- 1Department of Life Sciences, Independent University Bangladesh (IUB), Dhaka, Bangladesh
- 2Department of Physical Sciences, Independent University Bangladesh (IUB), Dhaka, Bangladesh
- 3Institute of Technology Transfer and Innovation (ITTI), Bangladesh Council of Scientific and Industrial Research (BCSIR), Dhaka, Bangladesh
- 4Biotechnology Division, Bangladesh Agricultural Research Institute (BARI), Gazipur, Bangladesh
- 5Plasma Plus Laboratory, School of Pharmacy and Public Health, Independent University Bangladesh (IUB), Dhaka, Bangladesh
Introduction: In Bangladesh, rice straw (RS) and sawdust (SD) substrates have traditionally been used in the production of oyster mushrooms (Pleurotus ostreatus). However, the rising costs of these substrates have led many to look for alternatives.
Objectives: The present study thus focuses on the potential of waste tea leaves (WTL) for mushroom farming.
Methods: We prepared various substrate mixtures by combining WTL with SD and RS, subsequently evaluating mushroom yield and various quality parameters such as amino acid concentration, mineral content, and biological efficiency.
Results and discussion: Our investigation revealed that WTL alone is not a suitable substrate for mushroom (Pleurotus ostreatus) growth. However, when combined with SD at a 50% ratio, it significantly boosts mushroom yield and biological efficiency (BE). Conversely, a reduction in yield was noted when WTL was mixed with RS in all tested treatments, although BE surpassed 50%. In summary, incorporating WTL into both substrates proves economically viable from the BE standpoint. According to PCA analysis, the minerals and amino acid content varied based on the different substrate formulations involving WTL blending with both SD and RS at different ratios. Remarkably, mushroom fruiting bodies exhibited lower levels of Na and Fe despite these elements being present in higher concentrations in the growing substrates, suggesting the inability of P. ostreatus to bioaccumulate Na and Fe. Conversely, we observed higher bioaccumulation of Zn and P, even exceeding substrate levels. Importantly, our findings showed that mushrooms cultivated on WTL-based formulations consistently contained elevated Zn levels irrespective of substrate types, indicating that WTL enriched Zn in mushrooms. Additionally, the Fe level increased specifically in RS + WTL-based formulations. All essential and non-essential amino acids were detected, with the highest concentration of histidine, isoleucine, and methionine found in the WTL + SD formulation. Non-essential amino acids (NEAA) like alanine and glutamic acid were more prominent in formulations combining WTL with RS. This study represents the first documented exploration of the impact of WTL on the accumulation of intracellular metabolites including minerals and amino acids, in P. ostreatus.
1 Introduction
Oyster mushrooms, known scientifically as Pleurotous spp. are well recognized as primary decomposers, and their ability to thrive in fruiting bodies on a diverse range of substrates. Mushroom production is one of the most effective biotechnological approaches for recycling lignocellulosic organic waste. It can secret an array of lignocellulosic enzymes for degrading environmental pollutants (Kabel et al., 2017; Carrasco et al., 2018).
In addition to converting environmental pollutants into protein-rich human food, mushrooms also produce notable nutraceutical compounds like biologically active proteins, antioxidants, terpenoids, and sterol-which makes mushrooms a great choice for human health (Bell et al., 2022; Jana and Acharya, 2022; Kour et al., 2022). Due to the saprophytic nature of mushrooms, they derive their nourishment from the growing substrate, which has an impact on the chemical, functional, and sensory properties of mushroom fruit bodies (Saba et al., 2016). Additionally, mushrooms can accumulate various metals from their substrates, including essential nutrients like Cu, Fe, Mn, and Zn (Damodaran, 2014; Lalotra et al., 2016; Seyfferth et al., 2016; Demková et al., 2021).
In Bangladesh, Oyster mushrooms (Pleurotous ostreatous) are primarily produced on sawdust and rice straw. Currently, commercial mushroom farmers are finding it more difficult and expensive to obtain due to the rising demand in the livestock sector and mushroom farming. In light of this problem, finding substitute substrates or reducing the use of sawdust (SD) and rice straw (RS) for mushroom growing are now beginning to receive much-deserved attention.
Like other nations, tea stands out as one of the most widely consumed beverages in Bangladesh. The consumer markets for tea in the country are currently expanding, showcasing a variety of tea products. Among these, black tea holds greater popularity in local tea stalls compared to other types like oolong, green, and white tea. However, a significant challenge arises in the form of tea waste generated after extracting water-soluble components from tea leaves. The growing amount of tea waste not only poses a threat to the environment but also requires considerable labor and space for proper disposal. Consequently, finding effective solutions to manage this issue has become an urgent priority. Various measures have been proposed to address the challenge of tea waste, including utilizing it as livestock feed or converting it into fertilizer. However, implementing these solutions on a practical scale to meet the demands of tea processing businesses has proven to be a challenging task. As a result, it is crucial to critically assess and explore viable options to tackle this issue and ensure sustainable practices within the tea industry.
Interestingly, tea waste is characterized by a composition of cellulose (28–30%), hemicellulose (10–20%), and lignin (28–30%) (Sharma et al., 2015; Mahmoud et al., 2020). In contrast, a commonly used mushroom growing substrate such as rice straw and sawdust typically consists of cellulose (32–39%), hemicellulose (23–24%), and Lignin 18–36%, for rice straw (El-Tayeb et al., 2012; Limayem and Ricke, 2012) and cellulose (40–55%), Hemicellulose (24–40%), and Lignin (18–25%) for hardwood sawdust (Sun and Cheng, 2002).
Numerous studies have delved into incorporating tea waste with various substrates like sugarcane bagasse and tea leaves (Chukowry et al., 2009) cottonseed hull and tea waste (Yang et al., 2016), tea waste combined with bran (Gökhan et al., 2018). Notably, In Bangladesh, cottonseed hull, or sugarcane bagasse are not utilized for commercial mushroom growing as these are not available at farmers’ level. Therefore, it is important to consider tea waste as an alternative to sawdust and rice straw in mushroom production, either independently or in combination. Furthermore, the absence of published research on the influence of tea waste combined with sawdust and rice straw on the yield and quality of oyster mushrooms underscores the need for further investigation. In addition, it is crucial to develop and optimize a detailed substrate formula for practical large-scale production.
Therefore, this study aims to assess the commercial feasibility of using tea waste either alone or combined with RS and SD as an alternative substrate for oyster mushroom production. Additionally, our study investigates how these substrates influence essential mushroom attributes such as major mineral content, heavy metal status, and amino acid composition. Our data establishes a foundation for the utilization of tea waste for commercial oyster mushroom cultivation in tropical countries. This innovative approach has the potential to convert tea waste into valuable, marketable, edible biomass and offer a cost-effective substrate option for mushroom growers.
2 Materials and methods
2.1 Materials
Rice straw and Sawdust were sourced from local farmers and sawmills, while waste black tea leaves were collected from the nearby tea stalls. At the tea stall, black tea leaves underwent boiling with water to extract the water-soluble compound. This extract was later mixed with sugar and milk, forming the beverage served to consumers. The remaining insoluble tea leaves were discarded as waste tea leaves. The mushroom inoculum (Pleurotus ostreatus) was obtained from the National Mushroom Development Institute in Savar., Dhaka.
2.2 Treatment
Different ratios of waste tea leaves (WTL), sawdust (SD), and rice straw (RS) (detailed in Table 1) were used as substrate for mushroom cultivation. It is noteworthy that in Bangladesh, the exclusive use of SD as a substrate at the farmers’ level is not practiced due to its low yield potential, rendering it non-profitable from a business standpoint. Thus, in the experiment, one control group used SD supplemented with wheat bran, while another control group used RS without any supplement.
2.3 Packet preparation, mushroom cultivation, and data collection
2.3.1 Sawdust packet preparation and incubation
The preparation of sawdust packets was initiated by combining SD and WTL in a mixing machine. We gradually added tap water to adjust the moisture to 65–70%. Subsequently, 500 g of this moistened substrate was placed in a polypropylene bag, which was then autoclaved at 121°C for 1 h. After cooling, each bag was spawned with fungal seed and incubated at 25 ± 2°C with a humidity level of 50–60%. The packets were allowed to become densely colonized with mycelium before transferring to the culture room to induce fructification.
2.3.2 Rice straw packet preparation and incubation
Before use, dried RS was chopped into small pieces (3–5 cm in length) using a chaff cutter. These pieces of RS were completely moistened by soaking them in tap water for 24 h. Both RS and WTL were pasteurized in a clean steel drum. To briefly describe the pasteurization process, the water was heated at 60°C initially. Then the substrate was added and maintained in warm water for 30 min. Following pasteurization, both substrates were wrapped in a sterile plastic sheet (2 m × 4 m) and placed on a steep concrete surface to drain excess water, reaching a moisture level of 65–75%. Layer spawning was employed to mix fungal seed with the RS and WTL before tightly sealing in polypropylene bags (35 × 17 cm2). These bags were incubated at a temperature of 25 ± 2°C, and humidity 50–60%. Once the mycelium fully colonized and attained a dense, and white appearance, the plastic bags were transferred to the cropping room to initiate the formation of fruiting bodies.
2.3.3 Mushroom cultivation
After transferring fully colonized bags to the culture house, a few holes are punctured on the sides to facilitate the emergence of fruiting bodies. Within the cropping room, environmental conditions are carefully maintained to stimulate the formation of fruit bodies. The temperature was maintained within a range of 25 to 30°C (Agustianto et al., 2021), the humidity was 80–90% by spraying a fine mist of water three times a day (Girmay et al., 2016), and the illumination level was controlled within the ranged between 150 and 200 lux (Wu et al., 2019).
2.3.4 Data collection for yield performance
The growth and development of mushrooms were monitored daily. The time (numbers of days) required from inoculation to completion of mycelium running and time elapsed between opening the plastic bags to pinhead formation were recorded. Mushrooms were harvested when the mushroom cap surface was flat to slightly up-rolled at the cap margins. For the collection of data for yield parameters and biological efficiency, the harvested fruiting bodies in each bag were then counted and weighed. A total of two flushes were recorded. Accordingly, biological yield (g) was determined by weighing the entire cluster of fruiting bodies without removing the base of stalks. Finally, the biological efficiency (%) was calculated as follows: BE = (wf/wd) × 100, where BE represents biological efficiency, wf is the weight of the freshly harvested fruiting body, and wd is the weight of dry matter of the substrate.
2.4 Minerals and toxic metals analysis
2.4.1 Sample preparation
The obtained mushroom samples and the substrates were oven-dried at 60 ± 2°C until a constant weight was achieved. The dried samples were ground into a fine powder using a mortar & pestle. All mushroom samples were mineralized prior to analysis. In this regard, 0.5 g of powdered samples were placed into the Teflon vessels with 5 mL 65% Nitric Acid (Analar Grade, Merck, Germany) and 2 mL 30% Hydrogen peroxide (Merck, Germany). The digestion was completed in Microwave Digester (Model: Ethos One, Milestone, United States) at 180°C for 45 min (Lao et al., 2023). After the completion of digestion, the digests were transferred into 50 mL volumetric flasks and made up the volume by Class I (18 MΩ) deionized water. All reagents used for heavy metal analysis were of Analar grade.
2.4.2 Analytical methods
Analyses of mineral elements (Na, Mg, P, Zn, Fe, Cu) were performed using Flame Atomic Absorption Spectrophotometry (FAAS) (Brzezicha et al., 2019). The toxic heavy metals (Pb, and As) were performed using Graphite Furnace Atomic Absorption Spectrophotometry (GFAAS) (Dowlati et al., 2021). Both flame and electrothermal modes were operated by the Shimadzu Instrument, Model AA-7000 (Japan origin). The analytical conditions of the AAS instrument such as detection limits, wavelength (nm), cathode lamp current (mA), slit width (0.2–0.7), and flame (air-acetylene mixture) were adjusted based on the heavy metals according to available literatures. The standard recovery percentages of the analytes ranged to be from 95 to 105%.
2.5 Amino acids analysis
The mushroom samples were subjected to amino acid analysis using an Automatic Amino Acid Analyzer (Model: S4300, Sykam GmbH, Germany). The analysis followed chromatographic techniques according to Ferré et al. (2019), Isildak et al. (2023). In brief, 0.20 g of powdered mushroom samples underwent initial acidification with 25 mL of 7 N HCl, ensuring thorough homogenization, followed by filtration to remove acid-insoluble components. Subsequently, this mixture underwent a 24-h hydrolysis period within a hydrolyzer. After complete hydrolysis, any excess HCl was neutralized using a 7.5 N NaOH solution, with confirmation of the neutralization point using a pH meter (Model: Sension TM 156, HACH, United States). The final sample volume was adjusted to 250 mL in a calibrated volumetric flask containing a specialized buffer solution designed for sample dilution, maintaining a pH level of 3.4. Following this, the sample underwent a secondary filtration step using a microfilter (0.45 mm) to eliminate any unwanted foreign particles. Subsequently, a mixture comprising 100 μL of the sample solution and 900 μL of the sample dilution buffer (pH 3.4) was placed into a sample vial, resulting in a total sample volume of 1 mL (representing a 10-fold sample dilution). This diluted sample was then sequentially analyzed for amino acid content. Additionally, a standard solution of amino acids was simultaneously analyzed to ensure dependable sample quantification, quality control, and data comparisons.
2.6 Statistical analysis
One-way analysis of variance (ANOVA) was used to analyze significant differences and mean values at the 5% level of significance. One-way ANOVA was performed using SPSS software version 20 (SPSS, Inc., Chicago, Illinois, United States). DMRT test for the separation of means was applied for p < 0.05 when the ANOVA was significant (p < 0.05). Additionally, a principal component analysis (PCA) was also carried out using R-program.
3 Results
3.1 Substrate qualities
Regarding substrate qualities, we observed distinct concentration patterns of macro-nutrients such as Magnesium (Mg), Sodium (Na), and phosphorus (P). Specifically, in the SD substrate, the pattern is Mg > Na > P, while in the WTL and RS substrates, it followed Na > Mg > P. According to our analysis, trace elements including Fe, Zn, and Cu were detected in the substrate, which is significant differences, showing a clear trend of Fe > Zn > Cu. Furthermore, WTL content has a lower C/N ratio than the other two substrates as detailed in Table 2.
3.2 Time elapsed for mycelium running and pin-head formation
The total colonization time and the duration from bag opening to primordia formation of oyster mushroom cultivation on different substrates are illustrated in Figures 1A,B. Notably, when the WTL ratio was set at 75% and combined with both SD and RS substrates, a cessation of mycelium growth was observed in all samples. This suggests that WTL alone is not conducive to the growth of Pleurotus Ostreatus. Furthermore, no discernible changes were found in the number of days needed for mycelium completion (MCD) among the various substrate formulae. The time needed for completing spawn running ranged from 27 to 30 days for SD + WTL-based substrate formulations including control and 30 to 31 days for RS + WTL-based substrate formulations including control. This finding suggests that the presence of tea waste did not have a notable impact on either slowing down or speeding up mycelial growth, as the control also showed a similar timeframe. Figures 1A,B explain the time taken for the appearance of pinhead following the opening of fully colonized spawn packets. The time for pinhead initiation (TPI) varied significantly (p < 0.05) among the treatment groups. In the case of SD+ WTL preparation, T1.2 (50% WTL + 50% SD) showed the shortest TPI ranging between 6 to 8 days. To the contrary, in RS + WTL, the lowest TPI (ranging from 4 to 5 days) was observed in control (100% RS).
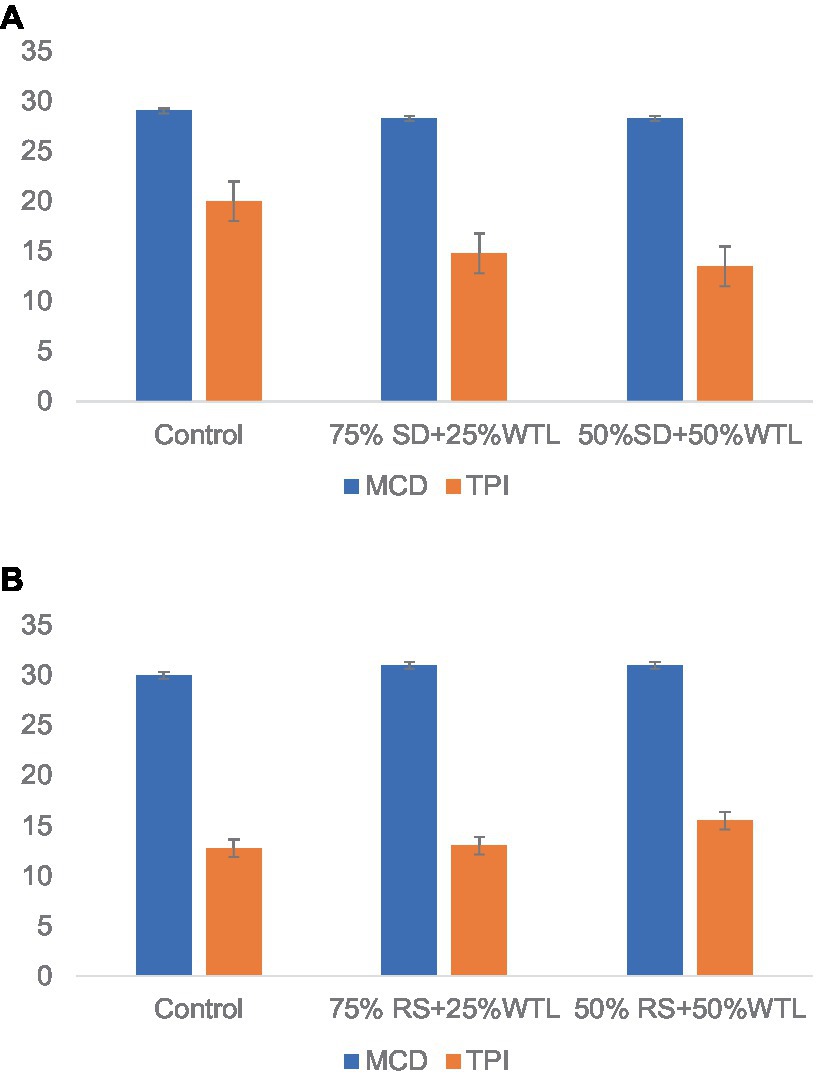
Figure 1. Time elapsed for mycelium completion (MCD) and Pinhead initiation (TPI) of oyster mushrooms. (A) SD + WTL-based formulation; (B) RS+ WTL-based formulation.
3.3 Yield and biological efficiency
The yield from two flushes was carefully recorded and analyzed as presented in Table 3. The yield significantly (p < 0.05) varied among the different WTL ratios, regardless of whether SD or RS was used as a substrate. Specifically, when 50% WTL was added to SD (T1.2), a substantial increase in yield was observed, totaling 189 g fresh mushroom per packet, with a corresponding biological efficiency (BE) of 79%. In contrast, the control group of SD exhibited the lowest yield, producing 130 g per packet and a BE of 62%. Unlikely, the RS control group showed the highest yield 357.4 g/packet, along with a remarkable BE of 89%. The lowest yield, on the other hand, was observed in 50% of RS + 50% of WTL, which also displayed a lower BE of 64%.
Biological efficiency (BE) serves as a valuable metric for assessing the efficiency of substrate conversion in the mushroom cultivation process. This metric is computed by dividing the fresh weight of harvested mushrooms by the dry weight of the cultivation substrate. A higher BE score indicates a greater likelihood of efficient substrate utilization for mushroom growth. To assess the economic viability of a particular substrate formulation for mushroom farming, it is important to note that the BE value ideally surpasses 50% as suggested by Wakchaure (2011). Moreover, it’s important to acknowledge that BE is influenced by various factors, including mushroom species, substrate composition, and environmental conditions, as highlighted by Girmay et al. (2016), Sadh et al. (2018). In our study, it was observed that the BE value exceeded 50% in all tested treatments. This implies that using WTL in the tested ratio is viable from a business standpoint, regardless of the presence of both RS and SD.
3.4 Minerals composition of mushroom fruiting bodies
Table 4 presents the mineral profile, based on dry weight, encompassing both macronutrients Sodium (Na), Magnesium (Mg), and Phosphorous (P) as well as micronutrients Zinc (Zn), Iron (Fe), and Copper (Cu) in the fruiting bodies of oyster mushrooms cultivated using different substrate formulation. The concentrations of macronutrients in the mushroom fruiting bodies varied significantly across different substrate formulations. For sodium (Na) the range was 680.95 mg/100 g to 828.24 mg/100 g, magnesium (Mg) ranged from 1594.3 mg/100gm to 2104.1 mg/100gm, while phosphorous (P) concentration ranged from 1.29 mg/100gm to 2.39 mg/100gm. Notably, formulation 75% SD+ 25% WLT tended to have the highest concentration of all studied macronutrients, except for P, which was highest in the control group. Likewise, the fruiting bodies in 75% RS + 25% WTL displayed the highest Na (4249.43 mg/100 g) and Mg (2196.29 mg/kg), while the control (100% RS) had the lowest concentration of Na (1196.33 mg/100 g) and Mg (2120.09 mg/100 g). Contrarily, P concentration was highest (2.77 mg/100 g) in control and lowest (1.83 mg/100 g) in 50% RS + 50% WTL.
Our analysis of trace elements, including Fe, Zn, and Cu in mushroom fruiting bodies exhibited significant differences, with a general trend of Cu < Fe and < Zn. Our observation aligns with the findings of Falandysz and Borovička (2013) who also asserted higher Zn concentration compared to Cu in mushrooms. In formulations based on SD + WTL, the highest Zn concentration (14.61 mg/100 g) was found in 75% SD + 25% WTL, while Fe (5.56 mg/100 g) and Cu (1.58 mg/100 g) concentration were maximum in control. Conversely, Control had the lowest Zn (8.82 mg/100 g) concentration, while 50% SD + 50% WTL had the lowest Fe (2.89 mg/100 g) and Cu (1.09 mg/100 g) concentration. In formulation using RS + WTL, 50% RS +50% WTL exhibited the highest Zn (11.54 mg/100 g), Fe (5.30 mg/100 g), and Cu (1.50 mg/100 g) concentration, while the control had the lowest Zn (5.41 mg/100 g), Fe (1.77 mg/100 g), and Cu (0.72 mg/100 g) concentration. These findings indicate that the incorporation of WTL effectively enriched the microelement content of mushroom fruiting bodies when combined with RS. In contrast, the SD+ WTL formulation yielded opposite outcomes except for Zn, where the results differed.
In our study, the levels of lead and Arsenic in the mushroom samples were found to be very low, indicating that these mushrooms are safe for consumption.
3.5 Amino acid profile of mushroom fruiting bodies
The distribution of the amino acid (AA) concentration (in g/100gm of dry matter) in mushrooms cultivated using different substrate formulations is presented in Table 5. Significant differences were observed among the tested treatments for all measured AAs. In the case of SD and WTL formulation, concentration range of AA in were as follows: Histidine (2.01–2.40), Isoleucine (0.23–0.53), Leucine (0.67–0.93), Methionine (0.19–0.53), Phenylalanine (0.49–1.04), Valine (0.73–1.05), Lysine (0.81–1.04), Threonine (0.81–1.18), Alanine (0.81–1.56), Aspartic acid (1.79–2.76), Glutamic acid (3.81–4.48), Cystine (1.35–2.25), Glycine (0.65–1.23), Proline (0.60–1.01), Argine (0.99–1.44), Serine (0.98–1.45) and Tyrosine (0.44–0.95). For the RS and WTL preparation, the AA concentration ranges were as follows: Histidine (0.97–1.99), Isoleucine (0.34–0.62), Leucine (0.93–1.11), Methionine (0.20–0.33), Phenylalanine (0.36–1.11), Valine (0.77–1.05), Lysine (0.41–0.75), Threonine (0.66–0.89), Alanine (0.98–1.17), Aspartic acid (1.74–2.66), Glutamic acid (2.96–3.11), Cystine (1.07–1.30), Glycine (0.73–0.93), Proline (0.62–0.93), Argine (0.78–0.95), Serine (0.65–0.95) and Tyrosine (0.41–0.82). Interestingly, when incorporating WTL with SD, there was a notable increase of EAA such as Histidine, Isoleucine, and Methionine. Non-essential amino acids (NEAA) like Alanine and Glutamic acid also exhibited an increase in concentration when WTL was combined with RS.
3.6 Principal components analysis
To enhance visual interpretation and simplicity in understanding the data, a principal component analysis was conducted (Figure 2).
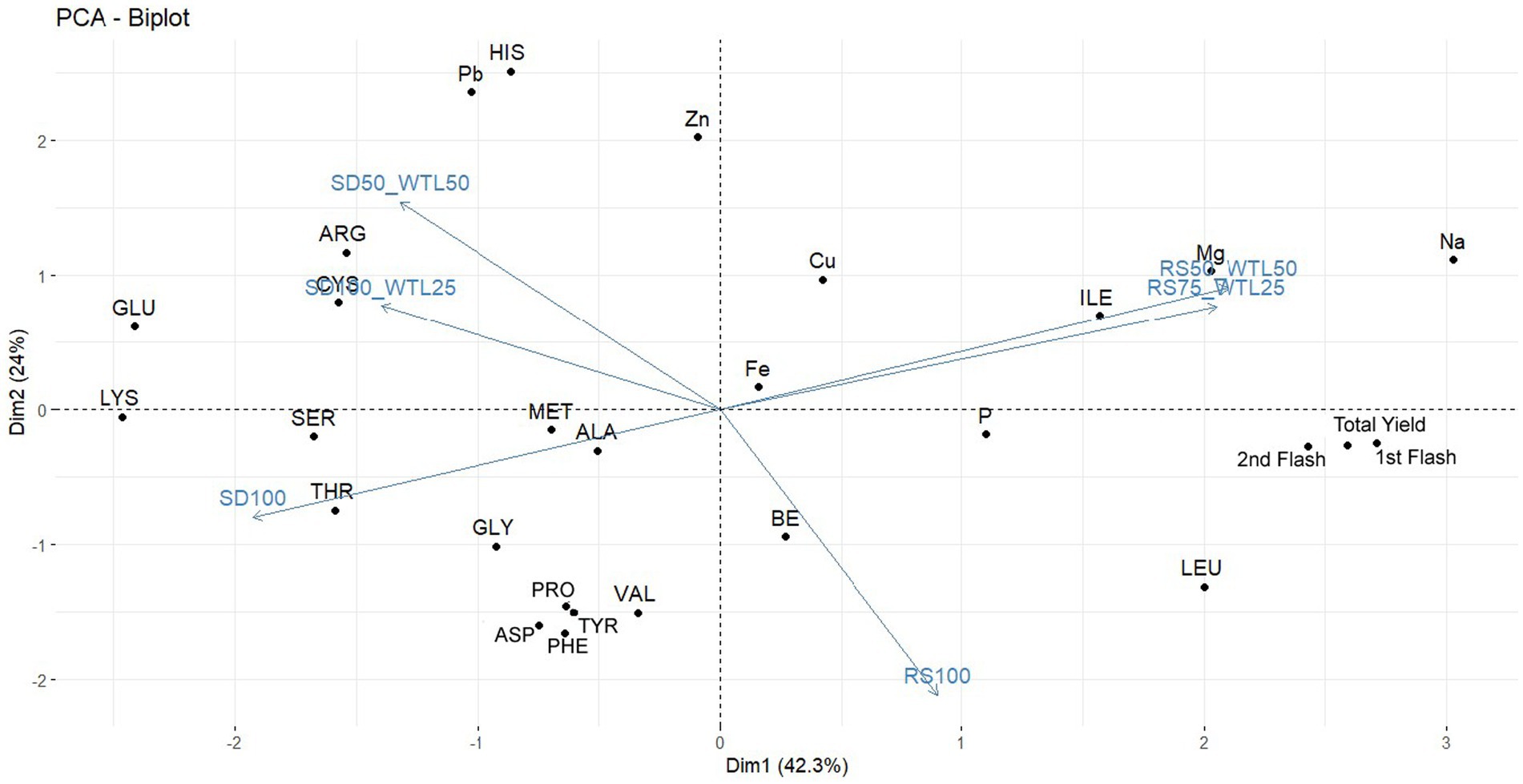
Figure 2. Principal component biplot of some qualitative traits of mushrooms among the substrate formulations.
The first principal component (PC1) explained 42.3% of the variability, while the second principal component (PC2) contributed a modest 24% to the overall variation. These outcomes differed in magnitude and signified a stronger positive relationship with all the examined traits when integrating WTL in both RS and SD. The elongated arrows, representing 50% of the WTL ratio, signify the highest observed variabilities in mushroom qualities across both substrates.
4 Discussion
According to Lu et al. (2021), the substrate carbon-nitrogen ratio (C/N) significantly influences the mycelial growth and fruiting body development of edible fungi. In our study, a substantial variation in the C/N ratio among the materials was observed, with WTL exhibiting a low C/N ratio (25.2) compared to the other two substrates, SD (244) and RS (63). The control group using RS outperformed all tested treatments in our study, including formulations based on RS + WTL, SD control, and SD + WTL-based formulation, in terms of mycelium growth, biological yield, and biological efficiency. Noteworthy, no mushroom mycelium growth was observed in a 75% WTL combination, regardless of substrate, which aligns with the findings by Yang et al. (2016). This lack of growth may be attributed to the content less amount of cellulose, hemicelluloses, low C/N, and greater amount of lignin as described (Sharma et al., 2015; Mahmoud et al., 2020). It is essential to note that the variation in the yield of Pleurotus spp. was primarily driven by differences in the physical and chemical composition of substrate formulations, including cellulose, hemicellulose, lignin, mineral content, and C/N ration (Suwannarach et al., 2022). Furthermore, research by Yang (2000) and Mintesnot et al. (2014) suggested that substrates having greater C/N ratio are conducive to mycelial growth and less favorable for fruiting body formation. Additionally, a substrate with a high lignin concentration impedes mushroom growth by limiting enzyme accessibility (Kaya and Cam, 2022). Noteworthy, our study revealed a significant increase in yield and biological efficiency when combining WTL and SD, suggesting that WTL may aid in reducing the substrate’s C/N ratio.
Mushrooms are important sources of minerals and play a vital role in the proper development of the human body. Thus, we evaluated whether the mineral contents of the substrate influenced the yield and quality of Pleurotus ostreatus. The analysis of minerals elements (Na, Mg, P, Zn, Fe, and Cu) in three substrates used in the study revealed macronutrient Na and Mg concentrations were high while P concentration was significantly lower (Table 2). Similar trends were also observed in mushroom fruiting bodies. Furthermore, our result established that mushrooms possess the ability to accumulate minerals in substantial amounts, often exceeding those present in the substrate which is supported by the line findings (Florczak et al., 2014; Mleczek et al., 2016). Surprisingly, the P concentration in the SD substrate was significantly lower than RS and WTL substrates. However, this difference was not reflected in the elemental composition of the fungal fruiting bodies, as a significantly higher p value was observed in the fruiting bodies produced on control SD.
Our result also showed that Na content in the substrate is higher than that found in the mushroom fruiting bodies. This suggested that mushrooms lack the capacity for bioaccumulation of Na. In contrast, multiple investigations have shown that mushrooms bioconcentrate Mg and P in their fruiting bodies (Frankowska et al., 2010; Kojta and Falandysz, 2016; Li et al., 2017; Malinowski et al., 2021; Hultberg et al., 2023). The biological conversion factor (BCF) of Mg ranged from 1.5 to 7.2, and P ranged from 10 to 50 times (Rudawska and Leski, 2005; Frankowska et al., 2010; Falandysz and Borovička, 2013). Our research finding confirmed that Pleurotus ostreatus exhibits the ability to bioconcentrate Mg and P but not Na. In RS + WTL formulas, micronutrients like Zn, Fe, and Cu concentration are significantly increased with the addition of tea leaves, while interestingly significantly decreased by the addition of WTL to SD except Zn. Consistent with other studies, we found that in mushroom fruiting bodies, the amount of Zn is higher than that of Cu irrespective of the substrate. Outstandingly, zinc (Zn) levels in fruiting bodies were significantly increased in both SD and RS substrates blended with WTL in all tested ratios. So, our study claimed, that the enrichment of Zn in mushrooms can be achieved by incorporating WTL as a supplement in the substrate. Zinc stands out as a crucial trace element essential for various aspects of human metabolism including transcription of DNA, translation of RNA, and cellular division (Brown et al., 2004). The introduction of Zn supplementation has shown substantial and positive effects on both growth and weight gain, as evidenced by the work of Rahman et al. (2016). Furthermore, it is noteworthy to note that the substrate’s Fe content was significantly higher than that of mushroom fruiting bodies. Our findings contradict those made by Almeida et al. (2015), who claimed that P. ostreatus was capable of bioaccumulation of Fe. It is well known that Fe uptake by fungus is a complex process, and it depends on the speciation of Fe (II) and Fe (III) forms (Philpott, 2006). The present study reveals that the WTL had a remarkable impact on the mineral content in the fruiting bodies of the mushrooms. More importantly, our results for trace elements falling within acceptable nutritional levels for human consumption are shown in Table 6.
When comparing mushrooms to fruits and vegetables, mushrooms emerge as superior sources of both essential amino acids (EAAs) and non-essential amino acids (NEAAs) as reported in numerous literature (Yang et al., 2001). The data of AA composition shows that mushrooms particularly rich in EAA like histidine and NEAA such as glutamic, aspartic acids, and cystine irrespective of the growing substrate. In contrast, methionine and isoleucine are presented in relatively lower quantities, categorizing them as limiting amino acids. The significant increase in glutamic and aspartic acid content in mushrooms is attributed to their roles as precursors for other amino acids such as serine, alanine, lysine, and proline, among others (Akindahunsi and Oyetayo, 2006). Markedly, the umami flavor associated with certain foods including mushrooms can be attributed to the presence of glutamic and aspartic acids. Furthermore, our study observed that the addition of WTL to substrate formulations resulted in a significant reduction in the concentration of both essential and non-essential amino acids, except for histidine in SD-WTL formulates and isoleucine, alanine in RS-WTL-based preparation. It is important to highlight that the amino acid concentration in WTL-based formulation exceeded the levels reported in the study involving Pleurotus spp. grown on perilla stalks (Li et al., 2017) and a substrate composed of wheat straw combined with grape marc (Tagkouli et al., 2020).
5 Limitations and future research
Our investigation explored the impact of WTL on the yield and qualities of oyster mushrooms (Pleurotus ostreatus). Nevertheless, certain limitations should be acknowledged. Firstly, our study concentrated exclusively on fresh mushrooms of Pleurotus ostreatus. Secondly, we utilized black tea waste sourced from the local tea stall. Future research endeavors could extend to other oyster mushroom species and consider diverse types of tea waste including industrial sources. Furthermore, assessing the applicability of our findings in varied geographical contexts, such as Bangladesh or other countries, would be valuable for future studies.
Data availability statement
The original contributions presented in the study are included in the article/supplementary material, further inquiries can be directed to the corresponding author.
Author contributions
RA: Writing – original draft, Investigation. MN: Investigation, Writing – original draft. MI: Data curation, Formal analysis, Writing – review & editing. MR: Writing – review & editing. SY: Data curation, Writing – review & editing, Methodology. SR: Data curation, Writing – review & editing, Methodology. JK: Writing – review & editing, Conceptualization, Project administration, Supervision, Writing – original draft.
Funding
The author(s) declare financial support was received for the research, authorship, and/or publication of this article. This work was supported by a sponsored research grant (project number 2022-SELS-03) from Independent University Bangladesh.
Conflict of interest
The authors declare that the research was conducted in the absence of any commercial or financial relationships that could be construed as a potential conflict of interest.
Publisher’s note
All claims expressed in this article are solely those of the authors and do not necessarily represent those of their affiliated organizations, or those of the publisher, the editors and the reviewers. Any product that may be evaluated in this article, or claim that may be made by its manufacturer, is not guaranteed or endorsed by the publisher.
References
Agustianto, K., Wardana, R., Destarianto, P., Mulyadi, E., and Wiryawan, I. G. (2021). Development of automatic temperature and humidity control system in kumbung (oyster mushroom) using fuzzy logic controller. IOP Conf. Ser.: Earth Environ. Sci. 672:12090. doi: 10.1088/1755-1315/672/1/012090
Akindahunsi, A. A., and Oyetayo, F. L. (2006). Nutrient and antinutrient distribution of edible mushroom, Pleurotus tuber-regium (fries) singer. LWT Food Sci. Technol. 39, 548–553. doi: 10.1016/j.lwt.2005.04.005
Almeida, S. M., Umeo, S. H., Marcante, R. C., Yokota, M. E., Valle, J. S., Dragunski, D. C., et al. (2015). Iron bioaccumulation in the mycelium of Pleurotus ostreatus. Braz. J. Microbiol. 46, 195–200. doi: 10.1590/S1517-838246120130695
Bell, V., Silva, C. R. P. G., Guina, J., and Fernandes, T. H. (2022). Mushrooms as future generation healthy foods. Front. Nutr. 9:1050099. doi: 10.3389/fnut.2022.1050099
Brown, K. H., Rivera, J. A., Bhutta, Z., Gibson, R. S., King, J. C., Lönnerdal, B., et al. (2004). Assessment of the risk of zinc deficiency in populations and options for its control. Food Nutr. Bull. 25, S99–S203.
Brzezicha, J., Grembecka, M., Grochowska, I., Falandysz, J., and Szefer, P. (2019). Elemental composition of selected species of mushrooms based on a chemometric evaluation. Ecotoxicol. Environ. Saf. 173, 353–365. doi: 10.1016/j.ecoenv.2019.02.036
Carrasco, J., Zied, D. C., Pardo, J. E., Preston, G. M., and Pardo-Giménez, A. (2018). Supplementation in mushroom crops and its impact on yield and quality. AMB Express 8:146. doi: 10.1186/s13568-018-0678-0
Chukowry, N. D., Nowbuth, R., and Lalljee, B. (2009). Evaluation of tea wastes as an alternative substrate for oyster mushroom cultivation. Univ. Maurit. Res. J. 15, 458–473.
Damodaran, D. (2014). Uptake of certain heavy metals from contaminated soil by mushroom—Galerina vittiformis. Ecotoxicol. Environ. Saf. 104, 414–422. doi: 10.1016/j.ecoenv.2013.10.033
Demková, L., Árvay, J., Hauptvogl, M., Michalková, J., Šnirc, M., Harangozo, L., et al. (2021). Mercury content in three edible wild-growing mushroom species from different environmentally loaded areas in Slovakia: an ecological and human health risk assessment. J. Fungi Open Access Mycol. J. 7:434. doi: 10.3390/jof7060434
Dowlati, M., Sobhi, H. R., Esrafili, A., Farzadkia, M., and Yeganeh, M. (2021). Heavy metals content in edible mushrooms: a systematic review, meta-analysis and health risk assessment. Trends Food Sci. Technol. 109, 527–535. doi: 10.1016/j.tifs.2021.01.064
El-Tayeb, T. S., Abdelhafez, A. A., Ali, S. H., and Ramadan, E. M. (2012). Effect of acid hydrolysis and fungal biotreatment on agro-industrial wastes for obtainment of free sugars for bioethanol production. Braz. J. Microbiol. 43, 1523–1535. doi: 10.1590/S1517-838220120004000037
Falandysz, J., and Borovička, J. (2013). Macro and trace mineral constituents and radionuclides in mushrooms: health benefits and risks. Appl. Microbiol. Biotechnol. 97, 477–501. doi: 10.1007/s00253-012-4552-8
Ferré, S., González-Ruiz, V., Guillarme, D., and Rudaz, S. (2019). Analytical strategies for the determination of amino acids: past, present and future trends. J. Chromatogr. B 1132:121819. doi: 10.1016/j.jchromb.2019.121819
Florczak, J., Chudy, J., and Barasi, M. (2014). wybrane składniki odżywcze grzybów dziko rosnących uszaka bzowego (hirneola auricula judae), boczniaka ostrygowatego (pleurotus ostreatus) i zimówki aksamitnotrzonowej (flammulina Velutipes).
Frankowska, A., Ziółkowska, J., Bielawski, L., and Falandysz, J. (2010). Profile and bioconcentration of minerals by King bolete (boletus edulis) from the Płocka dale in Poland. Food Addit. Contam. Part B 3, 1–6. doi: 10.1080/19440040903505232
Girmay, Z., Gorems, W., Birhanu, G., and Zewdie, S. (2016). Growth and yield performance of Pleurotus ostreatus (Jacq. Fr.) Kumm (oyster mushroom) on different substrates. AMB Expr. 6:87. doi: 10.1186/s13568-016-0265-1
Gökhan, B., Taşkin, H., Güzelel, Y. E., Büyükalaca, O., and Akilli, H. (2018). Use of the tea wastes in Pleurotus cultivation as an alternative substrate material in Turkey under conventional controlled climate. Int. J. Adv. Sci. Eng. Technol. 6, 13–16.
Hultberg, M., Asp, H., Bergstrand, K. J., and Golovko, O. (2023). Production of oyster mushroom (Pleurotus ostreatus) on sawdust supplemented with anaerobic digestate. Waste Manag. 155, 1–7. doi: 10.1016/j.wasman.2022.10.025
Isildak, Ö., Güneş, G., and Özbek, O. (2023). Use of some amino acid potentiometric biosensors as detectors in ion chromatography. Chem. Pap. 77, 3317–3325. doi: 10.1007/s11696-023-02706-6
Jana, P., and Acharya, K. (2022). Mushroom: a new resource for anti-Angiogenic therapeutics. Food Rev. Intl. 38, 88–109. doi: 10.1080/87559129.2020.1721529
Kabel, M. A., Jurak, E., Mäkelä, M. R., and de Vries, R. P. (2017). Occurrence and function of enzymes for lignocellulose degradation in commercial Agaricus bisporus cultivation. Appl. Microbiol. Biotechnol. 101, 4363–4369. doi: 10.1007/s00253-017-8294-5
Kaya, M., and Cam, M. (2022). Eritadenine: pressurized liquid extraction from Lentinula edodes and thermal degradation kinetics. Sustain. Chem. Pharm. 29:100809. doi: 10.1016/j.scp.2022.100809
Kojta, A. K., and Falandysz, J. (2016). Metallic elements (ca, hg, Fe, K, mg, Mn, Na, Zn) in the fruiting bodies of boletus badius. Food Chem. 200, 206–214. doi: 10.1016/j.foodchem.2016.01.006
Kour, H., Kour, D., Kour, S., Singh, S., Jawad Hashmi, S. A., Yadav, A. N., et al. (2022). Bioactive compounds from mushrooms: emerging bioresources of food and nutraceuticals. Food Biosci. 50:102124. doi: 10.1016/j.fbio.2022.102124
Lalotra, P., Gupta, D., Yangdol, R., Sharma, Y., and Sk, G. (2016). Bioaccumulation of heavy metals in the sporocarps of some wild mushrooms. Curr. Res. Environ. Appl. Mycol. 6, 159–165. doi: 10.5943/cream/6/3/2
Lao, Y. M., Qu, C. L., Zhang, B., and Jin, H. (2023). Development and validation of single-step microwave-assisted digestion method for determining heavy metals in aquatic products: health risk assessment. Food Chem. 402:134500. doi: 10.1016/j.foodchem.2022.134500
Li, H., Zhang, Z., Li, M., Li, X., and Sun, Z. (2017). Yield, size, nutritional value, and antioxidant activity of oyster mushrooms grown on perilla stalks. Saudi J. Biol. Sci. 24, 347–354. doi: 10.1016/j.sjbs.2015.10.001
Limayem, A., and Ricke, S. C. (2012). Lignocellulosic biomass for bioethanol production: current perspectives, potential issues and prospects. Prog. Energy Combust. Sci. 38, 449–467. doi: 10.1016/j.pecs.2012.03.002
Lu, M., Chi, Y., Dong-Lai, X., Xiao-Yu, L., Xiao-Ling, J., Zheng-He, Y., et al. (2021). Effects of different substrate carbon to nitrogen ratio (C/N) on the growth and development of Sparassis latifolia. Mycosystema 40, 3196–3213. doi: 10.13346/j.mycosystema.210331
Mahmoud, D. A. R., Allam, M. A., and Mohammad, M. (2020). Tea wastes as an alternative sustainable raw material for ethanol production. Egypt. J. Chem. 63, 5–6. doi: 10.21608/ejchem.2020.21785.2293
Malinowski, R., Sotek, Z., Stasińska, M., Malinowska, K., Radke, P., and Malinowska, A. (2021). Bioaccumulation of macronutrients in edible mushrooms in various habitat conditions of NW Poland—role in the human diet. Int. J. Environ. Res. Public Health 18:8881. doi: 10.3390/ijerph18168881
Mintesnot, B., Ayalew, A., and Kebede, A. (2014). Evaluation of biomass of some invasive weed species as substrate for oyster mushroom (Pleurotus spp.) cultivation. Pak. J. Biol. Sci. 17, 213–219. doi: 10.3923/pjbs.2014.213.219
Mleczek, M., Magdziak, Z., Gąsecka, M., Niedzielski, P., Kalač, P., Siwulski, M., et al. (2016). Content of selected elements and low-molecular-weight organic acids in fruiting bodies of edible mushroom boletus badius (Fr.) Fr. From unpolluted and polluted areas. Environ. Sci. Pollut. Res. 23, 20609–20618. doi: 10.1007/s11356-016-7222-z
Philpott, C. C. (2006). Iron uptake in fungi: a system for every source. Biochim. Biophys. Acta 1763, 636–645. doi: 10.1016/j.bbamcr.2006.05.008
Rahman, S., Ahmed, T., Rahman, A. S., Alam, N., Ahmed, A. M. S., Ireen, S., et al. (2016). Status of zinc nutrition in Bangladesh: the underlying associations. J. Nutr. Sci. 5:e25. doi: 10.1017/jns.2016.17
Rudawska, M., and Leski, T. (2005). Macro-and microelement contents in fruiting bodies of wild mushrooms from the Notecka forest in west-Central Poland. Food Chem. 92, 499–506. doi: 10.1016/j.foodchem.2004.08.017
Saba, M., Falandysz, J., and Nnorom, I. C. (2016). Mercury bioaccumulation by Suillus bovinus mushroom and probable dietary intake with the mushroom meal. Environ. Sci. Pollut. Res. Int. 23, 14549–14559. doi: 10.1007/s11356-016-6558-8
Sadh, P. K., Duhan, S., and Duhan, J. S. (2018). Agro-industrial wastes and their utilization using solid state fermentation: a review. Bioresour. Bioprocess. 5:1. doi: 10.1186/s40643-017-0187-z
Seyfferth, A. L., McClatchy, C., and Paukett, M. (2016). Arsenic, Lead, and cadmium in U.S. mushrooms and substrate in relation to dietary exposure. Environ. Sci. Technol. 50, 9661–9670. doi: 10.1021/acs.est.6b02133
Sharma, N., Rai, A., Rai, P., and Singh, S. (2015). Environmental factors affecting edible and medicinal mushroom production (p. NA).
Sun, Y., and Cheng, J. (2002). Hydrolysis of lignocellulosic materials for ethanol production: a review. Bioresour. Technol. 83, 1–11. doi: 10.1016/S0960-8524(01)00212-7
Suwannarach, N., Kumla, J., Zhao, Y., and Kakumyan, P. (2022). Impact of cultivation substrate and microbial community on improving mushroom productivity: a review. Biology 11:569. doi: 10.3390/biology11040569
Tagkouli, D., Kaliora, A., Bekiaris, G., Koutrotsios, G., Christea, M., Zervakis, G. I., et al. (2020). Free amino acids in three Pleurotus species cultivated on agricultural and agro-industrial by-products. Molecules 25:4015. doi: 10.3390/molecules25174015
Wakchaure, G. C. (2011). Production and Marketing of Mushrooms: global and National Scenario, 15–22. doi: 10.13140/RG.2.1.5012.3682
World Health Organization (1996). Trace Elements in Human Nutrition and Health. Geneva, Switzerland: World Health Organization.
Wu, N., Tian, F., Moodley, O., Song, B., Jia, C., Ye, J., et al. (2019). Optimization of agro-residues as substrates for Pleurotus pulmonarius production. AMB Expr. 9:184. doi: 10.1186/s13568-019-0907-1
Yang, X. M. Cultivation of edible mushroom. Beijing: China Agriculture Press (2000). [Google Scholar]
Yang, D., Liang, J., Wang, Y., Sun, F., Tao, H., Xu, Q., et al. (2016). Tea waste: an effective and economic substrate for oyster mushroom cultivation. J. Sci. Food Agric. 96, 680–684. doi: 10.1002/jsfa.7140
Keywords: tea waste, oyster mushroom, yield, biological efficiency, mineral, amino acid, PCA
Citation: Ahmed R, Niloy MAHM, Islam MS, Reza MS, Yesmin S, Rasul SB and Khandakar J (2024) Optimizing tea waste as a sustainable substrate for oyster mushroom (Pleurotus ostreatus) cultivation: a comprehensive study on biological efficiency and nutritional aspect. Front. Sustain. Food Syst. 7:1308053. doi: 10.3389/fsufs.2023.1308053
Edited by:
Ilija Djekic, University of Belgrade, SerbiaReviewed by:
Tyler John Barzee, University of Kentucky, United StatesPoonam Yadav, Banaras Hindu University, India
Copyright © 2024 Ahmed, Niloy, Islam, Reza, Yesmin, Rasul and Khandakar. This is an open-access article distributed under the terms of the Creative Commons Attribution License (CC BY). The use, distribution or reproduction in other forums is permitted, provided the original author(s) and the copyright owner(s) are credited and that the original publication in this journal is cited, in accordance with accepted academic practice. No use, distribution or reproduction is permitted which does not comply with these terms.
*Correspondence: Jebunnahar Khandakar, ZHIua2hhbmRha2FyQGl1Yi5lZHUuYmQ=