- 1Genetic Engineering and Society Center, North Carolina State University, Raleigh, NC, United States
- 2Department of Agricultural and Human Sciences, North Carolina State University Raleigh, NC, United States
- 3School of Public and International Affairs, North Carolina State University, Raleigh, NC, United States
- 4Department of Plant and Microbial Biology, North Carolina State University, Raleigh, NC, United States
- 5Department of Economics, Duke University, Durham, NC, United States
- 6Department of Biological and Agricultural Engineering, North Carolina State University, Raleigh, NC, United States
- 7Department of Crop and Soil Sciences, North Carolina State University, Raleigh, NC, United States
- 8Department of Forestry and Environmental Resources, North Carolina State University, Raleigh, NC, United States
Bioengineered/genome-edited carbon capture and sequestration (BE/GEd-CCS) crops are being developed to mitigate climate change. This paper explores how technology, regulation, funding, and social implications, could shape the development and deployment of these crops. We conclude that some of the technological efforts to create BE/GEd-CCS crops may work. Still, stakeholders must agree on generally accepted methods of measuring how much carbon is captured in the soil and its value. The regulatory space for BE/GEd-CCS crops remains fluid until the first crops are reviewed. BE/GEd-CCS crops have received considerable initial funding and may benefit financially more from other federal programs and voluntary carbon markets. BE/GEd-CCS crops may continue perpetuating social equity concerns about agricultural biotechnology due to a lack of oversight. We argue that stakeholders need to pursue a multidisciplinary view of BE/GEd-CCS crops that draw in varying perspectives for effective development and deployment. Communication is needed between researchers and policymakers involved in either developing BE/GEd-CCS crops or developing voluntary carbon markets. We argue for the start of a conversation both across disciplines and between researchers and policymakers about the development and deployment of BE/GEd-CCS crops.
Introduction
Agriculture accounts for 29% of global greenhouse gas (GHG) emissions (IPCC, 2023). The sector is also vulnerable to the effects of climate change, which has led to a reduction in the rate of agricultural growth globally (CCIAGR, 2012; IPCC, 2023). To reduce GHG emissions, bioengineered1/genome edited2 carbon capture and sequestration (BE/GEd-CCS) crops are being developed to remove carbon from the atmosphere for long-term storage in soils and plants (IGI, 2021; Yield10 Bioscience, 2023; Salk Institute, 2023a). Bioengineered (BE) crops are plants that have been modified through lab techniques to produce traits that cannot be created through conventional breeding and are not found in nature (USDA, AMS, 2023). Genome-edited (GEd) crops are plants that have been modified through lab techniques to produce traits that could be created through conventional breeding or are found in nature (Puchta, 2017). This distinction is important because both technologies are regulated differently in the United States.
Multiple institutions are developing BE/GEd-CCS crops. These crops improve carbon sequestration in two ways. The first is modifying photosynthetic tissues so the plant uses more carbon dioxide (IGI, 2021). The second is modifying root systems to achieve a larger root system for enhanced carbon storage capacity. Under the “Harnessing Plants Initiative,” the Salk Institute is developing model plants with root traits that increase carbon storage in the soil (Salk Institute, 2023a). The genes encoding these root traits will then be transferred into major crops (Salk Institute, 2023a). Yield10 Biosciences is improving performance traits in camelina plants to increase yield and soil carbon deposits using genome editing (Yield10 Bioscience, 2023). The Innovative Genomics Institute (IGI) is using genome editing to develop sorghum and rice varieties with better photosynthetic capacity and deeper root structures to store carbon (IGI, 2023c,d). It is unclear where BE/GEd-CCS crops will fit within the current US climate change policy landscape and what their scientific, regulatory, and socioeconomic impacts will be. However, most of the current research on BE/GEd-CCS crops has focused on the technology itself without consideration for the social, economic, legal, and ethical implications (Salk Institute, 2019, 2020, 2023; IGI, 2022). IGI has been the exception with their project “Developing a deployment roadmap for Carbon Dioxide Removal (CDR) crops,” which pays attention to “societal considerations and policies” (IGI, 2023b). We argue that BE/GEd-CCS crops would benefit from cross-sector communication between researchers and policymakers, as well as interdisciplinary communication between technical, regulatory, socioeconomic, legal, and ethics researchers. Particularly between those developing BE/GEd-CCS crops and those developing carbon capture programs in the United States.
This paper focuses on four different fields reflecting our areas of expertise, to highlight the complex environment within which BE/GEd-CCS crops would be deployed. The four fields are technology, regulation, funding, and social implications. The following sections discuss each of these areas.
Technology
There are two main technological challenges to developing and deploying BE/GEd-CCS crops. The first is developing crops that are better at capturing and/or storing carbon in the soil. The second is the ability to accurately measure carbon in the soil and carbon’s impact on the soil. The success of BE/GEd-CCS crops will depend on both crops and microbes that can capture more carbon and consistent means to measure carbon in the soil.
The first technological challenge of BE/GEd-CCS crops is the bioengineering or genome editing of photosynthetic organs, root structures, and soil microbes (Liu et al., 2021; IGI, 2023a; Salk Institute, 2023a). The goal is to make plants that are better at capturing carbon and/or storing it in the soil. To improve photosynthesis, one major target has been improving the CO2-fixing enzyme, Rubisco (Erb and Zarzycki, 2018). The overall goal is to increase the CO2 fixation efficiency of Rubisco in the photosynthetic system of C3 plants like cotton and soybean, to that of C4 plants like corn. Converting C3 plants to function as C4 plants will likely be a challenge judging from previous attempts to modify C3 plants, but once successful, will result in plants that capture more carbon from the air, have higher yields, and improve soil health (Ruan et al., 2012; Sharwood, 2017; Cui, 2021; Badger and Sharwood, 2023). To get more carbon to stay in the soil, plants will need larger root systems and the soil will need a better microbiome. Researchers are investigating ways to enhance the root systems in model organisms like Arabidopsis through a series of Genome-Wide Association Studies (GWAS) (Dimitrov and Tax, 2018; Ogura et al., 2019; Boatwright et al., 2022). These GWAS will identify genes for enhanced root systems that can be put into crops. Similarly, microbiomes in soil are being studied for genes and environments that can be modified to improve a plant’s ability to keep carbon in the soil (IGI, 2023e). Figure 1 provides an overview of the different modifications that can enhance CCS in crops.
The second technological challenge is measuring and understanding the impact of carbon in the soil. The effectiveness of BE/GEd-CCS crops are limited if the impacts of their increase in CCS is not accurately measured and understood. Different types of carbon can be stored in the soil and there is no single approach to measuring carbon’s presence (Cotrufo et al., 2019; Lavallee et al., 2020; Oldfield et al., 2022; Whalen et al., 2022). The two main kinds of carbon are particulate organic matter (POM) and mineral-associated organic matter (MAOM) (Cotrufo et al., 2019). Different methods can lead to different findings for both POM and MAOM in the soil (Whalen et al., 2022), and this has resulted in disputes about the amount of carbon credits companies have claimed to sequester. As more growers try to sell carbon credits, the inability to get consistent measures of sequestered carbon will become increasingly problematic (Oldfield et al., 2022). Practices to ensure consistency in how agricultural carbon is measured will lead to better carbon measurement, make for more accurate assessments of the GHG mitigation potential of these technologies, and will reduce uncertainty and friction in voluntary carbon markets.
Communication between those developing BE/GEd-CCS crops and solutions for measuring and valuing carbon in soil will be important for this technology and carbon capture programs. Genetic modification without context has a history of overpromising and underdelivering how much it can improve the capabilities of crops (Khaipho-Burch et al., 2023). Being grounded in reality through interdisciplinary communication and teamwork will increase the odds of BE/GEd-CCS crops fulfilling the promise of capturing and storing more carbon in the soil.
Regulation
This section focuses on how US regulations will affect bringing BE/GEd-CCS crops to market. In the US, the regulation of agricultural biotechnology is overseen by the Coordinated Framework which consists of the Environmental Protection Agency (EPA), Food and Drug Administration (FDA), and the United States Department of Agriculture (USDA) (EPA, FDA, USDA, 2023). Under the Coordinated Framework, a BE/GEd-CCS crop may require regulation if it either contains pesticides (EPA jurisdiction), is a food or drug for animals or humans (FDA jurisdiction), or is considered a plant pest (USDA jurisdiction). The USDA, FSA, 2020 SECURE Rule will likely streamline the regulation of many CCS crops due to the distinction that desired traits that could emerge through conventional breeding methods have less regulatory requirements (Hoffman, 2021). Genome editing has become more common and since traits from genome editing are considered possible through conventional breeding methods, any GEd-CCS crop will face less regulatory burden from the USDA.
Other traits might be stacked with these CCS plants to make them more viable like expressing Bt or glyphosate resistance. These additional traits are not likely to increase the regulatory burden of stacked BE/GEd-CCS crops as long as they are traits that have already been reviewed by regulators. These additional traits would likely fall under the EPA’s jurisdiction and like the USDA will not require an in-depth review for genome-edited crops “if the change already exists in a sexually compatible plant” (Stokstad, 2023). The EPA is unlikely to regulate the CCS trait itself since it should not cause an increase in “pesticide levels beyond those found in food from conventional crops” (Stokstad, 2023). Overall, the regulation of BE/GEd-CCS crops in the US will likely matter to the USDA and possibly the EPA with genome edited variants facing less regulatory burden.
Public and private funding
As a biotechnology and a CCS tool, BE/GEd-CCS crops have the potential to receive support from a suite of public and private funding opportunities. This section focuses on three areas of funding: research and development (R&D), conservation, and voluntary carbon markets. At the R&D stage, BE/GEd-CCS crop research has already received funding from a number of private entities and has the potential to receive funding from the National Biotechnology and Biomanufacturing Initiative (NBBI) (Salk Institute, 2019, 2020, 2023; IGI, 2021, 2022; Exec, 2023). Based on the first report from the NBBI, BE/GEd-CCS crops would align with “Goal 4.1: Develop Landscape-Scale Biotechnology Solutions” which calls for funding research that will produce crops that sequester carbon into the soil (OSTP, 2023).
For conservation, growers using BE/GEd-CCS crops may benefit from current programs like the Environmental Quality Incentives Program (EQIP), Conservation Stewardship Program (CSP), and Conservation Reserve Program (CRP) (USDA, FSA, 2020; USDA, NRCS, 2023a,b). BE/GEd-CCS crops may disrupt the current landscape similar to the effect biofuel crops have had on Conservation Research Program (CRP) lands (Abraha et al., 2019). Depending on the strength of government incentives, there might be a repeat in behavior where land that was once conserved is turned into BE/GEd-CCS crop farmlands because of the economic incentive. If this happened, depending on how much carbon BE/GEd-CCS crops can sequester and how much land is converted into farmland, there could either be a net loss in carbon capture or a balancing effect where total atmospheric carbon stays the same. This would ignore any other environmental changes due to converting conserved land to farmland.
For voluntary carbon markets, BE/GEd-CCS crops are timely and could be a tool to help meet the growing desire to generate carbon credits through climate-conscious agriculture (Jiang et al., 2021; Oldfield et al., 2022). Voluntary carbon markets are private institutions enabling parties to create and trade contracts where one party sequesters carbon (offsets) in exchange for a payment from the buyer. Companies can participate in voluntary markets for various reasons and these voluntary markets do not overlap with state-created markets. Since these offsets are not state-mandated, there are inconsistencies in the quality and amount of carbon captured and stored by different projects (Popkin, 2023; White, 2023). Voluntary markets do not have a set of industry standards and there is not a generalized federal carbon reduction mandate in the US. In addition, BE/GEd-CCS crops may only be applicable to voluntary markets because of how restrictive state-created markets are on what projects can count. Due to all the above, those involved in either the development of BE/GEd-CCS crops or voluntary markets should be communicating with each other since they have complementary roles.
Lastly, US farmers may seek support in entering voluntary carbon markets from the USDA starting in 2024 (117th Congress (2021–2022), 2022; Carbon Credits, 2022). The USDA will develop best practices, provide guidance on accreditation, and help growers find potential buyers of carbon credits (Homeland Security and Governmental Affairs, 2022; Fischler, 2023). The USDA’s assistance will help as a lack of a centralized, voluntary market will make it harder for growers to use BE/GEd-CCS crops to sell carbon credits. This is another point where communication between developers of BE/GEd-CCS crops and policymakers in the USDA would be beneficial. Figure 2 provides an overview of the stakeholders involved in the development of either BE/GEd-CCS crops or a carbon economy. It shows that these two sectors are not interacting with each other.
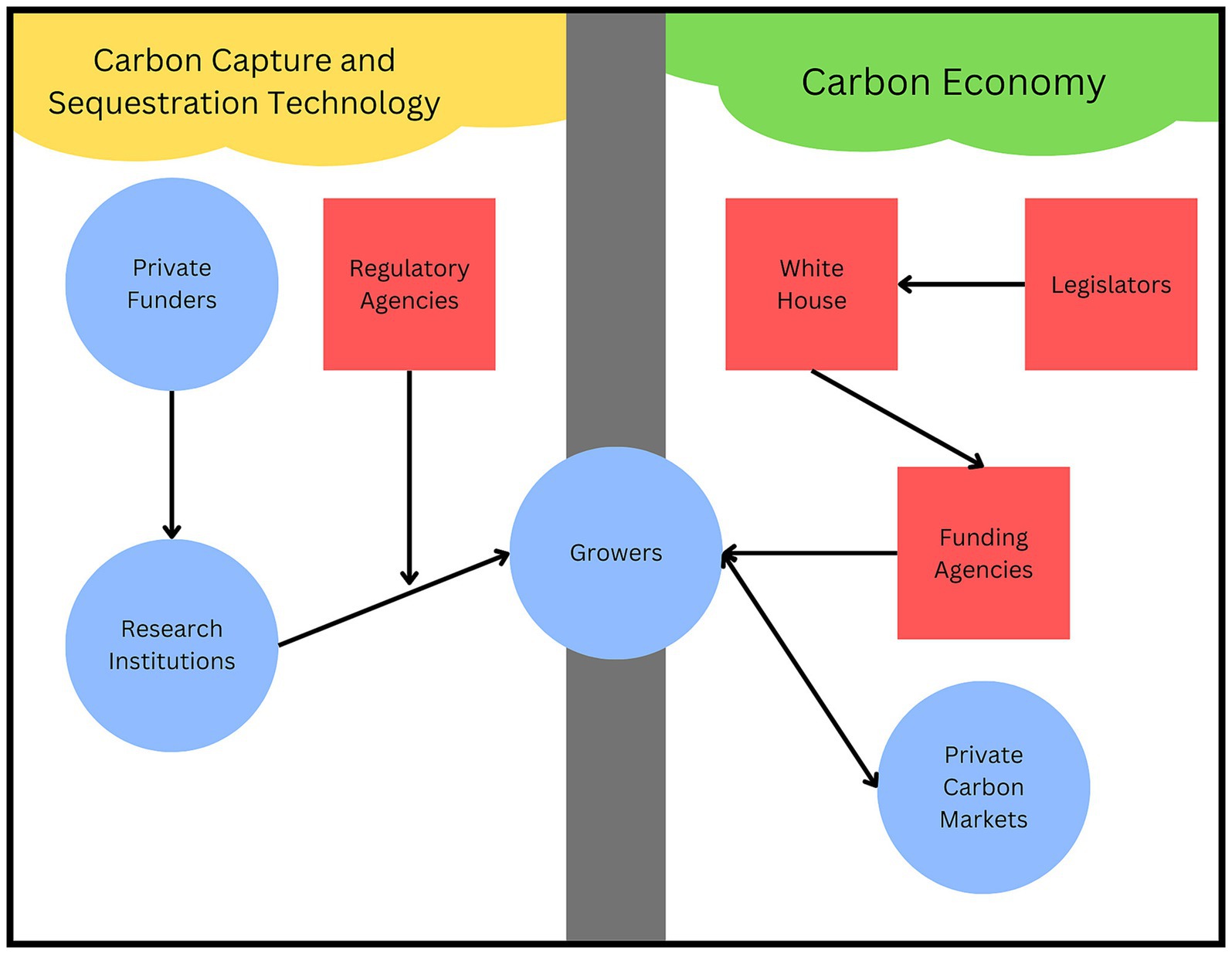
Figure 2. Policy landscape for the development of carbon capture and sequestration crops and the development of a carbon economy.
Social implications
The section on social implications focuses on two areas. The first area is historical precedents, like race, that will likely influence who will equitably benefit from the commercialization of BE/GEd-CCS crops. The second area is government programs that show a commitment to social equity and could influence who will benefit from BE/GEd-CCS crops. We define social equity as the fair distribution of benefits from technology (Stone, 2011). What is fair will depend upon the language used in already enacted and yet to be made policies applicable to BE/GEd-CCS crops. Due to the number of uncertainties, we argue BE/GEd-CCS crops may or may not perpetuate current and growing inequalities in US farming. This is a chance for developers of BE/GEd-CCS crops and policymakers/researchers to communicate with each other on how this technology could help address inequalities in United States agriculture.
In this social implication analysis, we focus specifically on farmers in the US because adequate data exists on that population. The US farming population is not uniform and various agriculture policies have resulted in varied outcomes. Over the years, mid-sized (9–999 acres) farms in the US have continued to disappear while the number of small farms has grown and the number of large farms remains stable (USDA, NASS, 2017; Rafter, 2021). This development has disproportionately harmed black farmers more and BE/GEd crops, alongside other technological innovations, could drive more consolidation (MacDonald, 2020; Rafter, 2021).3 BE/GE-CCS crops may not introduce new equity concerns, but they can amplify pre-existing ones if implemented without forethought. For example, transaction costs for participating in the voluntary carbon market could make it more easy for larger farms to participate than smaller ones. Buyers from the carbon market may seek a few larger contracts rather than many little contracts because it would be easier to work with a fewer large farms on monitoring and verification, as opposed to many small farms. Small contracts could be repackaged into larger composite securities, but that would involve middlemen and higher transaction costs. So, without proper forethought about how small or mid-sized farms could be included in carbon market contracts, the benefits of BE/GEd-CCS crops may end up being consolidated in large farms like what can happen with other biotech crops. BE/GEd-CCS crop developers can address this issue by communicating with other disciplines and policymakers as the IGI is doing through their deployment roadmap project which includes “a community engagement pilot using community-based participatory research and citizen assembly methods” (IGI, 2023b). Effective communication will ensure the interests of small and medium-scale farms are incorporated in the development of CCS crop policies.
There are also public policies that may support the equitable implementation of BE/GEd-CCS crops. The Biden-Harris Administration in 2022 began the Justice40 Initiative which aims to address environmental justice through making sure that “40% of the overall benefits of certain federal investments flow to disadvantaged communities that are marginalized, underserved, and overburdened by pollution” (White House, 2023). Under the USDA, programs affected by this initiative that may influence the implementation of BE/GEd-CCS crops are the Conservation Reserve Program (CRP) and farm loans. The Growing Climate Solutions Act also intends to increase climate smart farming like BE/GEd-CCS crops and includes language for assisting “socially disadvantaged farmers” (Homeland Security and Governmental Affairs, 2022). Lastly, the report “Bold Goals for U.S. Biotechnology and Biomanufacturing…” contains justice focused language like “equity” and “disability” (Exec, 2023; OSTP, 2023). Together, these federal initiatives could encourage the equitable implementation of BE/GEd-CCS crops. However, more work is needed to understand where exactly this biotechnology could fit into these different programs. We argue that there is a general lack of knowledge about how BE/GEd-CCS crops could either change or reinforce current inequalities among farmers in the US and discussions between researchers and policymakers are needed.
Conclusion and next steps
Based on this review, the development and future deployment of BE/GEd-CCS crops will be affected by multiple factors including technological interactions, economic incentives, regulatory oversight, agricultural policies, etc. We have yet to see multidisciplinary or interdisciplinary reviews of this topic. Researchers and policymakers need to engage in discussions about the various programs of the US agricultural system that BE/GEd-CCS crops will fit into. Doing so will allow for a systems-wide view of what challenges and opportunities exist for the development and deployment of BE/GEd-CCS crops. For this technology to be effectively deployed to address climate change, it will need a multidisciplinary approach that avoids piecemeal perspectives.
There are many ways to build upon this work. This paper focuses on the US, but other nations will have unique qualities that affect the deployment of BE/GEd-CCS crops. Going forward, systems-wide frameworks like the Responsible Research and Innovation or Ethical, Legal, and Social Implication frameworks could help evaluate the development and implementation of BE/GEd-CCS crops (Stilgoe et al., 2013; Trump et al., 2023). Lastly, researchers can explore publics’ opinions on BE/GEd-CCS crops since including the opinions of different groups are needed for the democratic governance of a technology (Stilgoe et al., 2014).
Based on this review, we recommend the following:
• Researchers working on the technological, socioeconomic, regulatory, legal, and ethical aspects of BE/Ged-CCS crops should collaborate in an interdisciplinary manner in order to better understand the impacts this technology will have.
• Researchers, policymakers, and other stakeholders working on BE/Ged-CCS crops and/or the US carbon economy should communicate between these two sectors so there is a systems-wide approach to deploying these crops.
Author contributions
JG: Writing – original draft, Writing – review & editing. AH: Writing – original draft, Writing – review & editing. JA: Writing – original draft, Writing – review & editing. JC: Writing – original draft, Writing – review & editing. SM: Writing – original draft, Writing – review & editing. JM: Writing – original draft, Writing – review & editing. SE: Writing – original draft, Writing – review & editing. DU: Writing – original draft, Writing – review & editing. SZ: Writing – original draft, Writing – review & editing.
Funding
The author(s) declare financial support was received for the research, authorship, and/or publication of this article. This paper was funded through the National Science Foundation (NSF) Research Traineeship (NRT) award #1828820.
Acknowledgments
We would like to express gratitude to Zachary Brown, Jason Delborne, Fred Gould, and Dawn Rodriguez-Ward of the Genetic Engineering and Society Center, North Carolina State University, as well as the entire team at the center, for the guidance and support that made this paper possible. We would like to thank Catalina Morris for her help in developing the figures found in this paper.
Conflict of interest
The authors declare that the research was conducted in the absence of any commercial or financial relationships that could be construed as a potential conflict of interest.
Publisher’s note
All claims expressed in this article are solely those of the authors and do not necessarily represent those of their affiliated organizations, or those of the publisher, the editors and the reviewers. Any product that may be evaluated in this article, or claim that may be made by its manufacturer, is not guaranteed or endorsed by the publisher.
Footnotes
1. ^In place of “genetically engineered” we use “bioengineered” to match US regulatory language.
2. ^We use bioengineered and genome edited to describe CCS crops as both techniques may be used to develop CCS crops, but have different regulations in the United States.
3. ^The degree to which BE/GEd crops are scale neutral is contested.
References
Abraha, M., Gelfand, I., Hamilton, S. K., Chen, J., and Robertson, G. P. (2019). Carbon debt of field-scale conservation reserve program grasslands converted to annual and perennial bioenergy crops. Environ. Res. Lett. 14:24019. doi: 10.1088/1748-9326/aafc10
Badger, M. R., and Sharwood, R. E. (2023). Rubisco, the imperfect winner: It’s all about the base. J. Exp. Bot. 74, 562–580. doi: 10.1093/jxb/erac458
Boatwright, J. L., Sapkota, S., Myers, M., Kumar, N., Cox, A., Jordan, K. E., et al. (2022). Dissecting the genetic architecture of carbon partitioning in Sorghum using multiscale phenotypes. Front. Plant Sci. 13:5. doi: 10.3389/fpls.2022.790005
Carbon Credits . (2022). What is the voluntary carbon market? Carbon credits. Available at: https://carboncredits.com/what-is-the-voluntary-carbon-market/.
CCIAGR . (2012). Agriculture and food production contribute up to 29 percent of global greenhouse gas emissions according to comprehensive research papers. Available at: https://ccafs.cgiar.org/media/press-release/agriculture-and-food-production-contribute-29-percent-global-greenhouse.
Cotrufo, M. F., Ranalli, M. G., Haddix, M. L., Six, J., and Lugato, E. (2019). Soil carbon storage informed by particulate and mineral-associated organic matter. Nat. Geosci. 12, 989–994. doi: 10.1038/s41561-019-0484-6
Cui, H. (2021). Challenges and approaches to crop improvement through C3-to-C4 engineering. Front. Plant Sci. 12:391. doi: 10.3389/fpls.2021.715391
Dimitrov, I., and Tax, F. E. (2018). Lateral root growth in Arabidopsis is controlled by short and long distance signaling through the LRR RLKs XIP1/CEPR1 and CEPR2. Plant Signal. Behav. 13:e1489667. doi: 10.1080/15592324.2018.1489667
EPA, FDA, USDA . (2023). About the coordinated framework. Unified website for biotechnology regulation. Available at: https://usbiotechnologyregulation.mrp.usda.gov/biotechnologygov/about/about (Accessed May 25, 2023).
Erb, T. J., and Zarzycki, J. (2018). A short history of RubisCO: the rise and fall (?) of Nature’s predominant CO2 fixing enzyme. Curr. Opin. Biotechnol. 49, 100–107. doi: 10.1016/j.copbio.2017.07.017
Fischler, J. (2023). U.S. Department of Agriculture to spend $300M to boost climate data in farming, forestry. NC Newsline. Available at: https://ncnewsline.com/briefs/u-s-department-of-agriculture-to-spend-300m-to-boost-climate-data-in-farming-forestry/.
Hoffman, N. E. (2021). Revisions to USDA biotechnology regulations: the SECURE rule. Proc. Natl. Acad. Sci. 118:e2004841118. doi: 10.1073/pnas.2004841118
Homeland Security and Governmental Affairs . (2022). H. R. 2617—117th Congress (2021-2022): Consolidated Appropriations Act, 2023. Available at: https://www.congress.gov/bill/117th-congress/house-bill/2617.
IGI . (2021). IGI launches new research in net-Zero Farming & Carbon Capture. Innovative genomics institute (IGI). Available at: https://innovativegenomics.org/news/net-zero-farming-carbon-capture/.
IGI . (2022). Supercharging plants and soils to remove carbon from the atmosphere. Innovative genomics institute (IGI). Available at: https://innovativegenomics.org/news/crispr-carbon-removal/.
IGI . (2023a). Cultivating soil microbial communities to promote carbon persistence. Innovative genomics institute (IGI). Available at: https://innovativegenomics.org/projects/cultivating-soil-microbial-communities-to-promote-carbon-persistence/. (Accessed May 30, 2023).
IGI . (2023b). Developing a deployment roadmap for CDR crops. Innovative genomics institute (IGI). Available at: https://innovativegenomics.org/projects/developing-a-deployment-roadmap-for-cdr-crops/. (Accessed May 30, 2023).
IGI . (2023c). Developing next-generation crops for carbon dioxide removal. Innovative genomics institute (IGI). Available at: https://innovativegenomics.org/projects/developing-next-generation-crops-for-carbon-dioxide-removal/. (Accessed May 30, 2023).
IGI . (2023d). Developing targeted gene insertion technology in rice. Innovative genomics institute (IGI). Available at: https://innovativegenomics.org/projects/developing-targeted-gene-insertion-in-rice/. (Accessed May 30, 2023).
IGI . (2023e). Investigating the role of soil microbes in greenhouse gas emissions. Innovative genomics institute (IGI). Available at: https://innovativegenomics.org/projects/soil-microbes-greenhouse-gas/. (Accessed May 30, 2023).
IPCC . (2023). Climate change 2022–impacts, adaptation and vulnerability: Working group II contribution to the sixth assessment report of the intergovernmental panel on climate change. 1st Edn. Cambridge: Cambridge University Press.
Jiang, Y., Luo, L., Xu, J., and Shao, X. (2021). The value relevance of corporate voluntary carbon disclosure: evidence from the United States and BRIC countries. J. Contemp. Account. Econ. 17:100279. doi: 10.1016/j.jcae.2021.100279
Khaipho-Burch, M., Cooper, M., Crossa, J., de Leon, N., Holland, J., Lewis, R., et al. (2023). Genetic modification can improve crop yields—but stop overselling it. Nature 621, 470–473. doi: 10.1038/d41586-023-02895-w
Lavallee, J. M., Soong, J. L., and Cotrufo, M. F. (2020). Conceptualizing soil organic matter into particulate and mineral-associated forms to address global change in the 21st century. Glob. Chang. Biol. 26, 261–273. doi: 10.1111/gcb.14859
Liu, D., Hu, R., Zhang, J., Guo, H.-B., Cheng, H., Li, L., et al. (2021). Overexpression of an Agave phosphoenolpyruvate carboxylase improves plant growth and stress tolerance. Cell 10:582. doi: 10.3390/cells10030582
MacDonald, J. M. (2020). Tracking the consolidation of U.S. agriculture. Appl. Econ. Perspect. Policy 42, 361–379. doi: 10.1002/aepp.13056
Ogura, T., Goeschl, C., Filiault, D., Mirea, M., Slovak, R., Wolhrab, B., et al. (2019). Root system depth in Arabidopsis is shaped by EXOCYST70A3 via the dynamic modulation of auxin transport. Cell 178, 400–412.e16. doi: 10.1016/j.cell.2019.06.021
Oldfield, E. E., Eagle, A. J., Rubin, R. L., Rudek, J., Sanderman, J., and Gordon, D. R. (2022). Crediting agricultural soil carbon sequestration. Science 375, 1222–1225. doi: 10.1126/science.abl7991
OSTP . (2023). Bold goals for U.S. biotechnology and biomanufacturing: harnessing Research and Development to further societal goals. Executive Office of the President of the United States. pp. 1–61.
Popkin, G. (2023). Farmers are being paid millions to trap carbon in their soils. Will it actually help the planet? Available at: https://www.science.org/content/article/farmers-paid-millions-trap-carbon-soils-will-it-actually-help-planet.
Puchta, H. (2017). Applying CRISPR/Cas for genome engineering in plants: the best is yet to come. Curr. Opin. Plant Biol. 36, 1–8. doi: 10.1016/j.pbi.2016.11.011
Rafter, F. (2021). Losing ground: Farmland consolidation and threats to new farmers, black farmers, and the future of farming. Cambridge, MA: Union of Concerned Scientists.
Ruan, C.-J., Shao, H.-B., and Teixeira da Silva, J. A. (2012). A critical review on the improvement of photosynthetic carbon assimilation in C3 plants using genetic engineering. Crit. Rev. Biotechnol. 32, 1–21. doi: 10.3109/07388551.2010.533119
Salk Institute . (2019). Salk institute initiative to receive more than $35 million to fight climate change. Salk Institute for Biological Studies. Available at: https://www.salk.edu/news-release/salk-institute-initiative-to-receive-more-than-35-million-to-fight-climate-change/.
Salk Institute . (2020). Bezos earth fund donates $30 million to Salk institute for innovative climate change research. Salk Institute for Biological Studies. https://www.salk.edu/news-release/bezos-earth-fund-donates-30-million-to-salk-institute-for-innovative-climate-change-research/.
Salk Institute . (2023a). Harnessing plants initiative. Salk Institute for Biological Studies. Available at: https://www.salk.edu/harnessing-plants-initiative/. (Accessed May 30, 2023).
Salk Institute . (2023b). Salk institute receives $50 million from Hess Corporation to mitigate climate change through plant science. Salk Institute for Biological Studies. Available at: https://www.salk.edu/news-release/salk-institute-receives-50-million-from-hess-corporation-to-mitigate-climate-change-through-plant-science/.
Sharwood, R. E. (2017). Engineering chloroplasts to improve Rubisco catalysis: prospects for translating improvements into food and fiber crops. New Phytol. 213, 494–510. doi: 10.1111/nph.14351
Stilgoe, J., Lock, S. J., and Wilsdon, J. (2014). Why should we promote public engagement with science? Public Underst. Sci. 23, 4–15. doi: 10.1177/0963662513518154
Stilgoe, J., Owen, R., and Macnaghten, P. (2013). Developing a framework for responsible innovation. Res. Policy 42, 1568–1580. doi: 10.1016/j.respol.2013.05.008
Stokstad, E. (2023). EPA decision to tighten oversight of gene-edited crops draws mixed response. Science. Available at: https://www.science.org/content/article/epa-decision-tighten-oversight-gene-edited-crops-draws-mixed-response.
Stone, D. (2011). Policy paradox: The art of political decision making (third edition). New York: W. W Norton and Company.
Trump, B., Cummings, C., Klasa, K., Galaitsi, S., and Linkov, I. (2023). Governing biotechnology to provide safety and security and address ethical, legal, and social implications. Front. Genet. 13:1052371. doi: 10.3389/fgene.2022.1052371
USDA, AMS . (2023). BE disclosure. Available at: https://www.ams.usda.gov/rules-regulations/be. (Accessed May 25, 2023).
USDA, FSA . (2020). Conservation reserve program [page]. National-Content. Available at: https://fsa.usda.gov/programs-and-services/conservation-programs/conservation-reserve-program/index.
USDA, NASS . (2017). 2017 census of agriculture. Available at: https://www.nass.usda.gov/Publications/AgCensus/2017/#full_report.
USDA, NRCS . (2023a). Environmental quality incentives program. Natural Resources Conservation Service. Available at: https://www.nrcs.usda.gov/programs-initiatives/eqip-environmental-quality-incentives.
USDA, NRCS . (2023b). Conservation stewardship program. Available at: https://www.nrcs.usda.gov/programs-initiatives/csp-conservation-stewardship-program.
Whalen, E. D., Grandy, A. S., Sokol, N. W., Keiluweit, M., Ernakovich, J., Smith, R. G., et al. (2022). Clarifying the evidence for microbial- and plant-derived soil organic matter, and the path toward a more quantitative understanding. Glob. Chang. Biol. 28, 7167–7185. doi: 10.1111/gcb.16413
White, N. (2023). Carbon-credit traders find their CO2 offsets may be worth nothing. Los Angeles Times. Available at: https://www.latimes.com/business/story/2023-08-22/carbon-trading-co2-credits.
White House . (2023). Justice40 initiative. The White house. Available at: https://www.whitehouse.gov/environmentaljustice/justice40/. (Accessed May 30, 2023).
Yield10 Bioscience . (2023). Novel crop traits. Available at: https://www.yield10bio.com/crop-science/novel-crop-traits. (Accessed July 19, 2023).
Keywords: genetic engineering, genome editing, bioengineered crops, climate change, carbon capture sequestration
Citation: Gakpo JO, Hardwick A, Ahmad J, Choi J, Matus SC, Mugisa JD, Ethridge S, Utley D and Zarate S (2024) The need for communication between researchers and policymakers for the deployment of bioengineered carbon capture and sequestration crops. Front. Sustain. Food Syst. 8:1329123. doi: 10.3389/fsufs.2024.1329123
Edited by:
Andy Morse, University of Liverpool, United KingdomReviewed by:
Rakesh S., National Academy of Agricultural Research Management (ICAR), IndiaCopyright © 2024 Gakpo, Hardwick, Ahmad, Choi, Matus, Mugisa, Ethridge, Utley and Zarate. This is an open-access article distributed under the terms of the Creative Commons Attribution License (CC BY). The use, distribution or reproduction in other forums is permitted, provided the original author(s) and the copyright owner(s) are credited and that the original publication in this journal is cited, in accordance with accepted academic practice. No use, distribution or reproduction is permitted which does not comply with these terms.
*Correspondence: Joseph Opoku Gakpo, jogakpo@ncsu.edu; Andrew Hardwick, arhardwi@ncsu.edu