- 1Department of Environmental Science, Policy, and Management, University of California, Berkeley, Berkeley, CA, United States
- 2School of Agriculture, Food, and Wine, University of Adelaide, Glen Osmond, SA, Australia
Farmers faced with increasingly limited access to freshwater for irrigation are utilizing dry farming techniques to produce fruit, vegetable, and grain crops. Defined as crop production during a dry season using little or no irrigation, dry farming relies on water stored in the soil and requires specialized soil management, healthy soil biology, and careful selection of crop varieties. We investigated these three factors in a dry-farmed tomato (Solanum lycopersicum) field trial with three indeterminate varieties (Early Girl, Momotaro, and Zapotec) and two determinate genotypes (76R and rmc), the latter serving as a model system for studying the role of arbuscular mycorrhizal (AM) fungi. While five prior years of contrasting surface tillage practices changed soil properties, they did not affect marketable tomato yields or quality, nor affect plant physiology, including plant water use efficiency [intrinsic (WUEi) and long-term (δ13C)], gas exchange, stem water potential (Ψstem), and leaf relative water content (RWC). Early Girl produced a greater number of fruits and fewer non-marketable fruits than Momotaro and Zapotec. Although AM fungi root colonization was very low for both wildtype mycorrhizal genotype 76R and its reduced mycorrhizal mutant rmc, it differed significantly depending on genotype and tillage treatment. 76R produced slightly greater marketable fruit, but physiological responses did not differ significantly between genotypes. This study does not provide field-based evidence that soil chemical and biological changes resulting from no-till influence dry-farmed tomato productivity, while genotype had a clear impact on tomato yield and quality. Field-scale tillage experiments may better capture soil physical changes that affect water availability, especially at depth, and should be examined.
1 Introduction
With the frequency and intensity of droughts projected to increase with climate change (IPCC, 2023), future crop production will be heavily constrained by the availability of water (Caretta et al., 2022), especially in regions where current crop production relies on irrigation. Faced with increasingly limited access to freshwater for irrigation (Acevedo et al., 2022), farmers are utilizing dry farming techniques as a climate resilience strategy to conserve soil moisture and produce food crops in water-limited regions globally (Garett, 2014).
For centuries, agricultural practices in arid regions have demonstrated the ability to thrive in the absence of irrigation, and farming in these conditions can take a variety of forms. For instance, indigenous people historically produced food in areas with minimal rainfall (Nabhan and McKibben, 2013), while much of the global grain production utilizes dryland farming systems that rely on precipitation during crop growth without supplemental irrigation (Parr et al., 1990; CAWSI, 2023). In Mediterranean climates, dry farming is practiced in regions that typically receive at least 500 mm of mean annual precipitation and have mild marine summers. Dry farming is defined as crop production during a dry season using little or no irrigation, relying instead on water stored in the soil from previous wet season rains (Leap et al., 2017; Garett, 2019). To date, fruit and vegetable dry farming in Mediterranean regions of the Western United States has not been well represented in scientific literature. In this region, tomatoes are becoming a popular choice for dry farming.
The benefits of dry farming include reducing water reliance and costs. Farmers select fields with deep, clay-rich soil and work to build water-holding capacity. With climate change and regulations making access to freshwater increasingly variable, expensive, or even unavailable (Hoegh-Guldberg et al., 2018; Acevedo et al., 2022), dry farming allows farmers to dramatically reduce irrigation needs. With little to no irrigation inputs, dry farming reduces weeds and may require fewer inputs than irrigated production since yield potential is reduced (Garett, 2019). Reductions in yields can be offset by the enhanced flavor of dry-farmed produce, making them prized by consumers and chefs and increasing selling prices for farmers (Leap et al., 2017; Acevedo et al., 2022). These cost savings from reduced irrigation water needs, as well as high prices for dry-farmed tomatoes in local markets (e.g., $8.78 USD/kg in a local Berkeley, CA market), make this a desirable system for some farmers. While dry farming can reduce input costs for irrigation and fertilizer, whether this leads to profitability depends on the balance between yield reduction and an increase in selling price from improved quality.
The productivity of dry farming, in terms of crop yield and quality, is dependent on optimal soil health, achieved through thoughtful management and careful selection of crop varieties. Farmers implementing dry farming employ a wide range of principles to improve soil health, including maximizing soil cover (e.g., cover cropping), minimizing soil disturbance, and increasing on-farm biodiversity (Socolar et al., in preparation). In particular, minimizing soil disturbance may offer opportunities for dry farming. While dry farmers implement diverse management techniques, such as dust mulch cultivation, lower planting density, and earlier planting times, to conserve soil moisture and minimize evaporation, the effect of soil disturbance on dry-farmed fruit and vegetable production is understudied (Leap et al., 2017; Dry Farming Institute, 2022). No-till most rapidly and distinctly impacts surface soil (Shaver et al., 2002; Blanco-Canqui and Ruis, 2018), including soil biological and chemical properties, such as soil organic matter, microbial activity, and diversity, and nutrient cycling (Pittelkow et al., 2015). While previous studies indicate that no-till impacts on crop yields are variable, no-till performs best under rainfed conditions in dry climates (Pittelkow et al., 2015), so no-till farming may improve dry farming outcomes (Peterson et al., 2020). However, if dry-farmed crops rely mainly on deep soil moisture, changes in surface soil properties from no-till may not have a strong effect on dry farming productivity.
Improved soil health may also improve dry farming outcomes through specific plant-microbe interactions with arbuscular mycorrhizal (AM) fungi. AM fungi affect a range of plant processes that mediate growth under drought, including nutrient acquisition and water relations, and can help to improve structure and water-holding capacity in the soil (Augé, 2001; Bowles et al., 2016; Acevedo et al., 2022). Reduced tillage increases AM fungal root colonization of annual cash crops by about 30% on average (Bowles et al., 2017). However, whether potential changes in AM fungi under reduced tillage mitigate the significant water stress experienced by dry-farmed crops, thereby potentially altering fruit quality and taste, is not known.
Varietal selection is another key element of dry farming success which warrants further study. Dry farmers carefully select drought-resistant varieties that can produce high-quality fruits with improved water use efficiency. In California, however, commercial dry farming of tomatoes has predominantly relied on one variety of hybrid, indeterminate tomato, the Early Girl (Leap et al., 2017). This approach poses a significant risk for farmers, as relying solely on a single variety of tomato, especially a hybrid, can leave them vulnerable to crop failure or changes in the seed industry that affect seed availability. Adoption of heirloom varieties may mitigate risks due to their increased genetic diversity and ability to better adapt to changing environments (Dwivedi et al., 2019), as well as enable seed saving by farmers.
Due to the changes in soil properties that affect water availability under no-till, our first hypothesis was that no-till would decrease tomato water stress when dry-farmed. We hypothesized that this decrease in water stress would impact fruit yield and quality and may be quantifiable through photosynthetic measurements. Changes in the plant’s available water, in this case, might affect fruit development issues such as blossom end rot, a physiological disorder that causes sunken necrotic lesions in tomatoes resulting from calcium (Ca) deficiency (Saure, 2001). Water restriction is one of the main causes of limited Ca uptake by roots and can thus lead to Ca deficiency and blossom end rot (Balate et al., 2018). Yields are likely to increase as water stress is lowered. However, fruit quality—critical for the price premium paid by consumers for dry-farmed produce—might also decrease if flavor is reduced due to increasing fruit moisture content. We also hypothesized that AM fungi would improve tomato water stress tolerance, leading to increased fruit production when dry-farmed. Finally, we hypothesized that there would be significant differences in fruit and physiological responses among tomato varieties under dry-farming conditions.
To test these hypotheses, we chose three indeterminate tomato varieties and two paired genotypes of determinate tomatoes that serve as a research model system for AM fungi. Early Girl was chosen as the current commercial standard. Momotaro, a hybrid, indeterminate variety, which has not been explored in dry-farming settings but is grown commercially in California and shares similar traits with Early Girl, was recommended by a dry farmer. We also selected an heirloom, indeterminate variety, Zapotec, that has shown promising performance in our preliminary research on dry farming. Wildtype mycorrhizal tomato genotype 76R and its reduced mycorrhizal mutant rmc were selected as a research model system to isolate the contribution of AM fungi to dry-farmed tomato production without other interventions (Bowles et al., 2016). rmc is a well-characterized tomato mutant with reduced mycorrhizal colonization that has comparable nutrient uptake and growth when not inoculated with AM fungi to its nearly isogenic mycorrhizal wildtype progenitor, 76R (Cavagnaro and Martin, 2011; Cavagnaro et al., 2012; Watts-Williams and Cavagnaro, 2012). This study takes inspiration from two farming systems that are farmer innovations in the region—dry farming and no-till systems—and combines them to explore possible effects of soil health on the success of dry farming.
2 Materials and methods
2.1 Field site and experimental design
The experiment was conducted at the University of California, Berkeley Oxford Tract Research Station, a 0.5-ha urban field site in Berkeley, California, United States (37.876187, −22.267345) with an average annual rainfall of 64 cm, during the 2022 growing season. The dominant soil type is a Terra complex, a fine, montmorillonitic, thermic Mollic Palexeralfs. In Fall 2017, a long-term field experiment was initiated to assess the multiyear effects of tillage and other agroecological management techniques on soil (Rainey et al., in preparation). The experiment was arranged in a randomized block design with tillage as treatment. Given the small size of this urban research field station, plots were necessarily limited in size, at 30 × 1 m (i.e., one bed wide). Thus, we focus on changes apparent at this small scale, including soil biological and chemical properties, rather than changes that may only be seen with much larger experimental plots, such as differential access to subsurface water and aboveground arthropod communities.
Taken together, the experimental treatments in this study represent whole farming systems with distinct residue management, field schedules, spatial scales, and levels of mechanization. While our field experiment cannot fully capture the wide range of farming practices used by fruit and vegetable farmers in the region, it can capture the impacts of changes in soil chemical and biological properties apparent after 5 years (Table 1; Rainey et al., in preparation). Soil in the no-till (“NT”) system was not disturbed, aside from occasional broad-forking to work in compost (see below), whereas the conservation till (“T”) system was managed by mechanized mowing, discing, roto-tilling, and bed forming via tractor after each crop cycle. Fallow periods were inevitable in the tilled systems due to delays from wet soil, whereas new crops were transplanted or sowed immediately following crop harvest and removal of aboveground biomass in the NT system. Compost (a 50:50 mixture of vegetable-based and chicken manure-based compost) was applied at a rate of 7.5 tons/acre under NT and 3.75 tons/acre in the T system. Higher application in the NT system accounted for the incorporation of cash crop residues in the tilled system (vs. removal in the NT system). A diverse polyculture of vegetables, beans, maize, and cover crop mixes was grown in all plots from Fall 2017 through Spring 2022 when this dry farming experiment began.
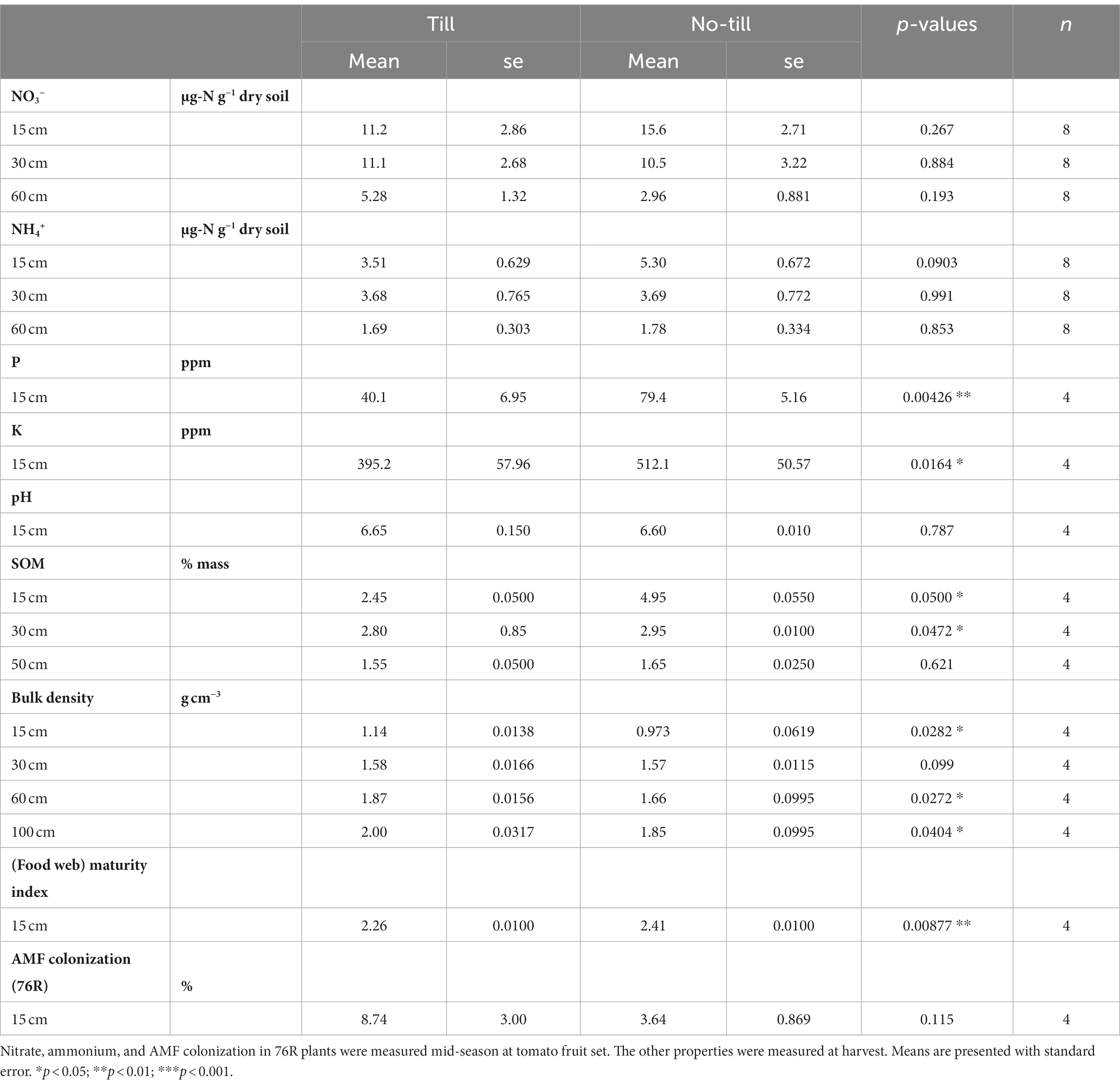
Table 1. Soil chemical and biological properties [nitrate (NO3−), ammonium (NH4+), phosphorus (P), potassium (K), pH, soil organic matter (SOM), bulk density, (food web) maturity index, and AMF colonization] of till and no-till plots measured during the 2022 dry-farmed tomato growing season.
Preparation of the field in Spring 2022 (27.4 m × 15.2 m) included cutting previous crops at the soil surface, bed formation, and incorporation of 50 kg/ha nitrogen (N) as Neem Gold (4–1-2), 100 kg/ha potassium (K) as Langbeinite (0–0-22), and 430 kg/ha lime dolomite as Lhoist #65. From 10 May to 7 October 2022 (transplanting and harvest, respectively), mean temperatures were 20.4°C (maximum) and 12°C (minimum), with a maximum of 40°C and a minimum of 5.4°C. The only precipitation events >1.5 mm were on 5 June (7.6 mm) and 18 September (4.9 mm) (Supplementary Figure S1; CIMIS, 2022).
For this experiment, we used the main tillage plots (four replicate blocks) and added subplots for the genotype, with two or three levels (Early Girl, Momotaro, and Zapotec; OR 76R and rmc, see below), replicated four times within each main plot (Supplementary Figure S2). Thus, there were four experimental units for each tillage and genotype combination.
Surface sterilized seeds of Solanum lycopersicum cv. Early Girl (EG), Momotaro (M), and Zapotec (Z) (Johnny’s Selected Seeds, Kitazawa Seeds, and TomatoFest, respectively), and 76R and rmc were germinated and grown in a glasshouse, followed by a lathhouse for seven weeks total. Seedlings were transplanted by hand on 10 May 2022 at a spacing of 0.45 m between plants (a total of 10 plants per plot, except for Zapotec plots, which had eight plants per plot due to limitations in germination and seed availability). In line with common dry farming practices, transplants were buried just below their first true leaves. Subplots for the genotype were 4.6 m long, with one buffer plant at each end. The buffer plants were intentionally not sampled to mitigate potential inaccuracies arising from edge effects. As a result, there were two plants per plot that were excluded from the sampling process. At 6 DAP, soils were sampled to assess water content, and irrigation was added to achieve 21.2 cm of water in the top 60 cm in all plots. Thus, we eliminated any effects of the management system on soil moisture content at the beginning of the tomato dry farming season. Irrigating in the transplants (i.e., just once at the start of the season, depending on winter rains) is a typical practice of dry-farmers in the region. After this, drip lines were removed, and irrigation did not occur again during the growing period.
2.2 Soil sampling for chemical and biological properties
The soil was sampled for moisture and inorganic nitrogen at three depths (0–15, 15–30, and 30–60 cm) at transplant (6 DAP), anthesis (53 DAP), and fruit set (80 DAP). Two cores per plot were composited at transplant, with one core per plot for anthesis and fruit set. Gravimetric water content (GWC) was measured on all samples by drying a subsample at 105°C for 48 h. Inorganic N was extracted from moist soils with 1 M KCl (soil:solution ratio of 2.5) and analyzed colorimetrically for NH4+ and NO3− (Foster, 1995; Miranda et al., 2001; Bowles et al., 2022).
For determination of AM root colonization, roots to a depth of approximately 15 cm were collected at 80 DAP. After washing with water and ethanol and clearing in KOH, roots were stained with black ink, and colonization was determined using the magnified intersections method (McGonigle et al., 1990).
Soil samples were collected at harvest down to a depth of 100 cm. Soil phosphorus (P) and K were evaluated using the Morgan extraction procedure. Total organic matter was evaluated by loss on ignition. Bulk density was evaluated using an equivalent soil mass technique for evaluating multiple soil depths (Wendt and Hauser, 2013). Soil food web indices were calculated by first extracting nematodes from 200 mL of field moist soil using a sieving and decanting technique followed by sugar centrifugation (Barker, 1985). The first 200 nematodes on the slide were identified to the genus level, and the total number of nematodes in each sample was counted. Indices of ecosystem functioning were calculated using the abundance of nematode groups of particular trophic levels, feeding types, or metabolic functioning. In particular, the maturity index is a quantitative representation of the spread of colonizer (rapid growth and high nutrient turnover) versus persister (slow growth with extended nutrient metabolism) species within the soil food web (Bongers, 1990; Ferris and Bongers, 2009). Each nematode genera is assigned a score based on its lifecycle, and an overall score is assigned to the sample.
2.3 Leaf gas exchange, water, and nitrogen status
Leaf gas exchange measurements (CO2 assimilation, An, and stomatal conductance, gs) were taken on two mature, fully expanded leaflets per plot with a field portable open flow infrared gas analyzer (model 6400, LI-COR Inc., United States) at anthesis (48 DAP) and fruit set (77 DAP). Measurements were taken between 9:30 and 14:30 h with a 6 cm2 leaf chamber, with the CO2 reference set at 420 μmol mol−1 and with a light intensity of 2,000 μmol m−2 s−1 using a light-emitting diode source. The mean of the two leaves was calculated from two different plants within each plot. Each measured leaflet was sampled for analysis of relative water content (RWC) and specific leaf area (SLA). Leaf relative water content (RWC) was measured at midday according to:
Where FW is leaf fresh weight, DW is leaf dry weight after 72 h at 60°C, and TW is leaf turgid weight after submergence in water overnight at 4°C (Bowles et al., 2016). The mean of the two leaves was taken for each plot for a total of four replicates per treatment (n = 4). The leaf area of one of the hydrated leaves per plot was measured using ImageJ. SLA was then calculated as the hydrated leaf area divided by the dry mass (m2/kg) (Laliberté, 2018) (n = 4).
Leaflets collected at fruit set (77 DAP) (n = 4) and during senescence (111 DAP) (n = 4) were oven dried for 48 h at 60°C, then ground using a cell disrupter (Mini-beadbetter-16, Biospec Products, United States). For the stable isotope analysis, methods were modified from DeVries et al. (2016). Ground leaf material was placed in a 3 x 5 mm tin capsule and weighed to a precise mass. Carbon (δ13C) and nitrogen (δ15N) stable isotope ratios and the elemental concentrations of C and N were analyzed using a continuous-flow isotope ratio mass spectrometry at the University of California, Berkeley Center for Stable Isotope Biogeochemistry, using a CHNOS Elemental Analyzer (Vario ISOTOPE cube analyzer, Elementar, Germany) coupled to an IsoPrime100 Isotope Ratio Mass Spectrometer (Isoprime, UK). Isotope ratios are expressed in parts per thousand (‰) using delta-notation as:
Where h is the high mass number, X is the element, R is the high mass-to-low mass isotope ratio, and Rstandard is Vienna Pee Dee belemnite (VPDB) for carbon and air for nitrogen. A peach leaf standard [Standard Reference Material (SRM) No. 1547, n = 60, SD of δ13C = 0.1 ‰ and δ15N = 0.2‰] as well as a bovine liver standard (SRM No. 1577, n = 7, SD of δ13C and δ15N was = 0.1‰) were used as references and standards and to correct for instrument drift and linearity.
Stem water potential (ΨStem) was measured at mid-morning on one day during the anthesis (50 DAP) and fruit set (83 DAP) samplings. Two mature leaflets per plot were covered for at least 20 min in reflective bags to prevent leaf transpiration, excised, and measured with a Scholander pressure chamber (Model 615, PMS Instruments, United States). The mean of the two leaflets was taken for each plot for a total of four replicates per treatment (n = 4).
Leaf chlorophyll content was measured on one day during the anthesis (48 DAP) and fruit set (76 DAP) samplings. Three mature leaves per plot were measured using a chlorophyll meter (SPAD-502, Minolta, United States). The mean of the three leaves was taken for each plot for a total of four replicates per treatment (n = 4).
2.4 Fruit sampling
For the indeterminate varieties, fruits were harvested as they ripened, approximately once a week, from 80 DAP (29 July 2022) to 136 DAP (23 September 2022). All fruits were weighed, counted, and split into four categories (marketable, blossom end rot, sunburnt, and other non-marketable). At 106 and 114 DAP, when all plots were producing fruit, sub-samples were taken. Three to five fruits, depending on fruit size and availability, were cut, weighed, and dried at 65°C for at least five days for moisture content. Three to five fruits were juiced by hand for immediate Brix measurements using a digital refractometer (MA871, Milwaukee Instruments, United States) and then frozen for subsequent pH measurements (Accumet AE150, Fisher Scientific, United States). Tomato aboveground biomass was harvested at 151 DAP.
For the determinate varieties, fruit subsamples were taken at harvest at 107 DAP. Five fruits were cut, weighed, and dried at 65°C for five days for moisture content. Five fruits were juiced by hand for immediate Brix measurements and then frozen for subsequent pH measurements. After fruit harvest, tomato plants were harvested.
For both indeterminate and determinate varieties, dried fruits were ground by hand in a small mortar and pestle, followed by grinding in a ball mill (MM 400, Retsch, Germany) for 2 min with two small ball bearings. The fruits were analyzed for C and N contents using an elemental analyzer (Vario EL cube, Elementar, United States). For EG fruits, concentrations of phosphorus, potassium, sulfur, boron, calcium, magnesium, zinc, manganese, iron, and copper were determined by nitric acid/hydrogen peroxide microwave digestion followed by Inductively Coupled Plasma Atomic Emission Spectrometry (ICP-AES) (iCAP 6,500, Thermo Scientific, United States) (n = 4). Only EG fruits were sampled due to logistical reasons, as they are the current commercial standard.
2.5 Statistical analysis
We tested the effect of tillage (till vs. no-till), tomato genotype (Early Girl, Momotaro vs. Zapotec; or 76R vs. rmc), and their interaction on dry-farmed tomato plant physiology, water status, and fruit traits, and for 76R and rmc, AMF colonization, using generalized linear mixed models with the nlme and lmerTest packages in R (Kuznetsova et al., 2017; Pinheiro et al., 2021; R Core Team, 2021). Mixed effect analysis of variance (ANOVA) was performed using these packages with 95% confidence intervals calculated, followed by estimated marginal means (EMMs) using pairwise comparisons in the emmeans package. Genotype and tillage treatment were treated as fixed effects, while block and block × tillage treatment were considered random effects to account for the split-plot experimental design. Transformations were used as needed to meet assumptions of homoscedasticity and normality. Graphs were plotted in R. The indeterminate and determinate tomato data were analyzed and are presented separately.
3 Results
3.1 Soil properties
Soil biological and chemical properties, measured during the dry-farmed tomato growing season, differed between the till and no-till treatments. Surface soil P and K were significantly higher in no-till compared to till (p < 0.01 and p < 0.05, respectively, Table 1), and soil organic matter (SOM) was also higher in no-till at 0–15 cm and 15–30 cm depths (Table 1). Bulk density was lower in no-till soils at the surface (0–15 cm) and below 60 cm. The food web maturity index was significantly greater in no-till plots, demonstrating a nematode community that has shifted toward a more persistent, slower-growing, and less disturbed food web assemblage. Tillage did not significantly affect soil pH or AMF colonization.
Soil gravimetric water content decreased from transplant to anthesis and from anthesis to fruit set at all three depths (p < 0.01). The mean for till plots from 0 to 15 cm was originally 0.29 g g−1 at transplant, dropping to 0.14 and 0.12 g g−1 at anthesis and fruit set, respectively (Figure 1). No-till plots showed similar trends but started slightly drier at 0.26 g g−1, before water was added to the soil to bring all beds to 21.2 cm moisture in the profile. Deeper depths, 15–30 cm and 30–60 cm, also showed similar trends, with the greatest gravimetric water content at transplant and statistically similar gravimetric water contents at anthesis and fruit set (Figure 1A).
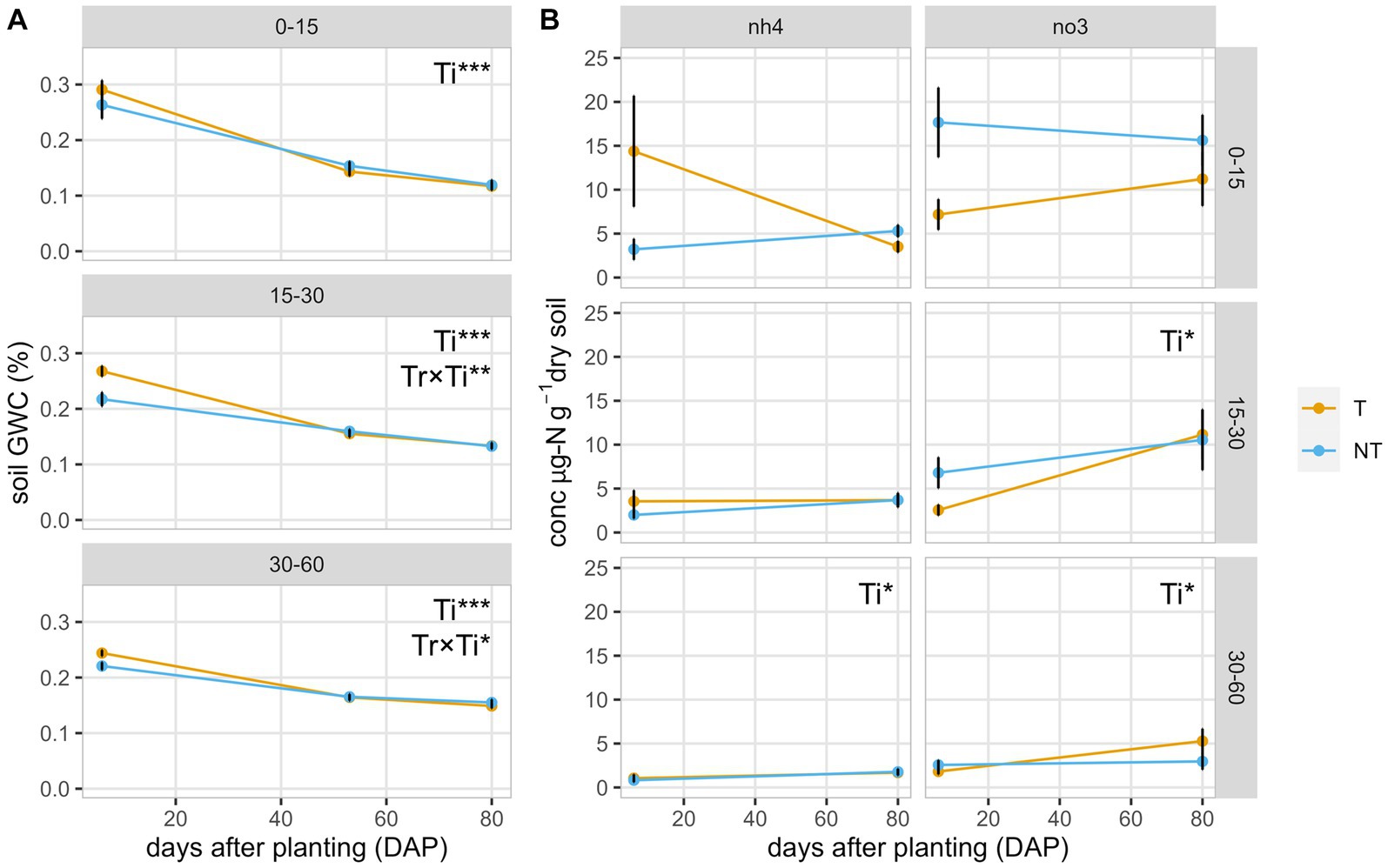
Figure 1. Soil parameters in the field during the dry-farmed tomato experiment with two tillage regimes, no-till (NT) and till (T). (A) Soil gravimetric water content (GWC) and (B) soil ammonium (NH4+) and nitrate (NO3−) concentrations at three timepoints during the growing season: transplant (6 DAP), anthesis (53 DAP), and fruit set (80 DAP), measured at three depths. Significant treatment effects and observed means ± se (n = 4) are shown. Data were transformed for 30–60 cm NH4+ using the natural log function and 30–60 cm NO3− using the square root function to meet model assumptions, so back-transformed means ± se (n = 4) are shown instead. Tr, tillage treatment; Ti, timepoint. *p < 0.05; **p < 0.01; ***p < 0.001.
When comparing soil inorganic nitrogen concentrations across sampling timepoints, soil nitrate concentrations were greater in the no-till treatment at transplant (6 DAP) at 0–15 cm (Figure 1B). Ammonium showed the opposite trend in the top 15 cm of soil at transplant, with higher ammonium in the till treatment (Figure 1B). At fruit set (80 DAP), there were no significant differences between ammonium or nitrate for each depth and tillage treatment. Nitrate concentration was greater at all depths at fruit set compared to at transplant, except for 0–15 cm no-till, where it was slightly lower. Soil ammonium concentration remained constant from transplant to fruit set at 15–30 cm. While it increased slightly for no-till at 0–15 cm depth, the mean ammonium concentration declined by 76% from transplant to fruit set at the same depth in the till treatment (Figure 1B).
3.2 Indeterminates
Significantly different results will be noted explicitly in the text with p-values, and otherwise comparisons are numerical.
3.2.1 Fruit yield and quality
The indeterminate fruits were harvested as needed throughout the growing season, with fruit production peaking between 16 and 34 days after the first harvest (Figure 2). Marketable fruit yield combined over the 10 harvests was greatest for EG no-till (mean = 1.85 kg/plant) and lowest for Z till (mean = 1.09 kg/plant, Figure 3). Tillage did not significantly impact marketable fruit (95% CI: −0.9 to 0.3 kg/plant). Marketable fruit number was significantly greater for EG than M or Z (p < 0.001). While Z produced the lowest fruit number over the season, Z fruits were greatest in weight (till mean = 111 g, no-till mean = 101 g, p < 0.001, Figure 3). Percentage non-marketable was under 6% for EG and M, and was greater for Z with 41% and 25% for till and no-till, respectively (p < 0.001, Figure 3). Fruit differences between genotypes were more pronounced than differences between till and no-till treatments.
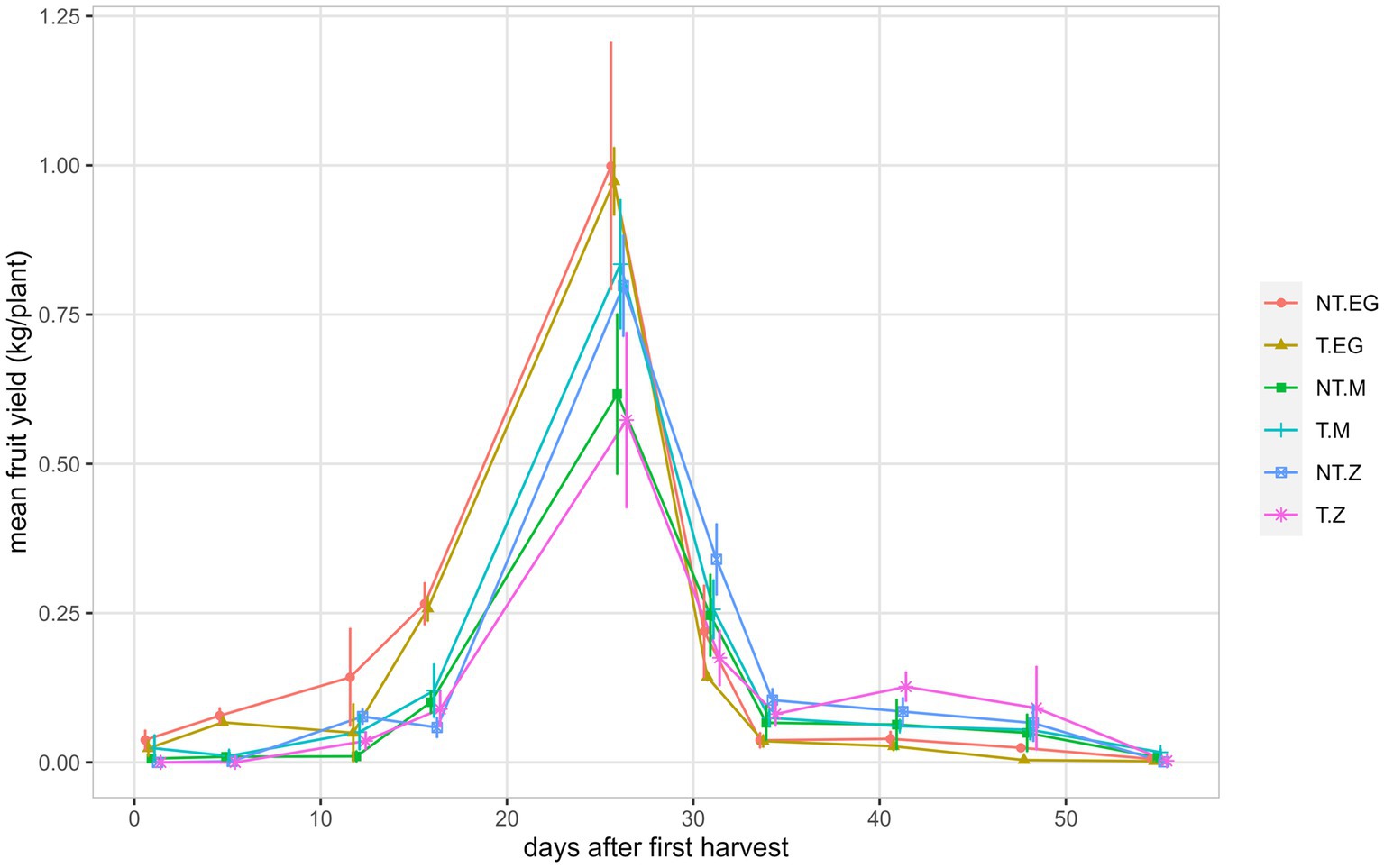
Figure 2. Mean fruit yield per plant for each harvest of the indeterminate tomato genotypes Early Girl (EG), Momotaro (M), and Zapotec (Z), grown with either no-till (NT) or till (T) soil regimes under field conditions. The first harvest occurred at 80 DAP on 29 July 2022. Shown are observed means ± se (n = 4). Means, error bars, and lines have been slightly dodged horizontally to avoid overlapping and for better visualization.
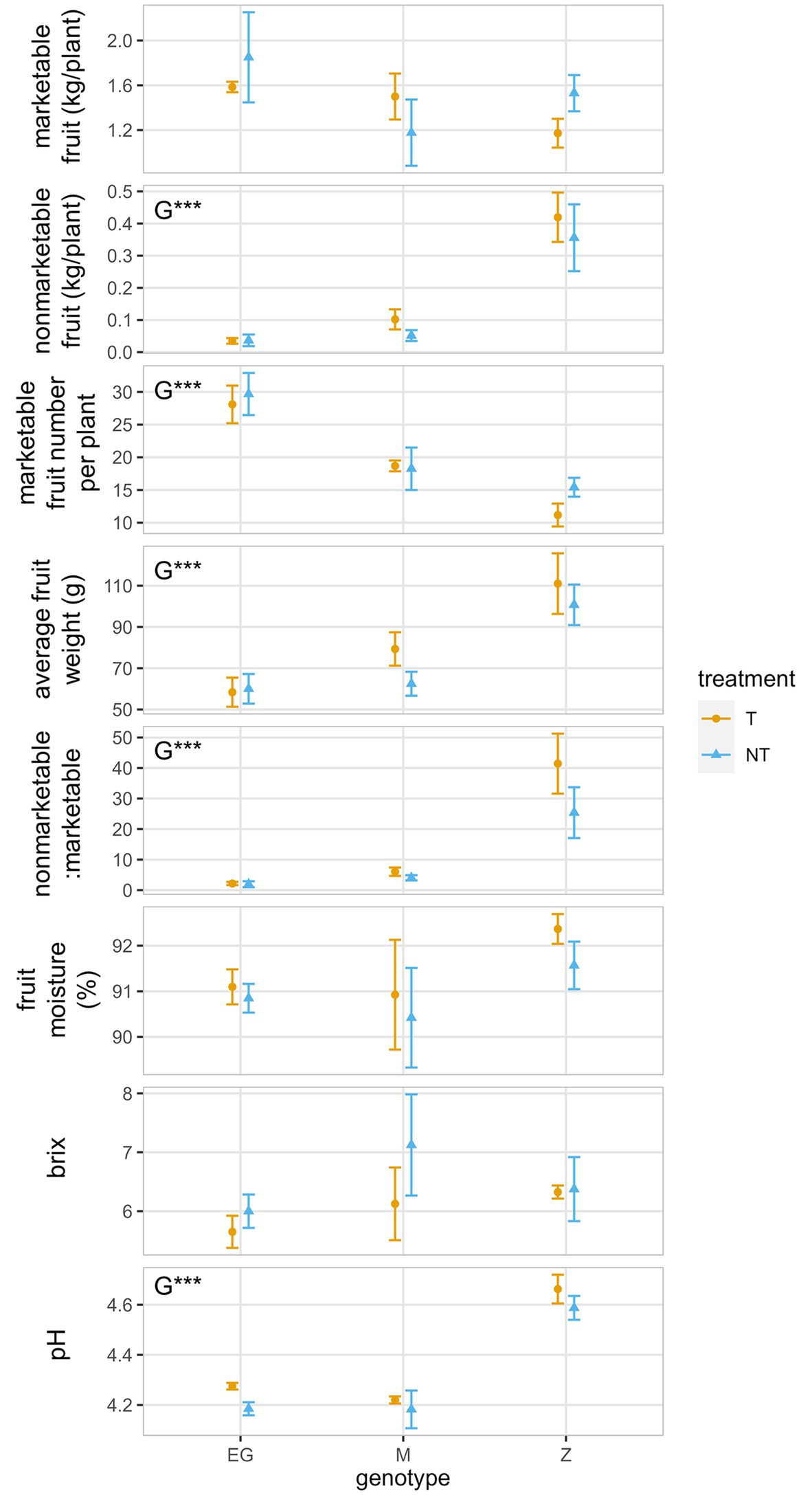
Figure 3. Fruit yield and quality attributes for indeterminate tomato genotypes Early Girl (EG), Momotaro (M), and Zapotec (Z), which were grown with two tillage regimes (NT, no-till; T, till) under field conditions. Marketable fruit is suitable for sale, whereas non-marketable fruit is damaged, decayed, or rotten. Significant treatment effects and observed means ± se (n = 4) are shown. Data were transformed for non-marketable fruit and percentage non-marketable using the square root function to meet model assumptions, so back-transformed means ± se (n = 4) are shown instead. Means and error bars have been slightly dodged horizontally to avoid overlapping and for better visualization. G, genotype. *p < 0.05; **p < 0.01; ***p < 0.001.
While fruit Brix and moisture content did not differ significantly between genotypes, Z pH was significantly greater than both EG and M, with pH increased by 10% (till mean = 4.7, no-till mean = 4.6, p < 0.001, Figure 3). C/N ratio, C%, and N% did not differ significantly between genotypes (Supplementary Table S2).
EG fruit nutrient concentrations did not differ significantly between till and no-till (Supplementary Table S3). For the treatments combined, the average phosphorus was 0.57%, potassium was 4.53%, calcium was 0.15%, and magnesium was 0.24%. M and Z were not sampled for nutrient concentrations.
3.2.2 Plant water status and leaf physiological responses
At anthesis and fruit set timepoints, stem water potential (Ψstem) at mid-morning was similar in all three genotypes (Table 2). Ψstem was significantly lower at fruit set than anthesis (p < 0.01). Leaf RWC was similar in all groups at anthesis and fruit set. Combining the timepoints and treatments, EG, M, and Z had mean leaf RWCs of 64%, 67%, and 68%, respectively.
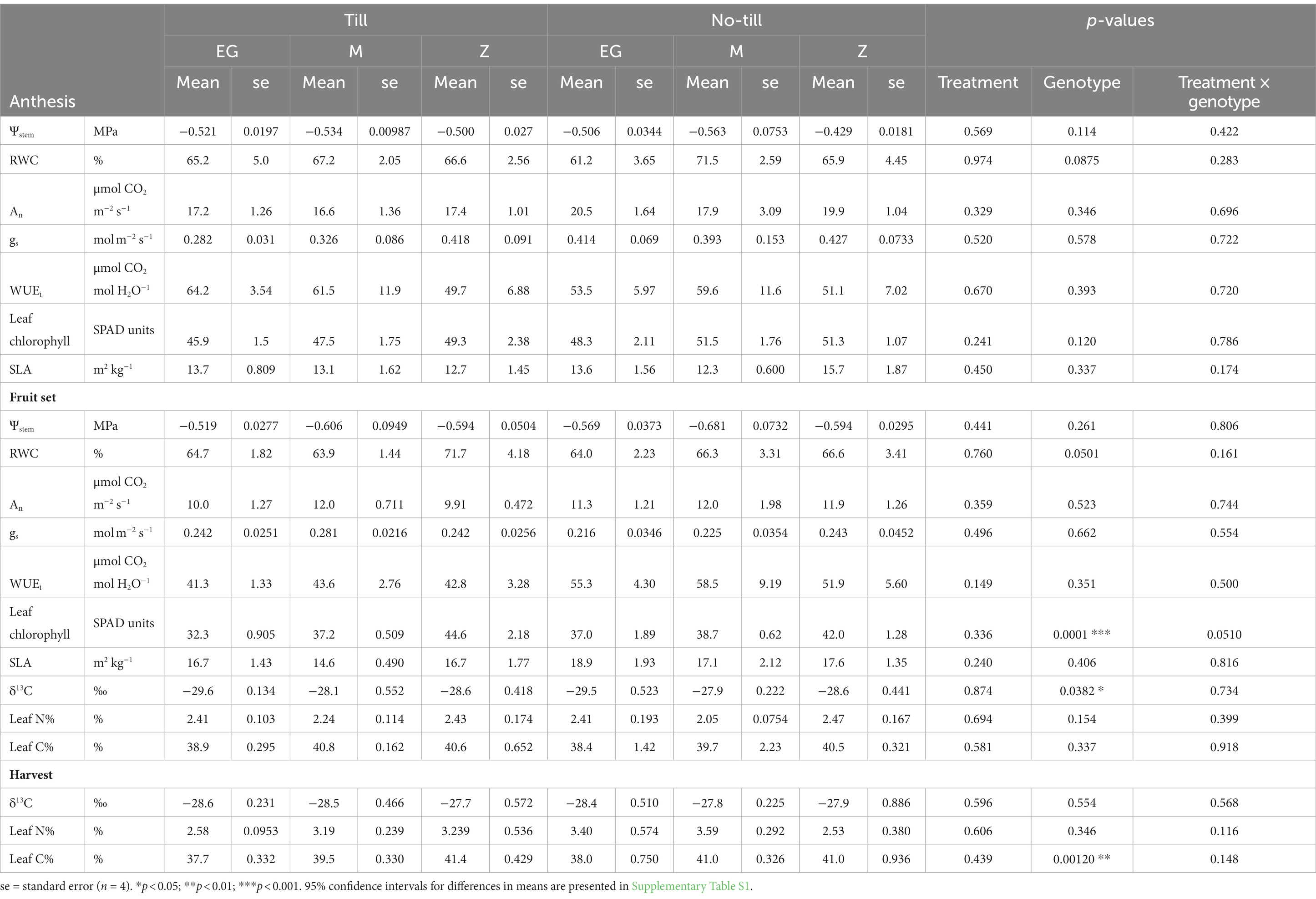
Table 2. Leaflet physiological characteristics [stem water potential (Ψstem), relative water content (RWC), CO2 assimilation (An), stomatal conductance (gs), intrinsic water use efficiency (WUEi), leaf chlorophyll, specific leaf area (SLA), δ13C, leaflet N, and leaflet C] at the anthesis, fruit set, and harvest samplings of Early Girl (EG), Momotaro (M), and Zapotec (Z) tomato genotypes grown with two tillage regimes (no-till and till) under field conditions.
At anthesis, An and gs were lower in till treatments for all three genotypes (An 95% CI: −11 to 4.5 μmol CO2 m−2 s−1; gs 95% CI: −0.5 to 0.3 mol m−2 s−1). An and gs decreased from anthesis to fruit set in all groups (p < 0.001). For EG at anthesis, An and gs were 19% and 47% greater for no-till than till. At fruit set, differences in gs between till and no-till were less pronounced. WUEi, the amount of CO2 fixed per unit of H2O lost, remained consistent over the timepoints and genotypes, except for the till treatment at fruit set, which was numerically lower in all three varieties (EG mean = 41.3 μmol CO2 mol H2O−1, M mean = 43.6 μmol CO2 mol H2O−1, Z mean = 42.8 μmol CO2 mol H2O−1). WUEi was 36%, 29%, and 14% lower at fruit set compared to anthesis for EG, M, and Z, respectively (p < 0.05). At fruit set, there was a significant effect of genotype on leaf δ13C (p < 0.05) (Table 2). Taking the mean of the tillage treatments for each genotype, δ13C was −29.6‰, −28.0‰, and −28.6‰ for EG, M, and Z, respectively.
Leaf chlorophyll content was greater at anthesis than at fruit set (p < 0.001). At each timepoint for EG and M, till resulted in lower leaf chlorophyll content than no-till, while the opposite was true for Z at fruit set (Table 2). Specific leaf area (SLA) showed the opposite trend and was greater at fruit set than at anthesis for all three varieties (p < 0.001).
3.3 Determinates
76R and rmc were used as a model system to compare how AM fungi influence tomato fruit and plant production when dry farmed. Significantly different results will be noted explicitly in the text, and otherwise comparisons are numerical.
3.3.1 AM fungi colonization
Tillage influenced the extent of root colonization by AM fungi, with the mycorrhizal wildtype progenitor 76R having significantly greater AM root colonization (mean = 8.7%) than the mycorrhiza defective mutant rmc (mean = 0%) when tilled (p < 0.05) (Figure 4). For the no-till treatment, both 76R and rmc had statistically similar AM fungi colonization (76R mean = 3.6%, rmc mean = 2.5%).
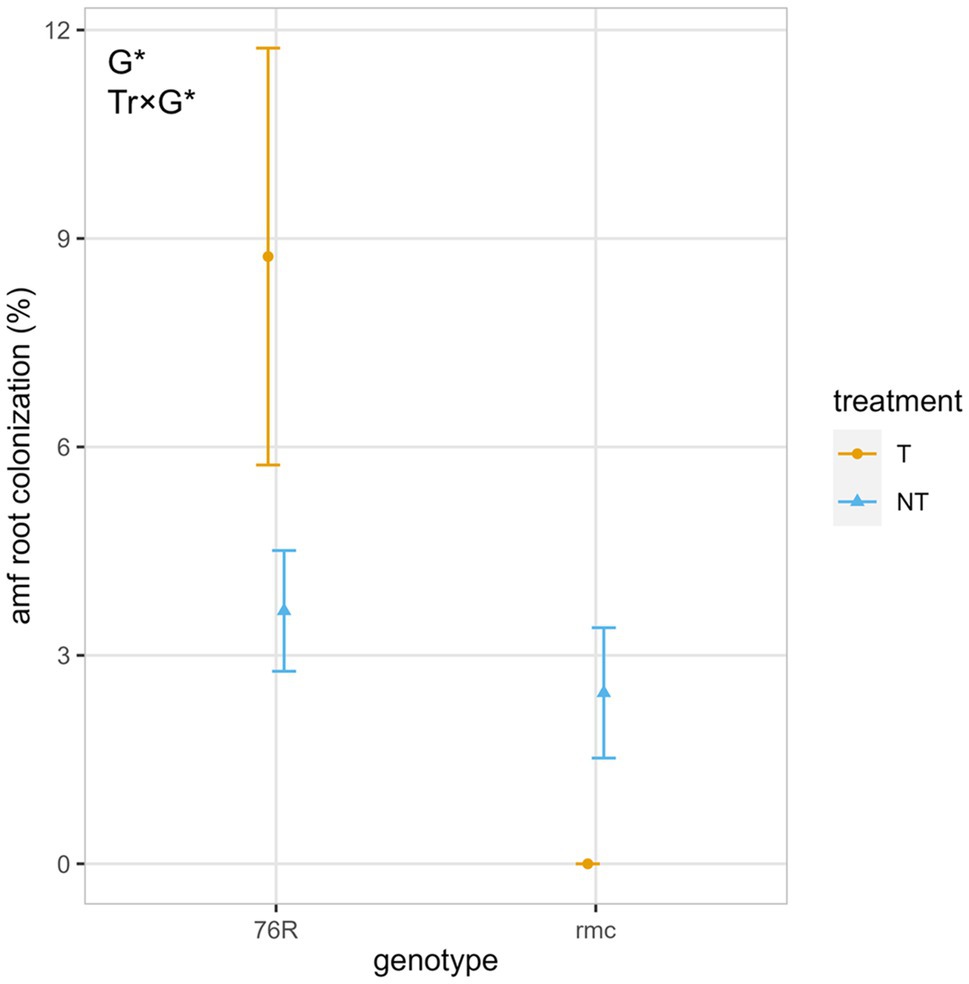
Figure 4. AM fungi root colonization for mycorrhizal (76R) and reduced mycorrhizal (rmc) tomato genotypes grown with two tillage regimes (NT, no-till; T, till) under field conditions. Shown are observed means ± se (n = 4). Means and error bars have been slightly dodged horizontally to avoid overlapping and for better visualization. Tr, tillage treatment; G, genotype. *p < 0.05; **p < 0.01; ***p < 0.001.
3.3.2 Fruit yield and quality
At harvest, marketable fruit biomass was lower in rmc compared to 76R for both till and no-till treatments (95% CI: −0.5 to 0.3 kg/plant). In the till treatment, rmc produced 38% lower marketable fruit biomass than 76R (Supplementary Table S4). Total fruit production was greatest in till 76R (mean = 1.39 kg/plant) and lowest in no-till rmc (mean = 0.89 kg/plant). Green fruit yield was slightly higher in 76R than rmc (95% CI: −0.1 to 0.1 kg/plant). Percentage non-marketable was greatest for till rmc (mean = 89%), while the other groups were under 53% (95% CI for tillage is −35.6 to 59.4%, 95% CI for genotype is −38.2 to 34.8%, Supplementary Table S4). In this case, non-marketable includes all categories other than marketable, including green, decaying, and otherwise non-marketable fruit.
pH was significantly greater in rmc than 76R under both till and no-till (p < 0.01, Supplementary Table S4). Fruit moisture content did not differ significantly between tillage and tomato genotypes, with a mean of 89% (Supplementary Table S3). Both genotypes under till had the same mean Brix content of 5.60 (95% CI: −1.4 to 0.03). Brix was greatest for 76R no-till (mean = 6.15). C/N ratio, fruit N%, and C% did not differ significantly between treatments (Supplementary Table S4).
3.3.3 Plant water status and leaf physiological responses
There were minimal significant differences in leaflet physiological characteristics between varieties and treatments, but there were trends across the timepoints (Supplementary Table S5). Ψstem at anthesis was greater than at fruit set (p < 0.001), indicating higher water stress later in the growing season. Correspondingly, An and gs decreased from anthesis to fruit set in all groups, as did leaf chlorophyll content (p < 0.001). SLA showed the opposite trends and was greater at fruit set than anthesis (p < 0.001). Since gs was lower in the no-till treatments at anthesis (76R mean = 0.314 mol m−2 s−1, rmc mean = 0.334 mol m−2 s−1, 95% CI: −0.2 to 0.4), the opposite trend was true for WUEi, and thus WUEi was higher in the no-till treatments. At harvest, there was an interaction effect of genotype × tillage for leaf δ13C (p < 0.05).
4 Discussion
All the plots in this experiment have a five year history of organic, agroecological management designed to increase soil health by incorporating compost applications, diverse crop rotations, cover cropping, and rising on-farm biodiversity. Plots were additionally split into either till or no-till. After five years of management, no-till significantly increased soil organic matter in the 0–30 cm range, reduced bulk density, increased soil P and K at the surface, and increased food web maturity index when compared to tilled plots (Table 1). Agroecological management improved these soil surface properties, and thus, we were interested in how tomatoes respond to extreme water deficit in this system.
4.1 Changes in surface soil properties from no-till may not influence dry-farmed indeterminate tomato productivity
We did not detect differences in dry-farmed indeterminate tomato water stress between till and no-till treatments. Photosynthesis, stomatal conductance, and stem water potential, all of which can be used as proxies for drought stress, were statistically similar between tillage regimes at each timepoint. WUE, in the form of intrinsic (WUEi) and long-term WUE (δ13C), often increases under drought as plants limit transpiration by closing stomata and use available water more efficiently (Gill et al., 2022). In this study, we did not find evidence that no-till can alleviate water stress brought about by dry farming. Future exploration is warranted with larger plots, greater replication, and additional precise measurements of soil and plant water status.
The effect of tillage on marketable fruit yield was variable and not statistically significant for all three varieties, supporting previous studies that have found that no-till may increase or decrease yields in certain scenarios (Pittelkow et al., 2015). Here, no-till was not detrimental to fruit yields, and Brix, a refractive index measure that is commonly used as an indicator of sugar content in fruits and used by producers to evaluate fruit quality (Baxter et al., 2005), was not increased under no-till. Taken together, indeterminate tomato plants grown in the no-till system did not cope better when exposed to significant water stress imposed by dry farming.
As comparisons in plant physiology, fruit yields, and fruit quality between no-till and tilled plots were not often statistically different, plants may be sending roots very deep in the soil profile, and thus, the tillage regime, which is typically thought to impact only the top 30 cm of the profile, may not strongly influence tomato performance. While the moisture in the top 15 cm of soil was more than halved from transplant to fruit set, the moisture in the deeper layer from 30 to 60 cm remained constant, indicating that plants may be reaching below this layer for water access. Factors such as soil properties, moisture, and nutrient availability strongly influence rooting depth (Sainju et al., 2000), with plants often increasing root production and root:shoot ratio to optimize water acquisition when water is limited (Galviz et al., 2021). Deficit irrigation has been reported to enhance root growth and increase rooting depth up to 75 cm in tomatoes (Nangare et al., 2016), suggesting that extensive root systems under dry farming are likely. However, the small plot sizes may limit inference, as the roots may have spread out laterally between plots and rows. Furthermore, additional field-scale research is needed to determine if we did not detect differences because there were no differences to detect or because we were unable to detect them with our experimental design.
Prior research on the same experimental plots showed that no-till increased soil moisture content during drought conditions, reduced stem water potential in black beans (Phaseolus vulgaris), and increased bean yield (Rainey et al., 2020). No-till promotes improvements in soil structure as well as healthy soil and root systems (Pittelkow et al., 2015; Acevedo et al., 2022), which we hypothesized would create conditions in which plants were better able to withstand drought conditions and potentially increase yield. Dynamic soil properties are influenced by management strategies, such as tillage, on seasonal to decadal timescales. While these properties affect water flow and soil water storage (Acevedo et al., 2022), and thus plant water availability, our small plot sizes and deep tomato roots likely limited our ability to test for these changes.
Future studies are warranted to establish dry-farmed tomato root length and root morphology patterns, as well as water uptake dynamics. Deuterium-based labeling techniques have previously been used to investigate root depth, spread, and water uptake dynamics in field studies (Beyer et al., 2016) and could prove valuable to investigate soil water dynamics in dry-farming scenarios.
With all of this in mind, dry farming soil management may be more flexible than originally established. While we acknowledge the limitations of this study due to plot size, our study may indicate the potential for farmers to make decisions based on variables other than tillage. In other words, while we do not identify a no-till benefit in this study, farmers could implement no-till for other motivations and not likely experience negative impacts on dry farm tomato production. However, other challenges related to no-till, such as increased weed pressure, fertilization placement and nutrient stratification, pest management, and soil structural issues, are important considerations (Dang et al., 2020). Further rigorous research is needed to compare management strategies and their impacts on tomato dry farming in California.
4.2 Indeterminate tomato physiological responses to water stress in an agroecologically-managed system
Soil moisture decreased from anthesis to fruit set, and the tomato plants correspondingly showed increased water stress. The stem water potential at fruit set was comparable to or more negative than previous tomato water-deficit field studies in California (Rudich et al., 1981; Bowles et al., 2016). Photosynthesis and stomatal conductance also decreased from anthesis to fruit set, likely because tomato plants closed their stomata to limit water loss through transpiration (Seleiman et al., 2021). Plants maintain water potential under stress by reducing stomatal transpiration losses and increasing water uptake from established root systems. In this study, photosynthesis and stomatal conductance were reduced compared to a previous field study (Nemeskéri et al., 2019), suggesting severe, prolonged water stress. The agroecologically-managed system, therefore, may not negate the significant water stress experienced by the tomato plants in this scenario, even if it improved other soil health parameters such as soil organic matter, bulk density, and food web maturity index.
Furthermore, WUEi decreased at fruit set, indicating that the water stress was significant enough to lose efficiency in photosynthesis, but this may also be a result of the aging plants (Nangare et al., 2016). As water is so limited in dry farming systems, increasing WUE may increase yields, but this could come at the expense of quality and flavor, on which dry farming marketing success relies. Leaf chlorophyll content also decreased, likely due to oxidative damage and potential leaf nutrient deficiencies, as water-stressed plants attempt to maintain cellular water potential at the expense of photosynthetic efficiency (Sun et al., 2021).
4.3 Early Girl was the superior indeterminate genotype for fruit yield and quality
EG is currently the leading commercial standard for dry-farmed tomatoes in the Western United States, chosen for its deep roots and consistent production of high-quality, flavorful, and marketable fruits (Leap et al., 2017). Our results were consistent with these previous findings, with EG producing the greatest marketable fruit yield and number, as well as the lowest non-marketable yield. Rotted or decaying fruits were rare for EG, supporting consistency in marketable fruit yields. While Brix was not greater in EG tomatoes, EG was, anecdotally, far tastier and more flavorful than both M and Z. This is likely a result of the reduced moisture content and small size of EG fruits, ensuring that the sugars, organic acids, and amino acids were concentrated for increased taste. The variability, indicated by standard error bars, was greater for M than EG for Brix and moisture. In this way, M was a less predictable variety, lowering its appeal as a dry farming varietal choice for farmers. Z fruits were larger, less flavorful, and often non-marketable due to rotting or decay. While this heirloom variety was not competitive in this scenario, other heirloom varieties should be investigated in future field trials.
The differences in EG fruit yields compared to M and Z may not be due to differences in physiology. Instead, EG, M, and Z showed similar gas exchange, water status, and nutrient responses when dry-farmed. Thus, while EG is evidently a superior choice commercially, these differences may be due to inherent phenological variations, variations in morphology, or flower-to-fruit conversion differences. As relying solely on a single variety of tomato, especially a hybrid, can leave farmers vulnerable to crop failure or limitations in seed availability, breeding efforts should focus on dry farming specifically to ensure that farmers have a suite of reliable options. One such de-hybridized dry-farmed tomato variety, called Dirty Girl, has been bred from EG stock over 13 growing seasons in Santa Cruz County, California (Dry Farming Institute, 2023). In some scenarios, Dirty Girl performs better than other commercially available varieties; thus, breeding shows potential to improve dry farming cultivars and reduce risks for farmers. Variety trials focused on identifying traits, such as fruit flavor and moisture content, fruit marketability, plant root depth, and resistance to water stress, for breeding new dry-farmed tomato varieties are therefore warranted.
4.4 AM fungi did not increase determinate tomato fruit yield or quality under dry farming
Root colonization of rmc by AM fungi was lower than its wildtype progenitor 76R but only in the tilled treatments, providing an effective non-AM control under these specific field conditions. However, AM fungi root colonization in 76R was low overall in both treatments, compared to previous studies. Watts-Williams and Cavagnaro (2012) found AM fungi colonization of 76R was between 6% and 50%, depending on soil phosphorus addition, while Cavagnaro, Barrios-Masias, and Jackson (Cavagnaro et al., 2012) reported mean AM fungi colonization of 76R of approximately 20% in an organic field in California, suggesting that our colonization results for 76R are lower than expected. Generally, reduced tillage increases AM fungi root colonization by an average of 30% (Bowles et al., 2017), but in this field study, no-till did not increase AM fungi root colonization. Phosphorus in the soil was at mean levels of 40.1 ppm in the T system and 79.4 ppm under NT, due to the applications of fertilizer and compost. These levels are above recommended levels of soil phosphorus for agricultural applications, and high soil phosphorus has previously been reported to repress AM colonization (Watts-Williams and Cavagnaro, 2012), which might explain the generally low AM fungi root colonization. Thus, future experiments on dry-farmed fruits or vegetables should explore AM fungi root colonization in soils with low phosphorus, as root colonization is likely to increase.
76R and rmc were chosen as a model system to compare how AM fungi influence dry-farmed tomato production and quality. As AM fungi root colonization was comparable between the genotypes under no-till, we would expect to see more pronounced differences within the till treatment. While yields tended to be higher for 76R vs. rmc in tilled conditions, these differences were not found to be statistically significant. 76R plants did not have significantly greater RWC, gas exchange parameters, or stem water potential than rmc within the tilled treatment.
Compared to previous drought studies on AM fungi tomato model systems, stem water potential and δ13C were greater, but RWC was lower, in our field experiment (Bowles et al., 2016). Photosynthesis and stomatal conductance were lower under this water deficit, with WUEi increasing, suggesting that the plants were under significant water stress. Although other studies, including in controlled environments (Duc et al., 2023), have shown that AM fungi mediate water or heat stress, AM fungi may not have contributed to yield or physiological gains under water stress in this case. The impacts of water stress on gas exchange, carbon assimilation, and nutrient content likely resulted in lower total and red fruit tomato fruit production.
5 Conclusion
In this field study, we did not find evidence of no-till affecting dry-farmed tomato fruit yield, quality, or plant physiology, despite observed changes in surface soil properties, likely because tomato roots acquired most resources from soil depths below the region that tillage influences. Indeterminate tomato variety did influence fruit properties, including non-marketable yield, marketable number of fruit, and average fruit weight. While plants were certainly water-stressed, we did not detect that AM fungi significantly mediated these stress responses. Early Girl was a superior genotype, with future research needed to investigate the dry farming potential of other hybrid and heirloom genotypes. To optimize dry farming productivity as a meaningful option for food production in drier climates, strategies that increase and retain soil moisture should be combined with the selection of drought-tolerant, water-efficient varieties.
Data availability statement
The raw data supporting the conclusions of this article will be made available by the authors, without undue reservation.
Author contributions
AG: Conceptualization, Data curation, Formal analysis, Funding acquisition, Investigation, Methodology, Visualization, Writing – original draft, Writing – review & editing. CR: Conceptualization, Investigation, Methodology, Writing – review & editing. YS: Conceptualization, Funding acquisition, Investigation, Writing – review & editing, Methodology. YG-S: Investigation, Writing – review & editing. TB: Conceptualization, Funding acquisition, Investigation, Methodology, Project administration, Resources, Supervision, Writing – review & editing.
Funding
The author(s) declare financial support was received for the research, authorship, and/or publication of this article. YS and TB acknowledged grant GW21-224 from the Western Sustainable Agriculture Research and Education program. AG acknowledged financial support from the Fulbright Association, which was sponsored by the US Department of State and the Australian Fulbright Commission. AG was also supported by the University of Adelaide Charles John Everard Scholarship, AW Howard Memorial Trust—Tim Healey Supplementary Scholarship, and Playford Memorial Trust PhD Scholarship.
Acknowledgments
The authors gratefully acknowledge Emma Hughes, Kenzo Esquivel, Bethany Andoko, Amiri Taylor, and Khondoker Dastogeer for field work and technical assistance. The authors also wish to express our gratitude to Todd Dawson (Department of Integrative Biology, University of California, Berkeley) for providing plant physiology and stable isotope expertise and resources throughout the experiment. The authors acknowledge the many different groups working at Oxford Tract who helped manage this and previous experiments. AG acknowledges her supervisors at the University of Adelaide—Rachel Burton, Beth Loveys, and Tim Cavagnaro—for their support and encouragement to undertake the Fulbright Scholarship. The authors thank the editor and two reviewers for their insightful comments on the manuscript.
Conflict of interest
The authors declare that the research was conducted in the absence of any commercial or financial relationships that could be construed as a potential conflict of interest.
Publisher’s note
All claims expressed in this article are solely those of the authors and do not necessarily represent those of their affiliated organizations, or those of the publisher, the editors and the reviewers. Any product that may be evaluated in this article, or claim that may be made by its manufacturer, is not guaranteed or endorsed by the publisher.
Supplementary material
The Supplementary material for this article can be found online at: https://www.frontiersin.org/articles/10.3389/fsufs.2023.1301434/full#supplementary-material
References
Acevedo, S. E., Waterhouse, H., Barrios-Masias, F., Dierks, J., Renwick, L. L. R., and Bowles, T. M. (2022). How does building healthy soils impact sustainable use of water resources in irrigated agriculture? Elementa 10, 1–29. doi: 10.1525/elementa.2022.00043
Augé, R. M. (2001). Water relations, drought and vesicular-arbuscular mycorrhizal symbiosis. Mycorrhiza 11, 3–42. doi: 10.1007/s005720100097
Balate, C. A., Souza, D. C. D., Resende, L. V., and Freitas, S. T. D. (2018). Effect of abscisic acid on the calcium content for controlling blossom-end rot in tomato under water stress. Pesqui. Agropecu. Trop. 48, 414–419. doi: 10.1590/1983-40632018v4852048
Barker, K. (1985). “Nematode extraction and bioassays” in An advanced treatise on meloidogyne: volume II: methodology. eds. K. Barker, C. Carter, and J. Sasser (Raleigh, NC: Department of Plant Pathology, North Carolina State University)
Baxter, C. J., Carrari, F., Bauke, A., Overy, S., Hill, S. A., Quick, P. W., et al. (2005). Fruit carbohydrate metabolism in an introgression line of tomato with increased fruit soluble solids. Plant Cell Physiol. 46, 425–437. doi: 10.1093/pcp/pci040
Beyer, M., Koeniger, P., Gaj, M., Hamutoko, J. T., Wanke, H., and Himmelsbach, T. (2016). A deuterium-based labeling technique for the investigation of rooting depths, water uptake dynamics and unsaturated zone water transport in semiarid environments. J. Hydrol. 533, 627–643. doi: 10.1016/j.jhydrol.2015.12.037
Blanco-Canqui, H., and Ruis, S. J. (2018). No-tillage and soil physical environment. Geoderma 326, 164–200. doi: 10.1016/j.geoderma.2018.03.011
Bongers, T. (1990). The maturity index: an ecological measure of environmental disturbance based on nematode species composition. Oecologica 83, 14–19. doi: 10.1007/BF00324627
Bowles, T. M., Barrios-Masias, F. H., Carlisle, E. A., Cavagnaro, T. R., and Jackson, L. E. (2016). Effects of arbuscular mycorrhizae on tomato yield, nutrient uptake, water relations, and soil carbon dynamics under deficit irrigation in field conditions. Sci. Total Environ. 566–567, 1223–1234. doi: 10.1016/j.scitotenv.2016.05.178
Bowles, T. M., Jackson, L. E., Loeher, M., and Cavagnaro, T. R. (2017). Ecological intensification and arbuscular mycorrhizas: a meta-analysis of tillage and cover crop effects. J. Appl. Ecol. 54, 1785–1793. doi: 10.1111/1365-2664.12815
Bowles, T. M., Jilling, A., Morán-Rivera, K., Schnecker, J., and Grandy, A. S. (2022). Crop rotational complexity affects plant-soil nitrogen cycling during water deficit. Soil Biol. Biochem. 166:108552. doi: 10.1016/j.soilbio.2022.108552
Caretta, M. A., Mukherji, A., Arfanuzzaman, M., Betts, R. A., Gelfan, A., Hirabayashi, Y., et al. (2022). “Water” in Climate change 2022: impacts, adaptation and vulnerability: contribution of working group II to the sixth assessment report of the intergovernmental panel on climate change. eds. H.-O. Pörtner, D. C. Roberts, M. Tignor, E. S. Poloczanska, K. Mintenbeck, and A. Alegría, et al. (Cambridge: Cambridge University Press)
Cavagnaro, T. R., Barrios-Masias, F. H., and Jackson, L. E. (2012). Arbuscular mycorrhizas and their role in plant growth, nitrogen interception and soil gas efflux in an organic production system. Plant Soil 353, 181–194. doi: 10.1007/s11104-011-1021-6
Cavagnaro, T. R., and Martin, A. W. (2011). Arbuscular mycorrhizas in southeastern Australian processing tomato farm soils. Plant Soil 340, 327–336. doi: 10.1007/s11104-010-0603-z
CAWSI . (2023). Dry farming. Available at: http://agwaterstewards.org/practices/dry_farming/
Dang, Y. P., Page, K. L., Dalal, R. C., and Menzies, N. W. (2020). “No-till farming systems for sustainable agriculture: an overview” in No-till farming systems for sustainable agriculture. eds. Y. Dang, R. Dalal, and N. Menzies (Cham: Springer)
DeVries, M. S., Stock, B. C., Christy, J. H., Goldsmith, G. R., and Dawson, T. E. (2016). Specialized morphology corresponds to a generalist diet: linking form and function in smashing mantis shrimp crustaceans. Oecologia 182, 429–442. doi: 10.1007/s00442-016-3667-5
Dry Farming Institute . (2022). What is dry farming? Available at: https://dryfarming.org/about/what-is-dry-farming/
Dry Farming Institute . (2023). Dirty girl tomato. Available at: https://dryfarming.org/product/dirty-girl-tomato-starts/
Duc, N. H., Szentpéteri, V., Mayer, Z., and Posta, K. (2023). Distinct impact of arbuscular mycorrhizal isolates on tomato plant tolerance to drought combined with chronic and acute heat stress. Plant Physiol. Biochem. 201:107892. doi: 10.1016/j.plaphy.2023.107892
Dwivedi, S., Goldman, I., and Ortiz, R. (2019). Pursuing the potential of heirloom cultivars to improve adaptation, nutritional, and culinary features of food crops. Agronomy 9, 1–21. doi: 10.3390/agronomy9080441
Ferris, H., and Bongers, T. (2009). “Indices developed specifically for analysis of nematode assemblages” in Nematodes as environmental indicators. eds. M. Wilson and T. Kakouli-Duarte (Wallingford: CABI)
Foster, J. (1995). “Soil nitrogen” in Methods in applied soil microbiology and biochemistry. eds. K. Alef and P. Nannipieri (San Diego, CA: Academic Press)
Galviz, Y. C., Bortolin, G. S., Guidorizi, K. A., Deuner, S., Reolon, F., and de Moraes, D. M. (2021). Effectiveness of seed priming and soil drench with salicylic acid on tomato growth, physiological and biochemical responses to severe water deficit. J. Soil Sci. Plant Nutr. 21, 2364–2377. doi: 10.1007/s42729-021-00528-7
Garett, A. (2014). Common misconceptions and key points about dry farming: case study of dry farmer with more than 40 years of experience. Available at: https://smallfarms.oregonstate.edu/common-misconceptions-and-key-points-about-dry-farming-case-study-dry-farmer-more-40-years
Garett, A. (2019). Intro to dry farming organic vegetables. Available at: https://catalog.extension.oregonstate.edu/sites/catalog/files/project/pdf/em9229.pdf
Gill, A. R., Loveys, B. R., Cowley, J. M., Hall, T., Cavagnaro, T. R., and Burton, R. A. (2022). Physiological and morphological responses of industrial hemp (Cannabis sativa L.) to water deficit. Ind. Crop. Prod. 187, 115331–115311. doi: 10.1016/j.indcrop.2022.115331
Hoegh-Guldberg, O., Jacob, D., Taylor, M., Bindi, M., Brown, S., Camilloni, L., et al. (2018). “Impacts of 1.5°C of global warming on natural and human systems”, in Global Warming of 1.5°C. An IPCC Special Report on the impacts of global warming of 1.5°C above pre-industrial levels and related global greenhouse gas emission pathways, in the context of strengthening the global response to the threat of climate change, sustainable development, and efforts to eradicate poverty. (Eds) V. P. Masson-Delmotte, H.-O. Zhai, D. Pörtner, J. Roberts, P. R. Skea, and A. Shukla, et al. (Cambridge, UK and New York, NY, USA: Cambridge University Press), pp. 175–312.
IPCC . (2023). Climate change 2023: synthesis report: contribution of working groups I, II and III to the sixth assessment report of the intergovernmental panel on climate change. Geneva: IPCC.
Kuznetsova, A., Brockhoff, P. B., and Christensen, R. H. B. (2017). lmerTest package: tests in linear mixed effects models. J. Stat. Soft. 82, 1–26. doi: 10.18637/jss.v082.i13
Laliberté, E. (2018). Measuring specific leaf area and water content. Protocols, 1–14. doi: 10.17504/protocols.io.p3tdqnn
Leap, J., Wong, D., and Yong, C. K. (2017). Organic dry-farmed tomato production on California’s central coast: a guide for beginning specialty crop growers. Santa Cruz: Center for Agroecology.
McGonigle, T. P., Miller, M. H., Evans, D. G., Fairchild, G. L., and Swan, J. A. (1990). A new method which gives an objective measure of colonization of roots by vesicular—arbuscular mycorrhizal fungi. New Phytol. 115, 495–501. doi: 10.1111/j.1469-8137.1990.tb00476.x
Miranda, K. M., Espey, M. G., and Wink, D. A. (2001). A rapid, simple spectrophotometric method for simultaneous detection of nitrate and nitrite. Nitric Oxide 5, 62–71. doi: 10.1006/niox.2000.0319
Nabhan, G., and McKibben, B.. (2013). Growing food in a hotter, drier land: lessons from desert farmers on adapting to climate uncertainty. Chelsea, VT: Chelsea Green Publishing.
Nangare, D. D., Singh, Y., Kumar, P. S., and Minhas, P. S. (2016). Growth, fruit yield and quality of tomato (Lycopersicon esculentum mill.) as affected by deficit irrigation regulated on phenological basis. Agric. Water Manag. 171, 73–79. doi: 10.1016/j.agwat.2016.03.016
Nemeskéri, E., Neményi, A., Bőcs, A., Pék, Z., and Helyes, L. (2019). Physiological factors and their relationship with the productivity of processing tomato under different water supplies. Water 11:586. doi: 10.3390/w11030586
Parr, J., Stewart, B., Hornick, S., and Singh, R. (1990). “Improving the sustainability of dryland farming systems: a global perspective” in Advances in soil science, dryland agriculture: strategies for sustainability. eds. R. Singh, J. Parr, and B. Stewart (New York, NY: Springer-Verlag)
Peterson, G. A., Westfall, D. G., Schipanski, M. E., and Fonte, S. J. (2020). Soil and crop management systems that ameliorate damage caused by decades of dryland agroecosystem mismanagement. Agron. J. 112, 3227–3238. doi: 10.1002/agj2.20257
Pinheiro, J., Bates, D., Debroy, S., Sarkar, D., Heisterkamp, S., Van Willigen, B., et al. (2021). nlme: linear and nonlinear mixed effects models. Version 3.1-163. Available at: https://cran.r-project.org/web/packages/nlme/nlme.pdf (Accessed August 16, 2023)
Pittelkow, C. M., Linquist, B. A., Lundy, M. E., Liang, X., van Groenigen, K. J., Lee, J., et al. (2015). When does no-till yield more? A global meta-analysis. Field Crop Res. 183, 156–168. doi: 10.1016/j.fcr.2015.07.020
R Core Team . (2021). R: a language and environment for statistical computing. Version 4.1.1. Available at: https://www.R-project.org/ (Accessed August 17, 2023).
Rainey, C., Egerer, M., Herrmann, D., and Bowles, T. (2020). “Restoring soil and supporting food sovereignty across urban–rural landscapes: an interdisciplinary perspective”, in Urban Agroecology: Interdisciplinary Research and Future Directions, (Eds). M. Egerer and H. Cohen (USA: CRC Press), 95–126.
Rainey, C., Varas, T., Kaiser, P., Kaiser, E., Smith, W., Guzman, A., et al. (in preparation). Rapid changes in soil structure, ecology, and functioning under labor and knowledge intensive no-till farming.
Rudich, J., Rendon-Poblete, M., Stevens, M., and Ambri, A.-I. (1981). Use of leaf water potential to determine water stress in field-grown tomato plants. J. Am. Soc. Hortic. Sci. 106, 732–736. doi: 10.21273/JASHS.106.6.732
Sainju, U., Singh, B., Rahman, S., and Reddy, V. (2000). Tomato root growth is influenced by tillage, cover cropping, and nitrogen fertilization. HortScience 35, 78–82. doi: 10.21273/HORTSCI.35.1.78
Saure, M. C. (2001). Blossom-end rot of tomato (Lycopersicon esculentum mill.) - a calcium- or a stress-related disorder? Sci. Hortic. 90, 193–208. doi: 10.1016/S0304-4238(01)00227-8
Seleiman, M. F., Al-suhaibani, N., Ali, N., Akmal, M., Alotaibi, M., and Refay, Y. (2021). Drought stress impacts on plants and different approaches to alleviate its adverse effects. Plan. Theory 10:259. doi: 10.3390/plants10020259
Shaver, T. M., Peterson, G. A., Ahuja, L. R., Westfall, D. G., Sherrod, L. A., and Dunn, G. (2002). Surface soil physical properties after twelve years of dryland no-till management. Soil Sci. Soc. Am. J. 66, 1296–1303. doi: 10.2136/sssaj2002.1296
Socolar, Y., Matta, T. J., Rodríguez Fuentes, M., Andoko, B., Cook, J., Schirmer, J., et al. (in preparation). Deep nutrients and fungal communities support tomato fruit yield and quality in dry farm management systems.
Sun, J., Yang, L., Yang, X., Wei, J., Li, L., Guo, E., et al. (2021). Using spectral reflectance to estimate the leaf chlorophyll content of maize inoculated with arbuscular mycorrhizal fungi under water stress. Front. Plant Sci. 12, 1–12. doi: 10.3389/fpls.2021.646173
Watts-Williams, S. J., and Cavagnaro, T. R. (2012). Arbuscular mycorrhizas modify tomato responses to soil zinc and phosphorus addition. Biol. Fertil. Soils 48, 285–294. doi: 10.1007/s00374-011-0621-x
Keywords: agroecology, arbuscular mycorrhizal fungi, dry farming, no-till agriculture, tillage, tomato, water stress
Citation: Gill AR, Rainey C, Socolar Y, Gil-Santos Y and Bowles TM (2024) Comparing dry farming of tomatoes across varieties and soil management history. Front. Sustain. Food Syst. 7:1301434. doi: 10.3389/fsufs.2023.1301434
Edited by:
Ali Shahnazari, Sari Agricultural Sciences and Natural Resources University, IranReviewed by:
Prosanta K. Dash, Texas A&M University at Qatar, QatarEmmanuel Torres Quezada, Virginia Tech, United States
Copyright © 2024 Gill, Rainey, Socolar, Gil-Santos and Bowles. This is an open-access article distributed under the terms of the Creative Commons Attribution License (CC BY). The use, distribution or reproduction in other forums is permitted, provided the original author(s) and the copyright owner(s) are credited and that the original publication in this journal is cited, in accordance with accepted academic practice. No use, distribution or reproduction is permitted which does not comply with these terms.
*Correspondence: Alison R. Gill, YWxpc29uLmdpbGxAYWRlbGFpZGUuZWR1LmF1