- 1Institute of Nutrition, Mahidol University, Salaya, Thailand
- 2Internaitonal Center of Excellence in Seafood Science and Innovation, Faculty of Agro-Industry, Prince of Songkla University, Songkhla, Thailand
- 3Faculty of Science, Mahidol University, Bangkok, Thailand
- 4Department of Food Science and Nutrition, College of Agriculture and Food Sciences, King Faisal University, Al-Ahsa, Saudi Arabia
The present study investigated the antioxidant and antimicrobial activities of Bao mango and daikon radish peel (Thai variety) ethanolic extracts. The percentage extraction yield of radish peel was higher compared to the mango peel (p < 0.05). However, Mango peel extract (MPE) contained higher total phenolic content (TPC) than radish peel extract (RPE) (p < 0.05). Similar to the TPC value, antioxidant activities including DPPH radical scavenging activity, reducing power, and peroxidation inhibition were higher in the MPE, compared to the RPE, except for metal chelating activity. The antimicrobial analyses suggested that the MPE showed lower minimum inhibitory concentration (MIC) and minimum bactericidal concentration (MBC) values compared to the RPE (p < 0.05) against Gram-positive and Gram-negative bacteria. High-performance liquid chromatography (HPLC) revealed that mangiferin was the major phenolic compound present in MPE, followed by 3, 4 dihydroxybenzoic acid. Therefore, MP could be used as a promising natural antioxidant and antimicrobial agent for food applications.
1 Introduction
Fruits and vegetables are the most commonly consumed commodities among all horticultural crops. Hence, the production and processing of these horticulture products have increased exponentially to fulfill their increasing demands. In Thailand, an increase in agricultural production has been reported by the Office of Agricultural Economics (OAE), and it was reported that the agricultural gross domestic product (GDP) of 2021 expanded by 1.7 to 2.7%, compared to the year 2020 (OAE, 2019; Nation, 2021). Similarly, these industries generated 10–60% of waste in the form of peels, seeds, pomace, etc. (Kaur et al., 2019). The Food and Agriculture Organization of the United Nations (FAO) reported that annual global food waste is at least one-third (1.3 billion tons) of the food produced, and most of the food loss occurs in the horticulture sector. An increase in agricultural production will bring about increased waste. Most of these wastes are simply dumped in municipal landfills, causing health hazards due to environmental pollution. The utilization of this waste for the recovery of added-value compounds offers a new avenue for waste management, industrial growth, and the country’s economy. Generally, these wastes contain large amounts of polysaccharides, fibers, pigments, minerals, and phytochemicals (Kumar et al., 2017). The extraction of phytochemical compounds can be a sustainable and lucrative alternative due to their broad spectrum of bioactivities (Nirmal et al., 2015). The phytochemicals mostly present in fruit and vegetable waste (FVW) are polyphenols, flavonoids, carotenoids, isoflavones, phenolic acid, tannins, isothiocyanates, lignans, saponins, etc. (Nirmal et al., 2023b). Many in-vitro and in-vivo studies provide evidence that various phytochemicals extracted from plant waste material showed prevention or control of various diseases, including diabetes, cancer, cardiovascular disorders, hypertension, etc. (Nirmal et al., 2015; Rajput et al., 2022). In addition, these plant waste extracts or phytochemicals act as natural preservatives and increase the shelf life of various food systems, including shrimp, mincemeat, fish fillets, etc. (Rathod et al., 2023; Nirmal et al., 2023b). Thus, for value addition to waste, the extraction of bioactive and functional compounds is the best approach (Leong and Chang, 2022).
Globally, a large amount of mango (Mangifera indica) peel waste is generated during mango juice processing. As per the 2020 report of the FAO, the total quantity of mangoes exported from Thailand was nearly 339,917 tonnes in 2022 (FAO, 2023), and during the processing of mangoes, fruit culls, peels, and seeds make 35–60% of the total weight that is discarded as waste (Manhongo et al., 2021). At the same time, mango peel has been frequently reported to have a high bioactive compound content, including flavonoids, phenolic acids, fiber, vitamins, carotenoids, etc. (Okino-Delgado et al., 2020). Mangiferin (a xanthone), one of the most abundant and potent antioxidants, has been identified in Mangifera indica L. var. (Zafra Ciprián et al., 2023). On the other hand, radish extract is reported to have strong antimicrobial activity (Maqbool et al., 2020). Primarily, radish contains flavonoids and isothiocyanate (4-(methylthio)-3-butenyl isothiocyanate, benzyl isothiocyanate, allyl isothiocyanate, and phenethyl isothiocyanate). Lugasi et al. (2005) investigated the in vitro antioxidant activities of radish peel, and L-tryptophan was identified to possess antioxidant activity. Moreover, radish root extract prevents membrane alterations induced by a high-fat diet and stimulates scavenging activity in rats, thus safeguarding cell membranes from lipid peroxidation (Sipos et al., 2002). However, a few or no reports on the antioxidant and antimicrobial activities of the ethanolic extracts from Bao mango (Mangifera indica L. var.) from the south of Thailand and from Daikon radish (Raphanus sativus var. Longipinnatus) grown in Thailand exist. Therefore, the present study investigated total phenolic content, antioxidant and antimicrobial potential of Bao mango peel and Daikon radish peel ethanolic extracts. Furthermore, the major phytochemicals present in Bao mango peel extract were also determined.
2 Materials and methods
2.1 Raw material
Radish peel (Daikon/White Radish, Raphanus sativus var. Longipinnatus) waste was collected from MK restaurant in Nakhon Pathom province, Thailand, whereas Thai indigenous mango peel (Bao mango, Mangifera indica L. var.) waste was received from Promsap (Thailand) Company Limited, Hat Yai, Songkla, Thailand. Only peels from either mango and radish were collected and washed to remove any dust or foreign particles.
2.2 Preparation and extraction of mango and radish peel
The peel was dried overnight in a hot air oven at 50°C until the moisture content was reduced to less than 10%. Afterward, each dried sample was ground to powder with the blender and sieved with a stainless-steel sieve of mesh size 35. Each sample was extracted with 80% ethanol solvent (5 g/250 mL) at 40°C for 2 h using a temperature-controlled hot plate magnetic stirrer (IKA C-MAG HS7, Don Mueng, Bangkok, Thailand). Then, the mixture was filtered using Whatman filter paper no. 1, and the filtrate was concentrated using an Eyela rotary evaporator (Eyela USA, NY, United States) at 40°C. The concentrated extract was collected and lyophilized.
The extraction yield was calculated by dividing the weight of the extract powder by the weight of the initial sample and multiplying by 100.
2.3 Total phenolic content
Initially, properly diluted extract (200 μL) was added with 10% Folin–Ciocalteu reagent (1 mL) and stood for 3 min at room temperature. Thereafter, 1 mL of a 2% sodium carbonate solution was added to the mixture and left to stand for 30 min under dark conditions. The absorbance of the reaction mixture was read at 760 nm using a spectrophotometer (Perkin Elmer LS 55, United States). For the blank, deionized water was used instead of the Folin–Ciocalteu reagent in the sample blank, and the extract was excluded and ethanol was used instead in the control. The concentration of total phenolic compound in the extracts was calculated from the standard curve of quercetin with a range of 1–10 μg/mL and expressed as μg QE/ g of extract powder.
2.4 DPPH radical scavenging assay
Briefly, diluted extract (1 mL) was added to 1 mL of DPPH (0.15 mM) prepared in 95% ethanol and incubated for 30 min at room temperature in dark conditions (Nirmal and Benjakul, 2011). The absorbance of the mixture was read at 517 nm using the UV spectrophotometer. The sample blank was prepared using ethanol instead of a DPPH solution, while the control consisted of a DPPH solution and ethanol. The percentage inhibition of DPPH radical was calculated using the following equation and results were expressed as the IC50 value (μg/ mL):
where Asample and Acontrol are the absorbance of sample and control, respectively.
2.5 Reducing power assay
A diluted extract in phosphate buffer (500 μL, 0.2 M, pH 6.6) was mixed with 1% potassium ferric cyanide (500 μL), and the mixtures were incubated at 50°C for 20 min (Nirmal and Panichayupakaranant, 2015). Thereafter, 500 μL of 10% trichloroacetic acid was added and cooled at room temperature. The mixtures were centrifuged at 1000 × g for 10 min and the supernatant (500 μL) was added to the deionized water (500 μL) and 0.1% ferric chloride (100 μL) mixture. The preparation of each sample blank and the control were managed similarly. The phosphate buffer was used instead of potassium ferric cyanide in the sample blank and instead of extract in the control. The absorbance of the reaction was read at 700 nm. A higher absorbance of the sample indicated a higher reducing capacity.
2.6 Metal chelating assay
The assay was conducted as mentioned by Nguyen et al. (2023). Briefly, 1 mL of each sample (0.25–4 mg/mL) was added to the 25 μL of 2 mM FeCl2 · 4H2O. Afterward, 5 mM ferrozine (100 μL) was mixed with the mixture and vortexed. After incubation at room temperature for 10 min, the absorbance of the sample was recorded at 562 nm. EDTA was used as the positive control in this assay. The percentage of the chelating ability of the sample was determined using the following equation and the results were expressed as the IC50 value (mg/mL).
Chelating ability (%) =
where Ao = Abs of control sample (95% Ethanol + FeCl2 · 4H2O + Ferrozine)
As = Abs of a sample
2.7 Linoleic acid peroxidation method
The fatty acid emulsion was prepared using linoleic acid (0.28 g), Tween-40 (0.28 g), and 50 mL of phosphate buffer (0.2 M, pH 7.0) as mentioned by Jayaprakasha et al. (2001). Each diluted sample in 75% ethanol (0.5 mL) was mixed with emulsion (2.5 mL) and phosphate buffer (2.5 mL) and incubated at 37°C for 120 h. The peroxidation of fatty acid emulsion was examined using the thiocyanate method. Briefly, 0.1 mL of the emulsion mixture was taken every 24 h to mix with 5.0 mL of ethanol (75%), 0.1 mL of ammonium thiocyanate (30%), and 0.1 mL of FeCl2 (20 mM in 3.5% HCl). The absorbance of the reaction mixture was read at 500 nm after standing at room temperature for 3 min. The control was prepared using 75% ethanol instead of the sample in the same manner.
2.8 Gram-positive and gram-negative bacteria inhibition assay
Minimal inhibitory concentration (MIC) was determined using the broth microdilution assay as described by Htoo et al. (2019). Bacterial cultures Gram-positive (Staphylococcus aureus-ATCC 29213 and Bacillus subtills-PY 79), and Gram-negative (Escherichia coli-ATCC 25922, and Salmonella typhimurium-DMST 562) were grown on trypticase soy agar (TSA) plates. The freshly grown colonies were transferred to tryptic soy broth (TSB) until the absorbance of 0,2 was achieved at 600 nm. A 96-well plate was prepared with mango peel extract (MPE) or radish peel extract (RPE) with serial dilution. Subsequently, bacterial culture was diluted to 1:100 into each 96-well plate to obtain the final bacterial concentration of 105 CFU/mL. The plates were incubated at 30°C for 24 h and were visually observed for bacterial growth. The MIC of the sample was noted where there was no bacterial growth.
The medium from each MIC well that showed no apparent growth was removed and subculture onto Mueller-Hinton agar (MHA) plates. To determine the minimal bactericidal concentration (MBC), the MHA plates were incubated at 30°C for 24 h to observe the growth of bacteria.
2.9 Determination of phenolic profile
The sample with higher antioxidant and antimicrobial activities was subjected to high-performance liquid chromatography-electrospray ionization tandem mass spectrometry (HPLC-ESI-MS/MS) to examine the phytochemicals in the selected extract. The procedures mentioned by Sirichai et al. (2022) and Chupeerach et al. (2022) were followed. The standard phenolic compounds include 3,4-dihydroxybenzoic acid, genistein, caffeic acid, hesperidin, (−)-epigallocatechin gallate, 4-hydroxybenzoic acid, apigenin, kaempferol, cinnamic acid, chlorogenic acid, naringenin, p-coumaric acid, ferulic acid, mangiferin, sinapic acid, syringic acid, quercetin, myricetin, vanillic acid, gallic acid, rutin, and galangin and rosmarinic acid (Sigma-Aldrich, St. Louis, MO, United States) were used as the standards.
2.10 Statistical analysis
All experiments were performed in triplicates and the results are expressed as mean ± standard deviation. One-way analysis of variance (ANOVA) followed by Duncan’s tests was used to evaluate significant differences. All the statistical analysis was conducted using SPSS, and significant differences between treatments were considered significant at p < 0.05.
3 Results and discussion
3.1 Extraction yield and total phenolic content (TPC)
The percentage extraction yield of radish peel (39.12%) was higher compared to the mango peel extract powder (18.41%) (p < 0.05). Safdar et al. (2017) reported that mango peel extracted using 80% methanol achieved higher extraction yield, but higher TPC was noted in 80% ethanol solvent. On the other hand, Janjua and Shahid (2013) reported that radish peel (R. sativus) extracted using 80% ethanol achieved a higher extraction yield (5.6%) compared to the petroleum ether (5%), and methanol (4.2%) extract. The extraction yield of radish peel in this study was higher compared to the report of Janjua and Shahid (2013). It was noted that Janjua and Shahid (2013) extracted radish peel samples at room temperature, whereas this study used 40°C temperature for the extraction. Hence, the extraction yield of any plant material is affected by the type of solvent, temperature, time of incubation, and most importantly plant material structural integrity and presence of phytochemicals (Nirmal et al., 2023b).
TPC in the mango peel powder was significantly (p < 0.05) higher than that of the radish peel powder. The TPC in the MPE sample was 280.5 ± 7.95 μg quercetin/ g of extract powder, whereas 25.92 ± 0.32 μg quercetin/ g of extract powder was found in the RPE sample (Table 1). Earlier, Noor et al. (2015) reported that the Mangifera indica L. var. Perlis Sunshine mango peel contained a TPC value of 21.7 mg/g of peels. The mango peel is known to have a higher TPC and free radical scavenging activity compared to the mango pulp (Abbasi et al., 2015; Agatonovic-Kustrin et al., 2018). On the other hand, Noman et al. (2021) reported that the TPC content in white radish leaves (125.3 GAE/g) was higher than the root (95.8 GAE/g). Also, extraction methods have a significant effect on the TPC of fruits and vegetable wastes, as Martínez-Ramos et al. (2020) documented that the optimal extraction is dependent on the solvent employed. Ethanol-acetone blend yielded the extract exhibiting the highest activity compared to the extract obtained from conventional extraction of mango (Mangifera indica L.) peel. Therefore, MPE had higher TPC content than RPE.

Table 1. Total phenolic contents and antioxidant potential of mango peel and radish peel extract powder.
3.2 Antioxidant activities
3.2.1 DPPH radical scavenging activity
The IC50 values of MPE and RPE for DPPH free radical scavenging capacity are presented in Table 1. The lower the IC50 value, the higher the inhibitory activity of the compound was. For DPPH radical scavenging activity, the IC50 value of MPE was 35.09 ± 0.00 μg/mL, exhibiting higher scavenging activity, compared to the RPE (2,552.1 ± 17.9 μg/mL) (p < 0.05). This result was in agreement with the TPC value of respective samples where higher TPC content was noted in MPE compared to the RPE. Ajila et al. (2007) reported that IC50 values of different varieties of Indian mango peel vary from 1.83 to 4.54 μg/mL of GAE, where the concentration-dependent scavenging of DPPH radical was noted. Contrary to the present study, Noman et al. (2021) reported higher scavenging activity of Raphanus sativus L. roots extract, which possesses DPPH scavenging activity with an IC50 value of 359.7 μg/mL. Thakare et al. (2023) reported that mango peel extract exhibited DPPH activity of 76.58 and 72.37% by sodium cumene sulfonate and methanolic (50%, v/v) extraction, respectively. Nevertheless, Dorta et al. (2012) reported that the antioxidant activities (DPPH, ABTS radical scavenging, and lipid peroxidation inhibition) of mango peel and seed extract were affected by different drying treatments. However, higher antioxidant activities were reported in mango peel extract compared to the mango seed extract. Furthermore, the antioxidant activities of both samples were related to the phenols and anthocyanin content. Antioxidant activity evaluation by scavenging the DPPH free radical is attributed to the sample’s ability to donate hydrogen atoms (Nirmal et al., 2021). When phenolic compounds interact with DPPH, it produces 2,2-diphenyl-1-picrylhydrazine, whose color shift reveals the scavenging ability of the antioxidants present in the extract (Dahal and Koirala, 2020). The reactive potentiality of the phenol moiety, i.e., the hydroxyl group on their aromatic ring, gives phenolics their antioxidant properties. Phenols can neutralize free radicals by either electron or hydrogen donation (Nirmal et al., 2022).
3.2.2 Metal chelating assay
As metals are involved in reactive oxygen species (ROS) generation, metal chelation is one of the routes to inhibit ROS production. Iron is one of the major transition metals involved in ROS formation; thus, iron chelators can inhibit metal-dependent oxidation and have the possibility of mitigating disorders caused by ROS (Finefrock et al., 2003). The metal chelating capacities of both extracts (mango peel and radish peel) are presented in Table 1. The result indicated that RPE had a significantly higher metal chelating capacity compared to MP (p < 0.05). The MPE metal chelating IC50 value was calculated to be 3.86 ± 0.035 mg/ mL, while the RPE sample showed 2.39 ± 0.016 mg/mL. The results of the metal chelating abilities of MPE and RPE were contradictory to the TPC and DPPH radical scavenging activities of the respective samples. The difference in metal chelating activity could be related to the presence of different phenolic compounds having varying metal chelating capacities. Wang et al. (2010) mentioned that acylated pelargonidin derivatives, a major compound in the extract from red radish, showed metal chelation potential. Mango peel extract is rich in mangiferin, possesses potent metal-chelating properties (Safdar et al., 2017), and possibly acts as an antioxidant. This could prevent oxidative damage caused by metal-catalyzed decomposition processes (Joel et al., 2017). Besides their major active compound, crude extract samples plausibly consisted of various phenolic compounds, which might react toward metals differently, thereby influencing the particular bioactivities or mechanism of action. Stoilova et al. (2008) reported that mangiferin could chelate the Fe ions thereby inhibiting the degradation of deoxyribose and the production of hydroxyl radicals.
3.2.3 Reducing power assay
The potential antioxidant activity of a compound can also be strongly predicted by the reducing capacity of its component (Nirmal et al., 2022). The reducing abilities of the compounds are due to the presence of reductants, like ascorbic acid, which can break the chain of free radicals by donating a hydrogen atom. Some peroxide precursors react with reductants, blocking the formation of peroxide (Nirmal and Panichayupakaranant, 2015). Ferric ion-reducing capacities of MPE and RPE are presented in Table 2. The results indicated that the MPE had significantly higher reducing power than the RPE (p < 0.05). In general, increasing the concentration of phenolic compounds showed increasing reducing properties of the compounds (p < 0.05). This result agreed with the report of Ajila et al. (2007) who indicated increasing reducing power while increasing mango peel extract concentration. It was noted that RPE required a higher concentration of the sample to exhibit the reducing ability compared to the MPE (p < 0.05). The reducing powers of RPE and MPE could be co-related to the TPC of the extracts of the different varieties of mango and radish, which also serves as a significant indicator of antioxidant activity (Wang et al., 2010; Safdar et al., 2017). With regards to the solvent concentration, Safdar et al. (2017) documented that mango peel extracts obtained from 80% ethanol had the highest reducing ability (31.51 ± 0.79 mmol/100 g of extract). While recently, García-Villegas et al. (2023) reported that mango peel showed a FRAP value of 0.66 mmol FeSO4/g dry extract which was higher than the Safdar et al. (2017) report. On the other hand, Liu et al. (2017) showed that the FRAP value of eight different mango cultivars varied from 0.02–0.12 mmol FeSO4/g dry extract. The FRAP of mango peel extract was correlated with the presence of various phenolic compounds in mango peel extract. Also, Enkhtuya and Tsend (2020) reported that Fe3+ ion reduction was significantly (p < 0.05) higher in the dried unpeeled radish root compared to the dried peeled root.
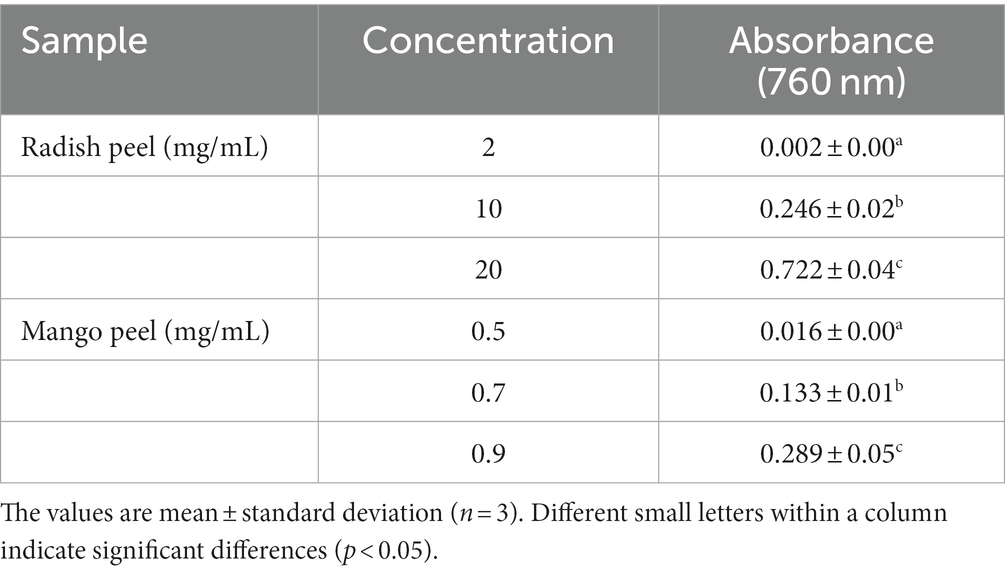
Table 2. Reducing power of the different concentrations of radish peel and mango peel extract powder.
3.2.4 Linoleic acid peroxidation
A linoleic acid peroxidation inhibition assay is usually conducted to understand the potentiality of extracts to inhibit peroxidation in lipids. Linoleic acid, a polyunsaturated fatty acid, undergoes auto-oxidation, which results in the production of peroxyl radicals. These radicals are subsequently used to oxidize ferrous ions into ferric ions, which combine with thiocyanate (SCN) ions and form a colored complex (Nguyen et al., 2023). A lower absorbance value indicates the high peroxide inhibition properties of the sample. Herein, it was noted that for a certain time exposure, MPE possesses strong lipid peroxide inhibition compared to RPE. Subsequently, the potentiality of both extracts to inhibit peroxidation in lipids declined. Lizárraga-Velázquez et al. (2018) reported that the hydrophilic extract from mango peel significantly inhibited the peroxidation of fish oil over time (p < 0.05). The capacity of mango peel extract to inhibit lipid peroxidation was examined by Dorta et al. (2013) and there were several factors affecting the aforementioned capacity. Regarding the lipid peroxidation inhibitory activity of RPE, Enkhtuya and Tsend (2020) found that black radish peel reduced the formation of oxidized intermediates in the lipid peroxidation process. The MPE at 0.1 mg/mL efficiently lowered the lipid peroxidation compared to the RPE at 2 mg/mL. Stoilova et al. (2008) indicated that mangiferin at all concentrations (0.01–0.1%) inhibited the formation of hydroperoxides at the early stage of linoleic acid oxidation, while a higher concentration (0.1%) was needed to inhibit the secondary oxidation product. Furthermore, Ajila et al. (2007) indicated that ripe mango peel extract possesses higher inhibitory action against lipid peroxidation compared to the raw peel extract which was related to the higher TPC value, carotenoid, and anthocyanin content.
3.3 Antibacterial activities
Table 3 displays the antibacterial activities of MPE and RPE against Gram-positive and Gram-negative bacteria. The MIC and MBC values of MPE showed a stronger inhibitory capacity than those of RPE toward all tested bacteria (p < 0.05). In general, Gram-positive bacteria showed higher sensitivity to natural compounds than Gram-negative bacteria due to their different cell wall compositions (Nirmal et al., 2023a). Loan et al. (2021) examined that a crude extract of mango peel waste inhibited E. coli growth with a zone of inhibition ranging from 7.7 ± 0.3 to 15.6 ± 0.5 (mm), and a dose-dependent inhibition manner was observed. Previous studies stated that mangiferin possesses excellent antibacterial activity against a wide range of Gram-positive and Gram-negative bacteria (Thambi et al., 2016; Kanatt and Chawla, 2018; Loan et al., 2021). Stoilova et al. (2005) also documented that mangiferin demonstrated antibacterial activity against Staphylococcus, Bacillus, Escherichia coli, and certain Salmonella spp. Also, Kanatt and Chawla (2018) found that mango peel extracted with 70% ethanol showed the maximum zone of inhibition against Gram-positive and Gram-negative bacteria. Radish peel, on the other hand, had a lower potential to inhibit bacterial growth. As illustrated in Table 3, the MBC of RPE to inhibit different bacteria was 5 mg/mL or higher, which was 20-fold higher than mango peel (0.25 mg/mL). It was reported that the ethanolic extracts of R. raphanistrum subsp. sativus powder possesses antimicrobial activity against specific foodborne pathogens, such as Bacillus cereus ATCC 10876, Salmonella enteritidis 110, and Staphylococcus aureus ATCC 6538. However, no inhibitory activity was observed against Escherichia coli 23,716 (Lim et al., 2019) (see Figure 1).
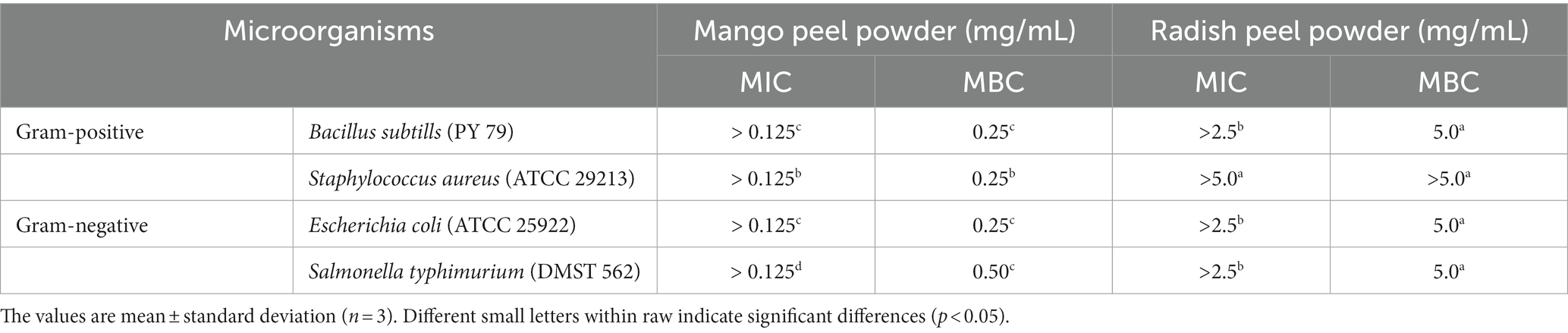
Table 3. Minimal inhibitory and minimal bactericidal concentration of mango peel and radish peel extract powder.
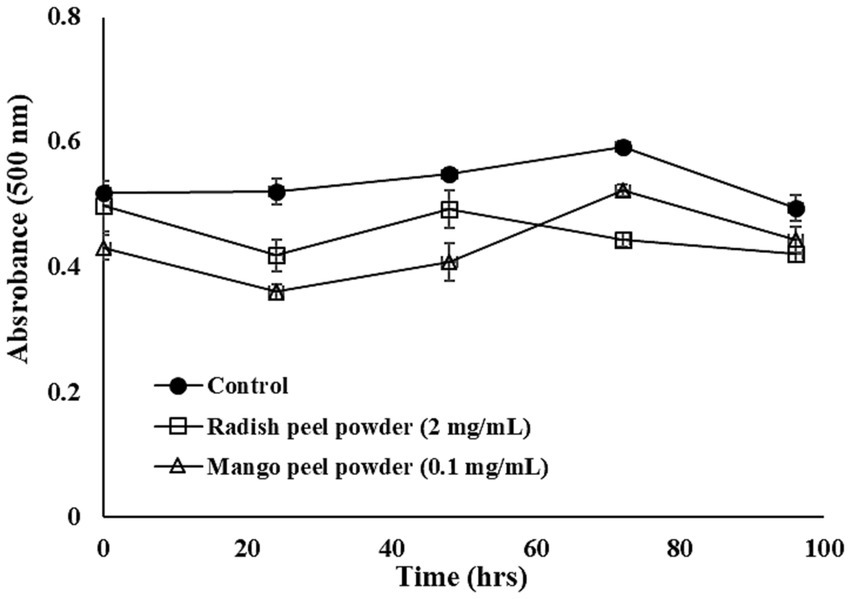
Figure 1. Linoleic acid peroxidation inhibitory activity of mango peel and radish peel extract powder. The bar represents the standard deviation (n = 3).
3.4 Phenolic profile of mango peel extract
The polyphenolic compounds in MPE, which showed higher antioxidant and antimicrobial potentials, were determined using LC-ESI-MS/MS. Three major and three minor compounds were identified in the mango peel extract as shown in Table 4. The major phenolic groups were xanthonoids, gallotannins, and flavonoids. Mangiferin, a xanthonoids, was the main phenolic, which was 82,806.97 μg/ g of mango peel extract powder, followed by 3,4-Dihydroxybenzoic acid, and gallic acid. Figure 2 represents the LC-ESI-MS/MS chromatogram of mango peel extract indicating three major peaks. Also, trace amounts of naringenin, catechin, and epicatechin gallate were identified. The identification and quantification of the phenolic profile of different Brazilian mango cultivars byproducts (barks, kernels, peel, and old and young leaves) was conducted by Barreto et al. (2008). The authors indicated that mangiferin, penta-O-galloyl-glucoside, gallic acid, and methyl gallate were the four major compounds identified in all samples. Among them, mangiferin was reported in higher concentration in all byproducts and showed higher antioxidant activities (DPPH radical scavenging, FRAP, and ORAC). Marcillo-Parra et al. (2021) investigated the presence of a phytochemical profile in MPE and reported that mangiferin ranged from 5.72 mg to 314 mg/100 g, gallic acid from 28.6 to 43.1 mg/100 g, and epicatechin from 9.01 to 9.24 mg/100 g of the dry weight of MPE. The variety, the region where the mango is grown, and the methods of extraction significantly affect the phenolic content of mango and its peel extracts (Coelho et al., 2019; Marcillo-Parra et al., 2021). The compounds identified in the present study have been recognized for their vital biological and health-beneficial properties, such as antioxidant, anti-inflammatory, anticancer capacity, antidiabetic, and antimicrobial properties (Ordoñez-Torres et al., 2021; Tacias-Pascacio et al., 2022). Therefore, the byproduct of mango, i.e., peel, has a wide range of applications in the food, pharmaceutical, and nutraceutical industries.
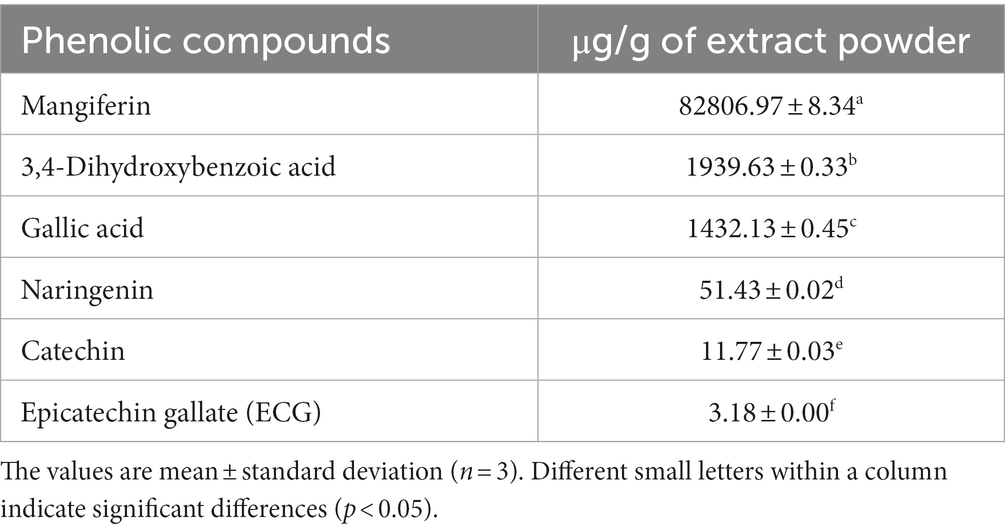
Table 4. Identification and quantification of major phenolic compounds in mango peel extract powder.
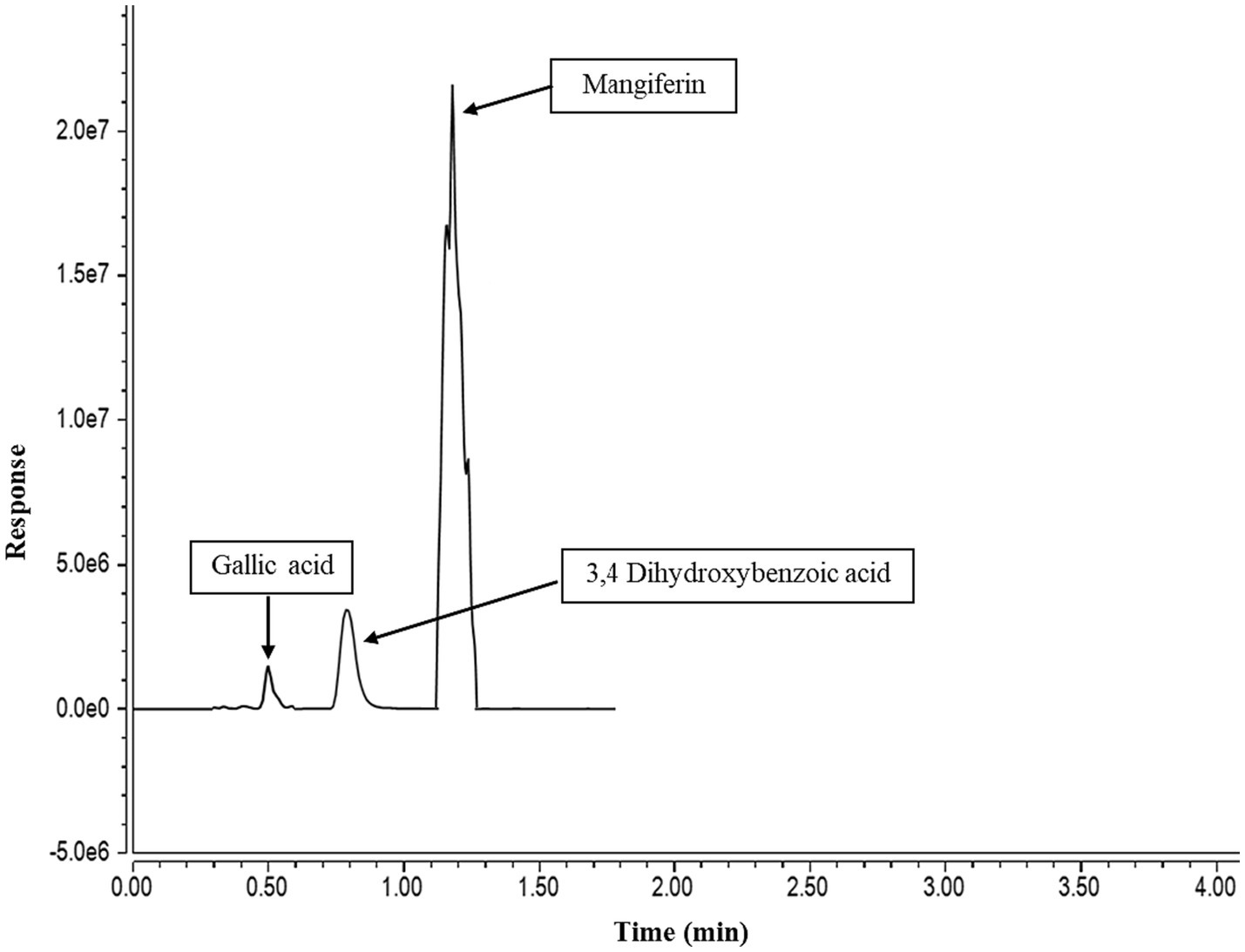
Figure 2. A liquid chromatography-electrospray ionization tandem mass spectrometry (LC-ESI-MS/MS) chromatogram of mango peel extract.
3.5 Correlations
Considering that several antioxidant mechanisms contribute to the total antioxidant activity, it is unclear whether the TPC results are responsible for the actual antioxidant capacity. Previous studies revealed a significant relationship between the total phenolic components of peel extracts and their antioxidant activities. Table 5 displayed correlation coefficient matrices between TPC in the mango peel extract and their antioxidant activities. In the mango peel samples, significant coefficients of determination were calculated between mangiferin-TPC, mangiferin-DPPH, and mangiferin-lipid peroxidation. These findings supported earlier research by Abbasi et al. (2015) which showed that phenolic compounds from mango peel play a key role in antioxidant capacity. According to our research, mango peel is one of the promising sources of phenolic compounds, which are a key source of naturally occurring antioxidants.
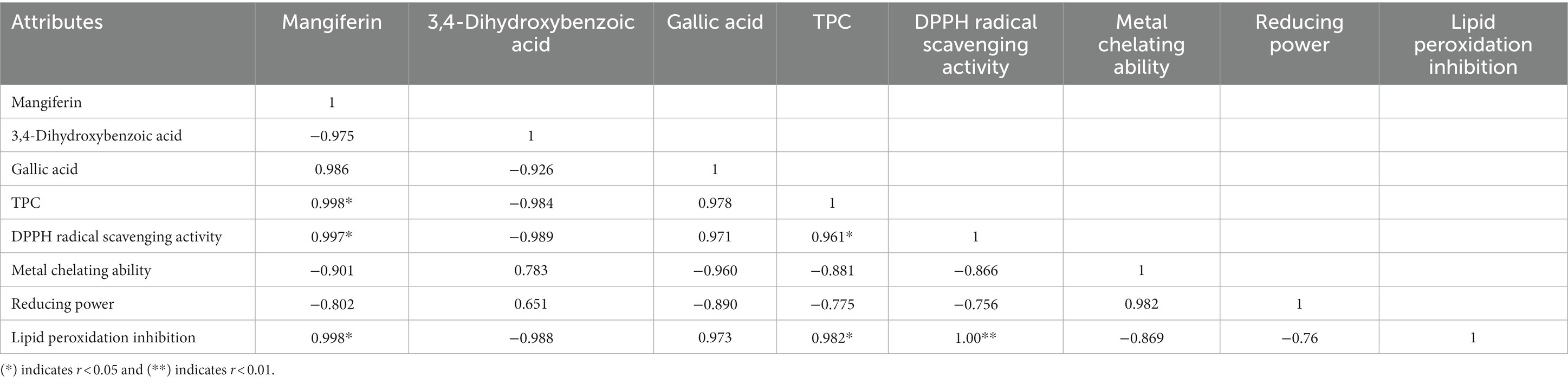
Table 5. Correlation coefficient matrix between total phenolic content and antioxidant properties in mango peel extract powder.
4 Conclusion
Mango peel and radish peel waste were extracted to obtain phenolic-rich extract. The extraction yield of radish peel was higher than that of mango peel. However, mango peel showed higher total phenolic content and antioxidant activities than radish peel (p < 0.05). Similarly, mango peel showed higher inhibitory activity against Gram-positive and Gram-negative bacteria compared to the radish peel (p < 0.05). The variation in antioxidant and antimicrobial activities of both samples could be related to the presence of active phytochemicals. Mangiferin was the major bioactive compound detected in the mango peel extract powder. TPC and phenolic profile of mango peel were very well correlated with the antioxidant activities of the extract. These results offer valuable insights into the potential use of native Thai mango peel waste as a source of phenolic compounds with stronger antioxidant and antibacterial properties.
Data availability statement
The raw data supporting the conclusions of this article will be made available by the authors, without undue reservation.
Author contributions
PK: Data curation, Formal analysis, Investigation, Methodology, Software, Writing – original draft. PC: Data curation, Formal analysis, Investigation, Software, Writing – original draft. US: Formal analysis, Investigation, Methodology, Writing – original draft. SB: Validation, Writing – review & editing. KK: Investigation, Methodology, Writing – original draft. FA-A: Validation, Visualization, Writing – review & editing. NN: Conceptualization, Funding acquisition, Methodology, Project administration, Resources, Supervision, Validation, Visualization, Writing – review & editing.
Funding
The author(s) declare financial support was received for the research, authorship, and/or publication of this article. This research project is supported by Mahidol University (grant number: MRC-IM 06/2565).
Acknowledgments
The author FA-A would like to thank the Deanship of Scientific Research, Vice Presidency for Graduate Studies and Scientific Research, King Faisal University, Saudi Arabia for research grant support (grant no. 5228).
Conflict of interest
Radish peel waste was donated by MK restaurant in Nakhon Pathom province, Thailand. Whereas Thai indigenous mango peel (Bao, Mangifera indica L. var.) waste was received from Promsap (Thailand) Company Limited, Hat Yai, Songkla, Thailand.
The authors declare that the research was conducted in the absence of any commercial or financial relationships that could be construed as a potential conflict of interest.
The author(s) declared that they were an editorial board member of Frontiers, at the time of submission. This had no impact on the peer review process and the final decision.
Publisher’s note
All claims expressed in this article are solely those of the authors and do not necessarily represent those of their affiliated organizations, or those of the publisher, the editors and the reviewers. Any product that may be evaluated in this article, or claim that may be made by its manufacturer, is not guaranteed or endorsed by the publisher.
References
Abbasi, A. M., Guo, X., Fu, X., Zhou, L., Chen, Y., Zhu, Y., et al. (2015). Comparative assessment of phenolic content and in vitro antioxidant capacity in the pulp and Peel of mango cultivars. Int. J. Mol. Sci. 16, 13507–13527. doi: 10.3390/ijms160613507
Agatonovic-Kustrin, S., Kustrin, E., and Morton, D. W. (2018). Phenolic acids contribution to antioxidant activities and comparative assessment of phenolic content in mango pulp and Peel. S. Afr. J. Bot. 116, 158–163. doi: 10.1016/j.sajb.2018.03.013
Ajila, C. M., Naidu, K. A., Bhat, S. G., and Rao, U. J. S. P. (2007). Bioactive compounds and antioxidant potential of mango Peel extract. Food Chem. 105, 982–988. doi: 10.1016/j.foodchem.2007.04.052
Barreto, J. C., Trevisan, M. T., Hull, W. E., Erben, G., De Brito, E. S., Pfundstein, B., et al. (2008). Characterization and quantitation of polyphenolic compounds in bark, kernel, leaves, and peel of mango (Mangifera indica L.). J. Agric. Food Chem. 56, 5599–5610. doi: 10.1021/jf800738r
Chupeerach, C., Temviriyanukul, P., Thangsiri, S., Inthachat, W., Sahasakul, Y., Aursalung, A., et al. (2022). Phenolic profiles and bioactivities of ten original lineage beans in Thailand. Food Secur. 11:3905. doi: 10.3390/foods11233905
Coelho, E. M., Souza MEAO, D., Corrêa, L. C., Viana, A. C., De Azevêdo, L. C., and Dos Santos Lima, M. (2019). Bioactive compounds and antioxidant activity of mango Peel liqueurs (Mangifera Indica L.) produced by different methods of maceration. Antioxidants 8:102. doi: 10.3390/antiox8040102
Dahal, M., and Koirala, P. (2020). Effect of roasting on bioactive and Antinutritional components of flaxseed (Linum usitatissimum). J. Food Nutr. Dis. 3:2.
Dorta, E., Lobo, M. G., and González, M. (2012). Using drying treatments to stabilise mango peel and seed: effect on antioxidant activity. LWT Food Sci. Technol. 45, 261–268. doi: 10.1016/j.lwt.2011.08.016
Dorta, E., Lobo, M. G., and González, M. (2013). Improving the efficiency of antioxidant extraction from mango Peel by using microwave-assisted extraction. Plant Foods Hum. Nutr. 68, 190–199. doi: 10.1007/s11130-013-0350-4
Enkhtuya, E., and Tsend, M. (2020). The effect of peeling on the antioxidant capacity of black radish root. Italian. J. Food Sci. 32, 701–711.
Finefrock, A. E., Bush, A. I., and Doraiswamy, P. M. (2003). Current status of metals as therapeutic targets in Alzheimer's disease. J. Am. Geriatr. Soc. 51, 1143–1148. doi: 10.1046/j.1532-5415.2003.51368.x
García-Villegas, A., Fernández-Ochoa, Á., Rojas-García, A., Alañón, M. E., Arráez-Román, D., Cádiz-Gurrea, M. D. L. L., et al. (2023). The potential of Mangifera indica L. Peel extract to be revalued in cosmetic applications. Antioxidants (Basel) 12:1892. doi: 10.3390/antiox12101892
Htoo, H. H., Brumage, L., Chaikeeratisak, V., Tsunemoto, H., Sugie, J., Tribuddharat, C., et al. (2019). Bacterial cytological profiling as a tool to study mechanisms of action of antibiotics that are active against Acinetobacter baumannii. Antimicrob. Agents Chemother. 63:e02310. doi: 10.1128/aac.02310-18
Janjua, S., and Shahid, M. (2013). Phytochemical analysis and in vitro antibacterial activity of root Peel extract of Raphanus Sativus L. Var. Niger. Adv. Med. Plant Res. 1, 1–7.
Jayaprakasha, G. K., Singh, R., and Sakariah, K. (2001). Antioxidant activity of grape seed (Vitis Vinifera) extracts on peroxidation models in vitro. Food Chem. 73, 285–290. doi: 10.1016/S0308-8146(00)00298-3
Joel, J., Sheena, O., Martins, O., Onyemauche, N., and Emmanuel, A. (2017). Comparative antioxidant capacity of aqueous and ethanol fruit extracts of Tetrapleura Tetraptera. J. Biol. Sci. 17, 185–193. doi: 10.3923/jbs.2017.185.193
Kanatt, S. R., and Chawla, S. (2018). Shelf life extension of chicken packed in active film developed with mango Peel extract. J. Food Saf. 38:e12385. doi: 10.1111/jfs.12385
Kaur, P., Ghoshal, G., and Jain, A. (2019). Bio-utilization of fruits and vegetable waste to produce β-carotene in solid-state fermentation: characterization and antioxidant activity. Process Biochem. 76, 155–164. doi: 10.1016/j.procbio.2018.10.007
Kumar, K., Yadav, A. N., Kumar, V., Vyas, P., and Dhaliwal, H. S. (2017). Food waste: a potential bioresource for the extraction of nutraceuticals and bioactive compounds. Bioresour. Bioprocess. 4:18. doi: 10.1186/s40643-017-0148-6
Leong, Y. K., and Chang, J.-S. (2022). Valorization of fruit wastes for circular bioeconomy: current advances, challenges, and opportunities. Bioresour. Technol. 359:127459. doi: 10.1016/j.biortech.2022.127459
Lim, H.-W., Song, K.-Y., Chon, J.-W., Jeong, D., and Seo, K.-H. (2019). Antimicrobial action of Raphanus Raphanistrum Subsp. Sativus (radish) extracts against foodborne Bacteria present in various Milk products: a preliminary study. J. Dairy Sci. Biotechnol. 37, 187–195. doi: 10.22424/jmsb.2019.37.3.187
Liu, Y.-G., Zhang, X.-M., Ma, F.-Y., and Fu, Q. (2017). The antioxidant activitives of mango peel among different cultivars. IOP Conf. Ser. Earth Environ. Sci. 61:012065.
Lizárraga-Velázquez, C. E., Hernández, C., González-Aguilar, G. A., and Heredia, J. B. (2018). Effect of hydrophilic and lipophilic antioxidants from mango Peel (Mangifera Indica L. cv. Ataulfo) On lipid peroxidation in fish oil. CyTA J. Food 16, 1095–1101. doi: 10.1080/19476337.2018.1513425
Loan, N. T. T., Long, D. T., Yen, P. N. D., Hanh, T. T. M., Pham, T. N., and Pham, D. T. N. (2021). Purification process of Mangiferin from Mangifera Indica L. leaves and evaluation of its bioactivities. Processes 9:852. doi: 10.3390/pr9050852
Lugasi, A., Blázovics, A., Hagymási, K., Kocsis, I., and Kéry, Á. (2005). Antioxidant effect of squeezed juice from black radish (Raphanus Sativus L. Var Niger) in alimentary Hyperlipidaemia in rats. Phytother. Res. 19, 587–591. doi: 10.1002/ptr.1655
Manhongo, T. T., Chimphango, A., Thornley, P., and Röder, M. (2021). An economic viability and environmental impact assessment of mango processing waste-based biorefineries for co-producing bioenergy and bioactive compounds. Renew. Sust. Energ. Rev. 148:111216. doi: 10.1016/j.rser.2021.111216
Maqbool, H., Visnuvinayagam, S., Zynudheen, A., Safeena, M., and Sathish Kumar, K. (2020). Antibacterial activity of beetroot Peel and whole radish extract by modified well diffusion assay. Int. J. Curr. Microbiol. App. Sci. 9, 1222–1231. doi: 10.20546/ijcmas.2020.901.135
Marcillo-Parra, V., Anaguano, M., Molina, M., Tupuna-Yerovi, D. S., and Ruales, J. (2021). Characterization and quantification of bioactive compounds and antioxidant activity in three different varieties of mango (Mangifera Indica L.) Peel from the Ecuadorian region using HPLC-UV/VIS and UPLC-PDA. NFS Journal 23, 1–7. doi: 10.1016/j.nfs.2021.02.001
Martínez-Ramos, T., Benedito-Fort, J., Watson, N. J., Ruiz-López, I. I., Che-Galicia, G., and Corona-Jiménez, E. (2020). Effect of solvent composition and its interaction with ultrasonic energy on the ultrasound-assisted extraction of phenolic compounds from mango peels (Mangifera Indica L.). Food Bioprod. Process. 122, 41–54. doi: 10.1016/j.fbp.2020.03.011
Nation, T. (2021). "Agriculture GDP in first quarter grows 1.4% by the Nation ". (Thailand: Nation group).
Nguyen, C. N. M., Nirmal, N. P., Sultanbawa, Y., and Ziora, Z. M. (2023). Antioxidant and antibacterial activity of four tannins isolated from different sources and their effect on the shelf-life extension of vacuum-packed minced meat. Food Secur. 12:354. doi: 10.3390/foods12020354
Nirmal, N. P., and Benjakul, S. (2011). Use of tea extracts for inhibition of Polyphenoloxidase and retardation of quality loss of Pacific white shrimp during iced storage. LWT Food Sci. Technol. 44, 924–932. doi: 10.1016/j.lwt.2010.12.007
Nirmal, N. P., Chunhavacharatorn, P., Chandra Khanashyam, A., Li, L., and Al-Asmari, F. (2023a). Cinnamon bark oil in water Nanoemulsion formulation, characterization, and antimicrobial activities. LWT 179:114671. doi: 10.1016/j.lwt.2023.114671
Nirmal, N. P., Khanashyam, A. C., Mundanat, A. S., Shah, K., Babu, K. S., Thorakkattu, P., et al. (2023b). Valorization of fruit waste for bioactive compounds and their applications in the food industry. Food Secur. 12:556. doi: 10.3390/foods12030556
Nirmal, N. P., Mereddy, R., Webber, D., and Sultanbawa, Y. (2021). Biochemical, antioxidant, and sensory evaluation of Davidsonia Pruriens and Davidsoina Jerseyana fruit infusion. Food Chem. 342:128349. doi: 10.1016/j.foodchem.2020.128349
Nirmal, N. P., Mereddy, R., Webber, D., and Sultanbawa, Y. (2022). Biochemical composition and aroma of Melaleuca Citrolens Barlow (Myrtaceae) leaves from different regions of the Australian Northern Territory. S. Afr. J. Bot. 145, 78–84. doi: 10.1016/j.sajb.2022.01.037
Nirmal, N. P., and Panichayupakaranant, P. (2015). Antioxidant, antibacterial, and anti-inflammatory activities of standardized brazilin-rich Caesalpinia Sappan extract. Pharm. Biol. 53, 1339–1343. doi: 10.3109/13880209.2014.982295
Nirmal, N. P., Rajput, M. S., Prasad, R. G. S. V., and Ahmad, M. (2015). Brazilin from Caesalpinia Sappan heartwood and its pharmacological activities: a review. Asian Pac J Trop Med 8, 421–430. doi: 10.1016/j.apjtm.2015.05.014
Noman, O. M., Nasr, F. A., Alqahtani, A. S., al-zharani, M., Cordero, M. A. W., Alotaibi, A. A., et al. (2021). Comparative study of antioxidant and anticancer activities and HPTLC quantification of Rutin in white radish (Raphanus Sativus L.) leaves and root extracts grown in Saudi Arabia. Open Chem. 19, 408–416. doi: 10.1515/chem-2021-0042
Noor, S. A. A., Siti, N. M., and Mahmad, N. J. (2015). Chemical composition, antioxidant activity and functional properties of mango (Mangifera indica L. var Perlis sunshine) Peel flour (MPF). Appl. Mech. Mater. 754-755, 1065–1070. doi: 10.4028/www.scientific.net/AMM.754-755.1065
OAE , O.O.a.E. (2019). "Agricultural statistics of Thailand-2019 ". (Thailand: Office of Agricultural Economics).
Okino-Delgado, C. H., Prado, D. Z., Pereira, M. S., Camargo, D. A., Koike, M. A., and Fleuri, L. F. (2020). “Chapter 8—mango” in Valorization of fruit processing by-products. ed. C. M. Galanakis (Cambridge, Massachusetts, United States: Academic Press), 167–181.
Ordoñez-Torres, A., Torres-León, C., Hernández-Almanza, A., Flores-Guía, T., Luque-Contreras, D., Aguilar, C. N., et al. (2021). Ultrasound-microwave-assisted extraction of polyphenolic compounds from Mexican “Ataulfo” mango peels: antioxidant potential and identification by HPLC/ESI/MS. Phytochem. Anal. 32, 495–502. doi: 10.1002/pca.2997
Rajput, M. S., Nirmal, N. P., Nirmal, S. J., and Santivarangkna, C. (2022). Bio-actives from Caesalpinia Sappan L.: recent advancements in Phytochemistry and pharmacology. S. Afr. J. Bot. 151, 60–74. doi: 10.1016/j.sajb.2021.11.021
Rathod, N. B., Nirmal, N. P., Abdullah, S., Surasani, V. K. R., Ranveer, R. C., Kumar, S., et al. (2023). Extraction of natural bioactive compounds using clean label technologies and their application as muscle food preservatives. Front. Sustain. Food Syst. 7:1207704. doi: 10.3389/fsufs.2023.1207704
Safdar, M. N., Kausar, T., and Nadeem, M. (2017). Comparison of ultrasound and maceration techniques for the extraction of polyphenols from the mango Peel. J. Food Proc. Preserv. 41:e13028. doi: 10.1111/jfpp.13028
Sipos, P., Hagymási, K., Lugasi, A., Fehér, E., and Blázovics, A. (2002). Effects of black radish root (Raphanus Sativus L. Var. Niger) on Colon mucosa in rats fed a fat rich diet. Phytother. Res. 16, 677–679. doi: 10.1002/ptr.950
Sirichai, P., Kittibunchakul, S., Thangsiri, S., On-Nom, N., Chupeerach, C., Temviriyanukul, P., et al. (2022). Impact of drying processes on Phenolics and in vitro health-related activities of indigenous plants in Thailand. Plan. Theory 11:294. doi: 10.3390/plants11030294
Stoilova, I., Gargova, S., Stoyanova, A., and Ho, I. (2005). Antimicrobial and antioxidant activity of the polyphenol Mangiferin. Herba Polonica 1, 37–44.
Stoilova, I., Jirovetz, L., Stoyanova, A., Krastanov, A., Gargova, S., and Ho, L. (2008). Antioxidant activity of the polyphenol mangiferin. EJEAFChe 12, 2706–2716.
Tacias-Pascacio, V. G., Castañeda-Valbuena, D., Fernandez-Lafuente, R., Berenguer-Murcia, Á., Meza-Gordillo, R., Gutiérrez, L.-F., et al. (2022). Phenolic compounds in mango fruit: a review. J. Food Meas. Charact. 16, 619–636. doi: 10.1007/s11694-021-01192-2
Thakare, V. B., Jadeja, G. C., and Desai, M. A. (2023). Extraction of Mangiferin and pectin from mango peels using process-intensified tactics: a step towards waste valorization. Chem. Eng. Res. Des. 192, 280–288. doi: 10.1016/j.cherd.2023.02.036
Thambi, P. A., John, S., Lydia, E., Iyer, P., and Monica, S. (2016). Antimicrobial efficacy of mango Peel powder and formulation of recipes using mango Peel powder (Mangifera Indica L.). Int. J. Home Sci. 2, 155–161.
Wang, L.-S., Sun, X.-D., Cao, Y., Wang, L., Li, F.-J., and Wang, Y.-F. (2010). Antioxidant and pro-oxidant properties of Acylated Pelargonidin derivatives extracted from red radish (Raphanus Sativus Var. Niger, Brassicaceae). Food Chem. Toxicol. 48, 2712–2718. doi: 10.1016/j.fct.2010.06.045
Keywords: recycling, sustainability, waste utilization, antioxidant, antimicrobial
Citation: Koirala P, Chunhavacharatorn P, Suttisansanee U, Benjakul S, Katewongsa K, Al-Asmari F and Nirmal N (2024) Antioxidant and antimicrobial activities of mango peel and radish peel-a comparative investigation. Front. Sustain. Food Syst. 8:1354393. doi: 10.3389/fsufs.2024.1354393
Edited by:
Lourdes Maria Correa Cabral, Brazilian Agricultural Research Corporation (EMBRAPA), BrazilReviewed by:
Rodolfo Ramos-González, National Council of Science and Technology (CONACYT), MexicoMaria Simona Chiş, University of Agricultural Sciences and Veterinary Medicine of Cluj-Napoca, Romania
Copyright © 2024 Koirala, Chunhavacharatorn, Suttisansanee, Benjakul, Katewongsa, Al-Asmari and Nirmal. This is an open-access article distributed under the terms of the Creative Commons Attribution License (CC BY). The use, distribution or reproduction in other forums is permitted, provided the original author(s) and the copyright owner(s) are credited and that the original publication in this journal is cited, in accordance with accepted academic practice. No use, distribution or reproduction is permitted which does not comply with these terms.
*Correspondence: Nilesh Nirmal, bmlsZXNoLm5pckBtYWhpZG9sLmFjLnRo