynthesis pathway. However, the metabolites of pinobanksin 3-acetate and vitexin exhibited significant enrichment and upregulation in the flavonoid biosynthesis pathway. Isovitexin, vitexin, and chrysoeriol experienced enrichment and significant upregulation in the flavone and flavonol biosynthesis pathways. The compounds of 15-OxoETE, arachidonate, 9,10-DHOME, and 9,12,13-triHOME were found to be enriched and significantly reduced in the pathways of arachidonic acid metabolism and linoleic acid metabolism, except for 9,12,13-triHOME (Figure 7).
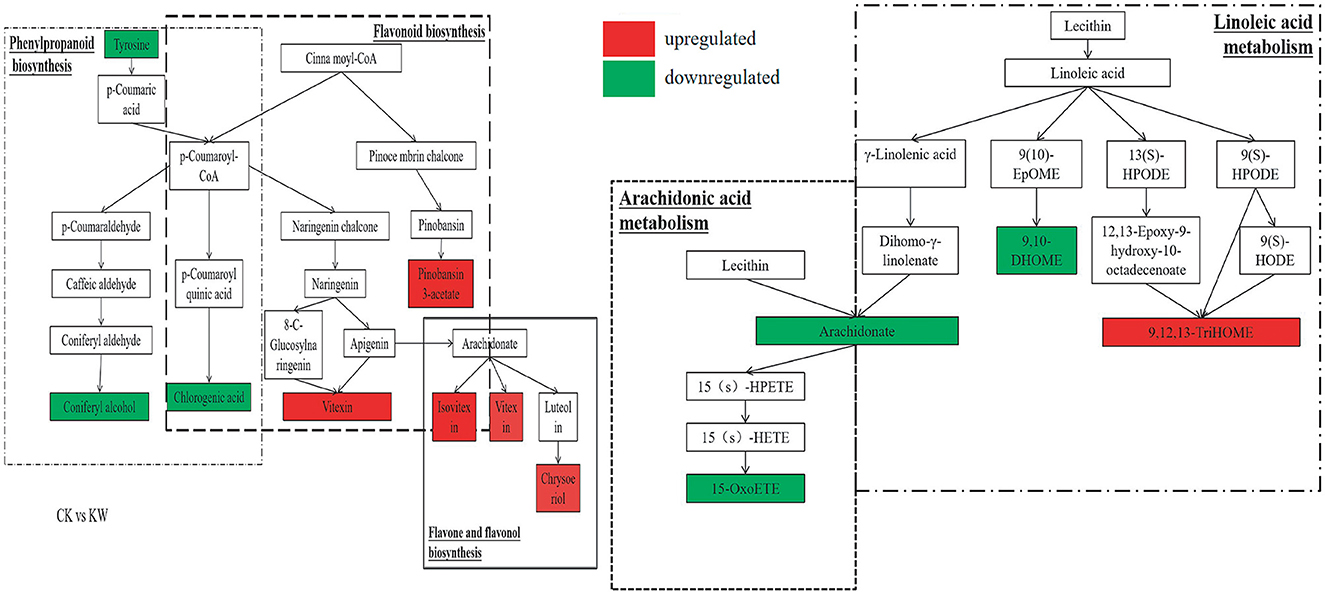
Figure 7. Changes in key metabolites mapped to metabolic pathways in melon samples pairwise comparisons (CK, VS, and KW). The red small rectangle indicates that the metabolite content is significantly upregulated; the green small rectangle indicates that metabolite content is significantly downregulated.
With the KW vs. MM pairwise comparison, glycerone-P, glycerate-3P, shikimate, phenylpyruvate, and phosphoenolpyruvate were enriched, which were significantly upregulated in glycolysis/gluconeogenesis phenylpropanoid pathway. Nicotinamide, NAD, and NADP+ exhibited an increase in abundance and upregulation in the biosynthesis of the cofactor pathway. The compounds of caffeic acid, caffeoyl quinic acid, coniferyl alcohol, syringin, luteolin, eriodictyol, neohesperidin, and coumarin were enriched, which were upregulated significantly except for coumarin in the phenylpropanoid biosynthesis pathway. In the phenylalanine metabolism pathway, the compounds 4-hydroxy-phenylacetate, 2-hydroxy-phenylacetate, phenylpyruvate, and tyrosine were enriched, which were downregulated except for phenylpyruvate. In amino acid pathway biosynthesis, LL-2,6-diaminohepta nedioate, citruline, and oxaloacetate were enriched, which were downregulated except for oxaloacetate (Supplementary Figure 3).
With the CK vs. MM pairwise comparison, glycerate-3P, shikimate, phenylpyruvate, and phosphoenolpyruvate were enriched, which were significantly upregulated in the glycolysis/gluconeogenesis phenylpropanoid pathway. In amino acids pathway biosynthesis, the compounds of (s)-2-aceto-2-hydroxybutanoate, 2,6-diaminoheptanedioate, citruline, and oxaloacetate were found to be enriched, which were downregulated except for oxaloacetate and (s)-2-aceto-2-hydroxybutanoate (Supplementary Figure 4).
4 Discussion
Fusarium wilt, caused by Fusarium oxysporum f. sp. melonis (FOM), posed a significant challenge to melon production due to its persistence in monocropping systems. Utilizing pesticides in the production process may reduce the prevalence of Fusarium wilt, but it poses risks to both human and ecological wellbeing. Previous studies showed that the application of Trichoderma could reduce the intensity of Fusarium wilt in melon. However, the specific mechanism behind the reduction in wilt severity has not been fully understood.
The phenylpropanoid biosynthesis pathway was the major secondary metabolism in various plants when plants suffered from abiotic or biotic stress (Sharma et al., 2019). In this pathway, numerous antioxidants in plants were generated, including flavonoids, phenols, and lignins, preventing Fusarium from attacking the melon plants (Anjali et al., 2023). In this study, the levels of compounds in the phenylpropanoid biosynthesis pathway were found to be significantly increased in KW compared to MM, with the exception of coumarin compounds. Conversely, caffeoyl quinic acid, caffeic acid, coniferylalcohol, syringin, and chlorogenic acid were significantly decreased in CK compared to KW comparison pairs. Chlorogenic acid is an important signaling molecule and plant activator with various physiological functions, especially in induced resistance and in the presence of both biotic and abiotic stress conditions (Zhang Y. et al., 2023). Caffeic acid and syringic acid were the precursors of lignin, which could inhibit the growth of pathogens and enhance plant resistance (König et al., 2014). Plant cell walls were strengthened by the deposition of lignin, referred to as phytoalexin, allowing them to withstand the mechanical pressure caused by pathogen invasion (Liu et al., 2018). In this study, these results suggested that Trichoderma harzianum inoculation would increase the defensive capabilities of oriental melon in comparison to FOM inoculation, implying that Trichoderma harzianum not only inhibited the growth of FOM but also had a tendency to induce the resistance of oriental melon to the pathogen infection. These results may explain the intuitive outcome of Trichoderma harzianum treatment in alleviating the incidence of Fusarium wilt.
Several studies indicated that coumarins were antimicrobial substances that controlled the microbiomes in the rhizosphere and were characterized as phytoalexin agents (Stringlis et al., 2019). Our study revealed that the application of Trichoderma harzianum resulted in a decrease in the secretion of coumarins in the roots of oriental melon. P-coumaric acid, a phenolic acid produced by Cucurbitaceae crops, plays a crucial role in the occurrence of continuous cropping obstacles in these crops (Zhou and Wu, 2012). According to reports, P-coumaric acid negatively affects the growth of cucumbers by altering the composition of microorganisms in the rhizosphere. Zhou et al. (2018) demonstrated the conversion of p-coumaric acid into coumarin using multiple enzymes. However, we could not exclude the possibility that the application of Trichoderma harzianum inhibited the synthesis of self-toxic chemicals and boosted the production of antifungal compounds, thereby reducing the challenges associated with continuous cropping. This could potentially account for the observed decrease in coumarin concentration; however, further studies are needed to confirm these findings.
Flavonoids, acting as phytoalexins or antioxidants, are synthesized from the phenylpropanoid metabolic pathway. They possess the capability to scavenge reactive oxygen species (ROS) and provide protection to plants against both biotic and abiotic stresses (Iwashina, 2003; Pourcel et al., 2007; Cavaiuolo et al., 2013; Zhang et al., 2020). Sakuranetin, a flavanone phytoalexin, was synthesized in rice plants as a defense mechanism against pathogens. In mutant pal06, which cannot produce sakuranetin, there was an increased production of flavonoids and a heightened susceptibility to the rice blast fungus Magnaporthe oryzae (Duan et al., 2014; Hasegawa et al., 2014; Singh et al., 2023). In this study, the content of flavonoids was found to increase in the roots of melon inoculated with FOM or Trichoderma harzianum. However, the treatment of Trichoderma harzianum resulted in a significant decrease in the flavonoid content compared to the treatment with FOM inoculation. Additionally, out of the six different varieties, it was observed that “Yangjiaomi,” an oriental melon, was susceptible to FOM, suggesting that Trichoderma harzianum had the potential to safeguard oriental melon from pathogen infection. However, further studies are required to investigate the specific regulatory mechanisms.
The glycolysis/gluconeogenesis pathway is a metabolic process that involves the conversion of 6-carbon glucose into 2-carbon molecules, which then bond with the tricarboxylic acid cycle. This process leads to the release of ATP (Rui, 2014), which serves as the kinetic energy for plant growth (He et al., 2023). Accumulating evidence shows that the glycolysis/gluconeogenesis pathway is usually upregulated in plants as a response to abiotic and biotic stress conditions, leading to the development of resistance (Li et al., 2020b). In this study, the tricarboxylic cycle and phenylpropanoid biosynthesis nodes were connected via the glycolysis/gluconeogenesis pathway, which was accumulated in the comparison pairs of CK vs. MM and KW vs. MM. Treatment with Trichoderma harzianum stimulated the synthesis of various intermediate metabolites in the glycolysis/gluconeogenesis pathway, such as shikimate, glycerone-3p, phosphoenolpyruvate, and other key compounds (Figure 8). These results showed that Trichoderma harzianum, used as a plant growth-promoting rhizobacterium (PGPR), improved the resistance against FOM and facilitated the growth of oriental melon by providing energy. Nicotinamide adenine dinucleotide (NAD) is a chemical elicitor that regulates plant defense responses to multiple biotic stresses (Abdelsamad et al., 2019; Sidiq et al., 2021). The application of NAD+ to citrus tissues prepared them for more rapid and robust activation of genes in multiple salicylic acid pathways when subsequently infected with Xcc (Alferez et al., 2018). In this study, treatment with MM resulted in higher levels of NAD+, NADP+, and nicotinamide compared to KW. However, in the other comparisons, there was no change in the levels of these compounds, which suggested that the application of Trichoderma harzianum could prime the resistance of melon against pathogen infection, implying that oriental melon suffered from infection with pathogens only.
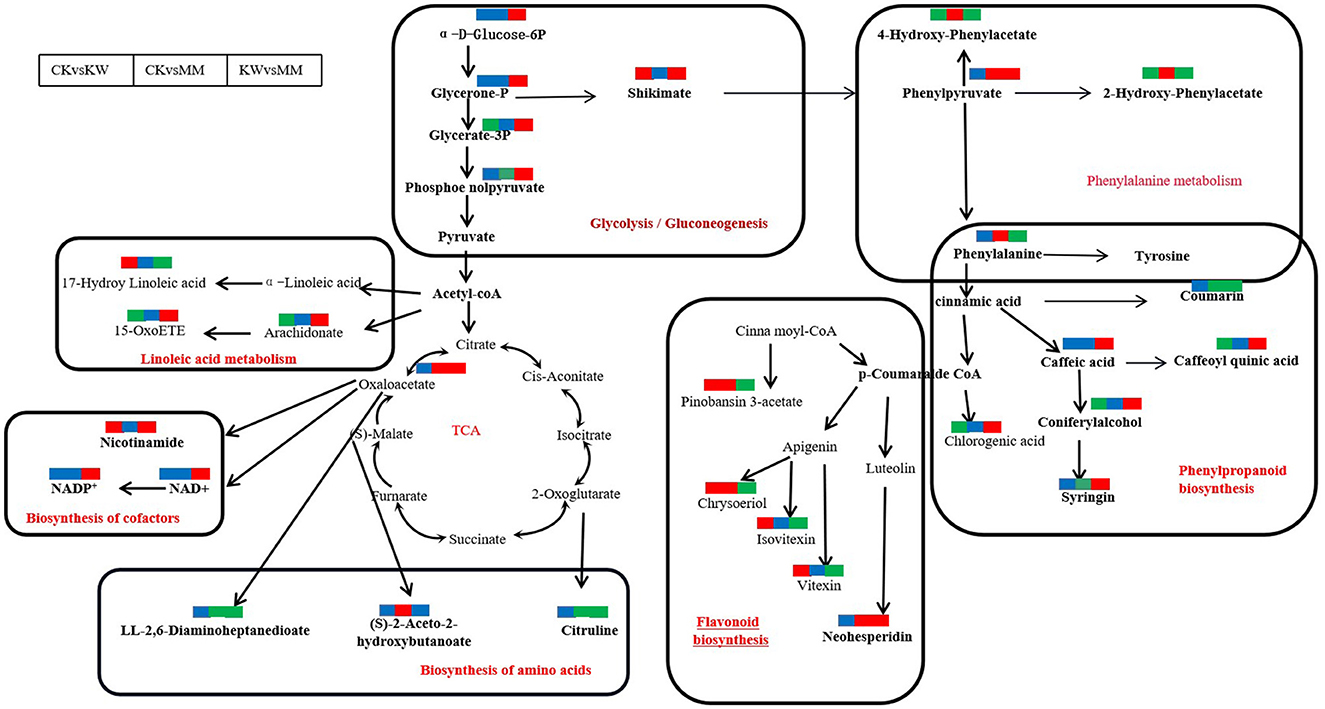
Figure 8. Changes in key metabolites mapped to metabolic pathways in melon samples by pairwise comparisons. The red small rectangle indicates that the metabolite content is significantly upregulated; the green small rectangle indicates that metabolite content is significantly downregulated; the blue small rectangle indicates no significant difference in that metabolite content.
Cucurbitin, referred to as a “green pesticide,” is a potent tool for plant defense against pests and diseases (Balkema-Boomstra et al., 2003). In this investigation, it was discovered that the content of isocurbitin D increased with CK vs. KW comparison pairs. However, in KW vs. MM comparison pairs, isocurbitin A, E, and D contents dramatically decreased, and cucurbitin A and isocucurbitin D levels were dramatically reduced in CK vs. MM comparison pairs. Therefore, the application of Trichoderma harzianum resulted in a considerable reduction in isocucurbitin D levels. Nevertheless, Zhong et al. (2022) reported that cucurchinin B was the primary constituent responsible for melon disease resistance, which may be associated with the differences in cucurbitin production among different oriental melon varieties. Utilizing cucurchinin has the potential to improve melon's resistance to Fusarium wilt while simultaneously enhancing the quality of melon vegetables. However, further studies and analysis will be required to comprehend the varying responses of different cucurbitins to Fusarium wilt.
5 Conclusion
From the metabolic pathway of the KEGG analysis, Trichoderma treatment could alleviate the incidence of Fusarium wilt for its inhibiting effect and change the metabolites in oriental melon under the infection of pathogens, mainly through regulating the phenylpropanoid biosynthesis pathway and the flavone and flavonol biosynthesis pathways, whereas Trichoderma harzianum application decreased the infection of pathogens, increased the biosynthesis of glycolysis/gluconeogenesis, and increased the biosynthesis of cofactors related to growth. This study can provide new insights into the understanding of the response of oriental melon against the infection of FOM and the mechanisms of improving the resistance of oriental melon treated with Trichoderma harzianum to Fusarium wilt exerted by the metabolism level.
Data availability statement
The original contributions presented in the study are included in the article/Supplementary material, further inquiries can be directed to the corresponding authors.
Author contributions
CL: Writing – original draft, Data curation. JN: Investigation, Writing – review & editing. XingW: Investigation, Writing – review & editing. YZ: Project administration, Writing – review & editing. XL: Resources, Writing – review & editing. XiaW: Methodology, Writing – review & editing. KY: Writing – review & editing. YJ: Writing – review & editing.
Funding
The author(s) declare financial support was received for the research, authorship, and/or publication of this article. This work was supported by the Heilongjiang Postdoctoral Science Foundation (LBH-Z21225) and the Research Foundation for Advanced Talents (XYB201924).
Acknowledgments
We would like to thank the agency of the People's Republic of China for financing the project.
Conflict of interest
The authors declare that the research was conducted in the absence of any commercial or financial relationships that could be construed as a potential conflict of interest.
Publisher's note
All claims expressed in this article are solely those of the authors and do not necessarily represent those of their affiliated organizations, or those of the publisher, the editors and the reviewers. Any product that may be evaluated in this article, or claim that may be made by its manufacturer, is not guaranteed or endorsed by the publisher.
Supplementary material
The Supplementary Material for this article can be found online at: https://www.frontiersin.org/articles/10.3389/fsufs.2024.1354468/full#supplementary-material
Supplementary Figure 1. TIC overlap was detected by QC sample mass spectrum [(A) negative ion mode and (B) positive ion mode].
Supplementary Figure 2. The model plots of OPLS-DA pairwise comparisons of differential metabolites. (A) CK vs. KW; (B) CK vs. MM; and (C) KW vs. MM.
Supplementary Figure 3. Changes in key metabolites mapped to metabolic pathways in melon samples pairwise comparisons KW vs. MM. The red color small rectangle indicates that the metabolite content is significantly upregulated, and the green small rectangle indicates that the metabolite content is significantly downregulated.
Supplementary Figure 4. Changes in key metabolites mapped to metabolic pathways in melon samples pairwise comparisons CK vs. MM. The red color small rectangle indicates that the metabolite content is significantly upregulated, and the green small rectangle indicates that the metabolite content is significantly downregulated.
References
Abdelsamad, N., Regmi, H., Desaeger, J., and DiGennaro, P. (2019). Nicotinamide adenine dinucleotide induced resistance against root-knot nematode Meloidogyne hapla is based on increased tomato basal defense. J. Nematol. 51, 1–10. doi: 10.21307/jofnem-2019-022
Alferez, F. M., Gerberich, K. M., Li, J. L., Zhang, Y., Graham, J. H., and Mou, Z. (2018). Exogenous nicotinamide adenine dinucleotide induces resistance to citrus canker in citrus. Front. Plant Sci. 9:1472. doi: 10.3389/fpls.2018.01472
Amdan, N. L., Shalaby, S., Ziv, T., Kenerley, C. M., and Horwitz, B. A. (2015). Secretome of the biocontrol fungus Trichoderma virens co-cultured with maize roots: role in induced systemic resistance. Mol. Cell. Proteom. 14, 1054. doi: 10.1074/mcp.M114.046607
Anjali, K. S., Korra, T., Thakur, R., Arutselvan, R., Kashyap, A. S., Nehela, Y., et al. (2023). Role of plant secondary metabolites in defence and transcriptional regulation in response to biotic stress. Plant Stress 2023, 100154. doi: 10.1016/j.stress.2023.100154
Bader, A. N., Salerno, G. L., Covacevich, F., and Consolo, V. F. (2020). Native Trichoderma harzianum strains from Argentina produce indole-3 acetic acid and phosphorus solubilization, promote growth and control wilt disease on tomato (Solanum lycopersicum L.). J. King Saud Univ. Sci. 32, 867–873. doi: 10.1016/j.jksus.2019.04.002
Balkema-Boomstra, A. G., Zijlstra, S., Verstappen, F. W. A., Inggamer, H., Mercke, P. E., and Jongsma, M. A. (2003). Role of cucurbitacin C in resistance to spider mite (Tetranychus urticae) in cucumber (Cucumis sativus L.). J. Chemic. Ecol. 29, 225–235. doi: 10.1023/A:1021945101308
Carrion, V. J., Perez-Jaramillo, J., Cordovez, V., Tracanna, V., de Hollander, M., Ruiz-Buck, D., et al. (2019). Pathogen-induced activation of disease-suppressive functions in the endophytic root microbiome. Science 366, 606–612. doi: 10.1126/science.aaw9285
Cavaiuolo, M., Cocetta, G., and Ferrante, A. (2013). The antioxidants changes in ornamental flowers during development and senescence. Antioxidants 2, 132–155. doi: 10.3390/antiox2030132
Chen, H., Wu, H., Yan, B., Zhao, H., Liu, F., Zhang, H., et al. (2018). Core microbiome of medicinal plant Salvia miltiorrhiza seed: a rich reservoir of beneficial microbes for secondary metabolism? Int. J. Mol. Sci. 19, 672. doi: 10.3390/ijms19030672
Chen, W., Gong, L., Guo, Z., Wang, W., Zhang, H., and Liu, X. (2013). A novel integrated method for large-scale detection, identification, and quantification of widely targeted metabolites: application in the study of rice metabolomics. Mol. Plant 6, 1769–1780. doi: 10.1093/mp/sst080
Duan, L., Liu, H., Li, X., Xiao, J., and Wang, S. (2014). Multiple phytohormones and phytoalexins are involved in disease resistance to Magnaporthe oryzae invaded from roots in rice. Physiol. Plant 152, 486–500. doi: 10.1111/ppl.12192
Gordon, T. R. (2017). Fusarium oxysporum and the Fusarium wilt syndrome. Annu. Rev. Phytopathol. 55, 23–39. doi: 10.1146/annurev-phyto-080615-095919
Hasegawa, M., Mitsuhara, I., Seo, S., Okada, K., Yamane, H., Iwai, T., et al. (2014). Analysis on blast fungus-responsive characters of a flavonoid phytoalexin sakuranetin; accumulation in infected rice leaves, antifungal activity and detoxification by fungus. Molecules 19, 11404–11418. doi: 10.3390/molecules190811404
He, M. Y., Ren, T. X., Jin, Z. D., Deng, L., Liu, H. J., Cheng, Y. Y., et al. (2023). Precise analysis of potassium isotopic composition in plant materials by multi-collector inductively coupled plasma mass spectrometry. Spectrochim. Acta B At. Spectrosc. 209:106781. doi: 10.1016/j.sab.2023.106781
Huang, W., Long, C., and Lam, E. (2018). Roles of plant-associated microbiota in traditional herbal medicine. Trends Plant Sci. 23, 559–562. doi: 10.1016/j.tplants.2018.05.003
Iwashina, T. (2003). Flavonoid function and activity to plants and other organisms. Biol. Sci. Space 17, 24–44. doi: 10.2187/bss.17.24
Kan, Y., Kan, H., Bai, Y., Zhang, S., and Gao, Z. (2023). Effective and environmentally safe self-antimildew strategy to simultaneously improve the mildew and water resistances of soybean flour-based adhesives. J. Clean. Prod. 392, 136319. doi: 10.1016/j.jclepro.2023.136319
König, S., Feussner, K., Kaever, A., Landesfeind, M., Thurow, C., Karlovsky, P., et al. (2014). Soluble phenylpropanoids are involved in the defense response of Arabidopsis against Verticillium longisporum. New Phytol. 202, 823–837. doi: 10.1111/nph.12709
Li, Y., Guo, Q., He, F., Li, Y., Xue, Q., and Lai, H. (2020a). Biocontrol of root diseases and growth promotion of the tuberous plant Aconitum carmichaelii induced by Actinomycetes are related to shifts in the rhizosphere microbiota. Microb. Ecol. 79, 134–147. doi: 10.1007/s00248-019-01388-6
Li, Y., Mo, X., Xiong, J., Huang, K., Zheng, M., Jiang, A., et al. (2023). Deciphering the probiotic properties and safety assessment of a novel multi-stress-tolerant aromatic yeast Pichia kudriavzevii HJ2 from marine mangroves. Food Biosci. 56:103248. doi: 10.1016/j.fbio.2023.103248
Li, Y., Zhao, M., Chen, W., Du, H., Xie, X., Wang, D., et al. (2020b). Comparative transcriptomic analysis reveals that multiple hormone signal transduction and carbohydrate metabolic pathways are affected by Bacillus cereus in Nicotiana tabacum. Genomics 112, 4254–4267. doi: 10.1016/j.ygeno.2020.07.022
Liu, Q., Luo, L., and Zheng, L. (2018). Lignins: biosynthesis and biological functions in plants. Int. J. Mol. Sci. 19:335. doi: 10.3390/ijms19020335
López-Bucio, J., Pelagio-Flores, R., and Herrera-Estrella, A. (2015). Trichoderma as biostimulant: exploiting the multilevel properties of a plant beneficial fungus. Sci. Hortic. 196:109–123. doi: 10.1016/j.scienta.2015.08.043
Malmierca, M. G., Barua, J., McCormick, S. P., Izquierdo-Bueno, I., Cardoza, R. E., Alexander, N. J., et al. (2015). Novel aspinolide production by Trichoderma arundinaceum with a potential role in Botrytis cinerea antagonistic activity and plant defence priming. Environ. Microbiol. 17, 1103–1118. doi: 10.1111/1462-2920.12514
Pang, Z., Chen, J., Wang, T., Gao, C., Li, Z., Guo, L., et al. (2021). Linking plant secondary metabolites and plant microbiomes: a review. Front. Plant Sci. 12:621276. doi: 10.3389/fpls.2021.621276
Pascale, A., Proietti, S., Pantelides, I. S., and Stringlis, I. A. (2019). Modulation of the root microbiome by plant molecules: the basis for targeted disease suppression and plant growth promotion. Front. Plant Sci. 10:1741. doi: 10.3389/fpls.2019.01741
Peng, M., Shahzad, R., Gul, A., Subthain, H., Shen, S., Lei, L., et al. (2017). Differentially evolved glucosyltransferases determine natural variation of rice flavone accumulation and UV-tolerance. Nat. Commun. 17, e02168. doi: 10.1038/s41467-017-02168-x
Pourcel, L., Routaboul, J. M., Cheynier, V., Lepiniec, L., and Debeaujon, I. (2007). Flavonoid oxidation in plants: from biochemical properties to physiological functions. Trends Plant Sci. 12, 29–36. doi: 10.1016/j.tplants.2006.11.006
Qin, X., Zhang, K., Fan, Y., Fang, H., Nie, Y., and Wu, X. L. (2022). The bacterial MtrAB two-component system regulates the cell wall homeostasis responding to environmental alkaline stress. Microbiol. Spectr. 10, e0231122. doi: 10.1128/spectrum.02311-22
Rui, L. Y. (2014). Energy metabolism in the liver. Compr. Physiol. 4, 177–197. doi: 10.1002/cphy.c130024
Sharma, A., Shahzad, B., Rehman, A., Bhardwaj, R., Landi, M., and Zheng, B. (2019). Response of phenylpropanoid pathway and the role of polyphenols in plants under abiotic stress. Molecules 24, 2452. doi: 10.3390/molecules24132452
Shi, W., Li, M., Wei, G., Tian, R., Li, C., Wang, B., et al. (2019). The occurrence of potato common scab correlates with the community composition and function of the geocaulosphere soil microbiome. Microbiome 7, 14. doi: 10.1186/s40168-019-0629-2
Sidiq, Y., Nakano, M., Mori, Y., Yaeno, T., Kimura, M., and Nishiuchi, T. (2021). Nicotinamide effectively suppresses fusarium head blight in wheat plants. Int. J. Mol. Sci. 22:2968. doi: 10.3390/ijms22062968
Singh, G., Argawal, H., and Bednarek, P. (2023). Specialized metabolites as versatile tools in shaping plant-microbe associations. Mol. Plant 12, 6. doi: 10.1016/j.molp.2022.12.006
Snelders, N. C., Rovenich, H., Petti, G. C., Rocafort, M., van den Berg, G. C. M., Vorholt, J. A., et al. (2020). Microbiome manipulation by a soil-borne fungal plant pathogen using effector proteins. Nat. Plants 6, 1365–1374. doi: 10.1038/s41477-020-00799-5
Stringlis, I. A., de Jonge, R., and Pieterse, C. M. J. (2019). The age of coumarins in plant-microbe interactions. Plant Cell Physiol. 60, 1405–1419. doi: 10.1093/pcp/pcz076
Thakur, H., Sharma, S., and Thakur, M. (2019). Recent trends in muskmelon (Cucumis melo L.) research: an overview. J. Hort. Sci. Biotechnol. 94, 533–547. doi: 10.1080/14620316.2018.1561214
Wang, Y., Li, C., Shen, B., Zhu, L., Zhang, Y., and Jiang, L. (2023). Ultra-small Au/Pt NCs@GOX clusterzyme for enhancing cascade catalytic antibiofilm effect against F. nucleatum-induced periodontitis. Chem. Eng. J. 466, 143292. doi: 10.1016/j.cej.2023.143292
Wen, T., Yuan, J., He, X., Lin, Y., Huang, Q., and Shen, Q. (2020). Enrichment of beneficial cucumber rhizosphere microbes mediated by organic acid secretion. Hortic. Res. 7, 154. doi: 10.1038/s41438-020-00380-3
Xue, M., Wang, R., Zhang, C., Wang, W., Zhang, F., Chen, D., et al. (2021). Screening and identification of Trichoderma strains isolated from natural habitats in China with potential agricultural applications. BioMed Res. Int. 2021, 7913950. doi: 10.1155/2021/7913950
Zhang, P., Du, H., Wang, J., Pu, Y., Yang, C., Yan, R., et al. (2020). Multiplex CRISPR/Cas9-mediated metabolic engineering increases soya bean isoflavone content and resistance to soya bean mosaic virus. Plant Biotechnol. J. 18, 1384–1395. doi: 10.1111/pbi.13302
Zhang, T., Yu, S., Pan, Y., Li, H., Liu, X., and Cao, J. (2023). Properties of texturized protein and performance of different protein sources in the extrusion process: a review. Food Res. Int. 174, 113588. doi: 10.1016/j.foodres.2023.113588
Zhang, Y., Zhang, W., Wang, H., Shu, C., Chen, L., Cao, J., et al. (2023). The combination treatment of chlorogenic acid and sodium alginate coating could accelerate the wound healing of pear fruit by promoting the metabolic pathway of phenylpropane. Food Chem. 414, 135689. doi: 10.1016/j.foodchem.2023.135689
Zhong, Y., Xun, W., Wang, X., Tian, S., Zhang, Y., Li, D., et al. (2022). Root-secreted bitter triterpene modulates the rhizosphere microbiota to improve plant fitness. Nat. Plants 22, 2. doi: 10.1038/s41477-022-01201-2
Zhou, X., and Wu, F. (2012). p-Coumaric acid influenced cucumber rhizosphere soil microbial communities and the growth of Fusarium oxysporum f.sp. cucumerinum Owen. PLoS ONE 7, e48288. doi: 10.1371/journal.pone.0048288
Zhou, X., Zhang, J., Pan, D., Ge, X., Jin, X., Chen, S., et al. (2018). p-Coumaric can alter the composition of cucumber rhizosphere microbial communities and induce negative plant-microbial interactions. Biol. Fertil. Soils 54, 363–372. doi: 10.1007/s00374-018-1265-x
Keywords: Trichoderma harzianum, Fusarium oxysporum f. sp. melonis, oriental melon, UPLC-ESI-MS, secondary metabolites
Citation: Li C, Nie J, Wu X, Zhang Y, Li X, Wu X, Yin K and Jin Y (2024) Oriental melon roots metabolites changing response to the pathogen of Fusarium oxysporum f. sp. melonis mediated by Trichoderma harzianum. Front. Sustain. Food Syst. 8:1354468. doi: 10.3389/fsufs.2024.1354468
Received: 12 December 2023; Accepted: 05 January 2024;
Published: 25 January 2024.
Edited by:
Muhammad Saqlain Zaheer, Khwaja Fareed University of Engineering and Information Technology (KFUEIT), PakistanReviewed by:
Salim Manoharadas, King Saud University, Saudi ArabiaFatima Rasool, Islamia University of Bahawalpur, Pakistan
Copyright © 2024 Li, Nie, Wu, Zhang, Li, Wu, Yin and Jin. This is an open-access article distributed under the terms of the Creative Commons Attribution License (CC BY). The use, distribution or reproduction in other forums is permitted, provided the original author(s) and the copyright owner(s) are credited and that the original publication in this journal is cited, in accordance with accepted academic practice. No use, distribution or reproduction is permitted which does not comply with these terms.
*Correspondence: Chunxia Li, lcx198238@163.com; Kuide Yin, yinkuide@sohu.com; Yazhong Jin, jyz_hsp@126.com