- 1ICAR-Central Soil Salinity Research Institute (CSSRI), Karnal, India
- 2CCS Haryana Agricultural University (CCS HAU), Hisar, India
- 3ICAR-Indian Institute of Maize Research (IIMR), Ludhiana, India
- 4ICAR-Central Institute for Research on Buffaloes, Sub Campus, Nabha, Punjab, India
- 5ICAR-Central Arid Zone Research Institute, Regional Research Station, Bikaner, Rajasthan, India
- 6International Maize and Wheat Improvement Center (CIMMYT), Dhaka, Bangladesh
- 7International Maize and Wheat Improvement Center (CIMMYT), SARO, Kathmandu, Nepal
- 8International Crops Research Institute for Semi-Arid Tropics (ICRISAT), Patancheru, India
The future of South Asia’s major production system (rice–wheat rotation) is at stake due to continuously aggravating pressure on groundwater aquifers and other natural resources which will further intensify with climate change. Traditional practices, conventional tillage (CT) residue burning, and indiscriminate use of groundwater with flood irrigation are the major drivers of the non-sustainability of rice–wheat (RW) system in northwest (NW) India. For designing sustainable practices in intensive cereal systems, we conducted a study on bundled practices (zero tillage, residue mulch, precise irrigation, and mung bean integration) based on multi-indicator (system productivity, profitability, and efficiency of water, nitrogen, and energy) analysis in RW system. The study showed that bundling conservation agriculture (CA) practices with subsurface drip irrigation (SDI) saved ~70 and 45% (3-year mean) of irrigation water in rice and wheat, respectively, compared to farmers’ practice/CT practice (pooled data of Sc1 and Sc2; 1,035 and 318 mm ha−1). On a 3-year system basis, CA with SDI scenarios (mean of Sc5–Sc8) saved 35.4% irrigation water under RW systems compared to their respective CA with flood irrigation (FI) scenarios (mean of Sc3 and Sc4) during the investigation irrespective of residue management. CA with FI system increased the water productivity (WPi) and its use efficiency (WUE) by ~52 and 12.3% (3-year mean), whereas SDI improved by 221.2 and 39.2% compared to farmers practice (Sc1; 0.69 kg grain m−3 and 21.39 kg grain ha−1 cm−1), respectively. Based on the 3-year mean, CA with SDI (mean of Sc5–Sc8) recorded −2.5% rice yield, whereas wheat yield was +25% compared to farmers practice (Sc1; 5.44 and 3.79 Mg ha−1) and rice and wheat yield under CA with flood irrigation were increased by +7 and + 11%, compared to their respective CT practices. Mung bean integration in Sc7 and Sc8 contributed to ~26% in crop productivity and profitability compared to farmers’ practice (Sc1) as SDI facilitated advancing the sowing time by 1 week. On a system basis, CA with SDI improved energy use efficiency (EUE) by ~70% and partial factor productivity of N by 18.4% compared to CT practices. In the RW system of NW India, CA with SDI for precise water and N management proved to be a profitable solution to address the problems of groundwater, residue burning, sustainable intensification, and input (water and energy) use with the potential for replication in large areas in NW India.
1 Introduction
In South Asia, a widespread cereal-based agricultural system known as rice–wheat (RW) ensures the survival of billions of people (Nawaz et al., 2019; Bhatt et al., 2021). At 13.5 million hectares in size, the Indo-Gangetic plains (IGPs) represent over 85% of the territory covered by the RW system (Saharawat et al., 2012; Bhatt et al., 2020). In India, 76% of the total area is land, and 50% of it is in the northwest (NW) region. The output of wheat and rice was at its peak in 2020–21, reaching 122.3 and 109.5 million tons (MT), respectively (PIB, 2021). In India, rice and wheat are widely distributed to guarantee the nation’s right to food security. Nonetheless, the nation has also been spending a sizable amount on the importation of pulses, oilseeds, and other nutrient-dense food products. According to the anticipated climate alteration scenario, the demand for irrigation in the future will emerge by 10% for every °C that the air temperature rises, but the availability of irrigation will primarily downturn where it is most needed, particularly in northwest India (Fischer et al., 2007; Singh et al., 2014). In India, the agricultural sector utilizes over 80% of all fresh water, which has a direct impact on the pattern of precipitation (Teri Report, 2022). More than 450 million children worldwide risk high to extremely high-water vulnerability because of rapid population growth, urbanization, climate change, and extreme weather events, according to a UNICEF Report (2021). According to Mukherjee et al. (2015), India consumes more groundwater than any other country in the world, making up over a quarter of global groundwater use.
The long-term sustainability and resilience of India’s intensive RW systems have also been challenged and strongly debated in the policy and scientific forums (Kakraliya et al., 2018; Jat et al., 2018a; Bhatt et al., 2021). The necessity to produce more food and feed from a smallholder system is always a focus in this specialized field. Natural resource sustainability in this area has grown to be a major problem over time for system efficiency and farmer profits. RW cropping system’s long-term viability in the face of current obstacles such as repeated tillage, free electricity, crop residue burning, water table depletion (Rodell et al., 2009; Humphreys et al., 2010), soil degradation and multiple nutrient deterioration (Bhandari et al., 2002; Tripathi and Das, 2017), labor scarcity, pollution (Singh et al., 2008), stagnant yields (Ladha et al., 2003a), and higher cultivation costs (Choudhary et al., 2018; Jat et al., 2019c; Kumar et al., 2021) is a daunting task.
The decline in the groundwater table in NW India between 1973 and 2001 was approximately 0.2 m yr.−1 which has accelerated 5-fold (1.0 m yr.−1) between 2000 and 2006 (Jat et al., 2019c). Generally, the current water table depth ranges from 10 to 40 m below ground level across the region (Macdonald et al., 2016; Van Dijk et al., 2016) as opposed to 1 to 8 m below the level of groundwater in the rest of India (Sekhri, 2013). In Punjab and Haryana, it was decreasing at a rate of approximately 1 m per year, and it is anticipated to decrease even faster in the upcoming years (Humphreys et al., 2010; Singh et al., 2014), which will have a significant negative impact on future food security and cause social unrest. Future irrigated agriculture will be entirely dependent on “More Crop Per Drop” to produce sustainably and protect the soil and water resources for the future (Skaggs et al., 2004). New effective production technologies that are adapted to current scenarios, such as tillage, crop establishment, and fertilizer and water management, including legume crops, must be re-designed or developed to replace the old way of doing things to achieve overall sustainability in the irrigated RW system of NW India (Singh et al., 2014; Bhatt et al., 2020).
The conventional puddled transplanted rice (PTR) and wheat were replaced with conservation agriculture (CA) management techniques such as zero tillage (ZT), dry seeded rice (DSR), precision water and residue management, and others to boost farmer productivity and profitability (Ladha et al., 2009; Choudhary et al., 2018). We are concentrating more on precise water management with drip irrigation to meet future water shortages. Drip irrigation provides a number of benefits, including water efficiency and savings, as well as enhanced quality and output in horticultural and vegetable crops (Mohammad, 2015). Surface drip irrigation was discovered to be a practical solution for rice and wheat in cereal systems to address water scarcity (Chen et al., 2015; Sharda et al., 2017); however, anchoring the lateral lines at the start and removing them at the end of the growing season are a laborious process under both conventional and CA management practices. Subsurface drip irrigation (SDI), which lessens water loss via evaporation, enhances water and nutrient usage efficiency directly to the root zone, decreases weed emergence, and lowers labor costs, therefore constitutes a step forward in the acceptability of drip irrigation in cereal systems (Ayars et al., 1999). Jat et al. (2019c) and Sidhu et al. (2019) have recently standardized this SDI system in a CA-based RW system, resulting in increased irrigation water and its use efficiency.
At present, the domestic per capita supply of protein-containing foods such as legumes has declined (Humphreys et al., 2010; Jat et al., 2016). Pulses are the main sources of protein and are crucial for nutritional security and also for the growth and development of the human body. Hence, adding a short-duration pulse crop, such as mung bean, to the RW system also is required to break the monotony of systems and also for enhancing soil quality (Gathala et al., 2013; Jat et al., 2018a). In the RW system of NW India, a fallow period (65–70 days) is available which could be utilized for the cultivation of a short-duration pulse crop (mung bean). Previous research reports suggest that CA-based agronomic management options improved crop yields, saved irrigation water, and increased the economic benefits (Gathala et al., 2013; Jat et al., 2016), but SDI system advances the mung bean sowing by ~7 days and provides more windows to mature the crop (Authors personal observations) compared to flood system. Our research hypothesis is that combining CA-based innovative agronomic management approaches with precision water management (SDI) and the use of crop residues with mung bean integration in the IGP of NW India can help in achieving sustainable crop (cereal and pulse) production. Precise water management and integration of mung bean into a CA-based RW system would boost farm system profitability while enhancing nutrient, water, and energy efficiency in cereal-based systems of NW India.
2 Materials and methods
2.1 Experimental design and treatment description
The portfolio of practices used in the study includes more than two treatments/agronomic interventions in each treatment under RW to see the combined effect of diverse management practices during both kharif and rabi seasons of 2019–20 to 2021–22. The management practices related to varied indicators, viz., tillage, crop establishment, residue management, irrigation strategies, and mung bean integration, are presented in Table 1. Eight scenarios (Sc) included, i.e., Sc1 (FP; farmers’ practice)-puddled transplanted rice (PTR) followed by (fb) conventional tillage wheat (CTW) without residue (−R) with flood irrigation; Sc2-PTR fb CTW with residue (+R) with flood irrigation; Sc3-zero tillage direct seeded rice (ZTDSR) fb zero tillage wheat (ZTW) (−R) with flood irrigation; Sc4-ZTDSR fb ZTW (+R) with flood irrigation; Sc5-ZTDSR fb ZTW (−R) with subsurface drip irrigation (SDI); Sc6-ZTDSR fb ZTW (+R) with SDI; Sc7-ZTDSR fb ZTW fb zero tillage mung bean (ZTMb) (−R) with SDI; Sc8-ZTDSRfb ZTW fb ZTMb (+R) with SDI, were evaluated for productivity, profitability, energy, and water efficiency. Each scenario was replicated thrice in a plot size of 4.05 m x 40 m = 162 m2 in a randomized complete block design. Details of all the scenarios along with different crop management practices are presented in Table 1.
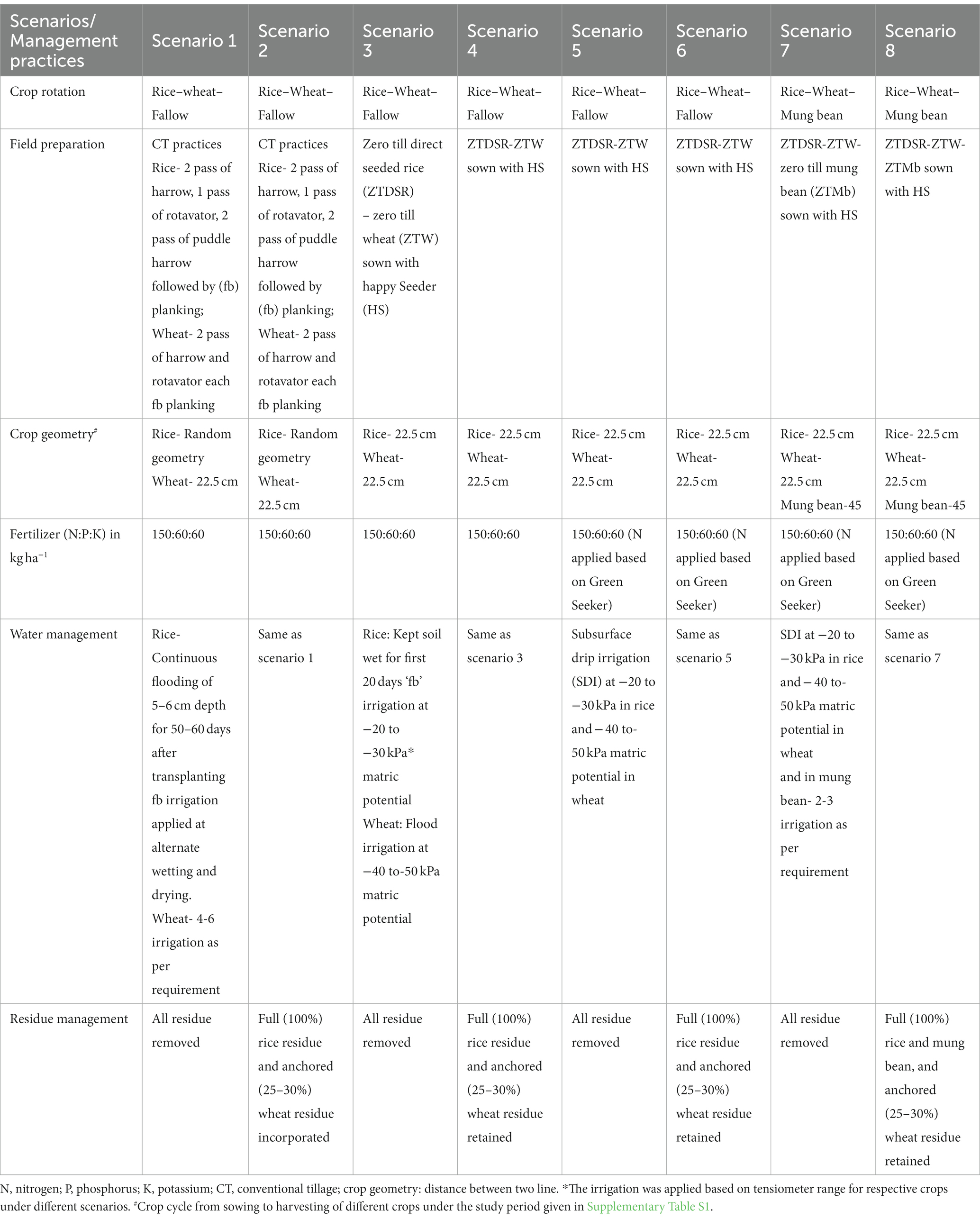
Table 1. Crop rotation, field preparation, tillage, crop geometry, fertilizer, water, and residue management under different scenarios.
2.2 Experiment site and characteristics
The experiment was carried out for 3 years (from 2019–20 to 2021–22) at the ICAR-Central Soil Salinity Research Institute (CSSRI) experimental farm in Karnal, Haryana, India, which is located in the sub-tropical region at 29° 43’ N latitude and 76° 58′ E longitude with an elevation of 240 m above mean sea level in Haryana state of India. The Karnal region’s climate is semi-arid tropical and sub-tropical, with hot and dry summers and freezing winters. The area receives approximately 756 mm of yearly rainfall from the south-west monsoon, with 80% of it falling between June and September and the rest falling during the winter months (December to March). The average annual maximum temperature is 30°C, while the average annual minimum temperature is 17°C. Temperature begins to rise in February and regularly approaches +40°C in May or June, with relative humidity (RH) remaining between 60 and 90% throughout the year. The experimental site’s soil is clay loam with little organic carbon (0.53%), an alkaline pH of 8.1, and a poor nutrient content. Table 2 shows the soil parameters of the experiment site during the preliminary stage.
2.3 Crop residue management
All crop residues of the rice–wheat crop were removed from the surface in the farmer’s practice (Sc1). Approximately 50% rice and 25–30% anchored wheat residue were absorbed or incorporated into the soil before the next harvest in the improved farmer’s practice (Sc2). All crop residues were eliminated in ZT-based scenarios (Sc3, Sc5, and Sc7); however, in Sc4 and Sc6, the entire residue (100%) of rice and anchored (25–30%) of wheat stubbles residue remained on the soil surface, and in Sc8, 100% mung bean residue was also retained. The residue data of each scenario are given in Table 3.
2.4 Fertilizer management
Nitrogen, phosphorus, and potash (N-150: P2O5–60: K2O-60 kg ha−1) fertilizers were applied as per the recommendation given by CCS Haryana Agricultural University, Hisar, India for both rice and wheat crops (Table 1). N was supplied in basal through NPK (12,32,16) complex fertilizer, and the remaining N was applied through urea (46% N) based on their recommendation under different scenarios. However, the entire amount of P and a half dose of K were applied as basal through NPK and the remaining K through MoP (muriate of potash- 60% K2O), respectively, in all the scenarios. In Sc1 and Sc2 (PTR), 187.5 kg NPK complex as basal with remaining 33.3 kg MoP and 277 kg urea ha−1 as top-dressed in three equal splits were applied at establishment (15–20 DAT, days after transplanting), tillering (25–30 DAT), and panicle initiation stage (45–50 DAT) in rice. In CT wheat, same as with rice, was applied at basal and two top-dressed of N at crown root initiation (21–23 DAS days after sowing) and maximum tillering stage (45 DAS). In Sc3 and Sc4 (zero tillage), same as PTR, dose was drilled as basal and top-dressed of N in three equal splits as 20, 40, and 60 DAS in rice. However, in ZT wheat, same as CT wheat, basal and urea were top-dressed.
In subsurface drip-irrigated scenarios (Sc5 to Sc8), same dose of basal as PTR and ZTDSR scenarios was drilled, and the remaining N was applied based on green seeker (GS) reading through fertigation (Supplementary Table S2) at 10- and 15-day intervals in eight equal splits staring from 15 DAS (1st four intervals’ days 10 and remaining interval days 15) in rice. However, same as rice, in wheat based on GS, it was applied in five equal splits at 20, 35, 50, 65, and 80 DAS. In mung bean, 111 kg DAP ha−1 fertilizer was applied as basal in both scenarios. For better estimation of nitrogen (N), the nitrogen nutrition index (NNI) and partial factor productivity of N (PFPN) were calculated using the following equations. Based on the grain % N dilution curve, the N nutrition index of grain (NNI) was calculated using Eq. 1.
where %NG is the grain N concentration, if NNI < 1 would indicate that grain yield is limited by N supply; NNI = 1 would indicate that grain N status is non-limiting for the grain yield; and NNI > 1 would indicate that luxury N is accumulated in grains. For determining the nitrogen nutrition index (NNI), He et al. (2022) described the critical N dilution curve (%NC) of rice = 3.37 W −0.44, while Justes et al. (1994) described the critical N dilution curve (%NC) of wheat = 2.85 W −0.17, where NC is critical N concentration, and W is grain yield (Mg ha−1).
The partial factor productivity of N (PFPN) was calculated using Eq. 2:
2.5 Enlistment of subsurface drip irrigation system
The main line, sub-mains, and laterals of the subsurface drip irrigation (SDI) system had inner diameters of 90, 63, and 16 mm, respectively. The volume of water applied in flood and SDI plots for each crop in each irrigation was measured using a water meter (Landforce Dasmesh Mechanical Works, Punjab, India) fitted on the delivery pipe. By adding the irrigation water and rainfall, the total amount of water consumed by the crop was computed. The tensiometer was installed at a 15-cm soil depth between the laterals and/or crop rows. Parallel to the planting rows, the plastic laterals were laid. The drip irrigation system was kept running at a 1.5 to 2.0 kg cm2 pressure. The emitters were in-line, with a capacity of 2.0 L h−1 at 135 kPa and a distance of 40 cm between them. In rice, wheat, and mung bean crops, mains and sub-mains were set out at 100 cm, while laterals were laid out at 15 and 45 cm depth and spacing, respectively. The drip system consisted of a hydrocyclone filter fitted at the source of irrigation. An upstream and downstream suction was created by a Venturi injector system during fertigation.
2.6 Water management
Irrigation scheduling under different scenarios/practices is explained in Table 1. Rainfall was monitored using a rain gauge located near the site in a meteorological laboratory. The amount of irrigation water applied was quantified (in mm ha−1) using Eqs. 3, 4, and irrigation water productivity (WPI) using Eq. 5 as follows:
The water use efficiency was calculated using Eq. 6.
where total water use (TWU) is calculated using irrigation water (cm) and rainfall (cm), while 1 ha-mm irrigation depth = 10 kiloliter = 10 m3; 1 m3 = 1,000 liter.
Water management protocols for each scenario are presented in Table 1. For rice, 14, 9, and 7 irrigations were applied in PTR scenarios, whereas 12, 11, and 9, and 25, 18, and 14 irrigations were applied in ZTDSR under flooded and SDI conditions based on tensiometer readings, respectively, during the respective years. However, for wheat, the total number of irrigations required was 3, 2, and 2 under CT scenarios, and 2 and 5 in ZTW under FI and SDI conditions based on tensiometer readings, respectively. The mung bean crop in Sc7 and Sc8 required 3, 3, and 2 irrigations during the 1st, 2nd, and 3rd year, respectively.
2.7 Crop yields and system productivity
All three crops were determined on a total area of 27 m2 by sampling from three locations of 9 m2 each. To compare the productivity of rice, wheat, and mung bean and the total system productivity of the different scenarios, the yield of non-rice crops (wheat and mung bean) was converted into rice equivalent yield (REY) (Mg ha−1) and calculated using Eq. 7.
where MSP is the minimum support price (Table 4); (1 USD = 70 INR).
2.8 Energy analysis
Seed, fertilizer, irrigation, labor, diesel, pesticides, machinery, and other crop inputs were utilized to determine the total energy input for rice, wheat, and mung bean, whereas grain and straw were estimated for output energy using energy equivalents (MJ unit−1) values given by Gathala et al. (2016), Argiro et al. (2006), and Ozkan et al. (2004). The ratio between total energy outputs and total energy inputs was used to calculate the energy use efficiency (EUE) of crops under various situations using Eq. 8.
2.9 Economic analysis
The calculation of variable costs was calculated by different crop operations, i.e., nursery of rice, tillage, seeding, harvesting, and threshing (tractor and combine on hours ha−1 basis), while input materials and labor (8 h = one person day’s ha−1) for raising the crops. The prices of all the inputs used for crop production were recorded, with irrigation water being charged at INR 0.30 per kWh of electricity fixed by the local government of Haryana including extra labor charges for application (Table 4). The cost of a drip irrigation system was calculated after considering the 80% subsidy provided by the government of India on the actual cost (INR 250 × 103 ha−1). The cost incurred on the drip system was calculated considering the life span of 20 years for the main line, sub-main line, Venturi, and pump. The life of the subsurface drip irrigation system was considered 15 years. The depreciation cost of the drip irrigation system was considered as 10%. The minimum support price (MSP) of grain crops fixed by the Govt. of India and prevailing local market rates every year were used for crop straw (Table 4). The net return from each crop was calculated after subtracting variable costs/cultivation costs from the calculated gross returns. The system net returns were estimated by summing the net returns of all crops harvested within a calendar year.
2.10 Statistical analysis
The data collected for various crop factors were analyzed using the analysis of variance (ANOVA) technique using SAS 9.1 software (Gomez and Gomez, 1984) for randomized complete block design (SAS Institute, 2001). Tukey’s honestly significant difference was used to compare the treatment means (HSD at a 5% level of significance).
3 Results
3.1 Weather conditions
All the weather parameters measured during the study period are presented in Figure 1. Crops received a total rainfall of 769, 1,079, and 1,371 mm in 2019–20, 2020–21, and 2021–22, respectively. Though the distribution was not uniform throughout the crop season and year, the maximum rainfall was received in July and August (Figure 1A). During the 1st year of the kharif (rice) season, 50% rainfall was received in 2 consecutive months, i.e., July—245 mm and August—101 mm with total 380 mm; however, it was 928.5 mm and 1,044 mm during the 2nd and 3rd year, respectively (Figure 1A). During rabi season, 297, 110, and 217 mm rainfall were received during the 3 consecutive years, whereas the mung bean crop was received 92.4, 40.2, and 111 mm, respectively. The maximum and minimum temperatures were almost the same during the reported years (Figure 1B).
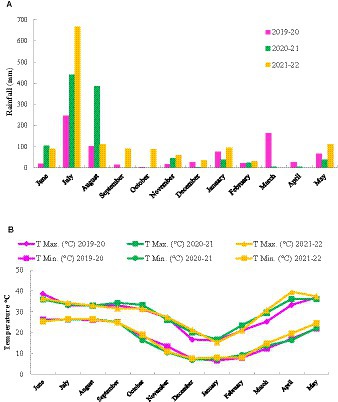
Figure 1. Monthly mean weather data of (A) total rainfall and (B) maximum and minimum temperature during 2019–20, 2020–21, and 2021–22.
3.2 Crop productivity
In the current study, CA practices irrespective of flood and subsurface drip irrigation (SDI) influenced the grain yields of rice over the years (2019–20 to 2021–22) (Table 5). CA-based Scenario 3 [ZTDSR (−R)] recorded 8% higher rice grain yield, while Sc4 [ZTDSR (+R)] recorded 6% higher with flood irrigation (FI) system than the farmer’s practice (Sc1; 5.44 Mg ha−1). With the SDI system, similar grain yield of rice was recorded across the scenarios and lower as compared to Sc3 and Sc4 over the years. In contrast, CA-based scenarios with the SDI (Sc5–Sc8) system produced significantly (p ≤ 0.05) lower yield than FI during the years (Table 6). However, CT with residue incorporation (Sc2) improved the rice yield by 4% compared to Sc1 in all 3 years.
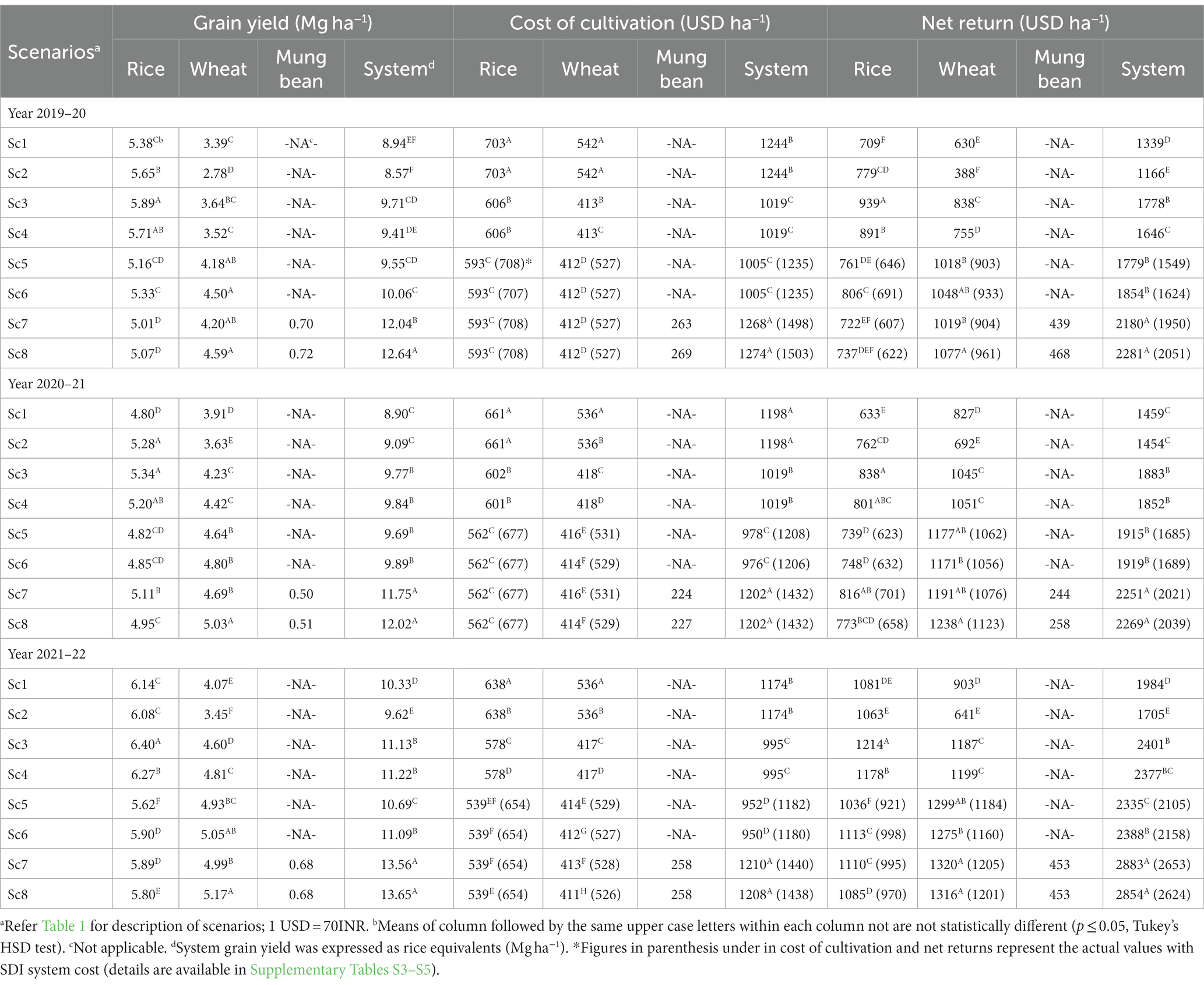
Table 5. Effect of management practices on grain yield, cost of cultivation, and net returns under different management scenarios during 2019–20 to 2021–22.
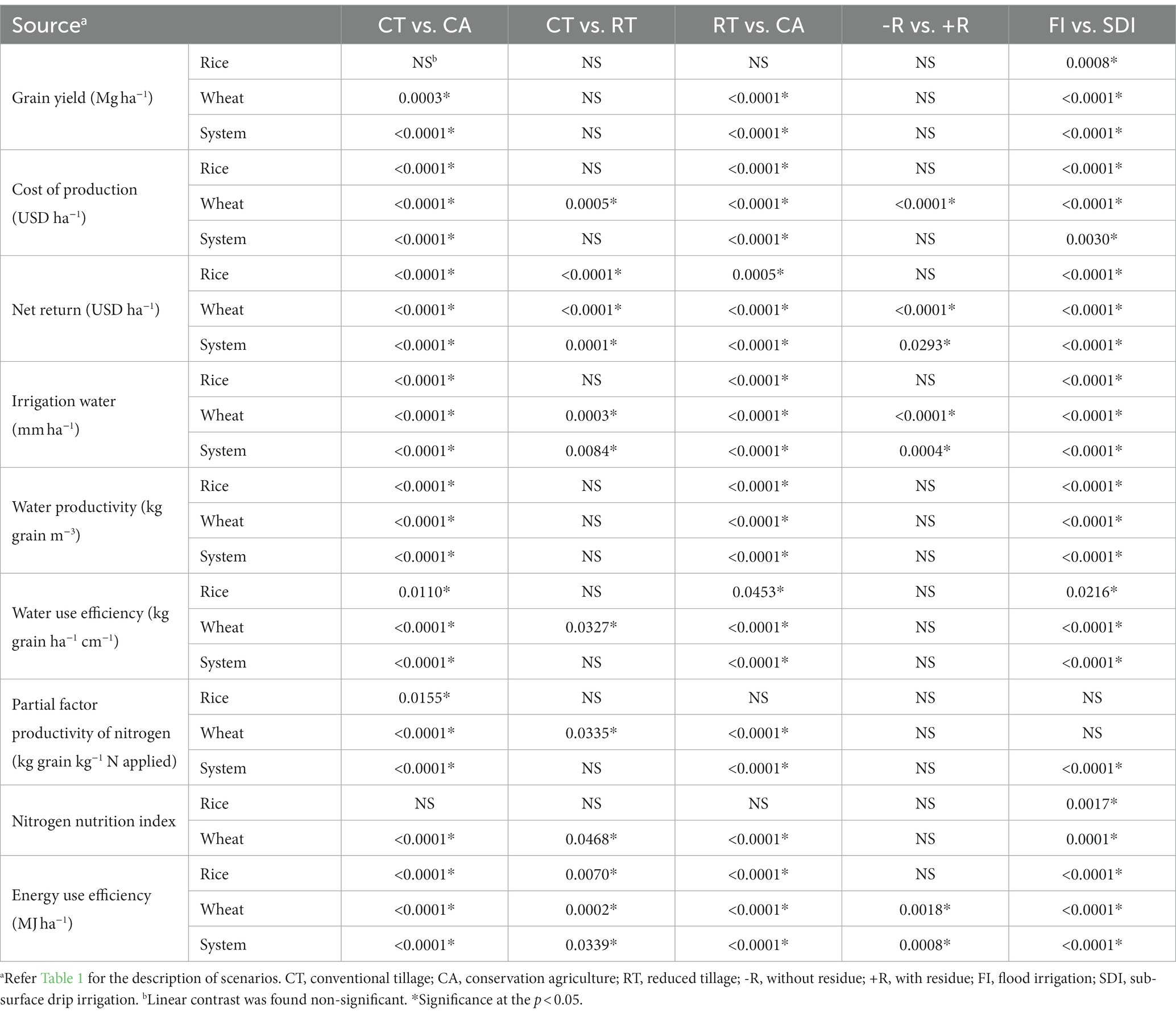
Table 6. Significance effects of different agronomic management practices and their linear contrast on different sources under different scenarios during the 3 year mean (2019–20 to 2021–22).
On a 3-year mean basis, Sc8 (ZT rice–wheat–mung bean) recorded a 30% higher wheat grain yield over Sc1 (3.79 Mg ha−1) (Table 5), while CA with FI (Sc3 and Sc4) improved the wheat yield by 10 and 12% (a 3-year mean), respectively, compared to Sc1. However, scenarios with SDI (Sc5–Sc8) produced 21–30% higher wheat grain yield during the 3 consecutive years than Sc1 (3.79 Mg ha−1). In contrast, precise water and N management through SDI significantly (p ≤ 0.05) improved the wheat grain yield by 10–19% across the years compared to FI irrespective of management practices (Table 6). The improved farmer’s practices (Sc2) were recorded as 13% lower grain yield over FP (Sc1) based on a 3-year mean. The grain yield was lowered by ~18% during 2019–20 due to unfavorable weather conditions throughout the year. On a 3-year mean basis, the mung bean crop recorded yields of 0.60 and 0.62 Mg ha−1 in Sc7 and Sc8, respectively.
System yield (rice equivalents; RE) varied from 8.57 to 13.65 Mg ha−1 during the 3 years of study (Table 5). The integration of mung bean in the rice–wheat system with SDI (Sc8) recorded a 36% (3 years’ mean) higher system yield over Sc1 (9.38 Mgha−1), and it was closely followed by Sc7 (12.47 Mgha−1). The lowest (9.09 Mg ha−1) system yield (a 3-year mean basis) was recorded with the CT rice–wheat system, where crop residues were incorporated (Sc2). The CA with SDI without mung bean (Sc5 and Sc6) integration increased the system productivity by 7 and 10% (a 3-year mean) compared to Sc1, whereas with SDI with mung bean integration (Sc7 and Sc8), the system yield was increased by 33 and 36% compared to Sc1 (9.38 Mg ha−1) (Table 5). CA-based system (mean of Sc3–Sc8) increased the system productivity by 19% (a 3-year mean) compared to the CT-based system (mean of Sc1 and Sc2; 9.24 Mg ha−1) (Table 5). The contrast effects were significant to system productivity associated with different CA-based flood and SDI management practices (Table 5).
3.3 Economic profitability
The cultivation cost depends upon the different management practices starting from field preparation to harvesting/threshing, input cost, and related man days involved in crop production (Table 5). Scenarios 1 and 2 associated with PTR (USD 667 ha−1) and CT wheat (USD 538 ha−1) led to significantly (p ≤ 0.05) higher cost of cultivation during the study. In contrast, CA-based scenarios with SDI (mean of Sc5–Sc8) significantly (p ≤ 0.05) recorded (15.43 and 23.20%; 3 years’ mean) lower cultivation costs in rice and wheat, respectively (Table 6). However, CA-based scenarios with FI (Sc3 and Sc4) recorded 10.85 and 22.68% (3-year mean) lower cultivation costs for rice and wheat production, respectively, compared to CT-based scenarios (Sc1 and Sc2). On a 3-year basis, higher (1.81%) system cultivation cost was recorded under the CA-based scenario with SDI and mung bean integration (Sc7 and Sc8; USD 1227 ha−1) than the CT-based scenario (USD 1205 ha−1), while lowest was found under Sc5 and Sc6 (USD 978 ha−1), respectively, based on the 3-year mean (Table 5).
Among the different scenarios of rice, Sc3 recorded a 23.5% higher net return, which was closely followed by Sc4 (18.49%), and the lowest net return was recorded with farmers’ practice (Sc1; 807 USD ha−1) (Table 5). A higher net return was also recorded with SDI scenarios, and it was 4.66, 10.07, 9.27, and 7.10% higher in Sc5, Sc6, Sc7, and Sc8, respectively, than Sc1 (USD 807 ha−1). In wheat, Sc8 (CA-based ZTW + R with SDI) recorded the highest net return of 53.9% and was closely followed by Sc7 (49.6%), whereas the lowest was fetched with CTPTR+R (Sc2; USD 573 ha−1) on a 3-year mean basis. CA-based ZT wheat irrespective of residue management (mean of Sc3 and Sc4) recorded 28.73% higher net returns than Sc1 (786 USD ha−1). The mung bean crop in Sc7 and Sc8 improved the net return by USD 379 and 393 ha−1, on the cultivation cost of USD 248 and 251 ha−1 (3-year mean). CA-based RW integrated with mung bean under SDI system (Sc7 and Sc8) improved the crop net returns/profitability by 53.88% (3-year mean) over farmers’ practice (Sc1; USD 1594 ha−1) irrespective of residue management practices (Table 5). The CA-based scenarios (Sc5–Sc8) with the SDI system recorded higher system net returns by 26.09, 28.83, 52.93, and 55.83%, respectively, than the farmer’s practice (Sc1). In contrast, all the CA-based scenarios (mean of Sc3 to Sc8) significantly (p ≤ 0.05) recorded 42.16% (3-year mean) higher system net returns over CT-based scenarios (USD 1518 ha−1; mean of Sc1 and Sc2 (Table 5). Moreover, the CA-based scenarios (Sc5–Sc8) including SDI amount increased net return by 11.7 to 40.4 and 17.3 to 47.4% as compared to the Sc1 and CT-based scenarios, respectively.
3.4 Irrigation water use
Rice and wheat crops need variable irrigation water requirements to meet their evapo-transpiration demand for completing their lifecycle. Rice water requirement was mainly influenced by crop establishment (puddled vs. direct seeding) and irrigation methods (flood vs. drip). The amount of irrigation water used in rice varied from 527 to 1,177 and 195 to 494 mm ha−1 in flood and subsurface drip irrigation systems, respectively, over the 3 years of study (Table 7). Farmers’ practice (CTPTR-R) used the highest (1,035 mm ha−1) amount of irrigation water, and it was ~35% (3-year mean) higher than CA-based ZTDSR with flood irrigation (mean of Sc3–Sc4). However, CA-based ZTDSR with SDI (Sc5–Sc8) saved ~69% of irrigation water compared to Sc1 (1,035 mm ha−1) (3-year mean). Based on the 3-year mean of wheat, conventional tillage (Sc1 and Sc2) in wheat recorded 12.45 and 44.15% higher irrigation water use than CA-based FI and SDI-based scenarios (Sc3–Sc4 and Sc5–Sc8), respectively. With the SDI system, the CA-based scenarios (Sc5–Sc8) saved 52.19% (1st year), 30.12% (2nd year), and 54.00% (3rd year) irrigation water compared to the CT wheat scenarios (mean of Sc1 and Sc2; 182, 395, and 376 mm ha−1, respectively). The CA-based FI scenarios (Sc3 and Sc4) saved 11.60% (3-year mean) irrigation water than farmer’s practice (Sc1). With the SDI system, scenarios 5, 6, 7, and 8 saved 63.10, 63.30, 58.50, and 58.42% of irrigation water (3-year mean), respectively, compared to farmer’s practice (Sc1; 1,355) in RW rotation (Table 7). However, CA-based ZTRW with FI scenarios (mean of Sc3 and Sc4) saved 29.52% (3 years’ mean) irrigation water compared to CTRW scenarios (mean of Sc1 and Sc2; 1,353 mm). In contrast, CA-based practices significantly (p ≤ 0.05) saved the irrigation water compared to conventional practices in rice, wheat, and RW systems, but were non-significant in CT vs. RT and –R vs. +R in rice crop during the 3-year mean (Table 6).
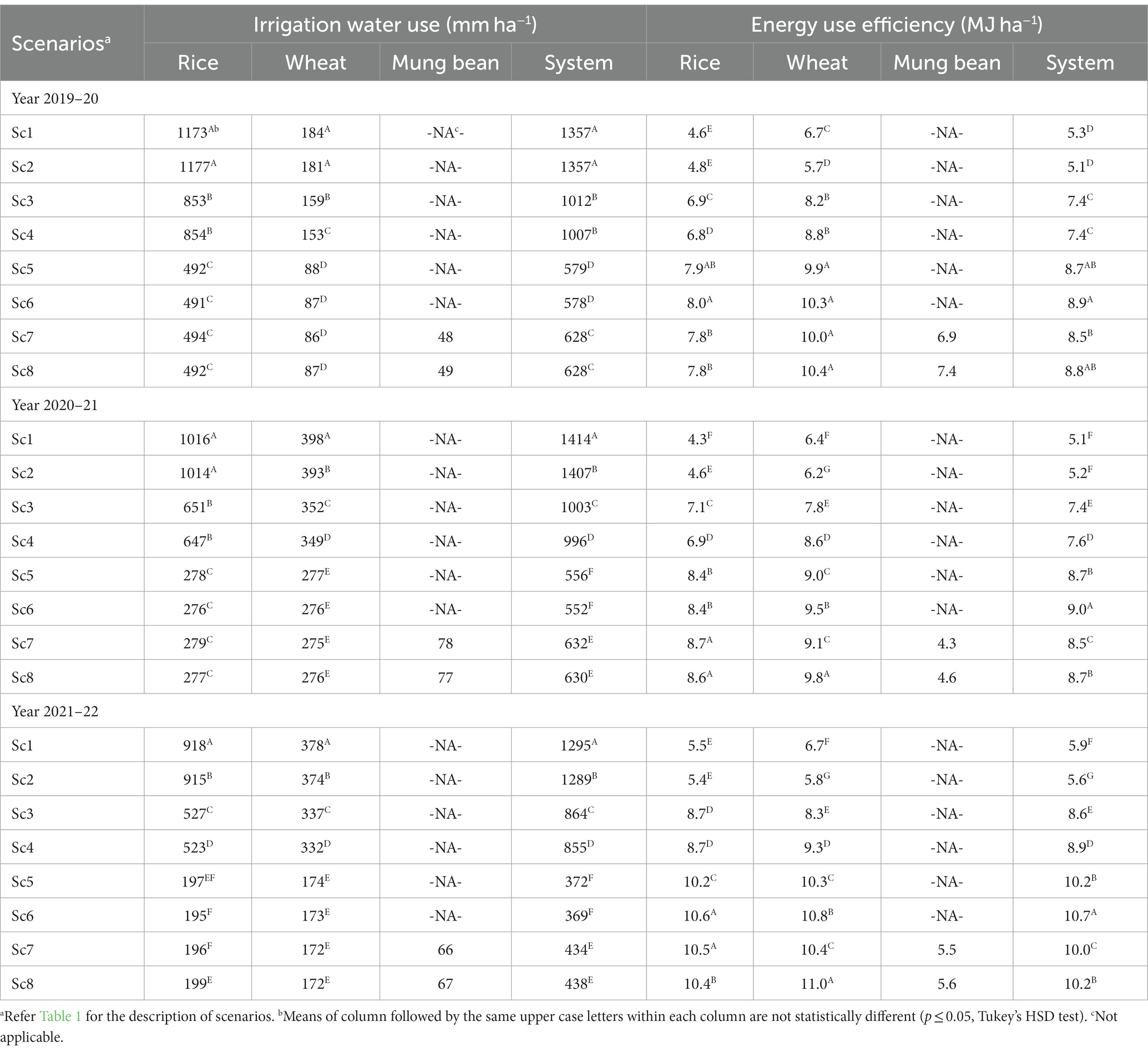
Table 7. Effect of management scenarios on irrigation water use and energy use efficiency (EUE) during 2019–20 to 2021–22.
3.5 Water productivity of irrigation (WPI)
The lowest irrigation water productivity (WPI) was recorded with Sc1 (0.53 kg grain m−3) and the highest (266.42% of Sc1) with Sc6. CA-based practices with the SDI system improved the WPI of rice by 266.42% (3-year mean) compared to Sc1 (Figure 2). In wheat, CA-based ZT wheat with the SDI system (Sc5, Sc6, Sc7, and Sc8) recorded significantly higher WPI by 158–188, 70–86, and 163–178% during 1st to 3rd year, respectively, than CT wheat (Sc1). CT practices (Sc1 and Sc2) in wheat decreased the WPI by 35.9, 29.9, and 40.9% compared to CA-based ZT wheat with FI (mean of Sc3 and Sc4), respectively, over the year (Figure 2). Farmers’ practice (Sc1; 1.84, 0.98, and 1.08 kg grain m−3) also recorded higher WPI at 16.3, 5.8, and 14.7% than improved farmer’s practice (Sc2) over the years. CA-based ZT wheat with SDI (mean of Sc5–Sc8) increased the WPI by 147.8% (3-year mean) compared to Sc1 (1.30 kg grain m−3).
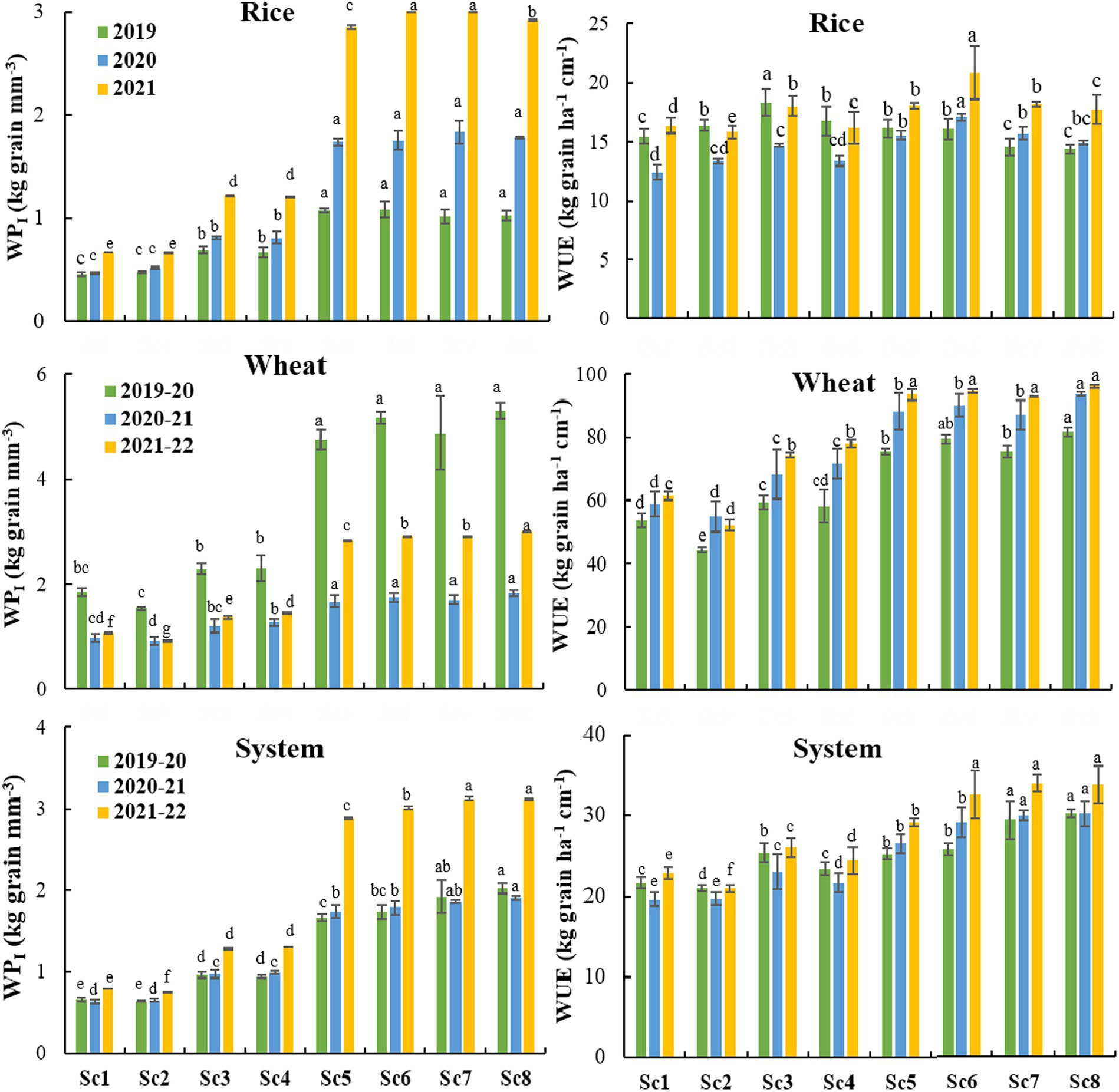
Figure 2. Effect of management practices on irrigation water productivity (WPI) and water use efficiency (WUE) under different management scenarios from 2019–20 to 2021–22. Rainfall data of rice, wheat, and mung bean season are present in Figure 1A.
System-based irrigation water productivity (WPI) was lower in CT-based scenarios (Sc1; 0.69 and Sc2; 0.67 kg grain m−3) during all the 3 years. However, CA-based scenarios (Sc3–Sc8) recorded significantly higher WPI to the tune of 42–207, 54–204, and 62–292% during 1st to 3rd year than Sc1 (0.63–2.02, 0.63–1.90, and 0.75–3.12 kg grain m−3), respectively (Figure 2). CA-based scenarios with SDI system (Sc5–Sc8) improved WPI by 221.2 (3-year mean) compared to farmers’ practice (Figure 2).
3.6 Water use efficiency
In rice, the efficiency of water use varied from 14.38 to 18.34, 12.42 to 17.05, and 15.79 to 20.80 kg grain ha−1 cm−1 during the 1st, 2nd, and 3rd year, respectively (Figure 2). Scenario 3 (ZTDSR-R with FI) recorded highest water use efficiency (18.34 kg grain ha−1 cm−1) during the 1st year, while it was higher by 37.29 and 27.34% in Sc6 (ZTDSR+R with SDI system) than farmer’s practice/Sc1 (12.42 and 16.33 kg grain ha−1 cm−1) during the 2nd and 3rd year, respectively. The efficiency of water applied in CA-based scenarios with the SDI system was significantly (p < 0.05) lowered by 0.9% in the 1st year, but it was 27.21 and 14.39% higher during the 2nd and 3rd year, respectively, than Sc1 (Figure 2). In wheat, compared to CT, wheat without residue (Sc1; 53.56, 58.83 and 61.28 kg grain ha−1 cm−1) recorded lower WUE by 9.74, 18.82, and 24.07% than CA-based ZT wheat with flood irrigation (3-year mean of Sc3 and Sc4) during the 1st to 3rd year, respectively. With the SDI system, WUE was higher by 53.61% during the 3rd year, which was 8.14% higher than the 1st year than CT wheat (Sc1). The lowest (50.48 kg grain ha−1 cm−1) WUE was recorded in CT wheat with residue (Sc2) based on the 3-year mean (Figure 2).
On a system basis, the highest WUE was recorded with Sc8 by 52.99% compared to Sc1 (21.39 kg grain ha−1 cm−1) and the lowest to be recorded by 3.89% in Sc2 during the 3-year mean (Figure 2). With the SDI system, CA-based scenarios (mean of Sc5–Sc8) improved the WUE by 28.01, 47.87, and 41.61%, respectively (3-year mean) than Sc1 (21.66, 19.63, and 22.89 kg grain ha−1 cm−1), while CA-based scenarios with FI (mean of Sc3 and Sc4) improved the WUE by 12.27% (3-year mean) compared to Sc1 (Figure 2). In contrast, CA-based practices significantly (p ≤ 0.05) improved the WUE compared to conventional practices in rice, wheat, and RW system but was non-significant in CT vs. RT and –R vs. +R in rice and RW systems during the 3-year mean (Table 6).
3.7 Nitrogen nutrition index
The nitrogen nutrition index ranged from 0.53 to 0.69, 0.48 to 0.59, and 0.64 to 0.82 during the 1st, 2nd, and 3rd year, respectively, in rice (Figure 3). In ZTDSR with FI (3-year mean of Sc3 and Sc4), the nitrogen nutrition index (NNI) was increased by 19.5% compared to farmer’s practice (0.58). During the rice season in the 3 years, the NNI value of CTPTR scenarios (Sc1 and Sc2) ranged from 0.48 to 0.71 (lower than 1) indicating that N limited the grain yield, while both scenarios recorded higher NNI value to the SDI system (Figure 3). In the SDI system, CA-based scenarios (mean of Sc5–Sc8) improved the NNI value by ~10% during the 3rd year compared to the 1st year of the study. In contrast, FI vs. SDI significantly (p ≤ 0.05) improved the NNI in rice crop during the 3 years (Table 6).
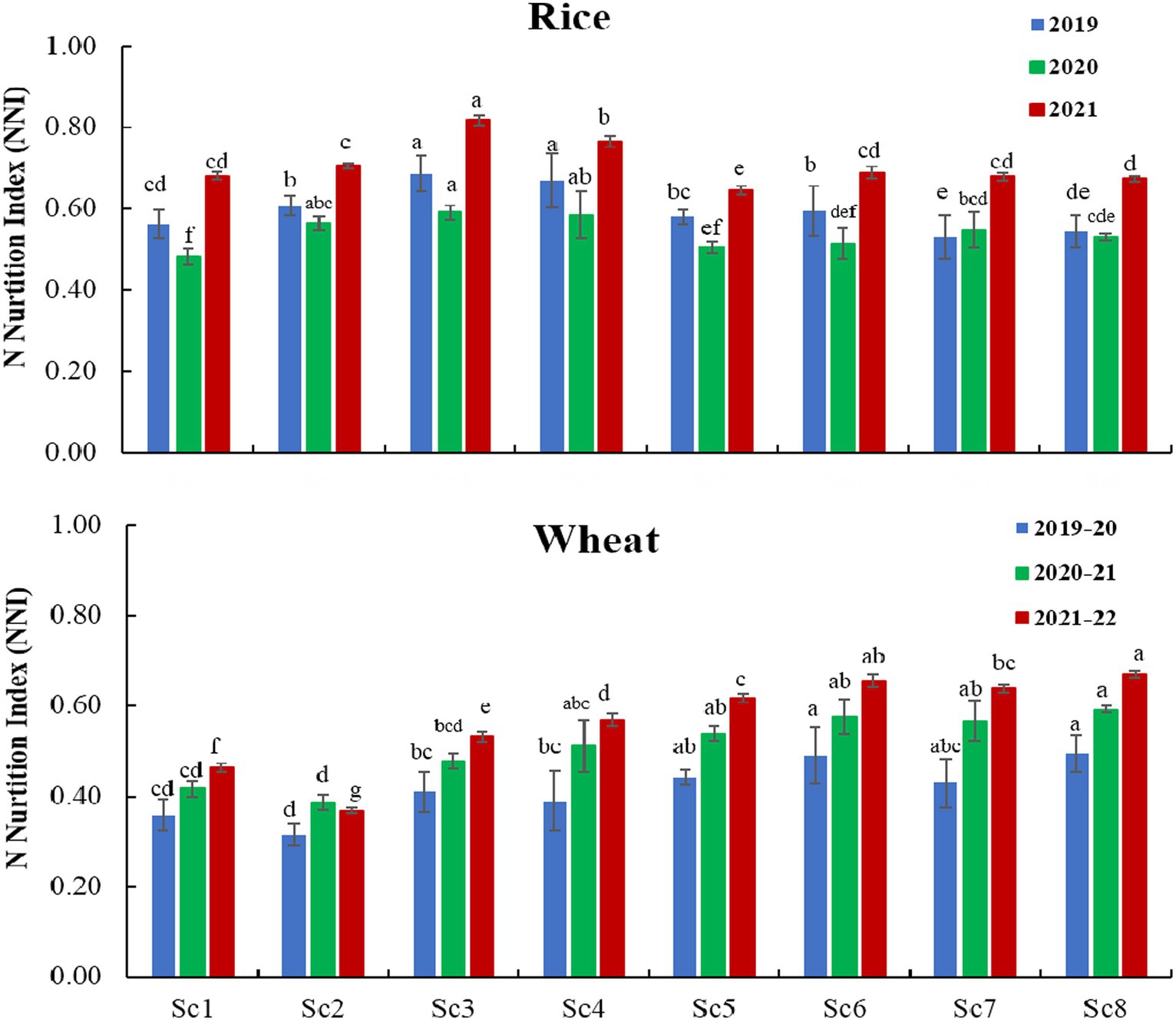
Figure 3. Effect of management practices on nitrogen nutrition index (NNI) under different management scenarios of rice and wheat crop from 2019–20 to 2021–22.
In wheat, conventional tillage (Sc1 and Sc2) practices recorded 26.32 and 47.37% lowered NNI values compared to CA-based FI and SDI scenarios (Sc3–Sc4 and Sc5–Sc8), respectively, during all the 3 years (Figure 3). The NNI values in wheat ranged from 0.31 to 0.49, 0.39 to 0.59, and 0.37 to 0.67 during the 1st, 2nd, and 3rd year, respectively. During the wheat season in the 3 years, the NNI value of SDI scenarios (Sc5–Sc8) ranged from 0.43 to 0.67 (lower than 1.0) but slightly increased the grain yield over the years than CT-based scenarios. CA-based ZT wheat with SDI including summer mung bean (Sc8) increased the NNI by 41.3% (3-year mean) compared to farmer’s practice (0.41) and was the lowest to be recorded under CTW + R scenario (Sc2; 0.36). Contrast effects of CT vs. CA, CT vs. RT, RT vs. CA, and FI vs. SDI significantly affected NNI under different management scenarios (Table 6).
3.8 Fertilizer-N use efficiency
The partial factor productivity of N (PFPN) and an index of N use efficiency (NUE) varied from 33.44 to 39.29, 31.45 to 35.35, and 37.66 to 42.66 kg grain kg−1 N applied in rice crop over the 3 years of study (Figure 4). The ZTDSR-R (Sc3) recorded the highest (39.10 kg grain kg−1 N) PFPN compared to CT-based scenarios (mean of Sc1–Sc2) on 3-year basis. However, CA-based ZTDSR with SDI (mean of Sc5–Sc8) increased PFPN by 0.3% compared to Sc1 (36.26 kg grain kg−1 N) during the 2nd year. In wheat, partial factor productivity of N varied from 18.56 to 30.62, 24.24 to 36.70, and 22.99 to 38.48 kg grain kg−1 N applied during the 1st, 2nd, and 3rd year, respectively (Figure 4). In contrast, CT vs. CA significantly (p ≤ 0.05) improved PFPN in rice crop during the years under investigation (Table 6).
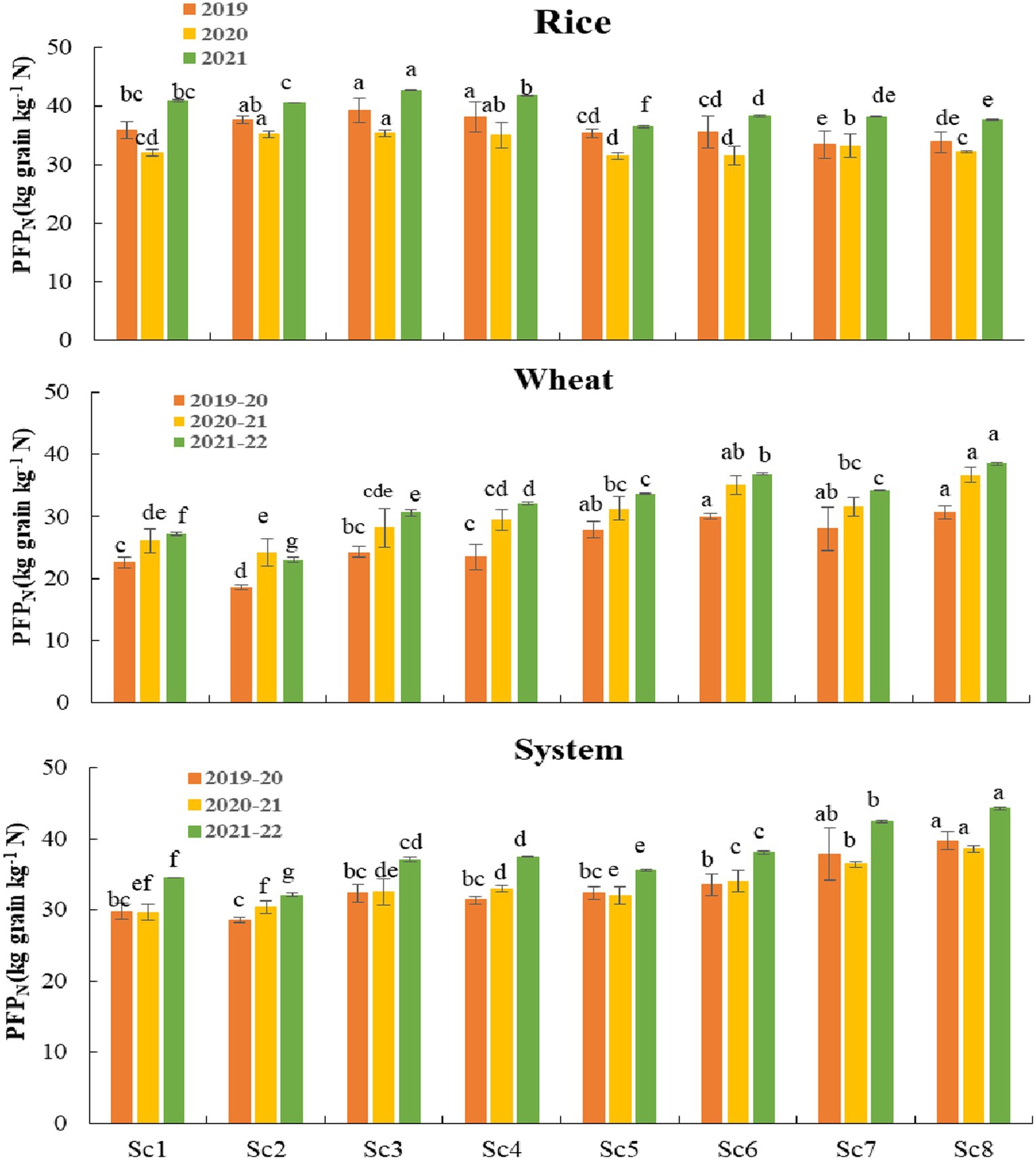
Figure 4. Effect of management practices on partial factor productivity of nitrogen (PFPN) under different management scenarios from 2019–20 to 2021–22.
Based on the 3-year mean, Sc8 recorded 49.39% higher PFPN over conventional tillage (23.60 kg grain kg−1 N) followed by 44.03% over Sc6 (Figure 4). With the SDI system, CA-based scenarios (Sc5–Sc8) recorded 28.99% (1st year), 28.99% (2nd year), and 31.61% (3rd year) higher PFPN than farmer’s practice (22.58, 26.08, and 27.17 kg grain kg−1 N, respectively). In flood conditions, CA-based scenarios (Sc3 and Sc4) also improved PFPN by 10.63% (3-year mean) than farmer’s practice (Sc1). The contrast effects were significant to partial factor productivity of N associated with different CT and CA-based management practices (CT vs. CA, CT vs. RT, and RT vs. CA) during the 3-year mean (Table 6).
On a system basis, scenarios 5, 6, 7, and 8 improved 6.60, 12.59, 24.26, and 30.72% PFPN (3-year mean), respectively, compared to Sc1 (31.28 kg grain kg−1 N) in RW rotation (Figure 4). However, CA-based ZT rice–wheat with FI (mean of Sc3 and Sc4) also recorded higher PFPN by 10.10% (3-year mean) than the CT rice–wheat scenario (mean of Sc1 and Sc2; 31.28 and 30.30 kg grain kg−1 N) (Figure 4).
3.9 Energy use efficiency
Energy use efficiency (EUE) was significantly (p < 0.05) influenced by tillage, crop establishment method, residue, water, and nutrient management practices in the RW system (Table 7). The CA-based scenarios with flood irrigation (Sc3 and Sc4) recorded the higher EUE of rice by 56.2% (3-year mean) than Sc1 (4.8 MJ ha−1) during the 3 years of experimentation. In wheat, CA-based scenarios with SDI (Sc5–Sc8) increased the EUE by approximately 45.2–57.8% compared to Sc1 (6.43–6.73 MJ ha−1) during the 1st to 3rd year, respectively. Scenario 8 recorded 57.3% higher EUE than Sc1 (6.63 MJ ha−1) based on the 3-year mean data. The CA-based scenarios with FI (Sc3 and Sc4) improved EUE by 40.4% compared to farmers’ practice (Sc1).
On a system basis, CA-based scenarios with SDI system recorded 69.4% higher EUE than farmers’ practice (5.45 MJ ha−1) over the years (Table 7). However, CA-based scenarios with FI (mean of Sc3 and Sc4) increased EUE by 44.7% (7.89 MJ ha−1) compared to Sc1. On a system basis, Sc8 increased the energy EUE by 69.4% (3-year mean) compared to Sc1 (5.45 MJ ha−1) during all the years (Table 7). In 3 years, contrast effects were significant to energy use efficiency associated with different CA-based management practices (CT vs. CA, CT vs. RT, RT vs. CA, −R vs. +R, and FI vs. SDI) in rice, wheat, and RW systems except –R vs. +R in rice crop (Table 6).
4 Discussion
4.1 Crop productivity
The rice–wheat system of western IGP mainly depends on groundwater aquifers to meet their evaporative demand. In the present study, higher rice grain yield was recorded with CA-based flood irrigation scenarios (Sc3 and Sc4) which might be due to the more favorable micro-climatic conditions throughout the season as well as due to the residual effect of previous crop residues (Table 5). Based on recorded datasets, under flood irrigated circumstances, direct seeded rice (DSR) production was greater due to a higher number of panicles, 1,000-grain weight, and a lower sterility percentage (Sarkar et al., 2003). Zero tillage direct seeded rice (ZTDSR) also provides additional benefits of time savings as it is harvested 7–10 days earlier and benefits the following crop in the cycle (Kumar and Ladha, 2011). Sidhu et al. (2019) showed similar outcomes in comparable ecologies with lateral spacing of 45–60 cm. In accordance with similar findings, other researchers (Yadav et al., 2011; Nagaraju et al., 2014; Sharda et al., 2017; Parthasarathi et al., 2018) hypothesized that drip irrigation might increase rice yield due to higher dry matter accumulation, tiller m−2, panicle weight, and harvest index compared to conventional flood irrigation. In wheat, SDI with CA-based management practices recorded a higher grain yield over Sc1 (Table 5). The SDI scenarios (Sc5–Sc8) produced 21–30% (3-year mean) higher grain than Sc1 (3.79 Mg ha−1), and this may be attributable to increased soil moisture availability, moderated soil temperature, improved soil fertility brought about by continuous nutrient supply, particularly nitrogen through N-fertigation, decreased weed population, mineralization brought about by crop residue retention on the soil surface, and integration of legumes (Kakraliya et al., 2018; Sandhu et al., 2019; Jat et al., 2019b, 2021a).
Several studies in the IGP region have demonstrated that ZT wheat enables early sowing by almost 2 weeks coupled with residual mulch for improved temperature regulation and protection of crop from heat stress during the reproductive stage (Gathala et al., 2011; Choudhary et al., 2018), and by preventing soil compaction, rice produced under aerobic conditions (DSR) benefits the next wheat crop as well (Jat et al., 2019a, 2021a). The combined impacts of CA techniques and precise water and N management may be to blame for the current findings of increased wheat output under SDI in both years (Sidhu et al., 2019; Jat et al., 2019a). The crop residue mulch on the soil surface delivered favorable conditions for crop growth and development resulting in higher grain yields of wheat in the Western IGP of India and these findings are consistent with other studies (Gao et al., 2014; Jat et al., 2018a; Sidhu et al., 2019; Umair et al., 2019; Jat et al., 2019a). Using SDI to integrate deficit irrigation will not increase soil water extraction from deep soil layers, but it will increase output by promoting crop development (Yang et al., 2020). Subsurface drip irrigation has a preventive impact on both grain and straw yield in wheat crops, leading to a greater yield (~7%) than flood irrigation of CT-based management methods.
In our study, sustainable intensification of RW with mung bean integration improved the system productivity by ~26% (3-year mean) with SDI compared to the farmer’s practice of conventional RW system (9.38 Mg ha−1) in the IGP (Table 5). Jat et al. (2019b, 2021b) also reported similar findings with mung bean integration in the RW system under silty loam soils of IGP. Kakraliya et al. (2021) compiled the data of many researchers and estimated an increase of 10–15% higher system yield under CA-based SDI than the RW system with flood irrigation.
4.2 Economic profitability
Conventional management-based RW systems (Sc1 and Sc2) led to higher cost of cultivation and lower profitability than the CA-based flood and SDI scenarios (Sc3–Sc8) in both rice and wheat during all the 3 years (Table 5). Consistent with our study, Gathala et al. (2015), Kakraliya et al. (2018), and Jat et al. (2019b) observed that adopting CA techniques through ZT reduced 79–85% of the production cost compared to CT systems of manually transplanted rice and broadcasted wheat. The additional findings (Nagaraju et al., 2014; Choudhary et al., 2018; Kumar et al., 2018; Jat et al., 2019c) in NW India are consistent with the lower cost and higher profitability of production in the CA-based system than CT. The higher profitability under CA-based scenarios with SDI including summer mung bean (Sc7 and Sc8) was mainly due to the combined effect of fertilizer costs and lesser amount of irrigation that improved the crop profitability by ~50% through mung bean than CT management practices (Sc1 and Sc2). In earlier studies, Sivanappan (1994) and Sharda et al. (2017) on rice and Sidhu et al. (2019) and Jat et al. (2019b, 2021b) on rice and wheat reported higher profit under SDI than with flood irrigation.
4.3 Irrigation water use, efficiency, and productivity
The amounts of irrigation water applied in CA-based scenarios with flood irrigation (Sc3 and Sc4) were lower compared to CT-based scenarios (Sc1 and Sc2) (Table 7). It was mostly because of differences in irrigation scheduling and the availability of water and nutrients to crop plants under CT and ZT conditions (Ram et al., 2013; Kakraliya et al., 2018; Kumar et al., 2018; Jat et al., 2018b; Sidhu et al., 2019). However, the SDI system saved more irrigation water compared to CT and ZT in flooded conditions of rice and wheat as we are directing the water as per need to achieve ‘Per Drop More Crop’. In a similar vein, several studies by Jat et al. (2019c) found irrigation water savings using CA methods including SDI under the RW system as compared to CA with flood irrigation in similar ecologies. Lower irrigation water use in CA with flood and SDI conditions with higher or at par crop yields resulted in higher WPI in rice and wheat than the flood irrigation system under conventional tillage (Sc1) during all the years of experimentation. Jat et al. (2019c), Ramulu et al. (2016), and Sharda et al. (2017) also recorded similar water savings of 40–50% and higher WP of 90% with drip irrigation in DSR than PTR. Due to higher irrigation rates than the soil’s ability to store water, flood irrigation causes water delivered to the field at a depth of 5–8 cm (in each event) to be more susceptible to evaporation and percolation losses (Ayars et al., 1999; Sidhu et al., 2019).
In the SDI system, CA-based scenarios were found to have significantly higher WPI and WUE during all the 3 years than CT-based Sc1 (Figure 2). When irrigation water was given by SDI in modest amounts (1–2 cm ha−1) and directly to the root zone of the crop at regular intervals (2–5 days) depending on the tensiometer readings conserved more water by reducing evaporation and seepage losses (Jat et al., 2019a). The SDI enables consistent soil moisture and nitrogen delivery in the root zone of both crops (rice and wheat). Some researchers (Camp, 1998; Chen et al., 2015; Chouhan et al., 2015; Sidhu et al., 2019; Jat et al., 2019b) also reported similar results of irrigation water saving and higher WP. The SDI system benefits the farmers by boosting the efficiency of water through significant savings of irrigation water with comparable or higher yields of cereal-based systems (Nagaraju et al., 2014; Sidhu et al., 2019). The SDI system has limited adoption in the IGP due to free power for groundwater pumping, greater initial investment rather than flood systems, fine-tuning in drip line spacing and depth (Chen et al., 2015), and light soil texture.
4.4 Fertilizer-N nutrition index and efficiency
The real gain of N use and differences in grain yield can be calculated using both the factor of nitrogen nutrition index (NNI) (Lemaire and Ciampitti, 2020; Zhao et al., 2022) and the partial factor productivity of nitrogen (PFPN) (Figure 4). To minimize the N losses through leaching, volatilization and denitrification were managed by the placement and timing of fertilizer N in tiny doses and with more splits through SDI utilizing fertigation to meet the crop need and enhanced NNI, as well as its efficiency in RW system. However, the NNI and PFPN are helpful to better assess the N requirement and optimize crop N management for increasing NUE. In our study, N was tested in 8 and 5 equal splits dose in rice and wheat at 10- to 15-day intervals in the SDI system which parallel to that of 3 and 2 splits dose under flood irrigation system, respectively. The higher efficiency of nitrogen under CA-based systems was mainly due to the proper N doses and scheduling which resulted in saving of approximately 10% nitrogen fertilizer in both rice and wheat compared to CT-based flood irrigation systems (Jat et al., 2019c). Some researchers showed that the application of fertilizer N through SDI saved ~50% of the recommended fertilizer N in rice over the flood irrigation system (Nagaraju et al., 2014). Sidhu et al. and Jat et al. (2019c) have reported a significant increase in N use efficiency under the SDI system compared to flood irrigation in the RW system.
4.5 Energy use efficiency
Energy use efficiency (EUE) was higher under the CA-based management system with SDI (Sc6 and Sc8) due to lower energy input because of less tillage, fertilizer, irrigation, and similar or higher energy output as than the rest of the scenarios (Table 7). Lower EUE in the CT rice–wheat system was mostly caused by more extensive tillage techniques, which account for 33–40% of the total energy used and can only be avoided by ZT without reducing crop yields. Many researchers have found similar energy-saving figures for CA-based management systems in the RW systems (Choudhary et al., 2018; Kumar et al., 2018; Jat et al., 2019c). Laik et al. (2015) found comparable EUE values in the RW system of the IGP, which lie between 3.94 and 1.31 MJ MJ−1. Compared to CT-based scenarios, CA-based scenarios (Sc3–Sc8) resulted in reduced input and higher output of energy, which enhanced the EUE in the RW system (Sc1–Sc2). The same CA-based management strategies have been detailed by Laik et al. (2015), Chaudhary et al. (2017), Parihar et al. (2017), and Kakraliya et al. (2022) as being able to minimize energy input while improving energy output.
5 Conclusion
In the current scenario of declining groundwater table and weather abnormalities, developing effective and scalable techniques for rice cultivation is of utmost importance in the RW domain. In comparison with farmers’ practices, the SDI under CA-based management systems with mung bean integration enhanced crop productivity and economic profitability by 22 and 41%, respectively while using 61% less irrigation water (FP). The RW system with CA in a flooded condition also recorded 7 and 11% higher productivity and profitability, respectively, irrespective of the conventional RW system. The mung bean integration in RW systems contributed ~26% to the systems’ productivity and profitability irrespective of different management practices. Applied fertilizer N through SDI in the CA-based systems increased ~28% partial factor productivity of N and improved N nutrition index (NNI) value through proper N doses and timely scheduling which helped in reducing the N losses during fertigation under the SDI system in CA-based RW system. On a system basis, CA with SDI improved the energy use efficiency (EUE) by ~70% compared to farmers’ practice. This study clearly demonstrates that bundling of CA-based management practices allowed early wheat planting which can enhance wheat yields in NW India by mitigating the negative effects of terminal heat stress compared to when wheat is sown in mid-November under CT-based practices. The ZT direct seeded rice appears to be an economically viable alternative to PTR to overcome the emerging problems of labor, water scarcity, and rising production cost. Irrigation water and energy use declined significantly with ZT DSR compared to conventional rice. Despite these advantages of ZTDSR, however, there are some concerns about its low yields and sustainability, and this calls for additional targeted research to understand the processes contributing to this decline. Current water and energy pricing policies as well as the public procurement system for rice (i.e., provision of a stable market) favor transplanted rice cultivation. These results provide strong evidence that the dual goals of enhancing productivity and profitability of cereal-based cropping systems in NW India can be reliably achieved through proper bundling of CA principles with precise water and nutrient management and through the adoption of efficient genotypes in the RW system.
Data availability statement
The original contributions presented in the study are included in the article/Supplementary material, further inquiries can be directed to the corresponding author.
Author contributions
MK: Data curation, Formal analysis, Methodology, Writing – original draft, Writing – review & editing. HJ: Conceptualization, Data curation, Resources, Supervision, Writing – original draft, Writing – review & editing. SK: Data curation, Formal analysis, Methodology, Supervision, Writing – review & editing. SKK: Data curation, Formal analysis, Investigation, Methodology, Writing – review & editing. MGo: Formal analysis, Investigation, Writing – review & editing. TP: Formal analysis, Investigation, Writing – review & editing. SaK: Data curation, Formal analysis, Software, Supervision, Validation, Writing – review & editing. MC: Formal analysis, Resources, Supervision, Visualization, Writing – review & editing. MGa: Formal analysis, Funding acquisition, Resources, Supervision, Writing – review & editing. PS: Conceptualization, Resources, Supervision, Visualization, Writing – review & editing. MJ: Conceptualization, Methodology, Project administration, Resources, Supervision, Visualization, Writing – review & editing.
Funding
The author(s) declare financial support was received for the research, authorship, and/or publication of this article. The authors acknowledge the support received from the ICAR-CSSRI, Karnal, CIMMYT Academy, and CCS Haryana Agricultural University, Hisar. The research was financed and supported by the CGIAR Research Program (CRPs) on Climate Change, the Agriculture and Food Security (CCAFS), and the Indian Council of Agricultural Research (ICAR) and a grant to CIMMYT for CA research in the country.
Conflict of interest
The authors declare that the research was conducted in the absence of any commercial or financial relationships that could be construed as a potential conflict of interest.
Publisher’s note
All claims expressed in this article are solely those of the authors and do not necessarily represent those of their affiliated organizations, or those of the publisher, the editors and the reviewers. Any product that may be evaluated in this article, or claim that may be made by its manufacturer, is not guaranteed or endorsed by the publisher.
Supplementary material
The Supplementary material for this article can be found online at: https://www.frontiersin.org/articles/10.3389/fsufs.2024.1292284/full#supplementary-material
References
Argiro, V., Strapatsa, A., George, D., and Nanos, A.Constantinos (2006). Energy flow for integrated apple production in Greece. Agric. Ecosyst. Environ. 116, 176–180. doi: 10.1016/j.agee.2006.02.003
Ayars, J. E., Phene, C. J., Hutmacher, R. B., Davis, K. R., Schoneman, R. A., Vail, S. S., et al. (1999). Subsurface drip irrigation of row crops: a review of 15 years of research at the water management research laboratory. Agric. Water Manag. 42, 1–27. doi: 10.1016/S0378-3774(99)00025-6
Bhandari, A. L., Ladha, J. K., Pathak, H., Padre, A. T., Dawe, D., and Gupta, R. K. (2002). Yield and soil nutrient changes in a long-term rice–wheat rotation in India. Soil Sci. Soc. Am. J. 66, 162–170. doi: 10.2136/sssaj2002.1620a
Bhatt, R., Hossain, A., Busari, M. A., and Meena, R. S. (2020). “Water footprints in rice-based systems of South Asia” in Footprints in agro ecosystem. eds. A. Barnarjee, R. S. Meena, and M. K. Jhariya (Singapore: Springer), 272–308.
Bhatt, R., Singh, P., Hossain, A., and Timsina, J. (2021). Rice–wheat system in the northwest indo-Gangetic plains of South Asia: issues and technological interventions for increasing productivity and sustainability. Paddy Water Environ. 19, 345–365. doi: 10.1007/s10333-021-00846-7
Camp, C. R. (1998). Sub-surface drip irrigations: a view. Trans. ASAE 41, 1353–1367. doi: 10.13031/2013.17309
Chaudhary, V. P., Singh, K. K., Pratibha, G., Bhattacharyya, R., Shamim, M., Srinivas, I., et al. (2017). Energy conservation and greenhouse gas mitigation under different production systems in rice cultivation. Energy 130, 307–317. doi: 10.1016/j.energy.2017.04.131
Chen, R., Cheng, W., Liao, J., Fan, H., Zheng, Z., and Ma, F. (2015). Lateral spacing in drip-irrigated wheat: the effects on soil moisture, yield, and water use efficiency. Field Crop Res. 179, 52–62. doi: 10.1016/j.fcr.2015.03.021
Chopra, S. L., and Kanwar, J. S. (1991). Analytical agriculture chemistry. 4th Edn, Kalyani Publishers: New Delhi, pp.301.
Choudhary, K. M., Jat, H. S., Nandal, D. P., Bishnoi, D. K., Sutaliya, J. M., Choudhary, M., et al. (2018). Evaluating alternatives to rice-wheat system in western indo-Gangetic Plains: crop yields, water productivity and economic profitability. Field Crop Res. 218, 1–10. doi: 10.1016/j.fcr.2017.12.023
Chouhan, S. S., Awasthi, M. K., and Nema, R. K. (2015). Studies on water productivity and yields responses of wheat based on drip irrigation systems in clay loams soil. Indian J. Sci. Technol. 8, 650–654. doi: 10.17485/ijst/2015/v8i7/64495
Fischer, G., Tubiello, F. N., Van Velthaizen, H., and Wiberg, D. A. (2007). Climate change impacts on irrigation water requirements: effects of mitigation, 1990–2080. Technol. Forecast. Soc. Change 74, 1083–1107. doi: 10.1016/j.techfore.2006.05.021
Gao, Y., Yang, L., Shen, X., Li, X., Sun, J., Duan, A., et al. (2014). Winter wheat with subsurface drip irrigation (SDI): crop coefficients, water-use estimates, and effects of SDI on grain yield and water use efficiency. Agric. Water Manag. 146, 1–10. doi: 10.1016/j.agwat.2014.07.010
Gathala, M. K., Kumar, V., Sharma, P. C., Saharawat, Y. S., Jat, H. S., Singh, M., et al. (2013). Optimizing intensive cereal based cropping systems addressing current and future drivers of agricultural change in the north-western indo-Gangetic plains of India. Agric. Ecosyst. Environ. 177, 85–97. doi: 10.1016/j.agee.2013.06.002
Gathala, M. K., Ladha, J. K., Kumar, V., Saharawat, Y. S., Kumar, V., Sharma, P. K., et al. (2011). Tillage and crop establishment affects sustainability of south Asian rice–wheat system. J. Agron. 103, 961–971. doi: 10.2134/agronj2010.0394
Gathala, M. K., Timsina, J., Islam, M. S., Krupnik, T. J., Bose, T. R., Islam, N., et al. (2016). Productivity, profitability, and energetics: a multi-criteria assessment of farmers’ tillage and crop establishment options for maize in intensively cultivated environments of South Asia. Field Crop Res. 186, 32–46. doi: 10.1016/j.fcr.2015.11.008
Gathala, M. K., Timsina, J., Islam, S., Rahman, M., Hossain, I., Harun-Ar-Rashid, M., et al. (2015). Conservation agriculture-based tillage and crop establishment options can maintain farmers’ yields and increase profits in South Asia’s rice–maize systems: evidence from Bangladesh. Field Crop Res. 172, 85–98. doi: 10.1016/j.fcr.2014.12.003
Gomez, K. A., and Gomez, A. A. (1984). Statistical procedures for agricultural research. Hoboken, NJ: John Wiley & Sons.
Haynes, R. J. (1993). Effect of sample pre-treatment on aggregate stability measured by wet sieving or turbidimetry on soils of different cropping history. J. Soil Sci. 44, 261–270. doi: 10.1111/j.1365-2389.1993.tb00450.x
He, J., Ma, J., Cao, Q., Wang, X., Yao, X., Cheng, T., et al. (2022). Development of critical nitrogen dilution curves for different leaf layers within the rice canopy. Eur. J. Agron. 132:126414. doi: 10.1016/j.eja.2021.126414
Humphreys, E., Kukal, S. S., Christen, E. W., Hira, G. S., Balwinder, S., Yadav, S., et al. (2010). Halting the groundwater decline in north-West India-which crop technologies will be winners. Adv. Agron. 109, 155–217. doi: 10.1016/B978-0-12-385040-9.00005-0
Jackson, M. L. (1973). Soil chemical analysis, 2nd Edn. Prentice Hall of India Pvt. Ltd: New Delhi, pp. 498.
Jat, H. S., Datta, A., Choudhary, M., Sharma, P. C., and Jat, M. L. (2021a). Conservation agriculture: factors and drivers of adoption and scalable innovative practices in indo- Gangetic plains of India–a review. Int. J. Agric. Sustain. 19, 40–55. doi: 10.1080/14735903.2020.1817655
Jat, H. S., Datta, A., Choudhary, M., Sharma, P. C., Yadav, A. K., Choudhary, V., et al. (2019a). Climate smart agriculture practices improve soil organic carbon pools, biological properties and crop productivity in cereal-based systems of north-West India. Catena 181:104059. doi: 10.1016/j.catena.2019.05.005
Jat, H. S., Datta, A., Sharma, P. C., Kumar, V., Yadav, A. K., Choudhary, M., et al. (2018a). Assessing soil properties and nutrient availability under conservation agriculture practices in a reclaimed sodic soil in cereal-based systems of north-West India. Arch. Agron. Soil Sci. 64, 531–545. doi: 10.1080/03650340.2017.1359415
Jat, R. D., Jat, H. S., Nanwal, R. K., Yadav, A. K., Bana, A., Choudhary, K. M., et al. (2018b). Conservation agriculture and precision nutrient management practices in maize-wheat system: effects on crop and water productivity and economic profitability. Field Crop Res. 222, 111–120. doi: 10.1016/j.fcr.2018.03.025
Jat, H. S., Jat, R. K., Sing, Y., Parihar, C. M., Jat, S. L., Tetarwal, J. P., et al. (2016). Nitrogen management under conservation agriculture in cereal-based systems. Indian J. Ferti. 12, 76–91.
Jat, H. S., Kumar, V., Kakraliya, S. K., Abdallah, A. M., Datta, A., Choudhary, M., et al. (2021b). Climate-smart agriculture practices influence weed density and diversity in cereal-based Agri-food systems of western indo-Gangetic plains. Sci. Rep. 11, 15901–15915. doi: 10.1038/s41598-021-95445-1
Jat, H. S., Sharma, P. C., Datta, A., Choudhary, M., Kakraliya, S. K., Singh, Y., et al. (2019b). Re-designing irrigated intensive cereal systems through bundling precision agronomic innovations for transitioning towards agricultural sustainability in north-West India. Sci. Rep. 9, 17929–17914. doi: 10.1038/s41598-019-54086-1
Jat, R. K., Singh, R. G., Gupta, R. K., Gill, G., Chauhan, B. S., and Pooniya, V. (2019c). Tillage, crop establishment, residue management and herbicide applications for effective weed control in direct seeded rice of eastern indo–Gangetic Plains of South Asia. Crop Prot. 123, 12–20. doi: 10.1016/j.cropro.2019.05.007
Justes, E., Mary, B., Meynard, J. M., Machet, J. M., and Thelier-Huché, L. (1994). Determination of a critical nitrogen dilution curve for winter wheat crops. Ann. Bot. 74, 397–407. doi: 10.1006/anbo.1994.1133
Kakraliya, M., Jat, H. S., Kumar, S., Sidhu, H. S., and Sharma, P. C. (2021). Influence of subsurface drip irrigation in rice-wheat system on water saving, crop productivity and profitability under conservation agriculture-based system. Extended summaries: 5th international agronomy congress, Hyderabad, India, pp. 397–398.
Kakraliya, S. K., Jat, H. S., Singh, I., Gora, M. K., Kakraliya, M., Bijarniya, D., et al. (2022). Energy and economic efficiency of climate-smart agriculture practices in a rice–wheat cropping system of India. Sci. Rep. 12:8731. doi: 10.1038/s41598-022-12686-4
Kakraliya, S. K., Jat, H. S., Singh, I., Sapkota, T. B., Singh, L. K., Sutaliya, J. M., et al. (2018). Performance of portfolios of climate smart agriculture practices in a rice-wheat system of Western indo-Gangetic plains. Agric. Water Manag. 202, 122–133. doi: 10.1016/j.agwat.2018.02.020
Kumar, N., Chhokar, R. S., Meena, R. P., Kharub, A. S., Gill, S. C., Tripathi, S. C., et al. (2021). Challenges and opportunities in productivity and sustainability of rice cultivation system: a critical review in Indian perspective. Cereal Res. Commun., 1–29. doi: 10.1007/s42976-021-00214-5
Kumar, V., Jat, H. S., Sharma, P. C., Gathala, M. K., Malik, R. K., Kamboj, B. R., et al. (2018). Can productivity and profitability be enhanced in intensively managed cereal systems while reducing the environmental footprint of production? Assessing sustainable intensification options in the breadbasket of India. Agric. Ecosyst. Environ. 252, 132–147. doi: 10.1016/j.agee.2017.10.006
Kumar, V., and Ladha, J. K. (2011). Direct seeding of rice: recent developments and future research needs. Adv. Agron. 111, 29z–413z. doi: 10.1016/B978-0-12-387689-8.00001-1
Ladha, J. K., Dawe, D., Pathak, H., Padre, A. T., Yadav, R. L., Singh, B., et al. (2003a). How extensive are yield declines in long-term rice–wheat experiments in Asia? Field Crop Res. 81, 159–180. doi: 10.1016/S0378-4290(02)00219-8
Ladha, J. K., Singh, Y., Erenstein, O., and Hardy, B. (2009). Integrated crop and resource Management in the Rice–Wheat Systems of South Asia. International Rice Research Institute: Los Banos, Philippines, pp. 1–395.
Laik, R., Sharma, S., Idris, M., Singh, A. K., Singh, S. S., Bhatt, B. P., et al. (2015). Integration of conservation agriculture with best management practices for improving system performance of the rice-wheat rotation in the eastern indo-Gangetic Plains of India. Agric. Ecosyst. Environ. 195, 68–82. doi: 10.1016/j.agee.2014.06.001
Lemaire, G., and Ciampitti, I. (2020). Crop mass and N status as prerequisite Covariables for unraveling nitrogen use efficiency across genotype-by-environment-by-management scenarios: a review. Plan. Theory 9:1309. doi: 10.3390/-plants9101309
MacDonald, A. M., Bonsor, H. C., Ahmed, K. M., Burgess, W. G., Basharat, M., Calow, R. C., et al. (2016). Groundwater quality and depletion in the indo-Gangetic Basin mapped from in situ observations. Nat. Geo sci. 9, 762–766. doi: 10.1038/ngeo2791
Mohammad, A. Z. (2015). Effects of trickle irrigation system from southern Iran District Chabahar free zone. Irrig. Drain. Syst. Engin. 4:152. doi: 10.4172/2168-9768.1000152
Mukherjee, A., Saha, D., Harvey, C. F., Taylor, R. G., Ahmed, K. M., and Bhanja, S. N. (2015). Groundwater systems of the Indian sub-continent. J. Hydrol. Reg. Stud. 4, 1–14. doi: 10.1016/j.ejrh.2015.03.005
Nagaraju, S., Anusha Gururaj, K., Rekha, B., Sheshadri, T., and Shankar, M. A. (2014). Drip irrigation and fertigation in direct seeded aerobic rice in Karnataka, India: research status. 4th international Rice congress Ċ, 7 workshop, Bangkok, pp. 8–9.
Nawaz, A., Farooq, M., Nadeem, F., Siddique, K. H. M., and Lal, R. (2019). Rice-wheat cropping systems in South Asia: issues, options and opportunities. Crop Pasture Sci. 70, 395–427. doi: 10.1071/CP18383
Olsen, B. C., Cole, C. V., Watenabe, F. S., and Dean, L. A. (1954). Estimation of available phosphorus by extraction with sodium carbonate. USDA Circular No. 939:19.
Ozkan, B., Akcaoz, H., and Fert, C. (2004). Energy requirement input-output analysis in Turkish agriculture. Renew. Energ. 29, 39–51. doi: 10.1016/S0960-1481(03)00135-6
Parihar, C. M., Jat, S. L., Singh, A. K., Majumdar, K., Jat, M. L., Saharawat, Y. S., et al. (2017). Bioenergy, biomass water-use efficiency and economics of maize-wheat mungbean system under precision-conservation agriculture in semi-arid agro-ecosystem. Energy 119, 245–256. doi: 10.1016/j.energy.2016.12.068
Parthasarathi, T., Vanitha, K., Mohandass, S., and Vered, E. (2018). Evaluation of drip irrigation system for water productivity and yield of rice. Agron. J. 110, 2378–2389. doi: 10.2134/agronj2018.01.0002
PIB . (2021). Fourth advance estimates of production of foodgrains for 2020–21. Available at: https://static.pib.gov.in/WriteReadData/specificdocs/documents/2021/aug/doc202181121.pdf (Accessed 20 Sep 2021)
Ram, H., Dadhwal, V., Vashist, K. K., and Kaur, H. (2013). Grain yield and water use efficiency of wheat (Triticum aestivum L.) in relation to irrigation levels and rice straw mulching in north West India. Agric. Ecosyst. Environ. 128, 92–101. doi: 10.1016/j.agwat.2013.06.011
Ramulu, V., Praveen Rao, V., Uma Devi, M., Avil Kumar, K., and Radhika, K. (2016). Evaluation of drip irrigation and fertigation levels in aerobic rice for higher water productivity. 2nd world irrigation forum (WIF2), 6–8 November, 2016, Chiang Mai, Thailand.
Richards, L. A. (1954). Diagnosis and improvement in saline, alkali soils. Soil Sci. 78:154. doi: 10.1097/00010694-195408000-00012
Rodell, M., Velicogna, I., and Famiglietti, J. S. (2009). Satellite-based estimates of groundwater depletion in India. Nature 460, 999–1002. doi: 10.1038/nature08238
Saharawat, Y. S., Ladha, J. K., Pathak, H., Gathala, M., Chaudhary, N., and Jat, M. L. (2012). Simulation of resource-conserving technologies on productivity, income and greenhouse gas GHG emission in rice-wheat system. J. Soil Sci. Environ. Manag. 3, 9–22. doi: 10.5897/JSSEM11.108
Sandhu, O. S., Gupta, R. K., Thind, H. S., Jat, M. L., and Sidhu, H. S. (2019). Drip irrigation and nitrogen management for improving crop yields, nitrogen use efficiency and water productivity of maize-wheat system on permanent beds in North-West India. Agric. Water Manag. 219, 19–26. doi: 10.1016/j.agwat.2019.03.040
Sarkar, R. K., Sanjukta, D., and Das, S. (2003). Yield of rainfed lowland rice with medium water depth under anaerobic direct seeding and transplanting. Trop. Sci. 43, 192–198. doi: 10.1002/ts.117
SAS Institute . (2001). SAS/STAT User’s guide. Version 8–1; SAS Inst Cary, NC, USA. Available at: http://stat.iasri.res.in/sscnarsportal
Sekhri, S. (2013). Sustaining groundwater: role of policy reforms in promoting conservation in India. Shekhar Shah Barry Bosworth Arvind Panagariya 149, 149–187.
Sharda, R., Mahajan, G., Siag, M., Singh, A., and Chauhan, B. S. (2017). Performance of drip-irrigated dry-seeded rice (Oryza sativa L.) in South Asia. Paddy Water Environ. 15, 93–100. doi: 10.1007/s10333-016-0531-5
Sidhu, H. S., Jat, M. L., Singh, Y., Sidhu, R. K., Gupta, N., Singh, P., et al. (2019). Sub-surface drip fertigation with conservation agriculture in a rice-wheat system: a breakthrough for addressing water and nitrogen use efficiency. Agric. Water Manag. 216, 273–283. doi: 10.1016/j.agwat.2019.02.019
Singh, Y., Kukal, S. S., Jat, M. L., and Sidhu, H. S. (2014). Improving water productivity of wheat-based cropping systems in South Asia for sustained productivity. Adv. Agron. 127, 157–258. doi: 10.1016/B978-0-12-800131-8.00004-2
Singh, B., Shan, Y. H., Johnson-Beebout, S. E., Singh, Y., and Buresh, R. J. (2008). Crop residue management for lowland rice-based cropping systems in Asia. Adv. Agron. 98, 117–199. doi: 10.1016/S0065-2113(08)00203-4
Sivanappan, R. K. (1994). Prospects of micro-irrigation in India. Irri. Drain. Syst. 8, 49–58. doi: 10.1007/BF00880798
Skaggs, T. H., Trout, T. J., Simunek, J., and Shouse, P. G. (2004). Comparison of HYDRUS-2D simulations of drip irrigation with experimental observations. J. Irri. Drain. Engine. 130, 304–310. doi: 10.1061/(ASCE)0733-9437(2004)130:4(304)
Subbiah, B. V., and Asija, G. L. (1956). A rapid procedure for the estimation of available nitrogen in soils. Curr. Scie. 25, 259–260.
Teri Report . (2022). Water-key facts for its sustainable management in India. World sustainable development summit 2022: towards a resilient planet. The Energy and Resources Institute: New Delhi.
Tripathi, S. C., and Das, A. (2017). Bed planting for resource conservation, diversification and sustainability of wheat based cropping system. J. Wheat Res. 9, 1–11. doi: 10.25174/2249-4065/2017/62285
Umair, M., Hussain, T., Jiang, H., Ahmad, A., Yao, J., Qi, Y., et al. (2019). Water-saving potential of subsurface drip irrigation for winter wheat. Sustain. For. 11:2978. doi: 10.3390/su11102978
UNICEF Report . (2021). One in five children globally does not have enough water to meet their everyday needs-Unicef. New York. Available at: https://www.unicef.org/press-releases/one-five-children-globally-does-not-have-enough-water-meet-their-everyday-needs (Accessed March 18, 2021)
Van Dijk, W. M., Densmore, A. L., Singh, A., Gupta, S., Sinha, R., Mason, P. J., et al. (2016). Linking the morphology of fluvial fan systems to aquifer stratigraphy in the Sutlej-Yamuna plain of Northwest India. J. Geophys. Res. Earth Surf. 121, 201–222. doi: 10.1002/2015JF003720
Walkley, A., and Black, C. A. (1934). An examination of the method for determination of soil organic matter and proposed medication at the chromic acid titration method. Soil Sci. 37, 29–38. doi: 10.1097/00010694-193401000-00003
Yadav, S., Gill, G., Humphreys, E., Kukal, S. S., and Wallia, U. S. (2011). Effect of water management on dry seeded and puddled transplanted rice part-1. Field Crop Res. 120, 112–122. doi: 10.1016/j.fcr.2010.09.002
Yang, M. D., Leghari, S. J., Guan, X. K., Ma, S. C., Ding, C. M., Mei, F. J., et al. (2020). Deficit subsurface drip irrigation improves water use efficiency and stabilizes yield by enhancing subsoil water extraction in winter wheat. Front. Plant Sci. 11:508. doi: 10.3389/fpls.2020.00508
Keywords: conservation agriculture, direct seeded rice, subsurface drip irrigation, system irrigation and water productivity, economic profitability, energy and nitrogen efficiency
Citation: Kakraliya M, Jat HS, Kumar S, Kakraliya SK, Gora MK, Poonia T, Kumar S, Choudhary M, Gathala MK, Sharma PC and Jat ML (2024) Bundling subsurface drip irrigation with no-till provides a window to integrate mung bean with intensive cereal systems for improving resource use efficiency. Front. Sustain. Food Syst. 8:1292284. doi: 10.3389/fsufs.2024.1292284
Edited by:
Emmanuel Donkor, Humboldt University of Berlin, GermanyReviewed by:
Vinay Sindhu, Punjab Agricultural University, IndiaArpna Bajpai, Krishi Vigyan Kendra (Madhya Pradesh), India
Copyright © 2024 Kakraliya, Jat, Kumar, Kakraliya, Gora, Poonia, Kumar, Choudhary, Gathala, Sharma and Jat. This is an open-access article distributed under the terms of the Creative Commons Attribution License (CC BY). The use, distribution or reproduction in other forums is permitted, provided the original author(s) and the copyright owner(s) are credited and that the original publication in this journal is cited, in accordance with accepted academic practice. No use, distribution or reproduction is permitted which does not comply with these terms.
*Correspondence: Hanuman S. Jat, hsjat_agron@yahoo.com