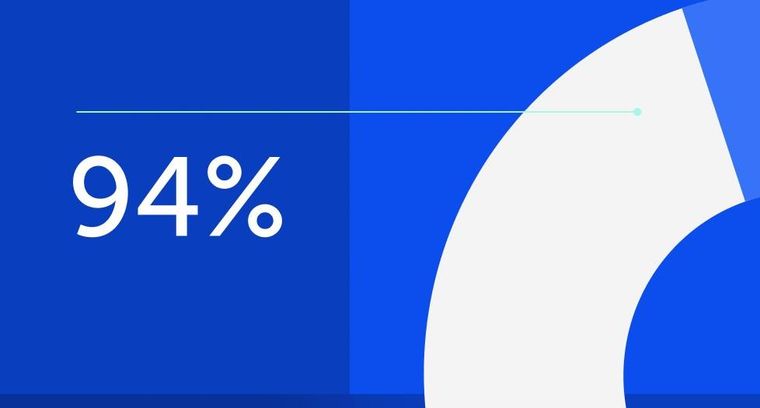
94% of researchers rate our articles as excellent or good
Learn more about the work of our research integrity team to safeguard the quality of each article we publish.
Find out more
ORIGINAL RESEARCH article
Front. Sustain. Food Syst., 23 November 2023
Sec. Nutrition and Sustainable Diets
Volume 7 - 2023 | https://doi.org/10.3389/fsufs.2023.1310426
This article is part of the Research TopicSustainable Food Consumption and Production in the 21st CenturyView all 22 articles
Introduction: In the face of escalating apprehensions surrounding food security, the sustainability of food systems, and food quality, the ingenuity of resource management strategies becomes paramount. A key component within these strategies is the enhancement of chemical fertilizer utilization, an element that bears significant weight on agricultural yields and the preservation of our environment. The emergence of Integrated Water-Fertilizer Systems (IWFS) thus presents a significant innovation in boosting the efficiency of chemical fertilizer usage, necessitating in-depth examination.
Methods: Utilizing a rigorous analytical framework that combines meta-frontier production function with a Two-Stage Residual Inclusion model, this study delves into the multi-dimensional impacts of IWFS adoption on fertilizer use efficiency among cotton growers in Xinjiang, China.
Results: Empirical evidence demonstrates that those who have adopted IWFS achieve a fertilizer use efficiency score of 0.452, markedly outstripping the mean score of 0.382 among non-adopters. Intriguingly, efficiency increases proportionately with the size of the farm, hinting at a sophisticated interplay between the adoption of technology and operational parameters.
Discussion: The research further unveils additional benefits including augmented cotton yields and diminished labor inputs among adopters. These multifaceted outcomes bear significant policy implications, highlighting the transformative potential of IWFS in promoting sustainable food systems, bolstering food security, and enhancing food quality.
Chemical fertilizers serve a dual role in contemporary society: they are essential yet problematic. On one hand, they have significantly increased global crop yields by 30–50%, supporting the livelihoods of approximately 4 billion people born in the last century (Stewart et al., 2005; Erisman et al., 2008; Zhang et al., 2020). However, their usage has also led to various environmental issues (Wu, 2011; Zhang et al., 2015; Hu et al., 2019; Yuan and Zhang, 2021; Zhang and Yu, 2021). These adverse environmental effects primarily result from the excessive release of reactive nitrogen, phosphorus, and potassium into the environment. For example, ammonia gas, nitrous oxide, and nitrogen oxidesescape into the atmosphere, contributing to air pollution and tropospheric ozone degradation (Liu et al., 2013). Likewise, the leaching and runoff of ammonium and nitrate contaminate groundwater and promote eutrophication in aquatic ecosystems (Zhang et al., 2015). Therefore, it is crucial to carefully consider the benefits and drawbacks of chemical fertilizer use in order to address both food security and environmental concerns. This study aims to explore strategies for optimizing the efficiency of chemical fertilizer use.
The concept of chemical fertilizer use efficiency has different interpretations in scholarly discourse. Agricultural scientists commonly define it as the proportion of chemical fertilizer converted into harvested crop products, a metric adopted by over 130 countries to achieve Sustainable Development Goals (Zhang et al., 2015). This efficiency is calculated using inputs and outputs either estimated from historical data and key variables (Zhang et al., 2015) or measured in the field trials (Zhang et al., 2016; Cui et al., 2018). However, this method relies on multiple parameters, and can be influenced by factors like weather conditions, soil quality, measurement errors, and farming practices (Zhang G. et al., 2014; Zhang and Yu, 2021). In contrast, economists in the agricultural sector often define it as the ratio of minimum required chemical fertilizer to the actual amount used (Wu, 2011; Hu et al., 2019). This definition considers technological constraints and other determinants. Some literature also conceptualizes it as allocation efficiency, examining the marginal utility of chemical fertilizer relative to its cost (Zhang et al., 2017; Yuan and Zhang, 2021; Zhang and Yu, 2021). For this research, we adopt the economist’s perspective and measure efficiency as the ratio of minimum required to actual chemical fertilizer use.
Integrated Water-Fertilizer Systems (IWFS) present a promising approach to enhance fertilizer use efficiency. Extensive literature has explored various factors influencing chemical fertilizer efficiency, including technological advancements and socio-economic variables. Among technological innovations, integrated soil-crop system management has been identified as a crucial element in improving fertilizer use efficiency (Chen et al., 2014). Socio-economic determinants, such as management practices, farm size, policy distortions, land leasing, and pricing, have also received significant attention in scholarly research (Zhang et al., 2015; Wu et al., 2018; Hu et al., 2019; Zhang et al., 2023a). Research by Zhang et al. (2016) suggests that platforms like the Science and Technology Backyard, which embed agricultural scientists in farming communities to enhance farming practices and technology adoption, can reduce nitrogen use by 32% in wheat and 7.5% in maize. IWFS is a notable technological innovation that combines water and fertilizer, delivering them directly to crops through drip or sprinkler irrigation systems, thus minimizing fertilizer loss through volatilization and soil residue (Zhong et al., 2012; Gao et al., 2015). IWFS integrates the advantages of mulched drip irrigation (Hu et al., 2009; Wang et al., 2014; Zhang Z. et al., 2014; Qi et al., 2018) and integrated soil-crop system management (Chen et al., 2014), offering the potential to mitigate the negative impacts of socio-economic factors, weather conditions, and soil conditions on fertilizer efficiency.
Empirical evaluations of IWFS heavily rely on field trials, which provide valuable insights into its impact on fertilizer efficiency across various crop types. While the global average for chemical fertilizer use efficiency is approximately 40%, China’s rate lags behind at around 30%, which is 15–30% lower than that of developed countries (Zhang et al., 2015; Liu et al., 2019). Zhang G. et al. (2014) demonstrated that the adoption of IWFS with drip irrigation couldincrease phosphorus fertilizer efficiency to 40.6% for corn production. Similarly, Wu et al. (2016) reported that implementing IWFS through mulched drip irrigation not only enhanced the yield and quality of potatoes but also improved the efficiencies of nitrogen, phosphorus, and potassium fertilizers by 22.7, 20.5, and 23.5%, respectively. It is important to note that these field trials are typically conducted by experts, setting an upper limit on efficiency that may not be fully attainable for average farmers. This study aims to assess the effectiveness of IWFS in enhancing chemical fertilizer use efficiency from the perspective of farmers.
This study makes two primary contributions to the existing literature. Firstly, it sheds light on the impact of IWFS on chemical fertilizer efficiency, using data from cotton farmers in Xinjiang, China. Xinjiang, known for its arid climate with high water evaporation rates, low fertilizer efficiency, and delicate ecosystems, accounts for nearly 90% of China’s cotton production (Zhang et al., 2023b). Given the significance of cotton in the region and the ecological challenges poses, it is crucial to explore the potential benefits of IWFS in improving chemical fertilizer efficiency.
Secondly, this research addresses the potential endogeneity of IWFS adoption by employing a two-stage residual inclusion approach (2SRI) and utilizes a meta-frontier analysis to accurately assess chemical fertilizer use efficiency, considering differentiated production frontiers between IWFS adopters and non-adopters (Zhang et al., 2023c). The decision to adopt IWFS is not random, as farmers self-select based on unobservable factors such as environmental motivation or inherent capabilities (Ma et al., 2018). Furthermore, IWFS not only enhances fertilizer efficiency but also crop yield, potentially leading to a shift in the production frontier (Bravo-Ureta et al., 2020).
We find that the chemical fertilizer use efficiency of IWFS adopters is 0.452, 1.6 times larger than that of non-adopters, with a mean value of 0.382. Adopting IWFS can contribute to 0.223 increases in chemical fertilizer use efficiency, and efficiency gains increase with farm size. In the context of sustainable food production and consumption, the findings of this paper have significant implications. Efficient fertilizer use facilitated by IWFS adoption can contribute to improved food security, food quality, and safety, while mitigating environmental degradation. This aligns with broader goals of agricultural sustainability, particularly in regions like Xinjiang that face complex ecological challenges.
The remainder of this paper is structured as follows: Section 2 provides background information on chemical fertilizer consumption and IWFS adoption in China. Section 3 outlines the meta-frontier production function and describes the estimation strategy using the 2SRI approach. Section 4 presents the data and descriptive statistics, followed by a discussion of empirical results in Section 5. Finally, Section 6 offers the conclusion.
China holds the position of the world’s largest consumer of chemical fertilizers. The country’s global share of nitrogen usage peaked at 30.6% in 1995, but has declined to 19.6% in 2021. Specifically, nitrogen consumption in China has risen from 0.54 million tons in 1961 to 21.27 million tons in 2021, with a peak of 30.98 million tons in 2014. This represents an average annual growth rate of 6.3% (Figure 1). Furthermore, the intensity of nitrogen use in China has significantly increased, with per hectare usage rising from 5.21 kilograms in 1961 to 166.4 kilograms in 2021, which is 2.54 times higher than the global average (Figure 2). This significant expansion in chemical fertilizer application has contributed to a 40% increase in China’s grain production (MOARA, 2015).
The intensity of chemical fertilizer application has shown an upward trend, increasing from 382.50 kilograms/hm2 in 2004 to 622.05 kilograms/hm2 in 2021 (Figure 3). However, the amount of chemical fertilizer used per kilogram of cotton has remained relatively stable at around 0.33 kilograms, primarily due to consistent increases in yield per hectare (Figure 4). It is worth noting that while fertilizer use per unit area in Xinjiang exceeds the national average, the opposite is true for fertilizer use per kilogram of cotton. The climatic conditions in Xinjiang contribute to higher cotton yields per unit area, but they may also result in lower fertilizer use efficiency. Nevertheless, the widespread adoption of IWFS has the potential to alleviate this effect.
Figure 3. Chemical fertilizer use quantity per area for cotton production. Data is from China Agricultural Product Cost–Benefit Compilation.
Figure 4. Chemical fertilizer use quantity per kilogram cotton. Calculating by authors using data from China Agricultural Product Cost–Benefit Compilation.
A significant quantity of nitrogen fertilizer is utilized in crop production; however, a considerable portion remains unabsorbed, persisting in the soil and atmosphere. The global average for chemical fertilizer use efficiency is approximately 0.4, indicating that only 40% of applied fertilizers contribute to crop yields (Zhang et al., 2015). Although China’s efficiency rate falls below the global average, there has been an upward trend, with an estimated 35.2% efficiency rate for grain production in 2014 (MOARA, 2015; MOARA, 2016). Several factors influence this efficiency, providing substantial opportunities to bridge the gap between China and developed nations (Gao et al., 2015).
Conventional methods of applying chemical fertilizer involve the spreading of solid fertilizer over soil surfaces. In this approach, the fertilizer dissolves upon encountering soil moisture, allowing the nutrients (nitrogen, phosphorus, and potassium) to be absorbed by crop roots. This technique, however, has limitations. It requires specific environmental conditions, such as adequate soil moisture, and rapid dissolution of fertilizers to prevent nutrient evaporation and air dispersal. Additionally, this method results in nutrient dispersion across the field, reducing the likelihood of nutrient uptake by roots located at a distance. Consequently, this traditional method exhibits low use efficiency.
IWFS offers a more efficient alternative by integrating solid fertilizer with water (Geng et al., 2014). This combined solution is directly delivered to the roots or foliage of crops through a piping system, shifting the focus of application from soil to crops themselves. The IWFS system enhances both water and fertilizer efficiency, promoting sustainable agricultural practices by reducing fertilizer volatilization and soil residues. Furthermore, the integration of IWFS with information and intelligent technologies enables automated, on-demand fertilizer application, reducing labor requirements.
The concept of IWFS originated from soilless agriculture practices in the United Kingdom and has evolved alongside efficient irrigation technologies like plastic conduits (Gao et al., 2015). The United States leads in global micro-irrigation areas, with IWFS being employed in 60% of potato, 25% of corn, and 33% of fruit production (Gao et al., 2015). The Netherlands also implements IWFS in its greenhouses. In China, IWFS was initially applied to cotton production in Xinjiang due to the region’s arid climate and high evaporation rates. In 2002, China invested over 100 million RMB to promote IWFS, establishing demonstration bases in more than 20 provinces, covering an area of 3 million hectares. Subsequent policies, such as the National Agricultural Water Conservation Outlines (2012–2020) and the Implementation Plan for Promoting Integrated Water-Fertilizer System (2016–2020), have further encouraged the adoption of IWFS, with the aim of expanding its use to 150 million mu by 2020.
IWFS represents a technological innovation that could alter the relationship between inputs and outputs. Therefore, different production frontiers may exist for IWFS adopters and non-adopters in cotton production. Estimating a single production function could lead to biased technical efficiency assessments (Bravo-Ureta et al., 2020). Technical efficiency is calculated relative to the production frontier and constitutes a vital component in determining chemical fertilizer use efficiency. Consistent with prior research (Battese et al., 2004; O’Donnell et al., 2008; Zhang et al., 2023c), this paper estimates separate production functions for both groups and calculates comparative technical efficiencies via a meta-frontier encompassing both.
Battese et al. (2004) and O’Donnell et al. (2008) employed a mathematical programming technique to estimate the meta-frontier production function. Huang et al. (2014) argued that such techniques lack meaningful statistical interpretation and proposed a stochastic frontier regression method instead. In the present study, we employ the stochastic frontier regression technique developed by Huang et al. (2014) to estimate the meta-frontier production function and ascertain chemical fertilizer use efficiency. This method comprises two stages.
In the first stage, separate production frontiers are estimated for IWFS adopters and non-adopters.
where denotes the logarithm of cotton yield of farmer among IWFS adopters () and non-adopters (). denotes the logarithm of the input vectors for farmer , where correspond to land, labor, and intermediate input, respectively. is the logarithm of chemical fertilizer input for farmer . The parametric vectors are to be estimated associated with the inputs. The error term is independently and identically distributed as . refers to technical inefficiency and is independently and identically distributed as .
The optimal yield can then be predicted for both groups using the estimated parameters from Equation 1. Subsequently, these optimal yields replace observed yields with optimal yields to estimate the meta-frontier production function, which serves as a smooth envelope corresponding to the separate frontiers for both groups. The meta-frontier production function for both IWFS adopters and non-adopters is as follows:
All symbols retain their meanings from Equation 1. Huang et al. (2014) suggested that the comparable technical efficiency for both groups can be calculated as follows:
Where is defined as the production technology gap relative to the meta-frontier. The comparable technical efficiency metric specifically accounts for potential differences in production technologies between IWFS adopters and non-adopters.
Based on the estimated comparable technical efficiency , we extend our analysis to compute chemical fertilizer use efficiency. By definition, an environmentally efficient farmer minimizes fertilizer input to achieve a given cotton yield, with technology held constant. Thus, we substitute with , and set in Equation 2. Rewriting Equation 2 yields:
Chemical fertilizer use efficiency equates to the ratio of minimum fertilizer input to the observed input . When expressed in logarithmic form, this is . By combining Equations 2 and 4, we can derive a quadratic equation in terms of .
The solution for chemical fertilizer use efficiency can be found in Equation 5 as:
In theory, Equation 5 offers two possible solutions. However, following Zhou et al. (2015) suggestion, we only use the solution reported in Equation 6. It is noteworthy that in equation (2 is the technology gap between the individual frontiers and meta-frontier. For Equation 6, it is essential to use farmers’ technical inefficiency, gauged by the distances from production points to the meta-frontier, thus, .
Given that farmers make a self-selection choice to adopt IWFS, the IWFS variable is potentially endogenous. To address this issue, this study employs a Two-Stage Residual Inclusion (2SRI) model. In this paper, the treatment variable is the IWFS adoption decision which is a binary variable. Thus, a 2SRI model is used to address the endogeneity issue of a binary treatment variable, while 2SLS model is used for a continuous endogenous treatment variable. A 2SRI model consists of two steps.
Step 1: To estimate the probability of farmer adopting IWFS by using a logit model:
Where is the propensity of a farmer to adopt IWFS, which is observed by . Specifically, if the farmer is an IWFS adopter, and 0 otherwise. IV serves as an instrumental variable that isolates the part of the IWFS variable uncorrelated with unobservable factors. refers to a vector of control variables, including gender, nationality, age and education level of the household head, and agricultural training, price of cotton and fertilizer, cooperation organization participation, household size, subsidy, and share of agricultural income. is a dummy variable to control county fixed effect. is an error term. is a constant. , and are parameters to be estimated.
The ratio of IWFS adoption in the village, excluding the individual farmer, is used as the instrumental variable to estimate Equation 7. This instrument must be correlated with the endogenous variable and uncorrelated with unobservable factors. A higher ratio of IWFS adoption in the village likely influences a farmer’s choice to adopt IWFS due to peer effects. Moreover, this ratio is uncorrelated with the individual farmer’s motivation or inherent environmental conservation abilities.
Step 2: Estimating the 2SRI model to reveal the impacts of IWFS adoption on chemical fertilizer use efficiency. The empirical specification is as follows:
where is farmer ‘s chemical fertilizer use efficiency. , and are defined above. is the residual term in Equation 7. We add the residual term in Equation 8 to control the unobserved heterogeneity that is correlated with IWFS variable and will result in biased estimation for IWFS variable. is an error term. is a constant. , and are parameters to be estimated.
The farm-level data used in this paper was collected in 2019 from Xinjiang, China, by Xinjiang Agricultural University and China Agricultural University. The survey methodology, which involved multistage random sampling, is detailed in Zhang et al. (2023b). First, three counties in north Xinjiang and five counties in south Xinjiang were chosen based on their agricultural output values. Second, two towns from each county and three villages from each town were randomly selected. Finally, the dataset includes information from 352 cotton producers located in 41 villages across seven counties within Xinjiang. Within this sample, the adoption prevalence of IWFS stands at 75%.
Table 1 presents a statistical summary of the dataset, including the mean values for variables, as reported in Zhang et al. (2023b). This study primarily focuses on a comparative analysis between IWFS adopters and non-adopters, revealing statistically significant differences in both inputs and outputs. IWFS adopters, for instance, manage larger farms with an average size of 118.2 mu, which is nearly eight times larger than their non-adopter counterparts. In terms of demographic and household variables, IWFS adopters tend to be older, predominantly of Han ethnicity, and more likely to participate in cooperative organizations. They also have higher levels of educational attainment, smaller household sizes, and a decreased reliance on agricultural income. Despite these differences, gender distribution and agricultural training remain consistent across both groups. It is important to note that although IWFS adopters face higher fertilizer costs, they also benefit from increased subsidies.
The notable disparities outlined in Table 1 call for additional scrutiny since they do not accommodate potential confounding variables. Consequently, the succeeding section will focus on a more rigorous examination of the impact of IWFS adoption on fertilizer use efficiency, factoring in these variables.
This section is structured to provide a detailed explanation of the estimation of separate production frontiers and the meta-frontier, followed by the calculation of chemical fertilizer use efficiency. Subsequently, a Two-Stage Residual Inclusion (2SRI) model is deployed to assess the influence of IWFS adoption on both chemical fertilizer use efficiency and intensity, which is a fundamental measure of use efficiency. Additionally, the impact of IWFS adoption on yield and labor utilization will be elucidated.
The current study employs a translog production function, integrating linear, quadratic, and interaction terms of inputs. The selection of the translog function is driven by its flexibility and its capability to approximate any unknown function to the second order (Zhou et al., 2015). The estimators linked to both separate frontiers and the meta-frontier are displayed in Table 2. It merits emphasis that, among IWFS adopters, linear terms pertaining to labor, fertilizer, and intermediate inputs, the quadratic term for intermediate inputs and numerous interaction terms exert a considerable influence on cotton yields. In contrast, for IWFS non-adopters, linear terms of fertilizer and intermediate inputs, coupled with quadratic terms for farm size and fertilizer, significantly affect cotton production.
The discrepancy in estimators between columns (1) and (2) verifies that IWFS adopters and non-adopters function under unique production frontiers. Consequently, it is essential to estimate separate frontiers for each group, culminating in a meta-frontier that encompasses both. The results from the meta-frontier estimation reveal that all terms, encompassing linear, quadratic, and interaction terms–with the exception of the labor-fertilizer interaction term–make substantial contributions to cotton yields.
Before proceeding to the computation of chemical fertilizer use efficiency, it is imperative to estimate the comparative technical efficiency. This is gauged by two metrics: the distance from the separated frontiers to the meta-frontier (denoted as the technology gap ) and the distance from the operational point to the separated frontiers (termed incomparable technical inefficiency ). Table 3 reveals that IWFS adopters are proximate to the meta-frontier, signifying a higher level of technological sophistication. The calculated comparative technical efficiencies suggest potential increases of 24.8 and 41.6% in cotton yield for IWFS adopters and non-adopters, respectively, under existing technological and input conditions, if technical inefficiencies are fully eradicated. In simpler terms, IWFS adopters are capable of either producing the same cotton yield with fewer inputs or achieving higher yields with the same number of inputs compared to non-adopters.
Table 3. The differences of technical efficiency and fertilizer use efficiency between IWFS adopter and non-adopter.
Using the parameters outlined in Table 2 and the derived comparative technical efficiency, the calculation of chemical fertilizer use efficiency for both IWFS adopters and non-adopters is performed using Equation 6. The results, presented in Table 3, reveal an average chemical fertilizer use efficiency of 0.382 among cotton farmers in Xinjiang. This indicates that more than 60% of chemical fertilizers are not utilized effectively, contributing to environmental degradation. MOARA (2015), MOARA, 2016) states that the national average efficiency of chemical fertilizer use in grain production is 0.352. However, caution should be exercised when comparing these two efficiency metrics due to variations in definitions and measurement methods. In addition, Hu et al. (2019) reveals that the average chemical fertilizer use efficiency of rice in Jiangsu province is 0.6, which is higher than that of cotton production in Xinjiang China. The differentiated chemical fertilizer use efficiency may be arised from the different capacity of fertilizer absorption between cotton and rice, and geographical condition. Few literature studied cotton farmers chemical fertilizer use efficiency. Hu et al. (2009) argue that drip irrigation could promote cotton root growth. Geng et al. (2014) calculate the technical efficiency of cotton farmers in Xinjiang, and pointed out that 15% of potential yield could be achieved without any other inputs, which is 29.1% in this paper. We also find that the efficiency for IWFS adopters is 0.452, which is 1.6 times higher than that for non-adopters.
Although the summary statistics in Table 3 suggest that IWFS adoption enhances chemical fertilizer use efficiency, a direct comparison between IWFS adopters and non-adopters lacks causal validity. The decision to adopt IWFS is endogenous, and variations in chemical fertilizer use efficiency could also be influenced by other variables. Hence, the subsequent analysis employs a 2SRI model to ascertain the causal effect of IWFS adoption on chemical fertilizer use efficiency.
The first-stage results of the 2SRI model are provided in Supplementary Table A1, indicating that the instrumental variable, represented by the ratio of IWFS adoption in the village, as well as factors such as agricultural training, subsidies, farm size, and participation in cooperative organizations, significantly influence cotton farmers’ adoption of IWFS. The impact of IWFS adoption on chemical fertilizer use efficiency is elucidated in Table 4 through the 2SRI model. Columns (1) and (2) of Table 4 indicate that the coefficients of the residual terms are statistically significant, confirming the presence of unobserved factors correlated with the IWFS adoption variable. Consequently, addressing endogeneity becomes necessary.
The coefficient for IWFS adoption in Column (1) of Table 4 indicates that adopting IWFS enhances chemical fertilizer use efficiency by 0.146 units. It is noteworthy that irrigation method used to deliver the mixed solution of chemical fertilizer and water may have different impacts on chemical fertilizer use efficiency. We do not distinguish the differentiated effects of these two methods for the following reasons. First, the data used in this paper did not distinguish the irrigation method to deliver the mixed solution of chemical fertilizer and water. Second, we found that most of surveyed farmers use drip irrigation to deliver the mixed solution to cotton.
To examine the heterogeneous effects across different farm sizes, an interaction term between IWFS adoption and farm size is introduced into the empirical model. The coefficient of this interaction term suggests that efficiency gains increase with farm size. This finding diverges from Hu et al. (2019) who reported an inverse relationship between farm size and chemical fertilizer use efficiency. A plausible rationale is that IWFS reduces reliance on managerial capabilities, thereby preventing a decline in chemical fertilizer efficiency as farm size increases. Specifically, the marginal effect of adopting IWFS on chemical fertilizer use efficiency is 0.223, indicating an average increase of 0.223 units in a farmer’s efficiency upon IWFS adoption. MOARA (2015) noted that integrated soil-crop system management led to incremental efficiencies of 0.05, 0.12, and 0.1 for nitrogen, phosphorus, and potassium fertilizers in grain production, respectively, reaching levels of 0.33, 0.24, and 0.42 in 2015. Therefore, the IWFS effect surpasses that of integrated soil-crop system management, benefiting from the synergies of both systems.
The control variables also provide noteworthy insights. For example, higher prices for both fertilizer and cotton appear to enhance chemical fertilizer use efficiency, possibly due to increased diligence in production when input and output prices rise. Conversely, the negative coefficient associated with age implies a decrease in chemical fertilizer use efficiency among older farmers.
To ensure robustness, we extend our analysis to assess the impact of IWFS adoption on the quantity of chemical fertilizer used per unit land area, which serves as a valid proxy for fertilizer use efficiency. Columns (3) and (4) present the effects of IWFS adoption on this measure. The coefficient in Column (3) is −0.386, statistically significant at the 5% level, indicating a 38.6% reduction in fertilizer use per unit area resulting from IWFS adoption. This finding is supported by Cui et al. (2018), who observed an 8.5–15.6% reduction through integrated soil-crop system management. The inclusion of an interaction term between IWFS adoption and farm size in Column (4) reveals that larger farms experience an even greater reduction in chemical fertilizer use intensity upon IWFS adoption.
Table 5 presents the results pertaining to the influence of IWFS adoption on cotton yield per unit area. The coefficient for IWFS adoption is 0.222, indicating a 22.2% increase in cotton yield per mu with the adoption of IWFS. The findings from both Tables 4, 5 collectively demonstrate that IWFS adoption achieves the dual objectives of enhancing cotton production and promoting environmental sustainability. Notably, the interaction term between IWFS adoption and farm size lacks statistical significance, aligning with the classical inverse relationship between farm size and yield per unit area, as documented by (Zhang et al., 2019). Therefore, this inverse relationship counterbalances the positive effect of IWFS adoption on yield.
In theory, IWFS applies a combination of fertilizer and water directly to the cotton roots through tubing, thereby significantly reducing labor requirements. To empirically assess this, we examine the impact of IWFS adoption on labor inputs in cotton farming, as presented in Table 6. The coefficient for IWFS adoption is −0.536, suggesting a labor input reduction of 53.6% upon IWFS adoption. Furthermore, the coefficient of the interaction term indicates that this labor-saving effect is magnified with increasing farm size.
Chemical fertilizers have played a critical role in boosting crop yields in China, yet they have precipitated environmental challenges including soil salinization, air pollution, and water contamination. To address this conundrum, it is essential to improve chemical fertilizer efficiency to maintain yield levels and mitigate environmental damage. The Integrated Water-Fertilizer Systems present a promising technological innovation in this endeavor. Nevertheless, the existing body of knowledge primarily focuses on field trials, leaving an information gap regarding its impacts from the farmers’ perspective. Utilizing data from 352 cotton farms in Xinjiang, China, this study employs meta-frontier production functions and a two-stage residual inclusion (2SRI) model to assess the implications of IWFS adoption for chemical fertilizer efficiency.
The empirical findings suggest that IWFS adopters achieve a chemical fertilizer use efficiency of 0.452, a figure that is 1.6 times greater than their non-adopting counterparts, whose average efficiency is 0.382. IWFS adoption contributes to an efficiency increase of 0.223, a gain that magnifies with farm size. Notably, IWFS’s effectiveness in improving fertilizer use efficiency outperforms that of integrated soil-crop management systems due to the combined benefits of both techniques. In addition, IWFS adoption delivers ancillary benefits such as increased cotton yields and reduced labor inputs.
To effectively tackle the urgent issues of sustainable food production and consumption, food security, and environmental conservation, it is essential for policy-makers to champion the widespread adoption of IWFS. Tailored interventions are particularly crucial for small-scale farmers who often encounter hurdles to adopting such innovative technologies. Enhancing infrastructural support can encourage these farmers to adopt IWFS, contributing to an array of interconnected objectives. For example, improving fertilizer use efficiency through IWFS can reduce environmental pollutants, promoting ecological sustainability. In the context of food security, efficient fertilizer use can optimize crop yields, ensuring a stable food supply. Additionally, minimization of fertilizer over-application could result in safer, higher-quality food products by reducing residual chemical content in crops. Therefore, policy interventions that promote IWFS have the potential to create a ripple effect, concurrently advancing environmental sustainability, food security, and food quality. As such, it is vital for policy-makers to facilitate the adoption of IWFS, especially among small-scale farmers, through enhanced infrastructural support. These initiatives are likely to make a significant contribution to the broader goals of food security, food quality, and environmental sustainability.
While offering valuable insights, this study acknowledges certain limitations. First, the analysis draws upon cross-sectional data, which precludes control for individual heterogeneity—an issue that could be addressed with the use of panel data. Second, the study does not make distinctions among different fertilizer types, including nitrogen, phosphorus, and potassium. Third, soil quality will affect fertilizer use efficiency which was documented by the effects of integrated soil-crop system management adoption. However, we did not collect soil information in the survey. These consideration presents an opportunity for further exploration in our future research.
The original contributions presented in the study are included in the article/Supplementary material, further inquiries can be directed to the corresponding authors.
YY: Data curation, Funding acquisition, Writing – original draft, Writing – review & editing. CW: Data curation, Writing – original draft, Writing – review & editing. XuZ: Funding acquisition, Visualization, Writing – review & editing. YS: Funding acquisition, Investigation, Project administration, Resources, Supervision, Writing – review & editing. XiZ: Data curation, Formal analysis, Methodology, Software, Validation, Writing – review & editing.
The author(s) declare financial support was received for the research, authorship, and/or publication of this article. This study was supported by the Natural Science Foundation of the Jiangsu Higher Education Institutions of China (no. 22KJB210002), the Natural Science Foundation of Jiangsu Province in China (no. BK20220164), the Dr. Startup project of Jinling Institute of Technology (no. jit-b-202116), National Natural Science Foundation of China (no. 72003074), the Natural Science Foundation of Xinjiang (no. 2021D01A8081), Xinjiang Tianshan Talent Training Program (no. 2022TSYCCX0093) and the Fundamental Research Funds for the Central University (No. 2662022JGQD006).
We were grateful for the financial support mentioned in “Funding.” We also extend our gratitude to readers and reviewers for suggestions improving this article.
The authors declare that the research was conducted in the absence of any commercial or financial relationships that could be construed as a potential conflict of interest.
All claims expressed in this article are solely those of the authors and do not necessarily represent those of their affiliated organizations, or those of the publisher, the editors and the reviewers. Any product that may be evaluated in this article, or claim that may be made by its manufacturer, is not guaranteed or endorsed by the publisher.
The Supplementary material for this article can be found online at: https://www.frontiersin.org/articles/10.3389/fsufs.2023.1310426/full#supplementary-material
Battese, G. E., Rao, D. P., and O’Donnell, C. J. (2004). A metafrontier production function for estimation of technical efficiencies and technology gaps for firms operating under different technologies. J. Prod. Anal. 21, 91–103. doi: 10.1023/B:PROD.0000012454.06094.29
Bravo-Ureta, B. E., Higgins, D., and Arslan, A. (2020). Irrigation infrastructure and farm productivity in the Philippines: a stochastic Meta-frontier analysis. World Dev. 135:105073. doi: 10.1016/j.worlddev.2020.105073
Chen, X., Cui, Z., Fan, M., Vitousek, P., Zhao, M., Ma, W., et al. (2014). Producing more grain with lower environmental costs. Nature 514, 486–489. doi: 10.1038/nature13609
Cui, Z., Zhang, H., Chen, X., Zhang, C., Ma, W., Huang, C., et al. (2018). Pursuing sustainable productivity with millions of smallholder farmers. Nature 555, 363–366. doi: 10.1038/nature25785
Erisman, J. W., Sutton, M. A., Galloway, J., Klimont, Z., and Winiwarter, W. (2008). How a century of ammonia synthesis changed the world. Nat. Geosci. 1, 636–639. doi: 10.1038/ngeo325
Gao, X., Du, S., Zhong, Y., Wu, Y., and Zhang, G. (2015). The development status and future of integrated water-fertilizer system. China Agric. Inf. 2, 14–19. doi: CNKI:SUN:NXTS.0.2015-04-006
Geng, X., Zhang, X., and Song, Y. (2014). Measurement of irrigation water efficiency and analysis of influential factors: an empirical study based on stochastic production frontier and cotton farmers’ data in Xinjiang. J. Nat. Resour. 29, 934–943. doi: 10.11849/zrzyxb.2014.06.003
Hu, X., Chen, H., Wang, J., Meng, X., and Chen, F. (2009). Effects of soil water content on cotton root growth and distribution under mulched drip irrigation. Agr. Sci. China. 8, 709–716. doi: 10.1016/S1671-2927(08)60269-2
Hu, L., Zhang, X., and Zhou, Y. (2019). Farm size and fertilizer sustainable use: An empirical study in Jiangsu. China. J. Integr. Agr. 18, 2898–2909. doi: 10.1016/S2095-3119(19)62732-2
Huang, C. J., Huang, T., and Liu, N. (2014). A new approach to estimating the metafrontier production function based on a stochastic frontier framework. J. Prod. Anal. 42, 241–254. doi: 10.1007/s11123-014-0402-2
Liu, S., Shi, W., Ma, H., Wang, G., Chen, Q., and Xu, M. (2019). Advances in research on efficient utilization of fertigation in fruit trees. J. Fruit Sci. 36, 366–384. doi: 10.13925/j.cnki.gsxb.20180212
Liu, X., Zhang, Y., Han, W., Tang, A., Shen, J., Cui, Z., et al. (2013). Enhanced nitrogen deposition over China. Nature 494, 459–462. doi: 10.1038/nature11917
Ma, W., Abdulai, A., and Goetz, R. (2018). Agricultural cooperatives and Investment in Organic Soil Amendments and Chemical Fertilizer in China. Am. J. Agr. Econ. 100, 502–520. doi: 10.1093/ajae/aax079
MOARA. (2015). The action plan for zero growth in fertilizer use by 2020, and the action plan for zero growth in pesticide use by 2020. Ministry of Agriculture and Rural Affairs of the People’s Republic of China.
MOARA. (2016). Implementation plan for promoting integrated water-fertilizer system (2016-2020). Ministry of Agriculture and Rural Affairs of the People’s Republic of China.
O’Donnell, C. J., Rao, D. S. P., and Battese, G. E. (2008). Metafrontier frameworks for the study of firm-level efficiencies and technology ratios. Empir. Econ. 34, 231–255. doi: 10.1007/s00181-007-0119-4
Qi, Z., Feng, H., Zhao, Y., Zhang, T., Yang, A., and Zhang, Z. (2018). Spatial distribution and simulation of soil moisture and salinity under mulched drip irrigation combined with tillage in an arid saline irrigation district, Northwest China. Agric. Water Manage. 201, 219–231. doi: 10.1016/j.agwat.2017.12.032
Stewart, W., Dibb, D. W., Johnston, A. E., and Smyth, T. J. (2005). The contribution of commercial fertilizer nutrients to food production. Agron. J. 97, 1–6. doi: 10.2134/agronj2005.0001
Wang, Z., Jin, M., Šimůnek, J., and van Genuchten, M. T. (2014). Evaluation of mulched drip irrigation for cotton in arid Northwest China. Irrig. Sci. 32, 15–27. doi: 10.1007/S00271-013-0409-X
Wu, Y. (2011). Chemical fertilizer use efficiency and its determinants in China’s farming sector. China Agr. Econ. Rev. 3, 117–130. doi: 10.1108/17561371111131272
Wu, Y., Xi, X., Tang, X., Luo, D., Gu, B., Lam, S. K., et al. (2018). Policy distortions, farm size, and the overuse of agricultural chemicals in China. Proc. Natl. Acad. Sci. 115, 7010–7015. doi: 10.1073/pnas.1806645115
Wu, X., Zeng, L., Li, J., Fang, Z., Liang, B., and Wei, F. (2016). Effects of different fertilizer treatments on yield, quality and fertilizer utilization ratio of potato under mulching drip irrigation conditions. Acta Agric. Boreali-Sinica 31, 193–198. doi: 10.7668/hbnxb.2016.05.029
Yuan, Y., and Zhang, X. (2021). Comparison of agrochemicals allocation efficiency between greenhouse and open-field vegetables in China. Sci. Rep. 11:12807. doi: 10.1038/s41598-021-92316-7
Zhang, W., Cao, G., Li, X., Zhang, H., Wang, C., Liu, Q., et al. (2016). Closing yield gaps in China by empowering smallholder farmers. Nature 537, 671–674. doi: 10.1038/nature19368
Zhang, X., Davidson, E. A., Mauzerall, D. L., Searchinger, T. D., Dumas, P., and Shen, Y. (2015). Managing nitrogen for sustainable development. Nature 528, 51–59. doi: 10.1038/nature15743
Zhang, Z., Hu, H., Tian, F., Hu, H., Yao, X., and Zhong, R. (2014). Soil salt distribution under mulched drip irrigation in an arid area of Northwestern China. J. Arid Environ. 104, 23–33. doi: 10.1016/j.jaridenv.2014.01.012
Zhang, X., Hu, L., and Yu, X. (2023a). Farmland leasing, misallocation reduction, and agricultural total factor productivity: insights from rice production in China. Food Policy 119:102518. doi: 10.1016/j.foodpol.2023.102518
Zhang, X., Jiang, S., Song, Y., Mao, H., and Zheng, H. (2023b). Impacts of digital agricultural extension on allocation inefficiency costs: evidence from cotton farmers in China. Int J Sust Dev World 2023:205. doi: 10.1080/13504509.2023.2215205
Zhang, X., Ma, W., Vatsa, P., and Jiang, S. (2023c). Short supply chain, technical efficiency, and technological change: insights from cucumber production. Agribusiness 39, 371–386. doi: 10.1002/agr.21789
Zhang, G., Wang, J., Liu, T., William, G., and Chu, G. (2014). Effect of water and P fertilizer coupling on corn yield, P uptake, and P utilization efficiency with drip irrigation in a calcareous soil. J. Plant Nutr. Fertil. 20, 1103–1109. doi: 10.11674/zwyf.2014.0506
Zhang, X., Wang, Z., Qing, P., Koemle, D., and Yu, X. (2020). Wheat yield convergence and its driving factors in countries along the belt and road. Ecosyst. Health Sust. 6:1819168. doi: 10.1080/20964129.2020.1819168
Zhang, X., and Yu, X. (2021). Short supply chain participation, and agrochemicals’ use intensity and efficiency: evidence from vegetable farms in China. China Agr. Econ. Rev. 13, 721–735. doi: 10.1108/CAER-05-2020-0108
Zhang, X., Yu, X., Tian, X., Geng, X., and Zhou, Y. (2019). Farm size, inefficiency, and rice production cost in China. J. Prod. Anal. 52, 57–68. doi: 10.1007/s11123-019-00557-6
Zhang, X., Zhou, Y., and Yan, B. (2017). Farm size and rice cost of production: a case study in Jiangsu. Issues Agric. Econ. 38, 48–55. doi: 10.13246/j.cnki.iae.2017.02.007
Zhong, K., Zheng, X., Mao, X., Lin, Z., and Jiang, G. (2012). Sugarcane bagasse derivative-based superabsorbent containing phosphate rock with water-ertilizer integration. Carbohyd. Polym. 90, 820–826. doi: 10.1016/j.carbpol.2012.06.006
Keywords: chemical fertilizer, meta-frontier, 2SRI, integrated water-fertilizer system, cotton
Citation: Yuan Y, Wang C, Zai X, Song Y and Zhang X (2023) Optimizing fertilizer use for sustainable food systems: an evaluation of integrated water-fertilizer system adoption among cotton farmers in China. Front. Sustain. Food Syst. 7:1310426. doi: 10.3389/fsufs.2023.1310426
Received: 09 October 2023; Accepted: 08 November 2023;
Published: 23 November 2023.
Edited by:
Amar Razzaq, Huanggang Normal University, ChinaReviewed by:
Jin Liu, Shanghai University of Finance and Economics, ChinaCopyright © 2023 Yuan, Wang, Zai, Song and Zhang. This is an open-access article distributed under the terms of the Creative Commons Attribution License (CC BY). The use, distribution or reproduction in other forums is permitted, provided the original author(s) and the copyright owner(s) are credited and that the original publication in this journal is cited, in accordance with accepted academic practice. No use, distribution or reproduction is permitted which does not comply with these terms.
*Correspondence: Yulan Song, c3lsNDIxQDE2My5jb20=; Xueming Zai, enhtMUBqaXQuZWR1LmNu
Disclaimer: All claims expressed in this article are solely those of the authors and do not necessarily represent those of their affiliated organizations, or those of the publisher, the editors and the reviewers. Any product that may be evaluated in this article or claim that may be made by its manufacturer is not guaranteed or endorsed by the publisher.
Research integrity at Frontiers
Learn more about the work of our research integrity team to safeguard the quality of each article we publish.