- 1Wageningen Economic Research, Wageningen University and Research, Wageningen, Netherlands
- 2Wageningen Livestock Research, Wageningen University and Research, Wageningen, Netherlands
- 3Kenya Marine and Fisheries Research Institute, National Aquaculture Research and Development Centre, Sagana, Kenya
- 4Bioprocess Engineering, AlgaePARC, Wageningen University, Wageningen, Netherlands
- 5Wageningen Marine Research, Wageningen University and Research, IJmuiden, Netherlands
Hunger affected 278 million people in Africa in 2021, and in Kenya, the proportion of the population unable to afford a healthy diet is 81%. To combat hunger and increase resilience throughout the food system, spirulina (Arthrospira platensis) has been shown to have remarkable properties. The main aim of this study is to investigate whether protein from spirulina is a suitable alternative to protein sourced from fish meal in fish feed for juvenile Nile tilapia (Oreochromis niloticus). In a trial investigating the use of spirulina biomass for fish feed on juvenile Nile tilapia, it has been proven that tilapia fingerlings fed diets with spirulina, replacing up to 30% of fish meal protein, were not affected in terms of growth performance compared to the fish fed the commercial diet. This shows that, nutritionally, protein from spirulina can replace fish meal protein fed to juvenile Nile tilapia. This offers promising opportunities for enhanced food and nutrition security. If produced affordably, spirulina can have a significant impact on the income level of low-income fish farmers in Kenya, who often spend more than 50% of their income on commercial fish feed. Affordability can be made possible if small-scale farmers can produce spirulina using animal manure that is available on the farm instead of using highly priced commercial fertilizer. The additional environmental benefits of spirulina include carbon dioxide sequestration and the removal of nitrogenous and phosphorous waste from the wastewater. Spirulina can therefore increase the sustainability of a whole food system.
1 Introduction
In 2021, hunger affected 278 million people in Africa, and it has been estimated that nearly 670 million people worldwide will still be undernourished in 2030 (FAO, IFAD, UNICEF, WFP, WHO, 2022). For developing countries, accomplishing food and nutrition security for all is a major challenge (Graef et al., 2014; Müller et al., 2020). Food security is a function of food availability, food accessibility, food stability, and food utilization (Ziervogel and Ericksen, 2010). A food-secure situation is accomplished through access to sufficient, safe, and nutritious food to maintain a healthy and active life for all people at all times (FAO, 1996). In Kenya, the proportion of people unable to afford a healthy diet is 81% (FAO, IFAD, UNICEF, WFP, WHO, 2022).
The challenges of combating hunger are complex. Transformation of agriculture and food systems in line with the Sustainable Development Goals (SDGs) (Herrero et al., 2021) has become a commonly agreed upon imperative (Eyhorn et al., 2019; Niggli et al., 2023), including SDG1: No poverty; SDG2: Zero hunger; SDG6: Clean water and sanitation; and SDG12: Responsible consumption and production. A food system approach is applicable to transition pathways aiming for the SDGs because it conceptually explains how socioeconomic and climate factors operate as drivers within a food system, for which multiple (biophysical, economic, political, and social) factors and activities relate to the production, processing, distribution, preparation, and consumption of food and the outcomes of these activities (HLPE, 2014).
Fish farming and the demand for fish feed are growing rapidly worldwide (Karimi et al., 2021; Boyd et al., 2022; Rashidian et al., 2023). Fish is very dense in protein and contains important fatty acids that are essential to human health (Alp Erbay and Yeşilsu, 2021; Rasul et al., 2021). Aquaculture production in Kenya was at 21,076 MT in 2021 (KeFS, 2022), representing only 15% of the total fish production in Kenya, while per capita fish consumption is still at 4.5 kg per year, which is far below the global annual average of 13.3 kg per capita (Troell et al., 2023). Aquaculture production is affected by the high cost of feeds due to the high and rising costs of fish meal among other conventional protein sources such as soybean meal (Shati et al., 2022). Proteins are the most expensive feed components, and cheaper alternative protein sources would be desirable to cut down on feeding costs and make fish more affordable to low-income populations. For further sustainable growth, the fish feed industry is looking for alternative protein sources that are sustainable and face less competition from other sectors (Salter and Lopez-Viso, 2021). Among these alternatives, microalgae could play an important role due to their high crude protein values (up to 50% dry weight) and their profile of amino acid, which is comparable to that of fish meal. In addition, the presence of high levels of polyunsaturated fatty acids (PUFAs) makes their use in fish feed extremely interesting (Alagawany et al., 2021).
Spirulina is a well-known microalga whose production is mainly focused on human consumption as a nutraceutical (Soni et al., 2017). However, because spirulina contains useful amino acids, minerals, antioxidants, and immune-stimulating properties that can minimize the expense and risk of using medication in aquaculture, it has been acknowledged as a potential sustainable alternative protein source to fish meal in fish nutrition (FAO, 2008; Ungsethaphand et al., 2009; Onura et al., 2022; Al Mamun et al., 2023). Indeed, the benefits of using spirulina in fish feed have been highlighted in recent studies. For instance, improved immunity has been reported in cultured fish when the fish consume spirulina-based diets (Abdel-Tawwab et al., 2021; Shalata et al., 2021; El-Araby et al., 2022; Al Mamun et al., 2023), including immunological responses of Nile tilapia (O. niloticus) to Aeromonas hydrophila infections (Mabrouk et al., 2021). Moreover, the use of spirulina as a feed ingredient has resulted in significant improvements in the growth performance of cultivated fish (Tongsiri et al., 2010; Jahan et al., 2016) and in boosting the reproductive performance of tilapia by increasing the number and size of eggs as well as their hatchability (Wahbi and Sangak, 2017; Joshua and Zulperi, 2020). However, these fish feed trials have mostly been carried out in Asia, with only very few studies showing the effectiveness of spirulina in African countries. This is likely because spirulina is more often used as a human food supplement than as a livestock and fish feed ingredient. The possibility of using spirulina to replace fish meal as a protein source in feed can be an added advantage for rural communities in Africa. By using locally available animal manures, which are affordable, manure production can contribute to reducing natural resource degradation and carbon emissions and enhance sustainability (Soma et al., 2022; Tzachor et al., 2022).
Against this background, in this study, the main aim is to investigate whether protein from spirulina is a suitable alternative to protein from fish meal in fish feed for juvenile Nile tilapia. In particular, we investigate the impacts of replacing parts of commercial feed for juvenile tilapia with spirulina to measure fish growth performance, fish proximate composition, and the fatty acid profile of the whole fish. These trials can be duplicated all over the world.
2 Materials and methods
2.1 Experimental diets and fish stocking
The trial on the use of spirulina biomass as a protein source for fish feed for Nile tilapia fingerlings took place at Kenya Marine and Fisheries Research Institute (KMFRI), Hatchery in Sagana, Kirinyaga county, Kenya, in 2022, in cooperation with Wageningen University & Research (WUR). The dried spirulina powder was brought in from a firm in Portugal (SPAROS LDA). The nutritional composition of this batch of spirulina is provided in Table 1.
Four experimental diets were formulated, of which the protein fraction of the fish meal was replaced by protein stemming from spirulina at 0, 10, 20, and 30%, respectively. In addition to these four experimental feeds, a commercial feed (Skretting® Nutra tilapia 120 of 40% CP, 8% crude fat, 1.2 mm pellet) was used as a reference diet. The experimental diets were formulated and extruded to obtain 1.2 mm pellets at Sparos®, Olhão, Portugal, and shipped to KMFRI, Kenya. The feed was stored in airtight containers at room temperature before feeding. The composition of the feeds was isonitrogenous and isoenergetic based on the composition of the commercial diet (Table 2).
The experimental diets were tested in triplicate, summing up to a total of 15 experimental units with a stocking density of 35 fish/tank and adding up to 525 fish in total. Juvenile Nile tilapia (O. niloticus) (± 2 g) obtained from the KMFRI Sagana hatchery were randomly allocated to the 15 tanks (70 cm x 47 cm x 30 cm) integrated into a flow-through aquaculture system. During the acclimatization period, the fish were fed the commercial diet (Sketting® Nutra tilapia 120), 1.2 mm pellets. To achieve maximum feed intake after the acclimatization period, the fish were fed to apparent satiation with the experimental diets twice daily, in the morning (10,00 h) and afternoon (16,00 h). During the experiment, fish were held under natural daylight conditions.
2.2 Water quality monitoring
Water quality parameters were measured weekly using a multi-parameter water quality meter model H19828 (Hanna Instruments Ltd., Chicago, United States) and using standard methods (Boyd and Tucker, 1998). Temperature and oxygen were measured daily; pH, Total ammonium nitrogen (TAN), Nitrite (NO2−), and Nitrate (NO3−) content were measured twice a week.
2.3 Fish sampling and calculations
To determine growth performance, the fish were weighed to measure body weight (BW) at the start (BW0), body weight on day 42 (BW42), and body weight at the end of the trial on day 84 = (BWend). The fish were not fed one day prior to weighing. From the individual weight data, average body weight at the start (BW0), at day 42 (BW42), and at the end (BWend) were calculated per tank, which was considered the experimental unit. Growth rates expressed in % BW/d were calculated using the formula: (BW(t)-BW(0))/t, LN(BW(t)) – LN(BW(0))/(t) * 100, with t representing the duration of the growth period and LN the natural logarithm. Until day 42, the apparent feed intake in g/fish was calculated by the sum of the feed given, divided by the number of fish per tank. The tanks were cleaned every 2 days by siphoning out fecal matter and uneaten feed before feeding in the morning. A complete water exchange was carried out during the sampling days. The apparent feed conversion ratio until day 42 (aFCR42) was calculated by dividing the apparent feed intake (aFI42) by weight gain or growth of fish (aFCR = aFI42/WG42). At the end of the trial, the apparent feed intake in g/tank is the sum of the feed given (aFIend). The economic feed conversion rate (eFCR) was calculated by dividing the feed given per tank by biomass gain per tank (Biomass gain = harvest weight-stocking weight).
Growth performance, apparent feed conversion rate, and survival rate were determined at the end of the experiment. The optimal inclusion, i.e., the replacement of fish protein with spirulina protein was calculated based on the performance of the fish in relation to the inclusion of the spirulina in the diet. The optimal level is defined as the maximum inclusion level of spirulina without loss of production performance (growth, feed conversion rate, survival rate) relative to the commercial standard feed.
2.4 Proximate composition analysis of feeds and fish
Feeds and pooled whole fish homogenate samples were analyzed for crude protein, crude lipid, fiber, carbohydrates, ash, and gross energy using the standard methods of the Association of Official Analytical Chemists (AOAC, 1990). Pooled samples of 30 fish were taken from each treatment for analysis. Moisture content was assessed by oven drying at 105°C for 12 h to a constant weight, and ash content was determined by combusting dry samples in a muffle furnace (Thermolyne Corporation, Dubuque, IO, United States) at 550°C for 12 h. Crude protein content (N × 6.25) was determined using a micro-Kjeldahl apparatus (Labconco Corporation, Kansas City, USA), and lipid content was analyzed using a Soxhlet extractor (VELP Scientifica, Milano, Italy). Ash content was evaluated by burning dry samples for 12 h in a muffle furnace (Thermolyne Corporation, Dubuque, IO, United States) at 550°C.
2.5 Extraction of lipids and fatty acid analysis
Lipid extraction was done according to Bligh and Dyer (1959), for which total lipid was extracted from 0.5 g samples of pooled whole fish homogenates from every treatment. The samples were homogenized in 10 mL chloroform/methanol (2:1, v/v) containing 0.01% butylated hydroxytoluene (BHT) as an antioxidant, 2 mL cold isotonic saline, and 0.9% sodium chloride. Homogenates were mixed vigorously and allowed to stand for 20 min before being centrifuged at 3000 rpm for 10 min, and the upper aqueous layer was aspirated before the lower organic/chloroform layer was transferred to a 100 mL reflux flask and evaporated to dryness under vacuum. Fatty acid methyl esters (FAME) were synthesized from extracted total lipid and fatty acid standards using acid-catalyzed transmethylation. In a 50 mL reflux flask, 5 mL of 1% H2SO4 (v/v) in methanol was combined with 1 mL of extracted total lipid and refluxed at 70°C for 3 h. FAME was extracted in 750 mL of distilled water and 10 mL of hexane then dehydrated in anhydrous sodium sulfate (Na2SO4). The extracted FAME was concentrated to 0.5 mL in a vacuum evaporator and put into gas chromatography (GC) before GC analysis.
2.6 Gas chromatography analysis
FAME were separated and quantified by gas chromatography (GC) (Shimadzu Model GC14B, Japan) fitted with on-column injection and equipped with a fused silica capillary column (SUPELCO Column Omega waxtm 530, 30 m x 0.5 mm x 0.5 μm) with nitrogen as carrier gas. Temperature programming was from 170°C to 220°C at 1.8°C min−1, then 220°C for 47 min and a total run time of 75 min. Injection and detection temperatures were 240°C and 260°C, respectively. All GC analyses were performed under the same conditions. Individual methyl esters were identified by comparing the retention times of FAME with the Supelco 37 Component FAME Mix standard (Sigma-Aldrich, Munich, Germany).
2.7 Statistical analyses
The obtained data were analyzed using one-way analysis of variance (ANOVA) to test dietary effects. The Fisher Least Significant Difference (LSD) post hoc test was performed for means comparisons. The significance level was set at p < 0.05.
3 Outcomes of the spirulina feed trial on juvenile Nile tilapia
3.1 Growth performance
Fish growth performance data is presented in Table 3. Fish body weight at the start of the experiment (BWstart) was similar between treatments (p > 0.05). All diets were well eaten by the fish. Apparent feed intakes at day 42 (g/fish) (aFI42) and at the end (g/tank) (aFIend) were similar between treatments (p > 0.05), although numerically, the aFI at the end in g/tank increased with an increasing inclusion level of spirulina. Fish body weight in g at day 42 (BW42) and at the end (BWend) was not affected by diet. Growth was expressed in % BW d−1 at day 42 (SGR42) and at the end (SGRend). Weight gain at day 42 and at the end was not significantly different among the treatments (p > 0.05). Mortality was less than 6% in all the treatments of S0%, S10%, S20%, S30%, and CPEL. The mortality did not affect the economic feed conversion rate (eFCR) at the end. Weight gain at day 42 (WG42) and at the end (WGend), the apparent feed conversion rate at day 42 (aFCR42), biomass gain at the end, and the economic feed conversion rate (eFCR) were all similar across treatments (p > 0.05).
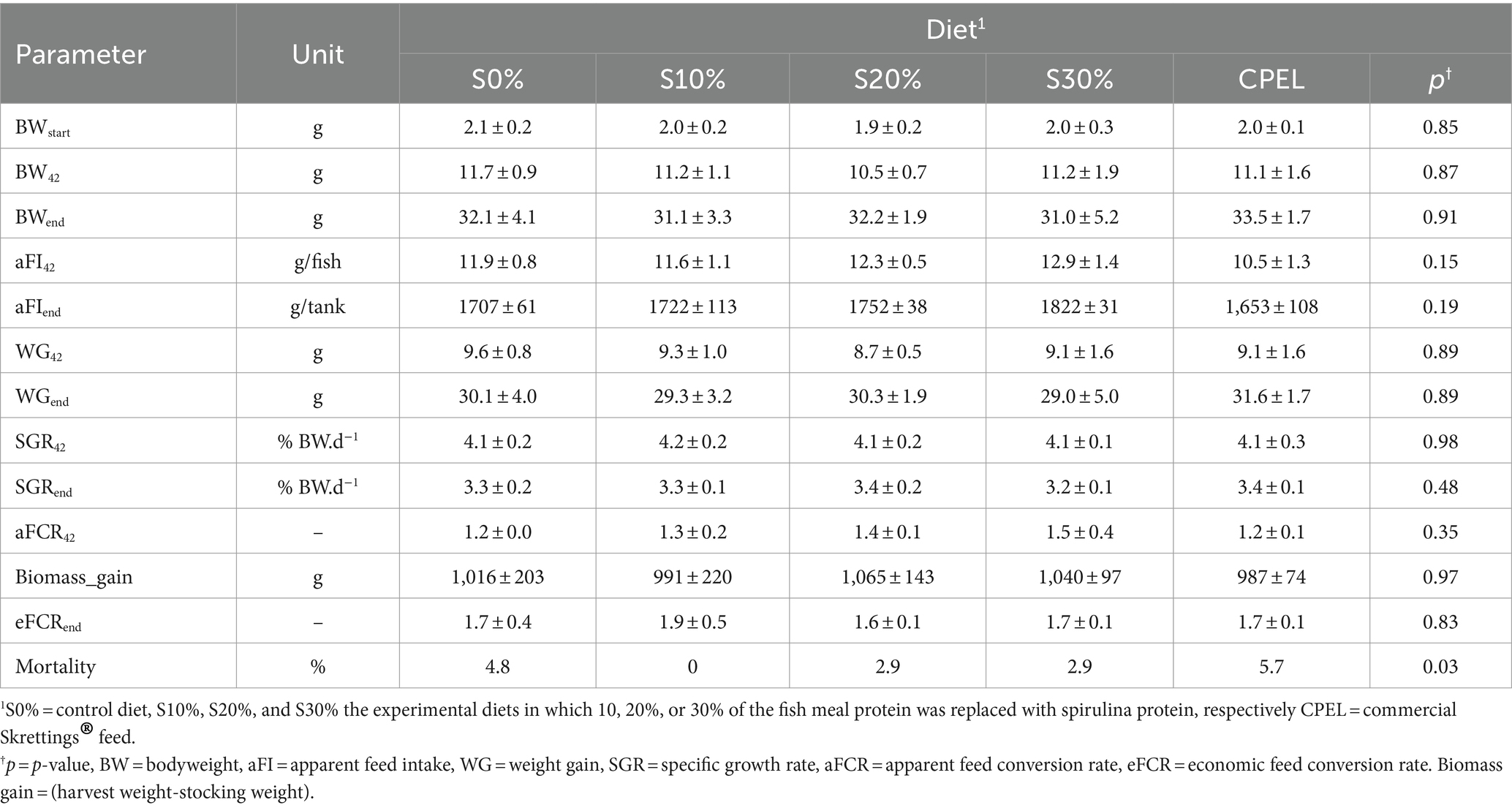
Table 3. Growth performance of Nile tilapia (O. niloticus) fingerlings fed graded levels of protein replacement in fish meal with protein stemming from spirulina (A. platensis) and commercial diet.
3.2 Water quality
During the experiment, temperature 26.4 ± 0.5°C and dissolved oxygen 5.0 ± 0.2 mg/L were similar between treatments (p > 0.05). The pH 6.7 ± 0.0, TAN 0.2 ± 0.0 mg/L, NO2–0.01 ± 0.01 mg/L, NO3–12.9 ± 1.4 mg/L, and salinity 0.13 ± 0.0 ppt were within the required range limits for tilapia culture (Boyd and Tucker, 1998).
3.3 Fish proximate composition
The proximate composition (Table 4) indicated that there were significant differences in the crude protein composition of fish fed spirulina-based diets. The fish fed 30% spirulina presented a significantly higher protein content (55.2%) compared to 10 and 20% spirulina diets (p < 0.05). Fat content was significantly higher in fish fed 20% spirulina (6.20%) compared to the other treatments. Ash content was significantly lower in fish fed the control diet (S0%) and fish fed S20%, while carbohydrates were significantly higher in the fish fed S20% (p < 0.05). Gross energy was significantly higher in fish fed the commercial diet (CPEL).
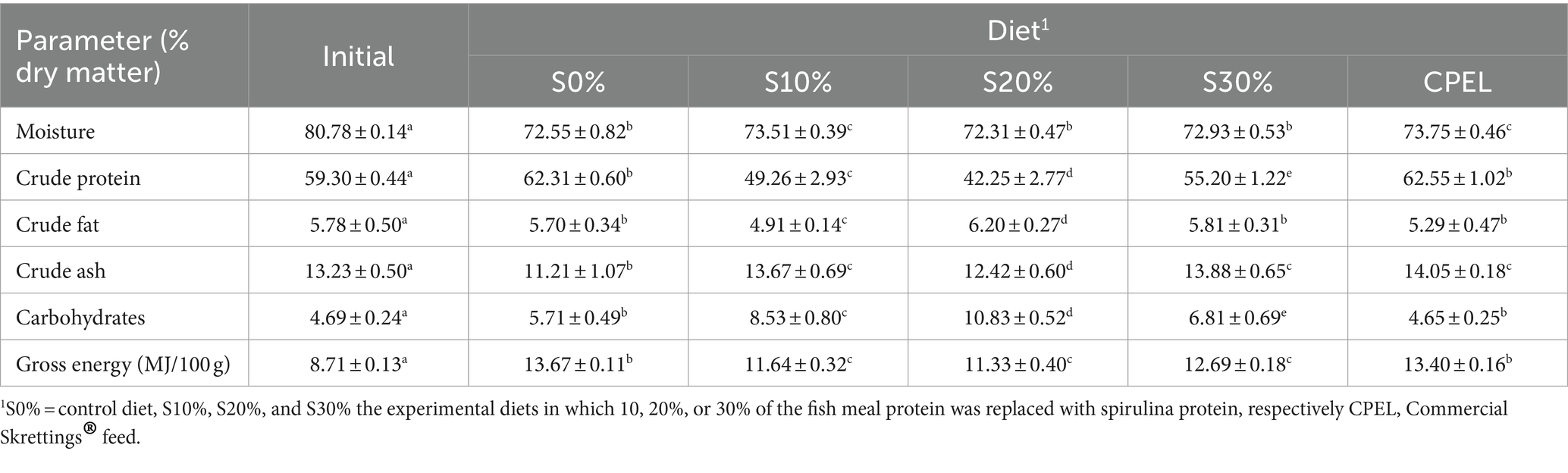
Table 4. Whole body composition of Nile tilapia fingerlings (O. niloticus) fed grade levels of Spirulina (A. platensis) and commercial diet.
The fatty acid profile of whole fish analyzed at the end of the experiment is presented in Table 5. The saturated fatty acids had lower values apart from palmitic acid, which had higher values across all the treatments but were not significantly different (p > 0.05). The total saturated fats significantly increased (p < 0.05) in the fish fed spirulina-based diets compared to the initial content. Among the monounsaturated fatty acids, oleic acid was significantly higher in the fish fed spirulina-based diets compared to the initial content and the fish fed commercial feed. Linoleic acid (LA) was significantly higher in fish fed commercial feed (p < 0.05). Three omega-3 fatty acids were detected, namely linolenic acid, eicosapentaenoic acid (EPA), and docosahexaenoic acid (DHA), which were significantly higher in fish fed 30% spirulina compared to the control. Linolenic acid was significantly higher in fish fed a commercial diet while the total omega-3 fatty acid was significantly higher in fish fed S30%. Total PUFA was also significantly higher in fish fed 30% spirulina (p < 0.05) but was not significantly different from the commercial diet (p > 0.05).
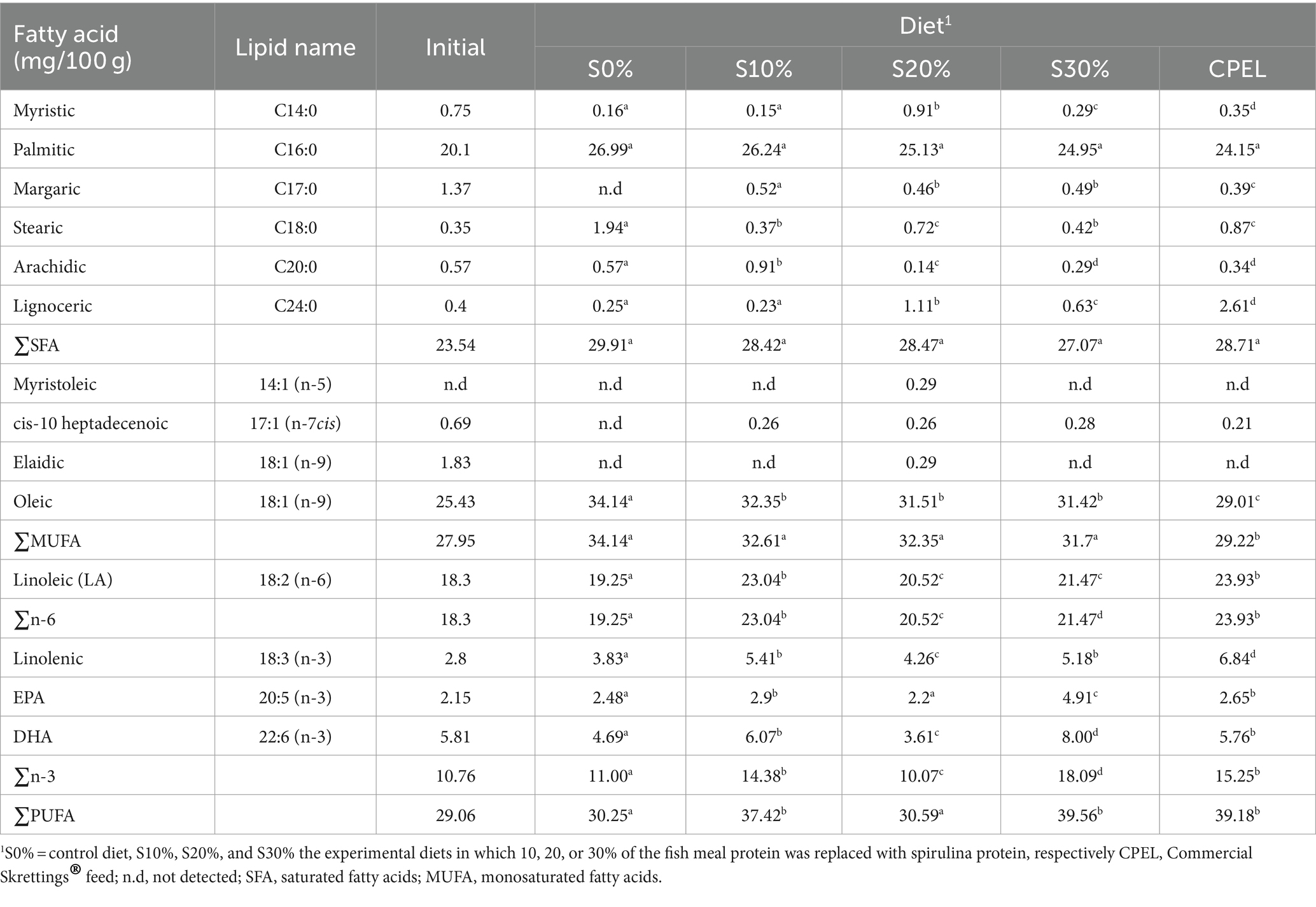
Table 5. Whole body fatty acid composition of Nile tilapia fingerlings (O. niloticus) fed graded levels of spirulina (A. platensis) and commercial diet.
4 Discussion – trial outcomes and potentials for enhanced livelihood in Africa
Even though the challenges of ensuring food and nutrition security are complex, there are many opportunities, for instance, using the edible microbial biomass derived from microalgae as a conventional source of food and feed (Linder, 2019). In this study, the main aim is to investigate whether protein from spirulina is a suitable alternative to protein sourced from fish meal in fish feed for juvenile Nile tilapia. By replacing parts of the fish meal protein of the control diet for juvenile tilapia with dried spirulina, we measured (1) the growth performance, (2) the fish proximate composition, and (3) the fatty acid profile of whole fish.
The trial in this study confirms that fingerlings can just as well be fed a diet with 30% of the fish meal protein replaced by protein from spirulina as the commercial diet. In particular, the body composition analysis shows that fish fed 30% spirulina protein (S30) had a significantly higher protein content while a higher lipid content was reported in fish fed 20% spirulina protein (S20). The increase in protein content could have resulted from increased nutrient deposition and effective conversion of ingested food into structural protein, building more muscle (Lara-Flores and Olvera-Novoa, 2013).
Moreover, the results show that the economic commercial feed diet (eFCR) of the fingerlings fed the different diets was similar across all trial groups. Also, the nutritive value did not vary significantly between diets, meaning that the growth of the fingerlings depended on the nutritive content of the diets and that the growth performance was not influenced by replacing fish meal protein with spirulina protein by up to 30%. The similarity in feed intake and mortality rates of fingerlings from all treatments also indicate that there was no influence on the palatability or toxicity of the inclusion of spirulina in feed, i.e., the replacement of fish protein with spirulina protein in the diet.
Furthermore, the substitution of fish meal and fish oil with plant sources led to a decrease in polyunsaturated fatty acids (n-3 LC-PUFA), eicosapentaenoic acid (EPA), and docosahexaenoic acid (DHA) levels in aquafeeds, lowering levels of these critical beneficial fatty acids in many farmed fish species (Sprague et al., 2016; Trevi et al., 2023). As a result, it is important to find novel ingredients that can meet the needs of the fish feed industry while also meeting the nutritional requirements of cultured fish and ultimately enriching the n-3 LC-PUFA contents of farmed fish (Tocher et al., 2019) to provide the required important nutrients to the consumers, thereby ensuring nutrition security. Spirulina has been documented to be rich in n-3 LC-PUFA, EPA, and DHA and its protein content is similar to marine fish meal, which makes it a suitable substitute for fish meal (Trevi et al., 2023). The presence of high levels of n-3 PUFA in fish fed 30% spirulina is an indicator that the fish digested PUFA better than the saturated fatty acids (SFA) (Raji et al., 2020). Similarly, PUFA levels were elevated in Siberian sturgeon (Acipenser baeri) fed a diet containing a high concentration of spirulina, of up to 60% (Palmegiano et al., 2005).
These outcomes confirm similar findings across the literature on spirulina trials as feed in the aquaculture sector, mostly in Asia. This includes experiments on growth performance, survival rate, fatty acid profiles, and fecundity of Nile tilapia (O. niloticus) conducted in Bangladesh (Hossain, 2017). In Japan, it has been found that tilapia fed solely raw spirulina has a high flesh quality (Lu et al., 2003). Moreover, the literature informs of a series of experiments with spirulina as feed for catfish. This includes research on; 1) replacing protein sourced from fish meal with protein sourced from spirulina to investigate the growth, carcass composition, and pigment of the Mekong giant catfish (Pangasius gigas) (Tongsiri et al., 2010) 2) the tolerance effect of spirulina against lead nitrate-induced cytotoxicity and genotoxicity in catfish (Clarias gariepinus) (Hamed et al., 2019), and 3) the effects of feeding African catfish (C. gariepinus) exposed to food shortage using spirulina diets to investigate growth and hematological parameters (Sayed and Fawzy, 2014). While, overall, the experiments inform of positive results, one experiment obtained more disadvantageous outcomes. Altmann and Rosenau (2022) inform that although spirulina overall provides a prospective protein source in poultry and pork production, as well as in aquaculture, it can have implications for system productivity and end-product quality, depending on the animal production system, although this needs further research. Other microalgae, which have been used as feed ingredients in similar trials, include Schizochytrium (Troell et al., 2023) and Chlorella (Alagawany et al., 2021).
Such findings are not completely new; As early as in 1975, spirulina feed trials for experimenting with the growth of tilapia, among other species, were proven to be successful (Stanley and Jones, 1976). In practice, it is not always easy to get hold of a starter culture to produce spirulina, and it is often very expensive. Although spirulina grows naturally on a few lakes in Kenya, it is not suited for feed production because it is highly contaminated with other microalgae species, some of which can be toxic. Although commercial spirulina contains trace concentrations of six typical heavy metals (Ni, Zn, Hg, Pt, Mg, and Mn), it has been revealed that concentrations of inorganic elements have not been found to exceed the present regulation levels, and they can be considered safe food (Al-Dhabi, 2013). To compare the production costs of spirulina as a protein source in feed at this stage is not possible for a series of reasons: (1) affordably produced spirulina is not available at this stage so there is no market price to compare, and (2) the cost prices of different sources of protein depend on the market levels: at the community level, at the city level, at the national level, at the regional level, or in the international market.
There are at least seven reasons for the increased urgency to make use of spirulina as a protein source in feed (Greene et al., 2016; Linder, 2019). (1) The use of microalgae biomass for animal feed, which can compete with conventional feed sources such as soy and fish meal, is not necessarily limited by access to low-cost growth substrates. (2) As the feed and aquaculture sectors are expanding rapidly, it is more necessary now than ever to make use of alternative sustainable feed ingredients, and microalgae have the potential to ensure sustainability standards by replacing fish meal and fish oil. (3) Given the need to reduce feed and production costs and feed waste, a highly digestible feed with low input costs is needed, such as microalgae. (4) The global food production system is threatened by the effects of climate change, and due to low carbon footprints and carbon credits from industrial CO2 conversion, microalgae-based feeds have the potential to provide significant climate benefits as well as economic benefits. (5) Given the urgency of future food and nutrition security, new sources of environmentally friendly and nutrient-rich feed ingredients for global food security in the bio-economy are enhanced. (6) Sustainable management of natural resources in food systems is critical to food and nutrition security, and microalgae have the potential to bypass many of the current environmental boundaries of food production while also reducing environmental impacts. Microalgae can also be cultivated for food and feed independently of arable land and can be used for wastewater treatment. (7) The knowledge and insights of microalgae as a protein replacement in feed and food are increasing.
As such, spirulina can have multiple functions following a food system approach and can contribute to transition pathways aiming for SDGs, including SDG1: No poverty; SDG2: Zero hunger; SDG6: Clean water and sanitation; and SDG12: Responsible consumption and production. According to the literature, there are many potential uses for spirulina in our food systems. The literature recommends that spirulina play a critical role in a circular economy context (Napolitano et al., 2022). For instance, Life Cycle Assessment (LCA) was applied to estimate the sustainability of “circular” fish farming growing spirulina on urban or agro-industrial wastewater, resulting in significant impacts as a wastewater treatment while improving fish welfare (Napolitano et al., 2022). Novel investigations have shown that spirulina can efficiently metabolize the nutrients and remove the heavy metals contained in aquaculture effluent (Alagawany et al., 2021), and as such, it can be used for treating wastewater, including effluent from fish culture activities (Zhang et al., 2020). Fish aquaculture could apply an integrated strategy of simultaneously treating aquaculture effluent while producing spirulina biomass to supplement fish diets (Zhang et al., 2020). Another study utilized fish waste in the form of fish protein hydrolysate as an organic nitrogen source for the cultivation of spirulina as a replacement for inorganic nitrogen. Because inorganic nitrogen bears high costs and energy use and causes severe environmental problems, it is concluded that both fish waste valorization and spirulina cultivation comprise sustainable and environment-friendly contributions (Shanthi et al., 2021). Spirulina has also been recommended in other studies for environmental reasons, including a study investigating spirulina growth on swine wastewater and a study on the removal of chemical oxygen demand, ammonia, and phosphorous (Mezzomo et al., 2010).
In Kenya, 26% of children below 5 years old suffer from malnutrition (Masibo et al., 2020) and increased fish consumption by such vulnerable groups could greatly improve their nutrition status. While spirulina has nutritional value as a feed ingredient, it can also become an affordable nutritious food ingredient in future, which can lead to substantially enhance food and nutrition security among the food insecure population in Africa. Moreover, affordable fish can have a huge impact on food and nutrition security among vulnerable groups in Kenya (Kawarazuka and Béné, 2010; Ogello and Munguti, 2016). However, fish prices affect consumption patterns in urban slums, where consumers tend to buy smaller and cheaper fish, such as the Lake Victoria sardine (Rastrineobola argentea), locally known as Omena when its price is reduced (Ayuya et al., 2021). This means that farmed fish could be more affordable to low-income families if it was produced and sold cheaper. In recent years, the consumption of Omena has been on the rise locally among poor rural communities (Aura et al., 2022). Spirulina could fill this gap and complement Omena as human food and animal feed since it is also utilized as a protein source for fish and other farmed livestock such as dairy cattle and pigs.
5 Concluding remarks
The main aim of this study is to investigate whether protein from spirulina is a suitable alternative to protein sources from fish meal in the feed of juvenile tilapia. The outcomes of a trial conducted in Kenya show that nutritionally, protein from spirulina is a suitable ingredient to replace fish meal protein in juvenile Nile tilapia feed. In particular, the body composition analysis shows that fish fed 30% spirulina protein (S30) had a significantly higher protein content, while a higher lipid content was reported in fish fed 20% spirulina protein (S20). This is interesting because it implies that non-sustainable protein sources in feed, such as soya and fish meal, can be replaced by highly environmentally friendly and climate-neutral alternatives.
Given the unique properties of spirulina, including its high protein, essential amino acids, and essential fatty acids content, it has a high potential to fight hunger and to contribute substantially to increasing food and nutrition security among the most vulnerable population groups in Kenya and elsewhere, both as feed for aquaculture (Alofa et al., 2020) and as food (Masibo et al., 2020). Thus, spirulina potentially has a series of impact areas throughout a food system. Furthermore, given its contributions to feed and food and value gained through processing and marketing throughout a value chain, it serves as an environmental driver in a circular economy, recommended to play a critical role as a side stream treatment while improving fish welfare (Napolitano et al., 2022). As such, it contributes to a series of SDGs, including SDG1: no poverty; SDG2: zero hunger; SDG6: clean water and sanitation; and SDG12: Responsible consumption and production.
The challenge is to ensure spirulina becomes available in affordable quantities to low-income groups. The high prices of fish meal, soybean, and fish oil lead farmers to often spend more than half of their earnings from livestock on feed (Chandra et al., 2010). For instance, making use of spirulina as feed in fish farming has great potential in Kenya to improve the livelihoods of farmers by generating income but also by improving nutrition (Amankwah et al., 2018; Munguti et al., 2022). As such, cheaper suitable alternative protein-rich ingredients in feed are being sought, and spirulina is recommended as a major plant protein source in farmed fish nutrition (Alagawany et al., 2021). To ensure the low costs of feed, it is recommended that spirulina is grown on animal manure available on farms (Ungsethaphand et al., 2009). While, in Kenya, spirulina is produced commercially for human consumption only, Wageningen University and Research, together with KMFRI in Sagana and Nasio Trust in Kakamega, Kenya, are carrying out trials using different types of animal manure to grow spirulina on fish farms. The incentive is to make spirulina available to small-scale farmers, to ensure the affordability of high-quality feed, and to combat hunger by increasing food production in sustainable, climate-neutral ways.
Data availability statement
The data that support the findings of this study are available from the corresponding author upon request.
Author contributions
KS: Conceptualization, Supervision, Writing – review & editing. JK: Conceptualization, Methodology, Supervision, Validation, Writing – original draft, Writing – review & editing. MO: Data curation, Formal analysis, Investigation, Methodology, Resources, Writing – review & editing. AN: Conceptualization, Writing – review & editing. RG-C: Supervision, Writing – review & editing. MB: Funding acquisition, Supervision, Writing – review & editing. ER: Writing – review & editing. AV: Conceptualization, Funding acquisition, Supervision, Writing – review & editing.
Funding
The author(s) declare financial support was received for the research, authorship, and/or publication of this article. This research is supported by two projects at Wageningen University & Research (WUR): (1) “Alternative fish feed sources from local resources in Lake Victoria region”: Food loss and dialogues project (KB-35-008-015)” and (2) “Food & nutrition security for low-income groups in rural–urban food systems (KB-35-102-001). These two projects are funded by the Dutch Ministry of Agriculture, Nature and Food Security.
Acknowledgments
The authors want to thank Sparos®, Olhão, Portugal, who provided the starter culture of spirulina used in the trials, as well as the Kenya Marine and Fisheries Research Institute (KMFRI) for the use of its research facility and technicians who helped in the experiment. Thanks also to the Dutch Ministry of Agriculture, Nature and Food Security, who funded this study.
Conflict of interest
The authors declare that the research was conducted in the absence of any commercial or financial relationships that could be construed as a potential conflict of interest.
Publisher’s note
All claims expressed in this article are solely those of the authors and do not necessarily represent those of their affiliated organizations, or those of the publisher, the editors and the reviewers. Any product that may be evaluated in this article, or claim that may be made by its manufacturer, is not guaranteed or endorsed by the publisher.
References
Abdel-Tawwab, M., El-Saadawy, H. A., El-Belbasi, H. I., Abd El-Hameed, S. A. A., and Attia, A. A. (2021). Dietary spirulina (Arthrospira platenesis) mitigated the adverse effects of imidacloprid insecticide on the growth performance, haemato-biochemical, antioxidant, and immune responses of Nile tilapia. Comp. Biochem. Physiol. 247:109067. doi: 10.1016/j.cbpc.2021.109067
Al Mamun, M., Hossain, M. A., Saha, J., Khan, S., Akter, T., and Banu, M. R. (2023). Effects of spirulina Spirulina platensis meal as a feed additive on growth performance and immunological response of Gangetic mystus Mystus cavasius. Aquac. Rep. 30:101553. doi: 10.1016/j.aqrep.2023.101553
Alagawany, M., Taha, A. E., Noreldin, A., El-Tarabily, K. A., and Abd El-Hack, M. E. (2021). Nutritional applications of species of Spirulina and Chlorella in farmed fish: a review. Aquaculture 542:736841. doi: 10.1016/j.aquaculture.2021.736841
Al-Dhabi, N. A. (2013). Heavy metal analysis in commercial Spirulina products for human consumption. Saudi J. Biol. Sci. 20, 383–388. doi: 10.1016/j.sjbs.2013.04.006
Alofa, C. S., Adite, A., and Abou, Y. (2020). Evaluation of Spirulina (Spirulina platensis) wastes and live housefly (Musca domestica) larvae as dietary protein sources in diets of Oreochromis niloticus (Linnaeus 1758) fingerlings. Aquatic Res. 3, 24–35. doi: 10.3153/AR20003
Alp Erbay, E., and Yeşilsu, A. F. (2021). Fish protein and its derivatives: functionality, biotechnology and health effects. Aquat. Food Stud. 1:AFS13. doi: 10.4194/AFS13
Altmann, B. A., and Rosenau, S. (2022). Spirulina as animal feed: Opportunities and challenges. Foods. 11:965. doi: 10.3390/foods11070965
Amankwah, A., Quagrainie, K. K., and Preckel, P. V. (2018). Impact of aquaculture feed technology on fish income and poverty in Kenya. Aquac. Econ. Manag. 22, 410–430. doi: 10.1080/13657305.2017.1413689
AOAC (1990). OFficial methods of analysis of the Association of Official Analytical Chemists. 15th Edn. Virginia, USA: Association of Official Analytical Chemists (AOAC).
Aura, C. M., Owiti, H., Nyamweya, C., Odoli, C. O., Obuya, J. A., Ogari, Z., et al. (2022). Aligning small indigenous fish species (SIS) in policy and management for enhanced food security and nutrition: the case of the Kenyan Lake Victoria Omena fishery. Lakes Reserv. Sci. Pol. Manag. Sustain. Use 27, 1–12. doi: 10.1111/lre.12399
Ayuya, O. I., Soma, K., and Obwanga, B. (2021). Socio-economic drivers of fish species consumption preferences in Kenya’s urban informal food system. Sustainability (Switzerland) 13:5278. doi: 10.3390/su13095278
Bligh, E. G., and Dyer, W. J. (1959). A rapid method of total lipid extraction and purification. Can. J. Biochem. Physiol. 37, 911–917. doi: 10.1139/y59-099
Boyd, C. E., McNevin, A. A., and Davis, R. P. (2022). The contribution of fisheries and aquaculture to the global protein supply. Food Secur. 14, 805–827. doi: 10.1007/s12571-021-01246-9
Boyd, C. E., and Tucker, C. S. (1998). Pond aquaculture water quality management. Springer New York, NY: Springer US.
Chandra, S., Chaudhury, U., and Banerjee, R. (2010). Development and assessment of a fish feed to assist in aquaculture nutrition management.
El-Araby, D. A., Amer, S. A., Attia, G. A., Osman, A., Fahmy, E. M., Altohamy, D. E., et al. (2022). Dietary Spirulina platensis phycocyanin improves growth, tissue histoarchitecture, and immune responses, with modulating immunoexpression of CD3 and CD20 in Nile tilapia Oreochromis niloticus. Aquaculture 546:737413. doi: 10.1016/j.aquaculture.2021.737413
Eyhorn, F., Müller, A., Reganold, J. P., Frison, E., Herren, H. R., Luttikholt, L., et al. (2019). Sustainability in global agriculture driven by organic farming. Nat. Sustain. 2, 253–255. doi: 10.1038/s41893-019-0266-6
FAO (2008). A review on culture, production and use of Spirulina as food for humans and feeds for. In aquaculture. Rome, Italy: FAO
FAO, IFAD, UNICEF, WFP, WHO (2022). The state of food security and nutrition in the world 2022; repurposing food and agricultural policies to make healthy diets more affordable ; Rome, Italy: FAO.
Graef, F., Sieber, S., Mutabazi, K., Asch, F., Biesalski, H. K., Bitegeko, J., et al. (2014). Framework for participatory food security research in rural food value chains. Glob. Food Secur. 3, 8–15. doi: 10.1016/j.gfs.2014.01.001
Greene, C. H., Huntley, M. E., Archibald, I., Gerber, L. N., Sills, D. L., Granados, J., et al. (2016). Marine microalgae: climate, energy, and food security from the sea. Oceanography 29, 10–15. doi: 10.5670/oceanog.2016.91
Hamed, M., Soliman, H. A., and Sayed, A. E. D. H. (2019). Ameliorative effect of Spirulina platensis against lead nitrate–induced cytotoxicity and genotoxicity in catfish Clarias gariepinus. Environ. Sci. Pollut. Res. 26, 20610–20618. doi: 10.1007/s11356-019-05319-3
Herrero, M., Thornton, P. K., Mason-D’Croz, D., Palmer, J., Bodirsky, B. L., Pradhan, P., et al. (2021). Articulating the effect of food systems innovation on the sustainable development goals. Lancet Planet. Health 5, e50–e62. doi: 10.1016/S2542-5196(20)30277-1
HLPE (2014). Food losses and waste in the context of sustainable food systems; a report by the high level of experts on food security and nutrition of the committee on world food security ; Rome, Italy: HLPE.
Hossain, M. B. (2017). Growth performance and fatty acid profile of Nile Tilapia Oreochromis niloticus (Linnaeus, 1758) fed with different phytoplankton (Doctoral dissertation, University of Dhaka).
Jahan, T., Ali, M. A., and Rahman, T. R.-F. (2016). Investigation on the growth performances of selected probiotic supplemented tilapia (Oreochromis niloticus) and walking catfish (Clarias batrachus) fingerlings. Fundam. Appl Agricul. 1, 75–81.
Joshua, W. J., and Zulperi, Z. (2020). Effects of spirulina platensis and chlorella vulgaris on the immune system and reproduction of fish in Pertanika. J. Trop. Agric. Sci. 43, 429–444. doi: 10.47836/PJTAS.43.4.01
Karimi, S., Soofiani, N. M., Mahboubi, A., Ferreira, J. A., Lundh, T., Kiessling, A., et al. (2021). Evaluation of nutritional composition of pure filamentous fungal biomass as a novel ingredient for fish feed. Fermentation 7:152. doi: 10.3390/FERMENTATION7030152
Kawarazuka, N., and Béné, C. (2010). Linking small-scale fisheries and aquaculture to household nutritional security: an overview. Food Secur. 2, 343–357. doi: 10.1007/s12571-010-0079-y
KeFS (2022). Republic of Kenya fisheries annual statistical bulletin 2020 Kenya Fisheries Service. 1–47.
Lara-Flores, M., and Olvera-Novoa, M. A. (2013). The use of lactic acid bacteria isolated from intestinal tract of Nile tilapia (Oreochromis niloticus), as growth promoters in fish fed low protein diets. Lat. Am. J. Aquat. Res. 41, 490–497. doi: 10.3856/vol41-issue3-fulltext-12
Linder, T. (2019). Making the case for edible microorganisms as an integral part of a more sustainable and resilient food production system. Food Secur. 11, 265–278. doi: 10.1007/s12571-019-00912-3
Lu, J., Takeuchi, T., and Ogawa, H. (2003). Flesh quality of tilapia Oreochromis niloticus fed solely on raw Spirulina. Fish. Sci. 69, 529–534. doi: 10.1046/j.1444-2906.2003.00653.x
Mabrouk, M. M., Ashour, M., Labena, A., Zaki, M. A. A., Abdelhamid, A. F., Gewaily, M. S., et al. (2021). Nanoparticles of Arthrospira platensis improves growth, antioxidative and immunological responses of Nile tilapia (Oreochromis niloticus) and its resistance to Aeromonas hydrophila. Aquac. Res. 53, 125–135. doi: 10.1111/are.15558
Masibo, P. K., Humwa, F., and MacHaria, T. N. (2020). The double burden of overnutrition and undernutrition in mother-child dyads in Kenya: demographic and health survey data, 2014. J. Nutrition. Sci. 9:e5. doi: 10.1017/jns.2019.39
Mezzomo, N., Saggiorato, A. G., Siebert, R., Tatsch, P. O., Lago, M. C., Hemkemeier, M., et al. (2010). Cultivation of microalgae Spirulina platensis (Arthrospira platensis) from biological treatment of swine wastewater. Food Sci. Technol. 30, 173–178. doi: 10.1590/S0101-20612010000100026
Müller, B., Hoffmann, F., Heckelei, T., Müller, C., Hertel, T. W., Polhill, J. G., et al. (2020). Modelling food security: bridging the gap between the micro and the macro scale. Glob. Environ. Chang. 63:102085. doi: 10.1016/j.gloenvcha.2020.102085
Munguti, J. M., Nairuti, R., Iteba, J. O., Obiero, K. O., Kyule, D., Opiyo, M. A., et al. (2022). Nile tilapia (Oreochromis niloticus Linnaeus, 1758) culture in Kenya: emerging production technologies and socio-economic impacts on local livelihoods. Aquac. Fish Fish. 2, 265–276. doi: 10.1002/aff2.58
Napolitano, G., Venditti, P., Agnisola, C., Quartucci, S., Fasciolo, G., Tomajoli, M. T. M., et al. (2022). Towards sustainable aquaculture systems: biological and environmental impact of replacing fishmeal with Arthrospira platensis (Nordstedt)(spirulina). J. Clean. Prod. 374:133978. doi: 10.1016/j.jclepro.2022.133978
Niggli, U., Sonnevelt, M., and Kummer, S. (2023). “Pathways to advance agroecology for a successful transformation to sustainable food systems” in In Science and Innovations for Food Systems Transformation [Internet] eds. J. von Braun, K. Afsana, and L. O. Fresco (Cham (CH): Springer), Available at: https://www.ncbi.nlm.nih.gov/books/NBK599641/
Ogello, E. O., and Munguti, J. M. (2016). Aquaculture: a promising solution for food insecurity, poverty and malnutrition in Kenya. Afr. J. Food Agric. Nutr. Dev. 16, 11331–11350. doi: 10.18697/ajfand.76.15900
Onura, C. N., Muthumbi, A. W., James, J. G., and Wang’ondu, V. W. (2022). Stress tolerance of African catfish (Clarias gariepinus, Burchell, 1822) larvae fed on Spirulina platensis or Eisenia fetida in partial replacement of Caridina nilotica in formulated diets. East African J. Agric. Biotechnol. 5, 195–212. doi: 10.37284/eajab.5.1.845
Palmegiano, G. B., Agradi, E., Forneris, G., Gai, F., Gasco, L., Rigamonti, E., et al. (2005). Spirulina as a nutrient source in diets for growing sturgeon (Acipenser baeri). Aquac. Res. 36, 188–195. doi: 10.1111/j.1365-2109.2005.01209.x
Raji, A. A., Jimoh, W. A., Bakar, N. H. A., Taufek, N. H. M., Muin, H., Alias, Z., et al. (2020). Dietary use of Spirulina (Arthrospira) and Chlorella instead of fish meal on growth and digestibility of nutrients, amino acids and fatty acids by African catfish. J. Appl. Phycol. 32, 1763–1770. doi: 10.1007/s10811-020-02070-y
Rashidian, G., Gabriel, N. N., and Dharmaraj, R. (2023). Editorial: new advances in functional aquafeeds. Front. Vet. Sci. 10:1187586. doi: 10.3389/fvets.2023.1187586
Rasul, M. G., Yuan, C., and Shah, A. K. M. A. (2021). Chemical composition and nutritional value of dried fish in Bangladesh. Egypt. J. Aquat. Biol. Fish. 25, 379–399. doi: 10.21608/EJABF.2021.189666
Salter, A. M., and Lopez-Viso, C. (2021). Role of novel protein sources in sustainably meeting future global requirements. Proc. Nutr. Soc. 80, 186–194. doi: 10.1017/S0029665121000513
Sayed, A. E. D. H., and Fawzy, M. A. (2014). Effect of dietary supplementation of Spirulina platensis on the growth and haematology of the catfish Clarias gariepinus. J Adv. Biol. 5, 625–635.
Shalata, H. A., Bahattab, O., Zayed, M. M., Farrag, F., Salah, A. S., Al-Awthan, Y. S., et al. (2021). Synergistic effects of dietary sodium butyrate and Spirulina platensis on growth performance, carcass composition, blood health, and intestinal histomorphology of Nile tilapia (Oreochromis niloticus). Aquac. Rep. 19:100637. doi: 10.1016/j.aqrep.2021.100637
Shanthi, G., Premalatha, M., and Anantharaman, N. (2021). Potential utilization of fish waste for the sustainable production of microalgae rich in renewable protein and phycocyanin-Arthrospira platensis/Spirulina. J. Clean. Prod. 294:126106. doi: 10.1016/j.jclepro.2021.126106
Shati, S. M., Opiyo, M. A., Nairuti, R. N., Shoko, A. P., Munyi, F., and Ogello, E. O. (2022). Black soldier fly (Hermetia illucens) larvae meal improves growth performance, feed utilization, amino acids profile, and economic benefits of Nile tilapia (Oreochromis niloticus, L.). Aquat. Res. 5, 238–249. doi: 10.3153/AR22023
Soma, K., Vernooij, A., Barbosa, M., Opiyo, M., Kals, J., Cubero, R., et al. (2022). The protein transition how to operationalise use of black soldier fly larvae (BSFL) and spirulina as feed ingrediencies in Kenya and Uganda?
Soni, R. A., Sudhakar, K., and Rana, R. S. (2017). Spirulina - from growth to nutritional product: a review. Trends Food Sci. Technol. 69, 157–171. doi: 10.1016/j.tifs.2017.09.0100924-2244/
Sprague, M., Dick, J. R., and Tocher, D. R. (2016). Impact of sustainable feeds on omega-3 long-chain fatty acid levels in farmed Atlantic salmon, 2006–2015. Sci. Rep. 6:21892. doi: 10.1038/srep21892
Stanley, J. G., and Jones, J. B. (1976). Feeding algae to fish. Aquaculture 7, 219–223. doi: 10.1016/0044-8486(76)90140-X
Tocher, D. R., Betancor, M. B., Sprague, M., Olsen, R. E., and Napier, J. A. (2019). Omega-3 long-chain polyunsaturated fatty acids, EPA and DHA: bridging the gap between supply and demand. Nutrients 11, 1–20. doi: 10.3390/nu11010089
Tongsiri, S., MangAmphan, K., and Peerapornpisal, Y. (2010). Effect of replacing fishmeal with Spirulina on growth, carcass composition and pigment of the Mekong giant catfish. Asian J. Agric. Sci. 2, 106–110.
Trevi, S., Uren Webster, T., Consuegra, S., and Garcia de Leaniz, C. (2023). Benefits of the microalgae Spirulina and Schizochytrium in fish nutrition: a meta-analysis. Sci. Rep. 13:2208. doi: 10.1038/s41598-023-29183-x
Troell, M., Costa-Pierce, B., Stead, S., Cottrell, R. S., Brugere, C., Farmery, A. K., et al. (2023). Perspectives on aquaculture’s contribution to the sustainable development goals for improved human and planetary health. J. World Aquacult. Soc. 54, 251–342. doi: 10.1111/jwas.12946
Tzachor, A., Smidt-Jensen, A., Ramel, A., and Geirsdóttir, M. (2022). Environmental impacts of large-scale Spirulina (Arthrospira platensis) production in Hellisheidi Geothermal Park Iceland: life cycle assessment. Mar. Biotechnol. 24, 991–1001. doi: 10.1007/s10126-022-10162-8
Ungsethaphand, T., Peerapornpisal, Y., and Whangchai, N. (2009). Production of spirulina platensis using dry chicken manure supplemented with urea and sodium bicarbonate. Maejo Int. J. Sci. Technol. 3, 379–387.
Wahbi, O. M., and Sangak, Y. (2017). Enhancement of reproductive performance of nile tilapia Oreochromis niloticus using phytobiotic Spirulina platensis. J. Biol. Sci. 17, 305–311. doi: 10.3923/jbs.2017.305.311
Zhang, F., Man, Y. B., Mo, W. Y., and Wong, M. H. (2020). Application of Spirulina in aquaculture: a review on wastewater treatment and fish growth. Rev. Aquac. 12, 582–599. doi: 10.1111/raq.12341
Keywords: alternative protein, spirulina (Arthrospira platensis), fish feed, fish nutrition, Kenya
Citation: Soma K, Kals J, Opiyo MA, Ndambi A, García-Cubero R, Barbosa MJ, Rurangwa E and Vernooij A (2024) Toward sustainable food systems: can spirulina (Arthrospira platensis) become a sustainable source of protein to enhance the nutritional benefits of cultured Nile tilapia (Oreochromis niloticus)? Front. Sustain. Food Syst. 8:1283150. doi: 10.3389/fsufs.2024.1283150
Edited by:
Brandy E. Phipps, Central State University, United StatesReviewed by:
Imana Pal, Durban University of Technology, South AfricaSimrat Kaur, Climate Survival Solutions, India
Copyright © 2024 Soma, Kals, Opiyo, Ndambi, García-Cubero, Barbosa, Rurangwa and Vernooij. This is an open-access article distributed under the terms of the Creative Commons Attribution License (CC BY). The use, distribution or reproduction in other forums is permitted, provided the original author(s) and the copyright owner(s) are credited and that the original publication in this journal is cited, in accordance with accepted academic practice. No use, distribution or reproduction is permitted which does not comply with these terms.
*Correspondence: Katrine Soma, a2F0cmluZS5zb21hQHd1ci5ubA==