- 1Environmental and Soil Microbiology Laboratory, Pontificia Universidad Javeriana, Bogotá, Colombia
- 2Instituto Nacional de Tecnología Agropecuaria, Córdoba, Argentina
- 3Centro Nacional de Recursos Genéticos, Instituto Nacional de Investigaciones Forestales, Agrícolas y Pecuarias, Boulevard de la Biodiversidad, Rancho las Cruces, Tepatitlán de Morelos, Jalisco, Mexico
- 4Instituto de Agrobiotecnologia y Biologia Molecular, IABIMO Conicet-INTA, Buenos Aires, Argentina
- 5Universidad de los Andes, Bogotá, Colombia
Fungi are essential players in the maintenance of global coffee productivity, but their taxonomic and functional diversity in tropical and subtropical soils of Latin America remains largely unexplored. To address this concern, soil fungi were surveyed in six farms in three traditional coffee-growing regions of Colombia (Cauca, Magdalena, and Risaralda). Five farms were organic and newly established (<1 to 15 years) with low shade, and one farm was under long-term conventional management (>30 years old) with higher shade cover. We used amplicon sequencing and functional prediction based on the FUNGuild annotation tool. Fungal community composition diverged among farms, with Mortierella (Mortierellomycota) and Saitozyma (Basidiomycota) among the most prevalent genera. Functional prediction revealed the predominance of saprotroph-symbiotroph and pathotroph fungi. The endophyte and litter decomposer Mortierella genus was dominant within the saprotrophs and symbiotrophs. The pathotroph community was characterized by insect pathogen species belonging to the Metarhizium (Ascomycota) genus. Indeed, M. anisopliae and M. marquandii were identified as indicator species in the conventional long-term shaded farm. This study revealed that coffee plantations studied sustain a diverse fungal community and nurture potentially beneficial species. Further studies are needed to elucidate how particular management practices can nourish beneficial fungi, suppress detrimental species, and promote more sustainable coffee production.
1 Introduction
Coffee (Coffea arabica L.—Rubiaceae) is the second most valuable commodity in the world after oil. It is a major crop in many countries in Latin America, Africa and Asia. Colombia is the third-largest global coffee producer after Brazil and Vietnam (de Oliveira Junqueira et al., 2019). Colombian coffee cultivation produces income that accounts for 15% of the agricultural GDP and employs 2.5 million people annually (Cano-Sánz et al., 2013). The success of coffee cultivation in Colombia is mainly due to rich volcanic-ash soil, abundant annual rainfall (200 mm per month on average), and high altitudes (1,800–2,000 m) where the Arabic coffee grows (Lara-Estrada and Vaast, 2005). However, such conditions overlap with areas of enormously rich biodiversity (Komar, 2006). Consequently, coffee represents a promising crop in the described environment but entails an undeniable impact on local and global biodiversity (Jha et al., 2014).
Different cropping strategies for coffee cultivation have a particular impact on biodiversity (Moguel and Toledo, 1999). Agroforestry, also known as shade-grown management, supports coffee plantations in the understory of native and exotic trees. Diversified shade management has been the basis of traditional coffee plantations and offers a refuge for biodiversity, such as arthropods, mammals, amphibians and birds (De Beenhouwer et al., 2013; Perfecto et al., 2014), with tree leaf litter being an important source of carbon that modulates soil biota structure and functions (Rao et al., 2020). Coffee farm management is gradually shifting away from shade-grown management in favor of sun-intensive, higher-yield farms (DaMatta, 2004; Bravo-Monroy et al., 2016; Velmourougane and Bhat, 2017; Harvey et al., 2021). Compared to the available information about the impact of coffee cultivation on aboveground diversity, relatively little is known about belowground microbial diversity.
Among soil-borne microorganisms, soil fungi regulate key ecosystem processes, including plant productivity and carbon mineralization and sequestration. They are essential decomposers, mutualists, and pathogens (Webster and Weber, 2006). Certain pathogenic and mutualistic fungi have been extensively studied in coffee (Lovera et al., 2022; Lu et al., 2022b). For example, in the period from 2008 to 2011, until the appearance of resistant cultivars, Hemileia vastatrix caused a massive outbreak of coffee leaf rust in C. arabica plantations with significant economic losses (Cristancho et al., 2012; Talhinhas et al., 2017; Gichuru et al., 2021). On the other hand, the mutualistic association between coffee and arbuscular mycorrhizal fungi (AMF) has been well documented (Urgiles-Gómez et al., 2021; Lovera et al., 2022). Nevertheless, there is scarce information about overall soil fungal communities and their ecological functions, which are of utmost importance for sustainable crop management (Duong et al., 2020; Rao et al., 2020).
Soil fungal responses are context-dependent, and local conditions determine the resultant assemblage and functions of fungal communities (Lekberg et al., 2021; Wang et al., 2021; Tedersoo et al., 2022). For example, fungal diversity showed high sensitivity to the legacy effects of land uses in the past (Turley et al., 2020; Correia et al., 2021) and along altitudinal gradients (Ogwu et al., 2019). In coffee plantations, earlier studies revealed that the soil fungal community is strongly influenced by agronomical management (i.e., conventional vs. organic) and the canopy composition in agroforestry systems on Nicaraguan farms (Jurburg et al., 2020), while altitude, the regional floristic domains and Coffea species were the main drivers of fungal diversity on Brazilian farms (Veloso et al., 2020, 2023). However, in situ surveys of soil fungi are missing in important and traditional coffee-growing regions of Colombia. Notably, unsustainable farm practices can favor pathogenic species (Jurburg et al., 2020; Rao et al., 2020), and impoverish the pool of mutualistic fungi from which plant roots can be colonized (Brinkmann et al., 2019; Prates Júnior et al., 2019; Lovera et al., 2022). In addition, valuable agents against pathogens and pests in coffee plantations, such as entomopathogenic and endophyte fungal species, are also sensitive to management practices (Vega et al., 2008; Duong et al., 2020; Bayman et al., 2021).
This study aimed to identify and describe the community composition and ecological functions of soil fungi in coffee farms in three traditional coffee-growing regions of Colombia. We conducted our study in six farms with divergent management practices (one conventional and five organic), which also varied in shade management, the plantation age and environmental properties. Certainly, we acknowledge that the multiplicity of conditions that characterize the farms across the studied regions can determine the existing fungal community (Lekberg et al., 2021; Wang et al., 2021; Tedersoo et al., 2022). For this reason, we focused on the identification of abundant genera, indicator species, and functional guilds. Despite the aforementioned sampling limitations, this investigation offers insight into the soil fungal taxonomy and ecological functions and provides an empirical baseline supporting the implementation of sustainable agricultural practices in Colombian coffee plantations.
2 Materials and methods
2.1 Description of the study area
The experimental sites were located in three Colombian coffee-growing regions: Cauca (Cau), Risaralda (Ris), and Magdalena (Mag) (Figure 1). The Cauca and Risaralda regions were characterized by Andosols while Acrisols prevailed in the Magdalena region (IUSS Working Group WRB, 2022, adapted from IGAC, 2022). In Cauca, the farm was located in the town of El Tambo. The region is part of the southwestern Andean mountains of Colombia, characterized by diverse topography and favorable edaphic and climatic conditions for producing top-tier coffee (Rekik et al., 2018). In Risaralda, the surveyed farms were located in the town of Quinchia, in the central Andean mountains. Risaralda stands as the preeminent coffee-producing region in Colombia, commonly denominated as the ‘Eje Cafetero’ or Coffee Central Axis. For over a century and a half, this expansive territory has played a pivotal role as the principal epicenter of coffee cultivation in Colombia. Risaralda’s abundant rainfall and fertile soils contribute to the production of high-quality Arabica coffee beans (García et al., 2014; Velandia-Silva, 2017). In Magdalena, the farms were in the town of Minca, in the northern part of the country. It harbors diverse ecosystems, including coastal areas, mountains, and tropical forests that differentiate it from any other coffee-producing area in Colombia (García et al., 2014). In Magdalena, studied coffee farms were small (less than one hectare) with low-tech coffee management.
The six coffee farms surveyed differed in the use of agrochemicals [conventional (1) vs. organic (5)] and shade management [low (3) vs. higher shade cover (3)] (Table 1). Cordia alliodora, Inga densiflora, and Eucalyptus grandis were the most prevalent tree species in shaded coffee plantations. In the organic farms certified by the National Coffee Federation, nutrient are replenished through the application of homemade organic amendments produced on-site and the cultivation of beneficial legumes. Pesticides for pest and disease control are prohibited. In the surveyed conventional farm, synthetic chemicals are used for fertilization, as well as for pest and weed control.
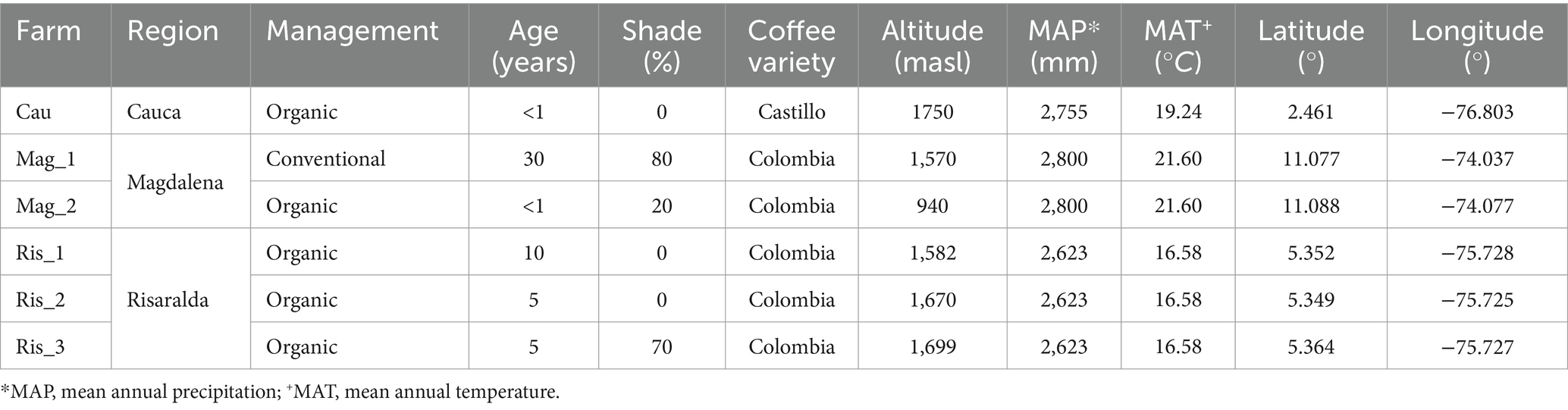
Table 1. Description of study sites (i.e., farms) belonging to three traditional coffee regions of Colombia located in Cauca (Cau), Magdalena (Mag_1; Mag_2), and Risaralda (Ris_1; Ris_2; Ris_3).
2.2 Soil sampling
The samples were collected between July and August 2021. Before collection, the surface of each sampling area was manually cleaned to remove organic material such as leaf litter, branches, stems of plants, inorganic debris, etc. In each farm, one transect of 500 plants was selected and five plants, each separated by 20 coffee shrubs, were sampled. A sample of 50–150 g of soil was collected using a sterile metal spatula at a depth of 0–20 cm and 30 cm from each coffee trunk. Samples were stored in sterile Ziploc-type plastic bags (4°C) and transported to the Laboratory of Molecular Interactions of Microorganisms in Agriculture (LIMMA), Universidad de Los Andes, for further processing.
2.3 Soil properties
Soil sampling for physicochemical analyses were performed in one composite sample per farm at the Soil and Foliar Laboratory, Universidad Tecnológica de Pereira, Colombia. In Mag_1 and Mag_2 farmers carried out sampling. Unfortunately, due to circumstances beyond our control, we were unable to conduct physicochemical analyses of the Mag_2 farm because the farmer did not provide the soil sample. In the remaining sites, pH was measured with a potentiometer in water (1:1), organic matter (OM) with the Walkley-Black photometric method, and electrical conductivity (EC) in paste saturated with water using a conductivity meter. Iron (Fe), manganese (Mn), zinc (Zn), and copper (Cu) ions were determined by the atomic absorption technique (acetate+EDTA). Boron (B) was determined by extraction with monocalcium phosphate, azomethine H., and photometric detection; sulfur (S) was determined by the turbidimetric method after extraction with monocalcium phosphate. Phosphorus (P) was analyzed using the Bray II photometric method. Texture was determined by Bouyoucos, and exchangeable acidity was determined by the volumetric method under KCl extraction. The bases – potassium (K), calcium (Ca), magnesium, (Mg), and sodium (Na) – were analyzed by the ammonium acetate method, using atomic absorption. Finally, cation exchange capacity (CEC) was quantified by atomic absorption spectrophotometry.
2.4 DNA extraction
Molecular identification of soil fungi was conducted using five soil replicates per individual farm. DNA was extracted from 250 mg of fresh soil using DNeasy® PowerSoil® Pro-Kit (Qiagen), following the manufacturer’ recommendations. DNA quality and quantity were checked by 1% agarose gel electrophoresis, and spectrophotometry was conducted using the NanoDrop™ 2000 (Thermo Fisher Scientific™). The DNA samples were stored at - 80°C until further processing.
2.5 Library preparation and high throughput sequencing
Amplification was performed using fungal-specific primers ITS3_KyO2F (5’-GATGAAGAACGYAGYRAA-3′) and ITS4R (5’-TCCTCCGCTTATTGATATGC-3′) targeting the internal transcribed spacer (ITS2) region (Toju et al., 2012). Library preparation and high-throughput, pair-end sequencing were performed on the Illumina MiSeq PE250 platform of the Genomics Unit of the IABIMO (INTA, Hurlingham, Buenos Aires, Argentina).
2.6 Bioinformatics analysis
Bioinformatics processing of the reads was performed with QIIME 2 version 2022.2 (Bolyen et al., 2019). Illumina adapters and primers were trimmed with the cutadapt function of QIIME2 pipeline. Next, denoising processing was performed using DADA2 version 2022.8.0 (Callahan et al., 2016). Amplicons with more than 100 bp were retained and amplicon sequence variants (ASVs) were generated. The taxonomic assignment of each ASV was annotated using the q2-classify-sklearn module against UNITE 8.3 database (Nilsson et al., 2019). Functional guilds were assigned at the genus level using the annotation tool FUNGuild (Nguyen et al., 2016). Only ‘highly probable’ and ´probable’ confidence rankings were considered for further analyses. Among the total number of ASVs, 75.2% were assigned to a specific functional group.
2.7 Statistical analysis
Sequencing efficacy was assessed with rarefaction analysis using the rarefy function from the R package VEGAN (Oksanen et al., 2015). For further analyses, the data matrix was standardized by rarefaction to the minimum read count per sample (12,174). This approach, which consists of randomly selecting reads in each sample until the minimum read count is reached, is optimal for reducing bias due to differences in sample size while retaining information (De Cárcer et al., 2011). Alpha diversity indexes were calculated using the diversity function from the R package VEGAN (Oksanen et al., 2015). Differences in alpha diversity indexes among farms were tested using Tukey post hoc analyses with Bonferroni correction after Kruskal-Wallis tests from the R package STATS (R Development Core Team, 2022). The effect of farms on soil fungal community composition was assessed by permutational multivariate analysis of variance (Anderson, 2001) using the adonis function with 9,999 permutations from the R package VEGAN (Oksanen et al., 2015). Variation in fungal taxonomic community composition was visualized by non-metric multidimensional scaling (three-dimensional NMDS, with 50 iterations) using the metaMDS function and Bray-Curtis distance from VEGAN package (Oksanen et al., 2015). We plotted ellipses representing communities belonging to the different farms with the ordiellipse function using the standard deviations of weighted averages (Oksanen et al., 2015). To identify fungal ASVs associated with a specific farm, we used indicator species analysis (Dufrêne and Legendre, 1997) as implemented by function indval from the R package LABDSV (Roberts, 2012). Only those ASVs with an indicator value of at least 0.25 were considered. To identify abundant genera, we detected those genera with ≥0.005 relative abundance per sample and present in at least 25% of sampling sites. Differences in the abundance of genera, phyla, and fungal trophic mode among farms were tested as described for alpha diversity indexes.
3 Results
3.1 Soil properties
Overall, soils had low nutrient content, considerably high levels of organic matter, and coarse textures with a predominance of sand among soil textural particles. Soils were characterized by moderate water retention (Supplementary Table S1), low cation exchange capacity (CEC), low P availability (except for Ris_1) and poor base saturation. The cationic complex was dominated by acidic metallic components, namely Fe and Al (Table 1 and Supplementary Table S1).
3.2 Sequencing
A total of 5,034,648 ITS sequences/reads were obtained from 27 out of 30 samples submitted for sequencing. Three samples of Cau and one of Ris_1 failed in the sequencing procedure. After cleaning and denoising processes, 316,524 reads were retained and 456 fungal ASVs were identified. All samples reached the asymptote when the number of reads was 12,174 (see Supplementary Figure S1). The raw reads were submitted to the European Nucleotide Archive and are available from BioProject PRJEB51624.
3.3 Alpha diversity
Alpha diversity indexes varied widely among farms with significant differences in observed richness and Shannon indexes (Table 2). The range of observed richness (ASVs) was around 30.6 (Mag_2) and 107.2 (Mag_1). Mag_1 presented the highest value of Shannon index with an average of 2.97 whereas the range presented by the other farms ranged from 0.89 (Mag_2) to 1.94 (Ris_2). Farms did not exhibit differences in evenness of fungal species, according to the invSimpson index (Table 2).
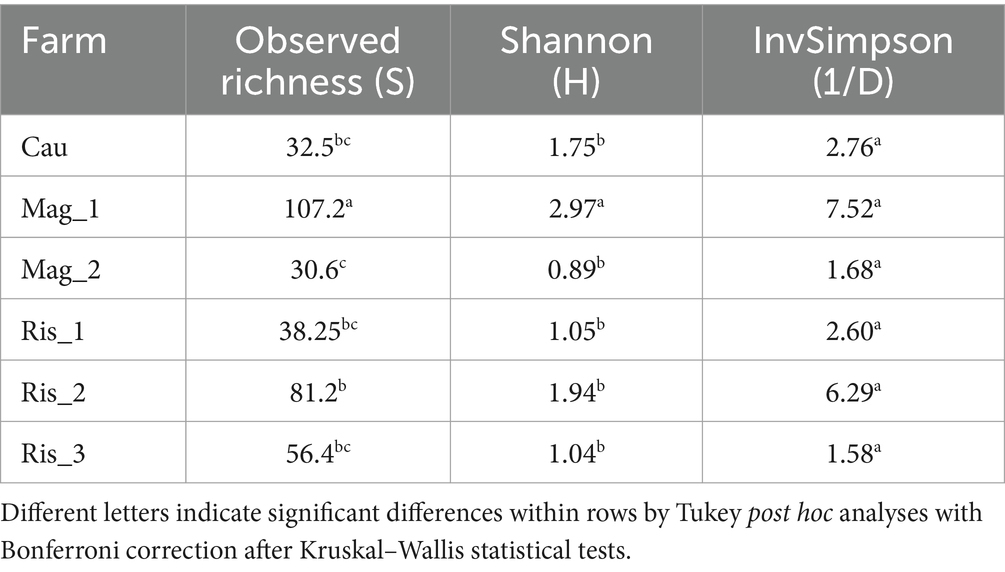
Table 2. Observed richness, Shannon diversity and inverse of the Simpson indexes based on the taxonomic diversity of soil fungi.
3.4 Community composition
Composition differences in soil fungal communities were assessed across farms based on the Bray-Curtis distances between samples [PERMANOVA, df = 5, R2 = 0.6221, p < 0.001]. This finding is further illustrated in the NMDS plot, where Mag_1 and Mag_2 formed separate and less dispersed groups onto the ordination plot (Figure 2).
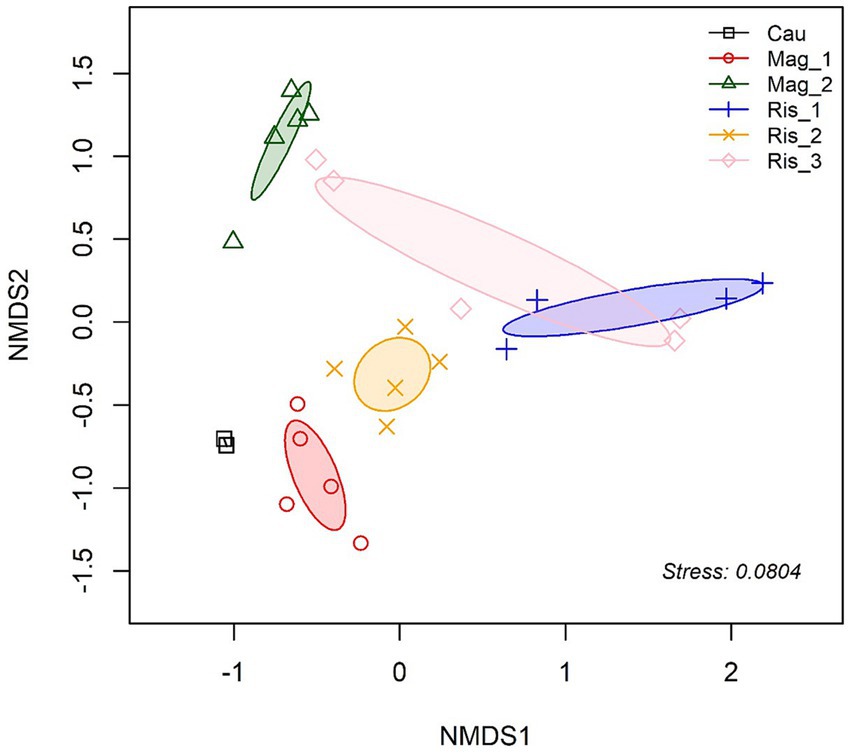
Figure 2. Non-metric multidimensional scaling (NMDS) ordination of fungal community composition based on Bray-Curtis distance (k = 2, stress 0.08). Ellipses indicate one SD around group centroids of each farm.
Indicator species analysis revealed that 21 fungal ASVs were distinctive of a particular farm (Table 3). Thirteen out of the 21 indicator fungal ASVs were identified at the species level. Notably, Cau and Mag_1 exhibited the highest number of indicator species, including pathotrophs, saprotrophs-symbiotrophs and pathotrophs-saprotrophs. Mag_2 was characterized by the saprotroph-symbiotroph Mortierella elongata (Table 3). The saprotroph Ascobolaceae and the pathotroph-saprotroph Septoriella sp. were indicators of Ris_1; while Ris_2 did not show any indicator species and the pathotroph-saprotroph Cordana bisbyi was an indicator of Ris_3 (Table 3).
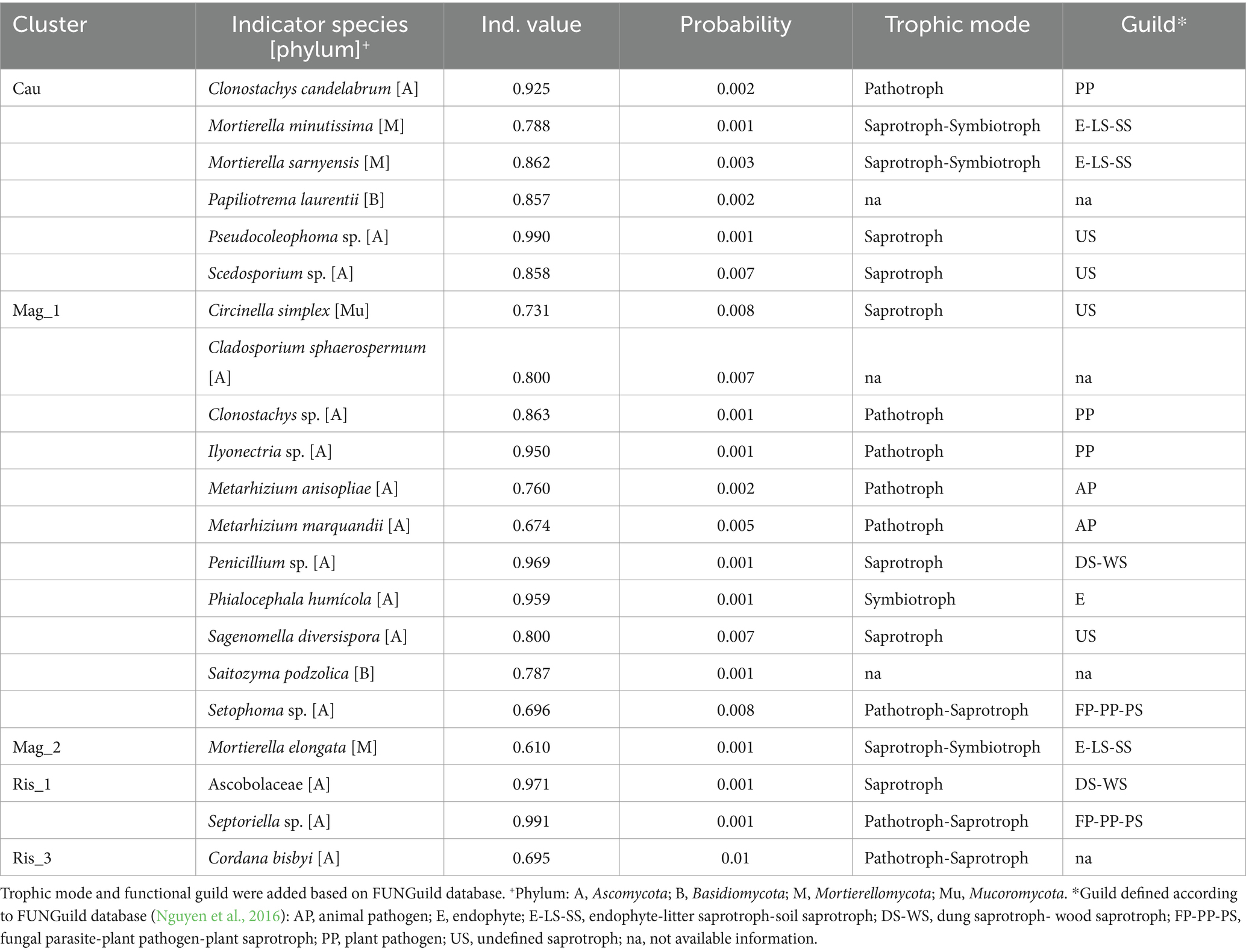
Table 3. Indicator species analysis showing characteristic fungal species (indicator value >0.25) of studied coffee farms.
Overall, 11 phyla were identified, and Mortierellomycota, Basidiomycota, and Ascomycota were the most abundant (Figure 3). The per sample abundance distribution and statistical comparisons among farms are presented in Supplementary Figure S2 and Supplementary Table S2, respectively. Mortierellomycota was dominant in Ris_3 (88.4%) and Mag_2 (88.2%) and less abundant in Mag_1 (16.6%) (Supplementary Table S2). Basidiomycota was mainly found in Mag_1 (43.8%) with a low abundance in Mag_2 and Ris_3 (both around 0.1%). Ascomycota was predominant in Mag_1 (33.7%), and rare in Ris_1 (0.7%) and Mag_3 (0.2%) (Supplementary Table S2). Chytridiomycota, Glomeromycota, Kickxellomycota, and Mucoromycota were mainly found in Mag_1, and Rozellomycota was detected in Cau (Supplementary Figure S2 and Supplementary Table S2).
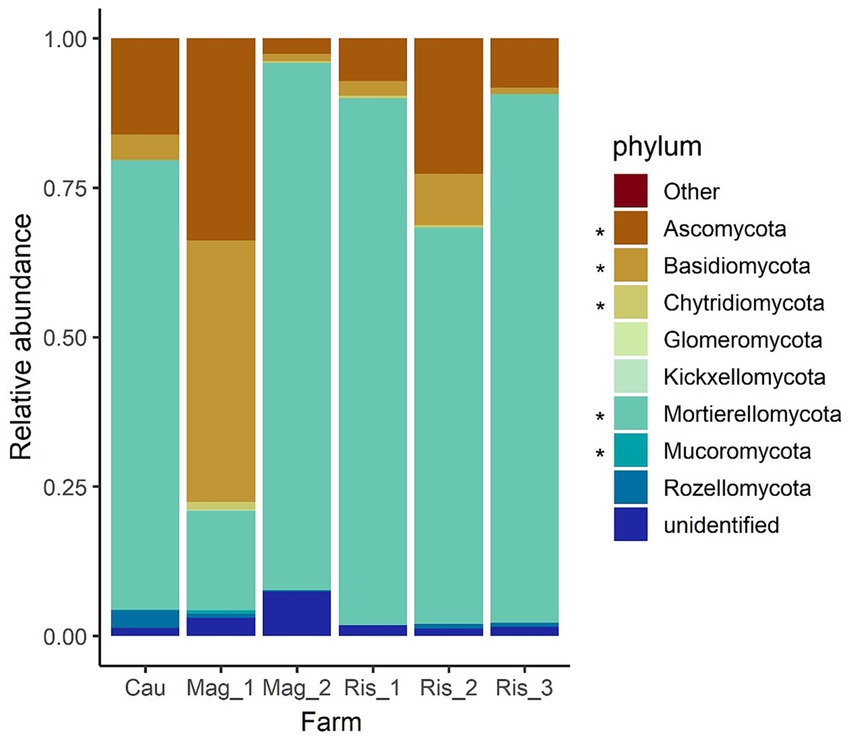
Figure 3. Relative abundances of soil fungal phyla in coffee farms. “Other” indicates the relative abundance of minor phyla Aphelidiomycota and Zoopagomycota. “Unidentified” indicates the relative abundance of ASVs belonging to Fungi but not associated with further taxonomic groups. Asterisks (*) indicate significant difference in phylum abundance according to Tukey post-hoc analyses with Bonferroni correction after Kruskal–Wallis statistical tests (Supplementary Table S2).
3.5 Abundant genera
Six genera were identified as predominant among the coffee farms (Figure 4). By far, Mortierella (Mortierellomycota) was the most abundant genus, reaching up to 88% abundance, followed by Saitozyma (Basidiomycota) with 30% abundance in Mag_1 (p < 0.001). Metarhizium (Ascomycota) accounted for 8% in Mag_1 and showed significant differences from the other five farms (p < 0.0003). Apiotrichum and Fusarium did not show significant differences among farms (Figure 4).
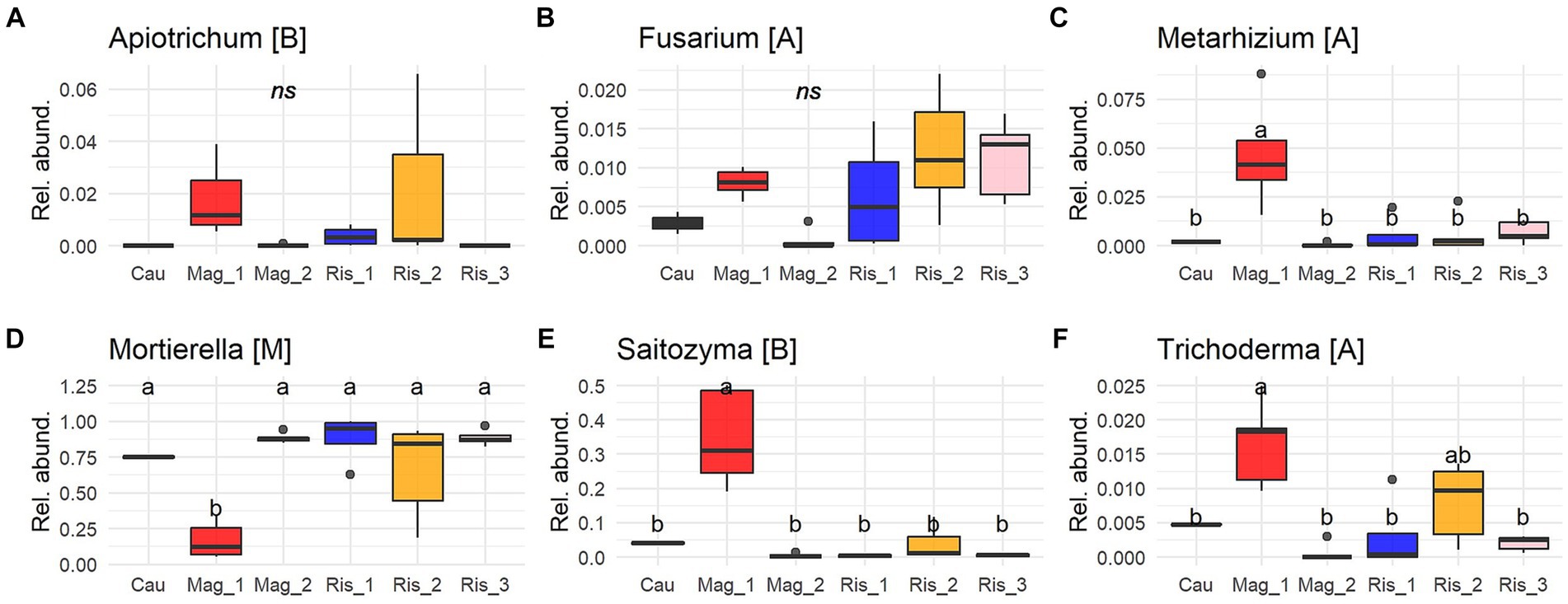
Figure 4. Proportion of reads (0 to 1) of the most abundant genus of soil fungi (A-F) (≥ 0.005 of relative abundance per sample and present in at least 25% of sampling sites). Solid lines indicate medians; boxes and whiskers indicate quartiles and ranges, respectively. Different letters indicate statistical differences among farms by Tukey post-hoc analyses with Bonferroni correction after Kruskal Wallis statistical tests.
3.6 Functional prediction
Saprotroph-symbiotroph was the most abundant trophic mode in the survey, accounting for up to 99% of detected functions in Mag_2, and 43% in Mag_1 (Figure 5). The genus Mortierella was representative of this trophic mode, with M. alpine, M. elongata, M. exigua, and M. minutissima identified at the species level (Figure 6). Potential pathotrophs were significantly more abundant in Mag_1 than in any other surveyed farms, with a 24% relative abundance (Figure 5). Among the genera belonging to Pathotroph, Metarhizium reached the highest abundance, particularly in Mag_1, with M. anisopliae and M. acridum identified at the species level (Figure 6). The potentially plant pathogens Clonostachys, Galactomyces, Giberella, Ilyonectria, and Thanatephorus were identified in low abundance considering the total counts (Supplementary Table S3 and Figures 5, 6). Saprotrophs reached up to 50% relative abundance in Mag_1 but varied highly within farms (Figure 5). Pseudocoleophoma and Talaromyces dominated the saprotrophic fungi in Cau, while species with low prevalence and abundance aggregated in the category “others” predominated on the remaining farms (Figure 6). The pathotroph-saprotroph-symbiotroph group exhibited a low relative abundance (i.e., <1% of total reads) compared to the previous groups (Figure 5). In this trophic mode, Trichoderma longipilis and Trichoderma sp. predominated in Cau and Mag_1, respectively (Figure 6). Pathotroph-saprotroph and pathotroph-symbiotroph fungi showed low abundance and were absent on the Cau farm (Figure 5).
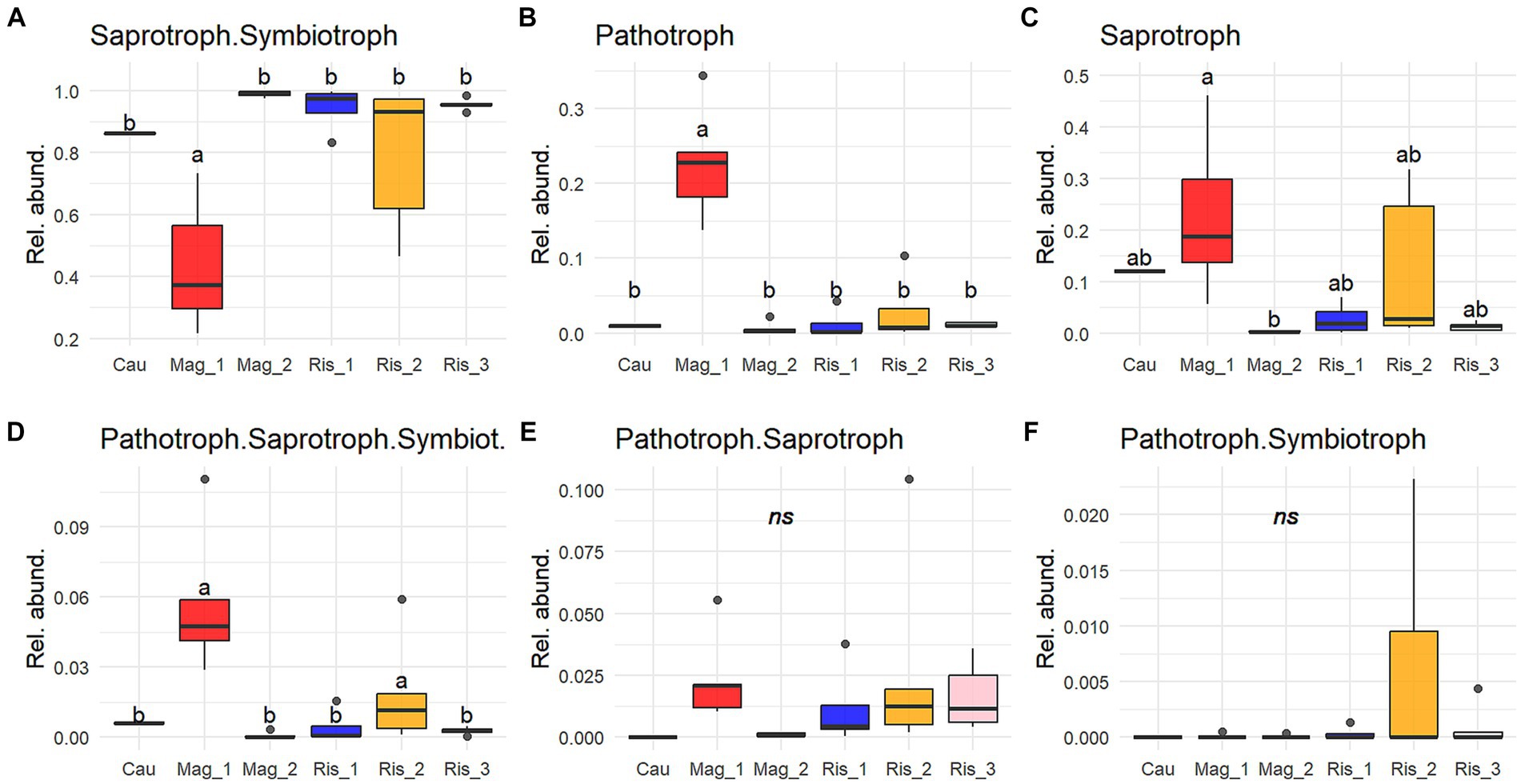
Figure 5. Proportion of reads (0 to 1) of the most abundant trophic modes (A-F) (≥ 0.005 of relative abundance per sample and present in at least 25% of sampling sites). Only trophic modes with “probable” and “highly probable” confidence were considered. Solid lines indicate medians; boxes and whiskers indicate quartiles and ranges, respectively. Different letters indicate statistical differences among farms by Tukey post-hoc analyses with Bonferroni correction after Kruskal Wallis statistical tests.
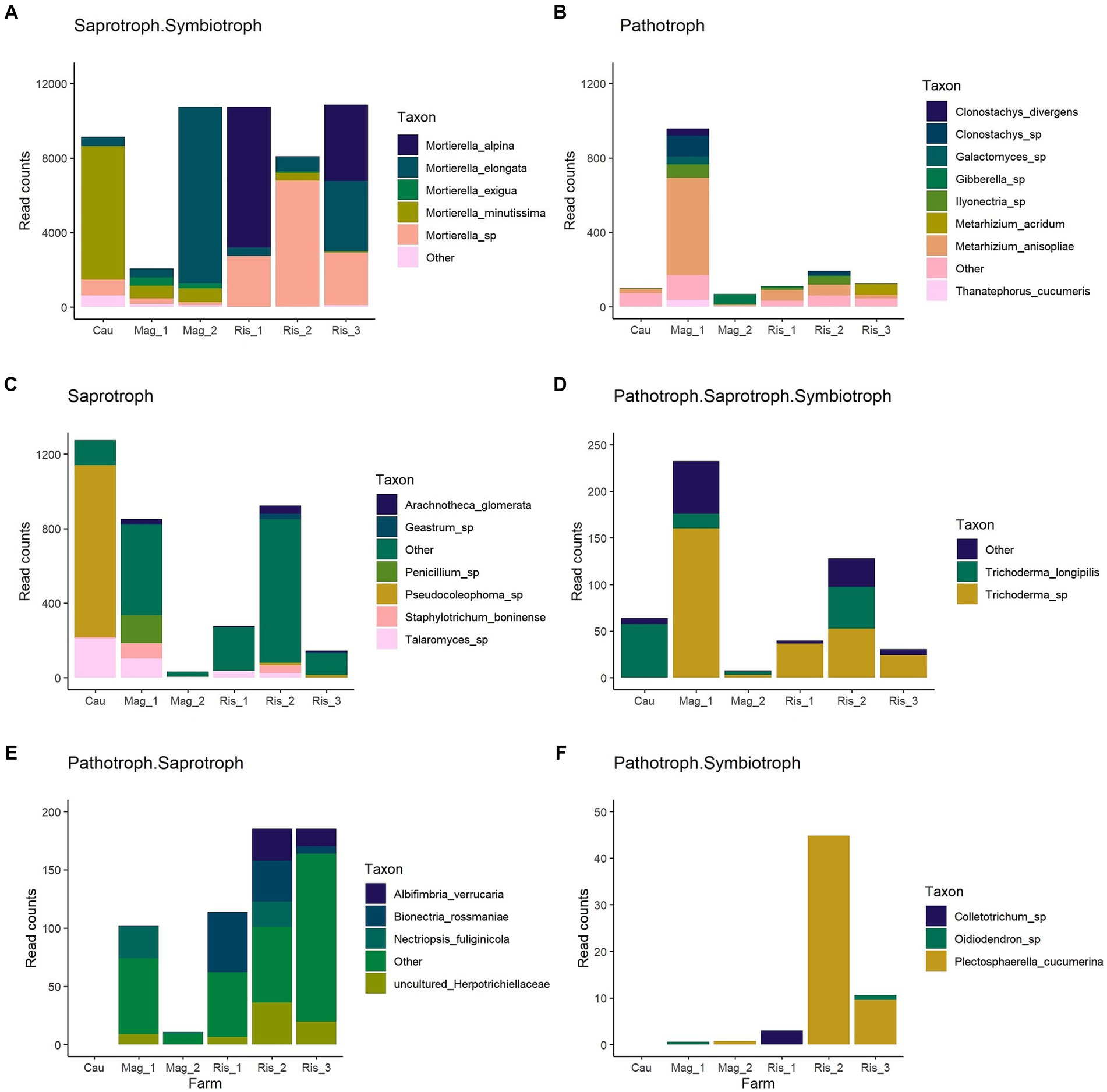
Figure 6. Abundances shown as read counts per farm of fungal taxon assigned as Saprotroph. Symbiotroph (A), Pathotroph (B), Saprotroph (C), Pathotroph. Saprotroph. Symbiotroph (D), Pathotroph. Saprotroph (E), and Pathotroph. Symbiotroph (F) trophic modes. “Taxon” represents the highest taxonomic level revealed by the functional prediction database FUNGuild. Note that the trophic mode was assigned based on fungal genus (Nguyen et al., 2016). “Other” is comprised by taxon with abundances lower than 0.5% of total counts of the group and a prevalence higher than 0.25.
4 Discussion
Agriculture generates artificial systems through multiple human activities, which greatly alter the resources and environment, and affects the biodiversity patterns of pre-existing biota (Sun et al., 2016; García-Delgado et al., 2019; Vukicevich et al., 2019). Here, we evaluated the composition of soil fungal communities in six coffee farms across three main coffee-growing regions in Colombia (Cauca, Risaralda, and Magdalena). These regions exhibited particular climatic and edaphic conditions, typical of soils intended for coffee production (Vaast et al., 2006). We found differences in community composition and ecological functions of soil fungi, which could be expected given the distinct geographical locations (Tedersoo et al., 2014; Nilsson et al., 2019), altitudinal gradients (Ogwu et al., 2019), topology (Veloso et al., 2020), floristic domains (Veloso et al., 2023), and inherent soil properties (Sun et al., 2016; Jurburg et al., 2020; Ning et al., 2021). However, to the best of our knowledge, the great predominance of the saprotroph-symbiotroph Mortierella and the genus Saitozyma is an unprecedented result that highlights the need for further investigation to disentangle the potential benefits as well as the consequences of this finding on the maintenance of sustainable coffee plantations (Duong et al., 2020).
We found that the conventional long-term shaded farm showed a diverse fungal community and high number of indicator species. Ascomycota and Basidiomycota exhibited the highest abundances (33.7 and 44%, respectively) in Mag_1, the farm under conventional management, and were non-dominant on the remaining farms (2–22% and 1–8%, respectively). On the other hand, Mortierellomycota dominated fungal abundance, with up to 88% of the total number of reads in a single sample belonging to the organic farms. Such differences in phyla distribution are consistent with further taxonomical and functional indicators evaluated in this study (i.e., indicator species, abundant genera, and abundant trophic modes).
Ascomycota, Basidiomycota, and Mortierellomycota are considered ubiquitous in natural and anthropogenic soils (Yang et al., 2019; Chen et al., 2020; Wang et al., 2021). Ascomycota and Basidiomycota comprise a great variety of species displaying a wide range of lifestyles (Webster and Weber, 2006). In a previous report on soil fungi in orchards in Boyacá Department, Colombia, Landinez-Torres et al. (2019) found that ~60% of the abundance belonged to Ascomycota followed by ~20% Basidiomycota and ~ 15% Zygomycota (formerly the phylum that contained Mortierellomycota). This study observed a similar pattern of relative abundance across the seven sites assessed in their survey (Landinez-Torres et al., 2019). Guevara (2005) found that conversion of natural forest to coffee plantation greatly affected the abundance of Basidiomycota, particularly mycelial cord-forming fungi, due to changes in soil micro-environmental conditions.
Mortierellomycota is frequently represented in investigations of soil fungi. It has been identified among generalist fungi of cropping systems (Toju et al., 2018; Grzadziel and Gałazka, 2019; Wang et al., 2021), and among the most characteristic micro-fungal species in montane cloud forest (Velez et al., 2021). Mortierellomycota became highly dominant in soils after the addition of organic amendments (Li et al., 2018, 2021), and high soil P availability negatively affected Mortierellomycota abundance (Detheridge et al., 2016; Orrù et al., 2021). Hence, considering that our study was conducted mostly in P-impoverished soils, we infer that such conditions might have created a favorable trophic niche for Mortierellomycota development (Sun et al., 2016; Li et al., 2021). Indeed, Mortierella was the predominant genus in five out of the six studied coffee farms, and it was identified as indicator species of organic farms. This result can be associated with the application of organic amendments on the organic farms (Li et al., 2018, 2021), which are not generally used on conventional farms as these rely on mineral fertilization (Perfecto et al., 2019).
The fungal functional guild analysis revealed a strong association between predicted functions and taxonomic composition. The great predominance of Mortierellomycota is consistent with the prevalence of the saprotroph-symbiotroph trophic mode and the representative species detected in this study (i.e., Mortierella alpina, M. elongata, M. exigua, and M. minutissima). It is noteworthy that the genus Mortierella comprises several phosphate-solubilizing species (Osorio Vega et al., 2015; Ceci et al., 2018), as well as species able to assist roots and mycorrhizal fungi in P acquisition (Tamayo-Velez and Osorio, 2017). Our results highlight the potential role of Mortierella in carbon turnover and P acquisition on the surveyed farms, particularly in recently established coffee plantations suggesting this genus can be considered a beneficial component of the soil fungal community.
Potentially pathogenic fungi were abundant in the studied soils and included species of crucial agronomical importance. Animal, plant, and insect pathogens are the main functional guilds of pathotrophs (Nguyen et al., 2016). Plant pathogens were found in very low abundance across the surveyed farms, and only Thanatephorus (teleomorph of Rhizoctonia solani) was found to be a potential coffee disease (Priyatmojo et al., 2001). This pathogen is not a menace in productive plantations and has rarely been reported in coffee plantations (Duong et al., 2020; Lu et al., 2022b). Apiotrichum and Fusarium were among the dominant genera, and this finding was previously reported in coffee plantations and montane forest (Arias and Abarca, 2014; Velez et al., 2021). Apiotrichum and Fusarium have been described as devastating pests affecting coffee production in Africa, where they usually infect mature trees and affect coffee quality by the premature ripening of coffee beans (Rutherford and Phiri, 2006). Although farmers did not report damage caused by the fungal species in this study, they are a potential menace that deserves further consideration.
Conversely, insect pathogen species belonging to Metarhizium, in particular M. anisopliae and M. acridum, were characteristic of the conventional long-term shaded farm (Mag_1) (Figures 4, 6). In addition to their well-documented entomopathogenic function, Metarhizium can also colonize plant root tissues as an endophyte, resulting in increased tolerance against pests and diseases (Behie and Bidochka, 2014; Altinok et al., 2019). In coffee plantations, M. anisopliae is used as a biopesticide to control nematodes (Tylenchida: Heteroderidae) and the coffee berry borer (Coleoptera: Cucurlionidae), which cause significant economic losses in coffee crops (Escobar-Ramírez et al., 2019; Cure et al., 2020; de Oliveira et al., 2021). It remains to be established whether the sequences identified in this study are vestiges of the commercial products applied on the farm in recent years or the native inhabitants of the soil microbiome.
Saprotrophs were among the abundant trophic modes with a high number of species with low prevalence (i.e., <25%) and low abundance per site (i.e., <10%) that were grouped as “other.” Dominance of saprotroph was reported earlier in tropical forests and coffee plantations suggesting that saprotrophs are key players in nutrient cycling (Looby and Treseder, 2018; Velez et al., 2021). Among the surveyed farms, we found that Ascomycotan Arachnotheca glomerata (Onygenaceae) and Basidiomycotan Geastrum spp. (Geastraceae) were prevalent with low abundance. A. glomerata possesses cellulose degradation abilities (Cano et al., 1987), while Geastrum has been reported to be an ectomycorrhizal fungus (Hibbett et al., 2000). The Cauca farm exhibited a distinct abundance of Pseudocoleophoma sp. that accounted for >75% of reads within the saprotrophic group and was identified as an indicator species of this site. Pseudocoleophoma is a genus belonging to Dictyosporiaceae and was recently isolated from decayed wood of Coffea arabica plantations in China (Lu et al., 2022a). According to the authors, their finding represents the first report of coffee-associated fungi in Dictyosporiaceae, and our results support this novelty. Probably, Pseudocoleophoma is a common saprotroph soil fungus without any record in coffee plantations due to the limited surveys of fungi on coffee debris.
We found a low abundance of Pathotroph-Saprotroph-Symbiotroph, Pathotroph-Saprotroph, and Pathotroph-Symbiotroph. Among these groups, we would like to highlight Trichoderma, which soared up to 2.5% of relative abundance in Mag_1 and was among the abundant genera. Trichoderma’s antimicrobial action has been used as a biocontrol agent against diverse phytopathogens in agroecosystems (Láng, 1936; Zamanizadeh et al., 2011). Mulaw et al. (2010) and Hoyos-Carvajal and Bissett (2011) isolated Trichoderma species inhabiting the rhizosphere of coffee plants. Arias and Abarca (2014) found Trichoderma as one of the most abundant and frequent genera among the saprotrophic fungi in coffee plantations. These authors suggest that its potential to produce large quantity of spores that are able to colonize a wide variety of substrates coupled with the ability to produce mycotoxins might constitute a competitive advantage of Trichoderma over other soil organisms in coffee plantations.
Among the fungi without a functional annotation, Papiliotrema laurentii (Basidiomycota), Cladosporium sphaerospermum, and Saitozyma podzolica were detected as indicators species. P. laurentii is an oleaginous yeast frequently found in soil, characterized by its ability to produce enzymes that degrade a wide diversity of carbon sources (de Almeida et al., 2022). In addition, P. laurentii can enhance mycorrhizal colonization of roots (Sampedro et al., 2004). Cladosporium sphaerospermum is usually found in soil air and has been reported to be a plant growth-promoting fungus (Hamayun et al., 2009). Dietzel et al. (2019) reported that C. sphaerospermum is a pathogenic fungus highly found in dust. Hamdouche et al. (2016) identified C. sphaerospermum among post-harvest fungi in Coffea arabica beans. Despite the ecological and agronomical role of P. laurentii and C. sphaerospermum in this survey, both were detected in limited abundance (i.e., <1%). In contrast, Saitozyma (Basidiomycota) was the second most dominant genus in our study (i.e., up to 50%). It has been recently cited as a common member of soils associated with Coffea arabica plantations in Brazil (Veloso et al., 2023). Although the FUNGuild database did not assign any ecological function to this genus, Veloso et al. (2023) propose that Saitozyma can be used as a starter culture in coffee fermentation to improve the sensory perception of the coffee beverage due to its ability to degrade particular lignocellulosic compounds. In addition, according to Starmer and Lachance (2011), Saitozyma species are key soil yeasts that can act as saprotrophs, mutualists and parasites. Yurkov et al. (2012) and Yurkov (2018) proposed this species as a potential indicator of acidic, well-drained, soils. Future investigations are necessary to disentangle the ecological role of Saitozyma in tropical and subtropical soils under anthropogenic use as well as the potential implications in further coffee beverage processing.
Admittedly, evident design constraints affected the generalizability of the findings of this study. One of the main limitations of this research was the restricted sampling design, associated with the absence of comparable farms in the sampled regions and scarce funding. We are aware that our results might not be representative of heterogeneous regional agroecosystems. Studying farms with comparable land practices is pivotal to disentangling agronomical-based causal effects, and here we attempted to explore the broadest area in order to include more sites from unexplored regions. Given the persistent lack of data on coffee soil microbiomes, our study makes a valuable contribution not only to local coffee farmers but also to global datasets of biodiversity from these poorly studied soils.
5 Concluding remarks
This exploratory survey sheds light on the composition of soil fungi in a poorly studied area with the goal of providing important information for ensuring a sustainable food production. Different fungal communities prevailed across the sampled regions. The saprotroph Mortierella was broadly frequent and dominant. Saitozyma was prevalent in the long-term agroforestry farm, and even though we could not assign an ecological function, this yeast may hold a valuable potential to improve the beverage quality of coffee. The identification of beneficial fungi such as Metarhizium and Trichoderma, widely used as biological control agents in Colombia’s coffee production, is an indication of the health of coffee soils in the country. In addition, no major pathogens of coffee roots, such as Rosellinia, were identified in any of the samples.
Data availability statement
The datasets presented in this study can be found in online repositories. The names of the repository/repositories and accession number(s) can be found in the article/Supplementary material.
Author contributions
VO-H: Investigation, Methodology, Writing – original draft, Writing – review & editing. VF: Conceptualization, Formal analysis, Funding acquisition, Investigation, Methodology, Supervision, Writing – original draft, Writing – review & editing. LG-G: Investigation, Methodology, Writing – original draft. MR: Funding acquisition, Investigation, Project administration, Resources, Supervision, Writing – original draft. MC: Conceptualization, Funding acquisition, Investigation, Methodology, Resources, Supervision, Writing – original draft, Writing – review & editing.
Funding
The author(s) declare that financial support was received for the research, authorship, and/or publication of this article. This research was funded by the grant “Capacity building for bioinformatics in Latin America- Network” (CABANA-net), funded by the agreement (2022–316296) between the Chan Zuckerberg Initiative (CZI) and the Universidad de Costa Rica.
Conflict of interest
The authors declare that the research was conducted in the absence of any commercial or financial relationships that could be construed as a potential conflict of interest.
Publisher’s note
All claims expressed in this article are solely those of the authors and do not necessarily represent those of their affiliated organizations, or those of the publisher, the editors and the reviewers. Any product that may be evaluated in this article, or claim that may be made by its manufacturer, is not guaranteed or endorsed by the publisher.
Supplementary material
The Supplementary material for this article can be found online at: https://www.frontiersin.org/articles/10.3389/fsufs.2024.1345383/full#supplementary-material
References
Altinok, H. H., Altinok, M. A., and Koca, A. S. (2019). Modes of action of entomopathogenic fungi. Curr. Trends Nat. Sci. 8, 117–124.
Anderson, M. J. (2001). A new method for non-parametric multivariate analysis of variance. Austral Ecol. 26, 32–46. doi: 10.1046/j.1442-9993.2001.01070.x
Arias, R. M., and Abarca, G. H. (2014). Fungal diversity in coffee plantation systems and in a tropical montane cloud forest in Veracruz, Mexico. Agrofor. Syst. 88, 921–933. doi: 10.1007/s10457-014-9736-z
Bayman, P., Mariño, Y. A., García-Rodríguez, N. M., Oduardo-Sierra, O. F., and Rehner, S. A. (2021). Local isolates of Beauveria bassiana for control of the coffee berry borer Hypothenemus hampei in Puerto Rico: virulence, efficacy and persistence. Biol. Control 155:104533. doi: 10.1016/j.biocontrol.2021.104533
Behie, S. W., and Bidochka, M. J. (2014). Nutrient transfer in plant-fungal symbioses. Trends Plant Sci. 19, 734–740. doi: 10.1016/j.tplants.2014.06.007
Bolyen, E., Rideout, J. R., Dillon, M. R., Bokulich, N. A., Abnet, C. C., Al-Ghalith, G. A., et al. (2019). Reproducible, interactive, scalable and extensible microbiome data science using QIIME 2. Nat. Biotechnol. 37, 852–857. doi: 10.1038/s41587-019-0209-9
Bravo-Monroy, L., Potts, S. G., and Tzanopoulos, J. (2016). Drivers influencing farmer decisions for adopting organic or conventional coffee management practices. Food Policy 58, 49–61. doi: 10.1016/j.foodpol.2015.11.003
Brinkmann, N., Schneider, D., Sahner, J., Ballauff, J., Edy, N., Barus, H., et al. (2019). Intensive tropical land use massively shifts soil fungal communities. Sci. Rep. 9:3403. doi: 10.1038/s41598-019-39829-4
Callahan, B. J., McMurdie, P. J., Rosen, M. J., Han, A. W., Johnson, A. J. A., and Holmes, S. P. (2016). DADA2: high-resolution sample inference from Illumina amplicon data. Nat. Methods 13, 581–583. doi: 10.1038/nmeth.3869
Cano, J., Guarro, J., and Figueras, M. J. (1987). Some keratinophilic fungi from Spain. Mycopathologia, 100, 163–167.
Cano-Sánz, C. G., Vallejo-Mejía, C., Caicedo-García, E., Amador-Torres, J. S., and Tique-Calderón, E. Y. (2013). El mercado mundial del café y su efecto en Colombia. Flujos de Capitales, Choques Externos y Respuestas de Política En Países Emergentes. Capítulo 10. El Mercado Mundial Del Café y Su Efecto En Colombia. Available at: http://repositorio.banrep.gov.co/handle/20.500.12134/6644
Ceci, A., Pinzari, F., Russo, F., Maggi, O., and Persiani, A. M. (2018). Saprotrophic soil fungi to improve phosphorus solubilisation and release: in vitro abilities of several species. Ambio 47, 30–40. doi: 10.1007/s13280-017-0972-0
Chen, Y., Tian, W., Shao, Y., Li, Y. J., Lin, L. A., Zhang, Y. J., et al. (2020). Miscanthus cultivation shapes rhizosphere microbial community structure and function as assessed by Illumina MiSeq sequencing combined with PICRUSt and FUNGUIld analyses. Arch. Microbiol. 202, 1157–1171. doi: 10.1007/s00203-020-01830-1
Correia, M., Espelta, J. M., Morillo, J. A., Pino, J., and Rodríguez-Echeverría, S. (2021). Land-use history alters the diversity, community composition and interaction networks of ectomycorrhizal fungi in beech forests. J. Ecol. 109, 2856–2870. doi: 10.1111/1365-2745.13674
Cristancho, M. A., Rozo, Y., Escobar, C., Rivillas, C. A., and Gaitán, A. L. (2012). Outbreak of coffee leaf rust (Hemileia vastatrix) in Colombia. New Dis. Rep. 25, 19–0588. doi: 10.5197/j.2044-0588.2012.025.019
Cure, J. R., Rodríguez, D., Gutierrez, A. P., and Ponti, L. (2020). The coffee agroecosystem: bio-economic analysis of coffee berry borer control (Hypothenemus hampei). Sci. Rep. 10, 12262–12212. doi: 10.1038/s41598-020-68989-x
DaMatta, F. M. (2004). Ecophysiological constraints on the production of shaded and unshaded coffee: a review. Field Crop Res. 86, 99–114. doi: 10.1016/j.fcr.2003.09.001
de Almeida, E. L. M., Ventorim, R. Z., de Moura Ferreira, M. A., and da Silveira, W. B. (2022). Papiliotrema laurentii: general features and biotechnological applications. Appl. Microbiol. Biotechnol. 106, 6963–6976. doi: 10.1007/s00253-022-12208-2
De Beenhouwer, M., Aerts, R., and Honnay, O. (2013). A global meta-analysis of the biodiversity and ecosystem service benefits of coffee and cacao agroforestry. Agric. Ecosyst. Environ. 175, 1–7. doi: 10.1016/j.agee.2013.05.003
De Cárcer, D. A., Denman, S. E., McSweeney, C., and Morrison, M. (2011). Evaluation of subsampling-based normalization strategies for tagged high-throughput sequencing data sets from gut microbiomes. Appl. Environ. Microbiol. 77, 8795–8798. doi: 10.1128/AEM.05491-11
de Oliveira Junqueira, A. C., de Melo Pereira, G. V., Coral Medina, J. D., Alvear, M. C., Rosero, R., de Carvalho Neto, D. P., et al. (2019). First description of bacterial and fungal communities in Colombian coffee beans fermentation analysed using Illumina-based amplicon sequencing. Sci. Rep. 9:8794. doi: 10.1038/s41598-019-45002-8
de Oliveira, C. M. G., Rosa, J. M. O., Pillat, R. R., and de Almeida, J. E. M. (2021). Efficiency of Metarhizium anisopliae in the control of Meloidogyne incognita in banana and coffee crops. Coffee Sci. 16, 1–6. doi: 10.25186/.v16i.1957
Detheridge, A. P., Brand, G., Fychan, R., Crotty, F. V., Sanderson, R., Griffith, G. W., et al. (2016). The legacy effect of cover crops on soil fungal populations in a cereal rotation. Agric. Ecosyst. Environ. 228, 49–61. doi: 10.1016/j.agee.2016.04.022
Dietzel, K., Valle, D., Fierer, N., U'Ren, J. M., and Barberán, A. (2019). Geographical distribution of fungal plant pathogens in dust across the United States. Front. Ecol. Evol. 7:304. doi: 10.3389/fevo.2019.00304
Dufrêne, M., and Legendre, P. (1997). Species assemblages and indicator species: the need for a flexible asymmetrical approach. Ecol. Monogr. 67, 345–366. doi: 10.1890/0012-9615(1997)067[0345,SAAIST]2.0.CO;2
Duong, B., Marraccini, P., Maeght, J. L., Vaast, P., Lebrun, M., and Duponnois, R. (2020). Coffee microbiota and its potential use in sustainable crop management. A review. Front. Sustain. Food Syst. 4:607935. doi: 10.3389/fsufs.2020.607935
Escobar-Ramírez, S., Grass, I., Armbrecht, I., and Tscharntke, T. (2019). Biological control of the coffee berry borer: main natural enemies, control success, and landscape influence. Biol. Control 136:103992. doi: 10.1016/j.biocontrol.2019.05.011
García, J. C., Posada-Suárez, H., and Läderach, P. (2014). Recommendations for the regionalizing of coffee cultivation in Colombia: a methodological proposal based on agro-climatic indices. PLoS One 9:e113510. doi: 10.1371/journal.pone.0113510
García-Delgado, C., Barba-Vicente, V., Marín-Benito, J. M., Mariano Igual, J., Sánchez-Martín, M. J., and Sonia Rodríguez-Cruz, M. (2019). Influence of different agricultural management practices on soil microbial community over dissipation time of two herbicides. Sci. Total Environ. 646, 1478–1488. doi: 10.1016/j.scitotenv.2018.07.395
Gichuru, E., Alwora, G., Gimase, J., and Kathurima, C. (2021). Coffee leaf rust (Hemileia vastatrix) in Kenya—a review. Agronomy 11:2590. doi: 10.3390/agronomy11122590
Grzadziel, J., and Gałazka, A. (2019). Fungal biodiversity of the most common types of polish soil in a long-term microplot experiment. Front. Microbiol. 10, 1–12. doi: 10.3389/fmicb.2019.00006
Guevara, R. (2005). Saprotrophic mycelial cord abundance, length and survivorship are reduced in the conversion of tropical cloud forest to shaded coffee plantation. Biol. Conserv. 125, 261–268. doi: 10.1016/j.biocon.2005.03.026
Hamdouche, Y., Meile, J. C., Nganou, D. N., Durand, N., Teyssier, C., and Montet, D. (2016). Discrimination of post-harvest coffee processing methods by microbial ecology analyses. Food Control 65, 112–120. doi: 10.1016/j.foodcont.2016.01.022
Hamayun, M., Afzal Khan, S., Ahmad, N., Tang, D. S., Kang, S. M., Na, C. I., et al. (2009). Cladosporium sphaerospermum as a new plant growth-promoting endophyte from the roots of Glycine max (L.) Merr. World J. Microbiol. Biotechnol, 25, 627–632. doi: 10.1007/s11274-009-9982-9
Harvey, C. A., Pritts, A. A., Zwetsloot, M. J., Jansen, K., Pulleman, M. M., Armbrecht, I., et al. (2021). Transformation of coffee-growing landscapes across Latin America. A review. Agron. Sustain. Dev. 41:62. doi: 10.1007/s13593-021-00712-0
Hibbett, D. S., Gilbert, L. B., and Donoghue, M. J. (2000). Evolutionary instability of ectomycorrhizal symbioses in basidiomycetes. Nature 407, 506–508. doi: 10.1038/35035065
Hoyos-Carvajal, L., and Bissett, J. (2011). “Biodiversity of trichoderma in neotropics” in The dynamical processes of biodiversity - case studies of evolution and spatial distribution
IGAC, (2022). Instituto Geográfico Agustín Codazzi. Gobierno de Colombia. Available at: https://www.igac.gov.co/ (Accessed November 21, 2022)
IUSS Working Group WRB (2022). "World Reference Base for soil resources, 4th edition". IUSS, Vienna.
Jha, S., Bacon, C. M., Philpott, S. M., Ernesto Méndez, V., Läderach, P., and Rice, R. A. (2014). Shade coffee: update on a disappearing refuge for biodiversity. Bioscience 64, 416–428. doi: 10.1093/biosci/biu038
Jurburg, S. D., Shek, K. L., and McGuire, K. (2020). Soil microbial composition varies in response to coffee agroecosystem management. FEMS Microbiol. Ecol. 96, 1–11. doi: 10.1093/femsec/fiaa164
Komar, O. (2006). Priority contribution. Ecology and conservation of birds in coffee plantations: a critical review. Bird Conserv. Int. 16, 1–23. doi: 10.1017/S0959270906000074
Landinez-Torres, A., Panelli, S., Picco, A. M., Comandatore, F., Tosi, S., and Capelli, E. (2019). A meta-barcoding analysis of soil mycobiota of the upper Andean Colombian agro-environment. Sci. Rep. 9, 10085–10012. doi: 10.1038/s41598-019-46485-1
Láng, P. H. (1936). Recent trends in mycological research. Bull Am Musicol Soc 1, 15–16. doi: 10.2307/829255
Lara-Estrada, L., and Vaast, P.. (2005). Effects of altitude, shade, yield and fertilization on coffee quality (Coffea arabica L. var. Caturra) produced in agroforestry systems of the Northern Central Zones of Nicaragua. In 2nd International Symposium on Multi-Strata Agroforestry Systems with Perennial Crops, 17–21.
Lekberg, Y., Arnillas, C. A., Borer, E. T., Bullington, L. S., Fierer, N., Kennedy, P. G., et al. (2021). Nitrogen and phosphorus fertilization consistently favor pathogenic over mutualistic fungi in grassland soils. Nat. Commun. 12:3484. doi: 10.1038/s41467-021-23605-y
Li, F., Chen, L., Redmile-Gordon, M., Zhang, J., Zhang, C., Ning, Q., et al. (2018). Mortierella elongata's roles in organic agriculture and crop growth promotion in a mineral soil. Land Degrad. Dev. 29, 1642–1651. doi: 10.1002/ldr.2965
Li, J., Gan, G., Chen, X., and Zou, J. (2021). Effects of long-term straw management and potassium fertilization on crop yield, soil properties, and microbial community in a rice–oilseed rape rotation. Agriculture 11, 1–18. doi: 10.3390/agriculture11121233
Looby, C. I., and Treseder, K. K. (2018). Shifts in soil fungi and extracellular enzyme activity with simulated climate change in a tropical montane cloud forest. Soil Biol. Biochem. 117, 87–96. doi: 10.1016/j.soilbio.2017.11.014
Lovera, M., Cuenca, G., Fajardo, L., Cáceres, A., and Guerra-Sierra, B. E. (2022). “AMF Diversity in Coffee and Cacao Agroforestry Systems: Importance for Crop Productivity and Forest Conservation”. In Mycorrhizal Fungi in South America: Biodiversity, Conservation, and Sustainable Food Production. Eds. M. A. Lugo and M. C. Pagano, (New York, NY, USA: Springer International Publishing). 107–127.
Lu, L., Karunarathna, S. C., Dai, D. Q., Xiong, Y. R., Suwannarach, N., Stephenson, S. L., et al. (2022a). Description of four novel species in Pleosporales associated with coffee in Yunnan, China. J Fungi 8:1113. doi: 10.3390/jof8101113
Lu, L., Tibpromma, S., Karunarathna, S. C., Jayawardena, R. S., Lumyong, S., Xu, J., et al. (2022b). Comprehensive review of Fungi on coffee. Pathogens 11, 1–17. doi: 10.3390/pathogens11040411
Moguel, P., and Toledo, V. M. (1999). Biodiversity conservation in traditional coffee systems of Mexico. Conserv. Biol. 13, 11–21. doi: 10.1046/j.1523-1739.1999.97153.x
Mulaw, T. B., Kubicek, C. P., and Druzhinina, I. S. (2010). The rhizosphere of Coffea arabica in its native highland forests of Ethiopia provides a niche for a distinguished diversity of Trichoderma. Diversity 2, 527–549. doi: 10.3390/d2040527
Nguyen, N. H., Song, Z., Bates, S. T., Branco, S., Tedersoo, L., Menke, J., et al. (2016). FUNGuild: an open annotation tool for parsing fungal community datasets by ecological guild. Fungal Ecol. 20, 241–248. doi: 10.1016/j.funeco.2015.06.006
Nilsson, R. H., Larsson, K. H., Taylor, A. F. S., Bengtsson-Palme, J., Jeppesen, T. S., Schigel, D., et al. (2019). The UNITE database for molecular identification of fungi: handling dark taxa and parallel taxonomic classifications. Nucleic Acids Res. 47, D259–D264. doi: 10.1093/nar/gky1022
Ning, Q., Chen, L., Zhang, C., Ma, D., Li, D., Han, X., et al. (2021). Saprotrophic fungal communities in arable soils are strongly associated with soil fertility and stoichiometry. Appl. Soil Ecol. 159:103843. doi: 10.1016/j.apsoil.2020.103843
Ogwu, M. C., Takahashi, K., Dong, K., Song, H. K., Moroenyane, I., Waldman, B., et al. (2019). Fungal elevational Rapoport pattern from a High Mountain in Japan. Sci. Rep. 9, 6570–6510. doi: 10.1038/s41598-019-43025-9
Oksanen, J., Blanchet, F. G., Kindt, R., Legendre, P., Minchin, P. R., O’hara, R. B., et al. (2015). Vegan: community ecology package. R package vegan, vers. 2.2-1. Worl. Agro. Cent. 3, 7–81.
Orrù, L., Canfora, L., Trinchera, A., Migliore, M., Pennelli, B., Marcucci, A., et al. (2021). How tillage and crop rotation change the distribution pattern of Fungi. Front. Microbiol. 12, 1–18. doi: 10.3389/fmicb.2021.634325
Osorio Vega, N. W., Habte, M., and León Peláez, J. D. (2015). Effectiveness of a rock phosphate solubilizing fungus to increase soil solution phosphate impaired by the soil phosphate sorption capacity. Rev. Fac. Nac. Agron. Medellín 68, 7627–7636. doi: 10.15446/rfnam.v68n2.50950
Perfecto, I., Jiménez-Soto, M. E., and Vandermeer, J. (2019). Coffee landscapes shaping the anthropocene: forced simplification on a complex agroecological landscape. Curr. Anthropol. 60, S236–S250. doi: 10.1086/703413
Perfecto, I., Vandermeer, J., and Philpott, S. M. (2014). Complex ecological interactions in the coffee agroecosystem. Annu. Rev. Ecol. Evol. Syst. 45, 137–158. doi: 10.1146/annurev-ecolsys-120213-091923
Prates Júnior, P., Moreira, B. C., da Silva, M., Veloso, T. G. R., Stürmer, S. L., Fernandes, R. B. A., et al. (2019). Agroecological coffee management increases arbuscular mycorrhizal fungi diversity. PLoS One 14, e0209093–e0209019. doi: 10.1371/journal.pone.0209093
Priyatmojo, A., Escopalao, V. E., Tangonan, N. G., Pascual, C. B., Suga, H., Kageyama, K., et al. (2001). Characterization of a new subgroup of Rhizoctonia solani anastomosis group 1 (AG-1-ID), causal agent of a necrotic leaf spot on coffee. Phytopathology 91, 1054–1061. doi: 10.1094/PHYTO.2001.91.11.1054
R Development Core Team (2022). R: A language and environment for statistical computing. R Foundation for Statistical Computing, Vienna, Austria.
Rao, M. V., Rice, R. A., Fleischer, R. C., and Muletz-Wolz, C. R. (2020). Soil fungal communities differ between shaded and sun-intensive coffee plantations in El Salvador. PLoS One 15, e0231875–e0231819. doi: 10.1371/journal.pone.0231875
Rekik, F., van Es, H., Hernandez-Aguilera, J. N., and Gómez, M. I. (2018). Soil health assessment for coffee farms on andosols in Colombia. Geoderma Reg. 14:e00176. doi: 10.1016/j.geodrs.2018.e00176
Roberts, D. W. (2012). Package “labdsv”: ordination and multivariate analysis for ecology. R package version 1.0–5. Available at: http://ecology.msu.montana.edu/labdsv/R
Rutherford, M. A., and Phiri, N. (2006). Pests and Diseases of Coffee in Eastern Africa: a Technical and Advisory Manual, Wallingford, UK: CAB International.
Sampedro, I., Aranda, E., Scervino, J. M., Fracchia, S., García-Romera, I., Ocampo, J. A., et al. (2004). Improvement by soil yeasts of arbuscular mycorrhizal symbiosis of soybean (Glycine max) colonized by Glomus mosseae. Mycorrhiza 14, 229–234. doi: 10.1007/s00572-003-0285-y
Starmer, W. T., and Lachance, M. A., (2011). Yeast ecology. The Yeasts, a Taxonomic Study, Vol. 1, 5th edn, Eds. C. P. Kurtzman, J. W. Fell, and T. Boekhout (Amsterdam: Elsevier Science Publishers). 65–83.
Sun, R., Dsouza, M., Gilbert, J. A., Guo, X., Wang, D., Guo, Z., et al. (2016). Fungal community composition in soils subjected to long-term chemical fertilization is most influenced by the type of organic matter. Environ. Microbiol. 18, 5137–5150. doi: 10.1111/1462-2920.13512
Talhinhas, P., Batista, D., Diniz, I., Vieira, A., Silva, D. N., Loureiro, A., et al. (2017). The coffee leaf rust pathogen Hemileia vastatrix: one and a half centuries around the tropics. Mol. Plant Pathol. 18, 1039–1051. doi: 10.1111/mpp.12512
Tamayo-Velez, A., and Osorio, N. W. (2017). Co-inoculation with an arbuscular mycorrhizal fungus and a phosphate-solubilizing fungus promotes the plant growth and phosphate uptake of avocado plantlets in a nursery. Botany 95, 539–545. doi: 10.1139/cjb-2016-0224
Tedersoo, L., Bahram, M., Põlme, S., Kõljalg, U., Yorou, N. S., Wijesundera, R., et al. (2014). Global diversity and geography of soil fungi. Science 346:1256688. doi: 10.1126/science.1256688
Tedersoo, L., Mikryukov, V., Zizka, A., Bahram, M., Hagh-Doust, N., Anslan, S., et al. (2022). Towards understanding diversity, endemicity and global change vulnerability of soil fungi. bioRxiv :484796. doi: 10.1101/2022.03.17.484796
Toju, H., Tanabe, A. S., and Sato, H. (2018). Network hubs in root-associated fungal metacommunities. Microbiome 6:116. doi: 10.1186/s40168-018-0497-1
Toju, H., Tanabe, A. S., Yamamoto, S., and Sato, H. (2012). High-coverage ITS primers for the DNA-based identification of ascomycetes and basidiomycetes in environmental samples. PLoS One 7:e40863. doi: 10.1371/journal.pone.0040863
Turley, N. E., Bell-Dereske, L., Evans, S. E., and Brudvig, L. A. (2020). Agricultural land-use history and restoration impact soil microbial biodiversity. J. Appl. Ecol. 57, 852–863. doi: 10.1111/1365-2664.13591
Urgiles-Gómez, N., Avila-Salem, M. E., Loján, P., Encalada, M., Hurtado, L., Araujo, S., et al. (2021). Plant growth-promoting microorganisms in coffee production: from isolation to field application. Agronomy 11:1531. doi: 10.3390/agronomy11081531
Vaast, P., Bertrand, B., Perriot, J. J., Guyot, B., and Génard, M. (2006). Fruit thinning and shade improve bean characteristics and beverage quality of coffee (Coffea arabica L.) under optimal conditions. J. Sci. Food Agric. 86, 197–204. doi: 10.1002/jsfa.2338
Vega, F. E., Posada, F., Aime, M. C., Pava-Ripoll, M., Infante, F., and Rehner, S. A. (2008). Entomopathogenic fungal endophytes. Biol. Control 46, 72–82. doi: 10.1016/j.biocontrol.2008.01.008
Velandia-Silva, C. A. (2017). The coffee cultural landscape of Colombia. J. World Herit. Stud., 44–50.
Velez, P., Tapia-Torres, Y., García-Oliva, F., and Gasca-Pineda, J. (2021). Small-scale variation in a pristine montane cloud forest: evidence on high soil fungal diversity and biogeochemical heterogeneity. PeerJ 9:e11956. doi: 10.7717/peerj.11956
Velmourougane, K., and Bhat, R. (2017). “Sustainability challenges in the coffee plantation sector” in Sustainability challenges in the agrofood sector, Ed. R. Bhat, (West Sussex, UK: John Wiley & Sons). 616–642. Available at: http://onlinelibrary.wiley.com/doi/book/10.1002/9781119072737.
Veloso, T. G. R., da Silva, M., Cardoso, W. S., Guarçoni, R. C., Kasuya, M. C. M., and Pereira, L. L. (2020). Effects of environmental factors on microbiota of fruits and soil of Coffea arabica in Brazil. Sci. Rep. 10, 14692–14611. doi: 10.1038/s41598-020-71309-y
Veloso, T. G. R., da Silva, M. D. C. S., Moreira, T. R., Da Luz, J. M. R., Moreli, A. P., and Kasuya, M. C. M. (2023). Microbiomes associated with Coffea arabica Coffea canephora in four different floristic domains of Brazil. Scientific Reports, 13, 18477. doi: 10.1038/s41598-023-45465-w
Vukicevich, E., Thomas Lowery, D., Bennett, J. A., and Hart, M. (2019). Influence of groundcover vegetation, soil physicochemical properties, and irrigation practices on soil fungi in semi-arid vineyards. Front. Ecol. Evol. 7, 1–10. doi: 10.3389/fevo.2019.00118
Wang, J. T., Shen, J. P., Zhang, L. M., Singh, B. K., Delgado-Baquerizo, M., Hu, H. W., et al. (2021). Generalist taxa shape fungal community structure in cropping ecosystems. Front. Microbiol. 12:678290. doi: 10.3389/fmicb.2021.678290
Webster, J., and Weber, R. (2006). Introduction to Fungi. (Third Edit). Cambridge, UK: Cambridge University Press.
Yang, J., Wang, Y., Cui, X., Xue, K., Zhang, Y., and Yu, Z. (2019). Habitat filtering shapes the differential structure of microbial communities in the Xilingol grassland. Sci. Rep. 9, 19326–19312. doi: 10.1038/s41598-019-55940-y
Yurkov, A. M. (2018). Yeasts of the soil – obscure but precious. Yeast 35, 369–378. doi: 10.1002/yea.3310
Yurkov, A. M., Kemler, M., and Begerow, D. (2012). Assessment of yeast diversity in soils under different management regimes. Fungal Ecol. 5, 24–35. doi: 10.1016/J.FUNECO.2011.07.004
Keywords: coffee mycobiome, sustainable agriculture, shade, microbiome, amplicon sequencing
Citation: Ochoa-Henriquez VH, Faggioli V, Gómez-Godínez LJ, Rivarola M and Cristancho M (2024) Colombian coffee (Coffea arabica L.) plantations: a taxonomic and functional survey of soil fungi. Front. Sustain. Food Syst. 8:1345383. doi: 10.3389/fsufs.2024.1345383
Edited by:
Robert Hunter Manson, Instituto de Ecología (INECOL), MexicoReviewed by:
Rosa Maria Arias Mota, Instituto de Ecología (INECOL), MexicoPatricia Velez, National Autonomous University of Mexico, Mexico
Copyright © 2024 Ochoa-Henriquez, Faggioli, Gómez-Godínez, Rivarola and Cristancho. This is an open-access article distributed under the terms of the Creative Commons Attribution License (CC BY). The use, distribution or reproduction in other forums is permitted, provided the original author(s) and the copyright owner(s) are credited and that the original publication in this journal is cited, in accordance with accepted academic practice. No use, distribution or reproduction is permitted which does not comply with these terms.
*Correspondence: Valeria Faggioli, faggioli.valeria@inta.gob.ar; faggiolivaleria@gmail.com
†Present addresses: Victor Hugo Ochoa-Henriquez, School of Life Sciences, Arizona State University, Arizona, AZ, United States
Marco Cristancho, Plant Pathology Department, National Center for Coffee Research - CENICAFE, Manizales, Colombia