- Mingde College, Henan University of Technology, Zhengzhou, China
This study investigates the impact of storage temperature and cold shock treatment (CST) on the postharvest quality and shelf life of guava fruits. Guavas were stored at varying temperatures (4°C, 6°C, 8°C, and 10°C) for 25 days to evaluate the incidence of chilling injury (CI) and determine optimal storage conditions. Results indicated that the best storage temperature was 6°C, which effectively maintained fruit quality by inhibiting CI, controlling weight loss, and preserving soluble solid content and soluble sugars. Further analysis on the effect of CST on guavas showed that longer cold shock durations (6, 9, and 12 h) before storage at a constant 4°C improved the storage quality and shelf life significantly by decreasing the CI rates, reducing weight loss, maintaining firmness, and improving the fruit’s nutritional quality. Notably, a 12-h CST yielded the best outcomes, evidenced by the lowest malondialdehyde (MDA) content and the highest increases in proline and soluble protein content - biomarkers of enhanced cold tolerance and reduced CI. Additionally, CST was found to modulate the reactive oxygen species (ROS) metabolism, increasing the activity levels of key antioxidant enzymes such as superoxide dismutase (SOD), peroxidase (POD), catalase (CAT), and ascorbate peroxidase (APX), alongside higher contents of ascorbic acid. The study identified a correlation between the length of cold shock and improved antioxidant capacity, crucial for extending guava’s shelf life and preserving quality during cold storage. These findings have significant implications for the postharvest management of guava, recommending 6°C storage and 12-h CST as effective strategies for extending shelf life and ensuring quality in cold storage settings.
1 Introduction
Guava (Psidium guajava L.), a significant fruit in tropical and subtropical regions (Rai et al., 2010), offers both refreshing taste and nutritious value, with an abundance of vitamin C, lycopene, dietary fiber, riboflavin, amino acids, among others (Murmu and Mishra, 2018). Notably, studies have also attributed medicinal properties to it, including hypoglycemic, anti-cancer, and immune-regulatory benefits (Sarah et al., 2021). Despite the fruit’s market demand, its thin and delicate skin poses substantial challenges for the guava industry, as it is prone to damage, dehydration, and deterioration, leading to a notably short postharvest shelf life. Addressing these issues through advanced postharvest treatments and preservation methods is thus crucial for enhancing the feasibility of guava industrialization (Jacomino et al., 2001).
So far, the post-harvest storage technology of guava is mainly to improve the shelf life of guava through different processing methods. These include refrigerated preservation, controlled air packaging storage (Singh and Pal, 2007), hot water treatment (Alvindia and Acda, 2015), coated preservation (Kapoor et al., 2023), material packaging and preservation (Yadav et al., 2022), melatonin treatment (Hongbin et al., 2022), ionizing radiation treatment (Singh and Pal, 2008), and other technologies. Among them, the most common treatment method, which is also the lowest cost and most widely used, is low-temperature refrigeration. However, improper low-temperature treatment will have a neglectable effect on the metabolism of reactive oxygen species (ROS) and protein expression of cells, causing cell decomposition, resulting in chilling injury (CI), affecting its quality and shelf life (Martinez et al., 2002). In the process of low temperature storage of fruits and vegetables, if cold damage occurs, cell metabolism will be disordered, resulting in fruit weight loss, stability reduction, and serious loss of nutrients (Zhuang et al., 1997). The decline in fruit and vegetable quality caused by CI is an urgent problem to be solved during low-temperature storage (Wang, 1989). Guava is a cold-sensitive fruit, which is prone to CI, skin browning and depression, resulting in quality decline and loss of commercial value. It is of great significance to study the appropriate storage temperature of guava for fresh-keeping and reducing CI.
Precooling is a critical step in the food cold chain, which can effectively delay the change of fruit quality after harvest (Yan J. et al., 2018). In recent years, cold shock treatment (CST) has been used as a way of precooling (Gu et al., 2020; Si et al., 2023). A physical treatment that puts fruits and vegetables under low temperatures for a short time to improve their stress resistance, which has an obvious anti-ageing effect on fresh-keeping fruits and vegetables (Zhang et al., 2009), enhanced cold resistance (Jia et al., 2023), is an emerging physical fresh-keeping method. It has been shown to effectively extend the shelf life of cucumis (Chen et al., 2015), sweet cherries (Gu et al., 2020), and peppers (Si et al., 2023). However, it is still questionable whether post-harvest pretreatment of freshly picked guava by CST can prolong its shelf life and shelf life.
Low temperature can quickly remove the field heat of fruits and vegetables, which is a crucial factor in the process of cold chain transportation and directly affects the quality and shelf life of fruits (Li et al., 2018; Bernardo and Maria, 2021). In addition, the firmness, total soluble solids (TSS) content and weight loss rate of fruits can directly reflect the freshness of fruits and vegetables during cold storage (Ahmed et al., 2020). Malondialdehyde (MDA) is a product of membrane lipid peroxidation that is harmful to fruit cells and accumulates with the occurrence of fruit cold injury (Feng et al., 2022). The contents of soluble protein and free proline were positively correlated with the cold tolerance of fruit. These quality changes can be used to evaluate the effect of CST on guava quality. In addition, cold injury is associated with cell membrane damage as well as physiological disorders associated with increased generation of ROS (Shadmani et al., 2015), and excessive ROS accumulation can lead to the accumulation of chemicals that affect plant growth and metabolism (Rehman et al., 2021). However, little is known about the effects of CST on postharvest physiological and oxidative properties of guava.
In this study, we aim to establish the optimal conditions for CST, including the ideal storage temperature and CST temperature, and to assess how these factors influence key quality attributes of guava, such as firmness, total soluble solids, and weight loss. Additionally, we investigated the effects of cold-air shock treatment on quality of guava fruit and its metabolism of ROS during storage, explored the feasibility of its method on guava, and analyzed its possible antioxidant mechanism, to provide theoretical guidance for extending the storage and preservation period of guava and commercial circulation, and provide theoretical basis for exploring fresh preservation methods for cold-sensitive fruits.
2 Materials and methods
2.1 Sample preparation
The fruit variety used in this study is known as Pearl Guava, which was collected from a farm in Changtai County, Zhangzhou, Fujian Province. The guava fruits were harvested at the commercial stage of ripeness (80% maturity, green color) and immediately transported to the laboratory through the cold chain at low temperatures. Guava fruits with uniform size and color and intact fruits were selected, and damaged and diseased fruits were discarded.
2.2 Effect of storage temperature on quality of guava
2.2.1 Sample handling
Guava was treated with different methods, including the temperature constant group and temperature fluctuation group. The temperature constant group was divided into four groups, which were stored in the cold storage at 4°C, 6°C, 8°C and 10°C for 25 days. In the temperature fluctuation group, the guava was first placed in cold storage at 6°C, and then the cold storage temperature was adjusted every day. According to the process of 6°C → 4°C → 2°C → 4°C → 6°C → 4°C → 2°C → 4°C, the guava was stored in the cold storage at 4°C for 18 days. The humidity of the cold storage was 85% ± 5%. Each treatment group was subjected to three biological replicates, each replicate contained 100 fruits, which were packed in perforated polyethylene (PE) bags with three fruits per bag. Samples were taken every 5 days for visual quality analyses, and the corresponding physiological and biochemical indexes were measured.
2.2.2 Measurement and methods of indices of fruits
Good fruit rate: The fruit quality was categorized based on the counting method proposed by Zhou et al. (2019) and presented in Table 1, while the calculation of good fruit rate was performed using Formula (1):
Firmness: According to the method of Río Segade et al. (2013). Fruit firmness was measured using a fruit durometer (Model GY-4, Handpi, China) equipped with a Φ11mm probe. The probe penetrated the fruit to a depth of 10 mm at a constant speed, ensuring uniform insertion for accurate firmness readings.
Total soluble solids (TSS): Guava fruits were juiced using a juicer (Model MJ-ZZ12W7–002, Midea, China), and then the TSS content of the extracted juice was measured using a hand-held refractometer (Model PAL-1, Atago, Japan).
Weight loss rate: According to the method of Dong et al. (2021). The weight of guava during different storage periods was measured using an analytical electronic balance (Model HT224R, Vibra, Japan). The weight loss rate of fruit was calculated according to Formula (2):
Respiration rate: According to the method of Wenyan et al. (2022). Each group randomly selected 15 fruits, divided into 3 times into 1 L container, allowing carbon dioxide and oxygen to accumulate in the box at 17°C. After 2 h, gas analyzer was measured using a portable O2/CO2 analyzer (Check point 3, Mocon, Denmark). Calculated according to Formula (3):
Note: m represents sample mass, M represents the relative molecular mass of gas, T represents ambient temperature, N represents CO2 concentration, V represents the volume of the container used for determination, and h represents measurement time.
Titratable acid: According to the method of Deng et al. (2023). The guava was minced and weighed to 10.0 g, ground in a mortar, transferred to a 100 mL volumetric flask and constant volume, and shaken on a rotary shaker (Model OS-20F, Oaic, China) to ensure thorough mixing. The solution was allowed to stand for 30 min and then filtered through filter paper. Titratable acid was determined by titrating 20 mL of the filtrate with 0.01 M NaOH to pH 8.3. The results were represented as percentage of malic acid (g of malic acid per 100 g fresh weight).
Soluble sugar: Determined by anthrone colourimetric method (Niels, 2010). In brief, the samples were boiled twice and filtered after grinding in a mortar, and the filtrate was collected twice and constant in 100 mL volumetric flasks. After dropping anthrone solution, distilled water, and concentrated sulfuric acid, the absorbance of guava was measured in a water bath for another 1.0 min, with distilled water as the control, and repeated three times. The standard curve was drawn to measure the soluble sugar content of guava.
2.3 Effect of CST on quality of guava
2.3.1 Cold shock treatment
Using the cold air cold shock method, fresh guava fruits picked from the farm were placed in 0°C cold storage for 6, 9, and 12 h of air cold shock, and then stored in 4°C cold storage. The control group did not do any treatment and was placed in 4°C cold storage. Each group of 100 guavas, packed in perforated PE bags with three fruits per bag. Samples were taken every 5 days for visual quality analyses and the corresponding physiological, and biochemical indexes were measured.
2.3.2 Measurement and methods of indices of fruits
2.3.2.1 Chilling injury incidence and CI index
The degree of CI in guava was graded according to the method proposed by Lian (2014) as shown in Table 2. The incidence of CI and CI index were calculated according to the following Formulas (4, 5):
2.3.2.2 Physiological and biochemical index
The measurement methods of Firmness, TSS and weight loss rate are the same as those described in section 2.2.2.
Soluble protein content: According to Coomassie brilliant blue staining method (Martins et al., 2022). In brief, the ground guava was centrifuged, and the extract was collected before the Coomassie Brilliant Blue G-250 solution was added. With distilled water as contrast, the use of ultraviolet visible spectrophotometer (Cary 60, Agilent, United States) determines the absorbance of the solution at 595 nm, repeat 3 times. The soluble protein content of guava was calculated from the standard curve.
Malondialdehyde (MDA) content: According to the thiobarbituric acid (TBA) method (Fan et al., 2023). In brief, guava tissue was finely homogenized under ice-cold conditions to minimize enzymatic activity. The homogenate was centrifuged at 10,000 ×g for 15 min at 4°C, and the supernatant was collected for analysis. A volume of 2 mL of 0.6% (w/v) TBA reagent, prepared in 20% trichloroacetic acid (TCA), was mixed with 2 mL of the supernatant in a centrifuge tube. The mixture was incubated in a boiling water bath for 15 min and then rapidly cooled on ice. Clarification was achieved by re-centrifugation at 10,000 ×g for 5 min. The absorbance of the supernatant was recorded at wavelengths of 532 nm, 600 nm (to correct for non-specific turbidity), and 450 nm. The measurements were conducted in triplicate for improved statistical reliability. The MDA concentration in the guava tissue was calculated using the following revised Formula (6):
Proline acid content: According to the method of Wang et al. (2007). Guava samples were homogenized in 3% (w/v) sulfosalicylic acid and centrifuged at 10,000 ×g for 10 min. The supernatant was subjected to a derivatization reaction. To 2 mL of supernatant, we added 2 mL of acid ninhydrin reagent and 2 mL of glacial acetic acid. The mixture underwent a heating step at 100°C for 1 h. Upon cooling to terminate the reaction, 4 mL of toluene was added to the reaction tube and vortexed for 15–20 s for phase separation. Once settled, the chromophore-containing toluene layer, now on top, was carefully collected. The absorbance of this toluene phase was measured at a wavelength of 520 nm against a toluene blank. This measurement too was replicated three times to ensure precision. The proline acid content was derived from a calibration curve plotted with known proline standards.
2.4 Effect of CST on reactive oxygen metabolism in guava
Ascorbic acid content: Determination by potassium iodate titration. Titrate the juice of the guava fruit using a standardized solution of 0.01 M KIO3 until the endpoint is reached, indicated by a persistent color change. Use a freshly prepared starch solution as an indicator. Perform the titration under acidic conditions by adding a small amount of dilute H2SO4 to the juice before titration starts. Calculate the ascorbic acid content using the volume of potassium iodate consumed and its molar concentration.
Superoxide Radica (O2−) production rate: According to the method of Lian (2014). Homogenize the peeled guava fruit and centrifuge at 4°C to obtain the supernatant. Prepare a reaction mixture containing the supernatant, phosphate buffer (pH 7.0), and hydroxylamine hydrochloride. Incubate at 25°C for 60 min. Add a solution of p-aminophenylsulfonic acid and α-naphthylamine to the mixture. Allow the reaction mixture to develop at room temperature in the dark for 20 min. Measure the absorbance at 530 nm against a reagent blank to nullify any background absorbance from the solutions themselves. Calculate the superoxide radical production rate using a standard curve, and perform the assay in triplicate.
Hydrogen peroxide (H2O2) content: According to the method of Lian (2014). Homogenize peeled guava fruit in ice-cold conditions and centrifuge to collect the supernatant. Add titanium tetrachloride-hydrochloric acid reagent to the supernatant, followed by concentrated ammonia to form a precipitate. After 15 min of reaction time, centrifuge and discard the supernatant. Wash the resulting pellet with an acetone solution multiple time. Re-dissolve the pellet in H2SO4 and measure the absorbance at 412 nm. Calculate the H2O2 content from a standard curve, conducting the assay in triplicate.
Superoxide dismutase (SOD) activity: Determination was performed according to the method described by Wisniewski and Dickinson (2003). Assemble a reaction mixture containing sodium phosphate buffer (500 μL, pH 7.8, 50 mmol/L), distilled water (500 μL), methionine (200 μL, 130 mmol/L), nitroblue tetrazolium (NBT, 100 μL, 750 μmol/L), EDTA-Na2 (100 μL, 100 μmol/L), riboflavin (50 μL, 20 μmol/L), and guava tissue extract (0.1 mL). Expose the reaction to UV radiation (60 μmol·m−2·s−1) for 20 min, then measure the absorbance at 560 nm alongside blank controls. Define one unit of SOD activity as the enzyme quantity causing 50% inhibition of NBT photoreduction and express it as units per gram of protein.
Catalase (CAT) activity: Determined according to the method described by Yin et al. (2012). Combine the supernatant (200 mL) with sodium phosphate buffer (0.8 mL, pH 7.5) and freshly prepared H2O2 solution (2 mL, 0.1 mol/L). Measure the change in absorbance at 240 nm every 30 s for a total duration of 3 min. Express CAT activity as a change of 0.01 in absorbance per minute per gram of fresh weight (U g−1 FW).
Ascorbate peroxidase (APX) activity: Determined according to the method described by Pasquariello et al. (2015). Start the reaction by adding the guava tissue extract (0.1 mL) to the reaction buffer (2.6 mL) and initiate the reaction with H2O2 solution (0.3 mL, 2 mmol/L). Monitor the decrease in absorbance at 290 nm and calculate APX activity. Define one unit of APX activity as the amount of enzyme that changes the absorbance by 0.01 units per minute per gram of protein.
Peroxidase (POD) activity: Determined according to the method described by Yan Q. et al. (2018). Prepare a reaction mixture consisting of phosphate buffer (50 mL, 0.05 mol/L, pH 6), guaiacol (28 mL), and H2O2 (19 mL). Add tissue supernatant to the reaction mixture (1 mL of the supernatant and 3 mL of the mixture were combined.) and monitor the increase in absorbance at 470 nm. Express the activity as a change in absorbance per unit time, ensuring the assay is conducted in triplicate for reproducibility.
2.5 Data processing
Data were processed and statistical analyses were conducted utilizing both SPSS 17.0 (SPSS Inc., United States) and Microsoft Excel 2019 (Redmond, USA) to compute the means and standard deviations (SD). All reported results represent the average of 3 replicates. The least significant difference (LSD) test was used to test the significant difference between groups, p < 0.05 threshold was used to indicate that the difference was statistically significant. Graphical representations were generated with Origin software (OriginLab, United States).
3 Results
3.1 Effect of storage temperature on quality of guava
3.1.1 Good fruit rate of guava
The effect of guava stored at varying temperatures over a 25-day period is shown in Figure 1. The good fruit rate can intuitively reflect the storage effect of guava, and the results are shown in Table 3. Guavas stored at 4 and 6°C maintained a 100% good fruit rate for the initial 10 days. After 10 days, the guavas kept at 4°C started to show signs of slight browning and water spots, which got worse. After 15 days, obvious symptoms of CI appeared, and the final good fruit rate was only 40%. At 6°C storage for 15 days, no chilling injuries were recorded, and the good fruit rate reached 73%. At both 8 and 10°C storage temperatures, chilling injuries were absent, but elevated storage temperatures led to disease spots surfacing on the fruit from day five, followed by pedicel detachment. Late storage periods were marked by significant mold, with the situation being more serious at 10 than at 8°C. Fruits stored at 4, 6, 8, and 10°C did not display chilling injury. Under fluctuating temperature storage, the guava cuticle did not show obvious water stains and brown depression but began to appear soft and rotten transparent phenomenon from the inside of the fruit, similar to the symptoms of water heart disease. By 25 days, 70% of guava showed severe CI, varying degrees of soft rot and transparency, suggesting that 6°C presents the optimal storage effect.
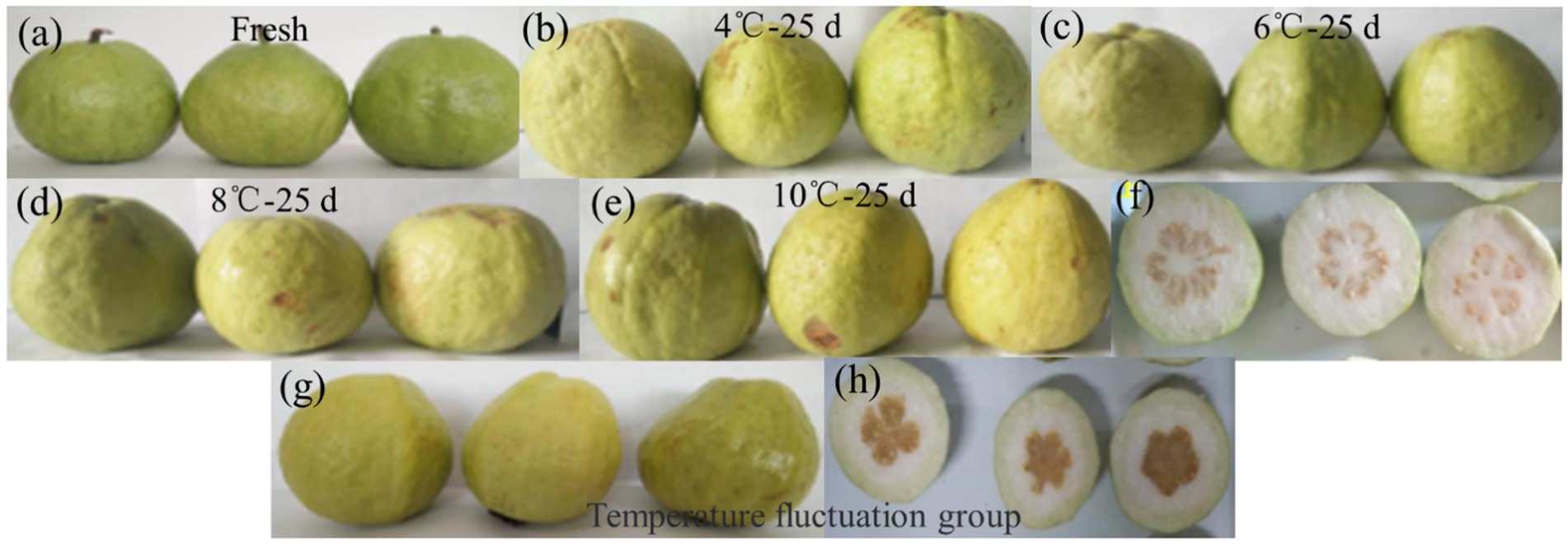
Figure 1. Rendering of guava storage at different temperatures for 25 days. (A) Fresh guava after harvest, stored for 0 day; (B,C) Renderings of guava stored in cold storage at 4°C and 6°C for 25 days, respectively. (B) Guava showed CI and brown depression on its epidermis. (C) Good storage. (D,E) Renderings of guava stored in cold storage at 8°C and 10°C for 25 days, respectively; although no CI occurred, mildew appeared on the surface of the fruit. (F) Section diagram of fruits A–D, without quality deterioration. (G,H) Renderings of the temperature fluctuation group for storage for 25 days. The fruit skin did not appear CI, but the inside appeared soft and transparent symptoms.
3.1.2 Effect of storage temperature on guava quality attributes
Attributes such as firmness, total soluble solids, respiration rate, weight loss rate, soluble sugar and titratable acid are commonly used to evaluate the storage effect and quality of postlarval fruits. The results of the effect of storage temperature on guava storage quality are shown in Figure 2.
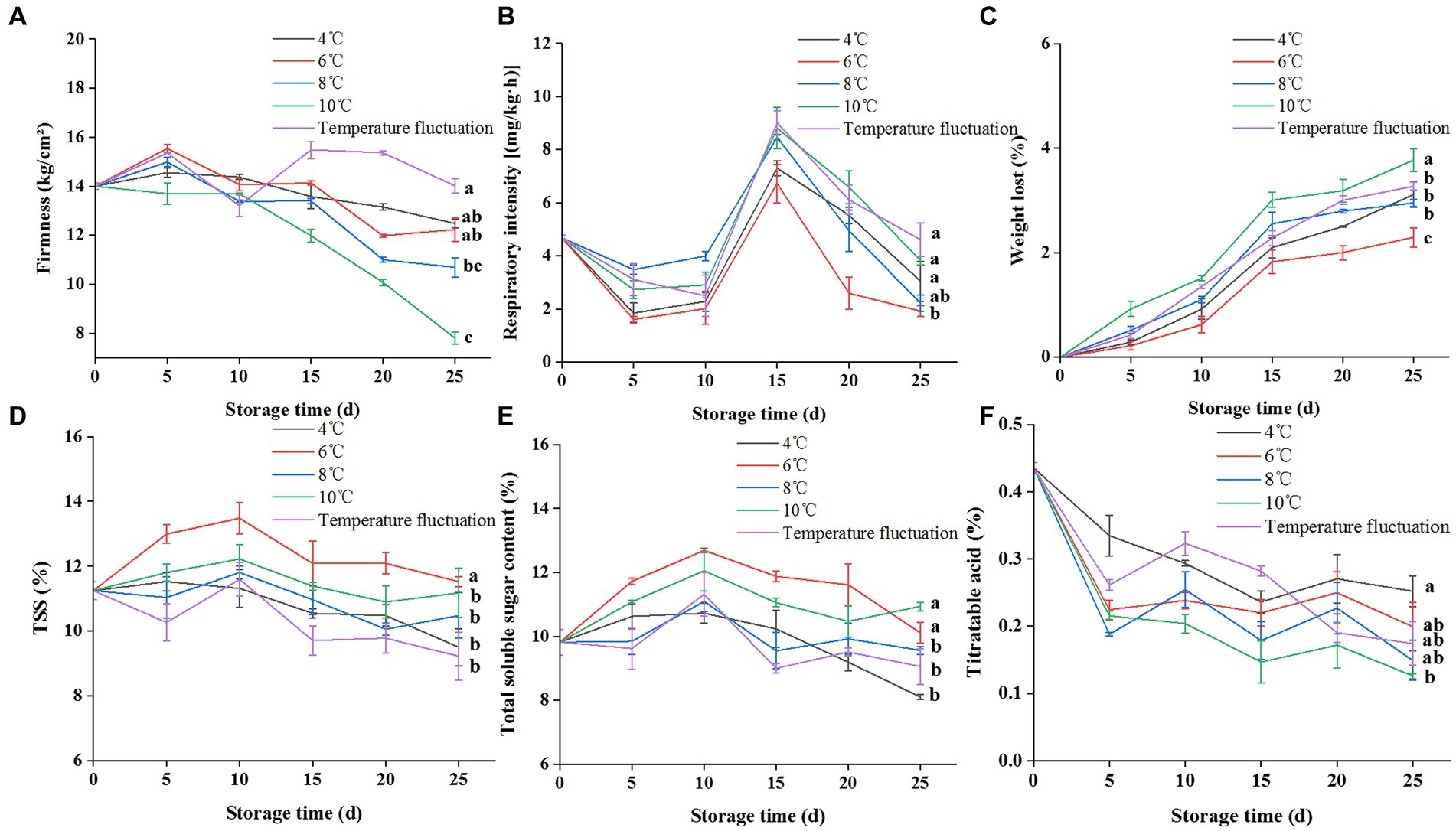
Figure 2. Effect of storage temperature on the storage quality of guava. (A) Effect of storage temperature on the firmness of guava. (B) Effect of storage temperature on the respiration rate of guava. (C) Effect of storage temperature on weight-loss ratio of guava. (D) Effect of storage temperature on total soluble solids of guava. (E) Effect of storage temperature on soluble sugar of guava. (F) Effect of storage temperature on titratable acid content of guava. The alphabets represent the significant difference at each time point (p < 0.05).
Storage temperature notably affected guava’s respiration intensity and soluble sugar content. In this study, the low-temperature storage group (4°C and variable temperature) was able to suppress early-stage weight loss rate and respiration intensity in fruits. Guava stored in 4°C cold storage showed slight CI on the 10th day, and many water spots or brown depressions appeared on the fruit epidermis after the 15th day, indicating serious CI. There was no obvious CI to the epidermis in the temperature fluctuation group during storage, but the inside of the fruit appeared soft and rotten and transparent from the 15th day, which was a more serious CI phenomenon from the inside of the fruit and seriously affected the storage quality. Guava stored at 6°C in cold storage has no CI, and 6°C can well inhibit the decline of soluble solids and soluble sugar, control the weight loss rate and respiration rate, effectively maintain the nutritional quality of the fruit, and the storage effect is good. Although guava stored in 8 and 10°C cold storage did not suffer from CI, the fruit will be precocious due to the high storage temperature.
In conclusion, low temperature refrigeration reduced the respiration rate of guavas fruit, reduced the loss of fruit nutrients, effectively maintained the stability of guavas fruit, reduced the weight loss rate, slowed down the reduction of TSS, soluble sugar, titratable acid, and other respiratory substrates in the fruit, and prolonged the shelf life of the fruit. In addition, we found that 6°C was the best storage temperature, and within the set temperature interval, the fruit showed slight CI at 4°C, which we identified as the critical temperature of CI of guava. Therefore, we speculated that inhibiting the CI degree of guava stored at 4°C would improve its storage quality even more than that of guava stored at 6°C. We next investigated the effect of pretreatment (cold shock) on the inhibition of CI in guava fruits stored at 4°C.
3.2 Effect of CST on postharvest storage CI of guava
3.2.1 Storage of guava after CST for different times
The effect of CST for different times after storage in cold storage at 4°C for 25 days is shown in Figure 3. Compared to without post-CST storage, guavas’ quality post-25 days of storage markedly improved, underscoring that CST effectively enhances guava freshness and extends its shelf life.
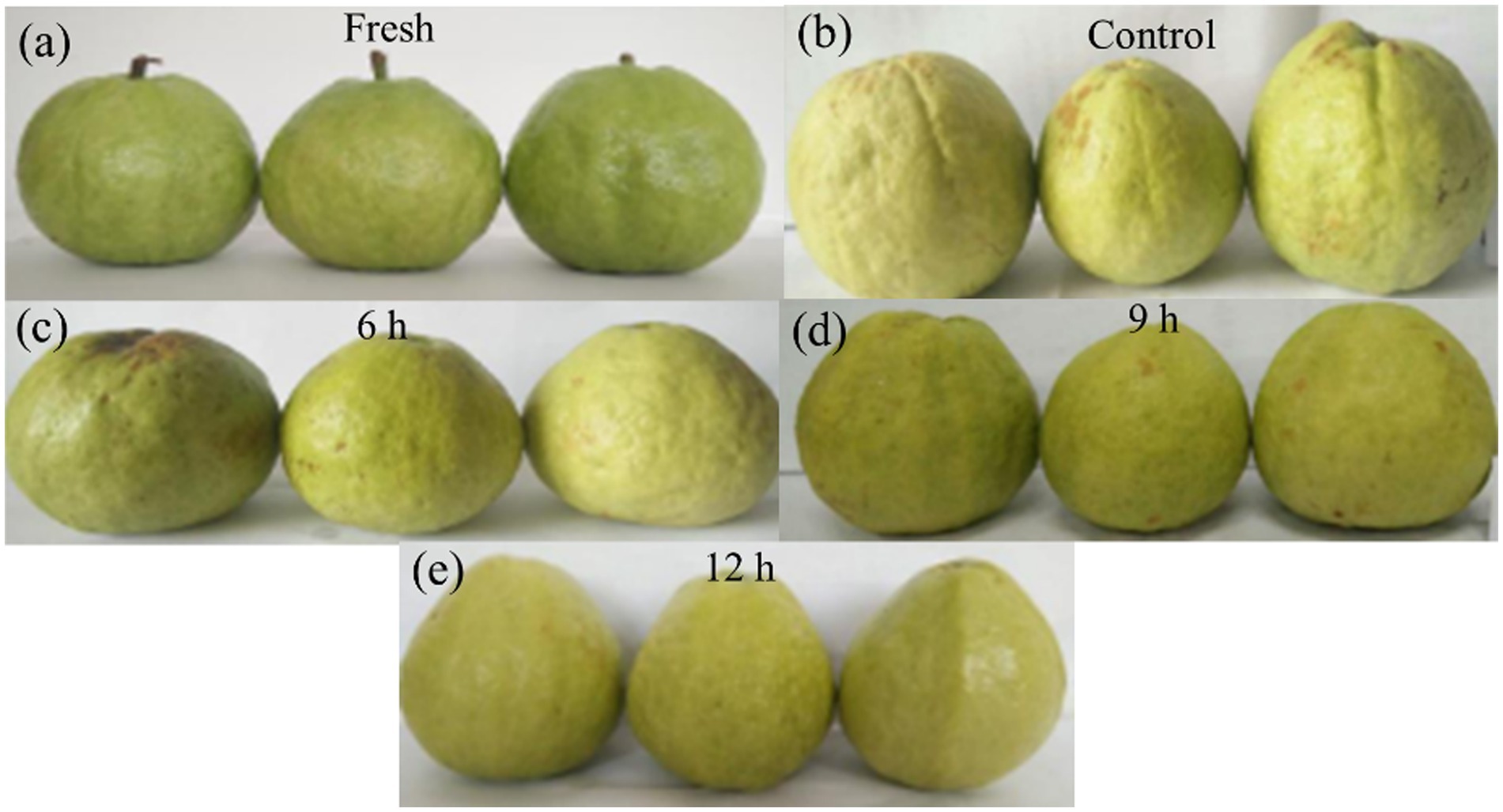
Figure 3. Rendering of the CST after storage at 4°C for 25 days after different times of CST. (A) Fresh guava after harvest, stored for 0 day; (B) Rendering of guava stored in cold storage at 4°C for 25 d without any treatment. (C–E) The rendering of guava after 6 h, 9 h, and 12 h CST for 25 days of storage, respectively.
3.2.2 Effect of CST on CI rate in guava
The area of the guava epidermis with CI is the most intuitive criterion to judge whether the fruit is CI, and the CI index and CI rate are the most intuitive evaluation indexes to judge whether the fruit is CI. The results are shown in Table 4. In the control group, the chilling damage rate reached 88%, and the chilling damage index reached 0.28. A serious CI occurred to the fruit. The CI rates of 12 h, 9 h and 6 h were 72, 56 and 42%, respectively. After CST, the rate of CI was decreased to different degrees. In the range of conditions set by the experiment, the storage effect was better with the increase of cold shock time. These results indicated that CST could inhibit the occurrence of CI of guava in different degrees.
3.2.3 Effect of CST on guava quality
This study applied CST to guavas for differing intervals, analyzing impacts on fruit quality, physiology, and biochemistry as depicted in Figure 4. All CST intervals conferred a degree of chilling injury prevention in the fruit. Within the experimentally established parameters, the protective effects were accentuated as cold shock duration increased.
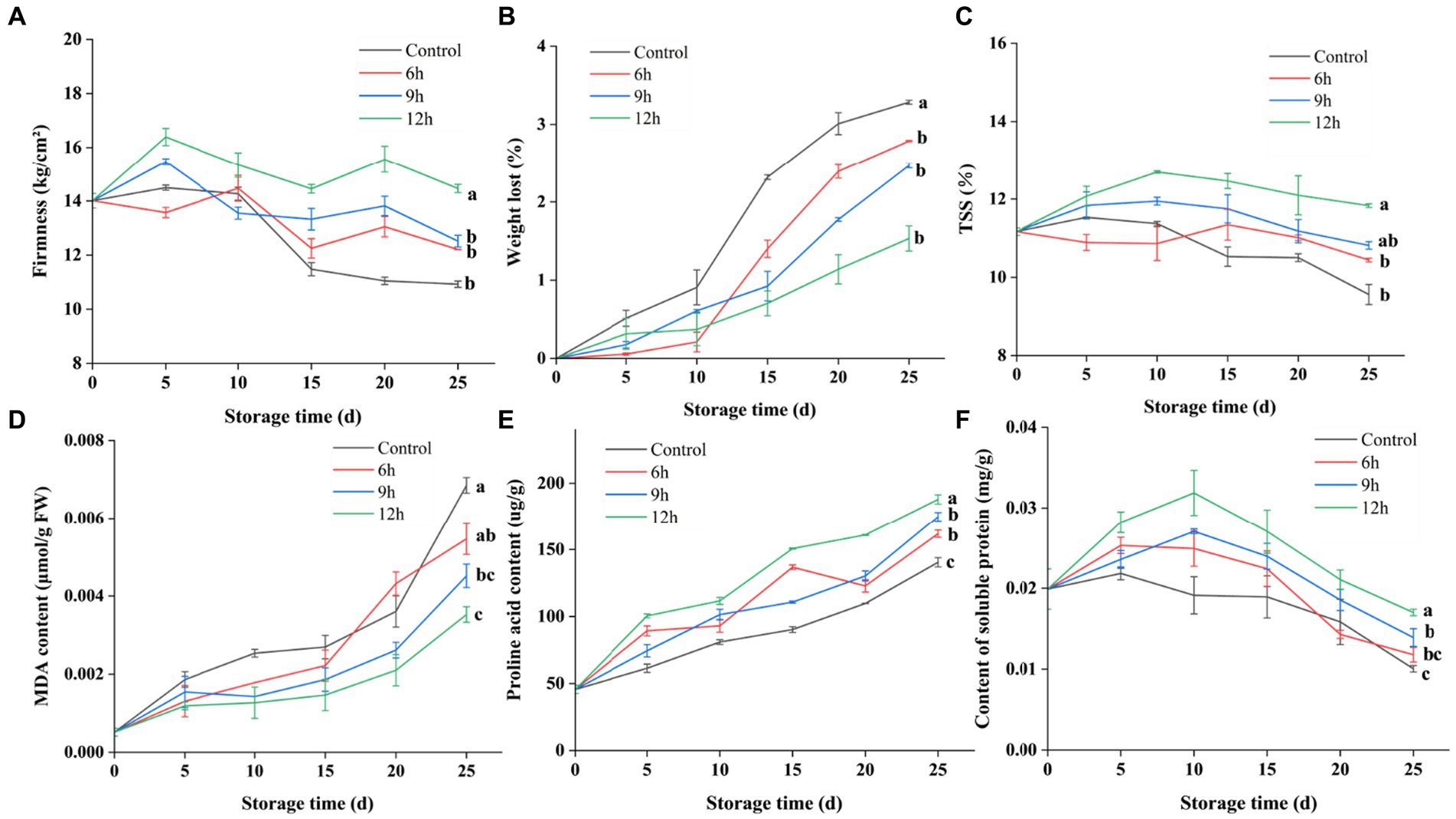
Figure 4. Effect of CST on guava quality. (A) Effect of CST on firmness development of guava during storage. (B) Effect of CST on weight-loss ratio of guava during storage. (C) Effect of CST on total soluble solids matter content of guava during storage. (D) Effect of CST on malondialdehyde content of guava during storage. (E) Effect of CST on proline acid content of guava during storage. (F) Effect of CST on soluble protein content of guava during storage. The alphabets represent the significant difference at each time point (p < 0.05).
CST reliably preserved the storage quality of guava, fruit firmness, total soluble solids content, weight loss rate and other aspects were better than the control group (p < 0.05). Cold shock for 6 h could also reduce the CI rate of fruits, but the nutritional quality and storage quality of fruits were not as good as other cold stress groups. CST could reduce the weight loss rate and malondialdehyde content of the fruit, maintain better firmness, reduce the loss of soluble solids, and increase the content of proline and soluble protein (p < 0.05). Among them, the CST for 9 h and 12 h had better effects, which could better maintain the storage quality of guava after harvest, and the CST for 12 h had the best effect.
Additionally, MDA, a by-product of membrane lipid peroxidation, is detrimental to fruit cells. As chilling injury’s severity increased, cellular MDA levels rose correspondingly. Free proline and soluble protein levels, positively correlated with fruit cold tolerance, were substantially higher in the 9-h and 12-h cold shock groups compared to the control group, while MDA content was significantly reduced. The heightened free proline accumulation correlated with the storage duration and potentially indicated cellular damage. The proteins and proline possibly work in synergy to maintain cell membrane integrity. The cold tolerance in the 9-h and 12-h cold shock groups was shown to be superior.
In conclusion, applying a 12-h CST demonstrated positive effects on guava fruit preservation. It effectively reduced the rate of weight loss, maintain better firmness (p < 0.05), and slow down the decline of TSS (p < 0.05), thus preserving the quality of guava fruit. Furthermore, CST improved the cold tolerance of guava fruit and inhibited the occurrence of chilling injury (CI) through several mechanisms. It was observed that CST inhibited the accumulation rate of malondialdehyde (MDA) (p < 0.05), increased the production rate of proline acid (p < 0.05), and enhanced the content of soluble protein (p < 0.05). These biochemical alterations contributed to the fruit’s enhanced cold resistance and delayed chilling injury manifestation. The efficacy of CST was influenced by both the temperature and duration of the treatment. Longer cold shock durations conferred better fruit storage quality under the experimental conditions set.
Moving forward, future research will concentrate on explicating the relationship between chilling injury in guava and ROS metabolism, alongside other indicators such as antioxidant enzyme activity, providing further insights into CST effects on guava preservation.
3.3 Effect of CST on reactive oxygen species metabolism in guava
The effect of CST on ROS metabolism in guava are shown in Figure 5. The CST group samples exhibited higher ascorbic acid, SOD, POD, CAT, and APX contents than the control group, substantiating CST’s role in bolstering guava’s antioxidant capacity. Throughout cold storage, levels of ascorbic acid, SOD, POD, and APX initially increased, then diminished, while CAT activity consistently declined. The degradation of ascorbic acid could impede the cold adaptability of guava under low-temperature storage. Cold shocks of 9 h and 12 h markedly elevated guava’s ascorbic acid content, with the 12-h group displaying the most significant enhancement. Low-temperature stress triggered the emergence of free radicals such as O2− and H2O2 within the fruit. Cold shocks of 9 h and 12 h notably decreased O2− production rate and H2O2 content. Concurrently, CST elevated activities of SOD and other antioxidative enzymes. Within the range of conditions in this experiment, the enzyme activity became stronger and stronger with the increase of cold shock time. Cold stress for 9 h and 12 h increased the enzyme activities of SOD, CAT, POD and APX, and cold stress for 12 h had the best effect. In conclusion, CST can improve the antioxidant capacity of guava, maintain the dynamic balance of ROS, and reduce the damage of CI to fruit.
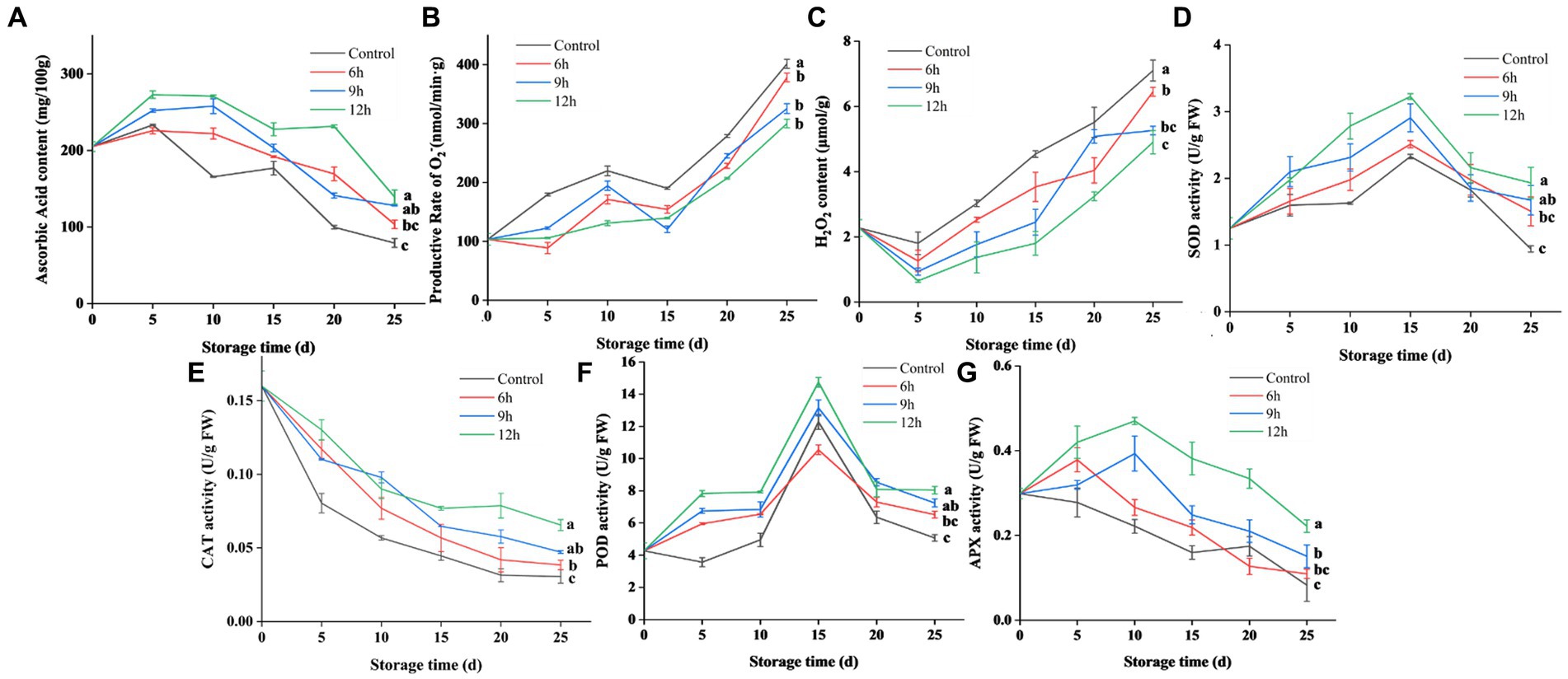
Figure 5. Effect of CST on reactive oxygen species metabolism in guava. (A) Effect of CST on ascorbic acid content of guava during storage. (B) Effect of CST on O2− production rate of guava during storage. (C) Effect of CST on H2O2 content of guava during storage. (D) Effect of CST on SOD activity of guava during storage. (E) Effect of CST on CAT activity of guava during storage. (F) Effect of CST on POD activity of guava during storage. (G) Effect of CST on APX activity of guava during storage. The alphabets represent the significant difference at each time point (p < 0.05).
4 Discussion
4.1 Physiological and biochemical effects of low temperature storage on guava fruit
Fruits and vegetables carry a large amount of field heat after harvest, with strong respiration and vigorous metabolism. Plant respiration will consume substrates, resulting in the loss of water, TSS and soluble sugar (Mendoza et al., 2016). Low temperature treatment can effectively reduce the physiological and biochemical reactions of fruits and vegetables after harvest. In this study, during the cold storage of guava fruits at low temperature, low temperature reduced the transpiration of water in the fruit, reduced the production of ethylene, kept the fruit tissues in a firm, brittle state, reduced the weight loss rate, and inhibited the senescence of the fruit. Moreover, low temperature reduced the activity of enzymes related to respiration in fruits, reduced the intensity of respiration, and reduced the loss of nutrients (TSS, soluble sugar, titratable acid, and other substrates of respiration) in fruits. In addition, low temperature can also inhibit the growth and reproduction of microorganisms, effectively reduce the production of mildew spots, and prolong the shelf life of guava.
4.2 Effects of CST on metabolism and cold resistance in guava
In view of the situation that CI will occur when guava is stored at 4°C, we designed a cold air CST for guava in a cold storage at 0°C, and refrigerated it in a cold storage at 4°C. Cold shock treatment slowed down the metabolic process by affecting the growth and metabolic activity of fruit, inhibited the synthesis and release of membrane lipid degradation product-MDA, and reduced the destruction of cell membrane. Moreover, low temperature will slow down the degradation rate of free proline. Because proline is highly hydrophilic and can stabilize the internal metabolic process, it can reduce the freezing point of protoplasmic colloids and tissues, prevent cell dehydration, and improve the cold resistance of plants. In addition, low temperature stress can regulate the gene expression of soluble protein, increase the content of soluble protein, increase the content of bound water in cells, improve the water retention capacity of cells, and increase the concentration in cytoplasm to reduce the occurrence of freezing. The results confirmed that CST could inhibit the production of MDA, increase the content of proline and soluble protein, increase the cold resistance of guavas fruit, and inhibit the occurrence of CI. Moreover, the CST technique has also been reported in bananas (Zhang et al., 2009), apricots (Yan J. et al., 2018), cherries (Alique et al., 2005), and avocados (Jiao et al., 2017) in could delay softening, reduce CI and maintain its quality in cold storage.
4.3 Effects of CST on ROS metabolism and antioxidant capacity in guava
ROS metabolism is closely related to the occurrence of cold injury in fruits and vegetables at unsuitable low temperature (Youxia et al., 2022). Low temperature causes cell membrane damage in cold-sensitive fruits through ROS accumulation (Vega-Alvarez et al., 2020). H2O2 and O2− free radicals can cause oxidative damage, stimulate senescence, and lead to fruit deterioration (Jiménez-Muñoz et al., 2021). In the present study (Figures 5B,C), H2O2 and O2− were increased in both control and CST groups, but the content of CST was lower than that of control, indicating that CST can inhibit the accumulation of H2O2 and O2− to alleviate CI.
The antioxidant system composed of SOD, POD, CAT, APX and other protective enzymes plays a vital role in the resistance of fruits to CI (Brennan and Frenkel, 1977). These enzymes cooperate with each other to form an antioxidant system, and then cooperates with non-enzymatic antioxidants such as ascorbic acid to remove excess reactive oxygen radicals in the body and avoid cell damage (Olga et al., 2003). In this study, the increase of ascorbic acid content and activities of SOD, POD and APX were related to low temperature stress, and the decrease of CAT content and activities of antioxidant enzymes was related to the destruction of enzyme proteins by low temperature (Feng et al., 2022). Low temperature cold shock treatment may increase ascorbic acid content by affecting the expression of proteins related to ascorbic acid metabolism pathway (Hui et al., 2018). Alternatively, cold shock may also increase the expression of antioxidant related genes and enhance the accumulation of antioxidant enzymes while inhibiting their reduction through low temperature stress. Low temperature stress, ascorbic acid, and antioxidant enzymes work together to inhibit the production rate of O2− and the content of H2O2 in guava fruit (Brennan and Frenkel, 1977), so free radicals are maintained at a low level, and thus the antioxidant capacity of the fruit is maintained and improved. Consistent with the findings, the enhanced antioxidant capacity of CST has also been found in green pepper, papaya, and avocados (Vincent et al., 2020; Feng et al., 2022; Nian et al., 2022). Our results confirmed that CST could reduce oxidative stress in fruits, prevent chilling injury, mitigate losses, reduce fruit senescence, and maintain better fruit quality by increasing the activity of antioxidant enzymes.
The preservation of guava freshness is crucial to ensure its quality and extend its shelf life. Cold storage techniques play a significant role in achieving these goals. We compared various CST methods applied to guava freshness preservation, including ice-water CST and cold air CST, as well as other non-cold storage techniques. Ice water CST is a commonly used technique in the industry (Nian et al., 2022). An advantage is its ability to quickly cool fruits and reduce their respiration rate and metabolic activity. However, this method requires a constant supply of ice and water, making large-scale applications less economical. Additionally, controlling and maintaining the required temperature can be challenging, which may result in potential temperature fluctuations that could affect fruit quality. On the other hand, the cold air CST applied in this study has several advantages over ice water CST (Yan J. et al., 2018). It involves circulating cold air around guava fruits to maintain low temperatures. Cold air CST is easier to control and operate, making it more suitable for large-scale promotion and application. It also provides a more uniform temperature distribution, minimizing temperature fluctuations and ensuring consistent fruit quality. Moreover, cold air CST is more cost-effective because it does not require a constant supply of ice and water.
Among non-cold storage techniques, antioxidant treatment is commonly used (Hongbin et al., 2022). This method involves using antioxidants such as ascorbic acid, polyphenolic compounds, melatonin, and natural extracts to inhibit oxidative reactions in guava fruits, reduce oxidative damage, and extend their shelf life. However, further studies are needed to determine the optimal concentration and treatment duration for effective preservation. Packaging technologies are another approach (Yadav et al., 2022), including film packaging, modified atmosphere packaging, and nano-packaging, among others. These techniques effectively slow the respiration rate of guava fruit, reduce water loss, and limit oxygen entry, thereby prolonging the fruit’s shelf life. However, the applicability and effect of different packaging technologies on guava fruit may be different, and the cost is high. Hormonal treatment is also an effective method, involving the use of hormones such as ethylene and gibberellin. These hormones can regulate the physiological activity of guava fruit, improving fruit quality, and enhancing resistance their cold resistance. However, strict control of hormone dosage and treatment time is necessary during the hormone treatment process to prevent hormone residue, which may pose potential risks to human health. The ability of ionizing radiation treatment to inhibit microbial growth and extend the shelf life of fruits has also been studied (Singh and Pal, 2008). However, concerns about the safety and public acceptance of this technology have limited its widespread application.
In conclusion, while various CST and non-cold storage techniques have been explored for guava freshness preservation, cold air CST demonstrates advantages in terms of control, operation, and cost-effectiveness. It provides a viable option for large-scale application in the industry. However, further research is needed to better understand the mechanisms underlying guava fruit’s chilling injury and explore genetic approaches to enhance its resistance to CI and prolong shelf life. Continued efforts in this field will contribute to the development of improved preservation techniques for guava and other fruits.
5 Conclusion
This study has demonstrated that appropriate storage temperature and CST are critical in preserving the postharvest quality of guava. It was found that maintaining guavas at a storage temperature of 6°C is the most favorable condition, effectively preventing CI while conserving the fruit’s nutritional attributes. Moreover, implementing a 12-h CST emerged as a particularly efficient approach for significantly reducing CI, sustaining the fruit’s nutritive value, and bolstering the antioxidant defenses which, in turn, decelerate the onset of CI. The synergy of CST and meticulous temperature regulation shows promise in extending the shelf life and quality of guava, pointing towards a need for further research to understand the biochemical and molecular mechanisms at play. The findings of this study provide valuable support for the development and deployment of cold shock technology, which could significantly enhance the storage quality, shelf life, and economic value of guava, offering a referential framework for the cold chain transportation of other cold-sensitive fruits.
Data availability statement
The original contributions presented in the study are included in the article/supplementary material, further inquiries can be directed to the corresponding author.
Author contributions
YZ: Conceptualization, Data curation, Formal analysis, Investigation, Methodology, Project administration, Resources, Software, Supervision, Validation, Visualization, Writing – original draft, Writing – review & editing.
Funding
The author(s) declare that no financial support was received for the research, authorship, and/or publication of this article.
Acknowledgments
I would like to thank the lab staff who helped me manage the experimental equipment.
Conflict of interest
The author declares that the research was conducted in the absence of any commercial or financial relationships that could be construed as a potential conflict of interest.
Publisher’s note
All claims expressed in this article are solely those of the authors and do not necessarily represent those of their affiliated organizations, or those of the publisher, the editors and the reviewers. Any product that may be evaluated in this article, or claim that may be made by its manufacturer, is not guaranteed or endorsed by the publisher.
References
Ahmed, A., Ali, S. W., Imran, A., Afzaal, M., Arshad, M. S., Nadeem, M., et al. (2020). Formulation of date pit oil-based edible wax coating for extending the storage stability of guava fruit. J. Food Proc. Preserv. 44:e14336. doi: 10.1111/jfpp.14336
Alique, R., Zamorano, J. P., Martínez, M. A., and Alonso, J. (2005). Effect of heat and cold treatments on respiratory metabolism and shelf-life of sweet cherry, type picota cv “Ambrunés”. Postharvest Biol. Technol. 35, 153–165. doi: 10.1016/j.postharvbio.2004.07.003
Alvindia, D. G., and Acda, M. A. (2015). Revisiting the efficacy of hot water treatment in managing anthracnose and stem-end rot diseases of mango cv. ‘Carabao’. Crop Prot. 67, 96–101. doi: 10.1016/j.cropro.2014.09.016
Bernardo, P., and Maria, C. (2021). Innovative preservation technology for the fresh fruit and vegetables. Foods 10:719. doi: 10.3390/foods10040719
Brennan, T., and Frenkel, C. (1977). Involvement of hydrogen peroxide in the regulation of senescence in pear. Plant Physiol. 59, 411–416. doi: 10.1104/pp.59.3.411
Chen, A., Yang, Z., Zhang, N., Zhao, S., and Chen, M. (2015). Effects of cold shock intensity on physiological activity of harvested cucumbers during storage. Sci. Hortic. 197, 420–427. doi: 10.1016/j.scienta.2015.09.056
Deng, H., Xuelian, L., Yang, W., Qiaoli, M., Yuge, Z., Yinchun, X., et al. (2023). Organic acid accumulation and associated dynamic changes in enzyme activity and gene expression during fruit development and ripening of common loquat and its interspecific hybrid. Foods 12:911. doi: 10.3390/foods12050911
Dong, Z., Li, R., and Gong, Y. (2021). Antibacterial and freshness-preserving mechanisms of chitosan-Nano-TiO2-Nano-ag composite materials. Coatings 11:914. doi: 10.3390/coatings11080914
Fan, W., Chen, S., Zhou, X., Zheng, Y., Zuo, J., and Wang, Q. (2023). The changes of postharvest quality and antioxidant enzyme activity of different fresh waxy corn. Sci. Technol. Food Ind. 45, 307–315. doi: 10.13386/j.issn1002-0306.2023030261
Feng, W., Qingzhen, Y., Qifeng, Z., and Xin, L. (2022). Cold shock treatment with oxalic acid could alleviate chilling injury in green bell pepper by enhancing antioxidant enzyme activity and regulating proline metabolism. Sci. Hortic. 295:110783. doi: 10.1016/j.scienta.2021.110783
Gu, S., Xu, D., Zhou, F., Gao, H., Hu, W., Gao, X., et al. (2020). Cold shock treatment maintains quality and induces relative expression of cold shock domain protein (CSDPs) in postharvest sweet cherry. Sci. Hortic. 262, –109058. doi: 10.1016/j.scienta.2019.109058
Hongbin, C., Hetong, L., Xuanjing, J., Mengshi, L., and Zhongqi, F. (2022). Amelioration of chilling injury and enhancement of quality maintenance in cold-stored guava fruit by melatonin treatment. Food Chem. X 14:100297. doi: 10.1016/j.fochx.2022.100297
Hui, L., Zhi-Wei, L., Zhi-Jun, W., Yong-Xin, W., Rui-Min, T., and Jing, Z. (2018). Differentially expressed protein and gene analysis revealed the effects of temperature on changes in ascorbic acid metabolism in harvested tea leaves. Hortic. Res. 5:65. doi: 10.1038/s41438-018-0070-x
Jacomino, A. P., de Luca Sarantopoulos, C. I. G., Sigrist, J. M. M., Kluge, R. A., and Minami, K. (2001). Sensorial characteristics of “Kumagai” guavas submitted to passive modified atmosphere in plastic packages. J. Plastic Film Sheet. 17, 6–21. doi: 10.1106/R06Q-QRR9-BMPJ-6Y9Y
Jia, Z., Bao, Y., Zhao, Y., Liu, Y., Zheng, Y., Feng, Z., et al. (2023). Cold shock treatment enhances cold tolerance in peach fruit through modulating PpbZIP9 and PpVIP1-mediated respiratory metabolism. Postharvest Biol. Technol. 204:112421. doi: 10.1016/j.postharvbio.2023.112421
Jiao, C., Xixia, L., Fenfang, L., Yixing, L., and Debao, Y. (2017). Cold shock treatment extends shelf life of naturally ripened or ethylene-ripened avocado fruits. PLoS One 12:e0189991. doi: 10.1371/journal.pone.0189991
Jiménez-Muñoz, R., Palma, F., Carvajal, F., Castro-Cegrí, A., Pulido, A., Jamilena, M., et al. (2021). Pre-storage nitric oxide treatment enhances chilling tolerance of zucchini fruit (Cucurbita pepo L.) by S -nitrosylation of proteins and modulation of the antioxidant response. Postharvest Biol. Technol. 171:111345. doi: 10.1016/j.postharvbio.2020.111345
Kapoor, S., Gandhi, N., Kaur, G., Khatkar, S. K., Bala, M., Nikhanj, P., et al. (2023). Electrospray application of guava seed oil for shelf life extension of guava fruit. Int. J. Food Sci. Technol. 58, 2669–2678. doi: 10.1111/ijfs.15833
Li, X., Yang, H., and Lu, G. (2018). Low-temperature conditioning combined with cold storage inducing rapid sweetening of sweetpotato tuberous roots (Ipomoea batatas (L.) lam) while inhibiting chilling injury. Postharvest Biol. Technol. 142, 1–9. doi: 10.1016/j.postharvbio.2018.04.002
Lian, L. (2014). Study on Optimum Storage Conditions and Chilling Injury Mechanism of Pitaya. Fuzhou City, Fujian Province, China: Fujian Agriculture and Forestry University.
Martinez, J., Perez-Serrano, J., Bernadina, W., and Rodriguez-Caabeiro, F. (2002). Oxidative and cold shock cause enhanced induction of a 50 kDa stress protein in Trichinella spiralis. Parasitol. Res. 88, 427–430. doi: 10.1007/s00436-002-0608-5
Martins, S. M., Keven, S. E., Srujana, M., Meireles, M. A., and Saldaña, M. D. (2022). Pulsed high-pressure processing of barley-based non-dairy alternative milk: β-carotene retention, protein solubility and antioxidant activity. Innov. Food Sci. Emerg. Technol. 82:103212. doi: 10.1016/j.ifset.2022.103212
Mendoza, R., Castellanos, D. A., García, J. C., Vargas, J. C., and Herrera, A. O. (2016). Ethylene production, respiration and gas exchange modelling in modified atmosphere packaging for banana fruits. Int. J. Food Sci. Technol. 51, 777–788. doi: 10.1111/ijfs.13037
Murmu, S. B., and Mishra, H. N. (2018). Selection of the best active modified atmosphere packaging with ethylene and moisture scavengers to maintain quality of guava during low-temperature storage. Food Chem. 253, 55–62. doi: 10.1016/j.foodchem.2018.01.134
Nian, Y., Wang, N., Li, R., Shao, Y., and Li, W. (2022). Cold shock treatment alleviates chilling injury in papaya fruit during storage by improving antioxidant capacity and related gene expression. Sci. Hortic. 294:110784. doi: 10.1016/j.scienta.2021.110784
Niels, M. (2010). Extraction and analysis of soluble carbohydrates. Methods Mol. Biol. 639, 341–370. doi: 10.1007/978-1-60761-702-0_22
Olga, B., Eija, V., and Fagerstedt, K. V. (2003). Antioxidants, oxidative damage and oxygen deprivation stress: a review. Ann. Bot. 91, 179–194. doi: 10.1093/aob/mcf118
Pasquariello, M. S., Patre, D. D., Mastrobuoni, F., Zampella, L., Scortichini, M., and Petriccione, M. (2015). Influence of postharvest chitosan treatment on enzymatic browning and antioxidant enzyme activity in sweet cherry fruit. Postharvest Biol. Technol. 109, 45–56. doi: 10.1016/j.postharvbio.2015.06.007
Rai, M. K., Asthana, P., Jaiswal, V. S., and Jaiswal, U. (2010). Biotechnological advances in guava (Psidium guajava L.): recent developments and prospects for further research. Trees 24, 1–12. doi: 10.1007/s00468-009-0384-2
Rehman, R. N., Malik, A. U., Khan, A. S., Ul Hasan, M., Anwar, R., Ali, S., et al. (2021). Combined application of hot water treatment and methyl salicylate mitigates chilling injury in sweet pepper (Capsicum annuum L.) fruits. Sci. Hortic. 283:110113. doi: 10.1016/j.scienta.2021.110113
Río Segade, S., Giacosa, S., Torchio, F., de Palma, L., Novello, V., Gerbi, V., et al. (2013). Impact of different advanced ripening stages on berry texture properties of ‘red globe’ and ‘crimson seedless’ table grape cultivars (Vitis vinifera L.). Sci. Hortic. 160, 313–319. doi: 10.1016/j.scienta.2013.06.017
Sarah, J. E. W. C., Niranjan, D., Piyali, B., and Anupam, B. (2021). Guava (Psidium guajava L.): a glorious plant with cancer preventive and therapeutic potential. Crit. Rev. Food Sci. Nutr. 63, 31–32. doi: 10.1080/10408398.2021.1945531
Shadmani, N., Ahmad, S. H., Saari, N., Ding, P., and Tajidin, N. E. (2015). Chilling injury incidence and antioxidant enzyme activities of Carica papaya L. ‘Frangi’ as influenced by postharvest hot water treatment and storage temperature. Postharvest Biol. Technol. 99, 114–119. doi: 10.1016/j.postharvbio.2014.08.004
Si, M., Tong, L., Quanying, S., Wenxuan, Z., and Xianghong, W. (2023). Cold shock precooling improves the firmness of chili pepper during postharvest storage and the molecular mechanisms related to pectin. Food Chem. 419, –136052. doi: 10.1016/j.foodchem.2023.136052
Singh, S. P., and Pal, R. K. (2007). Controlled atmosphere storage of guava (Psidium guajava L.) fruit. Postharvest Biol. Technol. 47, 296–306. doi: 10.1016/j.postharvbio.2007.08.009
Singh, S. P., and Pal, R. K. (2008). Ionizing radiation treatment to improve postharvest life and maintain quality of fresh guava fruit. Radiat. Phys. Chem. 78, 135–140. doi: 10.1016/j.radphyschem.2008.09.007
Vega-Alvarez, M., Salazar-Salas, N. Y., López-Angulo, G., Pineda-Hidalgo, K. V., López-López, M. E., Vega-García, M. O., et al. (2020). Metabolomic changes in mango fruit Peel associated with chilling injury tolerance induced by quarantine hot water treatment. Postharvest Biol. Technol. 169:111299. doi: 10.1016/j.postharvbio.2020.111299
Vincent, C., Mesa, T., and Munné-Bosch, S. (2020). Hormonal interplay in the regulation of fruit ripening and cold acclimation in avocados. J. Plant Physiol. 251:153225. doi: 10.1016/j.jplph.2020.153225
Wang, C. Y. (1989). Chilling injury of fruits and vegetables. Food Rev. Intl. 5, 209–236. doi: 10.1080/87559128909540850
Wang, S.-X., Xia, S.-T., Peng, K.-Q., Kuang, F.-C., Cao, Y., and Xiao, L.-T. (2007). Effects of formulated fertilizer synergist on abscisic acid accumulation, proline content and photosynthetic characteristics of Rice under drought. Rice Sci. 14, 42–48. doi: 10.1016/S1672-6308(07)60007-9
Wenyan, L., Yaping, L., Jianbing, D., Gang, R., Wei, W., Weichun, H., et al. (2022). Effects of 1-MCP treatment on physiology and storage quality of root mustard at ambient temperature. Foods 11:2978. doi: 10.3390/foods11192978
Wisniewski, L., and Dickinson, N. M. (2003). Toxicity of copper to Quercus robur (English oak) seedlings from a copper-rich soil. Environ. Exp. Bot. 50, 99–107. doi: 10.1016/S0098-8472(03)00005-4
Yadav, A., Kumar, N., Upadhyay, A., Fawole, O. A., Mahawar, M. K., Jalgaonkar, K., et al. (2022). Recent advances in novel packaging Technologies for Shelf-Life Extension of guava fruits for retaining health benefits for longer duration. Plan. Theory 11:547. doi: 10.3390/plants11040547
Yan, Q., Jianhui, B., Yuqing, W., Jinghui, L., Yongning, H., Zhi, D., et al. (2018). Comparative effects of salt and alkali stress on photosynthesis and root physiology of oat at anthesis. Arch. Biol. Sci. 70, 329–338. doi: 10.2298/ABS171124050Q
Yan, J., Song, Y., Li, J., and Jiang, W. (2018). Forced-air precooling treatment enhanced antioxidant capacities of apricots. J. Food Proc. Preserv. 42, –e13320. doi: 10.1111/jfpp.13320
Yin, J., Bai, S., Wu, F., Lu, G., and Yang, H. (2012). Effect of nitric oxide on the activity of phenylalanine ammonia-lyase and antioxidative response in sweetpotato root in relation to wound-healing. Postharvest Biol. Technol. 74, 125–131. doi: 10.1016/j.postharvbio.2012.06.011
Youxia, S., Dandan, Z., Zisheng, L., Taotao, L., Hongxia, Q., Xuewu, D., et al. (2022). Advances in chilling injury of postharvest fruit and vegetable: extracellular ATP aspects. Compr. Rev. Food Sci. Food Saf. 21, 4251–4273. doi: 10.1111/1541-4337.13003
Zhang, H., Yang, S., Joyce, D. C., Jiang, Y., Qu, H., and Duan, X. (2009). Physiology and quality response of harvested banana fruit to cold shock. Postharvest Biol. Technol. 55, 154–159. doi: 10.1016/j.postharvbio.2009.11.006
Zhou, X., Tan, J., Gou, Y., Liao, Y., Xu, F., Li, G., et al. (2019). The biocontrol of postharvest decay of table grape by the application of kombucha during cold storage. Sci. Hortic. 253, 134–139. doi: 10.1016/j.scienta.2019.04.025
Keywords: guava, chilling injury, cold shock treatment, postharvest storage, antioxidant enzyme activity
Citation: Zhang Y (2024) Post-harvest cold shock treatment enhanced antioxidant capacity to reduce chilling injury and improves the shelf life of guava (Psidium guajava L.). Front. Sustain. Food Syst. 8:1297056. doi: 10.3389/fsufs.2024.1297056
Edited by:
Pankaj B. Pathare, Sultan Qaboos University, OmanReviewed by:
Zinash A. Belay, Agricultural Research Council of South Africa (ARC-SA), South AfricaJayeeta Mitra, Indian Institute of Technology Kharagpur, India
Ruchika Zalpouri, Central Institute of Post-Harvest Engineering and Technology (ICAR), India
Copyright © 2024 Zhang. This is an open-access article distributed under the terms of the Creative Commons Attribution License (CC BY). The use, distribution or reproduction in other forums is permitted, provided the original author(s) and the copyright owner(s) are credited and that the original publication in this journal is cited, in accordance with accepted academic practice. No use, distribution or reproduction is permitted which does not comply with these terms.
*Correspondence: Yan Zhang, emhhbmd5YW4xMDE2QGhhdXQuZWR1LmNu