- 1Faculty of Agriculture, University of Zagreb, Zagreb, Croatia
- 2Institute of General and Physical Chemistry, University of Belgrade, Belgrade, Serbia
- 3Faculty of Forestry and Wood Technology, University of Zagreb, Zagreb, Croatia
The objective of this study was to investigate the changes in the nutrient and fatty acid profiles of hazelnuts (Corylus avellana) and walnuts (Juglans regia) subjected to continuous drying. Samples from two consecutive years (2020 and 2021) were analyzed for nutritional value both before and after conduction drying. Thermal conduction drying was performed at 60 and 80°C at intervals of 15, 30, 45, and 60 min. The results showed that hazelnuts had increased ash, protein (from 16.4 to 18.7%), carbohydrate and starch content, while walnuts had a higher pH and fat content (from 60.97 to 71.02%). After drying, increasing temperatures resulted in significant changes in nutrient concentrations for both nuts, including changes in ash, protein, fat (hazelnuts from 58.69 to 71.48% at 60°C for 60 min), carbohydrate and starch content, and pH. Monounsaturated and polyunsaturated fatty acid content varied by sample and year, with notable trends such as the increase in oleic acid in walnuts from 82.26 to 83.67%. Longer drying times and higher temperatures correlated with an increase in monounsaturated fatty acids and a decrease in polyunsaturated fatty acids in both nut types. In conclusion, conduction drying, especially at higher temperatures and longer durations, significantly affects the nutrient and fatty acid profiles of hazelnuts and walnuts. The study provides new insights into the effects of drying conditions on the nutrient composition and fatty acid profiles of hazelnuts and walnuts and reveals significant changes that warrant further investigation. It sets the stage for future research to extend these findings to other nut species and alternative drying processes and highlights the importance of optimizing processing parameters for improved health benefits and sustainability.
1 Introduction
The consumption of nuts is associated with numerous health benefits due to their nutrient density, as they are an important component of a sustainable diet. The risk of malnutrition increases with aging, which has a negative impact on health and quality of life. Nuts, with their high nutrient characteristics, can improve the nutritional status of older adults at risk of malnutrition (Tan et al., 2018). However, it is important to consider the significant differences in nutrient composition of different nut species in a sustainable diet (Cap et al., 2022). Walnuts are particularly rich in the omega-3 fatty acids linolenic acid (C 18:3) and omega-6 fatty acids linoleic acid (C 18:2). Nuts are rich in polyunsaturated fatty acids (PUFA), which have a positive effect on brain health (Carey et al., 2020; Müller et al., 2020), and eating walnuts can improve brain cell health and cognitive function (Nogales-Bueno et al., 2021). Hazelnuts contribute positively to human health due to their lipid content (Taş and Gökmen, 2015), and nut consumption has been associated with improved glycemic control and cardiovascular markers in type 2 diabetes (Nishi et al., 2014). In addition, African walnut oil provides an essential fatty acid composition that is ideal for culinary use (Nkwonta et al., 2016). The high proportion of monounsaturated and polyunsaturated fatty acids in this profile suggests that the oil could potentially offer nutritional benefits to consumers in their daily cooking habits. Nuts high in monounsaturated fatty acids, such as oleic acid, have a positive effect on lowering blood cholesterol levels, chronic heart disease and atherosclerosis (Phatanayindee et al., 2012). Post-harvest processing, including drying, is critical to maintaining the nutritional integrity of nuts as it minimizes moisture content, thereby increasing food safety and preserving quality during storage (Turan, 2018a). The drying process, a time-honored preservation technique for agricultural products, is particularly important in the context of hazelnuts. Studies have shown that different drying parameters such as ambient temperature, duration and energy input can significantly alter the physical and chemical properties of hazelnuts (Li et al., 2021). It is imperative to use modern drying methods that not only ensure efficient moisture removal, but also carefully modulate temperatures to preserve the sensory and nutritional value of the nuts (da Silva Simão et al., 2021). In their study of the effects of drying on hazelnuts, Alasalvar et al. (2010) found that drying, especially roasting, can increase the oil content and only slightly alter the fatty acid profile of the different Turkish hazelnut varieties. This underlines the importance of customized drying processes for maintaining the quality of the fatty acid compounds within the nut varieties.
Considering the current research on drying processes and nut composition, this study seeks to expand upon these insights by examining the effects of different drying conditions on hazelnuts and walnuts. The main objective of this study is to perform a comparative analysis between two different nut species — Corylus avellana (hazelnut) and Juglans regia (walnut) – focusing on elucidating differences in their chemical and fatty acid profiles. In addition, both nut species were subjected to a conduction drying procedure to systematically evaluate the effects of two different temperature settings (60°C and 80°C) over four specific time intervals (15, 30, 45, and 60 min) on the resulting compositional changes.
2 Materials and methods
2.1 Sample collection
The study was conducted over the course of two growing seasons (2020 and 2021) in Bjelovar-Bilogora County, Daruvar City. The orchard, which contains both hazelnut and walnut trees, is located at an altitude of 257 m above sea level, at a latitude of 45° and a longitude of 17°. The orchard was planted in 2003 with trees spaced 4.5 × 4 m apart and is cultivated in a vase-type nursery system. The orchard includes 1,250 hazelnut and walnut bushes. Harvesting was performed manually to avoid damaging. All collected samples were manually cleaned from remnants of the casings and impurities and prepared for further processing.
2.2 Conduction drying
Conduction drying was conducted in the laboratory model of the drying system. The toaster itself consists of a case with a door in which a perforated plate measuring 800 × 800 mm is installed. 3 PT 1000 probes are installed on the toaster itself to measure the air temperature at the entrance and exit from the toaster and the temperature of the hazelnuts in the air stream. The initial regulation of the air temperature is manual, and then it is switched to automatic. Thermal treatment of conduction drying has been carried out at temperatures of 60 and 80°C for times of 15-, 30-, 45-, and 60-min.
2.3 Moisture content determination
The moisture content (MC) is determined according to the protocol (CEN/TS 14774-2:2004, 2004) in the laboratory drying system. MC is determined by drying in an oven at 103°C (±2°C) for 3 h to a constant mass, if the sample does not contain volatile components or products other than moisture that could cause a change in the mass of the product under study. The samples were dried in a laboratory drying oven (INKO ST −40, Croatia) with the possibility of temperature regulation from 40 to 240°C according to the protocol. The measurement accuracy is ±0.1°C, and the volume of the working chamber is 20 L.
2.4 Determination of protein, oil, and carbohydrate content
Protein content was determined using AOAC Standard Methods (AOAC 950.48-1950; AOAC International, 2011) by the Kjeldahl method (Kjeldahl distillation unit Behrotest S1, Germany) and the conversion factor used to determine protein content was N% × 6.25. Oil content was determined according to AOAC Official Methods (ANON, 1990), and determined by extracting sample with petroleum ether, using a Soxhlet extractor R 304 (Germany). Carbohydrate content was calculated following (Oliveira et al., 2008) using the equation: carbohydrate content = 1,000 g kg − 1 of kernel dry weight − (protein content + fat content + ash content).
2.5 Determination of starch and ash content
To determine the total starch content, enzymatic tests were performed using Megazyme test kits. The procedure was performed in accordance with the American Association of Cereal Chemists (AACC) methods 76-13.01 and 76-30.02 (Determination of Damaged Starch – 76-30.02, 1999; Total Starch Assay Procedure, 2011). Briefly, the enzymatic tests involved the degradation of starch molecules into simpler glucose units, which were then quantified colorimetrically to estimate the total starch content. For ash content determination, samples were subjected to combustion in a muffle furnace (Nabertherm B170, Germany) (Association of Official Analytical Chemists (AOAC), 2000). The furnace was preheated to a temperature of 550°C and the samples were burned for a period of 12 h. This process was performed in accordance with AOAC Official Method 950.49.23. After combustion, the remaining ash was weighed, and its mass used to calculate the percentage ash content of the original sample.
2.6 Determination of fatty acids content
Prior to analysis, samples were ground and homogenized to ensure uniform distribution. The lipid fraction was extracted using a cold solution extraction. Gas chromatographic analyzes were performed according to the method (HRN EN ISO 5509:2004, 2004) using a CP -3800, Varian (United States), equipped with a flame ionization detector (FID CP -3800, Varian, USA) and a DB -23 fused silica capillary column (60 m × 0.25 mm, film thickness 0.25 μm). Helium served as the carrier gas at a flow rate of 1.5 mL/min. The hydrogen and air flow rates were set to 30 and 300 mL/min, respectively. The injector temperature was maintained at 250°C, and the oven temperature program ranged from 60 to 220°C at a rate of 7°C/min. The FID was operated at 250°C. The injection volume was 1.0 μL. To ensure reliability of the data, three replicates of the analysis were performed for each sample. Fatty acids were quantified using a standard calibration curve, and results are expressed as percent (%).
2.7 Determination of pH value
The pH value of the hazelnut and walnut samples was measured to evaluate the effects of drying on the physicochemical properties of the nuts, as pH is an indicator of potential microbial stability and product quality (Liu et al., 2022). Samples were prepared by weighing 10 g of the nut kernels and adding 100 mL of distilled water. The pH was then measured using a Mettler Toledo pH meter calibrated with standard buffer solutions of pH = 4 and pH = 7 before each analysis. It has been reported that drying processes can alter pH, affecting the overall quality of the product (Mechlouch et al., 2012). In this study, we focused on quantifying the pH values before and after drying to understand their effects on product quality. We refrained from detailing the operation of the pH meter but assure that it was operated according to standard analytical protocols.
2.8 Statistical analysis
Python 3.11.1 (Van Rossum and Drake, 2009) and associated packages, including pandas, NumPy, and matplotlib (Hunter, 2007; McKinney, 2010; Harris et al., 2020), were used for statistical analysis and data visualization. Data are presented as mean and standard deviation. Univariate analysis included analysis of variance (ANOVA) and Tukey’s post hoc HSD test, which were used to identify significant differences between the observed variables based on the assigned categories. In addition to the univariate methods, a multivariate analysis was performed to evaluate the effects of the parameters studied on the changes in fatty acid composition of the nut samples. This was done to gain a more comprehensive understanding of how the factors studied interacted to influence the dependent variables. Principal component analysis (PCA) was performed separately to explore underlying patterns in the data set, identify potential outliers, and provide a dimensionally reduced view of the multiple measured variables, facilitating interpretation of the data. All statistical analyzes, including ANOVA, Tukey’s HSD test, multivariate analysis, and PCA, were performed at the 95% confidence level.
3 Results
3.1 Moisture content
Figure 1 shows the average change in moisture content of the hazelnut samples before and after the application of temperature treatment and the duration of the process.
Figure 2 shows the change in moisture content of the walnut samples before and after the application of temperature treatment and the duration of the process.
Figures 1, 2 show that the moisture content (MC) of both hazelnuts and walnuts decreases significantly with increasing drying time and temperature, which is illustrated by a color gradient. This consistent decrease in moisture content is most pronounced at 80°C over a 60-min period, as shown by the data points. This emphasizes a significant inverse relationship between drying conditions and moisture content in these nut types.
3.2 Nutrient composition
Table 1 shows the differences in the composition of fresh hazelnut and walnut samples in two consecutive years, listing the differences in the percentages of ash, protein, fat, carbohydrate, starch and pH with statistical significance and indicators of variability.
Table 1 shows the results of the nutritional analysis for hazelnuts and walnuts in 2020 and 2021, comparing the percentages of ash, protein, fat, carbohydrates, starch and pH values for each nut type, along with indications of statistical significance. Hazelnuts had a higher fat content in the first year, while walnuts showed an increase in the second year, with a marginal increase in fat content from 2020 to 2021. Hazelnuts consistently had a higher protein content in both years. Conversely, walnuts had a higher carbohydrate content in both years. The pH values were stable in all samples, with walnuts having a slightly higher pH value in both years.
Table 2 shows the changes in the composition of the hazelnut and walnut after conduction drying, with differences in the percentage of ash, protein, fat, carbohydrates, starch, and pH value.
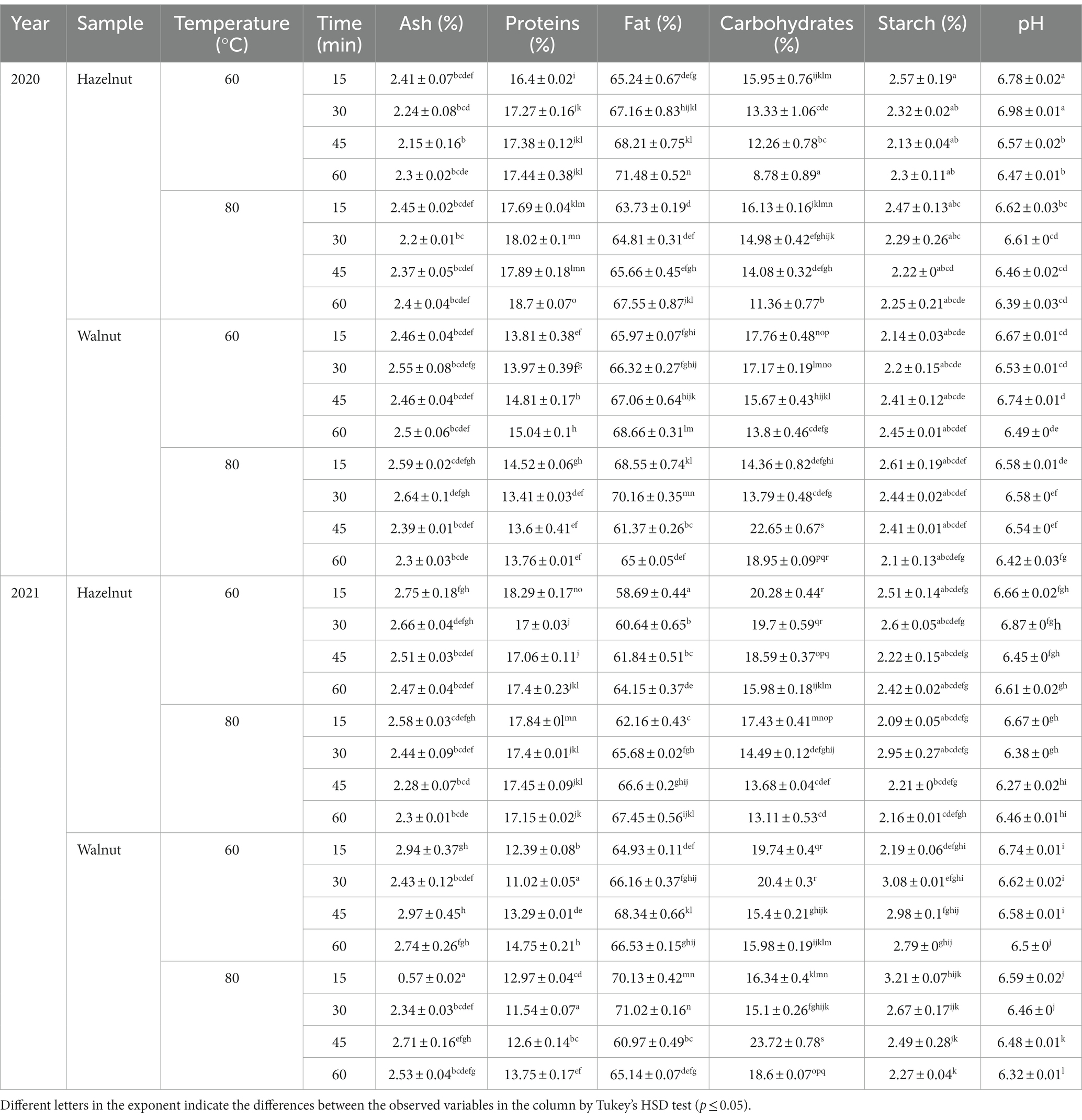
Table 2. Nutrient composition of hazelnuts and walnut samples over two years after conduction drying.
Table 2 shows that the carbohydrate, starch and pH values generally decrease with increasing temperature and drying time, while the ash, protein and fat content tend to increase. In particular, the lowest ash content was found in hazelnuts dried at 60°C for 15 min and the highest fat concentration in hazelnuts treated at 80°C for 60 min. The highest protein content was found in hazelnuts dried at 80°C for 60 min, while the lowest carbohydrate and starch contents were also found in hazelnuts, but at the same elevated temperature and a shorter drying time of 15 min. The pH value decreased the most in hazelnuts roasted at 80°C for 45 min.
Table 3 show the individual effects and interactions of the parameters year, sample, temperature, and time on the nutritional and chemical composition of hazelnuts and walnuts.
The table summarizes the effects of year, sample type, temperature and time on the nutritional properties of the nuts. It shows that the year significantly influences the fat content, while the sample type mainly influences the protein content. Temperature variations significantly change the ash content, and the interaction between sample type and temperature influences the starch content. Finally, the triple interaction of year, sample type and time has a notable effect on the pH value.
3.3 Fatty acid composition
Table 4 shows the percentage of the fatty acids in each nut, including palmitic acid (C16:0), palmitoleic acid (C16:1), stearic acid (C18:0), oleic acid (C18:1), linoleic acid (C18:2), and linolenic acid (C18:3). The table also shows the total percentage of saturated fatty acids (SFA), monounsaturated fatty acids (MUFA), and polyunsaturated fatty acids (PUFA) in each nut.
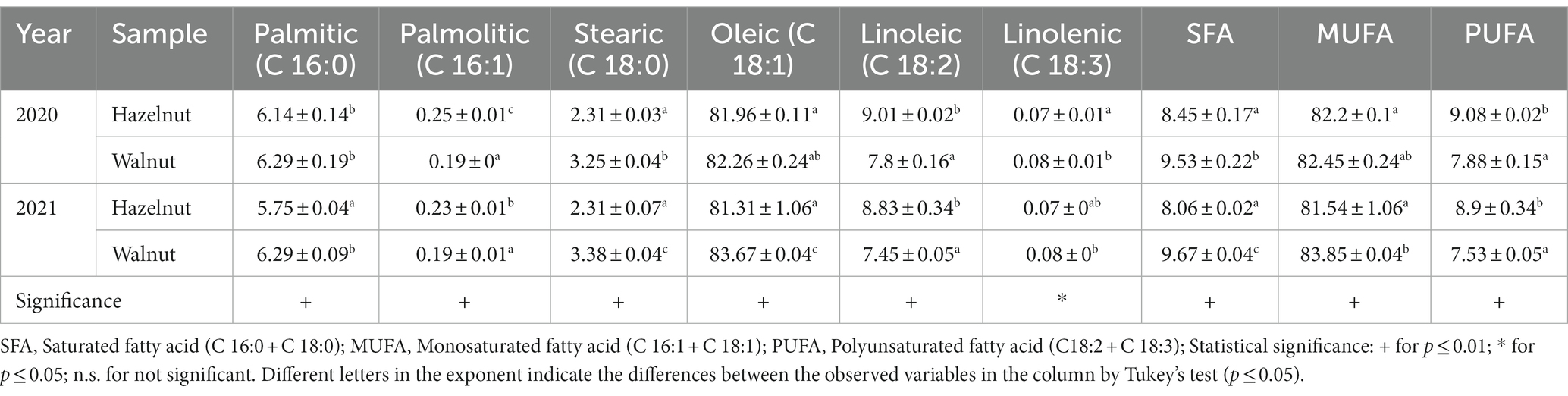
Table 4. Fatty acid composition of fresh samples of hazelnuts and walnuts during 2 years of research.
Figure 3 shows the principal component analysis (PCA) results for the unsaturated and polyunsaturated fatty acids in the nut samples. Principal Component Analysis (PCA) is a widely used dimensionality reduction technique in various fields, providing insights into the underlying structure of complex data sets by transforming the data into a new set of uncorrelated variables (Ringnér, 2008; Abdi and Williams, 2010; Jolliffe and Cadima, 2016). The principal component analysis (PCA) presented in the graph shows the fatty acid profiles of the hazelnut and walnut samples in two main components (PC1 and PC2), which together account for most of the variability in the data. Each sample is represented as a dot, with close dots indicating similar profiles and distant dots indicating different profiles. From the PCA, it is evident that hazelnuts and walnuts have different fatty acid profiles as the corresponding points are on opposite sides of the graph, emphasizing their different nutritional properties. Walnuts are associated with a higher concentration of unsaturated fatty acids — namely oleic acid, linoleic acid and linolenic acid — which is reflected in the alignment with these vectors in the first graph, where PC1 explains 85.37% of the variability. Hazelnuts, on the other hand, show a clustering of saturated and monounsaturated fatty acids (SFA and MUFA), suggesting that these species predominate in their composition. The second graph emphasizes this distinction, with walnuts being closely associated with polyunsaturated fatty acids (PUFA) showing greater variability than the unsaturated profiles, as PC1 covers a significant 91.54% of the variability. This separation and variability emphasizes the unique nutritional characteristics of hazelnuts and walnuts, with little overlap between their clusters, indicating the significant differences in their fatty acid compositions.
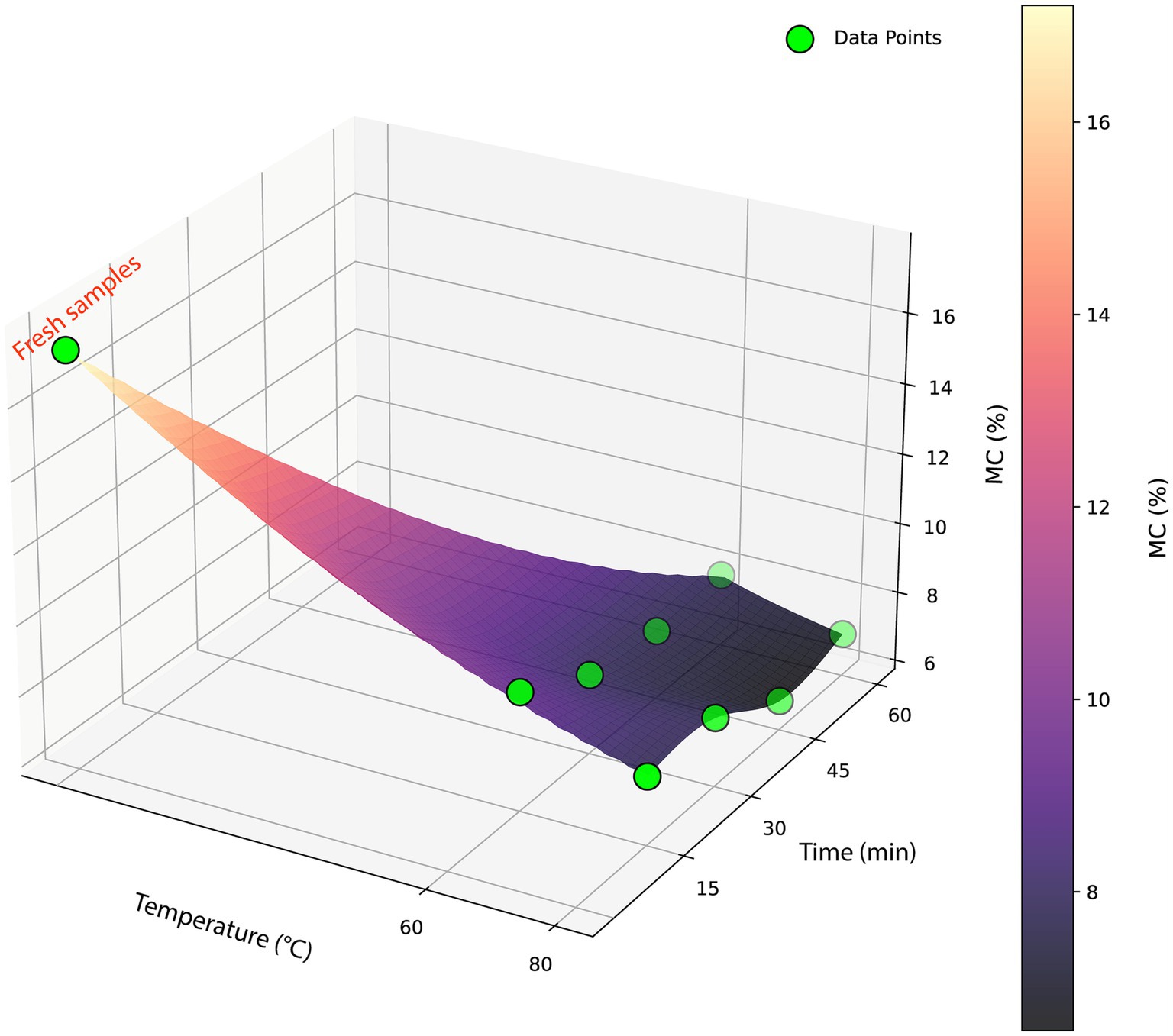
Figure 3. Principal component analysis of fatty acids of fresh samples (A) unsaturated and (B) semi-saturated fatty acids.
Table 5 shows the percentage of the fatty acids in each nut after conduction drying, including palmitic acid (C16:0), palmitoleic acid (C16:1), stearic acid (C18:0), oleic acid (C18:1), linoleic acid (C18:2), and linolenic acid (C18:3). The table also shows the total percentage of saturated fatty acids (SFA), monounsaturated fatty acids (MUFA) and poly-unsaturated fatty acids (PUFA) in each nut.
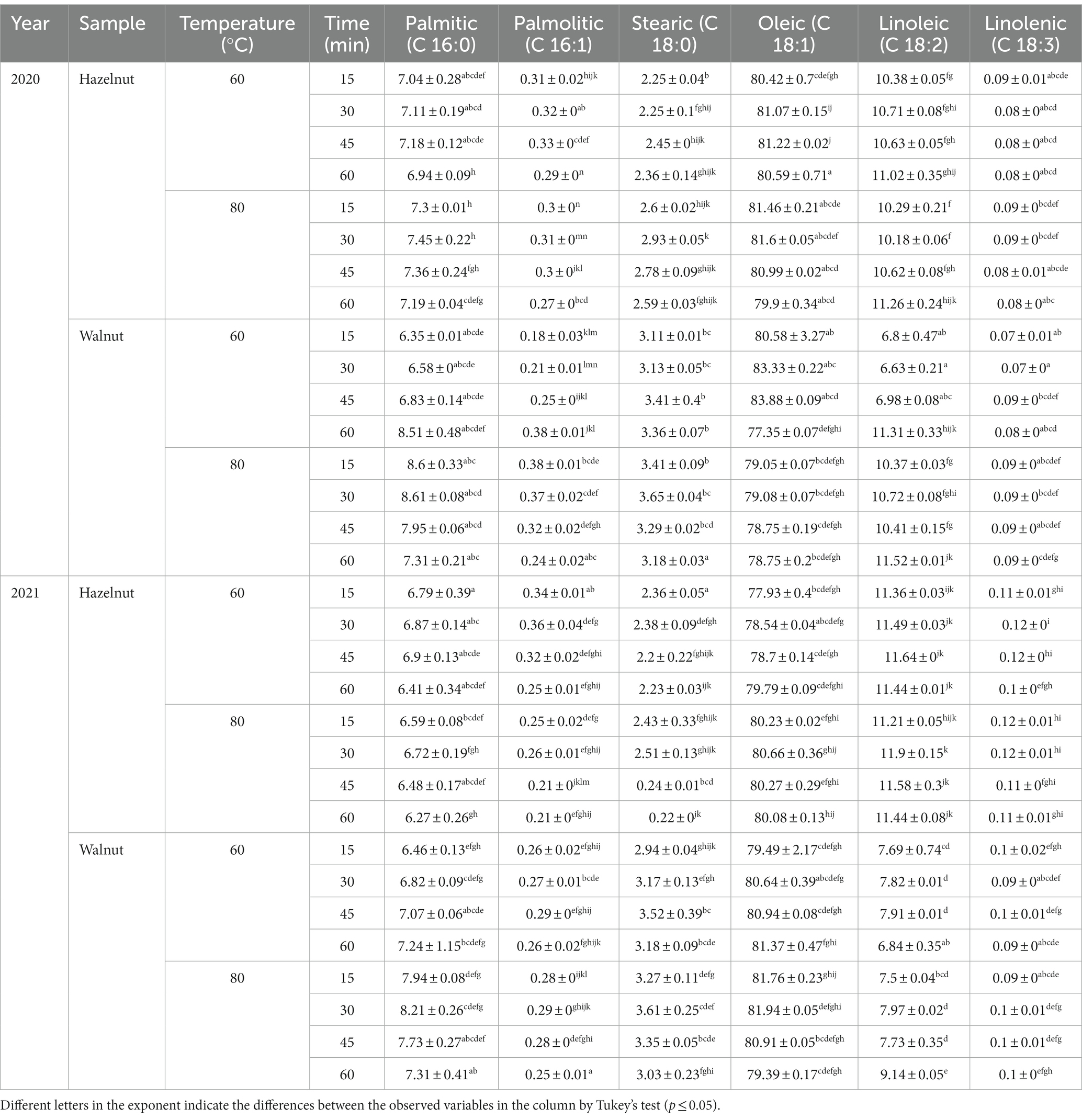
Table 5. Composition of fatty acids in hazelnuts and walnuts over two years after conduction drying.
The effects of drying temperature and drying time on the semi-saturated fatty acids content of hazelnuts and walnuts are shown in Table 6.
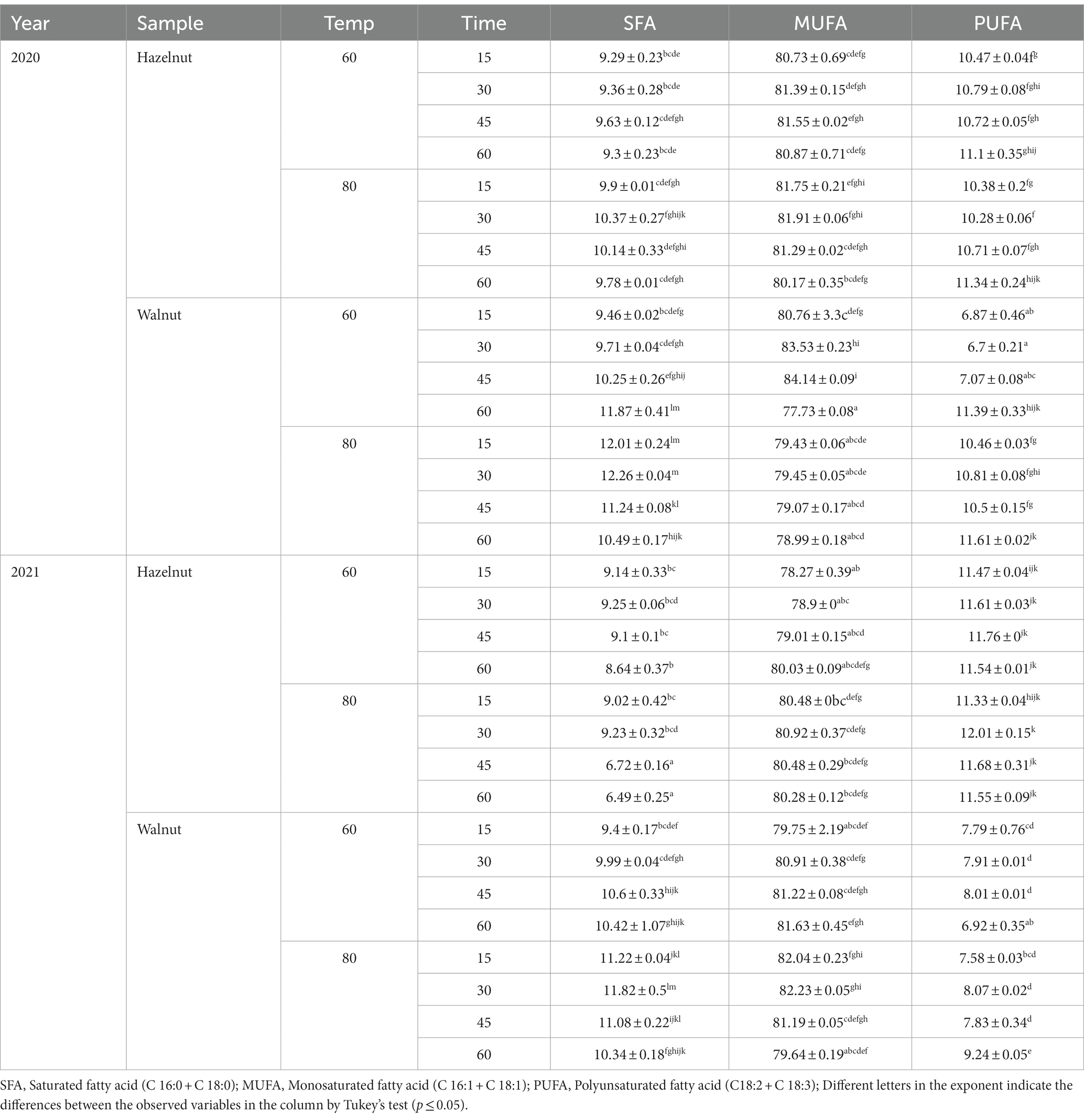
Table 6. Semi-saturated fatty acid profiles in hazelnuts and walnuts over a period of two years after conduction drying.
Table 7 show the individual effects and interactions of the parameters year, sample, temperature, and time on the nutritional and chemical composition of hazelnuts and walnuts.
4 Discussion
This study provides a comprehensive analysis of the nutrient composition and fatty acid profiles of two popular nut species, hazelnuts, and walnuts, over two years. The results highlight the effects of temperature and time variation on nutrient composition and provide valuable insight into the potential effects of environmental conditions on the quality of these nuts. The research also contributes to existing knowledge about the health benefits of these nuts, which are rich in essential nutrients and fatty acids (Ros, 2015).
Moisture content indicates how much water is contained in food. This variable is crucial because it affects the taste, texture, and shelf life of nuts. After drying, it was observed that either an increase in temperature or an increase in duration led to a decrease in moisture content (Elgin Kılıc and Cınar, 2019; Kutlu et al., 2022). Fresh hazelnut samples have a moisture content of 17.16%. After heat treatment at 60°C for 15 min, the moisture content drops to 9.29%. At 60°C, the moisture content decreases further to 7.71% after 30 min and to 7.67% after 60 min. At 80°C, the moisture content decreases further and reaches only 6.67% after 45 min. Fresh walnut samples start with a moisture content of 17.42%. At 60°C and a duration of 15 min, the moisture content drops to 9.11%, parallel to the trends observed for hazelnuts. Interestingly, walnut samples appear to stabilize in their moisture reduction at 7.7–7.8% when treated at 60°C for 45 and 60 min. At 80°C, moisture content drops to 6.58% after 45 min and then increases slightly to 6.95% after 60 min. This slight increase in moisture content could indicate that the drying process is nearing completion or that some reabsorption of moisture is occurring.
Comparative analysis of the nutrient composition of hazelnuts and walnuts over a two-year period revealed several important findings with nutritional implications. The data were consistent with existing literature in some respects, while providing new insights in others. In particular, the protein content of hazelnuts consistently exceeded that of walnuts, with values of 16.81% in 2020 and 16.76% in 2021 for hazelnuts, compared with 14.24% in 2020 and 12.00% in 2021 for walnuts. This higher protein concentration in hazelnuts is in general agreement with the results of Müller et al. (2020), who reported a protein range of 10.2–22.1% in different hazelnut species. A differentiated picture emerged for fat content. Although there were no significant differences, walnuts had a slightly higher fat content (62.83%) than hazelnuts (62.24%) in 2021. The carbohydrate and starch content of hazelnuts was also higher than that of walnuts in both years, complementing existing data from Dodevska et al. (2022) showing generally lower carbohydrate contents in various nuts. Minor but consistent differences were observed in pH value between the two nut species, with hazelnuts having slightly lower pH in both 2020 and 2021. The fatty acid composition of hazelnuts and walnuts demonstrated substantial variability between the years 2020 and 2021, a phenomenon also reported in other tree nut varieties (Taş and Gökmen, 2015; Müller et al., 2020). After analysis of nutrient composition in hazelnuts and walnuts after drying protein content in hazelnuts increased with temperature and time, from 16.4% at 60°C for 15 min to a peak of 18.7% after 60 min when dried at 80°C. In walnuts, the protein content remained relatively stable at different drying conditions, ranging from 11.02 to 15.04%. The fat content of hazelnuts increased significantly from 58.69% at 60°C for 15 min in 2021 to 71.48% at 60°C for 60 min in 2020. A similar trend, although less pronounced, was observed for walnuts, where the fat content increased from 60.97 to 71.02%. Notably, carbohydrates in hazelnuts decreased with increasing drying time and temperature, from 20.28% at 60°C for 15 min to 8.78% at 60°C for 60 min in 2020. In contrast, carbohydrates in walnuts showed a wider range, peaking at 23.72% at 80°C for 45 min in 2021. The starch content of both nut species varied only slightly, ranging from 2.1 to 3.21% at the different drying conditions. However, a significant decrease in pH was observed for hazelnuts, from 6.98 at 60°C for 30 min in 2020 to 6.27 at 80°C for 45 min in 2021.These data suggest that drying conditions have a significant effect on the nutrient composition of hazelnuts and walnuts, which is consistent with previous results demonstrating variability in nutrient composition as a function of environmental conditions (Müller et al., 2020; Dodevska et al., 2022).
In terms of palmitic acid (C16:0), hazelnuts showed a decrease from 6.14% in 2020 to 5.75% in 2021. In contrast, walnuts maintained a nearly constant level of palmitic acid (6.29% in both years). Palmitoleic acid (C16:1) content remained stable across both hazelnut and walnut samples in both years, which corroborates previous literature that shows a limited range of variation in this fatty acid among different cultivars (Müller et al., 2020). Stearic acid (C18:0) showed little fluctuation in hazelnuts but indicated a slight uptick in walnuts from 3.25 to 3.38%. One of the most critical fatty acids, oleic acid (C18:1), showed contrasting trends between the two types of nuts. The oleic acid content in hazelnuts declined marginally from 81.96% in 2020 to 81.31% in 2021, while it increased in walnuts from 82.26 to 83.67%. Similarly, linoleic acid (C18:2) levels showed a modest decline in both types of nuts, though the change was more significant in walnuts than in hazelnuts. These inter-annual variations could be influenced by environmental factors, genetic variability, or even differences in postharvest processing methods, warranting further investigation.
Examining the fatty acid composition of hazelnuts and walnuts roasted at different temperatures (60°C and 80°C) and time intervals revealed other noteworthy patterns. At 60°C, the palmitic acid content in hazelnuts ranged from 6.94 to 7.18%, whereas, at 80°C, it ranged from 7.19 to 7.45%. In walnuts, the range was more extensive, particularly at 60°C, where it ranged from 6.35 to 8.51%.
Oleic acid, one of the predominant fatty acids in these nuts, also demonstrated intriguing behavior. In hazelnuts, the oleic acid content showed minimal variations at both temperatures. However, in walnuts, it ranged from 77.35 to 83.88% at 60°C, showing greater variability than in hazelnuts. Similarly, other fatty acids like stearic acid, linoleic acid, and linolenic acid also showed variations, albeit in a narrower range, across different temperature and time conditions. These findings have significant implications for the health benefits associated with the consumption of hazelnuts and walnuts. Both types of nuts have been recognized for their high levels of monounsaturated fatty acids (MUFA) and polyunsaturated fatty acids (PUFA) (Taş and Gökmen, 2015). The observed fluctuations in the fatty acid composition, therefore, may have potential effects on their nutritional quality. Higher temperatures and drying times resulted in higher levels of monoun-saturated fatty acids and lower levels of polyunsaturated fatty acids for both nuts (Cristofori et al., 2008; Fu et al., 2016; Turan, 2018b). Analysis reveals significant variability in the fatty acid composition (Maguire et al., 2004) of hazelnuts and walnuts based on factors such as the year of harvest, sample type, temperature, and time. Most notably, the Sample and Temperature parameters had the highest influence, with Sample type significantly affecting stearic (C 18:0) and linoleic (C 18:2) acids (p ≤ 0.01), and Temperature notably influencing stearic acid levels (p ≤ 0.05). These findings are corroborated by statistical analysis, as summarized in Table 7, and have important implications for both the storage and utilization of these nuts in food products.
Given the observed data, this study provides indispensable insights into the intricate thermodynamic parameters that are critical for modulating moisture content and nutrient profile in hazelnuts and walnuts during the drying process (Chen and Pan, 2021). Detailed analyzes show that careful modulation of temperature and time during the drying process can lead to significant changes in critical macronutrients such as fats and proteins. This modulation also affects essential fatty acids such as oleic acid and linoleic acid, whose health benefits are well documented (Han et al., 2019; Chen et al., 2020). However, the research is not without limitations. Its focus is narrow and limited to only two nut species and a limited temperature range, calling into question the applicability of these results to other nut species and alternative drying conditions (Noszczyk et al., 2021). In terms of sustainability, the study highlights the fact that optimization of drying parameters can significantly improve energy efficiency. This, in turn, paves the way for more sustainable and resource-efficient practices in the food industry, an area of increasing importance given current climate constraints (Pycia and Juszczak, 2021). These advances are a critical step toward improving energy-efficient production of nutrient-rich foods and contributing to more sustainable agricultural practices.
5 Conclusion
This study conclusively demonstrates the complex effects of drying temperature and duration on the nutrient composition and fatty acid profiles of hazelnuts and walnuts. Both factors are shown to be significant variables affecting moisture content, protein content, and fatty acid composition. Despite contrary opinions, these results are not trivial; the changes in nutrient content can have critical implications for food storage and quality. Statistical validation underscores the pronounced effects of sample type and temperature, particularly on certain fatty acids. Considering that these nuts are often consumed in dried form, understanding, and optimizing these variables are critical for both food processing and quality control. Further studies should investigate the underlying mechanisms and extend these findings to other nut varieties. Thus, this work serves as an indispensable guide for refining drying protocols to maintain the nutritional quality of these widely consumed nuts. The results of this study underline the need for further research to optimize drying processes with the aim of maintaining and improving the nutritional profiles of hazelnuts and walnuts. Future research should focus on the wider applicability of these results to different nut varieties and drying conditions, which could lead to industry-wide improvements in food quality and energy efficiency.
Data availability statement
The original contributions presented in the study are included in the article/supplementary material, further inquiries can be directed to the corresponding author.
Author contributions
AM: Conceptualization, Writing – original draft. IB: Conceptualization, Data curation, Methodology, Writing – original draft. MG: Writing – review & editing. LP: Data curation, Software, Writing – original draft.TK: Validation, Writing – review & editing. BM: Validation, Writing – original draft. VJ: Writing – review & editing. AA: Funding acquisition, Project administration, Validation, Writing – review & editing.
Funding
The author(s) declare financial support was received for the research, authorship, and/or publication of this article. This research was funded by the European Regional Development Fund, under the Operational program competitiveness and cohesion 2014–2022, and project no. KK 01.2.1.02.0286, “Development of innovative pellets from forest and/or agricultural biomass—INOPELET.”
Acknowledgments
This research was supported by the Croatian Science Foundation within the project “Young Re-searchers’ Career Development Project—Training of Doctoral Students,” co-financed by the European Union, under the OP “Efficient Human Resources 2014–2020” from the ESF funds.
Conflict of interest
The authors declare that the research was conducted in the absence of any commercial or financial relationships that could be construed as a potential conflict of interest.
Publisher’s note
All claims expressed in this article are solely those of the authors and do not necessarily represent those of their affiliated organizations, or those of the publisher, the editors and the reviewers. Any product that may be evaluated in this article, or claim that may be made by its manufacturer, is not guaranteed or endorsed by the publisher.
References
Abdi, H., and Williams, L. J. (2010). Principal component analysis. Wiley Interdisc. Rev. Comput. Statis. 2, 433–459. doi: 10.1002/wics.101
Alasalvar, C., Pelvan, E., and Topal, B. (2010). Effects of roasting on oil and fatty acid composition of Turkish hazelnut varieties (Corylus avellana L.). Int. J. Food Sci. Nutr. 61, 630–642. doi: 10.3109/09637481003691820
ANON (1990). “Official methods of analysis” in Association of Official Analytical Chemists. ed. K. Herrich. 15th ed (Arlington, Virginia: ANON)
AOAC International. (2011). Official methods of analysis of AOAC international, 18 Washington: Gaithersburg.
Association of Official Analytical Chemists (AOAC), Official methods of analysis of AOAC international, 17th. Gaithersburg, MD: AOAC International (2000).
Cap, S., Bots, P., and Scherer, L. (2022). Environmental, nutritional and social assessment of nuts. Sustain. Sci. 18, 933–949. doi: 10.1007/s11625-022-01146-7
Carey, A. N., Fisher, D. R., Bielinski, D. F., Cahoon, D. S., and Shukitt-Hale, B. (2020). Walnut-associated fatty acids inhibit LPS-induced activation of BV-2 microglia. Inflammation 43, 241–250. doi: 10.1007/s10753-019-01113-y
CEN/TS 14774-2:2004. (2004). Solid biofuels - methods for the determination of moisture content - oven dry method - part 2: Total moisture
Chen, C., and Pan, Z. (2021). Heat and moisture transfer studies on walnuts during hot air drying in a fixed-bed column dryer. Appl. Therm. Eng. 199:117554. doi: 10.1016/j.applthermaleng.2021.117554
Chen, C., Venkitasamy, C., Zhang, W., Deng, L., Meng, X., and Pan, Z. (2020). Effect of step-down temperature drying on energy consumption and product quality of walnuts. J. Food Eng. 285:110105. doi: 10.1016/j.jfoodeng.2020.110105
Cristofori, V., Ferramondo, S., Bertazza, G., and Bignami, C. (2008). Nut and kernel traits and chemical composition of hazelnut (Corylus avellana L.) cultivars. J. Sci. Food Agric. 88, 1091–1098. doi: 10.1002/jsfa.3203
da Silva Simão, R., de Moraes, J. O., Monteiro, R. L., Schaidt, A. L., Carciofi, B. A. M., and Laurindo, J. B. (2021). Conductive drying methods for producing high-quality restructured pineapple-starch snacks. Innov. Food Sci. Emerg. Technol. 70:102701. doi: 10.1016/j.ifset.2021.102701
Dodevska, M., Kukic Markovic, J., Sofrenic, I., Tesevic, V., Jankovic, M., and Djordjevic, B. (2022). Similarities and differences in the nutritional composition of nuts and seeds in Serbia. Front. Nutr. 9:1003125. doi: 10.3389/fnut.2022.1003125
Elgin Kılıc, E., and Cınar, B. (2019). Convective hot air drying characteristics of selected vegetables. Int. Adv. Res. 3, 361–368.
Fu, M., Qu, Q., Yang, X., and Zhang, X. (2016). Effect of intermittent oven drying on lipid oxidation, fatty acids composition and antioxidant activities of walnut. LWT-Food Sci. Technol. 65, 1126–1132. doi: 10.1016/j.lwt.2015.10.002
Han, Y., Zheng, Y., Li, S., Mo, R., Long, X., and Liu, Y. (2019). Effects of drying process with different temperature on the nutritional qualities of walnut (Juglans regia L.). Food Sci. Technol. Res. 25, 167–177. doi: 10.3136/fstr.25.167
Harris, C. R., Millman, K. J., van der Walt, S. J., Gommers, R., Virtanen, P., Cournapeau, D., et al. (2020). Array programming with NumPy. Nature 585, 357–362. doi: 10.1038/s41586-020-2649-2
HRN EN ISO 5509:2004 (2004). Animal and vegetable fats and oils -- preparation of methyl esters of fatty acids (ISO 5509:2000; EN ISO 5509:2000)
Hunter, J. D. (2007). Matplotlib: a 2D graphics environment. Comp. Sci. Eng. 9, 90–95. doi: 10.1109/MCSE.2007.55
Jolliffe, I. T., and Cadima, J. (2016). Principal component analysis: a review and recent developments. Philos. Trans. R. Soc. A Math. Phys. Eng. Sci. 374:20150202. doi: 10.1098/rsta.2015.0202
Kutlu, K. N., Elbir, T., Yilmaz, Ö., and Kamiloğlu, A. (2022). Effect of different roasting methods on some properties of walnut kernels. Erzincan Üniversitesi Fen Bilimleri Enstitüsü Dergisi 15, 65–74. doi: 10.18185/erzifbed.1202053
Li, H., Niu, X., Chai, J., Guo, C., Sun, Y., Li, J. M., et al. (2021). Optimization of hot air-drying process for tiger nut and analysis of fatty acid composition of tiger nut oil. Int. J. Agric. Biol. Eng. 14, 228–236. doi: 10.25165/J.IJABE.20211406.6646
Liu, D., Zhang, C., Pu, Y., Chen, S., Liu, L., Cui, Z., et al. (2022). Recent advances in pH-responsive freshness indicators using fresh food colorants to monitor food freshness. Food Secur. 11:1884. doi: 10.3390/foods11131884
Maguire, L. S., O'Sullivan, S. M., Galvin, K., O'Connor, T. P., and O'Brien, N. M. (2004). Fatty acid profile, tocopherol, squalene and phytosterol content of walnuts, almonds, peanuts, hazelnuts, and the macadamia nut. Int. J. Food Sci. Nutr. 55, 171–178. doi: 10.1080/09637480410001725175
McKinney, W. (2010). Data structures for statistical computing in python. In Proceedings of the 9th Python in Science Conference.
Mechlouch, R. F., Elfalleh, W., Ziadi, M., Hannachi, H., Chwikhi, M., Aoun, A. B., et al. (2012). Effect of different drying methods on the physico-chemical properties of tomato variety Rio Grande. Int. J. Food Eng. 8. doi: 10.1515/1556-3758.2678
Müller, A. K., Helms, U., Rohrer, C., Möhler, M., Hellwig, F., Glei, M., et al. (2020). Nutrient composition of different hazelnut cultivars grown in Germany. Food Secur. 9, 1–11. doi: 10.3390/foods9111596
Nishi, S. K., Kendall, C. W. C., Bazinet, R. P., Bashyam, B., Ireland, C. A., Augustin, L. S. A., et al. (2014). Nut consumption, serum fatty acid profile and estimated coronary heart disease risk in type 2 diabetes. Nutr. Metab. Cardiovasc. Dis. 24, 845–852. doi: 10.1016/j.numecd.2014.04.001
Nkwonta, C. G., Alamar, M. C., Landahl, S., and Terry, L. A. (2016). Effects of postharvest storage and processing techniques on the main fatty acids in the profile of oil extracted from African walnut (Tetracarpidium conophorum Mull. Arg.). J. Food Compos. Anal. 45, 87–94. doi: 10.1016/j.jfca.2015.10.001
Nogales-Bueno, J., Baca-Bocanegra, B., Hernández-Hierro, J. M., Garcia, R., Barroso, J. M., Heredia, F. J., et al. (2021). Assessment of Total fat and fatty acids in walnuts using near-infrared hyperspectral imaging. Front. Plant Sci. 12:729880. doi: 10.3389/fpls.2021.729880
Noszczyk, T., Dyjakon, A., and Koziel, J. A. (2021). Kinetic parameters of nut shells pyrolysis. Energies 14, 1–22. doi: 10.3390/en14030682
Oliveira, I., Sousa, A., Morais, J. S., Ferreira, I. C., Bento, A., Estevinho, L., et al. (2008). Chemical composition, and antioxidant and antimicrobial activities of three hazelnut (Corylus avellana L.) cultivars. Food Chem. Toxicol. 46, 1801–1807. doi: 10.1016/j.fct.2008.01.026
Phatanayindee, S., Borompichaichartkul, C., Srzednicki, G., Craske, J., and Wootton, M. (2012). Changes of chemical and physical quality attributes of Macadamia nuts during hybrid drying and processing. Dry. Technol. 30, 1870–1880. doi: 10.1080/07373937.2012.703275
Pycia, K., and Juszczak, L. (2021). The influence of the addition of nuts on the thermal and rheological properties of wheat flour. Molecules 26, 1–15. doi: 10.3390/molecules26133969
Ringnér, M. (2008). What is principal component analysis? Nat. Biotechnol. 26, 303–304. doi: 10.1038/nbt0308-303
Tan, S. Y., Tey, S. L., and Brown, R. (2018). Can nuts mitigate malnutrition in older adults? A conceptual framework. Nutrients 10, 1–15. doi: 10.3390/nu10101448
Taş, N. G., and Gökmen, V. (2015). Profiling triacylglycerols, fatty acids and tocopherols in hazelnut varieties grown in Turkey. J. Food Compos. Anal. 44, 115–121. doi: 10.1016/j.jfca.2015.08.010
Turan, A. (2018a). Effect of drying methods on fatty acid profile and oil oxidation of hazelnut oil during storage. Eur. Food Res. Technol. 244, 2181–2190. doi: 10.1007/s00217-018-3128-y
Turan, A. (2018b). Effect of drying methods on nut quality of hazelnuts (Corylus avellana L.). J. Food Sci. Technol. 55, 4554–4565. doi: 10.1007/s13197-018-3391-8
Keywords: conduction drying, nutritional properties, fatty acid profile, hazelnuts (Corylus avellana), walnuts (Juglans regia), thermal treatment, fatty acids
Citation: Matin A, Brandić I, Gubor M, Pezo L, Krička T, Matin B, Jurišić V and Antonović A (2024) Effect of conduction drying on nutrient and fatty acid profiles: a comparative analysis of hazelnuts and walnuts. Front. Sustain. Food Syst. 8:1351309. doi: 10.3389/fsufs.2024.1351309
Edited by:
Poonam Rani, Teagasc Food Research Centre (Ireland), IrelandReviewed by:
Claudia Terezia Socol, University of Oradea, RomaniaSeydi Yıkmış, Namik Kemal University, Türkiye
Copyright © 2024 Matin, Brandić, Gubor, Pezo, Krička, Matin, Jurišić and Antonović. This is an open-access article distributed under the terms of the Creative Commons Attribution License (CC BY). The use, distribution or reproduction in other forums is permitted, provided the original author(s) and the copyright owner(s) are credited and that the original publication in this journal is cited, in accordance with accepted academic practice. No use, distribution or reproduction is permitted which does not comply with these terms.
*Correspondence: Ivan Brandić, aWJyYW5kaWNAYWdyLmhy