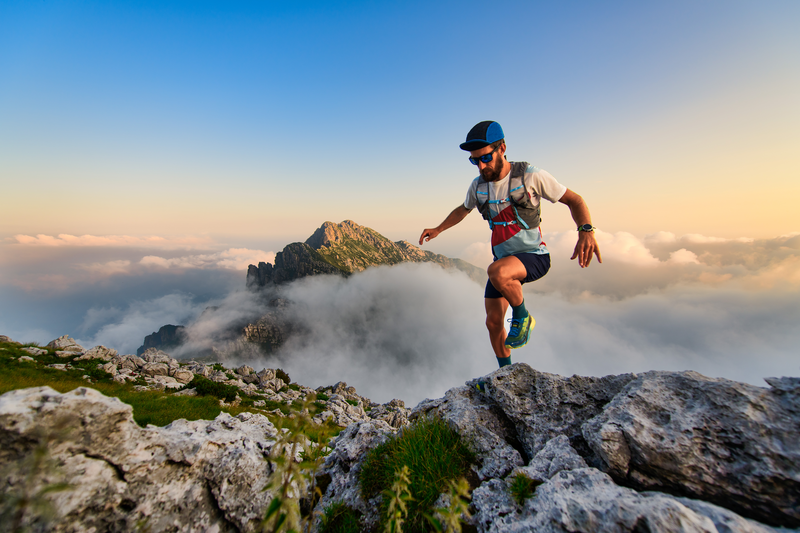
94% of researchers rate our articles as excellent or good
Learn more about the work of our research integrity team to safeguard the quality of each article we publish.
Find out more
ORIGINAL RESEARCH article
Front. Sustain. Food Syst. , 08 January 2024
Sec. Sustainable Food Processing
Volume 7 - 2023 | https://doi.org/10.3389/fsufs.2023.1326102
This article is part of the Research Topic Exploring Sustainable Strategies for Active Compounds from Low-Quality Crops: Extraction, Package, and Development View all 5 articles
Effects of steam explosion (SE) treatment (0.3–1.0 MPa, 30–90s) on the physicochemical and functional properties of dietary fiber (DF) extracted from tea residue were investigated. Results showed that SE led to the formation of porous and less compact microstructures of tea residues. Compared with the control sample (12.41 g/100 g), the content of soluble dietary fiber (SDF) was markedly increased by SE, reaching the maximum of 23.03 g/100 g when steam explored at 0.5 MPa for 90 s, due to the degradation of insoluble dietary fiber (IDF). The physicochemical and functional properties were significantly influenced by SE treatments. IDF exhibited decreased water holding capacity (WHC), swelling capacity (SC), oil holding capacity (OHC) after SE, whereas the physicochemical properties of SDF from SE-treated tea residues (0.3 MPa, 90s) were greatly improved. The glucose absorption capacity (GAC), cholesterol adsorption capacity (CAC), and nitrite ion adsorption capacity (NIAC) of both IDF and SDF showed trends of first increasing and then decreasing, indicating that excessive SE treatment resulted in poor properties of DF. These findings are of great value for the high-value utilization of agricultural by-product and development of functional foods.
Tea is widely consumed in the world as a non-alcoholic beverage, and its derived products, such as bottled tea drinks, instant tea powder and tea extracts have attracted increasing attentions due to its unique flavor, taste, and healthy benefits (Sui et al., 2019; Guo et al., 2021). As the by-product of the tea industry, the amount of tea residues rapidly increased. Owing to low quality and unsatisfactory physicochemical properties, tea residues are usually discarded or utilized as feed additive, which resulted in the waste of resources and increase of environmental stress (Wang et al., 2011; Miao et al., 2022).
Tea residues are rich in dietary fiber (DF), and numerous studies have demonstrated that DF has a variety of potential health benefits for our human bodies, such as the ability to lower cholesterol and regulate glucose metabolism, the promising regulatory effects on the intestinal flora for preventing gastrointestinal disorders (Barber et al., 2020; Gill et al., 2020). Moreover, DF is also of great significance for the stability of food during processing and storage, including effectively stabilizing the fat in food, improving gel properties, providing special taste and texture, and extending the shelf life, as well as being used as a fat substitute (Jiapan et al., 2021).
DF can be classified into insoluble dietary fiber (IDF, includes cellulose, lignin and insoluble hemicelluloses) and soluble dietary fiber (SDF, mainly consists of pectin, gums and soluble hemicelluloses) based on their solubility in water (Rodríguez et al., 2006). According to previous researches, it has been demonstrated that SDF exhibits more functional properties than IDF, such as emulsion capabilities, binding of toxic elements and inhibiting tumor cells (Guo et al., 2018; Xiaoqiang et al., 2019; Chen et al., 2020; Han et al., 2020). Zheng et al. found that the glucose adsorption ability of the SDF extracted from bamboo shoot shell was larger than that of IDF (Zheng et al., 2019). In total dietary fiber (TDF), the proportion of SDF should be greater than 10% for high-quality dietary fiber and the ratio of SDF/IDF is recommended to be approximately 1/2 as a right food ingredients (Jaime et al., 2002; Khanpit et al., 2021). Despite the high proportion of DF in tea residues, the SDF content is comparatively low, which limits its application in food manufacture, and it is crucial to develop modification technique to increase the content of SDF in tea residues to achieve dietary fibers with excellent functionalities.
The modification of DF to promote the conversion of IDF into SDF includes chemical (acid and alkali method), physical (extrusion), and biological methods (enzymatic hydrolysis and fermentation) (Niu et al., 2017; Chen et al., 2018, 2020). These methods, however, have certain disadvantages such as introduction of chemicals, high cost, time consuming, and environmentally unfriendly, etc. Steam explosion (SE), recognized as an effective, clean and economical technique to dissolute or degrade large molecular carbohydrate polymers to small ones, is defined as the steam hydrolysis at high temperature and pressure, followed by sudden decompression for physical tearing of hydrolyzed materials (Shen et al., 2019; Sui et al., 2019). During SE processing, insoluble macromolecular polysaccharides such as cellulose and hemicellulose can be converted into small-molecule soluble polysaccharides through thermal degradation, mechanical fracture and hydrogen bond destruction (Dechao et al., 2021), thus increasing the content of SDF. In recent years, SE technology has attracted much attention in food processing, such as enhancing extraction efficiency of phenolics, increasing the SDF content of plant material by-products (Li et al., 2019; Wan et al., 2022; Xi et al., 2022). In tea industry, SE has been adopted as an effective modification way to enhance the extractability of bioactive compounds and improve the quality of tea seed oil (Sui et al., 2019).
In this study, SE was applied to treat tea residue at different pressures (0.3, 0.5, and 1.0 Mpa) and holding times (30, 60 and 90s). The influences of SE treatment on the microstructures and DF composition of tea residue were analyzed. The changes in the physicochemical and functional properties of IDF and SDF before and after different SE treatments were also evaluated.
Green tea residue was purchased from a local market in Nanping (Fujian province, China), which contains 6.1% water, 25.8% protein, 2.1% fat, 61.8 dietary fiber and 3.2% ash. All analytical reagents were of analytical grade unless otherwise stated, which were purchased from Sinopharm Chemical Reagent Co., Ltd. (Beijing, China), Xilong Scientific Co., Ltd., Shanghai Yi En Chemical Technology Co., Ltd., and kits were purchased from Suzhou Keming Biotechnology Co., Ltd.
The tea residue (40 mesh) was loaded into the reactor of QB-300 steam explosion machine (Qingzheng Ecological Technology (Suzhou) Co., Ltd., China), and allowed to exploded at 0.3, 0.5 and 1.0 MPa for 30, 60, and 90 s, respectively. The exploded tea residue samples were collected and dried under 45°C, and stored at room temperature for further use.
The tea residue samples after SE treatments (80 mesh) were placed in glass culture dishes to completely cover the bottom, and then photographed and recorded under the same conditions. The color of the samples was analyzed with an ADCI-60-C colorimeter (Beijing Chentaike Instrument Technology Co., Ltd., China). The colorimeter was calibrated using standard white and black calibration plates. L* (lightness, 0 as black and 100 as white), a* (color range from green to red), and b* (color range from blue to yellow) values were recorded and analyzed.
The microstructures of the tea residue samples before and after SE treatments were observed with a scanning electron microscope (SEM, Quanta 250, FEI, Hillsboro, OR, United States) at an accelerating voltage of 15 kV. Before observation, the dried samples were sputter-coated with gold using an ion sputter coater (SCD 005, BAL-TEC, Switzerland).
The SE treatment tea residue was dispersed in 15 times volume of phosphate buffer and the pH was adjusted to 6.5. Then α-amylase (0.05 g/g) was added and allowed to hydrolyze at 95°C for 30 min. After the temperature of the hydrolysate was decreased to 60°C, 0.002 g/g papain was added to further hydrolyze for another 60 min at pH 6.0. The enzyme activity was inactivated in boiled water for 10 min. After that, the hydrolysate was centrifuged at 3,000 × g for 10 min at room temperature, and the sediment was washed with distilled water to get IDF. The supernatant was condensed to one-third in rotary evaporator, followed by mixing with 4 times 95% (v/v) ethanol for 12 h and subjecting to centrifugation at 3,000 × g for 5 min, SDF was defined as the precipitation after freeze-drying.
Cellulose, hemicellulose and lignin contents of DF were measured by the method according to Jiang et al. (2021). The AOAC methods (1990; method no. 973.18) was used to detected the neutral detergent fiber (NDF) and acid detergent fiber (ADF). Acid detergent lignin (ADL) were determined by treating samples with 72% H2SO4 (20°C) for 3 h, then the samples were washed and dried, followed by heating in a furnace. The lignin content (%) was defined as the weight loss (%) before and after heating. Cellulose was calculated as ADF-ADL (%) and hemicellulose was calculated as NDF-ADF (%).
The content of sugar in tea residue with or without SE was determined according to Geater and Fehr (2000). The phenol-sulfuric acid method was used to analyze the total sugar content and the 3,5-Dinitrosalicylic acid method was used for the determination of reducing sugar content.
The FTIR spectra of SDF and IDF were recorded according to Xi et al. (2022), all dry samples were mixed with KBr (FT–IR grade) before grinding and pressing. A FTIR spectrometer was used to measure the spectra of samples (Nicolet 6,700, Thermo Fisher, United States). FT-IR spectra in the wavenumber range of 400–5,000 cm−1 were recorded.
Water holding capacity was determined according to Yang et al. (2022) with minor modification. 0.5 g IDF and SDF were dissolved with 10 mL distilled water for 1 h at ambient temperature. The samples were centrifuged at 2,000 × g for 10 min and the supernatant was removed, tubes with precipitate were accurately weighed. WHC was calculated as follows:
0.1 g IDF and SDF were weighted and the initial volume was recorded. 5 mL of distilled water was mixed with sample for 24 h at room temperature, and the final volume of the hydrated sample was recorded. The swelling capacity (SC) was calculated by the following equation:
The OHC of the samples was measured based on a method reported by Jiang et al. (2021) with slight modifications. Briefly, 0.5 g IDF and SDF were mixed with 10 mL sunflower oil for 1 h. Then centrifuged at 1,000 × g for 30 min, supernatant and excess oil were removed. The weight of sample was recorded and the OHC was calculated as follows:
Based on the method described by Qianyun et al. (2021), the GAC of samples was determined using the DNS method. 0.2 g lyophilized IDF and SDF sample was mixed with 20 mL glucose solution (100 mmol/L), which was immersed into a 37°C water bath for 6 h. After the mixture was centrifuged at 3,000 × g for 10 min, the absorbance of the supernatant was determined at 540 nm, and the GAC was calculated according to the standard curve.
The CAC was measured based on a method reported by Si et al. (2022). 10 mL of egg york was added to 90 mL distilled water and mixed into the emulsion. 1 g of IDF and SDF were blended with 25 mL emulsion, respectively. Then the mixtures were adjusted to pH 2.0 (simulating the gastric environment) and pH 7.0 (simulating the intestinal environment) and shaken at 37°C for 2 h, and diluted yolk without DF was blank. The mixture was centrifuged at 2,200 × g for 10 min, and the supernatant was collected and distilled. The absorbance 0.4 mL sample was determined at 550 nm, and the cholesterol adsorption capacity was calculated according to the standard curve.
Analysis of NIAC was based on the previously established method Luo et al. (2017). In brief, the NaNO2 solution (100 μmol/L) was adjusted to pH 2.0 and pH 7.0, representing the simulated of gastric environment and small intestinal environment, respectively. 0.5 g IDF and SDF samples were weighed and mixed with 25 mL NaNO2 solution in a conical flask at 37°C water bath for 30 min. An ultraviolet spectrophotometer (GENESYS 10S, Thermo Fisher Scientific Inc., United States) was used to determine the absorption spectra of NaNO2 at wavelength of 538 nm, and the NIAC was estimated using the standard curve.
Each experiment was carried out in triplicate and results were expressed as mean values ± standard deviation (S.D.). Statistical analysis was performed with SPSS 20 and chart drawing were completed by Origin. Data were analyzed by analysis of variance (ANOVA) and the Duncan method, which used a significance level of p < 0.05.
Photographs of the tea residues after different SE treatments are shown in Figure 1. The color of the tea residues gradually changed from green to tawny with the increase in the steaming pressure and holding time. The darkening of colors may be related to the oxidation of tea polyphenols and Maillard reactions between proteins and reducing sugars caused by high temperatures and pressures (Zhang et al., 2019; Yan et al., 2021). The color properties (L*, a*, b*) of the tea residue samples are presented in Table 1. It can be found that the L* values decreased with the increase in the steaming temperature and holding time, indicating decreases in the lightness of the samples. A decrease in the b* (blue-yellow) value but increasing trend of a* (green-red) value were observed in the SE-treated samples when the steam intensity was elevated. This demonstrated that the color of the tea residues was closer to the red and blue regions (Minz and Saini, 2021).
Table 1. Effects of steam explosion on the color parameters of tea residues under different steaming conditions.
The microstructures of tea residues before and after SE treatment were observed using scanning electron microscopy (SEM), which are shown in Figure 2. The sample without SE treatment (Control, Figure 2A) exhibited a complete morphology with intact cell wall structure and smooth surface. SE treatment disrupted the integrity of the structure and led to the separation of leaf mesophyll and veins, accompanied by the formation of small fragments and large cavities were formed on the surface of tea residues, resulting much rougher texture. Particularly, when the tea residues were steam exploded under 0.5 MPa (60 and 90 s) and 1.0 MPa, vortex shaped cavities could be observed. This might be caused by the entrance of supersaturated steam and an instantaneous release of high pressure, which contributed to the rupture of the plant cell wall due to the expansion work and flashing effect during the instantaneous decompression (Nasir et al., 2020).
Figure 2. Effect of steam explosion on the microstructure of tea residues. (A), Control; (B), 0.3 MPa, 30 s; (C), 0.3 MPa, 60 s; (D), 0.3 MPa, 90s; (E), 0.5 MPa, 30 s; (F), 0.5 MPa, 60 s; (G), 0.5 MPa, 90 s; (H), 1.0 MPa, 30 s; (I), 1.0 MPa, 60 s; (J), 1.0 MPa; 90 s.
The content of SDF and ratio of SDF/IDF are comparably low in tea residue, which limit its application in food processing. Therefore, increasing the SDF content could improve the quality of tea residue. Figure 3 shows the changes in the SDF and IDF content in tea residues modified by SE. From the figure, the content of IDF and SDF in the control sample were 53.34 g/100 g and 12.41 g/100 g, respectively. SDF content was significantly increased by SE, reaching the maximum of 23.03 g/100 g (increased by 85.58% compared with the control) when exploded under 0.5 MPa for 90 s, and the IDF content was simultaneously decreased by 18.24% from 53.34 g/100 g to 43.61 g/100 g, indicating depolymerization of some material binding with fiber and partial degradation of high molecular weight polysaccharides (Gong et al., 2012). Meanwhile, the ratio of SDF/IDF increased from 1:4.3 to 1:1.9, which reached the recommended value (approximately 1:2) for using as food ingredients (Khanpit et al., 2021). However, further increasing the SE intensity (1.0 MPa) led to the decrease in the content of SDF, one reasonable explanation is that some polysaccharides in tea residue were over-degraded and converted into oligosaccharides with smaller molecular weight, which cannot be precipitated by ethanol when determining the SDF content (Li et al., 2019). The total content of DF did not differ significantly among the samples, indicating that SE did not cause obvious loss in essential ingredients of tea residue (Kong et al., 2020).
Table 2 shows the effects of SE (0.3, 0.5, and 1.0 MPa for 90 s.) on the content of cellulose, hemicellulose and lignin. These components decreased significantly with the increase in SE intensity. Under the condition of 1.0 MPa, 90 s, the content of cellulose, hemicellulose and lignin decreased by 30.35, 66.20, and 22.48%, respectively. The differences in the decrease extent of these three IDF components could be due to the fact that the chemical stability of hemicellulose and cellulose is weaker than that of lignin (Dai et al., 2017; He et al., 2019). The content of reducing sugar and total sugar in tea residue markedly increased after SE treatments, which confirmed that SE treatment degrade the IDFs into soluble polysaccharides. These findings were consistent with the DF compositions presented in section 3.3.
The FTIR spectra of DF from tea residue before and after SE are shown in Figure 4. The absorption peaks near 3,400 cm−1, 2,920 cm−1, 1,630 cm−1, and 1,060 cm−1 represented stretching vibration generated by O-H, C-H, C=O, and C-O-C/O-H of DF, respectively, which conformed to the typical infrared absorption spectrum characteristics of polysaccharides (Ma and Mu, 2015; Dong et al., 2020). After SE treatment, the IDF generated a red shift from 3,400 cm−1 to 3,357 cm−1 (Figure 4A), indicating partial degradation of cellulose and hemicellulose components in the raw material (Zheng et al., 2021). Figure 4B shows the FTIR spectra of SDF. We can find that when compared with the control sample (0-SDF), the intensity of the absorption peak near 3,440 cm−1 increased, indicating that SE promoted more exposure of -OH groups, which is beneficial for improving the hydrophilicity of the sample. Nevertheless, there were no new vibration peaks in the dietary fiber of tea residue after SE treatment, or significant differences in the peak shape, quantity, and position. Only the intensity of some absorption peaks was changed, indicating that the structure of DF was disrupted to some extent by SE, but without formation of new chemical groups, suggesting no changes in the composition of both IDF and SDF from tea residue.
Figure 4. FTIR spectra of insoluble dietary fiber (A) and soluble dietary fiber (B) from tea residue with or without steam explosion.
The influence of SE on the SC, WHC, and OHC of DFs from tea residue is shown in Figure 5. The SC of IDF decreased with the increase of SE intensity. At SE intensity of 0.3 MPa for 90 s, 0.5 MPa for 90 s and 1.0 MPa for 90 s, the swelling capacity decreased by 13.53, 21.10 and 46.33%, respectively, compared to the control. Comparatively, the SC of SDF increased first and then decreased, and at the SE intensity of 0.3 MPa for 90 s, the maximum value of 3.05 g/g was obtained, which was 41.86% higher than that of the SDF in control sample. The WHC and OHC of the IDF and SDF showed consistent variation trends with SC values. The improvement of SC, WHC and OHC of SDF might be attributed to the increase in the surface area of DF and short-chain fibers caused by appropriate SE treatment (Wang et al., 2017; Xi et al., 2022). On the other hand, cellulose and hemicellulose molecules contain hydrophilic groups with excellent water-holding and swelling capacities, and furthermore, highly hydrated cellulose and hemicellulose molecules can absorb oil and cholesterol (Shen et al., 2019). Therefore, the IDF from non-SE treated tea residue possessed the highest SC, WHC and OHC. SE may break the porous structure and channels in the raw fibers, thus causing the decrease in the physicochemical properties of IDF (Li et al., 2019; Zhu et al., 2021).
Figure 5. Effects of steam explosion on swelling capacity (SC), water holding capacity (WHC) and oil holding capacity (OHC) of dietary fiber from tea residue.
GAC is an important indicator for accessing the ability of DF to adsorb glucose in the intestine (Tang et al., 2023). The GAC values of DFs from tea residue were presented in Figure 6A. From the figure, we can observe that SE at 0.3 MPa for 90s significantly increased the GAC of both IDF and SDF with values of 5.02 mmol/g and 7.59 mmol/g. It has been demonstrated that SDF exhibited better GAC than IDF, probably due to increase in the viscosity of the system, which delayed the diffusion rate of glucose molecules (Qiao et al., 2021). It was also reported that the modification of SDF exposes more polar and non-polar groups on the surface of fiber, thus enhancing the interaction between SDF and glucose (Liu et al., 2021). Tang et al. (2023) and Huang et al. (2021) found that SE could increase the specific surface area and result in honeycomb structure of DF, which might be responsible for the improved GAC in the present study. However, excessive SE treatment may destroy the porous structure and lead to the decrease of glucose adsorption capacity.
It was reported that the hypolipidemic feature of DF could effectively alleviate the hazards caused by cholesterol accumulation in vivo (Li et al., 2022), and the CAC is an essential indicator of the DF’s capacity to lower serum cholesterol levels. According to the results shown in Figure 6B, appropriate SE treatment (0.3 or 0.5 MPa, 90 s) significantly enhances the CAC compared to the control sample, particularly for the SDF. It was reported that the surface of SE-treated SDF exhibits more binding sites, thus promoting the adsorption (Zhai et al., 2021). Moreover, the internal disordered structure of dietary fiber was disrupted by SE, thus increasing the exposure degree of polar groups on the surface, which favored its adsorption of cholesterol (Liu et al., 2021; Tang et al., 2023).
DF contains side chain groups such as carboxyl and hydroxyl groups, which have a certain adsorption effect on nitrite. The NIAC indicates the capacity of DF to prevent the toxicity caused by the over intake of nitrite and protect against gastric cancer development (Song et al., 2018). Gastric (pH 2.0) and small intestine (pH 7.0) environments were simulated to investigated the NIAC of the samples. As shown in Figure 7, the NIAC of DFs at pH 2.0 was higher than that at pH 7.0. This could be attributed to the fact that in acidic condition, HNO2 was formed by the reaction between H+ and nitrite ion, and nitrogen-oxide compounds were further generated that can readily combine with the DFs (Zhu et al., 2018). With the increase of SE intensity, the NIAC of the DFs from tea residue first increased and then decreased at both pH 2 and pH 7, the IDF and SDF pre-treated under steaming pressure of 0.3 MPa for 90 s displayed the highest NIAC. It has been demonstrated that the enlarged surface area and more active groups induced by SE contribute to the binding of nitrite ion, thus resulting in the improvement of NIAC of DFs (Xi et al., 2022). However, excessive SE caused the destruction of chemical groups in the IDF and the instability of the structure, thereby reducing the NIAC (Feng et al., 2022). Accordingly, the DFs obtained through moderate SE from tea residue can be used as a candidate for scavenging of nitrite ion.
Figure 7. Nitrite ion adsorption capacity (NIAC) of dietary fibers from tea residues. (A), pH=2; (B), pH=7.
In the present study, SE was used to modify the structural, physicochemical and functional properties of DFs from tea residue. The results suggested that SE changed the morphology of tea residue and lead to the formation of porous and loose microstructures. The content of SDF was effectively increased by 85.58% (up to 23.03 g/100 g) through appropriate SE treatments (0.5 MPa, 90s), resulting from the degradation of IDF. The ratio of SDF/IDF reached approximately 1:2 when the tea residue was exploded at this condition. The SC, WHC and OHC of IDF were impaired after SE treatments, whereas for SDF, these properties were significantly improved by SE at 0.3 MPa for 90 s. The GAC, CAC and NIAC of IDF and SDF showed trends of first increasing and then decreasing under various SE conditions, and SDF exhibited better functional properties than those of IDF. These results indicated that SE can be an effective technique for the modification of DF and improves the added value of tea residue, thereby promoting the application of agricultural processing by-products in food industry.
The original contributions presented in the study are included in the article/supplementary material, further inquiries can be directed to the corresponding author.
YX: Writing – original draft. YZ: Data curation, Methodology, Writing – review & editing. CK: Writing – review & editing. KL: Writing – review & editing. YC: Writing – review & editing. XW: Conceptualization, Funding acquisition, Supervision, Writing – review & editing. SW: Supervision, Writing – review & editing.
The author(s) declare financial support was received for the research, authorship, and/or publication of this article. The authors gratefully acknowledge the financial support provided by the Program of National Natural Science Foundation of China (32001681; 32101986).
The authors declare that the research was conducted in the absence of any commercial or financial relationships that could be construed as a potential conflict of interest.
All claims expressed in this article are solely those of the authors and do not necessarily represent those of their affiliated organizations, or those of the publisher, the editors and the reviewers. Any product that may be evaluated in this article, or claim that may be made by its manufacturer, is not guaranteed or endorsed by the publisher.
Barber, T. M., Kabisch, S., Pfeiffer, A. F. H., and Weickert, M. O. (2020). The health benefits of dietary fibre. Nutrients 12, 3209. doi: 10.3390/nu12103209
Chen, J., Huang, H., Chen, Y., Xie, J., Song, Y., Chang, X., et al. (2020). Effects of fermentation on the structural characteristics and in vitro binding capacity of soluble dietary fiber from tea residues. LWT Food Sci. Technol. 131:109818. doi: 10.1016/j.lwt.2020.109818
Chen, H., Zhao, C., Li, J., Hussain, S., Yan, S., and Wang, Q. (2018). Effects of extrusion on structural and physicochemical properties of soluble dietary fiber from nodes of lotus root. LWT Food Sci. Technol. 93, 204–211. doi: 10.1016/j.lwt.2018.03.004
Dai, L., He, C., Wang, Y., Liu, Y., Ruan, R., Yu, Z., et al. (2017). Hydrothermal pretreatment of bamboo sawdust using microwave irradiation. Bioresour. Technol. 247, 234–241. doi: 10.1016/j.biortech.2017.08.104
Dechao, N., Lingyun, Y., Xiaokai, X., Zhuo, Z., and Yanling, L. (2021). Promoting corn Stover degradation via sequential processing of steam explosion and cellulase/lactic acid bacteria-assisted ensilage. Bioresour. Technol. 337, 125392.
Dong, Y., Lu, X., Hu, C., Li, L., Hu, Q., Wang, D., et al. (2020). Pyrolysis products distribution of enzymatic hydrolysis lignin with/without steam explosion treatment by Py-GC/MS. Catalysts 10, 187. doi: 10.3390/catal10020187
Feng, X., Chen, H., Liang, Y., Geng, M., He, M., et al. (2022). Effects of electron beam irradiation treatment on the structural and functional properties of okara insoluble dietary fiber. J. Sci. Food Agric. 103, 195–204. doi: 10.1002/jsfa.12131
Geater, C. W., and Fehr, W. R. (2000). Association of Total Sugar Content with other seed traits of diverse soybean cultivars. Crop Sci. 40, 1552–1555. doi: 10.2135/cropsci2000.4061552x
Gill, S. K., Rossi, M., Bajka, B., and Whelan, K. (2020). Dietary fibre in gastrointestinal health and disease. Nat. Rev. Gastroenterol. Hepatol. 18, 101–116. doi: 10.1038/s41575-020-00375-4
Gong, L., Huang, L., and Zhang, Y. (2012). Effect of steam explosion treatment on barley bran phenolic compounds and antioxidant capacity. J. Agric. Food Chem. 60, 7177–7184. doi: 10.1021/jf301599a
Guo, S., Kumar Awasthi, M., Wang, Y., and Xu, P. (2021). Current understanding in conversion and application of tea waste biomass: a review. Bioresour. Technol. 338:125530. doi: 10.1016/j.biortech.2021.125530
Guo, Y., Liu, W., Wu, B., Wu, P., Duan, Y., Yang, Q., et al. (2018). Modification of garlic skin dietary fiber with twin-screw extrusion process and in vivo evaluation of Pb binding. Food Chem. 268, 550–557. doi: 10.1016/j.foodchem.2018.06.047
Han, Y., Cheng, Z., Zhang, Y., Zhang, N., Zhu, X., Chen, X., et al. (2020). Effect of metal ions and pH on the emulsifying properties of polysaccharide conjugates prepared from low-grade green tea. Food Hydrocoll. 102:105624. doi: 10.1016/j.foodhyd.2019.105624
He, L., Lv, H., Wang, C., Zhou, W., Pian, R., Zhang, Q., et al. (2019). Dynamics of fermentation quality, physiochemical property and enzymatic hydrolysis of high-moisture corn Stover ensiled with sulfuric acid or sodium hydroxide. Bioresour. Technol. 298:122510. doi: 10.1016/j.biortech.2019.122510
Huang, H., Chen, J., Chen, Y., Xie, J., Liu, S., Sun, N., et al. (2021). Modification of tea residue dietary fiber by high-temperature cooking assisted enzymatic method: structural, physicochemical and functional properties. LWT Food Sci. Technol. 145:111314. doi: 10.1016/j.lwt.2021.111314
Jaime, L., Mollá, E., Fernández, A., Martín-Cabrejas, M. A., López-Andréu, F. J., and Esteban, R. M. (2002). Structural carbohydrate differences and potential source of dietary fiber of onion (Allium cepa L.) tissues. J. Agric. Food Chem. 50, 122–128. doi: 10.1021/jf010797t
Jiang, G., Bai, X., Wu, Z., Li, S., Zhao, C., and Ramachandraiah, K. (2021). Modification of ginseng insoluble dietary fiber through alkaline hydrogen peroxide treatment and its impact on structure, physicochemical and functional properties. LWT Food Sci. Technol. 150:111956. doi: 10.1016/j.lwt.2021.111956
Jiapan, G., Liang, X., Guanyi, P., Jianhua, X., Yi, C., and Qiang, Y. (2021). Systematic review on modification methods of dietary fiber. Food Hydrocoll. 119:106872.
Khanpit, V., Tajane, S. P., and Mandavgane, S. A. (2021). Dietary fibers from fruit and vegetable waste: methods of extraction and processes of value addition. Biomass Conv. Biorefine. doi: 10.1007/s13399-021-01980-2
Kong, F., Wang, L., Chen, H., and Zhao, X. (2020). Improving storage property of wheat bran by steam explosion. Int. J. Food Sci. Technol. 56, 287–292. doi: 10.1111/ijfs.14630
Li, Y., Niu, L., Guo, Q., Shi, L., Deng, X., Liu, X., et al. (2022). Effects of fermentation with lactic bacteria on the structural characteristics and physicochemical and functional properties of soluble dietary fiber from prosomillet bran. LWT 154:112609. doi: 10.1016/j.lwt.2021.112609
Li, B., Yang, W., Nie, Y., Kang, F., Goff, H. D., and Cui, S. W. (2019). Effect of steam explosion on dietary fiber, polysaccharide, protein and physicochemical properties of okara. Food Hydrocoll. 94, 48–56. doi: 10.1016/j.foodhyd.2019.02.042
Liu, Y., Ao, H. P., Zheng, J. X., Liang, Y. X., and Ren, D. F. (2021). Improved functional properties of dietary fiber from Rosa roxburghii Tratt residue by steam explosion. J. Food Process. Preserv. 46:e16119. doi: 10.1111/jfpp.16119
Luo, X., Wang, Q., Zheng, B., Lin, L., Chen, B., Zheng, Y., et al. (2017). Hydration properties and binding capacities of dietary fibers from bamboo shoot shell and its hypolipidemic effects in mice. Food Chem. Toxicol. 109, 1003–1009. doi: 10.1016/j.fct.2017.02.029
Ma, M., and Mu, T. (2015). Modification of deoiled cumin dietary fiber with laccase and cellulase under high hydrostatic pressure. Carbohydr. Polym. 136, 87–94. doi: 10.1016/j.carbpol.2015.09.030
Miao, S., Wei, Y., Chen, J., and Wei, X. (2022). Extraction methods, physiological activities and high value applications of tea residue and its active components: a review. Crit. Rev. Food Sci. Nutr., 63, 12150–12168. doi: 10.1080/10408398.2022.2099343
Minz, P. S., and Saini, C. S. (2021). RGB camera-based image technique for color measurement of flavored milk. Meas. Food 4:100012. doi: 10.1016/j.meafoo.2021.100012
Nasir, A., Chen, H. Z., and Wang, L. (2020). Novel single-step pretreatment of steam explosion and choline chloride to De-lignify corn Stover for enhancing enzymatic edibility. Process Biochem. 94, 273–281. doi: 10.1016/j.procbio.2020.04.036
Niu, Y., Li, N., Xia, Q., Hou, Y., and Xu, G. (2017). Comparisons of three modifications on structural, rheological and functional properties of soluble dietary fibers from tomato peels. LWT Food Sci. Technol. 88, 56–63. doi: 10.1016/j.lwt.2017.10.003
Qianyun, M., Ziye, M., Wenxiu, W., Jianlou, M., Yaqiong, L., Jie, W., et al. (2021). The effects of enzymatic modification on the functional ingredient - dietary fiber extracted from potato residue. LWT Food Sci. Technol. 153:112511. doi: 10.1016/j.lwt.2021.112511
Qiao, H., Shao, H., Zheng, X., Liu, J., Liu, J., Huang, J., et al. (2021). Modification of sweet potato (Ipomoea batatas lam.) residues soluble dietary fiber following twin-screw extrusion. Food Chem. 335:127522. doi: 10.1016/j.foodchem.2020.127522
Rodríguez, R., Jiménez, A., Fernández-Bolaños, J., Guillén, R., and Heredia, A. (2006). Dietary fibre from vegetable products as source of functional ingredients. Trends Food Sci. Technol. 17, 3–15. doi: 10.1016/j.tifs.2005.10.002
Shen, M., Ge, Y., Kang, Z., Quan, Z., Wang, J., Xiao, J., et al. (2019). Yield and physicochemical properties of soluble dietary Fiber extracted from untreated and steam explosion-treated black soybean Hull. J. Chem. 2019, 1–9. doi: 10.1155/2019/9736479
Si, J., Yang, C., Ma, W., Chen, Y., Xie, J., Qin, X., et al. (2022). Screen of high efficiency cellulose degrading strains and effects on tea residues dietary fiber modification: structural properties and adsorption capacities. Int. J. Biol. Macromol. 220, 337–347. doi: 10.1016/j.ijbiomac.2022.08.092
Song, Y., Su, W., and Mu, Y. C. (2018). Modification of bamboo shoot dietary fiber by extrusion-cellulase technology and its properties. Int. J. Food Prop. 21, 1219–1232. doi: 10.1080/10942912.2018.1479715
Sui, W., Xiao, Y., Liu, R., Wu, T., and Zhang, M. (2019). Steam explosion modification on tea waste to enhance bioactive compounds' extractability and antioxidant capacity of extracts. J. Food Eng. 261, 51–59. doi: 10.1016/j.jfoodeng.2019.03.015
Tang, X., Wang, Z., Zheng, J., Kan, J., Chen, G., and Du, M. (2023). Physicochemical, structure properties and in vitro hypoglycemic activity of soluble dietary fiber from adlay (Coix lachryma-jobi L. var. ma-yuen Stapf) bran treated by steam explosion. Front. Nutr. 10:4012. doi: 10.3389/fnut.2023.1124012
Wan, F., Feng, C., Luo, K., Cui, W., Xia, Z., and Cheng, A. (2022). Effect of steam explosion on phenolics and antioxidant activity in plants: a review. Trends Food Sci. Technol. 124, 13–24. doi: 10.1016/j.tifs.2022.04.003
Wang, T., Liang, X., Ran, J., Sun, J., Jiao, Z., and Mo, H. (2017). Response surface methodology for optimisation of soluble dietary fibre extraction from sweet potato residue modified by steam explosion. Int. J. Food Sci. Technol. 52, 741–747. doi: 10.1111/ijfs.13329
Wang, R. R., Wang, H. L., Liu, X., and Xu, C. C. (2011). Effects of different additives on fermentation characteristics and protein degradation of green tea grounds silage. Anim. Biosci. 24, 616–622. doi: 10.5713/ajas.2011.10346
Xi, H., Wang, A., Qin, W., Nie, M., Chen, Z., He, Y., et al. (2022). The structural and functional properties of dietary fibre extracts obtained from highland barley bran through different steam explosion-assisted treatments. Food Chem. 406:135025. doi: 10.1016/j.foodchem.2022.135025
Xiaoqiang, C., Jianchun, X., Wei, H., Shengrong, S., Zhengqi, W., Long, W., et al. (2019). Comparative analysis of physicochemical characteristics of green tea polysaccharide conjugates and its decolored fraction and their effect on HepG2 cell proliferation. Ind. Crop. Prod. 131, 243–249. doi: 10.1016/j.indcrop.2019.01.061
Yan, Y., Jie, W., Xueyong, R., Anthony, L., Hamid, R., Masatsugu, T., et al. (2021). Steam explosion of lignocellulosic biomass for multiple advanced bioenergy processes: a review. Renew. Sust. Energ. Rev. 154:111871. doi: 10.1016/j.rser.2021.111871
Yang, C., Si, J., Chen, Y., Xie, J., Tian, S., Cheng, Y., et al. (2022). Physicochemical structure and functional properties of soluble dietary fibers obtained by different modification methods from Mesona chinensis Benth. Residue. Food Res. Int. 157:111489. doi: 10.1016/j.foodres.2022.111489
Zhai, X., Ao, H., Liu, W., Zheng, J., Li, X., and Ren, D. (2021). Physicochemical and structural properties of dietary fiber from Rosa roxburghii pomace by steam explosion. J. Food Sci. Technol. 59, 2381–2391. doi: 10.1007/s13197-021-05254-7
Zhang, S., Zheng, L., Zheng, X., Ai, B., Yang, Y., Pan, Y., et al. (2019). Effect of steam explosion treatments on the functional properties and structure of camellia (Camellia oleifera Abel.) seed cake protein. Food Hydrocoll. 93, 189–197. doi: 10.1016/j.foodhyd.2019.02.017
Zheng, Y., Wang, Q., Huang, J., Fang, D., Zhuang, W., Luo, X., et al. (2019). Hypoglycemic effect of dietary fibers from bamboo shoot shell: an in vitro and in vivo study. Food Chem. Toxicol. 127, 120–126. doi: 10.1016/j.fct.2019.03.008
Zheng, Y., Wang, X., Tian, H., Li, Y., Shi, P., Guo, W., et al. (2021). Effect of four modification methods on adsorption capacities and in vitro hypoglycemic properties of millet bran dietary fibre. Food Res. Int. 147:110565. doi: 10.1016/j.foodres.2021.110565
Zhu, Y., Chu, J., Lu, Z., Lv, F., Bie, X., Zhang, C., et al. (2018). Physicochemical and functional properties of dietary fiber from foxtail millet (Setaria italic) bran. J. Cereal Sci. 79, 456–461. doi: 10.1016/j.jcs.2017.12.011
Zhu, L., Yu, B., Chen, H., Yu, J., Yan, H., Luo, Y., et al. (2021). Comparisons of the micronization, steam explosion, and gamma irradiation treatment on chemical composition, structure, physicochemical properties, and in vitro digestibility of dietary fiber from soybean hulls. Food Chem. 366:130618. doi: 10.1016/j.foodchem.2021.130618
Keywords: tea residue, steam explosion, dietary fiber, structure, property
Citation: Xing Y, Zhou Y, Kuang C, Luo K, Cheng Y, Wang X and Wang S (2024) Structural, physicochemical and functional properties of dietary fibers from tea residue modified by steam explosion. Front. Sustain. Food Syst. 7:1326102. doi: 10.3389/fsufs.2023.1326102
Received: 22 October 2023; Accepted: 18 December 2023;
Published: 08 January 2024.
Edited by:
Tao Wu, Tianjin University of Science and Technology, ChinaReviewed by:
Lingxiao Gong, Beijing Technology and Business University, ChinaCopyright © 2024 Xing, Zhou, Kuang, Luo, Cheng, Wang and Wang. This is an open-access article distributed under the terms of the Creative Commons Attribution License (CC BY). The use, distribution or reproduction in other forums is permitted, provided the original author(s) and the copyright owner(s) are credited and that the original publication in this journal is cited, in accordance with accepted academic practice. No use, distribution or reproduction is permitted which does not comply with these terms.
*Correspondence: Xufeng Wang, eHVmZW5nd0Bjc3VzdC5lZHUuY24=; Shaoyun Wang, c2hhb3l1bndhbmdAMTI2LmNvbQ==
†These authors have contributed equally to this work
Disclaimer: All claims expressed in this article are solely those of the authors and do not necessarily represent those of their affiliated organizations, or those of the publisher, the editors and the reviewers. Any product that may be evaluated in this article or claim that may be made by its manufacturer is not guaranteed or endorsed by the publisher.
Research integrity at Frontiers
Learn more about the work of our research integrity team to safeguard the quality of each article we publish.