- 1Centre for Agri-Environmental Research, School of Agriculture, Policy and Development, University of Reading, Reading, United Kingdom
- 2Department of Agricultural, Forest and Food Sciences (DISAFA) and Interdepartmental Centre for the Innovation in the Agro-environmental Sector (AGROINNOVA), University of Turin, Turin, Italy
The development of integrated pest management (IPM) strategies, aimed at reducing pesticide use, has myriad ecological and agronomic benefits to terrestrial ecosystems and the environment, but can also lead to different biological and economic outcomes depending on the production system. The most common facet of IPM in apple is the reduction and/or alternative use of pesticides but also includes cultural, mechanical and biological controls. Using apple as a model system, we performed a meta-analysis of 55 studies from 20 countries to quantify the effects of IPM on beneficial invertebrates, pest and disease pressure, and crop productivity (i.e., fruit yield and quality). We also explored different feeding guilds (i.e., tissue-chewing, sap-sucking or boring/mining herbivores, and beneficial natural enemy predators or parasitoids) to determine whether invertebrate responses to IPM differ between feeding strategies. By scoring IPM adoption based on the relative number of facets of IPM used in each study, we also determined whether the level of IPM implemented in apple farming systems alters the responses of invertebrates and pathogens. Our results demonstrate how IPM adoption increases the performance of natural enemies, while simultaneously reducing pest and disease pressure overall. However, the effects of IPM on disease pressure may depend on the level of IPM adoption because disease pressure increased when multiple facets of IPM were adopted (i.e., as the level of IPM adoption increased). Apple quality was not limited by IPM adoption, yet fruit yield decreased overall. While both natural enemy feeding guilds (predators and parasitoids) responded positively to IPM adoption, only two of the three pest feeding guilds (tissue-chewing and sap-sucking herbivores) decreased under IPM, with boring/mining herbivores showing no response. These results demonstrate the complex benefits and limitations that can occur under IPM and call for economic risk assessments based on these differences. Effective IPM strategies rely on monitoring practices and pest/pathogen prevention but can provide real environmental value.
1 Introduction
Pest control in conventional fruit production is heavily dependent on artificial chemical pesticides (including insecticides, herbicides, fungicides, molluscicides, acaricides and nematicides), which have adverse impacts on the environment (Stehle and Schulz, 2015; Lamichhane et al., 2016) and pose health risks for producers and consumers (Mesnage et al., 2014; Kim et al., 2017). Insect resistance to pesticides has led to a vicious cycle for conventional farming systems, termed the ‘pesticide treadmill’ (van den Bosch, 1978), that requires increasing application rates, and thus additional costs, to prevent higher crop losses. Plant protection products (PPPs) contain an active substance (a chemical or micro-organism) that requires approval for use. Due to concerns over environmental (Sánchez-Bayo and Wyckhuys, 2019) and human health effects (European Food Safety Authority et al., 2021) of these active substances, the re-approvals of existing pesticides are being restricted in several countries, including the European Union (Regulation 128/2009) and the United States (Federal Insecticide, Fungicide, and Rodenticide Act). In the EU, the Directive 2009/128/EC aims to achieve a sustainable use of pesticides by reducing the risks and impacts of pesticide use and promoting the use of Integrated Pest Management (IPM). Moreover, the EU has adopted proposals for a new Regulation on the Sustainable Use of PPPs, including far reaching targets to reduce, by 50%, the use and risk of chemical pesticides by 2030, in line with the EU’s Farm to Fork and Biodiversity strategies.
Several farming system approaches aim to limit the reliance on chemical pesticides and to reduce their adverse impacts. These include ‘organic’, ‘zero residue’ and ‘IPM’, which are underpinned by similar principles and share many approaches. There are multiple definitions for IPM, with no standardised approach, but rather crop- and region-specific guidelines that promote ecologically-sound agricultural practices (Prokopy and Kogan, 2009; Barzman et al., 2015). These practices broadly rely on biological, cultural and mechanical techniques (e.g., using beneficial organisms, crop rotations and tillage, respectively) to prevent losses from pests, diseases and weeds, and the extent to which they are adopted has only recently been quantified (Creissen et al., 2019). IPM has the potential to deliver multiple benefits, including biodiversity protection, reduced environmental pollution and food contamination by synthetic pesticides, while maintaining crop productivity and profitability (Jacquet et al., 2011; Lechenet et al., 2017). However, IPM is a knowledge-intensive approach to agricultural production requiring an intimate understanding of the variables influencing pest control in crop systems.
Transitioning from a conventional to a more IPM-based agricultural approach can be limited by, among other things, a lack of knowledge of appropriate and effective tools as well as presenting economic risks for those producers who have somewhat limited uptake and expansion potential (Alwang et al., 2019; Deguine et al., 2021; Kansiime et al., 2021). The effective contribution of natural enemies to help control pests through conservation biocontrol (CBC) is fundamental to successful IPM schemes in open systems but there is a clear lack of evidence for the potential for CBC based approaches to deliver agronomic and economic benefits for growers, with research endpoints limited primarily to effects on abundance of pests and natural enemies (Kleijn et al., 2019; Johnson et al., 2021; Girling et al., 2022). Therefore, to provide the necessary evidence to support the transition to more IPM-based approaches it is critical to quantify how delivering better control by natural enemies and reducing undesirable inputs of pesticides influences crop yield and quality (Snyder, 2019). Ultimately, notwithstanding the political support in some parts of the world (Kuchheuser and Birringer, 2022), uptake of IPM will be determined in large part by its potential to maintain or improve production and economic outcomes for farmers in the absence of legislation or subsidies.
Fundamentally, IPM relies on understanding the ecology of pests and beneficials within the agroecological system and how to influence population dynamics to enhance production. The framework presented by current EU policies and defined in the Sustainable Use of Pesticides Directive (2009/128/EC) follows 8 key principles (reviewed by Barzman et al., 2015): (1) prevention and suppression; (2) monitoring; (3) decision making; (4–7) intervention (including reduced pesticide use and non-chemical methods including CBC); and (8) evaluation. This final step is important as it encourages reflection and evaluation of interventions that were most successful and establishes the notion that the system should be constantly developed and improved.
Foraging strategies employed by invertebrates can dictate how they respond to alternative pesticides or different management systems (Staton et al., 2021; Thabet et al., 2021) and it is therefore important to consider invertebrate functional groups or feeding guilds (e.g., whether they are tissue-chewing, sap-sucking, boring or mining herbivore pests, or whether they ecologically and/or economically benefit us by predating or parasitising herbivore pests) when assessing ecological responses to IPM. For example, tissue-chewing herbivores (chewers) may respond positively to reductions in pesticide use associated with IPM, whereas sap-sucking (suckers) and boring/mining herbivores (borers/miners), which, due to their feeding strategy, may avoid surface-level leaf defences, may be less responsive to pesticide reductions in general. However, chewers and suckers that remain on the surface of the plant to feed may be more negatively impacted by IPM-associated increases in biological control mechanisms compared with borers/miners that can hide within the plant for protection. As such, the response of different guilds to contrasting management approaches in a particular cropping system may depend on the presence and/or relative distribution of invertebrate feeding guilds within the population (Garratt et al., 2011).
Apples are one of the most economically important fruit crops globally, valued at more than US$45 billion per annum (FAOStat, 2022). In 2020, apples ranked as the third-highest fruit in global production, yielding a total of 86.4 million tons (Zaller et al., 2023). However, organic apple production output is significantly lower than conventional (Samnegård et al., 2019), and currently only covers ~114,000 ha, 2.5% of 4.62 M ha of total apple production worldwide (FAO, 2021; Willer et al., 2021). Apples face many significant pests and, as a result, pesticide application can be high, as can yield losses due to pests (Shaw et al., 2021). There is currently an overreliance on the use of insecticides in conventional apple systems at an estimated typical cost of €400 ha−1 per annum (Cross et al., 2015). Similarly, there are many beneficial organisms that deliver ecosystem services in apple orchards including natural enemies of pests (Albert et al., 2017; Cahenzli et al., 2017; Happe et al., 2019), so the potential for better IPM is clear (Cross et al., 2015; Demestihas et al., 2017). Furthermore, there has been considerable research on IPM in apples and its application in many different contexts, generating significant amounts of relevant data (Damos et al., 2015; Akotsen-Mensah et al., 2020). Therefore, apples offer an ideal model system as they present a key challenge in maintaining fruit yield and quality under significant pest and disease pressures but also have clear opportunities for control using IPM approaches, which could inform management strategies in other crops.
Using apples as a model system, we carried out a meta-analysis to (i) compare IPM systems with more conventional systems; (ii) quantify the effects of IPM on beneficial insects, pest and disease pressure, and crop output; (iii) explore to what extent these effects are moderated by different feeding guilds of pests and beneficials and the intensity to which IPM is being employed (i.e., how responses differ as IPM adoption level increases).
2 Materials and methods
2.1 Study selection and classification of predictors
Original research articles were identified via searches on Web of Science (BIOSIS Citation Index) following the approach by Cooke and Leishman (2012). On 4 September 2023, we identified studies comparing a baseline approach (i.e., conventional farming system) with an IPM or organic approach using the search terms ‘apple’ and ‘fruit’ in combination with terms indicative of IPM [TOPIC: (apple AND conventional AND IPM) OR (apple AND conventional AND integrated pest management) OR (fruit AND conventional AND IPM) OR (fruit AND conventional AND integrated pest management)]. Inspection of titles and abstracts of 543 records identified 101 studies of potential relevance that were obtained for detailed inspection. Our criteria for inclusion in the analysis was that the study must incorporate facets of IPM (cultural, mechanical, biological or reduced/alternative pesticide use; see Table 1 for details) and compare two or more farming approaches (most cases compared conventional and IPM, but we also included studies that compared conventional and organic, as well as those that compared IPM and low-IPM approaches). Forty-eight studies were excluded because they did not meet these criteria, resulting in 55 studies for the meta-analysis (Supplementary Supplementary Table S1; all studies included in reference list). Further details are provided in a PRISMA diagram (Supplementary Figure S1). Where variability information and the number of replicates were ambiguous, studies were excluded. Moreover, studies that were not translated to English were excluded. These were included in the exclusion category ‘no clear performance metric or comparison’ (Supplementary Figure S1).
Experimental work conducted in the extracted studies took place across 20 countries (Supplementary Figure S2). Where possible, an IPM score (1–4) was attributed to each farming approach based on the level of IPM adoption, whereby approaches that incorporated all four facets of IPM (Table 1) achieved a maximum score of four. The number of studies and effect sizes reported for each of the four facets are shown in Supplementary Table S1. For each pair of farming approaches, we calculated the difference between IPM scores to determine how the level of IPM adoption affected different response variables. The five response variables included beneficial invertebrate performance (natural enemy abundance and diversity, % parasitism), disease pressure (disease incidence and severity, infection rate), herbivore pressure (invertebrate pest abundance, infestation and damage intensity), fruit yield (fruit abundance, volume and mass per area) and fruit quality (soluble solids, vitamin C, moisture content, individual fruit mass, colour, firmness and diameter). Mean values, standard deviation (SD) and sample sizes (N) for the responses were recorded. Where studies with missing SDs included repeated measures (e.g., multiple apple cultivars or data points from multiple years), a single value was obtained by taking a mean of the repeated measures. Where SDs were missing and not able to be calculated from multiple data points (one study), they were imputed by averaging those from other studies using the same response variable, which is regarded as a suitable alternative to disregarding studies with missing SDs altogether (Furukawa et al., 2006). Numerical data were extracted from graphical figures using WebPlotDigitizer (Rohatgi, 2022).
2.2 Calculating effect sizes and meta-analytic models
Meta-analyses were performed using the package ‘metafor’ (Viechtbauer, 2010) in the R statistical platform v4.1.1. The effect size (Hedges’ d) was calculated for each pair of responses for the five variables (beneficial invertebrates, herbivore pressure, disease pressure, fruit yield and fruit quality). This effect size measure compares two means using a pooled SD and bias correction (Hedges and Olkin, 1985), with positive values indicating an increase in variable responses under IPM adoption and negative values indicating the opposite (i.e., variables decreased under IPM adoption). Random effects meta-analytic models (Berkey et al., 1998) were analyzed using the rma.mv function in the ‘metafor’ package, including ‘variable’ as a moderator. Study number was included as a random factor because some effect sizes arise from the same study. It was uncertain whether the inclusion of studies that incorporated organic management approaches (N = 19) would confound any effects on yield or other variables because organic approaches can include limitations on fertility practices or alternative fertility practices that may impact yield. Therefore, we compared two models initially; the first incorporated studies that used both IPM and organic management approaches and the second excluded those incorporating organic approaches. This enabled us to determine whether the inclusion of studies comparing organic management with more conventional approaches influenced the overall model predictions (i.e., the overall effects of IPM on the five selected variables). Additional models were run, incorporating invertebrate feeding guild (including predators, parasitoids, stem/leaf miners or borers, foliar tissue-chewers and cell-feeders or suckers) or the Order of invertebrates (including Araneae, Coleoptera, Dermaptera, Diptera, Hemiptera, Hymenoptera, Lepidoptera, Mesostigmata, Neuroptera, and Trombidiformes) as moderators. Specific invertebrate feeding guilds and Orders were identified from 43 and 44 studies, respectively. Only those included in two or more studies (listed above) were accounted for in analyses. Forest plots (Supplementary Figure S3) show the distribution of effect sizes between studies. Estimates with a lower to upper 95% confidence limit (LCL to UCL) not spanning zero were considered statistically significant.
2.3 Publication bias
Publication bias was assessed using a funnel plot across all study variables (Egger et al., 1997), which illustrates the overall relationship between the effect size and sample size. Egger’s regression was applied to determine whether funnel asymmetries were statistically significant (Nakagawa and Santos, 2012). Moreover, comparisons between effect sizes and year of publication were made to determine the presence of any time-lag bias (i.e., a decline in the magnitude of the effect over time) by including ‘Year’ as a moderator in regression models.
2.4 Accounting for changes in the level of IPM adoption
Variables that were recorded in eight or more studies (beneficial invertebrates, herbivore pressure and disease pressure; Supplementary Figures S3A–C, respectively) were compared with the level of IPM adoption using individual rma.mv models. Studies with a score of 3 or 4 were pooled due to the low number of studies that implemented four facets of IPM. The three selected variables were analysed by including ‘IPM score’ (classified as an ordered factor) as a moderator, with 1 and 3 as the lowest and highest adoption rate values, respectively. The random effect ‘study number’ was included in models to account for variation among studies.
3 Results
3.1 Effects of publication bias
There were no significant correlations between effect size and sample size (Supplementary Figure S4), which indicated a lack of publication bias, and year of publication did not affect the overall effect size (t425 = −0.01, p = 0.995), indicating there was no time-lag bias either.
3.2 Effects of IPM adoption on beneficial and herbivorous invertebrates, disease pressure and fruit characteristics
IPM adoption within apple production systems significantly increased beneficial invertebrate performance and decreased herbivore pest pressure, disease pressure and fruit yield. IPM adoption had no significant effect on fruit quality (Figure 1; full statistical results in Supplementary Table S2). When organic approaches were excluded from analyses, the response of the five variables to IPM adoption remained the same and did not influence the overall model predictions (Supplementary Figure S5).
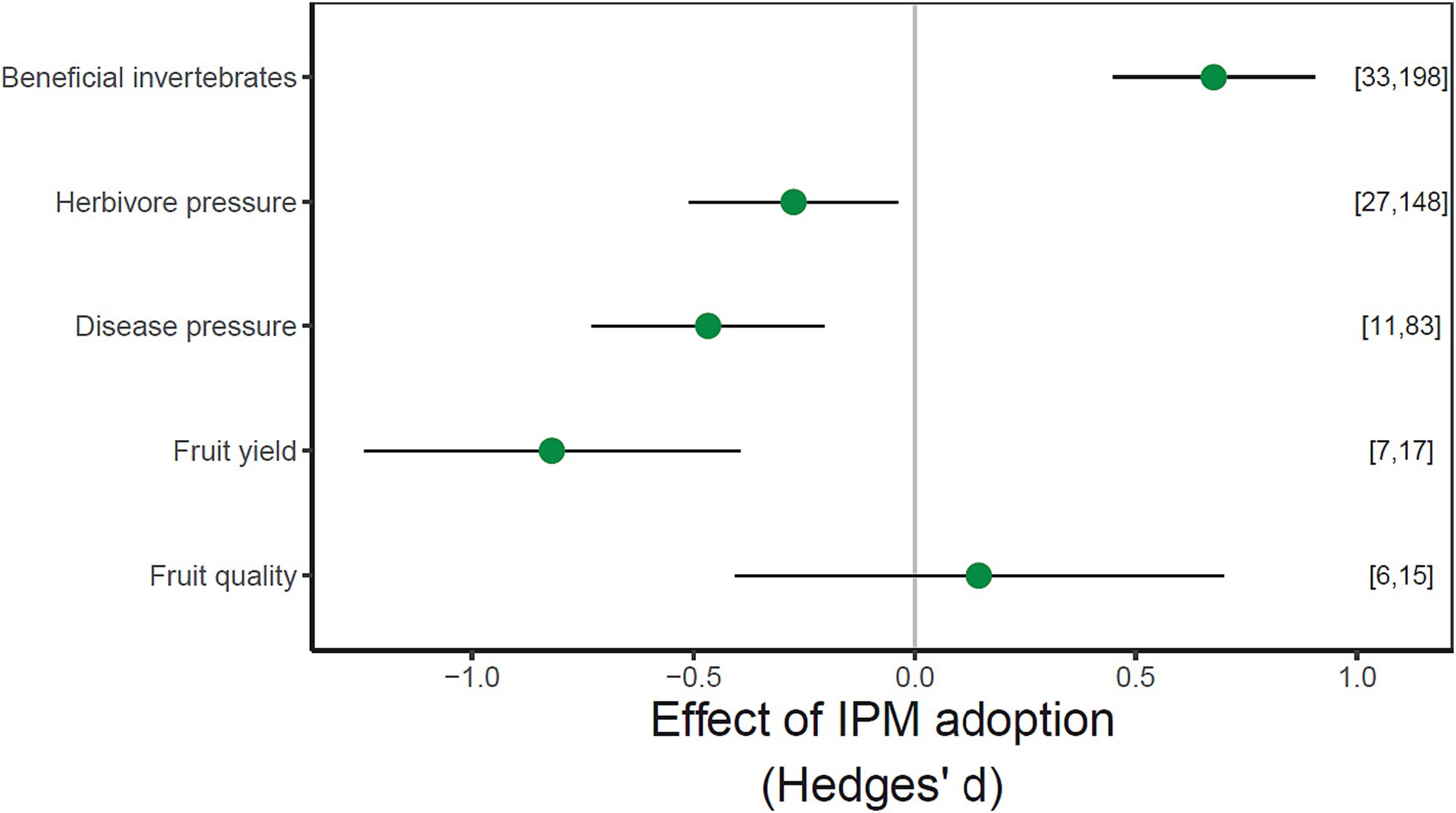
Figure 1. Effects of IPM adoption on the five response variables. Values are estimated mean effect sizes (±95% confidence intervals). Points to the left of the zero line indicate negative impacts and points to the right indicate positive impacts. The number of studies and observations for each response variable indicated in parentheses. Confidence intervals overlapping the zero line are not significantly different from zero (p < 0.05).
3.3 Effects of IPM adoption on invertebrate feeding guilds
IPM adoption significantly increased both types of beneficial invertebrates (predators and parasitoids), but predators increased more than parasitoids. Moreover, IPM adoption reduced the performance of chewing and sucking herbivore pests but had no significant effect on mining or boring herbivores (Figure 2; statistical results in Supplementary Table S3). The positive response of beneficial invertebrates to IPM adoption was driven primarily by Araneae, Hymenopteran parasitoids and Mesostigmata (mostly consisting of predatory mites), whereas Trombidiformes (mostly consisting of herbivorous mites) were responsible for driving the reduction in herbivore pressure under IPM (Supplementary Figure S6; Supplementary Table S3).
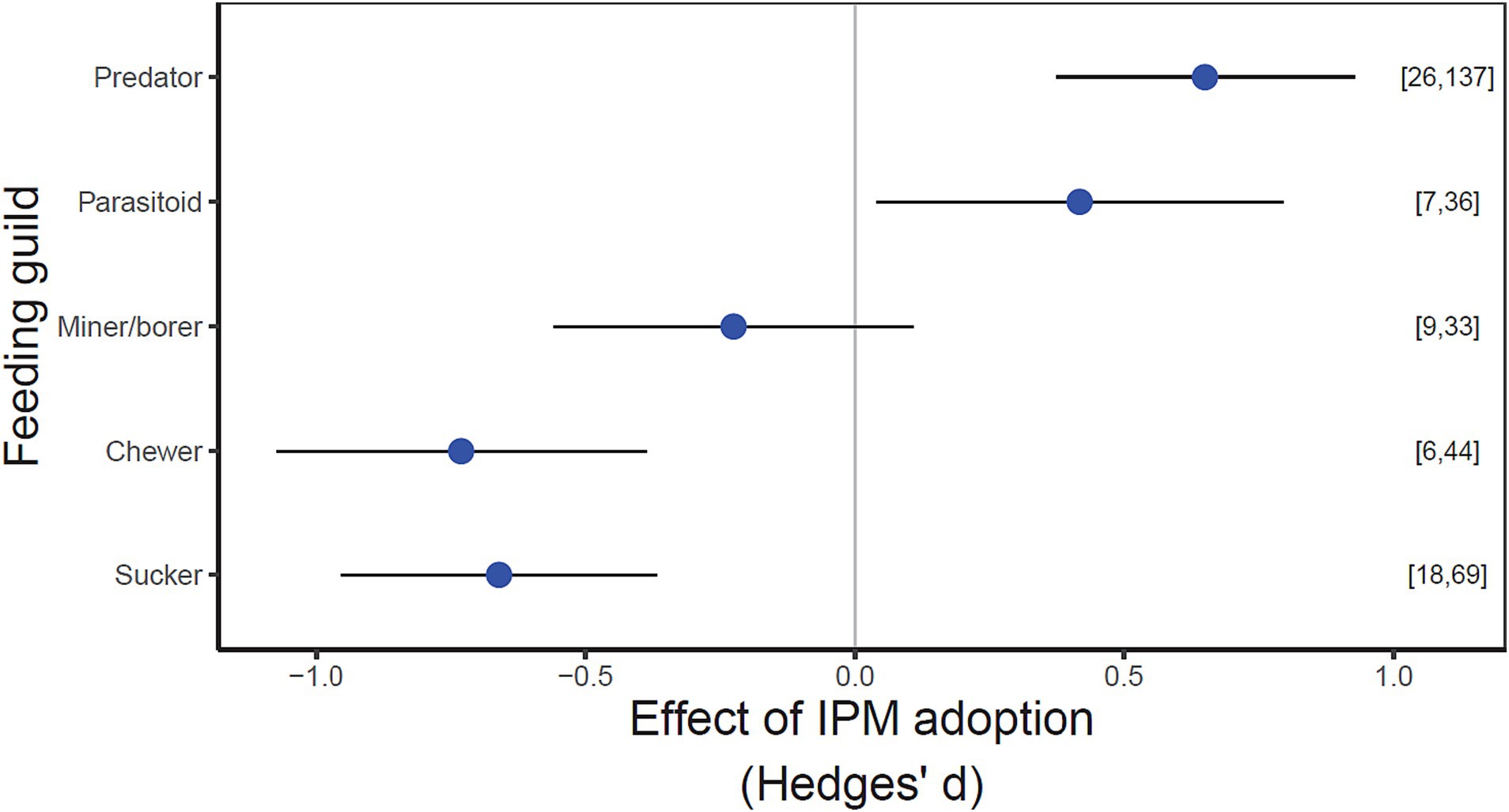
Figure 2. Effects of IPM adoption on different invertebrate feeding guilds. Beneficial invertebrates include predators and parasitoids. Herbivore pests include mining or boring (Miner/borer), cell-feeding (Sucker) and foliar tissue-chewing (Chewer) invertebrates. Values are estimated mean effect sizes (±95% confidence intervals). Points to the left of the zero line indicate negative impacts and points to the right indicate positive impacts. The number of studies and observations for each response variable indicated in parentheses. Confidence intervals overlapping the zero line are not significantly different from zero (p < 0.05).
3.4 How does the level of IPM adoption alter IPM-mediated changes in invertebrate performance and disease pressure?
Reduced/alternative pesticides was the most common IPM strategy or facet used by studies. By comparison, fewer studies incorporated cultural, mechanical and biological controls, and when studies incorporated two or more facets of IPM, they all included reduced/alternative pesticides (Supplementary Table S1). The variables selected for inclusion (beneficial invertebrate performance, herbivore pressure and disease pressure) varied significantly in their responses to the level of IPM adoption (Figure 3). In particular, the performance of beneficial invertebrates increased when studies implemented three or more facets of IPM, whereas the level of IPM adoption had no significant effect on herbivore pressure. While IPM adoption had a negative effect on disease pressure overall (Figure 1), results from studies that included a score of IPM adoption level showed that disease pressure generally increased as the level of IPM adoption increased (Figure 3; statistical results in Supplementary Table S4).
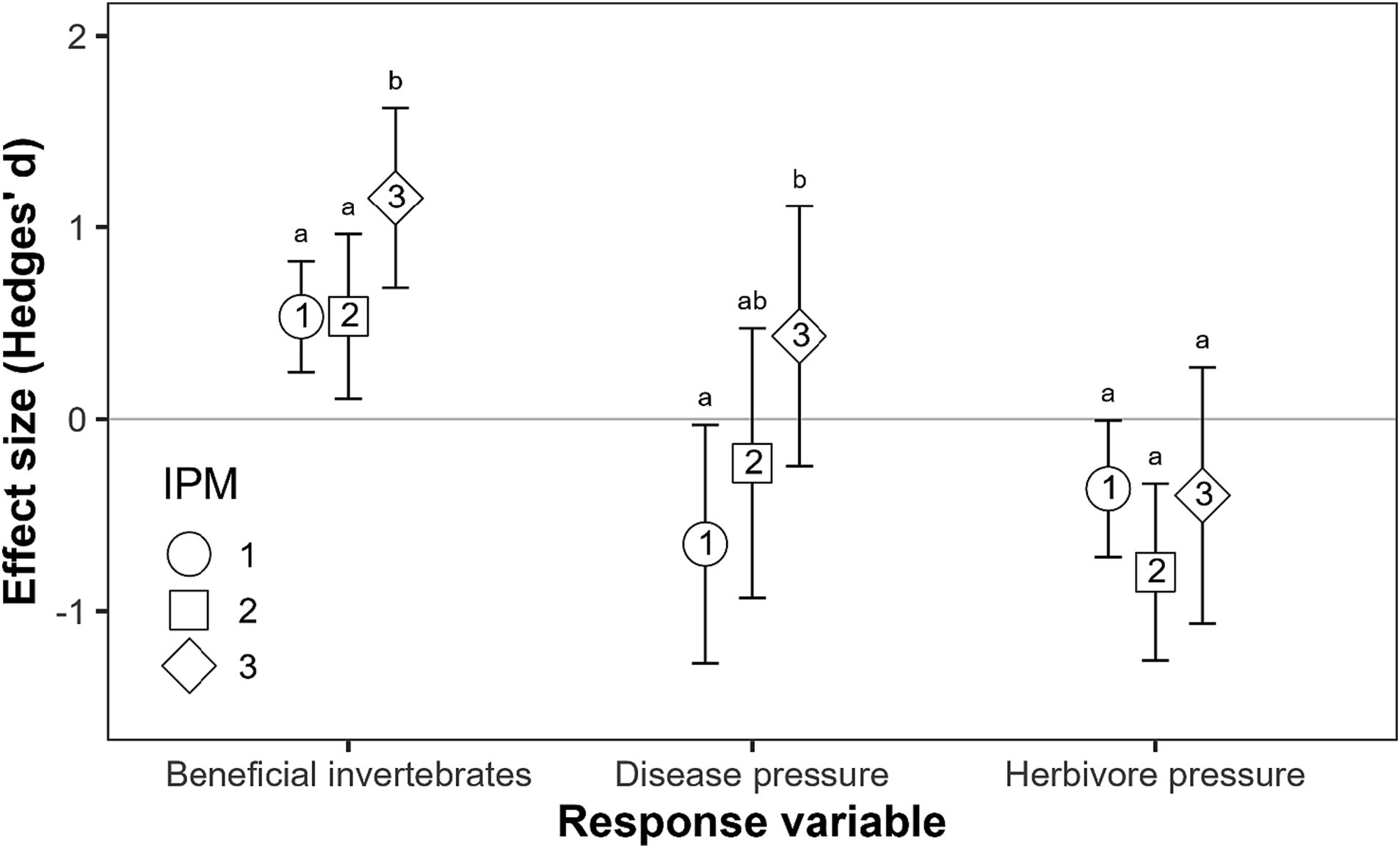
Figure 3. Mean effect size (± 95% confidence intervals) of IPM adoption score (i.e., studies scaled according to their level of IPM adoption) on selected response variables (those with a study N > 8). Numbers/shapes indicate IPM adoption score, with 1 and 3 as the lowest and highest adoption rate values, respectively. Adoption level 3 includes those with three or four facets of IPM. Note: no studies that measured beneficial invertebrate performance included four facets of IPM adoption. Bars with the same letters were not significantly different (p < 0.05) according to summary statistics of meta-analytic models.
4 Discussion
The value of conducting a meta-analysis is that it provides the ability to statistically combine the findings from multiple field trials into one study. Meta-analyses can provide more precise and stable estimates of the effects of experimental treatments, particularly in field studies where empirical sample sizes can be low, thereby providing increased confidence in the overarching results (Arnqvist and Wooster, 1995). In this study, we performed a series of meta-analyses to compare IPM apple production systems with more conventional systems to assess the effect size on a series of response variables.
Our analysis demonstrates a series of environmental and agronomic benefits of the adoption of IPM but, importantly for growers, there are also some production constraints to consider. IPM adoption benefitted apple production systems by (i) increasing the performance of beneficial invertebrates (ii) lowering herbivore pest pressure and (iii) lowering disease pressure generally. There was no difference in quality between fruit from conventional and IPM systems, however, there was a reduction in fruit yield in the IPM systems compared to conventional. This analysis of a global dataset indicates that there is real environmental value in adopting IPM approaches within apple production systems, but an economic assessment is required to determine whether the benefits of reduced pest and disease pressure are outweighed by the reductions in yield. Geographically, the majority of studies were undertaken in the United States and Europe (Supplementary Figure S1), with surprisingly few across South America, Africa, and SE Asia, where apple production is significant (Khan et al., 2022). Global models would benefit from published data from these regions, enabling economic assessments and management decisions that encompass the global supply chain and reflect variable income, production costs and commercial incentives.
Organic systems tend to have lower yields than conventional systems (Seufert et al., 2012), however management trade-off studies within apple systems have shown that IPM systems on average produce higher yields than organic systems (Samnegård et al., 2019) so the yield gap might be smaller for IPM adopters. When organic treatments were excluded from the meta-analysis, overall trends (i.e., the effects of IPM adoption on beneficial and pest invertebrates, disease pressure and fruit yield and quality) did not vary significantly, but IPM reduced both herbivore pressure and fruit yield to a lesser extent (i.e., mean effect sizes were closer to zero; Supplementary Figure S5). This suggests that organic production may have a more negative impact on fruit yield compared with IPM systems in general but may also decrease herbivore pest pressure. The reduction in synthetic fertiliser inputs in studies with organic treatments compared with conventional or IPM treatments may explain these differences. However, of the 18 studies included in the meta-analysis that implemented an organic treatment, only four and six measured changes in yield and herbivore pest pressure, respectively (Supplementary Table S1). Therefore, the inclusion of organic treatment effects were unlikely to alter the overall effects of IPM adoption on these variables. A wider environmental and economic assessment is needed to explore whether IPM apple systems can demonstrate enhanced public goods and services that could offset any reductions in yield. Available data on production metrics and disease pressure in apple lags significantly behind that available for beneficial invertebrate performance and pest pressure, and, as such, the research field would benefit from additional studies quantifying fruit production in IPM compared with non-IPM or reduced-IPM systems to aid economic assessments.
The positive effect of IPM adoption on natural enemy (NE) populations probably explains the simultaneous reduction in pest pressure through improved top-down control by more abundant and species-rich NEs (Dainese et al., 2019). Encouragingly, both NE feeding guilds, predators and parasitoids, responded positively to IPM, with corresponding positive responses seen across the predominantly NE orders Aranea, Hymenoptera and Mesostigmata (Supplementary Figure S6), highlighting the broadly positive effects of IPM for NEs. The most abundant NE species considered by studies were predatory mites (e.g., Typhlodromus spp.), beetles and spiders. A greater effect size was observed for predators compared with parasitoids and this could be explained by their ecology. While predators include groups with low to medium mobility that persist in the cropped area (predatory mites, beetles and spiders), parasitoids are almost exclusively alate hymenopterans which are relatively more mobile. Therefore, the abundance of predators could be determined by in-field management approaches as determined by IPM (Pearsons and Tooker, 2017), while relatively mobile parasitoid populations are more responsive to landscape context and the presence of non-crop areas (Martins et al. 2019), beyond the direct influence of an IPM treatment. To promote maximum pest control delivery by multiple natural enemy guilds both in-field and landscape-scale factors should be considered and manipulated as part of IPM (Ricci et al., 2019; Girling et al., 2022).
Two major pest feeding guilds (suckers and chewers, of which the wooly apple aphid (Eriosoma lanigerum) and obliquebanded leafroller (Choristoneura rosaceana) were the most abundant species considered, respectively) showed similar negative responses to IPM, despite the rather variable response among individual orders. However, this was not the case for miners/borers, for which the effect size was close to half that of other pest guilds. The majority of miners/borers considered were the codling moth (Cydia pomonella). With their larval stages often protected within the leaf or stem, miners and boring pests may be less susceptible to generalist predators, with some evidence indicating parasitoids are a greater mortality factor (Salvo and Valladares, 2007). Therefore, IPM approaches, which reduce pesticide inputs and seek to boost generalist natural enemies, may be less effective against these pests. The response to IPM varied between different feeding guilds and Orders of pest and beneficial invertebrates (Figure 1; Supplementary Figure S6). Therefore, IPM should be developed to incorporate approaches, which deliver the greatest benefits for pest control in the systems where it is deployed, i.e., by controlling key pests directly or by promoting NE populations best capable of controlling those pests (Barzman et al., 2015). However not disregarding the fact that more species rich or functionally diverse natural enemy communities often deliver greater pest control (Dainese et al., 2019; Snyder, 2019).
Analysing the effect of the IPM adoption score on selected response variables revealed that even low levels of IPM adoption (e.g., reduced or alternative pesticides alone) are likely to be effective against pest populations. Increasing levels of adoption of IPM strategies, including zero residue production systems, are potentially accompanied by positive effects on beneficial invertebrates, with even greater increases seen when more than two facets of IPM were adopted. However, the level of IPM adoption was positively correlated with disease pressure, such that disease pressure was significantly higher when studies incorporated three or more facets of IPM. This suggests that increasing the level of IPM adoption is also likely to be accompanied by a higher risk of pathogens and disease incidence, including apple scab, which was represented in the majority of studies, followed by sooty blotch and flyspeck. Thus, the development of IPM strategies, aimed at reducing pesticide use, should be accompanied by an increase in prevention and monitoring practices, together with strong training of farmers and technicians in pest and disease management strategies.
This study provides a useful overview, but further work is needed to understand the value of IPM in apple production systems. Methodologies for growers to explore and evaluate the economic benefits of IPM need to be developed (Girling et al., 2022) and viable monitoring systems are required to evaluate the effectiveness of IPM adoption (Barzman et al., 2015; Creissen et al., 2019). In addition, a universal approach to IPM adoption is needed to meet targets for sustainable food production systems and build ecological resilience within food systems to prevent future outbreaks of pests (Ortega-Ramos et al., 2022). Innovative technologies are in development that can help transform the way in which the food system operates (Herrero et al., 2020), but these require trust, incentives, and regulation for them to be widely adopted. A collaborative effort is now needed to bring growers, researchers, educators, advisors, industry consultants, policy makers and wider stakeholders together to develop IPM approaches that can support sustainable and regenerative food production systems (Baker et al., 2020).
Data availability statement
The raw data supporting the conclusions of this article will be made available by the authors, without undue reservation.
Author contributions
JR: Conceptualization, Methodology, Visualization, Writing – original draft, Writing – review & editing. MG: Conceptualization, Writing – original draft, Writing – review & editing. DS: Writing – original draft, Writing – review & editing. AM: Conceptualization, Writing – original draft, Writing – review & editing.
Funding
The author(s) declare financial support was received for the research, authorship, and/or publication of this article. This work was supported by the European Union within the EIT Food initiative (CLEANFRUIT - Standardization of innovative pest control strategies to produce zero residue fruit for baby food and other fruit produce - KAVA 20152) and a NERC Treescapes grant (NE/Y004191/1). JR was supported by a Leverhulme Trust Early Career Fellowship [grant number ECF-2020-017] and University of Reading’s National Institute for Research into Dairying (NIRD) Trust.
Acknowledgments
We would like to thank Michelle Felton, Richard Tranter, Lisa Bromfield, Louise Truslove and Philip Jones for their support and advice. We would also like to thank the articles from which we extracted the data for this meta-analysis (Cooley et al., 1997; Samu et al., 1997; van Driesche et al., 1998; Bogya and Markó, 1999; Suckling et al., 1999; Pekár, 1999a,b; Biggs et al., 2000; Bogya et al., 2000; Hardman et al., 2000; Epstein et al., 2001; Reganold et al., 2001; Agnello et al., 2003; Babadoost et al., 2004; Ramírez Legarreta et al., 2004; Trimble and Appleby, 2004; Markó and Kádár, 2005; Nicholas et al., 2005; Peck et al., 2006; Atlamaz et al., 2007; Garcia-Salazar et al., 2007; Sarvary et al., 2007; Cross and Berrie, 2008; Delate et al., 2008; Markó et al., 2009; Roussos and Gasparatos, 2009; Wearing et al., 2010, 2011a,b; Piñero et al., 2011; Mates et al., 2012; Ahmad et al., 2013; Monteiro et al., 2013; Rozman et al., 2013; Szabó et al., 2014; Tamošiūnas et al., 2014; Fountain and Harris, 2015; Funayama et al., 2015; Hedde et al., 2015; Mazzia et al., 2015; Schmidt-Jeffris et al., 2015; Warabieda, 2015; Dib et al., 2016; Givaudan et al., 2016; Holb et al., 2017; Lefebvre et al., 2017; Frank, 2018; Porcel et al., 2018; Tkaczuk et al., 2019; Orpet et al., 2020; Średnicka-Tober et al., 2020; Chen et al., 2021; Stupp et al., 2021; Erler and Tosun, 2023; Hulsmans et al., 2023).
Conflict of interest
The authors declare that the research was conducted in the absence of any commercial or financial relationships that could be construed as a potential conflict of interest.
The author(s) declared that they were an editorial board member of Frontiers, at the time of submission. This had no impact on the peer review process and the final decision.
Publisher’s note
All claims expressed in this article are solely those of the authors and do not necessarily represent those of their affiliated organizations, or those of the publisher, the editors and the reviewers. Any product that may be evaluated in this article, or claim that may be made by its manufacturer, is not guaranteed or endorsed by the publisher.
Supplementary material
The Supplementary material for this article can be found online at: https://www.frontiersin.org/articles/10.3389/fsufs.2024.1321067/full#supplementary-material
References
Agnello, A. M., Reissig, W. H., Kovach, J., and Nyrop, J. P. (2003). Integrated apple pest management in New York state using predatory mites and selective pesticides. Agric. Ecosyst. Environ. 94, 183–195. doi: 10.1016/S0167-8809(02)00026-9
Ahmad, S., Pozzebon, A., and Duso, C. (2013). Augmentative releases of the predatory mite Kampimodromus aberrans in organic and conventional apple orchards. Crop Prot. 52, 47–56. doi: 10.1016/j.cropro.2013.05.008
Akotsen-Mensah, C., Blaauw, B., Short, B., Leskey, T. C., Bergh, J. C., Polk, D., et al. (2020). Using IPM-CPR as a management program for apple orchards. J. Econ. Entomol. 113, 1894–1902. doi: 10.1093/jee/toaa087
Albert, L., Franck, P., Gilles, Y., and Plantegenest, M. (2017). Impact of agroecological infrastructures on the dynamics of Dysaphis plantaginea (Hemiptera: Aphididae) and its natural enemies in apple orchards in northwestern France. Environ. Entomol. 46, 528–537. doi: 10.1093/ee/nvx054
Alwang, J., Norton, G., and Larochelle, C. (2019). Obstacles to widespread diffusion of IPM in developing countries: lessons from the field. J. Integ. Pest Manag. 10, 1–8. doi: 10.1093/jipm/pmz008
Arnqvist, G., and Wooster, D. (1995). Meta-analysis: synthesizing research findings in ecology and evolution. Trends Ecol. Evol. 10, 236–240. doi: 10.1016/S0169-5347(00)89073-4
Atlamaz, A., Zeki, C., and Uludag, A. (2007). The importance of forecasting and warning systems in implementation of integrated pest management in apple orchards in Turkey. EPPO Bullet. 37, 295–299. doi: 10.1111/j.1365-2338.2007.01129.x
Babadoost, M., McManus, P. S., Helland, S. N., and Gleason, M. L. (2004). Evaluating a wetness-based warning system and reduced-risk fungicides to manage sooty blotch and flyspeck of apple. HortTechnology 14, 51–57. doi: 10.21273/HORTTECH.14.1.0051
Baker, B. P., Green, T. A., and Loker, A. J. (2020). Biological control and integrated pest management in organic and conventional systems. Biol. Control 140:104095. doi: 10.1016/j.biocontrol.2019.104095
Barzman, M., Bàrberi, P., Birch, A. N. E., Boonekamp, P., Dachbrodt-Saaydeh, S., Graf, B., et al. (2015). Eight principles of integrated pest management. Agron. Sustain. Dev. 35, 1199–1215. doi: 10.1007/s13593-015-0327-9
Berkey, C. S., Hoaglin, D. C., Antczak-Bouckoms, A., Mosteller, F., and Colditz, G. A. (1998). Meta-analysis of multiple outcomes by regression with random effects. Stat. Med. 17, 2537–2550. doi: 10.1002/(SICI)1097-0258(19981130)17:22<2537::AID-SIM953>3.0.CO;2-C
Biggs, A. R., Hogmire, H. W., and Collins, A. R. (2000). Assessment of an alternative IPM program for the production of apples for processing. Plant Dis. 84, 1140–1146. doi: 10.1094/PDIS.2000.84.10.1140
Bogya, S., and Markó, V. (1999). Effect of pest management systems on ground-dwelling spider assemblages in an apple orchard in Hungary. Agric. Ecosyst. Environ. 73, 7–18. doi: 10.1016/S0167-8809(99)00010-9
Bogya, S., Marko, V., and Szinetar, C. S. (2000). Effect of pest management systems on foliage- and grass-dwelling spider communities in an apple orchard in Hungary. Int. J. Pest Manag. 46, 241–250. doi: 10.1080/09670870050206000
Cahenzli, F., Pfiffner, L., and Daniel, C. (2017). Reduced crop damage by self-regulation of aphids in an ecologically enriched, insecticide-free apple orchard. Agron. Sustain. Dev. 37:65. doi: 10.1007/s13593-017-0476-0
Chen, L., Zhu, H., Horst, L., Wallhead, M., Reding, M., and Fulcher, A. (2021). Management of pest insects and plant diseases in fruit and nursery production with laser-guided variable-rate sprayers. HortSci. Horts 56, 94–100. doi: 10.21273/HORTSCI15491-20
Cooke, J., and Leishman, M. R. (2012). Tradeoffs between foliar silicon and carbon-based defences: evidence from vegetation communities of contrasting soil types. Oikos 121, 2052–2060. doi: 10.1111/j.1600-0706.2012.20057.x
Cooley, D. R., Gamble, J. W., and Autio, W. R. (1997). Summer pruning as a method for reducing flyspeck disease on apple fruit. Plant Dis. 81, 1123–1126. doi: 10.1094/PDIS.1997.81.10.1123
Creissen, H. E., Jones, P. J., Tranter, R. B., Girling, R. D., Jess, S., Burnett, F. J., et al. (2019). Measuring the unmeasurable? A method to quantify adoption of integrated pest management practices in temperate arable farming systems. Pest Manag. Sci. 75, 3144–3152. doi: 10.1002/ps.5428
Cross, J. V., and Berrie, A. (2008). Eliminating the Occurrence of Reportable Pesticide Residues in Apples”. Agricultural Engineering International: the CIGR Ejournal. Manuscript ALNARP 08 004.
Cross, J., Fountain, M., Markó, V., and Nagy, C. (2015). Arthropod ecosystem services in apple orchards and their economic benefits. Ecol. Entomol. 40, 82–96. doi: 10.1111/een.12234
Dainese, M., Martin, E. A., Aizen, M. A., Albrecht, M., Bartomeus, I., Bommarco, R., et al. (2019). A global synthesis reveals biodiversity-mediated benefits for crop production. Sci. Adv. 5:eaax0121. doi: 10.1126/sciadv.aax0121
Damos, P., Colomar, L.-A. E., and Ioriatti, C. (2015). Integrated fruit production and pest management in Europe: the apple case study and how far we are from the original concept? Insects 6, 626–657. doi: 10.3390/insects6030626
Deguine, J.-P., Aubertot, J.-N., Flor, R. J., Lescourret, F., Wyckhuys, K. A. G., and Ratnadass, A. (2021). Integrated pest management: good intentions, hard realities. A review. Agron. Sustain. Dev. 41:38. doi: 10.1007/s13593-021-00689-w
Delate, K., McKern, A., Turnbull, R., Walker, J. T. S., Volz, R., White, A., et al. (2008). Organic apple production in two humid regions: comparing progress in pest management strategies in Iowa and New Zealand. HortScience 43, 12–21. doi: 10.21273/HORTSCI.43.1.12
Demestihas, C., Plénet, D., Génard, M., Raynal, C., and Lescourret, F. (2017). Ecosystem services in orchards. A review. Agron. Sustain. Dev. 37:12. doi: 10.1007/s13593-017-0422-1
Dib, H., Sauphanor, B., and Capowiez, Y. (2016). Effect of management strategies on arthropod communities in the colonies of rosy apple aphid, Dysaphis plantaginea Passerini (Hemiptera: Aphididae) in South-Eastern France. Agric. Ecosyst. Environ. 216, 203–206. doi: 10.1016/j.agee.2015.10.003
Egger, M., Smith, G. D., Schneider, M., and Minder, C. (1997). Bias in meta-analysis detected by a simple, graphical test. BMJ 315, 629–634. doi: 10.1136/bmj.315.7109.629
Epstein, D. L., Zack, R. S., Brunner, J. F., Gut, L., and Brown, J. J. (2001). Ground beetle activity in apple orchards under reduced pesticide management regimes. Biol. Control 21, 97–104. doi: 10.1006/bcon.2001.0929
Erler, F., and Tosun, H. S. (2023). Mass-trapping the codling moth, Cydia pomonella (L.) (Lepidoptera: Tortricidae), using newly designed light trap reduces fruit damage in apple orchards. J. Plant Dis. Prot. 130, 795–807. doi: 10.1007/s41348-023-00735-7
European Food Safety AuthorityCarrasco Cabrera, L., and Medina Pastor, P. (2021). The 2019 European Union report on pesticide residues in food. EFSA J. 19:e06491. doi: 10.2903/j.efsa.2021.6491
FAO (2021). World food and agriculture – Statistical yearbook 2021. Rome: European Food Safety Authority.
FAOStat (2022). Crops and livestock products [online]. Food and Agriculture Organization of the United Nations. Available: https://www.fao.org/faostat/en/#data/QCL (Accessed November 02, 2022).
Fountain, M. T., and Harris, A. L. (2015). Non-target consequences of insecticides used in apple and pear orchards on Forficula auricularia L. (Dermaptera: Forficulidae). Biol. Control 91, 27–33. doi: 10.1016/j.biocontrol.2015.07.007
Frank, D. L. (2018). Evaluation of fruit bagging as a pest management option for direct pests of apple. Insects 9:178. doi: 10.3390/insects9040178
Funayama, K., Komatus, M., Sonoda, S., Takahashi, I., and Hara, K. (2015). Management of apple orchards to conserve generalist phytoseiid mites suppresses two-spotted spider mite, Tetranychus urticae (Acari: Tetranychidae). Exp. Appl. Acarol. 65, 43–54. doi: 10.1007/s10493-014-9850-7
Furukawa, T. A., Barbui, C., Cipriani, A., Brambilla, P., and Watanabe, N. (2006). Imputing missing standard deviations in meta-analyses can provide accurate results. J. Clin. Epidemiol. 59, 7–10. doi: 10.1016/j.jclinepi.2005.06.006
Garcia-Salazar, C., Gut, L. J., and Whalon, M. E. (2007). Hedgerow barriers and other reduced-risk controls for managing oriental fruit moth, Grapholitha molesta (Busck) (Lepidoptera: Tortricidae) in apples. Renew Agricul. Food Syst. 22, 181–188. doi: 10.1017/S174217050700172X
Garratt, M. P. D., Wright, D. J., and Leather, S. R. (2011). The effects of farming system and fertilisers on pests and natural enemies: a synthesis of current research. Agric. Ecosyst. Environ. 141, 261–270. doi: 10.1016/j.agee.2011.03.014
Girling, R. D., Breeze, T. D., and Garratt, M. P. (2022). “Advancing conservation biological control as a component of IPM of horticultural crops” in Improving integrated Pest management in horticulture. ed. R. Collier (Cambridge, UK: Burleigh Dodds)
Givaudan, N., Suchail, S., Rault, M., Mouneyrac, C., and Capowiez, Y. (2016). Impact of apple orchard management strategies on earthworm (Allolobophora chlorotica) energy reserves. Soil Biol. Biochem. 100, 252–254. doi: 10.1016/j.soilbio.2016.06.031
Happe, A.-K., Alins, G., Blüthgen, N., Boreux, V., Bosch, J., García, D., et al. (2019). Predatory arthropods in apple orchards across Europe: responses to agricultural management, adjacent habitat, landscape composition and country. Agric. Ecosyst. Environ. 273, 141–150. doi: 10.1016/j.agee.2018.12.012
Hardman, J. M., Moreau, D. L., Snyder, M., Gaul, S. O., and Bent, E. D. (2000). Performance of a pyrethroid-resistant strain of the predator mite Typhlodromus pyri (Acari: Phytoseiidae) under different insecticide regimes. J. Econ. Entomol. 93, 590–604. doi: 10.1603/0022-0493-93.3.590
Hedde, M., Mazzia, C., Decaëns, T., Nahmani, J., Pey, B., Thénard, J., et al. (2015). Orchard management influences both functional and taxonomic ground beetle (Coleoptera, Carabidae) diversity in south-East France. Appl. Soil Ecol. 88, 26–31. doi: 10.1016/j.apsoil.2014.11.014
Hedges, L.V., and Olkin, I. (1985). Statistical methods for Meta-analysis. New York, USA: Academic Press.
Herrero, M., Thornton, P. K., Mason-D’Croz, D., Palmer, J., Benton, T. G., Bodirsky, B. L., et al. (2020). Innovation can accelerate the transition towards a sustainable food system. Nat. Food 1, 266–272. doi: 10.1038/s43016-020-0074-1
Holb, I. J., Abonyi, F., Buurma, J., and Heijne, B. (2017). On-farm and on-station evaluations of three orchard management approaches against apple scab and apple powdery mildew. Crop Prot. 97, 109–118. doi: 10.1016/j.cropro.2016.11.023
Hulsmans, E., Daelemans, R., Cuypers, V., Van Der Straeten, E., Vanderlinden, M., De Blanck, T., et al. (2023). Cascading effects of management and landscape on insect pollinators, pollination services and yield in apple orchards. Agric. Ecosyst. Environ. 352:108509. doi: 10.1016/j.agee.2023.108509
Jacquet, F., Butault, J.-P., and Guichard, L. (2011). An economic analysis of the possibility of reducing pesticides in French field crops. Ecol. Econ. 70, 1638–1648. doi: 10.1016/j.ecolecon.2011.04.003
Johnson, A. C., Liu, J., Reynolds, O., Furlong, M. J., Mo, J., Rizvi, S., et al. (2021). Conservation biological control research is strongly uneven across trophic levels and economic measures. Pest Manag. Sci. 77, 2165–2169. doi: 10.1002/ps.6162
Kansiime, M. K., Girling, R. D., Mugambi, I., Mulema, J., Oduor, G., Chacha, D., et al. (2021). Rural livelihood diversity and its influence on the ecological intensification potential of smallholder farms in Kenya. Food Energy Secur. 10:e254. doi: 10.1002/fes3.254
Khan, S., Iqbal, M. Z., Bodhla, A., and Been, T. J. (2022). “History, distribution, production and taxonomic classification” in Apples. eds. R. Mushtaq, G. A. Nayik, and A. R. Malik (Abingdon, Oxon: CRC Press), 1–8.
Kim, K.-H., Kabir, E., and Jahan, S. A. (2017). Exposure to pesticides and the associated human health effects. Sci. Total Environ. 575, 525–535. doi: 10.1016/j.scitotenv.2016.09.009
Kleijn, D., Bommarco, R., Fijen, T. P. M., Garibaldi, L. A., Potts, S. G., and van der Putten, W. H. (2019). Ecological intensification: bridging the gap between science and practice. Trends Ecol. Evol. 34, 154–166. doi: 10.1016/j.tree.2018.11.002
Kuchheuser, P., and Birringer, M. (2022). Pesticide residues in food in the European Union: analysis of notifications in the European rapid alert system for food and feed from 2002 to 2020. Food Control 133:108575. doi: 10.1016/j.foodcont.2021.108575
Lamichhane, J. R., Dachbrodt-Saaydeh, S., Kudsk, P., and Messéan, A. (2016). Toward a reduced reliance on conventional pesticides in European agriculture. Plant Dis. 100, 10–24. doi: 10.1094/PDIS-05-15-0574-FE
Lechenet, M., Dessaint, F., Py, G., Makowski, D., and Munier-Jolain, N. (2017). Reducing pesticide use while preserving crop productivity and profitability on arable farms. Nat. Plants 3:17008. doi: 10.1038/nplants.2017.8
Lefebvre, M., Franck, P., Olivares, J., Ricard, J.-M., Mandrin, J.-F., and Lavigne, C. (2017). Spider predation on rosy apple aphid in conventional, organic and insecticide-free orchards and its impact on aphid populations. Biol. Control 104, 57–65. doi: 10.1016/j.biocontrol.2016.10.009
Martins, L. P., Medina, A. M., Lewinsohn, T. M., and Almeida-Neto, M. (2019). Trophic level and host specialisation affect beta-diversity in plant–herbivore–parasitoid assemblages. Insect Conservation and Diversity. 12, 404–413.
Markó, V., and Kádár, F. (2005). Effects of different insecticide disturbance levels and weed patterns on carabid beetle assemblages. Acta Phytopathol Entomol Hung 40, 111–143. doi: 10.1556/APhyt.40.2005.1-2.11
Markó, V., Keresztes, B., Fountain, M. T., and Cross, J. V. (2009). Prey availability, pesticides and the abundance of orchard spider communities. Biol. Control 48, 115–124. doi: 10.1016/j.biocontrol.2008.10.002
Mates, S. G., Perfecto, I., and Badgley, C. (2012). Parasitoid wasp diversity in apple orchards along a pest-management gradient. Agric. Ecosyst. Environ. 156, 82–88. doi: 10.1016/j.agee.2012.04.016
Mazzia, C., Pasquet, A., Caro, G., Thénard, J., Cornic, J. F., Hedde, M., et al. (2015). The impact of management strategies in apple orchards on the structural and functional diversity of epigeal spiders. Ecotoxicology 24, 616–625. doi: 10.1007/s10646-014-1409-1
Mesnage, R., Defarge, N., Spiroux de Vendômois, J., and Séralini, G.-E. (2014). Major pesticides are more toxic to human cells than their declared active principles. Biomed. Res. Int. 2014:179691, 1–8. doi: 10.1155/2014/179691
Monteiro, L. B., Souza, A., and Argenton, J. (2013). Mating disruption with low density diffusers for the management of oriental fruit moths (Lepidoptera: Tortricidae) in apple orchards under subtropical climate in southern Brazil. Rev. Bras. Frutic. 35, 1007–1016. doi: 10.1590/S0100-29452013000400011
Nakagawa, S., and Santos, E. S. A. (2012). Methodological issues and advances in biological meta-analysis. Evol. Ecol. 26, 1253–1274. doi: 10.1007/s10682-012-9555-5
Nicholas, A. H., Spooner-Hart, R. N., and Vickers, R. A. (2005). Abundance and natural control of the woolly aphid Eriosoma lanigerum in an Australian apple orchard IPM program. BioControl 50, 271–291. doi: 10.1007/s10526-004-0334-2
Orpet, R. J., Jones, V. P., Beers, E. H., Reganold, J. P., Goldberger, J. R., and Crowder, D. W. (2020). Perceptions and outcomes of conventional vs. organic apple orchard management. Agric. Ecosyst. Environ. 289:106723. doi: 10.1016/j.agee.2019.106723
Ortega-Ramos, P. A., Cook, S. M., and Mauchline, A. L. (2022). How contradictory EU policies led to the development of a pest: the story of oilseed rape and the cabbage stem flea beetle. GCB Bioenergy 14, 258–266. doi: 10.1111/gcbb.12922
Pearsons, K. A., and Tooker, J. F. (2017). In-field habitat management to optimize pest control of novel soil communities in agroecosystems. Insects 8:82. doi: 10.3390/insects8030082
Peck, G. M., Andrews, P. K., Reganold, J. P., and Fellman, J. K. (2006). Apple orchard productivity and fruit quality under organic, conventional, and integrated management. HortScience 41, 99–107. doi: 10.21273/HORTSCI.41.1.99
Pekár, S. (1999a). Effect of IPM practices and conventional spraying on spider population dynamics in an apple orchard. Agric. Ecosyst. Environ. 73, 155–166. doi: 10.1016/S0167-8809(99)00024-9
Pekár, S. (1999b). Side-effect of integrated pest management and conventional spraying on the composition of epigeic spiders and harvestmen in an apple orchard (Araneae, Opiliones). J. Appl. Entomol. 123, 115–120. doi: 10.1046/j.1439-0418.1999.00324.x
Piñero, J. C., Agnello, A. M., Tuttle, A., Leskey, T. C., Faubert, H., Koehler, G., et al. (2011). Effectiveness of odor-baited trap trees for plum curculio (Coleoptera: Curculionidae) monitoring in commercial apple orchards in the northeast. J. Econ. Entomol. 104, 1613–1621. doi: 10.1603/EC10310
Porcel, M., Andersson, G. K. S., Pålsson, J., and Tasin, M. (2018). Organic management in apple orchards: higher impacts on biological control than on pollination. J. Appl. Ecol. 55, 2779–2789. doi: 10.1111/1365-2664.13247
Prokopy, R., and Kogan, M. (2009). “Chapter 139 - integrated Pest management” in Encyclopedia of insects. eds. V. H. Resh and R. T. Cardé. 2nd ed (San Diego: Academic Press), 523–528.
Ramírez Legarreta, M. R., Jacobo Cuéllar, J. L., Ávila Marioni, M. R., and Gutiérrez González, R. (2004). Validación de un Sistema de Manejo Integrado de Plagas y Enfermedades en Huertos de Manzano [Malus sylvestris (L.) Mill. var. domestica (Borkh.) Mansf.] en Chihuahua, México. Revista Mexicana de Fitopatología 22, 277–289.
Reganold, J. P., Glover, J. D., Andrews, P. K., and Hinman, H. R. (2001). Sustainability of three apple production systems. Nature 410, 926–930. doi: 10.1038/35073574
Ricci, B., Lavigne, C., Alignier, A., Aviron, S., Biju-Duval, L., Bouvier, J. C., et al. (2019). Local pesticide use intensity conditions landscape effects on biological pest control. Proc. R. Soc. B Biol. Sci. 286:20182898. doi: 10.1098/rspb.2018.2898
Rohatgi, A. (2022). WebPlotDigitizer [Online]. Pacifica, California, USA. Available at: https://automeris.io/WebPlotDigitizer.
Roussos, P. A., and Gasparatos, D. (2009). Apple tree growth and overall fruit quality under organic and conventional orchard management. Sci. Hortic. 123, 247–252. doi: 10.1016/j.scienta.2009.09.011
Rozman, Č., Unuk, T., Pažek, K., Lešnik, M., Prišenk, J., Vogrin, A., et al. (2013). Multi criteria assessment of zero residue apple production. Erwerbs-obstbau 55, 51–62. doi: 10.1007/s10341-013-0186-y
Salvo, A., and Valladares, G. R. (2007). Leafminer parasitoids and pest management. Ciencia e Investigación Agraria 34, 125–142. doi: 10.7764/rcia.v34i3.396
Samnegård, U., Alins, G., Boreux, V., Bosch, J., García, D., Happe, A.-K., et al. (2019). Management trade-offs on ecosystem services in apple orchards across Europe: direct and indirect effects of organic production. J. Appl. Ecol. 56, 802–811. doi: 10.1111/1365-2664.13292
Samu, F., Rácz, V., Erdélyi, C., and Balázs, K. (1997). Spiders of the foliage and herbaceous layer of an IPM apple archard in Kecskemét-Szarkás, Hungary. Biol. Agric. Hortic. 15, 131–140. doi: 10.1080/01448765.1997.9755187
Sánchez-Bayo, F., and Wyckhuys, K. A. G. (2019). Worldwide decline of the entomofauna: a review of its drivers. Biol. Conserv. 232, 8–27. doi: 10.1016/j.biocon.2019.01.020
Sarvary, M. A., Nyrop, J., Reissig, H., and Gifford, K. M. (2007). Potential for conservation biological control of the obliquebanded leafroller (OBLR) Choristoneura rosaceana (Harris) in orchard systems managed with reduced-risk insecticides. Biol. Control 40, 37–47. doi: 10.1016/j.biocontrol.2006.09.006
Schmidt-Jeffris, R. A., Beers, E. H., and Crowder, D. W. (2015). Phytoseiids in Washington commercial apple orchards: biodiversity and factors affecting abundance. Exp. Appl. Acarol. 67, 21–34. doi: 10.1007/s10493-015-9927-y
Seufert, V., Ramankutty, N., and Foley, J. A. (2012). Comparing the yields of organic and conventional agriculture. Nature 485, 229–232. doi: 10.1038/nature11069
Shaw, B., Nagy, C., and Fountain, M. T. (2021). Organic control strategies for use in IPM of invertebrate pests in apple and pear orchards. Insects 12, 1106. doi: 10.3390/insects12121106
Snyder, W. E. (2019). Give predators a complement: conserving natural enemy biodiversity to improve biocontrol. Biol. Control 135, 73–82. doi: 10.1016/j.biocontrol.2019.04.017
Średnicka-Tober, D., Barański, M., Kazimierczak, R., Ponder, A., Kopczyńska, K., and Hallmann, E. (2020). Selected antioxidants in organic vs. conventionally grown apple fruits. Appl. Sci. 10:2997. doi: 10.3390/app10092997
Staton, T., Walters, R. J., Smith, J., Breeze, T. D., and Girling, R. D. (2021). Evaluating a trait-based approach to compare natural enemy and pest communities in agroforestry vs. arable systems. Ecol. Appl. 31:e02294. doi: 10.1002/eap.2294
Stehle, S., and Schulz, R. (2015). Agricultural insecticides threaten surface waters at the global scale. Proc. Natl. Acad. Sci. 112, 5750–5755. doi: 10.1073/pnas.1500232112
Stupp, P., Junior, R. M., Nora Cardoso, T. D., Padilha, A. C., Hoffer, A., Bernardi, D., et al. (2021). Mass trapping is a viable alternative to insecticides for management of Anastrepha fraterculus (Diptera: Tephritidae) in apple orchards in Brazil. Crop Prot. 139:105391. doi: 10.1016/j.cropro.2020.105391
Suckling, D. M., Walker, J. T. S., and Wearing, C. H. (1999). Ecological impact of three pest management systems in New Zealand apple orchards. Agric. Ecosyst. Environ. 73, 129–140. doi: 10.1016/S0167-8809(99)00022-5
Szabó, Á., Pénzes, B., Sipos, P., Hegyi, T., Hajdú, Z., and Markó, V. (2014). Pest management systems affect composition but not abundance of phytoseiid mites (Acari: Phytoseiidae) in apple orchards. Exp. Appl. Acarol. 62, 525–537. doi: 10.1007/s10493-013-9752-0
Tamošiūnas, R., Valiuškaitė, A., Survilienė, E., Duchovskienė, L., and Rasiukevičiūtė, N. (2014). Variety-specific population density and infestation levels of apple sawfly (Hoplocampa testudinea Klug) in two differently managed apple orchards in Lithuania. Žemdirbystė-Agricul. 101, 205–214. doi: 10.13080/z-a.2014.101.027
Thabet, A. F., Boraei, H. A., Galal, O. A., El-Samahy, M. F. M., Mousa, K. M., Zhang, Y. Z., et al. (2021). Silica nanoparticles as pesticide against insects of different feeding types and their non-target attraction of predators. Sci. Rep. 11:14484. doi: 10.1038/s41598-021-93518-9
Tkaczuk, C., Tipping, C., Majchrowska-Safaryan, A., Krol, A., Wyrzykowska, M., and Wegensteiner, R. (2019). The occurrence of entomopathogenic fungi in soils from apple orchards protected in different farming systems. Fresenius Environ. Bull. 28, 7906–7914.
Trimble, R. M., and Appleby, M. E. (2004). Comparison of efficacy of programs using insecticide and insecticide plus mating disruption for controlling the obliquebanded leafroller in apple (Lepidoptera: Tortricidae). J. Econ. Entomol. 97, 518–524. doi: 10.1093/jee/97.2.518
van Driesche, R. G., Mason, J. L., Wright, S. E., and Prokopy, R. J. (1998). Effect of reduced insecticide and fungicide use on parasitism of leafminers (Phyllonorycter spp.) (lepidoptera: Gracillariidae) in commercial apple orchards. Environ. Entomol. 27, 578–582. doi: 10.1093/ee/27.3.578
Viechtbauer, W. (2010). Conducting meta-analyses in R with the metafor package. J. Stat. Softw. vol. 36, 1–48.
Warabieda, W. (2015). The effect of methyl jasmonate and acibenzolar-S-methyl on the populations of the European red mite (Panonychus ulmi Koch) and Typhlodromus pyri Scheut. In apple orchards, as well as on the yield and growth of apple trees. Int. J. Acarol. 41, 100–107. doi: 10.1080/01647954.2015.1016104
Wearing, C. H., Attfield, B. A., and Colhoun, K. (2010). Biological control of woolly apple aphid, Eriosoma lanigerum (Hausmann), during transition to integrated fruit production for pipfruit in Central Otago, New Zealand. N. Z. J. Crop. Hortic. Sci. 38, 255–273. doi: 10.1080/01140671.2010.524189
Wearing, C. H., Attfield, B., and Colhoun, K. (2011a). Adult phenology and abundance of Froggatt's apple leafhoppper (Edwardsiana froggatti (Baker)) (Hemiptera: Cicadellidae) and its egg parasitoids, Anagrus spp. (Hymenoptera: Mymaridae), under three pest management programmes in Central Otago, New Zealand. New Zealand Entomol. 34, 56–64. doi: 10.1080/00779962.2011.9722210
Wearing, C. H., Colhoun, K., Attfield, B., and Marshall, R. R. (2011b). Diversity of natural enemies in Central Otago, New Zealand apple orchards: a practical measure of sustainability in pest management? Biocontrol Sci. Tech. 21, 1273–1296. doi: 10.1080/09583157.2011.615390
Willer, H., Trávníček, J., Meier, C., and Schlatter, B. (2021). The world of organic agriculture 2021-statistics and emerging trends. Bonn, Germany: Research Institute of Organic Agriculture FiBL, Frick, and IFOAM—Organics International.
Zaller, J. G., Oswald, A., Wildenberg, M., Burtscher-Schaden, H., Nadeem, I., Formayer, H., et al. (2023). Potential to reduce pesticides in intensive apple production through management practices could be challenged by climatic extremes. Sci. Total Environ. 872:162237. doi: 10.1016/j.scitotenv.2023.162237
Keywords: apple, biological control, disease pressure, fruit production, herbivore pests, natural enemies
Citation: Ryalls JMW, Garratt MPD, Spadaro D and Mauchline AL (2024) The benefits of integrated pest management for apple depend on pest type and production metrics. Front. Sustain. Food Syst. 8:1321067. doi: 10.3389/fsufs.2024.1321067
Edited by:
Klaus Birkhofer, Brandenburg University of Technology Cottbus-Senftenberg, GermanyReviewed by:
Pia Addison, Stellenbosch University, South AfricaCarlo Duso, University of Padua, Italy
Copyright © 2024 Ryalls, Garratt, Spadaro and Mauchline. This is an open-access article distributed under the terms of the Creative Commons Attribution License (CC BY). The use, distribution or reproduction in other forums is permitted, provided the original author(s) and the copyright owner(s) are credited and that the original publication in this journal is cited, in accordance with accepted academic practice. No use, distribution or reproduction is permitted which does not comply with these terms.
*Correspondence: James M. W. Ryalls, j.ryalls@reading.ac.uk