- 1Department of Crop Science, College of Agricultural Science, São Paulo State University (UNESP), São Paulo, Brazil
- 2Department of Agronomy, São Paulo Western University (UNOEST), São Paulo, Brazil
- 3Brazilian Agricultural Research Corporation (Embrapa), Sete Lagoas, Minas Gerais, Brazil
Grass intercropping under no-till is an option to increase crop residues on the soil surface and crop diversity. Urochloa spp. is frequently selected for intercropping to improve land use and agricultural production because of its high residue production, slow residue decomposition, as well as its vigorous, abundant, and deep root system. However, the effects of intercropping Urochloa and maize, especially the effects of Urochloa residues, on subsequent crops in rotation have not been established. To address this knowledge gap, a field experiment was carried out over 5 years (from 2014 to 2018) comprising 2 years of maize monocropping or intercropping and 3 years of crop rotation (common bean-wheat-common bean-wheat-maize). We evaluated the medium-term effects of monocropped maize or maize intercropped with Urochloa brizantha on soil fertility and the development, yields, and grain nutrient accumulation of subsequent common bean, wheat, and maize crops. The cultivation of U. brizantha in the intercropping system improved soil fertility over at least 4 years, with increases in soil pH; soil organic matter (SOM); phosphorus (P); exchangeable potassium (K), calcium (Ca), and magnesium (Mg); sulfur (S–SO42−); cation exchange capacity (CEC); and base saturation (BS) at all soil depths. The benefits of U. brizantha extended to root dry matter and distribution; 70–77% of the total roots were concentrated within a soil depth of 0.0–0.2 m. The intercropping system improved the root dry matter mass, yield components, and grain yields of subsequent common bean, wheat, and maize crops in all cultivation years. These findings indicate that intercropping maize and U. brizantha provides medium-term benefits for subsequent common bean, wheat, and maize crops, and improves nutrient cycling to increase soil P; exchangeable K, Ca, and Mg; S–SO42−; and organic matter content.
1. Introduction
Improvements in agricultural ecosystem services are necessary to meet future food production demands (Archer et al., 2019). To realize such improvements, smarter agricultural practices that improve soil attributes and crop production without excessive fertilization or new expansions of cultivated areas will become increasingly important (Fung et al., 2019).
No-till management, which maintains crop residues on the soil surface, is considered the most important sustainable agricultural practice (Gristina et al., 2018). However, no-till management can lead to plant deficiencies, such as poor crop development and reduced yields, when the crop rotation includes crops with low biomass production or when a fallow period reduces residue production (Pittelkow et al., 2015). If the agricultural system is not adequately deployed, several benefits of no-till management, such as increased soil quality, may not be fully realized (Nunes et al., 2020). These limitations can negatively impact crop production by decreasing vegetative metabolism and crop yields, especially in tropical regions such as the Brazilian Cerrado and African savanna (Pariz et al., 2017b). In these regions, the dry autumn/winter period hinders residue production, and the hot, rainy spring/summer period accelerates the decomposition of residues on the soil surface (Pariz et al., 2017b; Dias et al., 2018; Portugal et al., 2020). Therefore, no-till systems should include diverse crops with greater potential for litter production.
Increasing the diversity of an agricultural system by cultivating different crop species enhances its resilience to abiotic factors (Archer et al., 2019). In intercropping systems, two or more crops are cultivated simultaneously to take advantage of the positive interactions between crops and to efficiently increase resource availability (Archer et al., 2019). Intercropping can increase soil fertility, reduce erosion, and increase soil carbon (C) sequestration without compromising land productivity (Daryanto et al., 2019), particularly when tropical forages that can be maintained after the cereal harvest (Crusciol et al., 2020), such as Urochloa spp. (Yé et al., 2017), are used.
Intercropping maize with forages such as Urochloa spp. is a great alternative for increasing crop residue production in no-till systems and for maintaining soil coverage and/or forage production for animal feed in integrated crop-livestock systems (Crusciol et al., 2021). Urochloa spp. produce a large amount of roots, which improve soil physical quality, reduce the risks associated with water limitation (Calonego et al., 2011), promote nutrient cycling to improve soil chemical quality (Rosolem et al., 2017). and improve soil biological activity (Momesso et al., 2022). Ultimately, intercropping with Urochloa spp. improves plant nutrition and grain yield (Tanaka et al., 2019).
Studies have shown that Urochloa brizantha cultivation improves the performance of the subsequent crop (Calonego et al., 2017; Tanaka et al., 2019; Momesso et al., 2021). We hypothesized that intercropping U. brizantha with maize for 2 years would improve soil fertility. Urochloa brizantha is usually cultivated on non-agricultural land, and we anticipated that U. brizantha cultivation would improve the developmental performance of system components, grain nutrient concentration, and the yields of crops grown in rotation (common bean-wheat-common bean-wheat-maize) during the period of intercropping and in subsequent years. To validate this hypothesis, we studied the effects of 2 years of intercropping of U. brizantha and maize on soil fertility and the root biomass distribution, nutritional status, grain nutrient accumulation, and yield of subsequent crops in a common bean-wheat (2 years) and maize (1 year) rotation.
2. Materials and methods
2.1. Site description
A field experiment was carried out in a stable no-till system in Botucatu, São Paulo, Brazil (48°26’ W; 22°51’ S; 740 m asl) from 2014 to 2018. The climate is classified as Cwa according to Köppen’s system and is tropical with dry winters and hot, rainy summers. The historical annual maximum and minimum temperatures in this region are 26.1 and 15.3°C, respectively, with an average temperature of 20.7°C. The annual average rainfall is 1,359 mm and is mostly concentrated between October and March. The rainfall and mean temperatures recorded over the growing seasons of the experiment are shown in Figure 1. The soil is classified as a clayey-textured Typic Haplorthox (Soil Survey Staff, 2014) containing 620, 90, and 290 g kg−1 of clay, silt, and sand, respectively. The area was managed for 5 years with no-tillage using the systems described in Figure 2. Before installing the experiment, the soil was sampled (0–0.05, 0.05–0.1, 0.1–0.2, 0.2–0.4, and 0.4–0.6 m depths) to evaluate the soil chemical characteristics according to Van Raij et al. (2001) (Table 1). Based on the results of the soil chemical analysis, soil acidity was ameliorated by applying 3.4 t ha−1 of limestone prior to implementation of the treatments, in which the dolomitic lime rate was calculated to increase the base saturation in the topsoil (0–0.20 m) to 70%, according to the methodology proposed by Van Raij et al. (1997) due to aluminum (Al) toxicity of acidic soils.
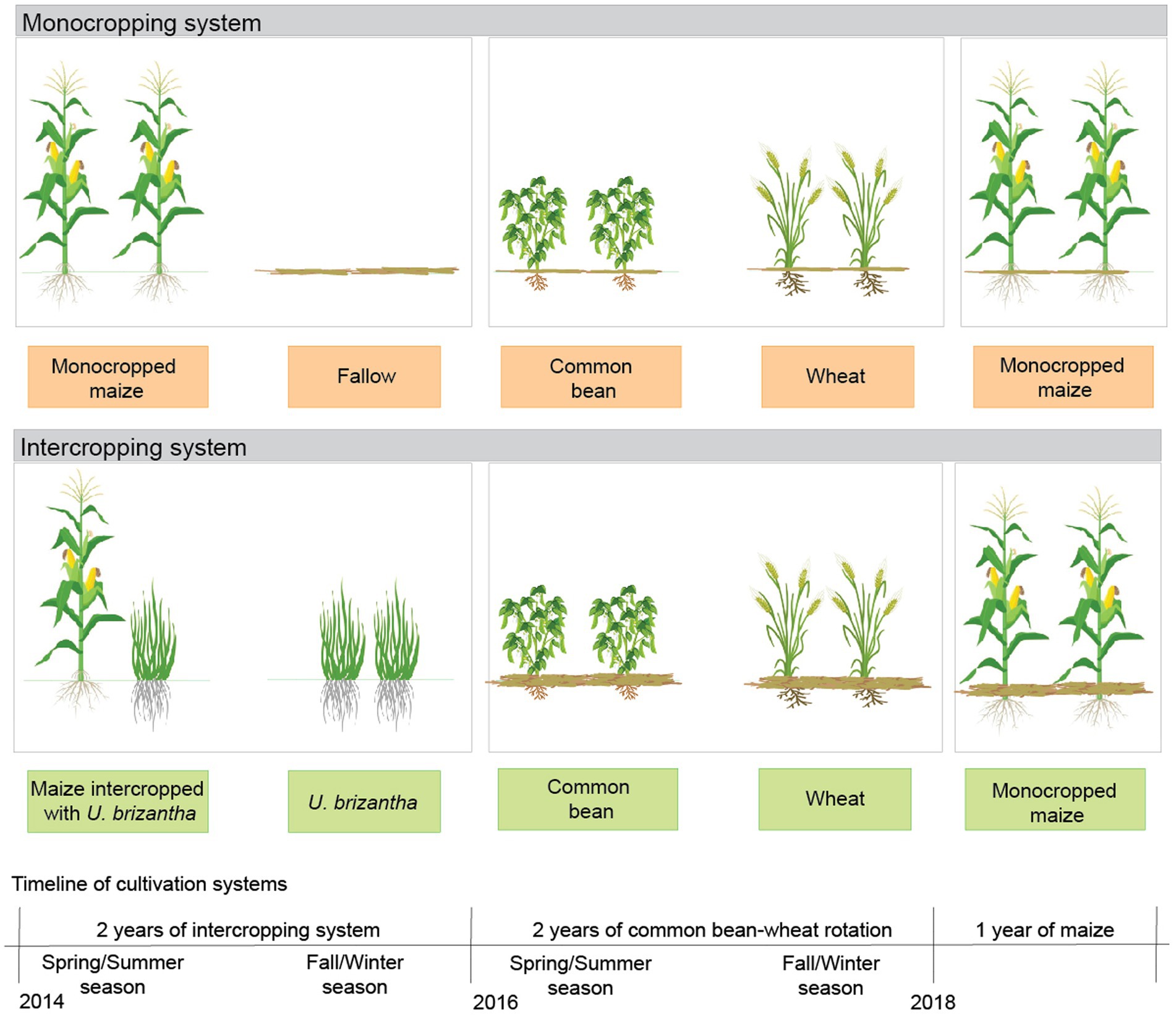
Figure 2. Scheme of cropping systems (monocropping and intercropping systems) and the experimental timeline.
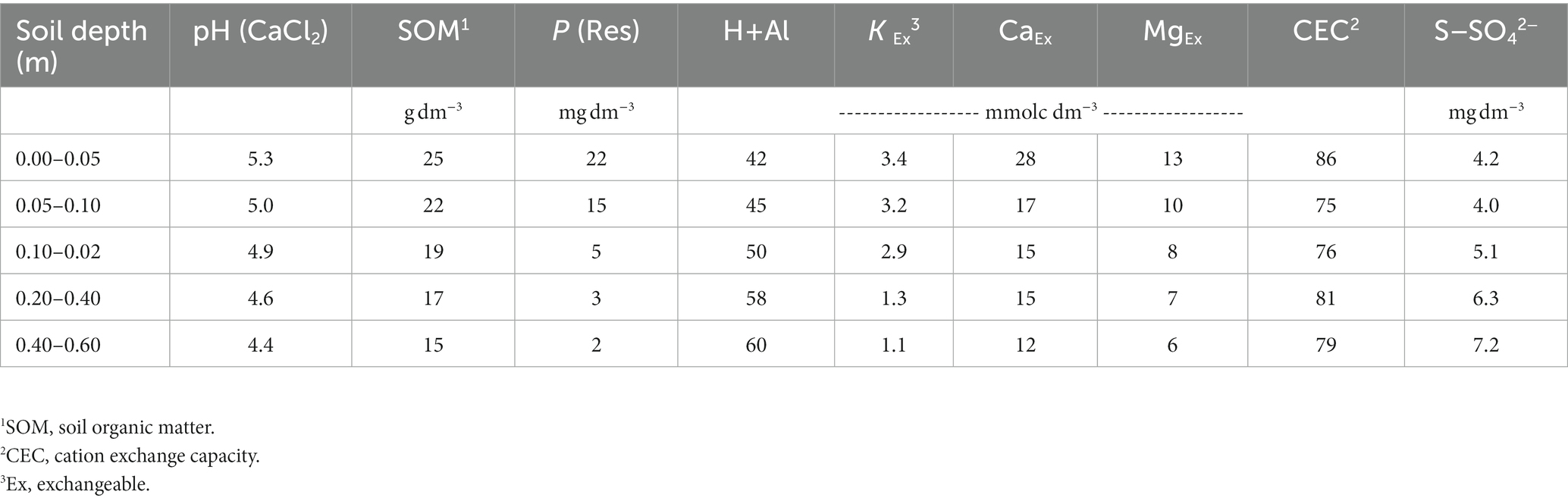
Table 1. Soil chemical characteristics in the experimental area before the establishment of the experiment in the 2014/2015 growing season.
2.2. Experimental design and treatments
The experiment was carried out in a randomized complete block design comprising two treatments with 12 replications. The experimental plots were 5.6 × 20 m in size. The treatments consisted of two distinct cropping systems:(i) a monocropping system (MS) with maize only and (ii) an intercropping system (IS) of maize and Urochloa brizantha cv. Marandu (palisade grass). Both cropping systems were grown during the spring/summer (2014/2015 and 2015/2016 seasons; Figure 2).
In both treatments, maize was sown 20 days after chemical desiccation with glyphosate at 1.96 kg a.i. ha−1 for weed control. The maize hybrid AG 9010 was sown at 55,000 seeds ha−1, with a row spacing of 0.45 m. Fertilization was performed in the sowing furrows with 20 kg N ha−1 as urea, 30.6 kg P ha−1 as triple superphosphate, and 33.3 kg K ha−1 as potassium chloride. In the IS treatment, U. brizantha was sown simultaneously with maize at a seed density of 3.4 kg ha−1. The palisade grass seeds were mixed with the maize fertilizer and sown 0.08 m from the maize seed (Mateus et al., 2007; Crusciol et al., 2012; Borghi et al., 2013). In both growing seasons, atrazine (0.5 kg a.i. ha−1) was applied 15 days after sowing (DAS) for weed control. When the maize plants had five expanded leaves (V5), topdressing was performed with 90 kg N ha−1 as ammonium sulfate. After the maize harvest, the plots remained fallow in the MS treatment but were occupied by palisade grass in the IS treatment until desiccation for sowing of the next spring–summer crop (maize intercropped with U. brizantha in 2015 and common bean in 2016; Figure 2).
2.3. Establishment and management of subsequent crops
After two seasons of the two treatments (MS and IS), five crops were cultivated: three in the spring/summer, i.e., common bean in 2016/2017 and 2017/2018 and maize in 2018, and two in the fall/winter, i.e., wheat in 2016 and 2017 (Figure 2).
The common bean cultivar Pérola was sown with a mechanical seeder at a density of 33 seeds m−2 and a row spacing of 0.45 m on 22 November 2016 and 27 November 2017. Basal fertilizer was applied in the sowing furrow with 10 kg N ha−1 as urea, 50 kg P ha−1 as triple superphosphate, 50 kg K ha−1 as potassium chloride, 1.25 kg Zn ha−1, and 11.25 kg S ha−1. Topdressing with 100 kg ha−1 N as ammonium nitrate (Quaggio et al., 2022) was applied when the plants reached stage V4 (third trifoliate leaf expanded).
The wheat cultivar CD 116 was sown at a density of 80 seeds m−2 and a row spacing of 0.17 m on 17 March 2016 and 22 March 2017. Fertilization was performed at sowing with 75 kg N ha−1 as urea, 70 kg P ha−1 as triple superphosphate, 40 kg K ha−1 as potassium chloride, 1.25 kg Zn ha−1, and 11.25 kg S ha−1. Weed control was performed 10 DAS with 0.8 kg a.i. ha−1 of 2,4-D.
The maize hybrid P30F90 was sown at a density of 60,000 seeds ha−1 and a row spacing of 0.80 m on 7 November 2018. Fertilizer was applied in the sowing furrow with 26 kg N ha−1 as urea, 45.9 kg P ha−1 as triple superphosphate, and 33.3 kg K ha−1 as potassium chloride (Cantarella et al., 2022). When the maize had five expanded leaves (V5), topdressing with 100 kg N ha−1 as ammonium nitrate was applied.
2.4. Sampling and analyzes
Before bean cultivation (November 2016), eight soil subsamples were randomly collected from each plot using a 50-mm-diameter core sampler at depths of 0–0.05, 0.05–0.1, 0.1–0.2, 0.2–0.4, and 0.4–0.6 m. Subsamples from the same depth were pooled to form one sample per depth. The samples were air dried and passed through a 2-mm sieve, and soil chemical properties [pH (CaCl2), soil organic matter (SOM), Presin (phosphorus extracted with ion exchange resin), H + Al, Kex (exchangeable potassium), Caex (exchangeable calcium), Mgex (exchangeable magnesium), CEC (cation exchange capacity), S–SO42−, and BS (base saturation)] were determined (Van Raij et al., 2001).
Leaves were sampled from all crops to determine leaf nutrient concentrations (Cantarella et al., 2022). For common bean, the third trifoliate leaves with the petiole were collected from randomly selected plants in each subplot at the flowering stage. For wheat, leaf samples were collected when 50% of the panicles in each plot were at the flowering stage. For maize, leaf samples were collected when 50% of the plants were at the full flowering stage (silking). The leaf samples were dried under forced-air circulation at 65°C for 72 h and ground, and N, P, K, Ca, Mg, and S were determined (Malavolta et al., 1997). Nitrogen determination was performed by digestion with sulfuric acid, and the remaining nutrients were extracted using nitro-perchloric acid solution.
Common bean was harvested at 95 DAS (26 February 2017) and 92 DAS (27 February 2018). The following yield components were determined: final population of plants, number of pods per plant, number of seeds per pod, 100-grain weight (W100G), and grain yield (GY) calculated at a moisture content of 13%. Additionally, grain from harvest in each plot was classified through a sieve with oblong holes numbers 12 (4.76 × 19.05 mm) to determine the sieve yield (SY) according to Farinelli and Lemos (2010).
Wheat was harvested at 124 DAS (19 July 2016) and 126 DAS (26 July 2017). The following yield components were measured: number of ears per m2 (ESPM), number of spikelets per ear (SPE), number of grains per spikelet (GPS), number of grains per ear, W100G, volumetric hectoliter mass corresponding to the mass of wheat grains in 100 l (HW), and GY (kg ha−1). W100G was determined by weighing four randomly collected samples of 100 grains from each plot.
Maize was harvested at 128 DAS (15 March 2019). The following yield components were determined: final population of plants, number of ears per plant, number of grains per ear, W100G, and GY (kg ha−1) calculated at a moisture content of 13%.
In the 2016/2017 season, root sampling of common bean and wheat was performed. Eight subsamples of roots were randomly collected from each plot and combined to form a composite sample. Four of the subsamples were collected from the plant rows and the other four were collected from the middle of the interrow. Soil was sampled by collection of five simple samples to compose one main sample from each of the following depths using a 69-mm-diameter galvanized steel probe: 0–0.05, 0.05–0.1, 0.1–0.2, 0.2–0.4, and 0.4–0.6 m. The roots were washed under a flow of swirling water over a 0.5-mm mesh sieve (Oussible et al., 1992), carefully separated from the soil, and dried in a forced-air oven at 60°C for 72 h. The root dry matter content (kg ha−1) was then estimated.
2.5. Statistical analysis
The variables were analyzed by ANOVA in the SAS statistical software package (SAS Institute, 1999). All data were tested for normality (Shapiro–Wilk, 1965) and heteroscedasticity using Bartlett’s test (Snecdecor and Cochran, 1991). Blocks and all block interactions were considered random effects. Effects were considered statistically significant at p ≤ 0.1 (n = 24).
3. Results
The concentrations of nutrients in leaves and grains of common bean, wheat, and maize were significantly higher under IS than under MS in all growing seasons (Table 2). Although the leaf concentrations of N, P, K, Ca, Mg, and S were higher under IS, all elements were within the reference value ranges proposed in the São Paulo State official soil fertility and plant nutrition bulletin (Cantarella et al., 2022) for common bean (g kg−1: N, 30–50; P, 2.5–4.0; K, 20–24; Ca, 10–25; Mg, 2.5–5.0; S, 2.0–3.0), wheat (g kg−1: N, 20–34; P, 2.1–3.3; K, 15–30; Ca, 2.5–10; Mg, 1.5–4.0; S, 1.5–3.0), and maize (g kg−1: N, 27–35; P, 2.0–4.0; K, 17–35; Ca, 2.5–8.0; Mg, 1.5–5.0; S, 1.5–3.0) in both treatments.
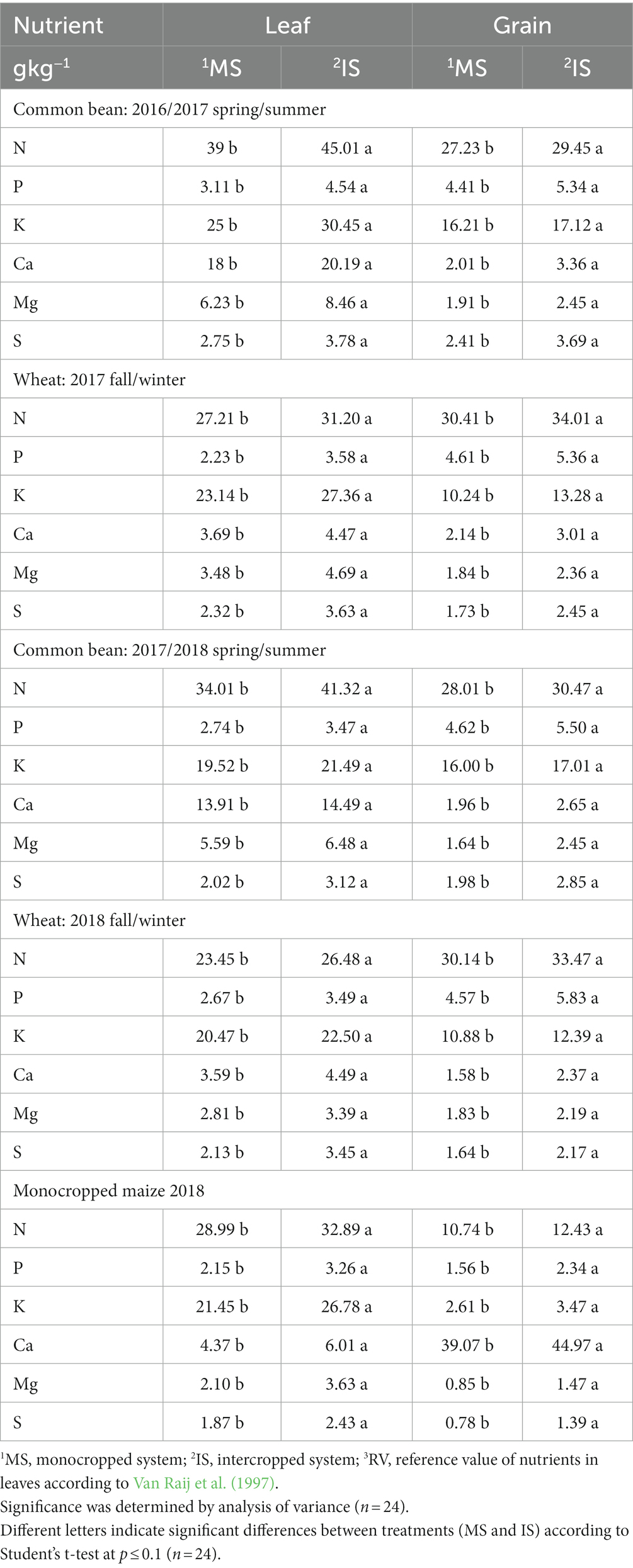
Table 2. Concentrations (g kg−1) of nutrients (N, P, K, Ca, Mg, and S) in leaves and grain of common bean, wheat, and corn from 2016 to 2018.
For common bean, both the root dry matter mass and root distribution percentage in the soil profile were influenced by the treatments (Figure 3). Approximately 70–77% of the total roots was concentrated within a soil depth of 0.0–0.2 m. Although the root distribution percentage was similar between the treatments, the accumulation of root dry matter mass in the soil profile was greater under IS (334 kg ha−1) than under MS (234 kg ha−1). For wheat, the root dry matter mass yield was approximately 340 kg ha−1 higher under IS than under MS (Figure 4). The percentage of roots in the uppermost surface layer (0.0–0.05 m) was 39% under MS and 23% under IS. Under MS, 72% of the roots were distributed within a depth of 0.0–0.2 m, whereas only 57% of the roots were found at this depth under IS. Approximately 23 and 5% of the total roots occupied depths of 0.2–0.4 m and 0.4–0.6 m, respectively, under MS; the corresponding shares were 43 and 15% under IS.
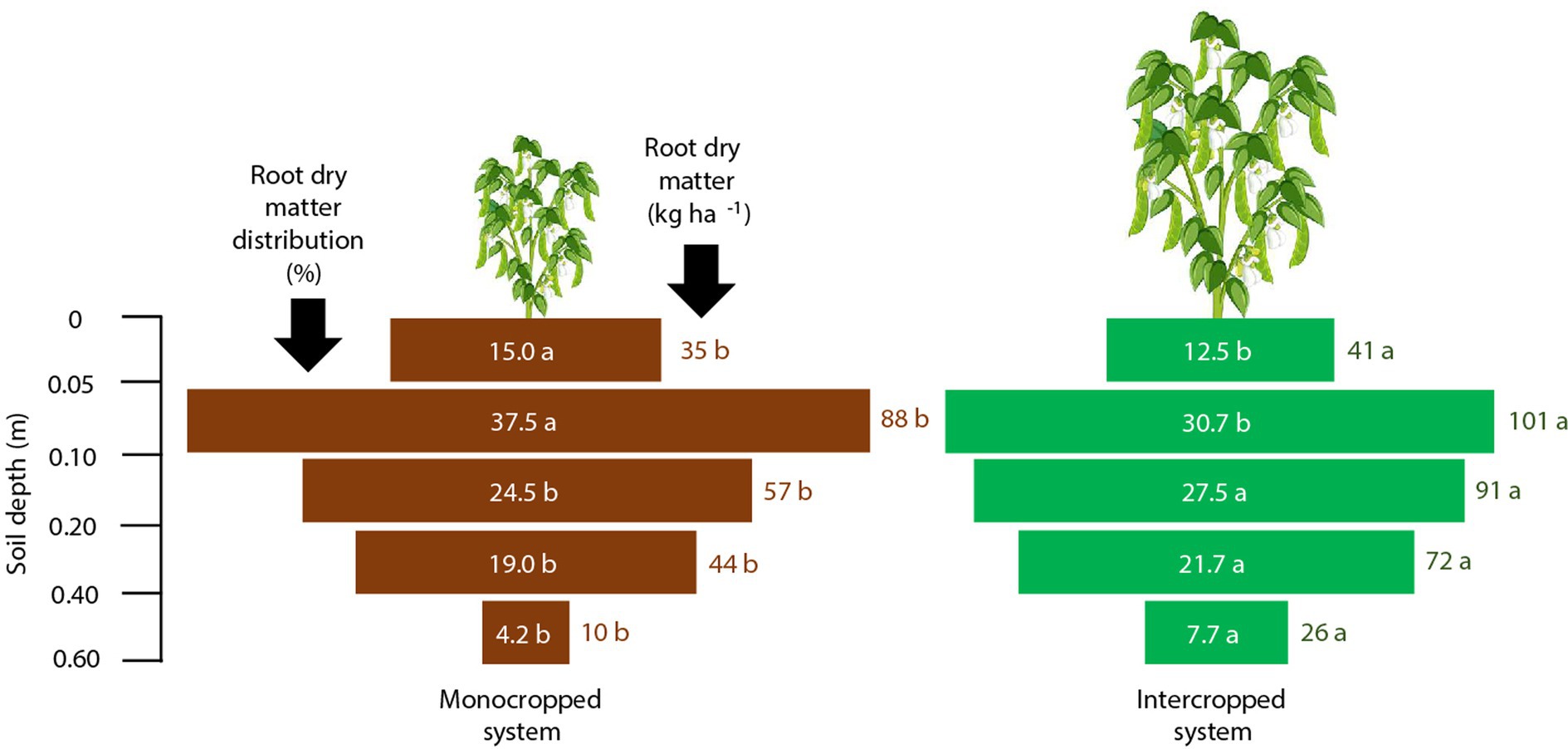
Figure 3. Root dry matter mass and root dry matter distribution of common bean in the different crop production systems (monocropped system and intercropped system). Different letters indicate significant differences between treatments according to Student’s t-test at p ≤ 0.1 (n = 24).
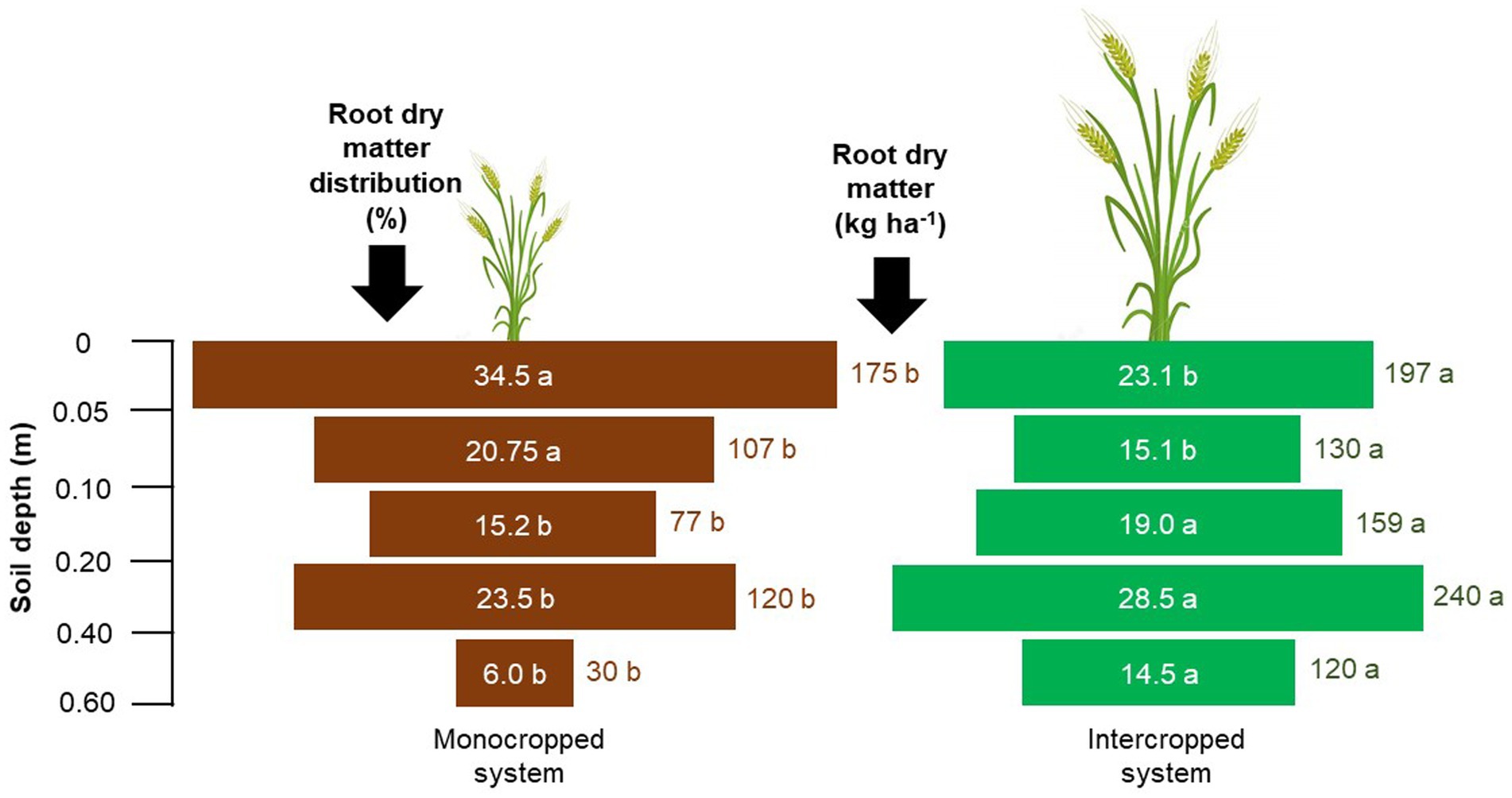
Figure 4. Root dry matter mass and root dry matter distribution of common wheat in the different crop production systems (monocropped system and intercropped system). Different letters indicate significant differences between treatments according to Student’s t-test at p ≤ 0.1 (n = 24).
The yield components of common bean were significantly influenced by the treatments (Figure 5). Compared with MS, IS increased shoot dry matter mass by 9 and 5% in the first and second growing seasons, respectively, and the number of pods per plant by 15% in both cropping seasons (Figures 5A,B). The W100G and sieve yield were higher under IS, indicating that IS induced the production of heavier and larger grains (Figures 5C,D), which directly increased GY. In the first and second growing seasons, the common bean GY was 606 and 414 kg ha−1 higher under IS than under MS, respectively (Figure 5E). In addition, the crude protein content was 14 and 13 g kg−1 higher under IS than under MS in the first and second growing seasons, respectively (Figure 5F).
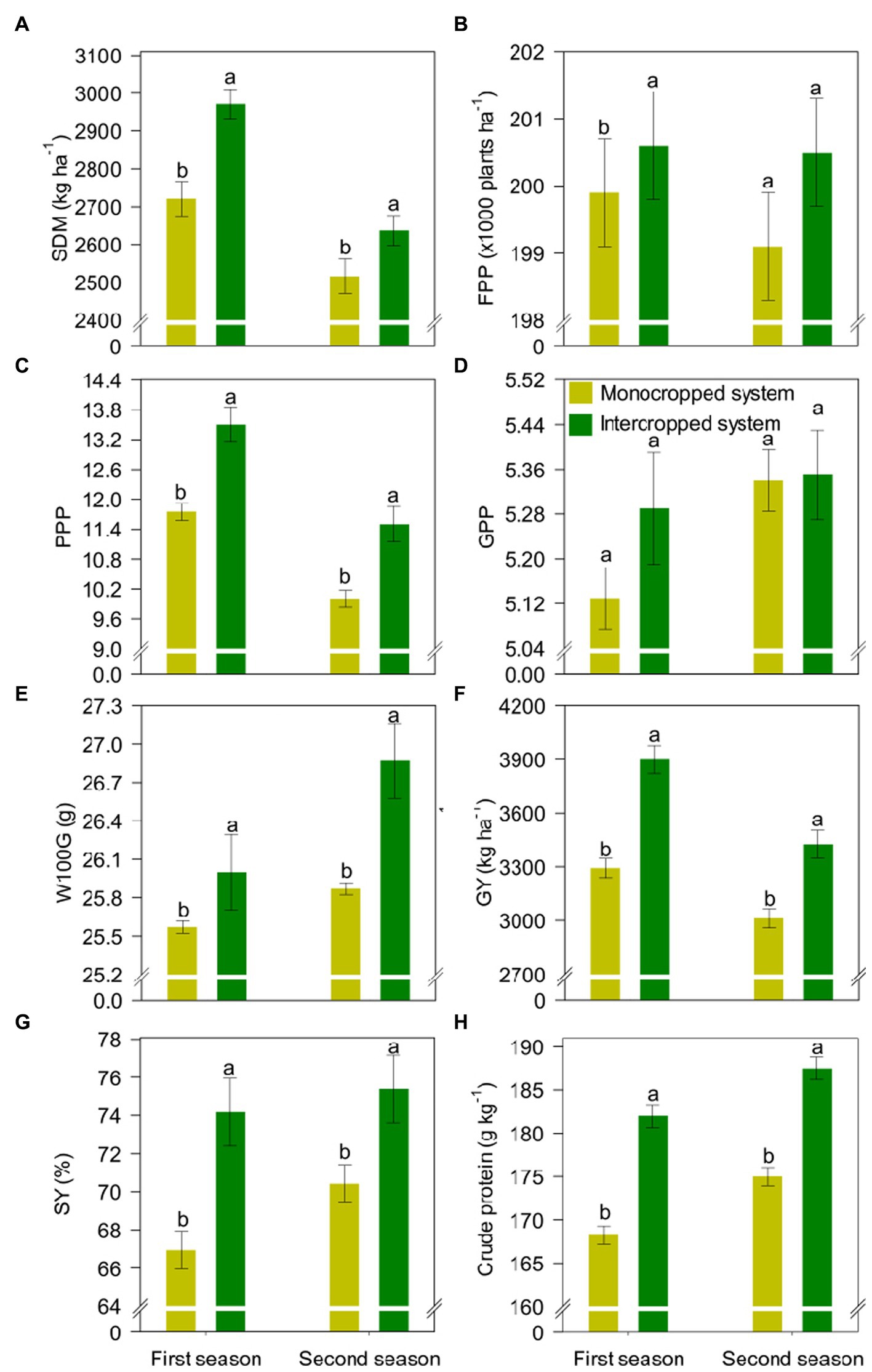
Figure 5. (A) Shoot dry matter (SDM), (B) final plant population (FPP), (C) pods per plant (PPP), (D) grains per pod (GPP), (E) hundred grain weight (W100G), (F) grain yield (GY), (G) sieve yield (SY), and (H) grain crude protein content of common bean in the different crop production systems (monocropped system and intercropped system). Different letters indicate significant differences between treatments according to Student’s t-test at p ≤ 0.1 (n = 24). The error bars express the standard error of the mean.
Previous cover crop management (i.e., the treatments) also affected the yield components of wheat (Figure 6). In the first and second growing seasons, IS increased wheat shoot dry matter mass by 19 and 17% (Figure 6A), ESPM by 7 and 6%, SPE by 15 and 8%, and GPS by 8 and 10%, respectively, compared with MS (Figures 6B–D). In accordance with these improvements in yield components, the wheat yield was 730 and 515 kg ha−1 higher under IS than under MS in the first and second growing seasons, respectively (Figure 6E). IS also enhanced wheat quality components; compared with MS, HW was 1.7 and 1 kg hct−1 higher and crude protein content was 1.3 and 1.5 g kg−1 higher under IS in the first and second growing seasons, respectively (Figures 6F,G).
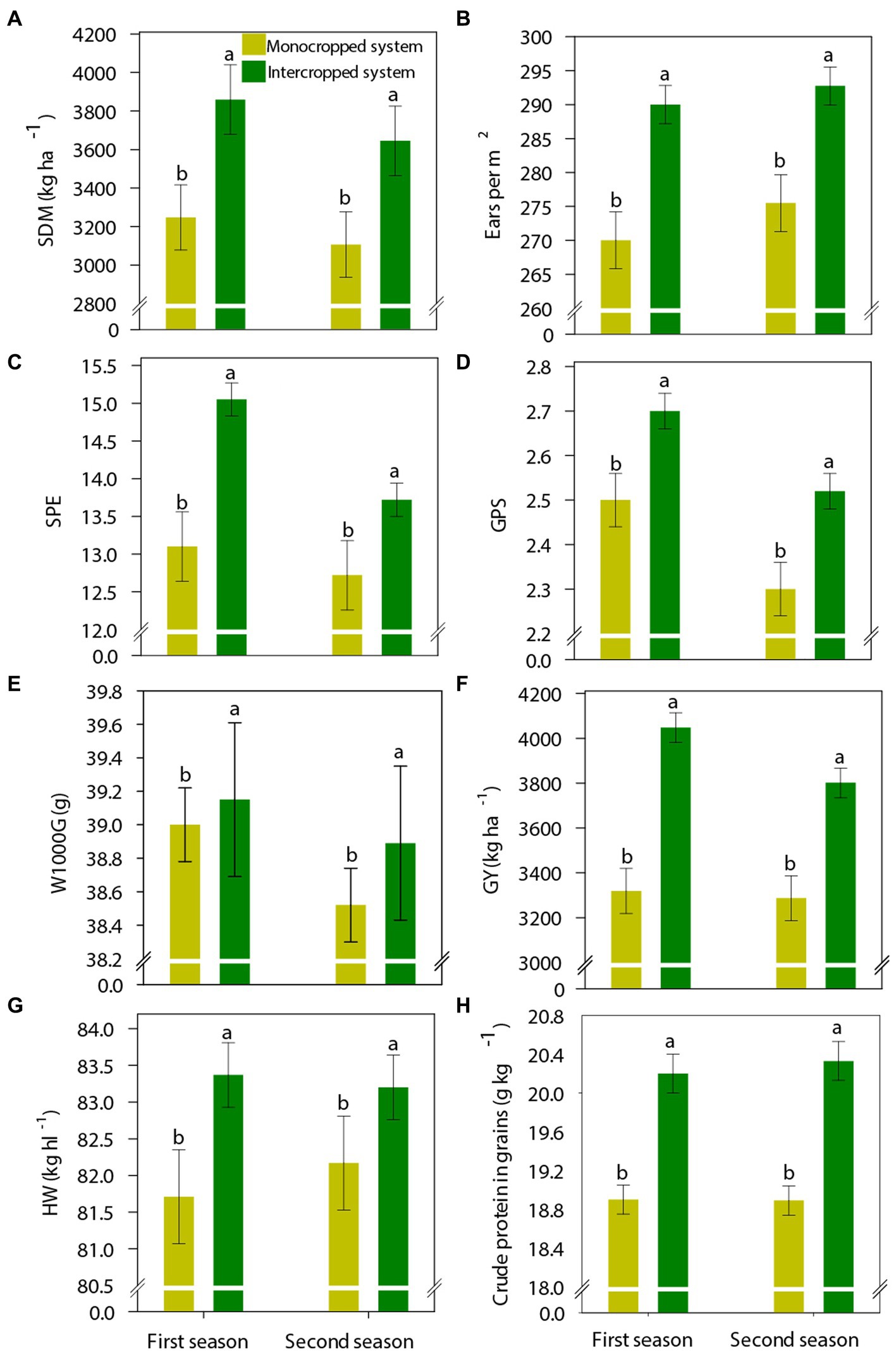
Figure 6. (A) Shoot dry matter (SDM), (B) ears per square meter, (C) spikelets per ear (SPE), (D) grains per spikelet (GPE), (E) hundred grain weight (W100G), (F) grain yield (GY), (G) hectoliter weight (HW), and (H) grain crude protein content of wheat in the different crop production systems (monocropped system and intercropped system). Different letters indicate significant differences between treatments according to Student’s t-test at p ≤ 0.1 (n = 24). The error bars express the standard error of the mean.
Similar to the results for common bean and wheat, the yield components of maize were significantly affected by the treatments (Figure 7). Compared with MS, shoot dry matter mass, GPE, and GY were 12, 10%, and 1930 kg ha−1 higher under IS (Figures 7B,C,F). IS also increased grain quality, as the crude protein content increased by 7 g kg−1 compared with that under MS (Figure 7G).
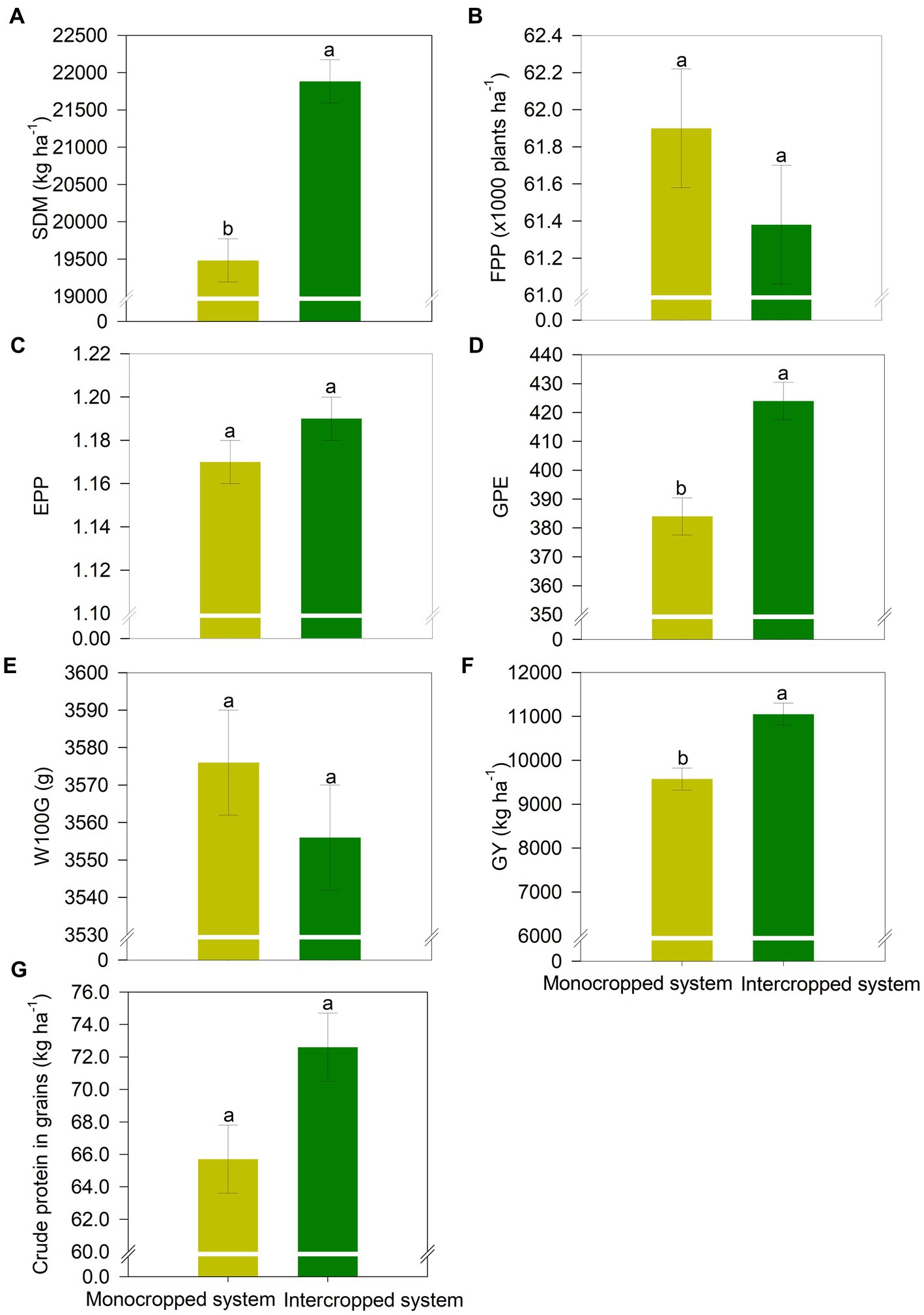
Figure 7. (A) Shoot dry matter (SDM), (B) final plant population (FPP), (C) ears per plant (EPP), (D) grains per ear (GPE), (E) hundred grain weight (W100G), (F) grain yield (GY), and (G) grain crude protein content of maize in the different crop production systems (monocropped system and intercropped system). Different letters indicate significant differences between treatments according to Student’s t-test at p ≤ 0.1 (n = 24). The error bars express the standard error of the mean.
Soil chemical and fertility parameters were significantly influenced by the treatments. Soil pH; SOM; P; exchangeable K, Ca, and Mg; S–SO42−; CEC; and BS were higher under IS than under MS regardless of soil depth (Figure 8). Under IS, soil pH was efficiently buffered in the uppermost surface layer (0.0–0.05 m) and was classified as “very slightly acidic” (pH > 6) according to the soil fertility recommendation bulletin of São Paulo State (Quaggio and van Raij, 1997). The soil pH was classified as “slightly acidic” (5.6–6.0) at a depth of 0.05–0.4 m and “moderately acidic” (5.1–5.5) at a depth of 0.4–0.6 m (Figure 8A). The higher accumulation of SOM and the increases in the concentrations of P, K, Ca, Mg, and S under IS (Figures 8B–G) were attributable to previous cover crop release from the straw and directly increased CEC and BS (Figures 8H,I).
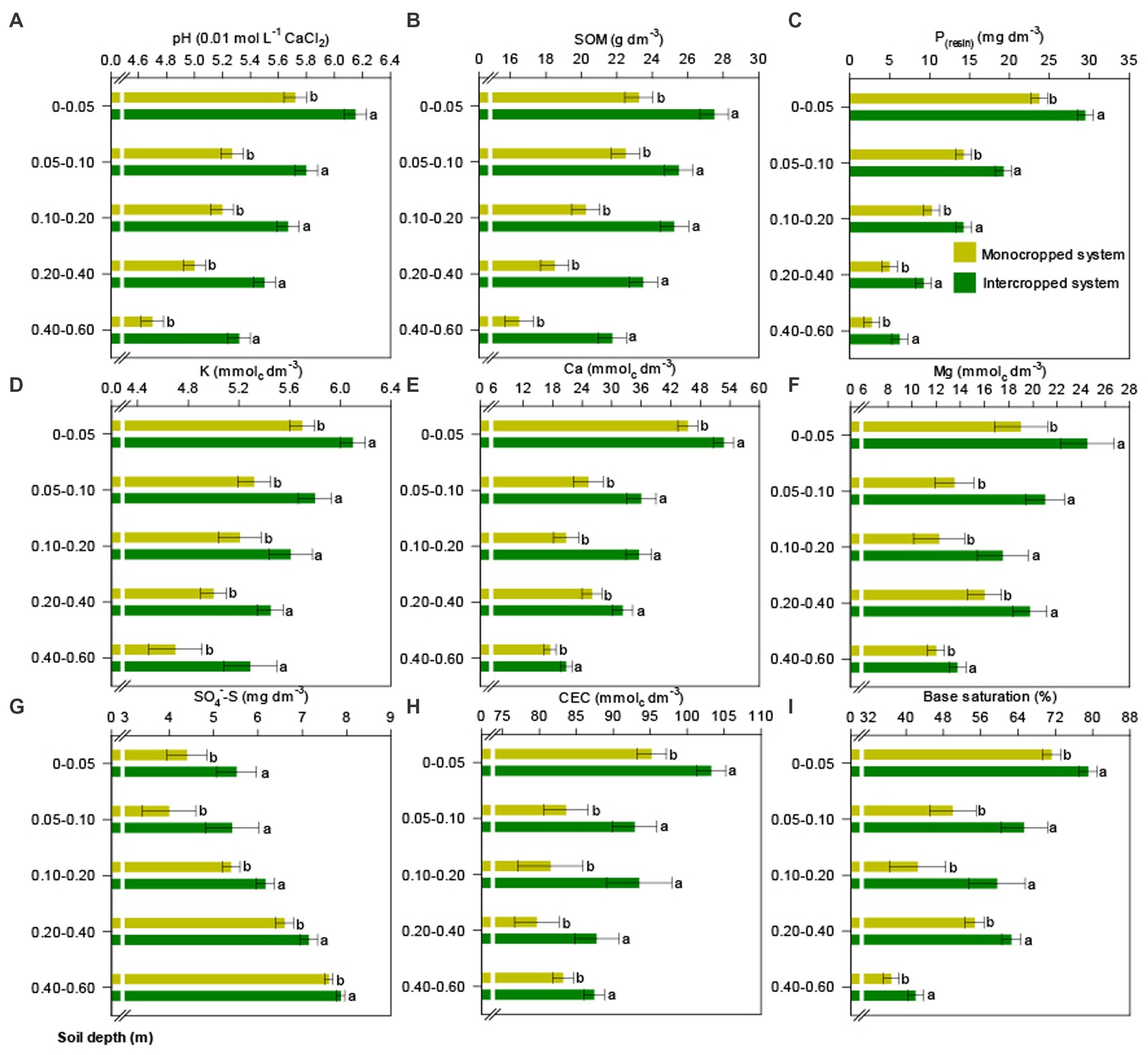
Figure 8. Soil chemical properties in the different crop production systems (monocropped system and intercropped system): (A) soil pH, (B) soil organic matter (SOM), (C) P, (D) K+, (E) Ca2+, (F) Mg2+, (G) S–SO42−, (H) cation exchange capacity (CEC), and (I) base saturation. Different letters indicate significant differences between treatments according to Student’s t-test at p ≤ 0.1 (n = 24). The error bars express the standard error of the mean.
4. Discussion
The higher nutrient recycling capacity of palisade grass enhanced soil chemical and fertility parameters. Soil pH was efficiently buffered 24 months after lime application, as evidenced by the increases in the concentrations of exchangeable Ca and Mg, which can reduce the activity of exchangeable Al in the soil solution (Fageria, 2001; Meriño-Gergichevich et al., 2010). Soil exchangeable Al can also be complexed by organic ligands, which is promoted by higher SOM concentrations such as those observed under IS (Martins et al., 2020; De Campos et al., 2022). Compared with other crops and forage species, palisade grass (U. brizantha) has more vigorous and deeper root growth, which can increase organic carbon accumulation throughout the soil profile. These benefits are magnified in no-till systems because of the large amounts of residues and organic compounds in the soil (Rosolem et al., 2017). Organic material degrades rapidly in weathered soils, which therefore typically have low organic carbon content (Vander Linden and Delvaux, 2019). Intercropping system management can be used as a tool to reduce this natural negative impact. After palisade grass (U. brizantha) is cultivated in the intercropping system, nutrients from the residues are released to the following crops (Nascente and Crusciol, 2012). In IS, U. brizantha continued to grow in the soil after maize harvest until desiccation and thus provided large amounts of dead material on the soil surface. These benefits explain the higher concentrations of nutrients under IS than under MS in this study.
The high quantity of biomass deposited on the soil surface (17.3 and 16.8 Mg ha−1 in the first and second growing seasons, respectively) under IS increased the concentrations of nutrients and SOM in the soil profile. Improved SOM content can lead to increased P and S–SO42− availabilities because these nutrients are primarily obtained by mineralizing organic compounds and recycling biomass residues (Vicensi et al., 2020). Additionally, the biopores formed by forage grasses can improve soil macroporosity and microporosity and can facilitate the displacement of fine lime particles throughout the soil profile, which explains the higher concentrations of Ca2+ and Mg2+ under IS (Bossolani et al., 2020; De Campos et al., 2022). The increase in the K concentration under IS can also be attributed to recycling of biomass residues because K is not associated with carbon skeletons and can return to the soil through biomass deposition (Rosolem et al., 2017; Soratto et al., 2021). SOM content is the most important determinant of CEC in weathered soils. Because of their oxidic mineralogy and the absence of permanent negative charges, weathered soils are classified as soils with variable or pH-dependent charge (Kosmulski, 2020). Thus, the higher CEC and BS under IS are attributable to increases in SOM content.
Root growth is subject to both physical and chemical impediments (Scarpari and de Beauclair, 2004; De Campos et al., 2022). The presence of adequate soil chemical properties probably explains the increases in root dry matter mass and homogeneous distribution up to a depth of 0.6 m under IS. The greater volume of root growth and soil exploration by palisade grass also enhance soil fertility by improving the biological and physical attributes of the soil (Costa and Rosolem, 2007; Carmeis Filho et al., 2017). By reducing the accumulation of roots in the uppermost surface layers, IS can reduce the susceptibility of plants to water stress.
The leaf concentrations of nutrients were in accordance with the proposed ranges for the crops (Cantarella et al., 2022; Quaggio et al., 2022). The nutrient concentrations in common bean grain were consistent with those previously reported (Soratto et al., 2014), and the higher crude protein contents of both crops under IS may be the result of increased nutrient concentrations.
The common bean, wheat, and maize crops were positively influenced by the previous cultivation of intercropped palisade grass and maize. The dead material from palisade grass supplied nutrients to common bean, mainly in the first season (2016). Forage plays a role in the cycling and release of nutrients, especially N and K (Pariz et al., 2017a; Momesso et al., 2021). In the subsequent wheat-common bean-wheat-maize rotation, the positive benefits of IS on residue nutrient release and soil fertility persisted and manifested as increased nutrient uptake by these crops, as observed in other studies (Crusciol et al., 2012; Nascente and Crusciol, 2012; Borghi et al., 2013).
These results indicate that forage residue, root decomposition, and physical and biological improvements in the soil can persist during crop cultivation and in subsequent years of crop rotations. Intercropping maize with palisade grass can be an important tool to minimize the effects of poor soils, such as those in the Brazilian Cerrado and African savanna (Oonyu, 2011). Although changes in the soil–plant system during common bean cultivation were not studied in detail, the results of this study showed that the residues from the intercropping system effectively supplied all nutrients to common bean. This is an important finding since common bean does not typically grow well in no-till systems with large amounts of straw (Momesso et al., 2021).
Under MS, spontaneous plants (weeds) provided poor soil coverage and nutrient release compared with IS. A few studies have shown that fallow periods do not reduce residue production (Pacheco et al., 2011, 2017). However, spontaneous plants do not grow uniformly in agricultural systems and can be hosts for pathogens and pests. We found that crops in succession to fallow had reduced nutrient availability. Momesso et al. (2022) suggested that compared to fallow periods, including palisade grass in the agricultural system stimulates microbial species that play essential roles in soil nutrient cycling and SOM decomposition. Therefore, the practice of using fallow periods to produce crop residues is not recommended for no-till systems.
In both systems, residues were not removed from the soil. Residue maintenance in no-till systems is essential for high development and yields of subsequent crops. Low soil coverage by straw, such as that in MS, favors soil degradation and reduced crop yield (Crusciol et al., 2012). In monocropping systems, in which monocropped maize is followed by a fallow period and annual species (common bean, wheat, and maize) growth, the return of crop residues to the soil is insufficient, leading to limited nutrient concentrations in the soil.
In the U. brizantha agricultural system (i.e., IS), the root systems of common bean and wheat reached deeper layers. When common bean and wheat were cultivated after a fallow period (i.e., MS), the root system was superficial, with an inferior distribution. Thus, IS benefitted subsequent cultivation by improving the root systems of crops in rotation. The U. brizantha root system includes fine roots that increase the complexity of the soil pore network, and the vast root architecture of U. brizantha positively alters soil structure throughout the soil layers (Galdos et al., 2020). In addition, a well-formed, deep root system improves nutrient and water absorption and plant resistance to adverse weather conditions.
In summary, the intercropping cultivation strategy proposed in this study for the spring/summer season may be an alternative practice for increasing soil fertility, nutrient cycling, as well as the growth, development, and yields of subsequent crops in rotation. Our results indicate that intercropping can enhance agrisystem services in both the short-term (2 years) and medium-term (5 years). Intercropping maize with forage species such as palisade grass can protect the soil for a longer period, ensuring high yields of beans, wheat, and maize in succession. Another advantage of this intercropping system is that planting forage guarantees farmers profitability over the years while providing initial benefits to the soil in the form of dead material as soil cover.
5. Conclusion
Cultivating maize intercropped with Urochloa brizantha for 2 years had positive medium-term effects on common bean, wheat, and maize in succession under no-till crop rotation over at least 3 years after U. brizantha desiccation. The intercropping system increased the soil nutrient concentrations of P; exchangeable K, Ca, and Mg; S–SO42−; soil fertility parameters such as pH, soil organic matter, cation exchange capacity, and base saturation; the dry matter mass content and distribution of roots of common bean, wheat, and maize; crop leaf and grain concentrations of N, P, K, Ca, Mg, and S; yield components and grain yield; and nutrient recycling potential.
Data availability statement
The original contributions presented in the study are included in the article/supplementary material, further inquiries can be directed to the corresponding author.
Author contributions
CC, JS, MV, EB, RS, and JC contributed to conceptualization, data acquisition, data analysis, and methodology design. LM, JP, and MC wrote and edited the manuscript. All authors contributed to the article and approved the submitted version.
Acknowledgments
The authors would like to thank the Coordination for the Improvement of Higher Level Personnel (CAPES/PROAP). The first, eighth, and ninth authors would like to thank the National Council for Scientific and Technological Development (CNPq) for an award for excellence in research.
Conflict of interest
The authors declare that the research was conducted in the absence of any commercial or financial relationships that could be construed as a potential conflict of interest.
Publisher’s note
All claims expressed in this article are solely those of the authors and do not necessarily represent those of their affiliated organizations, or those of the publisher, the editors and the reviewers. Any product that may be evaluated in this article, or claim that may be made by its manufacturer, is not guaranteed or endorsed by the publisher.
References
Archer, D. W., Franco, J. G., Halvorson, J. J., and Pokharel, K. P. (2019). “Integrated farming systems,” in Encyclopedia of ecology. ed. B. Fath . 2nd ed (Oxford: Elsevier), 508–514.
Borghi, E., Crusciol, C. A. C., Mateus, G. P., Nascente, A. S., and Martins, P. O. (2013). Intercropping time of corn and palisadegrass or guineagrass affecting grain yield and forage production. Crop Sci. 53, 629–636. doi: 10.2135/cropsci2012.08.0469
Bossolani, J. W., dos Santos, F. L., Meneghette, H. H. A., Sanches, I. R., Moretti, L. G., Parra, L. F., et al. (2020). Soybean in crop rotation with maize and palisade grass intercropping enhances the long-term effects of surface liming in no-till system. J. Soil Sci. Plant Nutr. 21, 119–130. doi: 10.1007/s42729-020-00347-2
Calonego, J. C., Borghi, E., and Crusciol, C. A. C. (2011). Least limiting water range and soil compactation as related to intercropped maize and brachiaria. Rev. Bras. Ciênc. Solo 35, 2183–2190. doi: 10.1590/S0100-06832011000600033
Calonego, J. C., Raphael, J. P. A., Rigon, J. P. G., de Oliveira Neto, L., and Rosolem, C. A. (2017). Soil compaction management and soybean yields with cover crops under no-till and occasional chiseling. Eur. J. Agron. 85, 31–37. doi: 10.1016/j.eja.2017.02.001
Cantarella, H., Zambrosi, F. C. B., Quaggio, J. A., and Duarte, A. P. (2022). “Cereals” in Lime and fertilizer recommendations for the state of Sao Paulo. eds. H. Cantarella, J. A. Quaggio, D. Mattos Junior, R. M. Boaretto and B. V. van Raij (Campinas, Brazil: Agronomic Institute of Campinas), 213–238.
Carmeis Filho, A. C. A., Crusciol, C. A. C., and Castilhos, A. M. (2017). Liming demand and plant growth improvements for an Oxisol under long-term no-till cropping. J. Agric. Sci. 155, 1093–1112. doi: 10.1017/S0021859617000235
Costa, A., and Rosolem, C. A. (2007). Liming in the transition to no-till under a wheat–soybean rotation. Soil Tillage Res. 97, 207–217. doi: 10.1016/j.still.2007.09.014
Crusciol, C. A. C., Mateus, G. P., Momesso, L., Pariz, C. M., Castilhos, A. M., Calonego, J. C., et al. (2020). Nitrogen-fertilized systems of maize intercropped with tropical grasses for enhanced yields and estimated land use and meat production. Front. Sustain. Food Syst. 4:544853. doi: 10.3389/fsufs.2020.544853
Crusciol, C. A. C., Mateus, G. P., Nascente, A. S., Martins, P. O., Borghi, E., and Pariz, C. M. (2012). An innovative crop-forage intercrop system: early cycle soybean cultivars and palisadegrass. Agron. J. 104, 1085–1095. doi: 10.2134/agronj2012.0002
Crusciol, C. A. C., Momesso, L., Portugal, J. R., Costa, C. H. M., Bossolani, J. W., Costa, N. R., et al. (2021). Upland rice intercropped with forage grasses in an integrated crop-livestock system: optimizing nitrogen management and food production. Field Crop Res. 261:108008. doi: 10.1016/j.fcr.2020.108008
Daryanto, S., Jacinthe, P.-A., Fu, B., Zhao, W., and Wang, L. (2019). Valuing the ecosystem services of cover crops: barriers and pathways forward. Agric. Ecosyst. Environ. 270-271, 76–78. doi: 10.1016/j.agee.2018.10.021
De Campos, M., Penn, C. J., Gonzalez, J. M., and Crusciol, C. A. C. (2022). Effectiveness of deep lime placement and tillage systems on aluminum fractions and soil chemical attributes in sugarcane cultivation. Geoderma 407:115545. doi: 10.1016/j.geoderma.2021.115545
Dias, D. S., Umburanas, R. C., and Sena Junior, D. G. (2018). Sowing date of palisadegrass intercropped with grain sorghum and soybean yield in succession. Comun. Sci. Hortic. J. 9, 194–201. doi: 10.14295/cs.v9i2.2487
Fageria, V. D. (2001). Nutrient interactions in crop plants. J. Plant Nutr. 24, 1269–1290. doi: 10.1081/PLN-100106981
Farinelli, R., and Lemos, L. B. (2010). Agronomic characteristics in common bean genotypes in dry and summer seasons. Bragantia 69, 361–366. doi: 10.1590/S0006-87052010000200013
Fung, K. M., Tai, A. P. K., Yong, T., Liu, X., and Lam, H.-M. (2019). Co-benefits of intercropping as a sustainable farming method for safeguarding both food security and air quality. Environ. Res. Lett. 14:044011. doi: 10.1088/1748-9326/aafc8b
Galdos, M. V., Brown, E., Rosolem, C. A., Pires, L. F., Hallett, P. D., and Mooney, S. J. (2020). Brachiaria species influence nitrate transport in soil by modifying soil structure with their root system. Sci. Rep. 10:5072. doi: 10.1038/s41598-020-61986-0
Gristina, L., Keesstra, S., and Novara, A. (2018). No-till durum wheat yield success probability in semi arid climate: a methodological framework. Soil Tillage Res. 181, 29–36. doi: 10.1016/j.still.2018.04.002
Kosmulski, M. (2020). The pH dependent surface charging and points of zero charge. VIII. Update. Adv. Colloid Interf. Sci. 275:102064. doi: 10.1016/j.cis.2019.102064
Malavolta, E., Vitti, G. C., and Oliveira, S. A. (1997). Evaluation of nutritional status of plant: Principles and applications. Piracicaba, SP, Brazil: Potafos.
Martins, D. S., Reis, V. M., Schultz, N., Alves, B. J. R., Urquiaga, S., Pereira, W., et al. (2020). Both the contribution of soil nitrogen and of biological N2 fixation to sugarcane can increase with the inoculation of diazotrophic bacteria. Plant Soil 454, 155–169. doi: 10.1007/s11104-020-04621-1
Mateus, G. P., Borghi, É., Marques, R. R., Bôas, R. L. V., and Crusciol, C. A. C. (2007). Sources and time of contact of mineral fertilizer with Brachiaria brizantha seeds as related with germination. Rev. Bras. Cienc. Solo 31, 177–183. doi: 10.1590/s0100-06832007000100018
Meriño-Gergichevich, C., Alberdi, M., Ivanov, A. G., and Reyes-Diaz, M. (2010). Al 3+ − Ca2+interaction in plants growing in acid soils: Al-phytotoxicity response to calcareous amendments. J. Soil Sci. Plant Nutr. 10, 217–243.
Momesso, L., Crusciol, C. A. C., Leite, M. F. A., Bossolani, J. W., and Kuramae, E. E. (2022). Forage grasses steer soil nitrogen processes, microbial populations, and microbiome composition in a long-term tropical agriculture system. Agric. Ecosyst. Environ. 323:107688. doi: 10.1016/j.agee.2021.107688
Momesso, L., Crusciol, C. A. C., Soratto, R. P., Tanaka, K. S., Costa, C. H. M., Bastos, L. M., et al. (2021). Cover crop and early nitrogen management for common bean in a tropical no-till system. Agron. J. 113, 5143–5156. doi: 10.1002/agj2.20815
Nascente, A. S., and Crusciol, C. A. C. (2012). Cover crops and herbicide timing management on soybean yield under no-tillage system. Pesq. Agropec. Bras. 47, 187–192. doi: 10.1590/S0100-204X2012000200006
Nunes, A. L. P., Bartz, M. L., Mello, I., Bortoluzzi, J., Roloff, G., Fuentes Llanillo, R., et al. (2020). No-till system participatory quality index in land management quality assessment in Brazil. Eur. J. Soil Sci. 71, 974–987. doi: 10.1111/ejss.12943
Oonyu, J. (2011). Upland rice growing: a potential solution to declining crop yields and the degradation of the Doho wetlands, Butaleja district-Uganda. Afr. J. Agric. Res. 6, 2774–2783. doi: 10.5897/AJAR10.806
Oussible, M., Crookston, R. K., and Larson, W. E. (1992). Subsurface compaction reduces the root and shoot growth and grain yield of wheat. Agron. J. 84, 34–38. doi: 10.2134/agronj1992.00021962008400010008x
Pacheco, L. P., Leandro, W. M., de Machado, P. L. O. A., de Assis, R. L., Cobucci, T., Madari, B. E., et al. (2011). Produção de fitomassa e acúmulo e liberação de nutrientes por plantas de cobertura na safrinha. Pesq. Agrop. Brasileira 46, 17–25. doi: 10.1590/S0100-204X2011000100003
Pacheco, L. P., Monteiro, M. M. D. S., Petter, F. A., Nóbrega, J. C. A., and Santos, A. S. D. (2017). Biomass and nutrient cycling by cover crops in Brazilian Cerrado in the state of Piaui. Rev. Caatinga 30, 13–23. doi: 10.1590/1983-21252017v30n102rc
Pariz, C. M., Costa, C., Crusciol, C. A. C., Castilhos, A. M., Meirelles, P. R. L., Roça, R. O., et al. (2017a). Lamb production responses to grass grazing in a companion crop system with corn silage and oversowing of yellow oat in a tropical region. Agric. Syst. 151, 1–11. doi: 10.1016/j.agsy.2016.11.004
Pariz, C. M., Costa, C., Crusciol, C. A. C., Meirelles, P. R. L., Castilhos, A. M., Andreotti, M., et al. (2017b). Production, nutrient cycling and soil compaction to grazing of grass companion cropping with corn and soybean. Nutr. Cycl. Agroecosyst. 108, 35–54. doi: 10.1007/s10705-016-9821-y
Pittelkow, C. M., Liang, X., Linquist, B. A., van Groenigen, K. J., Lee, J., Lundy, M. E., et al. (2015). Productivity limits and potentials of the principles of conservation agriculture. Nature 517, 365–368. doi: 10.1038/nature13809
Portugal, J. R., Arf, O., Buzetti, S., Portugal, A. R. P., Garcia, N. F. S., Meirelles, F. C., et al. (2020). Do cover crops improve the productivity and industrial quality of upland rice? Agron. J. 112, 327–343. doi: 10.1002/agj2.20028
Quaggio, J. A., Cantarella, H., and Zambrosi, F. C. B. (2022). “Legumes and oilseeds,” in Lime and fertilizer recommendations for the state of Sao Paulo. eds. H. Cantarella, J. A. Quaggio, D. Mattos Junior, R. M. Boaretto and B. V. van Raij (Campinas, Brazil: Agronomic Institute of Campinas), 239–258.
Quaggio, J. A., and van Raij, B. (1997). “Correção da acidez do solo,” in Recomendações de adubação e calagem para o Estado de São Paulo, eds. B. Raijvan, H. Cantarella, J. A. Quaggio, and A. M. C. Furlani (Campinas, Brazil: Instituto Agronômico), 14–19.
Rosolem, C. A., Ritz, K., Cantarella, H., Galdos, M. V., Hawkesford, M. J., Whalley, W. R., et al. (2017). “Chapter five - enhanced plant rooting and crop system Management for Improved N use Efficiency” in Advances in agronomy. ed. D. L. Sparks (Cambridge, Massachusetts: Academic Press), 205–239.
Scarpari, M. S., and de Beauclair, E. G. F. (2004). Sugarcane maturity estimation through edaphic-climatic parameters. Sci. Agric. 61, 486–491. doi: 10.1590/S0103-90162004000500004
Snecdecor, G. W., and Cochran, W. G. (1991). Statistical methods, 8th Edn. Hoboken, NJ: Wiley-Blackwell.
Soil Survey Staff . (2014). Keys to soil taxonomy. 14th Edn.. Washington, DC: USDA-Natural Resources Conservation Service.
Soratto, R. P., Crusciol, C. A. C., de Campos, M., da Costa, C. H. M., Gilabel, A. P., Castro, G. S. A., et al. (2021). Silicate rocks as an alternative potassium fertilizer for upland rice and common bean crops. Pesq. Agropec. Bras. 56:e01411. doi: 10.1590/s1678-3921.pab2021.v56.01411
Soratto, R. P., Perez, A. A. G., and Fernandes, A. M. (2014). Age of no-till system and nitrogen management on common bean nutrition and yield. Agron. J. 106, 809–820. doi: 10.2134/agronj13.0439
Tanaka, K. S., Crusciol, C. A. C., Soratto, R. P., Momesso, L., Costa, C. H. M., Franzluebbers, A. J., et al. (2019). Nutrients released by Urochloa cover crops prior to soybean. Nutr. Cycl. Agroecosyst. 113, 267–281. doi: 10.1007/s10705-019-09980-5
Van Raij, B., Andrade, J. C., Cantarella, H., and Quaggio, J. A. (2001). Chemical analysis for evaluation of fertility of tropical soils. Campinas, Brazil: Agronomic Institute of Campinas.
Van Raij, B., Cantarella, H., Quaggio, J. A., and Furlani, A. M. C. (1997). Recomedações de adubação e calagem para o Estado de São Paulo. 2nd Edn. Campinas, SP, Brazil: Instituto Agronômico (IAC).
Vander Linden, C., and Delvaux, B. (2019). The weathering stage of tropical soils affects the soil-plant cycle of silicon, but depending on land use. Geoderma 351, 209–220. doi: 10.1016/j.geoderma.2019.05.033
Vicensi, M., Lopes, C., Koszalka, V., Umburanas, R. C., Kawakami, J., Pott, C. A., et al. (2020). Gypsum rates and splitting under no-till: soil fertility, corn performance, accumulated yield and profits. J. Soil Sci. Plant Nutr. 20, 690–702. doi: 10.1007/s42729-019-00157-1
Keywords: palisade grass, tropical agriculture, intercropping grasses, no-tillage system, root system
Citation: Crusciol CAC, Santos Ferreira JH, Momesso L, Portugal JR, de Campos M, Volf MR, Borghi E, Soratto RP and Calonego JC (2023) Lasting effect of Urochloa brizantha on a common bean-wheat-maize rotation in a medium-term no-till system. Front. Sustain. Food Syst. 7:940996. doi: 10.3389/fsufs.2023.940996
Edited by:
Leonardo Deiss, The Ohio State University, United StatesReviewed by:
Milan Oldřich Urban, International Center for Tropical Agriculture (CIAT), ColombiaIdupulapati Madhusudana Rao, International Center for Tropical Agriculture (CIAT), Colombia
Copyright © 2023 Crusciol, dos Santos Ferreira, Momesso, Portugal, de Campos, Volf, Borghi, Soratto and Calonego. This is an open-access article distributed under the terms of the Creative Commons Attribution License (CC BY). The use, distribution or reproduction in other forums is permitted, provided the original author(s) and the copyright owner(s) are credited and that the original publication in this journal is cited, in accordance with accepted academic practice. No use, distribution or reproduction is permitted which does not comply with these terms.
*Correspondence: Carlos Alexandre Costa Crusciol, Y2FybG9zLmNydXNjaW9sQHVuZXNwLmJy