- 1Institute of Applied Technology and Sustainable Development, Nguyen Tat Thanh University, Ho Chi Minh City, Vietnam
- 2Faculty of Chemical Engineering and Food Technology, Nong Lam University, Ho Chi Minh City, Vietnam
- 3Faculty of Environmental and Food Engineering, Nguyen Tat Thanh University, Ho Chi Minh City, Vietnam
- 4College of Agriculture, Can Tho University, Can Tho City, Vietnam
- 5School of Graduate, Can Tho University, Can Tho City, Vietnam
- 6Department Chemistry, Soongsil University, Seoul, Republic of Korea
In this study, the heat pump drying technique was applied to develop the production process of dried mango from Tu Quy mango sources, Ben Tre province, Vietnam. The experiment was performed in three steps. First, mango slices were treated through the blanching process. Samples were pretreated with sizes from 6 to 12 mm at 80–95°C for 3–6 min, and then the mango slices were soaked in a syrup solution concentration range of 25–40°Bx, citric acid of 0.5–2%, and glycerol of 0.1–0.4% at 35–65°C for 90–180 min. Finally, the samples were dried using a pilot-scale heat pump dryer until the moisture content reached 14–16%. The results showed that the blanching and osmosis processes were carried out under optimal conditions to inactivate the Polyphenol oxidase (PPO) enzyme, obtain maximum polyphenol compounds, and conform to the product appearance. Additionally, the drying process was carried out at 30°C for 1,080 min with the polyphenol content retaining 11.71 mgGAE/gDW—This signifies a successful endeavor in developing production methodologies and expanding the range of mango-derived products. Employing innovative technological processes enhances both the quantity and quality of the products. Moreover, the manufacturing procedure was executed on a pilot device, streamlining the synchronization of large-scale production processes effortlessly. This advancement not only enhances economic value but also addresses the issue of congestion prevalent in the current state of the mango industry in Vietnam.
1 Introduction
Mango (Mangifera india L.) (Anacardiaceae) is native to Eastern India and tropical regions such as Myanmar, Vietnam, and Malaysia. Mango is one of the tropical fruits grown worldwide with a cultivation area of about 3.7 million hectares (Jahurul et al., 2015). The fruit flesh contains about 15% total sugars. Mango contains 1.5–5.5% total protein polyphenol content (96.27 mg GAE/ 100 g), Vitamin C (35.25 mg/ 100 g), and antioxidant activity (4.68%) (Maldonado-Celis et al., 2019; Tran et al., 2021). Notably, bioactive compounds (e.g., phenolic, flavonoids, chlorophyll, carotenoids, and other volatile compounds) are involved in disease prevention and treatment support are also identified in mangoes (Parvez, 2016). In addition, mangoes contain many powerful antioxidant compounds such as isoquercitrin, quercetin, fisetin, methyl gallate, gallic acid, and astragalin that prevent free radicals formation and cancer and decelerate the aging process. In addition, mangoes contain a high level of pectin and fiber, which reduce serum cholesterol levels, improve eyesight, prevent blood lipid disorders, strengthen the immune system, and support the excretory system secretion and health promotion (Maldonado-Celis et al., 2019; Thi Thanh Huong et al., 2022).
Currently, food processing is of interest to both scientists and manufacturers. The impact of pre-treatment and drying techniques on several properties of dried mango was investigated by Osunde (2017). The findings revealed that, compared to the ascorbic acid-treated, steam-blanched, and control samples, the mango samples treated with honey had the highest level of residual vitamin C (140.35 mg/100 g). Additionally, using drying techniques, the samples treated with honey obtained the maximum value of 5921.90 g/100 g for beta-carotene. Therefore, it can be concluded that using a honey and ascorbic acid solution in the pretreatment step helps to retain the biological activities in mango better. Nyangena et al. (2019) investigated how the antioxidant properties of dried mango slices were affected by pretreatment before drying. Dried mango slices showed the highest levels of vitamin C (78870.46 mg/100 g), β-carotene (ranging from 66540.88 mg/100 g), total polyphenols (5.83–29.9 mgGAE/100 g), and flavonoids (43.66–1.27 mg/100 g). The results demonstrated that pre-treatment of mango slices with 1% citric acid, steam blanching for 3 min, and drying at 50°C could improve the antioxidant retention in dried mango products (Nyangena et al., 2019). Indeed, pretreatment processes such as blanching methods are used to preserve the nutritional values of fruits well.
Drying technology is selected to support the processing of fruit and vegetable products due to the economic advantages and ease of implementation. One of the popular and highly effective drying techniques is known as hot air convection drying (HAD) (Nguyen et al., 2021; Dao et al., 2022). During drying, hot air serves as the heating source and the steam transport. This approach has more advantages than solar drying, such as regulating temperature, velocity, and relative humidity and reducing environmental pollution (Udomkun et al., 2020). The product is exposed to hot air for a prolonged time which is associated with lipid oxidation (Gang et al., 2019). Microwave drying is accomplished by continuously rotating water molecules due to the rapidly evolving electromagnetic fields. Moisture can evaporate from inside the surface due to the heat produced by the friction between water molecules (Punathil and Basak, 2016). Vacuum microwave drying dries products at a lower temperature and pressure than hot air drying. This method is intended for heat-sensitive foods. This method can significantly reduce lipid oxidation because the lipids are reduced or eliminated, and the product temperature is low. However, vacuum drying typically requires a lengthy period due to the slow drying rate (Wojdyło et al., 2014). Vacuum drying is frequently combined with microwave drying or other methods to boost drying efficiency. Previous studies have shown that vacuum microwave drying reduces product lipid oxidation (Ahmad et al., 2021). Shende and Datta (2020) optimized the refractive window (RW) drying of Langra mangoes. When drying at 95°C with a drying time of 22.5 min and mango slice thickness of 2.49 mm, the content of total ascorbic acid, total polyphenol, and hardness of the mango products were 62.33 mg/100gDW, 7.72 mgGAE/gDW, and 4.60 N, respectively. More nutrients were preserved in the microstructure of the mango skin and pulp. After the RW drying process, the mangoes were smooth and non-shedding with a uniform powder particle thickness, in contrast to tray and oven drying.
Recently, the heat pump drying method has been recognized as a drying approach that can improve energy efficiency while controlling drying temperature and air humidity (Jangam and Mujumdar, 2014). Tran et al. successfully produced mango using ultrasound-assisted cold drying. The optimized drying process, with parameters set at a temperature of 45°C, 2.07 W/g ultrasonic power, and 1 cm material thickness, yielded Total polyphenol (TP) and Total ascorbic acid (TA) values of 338.994 mg GAE/100 g and 36.783 mg AA/100 g, respectively. Validatory experiments confirmed the effectiveness of the ultrasound-assisted cold drying process, emphasizing the preservation of TP and TA content using low temperatures (Tran et al., 2021). Furthermore, Thao et al.’s (2023) research indicates that the heat pump drying method enhances the quality of dried mango slices by improving the kinetic parameters of the extraction process. Polyphenols are considered a quality indicator during drying, and the optimal mango sample, with a 10 mm thickness, was selected at 40°C for 1,080 min, retaining a total polyphenol content of 12.17 mgGAE/gDW. Indeed, when operating at low temperatures, products dried using this process typically have lower levels of total volatile base nitrogen, improved volatility, and less lipid oxidation. Therefore, it is appropriate for drying materials that are sensitive to heat. Infrared drying, freeze drying, and superheated steam drying are only a few current drying techniques. It is possible to make high-quality dried products with reduced energy costs by combining several drying procedures. Based on the properties of the sample, drying time, energy cost, and equipment investment, the drying process frequently needs to be optimized (Wang et al., 2011). Heat pump drying utilizes a system with a heat pump, auxiliary heater, and dehumidification system to precisely control drying temperature and humidity in a drying chamber. The heat pump serves two key functions: drying the air and utilizing the generated heat to further warm the drying air. The critical aspect is the dehumidification method, ensuring the air is thoroughly dried before being reintroduced to the chamber, facilitating faster product drying at various temperatures. However, because of technical factors, heat pump drying systems usually only work at a temperature range of 10–50°C, and the drying air has a low humidity of 10–20% (Wang et al., 2011; Minea, 2015).
Drying methods are selected to suit the materials depending on the characteristics of the material and the economic efficiency it brings. For example, mango has a high content of nutrients such as vitamins and antioxidants which are easily lost due to high temperatures during conventional drying, such as convection drying and contact drying. Therefore, using the heat pump drying method instead of conventional drying methods at a high temperature helps improve product quality and increase economic value. On the other hand, as the demand for domestic products increases, the diversification of processed products needs to be invested appropriately to promptly meet the domestic consumption of goods and the off-season periods of agricultural products. For the above reasons, the research and development of the production process of dried Tu Quy mangoes was experimentally produced. Tu Quy mango is a prominent fruit in the Mekong Delta region of Vietnam. It has been authorized as “Thanh Phu mango” 2021 by the National Office of Intellectual Property of the Ministry of Science and Technology. In this study, to actual production conditions, the effects of three key processes were assessed: drying, osmosis, and the influence of water blanching on the quality of mango slices. In particular, a pilot device that was similar to the actual production process was used to evaluate the tests. The dried mango product is invested and developed by the Department of Science and Technology of Ben Tre province to address the issues of surplus raw materials and difficulties in consuming fresh products, thereby enhancing the capacity for fresh mango production. This initiative also aims to improve preservation capabilities, minimize storage and transportation requirements, and increase revenue for enterprises adopting the technology and engaging in trade.
2 Experiment
2.1 Samples
Tu Quy mango (Mangifera indica L.) was harvested in December 2021 in Thanh Phu district, Ben Tre province. The selected samples were mature fruit at stage 3 with a yellow color of the skin of 41–70%, Total Soluble solids (TSS) of 20–22°Bx, and about 700 ± 100 g of weight, at which the highest polyphenol content was accumulated (Palafox-Carlos et al., 2012). After being transported to the laboratory, samples were stored at 5°C and used within no more than three days to maintain product quality.
2.2 Chemicals and agent
The chemicals used in the study, including gallic acid, 2,6-dichlorophenolindophenol, and the Folin–Ciocalteu reagent with a purity of 99.99%, were imported from Sigma-Aldrich Chemical, Co. Ltd. (United States). Methanol, Na2CO3, and 99.5% pure L-ascorbic acid were purchased from Xilong (China).
2.3 Technological process of drying products
After harvesting, Tu Quy mangoes were classified to remove damaged and unsatisfactory fruits. The fruit was subsequently rinsed with water, and a sharp 0.5 mm knife was employed to eliminate the seeds and peel. The mango sample was sliced to the appropriate size and blanched at 90°C for 4 min, which was previously evaluated as suitable for inactivating the PPO enzyme of mango slices. The osmosis process was then carried out at 35°C for 1.5 h with a syrup solution of 35°Bx. Finally, the sample was placed in the drying device at 50°C until the moisture content dropped to 14–16% (Figure 1).
The experiment was arranged and conducted with single-factor experiments.
• Blanching was carried out at fixed conditions of 90°C for 4 min. The survey processes were changed in size from 6 to 12 mm, temperature from 80 to 95°C, and time from 3 to 6 min.
• The osmosis process was carried out at fixed conditions of 35°C for 2 h. The survey processes were changed in the concentration of syrup from 25 to 40°Bx, citric acid from 0.5 to 2%, and glycerol from 0.1 to 0.4%. Survey parameters include temperatures of 35–65°C for 1.5–3 h.
• The drying process was carried out at the survey temperatures from 20 to 50°C and wind speeds from 40 to 55 Hz.
2.4 Determination of the ability to inactivate the PPO enzyme
Procedure: After water blanching, the mango slices were quickly cooled and pureed. To 1 g of the pureed sample, 10 mL of distilled water, 2 mL of guaniacol (1%), and 2 mL of H2O2 (3%) were added.
The color change was observed within 10 min. The color of the sample changes to red-brown if the PPO enzyme was present in the sample and gradually turns colorless if the mango slices inactivate the PPO enzyme (Shivhare et al., 2009).
2.5 Determination of total polyphenol content
First, weigh 0.5 g of mango juice and add it to a blender containing an alcohol solution. Adjust the volume to 50 mL with alcohol and then filter the mixture. Following filtration, transfer the samples into tubes, each containing 0.1 mL of extract and 0.5 mL of 10% Folin–Ciocalteu reagent. The reaction is initiated after 5 minutes, followed by the addition of 0.4 mL of 7.5% Na2CO3 into each tube. After stabilizing for 60 minutes, measure the absorbance of the samples using a UV–VIS spectrophotometer at a wavelength of 765 mm (Nguyen et al., 2020; Nhut et al., 2020; Tran et al., 2022).
The Total polyphenol content (TPC) of the dried material was measured in gallic acid equivalents (GAE) and was calculated by Equation (1):
where V1 = the volume of the cuvet, mL, V2 = the volume of the container of the extracted sample, mL, V3 = extract volume of fresh sample, mL, C1 = TPC measured from UV–vis, μg/mL, and h = the moisture in the sample.
2.6 Data processing
Each analytical experiment was repeated three times and expressed as mean ± standard deviation (S.D.). MS Excel software was used to process experimental data, and SPSS was used to perform a one-way analysis of variance (ANOVA) test with significance at 5%.
3 Results and discussion
3.1 Evaluation of the effect of blanching on the quality of Tu Quy mango slices
3.1.1 Effect of sample size on quality of mango slices during blanching
The blanching process is considered a pre-treatment to support the drying process. It is made to inactivate the browning enzyme (PPO) while preserving mango slices’ structure, color, and quality. However, during processing, the nutrient content, color, and texture of raw materials are significantly affected by heat (Dao et al., 2021). Therefore, sample size is one of the main factors that affect the blanching process. The examined size of mango slices ranged from 6 to 12 mm at a blanching temperature of 90°C for 4 min, as shown in Table 1 and Supplementary Figure S1. Results showed that the lower limit (6 mm) limited the structure of soft and fragile slices, while the upper limit (12 mm) was more suitable to maintain the shape of the dried mango product. The color and texture of mango slices tend to increase with increasing sample size (Supplementary Figure S1). Mango slices at 6 mm gave the highest value of soft appearance and color, as compared to fresh samples. In contrast, mango slices at 12 mm were firmer in size and brighter in color than the samples at 6 and 8 mm. Additionally, the 8 and 10 mm mango slices had a better structure than the 6 mm slices.
The results of ANOVA statistical analysis showed that a–c had a statistically significant difference (p < 0.05). The color parameters (L*, a*, b*, and ∆E*) are presented in Table 1, and the appearance change of the mango during blanching is shown in Figure 2. L-value (darkness/lightness), a-value (greenness/redness), and b-value (blueness/yellowness) were used to calculate ∆E of blanched samples at thicknesses of 6 mm, 8 mm, 10 mm, and 12 mm, which was 32.26 ± 0.75, 28.88 ± 0.61, 7.86 ± 0.89, and 16.19 ± 1.66, respectively. All color values of blanched samples were significantly reduced compared with fresh samples. It can be observed in Supplementary Figure S1 that thickness affects color during blanching (p < 0.05), and there is a significant difference between different levels of survey. The 10 mm sample with the lowest ∆E* (7.86 ± 0.89) is the best choice to preserve most of the color closest to the original sample. It shows that when blanching was fixed at 90°C for 4 min, the thinner the samples, the more fragile the ripe mango slices’ structure, leading to the color deterioration (Xu et al., 2015).
The thickness of mango slices significantly affects product quality and processing characteristics. The inactivation of enzymes in thin slices was faster with more rapid moisture loss than PPO enzymes when exposed to temperatures of 70–90°C, which destroy their catalytic activity and lead to inactivation (Zhu et al., 2010). Therefore, the sample had a reddish brown color when the PPO enzyme remained inactivated. When the PPO enzyme content was reduced, the reddish-brown color became lighter until the color disappeared, indicating that the PPO enzyme was completely inactivated. Figure 2 and Supplementary Table S1 show that when blanching mangoes at 90°C for 4 min, the samples at size 6, 8, and 10 mm inactivated the PPO enzyme. Meanwhile, at 90°C for 4 min, it was impossible to completely inactivate the PPO enzyme in 12 mm thick mango slices, which showed a reddish-brown color.
As shown in Figure 3, the TPC increases with the increasing size of mango slices. At 12 mm thickness, the highest TPC was obtained with the value of 105.31 ± 2.32 mgGAE/gDW. The opposite value was obtained at the size of 6 mm, the TPC was 66.12 ± 1.44 mgGAE/gDW, which was equal to the control sample. Furthermore, the values at 8 mm and 10 mm yielded TPC of 80.68 ± 2.50 mgGAE/gDW and 91.11 ± 1.97 mgGAE/gDW, respectively. At these sizes, the obtained TPC values were 129 and 146% higher than the control samples, respectively. The natural polyphenol oxidase can be totally inactivated by the increase in TPC during blanching, which can also cause the release of related phenolic acids from the breakdown of plant cell wall components. As a result, the size of 12 mm offers the maximum TPC and acceptable sensory and color characteristics. However, this size does not inactivate the PPO enzyme (Figure 3), a crucial marker for assessing how well the blanching procedure worked. Therefore, the mango slice size of 10 mm was selected for the following tests.
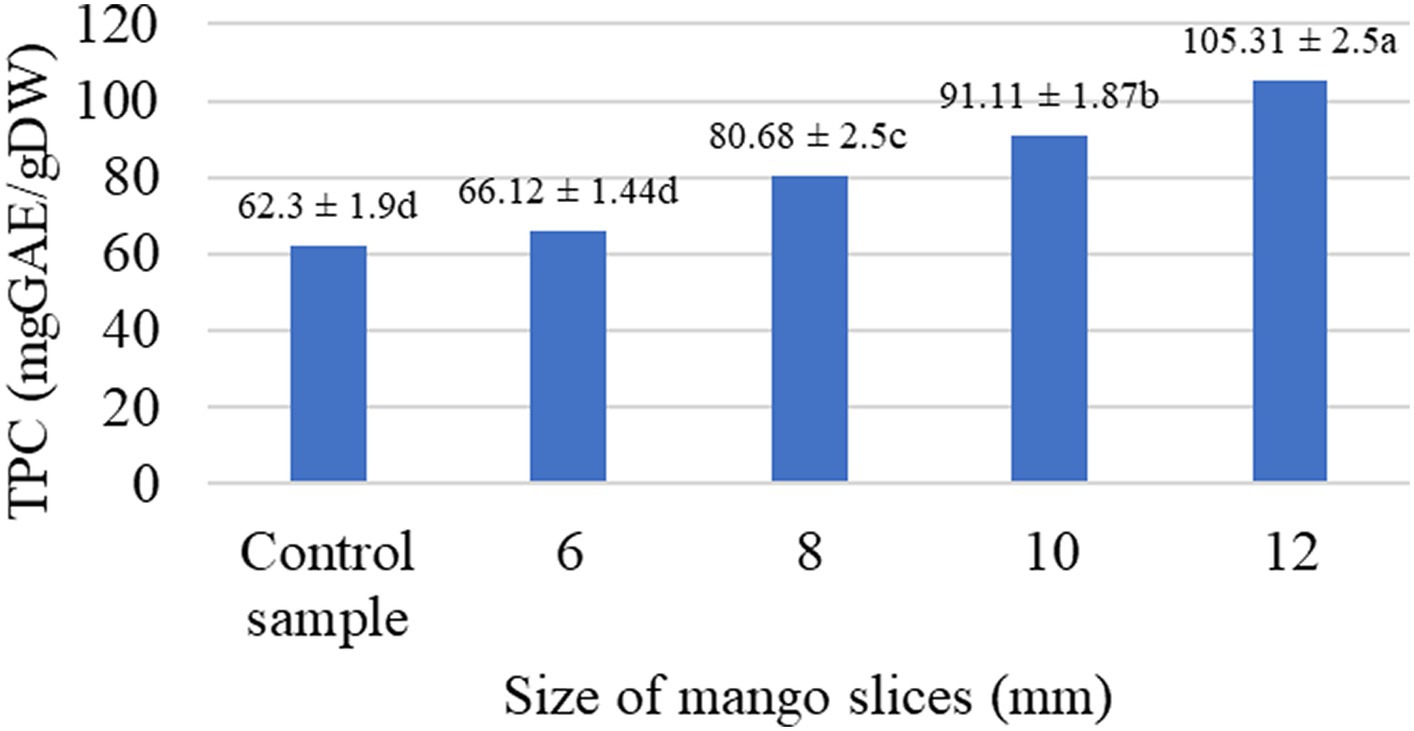
Figure 3. Effect of size on TPC during blanching. *(a-d) indicates a statistically significant difference (p-value<0.05).
3.1.2 Effect of temperature on quality of mango slices during blanching
Temperature is one of the main factors affecting the blanching process. The temperature was investigated from 80 to 95°C at fixed conditions such as 10 mm in size and 4 min blanching time, as shown in Table 2 and Supplementary Figure S2.
The temperature significantly affected product quality and the ability to inactivate PPO enzymes. The higher the temperature, the faster the inactivation of PPO took place. Table 2 and Supplementary Table S2 show that at 80°C and 85°C, the PPO enzyme had not been inactivated, as indicated by the reddish-brown color. In contrast, at 90°C and 95°C under the same conditions, PPO enzymes were inactivated (the test tube was clear, and no red-brown color appeared). Indeed, it can be seen that 95 o C for 4 min can inactivate the enzyme PPO. However, this range yielded the lowest color and TPC values compared with other temperatures (Figure 4). Therefore, the temperature of 90°C was selected as the best survey criterion.
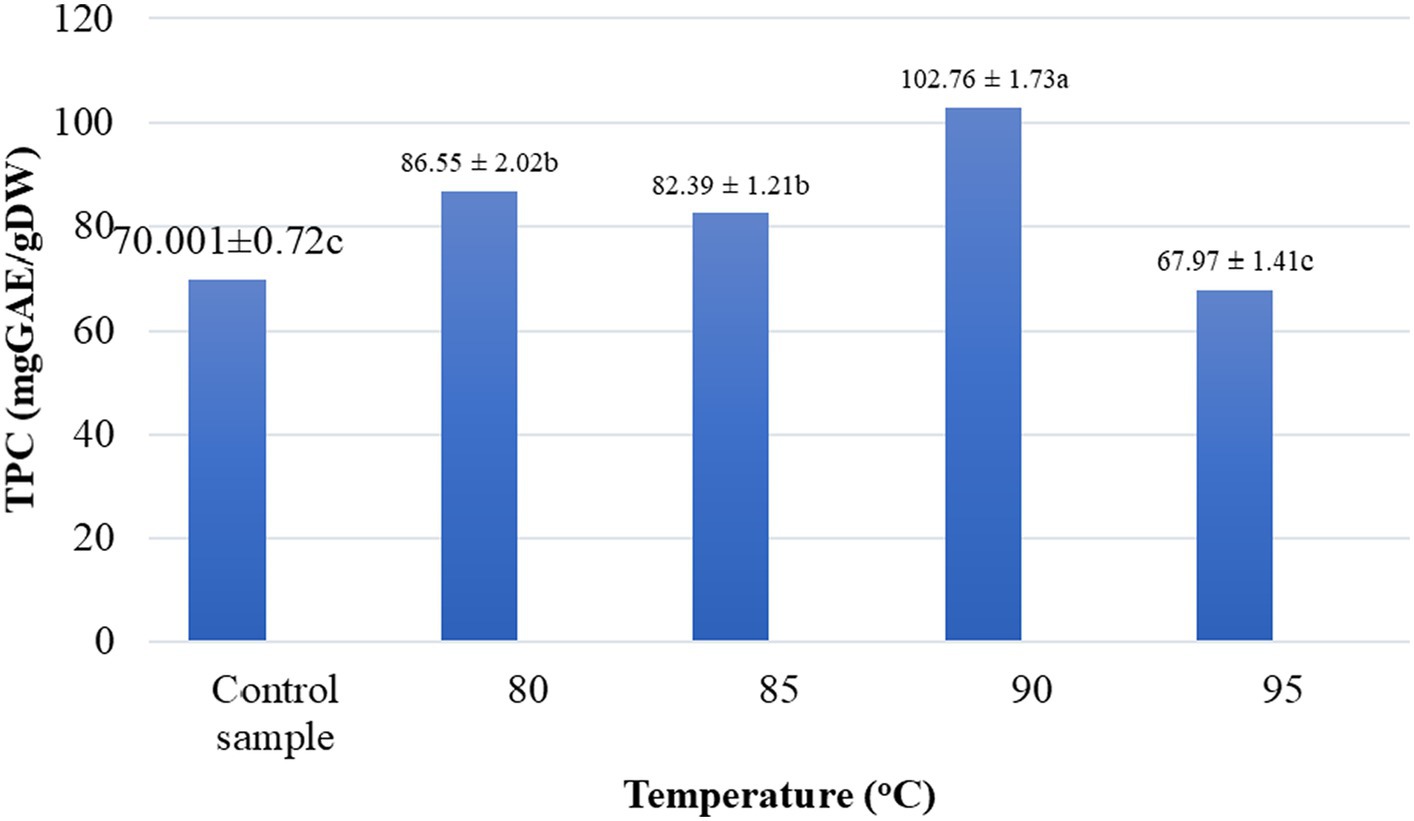
Figure 4. Effect of temperature on TPC during blanching. *(a-d) indicates a statistically significant difference (p-value<0.05).
The results of ANOVA analysis showed that TPC significantly affected the blanching temperature when increasing from 80–95°C at 95% confidence level (p < 0.05). Observing Figure 4, when the temperature increased from 80°C to 90°C, the total polyphenol content tended to increase. However, the temperature continued to rise to 95°C, and TPC tended to decrease to the lowest level (67.97 ± 1. 41 mgGAE/gDW), which was equivalent to the value obtained in fresh samples. Specifically, TPC values at 80°C and 85°C were obtained as 86.55 ± 2.02 mgGAE/gDW and 82.39 ± 1.21 mgGAE/gDW, respectively. At a blanching temperature of 90°C, the obtained TPC value was the highest with 102.76 ± 1.72 mgGAE/gDW. When blanching at higher temperatures, the faster diffusion of water molecules into the mango slices helped to retain the highest TPC at 90°C (Heras-Ramírez et al., 2012). The polyphenol compounds were degraded by heat (Abu-ghannam and Crowley, 2006; Gonçalves et al., 2010). Menon et al. (2015) showed that, at high temperatures, the TPC could be degraded or washed away. However, blanching with water at high temperatures minimizes the reduction of polyphenols in mango slices.
3.1.3 Effect of time on quality of mango slices during blanching
The process of blanching is significantly influenced by time. The mango slices’ structure, color, and polyphenol content will change if the blanching process is prolonged. The experiment was therefore evaluated at four time intervals, including 3, 4, 5, and 6 min fixed at 90°C (Supplementary Figure S3), based on prior documents (Bamidele et al., 2017).
Table 3 shows the color change when blanching at different times. The color change of Tu Quy mango slices tended to increase gradually with the ∆E* value from 25.45 ± 1 to 45.1 ± 1.16 when increasing the blanching time from 3 to 6 min. The sample with 3 min blanching time gave a color almost the same as the fresh sample with the ∆ E* value of 25.45 ± 1. However, under the condition of 3 min, the PPO enzyme could not be inactivated (Figure 5). Therefore, 3 min was not selected in the blanching time experiment. Additionally, the 4 min sample (27.82 ± 0.21) kept the best color compared to the 5 min and 6 min samples with values of 43.31 ± 1.61 and 45.1 ± 1.16, respectively. At 90°C, when the blanching time was prolonged, the color of mango slices became darker and more discolored than fresh samples, which was similar to the study of Gonçalves et al. (2007).
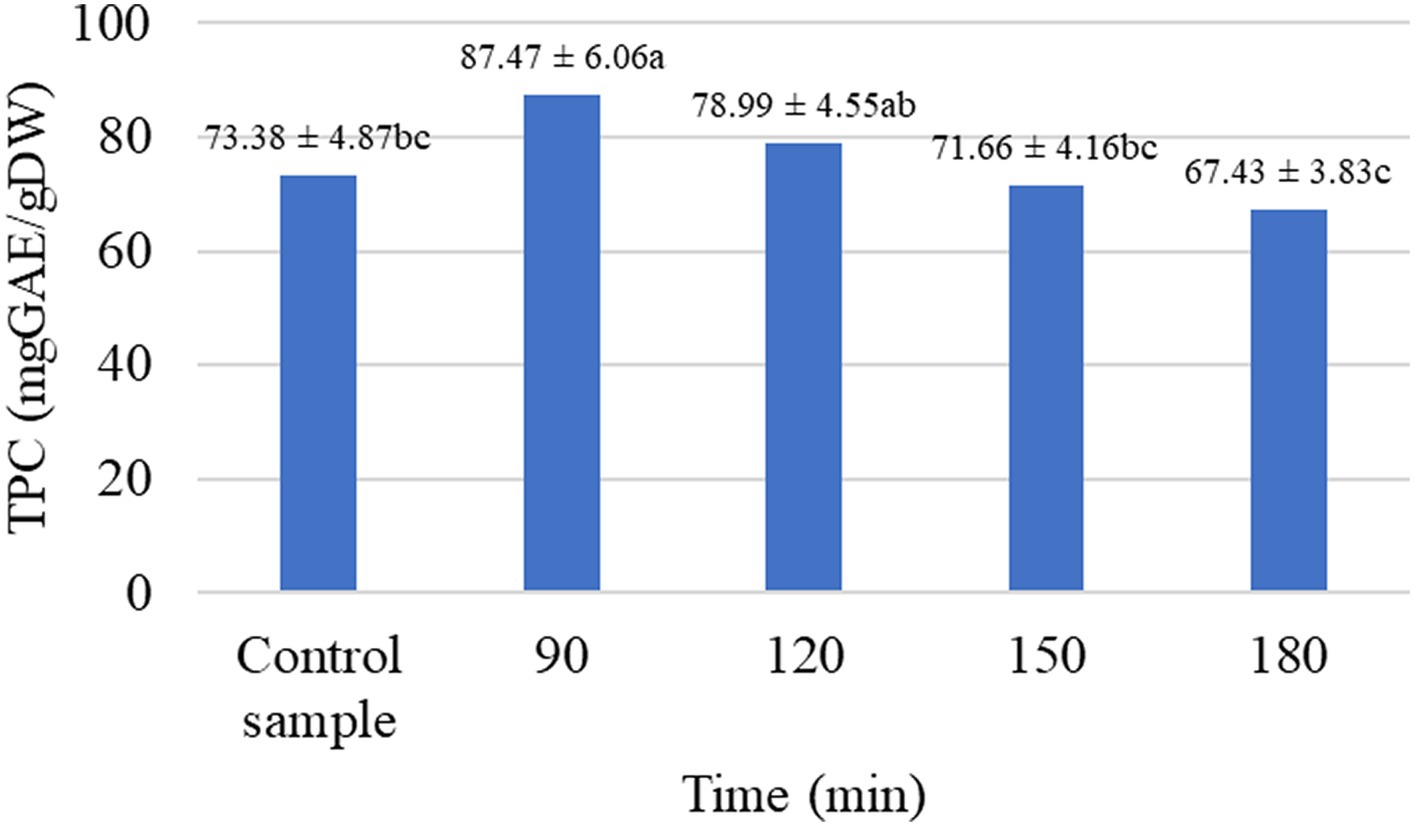
Figure 5. Effect of time on TPC during blanching. *(a-c) indicates a statistically significant difference (p-value<0.05).
Blanching time has a significant effect on product quality and PPO enzyme inactivation. Table 4 and Supplementary Table S3 show that as the time is prolonged, the ability to inactivate the enzyme is increased, specifically at 90°C for 3 to 6 min where the color changes from red brown to colorless. However, the short blanching time only partially inactivates the PPO enzyme, as shown by the reddish-brown color at 3 min. In contrast, blanching at 4, 5, and 6 min in the same conditions inactivated the PPO enzyme completely, as shown by the color disappearance. Overall, 4 min was also selected as it gives the highest color and TPC and inactivates the PPO enzyme.
The effect of blanching time on the TPC is shown in Figure 6. First, by increasing the blanching time from 3 to 4 min, the TPC increases from 74.25 ± 21.02 mgGAE/gDW to 91.33 ± 2.57 mgGAE/gDW. As the blanching time increased from 4 min to 5 min, TPC gradually decreased from 91.33 ± 2.57 mgGAE/gDW to 85.16 ± 2.17 mgGAE/gDW, which was similar at a blanching time of 6 min (85 ± 3.33 mgGAE/gDW). The reduced enzymatic breakdown of polyphenols after blanching may be the cause of the rise in TPC (complete inactivation of natural polyphenol oxidase). It can also be accounted for by the release of related phenolic acids from the degradation of plant cell wall constituents (Bamidele et al., 2017). Additionally, heat-sensitive active components such as polyphenols will degrade at the high temperatures produced during the prolonged blanching procedure (Thi Thanh Huong et al., 2022).
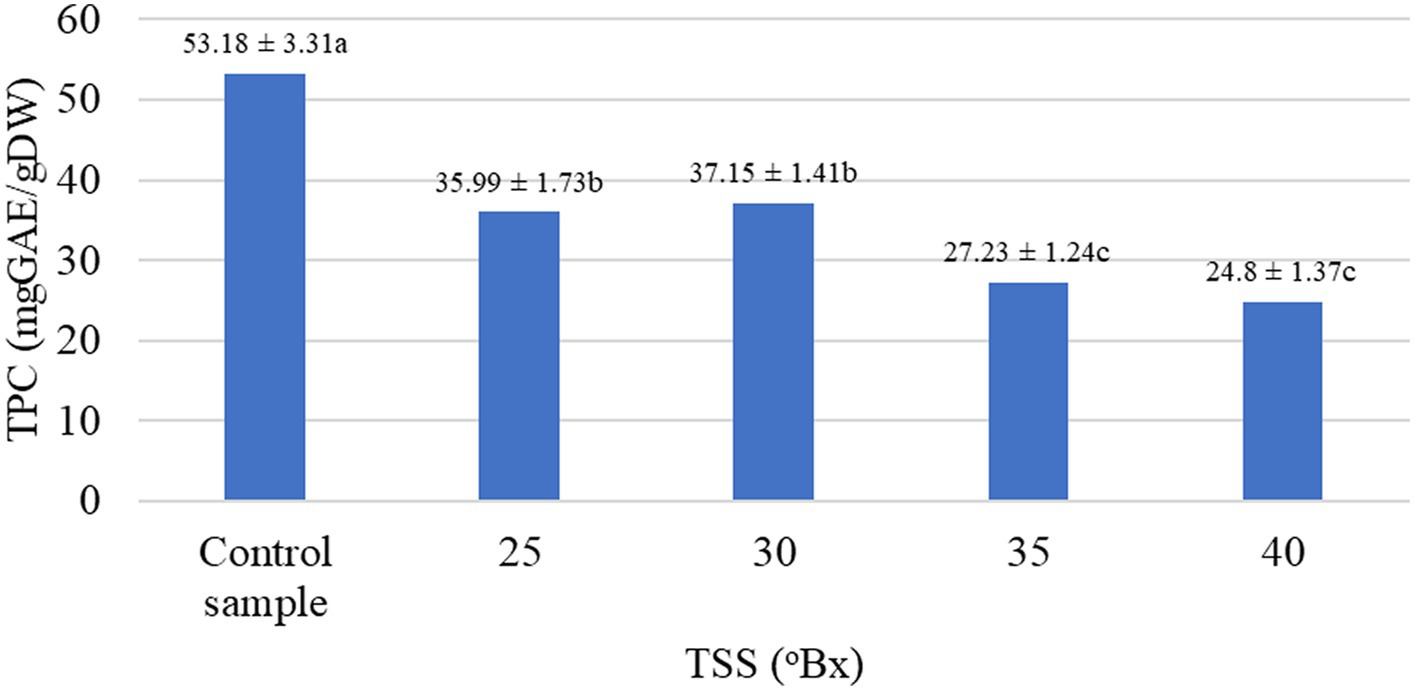
Figure 6. Effect of TSS on TPC during osmosis. *(a-d) indicates a statistically significant difference (p-value<0.05).
3.2 Evaluation of the effect of the osmosis process on the quality of Tu Quy mango slices
3.2.1 Effect of TSS on quality of mango slices during osmosis
The syrup solution plays a crucial role in the osmosis process and contributes to the final product’s flavor. Additionally, syrup helps to extend storage life by preventing microbial growth. As a result, it is vital to assess how syrup content affects the product quality and sensory perception. The total solute concentration in the syrup solution was investigated from 25°Bx to 40°Bx based on previous studies (Tabtiang et al., 2012). Observing Supplementary Figure S4, the color of mango slices was bright yellow with increasing concentrations of total dissolved solids. The lower limit (25°Bx) limits the darkening of mango slices, which is suitable for sensory evaluation. The upper limit (40°Bx) is applicable for the mango sample to have a nice color and slight sweet taste. Samples at 30°Bx and 35°Bx were reached in terms of sensory value.
The results of ANOVA analysis showed that TPC significantly affects the concentration of dissolved solids during osmosis at 95% confidence level (p < 0.05). As can be seen in Figure 7, TPC tends to decrease with increasing dissolved solids concentration from 25°Bx to 40°Bx. During osmosis of the samples at 25°Bx and 30°Bx, the TPC values reached their peak at 35.99 ± 1.73 mgGAE/gDW and 37.15 ± 1.41 mgGAE/gDW, respectively. These two samples had a lower TPC than fresh samples (32.32%). In contrast, TPC was the lowest at a total dissolved solids concentration of 40°Bx (24.8 ± 1.37 mgGAE/gDW), which was similar at 35°Bx (27.23 ± 1.24 mgGAE/gDW). According to Chavan, U. and R. Amarowicz, an increase in total dissolved solids concentration leads to a rise in the dehydration effect (Chavan and Amarowicz, 2022). Furthermore, the migration of water from mango slices to the syrup medium also carried polyphenol compounds. Thus, increasing the total solute concentration decreased TPC, which was consistent with the report by Grzelak-Błaszczyk et al. (2020). The permeation samples at 25°Bx and 30°Bx produce the highest TPC value. However, mango slices osmotically treated at 30°Bx exhibited a more consistent yellow color, along with a balanced sweet and sour taste, compared to those at 25°Bx. Therefore, the total solute concentration present in the 30°Bx immersion solution was selected as the fixative factor to conduct the following experiment.
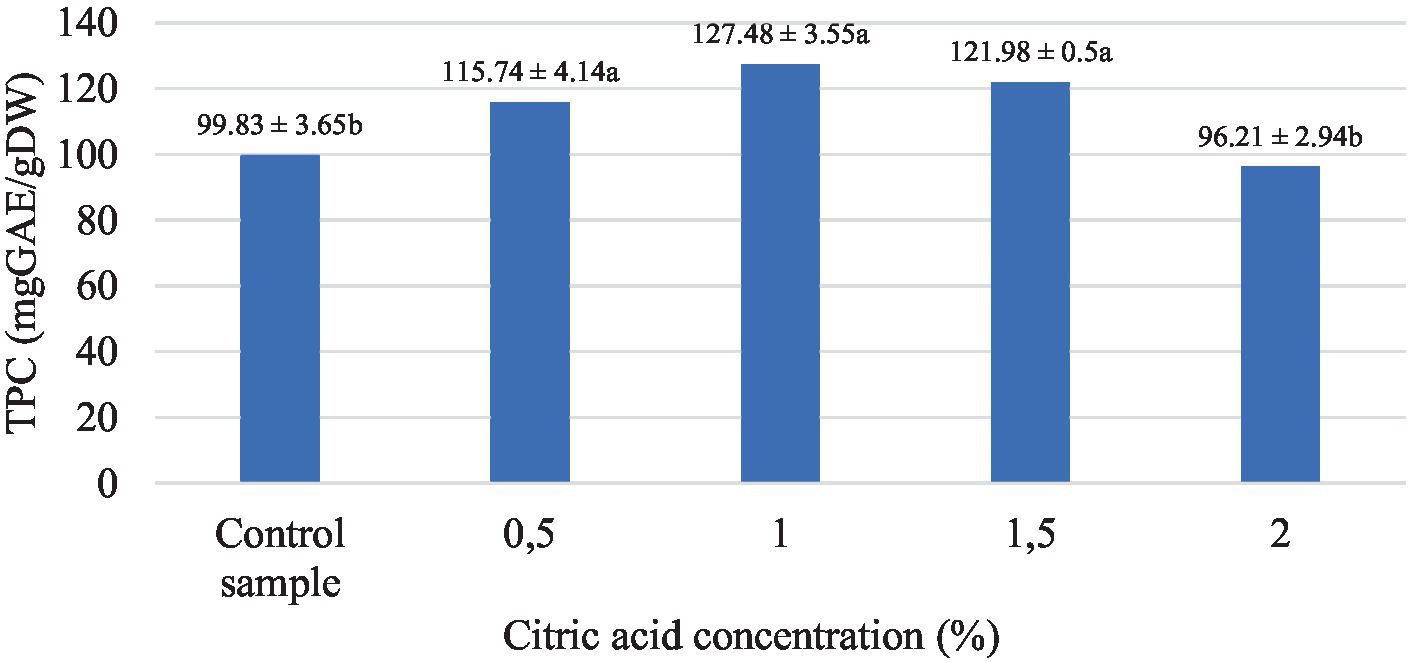
Figure 7. Effect of citric acid on TPC during osmosis of mango slices. *(a-d) indicates a statistically significant difference (p-value<0.05).
3.2.2 Effect of citric acid concentration on mango slices quality during osmosis
Citric acid is an additive used for flavoring products. It can be easily exchanged and excreted from the human body. Therefore, it is necessary to evaluate the effect of citric acid concentration on sensory and product quality. The survey range was performed at citric acid concentrations from 0.5 to 2% based on previous studies (Cảnh, 2012). As shown in Supplementary Figure S5, when increasing the concentration of citric acid, the yellow color of the mango slices was brighter and the hardness was better. At the concentration of 0.5% citric acid, mango slices had a mildly sour taste and uneven color. The 2% citric acid concentration made the mango slice taste sour and reduced the characteristic odor. Indeed, the citric acid concentrations of 1 and 1.5% are most suitable in terms of organoleptic evaluation.
The effect of citric acid concentration on TPC during osmosis is shown in Figure 5. When increasing the concentration of citric acid concentration from 0.5 to 1.5%, TPC increased by 128% higher than the control sample. However, when the citric acid concentration increased to 2%, the TPC tended to decrease to 96.21 ± 2.94 mgGAE/gDW, which was relatively equal to the control sample. This result is consistent with the report of Aliakbarian et al. (2009), which showed that TPC value decreased with increasing citric acid concentration. Figure 5 demonstrates that the TPC value at three concentrations of citric acid from 0.5 to 1.5% was not statistically different. At the same time, in terms of organoleptic, the sample had a high concentration of citric acid. Therefore, the concentration of 1% citric acid as the most optimal choice, exhibiting a vibrant yellow hue, subtly tangy taste, and retaining the distinctive essence of mango compared to the other samples. Thus, the concentration of 1% citric acid was selected to investigate the subsequent experiments.
3.2.3 Effect of glycerol concentration on mango slices quality during osmosis
Glycerol is colorless, odorless, non-toxic, soluble in water and some solvents, and has a specific sweet taste. It can absorb and retain moisture, dissolve flavorings, and has plasticity. Glycerol is added as an additive to increase the plasticity of dried mango products. In this study, the concentration of glycerol from 0.1 to 0.4% was selected to investigate the influence of this additive on the quality of mango slices during the osmosis process. The color and texture of mango slices increased with increasing glycerol concentration (Supplementary Figure S6). When the glycerol concentration increased from 0.2% to 0.4%, the mango slices exhibited a visually appealing, soft, and pliable texture, enhancing their sensory appeal. Conversely, lower glycerol concentrations (0.1%) yielded contrasting results, with mango slices displaying a darker color and inadequate flexibility, detracting from their overall desirability.
As shown in Figure 8, the concentration of glycerol affects the TPC. The glycerol concentration was investigated at four levels of 0.1–0.4%, showing an opposite trend with the TPC. When glycerol was increased from 0.1 to 0.4%, the TPC decreased from 108.3 ± 5.66 mgGAE/gDW to 69.27 ± 2.98 mgGAE/gDW. Additionally, glycerol has solvent properties for breaking down cells to release soluble proteins. Meanwhile, polyphenol compounds and proteins combine to form soluble, colloidal-sized complexes. Therefore, when the concentration of glycerol increases, more protein is released, leading to a decrease in polyphenols (Cassano et al., 2017). The TPC in the permeabilized sample with 0.1% glycerol concentration can be seen with the highest value. However, organoleptic samples with glycerol concentrations of 0.2 and 0.3% had better color, taste, and plasticity than the mango slices, which matched the organoleptic taste. Therefore, with a higher retention of TPC, the 0.2% glycerol sample was used to evaluate further experiments.
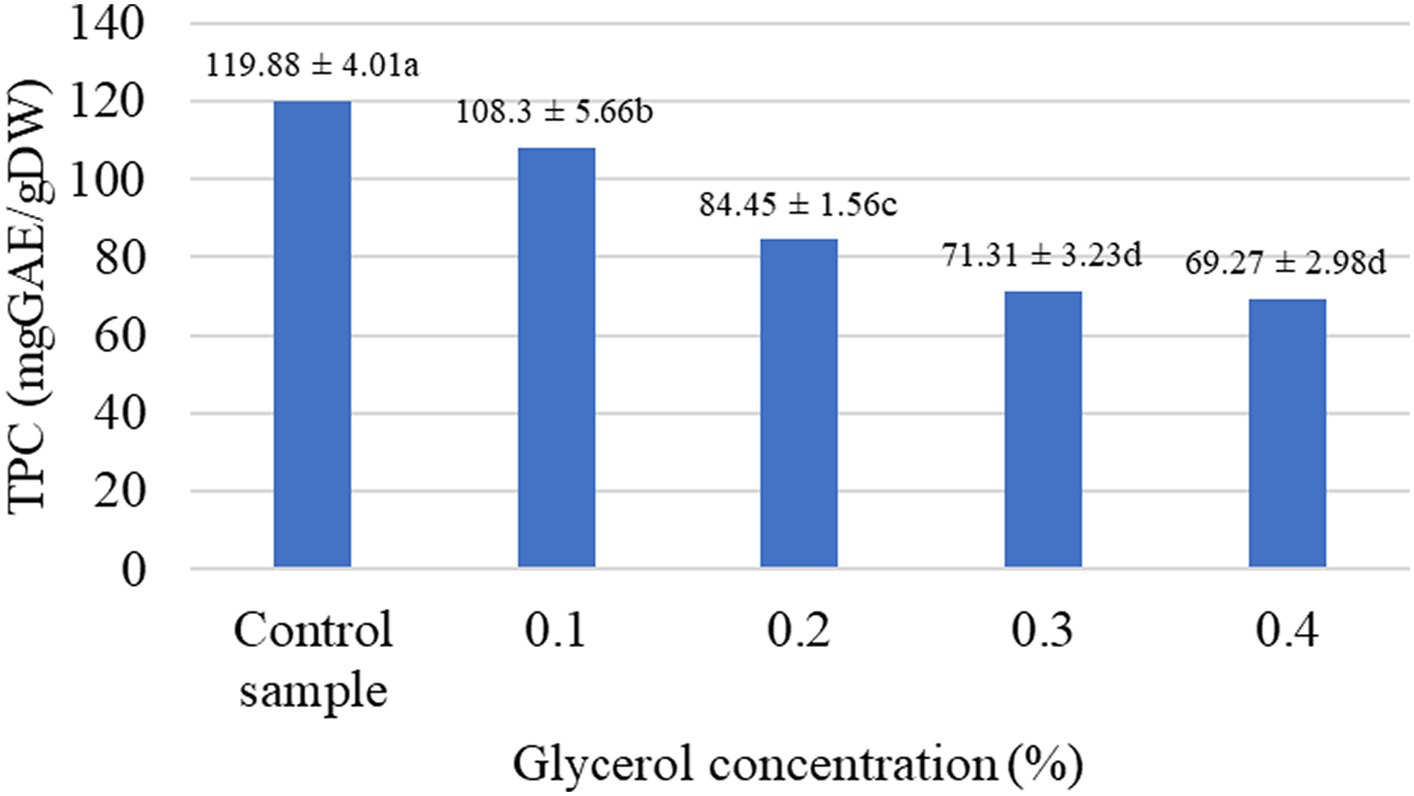
Figure 8. Effect of glycerol concentration on TPC during osmosis of mango slices. *(a-d) indicates a statistically significant difference (p-value<0.05).
3.2.4 Effect of temperature on mango slices quality during osmosis
Temperature is one of the main factors that affects the quality of mango slices during osmosis. Increasing the temperature reduces the viscosity of the osmotic solution leading to increased diffusion of water (Almeida et al., 2015). Based on previous studies, this experiment was investigated at four different temperatures: 35, 45, 55, and 65°C (Dao et al., 2022). At 35°C, the color of mango slices was not uniform, and the taste was mildly sweet (Supplementary Figure S8). On the contrary, mango slices were dark and uneven at the highest temperature of 65°C, leading to ripe samples’ soft texture that was unsuitable for organoleptic taste. Indeed, a temperature of 45–55°C produced mango slices with uniform color, moderate sweetness, and structure appropriate for organoleptic.
The results of ANOVA analysis showed that TPC had a significant effect on temperature during osmosis when increasing by 35–65°C, 95% confidence level (p < 0.05). As shown in Figure 9A, TPC tends to decrease with increasing osmotic temperature. When permeabilizing the sample at 35°C, the TPC was the highest value of 77.55 ± 2.81 mgGAE/gDW. This factor decreased by 13.20% compared to the control sample. When increasing the temperature from 35 to 45°C, TPC decreased from 77.55 ± 2.81 mgGAE/gDW to 66.68 ± 2.29 mgGAE/gDW. At the osmotic temperatures of 45–65°C, TPC did not change when analyzing its variance. This result is consistent with the report of Devic et al. (2010), that increasing the osmotic temperature will significantly reduce TPC. As the osmotic temperature increases, the intercellular distance also increases, leading to a faster osmosis rate and environmental TPC loss (Devic et al., 2010). Therefore, the temperature of 35°C gives the highest TPC content. However, in terms of sensory perception, the sample with a temperature of 45°C is the most suitable compared to the remaining mango slices because of its bright yellow color and uniform color, keeping the structure of mango slices. For this reason, the temperature of 45°C was chosen to investigate the following experiments.
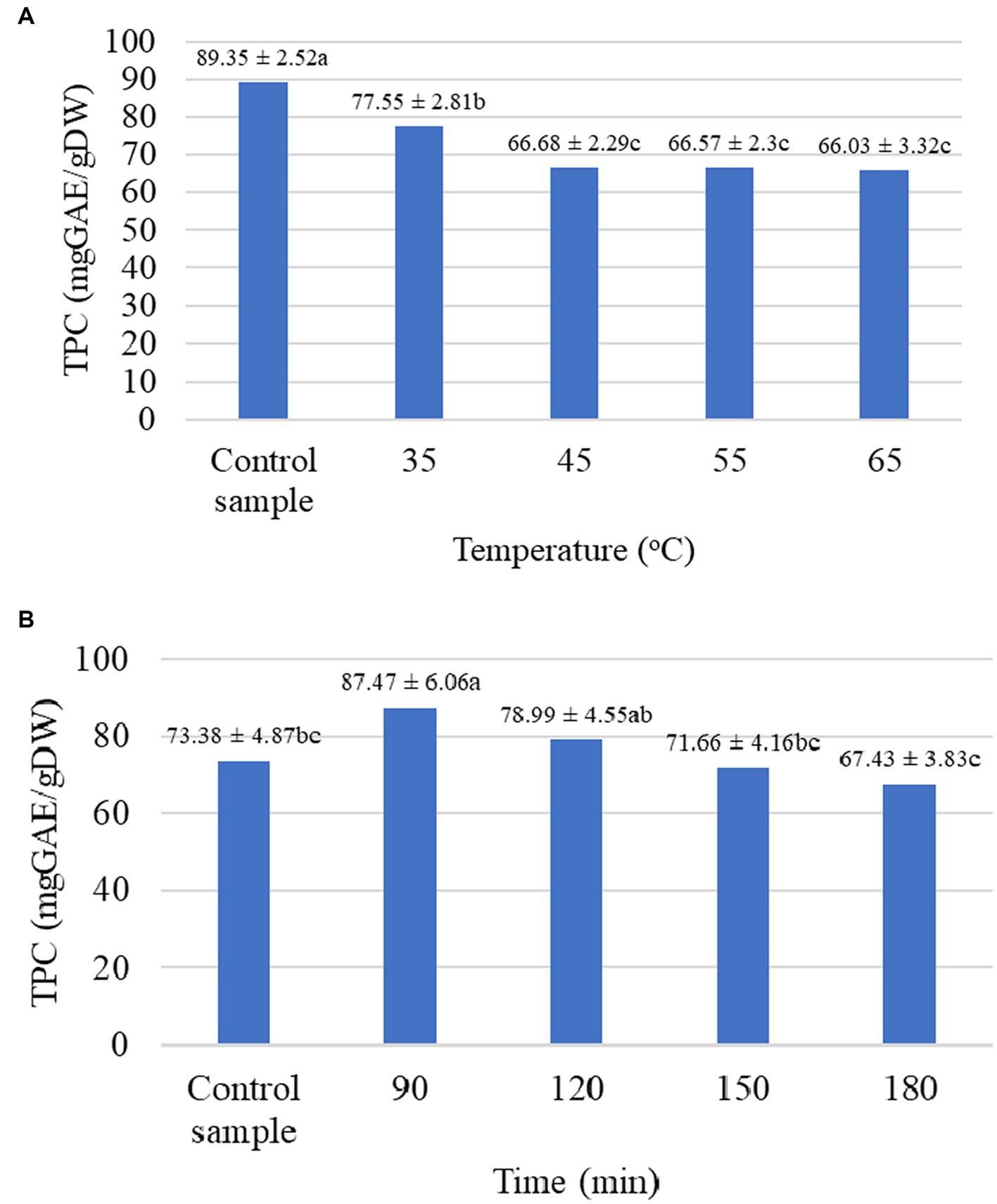
Figure 9. Effect of temperature and time on TPC during osmosis. *(a-c) indicates a statistically significant difference (p-value<0.05).
3.2.5 Effect of time on mango slices quality during osmosis
Osmosis time is a layer influencing factor on the chemical composition and quality of mango slices after soaking. This study investigated the effect of osmosis time on total polyphenol content from 90 min to 180 min (Dao et al., 2022). Observing Figure 9B, at 90 min, the color of the mango slices is uneven; the taste is mild. On the contrary, when subjected to a 90-minute osmotic process and unstable due to the short time leading to the inability to penetrate the inside of the mango slices with a light sweetness. Indeed, the time of 120–180 min for mango slices produced a uniform color, moderate sweetness, and suitable structure for the senses.
3.3 Evaluation of the effect of drying on the quality of Tu Quy mango slices
3.3.1 Effect of drying temperature on product quality
In this study, the mango slices’ heat pump drying process was carried out at 20°C, 30°C, 40°C, and 50°C. The initial moisture content of the mango fruit (dry weight) was 76.87 ± 0.30%. Figure 10A depicts the relationship between the moisture content of mango slices at various points during the drying process and under different drying temperature conditions. Figure 10A shows that the drainage rate increases with temperature and that the sample dries more quickly. The mango slice’s initial dry basis was decreased to a fixed value of 0. The varied drying times employed in the study were found to be 720 min at 50°C, 810 min at 40°C, 1,080 min at 30°C, and 1,455 min at 20°C under these circumstances. At 20°C, drying takes 2.02 times longer than at 50°C. The findings indicate that the drying temperature is the primary determinant of drying time. Higher drying temperatures requiring more heat with large airflow can shorten drying time (Zheng et al., 2011). The results indicate that a higher air-drying temperature leads to a more significant curve slope and a shorter drying process. There are two possible explanations for this. Firstly, as the moisture removal from the material occurs parallel to the diffusion of moisture from the center to the material’s surface and from the surface to the environment, the higher dry temperature in the air reduces the moisture content relative to the surface of the material, thus promoting surface evaporation during drying. Secondly, as air-dry temperatures rise, temperature gradients and surface evaporation rates also improve, speeding up the diffusion of moisture from the center to the surface. These findings are in agreement with another study, which found that the shorter drying time was caused by a rise in the air’s drying temperature (Chong et al., 2008).
The effect of drying on TPC in dried mango samples was observed during drying to constant mass (Figure 10B). From Figure 10B, in the range of 20–50°C, the process terminates faster with increasing temperature. However, TPC was significantly lost as these compounds are easily oxidized under long-term hot air, causing the water in the sample to escape quickly and reduce the nutritional content of substances in materials such as TPC. In the 26th cycle, corresponding to 750 minutes of drying, the TPC of mango slices subjected to temperatures of 20°C, 30°C, 40°C, and 50°C was recorded as 21.19, 24.53, 12.52, and 12.82 mgGAE/gDW, respectively. TPC values obtained at lower drying temperatures (20 and 30°C) were almost twice as significant than at 40 and 50°C. The different effects of drying on the retention or loss of polyphenol compounds are due to the further stability of polyphenol compounds under each drying condition. Based on Figure 10B, at 30°C, the highest TPC recovery is obtained and drying time is shortened, which determines the energy cost of the process.
3.3.2 Effect of the wind speed of the dryer on product quality
The wind speed is considered one of the factors that affect the efficiency and quality of the drying process, and the drying process was performed at 40–55 Hz. The change in moisture content during the drying process is shown in Figure 11. Results showed that the moisture content of mangoes during drying decreases gradually as the wind speed increases. The original mango which initially had a material moisture of 72.95%, reduced to 4.53% after drying at a wind speed of 40 Hz. The mangoes dried with a wind speed of 55 Hz had a moisture content of 1.58%, which was 2.95% lower than the dried mangoes at a wind speed of 40 Hz.
The variation of total polyphenol content is shown in the Figure 11. Research results showed that with wind speeds of 40–50 Hz, Polyphenol content is 8.92–29.82 mgGAE/g dry matter. In general, the TPC in mango was significantly less changed when drying at high wind speed. Specifically, with the initial range of mango polyphenols with a content of 8.92–29.82 mgGAE/g dry weight after drying with a wind speed of 50 Hz, the mango product with the lowest content changed with a value 29.82 mgGAE/g dry weight.
The increasing TPC value of mango slices during drying with time was similar to the study of Bamidele et al. (2017); the increase in TPC during drying may be due to decreased polyphenol-mediated degradation enzymes and complete inactivation of natural polyphenol oxidase. It can also be explained that as the cellular components of plant cell walls break down, there is a release of bound phenolic acids, contributing to an increase in the content of polyphenols obtained. This effect is more pronounced with prolonged processing (Bamidele et al., 2017). Additionally, the high temperatures generated during the prolonged blanching process decompose the heat-sensitive bioactive compounds and reduce polyphenol content, Specifically, at a wind speed of 55 Hz, there was a lower content of polyphenol compounds compared to those obtained at 45 and 50 Hz (Thi Thanh Huong et al., 2022).
4 Conclusion
The current investigation presents the Tu Quy Mango drying process data. Blanching was carried out with a sample size of 10 mm, blanching temperature of 90°C for 4 min for a bright color, and maximum ability to inactivate PPO enzyme and retained polyphenol content. In addition, the permeation process was carried out at 30oBx, 1% citric acid, 0.2% glycerol, and at 45°C for 150 min for the best product appearance, and the samples were dried at 30°C for 1,080 min with desirable product moisture content of 13–16% with total polyphenol content obtained at 12.17 mgGAE/gDW. The research aims to refine the dried mango production process using locally sourced mangoes from a specialty region in Vietnam. This endeavor contributes to enhancing the value of the local agricultural sector.
Data availability statement
The original contributions presented in the study are included in the article/Supplementary material, further inquiries can be directed to the corresponding authors.
Author contributions
BT: investigation, methodology, formal analysis, data curation, and writing – original draft. PT: investigation and methodology. NL: investigation and methodology. TTra: investigation, methodology, and formal analysis. NN: investigation and methodology. TP: investigation and methodology. TTru: methodology and formal analysis. LG: conceptualization, funding acquisition, and methodology. TD: conceptualization, data curation, funding acquisition, investigation, methodology, writing – original draft, and writing – review & editing. All authors contributed to the article and approved the submitted version.
Funding
The author(s) declare financial support was received for the research, authorship, and/or publication of this article. This study was supported by the Department of Science and Technology Ben Tre, Vietnam, and Nguyen Tat Thanh University, Ho Chi Minh City, Vietnam.
Conflict of interest
The authors declare that the research was conducted in the absence of any commercial or financial relationships that could be construed as a potential conflict of interest.
Publisher’s note
All claims expressed in this article are solely those of the authors and do not necessarily represent those of their affiliated organizations, or those of the publisher, the editors and the reviewers. Any product that may be evaluated in this article, or claim that may be made by its manufacturer, is not guaranteed or endorsed by the publisher.
Supplementary material
The Supplementary material for this article can be found online at: https://www.frontiersin.org/articles/10.3389/fsufs.2024.1204303/full#supplementary-material
References
Abu-ghannam, N., and Crowley, H. (2006). The effect of low temperature blanching on the texture of whole processed new potatoes. J. Food Eng. 74, 335–344. doi: 10.1016/j.jfoodeng.2005.03.025
Ahmad, N., Hussain, A., Khan, S., Korma, S. A., Hussain, G., Aadil, R. M., et al. (2021). Impact of thermal extrusion and microwave vacuum drying on fatty acids profile during fish powder preparation. Food Sci. Nutr. 9, 2743–2753. doi: 10.1002/fsn3.2236
Aliakbarian, B., Dehghani, F., and Perego, P. (2009). The effect of citric acid on the phenolic contents of olive oil. Food Chem. 116, 617–623. doi: 10.1016/j.foodchem.2009.02.077
Almeida, J. A. R., Mussi, L., Oliveira, D., and Pereira, N. R. (2015). Effect of temperature and sucrose concentration on the retention of polyphenol compounds and antioxidant activity of osmotically dehydrated bananas: bioactive compounds in the osmotic dehydration. J. Food Proc. Preservat. 39, 1061–1069. doi: 10.1111/jfpp.12321
Bamidele, O., Fasogbon, M. B., Adebowale, O. J., and Adeyanju, A. A. (2017). Effect of blanching time on Total phenolic, antioxidant activities and mineral content of selected green leafy vegetables. Curr. J. Appl. Sci. Technol. 24, 1–8. doi: 10.9734/CJAST/2017/34808
Cassano, A., De Luca, G., Conidi, C., and Drioli, E. (2017). Effect of polyphenols-membrane interactions on the performance of membrane-based processes. A review. Coord. Chem. Rev. 351, 45–75. doi: 10.1016/j.ccr.2017.06.013
Chavan, U., and Amarowicz, V. R. (2022). Osmotic dehydration process for preservation of fruits and vegetables. J. Food Res. 1. doi: 10.5539/jfr.v1n2p202
Chong, C. H., Law, C. L., Cloke, M., Hii, C., Chuah, A. L., and Daud, W. R. W. (2008). Drying kinetics and product quality of dried Chempedak. J. Food Eng. 88, 522–527. doi: 10.1016/j.jfoodeng.2008.03.013
Dao, T. P., Nguyen, D. V., Tran, T. Y. N., Pham, T. N., Nguyen, P. T. N., Bach, L. G., et al. (2021). Effects of tannin, ascorbic acid, and total phenolic contents of cashew (Anacardium occidentale L.) apples blanched with saline solution. Food Res. 5, 409–416. doi: 10.26656/FR.2017.5(1).454
Dao, T. P., Vu, D. N., Nguyen, D. V., Pham, V. T., and Tran, T. Y. N. (2022). Study of jelly drying cashew apples (Anacardium occidentale L.) processing. Food Sci. Nutr. 10, 363–373. doi: 10.1002/fsn3.2565
Devic, E., Guyot, S., Daudin, J. D., and Bonazzi, C. (2010). Effect of temperature and cultivar on polyphenol retention and mass transfer during osmotic dehydration of apples. J. Agric. Food Chem. 58, 606–614. doi: 10.1021/jf903006g
Gang, K.-Q., Wu, Z.-X., Zhou, D.-Y., Zhao, Q., Zhou, X., Lv, D.-D., et al. (2019). Effects of hot air drying process on lipid quality of whelks Neptunea arthritica cumingi Crosse and Neverita didyma. J. Food Sci. Technol. 56, 4166–4176. doi: 10.1007/s13197-019-03887-3
Gonçalves, E. M., Pinheiro, J., Abreu, M., Brandão, T., and Silva, C. L. M. (2010). Carrot (Daucus Carota L.) peroxidase inactivation, phenolic content and physical changes kinetics due to blanching. J. Food Eng. 97, 574–581. doi: 10.1016/j.jfoodeng.2009.12.005
Gonçalves, E. M., Pinheiro, J., Abreu, M., TRS, B., and CLM, S. (2007). Modelling the kinetics of peroxidase inactivation, colour and texture changes of pumpkin (Cucurbita Maxima L.) during blanching. J. Food Eng. 81, 693–701. doi: 10.1016/j.jfoodeng.2007.01.011
Grzelak-Błaszczyk, K., Czarnecki, A., and Klewicki, R. (2020). The effect of osmotic dehydration on the polyphenols content in onion. Acta Sci. Pol. Technol. Aliment. 19, 37–45. doi: 10.17306/J.AFS.0766
Heras-Ramírez, M. E., Quintero-Ramos, A., Camacho-Dávila, A. A., Barnard, J., Talamás-Abbud, R., Torres-Muñoz, J. V., et al. (2012). Effect of blanching and drying temperature on polyphenolic compound stability and antioxidant capacity of apple pomace. Food Bioprocess Technol. 5, 2201–2210. doi: 10.1007/s11947-011-0583-x
Jahurul, M. H. A., Zaidul, I. S. M., Ghafoor, K., Al-Juhaimi, F. Y., Nyam, K. L., Norulaini, N. A. N., et al. (2015). Mango (Mangifera Indica L.) by-products and their valuable components: a review. Food Chem. 183, 173–180. doi: 10.1016/j.foodchem.2015.03.046
Jangam, S. V., and Mujumdar, A. S. (2014). “Heat pump assisted drying technology—overview with focus on energy, environment and product quality” in Modern drying technology. eds. T. E. Tsotsas and A. S. Mujumdar (Wiley-VCH Verlag GmbH & Co. KGaA), 121–162.
Maldonado-Celis, M. E., Yahia, E. M., Bedoya, R., Landázuri, P., Loango, N., Aguillón, J., et al. (2019). Chemical composition of mango (Mangifera indica L.) fruit: nutritional and phytochemical compounds. Front. Plant Sci. 10:1073. doi: 10.3389/fpls.2019.01073
Menon, A. S., Hii, C. L., Law, C. L., Suzannah, S., and Djaeni, M. (2015). Effects of water blanching on polyphenol reaction kinetics and quality of cocoa beans. AIP Conf. Proc 1699:030006. doi: 10.1063/1.4938291
Minea, V. (2015). Overview of heat-pump–assisted drying systems, part ii: data provided vs results reported. Drying Technol. 33, 527–540. doi: 10.1080/07373937.2014.952378
Nguyen, D. V., Dao, T. P., Pham, T. N., Tran, T. Y. N., Nguyen, V. H., Trinh, K. D., et al. (2021). Kinetic of polyphenol losses during convection drying of cashew (Anacardium occidentale L.) apples. Food Res. 5, 342–349. doi: 10.26656/fr.2017.5(4).048
Nguyen, N. Q., Pham, T. N., Nguyen, V. T., Nguyen, M. T., Dung, L. T., and Bach, L. G. (2020). Phytochemical screening and antioxidant potential of crude drug “cao khai” in Ninh Thuan province, Vietnam. IOP Conf. Ser. Mater. Sci. Eng. 991:012016. doi: 10.1088/1757-899X/991/1/012016
Nhut, P. T., Quyen, N. T. N., Truc, T. T., Minh, L. V., An, T. N. T., and Anh, N. H. T. (2020). Preliminary study on phytochemical, phenolic content, flavonoids and antioxidant activity of Coriandrum Sativum l. originating in Vietnam. IOP Conf. Ser. Mater. Sci. Eng. 991:012022. doi: 10.1088/1757-899X/991/1/012022
Nyangena, I. O., Owino, W. O., Imathiu, S., and Ambuko, J. (2019). Effect of pretreatments prior to drying on antioxidant properties of dried mango slices. Sci. Afr. 6:e00148. doi: 10.1016/j.sciaf.2019.e00148
Osunde, Z. D. (2017). Effect of pretreatments and drying methods on some qualities of dried mango (Mangifera indica) fruit. Agric. Eng. Int. CIGR J. 19, 187–194.
Palafox-Carlos, H., Yahia, E., Islas-Osuna, M. A., Gutierrez-Martinez, P., Robles-Sánchez, M., and González-Aguilar, G. A. (2012). Effect of ripeness stage of mango fruit (Mangifera Indica L., cv. Ataulfo) on physiological parameters and antioxidant activity. Sci. Hortic. 135, 7–13. doi: 10.1016/j.scienta.2011.11.027
Parvez, G. M. M. (2016). Pharmacological activities of mango (Mangifera Indica): a review. J. Pharmacogn. Phytochem. 5, 1–7.
Punathil, L., and Basak, T. (2016). “Microwave processing of frozen and packaged food materials: experimental” in Trong reference module in food science (tr B9780081005965210000) (Elsevier).
Shende, D., and Datta, A. K. (2020). Optimization study for refractance window drying process of Langra variety mango. J. Food Sci. Technol. 57, 683–692. doi: 10.1007/s13197-019-04101-0
Shivhare, U. S., Gupta, M., Basu, S., and Raghavan, G. S. V. (2009). Optimization of blanching process for carrots. J. Food Process Eng. 32, 587–605. doi: 10.1111/j.1745-4530.2007.00234.x
Tabtiang, S., Prachayawarakon, S., and Soponronnarit, S. (2012). Effects of osmotic treatment and superheated steam puffing temperature on drying characteristics and texture properties of Banana slices. Dry. Technol. 30, 20–28. doi: 10.1080/07373937.2011.613554
Thao, B. T. T., Vo, T. T. K., Tran, T. Y. N., Le, D. T., Tran, T. T., Bach, L. G., et al. (2023). Application of mathematical techniques to study the moisture loss kinetics and polyphenol degradation kinetics of mango (Mangifera indica L.) slices during heat pump drying by pilot equipment. LWT 176:114454. doi: 10.1016/j.lwt.2023.114454
Thi Thanh Huong, N., Thinh, P., Vinh Long, D., Bao Long, H., Thanh Dat, U., Tan Phat, D., et al. (2022). Effects of microwave and ultrasound treatment on vitamin C, polyphenols and antioxidant activity of mango (Mangifera indica) during low temperature drying. Mater. Today Proc. 59, 781–786. doi: 10.1016/j.matpr.2021.12.581
Tran, T. Y. N., Dang, T. T., Do, T. N., Pham, V. T., Do, V. L., Chung, T. P. T., et al. (2021). Optimization of the cold drying process of mango (Mangifera indica L.) Tu Quy variety by response surface methodology. Food Res. 5, 386–393. doi: 10.26656/fr.2017.5(4).138
Tran, N. Y. T., Le, T. D., Dao, P. T., Bach, G. L., Huynh, P. X., and Tran, Q. N. (2022). Evaluation of different extraction methods on the polyphenols yield, flavonoids yield, and antioxidant activity of the pomelo flavedo extract from Da Xanh (Citrus maxima [burm] merr.) variety. Food Sci. Technol. 42:e97021. doi: 10.1590/fst.97021
Udomkun, P., Romuli, S., Schock, S., Mahayothee, B., Sartas, M., Wossen, T., et al. (2020). Review of solar dryers for agricultural products in Asia and Africa: An innovation landscape approach. J. Environ. Manag. 268:110730. doi: 10.1016/j.jenvman.2020.110730
Wang, Y., Zhang, M., and Mujumdar, A. S. (2011). Trends in processing technologies for dried aquatic products. Dry. Technol. 29, 382–394. doi: 10.1080/07373937.2011.551624
Wojdyło, A., Figiel, A., Lech, K., Nowicka, P., and Oszmiański, J. (2014). Effect of convective and vacuum–microwave drying on the bioactive compounds, color, and antioxidant capacity of sour cherries. Food Bioprocess Technol. 7, 829–841. doi: 10.1007/s11947-013-1130-8
Xu, C., Yu, C., and Li, Y. (2015). Effect of blanching pretreatment on carrot texture attribute, rheological behavior, and cell structure during cooking process. LWT Food Sci. Technol. 62, 48–54. doi: 10.1016/j.lwt.2015.01.033
Zheng, D.-J., Cheng, Y.-Q., Liu, H.-J., and Li, L.-T. (2011). Investigation of EHD-enhanced water evaporation and a novel empirical model. Int. J. Food Eng. 7:7. doi: 10.2202/1556-3758.1796
Keywords: Mangifera india L., heat pump drying, blanching process, osmosis process, enzyme polyphenol oxidase
Citation: Thao BTT, Thanh Hung P, Lai ND, Tran TYN, Nguyen NQ, Pham TN, Truc Tran T, Giang Bach L and Dao TP (2024) Application of heat pump drying technology to produce dried mango products from Tu Quy mango (Mangifera india L.), Vietnam, on a pilot scale. Front. Sustain. Food Syst. 8:1204303. doi: 10.3389/fsufs.2024.1204303
Edited by:
Samuel Ayofemi Olalekan Adeyeye, Hindustan Institute of Technology and Science, IndiaReviewed by:
Prerna Gupta, Lovely Professional University, IndiaGanlin Chen, Guangxi Academy of Agricultural Sciences, China
Copyright © 2024 Thao, Thanh Hung, Lai, Tran, Nguyen, Pham, Truc Tran, Giang Bach and Dao. This is an open-access article distributed under the terms of the Creative Commons Attribution License (CC BY). The use, distribution or reproduction in other forums is permitted, provided the original author(s) and the copyright owner(s) are credited and that the original publication in this journal is cited, in accordance with accepted academic practice. No use, distribution or reproduction is permitted which does not comply with these terms.
*Correspondence: Long Giang Bach, YmxnaWFuZ0BudHQuZWR1LnZu; Tan Phat Dao, ZGFvcGhhdDE0N0BnbWFpbC5jb20=