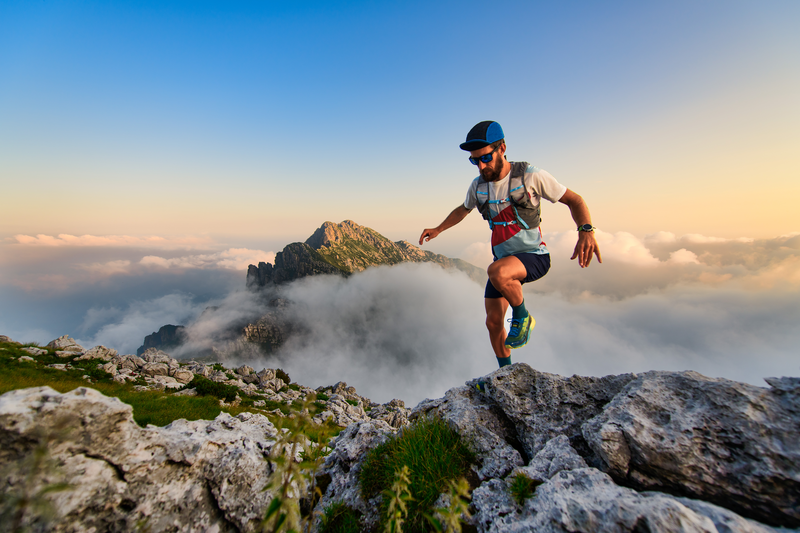
95% of researchers rate our articles as excellent or good
Learn more about the work of our research integrity team to safeguard the quality of each article we publish.
Find out more
REVIEW article
Front. Sustain. Food Syst. , 09 January 2024
Sec. Sustainable Food Processing
Volume 7 - 2023 | https://doi.org/10.3389/fsufs.2023.1331113
This article is part of the Research Topic The Aquatic Product Processing and By-product Utilization View all 10 articles
Fileting is a popular form of processing methods and in addition to being sold fresh or frozen, filets are used for preparation products battered and breaded filets. This generates considerable amount of side streams like skin, frames, and cut-offs which forms around 30–70% of the total body weight. The European Waste Framework Directive 2008 and recent amendments [EU WFD (2018/851)] stipulates comprehensive regulations which the manufacturers must follow while handling the side streams generated during the processing. This demands a detailed compilation of information regarding the yield, classification and valorization potential of side streams associated with the fileting operations of the cold-water finfishes. The side streams are a rich source of proteins including structural proteins like collagen and biominerals such as hydroxyapatite which find multiple application in food and pharmaceutical industry. Previously, the recovery of these components was performed by extensive chemical treatment with acids or bases, including subsequent washing steps. Nowadays, green extraction methods, defined as technologies with reduced energy and chemical consumption, should be considered to achieve a green shift in the food industry. Nevertheless, industrial upscaling of green extraction methods and subsequent refinement of the isolated compounds must be further evaluated and improved in order to achieve a green shift in food industry by using side-stream derived compounds as ingredients. Moreover, legislations as well as national and international regulations must be considered and evaluated. Even though a number of articles are recently available regarding seafood side stream valorization, this review focus on side streams generated predominantly from cold water fish species and also discusses sustainable green technologies to be included during the recovery process.
The utilization of marine resources has been shaping the lives of generations living along coastlines around the world. In 2020, the global fish production showed a total number of 176 million tons (FAO, 2022). Today, an increasing amount of fish is processed after capture, rather than being sold as whole fish. Further processing of fish into filets, which usually is the main product of fish, generates a high amount of by-products. These by-products can account for up to 70% of the fish weight (Ozogul et al., 2021). The post-harvest preparation of filets includes different steps, but involves the removal of intestines, heads, fins, backbones and skins (Ghaly et al., 2013; Maschmeyer et al., 2020). Moreover, significant amounts of cut-offs are generated during automated fileting, and together with other by-products show the possibility to be more efficiently utilized, aiming for circular utilization and valorization. The major portion of the fish side streams generated today are utilized for production of undervalued products like animal feed, fertilizer and biofuel (Välimaa et al., 2019; FAO, 2020). Even though the utilization of side streams as a feed ingredient facilitates its re-entry into the food chain, it causes an energy loss of up to 90% toward its conversion to meet rendering the use of side streams for human nutrition more significant (Venslauskas et al., 2021). Depending on fish species, sex, maturity, season etc. the secondary products, referred to as side streams contain varying amounts of proteins, lipids, ash, and minerals (Maschmeyer et al., 2020). The different side stream fractions contain a large number of valuable compounds, such as oils, including valuable omega-3 fatty acids and proteins, composing of bioactive peptides, essential amino acids and collagen. Moreover, frames and bones are an excellent source of hydroxyapatite (HAp) (Rustad et al., 2011). Proteins derived from marine resources are highly digestible, with good amino acid profiles and when broken down into its peptides, show multiple bioactive properties (Ucak et al., 2021). In addition, collagen has received attention due to its broad range of possible applications, ranging from food ingredients, skin care to the utilization as biomedical material (Regenstein and Zhou, 2007; Välimaa et al., 2019; Subhan et al., 2021).
The focus of this review article lies within the proteinaceous compounds of fileting by-products as new biomaterials, hence valuable compounds within the oil fraction are not further considered, but have been discussed previously in e.g., Shahidi (2007), Wu and Bechtel (2008), and Rustad et al. (2011). Specifically, the objective of this review article is to highlight the potential of biomaterials such as hydroxyapatite, collagen and bioactive peptides as innovative biomaterials in various applications. In addition, extraction methods, both conventional and green procedures to isolate these valuable biomaterials from the by-product fractions are described. Special emphasis is placed on highlighting the potential of newer, environmentally friendly methods described as green methods in literature. These methods offer the opportunity to enhance the value of the waste resources, while simultaneously reducing energy and/or chemical consumption in extraction processes.
Fileting by-products offer several high-value compounds for further utilization in various fields of applications. The importance of marine protein and proteinaceous compounds is marked by the amount of protein available in different by-product fractions. As an example, the protein contents of Alaska pollock, pink salmon and Pacific cod by-products were reported to range from 13.9 to 16.4% in heads, 13.0 to 15.3% in viscera, around 25% in skins and 15.8 to 16.3% in frames on a wet weight basis (Bechtel, 2003). Marine proteins are highly valuable not only in their intact structure as a macronutrient, but more so when broken down into their peptide constitutes. By extracting and isolating valuable compounds such as peptides, collagen or hydroxyproline from the by-product structures, the major part of fileting by-products can be utilized and is available for valorization into products (Siddiqui et al., 2023). Hereby likewise, the remaining lipid fractions can be considered for further utilization. Figure 1 indicates the major biomaterials that could be potentially extracted from fileting side streams.
Intensive research on bioactive peptides the past decades have lead to detection and characterization of a wide range of bioactive peptides (Pihlanto and Korhonen, 2003; Möller et al., 2008; Shahidi and Zhong, 2008). Bioactive peptides from marine origin with multiple positive effects on human health have been identified (Kim and Mendis, 2006; Kim and Wijesekara, 2010; Ozogul et al., 2021). In general, peptides display bioactivity at a chain length of 2–20 amino acids, and once released from the tightly packed protein structure, can exhibit their beneficial properties (Sun et al., 2021). Bioactive peptides were found to be rich in hydrophobic amino acids and determine their antioxidative and metal chelating activity (Mendis et al., 2005; Kim and Wijesekara, 2010). Moreover, the amino acid composition and distribution within the sequence influences functional properties such as the ability for emulsification and water holding capacity (Mendis et al., 2005). A commonly used method to acquire bioactive peptides from various by-product fractions is the application of enzymatic hydrolysis, hereby enzymatically deconstructing the proteins and release peptide chains. The possibility to generate fish protein hydrolysates including bioactive peptides from backbones, skins, heads and cut-offs from marine waste has been well-demonstrated previously (ŠliŽyte et al., 2009; Chalamaiah et al., 2012; Gajanan et al., 2016; Zamora-Sillero et al., 2018; Ozogul et al., 2021). A challenge for the application of marine peptides is their bitterness due to the presence of hydrophobic and alkaline amino acids (e.g., lysine, leucine) (Fan et al., 2019). Next to bitter taste, the bioactivity can be altered and decreased while passing through the gastrointestinal tract (GI), due to proteolytic degradation and the acidic environment. Here, innovative solutions such as the entrapment or encapsulation within different biomaterials (e.g., nanoliposomes) are applied to effective stabilize and increase the palatability of the peptides (Hosseini et al., 2017, 2021).
Collagen is one of the most abundant proteins and have a triple helical structure, with three polypeptide α-chains. Up-to date a total of 28 classes within the collagen family have been discovered (type I-XXVIII) (Ricard-Blum, 2011). The collagen superfamily includes multiple types, but common to all is the repeating sequence of (Gly-X-Y)n, indicating the repetition of proline (X) and hydroxyproline (Y) with Gly (glycine) being stringent in this sequence. Each member of the collagen superfamily includes at least one triple-helical domain (COL), being situated at the extracellular matrix (Zanaboni et al., 2000; Ricard-Blum and Ruggiero, 2005; Felician et al., 2018). Marine collagen is mainly found as type I collagen, whereas human collagen is prevalent (80–85%) in the form of type II (Felician et al., 2018). Marine collagen is regarded as a highly functional and useful material with potential for application in health-related sectors, including medical, pharmaceutical, cosmetic and the food industry (Salvatore et al., 2020). Especially marine collagen, in contrast to collagen from bovine or porcine origins, has the advantage of being appropriate for cultures restricting the consumption of bovine or porcine origin (Easterbrook and Maddern, 2008). This makes marine collagen attractive for a wide range of potential consumers (e.g., food applications) and patients (e.g., biomedical applications). Moreover, it is reported that the amino acid composition and biocompatibility of marine collagen is comparable to porcine or bovine origin, with glycine (< 30%) and hydroxyproline (35–48%) being the dominating compounds in the molecule (Yamada et al., 2014). According to Subhan et al. (2021), around 30% of marine side stream is rich in collagen. However, this will highly depend on e.g., the fish species, processing regimes and quality of obtained by-products. Moreover, the physicochemical characteristics such as hydration behavior and thermal properties can differ between cold water fish (e.g., Atlantic or baltic halibut, cod) and warm water fish (e.g., Pacific big-eye tuna, tilapia), which has to be considered for applications (Rose et al., 1988; Gauza-Włodarczyk et al., 2017). Marine collagen has been extracted from cold water fish by-product fractions such as skins, frames, heads and bones. Particularly, fish skins have been reported to be a good source to extract high yields of collagen with great potential for further utilization (Nagai and Suzuki, 2000; Sadowska et al., 2003; Kittiphattanabawon et al., 2005; Zelechowska et al., 2010; Sousa et al., 2020; Martins et al., 2022; Kendler et al., 2023). The source of collagen, type, the pre-treatment parameters, and the extraction methodologies adopted are the main factors which determine the properties of collagen such as molecular weight, amino acid profile, structure of the molecule, solubility properties and functional characteristics (Rajabimashhadi et al., 2023). Even though the major raw material used for extracting type I collagen is bovine (Salvatore et al., 2020), different health preferences, cultural, religious, and social stigma, has resulted in the exploration of alternate collagen raw material sources such as fish skin (Rajabimashhadi et al., 2023). Whereas, collagen from fish could be extracted from different side streams such as scale, bone, head, air bladder and viscera, skin remains the most important source (Felician et al., 2018; Maschmeyer et al., 2020; Subhan et al., 2021).
Hydroxyapatite (HAp), chemically a calcium phosphate mineral with the structure of Ca10 (PO4)6(OH)2 can be generated synthetically or is derived from biological sources such as bovine, coral, shells, algae or fish (Legeros and Legeros, 2008; Fernando et al., 2016; Mohd Pu'ad et al., 2019). HAp can be regarded as a bioceramic, showing great biocompatibility to human bones due to a similar Ca/P molar ratio of 1.67. This molar ratio indicates the amount of Ca+2 which is important for the growth and production of osteoblast cells, especially of significance for bone repair applications. HAp is hexagonal in its structure and when exposed to high temperatures (< 1,200°C) transforms into tricalcium phosphate (Ca3(PO4)2) (Khamkongkaeo et al., 2021). An advantage of natural (marine-derived) HAp over the synthetically produced HAp is the micro- and nano scale hierarchical structure, but also lower costs due to being generated out of waste materials. The chemical structure, characteristic and similarity to human hard tissue makes marine-derived HAp generated from fileting side streams an eco-friendly biomaterial (Khrunyk et al., 2020; Borciani et al., 2023). Fish are a good source of HAp, and previous studies on tilapia bones, fins and body parts have managed to generate HAp with similar Ca/P molar ratios to human HAp (Ahmad Fara and Abdullah, 2016; Khamkongkaeo et al., 2021). The primary by-product fractions after fileting are backbones, heads and fishbones, all of which contain HAp, which emphasizes the significant potential of further extracting HAp as a potential biomaterial.
Collagen extraction methods generally consists of three major steps. (1) pre-treatment stage consisting of separation of the target tissue, reduction of raw material size for increasing the surface area available for reaction, isolation of collagenous matter from non-collagenous matter for increasing the process efficiency, (2) treatment stage consisting of extraction of collagen peptides through hydrolysis of the protein matrix using chemical and enzyme aided process and finally the (3) recovery stage consisting of salt precipitation or dehydration (Rajabimashhadi et al., 2023). For extraction of cold water fish collagen the temperature should be kept between 4 and 10°C for minimizing the risk of denaturation of collagen peptides (Araujo et al., 2021; Luo et al., 2022; Son et al., 2022).
Collagen rich raw materials like skin usually contain a significant quantity of non-collagenous materials such as, fat, pigments, and non-collagenous proteins. Other raw materials used such as scales and bones also contain inorganic components such as ash contributed by minerals like calcium, zinc etc. (Schmidt et al., 2016; Ahmed et al., 2020). The initial process in pre-treatment of the raw materials is removing non-collagenous tissue adhered using mechanical means and reducing the size of the solid parts to facilitate higher surface area to volume ratio for better efficiency of the treatments to be followed (Safiya and Casparus, 2020). The non-collagenous matter present in the raw material could, if not properly eliminated, interfere with the extraction treatments and prevent proper breaking down of the collagen peptide bonds. Hereby significantly reducing the solubility of collagen peptides into the acid, alkali or enzymatic media used (Ahmed et al., 2020). The removal of the non-collagenous components are usually done under moderate conditions to prevent unnecessary breakdown of the collagen. For instance, the removal of non-collagenous protein is most commonly done using 0.05–0.1 M NaOH. At these concentrations, the collagen is not disturbed while most of the non-target protein is dissolved in the alkali (Hukmi and Sarbon, 2018). NaOH also contributes toward swelling of the tissue, therefore accelerating the rate of mass transfer during the extraction process (Ahmed et al., 2020). Fat is another important non-collagenous material which needs to be removed especially in the case of side streams from high fat fishes. The removal of fat is usually done with chemical treatment including acetone, 10–15% butanol and other alcohols or non-ionic detergents (Schmidt et al., 2016; Hukmi and Sarbon, 2018). Hard raw materials like bones and scales contain high levels of ash, contributed by the mineral content, and these need to be removed through a de-mineralization process. Calcium being the prominent mineral in these components, chelating agents like EDTA (Ethylene diaminetetraacetic acid) (0.5 M) and acids like HCl (0.5–1 M) are commonly used with minimal destruction of collagen (Ahmed et al., 2020). The decalcification process has also been found to make the raw material more permeable, as it increase the surface area and also simultaneously partially hydrolyzing collagen breaking the cross links while maintaining the collagen peptide chains intact, aiding the extraction process (Schmidt et al., 2016). The pre-treatment procedures are also reported to cause leaching of collagen peptides resulting in loss of yield (Bai et al., 2017) and hence are performed in batches with different combinations of treatment time, temperature, reactant concentration and raw material to chemical ratio (Muralidharan et al., 2013; Hukmi and Sarbon, 2018). Table 1 indicates different pre-treatment conditions followed for cold water fish collagen extraction.
The collagen extraction process depends on the solubility of collagen molecules into the extraction medium making it suitable for isolation and further recovery through filtration/dehydration. As the collagen molecule is water insoluble due to its triple helix structure, secured with inter/intra molecular hydrogen bonds, specific extraction techniques are required to solubilize and isolate collagen (Jafari et al., 2020). Depending on the solubility, collagen could be classified according to the extraction method utilized as acid, enzyme, or salt soluble (Oliveira et al., 2021).
Collagen extraction based on the saline solubility properties or salting out (SO) method utilizes neutral salts like NaCl and guanidine hydrochloride to precipitate collagen from the pre-treated raw material (Schmidt et al., 2016). In this method the salt concentration is gradually increased, which boosts the ionic strength of the solution, hereby increasing the solubility of the collagen (El Blidi et al., 2021). The collagen could be further isolated through dialysis or using ultrafiltration membranes (Saallah et al., 2021). The major drawback of this method is the lower yield, time consumption and resource dependency compared to other methods (Cao et al., 2019; Hong et al., 2019).
Acid hydrolysis can be employed for collagen extraction using organic acids such as acetic, lactic, citric, formic, and tartaric acid other than mineral acids like hydrochloric acid and sulfuric acid (Fassini et al., 2021). In this method, the acids involved, break down (depolymerize) the triple helix structure of the complex collagen molecules into shorter ones within a molecular weight range of 0.3–8 kDa (Bai et al., 2017). The acidic conditions also present an environment, predominant with positive charge, which further facilitates the aversion between the tropocollagen, accelerating the solubilization of collagen within the acid medium (Benjakul et al., 2012). Important parameters that influence the efficiency of the acid aided process are temperature, concentration, extraction period and solid to solvent ratio. The most common acid used for collagen extraction is acetic acid with a concentration of 0.5–1 M and for a time period of 24–48 h (Blanco et al., 2019; Jafari et al., 2020; Mohamad Razali et al., 2023). Blanco et al. (2019) also deliberated on the effect of acid concentration and process time on the choice of the optimum reaction temperature. Jafari et al. (2020) considered raw material to acid ratio as an important parameter to be considered while treating with acid for the extraction of collagen. They observed that an increase in the volume of the acidic solution enhances the free proton-amino acid interactions, which further speeds up fragmentation of the collagen molecules. The acid extraction method is also considered more effective than salt based extraction, resulting in better yield (Hong et al., 2019).
The low yield associated with the acid based method could be due to the partial solubility of collagen in the acid, including the likely formation of covalent crosslinks through condensation of aldehyde groups (Sinthusamran et al., 2013). This has led to the application of enzymes in collagen extraction which facilitate increased selectivity and lower deteriorative effect toward the amino acids in the collagen molecule (Hong et al., 2019). Different enzymes such as Flavourzyme, Alcalase®, papain, trypsin and pepsin could be employed (Schmidt et al., 2016). The most common type of enzyme employed for collagen extraction is pepsin (Nalinanon et al., 2010), which enables the breaking of peptide bonds among the collagen triple helix, facilitating the release of collagen molecules, increasing the total extraction yield (Schmidt et al., 2016; Bagus Fajar Pamungkas et al., 2019; Ahmed et al., 2020). The optimum temperature for pepsin reaction is estimated to be 60°C, and it is often used in combination with an acetic acid treatment to enhance the efficiency of the process resulting in a higher yield, quality, and free amine concentration with a lower denaturation temperature (Zhao et al., 2015; Delgado et al., 2017). Almost identical duration (24–48 h) and solvent to raw materials ratios (10–60 ml/g) are used in such combinations with an enzyme concentration between 1 and 1.5% (Hukmi and Sarbon, 2018; Cao et al., 2019). Even though the enzyme aided process is safe and sustainable compared to other chemical methods (Zhang et al., 2020), it is considered expensive due to the cost of enzymes (Schmidt et al., 2016). Table 2 indicates the conventional collagen extraction conditions followed for cold water fish side streams.
The extraction methods play a significant role in determining the quality of the collagen. The general alkali-acid-enzyme based collagen isolation methods have some inherent shortcomings such as multistage operational requirement, energy consumption, water dependency, chemical residues, extremely low yield, and non-ecofriendly nature (Ameer et al., 2017; Jafari et al., 2020; Faraz et al., 2021; Phon et al., 2023). Application of industry 4.0 technologies such as big data analysis, artificial intelligence, sensor based monitoring and advanced image processing in combination with green recovery methods could enhance the seafood side stream valorization process with respect to efficiency and lower carbon footprint (Hassoun et al., 2023). In this scenario, advanced eco-friendly or green technologies are proposed for effective extraction and isolation of collagen and collagen form marine resources resulting in minimal impact to the environment with emphasis on sustainability (Venugopal et al., 2023). Some of the emerging technologies considered for green extraction of collagen are Ultrasound-assisted extraction (UAE), Subcritical water extraction (SWE), High-pressure processing (HPP), and Microwave-Assisted Extraction (MAE). Figure 2 indicates the potential green technologies that could be adapted for collagen extraction from fileting side streams.
UAE is a non-thermal physical method which utilizes ultrasonic sound waves above 20 kHz to disintegrate macromolecules to simpler forms and therefore enhancing the reaction interface between the solvents and the targeted substrates (Faraz et al., 2021). In collagen extraction processes, the applied UAE, generally in the range of 200–500 kHz, destroys the complex collagen matrix through cavitation and facilitate collagen production. The sound waves traveling through the solvent creates alterations in pressure and temperature, enhancing the transfer rate of the solvent into the matrix. Hereby resulting in the isolation of collagen molecules (Torres et al., 2017). UAE could enhance the collagen extraction efficiency when utilized in combination with acidic or enzymatic methods. An acidic pre-treatment is observed to weaken the collagen matrix by removing non-collagenous proteins and weakens the peptide bonds further increasing the efficiency of the ultrasound frequencies (Mhd Sarbon et al., 2013). Non-protein denaturing frequencies of ultrasound could be used in combination with enzymes to enhance the extraction efficiency as in the case of acidic process where the enzymes partially hydrolyzing and weakening the peptide bonds increasing the extraction efficiency of the process (Nadar and Rathod, 2017). Lee et al. (2022) successfully utilized UAE in combination with enzyme application for preparing collagen hydrolysate from Alaska pollock skin (Theragra chalcogramma). They used 1% Alcalase® along with an ultrasound frequency of 20 kHz with 80% amplitude, for a period of 30 min. The extracted collagen fraction exhibited enhanced antioxidant properties when compared to the control. Moreover, Kendler et al. (2023) utilized UAE with or without enzymatic hydrolysis prior the extraction to generate collagen from different European plaice by-product fractions. Several studies have demonstrated the feasibility of UAE to extract marine collagen from different origins (Ali et al., 2018; Shaik et al., 2021; Lu et al., 2023).
SWE uses the changes in properties of water, generated in response to variations in temperature and pressure. It is the state that water attains between the temperature 100–374°C (Boiling and critical temperature of water) under a corresponding pressure range of 1–22.1 MPa (Zhang et al., 2020). The subcritical stage of water is observed to be capable of disrupting the molecule-molecule interaction by reducing the activation energy for desorption and simultaneously enhancing water penetration into the protein matrix. Hereby resulting in separation of protein molecules like collagen, based on polarity (Pillot et al., 2019). These intricate subcritical pressure and temperature combinations could result in partial or complete hydrolysis of collagen, resulting in collagen within a limited period of time (Jung et al., 2014; Park et al., 2015). Melgosa et al. (2021) utilized SWE to extract collagen from Atlantic codfish frames with an extraction yield of 5-% and 100% of protein recovery rate at a temperature-pressure combination of 90°C and 100 bar. The SWE extracted collagen showed highest anti-inflammatory properties. Similar attempts were made by Sousa et al. (2020) from cod skin, where SWE was employed for collagen extraction with a yield of 13.8%.
HPP is considered to be a sustainable, non-thermal, green technology which utilizes hydrostatic pressure within a range of 100–1,500 MPa (Naderi et al., 2017). Intermolecular bonds are disrupted under high pressure affecting the structure of the proteins making them more susceptible to extraction procedures without compromising on quality (Yang and Powers, 2016). The entire HPP process is accomplished through a system comprising of pressure vessel, pressure generation system, temperature, device management, pressure valves and a material handling system (Naderi et al., 2017). HPP has been utilized in the pre-treatment stage during collagen extraction from fish skins where acid and skin where packed in plastic bags and then placed in the high-pressure chamber (Jaswir et al., 2017; Yusof et al., 2017). This facilitated better acid penetration into the skin, breaking the non-covalent bond in the gelatin molecule. Hereby enabling swelling and subsequent extraction (Yusof et al., 2017). Noor et al. (2021) observed that during the HPP pre-treatment process, acid treatment at 10°C speeds up the weakening of crosslinks within the acid molecule and further extraction at 45°C in water accelerates the collagen hydrolysis. The extraction potential from fish skin were enhanced up to 25% along with additional advantages such as a solid gel forming capacity and elevated melting point when HPP was employed in the pre-treatment process (Jaswir et al., 2017). It was also observed that in the HPP aided collagen extraction process, despite of the extraction time and pressure conditions, for obtaining a high fish collagen yield, a higher volume of acid is required in comparison with mammalian sources due to the lower imino acid concentration resulting to its faster denaturation at lower temperatures (Yusof et al., 2017).
MAE is a thermal process which applies electromagnetic waves within a frequency of 300 MHz−300 GHz to elevate the temperature in order to evaporate fluids. Which causes cell deterioration releasing intracellular-bound compounds (Noor et al., 2021). In comparison with other green processes like ultrasound, MAE is observed to produce better yields with less consumption of solvents (Abdel-Aal et al., 2014). It also has advantages against conventional extraction processes such as reduced time of extraction, higher precision, and preservation of extract qualities (Delazar et al., 2012; Azmir et al., 2013). MAE has previously been successful in extracting collagen from European plaice by-products (Kendler et al., 2023) as well as chitin and chitosan from shrimp shells (El Knidri et al., 2019; Santos et al., 2019).
DES are suggested as possible alternatives to traditional ionic fluids and organic solvents (de Morais et al., 2015; van Osch et al., 2015; Zhao et al., 2015). They have many advantages against the conventional ones like biocompatibility (Wagle et al., 2014), convenience in preparation (Passos et al., 2016) eco-friendliness (Pérez and Ramón, 2015) and economical (Li et al., 2013). DES could be prepared by mixing hydrogen bond donor compounds (HBD) or hydrogen bond acceptor (HBA) compounds (Gouveia et al., 2016; Li et al., 2016) which makes the melting point of the mixture lower than the individual constituents (Carriazo et al., 2012; Tang et al., 2012; García-Argüelles et al., 2013). Choline chloride (ChCl) is one such widely used DES which is biodegradable and non-hazardous (Florindo et al., 2014). Bisht et al. (2021) utilized a DES prepared by mixing a solution of Urea: Lactic acid (Molar ratio 1:2) for extracting type-I collagen from Atlantic codfish (Gadus morhua). Compared to acetic acid, DES improved the extraction yield and quality of the collagen.
PEF is a non-thermal process which uses DC (Direct Current) voltage pulses in a range from micro to milliseconds through the intended medium placed between two electrodes, resulting in an electric field with an intensity proportional to the voltage applied and the distance between the electrodes (Luengo et al., 2016). The PEF process, when compared to conventional processes offers advantages such as swift and constant transmission rate, high penetration rate, reduced extraction time, reduced consumption of energy, operational continuity, and preservation of the innate properties of the extract (Dellarosa et al., 2017; Salvia-Trujillo et al., 2017). The PEF technology could be utilized for collagen extraction from seafood side streams as studies have demonstrated that a PEF ranging from 100–300 V/cm to 20–80 kV/cm can result in disintegrated cell membranes and thus enhance the extraction process (Barba et al., 2015; Puértolas and Barba, 2016). He et al. (2019) successfully utilized PEF of field strength of 20 kV/cm, and pulse number of 8 in combination with pepsin (1%) and raw material to liquid ratio of 1:10 for extracting collagen from fish bone side streams.
Nanomaterials (< 100 nm) are the key constituents in the cutting-edge science of nanotechnology which utilizes their properties such as high surface area, reactivity rate, absorption and strength compared to their massive counterparts enabling their incorporation into matrixes resulting in increased mechanical, physical and chemical properties of the respective composites (Khan et al., 2022). Evolution of bio-polymers for sustainable replacement of petroleum-based plastics has been fueled by the incorporation of nanomaterials into the biopolymer matrix for enhancing the barrier and physical properties (Garcia et al., 2018). But the drawbacks of inorganic nano-materials such as non-biodegradability and toxicity (Khalid et al., 2020) has inspired researchers to explore the possibilities of manufacturing nanomaterials from organic sources to overcome these obstacles. Seafood side streams like fileting discards such as frames and skin are an excellent source of nano-scalable biocomponents like collagen and hydroxyapatite. These biomaterials have a significant potential as nano-fillers in bio-polymer composites (Saini et al., 2018). Nano-collagen is basically the normal collagen reduced to nano-scale, which is below 100 nm. Such nano scaling of collagen enables it to be used as an ideal material for 3D printing bio-compatible materials due to the high surface area to size ratio and penetrability (Naskar and Kim, 2020). Nano-collagen fibers could be used for reinforcing biopolymers, making them breakage resistant with good elastic properties with potential for tissue engineering (Lo and Fauzi, 2021). As previously mentioned HAp is a potential material for biomedical applications. For scaffolings, bone fillers and orthopedic applications the formation into nano-scale is of particular interest. Here, powders, particles and rods in nano-scale generated from diverse marine by-products have been previously studied and listed in the review of Borciani et al. (2023).
Different techniques like Electrospinning (Law et al., 2017), Nano emulsion (Musazzi et al., 2018), Electrospray Deposition (Nagarajan et al., 2014), Milling (Sanz-Fraile et al., 2020) and Ultrasonication (Wu et al., 2021) are used for nano-scaling collagen molecules. Researchers have extracted nano-collagen from fish swim bladers by applying ultrasonication to the conventional acid extracted collagen (Trilaksani et al., 2020). Ultrasonication was applied at 200 watts, 20 kHz, under a temperature of 15°C with four different time periods such as 60, 90, 120, and 150 min followed by magnetic agitation while 96% ethanol being dripped at a ratio of 1:1 (v/v) at 1,500 rpm for 30 min at 15°C. The ultrasonication for 150 min produced collagen with a particle size of 404.1 nm with 0.446 polydispersity index. Dachi et al. (2020) applied ball milling process to extract nano-scale collagen from fish bone. Jia et al. (2020) used electrospinning technique to prepare fish collagen and polycaprolactone composite nanofibers in three different weight ratios (9:1, 7:3, and 5:5). The composite was prepared as a 12% solution in hexafluoro isopropanol and electrospun using a 1 mL syringe with a 27-gauge needle assisted by a syringe pump under 0.4 mL/h feeding rate with 45–55% humidity, electrospinning voltage of 9 kV DC and a temperature of 21–22°C.
Nano-Hydroxyapatite (n-HAP) has been demonstrated to be synthesized from natural as well as synthetic resources (Sadat-Shojai et al., 2013). The nano-size and the associated morphological features like increased surface area enhances the absorption and other functions of n-HAP compared to its normal form (Yan et al., 2016; Hokmabad et al., 2018). But the fragile nature, hazardous manufacturing process and unbalanced stoichiometric ratio of the synthetic n-HAP has encouraged the industry to explore the natural resources like fish bone (Zhou and Lee, 2011). Tri et al. (2020) prepared n-HAP from salmon bones employing hydrothermal and calcination methods with hydrothermal time/temperature parameters such as 8 h/120°C and calcination time/temperature parameters such as 2 h/800°C. The prepared n-HAP were observed to possess a spherical shape with 30–60 nm particle size, 13.8 m2.g−1 of BET surface area, 0.018 cm3.g−1 pore volume and 24.8 Å pore diameter. The n-HAP also reported a Ca/P molar ratio of 1.64 which was identical with the natural. Venkatesan et al. (2015) utilized alkaline hydrolysis for extracting n-HAP from salmon bones. The pre-treated bones were treated with 2 M NaOH at 200°C for a time period of 1 h. The process was repeated several times and the resultant n-HAP was isolated through centrifugation, washing, and drying. The n-HAP was observed to be in the size range of 6–37 nm with good biocompatibility.
The multitude of beneficial effects of marine derived bioactive peptides, such as e.g., their antithrombotic, anticancer, antimicrobial and antihypertensive properties have been previously identified by applying mainly in vitro biochemical assays (Ngo et al., 2012). To employ these properties in the human body, and being available at the cell location, peptides need to pass the gastrointestinal digestion (GI) in their intact form. However, peptides are highly susceptible to the GI digestion, hereby losing their structural integrity and further bioactive properties (Mohan et al., 2015). Encapsulation of susceptible compounds, such as bioactive peptides have been introduced to help preserving their functionality, in order to keep their physiological effects in the human body (Mohan et al., 2015; Sun et al., 2021). The encapsulation of bioactive peptides can increase multiple desired functions of the peptides, such as their bioaccessibility, bioavailability as well as the general digestibility of those peptides. Moreover, sensory properties can be increased, due to camouflaging of bitter and general fishy taste of marine peptides which often occurs after hydrolysis (Hosseini et al., 2021). Hereby, their general applicability in food and nutraceutical products can be increased.
Due to its preferable biological properties, such as biodegradability and low antigenicity as well as biocompatibility and safety, collagen has become an important resource in medical applications (Lee et al., 2001; Zhang et al., 2022). In addition, Lim et al. (2019) reports especially good water solubility, extractability as well as low immunogenicity and production costs for marine collagen. Moreover, review articles have emphasized on the specific benefits of applying marine collagen in diverse biomedical applications, such as applications in skin, vascular, dental, corneal and cartilage tissue engineering and regeneration, wound healing and skin repair (Lee et al., 2001; Felician et al., 2018; Lim et al., 2019; Lin et al., 2021).
Collagen's water holding capacity is essential for it being an effective and functional component in cosmetics, hereby contributing to the regulation of skin hydration (Alves et al., 2017). In their study, they concluded that collagen extracted from cod and salmon showed a good ability to retain water. In addition, they report that by exposing topical collagen in a human dermis reconstruction and investigating molecular markers for inflammation and irritation, no irritations were shown. Moreover, studies have shown that marine collagen peptides can be used for skin repair, showing positive effects skin texture, firmness and hydration (Lim et al., 2019).
Marine collagen has found its way into the food and beverage market already and it can be expected that the number of products with marine collagen as ingredient will rise in the future due to its current popularity. When looking into current products on the market, marine collagen is found in drinks (e.g., hydra.sci; B'EAU; PureWild; COLLAB, etc. …) and in diverse food supplements (e.g., Vild Nord; INLIFE; Edible Earth; etc. …). Due to its functionality and bioactive properties, collagen can be applied as a natural food additive. Moreover, studies reported the benefits of nano-encapsulating marine collagen to preserve the biological functionality and increase the stability of the compounds, especially important when applied in food products (e.g., fluctuating temperature, pH, …) (Mosquera et al., 2014).
The main field of application and potential for increased utilization of marine-derived HAp lies within biomedical applications, including bone tissue engineering. Previous studies have shown HAp's effect as a coating material for bone implant fixations as well as its strong connection to bone, caused by the production of bone-like apatite (Soballe et al., 1993). The study of Fernando et al. (2016) on chitosan silver/hydroxyapatite composite coatings for biomedical applications reported good bioactive, biocompatible and high osteoconductive properties of HAp. Maschmeyer et al. (2020) refer to biogenic (biological) HAp, as bio-HA and describes fish bones to be the major natural source of calcium phosphate, which constitutes mainly of inorganic matter and thereof a majority in the form of HAp. Moreover, Piccirillo et al. (2015) extracted HAp derived from cod fishbones. They concluded that HAp-based materials are suitable for commercial biomedical products due to great density at high sintering temperatures (1,250°C), good bioactive properties, no cytotoxicity and being compatible to blood (hemocompatibility). Good overall properties, biocompatability and possibilities for applications within the biomedical field (e.g., bioceramics as bone graft substitute; scaffolding materials within dentistry or orthopedics, …) of HAp derived from different fish bones were reported in several studies (Pal et al., 2017; Mohd Pu'ad et al., 2019; Khamkongkaeo et al., 2021; Venkatesan and Anil, 2021; Öteyaka et al., 2023).
As previously elaborated, a large number of highly valuable components are left as side streams after fileting of fish. So far, the marine seafood industry has been successful in implementing processes to further utilize those side streams as pet or animal feed, but has not yet managed to up-cycle these fractions into high-value products. To up-cycle side stream fractions by extraction of high-value components can be regarded as one of the greatest potentials, but also challenges in the marine seafood industry. Hanachi et al. (2022) refers to up-cycled products as second-degree valuated products, which includes the enhancement of biomaterials into products for human consumption, specifically pharmaceuticals, dietary or cosmetic products. The characteristics and positive effects of marine peptides on human health have been reported previously (Ngo et al., 2012; Ghanbari, 2019) and can be regarded as having a good potential in preventing diseases, as functional food ingredients and may help maintaining physical health when consumed frequently (Hanachi et al., 2022). Studies have also demonstrated through in silico analysis that the extracted peptides from seafood sources exhibits specific enzyme inhibiting bioactive properties which could be utilized in designing natural and non-toxic therapeutic measures (Jayant Singh et al., 2023). Moreover, further transformation of these up-cycled components into nano-biomaterials and encapsulated products offers the chance to enhance their functionality and positive effect on the human body. The bioactivity efficiency of these bio-nanocomponents could be enhanced if emphasis on molecular modeling prior to in-vivo or in-vitro applications is given in future research (Javan Nikkhah and Thompson, 2021).
The scale-up for green extraction procedures from lab to pilot/industry-scale has previously been investigated especially for the extraction of plant bioactive compounds. Belwal et al. (2020) state an increasing trend of research focusing on the scale-up of so called non-conventional extraction techniques, including e.g., UAE as well as MAE. Moreover, Belwal et al. (2020) highlights that the scale-up from bench to pilot/industry-scale keeps the efficient extraction for most processes. However, this efficiency will be necessary in order to keep extractions as short as possible and as long as necessary to guarantee high quality extracts, while limiting the energy consumption. It is crucial that next to process scalability, economic analysis must be considered. One great challenge for the utilization of marine by-products remains the question of how to guarantee stable quality and supply of fractions. For industry to refine by-products into the desired biomaterials, it is of utmost importance to have a stable supply and the same quality of products (Herrero and Ibañez, 2018). The application of green technologies becomes more relevant in this aspect as it influences the quality and reliability of the extracted components, especially those finding application in biomedical fields (Ozogul et al., 2021). As previously highlighted by Rustad et al. (2011), the reproducibility, characteristics of the by-product fraction as well as feasibility for industrial processes are major challenges to further up-cycle by-products into high value compounds for the market. This leads to an inconstant supply, which makes it difficult to establish a market with competitive prices, compared to synthetically produced compounds, which often pose the cheaper option (Olsen et al., 2014). Only by overcoming these known obstacles, will it be possible to introduce marine by-products into the markets. More awareness of waste reduction as well as the high quality of these fractions has led the industry to slowly move toward the holistic utilization of marine resources. However, it requires all stakeholders (governments, fishing industries, consumers, producers and sellers) of the respective countries to pull together for an increased utilization of valorized products for human consumption.
The sustainability goals setforth by the United Nations insists on maximum utilization of available resources limiting discards from the point of generation to consumption. Re-entry of valuable nutrients as well as biomaterials into human consumption trail through valorization of the respective side streams is a significant procedure to be adapted by the fishing industry. The cold water fish fileting side streams is such an important resource which provides unlimited valorization options in the form of protein and biominerals. By utilizing fileting by-products to generate and up-cycle biomaterials, a significant amount of solid side streams is valorized and re-enters the value chain for humans. The conventional extraction methods of these biocomponents when gradually replaced by greener processes shall provide a complete sustainable technology frame work for the industry to adapt inlight of the growing sustainable and blue-bio-economy concept. The technologies and resources are available to generate environmentally friendly, high value products for diverse sectors. However, big investments to introduce these green technologies, including appropriate facilities, adequate storage and availability of raw materials pose a big challenge, which has yet not been overcome, but will be necessary to reach the UN sustainability goals.
SK: Conceptualization, Writing—original draft, Writing—review & editing. AS: Conceptualization, Writing—original draft, Writing—review & editing. TR: Conceptualization, Funding acquisition, Supervision, Writing—review & editing.
The author(s) declare financial support was received for the research, authorship, and/or publication of this article. This article is part of the project PROFIUS (Preservation of underutilized fish biomasses for improved quality, stability, and utilization) funded by ERA-NET Co-fund on Blue Bioeconomy (BlueBio)—Unlocking the Potential of Aquatic Bioresources.
The authors declare that the research was conducted in the absence of any commercial or financial relationships that could be construed as a potential conflict of interest.
All claims expressed in this article are solely those of the authors and do not necessarily represent those of their affiliated organizations, or those of the publisher, the editors and the reviewers. Any product that may be evaluated in this article, or claim that may be made by its manufacturer, is not guaranteed or endorsed by the publisher.
Abdel-Aal, el-S. M., Akhtar, H., Rabalski, I., and Bryan, M. (2014). Accelerated, microwave-assisted, and conventional solvent extraction methods affect anthocyanin composition from colored grains. J. Food Sci. 79, C138–C146. doi: 10.1111/1750-3841.12346
Ahmad Fara, A. N. K., and Abdullah, H. Z. (2016). Influence of calcination temperature on the microstructure and crystallographic properties of hydroxyapatite from black tilapia fish scale. Mater. Sci. For. 840, 151–155. doi: 10.4028/www.scientific.net/MSF.840.151
Ahmed, M., Verma, A. K., and Patel, R. (2020). Collagen extraction and recent biological activities of collagen peptides derived from sea-food waste: a review. Sustain. Chem. Pharm. 18, 100315. doi: 10.1016/j.scp.2020.100315
Ali, A. M. M., Kishimura, H., and Benjakul, S. (2018). Extraction efficiency and characteristics of acid and pepsin soluble collagens from the skin of golden carp (Probarbus Jullieni) as affected by ultrasonication. Proc. Biochem. 66, 237–244. doi: 10.1016/j.procbio.2018.01.003
Alves, A. L., Marques, A. L. P., Martins, E., Silva, T. H., and Reis, R. L. (2017). Cosmetic potential of marine fish skin collagen. Cosmetics 4, 39. doi: 10.3390/cosmetics4040039
Ameer, K., Bae, S.-W., Jo, Y., Lee, H.-G., Ameer, A., and Kwon, J.-H. (2017). Optimization of microwave-assisted extraction of total extract, stevioside and rebaudioside-A from Stevia rebaudiana (Bertoni) leaves, using response surface methodology (RSM) and artificial neural network (ANN) modelling. Food Chem. 229, 198–207. doi: 10.1016/j.foodchem.2017.01.121
Araujo, J., Sica, P., Costa, C., and Márquez, M. C. (2021). Enzymatic hydrolysis of fish waste as an alternative to produce high value-added products. Waste Biomass Valor. 12, 847–855. doi: 10.1007/s12649-020-01029-x
Azmir, J., Zaidul, I. S. M., Rahman, M. M., Sharif, K. M., Mohamed, A., Sahena, F., et al. (2013). Techniques for extraction of bioactive compounds from plant materials: a review. J. Food Eng. 117, 426–436. doi: 10.1016/j.jfoodeng.2013.01.014
Bagus Fajar Pamungkas, S., Agnes, M., and Retno, I. (2019). Characterization of the acid- and pepsin-soluble collagens from haruan (Channa striatus) scales. Pakistan J. Nutr. 18, 324–332. doi: 10.3923/pjn.2019.324.332
Bai, C., Wei, Q., and Ren, X. (2017). Selective extraction of collagen peptides with high purity from cod skins by deep eutectic solvents. ACS Sustain. Chem. Eng. 5, 7220–7227. doi: 10.1021/acssuschemeng.7b01439
Barba, F. J., Parniakov, O., Pereira, S. A., Wiktor, A., Grimi, N., Boussetta, N., et al. (2015). Current applications and new opportunities for the use of pulsed electric fields in food science and industry. Food Res. Int. 77, 773–798. doi: 10.1016/j.foodres.2015.09.015
Bechtel, P. J. (2003). Properties of different fish processing by-products from Pollock, Cod and Salmon. J. Food Process. Preserv. 27, 101–116. doi: 10.1111/j.1745-4549.2003.tb00505.x
Belwal, T., Chemat, F., Venskutonis, P. R., Cravotto, G., Jaiswal, D. K., Bhatt, I. D., et al. (2020). Recent advances in scaling-up of non-conventional extraction techniques: Learning from successes and failures. TrAC Trends Anal. Chem. 127, 115895. doi: 10.1016/j.trac.2020.115895
Benjakul, S., Nalinanon, S., and Shahidi, F. (2012). “Fish collagen,” in Food Biochemistry and Food Processing, ed B. K. Simpson, 365–387. doi: 10.1002/9781118308035.ch20
Bisht, M., Martins, M., Dias, A. C. R. V., Ventura, S. P. M., and Coutinho, J. A. P. (2021). Uncovering the potential of aqueous solutions of deep eutectic solvents on the extraction and purification of collagen type I from Atlantic codfish (Gadus morhua). Green Chem. 23, 8940–8948. doi: 10.1039/D1GC01432C
Blanco, M., Vázquez, J. A., Pérez-Martín, R. I., and G. Sotelo, C. (2019). Collagen extraction optimization from the skin of the small-spotted catshark (S. canicula) by response surface methodology. Mar. Drugs 17, 40. doi: 10.3390/md17010040
Borciani, G., Fischetti, T., Ciapetti, G., Montesissa, M., Baldini, N., and Graziani, G. (2023). Marine biological waste as a source of hydroxyapatite for bone tissue engineering applications. Ceram. Int. 49, 1572–1584. doi: 10.1016/j.ceramint.2022.10.341
Cao, J., Duan, Q., Liu, X., Shen, X., and Li, C. (2019). Extraction and physicochemical characterization of pepsin soluble collagens from golden pompano (Trachinotus blochii) skin and bone. J. Aquat. Food Product Technol. 28, 837–847. doi: 10.1080/10498850.2019.1652216
Carriazo, D., Serrano, M. C., Gutiérrez, M. C., Ferrer, M. L., and del Monte, F. (2012). Deep-eutectic solvents playing multiple roles in the synthesis of polymers and related materials. Chem. Soc. Rev. 41, 4996–5014. doi: 10.1039/c2cs15353j
Carvalho, A. M., Marques, A. P., Silva, T. H., and Reis, R. L. (2018). Evaluation of the potential of collagen from codfish skin as a biomaterial for biomedical applications. Mar. Drugs 16. doi: 10.3390/md16120495
Chalamaiah, M., Dinesh kumar, B., Hemalatha, R., and Jyothirmayi, T. (2012). Fish protein hydrolysates: proximate composition, amino acid composition, antioxidant activities and applications: a review. Food Chem. 135, 3020–3038. doi: 10.1016/j.foodchem.2012.06.100
Chen, S., Chen, H., Xie, Q., Hong, B., Chen, J., Hua, F., et al. (2016). Rapid isolation of high purity pepsin-soluble type I collagen from scales of red drum fish (Sciaenops ocellatus). Food Hydrocol. 52, 468–477. doi: 10.1016/j.foodhyd.2015.07.027
Coscueta, E. R., Brassesco, M. E., and Pintado, M. (2021). Collagen-based bioactive bromelain hydrolysate from salt-cured cod skin. Appl. Sci. 11:8538. doi: 10.3390/app11188538
Dachi, K., Reveny, J., and Arianto, A. (2020). Isolation and characterization of collagen and nanocollagen from snakehead fish (Channa Striata) bone. Asian J. Pharm. Res. Dev. 8, 32–36. doi: 10.22270/ajprd.v8i5.791
de Morais, P., Gonçalves, F., Coutinho, J. A. P., and Ventura, S. P. M. (2015). Ecotoxicity of solvents cholinium-based deep eutectic. ACS Sustain. Chem. Eng. 3, 3398–3404. doi: 10.1021/acssuschemeng.5b01124
Delazar, A., Nahar, L., Hamedeyazdan, S., and Sarker, S. D. (2012). Microwave-assisted extraction in natural products isolation. Methods Mol. Biol. 864, 89–115. doi: 10.1007/978-1-61779-624-1_5
Delgado, L. M., Shologu, N., Fuller, K., and Zeugolis, D. I. (2017). Acetic acid and pepsin result in high yield, high purity and low macrophage response collagen for biomedical applications. Biomed. Mater. 12:65009. doi: 10.1088/1748-605X/aa838d
Dellarosa, N., Frontuto, D., Laghi, L., Dalla Rosa, M., and Lyng, J. G. (2017). The impact of pulsed electric fields and ultrasound on water distribution and loss in mushrooms stalks. Food Chem. 236, 94–100. doi: 10.1016/j.foodchem.2017.01.105
Easterbrook, C., and Maddern, G. (2008). Porcine and bovine surgical products: Jewish, Muslim, and Hindu perspectives. Arch. Surg. 143, 366–370; discussion 370. doi: 10.1001/archsurg.143.4.366
El Blidi, O., El Omari, N., Balahbib, A., Ghchime, R., El Menyiy, N., Ibrahimi, A., et al. (2021). Extraction methods, characterization and biomedical applications of collagen: a review. Biointerface Res. Appl. Chem. 11, 13587–13613. doi: 10.33263/BRIAC115.1358713613
El Knidri, H., Dahmani, J., Addaou, A., Laajeb, A., and Lahsini, A. (2019). Rapid and efficient extraction of chitin and chitosan for scale-up production: effect of process parameters on deacetylation degree and molecular weight. Int. J. Biol. Macromol. 139, 1092–1102. doi: 10.1016/j.ijbiomac.2019.08.079
Fan, W., Tan, X., Xu, X., Li, G., Wang, Z., and Du, M. (2019). Relationship between enzyme, peptides, amino acids, ion composition, and bitterness of the hydrolysates of Alaska pollock frame. J. Food Biochem. 43, e12801. doi: 10.1111/jfbc.12801
FAO (2022). The State of World Fisheries and Aquaculture 2022. Towards Blue Transformation. The State of World Fisheries and Aquaculture (SOFIA), Issue. Rome: FAO.
Faraz, N., Haq, H. U., Balal Arain, M., Castro-Muñoz, R., Boczkaj, G., and Khan, A. (2021). Deep eutectic solvent based method for analysis of Niclosamide in pharmaceutical and wastewater samples – A green analytical chemistry approach. J. Mol. Liq. 335, 116142. doi: 10.1016/j.molliq.2021.116142
Fassini, D., Wilkie, I. C., Pozzolini, M., Ferrario, C., Sugni, M., Rocha, M. S., et al. (2021). Diverse and productive source of biopolymer inspiration: marine collagens. Biomacromolecules 22, 1815–1834. doi: 10.1021/acs.biomac.1c00013
Felician, F. F., Xia, C., Qi, W., and Xu, H. (2018). Collagen from marine biological sources and medical applications. Chem. Biodivers. 15, e1700557. doi: 10.1002/cbdv.201700557
Fernando, S., McEnery, M., and Guelcher, S. A. (2016). 16 - Polyurethanes for bone tissue engineering,” in: Advances in Polyurethane Biomaterials, eds S. L. Cooper, and J. Guan (Woodhead Publishing; Elsevier), 481–501.
Florindo, C., Oliveira, F. S., Rebelo, L. P. N., Fernandes, A. M., and Marrucho, I. M. (2014). Insights into the synthesis and properties of deep eutectic solvents based on cholinium chloride and carboxylic acids. ACS Sustain. Chem. Eng. 2, 2416–2425. doi: 10.1021/sc500439w
Gajanan, P. G., Elavarasan, K., and Shamasundar, B. A. (2016). Bioactive and functional properties of protein hydrolysates from fish frame processing waste using plant proteases. Environ. Sci. Pollut. Res. Int. 23, 24901–24911. doi: 10.1007/s11356-016-7618-9
Garcia, C. V., Shin, G. H., and Kim, J. T. (2018). Metal oxide-based nanocomposites in food packaging: applications, migration, and regulations. Trends Food Sci. Technol. 82, 21–31. doi: 10.1016/j.tifs.2018.09.021
García-Argüelles, S., Serrano, M. C., Gutiérrez, M. C., Ferrer, M. L., Yuste, L., Rojo, F., et al. (2013). Deep eutectic solvent-assisted synthesis of biodegradable polyesters with antibacterial properties. Langmuir 29, 9525–9534. doi: 10.1021/la401353r
Gauza-Włodarczyk, M., Kubisz, L., Mielcarek, S., and Włodarczyk, D. (2017). Comparison of thermal properties of fish collagen and bovine collagen in the temperature range 298–670K. Mater. Sci. Eng. C, 80, 468–471. doi: 10.1016/j.msec.2017.06.012
Ghaly, A., Vasudevan Ramakrishnan, V., Brooks, M. S., Budge, S. M., and Dave, D. (2013). Fish processing wastes as a potential source of proteins, amino acids and oils: a critical review. J. Microb. Biochem. Technol. 5, 107–129. doi: 10.4172/1948-5948.1000110
Ghanbari, R. (2019). Review on the bioactive peptides from marine sources: indication for health effects. Int. J. Pept. Res. Ther. 25, 1187–1199. doi: 10.1007/s10989-018-9766-x
Gouveia, A. S. L., Oliveira, F. S., Kurnia, K. A., and Marrucho, I. M. (2016). Deep eutectic solvents as azeotrope breakers: liquid–liquid extraction and COSMO-RS prediction. ACS Sustain. Chem. Eng. 4, 5640–5650. doi: 10.1021/acssuschemeng.6b01542
Hanachi, A., Bianchi, A., Kahn, C. J. F., Velot, E., Arab-Tehrany, E., Cakir-Kiefer, C., et al. (2022). Encapsulation of salmon peptides in marine liposomes: physico-chemical properties, antiradical activities and biocompatibility assays. Mar. Drugs 20:249. doi: 10.3390/md20040249
Hassoun, A., Cropotova, J., Trollman, H., Jagtap, S., Garcia-Garcia, G., Parra-López, C., et al. (2023). Use of industry 4.0 technologies to reduce and valorize seafood waste and by-products: a narrative review on current knowledge. Curr. Res. Food Sci. 6, 100505. doi: 10.1016/j.crfs.2023.100505
He, G., Yan, X., Wang, X., and Wang, Y. (2019). Extraction and structural characterization of collagen from fishbone by high intensity pulsed electric fields. J. Food Process Eng. 42, e13214. doi: 10.1111/jfpe.13214
Herrero, M., and Ibañez, E. (2018). Green extraction processes, biorefineries and sustainability: recovery of high added-value products from natural sources. J. Supercrit. Fluids 134, 252–259. doi: 10.1016/j.supflu.2017.12.002
Hokmabad, V. R., Davaran, S., Aghazadeh, M., Alizadeh, E., Salehi, R., and Ramazani, A. (2018). A comparison of the effects of silica and hydroxyapatite nanoparticles on poly(ε-caprolactone)-poly(ethylene glycol)-poly(ε-caprolactone)/chitosan nanofibrous scaffolds for bone tissue engineering. Tissue Eng. Regener. Med. 15, 735–750. doi: 10.1007/s13770-018-0140-z
Hong, H., Fan, H., Chalamaiah, M., and Wu, J. (2019). Preparation of low-molecular-weight, collagen hydrolysates (peptides): current progress, challenges, and future perspectives. Food Chem. 301, 125222. doi: 10.1016/j.foodchem.2019.125222
Hosseini, S. F., Ramezanzade, L., and McClements, D. J. (2021). Recent advances in nanoencapsulation of hydrophobic marine bioactives: bioavailability, safety, and sensory attributes of nano-fortified functional foods. Trends Food Sci. Technol. 109, 322–339. doi: 10.1016/j.tifs.2021.01.045
Hosseini, S. F., Ramezanzade, L., and Nikkhah, M. (2017). Nano-liposomal entrapment of bioactive peptidic fraction from fish gelatin hydrolysate. Int. J. Biol. Macromol. 105(Pt 2), 1455–1463. doi: 10.1016/j.ijbiomac.2017.05.141
Hukmi, N. M. M., and Sarbon, N. M. (2018). Isolation and characterization of acid soluble collagen (asc) and pepsin soluble collagen (psc) extracted from silver catfish (pangasius sp.) skin. Int. Food Res. J. 25:1785–1791. Available online at: http://www.ifrj.upm.edu.my/25%20(05)%202018/(3).pdf (accessed October, 2023).
Jafari, H., Lista, A., Siekapen, M. M., Ghaffari-Bohlouli, P., Nie, L., Alimoradi, H., et al. (2020). Fish collagen: extraction, characterization, and applications for biomaterials engineering. Polymers 12, 2230. doi: 10.3390/polym12102230
Jaswir, I., Yusof, N., Jamal, P., and Jami, M. S. (2017). Novel Method for Gelatin Extraction of Various Local Fish Using High Pressure Processing (HPP). Int. Food Res. J. 533–539.
Javan Nikkhah, S., and Thompson, D. (2021). Molecular modelling guided modulation of molecular shape and charge for design of smart self-assembled polymeric drug transporters. Pharmaceutics 13, 141. doi: 10.3390/pharmaceutics13020141
Jayant Singh, P., Kumar Srivastava, S., and Batta, A. (2023). In silico study of myomere muscle proteins extracted from Indian walking catfish reveal angiotensin converting enzyme (ACE) inhibitory activity – a potential bioactive peptide. Food Chem. Adv. 4:100555. doi: 10.1016/j.focha.2023.100555
Jia, Z., Li, H., Cao, R., Xiao, K., Lu, J., Zhao, D., et al. (2020). Electrospun nanofibrous membrane of fish collagen/polycaprolactone for cartilage regeneration. Am. J. Transl. Res. 12, 3754–3766.
Jung, K. H., Choi, Y. C., Chun, J. Y., Min, S. G., and Hong, G. P. (2014). Effects of Concentration and reaction time of trypsin, pepsin, and chymotrypsin on the hydrolysis efficiency of porcine placenta. Kor. J Food Sci. Anim. Resour. 34, 151–157. doi: 10.5851/kosfa.2014.34.2.151
Kendler, S., Kobbenes, S. M. M., Jakobsen, A. N., Mukhatov, K., and Lerfall, J. (2023). The application of microwave and ultrasound technologies for extracting collagen from European plaice by-products. Front. Sustain. Food Syst. 7, 1257635. doi: 10.3389/fsufs.2023.1257635
Khalid, K., Tan, X., Mohd Zaid, H. F., Tao, Y., Lye Chew, C., Chu, D. T., et al. (2020). Advanced in developmental organic and inorganic nanomaterial: a review. Bioengineered 11, 328–355. doi: 10.1080/21655979.2020.1736240
Khamkongkaeo, A., Boonchuduang, T., Klysubun, W., Amonpattaratkit, P., Chunate, H. T., Tuchinda, N., et al. (2021). Sintering behavior and mechanical properties of hydroxyapatite ceramics prepared from Nile Tilapia (Oreochromis niloticus) bone and commercial powder for biomedical applications. Ceram. Int. 47, 34575–34584. doi: 10.1016/j.ceramint.2021.08.372
Khan, S., Mansoor, S., Rafi, Z., Kumari, B., Shoaib, A., Saeed, M., et al. (2022). A review on nanotechnology: properties, applications, and mechanistic insights of cellular uptake mechanisms. J. Mol. Liq. 348, 118008. doi: 10.1016/j.molliq.2021.118008
Khrunyk, Y., Lach, S., Petrenko, I., and Ehrlich, H. (2020). Progress in modern marine biomaterials research. Mar. Drugs 18, 589. doi: 10.3390/md18120589
Kim, S.-K., and Mendis, E. (2006). Bioactive compounds from marine processing byproducts – A review. Food Res. Int. 39, 383–393. doi: 10.1016/j.foodres.2005.10.010
Kim, S.-K., and Wijesekara, I. (2010). Development and biological activities of marine-derived bioactive peptides: a review. J. Funct. Foods 2, 1–9. doi: 10.1016/j.jff.2010.01.003
Kittiphattanabawon, P., Benjakul, S., Visessanguan, W., Nagai, T., and Tanaka, M. (2005). Characterisation of acid-soluble collagen from skin and bone of bigeye snapper (Priacanthus tayenus). Food Chem. 89, 363–372. doi: 10.1016/j.foodchem.2004.02.042
Law, J. X., Liau, L. L., Saim, A., Yang, Y., and Idrus, R. (2017). Electrospun collagen nanofibers and their applications in skin tissue engineering. Tissue Eng. Regener. Med. 14, 699–718. doi: 10.1007/s13770-017-0075-9
Lee, C. H., Singla, A., and Lee, Y. (2001). Biomedical applications of collagen. Int. J. Pharm. 221, 1–22. doi: 10.1016/S0378-5173(01)00691-3
Lee, J. E., Noh, S.-K., and Kim, M. J. (2022). Effects of enzymatic- and ultrasound-assisted extraction on physicochemical and antioxidant properties of collagen hydrolysate fractions from Alaska pollack (Theragra chalcogramma) skin. Antioxidants 11, 2112. doi: 10.3390/antiox11112112
Legeros, R. Z., and Legeros, J. P. (2008). “16 – hydroxyapatite,” in Bioceramics and Their Clinical Applications, ed T. Kokubo (Woodhead Publishing; Elsevier), 367–394.
Li, C., Li, D., Zou, S., Li, Z., Yin, J., Wang, A., et al. (2013). Extraction desulfurization process of fuels with ammonium-based deep eutectic solvents. Green Chem. 15, 2793–2799. doi: 10.1039/c3gc41067f
Li, G., Yan, C., Cao, B., Jiang, J., Zhao, W., Wang, J., et al. (2016). Highly efficient I2 capture by simple and low-cost deep eutectic solvents. Green Chem. 18, 2522–2527. doi: 10.1039/C5GC02691A
Lim, Y. S., Ok, Y. J., Hwang, S. Y., Kwak, J. Y., and Yoon, S. (2019). Marine collagen as a promising biomaterial for biomedical applications. Mar. Drugs 17:467. doi: 10.3390/md17080467
Lin, Z., Tao, Y., Huang, Y., Xu, T., and Niu, W. (2021). Applications of marine collagens in bone tissue engineering. Biomed. Mater. 16, 042007. doi: 10.1088/1748-605X/abf0b6
Lo, S., and Fauzi, M. B. (2021). Current update of collagen nanomaterials-fabrication, characterisation and its applications: a review. Pharmaceutics 13, 316. doi: 10.3390/pharmaceutics13030316
Lu, W.-C., Chiu, C.-S., Chan, Y.-J., Mulio, A. T., and Li, P.-H. (2023). Characterization and biological properties of marine by-product collagen through ultrasound-assisted extraction. Aquac. Rep. 29, 101514. doi: 10.1016/j.aqrep.2023.101514
Luengo, E., Martínez, J. M., Álvarez, I., and Raso, J. (2016). Effects of millisecond and microsecond pulsed electric fields on red beet cell disintegration and extraction of betanines. Ind. Crops Prod. 84, 28–33. doi: 10.1016/j.indcrop.2016.01.016
Luo, Q., Hossen, M. A., Zeng, Y., Dai, J., Li, S., Qin, W., et al. (2022). Gelatin-based composite films and their application in food packaging: a review. J. Food Eng. 313, 110762. doi: 10.1016/j.jfoodeng.2021.110762
Martins, E., Fernandes, R., Alves, A. L., Sousa, R. O., Reis, R. L., and Silva, T. H. (2022). Skin byproducts of Reinhardtius hippoglossoides (Greenland Halibut) as ecosustainable source of marine collagen. Appl. Sci. 12, 11282. doi: 10.3390/app122111282
Maschmeyer, T., Luque, R., and Selva, M. (2020). Upgrading of marine (fish and crustaceans) biowaste for high added-value molecules and bio(nano)-materials. Chem. Soc. Rev. 49, 4527–4563. doi: 10.1039/C9CS00653B
Melgosa, R., Marques, M., Paiva, A., Bernardo, A., Fernández, N., Sá-Nogueira, I., et al. (2021). Subcritical water extraction and hydrolysis of cod (Gadus morhua) frames to produce bioactive protein extracts. Foods 10, 1222. doi: 10.3390/foods10061222
Mendis, E., Rajapakse, N., and Kim, S.-K. (2005). Antioxidant properties of a radical-scavenging peptide purified from enzymatically prepared fish skin gelatin hydrolysate. J. Agric. Food Chem. 53, 581–587. doi: 10.1021/jf048877v
Mhd Sarbon, N., Badii, F., and Howell, N. K. (2013). Preparation and characterisation of chicken skin gelatin as an alternative to mammalian gelatin. Food Hydrocoll. 30, 143–151. doi: 10.1016/j.foodhyd.2012.05.009
Mohamad Razali, U. H., Ya'akob, H., Sarbon, N. M., Zainan, N. H., Dailin, D. J., and Zaidel, D. N. A. (2023). Improving collagen processing towards a greener approach: current progress. J. Chem. Technol. Biotechnol. 98, 1063–1082. doi: 10.1002/jctb.7332
Mohan, A., Rajendran, S. R. C. K., He, Q. S., Bazinet, L., and Udenigwe, C. C. (2015). Encapsulation of food protein hydrolysates and peptides: a review. RSC Adv. 5, 79270–79278. doi: 10.1039/C5RA13419F
Mohd Pu'ad, N. A. S., Koshy, P., Abdullah, H. Z., Idris, M. I., and Lee, T. C. (2019). Syntheses of hydroxyapatite from natural sources. Heliyon 5, e01588. doi: 10.1016/j.heliyon.2019.e01588
Möller, N. P., Scholz-Ahrens, K. E., Roos, N., and Schrezenmeir, J. (2008). Bioactive peptides and proteins from foods: indication for health effects. Eur. J. Nutr. 47, 171–182. doi: 10.1007/s00394-008-0710-2
Moreno, H. M., Montero, M. P., Gómez-Guillén, M. C., Fernández-Martín, F., Mørkøre, T., and Borderías, J. (2012). Collagen characteristics of farmed Atlantic salmon with firm and soft fillet texture. Food Chem. 134, 678–685. doi: 10.1016/j.foodchem.2012.02.160
Mosquera, M., Giménez, B., da Silva, I. M., Boelter, J. F., Montero, P., Gómez-Guillén, M. C., et al. (2014). Nanoencapsulation of an active peptidic fraction from sea bream scales collagen. Food Chem. 156, 144–150. doi: 10.1016/j.foodchem.2014.02.011
Muralidharan, N., Jeya Shakila, R., Sukumar, D., and Jeyasekaran, G. (2013). Skin, bone and muscle collagen extraction from the trash fish, leather jacket (Odonus niger) and their characterization. J. Food Sci. Technol. 50, 1106–1113. doi: 10.1007/s13197-011-0440-y
Musazzi, U. M., Franz,è, S., Minghetti, P., and Casiraghi, A. (2018). Emulsion versus nanoemulsion: how much is the formulative shift critical for a cosmetic product? Drug Deliv. Transl. Res. 8, 414–421. doi: 10.1007/s13346-017-0390-7
Nadar, S. S., and Rathod, V. K. (2017). Ultrasound assisted intensification of enzyme activity and its properties: a mini-review. World J. Microbiol. Biotechnol. 33, 170. doi: 10.1007/s11274-017-2322-6
Naderi, N., Pouliot, Y., House, J. D., and Doyen, A. (2017). High hydrostatic pressure effect in extraction of 5-methyltetrahydrofolate (5-MTHF) from egg yolk and granule fractions. Innovat. Food Sci. Emerg. Technol. 43, 191–200. doi: 10.1016/j.ifset.2017.08.009
Nagai, T., and Suzuki, N. (2000). Isolation of collagen from fish waste material — skin, bone and fins. Food Chem. 68, 277–281. doi: 10.1016/S0308-8146(99)00188-0
Nagarajan, U., Kawakami, K., Zhang, S., Chandrasekaran, B., and Unni Nair, B. (2014). Fabrication of solid collagen nanoparticles using electrospray deposition. Chem. Pharm. Bull. 62, 422–428. doi: 10.1248/cpb.c13-01004
Nalinanon, S., Benjakul, S., and Kishimura, H. (2010). Collagens from the skin of arabesque greenling (Pleurogrammus azonus) solubilized with the aid of acetic acid and pepsin from albacore tuna (Thunnus alalunga) stomach. J. Sci. Food Agric. 90, 1492–1500. doi: 10.1002/jsfa.3973
Naskar, A., and Kim, K. S. (2020). Recent advances in nanomaterial-based wound-healing therapeutics. Pharmaceutics 12, 499. doi: 10.3390/pharmaceutics12060499
Ngo, D.-H., Vo, T.-S., Ngo, D.-N., Wijesekara, I., and Kim, S.-K. (2012). Biological activities and potential health benefits of bioactive peptides derived from marine organisms. Int. J. Biol. Macromol. 51, 378–383. doi: 10.1016/j.ijbiomac.2012.06.001
Nilsuwan, K., Fusang, K., Pripatnanont, P., and Benjakul, S. (2022a). Properties and characteristics of acid-soluble collagen from salmon skin defatted with the aid of ultrasonication. Fishes 7:51. doi: 10.3390/fishes7010051
Nilsuwan, K., Patil, U., Tu, C., Zhang, B., and Benjakul, S. (2022b). Salmon skin acid-soluble collagen produced by a simplified recovery process: yield, compositions, and molecular characteristics. Fishes 7:330. doi: 10.3390/fishes7060330
Noor, N. Q. I. M., Razali, R. S., Ismail, N. K., Ramli, R. A., Razali, U. H. M., Bahauddin, A. R., et al. (2021). Application of green technology in gelatin extraction: a review. Processes 9:2227. doi: 10.3390/pr9122227
Oliveira, V. D. M., Assis, C. R. D., Costa, B. D. A. M., Neri, R. C. D. A., Monte, F. T. D., Freitas, H. M., et al. (2021). Physical, biochemical, densitometric and spectroscopic techniques for characterization collagen from alternative sources: a review based on the sustainable valorization of aquatic by-products. J. Mol. Struct. 1224, 129023. doi: 10.1016/j.molstruc.2020.129023
Olsen, R. L., Toppe, J., and Karunasagar, I. (2014). Challenges and realistic opportunities in the use of by-products from processing of fish and shellfish. Trends Food Sci. Technol. 36, 144–151. doi: 10.1016/j.tifs.2014.01.007
Öteyaka, M. Ö., Köse, E., Hoşgün, S., Aksu, S., Çinar, E., and Emiroglu, Ö. (2023). Chemical, structure and thermal characterisation of biowaste fish bone parts for orthopaedic coatings. Can. Metall. Q. 1–11. doi: 10.1080/00084433.2023.2259188
Ozogul, F., Cagalj, M., Šimat, V., Ozogul, Y., Tkaczewska, J., Hassoun, A., et al. (2021). Recent developments in valorisation of bioactive ingredients in discard/seafood processing by-products. Trends Food Sci. Technol. 116, 559–582. doi: 10.1016/j.tifs.2021.08.007
Pal, A., Paul, S., Choudhury, A. R., Balla, V. K., Das, M., and Sinha, A. (2017). Synthesis of hydroxyapatite from Lates calcarifer fish bone for biomedical applications. Mater. Lett. 203, 89–92. doi: 10.1016/j.matlet.2017.05.103
Park, S. H., Kim, J. H., Min, S. G., Jo, Y. J., and Chun, J. Y. (2015). Effects of ethanol addition on the efficiency of subcritical water extraction of proteins and amino acids from porcine placenta. Kor. J. Food Sci. Anim. Resour. 35, 265–271. doi: 10.5851/kosfa.2015.35.2.265
Passos, H., Tavares, D. J. P., Ferreira, A. M., Freire, M. G., and Coutinho, J. A. P. (2016). Are aqueous biphasic systems composed of deep eutectic solvents ternary or quaternary systems? ACS Sustain. Chem. Eng. 4, 2881–2886. doi: 10.1021/acssuschemeng.6b00485
Pérez, J. M., and Ramón, D. J. (2015). Synthesis of 3,5-disubstituted isoxazoles and isoxazolines in deep eutectic solvents. ACS Sustain. Chem. Eng. 3, 2343–2349. doi: 10.1021/acssuschemeng.5b00689
Phon, S., Pradana, A. L., and Thanasupsin, S. P. (2023). Recovery of collagen/gelatin from fish waste with carbon dioxide as a green solvent: an optimization and characterization. Recycling 8, 30. doi: 10.3390/recycling8020030
Piccirillo, C., Pullar, R. C., Costa, E., Santos-Silva, A., E. Pintado, M. M., and L. Castro, P. M. (2015). Hydroxyapatite-based materials of marine origin: a bioactivity and sintering study. Mater. Sci. Eng. C 51, 309–315. doi: 10.1016/j.msec.2015.03.020
Pihlanto, A., and Korhonen, H. (2003). Bioactive peptides and proteins. Adv. Food Nutr. Res. 47, 175–276. doi: 10.1016/S1043-4526(03)47004-6
Pillot, M., Lebeau, B., Nouali, H., Daou, T. J., Patarin, J., and Ryzhikov, A. (2019). High pressure intrusion of water and LiCl aqueous solutions in hydrophobic KIT-6 mesoporous silica: Influence of the grafted group nature. Micropor. Mesopor. Mater. 280, 248–255. doi: 10.1016/j.micromeso.2019.02.006
Puértolas, E., and Barba, F. J. (2016). Electrotechnologies applied to valorization of by-products from food industry: main findings, energy and economic cost of their industrialization. Food Bioprod. Process. 100, 172–184. doi: 10.1016/j.fbp.2016.06.020
Rajabimashhadi, Z., Gallo, N., Salvatore, L., and Lionetto, F. (2023). Collagen derived from fish industry waste: progresses and challenges. Polymers 15, 544. doi: 10.3390/polym15030544
Regenstein, J. M., and Zhou, P. (2007). “13 - Collagen and gelatin from marine by-products,” in Maximising the Value of Marine By-Products, ed F. Shahidi (Woodhead Publishing; Elsevier), 279–303.
Ricard-Blum, S. (2011). The collagen family. Cold Spring Harb. Perspect. Biol. 3, a004978. doi: 10.1101/cshperspect.a004978
Ricard-Blum, S., and Ruggiero, F. (2005). The collagen superfamily: from the extracellular matrix to the cell membrane. Pathol. Biol. 53, 430-442. doi: 10.1016/j.patbio.2004.12.024
Rose, C., Kumar, M., and Mandal, A. B. (1988). A study of the hydration and thermodynamics of warm-water and cold-water fish collagens. Biochem. J. 249, 127–133. doi: 10.1042/bj2490127
Rustad, T., Storrø, I., and Slizyte, R. (2011). Possibilities for the utilisation of marine by-products. Int. J. Food Sci. Technol. 46, 2001–2014. doi: 10.1111/j.1365-2621.2011.02736.x
Saallah, S., Roslan, J., Julius, F. S., Saallah, S., Mohamad Razali, U. H., Pindi, W., et al. (2021). Comparative study of the yield and physicochemical properties of collagen from sea cucumber (Holothuria scabra), obtained through dialysis and the ultrafiltration membrane. Molecules 26, 2564. doi: 10.3390/molecules26092564
Sadat-Shojai, M., Khorasani, M.-T., Dinpanah-Khoshdargi, E., and Jamshidi, A. (2013). Synthesis methods for nanosized hydroxyapatite with diverse structures. Acta Biomater. 9, 7591–7621. doi: 10.1016/j.actbio.2013.04.012
Sadowska, M., Kołodziejska, I., and Niecikowska, C. (2003). Isolation of collagen from the skins of Baltic cod (Gadus morhua). Food Chem. 81, 257–262. doi: 10.1016/S0308-8146(02)00420-X
Safiya, N., and Casparus, J. R. V. (2020). “Collagen: from waste to gold,” in Biotechnological Applications of Biomass, eds B. Thalita Peixoto, B. Thiago Olitta, and B. Luiz Carlos (IntechOpen), 12. doi: 10.5772/intechopen.94266
Saini, R. K., Bajpai, A. K., and Jain, E. (2018). “12 –Fundamentals of bionanocomposites,” in Biodegradable and Biocompatible Polymer Composites, ed N. G. Shimpi (Woodhead Publishing; Elsevier), 351–377.
Salvatore, L., Gallo, N., Natali, M. L., Campa, L., Lunetti, P., Madaghiele, M., et al. (2020). Marine collagen and its derivatives: versatile and sustainable bio-resources for healthcare. Mater. Sci. Eng. C 113, 110963. doi: 10.1016/j.msec.2020.110963
Salvia-Trujillo, L., Morales-de la Peña, M., Rojas-Gra,ü, A., Welti-Chanes, J., and Martín-Belloso, O. (2017). Mineral and fatty acid profile of high intensity pulsed electric fields or thermally treated fruit juice-milk beverages stored under refrigeration. Food Control 80, 236–243. doi: 10.1016/j.foodcont.2017.05.001
Santos, V. P., Maia, P., Alencar, N. D. S., Farias, L., Andrade, R. F. S., Souza, D., et al. (2019). Recovery of chitin and chitosan from shrimp waste with microwave technique and versatile application. Arq. Inst. Biol. 86, e0982018. doi: 10.1590/1808-1657000982018
Sanz-Fraile, H., Amoros, S., Mendizabal, I., Galvez-Monton, C., Prat-Vidal, C., Bayes-Genis, A., et al. (2020). Silk-reinforced collagen hydrogels with raised multiscale stiffness for mesenchymal cells 3D culture. Tissue Eng. Part A 26, 358–370. doi: 10.1089/ten.tea.2019.0199
Schmidt, M. M., Dornelles, R. C. P., Mello, R. O., Kubota, E. H., Mazutti, M. A., Kempka, A. P., et al. (2016). Collagen extraction process. Int. Food Res. J. 23, 913–922.
Shahidi, F. (2007). “12 - Marine oils from seafood waste,” in Maximising the Value of Marine By-Products, ed F. Shahidi (Woodhead Publishing; Elsevier), 258–278.
Shahidi, F., and Zhong, Y. (2008). Bioactive peptides. J. AOAC Int. 91, 914–931. doi: 10.1093/jaoac/91.4.914
Shaik, M. I., Chong, J. Y., and Sarbon, N. M. (2021). Effect of ultrasound-assisted extraction on the extractability and physicochemical properties of acid and pepsin soluble collagen derived from Sharpnose stingray (Dasyatis zugei) skin. Biocatal. Agric. Biotechnol. 38, 102218. doi: 10.1016/j.bcab.2021.102218
Shu, Y., Ren, H., Ao, R., Qi, W. C., and Zhang, Z. S. (2017). Comparison of physical and chemical characteristics of collagen from the skin of cod (Gadus macrocephaius). Genet. Mol. Res. 16. doi: 10.4238/gmr16027940
Siddiqui, S. A., Schulte, H., Pleissner, D., Schönfelder, S., Kvangarsnes, K., Dauksas, E., et al. (2023). Transformation of seafood side-streams and residuals into valuable products. Foods 12:422. doi: 10.3390/foods12020422
Sinthusamran, S., Benjakul, S., and Kishimura, H. (2013). Comparative study on molecular characteristics of acid soluble collagens from skin and swim bladder of seabass (Lates calcarifer). Food Chem. 138, 2435–2441. doi: 10.1016/j.foodchem.2012.11.136
ŠliŽyte, R., Mozuraityte, R., Martínez-Alvarez, O., Falch, E., Fouchereau-Peron, M., and Rustad, T. (2009). Functional, bioactive and antioxidative properties of hydrolysates obtained from cod (Gadus morhua) backbones. Proc. Biochem. 44, 668–677. doi: 10.1016/j.procbio.2009.02.010
Soballe, K., Hansen, E., Brockstedt-Rasmussen, H., and Bunger, C. (1993). Hydroxyapatite coating converts fibrous tissue to bone around loaded implants. J. Bone Joint Surg. Br. 75-B, 270–278. doi: 10.1302/0301-620X.75B2.8444949
Son, S.-A., Shin, E. S., Park, Y. M., Ma, A., Yang, H., Kim, S., et al. (2022). Composition of collagen extracted from the skin of three different varieties of fish. J. Kor. Soc. Food Sci. Nutr. 51, 71–81. doi: 10.3746/jkfn.2022.51.1.71
Sousa, R. O., Martins, E., Carvalho, D. N., Alves, A. L., Oliveira, C., Duarte, A. R. C., et al. (2020). Collagen from Atlantic cod (Gadus morhua) skins extracted using CO2 acidified water with potential application in healthcare. J. Polym. Res. 27, 73. doi: 10.1007/s10965-020-02048-x
Subhan, F., Hussain, Z., Tauseef, I., Shehzad, A., and Wahid, F. (2021). A review on recent advances and applications of fish collagen. Crit. Rev. Food Sci. Nutr. 61, 1027–1037. doi: 10.1080/10408398.2020.1751585
Sun, L., Li, B., Song, W., Si, L., and Hou, H. (2017). Characterization of Pacific cod (Gadus macrocephalus) skin collagen and fabrication of collagen sponge as a good biocompatible biomedical material. Proc. Biochem. 63, 229–235. doi: 10.1016/j.procbio.2017.08.003
Sun, X., Okagu, O. D., and Udenigwe, C. C. (2021). “Chapter 15 - Encapsulation technology for protection and delivery of bioactive peptides,” in Biologically Active Peptides, eds F. Toldrá, and J. Wu (Academic Press), 331–356.
Tang, S., Baker, G. A., and Zhao, H. (2012). Ether- and alcohol-functionalized task-specific ionic liquids: attractive properties and applications. Chem. Soc. Rev. 41, 4030–4066. doi: 10.1039/c2cs15362a
Tarigan, C. U. T. a. W. P. L. (2022). Extraction of functionally active collagen from salmon fish as formulation of clay mask. Best J. 5, 120–126.
Torres, O., Tena, N. M., Murray, B., and Sarkar, A. (2017). Novel starch based emulsion gels and emulsion microgel particles: design, structure and rheology. Carbohydr. Polym. 178, 86–94. doi: 10.1016/j.carbpol.2017.09.027
Tri, N., Trang, T. N. D., Trinh, N. H. D., Tung, L. T., Van, N. T. T., Anh, N. P., et al. (2020). Hydrothermal and calcination regimes and characteristics of nanohydroxyapatite synthesized from salmon bones. J. Biochem. Technol. 11, 82–87.
Trilaksani, W., Adnyane, I. K. M., Riyanto, B., and Safitri, N. (2020). Nano collagen of the grouper swim bladder in compliance with quality standard of cosmetics materials. IOP Conf. Ser. 404, 012050. doi: 10.1088/1755-1315/404/1/012050
Tylingo, R. M. S, Panek, A., Piatek, R., and Pawłowicz, R. (2016). Isolation and characterization of acid soluble collagen from the skin of African catfish (Clarias gariepinus), Salmon (Salmo salar) and Baltic Cod (Gadus morhua). J. Biotechnol. Biomater. 6:234. doi: 10.4172/2155-952X.1000234
Tziveleka, L.-A., Kikionis, S., Karkatzoulis, L., Bethanis, K., Roussis, V., and Ioannou, E. (2022). Valorization of fish waste: isolation and characterization of acid- and pepsin-soluble collagen from the scales of mediterranean fish and fabrication of collagen-based nanofibrous scaffolds. Mar. Drugs 20:664. doi: 10.3390/md20110664
Ucak, I., Afreen, M., Montesano, D., Carrillo, C., Tomasevic, I., Simal-Gandara, J., et al. (2021). Functional and bioactive properties of peptides derived from marine side streams. Mar. Drugs 19:71. doi: 10.3390/md19020071
Välimaa, A.-L., Mäkinen, S., Mattila, P., Marnila, P., Pihlanto, A., Mäki, M., et al. (2019). Fish and fish side streams are valuable sources of high-value components. Food Qual. Saf. 3, 209–226. doi: 10.1093/fqsafe/fyz024
van Osch, D. J. G. P., Zubeir, L. F., van den Bruinhorst, A., Rocha, M. A. A., and Kroon, M. C. (2015). Hydrophobic deep eutectic solvents as water-immiscible extractants. Green Chem. 17, 4518–4521. doi: 10.1039/C5GC01451D
Vate, N. K., Strachowski, P., Undeland, I., and Abdollahi, M. (2023). Structural and functional properties of collagen isolated from lumpfish and starfish using isoelectric precipitation vs salting out. Food Chem. 18:100646. doi: 10.1016/j.fochx.2023.100646
Venkatesan, J., and Anil, S. (2021). Hydroxyapatite derived from marine resources and their potential biomedical applications. Biotechnol. Bioprocess Eng. 26, 312–324. doi: 10.1007/s12257-020-0359-0
Venkatesan, J., Lowe, B., Manivasagan, P., Kang, K. H., Chalisserry, E. P., Anil, S., et al. (2015). Isolation and characterization of nano-hydroxyapatite from salmon fish bone. Materials 8, 5426–5439. doi: 10.3390/ma8085253
Venslauskas, K., Navickas, K., Nappa, M., Kangas, P., Mozuraityte, R., ŠliŽyte, R., et al. (2021). Energetic and economic evaluation of zero-waste fish co-stream processing. Int. J. Environ. Res. Public Health 18:2358. doi: 10.3390/ijerph18052358
Venugopal, V., Sasidharan, A., and Rustad, T. (2023). Green chemistry to valorize seafood side streams: an ecofriendly roadmap toward sustainability. J. Agric. Food Chem. 71, 17494–17509. doi: 10.1021/acs.jafc.3c03126
Wagle, D. V., Zhao, H., and Baker, G. A. (2014). Deep eutectic solvents: sustainable media for nanoscale and functional materials. Acc. Chem. Res. 47, 2299–2308. doi: 10.1021/ar5000488
Woonnoi, W., Chotphruethipong, L., Tanasawet, S., Benjakul, S., Sutthiwong, N., and Sukketsiri, W. (2021). Hydrolyzed collagen from salmon skin increases the migration and filopodia formation of skin keratinocytes by activation of FAK/Src pathway. Polish J. Food Nutr. Sci. 71, 323–332. doi: 10.31883/pjfns/141515
Wu, T. H., and Bechtel, P. J. (2008). Salmon by-product storage and oil extraction. Food Chem. 111, 868–871. doi: 10.1016/j.foodchem.2008.04.064
Wu, Z., Zhong, J., Yu, Y., Rong, M., and Yang, T. (2021). A rapid and convenient approach to construct porous collagen membranes via bioskiving and sonication-feasible for mineralization to induce bone regeneration. Front. Bioeng. Biotechnol. 9, 752506. doi: 10.3389/fbioe.2021.752506
Yamada, S., Yamamoto, K., Ikeda, T., Yanagiguchi, K., and Hayashi, Y. (2014). Potency of fish collagen as a scaffold for regenerative medicine. Biomed Res. Int. 2014, 302932. doi: 10.1155/2014/302932
Yan, J., Miao, Y., Tan, H., Zhou, T., Ling, Z., Chen, Y., et al. (2016). Injectable alginate/hydroxyapatite gel scaffold combined with gelatin microspheres for drug delivery and bone tissue engineering. Mater. Sci. Eng. C 63, 274–284. doi: 10.1016/j.msec.2016.02.071
Yang, J., and Powers, J. R. (2016). “Effects of high pressure on food proteins,” in High Pressure Processing of Food: Principles, Technology and Applications, eds V. M. Balasubramaniam, G. V. Barbosa-Cánovas, and H. L. M. Lelieveld (New York, NY: Springer), 353–389.
Yusof, N., Jaswir, I., Jamal, P., Jami, M. S., and Octavianti, F. (2017). Optimization of Process Condition for Extraction of Gelatin From Red Tilapia Skin (Oreochromis niloticus) by High Pressure Processing (HPP). Int. Food Res. J. 340-347.
Zamora-Sillero, J., Gharsallaoui, A., and Prentice, C. (2018). Peptides from fish by-product protein hydrolysates and its functional properties: an overview. Mar. Biotechnol. 20, 118–130. doi: 10.1007/s10126-018-9799-3
Zanaboni, G., Rossi, A., Onana, A. M. T., and Tenni, R. (2000). Stability and networks of hydrogen bonds of the collagen triple helical structure: influence of pH and chaotropic nature of three anions. Matrix Biol. 19, 511–520. doi: 10.1016/S0945-053X(00)00096-2
Zelechowska, E., Sadowska, M., and Turk, M. (2010). Isolation and some properties of collagen from the backbone of Baltic cod (Gadus morhua). Food Hydrocoll. 24, 325–329. doi: 10.1016/j.foodhyd.2009.10.010
Zhang, H., Wu, X., Quan, L., and Ao, Q. (2022). Characteristics of marine biomaterials and their applications in biomedicine. Mar. Drugs 20:372. doi: 10.3390/md20060372
Zhang, J., Wen, C., Zhang, H., Duan, Y., and Ma, H. (2020). Recent advances in the extraction of bioactive compounds with subcritical water: a review. Trends Food Sci. Technol. 95, 183–195. doi: 10.1016/j.tifs.2019.11.018
Zhao, B.-Y., Xu, P., Yang, F.-X., Wu, H., Zong, M.-H., and Lou, W.-Y. (2015). Biocompatible deep eutectic solvents based on choline chloride: characterization and application to the extraction of rutin from Sophora japonica. ACS Sustain. Chem. Eng. 3, 2746–2755. doi: 10.1021/acssuschemeng.5b00619
Keywords: fish fileting, side streams, collagen, hydroxyapatite, green technologies, extraction
Citation: Kendler S, Sasidharan A and Rustad T (2024) Extraction of proteinaceous components and biominerals from cold water fish fileting side streams: a review. Front. Sustain. Food Syst. 7:1331113. doi: 10.3389/fsufs.2023.1331113
Received: 31 October 2023; Accepted: 15 December 2023;
Published: 09 January 2024.
Edited by:
Dandan Ren, Dalian Ocean University, ChinaReviewed by:
Zhijian Tan, Chinese Academy of Agricultural Sciences (CAAS), ChinaCopyright © 2024 Kendler, Sasidharan and Rustad. This is an open-access article distributed under the terms of the Creative Commons Attribution License (CC BY). The use, distribution or reproduction in other forums is permitted, provided the original author(s) and the copyright owner(s) are credited and that the original publication in this journal is cited, in accordance with accepted academic practice. No use, distribution or reproduction is permitted which does not comply with these terms.
*Correspondence: Turid Rustad, dHVyaWQucnVzdGFkQG50bnUubm8=
Disclaimer: All claims expressed in this article are solely those of the authors and do not necessarily represent those of their affiliated organizations, or those of the publisher, the editors and the reviewers. Any product that may be evaluated in this article or claim that may be made by its manufacturer is not guaranteed or endorsed by the publisher.
Research integrity at Frontiers
Learn more about the work of our research integrity team to safeguard the quality of each article we publish.