- 1International Institute of Tropical Agriculture (IITA), Lusaka, Zambia
- 2Department of Agriculture Production, Kyambogo University, Kampala, Uganda
- 3Department of Plant Sciences, University of Zambia, Lusaka, Zambia
- 4Zambia Agriculture Research Institute (ZARI), Chilanga, Zambia
- 5National Institute for Scientific and Industrial Research (NISIR), Lusaka, Zambia
- 6IITA-Nigeria, Ibadan, Nigeria
Aflatoxin contamination in peanuts (Arachis hypogaea L.) by aflatoxin-producing Aspergillus spp. is a serious problem worldwide affecting human health and restricting trade. Recently, conservation agriculture practices such as minimum tillage, crop rotation, soil surface cover, intercropping, and planting in basins alongside trees that enhance soil fertility, have been introduced in Zambia as tools to conserve soil moisture, increase peanut yield, and reduce the risk of aflatoxin contamination. However, a limited understanding exists of the impact of conservation agriculture on Aspergillus population densities in the soil and aflatoxin concentrations in crops grown under conservation agriculture. This study assessed the impact of conservation agriculture on (i) Aspergillus communities profile in soil cropped to peanuts, (ii) peanut aflatoxin contamination levels, and (iii) influences of the practices on liver cancer risks as a result of consuming peanuts grown in basins, peanuts grown in basins in farms having Gliricidia sepium trees (agroforestry), and peanuts grown on ridges (conventional tillage). Densities of Aspergillus spp. in soils ranged from 10 to 7,400 CFU/g (mean = 295 CFU/g). The densities differed among farming techniques (p = 0.0011) with conventional tillage (357 CFU/g) supporting higher densities than agroforestry (296 CFU/g) and peanuts grown in basins (230 CFU/g). Aspergillus communities were dominated by fungi with S morphology (55%; which is composed of several morphologically similar species), followed by A. parasiticus (34%), and the L morphotype of A. flavus (11%). Over 60% of the peanut samples were contaminated but there were no differences among farming techniques. Mean aflatoxin contents in peanuts were 110, 99, and 87, μg/kg for agroforestry, conventional tillage, and peanuts grown in basins, respectively. Probable daily aflatoxin intake ranged from 12.7 to 767.1 ng/kg body weight/d, depending on the age of consumers. The estimated liver cancer risk due to consumption of raw peanuts (0.334 to 20.128 cases/100,000 persons/year) was highest among children below 5 years and least for young adults. The results showed that growing peanuts in basins has the potential to reduce Aspergillus populations in soil. Possibly, planting in basins and agroforestry had minimal effect on water runoff and did not support sufficient moisture retention in the soil to reduce Aspergillus spp. densities and aflatoxin accumulation in the peanuts. Thus, more research is needed to improve the effectiveness of peanut cultivation in basins in shaping the Aspergillus species profiles for ultimately reducing aflatoxin in peanuts to safe levels. Given the high exposure of Zambia’s population, especially children, and that contaminated food is the major source of exposure, it is essential that appropriate technical, policy and institutional measures are promoted for aflatoxin mitigation in Zambia.
1 Introduction
In many regions of the world, peanut (Arachis hypogaea L.) is an essential oil and food security crop. In Zambia, peanut is considered a “woman’s crop”, ranks second in cultivation after maize, and is highly valued because it helps meet the nutritional needs of people in rural areas (Mofya-Mukuka and Shipekesa, 2013; Tembo and Sitko, 2013; Mofya-Mukuka and Chisanga, 2014). Smallholder peanut production in Zambia is rain-fed with the largest production taking place in Eastern (31%), Northern (16%), and Southern (12%) provinces [Ross and de Klerk, 2012; MAL (Ministry of Agriculture and Livestock), 2013; Sichoongwe et al., 2014].
Despite its strategic food and nutrition security roles, peanut yields in Zambia are low with average yields ranging from 220 to 1,500 kg/ha (ZARI, 2018, 2019). Erratic rainfall patterns, prolonged dry spells and/or drought along with pest and disease pressures, poor soils and inaccessibility to high quality, improved seeds are factors limiting peanut production in Zambia (Mofya-Mukuka and Chisanga, 2014; Desmae and Sones, 2017). Furthermore, the quality, nutritional value, and safety of peanuts produced in Zambia are lowered by molds that often produce toxic metabolites, such as aflatoxins, in infected kernels (Bumbangi et al., 2016; Njoroge et al., 2017; Kachapulula et al., 2017a).
Aflatoxins, which are cancer-causing, growth-retardant and immune-suppressive toxic substances produced mainly by Aspergillus flavus (Link) and A. parasiticus (Speare), are the most common mycotoxins found in Zambian peanuts. About 12–25% of raw peanut grains or powder and 53–80% of processed peanut butter obtained at farmgate or markets contain aflatoxin exceeding levels considered safe for human consumption (10 μg/kg) (Bumbangi et al., 2016; Njoroge et al., 2017; Kachapulula et al., 2017a). High aflatoxin levels can worsen food insecurity and malnutrition. Coincidentally, in Zambia, high malnutrition rates occur in high peanut-producing provinces (Mofya-Mukuka and Shipekesa, 2013; Mofya-Mukuka and Kuhlgatz, 2016), where unacceptable aflatoxin levels have been found in children’s weaning and complementary foods (Alamu et al., 2020). Unfortunately, most farmers in Zambia have limited knowledge of aflatoxin health-related risks and do not employ effective aflatoxin management strategy to limit human exposure (Tembo et al., 2023).
Good agronomic practices (GAP), biocontrol using atoxigenic isolates of A. flavus, resistant crop varieties, food diversification, and the adoption of improved storage technologies can curtail aflatoxin in foodstuffs and prevent exposure among consumers (Ortega-Beltran and Bandyopadhyay, 2021). However, there are several other practices/techniques that may aid in reducing aflatoxin in peanuts that remain relatively unexplored. Conservation Agriculture (CA), which entails techniques that support minimal soil disturbance, permanent soil cover, and diversification of crop species, is currently being promoted across Southern Africa, including Zambia, as a climate-smart GAP for managing agroecosystems and preserving the environment for sustainable agricultural productivity, increased profits, and food security (Komarek et al., 2021; Ngoma et al., 2021; Mhlanga et al., 2022; Tufa et al., 2023).
Recently, CA practices (e.g., early planting, crop rotation, intercropping and planting in basins, alongside planting soil fertility enhancing trees) have been introduced in Zambia as tools for conserving soil moisture, increasing peanut yield, and reducing risks of aflatoxin (Kortei et al., 2022). However, CA may have both beneficial effects and some drawbacks (Andersson and D'Souza, 2014; Palm et al., 2014). For instance, mulching and using green manure as a CA practice may facilitate the build-up of soil- and crop residue-borne plant pathogens (Thomas et al., 2007). On the other hand, cultural practices such as manure application, mulching and tied ridges were reported as effective in enhancing peanut safety by reducing aflatoxin levels (Njoroge, 2018).
A recent study revealed that CA practices like tillage regimes had variable effects on soil microbiome without causing any significant change to fungal diversity (Li et al., 2020). In another study, different CA tillage regimes were found to alter soil fungal alpha diversity (Wang et al., 2016). However, limited literature exists on the effect of CA on the fungal component of soil microbial community structure under peanut cropping system. Furthermore, CA effects on Aspergillus species and subsequent aflatoxin levels in peanuts remain to be investigated. The objectives of the current study were to determine the impact of CA on (i) aflatoxin-producing Aspergillus species composition in soil cropped to peanuts, (ii) peanut aflatoxin contamination levels, and (iii) aflatoxin exposure risks. We hypothesized that CA practices in peanuts support more diverse fungal communities with reduced densities of aflatoxin-producing fungi leading to reduced aflatoxin contamination. The results can aid in enhancing peanut productivity and quality in different agro-ecological zones (AEZs) of Zambia using CA approaches.
2 Methodology
2.1 Survey sites
To assess the impact of the CA farming methods on aflatoxin-producing fungi and aflatoxin levels, a two-year survey was conducted in AEZs II and III of Zambia. AEZ II has the most fertile soils in Zambia with a growing period of 100–140 days and receives moderate rainfall (800–1,000 mm per annum). AEZ III receives the highest amount of rainfall (>1,000 mm per annum) and has a growing period of 120–150 days (Bunyolo et al., 1995). AEZ III has highly weathered, leached, and often extremely acidic soils that may be toxic to crops (Muimba-Kankolongo, 2018; Muimba-Kankolongo et al., 2021). In AEZ II, soil and crop samples were collected from seven districts in Eastern Province (i.e., Lundazi, Chipata, Katete, Mambwe, Sinda, Petauke, and Nyimba), while in AEZ III, samples were collected from Mpika and Chama districts of Muchinga Province (Figure 1). Usually, farmers in these districts sow peanuts from mid-November to late January and crop harvesting period stretches from May to July. The survey was carried out immediately after farmers harvested their crops between June and July 2017 and 2018 for the 2016/2017 and 2017/2018 cropping seasons, respectively.
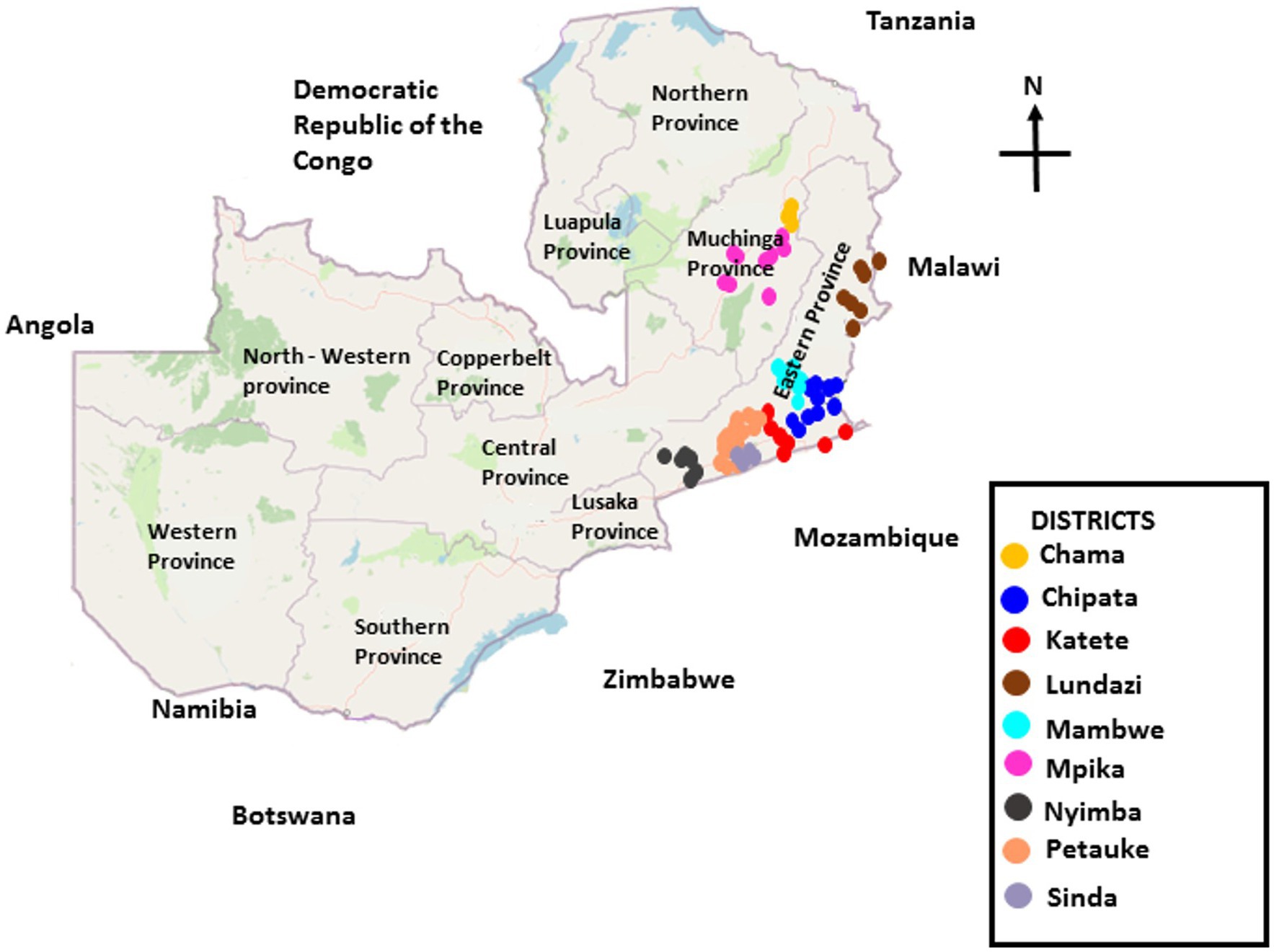
Figure 1. Locations in nine districts of two provinces of Zambia from where peanut samples were collected after crop harvest in 2016 and 2017.
2.2 Crop and soil sampling
Overall, 270 in-shell peanut samples were collected from female-managed and owned peanut fields. Farmers grew their crops in three types of cropping systems: (1) in basins without (CAB) or (2) with 2–5 year-old Gliricidia sepium agroforestry trees (AGF), and (3) on ridges in fields lacking G. sepium (CT). In each cropping season, five samples per farming technique were collected from each of the nine districts, giving a total of 135 samples (i.e., AGF = 45, CAB = 45, and CT = 45). Every sample consisted of 5 kg in-shell peanuts. The samples were labeled, geo-referenced, and transported to the pathology laboratory at Zambia Agriculture Research Institute (ZARI), Mt. Makulu, Chilanga for processing. The pods of each sample were hand-shelled and kernels ground using a laboratory blender (Waring Commercial, Springfield, MO) that was cleaned between samples with 70% methanol to prevent cross-contamination of aflatoxins and microorganisms.
For the soil samples, three peanut fields per cropping system (i.e., AGF, CAB, and CT) were randomly selected and sampled in each district in October 2018. From each field, a sample consisted of 30 subsamples of soil (~10 to 20 g each) collected from the surface to a depth of 5 cm at different sites across the entire field using a diagonal sampling framework. The 30 subsamples were mixed to make one composite sample. A total of 81 soil samples (3 fields × 3 cropping systems × 9 districts) were collected, placed in plastic Ziplock bags, stored in a cooler box, and transported to the laboratory. Samples were sieved to remove stones and debris. Grain and soil samples were stored at 4°C prior to Aspergillus isolation and aflatoxin quantification (only for kernels).
2.3 Aspergillus isolation and characterization
Aspergillus fungi were isolated from the 81 soil and 270 peanut samples on Modified Rose Bengal Agar (MRBA) using the dilution plate technique (Cotty, 1994; Probst et al., 2007). One liter of MRBA comprised of 3 g Sucrose, 3 g NaNO3, 0.75 g KH2PO4, 0.25 g K2HPO4, 0.5 g MgSO4,7H2O, 0.5 g KCl, 1 mL micronutrients, 5 mL Rose Bengal, 5 mL of chloramphenicol and 20 g agar. After sterilizing at 121°C for 20 min, the media was cooled, supplemented with 10 mL/L dichloran and 5 mL/L streptomycin sulfate, and then dispensed into 9 cm Petri dishes. One to five gram of soil or peanut flour sample was weighed and suspended in 10 mL sterile distilled water. Thereafter, 50–200 μL of the sample suspension was spread on MRBA plates and then incubated (dark, 31°C, 3 days). The number of Aspergillus colonies was counted and recorded before transferring 15 colonies onto 5/2 agar [5% V8 Juice (Campbell Soup Company, Camden, NJ, United States); 2% agar, pH 6.0] in 5.5 cm Petri dishes and incubated (dark, 31°C, 5 days). Species characterization was based on morphological features, colony pigmentation, and conidial size and appearance (Klich and Pitt, 1988; Cotty, 1989). On 5/2 agar, A. parasiticus colonies were dark green, while A. flavus and fungi with S-morphotype were light green and the latter produced numerous sclerotia (Cotty, 1994). Isolates with abundant small and smooth sclerotia (average < 400 μm) were classified as fungi with S-morphotype (Cotty and Cardwell, 1999). Isolates with smooth conidia and large sclerotia (average diameter > 400 μm) were classified as A. flavus L morphotype. Isolates with rough conidial surfaces were classified as A. parasiticus (Klich and Pitt, 1988).
2.4 Aflatoxin quantification in peanut
Total aflatoxin (AF) in each peanut sample was determined using the GIPSA-approved Neogen Reveal Q+ kit (Reveal Q+ for aflatoxin, Neogen Corporation, Lansing, United States). Ground peanut (50 g) was weighed and transferred to a 500 mL Pyrex bottle. Then, 250 mL 65% ethanol was added to the bottle, which was capped and shaken at 500 rpm for 3 min on a MaxQ 2000 orbital shaker (Thermo Fisher Scientific Inc., Bartlesville, United States). The extract was allowed to settle, the supernatant filtered through a Whatman No. 1 filter paper (Whatman Intl. Ltd., Maidstone, United Kingdom), and the AF in the extract was quantified using a Neogen AccuScan Reader. The reader can quantify AF from 2 μg/kg to 150 μg/kg. The extracts of samples containing AF levels exceeding the upper limit were diluted with 65% ethanol and re-assayed. Aflatoxin reference material (Neogen Corporation, Lansing, United States) with a predetermined AF level was included in the analyses after every 20 sample runs to serve as a quality control check.
2.5 Estimation of daily food consumption and body weight of consumers
A one-time survey was undertaken in August 2018 to determine the quantity of peanuts consumed by individuals within farming households of Chipata, Lundazi, and Katete districts of Eastern Province. The study focused on raw peanut grain, peanut butter, roasted peanuts, and peanut powder, the most typical forms of peanuts consumed in the province. Dietary intake was based on the weighing method for each selected peanut product (Alamu et al., 2020) following the farmers’ estimation of quantities consumed per day. The estimated quantity for consumption indicated by each farmer was weighed and recorded. For children, caretakers and/or mothers were asked to estimate the quantity given to each child per day. Overall, 322 respondents participated in the study, among which 138 respondents were from Chipata, 113 from Katete, and 71 from Lundazi. The body weight (bw) of each respondent was measured. Ethical approval was issued by the University of Zambia Biomedical Research Ethics Committee.
2.6 Risk assessment: dietary exposure and potential impact on health
Aflatoxin dietary exposure risk was assessed only for the three districts (i.e., Chipata, Lundazi, and Katete) in which peanut consumption data were collected. The average peanut consumption data for raw grain and peanut powder and the average bw for the different age groups used in the analyses are presented in the Results section. The AFB1 value was obtained for each sample by halving the AF value. Dietary exposure to AFB1 was estimated using the probable daily intake (PDI) approach. For this, the product of mean toxin concentration and peanut consumption rate was divided by the bw as indicated in Equation (1). The margin of exposure (MOE) was then calculated using a Benchmark Dose Lower Limit (BMDL) of 170 ng/kg/bw/day [European Food Safety Authority (EFSA), 2007] (Equation 2). The risk of public health concern was considered when the MOE was lower than 10,000 and/or aflatoxin exposure exceeded 0.017 ng/kg/bw/day.
Where;
PDI = probable daily intake for AFB1; μ = mean AFB1 level; C = quantity of peanuts consumed in each district; and Bw = average body weight for the different age groups.
The risk of developing liver cancer (i.e., aflatoxin-induced hepatocellular carcinoma (HCC) rates in the population) attributed to peanut consumption in the three districts across the different age groups was estimated for AFB1 by obtaining the product of AF potency and mean PDI. The average potency (AP) was computed using (Equation 3) from individual potencies of hepatitis B virus surface antigen (HBsAg)-positive and HBsAg-negative groups. In the analysis, the AFB1 carcinogen potency prior value of 0.02624 cases per year per 100,000 persons in ng/kg bw/d for Zambians aged 15–59 years was considered based on an estimated hepatitis B virus (HBV) prevalence of 5.6% (for this age group) and HBV negative groups of 94.4% (Zambian Ministry of Health, 2018; Nshimbi and Ngoma, 2019).
2.7 Data analysis
Aspergillus population densities were computed as colony-forming units (CFU)/g of sample using the formula (CFU = [dilution factor × number of colonies] divided by sample weight). The dilution factor was computed by dividing the suspension volume by the plated volume. For a species composition, the frequency was computed as the number of given species divided by the total number of isolated species × 100. All samples in the dataset containing AF values below 2 μg/kg were replaced with zero. The CFU and Aspergillus species characterization datasets were pooled for the two seasons, while that of aflatoxin level was not pooled between the two seasons before conducting statistical analyses. The datasets were then subjected to normality and homogeneity of variance tests using the Shapiro–Wilk and Levene’s test, respectively. Thereafter, the AF and CFU/g datasets were log-transformed before conducting an analysis of variance (ANOVA). Means were separated using Tukey’s studentized range test (α = 0.05). All the analyses were carried out using SAS v9.4. Descriptive analyses were also carried out to determine the mean (SD) for food consumption data. To assess the impact of AF on health (exposure potencies), Equations (1)–(3) were used.
3 Results
3.1 Effect of conservation agriculture practices on Aspergillus spp. population densities
Aspergillus spp. propagules were present in all soil and grain samples. Irrespective of the AEZ or farming technique, CFU/g of Aspergillus spp. in soil ranged from 10 to 7,400 (mean = 295 CFU/g) and 10 to 3,760,000 (mean = 67,726 CFU/g) in grain. Densities in the soil significantly varied by location (F = 9.55, df = 8; p < 0.0001), farming technique (F = 7.01, df = 2; p = 0.0011), and AEZ (F = 16.33, df = 1; p < 0.0001).
Overall, AEZ II soils contained significantly higher Aspergillus spp. densities than AEZ III, while conventional tillage systems on ridges (CT) supported higher densities than soils from CAB or AGF farms (Table 1). The densities were influenced by the interaction between farming technique and location (F = 3.86, df = 16, p < 0.0001), but not between AEZ and farming technique (p > 0.05). Apart from Mpika and Sinda, in which no statistical difference occurred in fungal population density among farming techniques, significant differences (p < 0.05) existed in the fungal densities among the farming techniques for the other seven districts. Compared to CAB, fungal density was higher in the CT system in Chama, Katete, Mambwe, Nyimba and Petauke (data not shown). In Lundazi, AGF contained higher fungal densities than CA but was not significantly different from CT (data not shown).
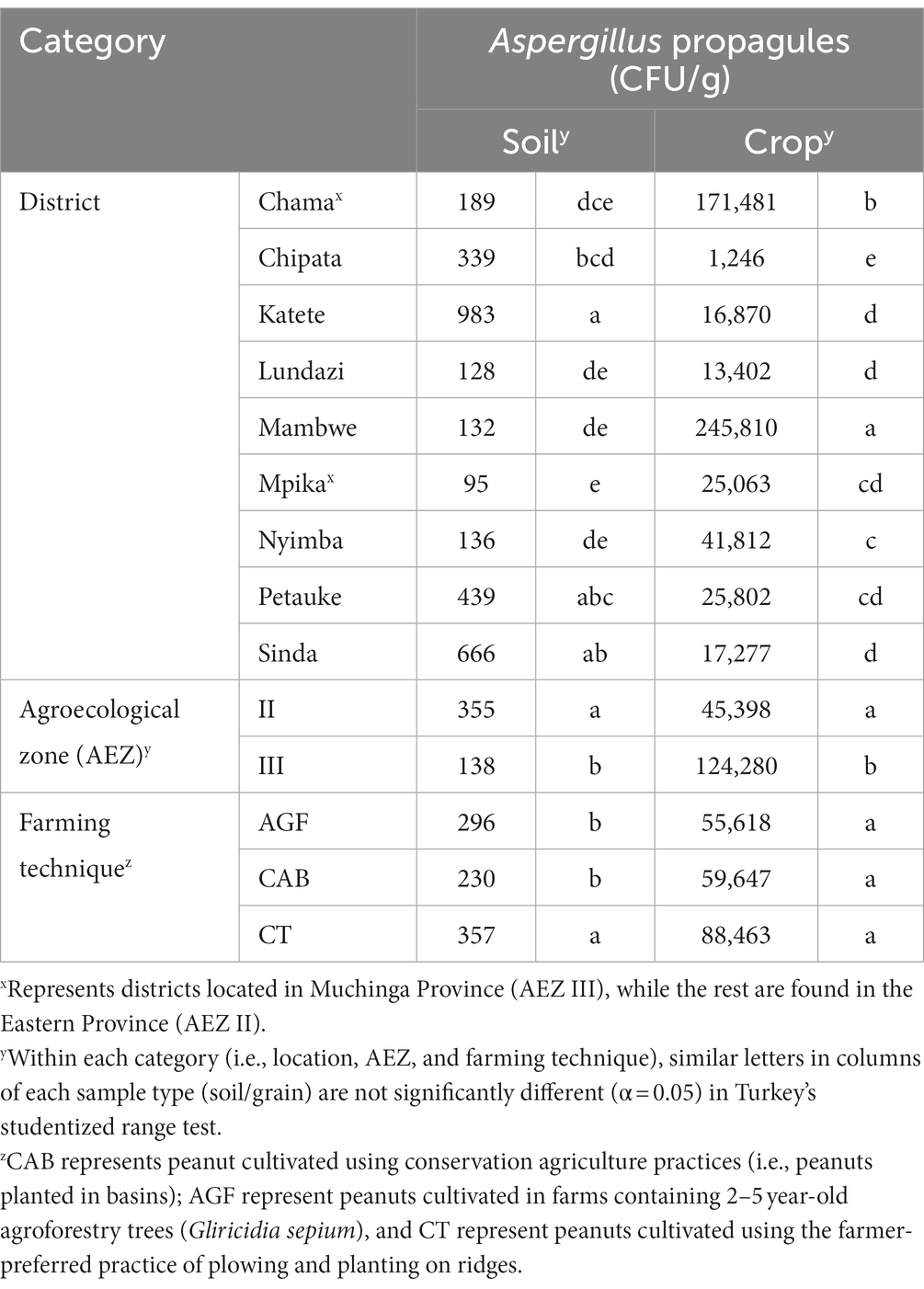
Table 1. Densities of Aspergillus spp. in soil samples cropped to peanuts and in harvested peanut grain samples during the 2017/2018 season expressed as colony forming units (CFU)/g.
Equally, fungal densities in grain depended on AEZ (F = 38.32, df = 1; p < 0.0001), location (F = 8.35, df = 8; p < 0.0001) but not on farming techniques (p > 0.05). Peanuts from AEZ II had significantly higher densities than peanuts from AEZ III. Mambwe peanuts had the highest densities, while Chipata peanuts had the least (Table 1). The densities in kernels were also affected by the interaction between AEZ and farming technique (F = 5.37, df = 2; p = 0.0049) and location and farming technique (F = 3.43, df = 16, p < 0.0001). In AEZ III, fungal densities were similar among farming techniques. In AEZ II, fungal densities were higher in CT soils compared with CAB farms (data not shown).
3.2 Effect of conservation agriculture on Aspergillus spp. profile
All soil and grain samples were associated with at least one of the main aflatoxin-producing Aspergillus spp. Among these were Aspergillus flavus L morphotyope, A. parasiticus, and fungi with S morphology. Overall, A. flavus was recovered from 49% of soil samples. In contrast, 89 and 88% of soil samples contained A. parasiticus and fungi with S morphology, respectively. Irrespective of farming technique, Aspergillus species composition was dominated by fungi with S morphology (55%), followed by A. parasiticus (34%) and A. flavus L morphotype (11%) (Figure 2).
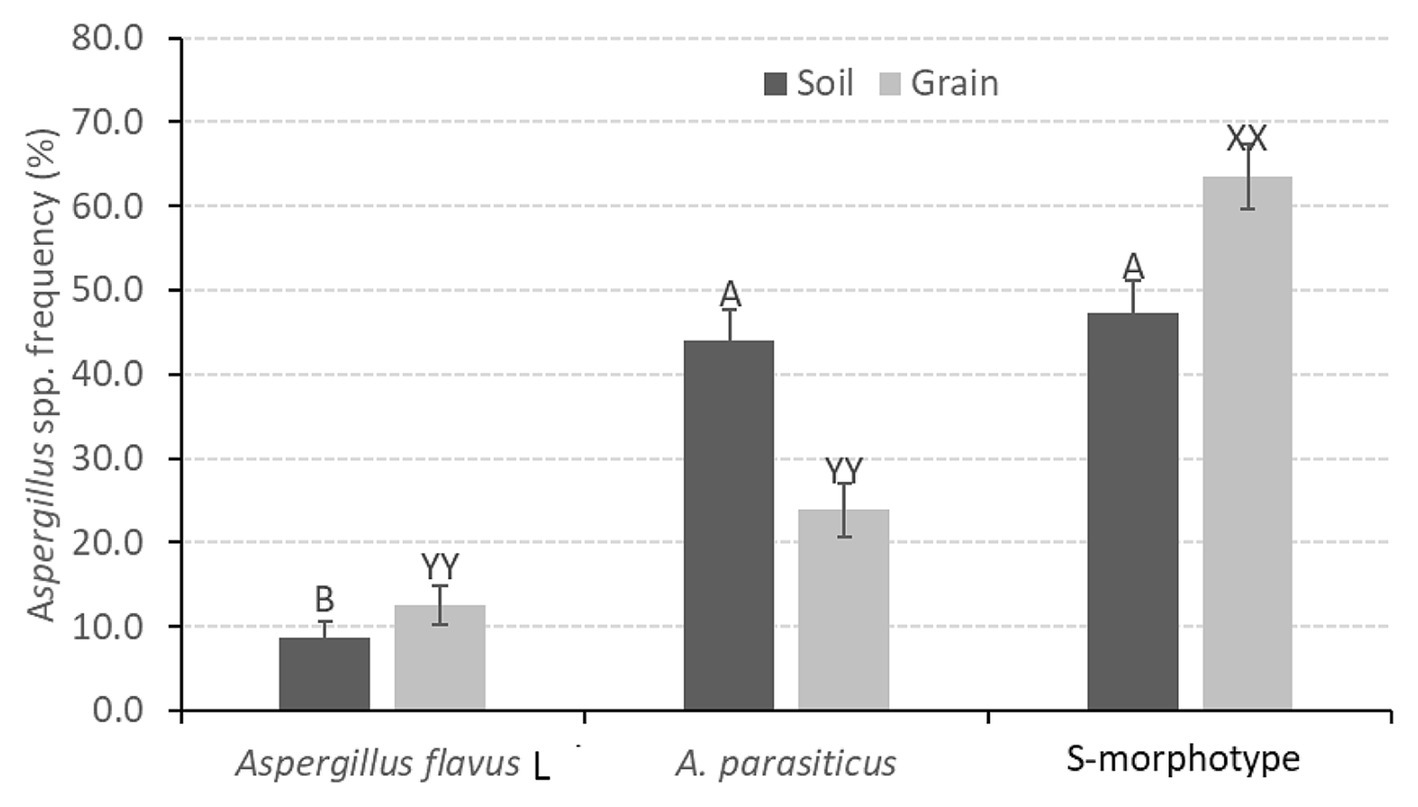
Figure 2. The distribution of Aspergillus spp. in soil and peanut grain samples collected from Eastern and Muchinga provinces of Zambia following the 2017/2018 cropping season. Bars followed by the same letter for the two sample types are not significantly different at p = 0.05, Tukey’s studentized range test.
In soil, Aspergillus spp. distribution depended on the fungal type (F = 18.36, df = 2; p < 0.0001) but not on location (F = 0.76, df = 8; p = 0.63) or farming technique (F = 0.94, df = 2; p = 0.39). The occurrence and distribution of fungi with S morphology and A. parasiticus were similar but significantly higher than that of A. flavus L morphotype (p < 0 0.0001) (Figure 3).
Alhough there was no interaction between location and farming technique (F = 0.62, df = 16; p = 0.86), Aspergillus spp. distribution in soil was influenced by the interaction between location and fungal type (F = 4.44, df = 16; p < 0.0001) as well as between fungal type and farming technique (F = 2.63, df = 4; p = 0.0376). In CT and CAB farms, the densities of A. parasiticus and fungi with S morphology were similar but statistically higher than that of A. flavus L morphotype. In AGF farms, the frequency of A. flavus was similar to A. parasiticus but significantly lower than fungi with S morphology (Figure 3).
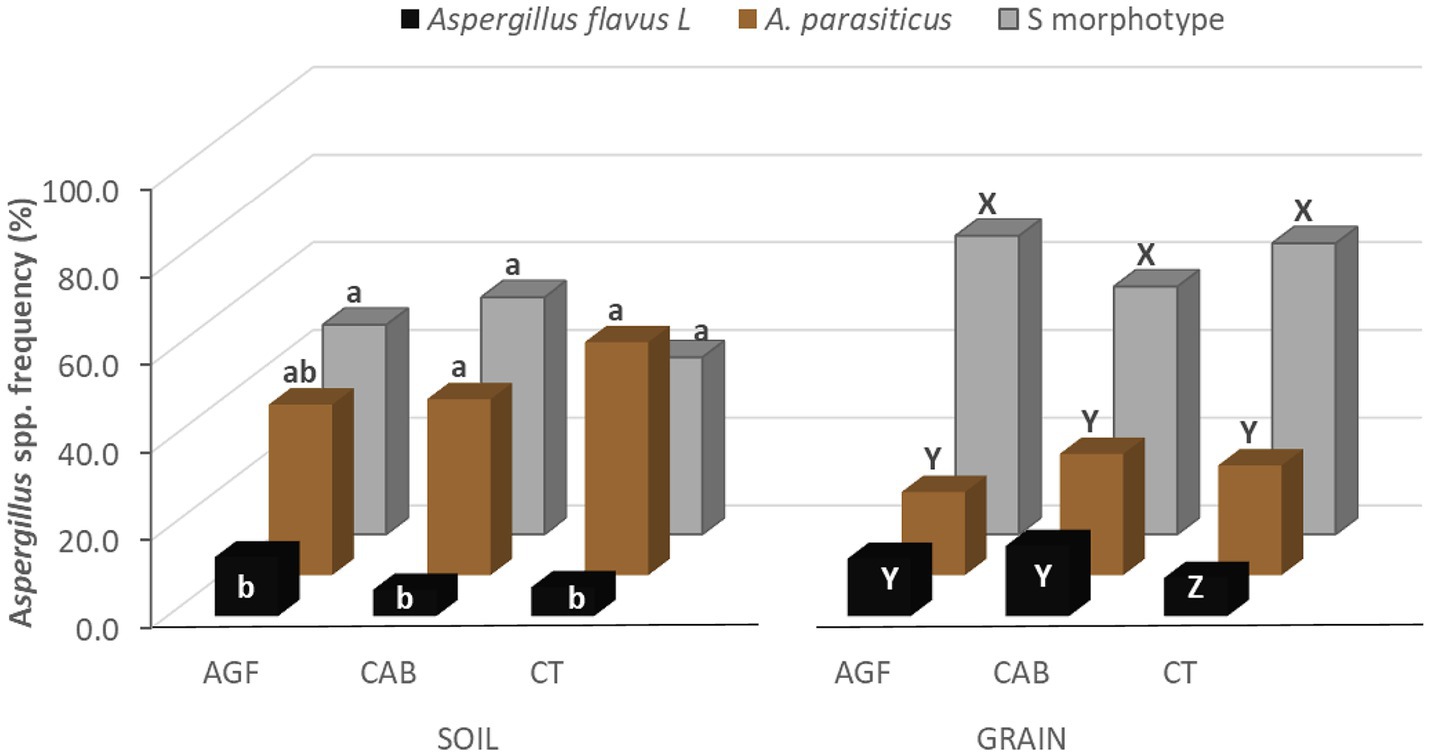
Figure 3. The effect of the farming technique on Aspergillus profile in soil and peanut samples collected from Eastern and Muchinga provinces of Zambia. The CAB represents peanuts cultivated using a conservation agriculture practice (i.e., peanuts planted in basins); AGF represents peanuts cultivated in farms containing 2–5-year-old agroforestry trees (Gliricidia sepium), and CT represents peanuts cultivated using the farmer-preferred practice of plowing and planting on ridges. For each sample type and farming technique, bars with the same letters are not significantly different at p = 0.05, Tukey’s studentized range test.
Across districts, the occurrence and distribution of the three Aspergillus types in soil samples from Chama, Katete, Chipata, Lundazi, and Mambwe were dominated by fungi with S morphology and significantly higher than A. flavus L morphotype (p < 0.0001). In Mpika, Nyimba, Petauke, and Sinda, A. parasiticus was the dominant Aspergillus spp. (data not shown).
Aspergillus flavus L morphotype was not recovered from 45% of the peanut samples (i.e., 10 CAB, 11 AGF, and 16 CT samples). Fungi with S morphology were detected in all samples except in 2 AGF, 2 CAB, and 3 CT kernel samples. Aspergillus parasiticus was not recovered from 22% of kernel samples (i.e., 9 AGF, 7 CAB, and 4 CT samples). Although statistically lower than the frequency of fungi with S morphology, the frequencies of A. parasiticus and A. flavus in kernels were not significantly different (Figure 3).
Equally, Aspergillus spp. distribution in kernel samples depended on the fungal type (F = 111.13, df = 2; p < 0.0001) but not on AEZ (F = 0.04, df = 1; p = 0.84), location (F = 1.06, df = 2; p = 0.38) or farming technique (F = 0.54, df = 2; p = 0.58). Aspergillus spp. distribution, however, depended on the interaction between location and fungal type (F = 12.57, df = 16; p < 0.0001), location and farming technique (F = 13.89, df = 16; p < 0.0001) and fungal type with farming technique (F = 7.75, df = 4; p < 0.0001). In peanuts from CT farms, the frequency of fungi with S morphology was significantly higher than that of A. parasiticus, which in turn was higher than that of A. flavus L morphotype (p < 0.0001). For the other AGF and CAB kernel samples, A. parasiticus and A. flavus frequencies were similar but statistically lower than fungi with S morphology (Figure 3). Across districts, the frequency of A. flavus L morphotype and A. parasiticus in peanuts were similar but significantly lower than that of the fungi with S morphology in Chama, Katete, Mambwe, and Mpika (p < 0.0001). In Chipata, Lundazi, and Petauke, the frequency of fungi with S morphology was significantly higher than that of A. parasiticus, which in turn was higher than the A. flavus L morphotype. In Nyimba, no significant difference was observed in fungal type distribution. In Sinda, the population of the S morphology fungi was similar to the A. flavus L morphotype but higher than A. parasiticus (data not shown).
3.3 Effect of CA on peanut aflatoxin levels
Aflatoxin incidence and contamination levels varied between the two sampling periods, with low incidence and severity recorded in the 2016/2017 cropping season (incidence = 37.8%) compared to the 2017/2018 cropping season (incidence = 83.0%) (F = 31.87, df = 1; p < 0.0001). Peanuts harvested following the 2017/2018 cropping season contained at least three times higher aflatoxin levels (range = ND-5,350 μg kg−1; mean = 165.2 μg kg−1) than those harvested in 2016/2017 (range = ND-1,308 μg kg−1; mean = 32.4 μg kg−1). The proportion of non-compliant peanut samples as per the European Union (EU; 4 μg kg−1), Common Market for Eastern and Southern Africa (COMESA (provisional) = 10 μg kg−1), and the US-Federal Drugs Administration (US-FDA; 20 μg kg−1) regulatory limits was moderate across the districts but depended on the season. Peanuts from 2016/2017 had less probability of rejection than those from 2017/2018 (Table 2).
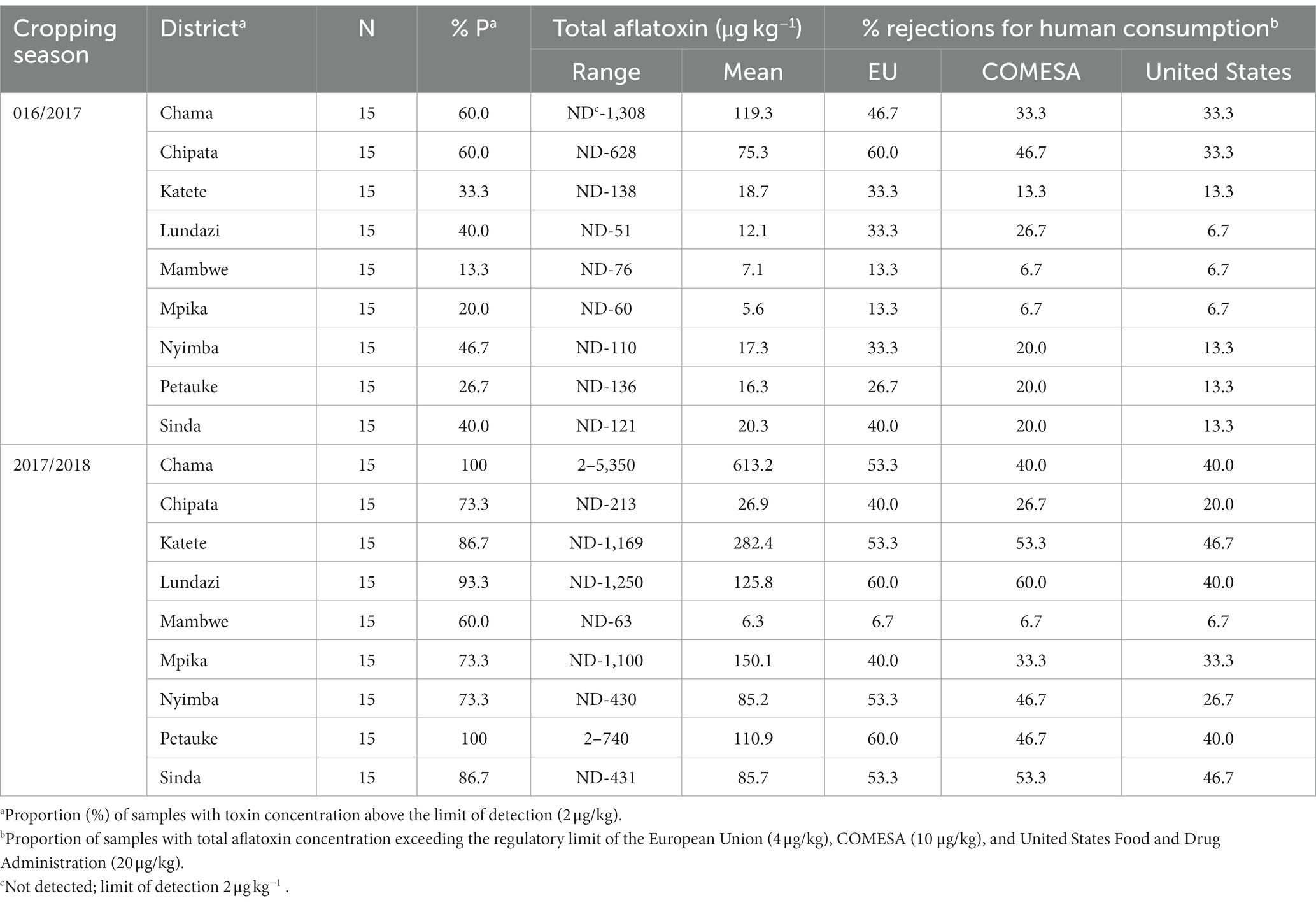
Table 2. Level of total aflatoxins detected in peanuts and the potential of rejection due to aflatoxin contamination in different districts of Zambia during the 2016/2017 and 2017/2018 cropping seasons.
Aflatoxin levels also depended on planting time (F = 31.6, df = 1; p < 0.0001), peanut variety (F = 2.69, df = 7, p = 0.0113), drying technique (F = 5.07, df = 3, p = 0.0018) and location (F = 2.68, df = 8; p = 0.0079), but not on the farming technique (p > 0.05). Late planting from 20th December onwards (mean AF = 98.3 ± 27.7 μg kg−1) supported higher AF than planting before mid-December (mean AF = 71.8 ± 9.6 μg kg−1) or in November (mean AF = 66.9 ± 13.6 μg kg−1). Across districts, peanuts from Chama and Katete had higher AF than peanuts from Mambwe (Table 2).
Though AF levels depended on the cropping season, no interaction was observed between farming technique and season (p > 0.05). Analysis of pooled data showed that farming techniques did not affect AF levels (p = 0.97). Irrespective of the season, 32% (AGF), 31% (CAB), and 29% (CT) of peanuts had AF exceeding the 20 μg kg−1 US-FDA acceptable limit (Figure 4). About 15% of the samples had high AF levels, i.e., >100 μg kg−1. In the two years, there were 33–43%, 26–33% and 18–25% of samples not meeting EU, COMESA, and US-FDA regulatory limits, respectively (Table 3).
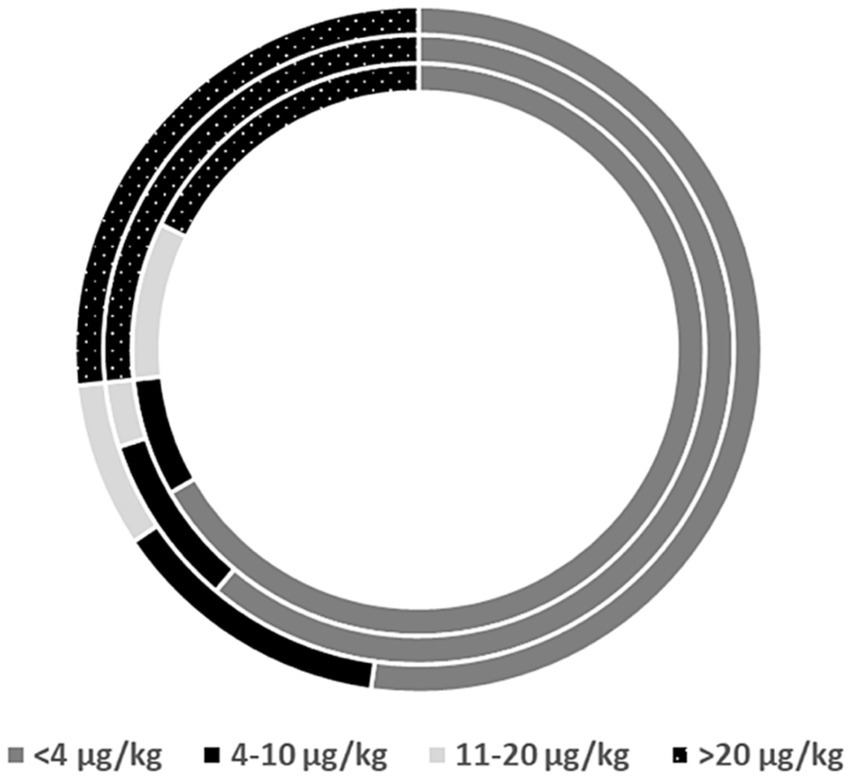
Figure 4. The proportion of peanut grain samples (inner circle AGF, middle circle CAB, outer circle CT) deemed safe as per the EU (4 μg/kg), COMESA (10 μg/kg), and United States (20 μg/kg) regulatory limits.
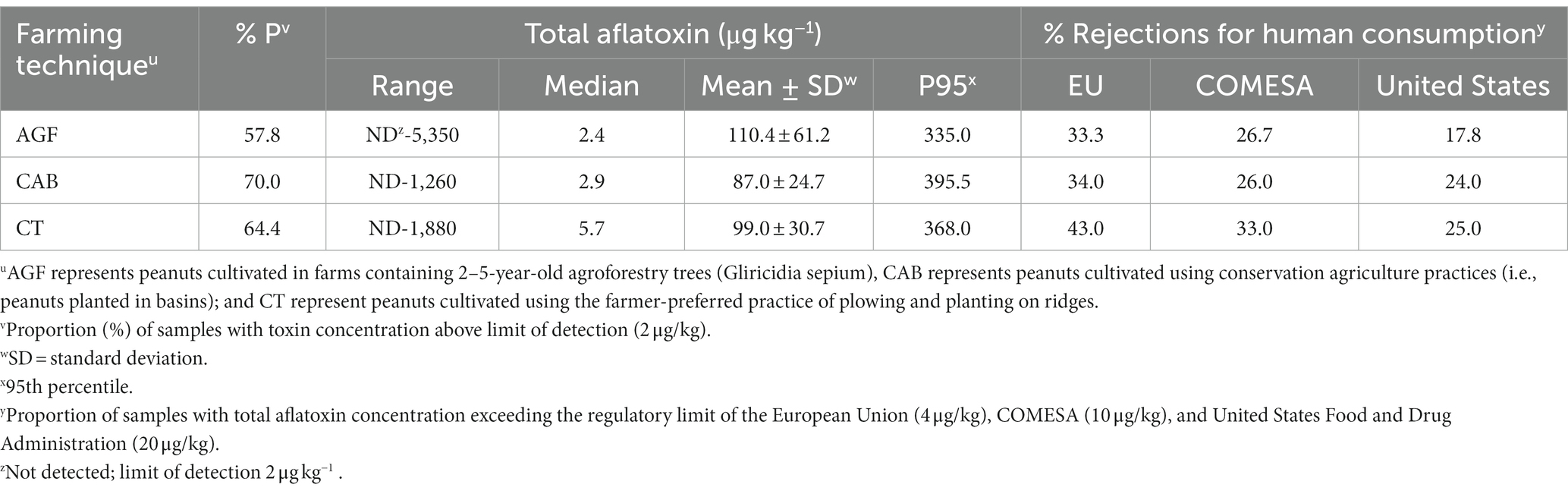
Table 3. The effect of conservation agricultural practices on aflatoxin levels in peanuts collected between 2017 and 2018 and the potential of rejection due to aflatoxin contamination.
3.4 Body weight of consumers
Of the 322 respondents persons, 138 were from Chipata, 113 from Katete, and 71 from Lundazi. There were 201 female and 121 male respondents. Their age ranged from 9 months to 87 years, with 13.7% children under 5 years of age, 4.3% children aged 6–9 year, 23% adolescents (10–19 year), 18.9% young adults (20–35 year), 24.2% adults (36–55 year), and 15.8% seniors (> 55 year). The average bw of respondents belonging to each category is presented in Figure 5.
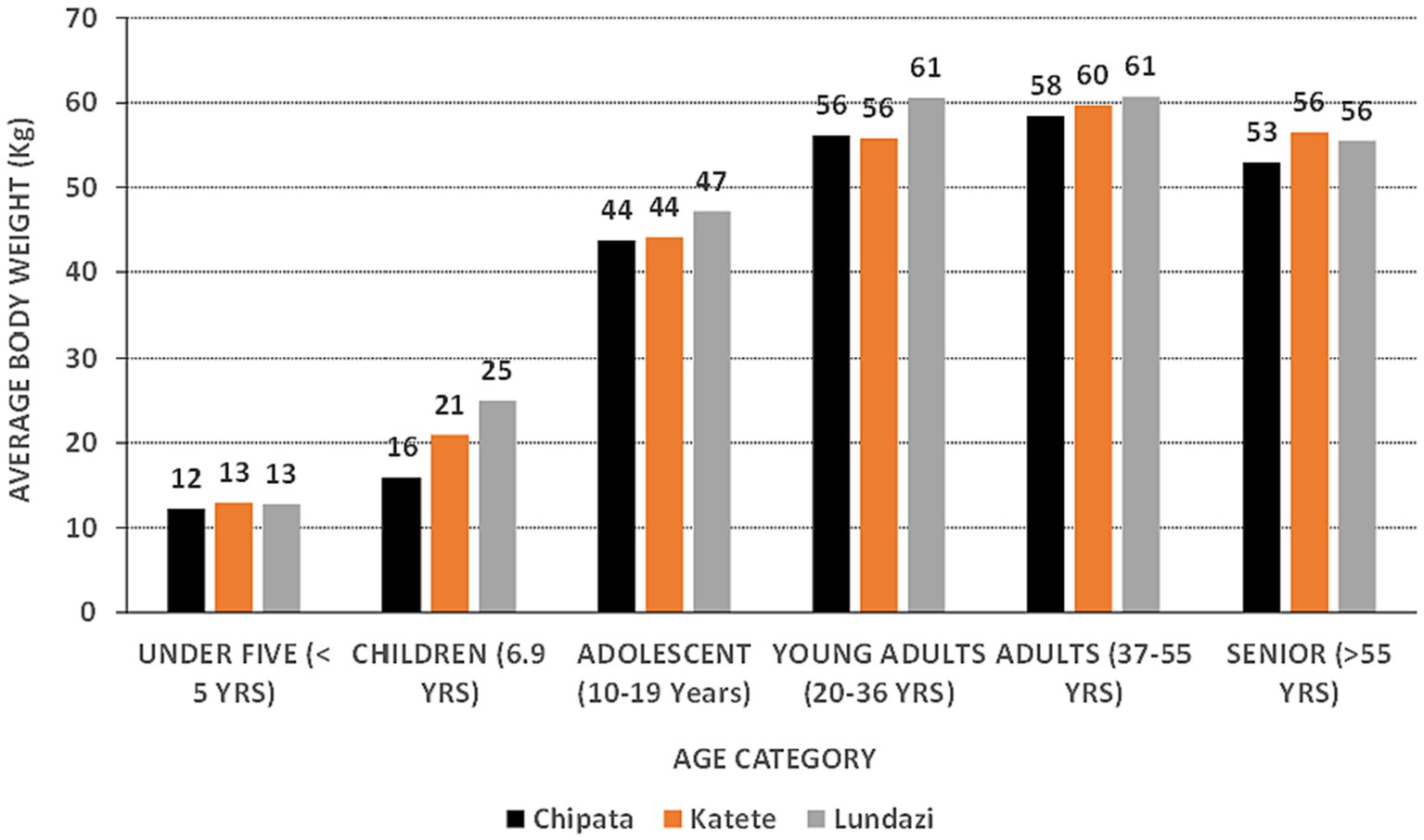
Figure 5. The estimated average body weights of peanut consumers in three districts of the Eastern Province of Zambia.
3.5 Consumption pattern of peanuts in Eastern Province
All respondents reported that peanut is part of their dietary and nutritional requirements. In the Eastern province, peanuts may be consumed every week in different forms (boiled, roasted, eaten fresh, pounded into paste and added to relish dishes or samp-breakfast dish made from pounded fresh maize), and occasionally when used to make cimponde (peanut butter). Most households utilize peanuts daily or 2–3 times a week at harvest periods when peanuts are in plenty. Peanut consumption patterns, however, varied with location, age group, and form. Table 4 shows the quantity of peanut-based products consumed by different age groups in the selected districts. In all the districts and age groups, peanut butter was the least consumed peanut-based product by all age groups, with a consumption rate of 56.4–116.9 g/d. In the Katete district, for under-five children, children, and adolescents, peanut powder was the most consumed product (154.3 to 180.9 g/d), while for young adults and senior adults, the highest consumption rate was with raw nuts. In this district, adults consumed roasted nuts more (Table 4).
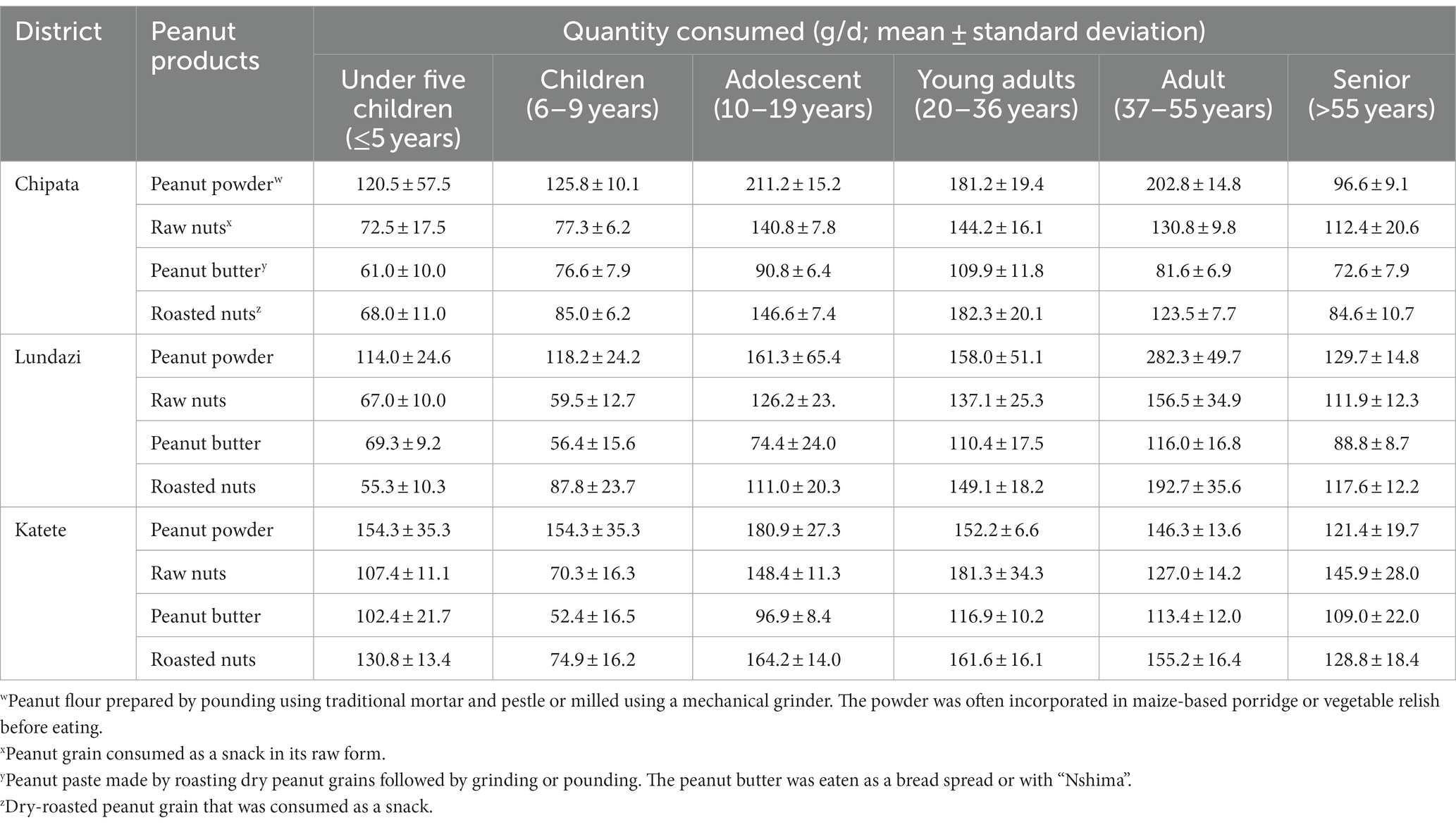
Table 4. Quantity of peanut-based products consumed by people of various age categories in farming households of three Eastern Province districts, Zambia.
3.6 Risk assessment: dietary exposure and potential health impact
AFB1 dietary exposure among consumers of peanut-based foods was in the range of 2 to 5,571 ng/kg bw/d but varied with the form of utilization, location, age group and season (Table 5). Generally, consumption of peanuts in its raw grain form resulted in lower exposure to AFB1 (avg. PDI = 12.7–724.4 ng/kg bw/d) than when utilized in powder form (PDI = 14.8–1,040.7 ng/kg bw/d) (Tables 5–7). In all districts and cropping seasons, children below five years of age exhibited the highest dietary exposure to aflatoxin, followed by those within the 5–9-year age bracket. On the other hand, the lowest dietary exposure in all districts within the two years was observed among those older than 55. Except for the Chipata district, consumer dietary exposure to aflatoxins was higher following the 2017/2018 cropping season than that of peanuts farmed during the 2016/2017 season (Tables 5–7).
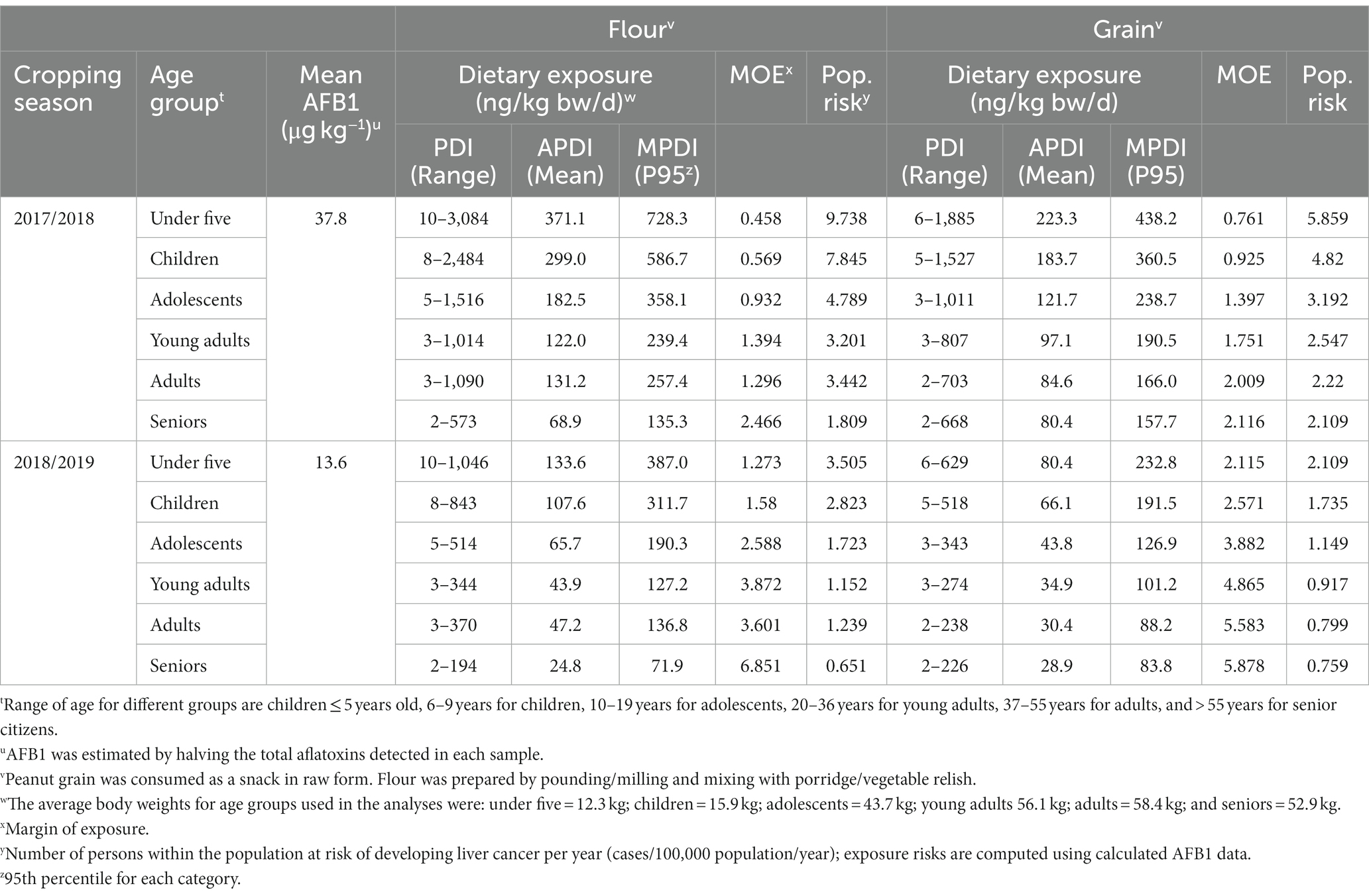
Table 5. Exposure risk assessment: probable daily intake (PDI), average PDI (APDI), and maximum PDI (MPDI) of aflatoxin B1 in peanuts produced and consumed as flour and grain by farming households in Chipata district of Eastern province of Zambia during 2017 and 2018.
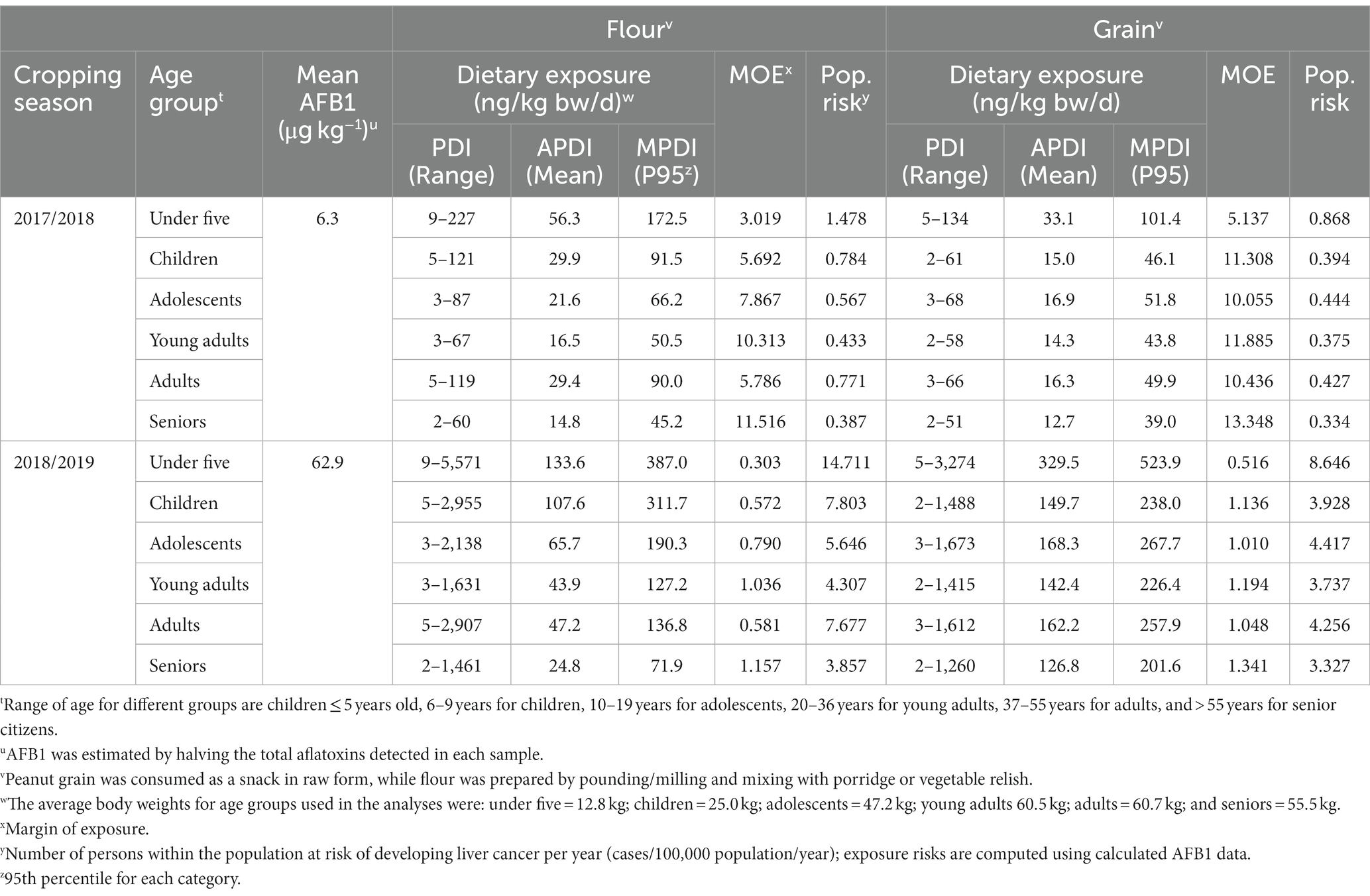
Table 6. Exposure risk assessment: probable daily intake (PDI), average PDI (APDI), and maximum PDI (MPDI) of aflatoxin B1 in peanuts produced and consumed as flour and grain by farming households in Lundazi district of the Eastern province of Zambia during 2017 and 2018.
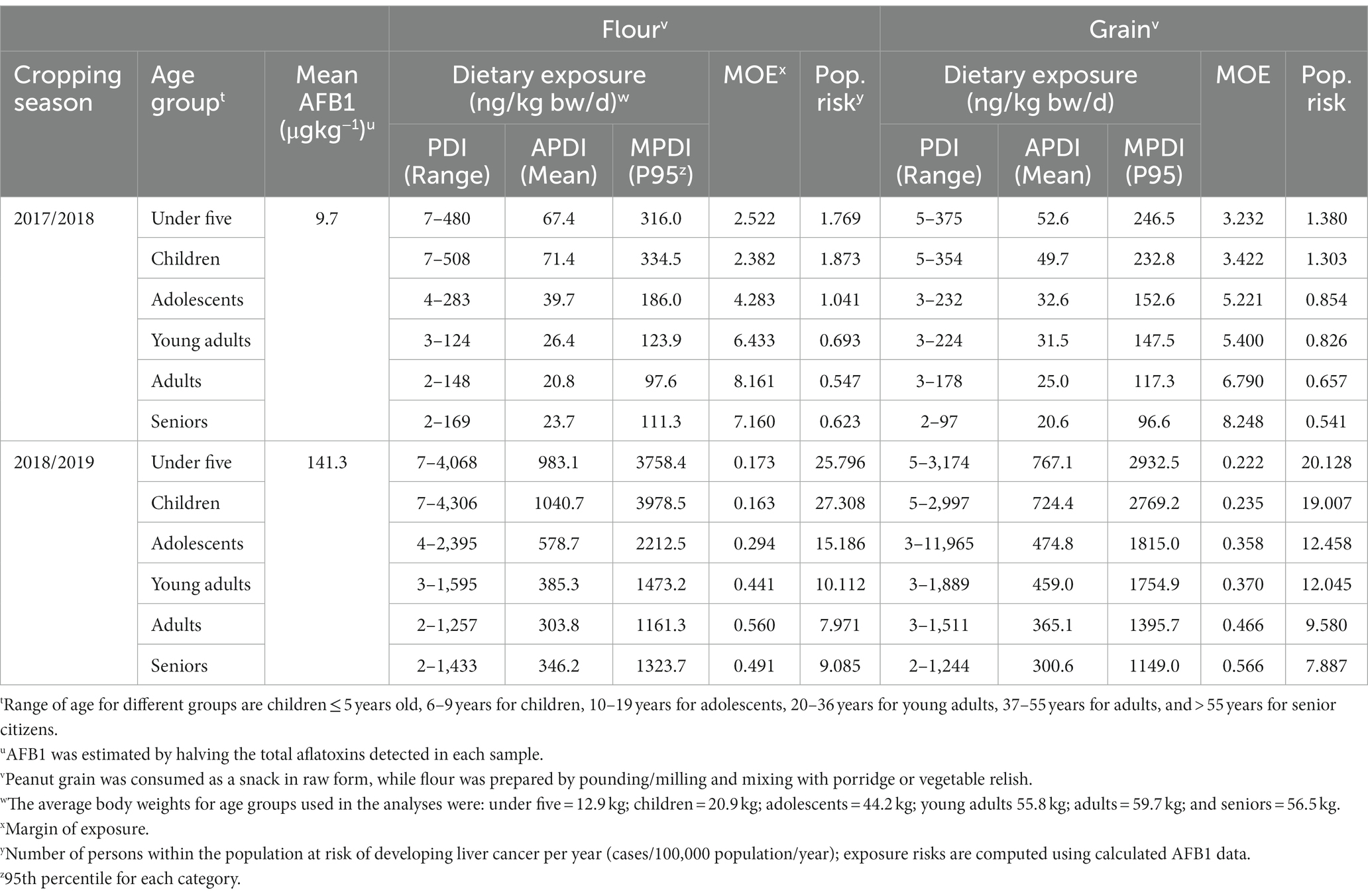
Table 7. Exposure risk assessment: probable daily intake (PDI), average PDI (APDI), and maximum PDI (MPDI) of aflatoxin B1 in peanuts produced and consumed as flour and grain by farming households in Katete district of the Eastern province of Zambia during 2017 and 2018.
The MOE (range = 0.222–13.348) exceeded the tolerable limit of 0.017 ng/kg bw/d for all age groups in all districts, greatly posing a hazardous health risk to the consumers. Thus, the consumption of peanuts in grain or powder form led to an estimated HCC rate of 0.387 to 27.308 cases/100,000 persons/yr (CPY) for powder and 0.334 to 20.128 CPY for kernels. Depending on the season and the form in which the peanut was consumed, children (1–9 years) with an estimated rate of up to 27.308 CPY were at the most significant risk of developing LC, followed by adolescents (up to 15 CPY). Across districts, peanuts collected from Katete (0.541–27.308 CPY) posed higher health risks to the public when compared to Chipata (0.651–9.738 CPY) and Lundazi (0.334–14.711 CPY) (Tables 5–7).
4 Discussion
Peanut production, spurred by private sector industry demand, remains one of the most important economic activities of smallholder farmers, especially women, in the Eastern and Muchinga Provinces of Zambia. The propagules of toxigenic Aspergillus spp. were highly prevalent in soil (95 to 983 CFU/g) and peanut samples (e.g., 1,246 to 245,810 CFU/g of peanut powder) collected from the two provinces of Zambia. Generally, the toxigenic Aspergillus species are soil-dwelling ubiquitous molds known to exist as saprophytes in different ecosystems (e.g., agricultural croplands) or as decay-causing molds on food grains, wild fruits and insects (Wilson et al., 2002; Horn, 2003; Kachapulula et al., 2017b). In Zambia, Aspergillus spp. are common in maize and peanut farms as well as in uncultivated forest soils in various AEZs (Kachapulula et al., 2017b). Such findings confirm the diverse ecologies in which these fungi can thrive.
In our study, it was observed that soils and peanut samples collected from AEZ II supported higher Aspergillus propagules than AEZ III. This is in line with the findings of Kachapulula et al. (2017a), wherein aflatoxin prevalence and severity were lower (probably due to low Aspergillus load in that environment) in the high-rainfall belt of Zambia when compared to the other AEZs. A study by Monyo et al. (2012) also showed that populations of aflatoxigenic fungi were higher in the hotter regions of neighboring Malawi. Contrary to our findings, Njoroge et al. (2016) reported higher Aspergilli populations from the cooler plateau regions compared to the hot Luangwa Valley; however, in that study, flooding had occurred in that Valley before soil sampling, which may have affected the fungal population densities in the area. Aspergillus spp. exist as aerobic fungi in the soil’s upper profiles. Environmental factors like waterlogging or deposition of alluvial soils from floods can significantly reduce the population in the soil (Horn, 2003). Soils collected from the conventional tillage of growing on ridges (CT) supported a higher Aspergillus population than those obtained from CAB or AGF farms (i.e., those containing G. sepium trees). Soil fertility factors such as organic matter content, nitrate and extractable phosphorus are generally known to affect Aspergillus spp., Fusarium spp., and the soil’s total fungal density. For instance, in Mississippi, Zablotowicz et al. (2007) observed the highest A. flavus populations in high organic matter plots that were under zero-tillage management systems.
The Aspergillus species profiling revealed that the soils and peanuts collected from the two provinces comprised A. flavus L-strain, A. parasiticus, and the S-morphotype, with A. flavus being the least encountered species. According to Kortei et al. (2021), peanut kernels habor a diversity of toxigenic and pathogenic fungal species including mycotoxigenic Aspergillus spp. which supports our current findings. The occurrence and distribution of the three Aspergillus spp. in soil samples were location-dependent. However, there was no effect of the farming technique on Aspergillus species occurrence and distribution, with all the tillage systems supporting relatively lower densities of A. flavus. This may suggest that under the current farming system, using agroforestry trees in combination with conservation practices like digging basins may have minimal effect on the Aspergillus population density or its composition within the soil environment.
Depending on the location, aflatoxin prevalence was observed to vary from 20 to 60% and 60 to 100% during the 2016/2017 and 2017/2018 cropping seasons, respectively. However, seasonal variability played a significant role in shaping peanut aflatoxin contamination levels, with peanuts cultivated during the drier farming season (i.e., 2017/2018) exhibiting higher incidence and contamination severity than the 2016/2017 cropping season-farmed peanuts that received between normal to above-average rainfall. Such seasonal effects, primarily attributed to climatic variability during the cropping season, are not unique and have been observed both in Zambia and elsewhere in the region (Mukanga et al., 2019; Akello et al., 2021). For instance, a study conducted in Zimbabwe showed that aflatoxin levels in maize harvested following a wet season were significantly lower than in crops harvested following a suboptimal rainfall season (Akello et al., 2021). Early planting of peanuts, i.e., before mid-December, supported less aflatoxin build-up in harvested peanuts, which was also earlier observed in the same province (Njoroge, 2018). An on-station experiment conducted in the Eastern Province of Zambia revealed that a delay in planting peanuts by about 2 weeks after the onset of rains significantly increased aflatoxin contamination levels in harvested peanuts (Njoroge, 2018). It has been postulated that the high risk of aflatoxin contamination in late-planted crops is attributed to erratic rains, especially if it ends before the crop has reached physiological maturity. Apart from aflatoxin contamination risks, the late-planted crop may also have reduced productivity attributed to drought stress. Generally, the smallholder farming communities of Malawi and Zambia prioritize the sowing of maize (a food security crop) and tobacco (a cash crop) over peanuts, which may be planted up to 2 weeks or more after the onset of the rainy season. Therefore, timely planting at the onset of the rains is important in peanut farming for maintaining crop productivity and food safety.
Even though higher densities of aflatoxin-producing Aspergillus were found in CT farms compared to the other techniques, planting in basins had no effect on peanut aflatoxin prevalence or severity. According to Mukanga et al. (2019), water harvesting techniques like tied ridges utilize “small ponds” between the rows for retaining moisture in the soil, especially during plant growth and pod development, thereby reducing drought stress and aflatoxin build-up in the harvested peanuts. In our study, planting in basins and incorporation of Gliricidia probably had minimal effect on water runoff nor supported sufficient moisture retention in the soil to reduce aflatoxin accumulation in the harvested peanuts.
For the first time, we document an estimated quantity of peanut-based products consumed in the Eastern province of Zambia, a parameter that is required for computing aflatoxin exposure risks attributed to the consumption of such products. Respondents reported consuming peanuts in their raw form or processed as powder and/or peanut butter. The high level of consumption of peanuts and their various forms agrees with a previous report (Mofya-Mukuka and Shipekesa, 2013).
Peanut plays an integral role in livelihood and is a vital food component in the healthy diets of most rural communities (Mofya-Mukuka and Shipekesa, 2013; Jung et al., 2020). Also, a significant increase in the nutritional quality of diets is expected with peanut consumption because peanuts are known to have cardio-protective, anti-inflammatory, and antioxidant properties that may influence blood lipid levels, endothelial function, and inflammatory biomarkers (Mahatma et al., 2016). However, variability in peanut consumption was observed across age groups and locations. Peanut powder, for instance, was observed as the most consumed peanut-based product in the three districts for under-five children (≤ 5 years), children (6–9 years), and adolescents (10–19 years). Zonal variability and consumption patterns of peanuts were also observed in Benin and Togo where peanut butter was least preferred (Gong et al., 2003) as in the Eastern provinces of Zambia. On the contrary, peanut butter is the most consumed peanut-based product in Ghana because the populace uses it for soup preparation (Jolly et al., 2008). In agreement with the findings of our study, He et al. (2013), Jolly et al. (2006), and Gama et al. (2018) observed that the age of the respondents significantly affects the rate of consumption of peanut butter as age groups younger than 22 or older than 35 are less likely to eat peanut butter.
The study revealed that the consumption of peanuts in various forms would lead to high exposure to AFB1 among consumers in the studied districts. With an estimated PDI ranging from 12.7 to 1040.7 ng/kg bw/d and a MOE of 0.222 to 13.348 ng/kg bw/d, consumption of peanuts in their raw or processed form posed a high health hazard to the different age groups. Similarly, a study by Kortei et al. (2021) reported that consumption of raw peanuts sourced from local markets could result in high exposure to aflatoxin B1 with risks of causing adverse health effects to Ghanaian consumers belonging to different age groups (i.e., infants (0–52 months, children (5–11 years), adolescents (12–18 years), and adults (18–60 years). According to several studies, exposure to aflatoxins at levels greater than 1 μg/kg bw/d through consumption of aflatoxin-contaminated food may have adverse health effects on consumers and lead to aflatoxin-related illnesses (Wu and Khlangwiset, 2010; Hove et al., 2016; Adetunji et al., 2017). This was evident in the current study in which a high risk of chronic exposure to aflatoxins was computed for all the different age groups in the sampled districts. During the two-year study period, cancer risks in the range of 0.334 to 27.308 cases/100,000 persons/yr. were calculated among consumers depending on cropping season, location and the different age groups. In a recent study, Ghanaian infants, children, adolescents and adults had an estimated population exposure risk of 1.19 × 10−3, 6.34 × 10−4, 3.56 × 10−4, and 2.69 × 10−4 CPY, respectively. While consumption of peanut powder posed a higher health risk than in its raw grain form, children followed by the adolescent category were at higher risk of developing aflatoxin-related illnesses. It has been reported that among the mothers from Eastern and Southern Provinces of Zambia, the addition of peanut flour to both porridge and vegetable dishes was a common practice and thus may be the primary exposure form for the children (Alamu et al., 2020). Generally, children are known to be more susceptible to diseases, including aflatoxin-related ill effects, because of their undeveloped and/or weak immune systems. The high exposure risk among children and low exposure risk among senior citizens could be attributed to the quantity of peanut-based foods consumed. The children receiving complementary foods or in the weaning stage are offered peanut-based porridge several times a day, increasing the quantity consumed. On the other hand, the elderly generally appeared to consume less peanut-based products, signifying less exposure. Also, the consumption of peanuts in Katete district posed the most significant health hazard. These results confirm the possible health risks across age groups associated with the consumption of aflatoxin-contaminated foods.
5 Conclusion
The current study demonstrated that Aspergillus propagules in conventionally tilled land is generally higher than those in conservation agriculture-farmed land. Overall, fungi with S morphology were the dominant Aspergillus fungal type followed by A. parasiticus and A. flavus L morphotype. We found no effect of farming technique on Aspergillus spp. occurrence and distribution in all tillage systems. Equally, there was no effect of the farming technique on aflatoxin level. For the first time, we document an estimated quantity of peanut-based products consumed in the Eastern province of Zambia, a critical parameter in computing exposure risks attributed to the consumption of such a product. Consumption of peanuts in various forms can lead to high exposure to AFB1 and cancer risks in Eastern and Muchinga Provinces. Given the high exposure of Zambia’s population, especially children, and that contaminated food is the major source of exposure, it is essential that appropriate technical, policy and institutional measures are promoted for aflatoxin mitigation in Zambia. Aflatoxin contamination is a multi-sectoral issue involving the agriculture, trade and health sectors. These three sectors must work together, using a Food Convergence Innovation concept (Ortega-Beltran and Bandyopadhyay, 2021), to reduce the high aflatoxin exposure and attendant cancer risks among children and other vulnerable populations.
Data availability statement
The raw data supporting the conclusions of this article will be made available by the authors, without undue reservation.
Ethics statement
The studies involving humans were approved by University of Zambia Biomedical Research Ethics Committee. The studies were conducted in accordance with the local legislation and institutional requirements. Written informed consent for participation in this study was provided by the participants and/or their legal guardians/next of kin.
Author contributions
JA: Writing – review & editing, Conceptualization, Funding acquisition, Project administration, Formal analysis, Investigation, Methodology, Resources, Supervision, Validation, Visualization, Writing – original draft, Data curation. EA: Formal analysis, Investigation, Methodology, Writing – original draft, Writing – review & editing, Data curation. CM: Investigation, Writing – original draft, Data curation. PK: Investigation, Writing – review & editing, Writing – original draft. MM: Writing – review & editing, Conceptualization, Methodology. HN: Conceptualization, Methodology, Writing – review & editing. DC: Conceptualization, Funding acquisition, Methodology, Writing – review & editing, Investigation, Project administration, Supervision, Writing – original draft. AO-B: Writing – review & editing, Data curation, Formal analysis. RB: Conceptualization, Writing – review & editing, Funding acquisition, Investigation, Methodology, Project administration, Supervision, Writing – original draft.
Funding
The authors declare financial support was received for the research, authorship, and/or publication of this article. This work was supported by the Deutsche Gesellschaft für Internationale Zusammenarbeit (GIZ) through grants from the Green Innovations Centers in Agricultural Systems, Zambia (Agreement No. 14.0967.1–114.00). Partial support was provided by the Bill & Melinda Gates Foundation (OPP1007117).
Acknowledgments
The authors appreciate the support provided by our national research partners, especially the assistance of the technical staff of COMACO and Zambia Agriculture Research Institute (ZARI), for the technical support rendered during the collection of crop samples and aflatoxin analyses. Equally, we are grateful to the government extension officers for the help with the identification of project sites as well as IITA staff for the technical support. The authors also thank Tunji Adejoro (IITA-Nigeria) for drawing the GIS map (Figure 1) in the manuscript.
Conflict of interest
The authors declare that the research was conducted in the absence of any commercial or financial relationships that could be construed as a potential conflict of interest.
Publisher’s note
All claims expressed in this article are solely those of the authors and do not necessarily represent those of their affiliated organizations, or those of the publisher, the editors and the reviewers. Any product that may be evaluated in this article, or claim that may be made by its manufacturer, is not guaranteed or endorsed by the publisher.
References
Adetunji, M. C., Atanda, O. O., and Ezekiel, C. N. (2017). Risk assessment of mycotoxins in stored maize grains consumed by infants and young children in Nigeria. Children 4:58. doi: 10.3390/children4070058
Akello, J., Ortega-Beltran, A., Katati, B., Atehnkeng, J., Augusto, J., Mwila, C. M., et al. (2021). Prevalence of aflatoxin-and fumonisin-producing fungi associated with cereal crops grown in Zimbabwe and their associated risks in a climate change scenario. Foods 10:287. doi: 10.3390/foods10020287
Alamu, E. O., Gondwe, T., Akello, J., Maziya-Dixon, B., and Mukanga, M. (2020). Relationship between serum aflatoxin concentrations and the nutritional status of children aged 6–24 months from Zambia. Int. J. Food Sci. Nutr. 71, 593–603. doi: 10.1080/09637486.2019.1689547
Andersson, J. A., and D'Souza, S. (2014). From adoption claims to understanding farmers and contexts: a literature review of conservation agriculture (CA) adoption among smallholder farmers in southern Africa. Agric. Ecosyst. Environ. 187, 116–132. doi: 10.1016/j.agee.2013.08.008
Bumbangi, N. F., Muma, J. B., Choongo, K., Mukanga, M., Velu, M. R., Veldman, F., et al. (2016). Occurrence and factors associated with aflatoxin contamination of raw peanuts from Lusaka district's markets, Zambia. Food Cont. 68, 291–296. doi: 10.1016/j.foodcont.2016.04.004
Bunyolo, A., Chirwa, B., and Muchinda, M. (1995). Agro-ecological and climatic conditions. Zambia Seed Technol. Handb., 19–23.
Cotty, P. J. (1994). Influence of field application of an atoxigenic strain of Aspergillus flavus on the populations of A. flavus infecting cotton bolls and on the aflatoxin content of cottonseed. Phytopathology 84, 1270–1277.
Cotty, P. J. (1989). Virulence and cultural characteristics of two Aspergillus flavus strains pathogenic on cotton. Phytopathology 79, 808–814.
Desmae, H., and Sones, H. (2017). Groundnut cropping guide. Nairobi, Kenya: Africa Soil Health Consortium.
European Food Safety Authority (EFSA) (2007). Opinion of the scientific panel on contaminants in the food chain on a request from the commission related to the potential increase of consumer health risk by a possible increase of the existing maximum levels for aflatoxins in almonds, hazelnuts and pistachios and derived products. EFSA J. 5:446. doi: 10.2903/j.efsa.2007.446
Gama, A. P., Adhikari, K., and Hoisington, D. A. (2018). Peanut consumption in Malawi: an opportunity for innovation. Foods 7:112. doi: 10.3390/foods7070112
Gong, Y. Y., Egal, S., Hounsa, A., Turner, P. C., Hall, A. J., Cardwell, K. F., et al. (2003). Determinants of aflatoxin exposure in young children from Benin and Togo, West Africa: the critical role of weaning. Int. J. Epidem. 32, 556–562. doi: 10.1093/ije/dyg109
He, J., Zhang, K. Y., Chen, D. W., Ding, X. M., Feng, G. D., and Ao, X. (2013). Effects of vitamin E and selenium yeast on growth performance and immune function in ducks fed maize naturally contaminated with aflatoxin B1. Livest. Sci. 152, 200–207. doi: 10.1016/j.livsci.2012.12.018
Horn, B. W. (2003). Ecology and population biology of aflatoxigenic fungi in soil. J. Toxicol. Toxin Rev. 22, 351–379. doi: 10.1081/TXR-120024098
Hove, M., De Boevre, M., Lachat, C., Jacxsens, L., Nyanga, L. K., and De Saeger, S. (2016). Occurrence and risk assessment of mycotoxins in subsistence farmed maize from Zimbabwe. Food Cont. 69, 36–44. doi: 10.1016/j.foodcont.2016.04.038
Jolly, P. E., Jiang, Y., Ellis, W. O., Sheng-Wang, J., Afriyie-Gyawu, E., Phillips, T. D., et al. (2008). “Modulation of the human immune system by aflatoxin” in Mycotoxins: Detection methods, management, public health and agricultural trade. eds. J. F. Leslie, R. Bandyopadhyay, and A. Visconti (Oxfordshire, UK: CAB International), 41–52.
Jolly, P., Jiang, Y., Ellis, W., Awuah, R., Nnedu, O., Phillips, T., et al. (2006). Determinants of aflatoxin levels in Ghanaians: sociodemographic factors, knowledge of aflatoxin and food handling and consumption practices. Int. J. Hyg. Environ. Health 209, 345–358. doi: 10.1016/j.ijheh.2006.02.002
Jung, M., Kim, J., and Ahn, S. M. (2020). Factors associated with frequency of peanut consumption in Korea: a national population-based study. Nutrients 12:1207. doi: 10.3390/nu12051207
Kachapulula, P. W., Akello, J., Bandyopadhyay, R., and Cotty, P. J. (2017a). Aflatoxin contamination of groundnut and maize in Zambia: observed and potential concentrations. J. App. Microbiol. 122, 1471–1482. doi: 10.1111/jam.13448
Kachapulula, P. W., Akello, J., Bandyopadhyay, R., and Cotty, P. J. (2017b). Aspergillus section Flavi community structure in Zambia influences aflatoxin contamination of maize and groundnut. Int. J. Food Microbiol. 261, 49–56. doi: 10.1016/j.ijfoodmicro.2017.08.014
Klich, M. A., and Pitt, J. I. (1988). Differentiation of Aspergillus flavus from A. parasiticus and other closely related species. Trans. Br. Mycol. Soc. 91, 99–108.
Komarek, A. M., Thierfelder, C., and Steward, P. R. (2021). Conservation agriculture improves adaptive capacity of cropping systems to climate stress in Malawi. Agric. Syst. 190:103117. doi: 10.1016/j.agsy.2021.103117
Kortei, N. K., Annan, T., Akonor, P. T., Richard, S. A., Annan, H. A., Wiafe-Kwagyan, M., et al. (2021). Aflatoxins in randomly selected groundnuts (Arachis hypogaea) and its products from some local markets across Ghana: human risk assessment and monitoring. Toxicol. Rep. 8, 186–195. doi: 10.1016/j.toxrep.2021.01.002
Kortei, N. K., Tetteh, R. A., Wiafe-Kwagyan, M., Amon, D. N. K., and Odamtten, G. T. (2022). Mycobiota profile, phenology, and potential toxicogenic and pathogenic species associated with stored groundnuts (Arachis hypogaea L.) from the Volta region, Ghana. Food Sci. Nutr. 10, 888–902. doi: 10.1002/fsn3.2719
Li, Y., Song, D., Liang, S., Dang, P., Qin, X., Liao, Y., et al. (2020). Effect of no-tillage on soil bacterial and fungal community diversity: a meta-analysis. Soil Tillage Res. 204:104721. doi: 10.1016/j.still.2020.104721
Mahatma, M. K., Thawait, L. K., Bishi, S. K., Khatediya, N., Rathnakumar, A. L., Lalwani, H. B., et al. (2016). Nutritional composition and antioxidant activity of Spanish and Virginia groundnuts (Arachis hypogaea L.): a comparative study. J. Food Sci. Technol. 53, 2279–2286. doi: 10.1007/s13197-016-2187-y
MAL (Ministry of Agriculture and Livestock). (2013). The crop forecasting survey report for the 2012/2013 agricultural season. Zambia: Lusaka.
Mhlanga, B., Pellegrino, E., Thierfelder, C., and Ercoli, L. (2022). Conservation agriculture practices drive maize yield by regulating soil nutrient availability, arbuscular mycorrhizas, and plant nutrient uptake. Field Crop Res. 277:108403. doi: 10.1016/j.fcr.2021.108403
Mofya-Mukuka, R., and Chisanga, B. (2014). October. Bridging the edible oil deficit: indaba agricultural policy research institute. In the 109th Zambia National Farmers Union National Congress, Mulungushi conference Centre, Lusaka).
Mofya-Mukuka, R., and Kuhlgatz, C. (2016). Impact of agricultural diversification and commercialization on child nutrition in Zambia: a dose response analysis. J. Agric. Sci. 8:60. doi: 10.5539/jas.v8n4p60
Mofya-Mukuka, R., and Shipekesa, A. M. (2013). Value chain analysis of the groundnuts sector in the Eastern Province of Zambia (no. 171869). Michigan State University, Department of Agricultural, food, and resource economics. https://ageconsearch.umn.edu/record/171869/files/wp78.pdf
Monyo, E. S., Njoroge, S. M. C., Coe, R., Osiru, M., Madinda, F., Waliyar, F., et al. (2012). Occurrence and distribution of aflatoxin contamination in groundnuts (Arachis hypogaea L) and population density of Aflatoxigenic aspergilli in Malawi. Crop Prot. 42, 149–155. doi: 10.1016/j.cropro.2012.07.004
Muimba-Kankolongo, A. (2018). Food crop production by smallholder farmers in southern Africa: Challenges and opportunities for improvement. London, U.K.: Academic Press.
Muimba-Kankolongo, A., Nkulu, C. B. L., Mwitwa, J., Kampemba, F. M., Nabuyanda, M. M., Haufroid, V., et al. (2021). Contamination of water and food crops by trace elements in the African Copperbelt: a collaborative cross-border study in Zambia and the Democratic Republic of Congo. Env. Adv. 6:100103. doi: 10.1016/j.envadv.2021.100103
Mukanga, M., Matumba, L., Makwenda, B., Alfred, S., Sakala, W., Kanenga, K., et al. (2019). Participatory evaluation of groundnut planting methods for pre-harvest aflatoxin management in Eastern Province of Zambia. Cah. Agric. 28:1. doi: 10.1051/cagri/2019002
Ngoma, H., Angelsen, A., Jayne, T. S., and Chapoto, A. (2021). Understanding adoption and impacts of conservation agriculture in eastern and southern Africa: a review. Front. Agron. 3:671690. doi: 10.3389/fagro.2021.671690
Njoroge, S. M. (2018). A critical review of aflatoxin contamination of peanuts in Malawi and Zambia: the past, present, and future. Plant Dis. 102, 2394–2406. doi: 10.1094/PDIS-02-18-0266-FE
Njoroge, S. M. C., Kanenga, K., Siambi, M., Waliyar, F., and Monyo, E. S. (2016). Identification and toxigenicity of Aspergillus spp. from soils planted to peanuts in eastern Zambia. Peanut Sci. 43, 148–156. doi: 10.3146/PS15-11.1
Njoroge, S. M., Matumba, L., Kanenga, K., Siambi, M., Waliyar, F., Maruwo, J., et al. (2017). Aflatoxin B1 levels in groundnut products from local markets in Zambia. Mycotoxin Res. 33, 113–119. doi: 10.1007/s12550-017-0270-5
Nshimbi, N., and Ngoma, A. (2019). Hepatitis B: vaccinate. Stop it in its tracks!!! Addressing the Hepatitis B prevalence in the adult population in Zambia. Health Press Zambia Bull. 3, 10–13.
Ortega-Beltran, A., and Bandyopadhyay, R. (2021). Contributions of integrated aflatoxin management strategies to achieve the sustainable development goals in various African countries. Global Food Sec. 30:100559. doi: 10.1016/j.gfs.2021.100559
Palm, C., Blanco-Canqui, H., DeClerck, F., Gatere, L., and Grace, P. (2014). Conservation agriculture and ecosystem services: an overview. Agric. Ecosyst. Environ. 187, 87–105. doi: 10.1016/j.agee.2013.10.010
Probst, C., Njapau, H., and Cotty, P. J. (2007). Outbreak of an acute aflatoxicosis in Kenya in 2004: identification of the causal agent. Appl. Environ. Microbiol. 73, 2762–2764.
Ross, S., and de Klerk, M. (2012). Groundnut value chain and marketing assessment in Eastern Province, Zambia. Lusaka, Zambia: Prepared for the conservation farming unit, 1–44.
Sichoongwe, K., Mapemba, L., Ng'ong'ola, D., and Tembo, G. (2014). The determinants and extent of crop diversification among smallholder farmers: A case study of Southern Province, Zambia. Working paper 5. Malawi Support Program. International Food Policy Research Institute, Washington DC, USA, 6.
Tembo, M., Lubungu, M., Singogo, F. K., Mwanza, M., Onyango, M., Sakala, P., et al. (2023). Maize and groundnut crop production among rural households in Zambia: implications in the management of aflatoxins. Food Control 154:109964. doi: 10.1016/j.foodcont.2023.109964
Tembo, S., and Sitko, N. (2013). Technical compendium: descriptive agricultural statistics and analysis for Zambia (no. 1093-2016-88013). doi: 10.22004/ag.econ.155988
Thomas, G. A., Titmarsh, G. W., Freebairn, D. M., and Radford, B. J. (2007). No-tillage and conservation farming practices in grain growing areas of Queensland–a review of 40 years of development. Aust. J. Exp. Agric. 47, 887–898. doi: 10.1071/EA06204
Tufa, A. H., Kanyamuka, J. S., Alene, A., Ngoma, H., Marenya, P. P., Thierfelder, C., et al. (2023). Analysis of adoption of conservation agriculture practices in southern Africa: mixed-methods approach. Front. Sustainable Food Syst. 7:1151876. doi: 10.3389/fsufs.2023.1151876
Wang, Z., Chen, Q., Liu, L., Wen, X., and Liao, Y. (2016). Responses of soil fungi to 5-year conservation tillage treatments in the drylands of northern China. Appl. Soil Ecol. 101, 132–140. doi: 10.1016/j.apsoil.2016.02.002
Wilson, D. M., Mubatanhema, W., and Jurjevic, Z. (2002). “Biology and ecology of mycotoxigenic Aspergillus species as related to economic and health concerns” in Mycotoxins and food safety. Advances in experimental medicine and biology. eds. J. W. DeVries, M. W. Trucksess, and L. S. Jackson, vol. 504 (Boston, MA: Springer)
Wu, F., and Khlangwiset, P. (2010). Health economic impacts and cost-effectiveness of aflatoxin-reduction strategies in Africa: case studies in biocontrol and post-harvest interventions. Food Addit. Contam. 27, 496–509. doi: 10.1080/19440040903437865
Zablotowicz, R. M., Abbas, H. K., and Locke, M. A. (2007). Population ecology of Aspergillus flavus associated with Mississippi Delta soils. Food Addit. Contam. 24, 1102–1108. doi: 10.1080/02652030701546198
Zambian Ministry of Health. (2018). Zambia consolidated guidelines for prevention and treatment of HIV infection. Zambia: Lusaka
ZARI. (2018). Report on the performance of technologies demonstrated under farmer field schools (FFS) in the 2017/2018 season.
Keywords: mycotoxin, agroforestry trees, conservation agriculture, exposure assessment, liver cancer
Citation: Akello J, Alamu EO, Mwila CM, Kachapulula PW, Mukanga M, Njapau H, Chikoye D, Ortega-Beltran A and Bandyopadhyay R (2024) Impact of conservation farming practices on Aspergillus population density, peanut aflatoxin level, and exposure risk in Zambia. Front. Sustain. Food Syst. 8:1331188. doi: 10.3389/fsufs.2024.1331188
Edited by:
Limbikani Matumba, Lilongwe University of Agriculture and Natural Resources, MalawiReviewed by:
Abhay K. Pandey, North Bengal Regional R & D Center, IndiaNii Korley Kortei, University of Health and Allied Sciences, Ghana
Copyright © 2024 Akello, Alamu, Mwila, Kachapulula, Mukanga, Njapau, Chikoye, Ortega-Beltran and Bandyopadhyay. This is an open-access article distributed under the terms of the Creative Commons Attribution License (CC BY). The use, distribution or reproduction in other forums is permitted, provided the original author(s) and the copyright owner(s) are credited and that the original publication in this journal is cited, in accordance with accepted academic practice. No use, distribution or reproduction is permitted which does not comply with these terms.
*Correspondence: Ranajit Bandyopadhyay, r.bandyopadhyay@cgiar.org