- 1Hubei Key Laboratory of Edible Wild Plants Conservation and Utilization, Hubei Normal University, Huangshi, China
- 2Hubei Engineering Research Center of Special Wild Vegetables Breeding and Comprehensive Utilization Technology, Hubei Normal University, Huangshi, China
Chromate [Cr(VI)] is a highly toxic heavy metal element, representing one of the most prevalent sources of wastewater contamination. It poses a significant threat to human health and food safety. Therefore, effective treatment before discharging wastewater is of paramount importance. In this study, termite nests (Coptotermes formosanus and Odontotermes formosanus), as natural biomass materials, were used to adsorb Cr(VI) ions in wastewater as a strategy to reduce environmental pollution and minimize poisoning by Cr. Structural and morphological characterizations were performed using scanning electron microscope (SEM), Fourier Transform Infrared Spectroscopy (FT-IR) and X-ray photoelectron spectroscopy (XPS) analyses. Batch adsorption experiments were conducted to evaluate the influence of termite nest dosage, coexisting ions, and pH. To gain further insight, detailed studies on adsorption kinetics, adsorption isotherms, and adsorption thermodynamics were undertaken. The results indicate that under acidic pH conditions, both termite nests exhibit the highest adsorption capacity for Cr(VI), with an optimal adsorbent dosage of 10 g/L. The maximum adsorption capacities of C. formosanus nest and O. formosanus nest for Cr(VI) were found to be 48.52 mg/g and 35.99 mg/g, respectively. Thermodynamic studies confirmed the spontaneous and endothermic nature of the adsorption process. In the rapeseed cultivation experiment, the growth status of Brassica chinensis L. post-adsorption treatment was markedly improved compared to the untreated group. Additionally, the concentration of Cr(VI) in the plants was significantly reduced. This demonstrates both the inhibitory effect of Cr(VI) on the growth of oilseed rape and the effectiveness of water remediation techniques. In addition, both types of termite nests can be effectively reused by 0.1 mol/L HCl. To the best of our knowledge, this is the first report of adsorption removal of Cr(VI) by C. formosanus nest and O. formosanus nest. Compared to traditional natural biomass adsorbents, termite nests exhibit a relatively higher adsorption capacity for Cr(VI). The results of this study demonstrate that subterranean termite nests can efficiently remove Cr(VI) from wastewater, offering the potential for a cost-effective and reusable bioremediation agent with the advantages of ease of operation.
1 Introduction
Water, crucial for human life, health, and agriculture, is a fundamental resource. Due to the rapid expansion of industrial production, water resources are facing severe threats, with particular attention being drawn to the issue of heavy metal pollution. Chromium is an element belonging to Group VIB of the periodic table, and it exists primarily in two stable forms: trivalent chromium [Cr(III)] and hexavalent chromium [Cr(VI)] (Sharma et al., 2022). It is commonly generated during industrial processes such as electroplating, leather manufacturing, wood preservativation, and steel production (Singh et al., 2022). The toxicity and mutagenicity of Cr(VI) are, 100 and 1,000 times greater than that of Cr(III), respectively (Benimeli et al., 2011). When irrigating farmland with chromium-contaminated water, chromium accumulates within the plant tissues. Furthermore, the breakdown of organic matter in the soil is inhibited, resulting in reduced agricultural yields (Hayat et al., 2012). The ingestion of hexavalent chromium by the human body can potentially lead to acute and chronic health issues, including disruptions in the digestive system, lung damage, carcinogenicity, and genetic toxicity (den Braver-Sewradj et al., 2021). Therefore, the removal of hexavalent chromium contamination is an urgent task in safeguarding food security and public health.
Different methods for the removal of heavy metals from aqueous solutions have been proposed (Qin et al., 2020). These methods include solvent extraction, ion exchange filtration, membrane separation, reverse osmosis, chemical precipitation, and coagulation. However, each of these methods has its drawbacks, including incomplete removal of metals, high energy consumption, costly reagents, disposal of large volumes of organic solvents, and inefficiency at metal concentrations below 10 mg/L (Amer et al., 2010). Consequently, the adsorption process is emerging as a viable alternative, devoid of the limitations associated with the previously mentioned techniques. Present research concentrates on adsorbents originating from natural or modified materials, alongside synthetic sources. These adsorbents are comprised of bacteria, agricultural products, and other nonliving biomass such as chitosan and clays (Loukidou et al., 2004; Baran et al., 2007). Compared to synthetic adsorbents, natural materials offer high efficiency and low operating costs, making them an economical alternative that can help minimize pollutant inputs to the environment. In natural ecosystems, termite nests constitute a widely prevalent yet underexploited biomass resource. However, research into the utilization of termite nests as biomass adsorbents remains in its nascent phase. Currently, there are no research reports on the use of C. formosanus nest and O. formosanus nest as biomass adsorbents for treating aqueous media containing Cr(VI). Exploring this area may provide new perspectives and methods for bioremediation of heavy metal pollution.
Termites are eusocial insects (Isoptera: Termitidae), and constitute 10% of all animal biomass (Van Huis, 2017), with approximately 3,105 species found worldwide (Lertlumnaphakul et al., 2022). Around 185 species have been identified as pests, posing a significant threat to crops and buildings, especially in tropical and subtropical regions (Verma et al., 2009). Termites possess the ability to break down cellulose, which is one of the primary components of wood (Ahmad et al., 2021a). It is estimated that each year termites cause a noteworthy economic loss of more than 40 billion USD worldwide (Subekti et al., 2015). Termites damage the agricultural and horticultural crops directly (through feeding on the bark and underlying tissue) and indirectly (the damaged plants become susceptible to pathogenic microbes) resulting in plant death (Paul et al., 2018). Infestations start from the root and then spreads to the whole plant. Furthermore, certain species of termites, such as O. formosanus, constitute a significant threat to the structural integrity of dams (Tian et al., 2009). Developing scientific methods for the prevention, control, and utilization of these hazardous termites represents a significant research challenge.
The management of termites using physical and mechanical means is a common practice worldwide to keep the population at a certain level (Ahmad et al., 2021b). According to the current Chinese local “Technical code of practice for prevention and control of termite in forest” (DB32/T 4588-2023) and “Technical specification for termite prevention and control in water conservancy projects” (DB42/T 768-2011), the nest-digging method involves digging out the termites from the nest, removing the termite kings and queens, and simultaneously killing the living organisms in the termite nest. The methods for termite control primarily involve nest excavation and the use of synthetic chemical agents, which often result in the generation of substantial amounts of discarded termite nests and additional environmental impacts. If these abandoned termite nests can be effectively utilized, it would not only achieve the reuse of resources but also help to reduce the use of synthetic chemical reagents, playing a positive role in environmental protection and resource recycling. Termite nests typically consist of cellulose components, saliva, excreta, and soil. The composition and unique structure make them potentially suitable as biomass adsorbents (Araújo et al., 2013), modified termite nests for the adsorption of cadmium ions, lead ions, and organic compounds (Cunha et al., 2018), and termite mounds for adsorbing lead and zinc ions, among others (Abdus-Salam and Bello, 2015). However, the exploration of termite nest biomass as an adsorbent material remains under-researched.
Therefore, this study proposes the use of termite nests from two common subterranean termite species (C. formosanus and O. formosanus) for remediating water contaminated with Cr(VI). The research involved the chemical characterization of this novel adsorbent and investigated its mechanism for Cr(VI) removal. This method transforms waste biomass into valuable components, giving new purpose to these bio-wastes while addressing the challenges of their safe disposal. This study aims to provide a novel, sustainable solution for overcoming heavy metal pollution issues while promoting the integrated development of environmental protection, resource recycling, and public health.
2 Materials and methods
2.1 Materials and chemicals
In this study, nest samples of the C. formosanus nests were collected from Nanning, Guangxi, while samples of the O. formosanus nests were obtained from Huangshi, Hubei. The termite nests were washed with deionized water, air-dried at room temperature (25 ± 2°C), and sieved through a 60-mesh screen (Araújo et al., 2013). The resulting material was used in the adsorption experiments.
Potassium dichromate (K2Cr2O7), sulfuric acid (H2SO4), hydrochloric acid (HCl), phosphoric acid (H3PO4), sodium hydroxide (NaOH), and diphenylcarbazide (C13H10N2O2) were all of the analytical grade and supplied by Sinopharm Chemical Reagent Co., Ltd. (Shanghai, China). In this research, the pH values of all solutions were measured using a pH meter (PHSJ-4F, Leici Shanghai) and adjusted with sodium hydroxide (NaOH) and hydrochloric acid (HCl) as needed. All solutions were prepared with deionized (DI) water.
2.2 Adsorption experiments
To investigate the adsorptive properties of termite nest material from C. formosanus and O. formosanus, all batch experiments were conducted in Erlenmeyer flasks maintained at a controlled temperature of 25 ± 2°C (Jing et al., 2021). In each flask, a designated amount of termite nest material was combined with a fixed concentration of Cr(VI) solution and agitated on a shaker at a speed of 150 rpm. The primary variables adjusted included the concentration of Cr(VI) affecting adsorption (100–1,000 mg/L), pH value (2–12), termite nest dosage (1–12 g/L), contact time (5–2,160 min), and coexisting ions (Cl−, NO, SO, PO). After the predetermined adsorption period, the aqueous solution was filtered through a membrane with a pore size of 0.45 um. Subsequently, a 5 mL sample of the filtered aqueous solution was taken for analysis. The initial and final concentrations of Cr(VI) were determined by the colorimetric diphenylcarbazide (DPC) method (Xia et al., 2018a,b). Each step of the experiment was repeated thrice for accuracy. The amount of Cr(VI) adsorbed by the termite nest material was calculated according to Equation (1):
where qt is the adsorbed capacity after time t, C0 and Ct are the initial and residual concentrations after time t, respectively, V is the volume of the solution, and m is the dry weight of the adsorbent.
2.3 Adsorption kinetics and adsorption isotherms experiments
The investigation of adsorption kinetics is critical as it characterizes the solute uptake rate, predicts the rate-determining steps, and elucidates the adsorption mechanism. For the adsorption kinetics analysis, C. formosanus and O. formosanus nests (each at 4 g/L) were incubated with Cr(VI) solutions at varying concentrations (50, 75, and 100 mg/L) under optimal pH conditions at 298 K for 24 h. Samples were collected at predetermined intervals. The quantification of residual Cr(VI) ions was conducted by Method 2.2.
Adsorbent capacity is determined through equilibrium studies. The Freundlich, Langmuir, and Temkin isotherms represent the simplest known models to describe the adsorption isotherms. In the analysis of adsorption isotherms, C. formosanus and O. formosanus nests (each at 4 g/L) were incubated with Cr(VI) solutions at various concentrations under optimal pH conditions and temperatures of 298, 308, and 318 K for 24 h. The concentration of residual Cr(VI) was quantified using the aforementioned method.
2.4 Characterization of the termite nest
Surface morphology was identified by a scanning electron microscope (SEM: HITACHI, Japan). The specific surface area (SBET), total pore volume (Vtot), and average pore size (Dp) were studied using a gas adsorption analyzer (Micromeritics, USA). All pH measurements were performed with a pH meter (INESA, China). The pH at the point of zero charges (pHpzc) was determined using the method described by Li et al. (2021). The functional groups of the material were studied using Fourier transform infrared spectroscopy (FT-IR: Thermo, USA). The surface elements of the adsorbent were analyzed qualitatively and quantitatively using X-ray photoelectron spectroscopy (XPS: Kratos, UK).
2.5 Desorption experiments
In the desorption study, 50 mg/L Cr(VI) solutions were adsorbed under optimized conditions using 4 g/L of both C. formosanus nests and O. formosanus nests. Subsequently, samples were collected through centrifugation at a speed of 12,000 rpm for 10 min. The samples were then resuspended and the adsorbents were regenerated using HCl (0.1 mol/L) (Xia et al., 2018a,b). The efficiency of desorption was calculated using the following equation:
Five consecutive adsorption/desorption cycles were performed to test the reusability of the adsorbents from C. formosanus and O. formosanus nests. The removal efficiency for each cycle was calculated as follows:
3 Results and discussion
3.1 Characterization of the termite nest
The porous structures of the C. formosanus nest and the O. formosanus nest are presented in Table 1. Both types of termite nests possess a considerable specific surface area, total pore volume, and average pore diameter. This can likely be attributed to the similarity in the primary components of the two nests, which both predominantly contain lignocellulosic materials. Notably, the C. formosanus nest exhibits greater porosity and accessible surface area, which may enhance its adsorption capacity for Cr(VI) (Song et al., 2016).
The surface morphology of the termite nest before and after the adsorption of Cr(VI) was examined using a scanning electron microscope (SEM). SEM images of the nests of C. formosanus and O. formosanus before the adsorption of Cr(VI) depicted a rough surface; the irregular texture and varying porosity within the termite nests provided high surface area and suitable sites for the adsorption of Cr(VI) (see Figures 1A, C). Following the adsorption of Cr(VI), a significant area of the termite nests was covered by adsorbed molecules. Post-adsorption SEM images of both types of termite nests displayed surfaces smoother than before, indicative of the trapping and adsorption of heavy metal ions on their surfaces (see Figures 1B, D) (Liu et al., 2020).
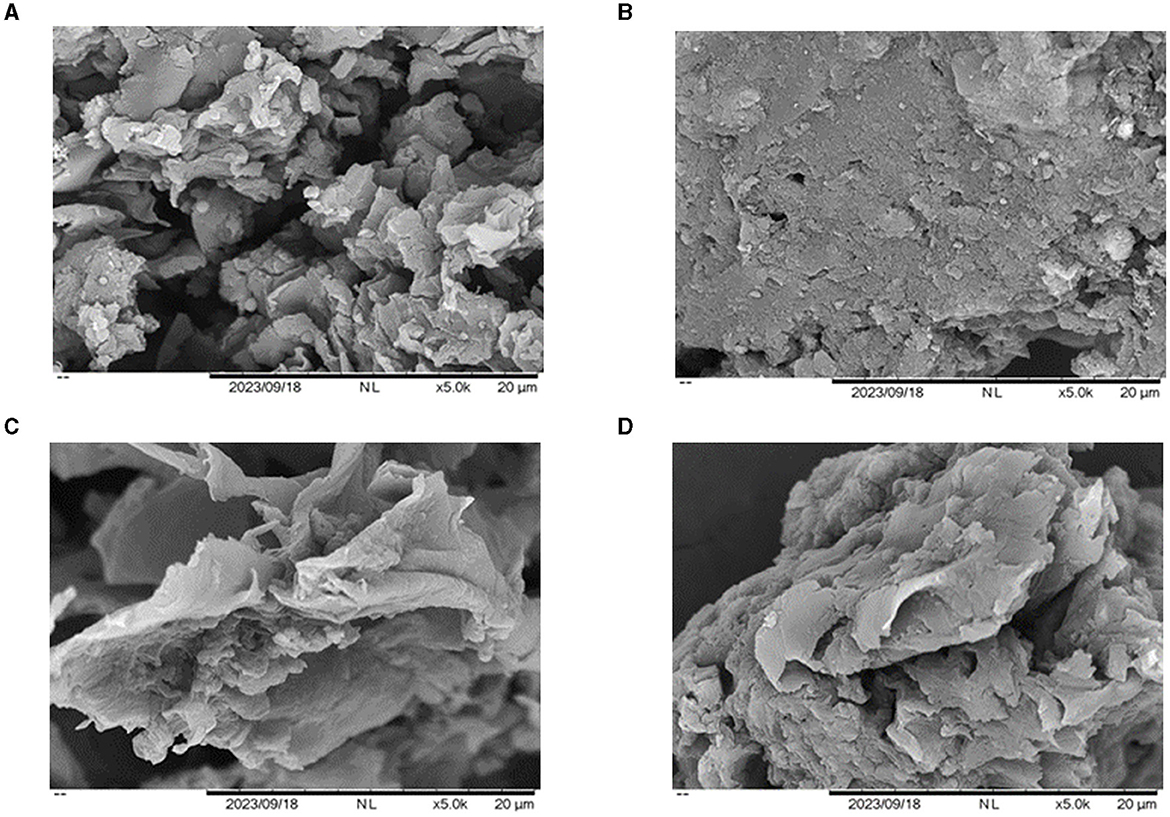
Figure 1. SEM micrographs of the termite nest adsorbent (×5,000). (A) C. formosanus nest before adsorption, (B) C. formosanus nest after Cr(VI) adsorption, (C) O. formosanus nest before adsorption, (D) O. formosanus nest after Cr(VI) adsorption.
The mode of the bonding in metal compounds can be determined by the infrared spectra. Comparative analysis of the infrared spectra of nests from C. formosanus and O. formosanus reveals that the primary absorption bands are fundamentally identical, with notable absorption peaks occurring near wavelengths of 3,400, 2,900, 1,400, and 1,050 cm−1 (see Figure 2A). However, certain variations in peak shapes and absorption intensities were observed. These suggested that while the samples share similar structures and functional groups, their relative concentrations vary. Examining the infrared spectra of both termite nests before and after adsorption shows slight shifts in the intensity and position of these absorption peaks, indicating the potential involvement of these functional groups in the adsorption process (Jing et al., 2021). The broad absorption peak between 3,500 and 3,200 cm−1, attributable to the stretching vibrations of phenolic hydroxyl groups, alters slightly in intensity and position post-adsorption, likely due to metal ions complexing with hydroxyl groups on the termite nest surfaces through surface adsorption (Meng et al., 2014). Absorption peaks near 1,750, 1,450, and 2,900 cm−1, originating from hydroxyl O-H or C-H stretching vibrations, indicate the presence of numerous hydroxyl and other oxygen-containing functional groups in the termite nests, conducive to ion exchange reactions (Mansour et al., 2020). A noticeable decrease in the intensity of these peaks post-adsorption suggests ion exchange between the termite nest and metal ions. The ion exchange between metal and mineral ions facilitates electrostatic outer-sphere complexation and co-precipitation/inner-sphere complexation (Qiu et al., 2008). The strong absorption peaks between 1,100 and 1,000 cm−1, associated with C-O bond stretching vibrations, change in intensity after heavy metal adsorption, indicating the involvement of ether-type oxygen-containing groups in the adsorption process (Shukla and Vankar, 2012). In conclusion, the positions of the absorption peaks of hydroxyl, carboxyl, and C-O oxygen-containing functional groups in both termite nests changed significantly after the adsorption of heavy metals, indicating that the adsorption process in the termite nests was primarily due to the complexation mechanism between Cr(VI) ions and these functional groups.
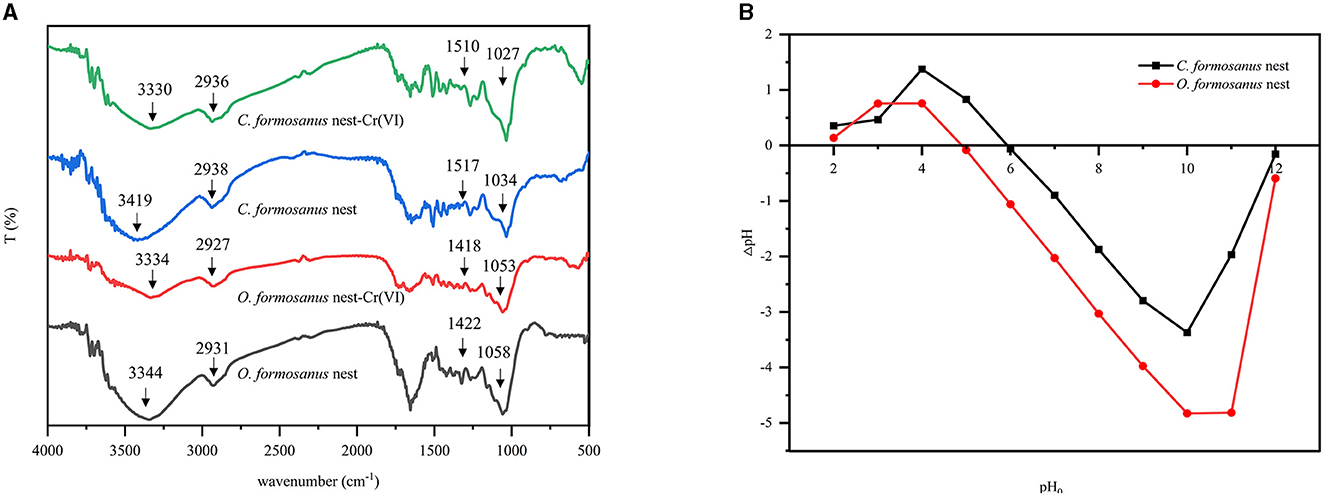
Figure 2. FTIR spectra and zero-charge points of termite nest. (A) FTIR spectra of fresh termite nest and Cr(VI) ions loaded termite nest, (B) determination of the point of zero charge of the termite nest.
The isoelectric points of C. formosanus nest and O. formosanus nest are shown in Figure 2B. From the graph, the isoelectric points of C. formosanus nest and O. formosanus nest are 5.93 and 4.90, respectively. This indicates that the surfaces of both termite nests have a relatively higher abundance of acidic functional groups. When the solution pH is below the isoelectric point, the adsorbent surfaces carry a positive charge, which is favorable for the electrostatic attraction-based adsorption of anions as the predominant mechanism. On the other hand, when the pH is above the isoelectric point, the adsorbent surfaces become negatively charged, facilitating the electrostatic attraction-based adsorption of cations as the predominant mechanism (Araújo et al., 2013).
The study employed X-ray Photoelectron Spectroscopy (XPS) to observe changes in the binding energy and valence states of elements within two types of termite nests before and after adsorption, as depicted in Figure 3. Compared to the pre-adsorption state, the XPS spectra of the termite nests post-adsorption of Cr(VI) revealed a new binding energy peak region, Cr2p, confirming the adsorption of Cr(VI) onto the surfaces of the termite nests (see Figure 3A). In the post-adsorption Cr2p spectra of both termite nests, a binding energy of 579 eV corresponding to Cr(VI) was observed, along with a weaker binding energy at 575 eV, indicative of a minor presence of Cr(III) (Shi et al., 2018). This observation suggests a partial reduction of Cr(VI) to Cr(III) during adsorption, likely facilitated by the interaction with functional groups on the termite nest surface (see Figure 3B). This reduction process is possibly attributable to the presence of hydroxyl and carbonyl groups in the adsorbent. Under acidic conditions, these groups can act as electron donors, thereby reducing Cr(VI) to its trivalent form (Suksabye et al., 2009).
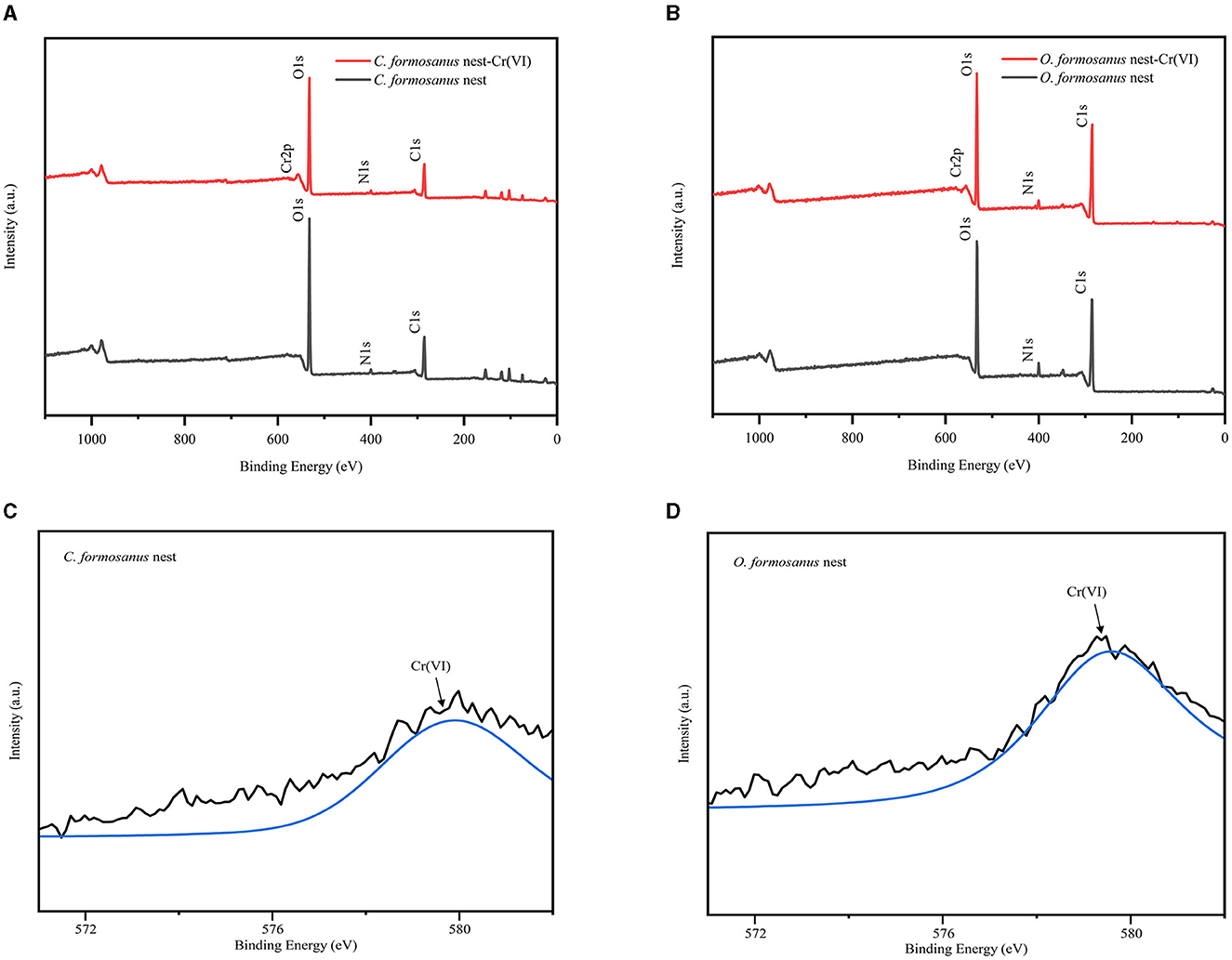
Figure 3. XPS wide scans and Cr 2p spectra of termite nest before and after Cr(VI) adsorption. (A, B) XPS wide scans, (C, D) Cr 2p XPS spectra.
In summary, the experimental findings suggest that the adsorption of Cr(VI) by the two types of termite nests primarily involves electrostatic interactions, complexation, and the partial reduction of Cr(VI) to Cr(III), which is then followed by complexation with surface functional groups.
3.2 Adsorption of Cr(VI) under different conditions
Figure 4A demonstrates that as the solution pH increases, the adsorption capacity for Cr(VI) by C. formosanus and O. formosanus termite nests initially decreases rapidly and then stabilizes. This trend can be explained by several factors. When the solution pH is below the isoelectric point, the high concentration of H+ ions protonates the functional groups on the surface of the termite nests, resulting in electrostatic attraction to Cr(VI) in the solution. The existence form of Cr(VI) varies with pH: as H2CrO4 at a pH below 1, as HCrO between pH 1 and 6, and as CrO at a pH above 6. HCrO requires fewer active adsorption sites compared to CrO, hence favoring adsorption at lower pH values (Araújo et al., 2013). As the pH increases, the adsorption of Cr(VI) decreases. However, even under strongly alkaline conditions, both types of termite nests still maintain a certain degree of Cr(VI) adsorption, indicating the involvement of specific functional groups that are complex with Cr(VI).
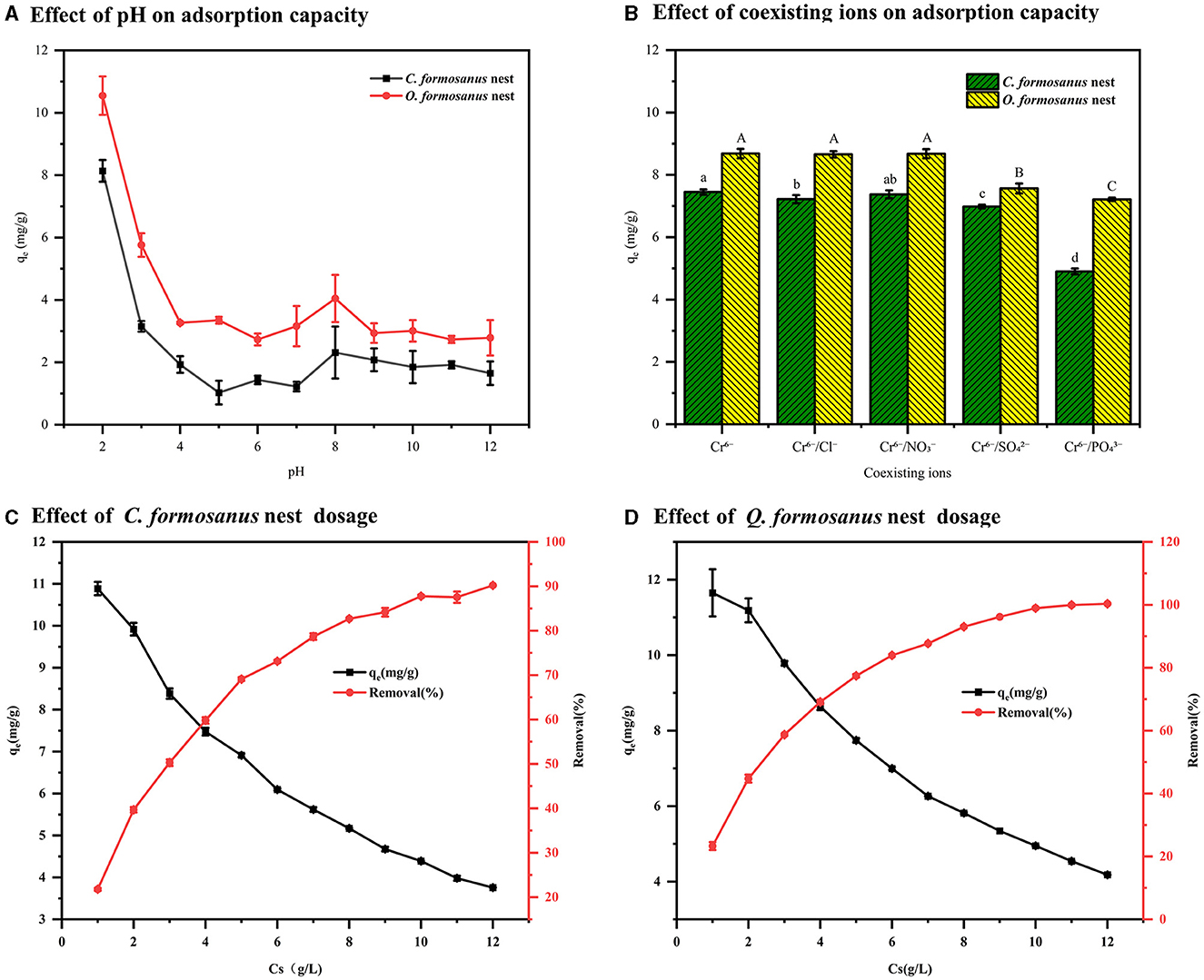
Figure 4. Effects of different factors on the adsorption capacity of termite nest. (A) pH, (B) coexisting ions, (C, D) termite nest dosage.
Industrial wastewater commonly contains various ions such as Cl−, NO, SO, and PO, making it crucial to study their impact on the adsorption process. As detailed in Figure 4B, our experiments were conducted with a constant ion concentration of 20 mg/L and an adsorbent dose of 4 g/L, under conditions of pH 2 and a temperature of 25°C. We found that Cl− and NO ions had minimal impact on the adsorption of Cr(VI) by both types of termite nests. In contrast, SO and PO significantly influenced the adsorption of Cr(VI): the removal efficiency of the C. formosanus nest decreased by 6.3% and 34.2%, respectively, while the O. formosanus nest showed a more pronounced decrease of 12.9% and 16.9%. This variation may be due to the molecular size of SO and PO anions being similar to the ionic radius of HCrO (Cai et al., 2019). Overall, the impact of coexisting ions on Cr(VI) adsorption by termite nests is relatively minor.
Figures 4C, D demonstrate that as termite nest dosage increases, the adsorption capacity decreases, but the removal efficiency of Cr(VI) ions from water improves. At a loading of 10 g/L, the removal efficiencies for C. formosanus and O. formosanus nests reach 87.77% and 98.95%, respectively, with adsorption capacities of 4.39 mg/g and 4.95 mg/g. This trend is attributed to the increased quantity of absorbance, offering more functional groups for adsorption and a larger specific surface area (Castro-Castro et al., 2020). However, removal efficiency plateaus with further loading increase, likely because excessive adsorbent leads to agglomeration, reducing the contact surface area and active adsorption sites (Chen et al., 2020). Additionally, reductive substances in the termite nests react with CrO, further enhancing Cr(VI) removal. Therefore, a 10 g/L loading is optimal for maximum removal efficiency, whereas a 4 g/L loading is more cost-effective.
3.3 Adsorption kinetics
To explore the adsorption kinetics of Cr(VI) by C. formosanus and O. formosanus nests, we examined the effect of contact time on adsorption capacity at three different Cr(VI) concentrations, using an optimal pH of 2. The findings, presented in Figure 5A, show that both nest types adsorbed Cr(VI) quickly at first but took longer to reach equilibrium. For C. formosanus nests, equilibrium was achieved at 12, 20, and 24 h for initial Cr(VI) concentrations of 50, 75, and 100 mg/L, respectively. O. formosanus nests reached equilibrium faster, within 6, 15, and 20 h at these concentrations. This suggests that the termite nests' Cr(VI) adsorption is influenced by the initial concentration (Xia et al., 2018a,b).
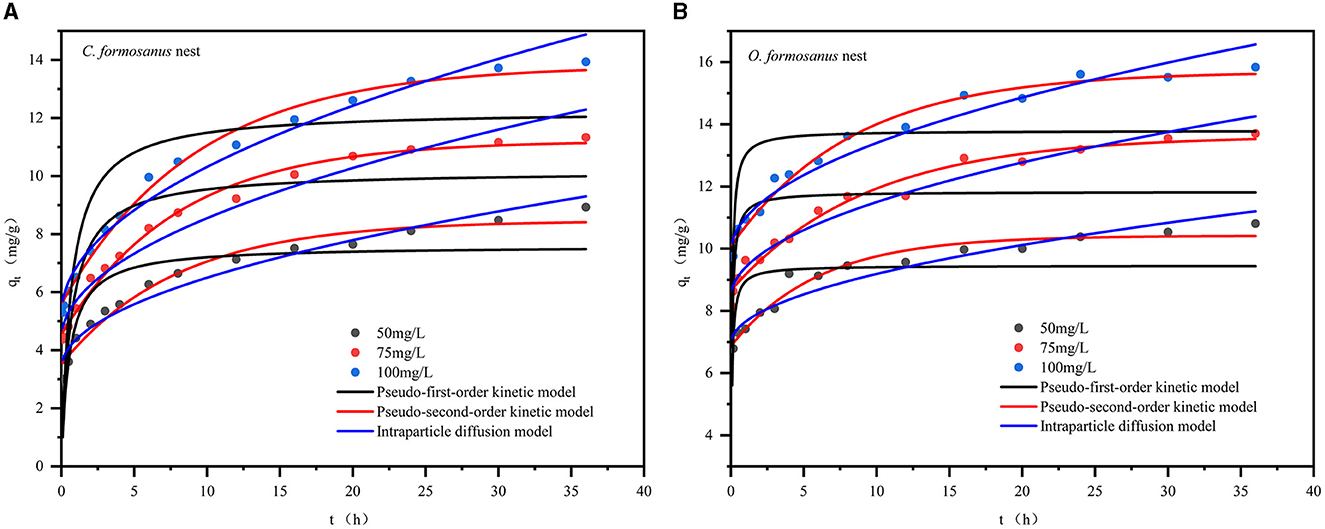
Figure 5. Non-linear fits of kinetic models to the Cr(VI) adsorption. (A) C. formosanus nest, (B) O. formosanus nest.
Furthermore, pseudo-first-order, pseudo-second-order and intraparticle diffusion models were performed to elucidate the adsorption kinetics. The three models were calculated as follows:
Pseudo-first-order kinetic model:
Pseudo-second-order kinetic model:
Intraparticle diffusion model:
where qe and qt are the amount of adsorbed Cr(VI) per unit mass of adsorbent (mg g−1) at equilibrium and time t, respectively. k1, k2, and k3 are the rate constants of the pseudo-first-order adsorption (h−1), pseudo-second-order adsorption (g mg−1 h−1) and intraparticle diffusion (mg g−1 h−0.5), respectively. c indicates the thickness of the boundary layer.
Table 2 presents the kinetic parameters, and Figure 5 displays the fitting curves for Cr(VI) concentrations of 50, 75, and 100 mg/L. The R2 values for the pseudo-second-order equation ranged from 0.9748 to 0.9991 for C. formosanus nests and 0.9661 to 0.9828 for O. formosanus nests, outperforming the pseudo-first-order and intraparticle diffusion models (see Table 2). Moreover, the equilibrium adsorption values derived from the pseudo-second-order model closely align with the experimental data. This indicates that Cr(VI) adsorption by both C. formosanus and O. formosanus nests adheres to a pseudo-second-order kinetic model. This model, which includes film diffusion, surface adsorption, and intraparticle diffusion stages, more accurately captures the complexity of the adsorption process (Sarfraz et al., 2022). Notably, similar kinetic behaviors are observed in the adsorption of heavy metals by tree termite nests, highlighting a shared characteristic among different types of nests (Araújo et al., 2013).
3.4 Adsorption isotherms
Comprehending the equilibrium adsorption isotherm is crucial for a thorough understanding of the termite nest's adsorption characteristics (Chen et al., 2020). To achieve this, we applied the experimental data of the termite nest to three key isotherm models: Langmuir, Freundlich, and Temkin. This approach allows for a comprehensive analysis of the adsorption properties.
Langmuir equation:
Freundlich equation:
Temkin equation:
where qe (mg/g) is the equilibrium adsorption capacity of Cr(VI) on the termite nest, Ce (mg/L) is the equilibrium Cr(VI) concentration in solution, qm (mg/g) is the maximum capacity of the adsorbent, KL (L/mg) is the Langmuir adsorption equilibrium constant related to the energy of adsorption, KF (L/mg) and 1/n are the Freundlich constant representing the adsorption capacity and adsorption intensity and A and B are Temkin constants.
Experimental adsorption data were collected at three temperatures: 298 K, 308 K, and 318 K, as shown in Figure 6. The isotherm fitting parameters are detailed in Table 3, with fitting curves for C. formosanus and O. formosanus nests displayed in Figures 6A, B. Correlation coefficients (R2 values, see Table 3) indicate that the adsorption behavior aligns more closely with the Freundlich model than with the Langmuir and Temkin models. The Langmuir model predicts maximum adsorption capacities (qm) of 48.5189 mg/g for C. formosanus and 35.9910 mg/g for O. formosanus. Additionally, the Freundlich constants (n), >1 for both termite nests, suggest favorable and heterogeneous adsorption. This finding implies that the adsorption of Cr(VI) on termite nests under our experimental conditions likely involves both monolayer biosorption and heterogeneous surface conditions (Charazińska et al., 2022). Consequently, the overall Cr(VI) adsorption process on termite nests appears complex, encompassing mechanisms such as ion exchange, electrostatic attraction, and surface complexation, as evidenced by the characterization results.
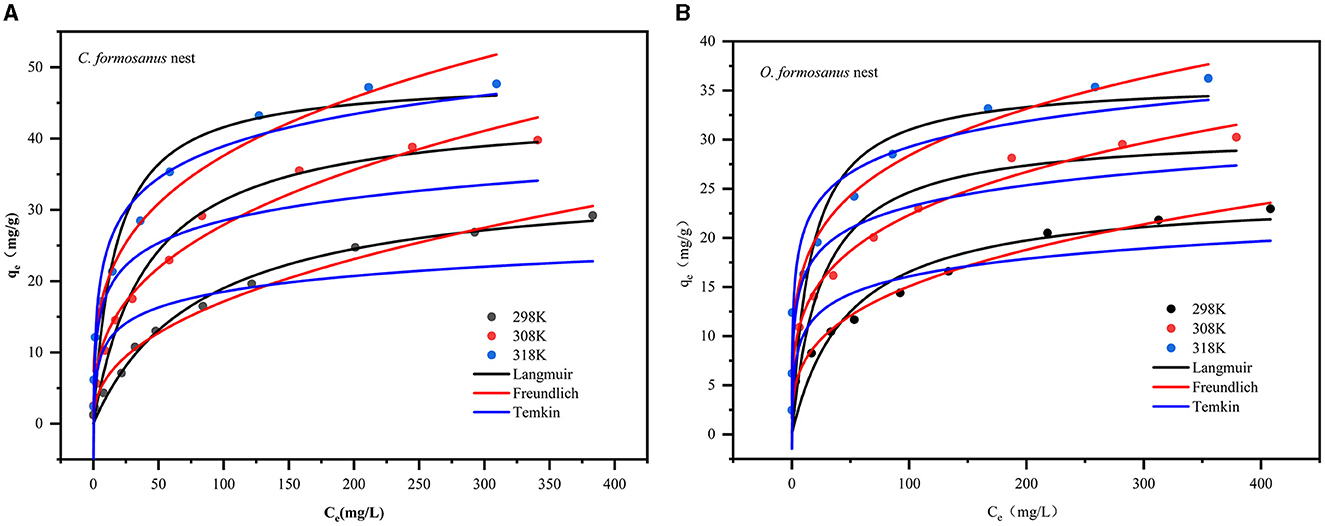
Figure 6. Non-linear fits of isotherm models to the Cr(VI) adsorption. (A) C. formosanus nest, (B) O. formosanus nest.
3.5 Desorption and reusability study
The desorption and reusability of adsorbents are crucial for practical applications. This study investigated the reusability of termite nests as adsorbents. We employed 0.1 mol/L HCl for desorbing Cr(VI) from the termite nests. Their reusability was evaluated through consecutive adsorption/desorption cycles. After five cycles, the Cr(VI) removal efficiency of C. formosanus and O. formosanus nests decreased by ~19.00% and 28.75%, respectively, as illustrated in Figure 7. The results show that both nests can be reused.
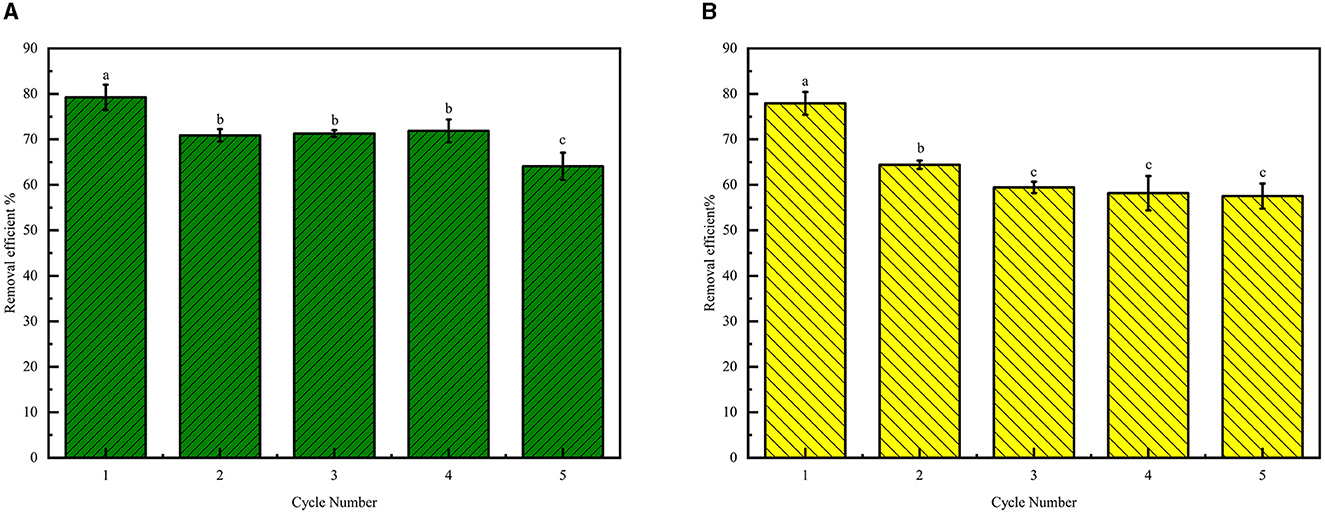
Figure 7. Reusability of the termite nest. (A) C. formosanus nest, (B) O. formosanus nest. Different letters over the bars denote significant differences (p < 0.05).
3.6 Pot cultivation of rapeseed
In the cultivation experiment, rapeseed (Brassia campestris L.) seeds were cultivated with untreated 50 mg/L Cr(VI) simulated wastewater and water treated with two types of termite nests (10 g/L) to assess their Cr(VI) adsorption or remediation efficiency. As shown in Figure 8, the rapeseed grown in the treated water exhibited notably longer stems and roots compared to the control (see Figure 8A). This suggests that Cr has an inhibitory effect on the growth of Brassia campestris L. The bioaccumulation of Cr by different parts of canola seedlings was further determined. The results showed that Cr accumulation was significantly reduced after termite nest adsorption treatment. For rapeseed grown in water associated with the control group, the Cr contents within roots and aerial parts were 15.6567 mg/kg and 6.4233 mg/kg, respectively; in the C. formosanus nest treatment group, these contents were 0.1267 mg/kg and 0.0267 mg/kg, respectively. In the O. formosanus nest treatment, the measured Cr contents in the roots and aerial parts of canola seedlings were 0.0733 mg/kg and 0.0067 mg/kg, respectively (see in Figure 8B). The substantial decrease in Cr levels, falling below the 0.5 mg/kg limit of China's national food safety standards, demonstrates the treatment's effectiveness (Yang et al., 2020).
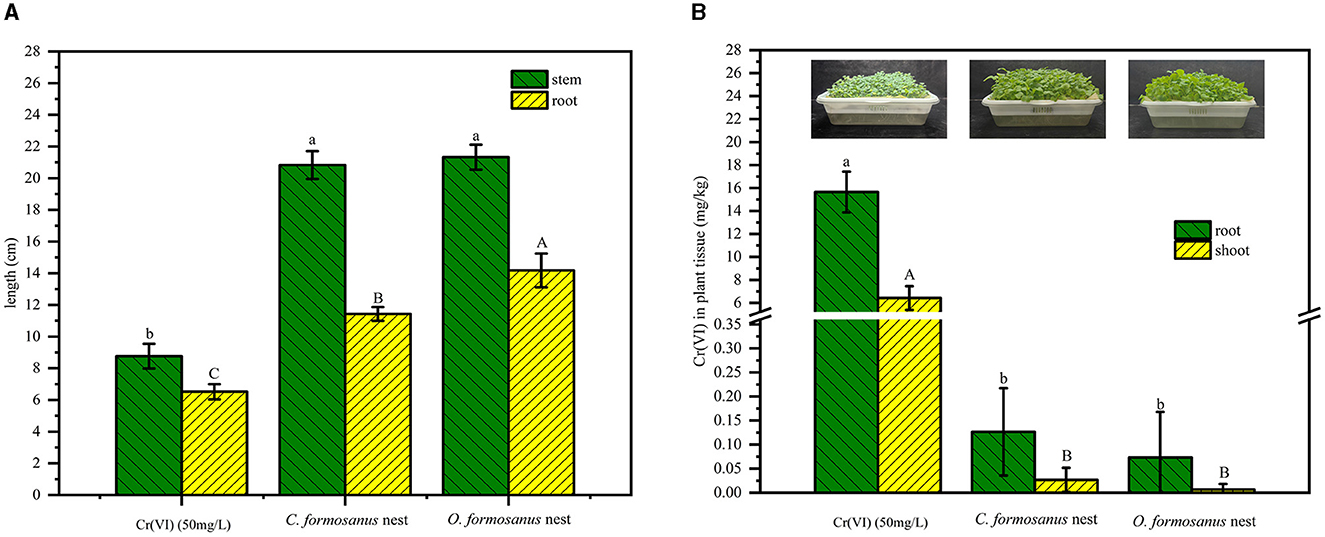
Figure 8. Effects of water on Brassica chinensis L. after termite nest restoration. (A) Growth of Brassica chinensis L. before and after water restoration in termite nests, (B) photos of rapeseed seedlings grown in untreated and remediated wastewater and the corresponding Cr content in roots and shoots of rapeseed. Different letters over the bars denote significant differences (p < 0.05; A. capital letters: root, lowercase letters: stem; B. capital letters: root; lowercase letters: shoot).
3.7 Comparison with other biosorbents
The study's findings reveal that nests of C. formosanus and O. formosanus have similar principles in Cr(VI) adsorption, yet differ in their capacities. The C. formosanus nest shows greater adsorption capacity and better regeneration, but is slower in adsorption rate and less efficient in removal compared to the O. formosanus nest. These variations may stem from the C. formosanus nest's larger surface area and more adsorption sites. However, C. formosanus nest's tendency to aggregate during adsorption may impede Cr(VI) access to these sites, leading to a prolonged time for reaching equilibrium. This underscores the need to choose the right termite nest type for practical use, considering specific adsorption needs and conditions (Chen et al., 2020).
The biosorption capability of termite nests for Cr(VI) was compared with other low-cost biosorbents, as detailed in Table 4 from various literature sources. While direct comparisons can be challenging due to differences in experimental conditions like temperature, pH, and adsorbent dosage, our findings indicate that C. formosanus and O. formosanus nests demonstrate a reasonable Cr(VI) adsorption capacity compared to other biosorbents. Notably, termite nests are often by-products of pest control efforts and are renewable and reusable. In the field of termite control, the nest excavation method is considered an effective approach for controlling large nesting termites, especially for controlling large soil-dwelling termites in dams. This process usually requires the use of synthetic chemical reagents with potential environmental risks. In this context, an innovative proposal was made in this study: instead of using synthetic chemical reagents to exterminate the living organisms in termite nests discarded during termite control, the nests were directly collected and used for the preparation of natural biomass adsorbents. This approach not only utilizes resources that would otherwise be wasted, but also helps to reduce the use of chemical reagents, thereby reducing potential risks to the environment.
4 Conclusions and perspectives
The study addresses the critical issue of Chromate [Cr(VI)], a highly soluble and environmentally harmful substance that poses significant risks to food safety and human health. It necessitates finding effective adsorbents for wastewater treatment. The research successfully developed an innovative, effective, and eco-friendly adsorbent using C. formosanus and O. formosanus nests, demonstrating excellent Cr(VI) adsorption. Key characteristics of these termite nests include their natural origin and construction by insects, rendering them environmentally benign and free of harmful chemicals. They show superior adsorption efficiency, especially at pH 2 and a Cr(VI) concentration of 50 mg/L, with an optimal adsorbent dose of 4 g/L. Kinetic analysis aligns their adsorption with the pseudo-second-order model, and their maximum adsorption capacities are 48.52 mg/g for C. formosanus and 35.99 mg/g for O. formosanus. Analytical techniques like SEM, point of zero charge, FTIR, and XPS reveal that chemical adsorption is the primary mechanism, involving electrostatic interactions, complexation, and partial reduction of Cr(VI) to Cr(III), followed by complexation with adsorbent surface groups. Hydroponic experiments with Brassica chinensis L. indicate both termite nests significantly mitigate the impact of Cr(VI) on plant growth and Cr content. The regeneration experiments reveal that both C. formosanus and O. formosanus nests maintain robust adsorption capabilities even after five cycles. This finding highlights termite nests significant reusability as adsorbents.
In summary, this research demonstrates that termite nests offer a practical, affordable, and reusable solution for removing Cr(VI) from wastewater. Their use as biological adsorption material leverages biomass waste and diminishes the need for pesticides in termite control. Future studies should delve into the optimization of termite nest treatment processes, exploring the potential of chemical or physical modification techniques to enhance adsorption efficiency. Investigating the interaction mechanisms between termite nests and various pollutants could also provide insights into broadening the scope of their application in environmental remediation. Additionally, the study's implications extend beyond mitigating chromium pollution, offering benefits in environmental protection, resource utilization, cost savings, and pesticide reduction. A deeper analysis of the economic and environmental impacts of large-scale implementation could further substantiate the practicality of this approach. Moreover, examining the regeneration and reuse cycles of termite nest-based adsorbents will be crucial in assessing their long-term viability and operational costs. These findings significantly bolster efforts toward sustainable development and environmental conservation, paving the way for innovative uses of termite nests in addressing complex environmental challenges.
Data availability statement
The original contributions presented in the study are included in the article/supplementary material, further inquiries can be directed to the corresponding author.
Author contributions
GL: Funding acquisition, Supervision, Writing – review & editing. MW: Writing – original draft, Data curation, Investigation, Methodology. NW: Writing – review & editing. JZ: Writing – review & editing. JYu: Writing – review & editing. LJ: Writing – review & editing. LL: Writing – review & editing. JYa: Writing – review & editing. SG: Writing – review & editing. YZ: Writing – review & editing. RZ: Writing – review & editing. LZ: Writing – review & editing.
Funding
The author(s) declare that financial support was received for the research, authorship, and/or publication of this article. This work was supported by the National Natural Science Foundation of China (31601891), the Natural Science Foundation of Hubei Province of China (2023AFB1005), and the Hubei Key Laboratory of Edible Wild Plants Conservation and Utilization (EWPL201615).
Conflict of interest
The authors declare that the research was conducted in the absence of any commercial or financial relationships that could be construed as a potential conflict of interest.
Publisher's note
All claims expressed in this article are solely those of the authors and do not necessarily represent those of their affiliated organizations, or those of the publisher, the editors and the reviewers. Any product that may be evaluated in this article, or claim that may be made by its manufacturer, is not guaranteed or endorsed by the publisher.
References
Abdus-Salam, N., and Bello, M. O. (2015). Kinetics, thermodynamics and competitive adsorption of lead and zinc ions onto termite mound. Int. J. Environ. Sci. Technol. 12, 3417–3426. doi: 10.1007/s13762-015-0769-2
Ahmad, F., Fouad, H., Liang, S., Hu, Y., and Mo, J. (2021a). Termites and Chinese agricultural system: applications and advances in integrated termite management and chemical control. Insect. Sci. 28, 2–20. doi: 10.1111/1744-7917.12726
Ahmad, F., Yang, G., Liang, S., Zhou, Q., Gaal, H. A., and Mo, J. (2021b). Multipartite symbioses in fungus-growing termites (Blattodea: Termitidae, Macrotermitinae) for the degradation of lignocellulose. Insect. Sci. 28, 1512–1529. doi: 10.1111/1744-7917.12890
Amer, M. W., Khalili, F. I., and Awwad, A. M. (2010). Adsorption of lead, zinc and cadmium ions on polyphosphate-modified kaolinite clay. J. Environ. Chem. Ecotoxicol. 2, 001008.
Araújo, B. R., Reis, J. O. M., Rezende, E. I. P., Mangrich, A. S., Wisniewski, A., Dick, D. P., et al. (2013). Application of termite nest for adsorption of Cr(VI). J. Environ. Manage. 129, 216–223. doi: 10.1016/j.jenvman.2013.07.004
Baran, A., Biçak, E., Baysal, S. H., and Önal, S. (2007). Comparative studies on the adsorption of Cr(VI) ions on to various sorbents. Bioresour. Technol. 98, 661–665. doi: 10.1016/j.biortech.2006.02.020
Benimeli, C. S., Polti, M. A., Albarracín, V. H., Abate, C. M., and Amoroso, M. J. (2011). Bioremediation potential of heavy metal–resistant actinobacteria and maize plants in polluted soil. Epol. 20, 459–477. doi: 10.1007/978-94-007-1914-9_20
Cai, W., Zhu, F., Liang, H., Jiang, Y., Tu, W., Cai, Z., et al. (2019). Preparation of thiourea-modified magnetic chitosan composite with efficient removal efficiency for Cr(VI). Chem. Eng. Res. Des. 144, 150–158. doi: 10.1016/j.cherd.2019.01.031
Castro-Castro, J. D., Sanabria-González, N. R., and Giraldo-Gómez, G. I. (2020). Experimental data of adsorption of Cr(III) from aqueous solution using a bentonite: optimization by response surface methodology. Data Brief. 28, 105022. doi: 10.1016/j.dib.2019.105022
Charazińska, S., Burszta-Adamiak, E., and Lochyński, P. (2022). The efficiency of removing heavy metal ions from industrial electropolishing wastewater using natural materials. Sci. Rep. 12, 17766. doi: 10.1038/s41598-022-22466-9
Chen, J., Liang, Q., Ploychompoo, S., and Luo, H. (2020). Functional rGO aerogel as a potential adsorbent for removing hazardous hexavalent chromium: adsorption performance and mechanism. Environ. Sci. Pollut. Res. 27, 10715–10728. doi: 10.1007/s11356-019-07365-3
Cunha, G. da C, dos Santos, B. T., Alves, J. R., Alves Silva, I. A., de Souza Cruz, D. R., et al. (2018). Applications of magnetic hybrid adsorbent derived from waste biomass for the removal of metal ions and reduction of 4-nitrophenol. J. Environ. Manage. 213, 236–246. doi: 10.1016/j.jenvman.2018.02.031
Dakiky, M., Khamis, M., Manassra, A., and Mer'eb, M. (2002). Selective adsorption of chromium(VI) in industrial wastewater using low-cost abundantly available adsorbents. Aer. 6, 533–540. doi: 10.1016/S1093-0191(01)00079-X
den Braver-Sewradj, S. P., van Benthem, J., Staal, Y. C. M., Ezendam, J., Piersma, A. H., and Hessel, E. V. S. (2021). Occupational exposure to hexavalent chromium. Part II. Hazard assessment of carcinogenic effects. Regul. Toxicol. Pharmacol. 126, 105045. doi: 10.1016/j.yrtph.2021.105045
Fernández-López, J. A., Angosto, J. M., and Avilés, M. D. (2014). Biosorption of hexavalent chromium from aqueous medium with opuntiabiomass. Sci. World J. 2014, 1–8. doi: 10.1155/2014/670249
Gupta, V. K., Pathania, D., Agarwal, S., and Sharma, S. (2013). Removal of Cr(VI) onto Ficus carica biosorbent from water. Environ. Sci. Pollut. Res. 20, 2632–2644. doi: 10.1007/s11356-012-1176-6
Hayat, S., Khalique, G., Irfan, M., Wani, A. S., Tripathi, B. N., and Ahmad, A. (2012). Physiological changes induced by chromium stress in plants: an overview. Protoplasma 249, 599–611. doi: 10.1007/s00709-011-0331-0
Idowu, S. O., Oni, S. O., and Adejumo, A. A. (2011). Biosorption of chromium (VI) from aqueous solution by biomass of plantain (Musa Paradisiaca) peel residue. Afr. J. Med. Phys. Biomed. Eng. Sci 3, 2227.
Jing, Y., Li, Z., Li, Y., Lei, G., Li, L., Yang, X., et al. (2021). The ability of edible fungi residue to remove lead in wastewater. Front. Environ. Sci. 9, 723087. doi: 10.3389/fenvs.2021.723087
Lertlumnaphakul, W., Ngoen-Klan, R., Vongkaluang, C., and Chareonviriyaphap, T. (2022). A review of termite species and their distribution in Thailand. Insects. 13, 186. doi: 10.3390/insects13020186
Li, W., Tang, Y., Zeng, Y., Tong, Z., Liang, D., and Cui, W. (2012). Adsorption behavior of Cr(VI) ions on tannin-immobilized activated clay. Chem. Eng. J. 193–194, 88–95. doi: 10.1016/j.cej.2012.03.084
Li, Z., Zou, P., Yang, J., Huang, M., Zhang, L., Huang, C., et al. (2021). A functionalized tannin-chitosan bentonite composite with superior adsorption capacity for Cr(VI). J. Polym. Eng. 41, 34–43. doi: 10.1515/polyeng-2020-0133
Liu, Q., Qu, L., and Ren, B. (2020). Effective removal of copper ions from aqueous solution by iminodiacetic acid-functionalized Paeonia ostii seed coats. J. Dispers. Sci. Technol. 41, 1126–1135. doi: 10.1080/01932691.2019.1614457
Loukidou, M. X., Karapantsios, T. D., Zouboulis, A. I., and Matis, K. A. (2004). Diffusion kinetic study of chromium(VI) biosorption by Aeromonas caviae. Ind. Eng. Chem. Res. 43, 1748–1755. doi: 10.1021/ie034132n
Malkoc, E., Nuhoglu, Y., and Dundar, M. (2006). Adsorption of chromium(VI) on pomace-an olive oil industry waste: batch and column studies. J. Hazard. Mater. 138, 142–151. doi: 10.1016/j.jhazmat.2006.05.051
Mansour, R. A., Atef, R., Elazaby, R. R., and Zaatout, A. A. (2020). Experimental study on the adsorption of Cr +6 and Ni +2 from aqueous solution using low-cost natural material. Int. J. Phytoremediat. 22, 508–517. doi: 10.1080/15226514.2019.1683716
Meng, J., Feng, X., Dai, Z., Liu, X., Wu, J., and Xu, J. (2014). Adsorption characteristics of Cu(II) from aqueous solution onto biochar derived from swine manure. Environ. Sci. Pollut. Res. 21, 7035–7046. doi: 10.1007/s11356-014-2627-z
Owlad, M., Aroua, M. K., Daud, W. A. W., and Baroutian, S. (2009). Removal of hexavalent chromium-contaminated water and wastewater: a review. Water. Air. Soil. Pollut. 200, 59–77. doi: 10.1007/s11270-008-9893-7
Paul, B., Khan, A., Paul, S., Shankarganesh, K., and Chakravorty, S. (2018). “Termite and Indian agriculture,” in Termites and Sustainable Management (Springer International Publishing), 51-96. doi: 10.1007/978-3-319-68726-1_3
Pehlivan, E., and Altun, T. (2008). Biosorption of chromium(VI) ion from aqueous solutions using walnut, hazelnut and almond shell. J. Hazard. Mater. 155, 378–384. doi: 10.1016/j.jhazmat.2007.11.071
Qin, H., Hu, T., Zhai, Y., Lu, N., and Aliyeva, J. (2020). The improved methods of heavy metals removal by biosorbents: a review. Environ. Pollut. 258, 113777. doi: 10.1016/j.envpol.2019.113777
Qiu, Y., Cheng, H., Xu, C., and Sheng, G. D. (2008). Surface characteristics of crop-residue-derived black carbon and lead(II) adsorption. Water. Res. 42, 567–574. doi: 10.1016/j.watres.2007.07.051
Sarfraz, S., Ameer, S., Javed, M., Iqbal, S., Aljazzar, S. O., Zahra, M., et al. (2022). Removal of hexavalent chromium ions using micellar modified adsorbent: isothermal and kinetic investigations. Rsc. Adv. 12, 23898–23911. doi: 10.1039/D2RA03906K
Sarin, V., and Pant, K. (2006). Removal of chromium from industrial waste by using eucalyptus bark. Bioresour. Technol. 97, 15–20. doi: 10.1016/j.biortech.2005.02.010
Sharma, P., Singh, S. P., Parakh, S. K., and Tong, Y. W. (2022). Health hazards of hexavalent chromium (Cr (VI)) and its microbial reduction. Bioengineered. 13, 4923–4938. doi: 10.1080/21655979.2022.2037273
Shi, S., Yang, J., Liang, S., Li, M., Gan, Q., Xiao, K., et al. (2018). Enhanced Cr(VI) removal from acidic solutions using biochar modified by Fe3O4@SiO2-NH2 particles. Sci. Total. Environ. 628–629, 499–508. doi: 10.1016/j.scitotenv.2018.02.091
Shukla, D., and Vankar, P. S. (2012). Efficient biosorption of chromium(VI) ion by dry Araucaria leaves. Environ. Sci. Pollut. Res 19, 2321–2328. doi: 10.1007/s11356-012-0741-3
Singh, V., Singh, N., Verma, M., Kamal, R., Tiwari, R., Sanjay Chivate, M., et al. (2022). Hexavalent-chromium-induced oxidative stress and the protective role of antioxidants against cellular toxicity. Antioxidants 11, 2375. doi: 10.3390/antiox11122375
Song, X., Jiang, Y., Rong, X., Wei, W., Wang, S., and Nie, S. (2016). Surface characterization and chemical analysis of bamboo substrates pretreated by alkali hydrogen peroxide. Bioresour. Technol. 216, 1098–1101. doi: 10.1016/j.biortech.2016.06.026
Subekti, N., Yoshimura, T., Rokhman, F., and Mastur, Z. (2015). Potential for subterranean termite attack against five bamboo speciesin correlation with chemical components. Procedia Environ. Sci. 28, 783–788. doi: 10.1016/j.proenv.2015.07.092
Suksabye, P., Nakajima, A., Thiravetyan, P., Baba, Y., and Nakbanpote, W. (2009). Mechanism of Cr(VI) adsorption by coir pith studied by ESR and adsorption kinetic. J. Hazard. Mater. 161, 1103–1108. doi: 10.1016/j.jhazmat.2008.04.064
Tian, W.-J., Ke, Y.-L., Zhuang, T.-Y., Wang, C.-X., Li, M., Liu, R.-Q., et al. (2009). Incipient colony development and biology of Odontotermes formosanus (Shiraki) and O. hainanensis (Light) (Isoptera: Termitidae). J. Agr. Urban. Entomol. 26, 147–156. doi: 10.3954/1523-5475-26.3.147
Van Huis, A. (2017). Cultural significance of termites in sub-Saharan Africa. J. Ethnobiol. Ethnomed. 13, 8. doi: 10.1186/s13002-017-0137-z
Verma, M., Sharma, S., and Prasad, R. (2009). Biological alternatives for termite control: a review. Int. Biodeter. Biodegr. 63, 959–972. doi: 10.1016/j.ibiod.2009.05.009
Xia, X., Wu, S., Li, N., Wang, D., Zheng, S., and Wang, G. (2018a). Novel bacterial selenite reductase CsrF responsible for Se(IV) and Cr(VI) reduction that produces nanoparticles in Alishewanella sp. WH16-1. J. Hazard. Mater. 342, 499–509. doi: 10.1016/j.jhazmat.2017.08.051
Xia, X., Zhou, Z., Wu, S., Wang, D., Zheng, S., and Wang, G. (2018b). Adsorption removal of multiple dyes using biogenic selenium nanoparticles from an Escherichia coli strain overexpressed selenite reductase CsrF. Nanomaterials-Basel. 8, 234. doi: 10.3390/nano8040234
Keywords: chromate, termite nest, adsorption, removal, Brassica chinensis L.
Citation: Wang M, Wang N, Zhou J, Yuan J, Jiang L, Lu L, Yan J, Gong S, Zeng Y, Zhang R, Zhi L and Li G (2024) Removal of chromate in aqueous solutions by termite nests and reduction chromate accumulation in Brassica chinensis L.. Front. Sustain. Food Syst. 8:1351964. doi: 10.3389/fsufs.2024.1351964
Received: 07 December 2023; Accepted: 08 January 2024;
Published: 23 January 2024.
Edited by:
Kaixiang Shi, Huazhong Agricultural University, ChinaCopyright © 2024 Wang, Wang, Zhou, Yuan, Jiang, Lu, Yan, Gong, Zeng, Zhang, Zhi and Li. This is an open-access article distributed under the terms of the Creative Commons Attribution License (CC BY). The use, distribution or reproduction in other forums is permitted, provided the original author(s) and the copyright owner(s) are credited and that the original publication in this journal is cited, in accordance with accepted academic practice. No use, distribution or reproduction is permitted which does not comply with these terms.
*Correspondence: Ganghua Li, ghli@hbnu.edu.cn