- 1Research in Social Sciences and Management (CSG), ISEG-Lisbon School of Economics and Management, Universidade de Lisboa, Lisbon, Portugal
- 2Center for Ecology, Evolution and Environmental Change (cE3c) & Global Change and Sustainability Institute (CHANGE), Faculdade de Ciências da Universidade de Lisboa, Lisbon, Portugal
Food insecurity is a multidimensional and intricate problem, known to have significant implications for individuals, communities, and countries worldwide. Africa has become the continent that is experiencing this uncertainty the most. Food Security (FS) encompasses several aspects such as availability, accessibility, nutrient use, and supply system stability with time and, more recently, other obliges to governance/agency and sustainability. Knowing the interconnection between these aspects and the Ecosystems Services (ES) and understanding the relationship and interactions between FS and ES is important. Moreover, this knowledge may contribute to supporting policies that promote long-term sustainable and secure food systems. Hereby, a conceptual framework is presented, that examines interactions between food insecurity drivers and ecosystem change drivers and the combined influence on ES. Our review further introduces existing trade-offs between ES on account of agricultural intensification vs. key existing strategies to promote sustainable agricultural production. These strategies include climate-smart agriculture, sustainably managed land, and effective handling of water resources. In the end, the potential of Payment for Ecosystem Services (PES), as a suitable approach to ensuring these strategies are adopted, especially in African countries where sustainable financial incentives are currently under-explored is discussed. In resume, this review aims to make a conceptual contribution to understanding how drivers of food insecurity influence drivers of ecosystem changes, the impact of these influences on the services of ecosystems, and how sustainable agro approaches and PES introduction can help to reduce such negative impacts.
1 Introduction
Having enough food and making sure there is year-round access to it, is one of the most important Sustainable Development Goals for 2030 (Atukunda et al., 2021). However, the likelihood of experiencing extreme food insecurity grew from 8.4 to 10.2% of global inhabitants (FAO, 2017). According to the 2024 report released on April 24, there was an increase of 1 million people facing emergency levels of acute food insecurity across 39 countries and territories (https://www.unicefusa.org/media-hub/reports/2024-Global-Report-Food-Crises). Food insecurity is quite a complex phenomenon owing to factors that differ in significance across regions and countries, and over time. According to the Food and Agriculture Organization (FAO), food insecurity is “a condition that results from people not having reliable access to adequate quantities of safe and healthy food.” (FAO and IFAD, 2013), or the economic and social conditions that limit access to safe, nutritious food—and sufficient amounts of it (https://www.mckinsey.com/featured-insights/mckinsey-explainers/what-is-food-insecurity). The likelihood of food insecurity in mostly of African countries is always extremely high because of the constant increase in population, hardship, violence, illness, erratic weather, low agricultural production, and malnutrition (WHES, 2015). There have been strong warnings by the FAO that, the Sustainable Development Goal (SDG) of ending world hunger, implemented by the United Nations (UN) would not be achieved if efforts are not increased (Brander et al., 2021).
In Sub-Saharan Africa, most farms owned by smallholder farmers are two hectares or smaller (70–80%) (Lowder et al., 2016). Small-scale farmers and their families rely on these small lands for food and revenue from harvests. Although global food and agricultural production have increased, associated with global value chains, they are quite insufficient in ensuring that the most vulnerable people can access food, or have stable access to sufficient food, which is one pillar that has made very little progress as a result of the volatile nature of global food prices (Swinnen and Squicciarini, 2012), as well as political instability, conflicts, and wars. Additionally, recognizing seasonal variations in food availability is essential to designing and implementing strategies for particular periods given the likelihood of catastrophic weather occurrences (Wilhite et al., 2014). Among other things, variables such as resource scarcity and ES deterioration are intrinsically related to food insecurity, hunger, and malnutrition (Cruz-Garcia et al., 2016). Moreover, increases in global food production, however, result in greater conversion of forest lands for agricultural production, which is causing the loss of vital ES and the extinction of species (UNEP, 2012).
Ecosystem Services are the “benefits people derive from ecosystems,” according to the Millennium Ecosystem Assessment (2005). The Intergovernmental Science-Policy Platform on Biodiversity and Ecosystem Services (IPBES, 2018) also describes these benefits as “Nature's contributions to mankind”. These advantages are divided into four categories: provisioning services, regulation services, cultural services, and support services. However, these categories have been boiled down to three major topics following multiple scientific discussions throughout the years. For example, Haines-Young and Potschin (2010) argue that supporting services are “structures, processes, and functions that characterize ecosystems” and so should not be included in ES categories.
The recent expansion of the studies on how ES is linked to food security is reflected in the recent increase in the number of ES research published (Vihervaara et al., 2010). However, details about the direct and indirect links between environmental services and food security are dispersed throughout numerous periodicals and academic publications. Food insecurity is a complex issue that has several driving factors that may influence food security pillars (Fyles and Madramootoo, 2016).
Human-induced and natural factors that bring about changes in the ecosystem directly are referred to as drivers of ecosystem change (Millennium Ecosystem Assessment, 2005). Regarding these drivers, several pieces of research (e.g., Emmerson et al., 2016; Watson et al., 2021; Pereira et al., 2022) assess the impact of agriculture intensification, mostly on increasing provisioning ES, however, literature on the existing trade-offs among the various ES is less frequent (e.g., Power, 2010; Howe et al., 2014) and even less when focusing on Africa. Moreover, there is a need to discuss potential ways “to feed the world” without destroying large ecosystems through, Climate Smart Agriculture (Lipper et al., 2015) and Payments for Ecosystem Services.
This review, therefore, specifically addresses the effects of agricultural intensification on ES and the resultant trade-offs overlooked. By highlighting the impact of such trade-offs and advocating for strategies like Climate Smart Agriculture and Payments for Ecosystem Services, the manuscript drives the conversation forward on sustainable agricultural measures and the need for financial incentives. This work may serve different stakeholders such as African governments, civil society, the commercial sector, and other parties involved in ecologically sensitive investments and land use choices. Ultimately, this study contributes to creating a more holistic understanding of sustainable agriculture needs, its positive impact on ES, and the importance of evolving adaptation strategies in the face of climate change and food insecurity more specifically for Africa.
This review begins with Sections 1, 2, being the introduction and methodology, respectively. Sections 3, 4 delve into the drivers of food insecurity and the drivers of ecosystem changes from the African perspective. Interactions and linkages between food insecurity drivers and drivers of ecosystem changes, and their impact on ecosystem services are discussed in Section 5. Section 6 highlights four key sustainable farming strategies that could be used to decrease the negative impact caused by the interactions of these drivers. Section 7 is a summary of future research trends and Section 8 is the conclusion with remarks and suggestions for future research.
2 Methodology
The focus of this review is on drivers of food insecurity and drivers of ecosystem changes and their influences on one another and related ES from the African perspective. Literature and publications were selected from three main databases, ScienceDirect, Google Scholar, and Web of Science, to obtain a wider pool of high-quality literature. Key words used include, “Sub-Saharan Africa,” “Africa,” “drivers of food insecurity,” “interactions between drivers,” “impact of food insecurity drivers,” “impact of agriculture intensification,” and “drivers of ecosystem changes.” To obtain an equilibrated relation of papers between both types of drivers, we selected publications that were between the years 2000 and 2023.
In all, twenty publications were selected from over two thousand results obtained from the various databases, after thorough screening with the help of inclusion and exclusion criteria. The criteria were to ensure that all selected papers were relevant, valid, and reliable for the best quality of review.
Inclusion criteria:
• The paper must be a study conducted in Africa or focus on the African context, mainly sub-Saharan Africa, and either food insecurity drivers or drivers of ecosystem changes, or both.
• The paper must be peer-reviewed and published.
• The paper must be published between the years 2000 and 2023.
• The paper must focus on at least one of the keywords used “Sub-Saharan Africa”,
“Africa”, “drivers of food insecurity,” “interactions between drivers,” “impact of food insecurity drivers,” “impact of agriculture intensification,” and “drivers of ecosystem changes”
• The paper must be published in English.
Exclusion criteria:
• All non-peer-reviewed articles were eliminated to ensure the highest quality of papers.
• Papers published before the year 2000 were eliminated.
• Papers published in languages other than English were removed.
With the help of these screening criteria, we were able to select the most reliable and relevant articles for this literature review. The analysis of the selected articles provided a reliable understanding of the drivers of food insecurity, how they interact with one another, their influence on drivers of ecosystem changes, and how they affect ecosystem services in Africa.
3 Food insecurity in Africa and its drivers
Regarding Africa, over 50% of Africans experience moderate or severe food insecurity, with East Africa having the highest rate (63% or 272 million people), Southern Africa coming in second (54% or 35 million people), West Africa coming in fourth (48% or 183 million people), and North Africa having the lowest rate (30% or 70 million people) (FAO et al., 2019). Specifically, in Sub-Saharan Africa (SSA), over 220 million people lack sufficient food, and about three-quarters of these people are inhabitants of rural communities (FAO, 2014). Farming being the main source of livelihood, they rely on the produce from their farming activities to feed themselves and generate income for other needs. They are however often susceptible to extreme weather such as drought and floods.
In the African context according to Wudil et al. (2022), the key drivers are high inflation affecting food prices, low crop productivity due to the reduced level of natural resources, climate change, inadequate funding for irrigated agriculture research, and rapid population increase. According to Fyles and Madramootoo (2016), the key food insecurity drivers globally are, increasing food demands, climatic change, natural resource availability, biofuel production, and no infrastructure investment from the public and private sector and development in agricultural research. The degree of importance may, however, vary between countries based on physical, economic, and social differences. An illustration of the interactions between the drivers of food insecurity and how they affect one another is shown in Figure 1. The influence of global food insecurity drivers on the African continent is then further discussed.
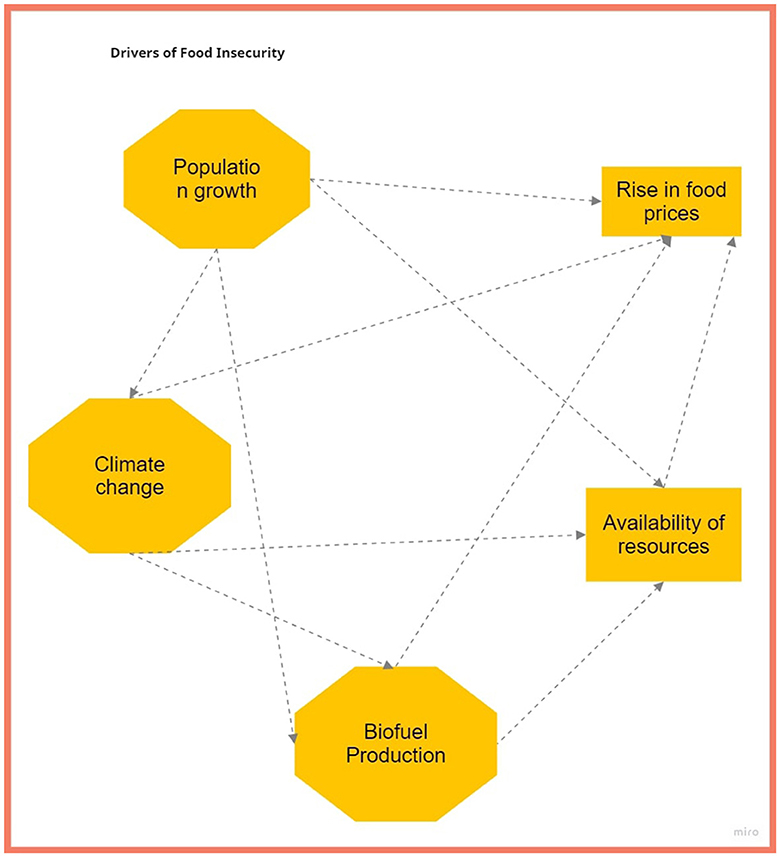
Figure 1. Interactions between drivers of food insecurity adapted from Fyles and Madramootoo (2016).
3.1 Population growth
Increased food demand is mainly driven by population increase, a primary driver of global food insecurity. In the last 20 years, the population has increased by 1.6 billion people worldwide, according to Mazzocchi et al. (2012), with low-income nations with food shortages accounting for 78% of this growth. Farmers will need to try to feed 40–86 million more people annually until 2050. The significant rise in living standards and dietary diversity in nations with a middle income, where more than 70% of people around the world reside, is increasing the strain on global food security and amplifying this need for food (World Bank, 2013a). Initially, gains in food production were mostly accomplished through increasing land area, having dramatically accelerated in the past 300 years, the rate of land use change. During the “Green Revolution,” improved genotypes, fertilizers, herbicides, water, and other agronomic practices were all used to intensify productivity. Environmental consequences (such as excessive fertilizing and leaching of pesticides) were generally disregarded throughout the Green Revolution. Ethiopia, for example, was reported by the World Bank (2019) to be the second most populated country in sub-Saharan Africa, with over 100 million people. The country's population growth rate was also 3% per annum, higher than in several African countries. The rapid population growth increases the level of competition among inhabitants for social amenities and food most importantly. The lack of adequate food to meet the nutritional needs of over 100 million people will result in malnutrition, stunted growth, starvation, and possibly death.
3.2 Natural resources availability
The quality and quantity of natural resources that are available for crop cultivation and raising animals are being affected by increased soil nutrient loss, increased land degradation and desertification, and reduced freshwater levels. Agriculture is responsible for the withdrawal of more than 90 percent of the freshwater used for consumption and 70% of the world's total freshwater (Hoekstra et al., 2012). Globally, water demand has been growing faster than population expansion, and agriculture and other uses are increasingly competing for access to freshwater resources (Palaniappan and Gleick, 2009). Attempts to produce more to satisfy the rising demand for food led to excessive fertilizer use, and bad irrigation systems, resulting in pollution. These practices have caused salinization and contamination of several water bodies where fresh water could be obtained (Fyles and Madramootoo, 2016). As noted by Bossio et al. (2010), the primary environmental issue limiting the production of small-scale farms in Africa is nutrient deficiency. According to Jones et al. (2013), annual yield losses in Sub-Saharan Africa are caused by a reduction in nutrients, soil erosion, and increasing desertification, and range from 2 to 40%, being an average loss of 8.2%. Population growth, urbanization, and industrialization have on the other hand caused an increase in cropland losses due to the conversion of prime agricultural lands to other uses (Fyles and Madramootoo, 2016). A considerable number of forests have been destroyed as well due to these conversions. Between 2000 and 2012, 1.5 million km2 of forests were estimated to be lost, with many of such losses occurring in tropical areas (Hansen et al., 2013). In addition, deforestation and bad management practices have contributed to increased cases of water run-off and soil nutrient losses.
3.3 Climatic change
Climatic change increases food insecurity both globally and locally, which has an impact on the volume of internationally traded food output, and local produce (Lobell and Gourdji, 2012). Predictions suggest, that more frequent and severe droughts are likely to increase, affecting the levels of available water globally, especially when 97% of Sub-Saharan African farmers rely on rain to irrigate their crops, indicating their level of vulnerability to the effects of variations in temperature and rainfall patterns (World Bank, 2013b). Prominent levels of weather variability have resulted from climate change, which negatively affects agricultural productivity. Due to the adverse consequences of climate change, it is expected that agricultural productivity in Africa will decline by 8–22%by 2050, which will worsen food insecurity in the area (Mthembu and Hlophe, 2021). Food insecurity brought on by climate change has affected several African nations that are the most susceptible to its effects (Mfwango et al., 2022). For example, Ethiopia is particularly sensitive to the immediate and accompanying effects of changes in climate since it has an agro-based economy and relies heavily on agriculture, which is responsible for 45% of its GDP (Ferede et al., 2013). Ethiopia has experienced repeated droughts in recent years, which have drastically reduced the nation's agricultural output led to food shortages, and also contributed to existing conflicts, resulting in a higher level of food insecurity.
3.4 Production of biofuels
Global biofuel production is being encouraged due to the urgent need to cut carbon emissions, reduce the extraction of fossil fuel, and global energy concerns. Demand for agricultural products like sugar, maize, and oilseeds is now largely driven by the developing biofuels sector. Previously mostly used as food, these crops are now also used in the production of both biodiesel and ethanol. Cultivation solely for biological fuel purposes takes away resources that could be used to produce food, which in turn decreases food supply and raises food prices. Global biofuel output rose between 2000 and 2012 from 17.8 to 105.6 billion liters as a result of public policies supporting the biofuels industry (REN21, 2013).
Energy and fuel transition, or a gradual shift toward the use of low-carbon energy sources like wind, solar, and bioenergy, is required in Africa because of the rising energy demand, which will grow twice as quickly as the global average due to an expanding population (IEA, 2019). South Africa, for instance, is actively establishing a biofuel business by utilizing the abundant agricultural resources and biodiversity of the country. The nation's energy policy covers a wide range of biofuels, including traditional bioethanol and biodiesel (Echendu et al., 2023). The potential for the biofuel industry in Africa could potentially shift the focus of farmers from production for consumption to production for fuel which has a much higher economic return due to the increasing demand. This creates more pressure on the already existing few farmers who are unable to produce enough to feed the increasing African population. Although it is currently not a direct cause of food insecurity in Africa, because the biofuel market is not very well-established, it could be argued that its potential to become one is undeniable and therefore requires the attention of researchers and policy leaders to find a way of preventing it, especially under a petrol-based world crisis due to recurrent geopolitical tensions.
3.5 Rise in food prices
To even out the distribution in the supply and the demand for food on a global and regional scale, trade and market dynamics are crucial. International unfair trade policies, agricultural assistance programs in wealthier nations, and market liberalization have increased poor countries' reliance on food imports and made them more susceptible to spikes in food prices (Madramootoo and Fyles, 2012).
To protect domestic food security after the dramatic increase in food prices in 2007–2008, numerous nations employed trade policies such as export taxes, quantitative export limitations, and lower import tariffs. In the end, these actions or trade barriers increased food prices and worsened the world's food security which affected the African continent the most (Rutten et al., 2013). Agricultural markets that do not operate effectively, commonly in African countries, are mostly a result of poor policies implemented by the regional and local governments and institutions and inappropriate infrastructures. Poor market performance, low local consumer demand, and a lack of export opportunities severely limit the chances for agricultural expansion in many African nations. Sub-Saharan Africa's agro-food policy needs to increase food security also focusing on the sector's rapid expansion (Fyles and Madramootoo, 2016).
4 Ecosystem services and drivers of ecosystem changes in Africa
There are considerable (sub)regional, and national variations in biodiversity throughout Africa, which are a result of the continent's long and diverse history of ecological interactions with humans as well as climatic and physical changes. A significant advantage of the region's efforts to achieve sustainable development is the immense natural resources, which have been collected over millions of years and are combined with the wealth of indigenous and local knowledge on the continent (IPBES, 2018).
Food items, drinks, living space, home furnishings, beauty products, and medications are examples of tangible goods that ecosystems may supply that improve our everyday lives (Provisioning ES) with the African continent being the source of many of these services. Despite being frequently neglected, the various sorts of ecosystem contributions (e.g., Regulating ES) are crucial in managing our living surroundings and preserving human civilizations. Regulating ES assists in maintaining clean water flow and safeguarding people from flooding and other dangers including landslides, tsunamis, and erosion of land (IPBES, 2018). The spiritual wellness of people, especially Africans, depends greatly on ecosystems which can have profound cultural or religious meaning (Cultural ES). Additionally, these offer chances for leisure activities or the pleasure of the outdoors (Haines-Young and Potschin, 2010). Ecosystems that are well-maintained have the potential to greatly enhance human health and wellbeing (Finlayson et al., 2015).
Studies suggest that regulating ES provided in the SSA region is on the decline due to agriculture intensification. For example, 152,000 t of nitrogen per year is transported by smaller rivers and dumped in an already eutrophied Lake Victoria in Eastern Africa (Zhou et al., 2014). This is associated with the lack of soil cover, which accelerates erosion, prevents rain infiltration, and promotes topsoil loss (Lal, 1987). These effects are frequently accompanied by nutrient transport and accumulation, such as into lakes and rivers (Zhou et al., 2014). Thus, the decrease in regulating ES will in turn cause a decrease in the provisioning of ES such as food production due to environmental degradation in the long run. Evidence has shown that humans participate in several land use variations which have led to several ecosystem changes. To better manage these ecosystem changes and optimize the supply of ES, it is necessary to comprehend the driving factors that influence these changes.
This paper discusses four main drivers and their direct negative impact on ES. These drivers are climate variability, the utilization of plant nutrients, land conversion, and lastly pests and diseases as well as invasive species (Millennium Ecosystem Assessment, 2005). These drivers were chosen based on Steffen et al. (2015) report on the Planetary Boundary Framework, which shows that these four boundaries have been crossed which amplifies the possibility of creating permanent environmental changes.
A more recent report by Richardson et al. (2023) suggests that six out of the nine boundaries have been crossed, increasing the risk of permanent environmental changes even higher. Figure 2, illustrates the various drivers of ecosystem changes and the influences they have on each other. This provides a better picture of the compounded effect of these drivers on the ecosystem and creates an opportunity to find suitable solutions or approaches for preventing these changes.
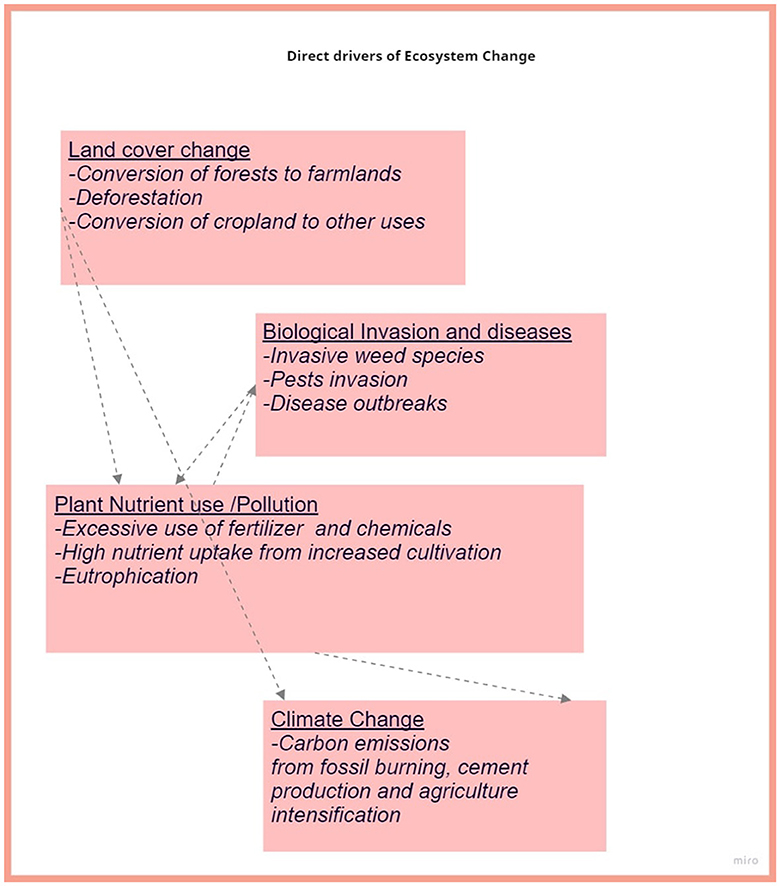
Figure 2. Drivers of ecosystem changes and their influence on one another, adapted from Millennium Ecosystem Assessment (2005).
4.1 Climate variability
According to reports from the IPCC (2014), about 78% of global greenhouse gas emissions have been from natural gas extraction, with the remaining 22% associated with changes in land use, mainly the destruction of forests and, agricultural production. Regarding climate change impacts the IPCC also indicates that there would likely be significant regional heterogeneity in the overall impact of climate change on yields of the key cereal crops in the African area (Niang et al., 2014). According to “worst-case” forecasts, losses of 27–32% are predicted for maize, sorghum, millet, and groundnuts by the middle of the century assuming a warming of around 2°C over levels before industrialization (Schlenker and Lobell, 2010). Precipitation differences and temperature influence the level of crop production and food availability directly. Precipitation being responsible for the supply of water and soil water content is essential for overall crop development. According to Reilly et al. (2003), even though a higher level of precipitation would lead to a reduced viable yield, i.e. a reduction in the yield gap between irrigating and relying on rainfall to produce, it could have an unfavorable effect during high precipitation levels which results in flooding and waterlogging (Falloon and Betts, 2009).
The temperature is also responsible for the duration of the growing seasons of crops, mainly controlling the plant development and water needs. Higher temperatures result in increased water level requirements and in most African countries where rainfed agriculture is commonly practiced, crop development and production in general is affected due to reduced amount of water. As stated by Niang et al. (2014), projected climate changes in Sub-Saharan Africa might shorten the growing seasons for crops and fodder by an average of 20% by 2050 in Western and Southern Africa, causing a 40% reduction in yields of grains and a reduction in the total quantity of cereal used in the production of livestock.
Climate changes have also increased the possibility of drought, enhanced insect resistance, and reduced insect growth and reproduction times, which have all made the extent of bug outbreaks worse (Raffa et al., 2008). These insect outbreaks result in invasions and the destruction of several crops and farm produce. Throughout the middle of the 21st century, estimated crop output losses due to global warming range from 18% in Southern Africa to 22% altogether in SSA (Niang et al., 2014).
4.2 Plant nutrient use
Extensive farming practices, like converting grassland into cultivated land, constant tillage, pesticides, and inorganic fertilizer application, have been shown to have a negative impact, e.g., on soil biodiversity, which is responsible for regulating ES. The result has been a decline in soil quality in many areas, including erosion, nutrient depletion, soil biodiversity losses, and a reduction in the quality of the structure of the soil (Tsiafouli et al., 2015). Agricultural productivity is affected by deteriorating soil conditions, which also reduces the ES that the landscape offers (Squire et al., 2015). Soil biodiversity assessments in recently converted areas show that intensive land use is associated with a lower soil faunal taxonomic richness and a reduced number of functional soil organisms (Tsiafouli et al., 2015). In the year 2000, annual nutrient depletion was believed to be 48 kilograms i.e., 26 kilograms of nitrogen, 3 kilograms of phosphorus, and 19 kilograms of potassium (FAO and ITPS, 2015).
Historically, the application of nitrogen fertilizers has been estimated to account for 30–50 percent improvements in yields (Zhu and Chen, 2002). Farming intensification has been made possible in large part with the use of inorganic fertilizers in production. Approximately 65% of SSA's agricultural land has undergone degradation as a result of inadequate management methods, which cause losses in soil biological, chemical, and physical standards, diminishing the soil's potential to sustain agricultural production and deliver other ES (Zingore et al., 2015). Degraded soils impair yearly harvests by roughly 3% (Zingore et al., 2015), which costs SSA around USD 68 billion per year.
4.3 Land use/conversion
Land use/cover change as defined by Antwi-agyei et al. (2019), is any human action or involvement that leads to the conversion of one land use/cover into another or the intensification of an existing piece of land. Increased extension into forested regions and cultivation on steep slopes are results of the loss of cropland. As stated by Tully et al. (2015), when land use is altered—for example, when it shifts from woodland to agriculture— important criteria for the regulating, provisioning, and cultural services provided by various ecosystems quickly decline.
Population increase has prompted the conversion of forests into cultivated lands resulting in an increase in agriculture intensification and the loss of tree species (Udo et al., 2011). According to Zhang et al. (2018), crop rotation is not being practiced, which results in nutrient mining from the soil, erosion, and a higher risk of pest and disease outbreaks. According to Elias (2017), soil erosion has caused land degradation, particularly in South Africa. As stated by Balabathina et al. (2020), various levels of soil erosion affect more than 70% of the nation. This erosion causes reservoirs to silt up and increases pollution by increasing suspended sediment concentrations in streams, affecting ecosystems and water consumption.
Research by Tata Ngome et al. (2019), to assess the lack of food, experienced by Cameroonian households in the context of deforestation, showed that higher deforestation led to a higher level of food insecurity. The results of the research suggest that deforestation and forest conversion into farmlands for production do not guarantee long-term food security for existing households.
According to Muyanga et al. (2013), farm sizes have gradually decreased in Sub-Saharan Africa over the past 50 years, resulting from rapid urban population growth which has led to converting often very fertile land to residences and homes. Additionally, because of the increased demand for resources, the growing number of cities significantly strains the adjacent ecosystems (Lambin et al., 2003). These conversions affect the amount of food that can be produced locally and in effect amplifies the pressure on available food.
4.4 Invasive species, pests, and diseases
Biological invasion usually occurs whenever organisms are deliberately or accidentally introduced into a new environment or ecosystem. Be it on land or underwater, to be able to invade an ecosystem, invasive species must go through a series of stages called the “invasion process” (Lockwood et al., 2005). Evidence has shown that human-driven movement of organisms, deliberate or accidental, has been responsible for a significant alteration of species ranges and the introduction of several invasive species. For instance, in Eastern Africa, the introduction of Prosopis juliflora was carried out to provide wood products and more fodder for livestock. However, an invasion of Prosopis juliflora amounted to reduced groundwater provisioning (Dzikiti et al., 2013) and availability of forage (Ndhlovu et al., 2011). In effect, invasive species caused a decrease in ecosystem services and also increased vectors of disease-causing organisms (Muller et al., 2017).
In the latest worldwide review of 1,297 invasive species, it was discovered that eleven of the twenty nations worldwide that are most susceptible to invasive species are in Africa (Paini et al., 2016). Numerous invasive species have an impact on food crops, particularly those native to Africa like sorghum (Sorghum bicolor), pearl millets (Pennisetum glaucum), and hunger rice (Digitaria exilis), which serve as dietary supplements or staples for a lot of low-income customers (Tadele and Assefa, 2012). The most dangerous pest of corn and sorghum in 18 African nations is the Chilo partelus (Spotted stem borer), a pest of Asian origins that spread to Africa's eastern part in the 1950s (Yonow et al., 2017). Native to the Indian subcontinent is the species known as Rastrococcus invadens (Mango mealy insect). After being discovered for the first time in Benin in 1980, this pest quickly expanded to both West and East Africa (Neuenschwander, 2010). Although mango is its main host, it has been seen on more than 100 plant species throughout the continent of Africa. Mango output decreased by 89–100% in places that were severely impacted (Bokonon-Ganta et al., 2002). Human transfer of seedlings from nurseries has been the primary contributor to its widespread introduction in the West African region.
5 Interactions between food insecurity drivers and ecosystem change drivers that affect ecosystem services
Drivers of food insecurity are seen to increase drivers of ecosystem change, thereby affecting the ecosystem in general and affecting its capacity to provide goods and services that benefit humans. Moreover, they interact with one another and potentiate impact on drivers of ecosystem change. Population growth, for example, increases the demand for food and the pressure on food production, which potentially leads to a rise in food prices. The food demand (indirectly) and production (directly) in turn increase land use changes and thereby potentially affect the ecosystem's ability to regulate atmospheric content as well as nutrient cycle in the soil. Moreover, over the years, food and fiber demand has been increasing rapidly, putting the greatest strain on soils. Not just population increase is to blame for this, but also higher per capita calorific consumption as a result of increased wealth and changing diets. As referred to above, evidence shows that current agriculture intensification practices lead to an increase in provisioning ES at the expense of regulating and cultural ES. The trade-off is observed over time with continuous environmental changes and reduction in ES such as biodiversity, erosion control, atmospheric control, and others.
Based on our review and the works from Dobson et al. (2005), Fyles and Madramootoo (2016), and Ofori et al. (2021) we propose and illustrate here a conceptual framework showing the linkages between the drivers of food insecurity and the drivers of ecosystem changes and their influence on ES (see Figure 3) considering key findings for Africa. Food insecurity drivers such as population growth and increase in food demand, have brought about land conversion rates rising together with inorganic fertilizer usage, and these are major drivers of ecosystem change as well. A major contributor to deforestation in Africa is the expansion of smallholder agriculture, which is further driven by population increase and the production of biological fuels (Millennium Ecosystem Assessment, 2005). These expansions result in habitat invasions that destroy pristine and culturally significant locations and have an impact on biodiversity, which includes big animals, birds, and reptiles that are also vital to tourism (Muhumuza and Balkwill, 2013). For instance, the conversion of significant portions of indigenous forest communities has impacted the hunting and gathering Ogiek community's traditional practices in Kenya's Mau Jungle (Chabeda-Barthe and Haller, 2018). These are examples of cultural ecosystem services that are lost because of the interactions between these two drivers.
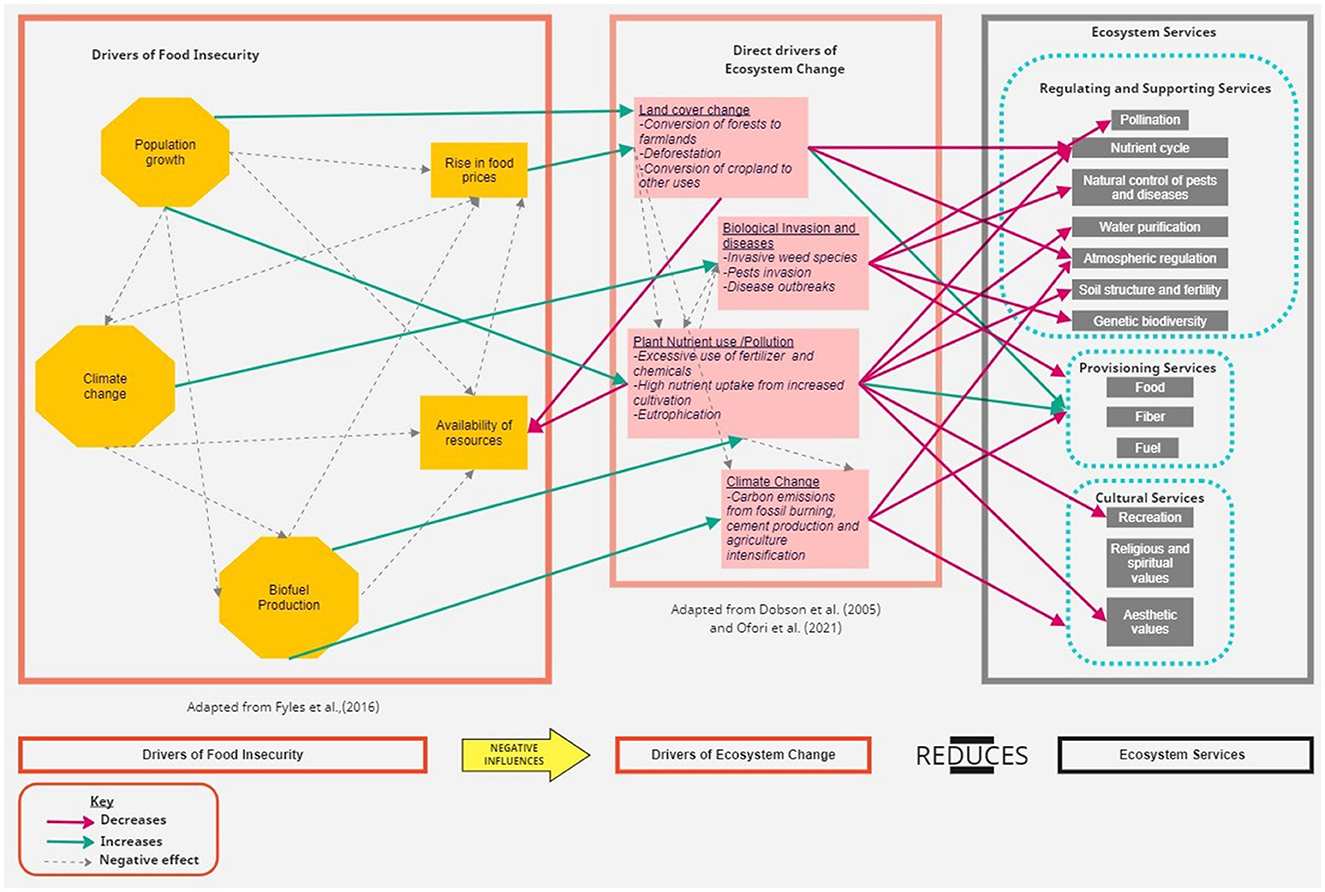
Figure 3. Linkages and interactions between drivers of food insecurity and ecosystem change drivers and how they affect ecosystem services.
Another significant cause of deforestation is the exploitation of fuelwood for residential purposes, which is influenced by the increase in population and the increased demand for food and available resources. Eighty percent of the energy used in SSA comes from fuelwood, with rural South Africa consuming an estimated 692 kilograms of fuelwood per year (Dovie et al., 2004). The gradual rise in biofuel production also increases the burden on producers and landowners. This adds up to the effects of climate change since the ecosystem provided by trees such as carbon absorption and the regulation of the atmosphere is removed or reduced. Climate variability and extremes are one of the major drivers of food insecurity globally and especially in Africa (World Health Organization, 2018). As the rate of nitrogen fertilizer application is increased, the rate at which nitrous oxide production and emitted from soils exponentially increases, accounting for around half of the total global anthropogenic nitrous oxide flux (Shcherbak et al., 2014).
Reports have shown that by 2050, Sub-Saharan Africa wants to utilize 7.7 million MT of fertilizer (Drescher et al., 2011). There will be an increase in carbon emissions from both the manufacture and usage of such a large amount of (extra) fertilizer. The predicted increase in fertilizer applications necessitates knowledge of possible consequences, even if the existing contributions to greenhouse emissions from the use of mineral fertilizers and manure in SSA are not nearly as large as in developed countries currently (van Loon et al., 2019). The excessive use of nitrogen-based fertilizers has other negative effects that are indirectly associated with the soil, for example neighboring water bodies becoming eutrophic. Eutrophication affects the quality of the water bodies making them unusable, unattractive, and inaccessible to humans. This leads to cultural ES losses like aesthetic value which could be obtained from recreational activities like swimming or for tourist purposes.
In addition, bio-regulators are lost due to the excessive use of chemicals and other human activities that affect the ecosystem. Bio-regulators are organisms that can influence the population levels of other soil creatures and also have a high potential for controlling pests and diseases in the soil, especially important in terms of tackling food insecurity. This regulating ES provided by bio-regulators is lost due to extensive pesticides and inorganic fertilizer application. Though the use of these chemicals may increase the amount of provisioning ES, e.g., food and fiber in the short term, the long-term effect is the loss of other ES. Insufficient soil quality, for instance, encourages expansion of the land (habitat encroachment) and inadequate groundwater recharge (Bob and Bronkhorst, 2010).
Soil is inextricably linked to food production and is also responsible for providing several ES. Humans consume ~98.8% of their daily calories from soil or land sources with just 1.2% from sources that are water (FAO, 2017). The pressure on the soils from intensification often leads to loss of fertility and destruction, limiting the soils' capacity to provide ES, e.g., the reduction or inability to provide food or fiber. As a result, more sustainable agronomic practices that match nitrogen supply are required.
In addition, agritourism, a rapidly expanding business where urban residents travel to rural areas to enjoy farming activities including farm restaurants, farm hostels, and farm walks, is one advantage of healthy soil (Rogerson and Rogerson, 2014). It has been also observed in Ghana (Appiah-Opoku, 2011) and Kenya (Gathogo, 2013) that, practices that enhance soil health also offer advantages for recreation and provide cultural ES.
6 Key strategies to promote food security and ecosystem services in African countries
Agriculture production levels or average yields only play a minor part in maintaining food security (Foley et al., 2011). Food insecurity levels continue to be on the rise in many African countries. This awareness has prompted an active response from a few African countries to adopt certain strategies to tackle food insecurity in a more sustainable way that also ensures the provision of ES. However, there is ongoing discussion regarding the best agro-production systems for maximizing food availability, meeting dietary needs, and protecting the environment, such as large-scale compared to small-scale, regional compared to global, monoculture compared to mixed, or organic compared to inorganic (FAO, 2016). Although there may be several strategies that have been adopted or documented, this paper will highlight four key ones; climate-smart agriculture, sustainable land use management, effective water resource management, and ecosystem services payments.
6.1 Climate-smart agricultural practices
The implementation of Climate Smart Agriculture (CSA) is becoming more crucial in policy and practice as long as climate change and variability continue to pose serious threats to food security (Müller et al., 2020) and CSA is offering an agro-paradigm for sustainable development that emphasizes productivity improvement, adaptation and resilience, mitigation of climate change, and protecting ecosystems in agricultural systems (Kebeda, 2019).
Farmers can boost output, adjust to changing weather patterns, and lower greenhouse gas emissions by using climate-wise agricultural methods. Additionally, these techniques can support sustainable water and land use, increase biodiversity, and boost soil health. Overall, CSA may significantly lessen agricultural practices' damaging environmental impact while maintaining food security for current and future generations (Fedele et al., 2018). The success of CSA initiatives in Africa, therefore, depends on removing the obstacles that smallholder farmers must overcome to use them.
The tendency for small-scale farmers to adopt sustainable farming in Africa has been the subject of several research works (e.g., Ighodaro et al., 2020). Senyolo et al.'s (2018) study revealed a variety of CSA technical advancements that may be used, including integrated soil management and conservation agriculture. There are a few challenges, however, regarding the adoption of CSA. For instance, smallholder farmers in Kenya encountered some difficulties in implementing CSA techniques, according to Mugure and Oino (2013), and these issues are mostly connected to difficulties in accessing capital, reduced technical expertise, and inadequate infrastructure. Despite the difficulties, implementing eco-friendly technology in agriculture can significantly improve small-scale farmers' capacity to withstand the consequences of changes in climatic conditions and eventually improve family welfare (Danso-Abbeam et al., 2020).
6.2 Sustainable land use management
It will be impossible to achieve food security without sustainable land use measures, which support the lasting viability of the ecosystems and soil resources that underpin agricultural activities (Wondie and Mekuria, 2018). According to Liu et al. (2016), this strategy uses techniques to maintain and improve soil fertility, lessen erosion, optimize water retention and drainage, boost biodiversity, and protect natural resources including wetlands and forests. Farmers may boost production and food security in a way that is ecologically benign by applying sustainable land use management strategies. Additionally, by encouraging carbon absorption in soil and plants, sustainable land use management can aid in reducing and adjusting to the consequences of climate change (Pandey and Bhusal, 2016).
Because technology cannot completely replace effective land management techniques, attempts to create a “green revolution” paradigm in Africa utilizing subsidies and inputs like fertilizers have been expensive and unsustainable (Zougmoré et al., 2014). Instead, soil management is a significant part of sustainable farming and offers a powerful tool for controlling climate and a strategy to protect ES and biodiversity (Ertiban, 2019).
Different sustainable land management techniques have been suggested. Agroforestry, conservation farming, intercropping, and crop rotation are some of these techniques (Masso et al., 2017). According to research, intercropping and crop rotation have the potential to increase soil productivity. Conservation farming focuses on lowering soil tillage, increasing crop residue cover, and improving soil organic matter, among other things (Zhichen et al., 2015).
6.3 Effective water resource management
Water is a crucial resource for human life, economic growth, and environmental sustainability, according to Komariah and Matsumoto (2019). Previous regional studies in Eastern Africa have shown that future drying will have a considerable influence on agriculture and the growing season duration (Owusu et al., 2019). To maximize water resource usage and ensure the effective disposal of dirty water, specific management measures are required.
Successful collaboration policies involving efforts to manage health, water resources, and the climate that focus on adaptive methods and manage the risk of drought and flooding have already been shown in projects in West Africa (Loucks, 2020). Policymakers must take a variety of issues into account, including socioeconomic growth, and environmental sustainability, and adapt to climate change to manage water resources in Africa successfully (Jones et al., 2017).
Agriculture and food systems, which account for 70% of worldwide freshwater withdrawals, are crucial factors in managing water resources in Africa (Cohim et al., 2021; Kishawi et al., 2022). Approximately 90% of all freshwater usage worldwide and in Africa is by irrigation so efficient water resource management is essential for guaranteeing food security.
A few used strategies in agriculture include efficient irrigation systems, rainwater gathering, and water recycling (Zhang et al., 2019). Agronomic techniques that improve soil moisture retention and the use of drought-resistant crop types can also help to lessen the adverse consequences of water scarcity on crop output (Kumar et al., 2022).
In sum, soil and water management, climate-smart agriculture, and sustainable land-use techniques are just a few of the interconnected strategies that must be considered, to achieve food security sustainably and to reduce the drivers that change ecosystems and the services they can provide. As part of the key explained technologies that can contribute to sustainable agricultural production, we also introduce a financial tool that could be used to obtain farmers' interest; Ecosystems Services Payment (ESP). ESP can play a role in contributing to improving food production and maintaining the needed ES. ESP related to conservation is now a relevant experiment taking place in some countries across African (Corbera, 2012; Weatherley-Singh and Gupta, 2015).
6.4 Introduction to Ecosystem Services Payments
According to Ecosystem Services Payments (ESP), those who get ES from the ecosystem remunerate landowners in cash or in-kind in exchange for them adopting certain sustainable land management methods/strategies that will lead to favorable ecological results (Waruingi et al., 2021). The recent changes to the agro-environmental policies of the EU and the UK have shown an increasing interest in ESP (Bieroza et al., 2021). For instance, the year 2005 marked the eleventh Conference of the Parties (COP) at the UNFCCC, where RED (Reducing Emissions from Deforestation) was first brought up. In 2007, at the 13th UNFCCC COP, REDD+1 was discussed and introduced. REDD+ is referred to as “the largest experiment in ESP in the history of the world” (Corbera, 2012), where it is anticipated that foreign transfers of funds to underdeveloped countries or poor nations will promote changes that will minimize deforestation and forest degradation. REDD+ projects have been carried out in some African countries including, Ghana, Tanzania, and Cameroon (Weatherley-Singh and Gupta, 2015). However, these projects focus on forest conservation and not on the adoption of sustainable farming practices.
In principle, ESPs compensate landowners (e.g., conservation) for the ES they render and charge the beneficiaries. Evidence shows (Mahanty et al., 2013) that the ecosystem regulating services are enhanced by this financial system, which also supports rural income generation and farmers' food security. ESP includes not only money made by the initiative as a reward for participants but also demands specialized support given by the administration of government to carry out operations effectively (Smith et al., 2013). The preferences of the surrounding communities have a significant impact on an ESP program's performance (Permadi et al., 2018). From empirical studies, it has however been demonstrated that the appropriate specification of ESP-related features influences the legitimacy and efficacy of ESP projects (Kang et al., 2019; Ola et al., 2019).
7 Future research trends
Sustainable farming practices such as climate-smart techniques, sustainable land use management, and effective and efficient water resource management are being studied (e.g., the SustInAfrica project and others financed by H2020) and the Task force rural Africa (TFRA) under the Africa-Europe Alliance for Sustainable Investment and Jobs is contributing for larger testing and escalation in more African countries to suit specific cultural and demographic settings, and most importantly, needs. It, however, requires a significant amount of research in designing and testing these techniques to suit various agroecological zones and socio-economic contexts. The way forward considers the need to carefully identify and value the ES provided by the adoption of specific sustainable farming practices. This will help generate more interest from buyers and stakeholders and create further awareness among policymakers of the potential implementation of ESP related to agricultural production in Africa. We hereby argue that ESP schemes apart support conservation, could also be used to motivate farmers to use sustainable farming methods. We believe this would not only serve as a form of compensation but also provide a sense of fulfillment and prestige to farmers for being rewarded for protecting the environment through these adoptions.
8 Conclusion
Food insecurity drivers have caused significant harm to the ecosystem due to measures adopted to mitigate existing food insecurity cases across the world. These measures have been shown to only improve one group of ES at the expense of others. These trade-offs cast a much larger shadow on the wellbeing of humans and other living creatures. In addition, these measures are only a short-term solution and therefore unsustainable in the long run. Current intensification methods will result in further ecosystem changes that will ultimately affect future yields and outputs from production. The neglect of the visible destruction of the ecosystems spells a total doom for several African countries already experiencing the most amount of food insecurity. Not only is it important to ensure that existing farming methods are changed, but sustainable approaches must be enforced and promoted in Africa.
In sum, to eliminate this compounding effect, policymakers must incorporate measures to promote sustainable agricultural practices. The financial mechanism of Ecosystem Services Payment is fairly new and still requires further research but seems promising in contributing to transforming agriculture intensification if we intend to ensure food security in Africa and across the world. Although our review paints a better picture of the interactions between drivers of food insecurity and drivers of ecosystem changes, it is limited to the African perspective and does not provide a global view of these interactions. Meta-analysis was not conducted which is another limiting factor of this review. For future research, we suggest that a larger systematic review and a meta-analysis be conducted in the context of other continents such as Asia and Latin America to identify possible similarities or differences that may exist in the interactions of these drivers.
Author contributions
GA: Conceptualization, Writing – original draft. IS: Funding acquisition, Supervision, Writing – review & editing. CB: Funding acquisition, Supervision, Writing – review & editing.
Funding
The author(s) declare financial support was received for the research, authorship, and/or publication of this article. The authors would like to acknowledge the SustInAfrica project, a supported initiative under the EU Horizon 2020 Research and Innovation Program under Grant Agreement 861924 and the Lisbon School of Economics and Management, Research in Social Sciences and Management (CSG), University of Lisbon, for funding the publication of this paper.
Acknowledgments
The authors would like to thank the Lisbon School of Economics and Management, Research in Social Sciences and Management (CSG), University of Lisbon for supporting this work.
Conflict of interest
The authors declare that the research was conducted in the absence of any commercial or financial relationships that could be construed as a potential conflict of interest.
Publisher's note
All claims expressed in this article are solely those of the authors and do not necessarily represent those of their affiliated organizations, or those of the publisher, the editors and the reviewers. Any product that may be evaluated in this article, or claim that may be made by its manufacturer, is not guaranteed or endorsed by the publisher.
References
Antwi-agyei, P., Kpenekuu, F., Hogarh, J. N., Obiri-danso, K., Abaidoo, R. C., Jeppesen, E., et al. (2019). Land use and land cover changes in the Owabi reservoir catchment, Ghana: implications for livelihoods and management. Geosciences 9:286. doi: 10.3390/geosciences9070286
Appiah-Opoku, S. (2011). Using protected areas as a tool for biodiversity conservation and ecotourism: a case study of Kakum National Park in Ghana. Soc. Nat. Resour. 24, 500–510. doi: 10.1080/08941920.2010.495108
Atukunda, P., Eide, W. B., Kardel, K. R., Iversen, P. O., and Westerberg, A. C. (2021). Unlocking the potential for achievement of the UN Sustainable Development Goal 2–‘Zero Hunger'–in Africa: targets, strategies, synergies and challenges. Food Nutr. Res. 65:7686. doi: 10.29219/fnr.v65.7686
Balabathina, V. N., Raju, R. P., Mulualem, W., and Tadele, G. (2020). Estimation of soil loss using remote sensing and GIS-based universal soil loss equation in the northern catchment of Lake Tana Sub-basin, Upper Blue Nile Basin, Northwest Ethiopia. Environ. Syst. Res. 9:35. doi: 10.1186/s40068-020-00203-3
Bieroza, M. Z., Bol, R., and Glendell, M. (2021). What is the deal with the Green Deal: will the new strategy help to improve European freshwater quality beyond the Water Framework Directive? Sci. Total Environ. 791:148080. doi: 10.1016/j.scitotenv.2021.148080
Bob, U., and Bronkhorst, S. (2010). Environmental conflicts: Key ISSUES and management implications. Afr. J. Conflict Resol. 10:63307. doi: 10.4314/ajcr.v10i2.63307
Bokonon-Ganta, A. H., de Groote, H., and Neuenschwander, P. (2002). Socio-economic impact of biological control of mango mealybug in Benin. Agric. Ecosyst. Environ. 93, 367–378. doi: 10.1016/S0167-8809(01)00337-1
Bossio, D., Geheb, K., and Critchley, W. (2010). Managing water for managing land: addressing land degradation to improve water productivity and rural livelihoods. Agric. Water Manag. 97, 536–542. doi: 10.1016/j.agwat.2008.12.001
Brander, M., Bernauer, T., and Huss, M. (2021). Improved on-farm storage reduces seasonal food insecurity of smallholder farmer households – Evidence from a randomized control trial in Tanzania. Food Policy 98:101891. doi: 10.1016/j.foodpol.2020.101891
Chabeda-Barthe, J., and Haller, T. (2018). Resilience of traditional livelihood approaches despite forest grabbing: ogiek to the west of mau forest, Uasin Gishu County. Land 7:140. doi: 10.3390/land7040140
Cohim, E., Leão, A. S., Neto, H. D. A. S., and Santos, G. M. (2021). Water loss associated with food loss and waste in Brazil. Rev. Bras. Ciênc. Ambientais 56, 305–317. doi: 10.5327/Z21769478885
Corbera, E. (2012). Problematizing REDD+ as an experiment in payments for ecosystem services. Curr. Opin. Environ. Sustain. 4, 612–619. doi: 10.1016/j.cosust.2012.09.010
Cruz-Garcia, G. S., Sachet, E., Vanegas, M., and Piispanen, K. (2016). Are the major imperatives of food security missing in ecosystem services research? Ecosyst. Serv. 19, 19–31. doi: 10.1016/j.ecoser.2016.04.001
Danso-Abbeam, G., Dagunga, G., and Ehiakpor, D. S. (2020). Rural non-farm income diversification: implications on smallholder farmers' welfare and agricultural technology adoption in Ghana. Heliyon 6:e05393. doi: 10.1016/j.heliyon.2020.e05393
Dobson, A., Dobermann, A., Janetos, A., Levy, M., Marco, D., Nakicenovic, N., et al. (2005). Drivers of Change in Ecosystem Condition and Services. Washington, DC: Island Press.
Dovie, D. B., Witkowski, E. T. F., and Shackleton, C. M. (2004). The fuelwood crisis in southern Africa- relating fuelwood use to livelihoods in a rural village. Geo. J. 60, 123–133. doi: 10.1023/B:GEJO.0000033597.34013.9f
Drescher, A., Glaser, R., Richert, C., and Nippes, K. R. (2011). Demand for Key Nutrients (NPK) in the Year 2050. Draft Report. University of Freiburg, Department of Geography, 77.
Dzikiti, S., Dzikiti, S., Schachtschneider, K., Naiken, V., Gush, M., and Moses, G. (2013). Water relations and the effects of clearing invasive prosopis trees on groundwater in an arid environment in the Northern Cape, South Africa. J. Arid Environ. 90, 103–113. doi: 10.1016/j.jaridenv.2012.10.015
Echendu, A. J., Hampo, C. C., Olatunde, D., Obasih, J. I., Oni, O., Ojo, D., et al. (2023). Biofuels as a key renewable energy source: a review of life cycle assessment studies in South Africa. Biofuels 15, 605–613. doi: 10.1080/17597269.2023.2264037
Elias, E. (2017). Characteristics of Nitisol profiles as affected by land use type and slope class in some Ethiopian highlands. Environ. Syst. Res. 6:20. doi: 10.1186/s40068-017-0097-2
Emmerson, M., Morales, M. B., Oñate, J. J., Batary, P., Berendse, F., Liira, J., et al. (2016). How agricultural intensification affects biodiversity and ecosystem services. Adv. Ecol. Res. 55, 43–97. doi: 10.1016/bs.aecr.2016.08.005
Ertiban, S. M. (2019). Soil fauna as webmasters, engineers, and bioindicators in ecosystems: implications for conservation ecology and sustainable agriculture. Am. J. Life Sci. 7:17. doi: 10.11648/j.ajls.20190701.14
Falloon, P. D., and Betts, R. A. (2009). Climate impacts on European agriculture and water management in the context of adaptation and mitigation – the importance of an integrated approach. Sci. Total Environ. 408, 5667–5687. doi: 10.1016/j.scitotenv.2009.05.002
FAO (2014). The State of Food and Agriculture: Innovation in Family Farming. Rome: Food and Agriculture Organization.
FAO (2016). Second International Conference on Nutrition (ICN2) Follow-up: Nutrition-related Implications for Agriculture and Livestock Development. COAG/2016/5. Rome. Available at: http://www.fao.org/3/a-mr235e.pdf (accessed June, 2024).
FAO (2017). Food-Based Dietary Guidelines. Available at: http://www.fao.org/nutrition/education/food-~dietaryguidelines/home/en/ (accessed May, 2024).
FAO and IFAD (2013). WFP, The State of Food Insecurity in the World 2013-The Multiple Dimensions of Food Security. Rome: FAO.
FAO IFAD, UNICEF, WFP, and WHO. (2019). The State of Food Security and Nutrition in the World 2019. Safeguarding Against Economic Slowdowns and Downturns. Rome: FAO.
FAO and ITPS (2015). Status of the World's Soil Resources (SWSR)- Main Report. Rome: Food and Agriculture Organization of the United Nations and Intergovernmental Technical Panel on Soils.
Fedele, G., Locatelli, B., Djoudi, H., and Colloff, M. J. (2018). Reducing risks by transforming landscapes: cross-scale effects of land-use changes on ecosystem services. PLoS ONE 13:e0195895. doi: 10.1371/journal.pone.0195895
Ferede, T., Ayenew, A. B., and Hanjra, M. A. (2013). Agroecology matters: Impacts of climate change on agriculture and its implications for food security in Ethiopia. Glob. Food Sec. 71–112. Retrieved from: http://www.oecd.org/env/cc/TADELE_AGROECOLOGY%20MATTERS%20(revised).pdf
Finlayson, C. M., Horwitz, P., and Weinstein, P. (2015). “A synthesis: wetlands as settings for human health,” in Wetlands and Human Health, eds. C. M. Finlayson, P. Horwitz, and P. Weinstein (Dordrecht: Springer), 251–263.
Foley, J. A., Ramankutty, N., Brauman, K. A., Cassidy, E. S., Gerber, J. S., Johnston, M., et al. (2011). Solutions for a cultivated planet. Nature 478, 337–342. doi: 10.1038/nature10452
Fyles, H., and Madramootoo, C. (2016). “1—Key drivers of food insecurity,” in Emerging Technologies for Promoting Food Security, ed. C. Madramootoo (Woodhead Publishing), 1–19. doi: 10.1016/B978-1-78242-335-5.00001-9
Gathogo, J. (2013). Historical developments of theological education in Eastern Africa– The example of Julius Krapf. Handb. Theol. Educ. Afr. 28–46. doi: 10.2307/j.ctv1ddcphf.13
Haines-Young, R., and Potschin, M. (2010). “The links between biodiversity, ecosystem services and human wellbeing,” in Ecosystem Ecology: A New Synthesis, eds. D. Raffaelli, and C. L. J. Frid (Cambridge: Cambridge University Press), 110–139.
Hansen, M. C., Potapov, P. V., Moore, R., Hancher, M., Turubanova, S. A., Tyukavina, A., et al. (2013). High-resolution global maps of 21st- century forest cover change. Science 342, 850–853. doi: 10.1126/science.1244693
Hoekstra, A. Y., Mekonnen, M. M., Chapagain, A. K., Mathews, R. E., and Richter, B. D. (2012). Global monthly water scarcity: blue water footprints versus blue water availability. PLoS ONE 7:e32688. doi: 10.1371/journal.pone.0032688
Howe, C., Suich, H., Vira, B., and Mace, G. M. (2014). Creating win-wins from trade-offs? Ecosystem services for human well-being: a meta-analysis of ecosystem service trade-offs and synergies in the real world. Glob. Environ. Change 28, 263–275 doi: 10.1016/j.gloenvcha.2014.07.005
IEA (2019). Africa Energy Outlook 2019, World Energy Outlook Special Report. Paris: International Energy Agency.
Ighodaro, I. D., Lewu, B. F., and Omoruyi, B. E. (2020). Smallholder farmers' adoption decision- making regarding soil erosion control on food security in South Africa. Ind. J. Agric. Res. 55, 42–50. doi: 10.18805/IJARe.A-533
IPBES (2018). “The IPBES regional assessment report on biodiversity and ecosystem services for Africa,” in Secretariat of the Intergovernmental Science-Policy Platform on Biodiversity and Ecosystem Services, eds. E. Archer, L. Dziba, K. J. Mulongoy, M. A. Maoela, and M. Walters (Bonn), 492.
IPCC (2014). “Climate change 2014: mitigation of climate change,” in Contribution of Working Group III to the Fifth Assessment Report of the Intergovernmental Panel on Climate Change, eds. O. Edenhofer, R. Pichs-Madruga, Y. Sokona, E. Farahani, S. Kadner, K. Seyboth, et al. (Cambridgel New York, NY: Cambridge University Press).
Jones, D. L., Cross, P., Withers, P. J. A., DeLuca, T. H., Robinson, D. A., Quilliam, R. S., et al. (2013). Nutrient stripping: the global disparity between food security and soil nutrient stocks. J. Appl. Ecol. 50, 851–862. doi: 10.1111/1365-2664.12089
Jones, J. W., Antle, J. M., Basso, B., Boote, K. J., Conant, R. T., Foster, I., et al. (2017). Toward a new generation of agricultural system data, models, and knowledge products: state of agricultural systems science. Agric. Syst. 155, 269–288. doi: 10.1016/j.agsy.2016.09.021
Kang, M., Siry, J., Colson, G., and Ferreira, S. (2019). Risk attitudes and conservation decisions: a case study of family forest owners in Georgia. For.Sci. 65, 201–210. doi: 10.1093/forsci/fxy047
Kebeda, B. T. (2019). Review on the expected role of climate smart agriculture on food system in Ethiopia. World J. Agric. Soil Sci. 2:548. doi: 10.33552/WJASS.2019.02.000548
Kishawi, Y., Mittelstet, A., Adane, Z., Shrestha, N., and Nasta, P. (2022). The combined impact of redcedar encroachment and climate change on water resources in the Nebraska Sand Hills. Front. Water 4:1044570. doi: 10.3389/frwa.2022.1044570
Komariah, I., and Matsumoto, T. (2019). Application of hydrological method for sustainable water management in the upper-middle ciliwung (UMC) River Basin, Indonesia. J. Water Environ. Technol. 17, 203–217. doi: 10.2965/jwet.18-003
Kumar, K., Pyare, R., Verma, V. K., Sachan, R., Niwas, R., Yadav, A., et al. (2022). Impact of moisture conservation practices, seed inoculation and zinc level on growth and yield of chickpea (Cicer arietinum L.). Int. J. Plant Soil Sci. 34, 546–556. doi: 10.9734/ijpss/2022/v34i2331618
Lal, R. (1987). Managing the soils of sub-Saharan Africa. Science 236, 1069–1076. doi: 10.1126/science.236.4805.1069
Lambin, E. F., Geist, H., and Lepers, E. (2003). Dynamics of land-use and landcover change in tropical regions. Annu. Rev. Environ. Resour. 28:105459. doi: 10.1146/annurev.energy.28.050302.105459
Lipper, L., Thorton, P., Campbell, B. M., Baedeker, T., Braimoh, A., Bwalya, M., et al. (2015). Climate-smart agriculture for food security. Nat. Clim. Change 4, 1068–1072. doi: 10.1038/nclimate2437
Liu, Q., Chen, Y., Li, V., Liu, Y., Han, J., Wen, X., et al. (2016). Plastic-film mulching and urea types affect soil CO2 emissions and grain yield in spring maize on the Loess Plateau, China. Sci. Rep. 6:28150. doi: 10.1038/srep28150
Lobell, D. B., and Gourdji, S. M. (2012). The influence of climate change on global crop productivity. Plant Physiol. 160, 1686–1697. doi: 10.1104/pp.112.208298
Lockwood, J. L., Cassey, P., and Blackburn, T. (2005). The role of propagule pressure in explaining species invasions. Trends Ecol. Evol. 20, 223–228. doi: 10.1016/j.tree.2005.02.004
Loucks, D. P. (2020). From analyses to implementation and innovation. Water 12:974. doi: 10.3390/w12040974
Lowder, S. K., Skoet, J., and Raney, T. (2016). The number, size, and distribution of farms, smallholder farms, and family farms worldwide. World Dev. 87, 16–29. doi: 10.1016/j.worlddev.2015.10.041
Madramootoo, C., and Fyles, H. (2012). Synthesis of findings from the four McGill conferences on global food security 2008–2011. Food Sec. 4, 307–317. doi: 10.1007/s12571-012-0185-0
Mahanty, S., Suich, H., and Tacconi, L. (2013). Access and benefits in payments for environmental services and implications for REDD+: lessons from seven PES schemes. Land Use Policy 31, 38–47. doi: 10.1016/j.landusepol.2011.10.009
Masso, C., Nziguheba, G., Mutegi, J., Galy-Lacaux, C., Wendt, J., Butterbach-Bahl, K., et al. (2017). “Soil fertility management in Sub-Saharan Africa,” in Sustainable Agriculture Reviews. Sustainable Agriculture Reviews, Vol 25, ed. E. Lichtfouse (Cham: Springer).
Mazzocchi, M., Shankar, B., and Traill, B. (2012). The Development of Global Diets since 1992: Influences of Agri-food Sector Trends and Policies. FAO Commodity and Trade Policy Research Working Paper No. 34. Second International Conference on Nutrition, Rome, Italy.
Mfwango, L. H., Ayenew, T., and Mahoo, H. F. (2022). Impacts of climate and land use/cover changes on streamflow at Kibungo Sub-Catchment, Tanzania. Heliyon 8:e11285. doi: 10.1016/j.heliyon.2022.e11285
Millennium Ecosystem Assessment (2005). Ecosystems and Human Well-being: Biodiversity Synthesis. Washington, DC: World Resources Institute.
Mthembu, A., and Hlophe, S. (2021). Building resilience to climate change in vulnerable communities: a case study of uMkhanyakude district municipality. Town Reg. Plan. 77, 42–56. doi: 10.18820/2415-0495/trp77i1.4
Mugure, A., and Oino, P. (2013). Farmer-oriented factors that influence adoption of agroforestry practices in Kenya: Experiences from Nambale District, Busia County. Int. J. Sci. Res. 4.
Muhumuza, M., and Balkwill, K. (2013). Factors affecting the success of conserving biodiversity in national parks: a review of case studies from Africa. Int. J. Biodivers. 2013:20. doi: 10.1155/2013/798101
Muller, G. C., Junnila, A., Traore, M. M., Traore, S. F., Doumbia, S., Sissoko, F., et al. (2017). The invasive shrub Prosopis juliflora enhances the malaria parasite transmission capacity of Anopheles mosquitoes: a habitat manipulation experiment. Malar. J. 16:237. doi: 10.1186/s12936-017-1878-9
Müller, M., Dembélé, S., Zougmoré, R. B., Gaiser, T., and Partey, S. T. (2020). Performance of three sorghum cultivars under excessive rainfall and waterlogged conditions in the Sudano- Sahelian Zone of West Africa: A Case Study at the Climate-Smart Village of Cinzana in Mali. Water 12:2655. doi: 10.3390/w12102655
Muyanga, M., Jayne, T. S., and Burke, W. J. (2013). Pathways into and out of poverty: a study of rural household wealth dynamics in Kenya. J. Dev. Stud. 49, 1358–1374. doi: 10.1080/00220388.2013.812197
Ndhlovu, T., Milton-Dean, S., and Esler, K. J. (2011). Impact of Prosopis (mesquite) invasion and clearing on the grazing capacity of semiarid Nama Karoo rangeland, South Africa. Afr. J. Range For. Sci. 28, 129–137. doi: 10.2989/10220119.2011.642095
Neuenschwander, P. (2010). Importance of biological control for tropical Africa. J. Kulturpflanzen 62,97–101.
Niang, I., Ruppel, O. C., Abdrabo, M. A., Essel, A., Lennard, C., Padgham, J., et al. (2014). Africa. Climate Change 2014: Impacts, Adaptation and Vulnerability: Part B: Regional Aspects: Working Group II Contribution to the Fifth Assessment Report of the Intergovernmental Panel on Climate Change, 1199–1266. New York, NY: Cambridge University Press. doi: 10.1017/CBO9781107415386.002
Ofori, S. A., Cobbina, S. J., and Obiri, S. (2021). climate change, land, water, and food security: perspectives from Sub-Saharan Africa. Front. Sustain. Food Syst. 5:680924. doi: 10.3389/fsufs.2021.680924
Ola, O., Menapace, L., Benjamin, E., and Lang, H. (2019). Determinants of the environmental conservation and poverty alleviation objectives of payments for ecosystem services (PES) programs. Ecosyst. Serv. 35, 52–66. doi: 10.1016/j.ecoser.2018.10.011
Owusu, K., Emmanuel, A. K., Musah-Surugu, I. J., and Yankson, P. W. (2019). The effects of 2015 El Nino on smallholder maize production in the transitional ecological zone of Ghana. Int. J. Clim. Change Strat. Manag. 11609–621. doi: 10.1108/IJCCSM-02-2018-0014
Paini, D. R., Sheppard, A. W., Cook, D. C., Barro, P. J., De Worner, S. P., and Thomas, M. B. (2016). Global threat to agriculture from invasive species. Proc. Natl. Acad. Sci. U. S. A. 113, 7575–7579. doi: 10.1073/pnas.1602205113
Palaniappan, M., and Gleick, P. (2009). “Peak water,” in The World's Water 20082009. The Biennial Report on Freshwater Resources, Vol. 36, ed. P. H. Gleick (Washington, DC: Island Press), 171. doi: 10.1017/s0376892909990099
Pandey, H. P., and Bhusal, M. (2016). A comparative study on carbon stock in Sal (Shorea robusta) forest in two different ecological regions of Nepal. Banko Janakari 26, 24–31. doi: 10.3126/banko.v26i1.15498
Pereira, P., Inacio, M., Kalinauskas, M., Bogdzevič, K., Bogunovic, I., and Zhao, W. (2022). Land-Use Changes and Ecosystem Services. Elsevier eBooks, 1–27.
Permadi, D., Burton, M., Pandit, R., Race, D., and Walker, L. (2018). Local community's preferences for accepting a forestry partnership contract to grow pulpwood in Indonesia: a choice experiment study. For.Policy Econ. 91, 73–83. doi: 10.1016/j.forpol.2017.11.008
Power, A. G. (2010). Ecosystem services and agriculture: tradeoffs and synergies. Philos Trans. Soc. B Biol. Sci. 365, 2959–2971. doi: 10.1098/rstb.2010.0143
Raffa, K. F., Aukema, B. H., Bentz, B. J., Carroll, A. L., Hicke, J. A., Turner, M. G., et al. (2008). Cross-scale drivers of natural disturbances prone to anthropogenic amplification: the dynamics of bark beetle eruptions. Bioscience 58, 501–517. doi: 10.1641/B580607
Reilly, J., Tubiello, F., McCarl, B., Abler, D., Darwin, R., Fuglie, K., et al. (2003). U.S. agriculture and climate change: new results. Clim. Change 57, 43–69. doi: 10.1023/A:1022103315424
REN21 (2013). Ethanol Industry Outlook. Battling for the Barrel. Renewables 2013 Global Status Report. REN21 Secretariat. Paris: Renewable Fuels Association. Available at: http://www.ethanolrfa.org/pages/annual-industry-outlook (accessed June, 2024).
Richardson, K., Steffen, W., Lucht, W., Bendtsen, J., Cornell, S. E., Donges, J. F., et al. (2023). Earth beyond six of nine planetary boundaries. Sci. Adv. 9:eadh2458. doi: 10.1126/sciadv.adh2458
Rogerson, C. M., and Rogerson, J. M. (2014). Agri-tourism and local economic development in South Africa. Bull. Geogr. Socio-Econ. Ser. 26, 93–106. doi: 10.2478/bog-2014-0047
Rutten, M., Shutes, L., and Meijerink, G. (2013). Sit down at the ball game: how trade barriers make the world less food secure. Food Policy 38, 1–10. doi: 10.1016/j.foodpol.2012.09.002
Schlenker, W., and Lobell, D. B. (2010). Robust negative impacts of climate change on African agriculture. Environ. Res. Lett. 5:014010. doi: 10.1088/1748-9326/5/1/014010
Senyolo, M. P., Long, T. B., Blok, V., and Omta, O. (2018). How the characteristics of innovations impact their adoption: an exploration of climate-smart agricultural innovations in South Africa. J. Clean. Prod. 172, 3825–3840. doi: 10.1016/j.jclepro.2017.06.019
Shcherbak, I., Millar, N., and Robertson, G. P. (2014). Global metaanalysis of the nonlinear response of soil nitrous oxide (N2O) emissions to fertilizer nitrogen. Proc. Nat. Acad. Sci. U. S. A. 111, 9199–9204. doi: 10.1073/pnas.1322434111
Smith, S., Rowcroft, P., Rogers, H., Quick, T., Eves, C., White, C., et al. (2013). Payments for Ecosystem Services: A Best Practice Guide. Available at: https://www.open-access.bcu.ac.uk/3319/ (accessed July, 2024).
Squire, G. R., Hawes, C., Valentine, T. A., and Young, M. W. (2015). Degradation rate of soil function varies with trajectory of agricultural intensification. Agric. Ecosyst. Environ. 202, 160–167. doi: 10.1016/j.agee.2014.12.004
Steffen, W., Richardson, K., Rockström, J., Cornell, S. E., Fetzer, I., Bennett, E. M., et al. (2015). Planetary boundaries: Guiding human development on a changing planet. Science 347:1259855. doi: 10.1126/science.1259855
Swinnen, J., and Squicciarini, P. (2012). Global food supply. Mixed messages on prices and food security. Science 335, 405–406. doi: 10.1126/science.1210806
Tadele, Z., and Assefa, K. (2012). Increasing food production in Africa by boosting the productivity of understudied crops. Agronomy 2:240283. doi: 10.3390/agronomy2040240
Tata Ngome, P. I., Shackleton, C., Degrande, A., Nosse, E. J., and Ngome, F. (2019). Assessing household food insecurity experience in the context of deforestation in cameroon. Food Policy 84, 57–65. doi: 10.1016/j.foodpol.2019.02.003
Tsiafouli, M. A., Thébault, E., Sgardelis, S. P., de Ruiter, P. C., van der Putten, W. H., Birkhofer, K., et al. (2015). Intensive agriculture reduces soil biodiversity across Europe. Glob. Chang. Biol. 21, 973–985. doi: 10.1111/gcb.12752
Tully, K., Sullivan, C., Weil, R., and Sanchez, P. (2015). The state of soil degradation in subsaharan africa: baselines, trajectories, and solutions. Sustainability 7, 6523–6552. doi: 10.3390/su7066523
Udo, H., Aklilu, H., Phong, L., Bosma, R., Budisatria, I., Patil, B., et al. (2011). Impact of intensification of different types of livestock production in smallholder crop-livestock systems. Livest. Sci. 139, 22–29. doi: 10.1016/j.livsci.2011.03.020
UNEP (2012). UNEP 2011 Annual Report. United Nations Environmen Programme. Available at: https://wedocs.unep.org/20.500.11822/8053 (accessed June, 2024).
van Loon, M. P., Hijbeek, R., Ten Berge, H. F., De Sy, V., Ten Broeke, G. A., Solomon, D., et al. (2019). Impacts of intensifying or expanding cereal cropping in sub-Saharan Africa on greenhouse gas emissions and food security. Glob. Change Biol. 25, 3720–3730. doi: 10.1111/gcb.14783
Vihervaara, P., Rönkä, M., and Walls, M. (2010). Trends in ecosystem service research: early steps and current drivers. Ambio 39, 314–324. doi: 10.1007/s13280-010-0048-x
Waruingi, E., Mbeche, R., and Ateka, J. (2021). Determinants of forest-dependent household's participation in payment for ecosystem services: evidence from plantation establishment livelihood improvement scheme (PELIS) in Kenya. Glob. Ecol. Conserv. 26:e01514. doi: 10.1016/j.gecco.2021.e01514
Watson, S. C. L., Newton, A. C., Ridding, L. E., Evans, P. M., Brand, S., McCracken, M., et al. (2021). Does agricultural intensification cause tipping points in ecosystem services? Landsc. Ecol. 36, 3473–3491. doi: 10.1007/s10980-021-01321-8
Weatherley-Singh, J., and Gupta, A. (2015). Drivers of deforestation and REDD+ benefit-sharing: a meta-analysis of the (missing) link. Environ. Sci. Policy 54, 97–105. doi: 10.1016/j.envsci.2015.06.017
WHES (2015). World Hunger and Poverty Facts and Statistics. World Hunger Education Service. Available at: http://www.worldhunger.org/2015-world-hunger-and-poverty-facts-and-~statistics (accessed July, 2024).
Wilhite, D. A., Sivakumar, M. V. K., and Pulwarty, R. (2014). Managing drought risk in a changing climate: the role of national drought policy. Weather Clim. Extremes 3, 4–13. doi: 10.1016/j.wace.2014.01.002
Wondie, M., and Mekuria, W. (2018). Planting of acacia decurrent and dynamics of land cover change in Fagita Lekoma District in the Northwestern Highlands of Ethiopia. Mt. Res. Dev. 38, 230–239. doi: 10.1659/MRD-JOURNAL-D-16-00082.1
World Bank (2013a). Middle Income Countries. Available at: http://www.worldbank.org/en/country/mic (accessed July, 2024).
World Bank (2013b). Turn Down the Heat: Climate Extremes, Regional Impacts, and the Case for Resilience. A report for the World Bank by the Potsdam Institute for Climate Impact Research and Climate Analytics., Washington, DC.
World Bank (2019). Data, Population Growth. The World Bank Group. Available at: https://data.worldbank.org/indicator/SP.POP.GROW (accessed October 16, 2019).
World Health Organization (2018). The State of Food Security and Nutrition in the World 2018. Building Climate Resilience for Food Security and Nutrition. Rome: FAO.
Wudil, A. H., Usman, M., Rosak-Szyrocka, J., Pilar, L., and Boye, M. (2022). Reversing years for global food security: a review of the food security situation in Sub-Saharan Africa (SSA). Int. J. Environ. Res. Public Health 19:14836. doi: 10.3390/ijerph192214836
Yonow, T., Kriticos, D. J., and Ota, N. (2017). The potential distribution of cassava mealybug (Phenacoccus manihoti), a threat to food security for the poor. PLoS ONE 12:e0173265. doi: 10.1371/journal.pone.0173265
Zhang, L., Xue, B., Yan, Y., Wang, G., Sun, W., Li, Z., et al. (2019). Model uncertainty analysis methods for semi-arid watersheds with different characteristics: a comparative SWAT case study. Water11:1177. doi: 10.3390/w11061177
Zhang, W., Kato, E., Bianchi, F. J. J. A., Bhandary, P., Gort, G., and van der Werf, W. (2018). Farmers' perceptions of crop pest severity in Nigeria are associated with landscape, agronomic and socio-economic factors. Agric. Ecosyst. Environ. 259, 159–167. doi: 10.1016/j.agee.2018.03.004
Zhichen, Y., Hong, L., and Jinshun, B. (2015). “Effects on soil organic carbon and microbial biomass carbon of different tillage,” in In 2015 AASRI International Conference on Circuits and Systems (CAS 2015) (Atlantis Press), 21–24.
Zhou, M., Brandt, P., Pelster, D., Rufino, M. C., Robinson, T., and Butterbach-Bahl, K. (2014). Regional nitrogen budget of the Lake Victoria Basin, East Africa: syntheses, uncertainties and perspectives. Environ. Res. Lett. 9:105009. doi: 10.1088/1748-9326/9/10/105009
Zhu, Z., and Chen, D. (2002). Nitrogen fertilizer use in China – Contributions to food production, impacts on the environment and best management strategies. Nutr. Cycl. Agroecosyst. 63, 117–127. doi: 10.1023/A:1021107026067
Zingore, S., Mutegi, J., Agesa, B., Tamene, L., and Kihara, J. (2015). Soil degradation in sub- Saharan Africa and crop production options for soil rehabilitation. Better Crops 99, 24–26. Available at: https://hdl.handle.net/10568/68702
Keywords: food security, payment for ecosystem services, sustainable agriculture intensification, climate change adaptation, smallholder farmers
Citation: Aryee GA, Sardinha ID and Branquinho C (2024) Linking drivers of food insecurity and ecosystem services in Africa. Front. Sustain. Food Syst. 8:1272332. doi: 10.3389/fsufs.2024.1272332
Received: 03 August 2023; Accepted: 25 July 2024;
Published: 15 August 2024.
Edited by:
Albie F. Miles, University of Hawaii–West Oahu, United StatesReviewed by:
Shalander Kumar, International Crops Research Institute for the Semi-Arid Tropics (ICRISAT), IndiaLilei Zhou, Chongqing Jiaotong University, China
Copyright © 2024 Aryee, Sardinha and Branquinho. This is an open-access article distributed under the terms of the Creative Commons Attribution License (CC BY). The use, distribution or reproduction in other forums is permitted, provided the original author(s) and the copyright owner(s) are credited and that the original publication in this journal is cited, in accordance with accepted academic practice. No use, distribution or reproduction is permitted which does not comply with these terms.
*Correspondence: Gregory Ayitey Aryee, gregoryaryee@iseg.ulisboa.pt; rhystika200@gmail.com