- 1Department of Horticulture, Michigan State University, East Lansing, MI, United States
- 2Department of Plant, Soil and Microbial Sciences, Michigan State University, East Lansing, MI, United States
Planting a cover crop living mulch between plastic mulch beds in fresh market vegetable production can reduce soil erosion and runoff, and offers an opportunity to grow an income generating cash crop alongside a soil building cover crop. However, potential negative impacts on yield, variable weed control, unclear impacts on soil health, and limited management recommendations challenge adoption of this practice, despite grower interest. In a two-year study in southwest Michigan, living mulches were evaluated in the production of plasticulture organic summer squash (Cucurbita pepo cv. Lioness) and bell pepper (Capsicum annuum cv. Paladin). Strategies evaluated included three common grower practices (cultivation, dead straw mulch, mowing ambient weeds) and three mowed living mulch treatments (Italian ryegrass [Lolium multiflorum] monoculture, rye [Secale cereale] monoculture, and a Dutch white clover [Trifolium repens]/rye mixture). We determined the impact of these strategies on weed control, organic matter inputs, vegetable crop performance, nitrogen retention, and soil microbial communities. We found that cultivation and dead mulch provided superior in-season weed control, reducing weed biomass by an average of 86% compared to 18% among living mulch treatments, with associated reductions in the weed seedbank compared to living mulches and mowed weeds. In most cases, living mulch establishment was a challenge and weed biomass on average accounted for 99, 74, and 94% of organic matter inputs in rye, ryegrass, and clover/rye treatments, respectively. Squash performance was unaffected by our treatments, but pepper yield reductions ranged from 41 to 54% in all treatments relative to cultivation in one of two years. Living mulches and the weedy treatment showed the ability to reduce end-of-season potentially leachable nitrogen by 61% compared to cultivation and dead mulching. Soil microbial abundance and functional diversity were similar across treatments, but extracellular enzyme activity was higher in dead mulch, living mulch and weedy treatments compared to cultivation. Results from this study help quantify tradeoffs between common grower practices for managing weeds and soil between plastic mulch beds, highlight key challenges with living mulch alternatives, and identify areas for future research.
1 Introduction
Since its introduction to agriculture in the 1950s, the use of plastic mulch film has become standard practice in the production of many organic and conventional fresh market vegetables (Lamont, 2017; Salama and Geyer, 2023). Its widespread adoption is often credited to effective in-row weed control and microclimate changes that improve crop quality and yield (Tarara, 2000; Kasirajan and Ngouajio, 2012; Wilhoit and Coolong, 2013). However, when roughly 50–75% of a field is covered with impermeable plastic, sediment loss, agrochemical runoff, and nutrient leaching during rain events can be greatly intensified between plastic mulched beds; especially when cultivation and herbicides used for weed control between beds leave the soil bare and highly susceptible to erosion (Wan and El-Swaify, 1999; Arnold et al., 2004; Rice et al., 2004; Zhang et al., 2013). As a result, yield gains in plasticulture production may be offset by associated environmental and soil degradation (Steinmetz et al., 2016).
Growing a cover crop living mulch in the exposed soil between plastic mulch beds can reduce soil erosion and runoff (Rice et al., 2004), simultaneously improving the environmental sustainability of the cropping system and offering an opportunity to grow a soil building cover crop alongside an income generating cash crop. Integrating living mulches into plasticulture production as an alternative between-bed weed control tactic may simultaneously suppress weeds, scavenge residual nutrients, and provide significant organic matter inputs where the ground would have otherwise been left bare (Tarrant et al., 2020). The potential for living mulches to control weeds and improve soil health make this an attractive strategy for growers, particularly those in northern climates where cover cropping windows are limited (Snapp et al., 2005). However, quantitative assessments of the impacts of between-bed living mulches on soil health metrics in plasticulture systems in particular represents a key knowledge gap limiting the adoption of this practice, despite grower interest.
The adoption of living mulches is constrained further by the often-cited challenge of establishing a living mulch stand that is competitive enough to suppress weeds, but not so competitive that it suppresses the cash crop through above- or below-ground resource capture (Hartwig and Ammon, 2002; Westbrook et al., 2021). In integrated plasticulture-living mulch systems, the plastic mulch serves as a spatial buffer and physical barrier that may provide protection against competition. However, previous studies in integrated plasticulture-living mulch systems demonstrate that between-bed living mulches still have variable effects on vegetable crop yields (Table 1). For example, among the 13 studies we identified comparing living mulch to cultivation in plasticulture production, vegetable yields were reduced in 69% of cases. The mechanisms behind such yield losses are often not distinguishable in these studies but are speculated to be the result of competition for water or nutrients between the crop and either the living mulch or associated weeds that are not adequately suppressed by the mulch (Hartwig and Ammon, 2002; Bhaskar et al., 2021). Elucidating the mechanisms causing yield reductions can aid in the development of best management practices to mitigate competition between cash crops and living mulches.
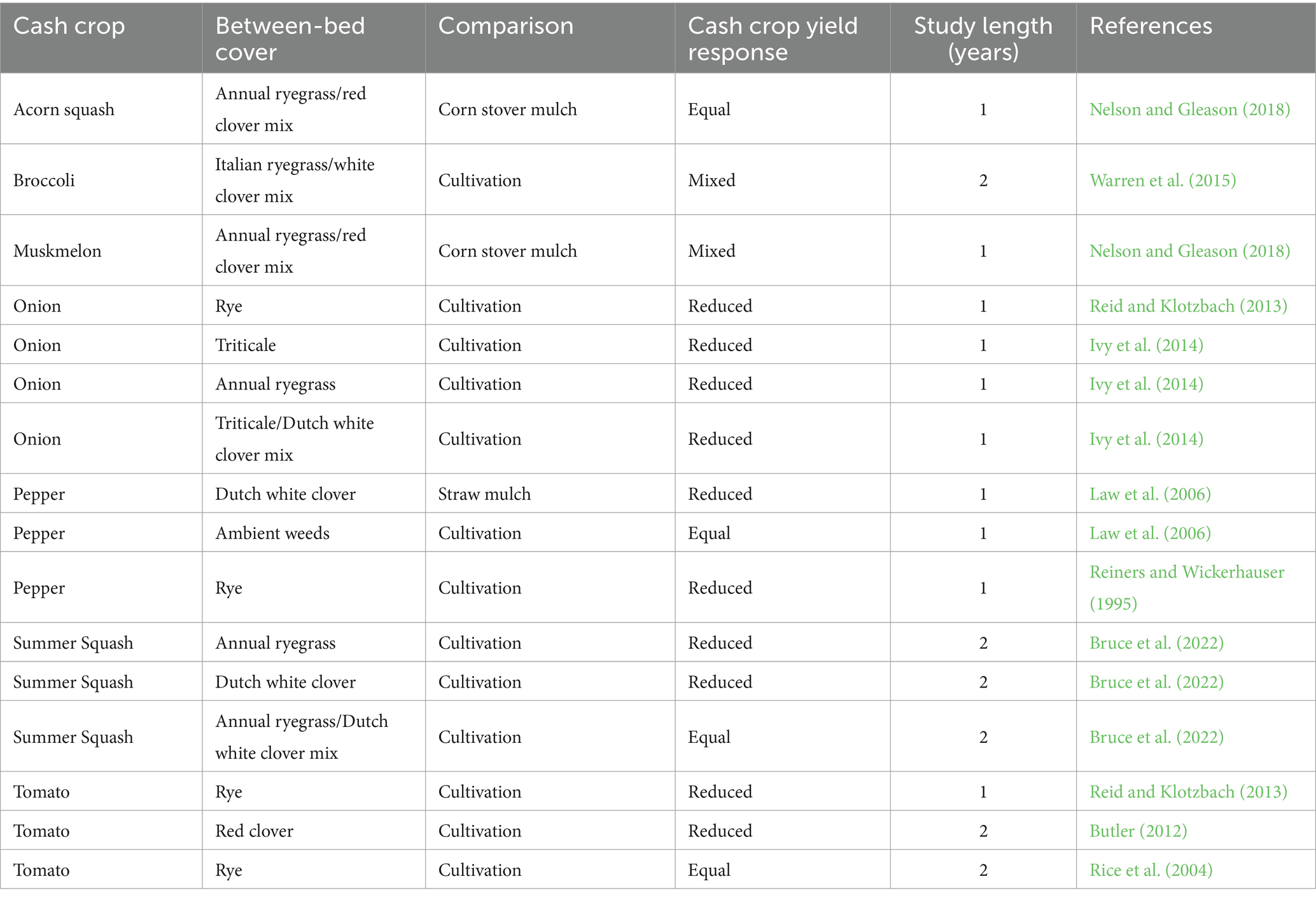
Table 1. Summary of previous studies evaluating the influence of between-bed living mulches on cash crop yields in plasticulture production.
Previous research investigating the use of living mulches between plastic mulch beds have focused on comparisons against a narrow set of common grower practices. For example, comparing living mulch treatments to cultivation (Reiners and Wickerhauser, 1995; Rice et al., 2004; Butler, 2012; Reid and Klotzbach, 2013; Ivy et al., 2014; Warren et al., 2015) or straw/dead mulches (Law et al., 2006; Nelson and Gleason, 2018), but few have compared these practices against one another within the same experiment (Law et al., 2006; Bruce et al., 2022). Furthermore, mowing ambient weeds between plastic mulch beds is a common grower practice, but has gone relatively uninvestigated in the literature (Law et al., 2006). Maintaining between-bed areas through cultivation, dead mulching, and mowing ambient weeds are likely to have distinct impacts on weed management, soil health, and crop yields compared to each other and to living mulch alternatives. Quantifying these system tradeoffs across diverse management practices, as well as understanding impacts in both an in-season production and long-term soil management context, is necessary for growers to make informed management decisions (DeDecker et al., 2014; Brown and Gallandt, 2018).
The objective of this research was to quantify the impact of between-bed management strategies on weed management, cash crop performance, and select soil health metrics in organic plasticulture production of green bell pepper (Capsicum annuum cv. Paladin) and yellow summer squash (Cucurbita pepo cv. Lioness). We included three cover crop living mulch treatments: Italian ryegrass (Lolium multiflorum) monoculture, rye (Secale cereale L.) monoculture, and a Dutch white clover (Trifolium repens)/rye mixture. We also included three control treatments representing common organic grower practice: cultivation, dead mulching, and mowing ambient weeds. We evaluated the impact of these between-bed management strategies on organic matter contributions, in-season and future weed control, vegetable crop performance, nitrogen retention, and soil microbial biomass and enzyme activities as a short-term indicator of soil health and internal nutrient cycling. Our overall hypothesis was that tradeoffs would be identified among between-bed management strategies with regards to their impact on weed management, cash crop performance, and soil health metrics. A more complete understanding of the influence of diverse between-bed management strategies on system performance in plasticulture production is needed to support extension recommendations and grower decision-making regarding living mulch adoption.
2 Materials and methods
2.1 Site description
In 2017 and 2018 a field experiment comparing different between-bed management strategies in organic plasticulture vegetable production was conducted at the Michigan State University (MSU) Southwest Michigan Research and Education Center (SWMREC) in Benton Harbor, Michigan (42.09°N, 86.36°W). The experimental treatments were repeated in the same field and plot boundaries across years of the study to investigate the combined in-season and cumulative impacts of between-bed management practices on soil quality parameters and weed management. The area used for the experimental site was a transitioning organic field that had been maintained as a rye-hairy vetch cover crop for five years prior to trial establishment. The soil at the experimental site was a Spinks (Sandy, mixed, mesic Lamellic Hapludalfs) and Selfridge (Loamy, mixed, active, mesic Aquic Arenic Hapludalfs) loamy sand (Soil Survey Staff, USDA Natural Resources Conservation Service, 2024). Initial soil chemical characteristics were as follows: organic matter 1.6% (loss on ignition); pH 5.9 (1,1 soil/water); CEC 4.0 cmol kg−1; and P (Bray-P1 extractant), K, Mg, and Ca (1 N ammonium acetate extractant) levels of 48, 133, 54, and 397 mg kg−1, respectively.
2.2 Experimental design
The experiment was arranged in a split-plot randomized complete block design with four replications. Between-bed management was the main plot factor and cash crop was the split-plot factor. Between-bed management strategies evaluated included cultivated bare ground, cereal rye residue dead mulch, mowed ambient weeds, and three cover crop living mulch treatments: rye (Secale cereale L.) monoculture, Italian ryegrass (Lolium multiflorum) monoculture, and a Dutch white clover (Trifolium repens)/rye mixture. Treatment names and description are listed in Table 2. Bell pepper (Capsicum annuum cv. Paladin) and summer squash (Cucurbita pepo cv. Lioness) were chosen as cash crops to represent a relatively long- and short-season crop commonly grown in plasticulture systems. While main plot (between-bed management) treatments were maintained in the same locations in both years of the study, the location of split-plots (cash crop factor) within main plots were rotated between years. Main plots measured 6.1 m wide × 5.0 m row length, contained four raised plastic mulch beds (each 0.61 m wide and 0.15 m tall), and three between-row soil areas that measured approximately 1 m wide.
2.3 Field management
Dates of key field operations are outlined in Table 3. Winter cover crops—a rye-vetch mixture in the first year and rye monoculture in the second—were mowed and incorporated with a rototiller in early May of both years. In mid-May, the field received 112 kg total N ha−1 as organic fertilizer (Nature Safe “All-Season 10-2-8,” Irving, TX) derived from feather meal, meat and bone meal, blood meal, and sulfate of potash. Plastic mulch beds (0.15 m bed height and 0.61 m bed top width) were laid on 1.67 m centers within 1 week of fertilizer application. Plastic mulch was black, 1 mil thick and 1.22 m wide (Trickl-Eez Irrigation Inc., St. Joseph, MI). Single drip irrigation lines with 0.30 m emitter spacing were laid under the plastic mulch using a combined plastic mulch layer and bed shaper (Reddick Equipment Company LLC, Williamston, NC).
Bell peppers were sown in 98 cell flats in a heated greenhouse at the end of March and transplanted into the field at the end of May. Summer squash were direct seeded in the field at pepper transplanting. Peppers were grown staked in staggered double rows with 0.30 m between-row spacing and 0.46 m in-row spacing. Squash were grown in single rows with 0.61 m in-row spacing. All beds were drip irrigated simultaneously twice daily with a single drip line offset from the row (squash) or between rows (peppers), based on crop demand and standard grower practice in the area. No fertilizer was applied through the drip.
Between-bed management strategies were established simultaneously with cash crop planting in both years. In 2017, plastic mulch beds were laid 1 week before implementing between-bed management treatments and a stale seedbed cultivation using a wheel-hoe was completed in all between-bed areas immediately before living mulch seeding to reduce potential weed competition. However, wetter spring conditions in 2018 did not allow for the same stale seedbed window between bed formation and treatment establishment.
Living mulch seed was broadcast sown by hand into between-bed areas and lightly incorporated using a rake. Italian ryegrass was seeded at 35.9 kg ha−1. Dutch white clover was seeded at 22.4 kg ha−1. In 2017, cereal rye was seeded at 168.1 kg ha−1, but was doubled to 336.3 kg ha−1 in 2018 in an attempt to address poor establishment in 2017. In both years, Dutch white clover was seeded with rye at half the monoculture rate (84.0 and 168.1 kg ha−1 in 2017 and 2018, respectively). Overhead irrigation was applied to benefit living mulch establishment in 2017, but not in 2018 due to higher precipitation around the time of seeding. Between-bed living mulches and weeds were mowed using a walk behind push mower with the mower deck set to 10.16 cm when the average height of weeds and living mulches were approximately 30 cm. Cultivated plots were maintained through hand cultivation using a wheel-hoe with a 20 cm blade (Glaser wheel hoe, Johnny’s selected seeds, Fairfield, ME). Dead mulch consisted primarily of cereal rye obtained from an adjacent field that had been established the previous fall and flail mowed two days before mulching. Dead mulch was applied at an approximate rate of 18 Mg ha−1.
At the end of September, plastic mulch was removed, and the entire experimental area rototilled to a depth of 20 cm. A rye cover crop was then planted at 180 kg ha−1.
2.4 Data collection
2.4.1 Living mulch and weed biomass production
Biomass samples in dead mulch, weedy, and living mulch plots were taken from one permanent 0.125 m−2 quadrat in each between-row area per plot prior to all mowing events and at experiment termination. Biomass was collected above 10.16 cm from ground level prior to mowing events (to reflect height of mowing) and to the ground at experiment termination. Weed and cover crop biomass were separated and dried to a constant weight at 60°C before dry weight determination. Ambient weeds in cultivated plots in 2017 were negligible, and thus biomass was not collected in cultivated plots in that year. However, in 2018, wet conditions caused delayed cultivation and greater weed escapes. Before the last cultivation event above-ground weed biomass was collected in only two of four replications from three 0.125 m−2 quadrats. The percent decrease in in-season weed biomass compared to the weedy treatment was calculated for each treatment.
2.4.2 Post-season weed seedbank
The readily germinable summer annual weed seedbank was evaluated after the 2017 and 2018 seasons using a greenhouse germination assay adapted from that described in Gallandt et al. (1998). Eight soil cores were taken in the spring to a depth of 20 cm (2.54 cm inside diameter), matching the fall tillage depth, in each between-bed area for a total of 24 cores per plot. Soil cores were homogenized by plot and stored at 4°C before transferring to a greenhouse for weed seed germination within a week of field collection. A subsample of 300–500 mL of field soil was mixed with equal parts potting mix (to improve water holding capacity of field soil) before the mixture was placed on top of a 25.4 cm × 25.4 cm tray filled with 3 L of potting soil. Noseeum mesh separated the bottom potting soil from the field soil mixture. Subsurface irrigation was used to maintain moisture at or near field capacity. The greenhouse temperature setpoint was 21°C, with a range of approximately 20–25°C depending on outside conditions. Weed seedlings were allowed to grow until they could be identified, counted by species, and removed. After emergence of new seedlings had stopped, soils were air dried for two weeks, stirred, rewetted and a second flush of germinated weed seedlings were counted and removed. Cumulative emergence by species was divided by soil volume and converted to germinable seeds per liter of field soil for comparison across treatments.
2.4.3 Cash crop performance
Peppers were harvested every 7–10 days, for a total of five harvest events in 2017 and four in 2018. Summer squash was harvested every other day for a total of 10 harvest events in each year. Squash and pepper were harvested from the center beds in each plot, with buffer plants between plots. This method resulted in a total of eight summer squash and 20 pepper data plants per plot. Harvested fruit were categorized as either marketable or unmarketable based on USDA standards (Agriculture Marketing Service, 2005). Cumulative yields in each season were obtained by summing individual harvests and adjusted to the number of surviving data plants in each plot for analysis on a per plant basis.
At the last harvest, a total of 20 pepper leaves per plot were collected for N analysis. In 2017, 16 squash leaves were collected per plot for N analysis at the last harvest event. Leaf samples were selected to represent the most recent mature leaves. In 2018, two whole squash plants per plot were collected at last harvest and analyzed for N content instead of leaves due to prior sample loss. Tissue samples were dried to a constant weight at 60°C, ground to pass a 1 mm sieve, and analyzed for total N concentration via combustion by A&L Great Lakes Laboratories (Fort Wayne, IN).
2.4.4 Soil nitrogen and water dynamics and soil organic matter
Soil samples were collected under plastic mulch beds in each subplot monthly starting at trial establishment and analyzed for inorganic N concentration (NO3− and NH4+). All soil samples consisted of 10 composited cores per bed taken to 20 cm depth (2.22 cm core diameter) and stored at 4°C until dried at 38°C for at least 36 h prior to passing through a 2 mm sieve. 10 g dried and sieved soil was extracted with 50 mL 1 M KCl, and extracts were analyzed for NO3− and NH4+ concentrations using a Lachat flow injection autoanalyzer (Lachat QuickChem, Hach Company, Loveland, CO).
In-bed soil volumetric water content in the top 20 cm of soil was also monitored regularly using a FieldScout TDR probe (Spectrum Technologies Inc., Aurora, IL). Measurements were taken at a consistent distance from drip irrigation lines, approximately midway between drip line and bed shoulder. 10 measurements were taken in each subplot and averaged before analysis.
To evaluate potentially leachable N remaining in the between-bed soil profile at the end of each season, one 2.54 cm diameter soil profile core per main plot was collected to 60 cm depth from representative between-bed areas in September of each year. Continuous cores were collected into plastic liners using a steel sampling tube driven into the ground by a portable gas-powered hammer (AMS Inc., American Fall, ID). Cores were transported from the field and stored at 4°C until being separated into 20 cm depth intervals and dried at 38°C for at least 36 h. Soil core sections were processed, and soil inorganic N was extracted and analyzed as previously described.
Soil organic matter (SOM) was evaluated at trial initiation in May 2017 and again at the last pepper harvest in August of 2018. Soil samples consisted of 12 composited cores per plot taken to 20 cm depth (2.22 cm core diameter) and were stored at 4°C until dried at 38°C for at least 36 h prior to passing through a 2 mm sieve. SOM was determined from a subsample of the sieved sample using the loss-on-ignition method (Combs and Nathan, 1998).
2.4.5 Microbial community assessment
Soil samples were taken from between-bed areas for microbial analysis at monthly intervals starting at trial establishment. Four samples were taken from each of three between-bed areas in each plot, for a composite sample of 12 cores (20 cm depth, 2.22 cm core diameter) that were homogenized in the field. Samples were placed in a cooler for temporary storage in the field and during transport to the lab where they were stored at 4°C until processing. Within 1 week of sampling, soil was passed through a 4 mm sieve, and evaluated for microbial biomass C and N, gravimetric water content, and a subsample frozen (−20°C) for later use in extracellular enzyme assays as described below.
A field moist sieved subsample was subjected to the chloroform-fumigation-extraction method adapted from Vance et al. (1987) to determine microbial biomass C. For each treatment replicate, 8 g of soil was weighed into two 50 mL centrifuge tubes. One sample was immediately extracted with 40 mL 0.5 M K2SO4 while the other was subjected to fumigation with chloroform stabilized with non-polar hydrocarbons for 24 h. After 24 h, lids were removed, and residual chloroform allowed to evaporate from fumigated samples for 2 h in a fume hood before extraction with 0.5 M K2SO4. Extracts were kept at −20°C until analysis using a Shimadzu total organic carbon analyzer with total N analyzer (TOC-V cpn, Shimadzu Corporation, Kyoto, Japan) in 2017 and using a Vario TOC/TN Select (Elementar Americas Inc., Ronkonkoma, NY) in 2018. Mass of extractable C in samples was calculated from the raw data using the equation:
Where C is the extractable C in a sample in μg g−1 soil, EC is the extractable C in a sample in μg mL−1 extractant, FW is the fresh weight of the sample; DW is the sample dry weight, and EV is the extractant volume.
Microbial biomass carbon was calculated from the equation:
Where MBC is the microbial biomass carbon, Cf is the extractable C in the fumigated sample, and Cu is the extractable C in the unfumigated sample. Since an extraction coefficient was not calculated from the sample, and the goal was to compare treatment means within our study and not between studies, an extraction coefficient was not used in calculating microbial biomass C.
Soil microbial community function was evaluated by measuring extracellular enzyme activities. We assessed the following seven enzymes: two cellulases ( -1,4-glucosidase [BG] and cellobiohydrolase [CBH]); a chitinase ( -1-2-N-acetylglucosaminidase [NAG]); a peptidase (leucine amino hydrolase [LAP]); urease; an enzyme responsible for releasing phosphorus from soil organic matter (phosphate-monoester phosphohydrolase [PHOS]); phenol oxidase; and peroxidase. Urease, phenol oxidase, and peroxidase were measured using colorimetric assays, all other enzymes were measured fluorometrically with corresponding methylumbelliferone (MUB) or methyl coumarin (MC) labeled substrates added to soil slurries in 96-well microplates as described in detail by Smercina et al. (2021). The Shannon diversity index was calculated from extracellular enzyme activity (Zak et al., 1994; Bending et al., 2002), using the equation:
Where pi is the ratio of activity of a particular enzyme relative to total (summed across all measured enzymes) extracellular enzyme activity.
2.5 Statistical analysis
All data were analyzed separately by year using a mixed model ANOVA with the MIXED procedure in SAS (Version 9.4, SAS Institute, Cary, NC). When necessary, data were square root or log transformed to meet assumptions of normality. Untransformed data are presented. Heterogeneous variance was corrected by using unequal variance models or with the appropriate variance–covariance structure in repeated measures analyses. Mean separations were conducted using Fisher’s protected least significant difference test at p < 0.05.
For data collected at a single time point, treatment was included as a fixed factor and block (replication) as a random factor. Since weed biomass in the cultivated treatment was negligible in 2017 and only collected in two of four treatment replicates in 2018, this treatment was excluded from total weed biomass statistical analysis. Weed seedbank data by species was summed across all sampling dates to get cumulative emergence as an estimate of the germinable seedbank by species. At the species level, high variability in estimates of germinable seedbank limited the statistical power to detect differences, so data was aggregated across all weed species.
A repeated measures mixed model ANOVA was used to analyze data collected at multiple time points or to multiple depths (i.e., soil cores). Treatment and sampling date or depth were included as fixed factors and block (replication) as a random factor. For inorganic N and soil moisture data, a first-order autoregressive variance–covariance structure was used given the roughly equally spaced timings of measurements. Soil microbial biomass, total extracellular enzyme activity, and the Shannon diversity index were determined in each treatment separately, but similarities in plant composition and the lack of significant differences in microbial response between weedy and living mulch treatments encouraged combining these treatments into a single category referred to as vegetative cover. Treatment group and sampling date were treated as fixed factors and block (replication) as a random factor. Variance–covariance structures were selected based on fit statistic results. For soil profile inorganic N, treatment and depth were treated as fixed factors and block (replication) as a random factor. An equal variance first-order autoregressive variance–covariance structure was used.
The relationships between marketable cash crop yield, cash crop tissue N, total between bed living mulch and weed biomass, soil inorganic N averaged over the growing season, and soil moisture averaged over the growing season were evaluated via Spearman’s rank correlation coefficient (rho) using the “cor.test” function in base R (Version 2023.03.1 + 446, R Core Team, 2023, Vienna, Austria).
3 Results
3.1 Weather
Average temperatures were similar in both years of the study and comparable to the 30-yr average for the location, except for higher temperatures in May 2018 (Table 4). Compared to seasonal precipitation averages, 2017 was a relatively dry season and 2018 a relatively wet season. In 2017, overhead irrigation was used for two weeks following living mulch sowing to encourage establishment. This was not necessary in 2018; heavy spring rain caused flooding in some areas of the field in that year and may have hindered living mulch establishment.
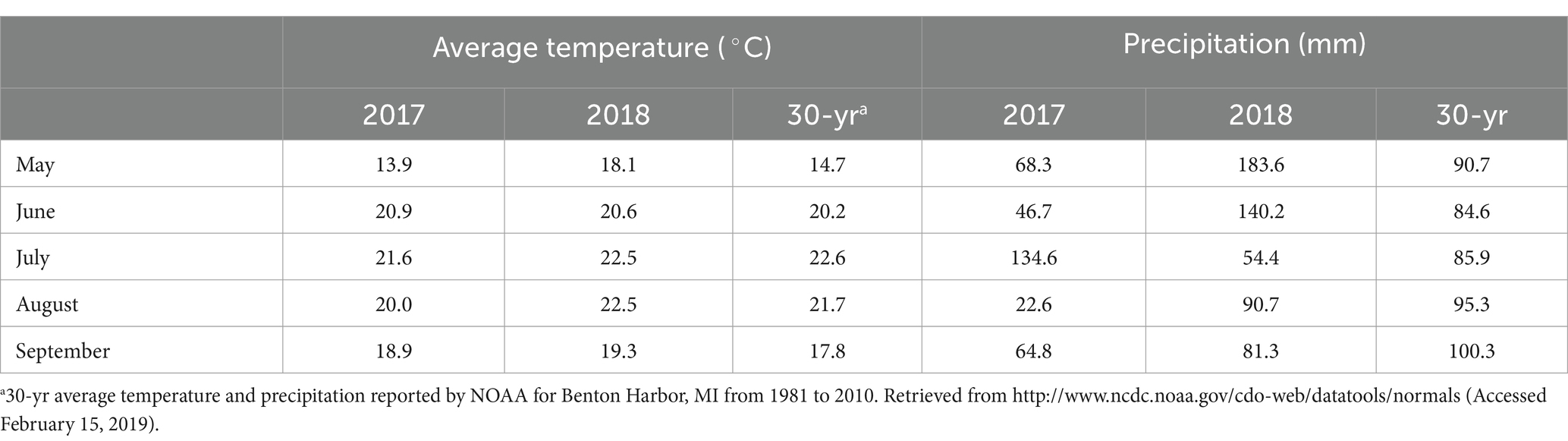
Table 4. Monthly temperature averages and precipitation totals during the 2017 and 2018 seasons and reported 30-year averages.
3.2 In-season weed control
Dominant summer annual weed species in the field included crabgrass species (Digitaria spp.), common lambsquarters (Chenopodium album L.), ladysthumb (Persicaria maculosa), oak-leaf goosefoot (Chenopodium glaucum), purslane (Portulaca oleracea L.), ragweed species (Ambrosia spp.), and wood sorrel species (Oxalis spp.). Perennial weeds including quackgrass (Elymus repens) and dock species (Rumex spp.) were less common than summer annual weeds, but present in localized areas of the field.
Cultivation and dead mulch reduced in-season weed biomass by an average of 94 and 78% respectively, more than any of the living mulch treatments (Figure 1). The cultivation schedule in 2017 resulted in these plots remaining weed-free during the season. However, in 2018, wet field conditions and delayed cultivation led to some weed growth in the cultivated treatment at the end of July. Although we are unable to statistically compare weed biomass in the cultivated treatment to the others, cumulative weed biomass production in the cultivated treatment was substantially less than weedy and living mulch treatments (Figure 1). In 2017, Italian ryegrass established quickly, producing 191 g m−2 biomass and reducing weed biomass by >50% compared to the weedy treatment (Table 5). However, heavy spring rain in 2018 contributed to poor Italian ryegrass establishment and modest biomass accumulation in that year (50.7 g m−2). In comparison, rye establishment was poor across years, and the development of leaf rust by mid-July nearly eliminated stands. The clover/rye treatment was slow to establish in 2017 and failed to establish in some plots in 2018. Neither rye nor clover/rye significantly reduced in-season weed biomass compared to the weedy treatment in either year (Table 5).
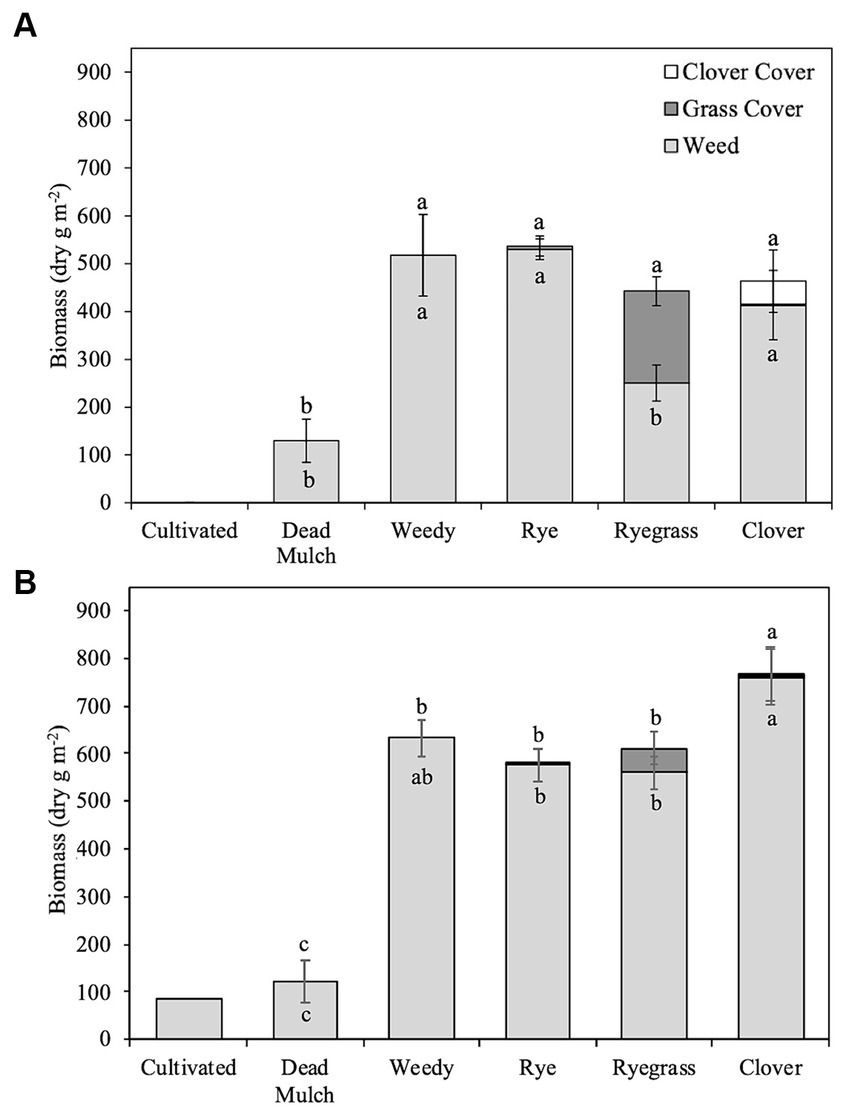
Figure 1. Mean cover and weed biomass production in (A) 2017 and (B) 2018. Error bars represent ± standard error around cumulative weed biomass mean and cumulative total (weed biomass + living mulch biomass) biomass mean. Different letters above bars represent significant differences related to cumulative total biomass production (p < 0.05). Different letters within bars represent significant differences related to cumulative weed biomass (p < 0.05).
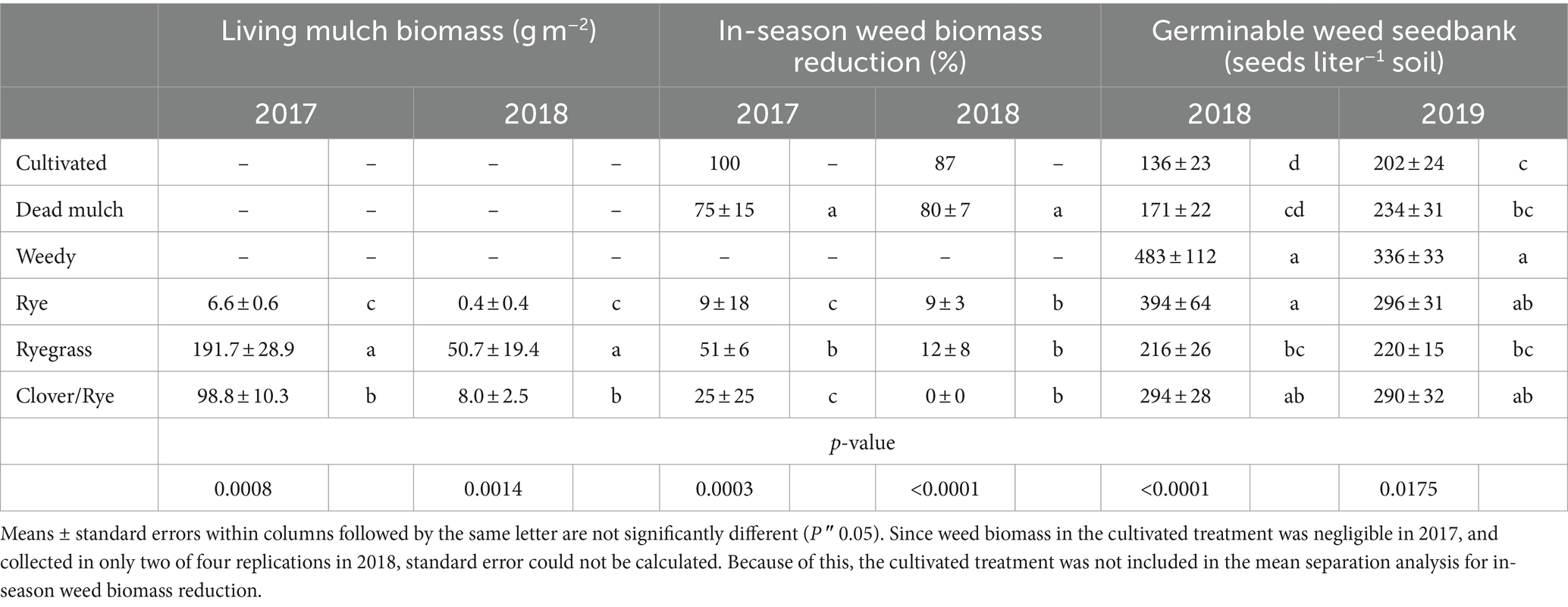
Table 5. Total living mulch biomass production and the effect of between-bed management strategy on in-season weed suppression and the germinable weed seedbank the following season.
3.3 Post-season weed seedbank
Dominant weed species found in the germinable seedbank were similar to those in the field, with the exception of perennials, which were excluded from seedbank evaluation. Overall, the most abundant species were crabgrass species, ladysthumb, and oak-leaf goosefoot.
After two years, reductions in the germinable seedbank relative to weedy treatment were detected only for the cultivation, dead mulch and Italian ryegrass treatments. The cultivated and dead mulch treatment had the smallest germinable weed seedbank, and the weedy treatment the largest after both seasons (Table 5). The 2017 pattern of in-season weed suppression was closely reflected in estimates of the germinable weed seedbank from between-bed areas in spring 2018. Compared to weedy, the reduction in the germinable seedbank increased from 18 to 72% as weed biomass suppression increased from 9 to 100% (Table 5). Among cover crops grown in 2017, the Italian ryegrass treatment had the greatest impact on weeds, reducing both weed biomass and subsequent weed seedbank density by more than half of that observed in the weedy treatment. Treatments applied in 2018 generally had less impact on the seedbank than those from 2017, and seedbank effects were not as closely associated with weed biomass suppression. Despite minimal in-season weed suppression by Italian ryegrass in 2018 (12%), the weed seedbank in that treatment was reduced by 36% relative to weedy and was comparable to that in both the dead mulch and cultivated treatments at experiment termination (Table 5). Although in-season weed control by Italian ryegrass living mulch was variable and small relative to cultivation and dead mulch, it still reduced the germinable weed seedbank across both years of our study compared to the weedy treatment. In contrast, rye and the clover/rye mixture living mulches did not reduce the weed seedbank compared to weedy in either year.
3.4 Cash crop performance
Squash performance metrics were unaffected by between-bed treatment in both years, and in 2018 pepper performed similarly across all treatments (Table 6). However, in 2017 living and dead mulch treatments resulted in pepper yield losses of between 41 and 54%, and we detected a negative correlation between total living mulch and weed biomass between plastic mulch beds and marketable pepper yield (Table 7). Total N concentration in pepper leaves sampled at the last harvest in 2017 was greater in the cultivated treatment relative to all other treatments (Table 6). Soil inorganic N levels in the crop row ranged from 28 to 57 mg N kg soil−1 in the weedy and rye treatments respectively, and no significant differences were resolved among treatments (Figure 2B). Correlation analysis revealed no relationship between marketable yield and in-row soil inorganic N, but a positive correlation between marketable pepper yield and in-row soil moisture averaged across sampling dates in 2017 (Table 7). Soil moisture under plastic mulch was numerically higher in the cultivated control compared to all other treatments during pepper fruit development in 2017 (Figure 2A). In 2018, pepper marketable yield was comparable across treatments, and no significant differences in in-bed soil moisture (Figure 2C) or soil inorganic N levels (Figure 2D) were detected in pepper beds.
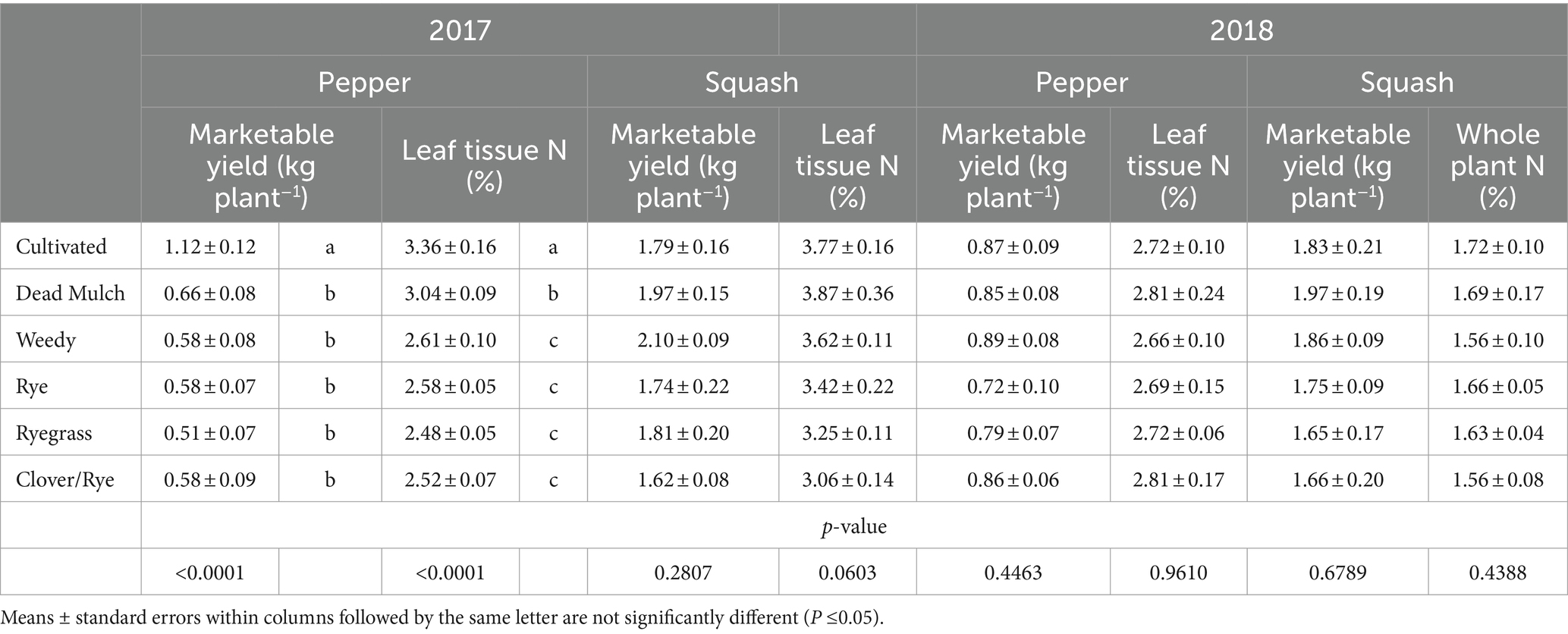
Table 6. Effect of between-bed management on marketable cash crop yield and leaf tissue N concentration at final harvest in 2017 and 2018.
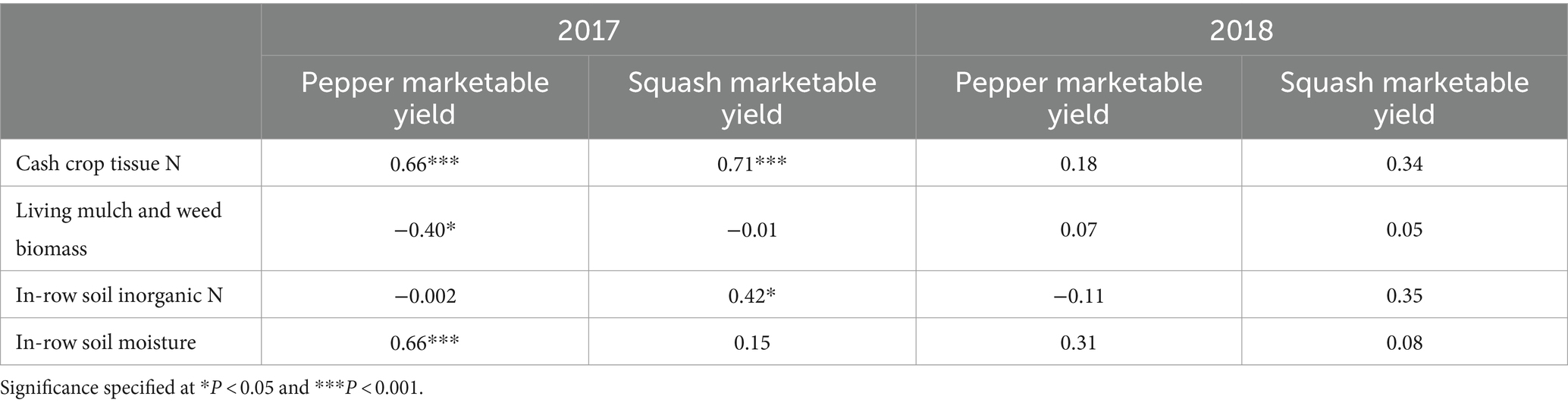
Table 7. Spearman’s rank correlations (rho) between pepper and squash marketable yield and cash crop tissue N, between-bed living mulch and weed biomass, average in-row soil inorganic N, and average in-row soil volumetric water content.
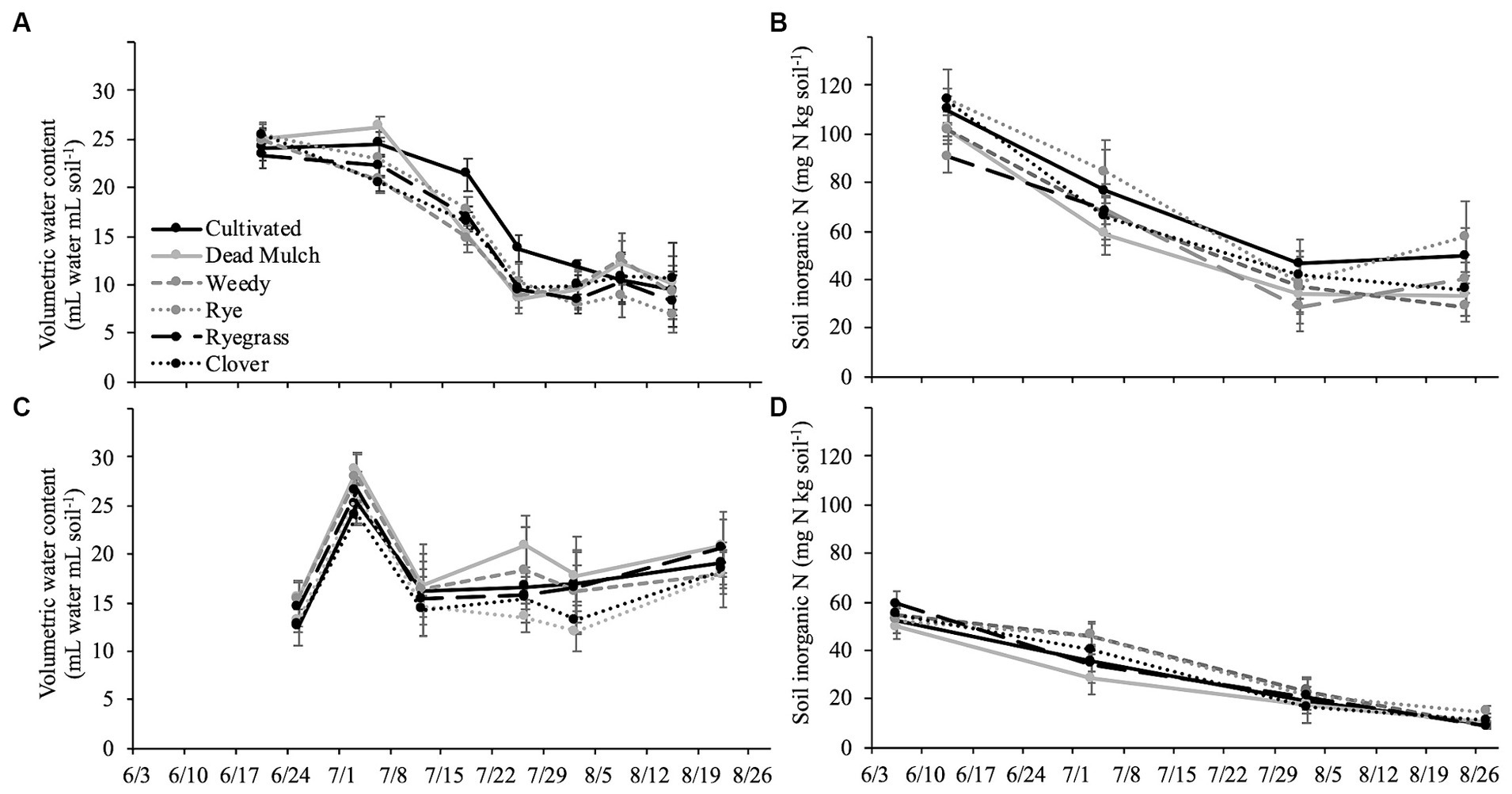
Figure 2. Mean of in-row soil moisture in top 20 cm of pepper beds in (A) 2017 and (C) 2018 and mean in-row inorganic nitrogen in (B) 2017 and (D) 2018. Error bars represent ± standard error.
3.5 Organic matter contributions and soil organic matter
Living mulch establishment was a challenge and weed biomass on average accounted for 99, 74, and 94% of organic matter inputs in rye, ryegrass, and clover/rye treatments, respectively. Italian ryegrass produced significantly more biomass than rye or clover/rye in both years (Table 5). Total shoot biomass inputs in the weedy and living mulch treatments averaged 490 g m−2 in 2017 and 647 g m−2 in 2018 (Figure 1). In comparison, dead mulch was applied at an average rate of 18 Mg ha−1 and had an additional input of 130 g m−2 of weed shoot biomass in 2017 and 122 g m−2 in 2018.
At trial initiation, we detected significantly greater SOM levels in weedy treatment plots relative to all others, a random spatial effect not attributable to treatments. SOM in the between-bed areas of the weedy treatment was again significantly greater than all other treatments at trial termination. However changes in SOM in between-bed areas over the course of the experiment were comparable across treatments, decreasing by an average of 0.2% (Table 8).
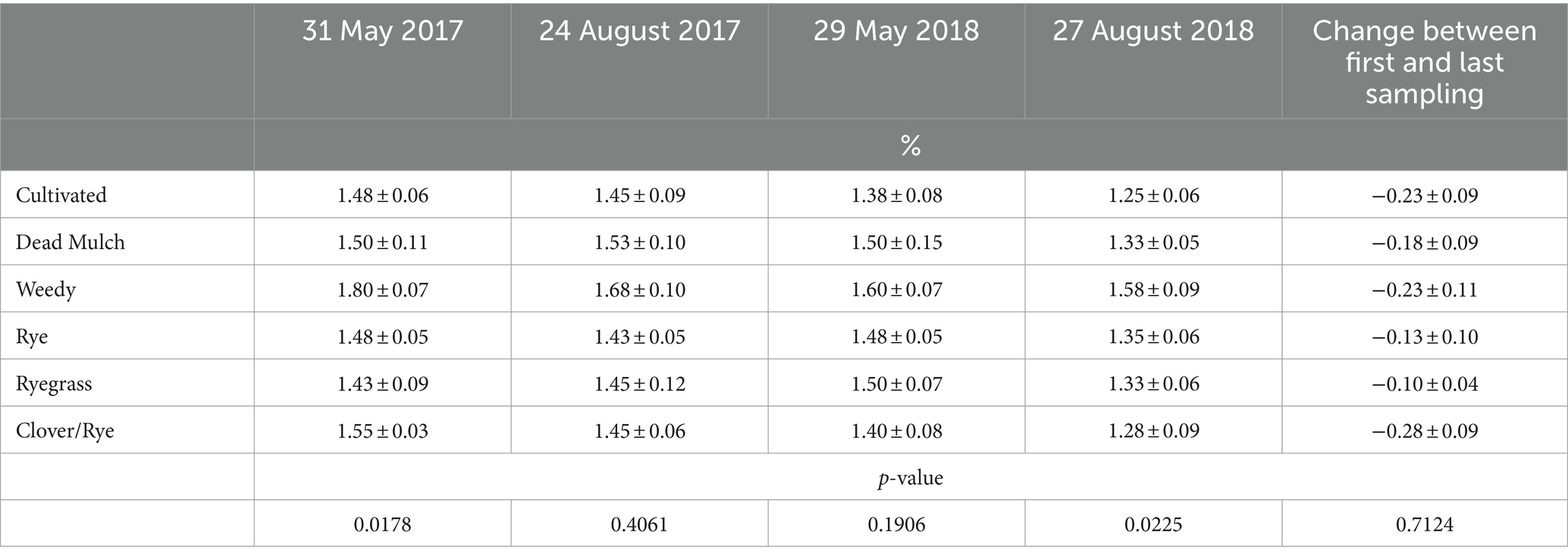
Table 8. Means ± standard errors of soil organic matter content in between-bed areas during the study.
3.6 Soil microbial community
Given similarities in plant community composition between living mulch treatments and the weedy treatment (i.e., dominated by the ambient weed community), these treatments were collapsed into a single treatment category referred to as “vegetative cover” from here forward.
No significant differences were observed in microbial biomass C between cultivated, dead mulch, or vegetative cover plots in either year (Figures 3A,B).
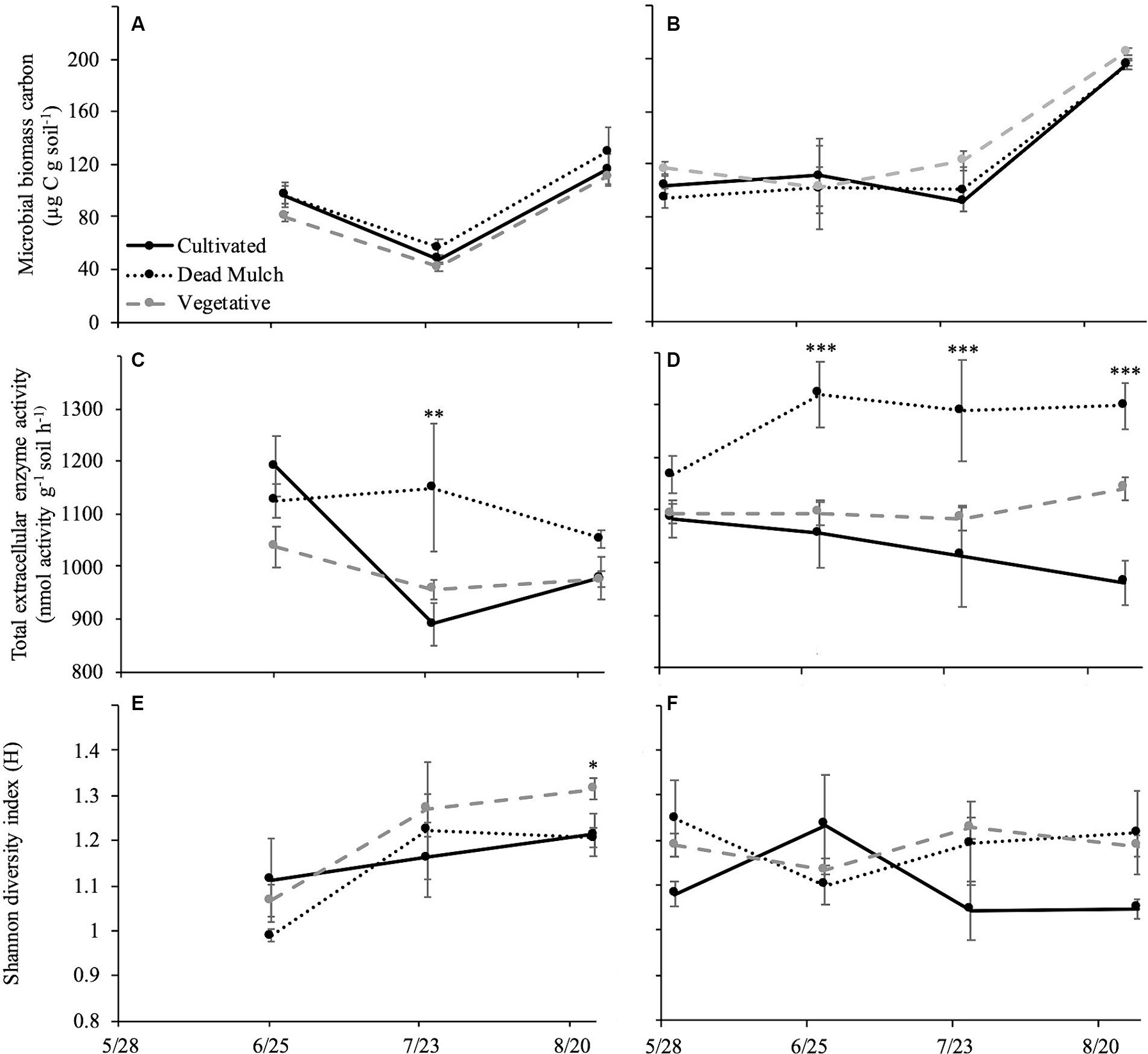
Figure 3. Mean of microbial biomass carbon in (A) 2017 and (B) 2018; total extracellular enzyme activity in (C) 2017 and (D) 2018; and Shannon diversity index in (E) 2017 and (F) 2018. Error bars represent ± standard error. Significant specified at *p < 0.05, **p < 0.01, ***p < 0.001.
By the end the of 2017 season, total extracellular enzyme activity was similar across treatments (Figure 3C). This was true at the beginning of the 2018 season too, but the treatments began separating in June (Figure 3D). As the season progressed, the dead mulch treatment consistently had the greatest total enzyme activity, with vegetative cover as an intermediate, and cultivation having the lowest (Figure 3D).
Significant differences in the Shannon Diversity Index were observed between treatments at the end of the 2017 season (Figure 3E). However, this pattern did not carry over into 2018. No significant differences between treatments were detected for the entirety of the 2018 season. However, in 2018 the diversity of enzyme activities was on average greater in dead mulch and the vegetative cover treatments than the cultivated treatment (Figure 3F).
3.7 End of season soil profile nitrogen
Where plants were actively growing between plastic mulch beds during the 2017 season, potentially leachable N in the soil profile (0–60 cm) was reduced by approximately 61% (Figure 4A). Differences in soil profile N were most pronounced in the top 20 cm and decreased with increasing soil depth. In 2018 no significant differences in potentially leachable nitrogen were observed (Figure 4B).
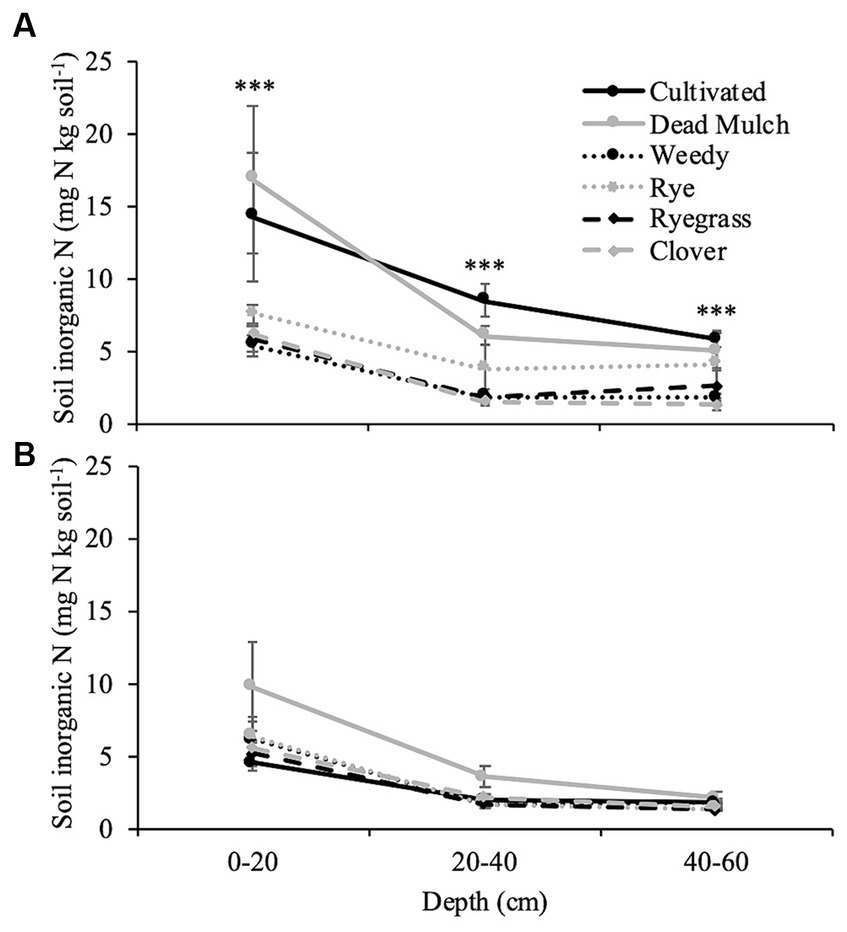
Figure 4. Mean of inorganic nitrogen at depth measured before fall tillage in (A) 2017 and (B) 2018. Error bars represent ± standard error. Significance specified at ***p < 0.001.
4 Discussion
4.1 Weed management
Our results confirm the importance of living mulch establishment and biomass accumulation in controlling weeds (e.g., Bybee-Finley et al., 2017; Alonso-Ayuso et al., 2018), and highlight the inherent risks of managing weeds with cover crops (Teasdale, 1996; Sarrantonio and Gallandt, 2003; Fernando and Shrestha, 2023). The relatively slow growth rate of clover and stress experienced by rye planted outside of its climatic niche made these species unable to compete with aggressive weeds, results confirmed elsewhere (Reid and Klotzbach, 2013). Italian ryegrass was better suited for this niche, but performance was still variable between years. In previous work we found that teff (Eragrostis tef) was the only living mulch treatment among nine to significantly reduce in-season weed biomass between plastic mulched beds (Tarrant et al., 2020). In both cases, weed suppression by Italian ryegrass and teff was variable across years, but these results suggest that vigorous summer annual grasses may be better suited for this niche than slower growing clovers and winter grains.
The close relationship between weed biomass suppression and weed seedbank suppression observed in the first year of this study suggests that competition effects on seed production played an important role in explaining seedbank effects. Reductions in weed seed production and seed viability in the presence of competition have been previously demonstrated in agronomic cropping systems (Nurse and DiTommaso, 2005) and in response to living mulches within vegetable cropping systems (Brainard and Bellinder, 2004; Brainard et al., 2005). However, treatment differences in the germinable seedbank may also have been influenced by indirect effects on the rates of predation, decay, or fatal germination of weed seeds. Seed burial and predation studies demonstrate that tillage and cover crops can accelerate the rate of seed loss through impacts on soil micro-organisms, seed predators, or fatal germination (Gallandt et al., 2004; Mohler et al., 2018; Frost et al., 2019; Fernando and Shrestha, 2023).
Not surprisingly, mowing ambient weeds in our study led to increases in the weed seedbank compared to cultivation, dead mulching, and Italian ryegrass living mulch. While the weed seed bank can serve as a “free” living mulch in subsequent seasons when managed this way (Hartwig and Ammon, 2002), unchecked weeds may be more competitive and harder to control than a cover crop living mulch (Westbrook et al., 2021). Mowing may also lead to a shift in the ambient weed community composition toward difficult to manage and competitive species (Butler et al., 2013). Because of the impact on future management, these potential drawbacks of mowing ambient weeds are important for growers to consider.
4.2 Cash crop performance
The challenge of competition between cash crops and living mulches in integrated plasticulture living mulch systems has been documented in several previous studies (Table 1). In our study, summer squash was particularly robust to competition, with yields unaffected by between-bed management in either year (Table 5). We observed that the squash canopy shaded plants most likely to compete for in-bed resources along bed edges (Figure 5). These results are aligned with previous work in plasticulture acorn squash (Cucurbita pepo cv. Table Ace) production (Nelson and Gleason, 2018), but contrast with results from Bruce et al. (2022) where researchers found that clover/ryegrass and ryegrass living mulch between plastic mulched beds reduced zucchini (Cucurbita pepo cv. Dunja F1) yields compared to a cultivated and straw mulch control. These diverging results highlight the complex interactions between cash crop and living mulch species, management, and environmental factors that mediate competitive dynamics (Dzvene et al., 2023).
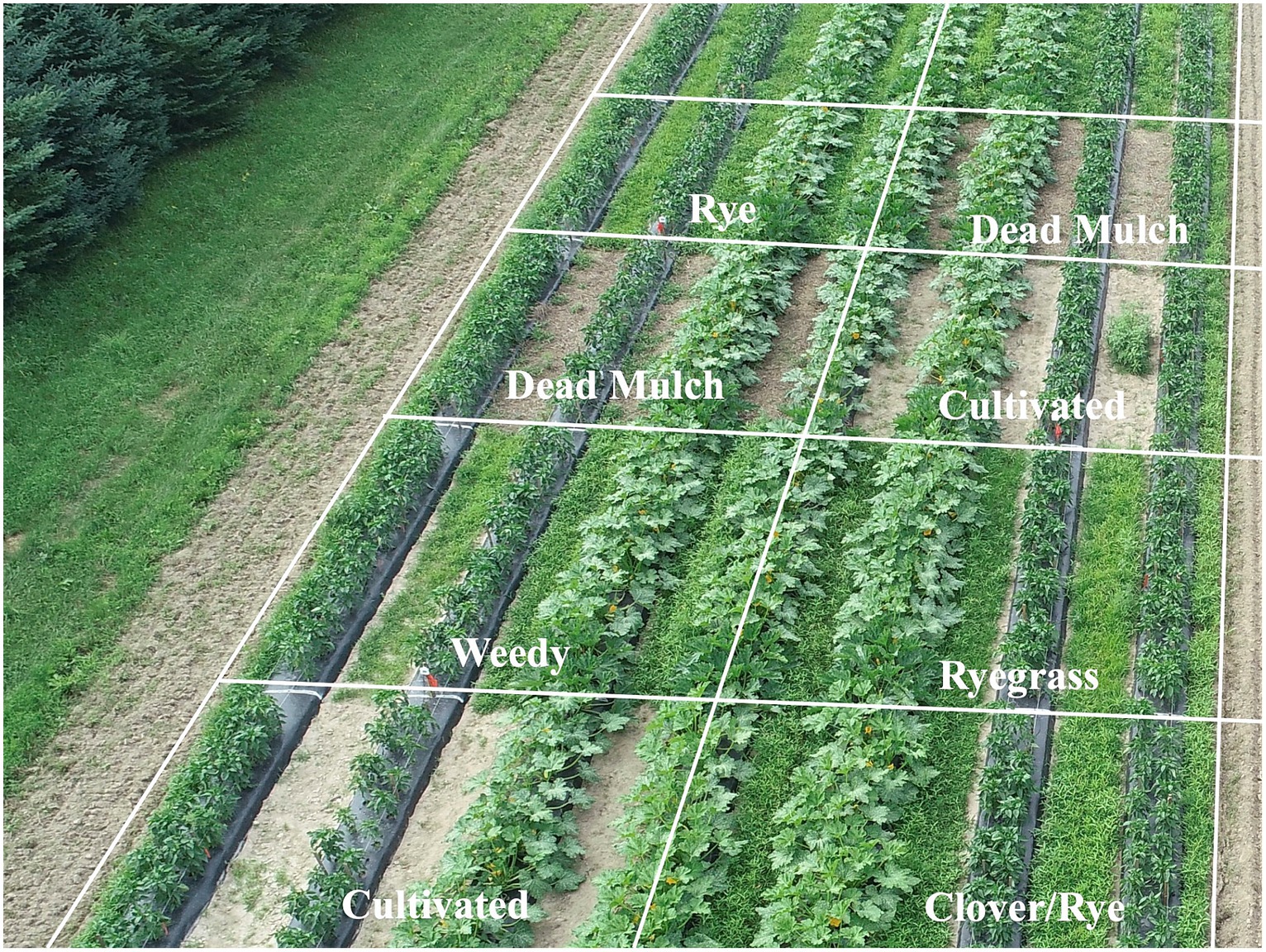
Figure 5. Overhead photograph of bell pepper and summer squash plants grown on black plastic mulch with different between-bed management treatments taken on July 12, 2018. Differences in cash crop stature, namely squash leaves reaching over bed edges shading between-bed weeds and living mulches, may have caused squash to be more robust to competition than pepper.
Pepper performance in our study was variable across study years, suggesting it may be more susceptible to competitive interference than squash. Law et al. (2006) also documented bell pepper yield reductions when living mulches or weeds were grown between plastic mulched beds. The researchers speculated yield reductions were driven by competition, but competitive mechanisms were not investigated. A negative relationship between marketable yield and between-bed living mulch and weed biomass suggest competition hindered pepper performance in 2017, driven by water limitations (Table 7 and Figure 2A). Reduced pepper leaf N content in all treatments compared to the cultivated control also suggest competition for N (Table 6). However, no significant differences in soil inorganic N levels in the crop row were resolved among treatments, and considerable plant available N remained under pepper plastic mulch beds at the end of the season. Pepper N uptake may therefore have been limited by soil moisture rather than N availability. Interestingly, the dead mulch treatment also reduced pepper yields in 2017. Weed escapes in dead mulch treatments were concentrated along bed edges where they may have been most likely to compete for in-bed resources, likely driving this result.
We would expect supplemental irrigation and/or fertility to help mitigate competition. However, Warren et al. (2015) found that even after increasing fertility to eliminate potential N competition and ensuring adequate irrigation, yields of broccoli (Brassica oleracea L. var. italica) grown on plastic mulched beds were reduced where living mulch was grown in the between-bed areas compared to cultivation. Alternative explanations for cash crop interference by living mulches and weeds in this case and in our study include a cooler microclimate caused by between-bed plant transpiration and the induction of a shade avoidance response.
Additional strategies could be employed to mitigate potential competition, for instance keeping the between-bed area weed-free for some critical period unique to plasticulture systems before planting living mulch, applying a dead mulch, or mowing ambient weeds. Research in inter-seeding living mulches in vegetable cropping systems has revealed great promise in delayed living mulch planting to mitigate yield penalties (Brainard and Bellinder, 2004; Gibson et al., 2011; Ciaccia et al., 2015). Though previous research has been done to evaluate the influence of in-bed weeds on plasticulture vegetable production (Buckelew et al., 2006; Norsworthy et al., 2008; Garvey et al., 2013; Chaudhari et al., 2016; Bertucci et al., 2019), research toward identifying the critical weed-free period for plants growing between plastic mulch beds is limited.
4.3 Soil health and nutrient cycling indicators
By contributing organic matter inputs, building microbial biomass, and physically protecting SOM from erosion, planting cover crops in fallow windows can increase SOM stocks over time (Kallenbach and Grandy, 2011; Peng et al., 2023). The ambient weed pressure at our study site was high enough to ensure complete soil coverage and resulted in biomass contributions comparable to our living mulch treatments, showing that in some cases the ambient weed community can be functionally like a cover crop in terms of biomass contributions. The maintenance of vegetative covers between plastic mulched beds in our trial led to average shoot residue contributions of 4,900 kg ha−1 and 6,460 kg ha−1 to between-bed areas (approximately 55% of the field) in 2017 and 2018 respectively, in addition to unmeasured root contributions. Considering typical biomass production of a productive summer cover crop like sorghum-sudangrass is 4,000–6,000 kg ha−1 in the north central United States (Clark, 2007), this represents a significant addition of organic material. However, we did not find a detectable difference in SOM changes between trial initiation and termination among treatments. The relatively short duration of our study (2 years) combined with the difficulty in detecting changes in bulk SOM on sandy soils (Tiemann and Grandy, 2015) may explain similar changes in SOM across our treatments. It should also be noted that SOM was evaluated before living and dead mulch incorporation at experiment termination in the second year of the study, which may have underestimated the impact of residues on SOM.
Since organic matter inputs act as substrate for soil microbes, we expected microbial abundance would be elevated in all treatments compared to the cultivated control. We further expected that the greatest increases may be observed in vegetative cover treatments based on past research showing that cover crop rhizo-deposition alone can stimulate microbial growth (Finney et al., 2017), in some cases more than surface applied dead mulches (Buyer et al., 2010). However, microbial biomass C was unaffected by our treatments at any sampling date (Figure 3). Discrepancies between our results and those from previous work may be explained by methodological differences including how MBC was measured and when it was measured in relation to cover crop growth. The chloroform-fumigation method is a course resolution assessment of microbial biomass and often does not capture small changes through time (Tiemann and Billings, 2011). Distinct soil types and the study site climate are also likely to mediate results, with the effects of cover crops on soil microbial communities more likely to accumulate on medium-textured compared to fine- or coarse-textured soils (Buyer et al., 2010; Muhammad et al., 2021).
Despite no differences in microbial abundance between our treatments, we did observe greater overall extracellular enzyme activity in dead mulch and vegetative cover plots at the last cash crop harvest. This result suggests improved nutrient recycling within these management strategies compared to cultivation (Xiao et al., 2018), and aligns with a meta-analysis that found removal of plant litter and living roots reduces extracellular enzyme activity in agroecosystems (Ai et al., 2023). This also provides some evidence for improved soil health, although additional time may be needed to realize and detect benefits. Based on the Shannon diversity index calculated from extracellular enzyme activity data, microbial communities appeared to be functionally similar across our treatments. Previous research has shown that organic matter input quality can influence enzyme activity diversity (Bending et al., 2002). However, Bending et al. (2002) evaluated extracellular enzyme activity following residue incorporation, whereas the smaller organic inputs derived from surface mulch and living roots during our sampling period may reduce the likelihood of detecting microbial community effects.
The presence of living cover between plastic mulch beds may also benefit system N cycling by scavenging mineral N unlikely to be accessed by the cash crop (Xie et al., 2017). In fact, end of season soil profile (0–60 cm) N sampling in 2017 demonstrated a 61% reduction in potentially leachable N in living mulch and weedy treatments compared to cultivated and dead mulch treatments (Figure 4A). While these results were not replicated in 2018, significant in-season N losses prior to sampling may have reduced the potential to resolve differences by season’s end. In particular, heavy spring rain in May and June of that year may have promoted early season N losses via leaching and denitrification. Nevertheless, greater N uptake by living mulches and weeds in between-bed areas are likely to reduce N losses relative to bare cultivation. However, the benefit of potential reductions in N losses from between-bed areas must be balanced with the potential for competition with cash crops for in-bed N resources (Xie et al., 2017).
4.4 Conclusions and future research
This research contributes to a limited body of literature quantifying systems tradeoffs between common organic grower practices for managing weeds and soil between plastic mulch beds versus living mulch alternatives. Within the context of our study, living mulches integrated into plasticulture production had limited potential to provide adequate weed control, introduced some risk to cash crop yields, and had minimal impacts on short-term soil health metrics despite notable organic matter inputs. However, the potential for soil health benefits to accrue over time, along with other possible benefits of living mulches not measured in this study, including biological pest control, increased farm biodiversity, reduced soil erosion, and improved harvesting conditions, continue to make this practice an attractive alternative to other between-bed management practices. Growers interested in planting living mulches between plastic mulch beds should proceed with caution, acknowledging variable weed control and potential risks to cash crop productivity. Future research identifying management levers on living mulch establishment, including species selection and appropriate establishment conditions could improve the weed suppressive ability of living mulches. In terms of living mulch species selection, summer annual grass species appear to be better suited than winter grasses or clovers to this niche. Additional research into irrigation and fertility management modifications could help mitigate competition between cash crops and living mulches. Identifying the critical weed-free period for between plastic mulch beds could help further mitigate competition and inform living mulch management.
Data availability statement
The original contributions presented in the study are included in the article/supplementary material, further inquiries can be directed to the corresponding authors.
Author contributions
AT: Conceptualization, Data curation, Formal analysis, Investigation, Methodology, Validation, Visualization, Writing – original draft, Writing – review & editing. DB: Conceptualization, Supervision, Writing – review & editing, Methodology, Funding acquisition, Resources. LT: Methodology, Writing – review & editing, Resources. ZH: Conceptualization, Data curation, Funding acquisition, Investigation, Methodology, Project administration, Resources, Supervision, Validation, Writing – review & editing.
Funding
The author(s) declare financial support was received for the research, authorship, and/or publication of this article. This research was supported by funding from the Organic Agriculture Research and Extension Initiative (OREI) Grant No. 2016-04458 from the U.S. Department of Agriculture National Institute of Food and Agriculture, North Central Region Sustainable Agriculture Research and Education (NCR-SARE) project number GNC17-251, and Michigan State University AgBioResearch.
Acknowledgments
We would like to thank Colin Phillippo, Michael Metiva, Victoria Lawless, Eryn Damon, Sam Callow, Genny Feister, Austin Green, and Patrick Squire for their help in the field and laboratory. Thank you too to Alexia Witcombe, Darian Smercina, and Andrew Curtright for their help specifically with soil microbial community analyses. Thanks also to Daniel Priddy for comments on an earlier version of this manuscript.
Conflict of interest
The authors declare that the research was conducted in the absence of any commercial or financial relationships that could be construed as a potential conflict of interest.
Publisher’s note
All claims expressed in this article are solely those of the authors and do not necessarily represent those of their affiliated organizations, or those of the publisher, the editors and the reviewers. Any product that may be evaluated in this article, or claim that may be made by its manufacturer, is not guaranteed or endorsed by the publisher.
References
Agriculture Marketing Service. (2005). United States Standards for Grades of Sweet Peppers. Available at: https://www.ams.usda.gov/grades-standards/sweet-peppers-grades-and-standards (Accessed January 3, 2019)
Ai, L., Wu, F., Fan, X., Yang, Y., Zhang, Y., Zheng, X., et al. (2023). Different effects of litter and root inputs on soil enzyme activities in terrestrial ecosystems. Appl. Soil Ecol. 183:104764. doi: 10.1016/j.apsoil.2022.104764
Alonso-Ayuso, M., Gabriel, J. L., García-González, I., Del Monte, J. P., and Quemada, M. (2018). Weed density and diversity in a long-term cover crop experiment background. Crop Prot. 112, 103–111. doi: 10.1016/j.cropro.2018.04.012
Arnold, G. L., Luckenbach, M. W., and Unger, M. A. (2004). Runoff from tomato cultivation the estuarine environment: biological effects of farm management practices. J. Exp. Mar. Biol. Ecol. 298, 323–346. doi: 10.1016/S0022-0981(03)00366-6
Bending, G. D., Turner, M. K., and Jones, J. E. (2002). Interactions between crop residue and soil organic matter quality and the functional diversity of soil microbial communities. Soil Biol. Biochem. 34, 1073–1082. doi: 10.1016/S0038-0717(02)00040-8
Bertucci, M. B., Jennings, K. M., Monks, D. W., Schultheis, J. R., Louws, F. J., Jordan, D. L., et al. (2019). Critical period for weed control in grafted and nongrafted watermelon grown in plasticulture. Weed Sci. 67, 221–228. doi: 10.1017/wsc.2018.76
Bhaskar, V., Westbrook, A. S., Bellinder, R. R., and DiTommaso, A. (2021). Integrated management of living mulches for weed control: a review. Weed Technol. 35, 856–868. doi: 10.1017/wet.2021.52
Brainard, D. C., and Bellinder, R. R. (2004). Weed suppression in a broccoli–winter rye intercropping system. Weed Sci. 52, 281–290. doi: 10.1614/WS-03-031R
Brainard, D. C., Bellinder, R. R., and DiTommaso, A. (2005). Effects of canopy shade on the morphology, phenology, and seed characteristics of Powell amaranth (Amaranthus powellii). Weed Sci. 53, 175–186. doi: 10.1614/WS-04-067R1
Brown, B., and Gallandt, E. R. (2018). A Systems Comparison of Contrasting Organic Weed Management Strategies. Weed Sci. 66, 109–120. doi: 10.1017/wsc.2017.34
Bruce, D., Silva, E. M., and Dawson, J. C. (2022). Suppression of weed and insect populations by living cover crop mulches in organic squash production. Front. Sustainable Food Syst. 6:995224. doi: 10.3389/fsufs.2022.995224
Buckelew, J. K., Monks, D. W., Hoyt, G. D., and Walls, R. F. Jr. (2006). Eastern Black nightshade (Solanum ptycanthum) reproduction and interference in transplanted Plasticulture tomato. Weed Sci. 54, 490–495. doi: 10.1614/WS-05-060R.1
Butler, R. A. (2012) Evaluation of cover crops and mowing as alternative approaches for weed control in organic tomato production. Purdue University, West Lafayette, IN, Master’s thesis. Available at: http://search.proquest.com.proxy1.cl.msu.edu/docview/1220693576/abstract/162EA7431ABF4BB1PQ/1 (Accessed April 1, 2019)
Butler, R. A., Brouder, S. M., Johnson, W. G., and Gibson, K. D. (2013). Response of four summer annual weed species to mowing frequency and height. Weed Technol. 27, 798–802. doi: 10.1614/WT-D-12-00112.1
Buyer, J. S., Teasdale, J. R., Roberts, D. P., Zasada, I. A., and Maul, J. E. (2010). Factors affecting soil microbial community structure in tomato cropping systems. Soil Biol. Biochem. 42, 831–841. doi: 10.1016/j.soilbio.2010.01.020
Bybee-Finley, K. A., Mirsky, S. B., and Ryan, M. R. (2017). Crop biomass not species richness drives weed suppression in warm-season annual grass–legume intercrops in the northeast. Weed Sci. 65, 669–680. doi: 10.1017/wsc.2017.25
Chaudhari, S., Jennings, K. M., Monks, D. W., Jordan, D. L., Gunter, C. C., McGowen, S. J., et al. (2016). Critical period for weed control in grafted and nongrafted fresh market tomato. Weed Sci. 64, 523–530. doi: 10.1614/WS-D-15-00049.1
Ciaccia, C., Montemurro, F., Campanelli, G., Diacono, M., Fiore, A., and Canali, S. (2015). Legume cover crop management and organic amendments application: effects on organic zucchini performance and weed competition. Sci. Hortic. 185, 48–58. doi: 10.1016/j.scienta.2015.01.011
Clark, A. J. (2007). Managing cover crops profitably (3rd ed.). Sustainable Agriculture Network, Beltsville, MD.
Combs, S. M., and Nathan, M. V. (1998). “Soil organic matter” in Recommended chemical soil test procedures for the north central region. ed. J. R. Brown (Columbia: Missouri Agricultural Experiment Station), 53–58.
DeDecker, J. J., Masiunas, J. B., Davis, A. S., and Flint, C. G. (2014). Weed Management Practice Selection Among Midwest U.S. Organic Growers. Weed Sci. 62, 520–531. doi: 10.1614/WS-D-13-00138.1
Dzvene, A. R., Tesfuhuney, W. A., Walker, S., and Ceronio, G. (2023). Management of cover crop intercropping for live mulch on plant productivity and growth resources: a review. Air Soil Water Res. 16:11786221231180079. doi: 10.1177/11786221231180079
Fernando, M., and Shrestha, A. (2023). The potential of cover crops for weed management: a sole tool or component of an integrated weed management system? Plants 12:752. doi: 10.3390/plants12040752
Finney, D. M., Buyer, J. S., and Kaye, J. P. (2017). Living cover crops have immediate impacts on soil microbial community structure and function. J. Soil Water Conserv. 72, 361–373. doi: 10.2489/jswc.72.4.361
Frost, M. D., Haramoto, E. R., Renner, K. A., and Brainard, D. C. (2019). Tillage and cover crop effects on weed seed persistence: do light exposure and fungal pathogens play a role? Weed Sci. 67, 103–113. doi: 10.1017/wsc.2018.80
Gallandt, E. R., Fuerst, E. P., and Kennedy, A. C. (2004). Effect of tillage, fungicide seed treatment, and soil fumigation on seed bank dynamics of wild oat (Avena fatua). Weed Sci. 52, 597–604. doi: 10.1614/WS-03-078R
Gallandt, E. R., Liebman, M., Corson, S., Porter, G. A., and Ullrich, S. D. (1998). Effects of pest and soil management systems on weed dynamics in potato. Weed Sci. 46, 238–248. doi: 10.1017/S0043174500090482
Garvey, P. V., Meyers, S. L., Monks, D. W., and Coble, H. D. (2013). Influence of palmer Amaranth (Amaranthus palmeri) on the critical period for weed control in plasticulture-grown tomato. Weed Technol. 27, 165–170. doi: 10.1614/WT-D-12-00028.1
Gibson, K. D., McMillan, J., Hallett, S. G., Jordan, T., and Weller, S. C. (2011). Effect of a living mulch on weed seed banks in tomato. Weed Technol. 25, 245–251. doi: 10.1614/WT-D-10-00101.1
Hartwig, N. L., and Ammon, H. U. (2002). Cover crops and living mulches. Weed Sci. 50, 688–699. doi: 10.1614/0043-1745(2002)050[0688:AIACCA]2.0.CO;2
Ivy, A., Davis, M., Reid, J., and Reiners, S. (2014). Inter-row cover crops for plasticulture vegetables. Available at: http://www.nnyagdev.org/wp-content/uploads/2011/12/NNYADP2014IvyInterRowCCReportText.pdf (Accessed ‑April 1, 2019)
Kallenbach, C., and Grandy, A. S. (2011). Controls over soil microbial biomass responses to carbon amendments in agricultural systems: a meta-analysis. Agric. Ecosyst. Environ. 144, 241–252. doi: 10.1016/j.agee.2011.08.020
Kasirajan, S., and Ngouajio, M. (2012). Polyethylene and biodegradable mulches for agricultural applications: a review. Agron. Sustain. Dev. 32, 501–529. doi: 10.1007/s13593-011-0068-3
Lamont, W. J. (2017). “Plastic mulches for the production of vegetable crops” in A guide to the manufacture, performance, and potential of plastics in agriculture. ed. M. D. Orzolek (Cambridge, MA: Elsevier), 45–60.
Law, D. M., Rowell, A. B., Snyder, J. C., and Williams, M. A. (2006). Weed control efficacy of organic mulches in two organically managed bell pepper production systems. HortTechnology 16, 225–232. doi: 10.21273/HORTTECH.16.2.0225
Mohler, C. L., Taylor, A. G., DiTommaso, A., Hahn, R. R., and Bellinder, R. R. (2018). Effects of incorporated rye and hairy vetch cover crop residue on the persistence of weed seeds in the soil. Weed Sci. 66, 379–385. doi: 10.1017/wsc.2017.80
Muhammad, I., Wang, J., Sainju, U. M., Zhang, S., Zhao, F., and Khan, A. (2021). Cover cropping enhances soil microbial biomass and affects microbial community structure: a meta-analysis. Geoderma 381:114696. doi: 10.1016/j.geoderma.2020.114696
Nelson, H., and Gleason, M. (2018). Comparing between-row mulches in organic muskmelon and squash – year 2. Farm Prog. Rep. 2017 16–18. doi: 10.31274/farmprogressreports-180814-1942
Norsworthy, J. K., Oliveira, M. J., Jha, P., Malik, M., Buckelew, J. K., Jennings, K. M., et al. (2008). Palmer amaranth and large crabgrass growth with plasticulture-grown bell pepper. Weed Technol. 22, 296–302. doi: 10.1614/WT-07-043.1
Nurse, R. E., and DiTommaso, A. (2005). Corn competition alters the germinability of velvetleaf (Abutilon theophrasti) seeds. Weed Sci. 53, 479–488. doi: 10.1614/WS-04-185R1
Peng, Y., Rieke, E. L., Chahal, I., Norris, C. E., Janovicek, K., Mitchell, J. P., et al. (2023). Maximizing soil organic carbon stocks under cover cropping: insights from long-term agricultural experiments in North America. Agric. Ecosyst. Environ. 356:108599. doi: 10.1016/j.agee.2023.108599
R Core Team (2023). R: A language and environment for statistical Computing. R Foundation for Statistical Computing, Vienna, Austria. Available at: https://www.R-project.org/.
Reid, J., and Klotzbach, K. (2013). Final Report for ONE12-171. Available at: https://projects.sare.org/project-reports/one12-171/ (Accessed April 1, 2019)
Reiners, S., and Wickerhauser, O. (1995). The use of Rye as a living mulch to control weeds in bell pepper production. HortScience 30:892D. doi: 10.21273/HORTSCI.30.4.892D
Rice, P. J., Harman-Fetcho, J. A., Teasdale, J. R., Sadeghi, A. M., McConnell, L. L., Coffman, C. B., et al. (2004). Use of vegetative furrows to mitigate copper loads and soil loss in runoff from polyethylene (plastic) mulch vegetable production systems. Environ. Toxicol. Chem. 23, 719–725. doi: 10.1897/03-14
Salama, K., and Geyer, M. (2023). Plastic mulch films in agriculture: their use, environmental problems, recycling and alternatives. Environments 10:179. doi: 10.3390/environments10100179
Sarrantonio, M., and Gallandt, E. (2003). The role of cover crops in North American cropping systems. J. Crop. Prod. 8, 53–74. doi: 10.1300/J144v08n01_04
Smercina, D. N., Evans, S. E., Friesen, M. L., and Tiemann, L. K. (2021). Temporal dynamics of free-living nitrogen fixation in the switchgrass rhizosphere. GCB Bioenergy 13, 1814–1830. doi: 10.1111/gcbb.12893
Snapp, S. S., Swinton, S. M., Labarta, R., Mutch, D., Black, J. R., Leep, R., et al. (2005). Evaluating cover crops for benefits, costs and performance within cropping system niches. Agron. J. 97, 322–332. doi: 10.2134/agronj2005.0322a
Soil Survey Staff, USDA Natural Resources Conservation Service. (2024) Official Soil Series Descriptions. Available at: https://soilseries.sc.egov.usda.gov/osdname.aspx (Accessed February 1, 2024).
Steinmetz, Z., Wollmann, C., Schaefer, M., Buchmann, C., David, J., Tröger, J., et al. (2016). Plastic mulching in agriculture. Trading short-term agronomic benefits for long-term soil degradation? Sci. Total Environ. 550, 690–705. doi: 10.1016/j.scitotenv.2016.01.153
Tarara, J. M. (2000). Microclimate modification with plastic mulch. HortScience 35, 169–180. doi: 10.21273/HORTSCI.35.2.169
Tarrant, A. R., Brainard, D. C., and Hayden, Z. D. (2020). Cover crop performance between plastic-mulched beds: impacts on weeds and soil resources. HortScience 55, 1069–1077. doi: 10.21273/HORTSCI14956-20
Teasdale, J. R. (1996). Contribution of cover crops to weed management in sustainable agricultural systems. J. Prod. Agric. 9, 475–479. doi: 10.2134/jpa1996.0475
Tiemann, L. K., and Billings, S. A. (2011). Indirect effects of nitrogen amendments on organic substrate quality increase enzymatic activity driving decomposition in a mesic grassland. Ecosystems 14, 234–247. doi: 10.1007/s10021-010-9406-6
Tiemann, L. K., and Grandy, A. S. (2015). Mechanisms of soil carbon accrual and storage in bioenergy cropping systems. GCB Bioenergy 7, 161–174. doi: 10.1111/gcbb.12126
Vance, E. D., Brookes, P. C., and Jenkinson, D. S. (1987). An extraction method for measuring soil microbial biomass C. Soil Biol. Biochem. 19, 703–707. doi: 10.1016/0038-0717(87)90052-6
Wan, Y., and El-Swaify, S. A. (1999). Runoff and soil erosion as affected by plastic mulch in a Hawaiian pineapple field. Soil Tillage Res. 52, 29–35. doi: 10.1016/S0167-1987(99)00055-0
Warren, N. D., Smith, R. G., and Sideman, R. G. (2015). Effects of living mulch and fertilizer on the performance of broccoli in plasticulture. HortScience 50, 218–224. doi: 10.21273/HORTSCI.50.2.218
Westbrook, A. S., Bhaskar, V., and DiTommaso, A. (2021). Weed control and community composition in living mulch systems. Weed Res. 62, 12–23. doi: 10.1111/wre.12511
Wilhoit, J., and Coolong, T. (2013). Mulching with large round bales between plastic-covered beds using a newly developed offset round-bale unroller for weed control. HortTechnology 23, 511–516. doi: 10.21273/HORTTECH.23.4.511
Xiao, W., Chen, X., Jing, X., and Zhu, B. (2018). A meta-analysis of soil extracellular enzyme activities in response to global change. Soil Biol. Biochem. 123, 21–32. doi: 10.1016/j.soilbio.2018.05.001
Xie, Y., Tittarelli, F., Fragstein, P.Von, Bavec, M., Canali, S., and Kristensen, H. L. (2017). Can living mulches in intercropping systems reduce the potential nitrate leaching? Studies of organic cauliflower (Brassica oleracea L. var. botrytis) and leek (Allium porrum L.) production across European conditions. Renewable Agric. Food Syst., 32, 224–239. doi: 10.1017/S1742170516000211
Zak, J., Willig, M., Moorhead, D., and Wildman, H. (1994). Functional diversity of microbial communities: a quantitative approach. Soil Biol. Biochem. 26, 1101–1108. doi: 10.1016/0038-0717(94)90131-7
Keywords: bell pepper, living mulch, organic agriculture, plastic mulch, soil health, summer squash, weed management
Citation: Tarrant AR, Brainard DC, Tiemann LK and Hayden ZD (2024) Weed control, soil health, and yield tradeoffs of between-bed management strategies in organic plasticulture vegetable production. Front. Sustain. Food Syst. 8:1276415. doi: 10.3389/fsufs.2024.1276415
Edited by:
Rishi Raj, Indian Agricultural Research Institute (ICAR), IndiaReviewed by:
David Butler, The University of Tennessee, Knoxville, United StatesAnil K. Choudhary, ICAR–Central Potato Research Institute, Shimla, India
Copyright © 2024 Tarrant, Brainard, Tiemann and Hayden. This is an open-access article distributed under the terms of the Creative Commons Attribution License (CC BY). The use, distribution or reproduction in other forums is permitted, provided the original author(s) and the copyright owner(s) are credited and that the original publication in this journal is cited, in accordance with accepted academic practice. No use, distribution or reproduction is permitted which does not comply with these terms.
*Correspondence: Alyssa R. Tarrant, dGFycmFuMTlAbXN1LmVkdQ==; Zachary D. Hayden, aGF5ZGVuemFAbXN1LmVkdQ==