- 1Embrapa Genetic Resources and Biotechnology, Embrapa Cenargen, Brasília, Brazil
- 2Biotechnological Center, University of Western Santa Catarina, UNOESC, Videira, Brazil
- 3Faculty of Economics, Administration and Accounting of Ribeirão Preto, University of São Paulo, USP, São Paulo, Brazil
- 4EAESP Business School, FGV, São Paulo, Brazil
- 5Genomic Sciences and Biotechnology, Catholic University of Brasília, Brasília, Brazil
- 6National Institute of Science and Technology, INCT PlantStress Biotech, Embrapa Cenargen, Brasília, Brazil
The world’s population is expected to grow by 30%–35% over the next 60 years. Forecasts indicate that the world’s population will reach almost 10 billion by 2050, with India and China as the most populous countries. As a result, the demand for global food production, particularly protein and dairy products, and their nutritional quality will need to increase by 50%–75%. In addition to increasing food production, it is also necessary to consider and reduce the impact on the environment and ecosystem. On the one hand, the threat of climate change, the reduction of arable land for agricultural expansion, the economic impact of geopolitical conflicts, the human and animal health pandemics, the conjuncture of the domestic political environments, and the demand for new technologies are the main bottlenecks to increasing sustainable food production worldwide. In contrast, notable technological advances have been achieved in current agriculture through basic and advanced scientific research, development, innovation, and technology transfer to the agribusiness sector. Technological advances in various sectors will become increasingly important to increase food production and minimize environmental impacts. This review study briefly highlights the major technological advances in world agriculture that have contributed to the substantial increase in food production from the early days of extractive agriculture to high-performance agriculture. It then highlights the key breakthroughs, disruptive technologies, the impact of climate change on agriculture, and contributions from molecular sciences that are revolutionizing global agriculture, focusing on Brazilian agriculture, livestock, and agribusiness. Subsequently, the evolution of Brazilian agriculture is highlighted based on the market share of agricultural products and its relevance to the national GDP. Finally, the potential decision-making that could have a positive impact on the Brazilian agribusiness sector and that will affect the import and export of agribusiness products were addressed. Therefore, the importance of supporting the agribusiness sector to increase healthy food production with higher nutritional quality and with less impact on the environment and human life was highlighted.
1 Introduction
The world’s population is expected to grow by 30%–35% over the next 50 to 100 years. It is projected to reach nearly 9.7 and 10.4 billion by 2050 and 2,100, respectively, with India and China as the most populous countries (United Nations, 2023). As an immediate consequence of this, the demand for global food production and supply, especially protein and dairy products, and their nutritional quality will need to increase by 35%–56% between 2010 and 2050 (van Dijk et al., 2021). Furthermore, the supply of these products at stable and fair prices to the low-income population will be crucial to minimizing the effects of food shortages and hunger (Falcon et al., 2022). Among these human foods, the vast majority depend on agriculture for their direct or indirect production. In the group of directly produced foods are those offered in natura, minimally processed, processed, or as by-products, while in the group of indirect foods are those that depend entirely on agricultural raw materials for their production, such as pork, poultry, beef, eggs, milk, among others. In addition, agriculture is also prominent in bioenergy production (such as biodiesel, biomethane, bioethanol, and biohydrogen) and other non-food by-products that contribute to the planet’s economic and social stability (Nair et al., 2022; Santos et al., 2023). Fortunately, since the origin of human civilization and with the exponential growth of the population over time, agriculture has evolved in parallel with a special highlight on growing food production (Thrall et al., 2010; Grossi-de-Sa and Basso, 2024). In this context, several technological advances have brought remarkable contributions on different fronts to global agriculture and livestock.
Despite these essential technological advances in agriculture, related environmental factors have brought to the discussion a scenario of concern that challenges the production and supply of food for this growing global population. The eminent climate changes have impacted the entire production system, people’s lives, and global agribusiness as a whole in recent years due to direct effects and as a result of decision-making as measures to mitigate these effects (Wake, 2023). These direct impacts of climate change are increasingly extreme and have a negative impact on the global production system (Bibi and Rahman, 2023). Therefore, the adoption of agricultural and urban measures, as well as technologies that enable more sustainable agriculture, are essential for human life on Earth planet in terms of food production and stopping the mass extinction of people as a result of climatic and environmental disasters (Neves, 2014; Neves, 2020).
The current adoption of various eco-friendly technologies is completely transforming global agribusiness, clinging to the sustainability of the ecosystem and agriculture (Bolfe et al., 2023; Neves et al., 2023). In particular, molecular sciences through basic and applied scientific research in the development of products and solutions have brought numerous contributions to agriculture (Reis et al., 2023). These contributions from molecular sciences range from cultivars better adapted to the climatic conditions of each producing region, improved resistance to pathogens and insect pests, greater productivity and yield, to better-adapted cultivars to climate changes (Das et al., 2023). Therefore, the investment in scientific research and the development of disruptive technologies that allow for more environmentally sustainable agriculture and livestock production is strongly necessary for the current scenario global.
Herein, this review study addressed (i) the main issues related to the evolution of agriculture, (ii) the technologies that have emerged to help meet the growth in demand for sustainable production, (iii) the impacts of climate changes on agriculture, and (iv) the contributions of molecular sciences for high-performance agriculture, focusing on Brazilian agribusiness. Furthermore, this review study also addressed and discussed (v) the current status of Brazilian agribusiness, (vi) the importance of scientific research, development, and innovation to make it possible to supply food, bioenergy, and other agri-based products in a sustainable way, and (vii) the major governmental, public, and private initiatives necessary to achieve these goals.
2 Materials and methods of data explored
The literature used in this review study is based on scientific research and public data mining, which were duly cited and bibliographic references were listed. The genetically modified events and agronomic traits approved by the National Technical Biosafety Commission (CTNBio) to date for commercial use in Brazil (Supplementary File S1) were retrieved from the CTNBio open access website (CTNBio, 2023)1 and, subsequently, these data were manually curated. The number of biological products registered in Brazil for commercial use, the amount of agricultural areas treated with biodefensives, and the number of biological products registered by target organism were retrieved from the Ministry of Agriculture, Livestock and Food Supply open access website (MAPA 2023a)2 and from CropLife Brazil open access website (CropLife Brasil, 2023).3 The agricultural land use, ranking of crops and livestock, and Brazilian agricultural production data were retrieved from the Brazilian Institute of Geography and Statistics open access webpage (IBGE, 2023)4 and MAPA open access (MAPA 2023b).5 The trade balance data between imports and exports and the Brazilian agribusiness contribution data were retrieved from the Comex Stat/SECINT open access webpage, which was elaborated by the Institute for Applied Economic Research (IPEA/COMEX Stat, 2023).6 Data on Brazilian agribusiness export by sector and importation ranking in gross production value were retrieved from the Observatory of Economic Complexity open access webpage (OEC, 2023).7 The Gross Domestic Product (GDP) data of the Brazilian agribusiness, agriculture, and livestock were retrieved from the Center for Advanced Studies on Applied Economics Department of Economy, Administration and Sociology open access webpage (CEPEA USP/ESALQ, 2023).8 Meanwhile, the world agricultural production ranking data were retrieved from the United States Department of Agriculture Foreign Agricultural Service open access webpage (USDA, 2023a)9 and from the Brazilian Sugarcane Industry Association open access webpage (UNICA, 2023).10 The world ranking data of livestock and poultry production and exports were retrieved from the USDA open access webpage (USDA, 2023b).11
3 Evolution of agriculture and major technological achievements
The first humans were reported to have appeared in East Africa about 2.5 million years ago. In the Paleolithic period (500,000 B.C.), they were characterized by a totally nomadic lifestyle, surviving mainly by hunting, fishing, and gathering plants, fruits, and seeds (Figure 1). In the Neolithic period (10,000 B.C.), humans began to experiment with agriculture, and although they still lived as typical nomads, they discovered that planting seeds was a safer way of producing and harvesting their food source. These early advances in primitive agriculture, highlighted by the planting of seeds, led to the first Agricultural Revolution or Neolithic Revolution (8,500 B.C.). This first revolution was based on the possibility of not only extracting but also planting and raising domestic animals for human survival. Soon after observing these possibilities of cultivating green plants and raising animals, prehistoric humans understood that a sedentary and more civilized lifestyle would be the most viable way in this scenario. The first proper human civilizations were reported in ancient China (8,000 B.C.), in the valleys of the Huang-Ho (also called Yellow River) and Yangtze (also called Blue River) rivers. However, it was in Ancient Egypt (5,000 B.C.) in Northeast Africa, on the banks of the Nile River, that agriculture was of paramount importance in sustaining human civilization. In addition to the flooding of the Nile Valley, which was considered very important for local agriculture because it made the land more fertile and productive, animal traction gained great importance for agricultural activity (Diamond, 2002). However, it was in the Middle Ages (500–1,000 A.D.) that agriculture regained its importance, due to its greater dominance, through the use of land management techniques and cultivation systems. Although it did not have major technologies, this fact characterized agriculture at that time, which was totally dependent on human and animal labor. The first technological milestones in agriculture occurred in the 17th century, such as with the invention of the first seed planter, which was first driven by human power and later moved by animal power (Jethro Tull, 1976). Among other advances, this invention served as a reference and incentive for the development and application of new technologies in agriculture, with the aim of reducing dependence on human labor and increasing crop productivity and yields. The practical application of these first technologies in agriculture marked the well-known second Agricultural Revolution, which took place in the 17th century. Later, another remarkable technological milestone that shaped world agriculture was the invention of the first seed harvester in the 19th century (Cyrus McCormick, 1809). This technological milestone demonstrated that in addition to planting seeds, harvesting could also be mechanized and optimized. In addition to mechanization, other factors began to play an important role in the development and evolution of agriculture, such as organic and chemical fertilization of agricultural land. In particular, soil fertilization in the Middle Ages was basically characterized by the use of organic waste, manure, carcasses, and river humus. However, the most important milestone in soil fertilization was the advent of mineral fertilization using NPK (nitrogen, phosphorus, and potassium) formulations in the 19th century (Justus von Liebig, 1842). The supplementation of land soil nutrients, such as the use of NPK, was of great relevance as the nutrition provided by organic waste was no longer sufficient for stable production. Despite the momentary dominance of planting, handling, and harvesting technologies, it was also demonstrated in the 19th century that it was possible to make targeted crosses between sexually compatible plant species (Gregor J. Mendel, 1865). This first plant breeding approach indicated that genetic characteristics of interest could be combined or transferred between different compatible species to produce superior cultivars (Miko, 2008). Shortly before Mendel’s three laws were scientifically validated, the appearance of potato late blight disease in Ireland (1845–1850) devastated the potato crop and caused 2 million people to starve and 1 million to emigrate. Subsequently, this potato disease was characterized by the scientist Heinrich Anton de Bary (1853) as being caused not by nutritional or abiotic stress conditions but by the phytopathogenic fungus Phytophthora infestans (Bourke, 1964).
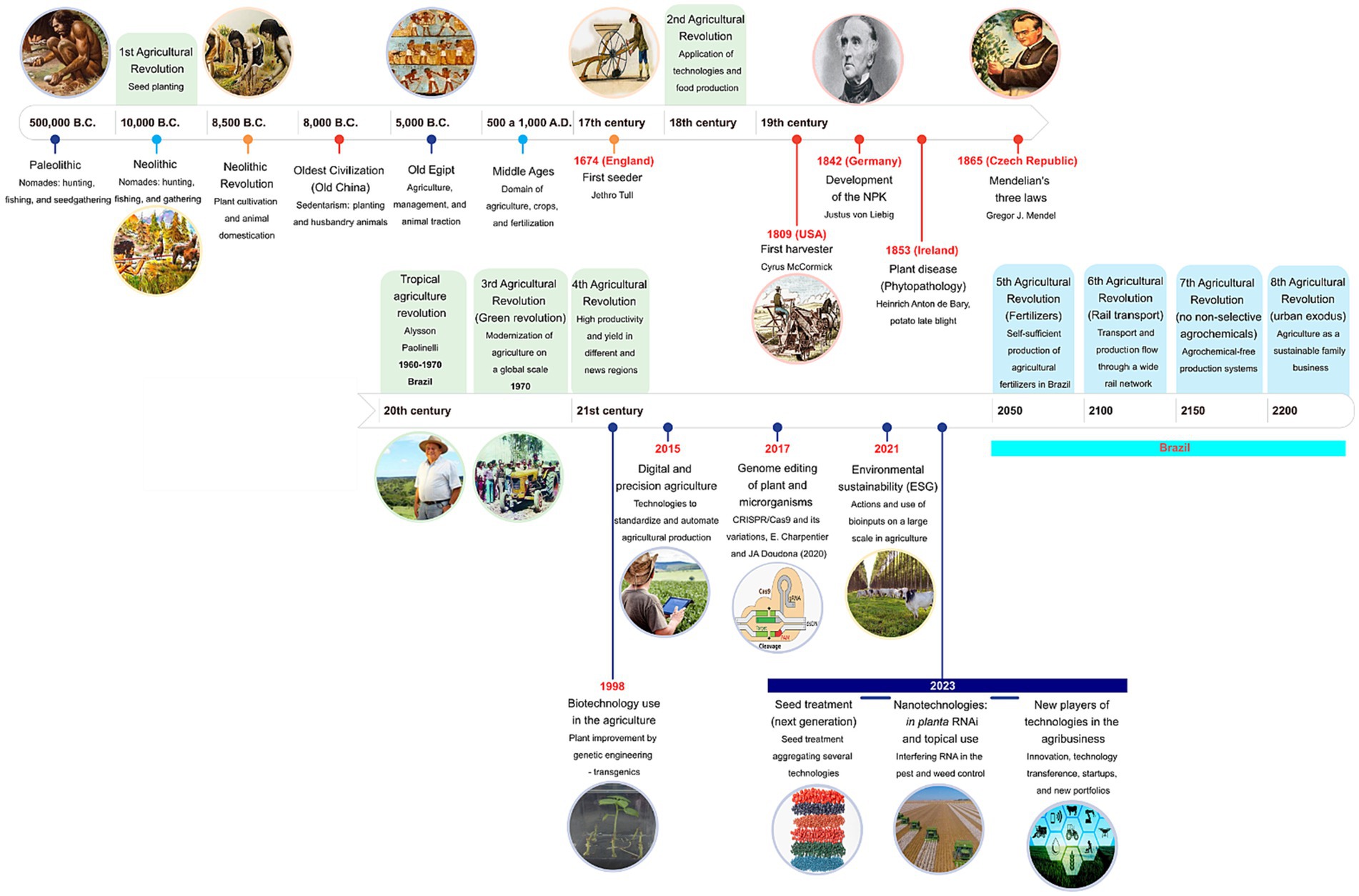
Figure 1. Evolution of global agriculture and its techniques over the years to the present day, and optimistic forecasts for the next 200 years of Brazilian agriculture based on demand and benefits for agribusiness that can have an impact on self-sufficient food production. The timeline presented highlights the major milestones in worldwide agriculture, with an emphasis on the technological advances made in Brazilian agriculture in recent years. B.C., Before Christ; A.C., After Christ; NPK, macronutrients nitrogen, phosphorus, and potassium; CRISPR/Cas9, Clustered Regularly Interspaced Short Palindromic Repeats-associated Cas9 endonuclease; ESG, Environmental, Social And Governance. Credits: Photos retrieved from the Google platform (original authors not mentioned in this source). Figure scheme adapted from Grossi-de-Sa and Basso (2024).
These collective discoveries warned that world agriculture had great potential for expansion of agricultural area and growth of production and yield, but that this activity depended on constant technological and scientific development and advances. This noticeable message brought challenges and good results over the years, marked by outstanding multidisciplinary advances, especially in Brazilian tropical agriculture, through the application of scientific knowledge and practical concepts for better agriculture exploration, taking into account its geographic specificities. In particular, the generation and application of scientific knowledge in Brazilian regional agriculture marked the Sustainable Tropical Agriculture Revolution in the 20th century (Alysson Paulinelli, 1936–2023). Among the most important advances of this revolution are the direct planting of major crops without disturbing the soil (no-till farming), planting windows for each region based on climatic conditions, and irrigation management. The adoption of these measures allowed at least two harvests per agricultural year in certain regions, more efficient land use, complete and balanced fertilization, livestock-forestry integration, consolidation of scientific research centers truly focused on solutions for regional agriculture, among others, which led Brazil to food self-sufficiency. These collective advances at the global level contributed to the third Agricultural Revolution that occurred in the 20th century (1960–1970). This third agricultural revolution was mainly marked by the modernization of agriculture through the application of technologies on various fronts, in particular from mechanization and automation-related technologies. Soon after, with the remarkable advances in crop and animal breeding and in technologies and cropping systems in different growing regions, the fourth Agricultural Revolution occurred in the 21st century. This fourth revolution was mainly characterized by high productivity and yield in different regions of each country, high quality of harvested products, traceability of agricultural processes, use of artificial intelligence, big data, and plant or crop phenotyping by unmanned aerial vehicles coupled with cameras containing RGB (Red, Green, and Blue), thermal, and multispectral systems for aerial images. Consequently, this era of digital technologies in agriculture has been conceptualized as precision agriculture, which has come to automate agricultural production and transform it into a more dynamic, user-friendly, and networked environment. In particular, precision agriculture uses information technology and is summarized in a large set of tools that collect, store, process, and analyze data from different agricultural operations to help the farmer make more confident decisions (Akmal et al., 2020; Bharadiya et al., 2023). Similar to these digital technologies used in agriculture, additional analyses have focused on the genomic DNA of target organisms by using large-scale DNA or RNA sequencing techniques from platforms that allow to generate large amounts of highly informative data in a few hours or days at a low cost. These genomic analyses make it possible to obtain and use the information at the DNA level of plants, animals, pathogens, and beneficial microorganisms in general to produce genetically better crops or animals (Marks et al., 2021).
In the last three decades, the use of plant genetic engineering in agriculture [e.g., transgenic soybean tolerant to the herbicides glyphosate (Monsanto/Bayer, event GTS 40-3-2, 1994) and imidazolinone (Embrapa/BASF, event BPS-CV127-9, Brazilian CTNBio Technical Opinion N. 2236/2009)] through transgenesis has demonstrated that new biotechnological tools can be developed by manipulating the genetic material of a plant to develop new traits (Rolla et al., 2014; Ribeiro et al., 2017, 2019; Basso et al., 2021). In addition to herbicide tolerance, other already commercial biotechnologies using transgenic approaches have been developed to improve plant resistance to insect pests. For example, plants overexpressing the Cry and/or Vip proteins or the HaHB4 (Helianthus annuus Homeobox 4) transcription factor (Ribichich et al., 2020; CTNBio, 2023) demonstrated improved resistance to insect pests and tolerance to drought stress, respectively. In particular, a transgenic common bean (Phaseolus vulgaris) was developed by Embrapa Genetic Resources and Biotechnology (Brasília, DF, Brazil), carrying interfering RNA (RNAi) technology targeting Bean golden mosaic virus (BGMV, Begomovirus) and conferring an improved plant resistance against this virus (Aragão et al., 2013). Interestingly, this transgenic event was the first RNAi-based crop (commercial name: BRS-FC401-RMD) produced by a completely Brazilian initiative and autonomy and, subsequently, approved for commercial use in Brazil (ISAAA, 2023). Brazil currently ranks second in the adoption of transgenic crops (soybean, maize, sugarcane, wheat, eucalyptus, cotton, and common bean), surpassed by the United States and followed by Argentina, Canada, and India (ISAAA, 2023). In a similar scenario, there are no sources of varietal resistance or transgenic cotton events with resistance to the cotton boll weevil (CBW, Anthonomus grandis) that have been commercially released to date. Thus, the development of a transgenic plant with high resistance to CBW could mean less use of agrochemicals and higher yields in cotton crops. In view of these possibilities, Embrapa Genetic Resources and Biotechnology is developing several scientific research projects aimed at developing transgenic-based technologies capable of controlling the CBW. In brief, these new technologies for cotton to control CBW use the expression of Cry toxins or the combination of Cry toxins with RNAi, both of which have already shown promising results in transgenic cotton lines evaluated under greenhouse conditions (Ribeiro et al., 2017, 2019, 2022; Vasquez et al., 2023).
In parallel with transgenesis, the clustered regularly interspaced short palindromic repeats (CRISPR)/CRISPR-associated protein 9 (Cas9) genome editing technology has been successfully established and applied in various important crops (Jinek et al., 2012; Basso et al., 2019). This new genome editing technology, which is considered simpler and more effective, has opened up new alternatives for plant improvement through genetic engineering, allowing desired genes to be switched on or off in the plant itself without the need to introduce a transgene (Basso et al., 2020; Távora et al., 2022). In this way, the use of this genome editing technology makes it possible to create superior cultivars with unprecedented agronomic traits, while reducing development time and costs, as well as fast regulation of new cultivars or traits. For example, genome editing can be used to develop crops with improved tolerance to abiotic stresses (e.g., cultivars with improved tolerance to rainfall deficiency or excess, soil acidity, salinity, cold, heat, and heavy metals) and resistance to biotic stresses (e.g., cultivars with improved resistance to insect pests and pathogens), thereby increasing crop productivity, yield, health, and nutritional quality (Yin et al., 2017). More specifically, as already real examples, CRISPR/Cas9 genome editing technology has been used to obtain sugarcane, soybean, and maize with improved agronomical features. Between among, sugarcane with better cell wall digestibility and improved conversion to 2G ethanol developed by Embrapa Agroenergy (Brasília, DF, Brazil), soybean with better tolerance to drought also developed by Embrapa Soybean (Londrina, PR, Brazil), maize with increased amylopectin developed by Corteva and commercially approved by CTNBio in 2018 as non-transgenic (Gao et al., 2020), and soybean with better yield or without antinutritional factors developed by GDM Seeds (Londrina, PR, Brazil). As already well observed in plants, genome editing of beneficial microorganisms for crops has also been explored, with the aim of improving their symbiotic relationship with plants (Yi et al., 2018; Wang et al., 2021). In addition to the outstanding results with plant genome editing, RNAi technology delivered by using transgenic plants or by topical RNAi delivery has also gained considerable prominence in the field of plant biotechnology. In a particular example, post-transcriptional gene silencing or downregulation of the AIP10 (ABAP1-interacting protein 10) gene in soybean and cotton by using RNAi has been shown to increase the earliness and yield of biomass, grain, and flower buds (Ferreira et al., 2014; Hemerly et al., 2022).
4 Impact of climate changes on Brazilian agriculture
The most important meteorological elements for agriculture are air temperature, solar radiation, air humidity, wind speed and direction, and the level of spatiotemporal precipitation (Howden et al., 2007). The balance of these factors in conditions closer to ideal allows agriculture to be carried out close to its maximum potential and uniformity. Rainfed agriculture is the prevalent method in agricultural countries around the world, with irrigation systems being used in a few cases, generally in small areas and crops with a higher financial return per cultivated area (He and Rosa, 2023). Therefore, most agriculture worldwide is prone to adversities imposed by climate changes, such as lack or excess of rain, hailstorms, cross-stress due to this imbalance (e.g., nutritional, pathogens, insect pests, others), weakening of the soil and microbiota, changes in planting, pruning, and harvesting windows, and incompatibility of some commercial cultivars to the production regions with seasonally changed climatic conditions (Howden et al., 2007; Elli et al., 2022). On the one hand, the regional climatic balance and uniformity have enabled the development and consolidation of commercial cultivars with highly adapted agronomic characteristics for each of these regions, but with these severe and non-linear climate changes, cultivars and the plant breeding pipeline need to be often optimized (Tillman et al., 2011; Rezaei et al., 2023). Furthermore, the cultural management system, such as planting, harvesting, fertilization, row spacing, seeding rate, use of inputs and by-inputs, application of pesticides, and other factors, needs to be reviewed taking into account the expected regional climatic conditions (Souza et al., 2019).
Within global climate changes, there are some regional climate phenomena that have resulted from this change set. A particular example, “El-Niño” is a seasonal atmospheric-oceanic phenomenon in which warming occurs beyond the normal temperatures of superficial waters in the equatorial position of the Pacific Ocean, altering wind patterns, rainfall, and average temperatures of South America (Moura et al., 2019). Data from the National Oceanic and Atmospheric Administration revealed an increase up to 2.2°C in the sea surface temperature (SST) index from February to November of 2023 in determined regions of the equatorial Pacific Ocean, compared with previous years, particularly the last more intense El ñino (2015/16 years) with 2.6°C SST index (NOAA, 2023). Since “El niño” causes strong excess rainfall in the Brazilian subtropical region and water deficit in the Brazilian tropical and equatorial regions, the phenomenon “La niña” is the opposite of “El niño” (Lin and Qian, 2019). The “La niña” phenomenon is characterized by the cooling of superficial waters in the equatorial position of the Pacific Ocean and consequent strong lack of rainfall in the Brazilian subtropical region while rainfall excess in the Brazilian tropical and equatorial regions (Lin and Qian, 2019; Chand et al., 2023). The “El niño” versus “La niña” phenomena last from nine to 12 months but sometimes last for years, and commonly occur at intervals of two to seven years on an irregular schedule, with “El Niño” occurring more frequently than “La Niña” (Wang et al., 2023). The “El Niño” versus “La niña” impacts on the global economy equates to multi-trillion US dollars in loss, ranging US$ 2.1 and 3.9 trillion globally, respectively, to the 1997/98 and 2015/16 years caused by “El Niño” extreme events (Liu et al., 2023). Therefore, these damages and losses are causes and effects under both urban and rural infrastructure as well as agriculture and livestock in general, impacting severely human life and the ecosystem worldwide.
Deforestation and general urbanization are considered the main trigger of the climate changes (Alves de Oliveira et al., 2021; Li et al., 2022; Smith et al., 2023). The greenhouse gas (GHG) emissions is one of the major factors that drive climate change worldwide, where China (12,295 Gt), the United States (5,289), India (3,166), Russia (1,799), Indonesia (1,475), Brazil (1,469), and Japan (1,062) are the most emitting countries in gigatonnes of CO2 equivalent (Reference year 2020; ClimateWatch, 2023). However, GHG emissions from agricultural activity correspond to only 20%–30% of total emissions per country. On the one hand, this increase in CO2 can lead to photosynthetic gain both in C3 (approx. 20%–30%) and C4 (approx. 5%–10%) plants, and better water use efficiency with greater emphasis on crops kept under the rainfed system (Ainsworth and Long, 2005). Meanwhile, the gradual increase in temperature can improve the yield of some crops in particular, such as cotton, grasses, and sugarcane (Silva et al., 2021b; Sharma et al., 2022). However, a linear or non-linear increase in minimum and maximum air temperature and the consequent reduction in the length of the crop cycle or phenological stages, clearly suggest, in general, a decline in agricultural production and yield if current agricultural systems remain unchanged (Souza et al., 2019; Liu and Dai, 2020; Zilli et al., 2020; Sharma et al., 2022). In addition, the rainfall shortages or excess can represent an estimated decline of up to 24 and 29% in soybean and maize yield from their normal upward trends by the year 2,100, which are estimated to still have an increased yield of 30%–66% and 30%–57%, respectively (Osei et al., 2023). In particular, the average annual losses in Brazilian gross domestic product due to climate change were predicted from 0.4% to 1.8% until 2050 (Souza and Haddad, 2022). Therefore, the use of technologies ranging from crop management, agrometeorological monitoring, agricultural zoning, good agricultural practices, and by-inputs to plant genetics, such as early genotypes or with long cycles, containing traits associated with improved resistance or tolerance, or for greater yield or biofortified, can minimize the reduction in crop productivity and yield as a consequence of climate change (Basso et al., 2019; Munaweera et al., 2022). Despite all this, an optimistic scenario is envisioned for the next 50 to 100 years for global agriculture, mainly the major agricultural players, with the implementation of eco-sustainable and disruptive technologies. Likewise, the challenges of this process are irreversible and necessary to achieve the goals of sustainable food production.
5 Major contributions of molecular sciences to Brazilian agriculture
Traditional plant breeding has strongly contributed greatly to the expansion and sustainability of agriculture in Brazil over the years. Through these improvement methods, high-performance cultivars have been achieved, with improved productivity, yield, resistance to pathogens, tolerance to abiotic stresses, and better adaptation to the climatic conditions of each region of the country. Traditional plant breeding programs have, over the years, incorporated several major crops in their pipeline, as well as crops of lesser importance in recent years, making it possible to diversify and expand agricultural production in all Brazilian regions (Greschuk et al., 2023; Martha Junior and Lopes, 2023). The maintenance and exploitation of germplasm banks by different private and public companies have led to the development of conventional cultivars and traits that are still quite relevant to date for different crops. These directional crossing approaches of contrasting cultivars assisted by phenotypic analyses have been supported over the years by molecular analyses that have ensured a faster and more robust development of superior cultivars with desirable agronomic traits. Among these analyses included molecular marker-assisted selection (e.g., RAPD, RFLP, AFLP, and microsatellites), which focuses on the information contained in the plant’s genomic DNA and allows greater assertiveness and shorter development times compared to the phenotypic method alone (Salgotra and Stewart, 2020). More recently, more robust genomic analyses have gained prominence due to advances in genomic DNA sequencing technologies and bioinformatics expertise, allowing low cost per project, high quality of generated data, and short generation and assembly time (Hu et al., 2018). Finally, molecular markers based on SNPs (Single Nucleotide Polymorphisms) have made a major contribution in recent years to consolidating plant breeding based on genomic selection, supported by targeted genomic region sequencing and real-time PCR analysis using allele-specific TaqMan probes (Sinha et al., 2023). Thus, SNP markers linked with agronomic traits of interest, or QTLs (Quantitative Trait Loci), have been widely used in conjunction with TaqMan probes as a reference for transferring these traits to superior cultivars of different crops. In particular, the use of these TaqMan probes has made it possible to monitor the insertion, segregation, homozygosity, and stability of these traits in segregating populations effectively and at a reduced cost (Woodward, 2014). Additionally, this technology based on molecular markers and TaqMan probes has also been successfully used for varietal analysis and trait purity in commercial cultivars.
Among the main agronomic traits that Brazilian plant breeders focus on include the highest grain yield (e.g., soybean, maize, rice, sesame, bean, and castor bean), plant biomass (e.g., sugarcane, energy cane, silage maize, and sorghum), sugar (e.g., sugarcane), textile fiber (e.g., cotton), resistance to the most relevant biotic diseases (e.g., fungi, nematodes, bacterial, and viral diseases in different crops), lower requirement for winter cold (e.g., temperate fruit trees), and adaptation to the different micro-regional environments of the country. More recently, the range has been extended to include traits associated with better tolerance to abiotic stresses (e.g., drought, flooding, and low-temperature tolerance), resistance to insect pests, and nutritional improvements in grain and biomass. However, some important agronomic traits, such as resistance to herbicides and insect pests, could not be obtained using these conventional methods. In this context, genetic engineering methods based on transgenic plants have been used to develop agronomic traits related to these features (Figures 2A–D) (Basso et al., 2019, 2020). The use of transgenic approaches in the major crops for the stable overexpression of desirable genes conferring resistance to widely used herbicides has contributed significantly to weed management (Wong et al., 2022). Among these engineered genes used in transgenic plants are included pat/bar, cp4 epsps, dmo, hppd, aad-12, and csr1-2, which confer resistance to the herbicides glufosinate-ammonium, glyphosate, dicamba, inhibitors of the HPPD enzyme, 2,4-D, and imidazolinones, respectively (Supplementary File S1; ISAAA, 2023). Similarly, transgenic plants overexpressing different Cry or VIP3A genes isolated from Bacillus thuringiensis that confer resistance to insect pests have also been successfully generated for different major crops. The main insect pests targeted by these technologies are caterpillars (e.g., Spodoptera frugiperda, Helicoverpa armigera, and Diabrotica virgifera virgifera) in different crops (ISAAA, 2023). New versions of previously consolidated Cry or VIP3A proteins, as well as new molecules, have recently been released to ensure greater control efficacy and reduce the rate of resistance development of these technologies (Supplementary File S1; ISAAA, 2023). Also, the search for active molecules that have an effect against other insect pest orders is also ongoing, such as molecules for transgenic control of CBW in cotton (Ribeiro et al., 2017, 2019, 2022; Vasquez et al., 2023). The main crops using these transgenic-based technologies in Brazil are soybean, maize, and cotton, with recent highlights also for eucalyptus, sugarcane, wheat, and common bean (Figure 2A). In addition to transgenic plants with resistance to herbicides and insect pests, transgenic traits associated with resistance/tolerance to biotic and abiotic stresses are currently being focused on. Unfortunately, the many barriers to technologies using transgenic plants have made the introduction of new traits slow and costly worldwide. Obviously, the use of these technologies can guarantee an increase in the production and yield of crops, less need for expansion of cultivated areas, and a reduction in the use of insecticides and fungicides. Similar to other countries, Brazil has the CTNBio (National Technical Commission on Biosafety), under the Ministry of Science and Technology, as a competent institution to evaluate and decide whether or not to approve transgenic plants in the country based on scientific information, through the Biosafety Law No. 11.105 of March 24, 2005. In particular, it is worth highlighting the remarkable contributions made by multinational and national companies in the field of plant biotechnology to achieve this outstanding scenario in Brazilian agriculture.
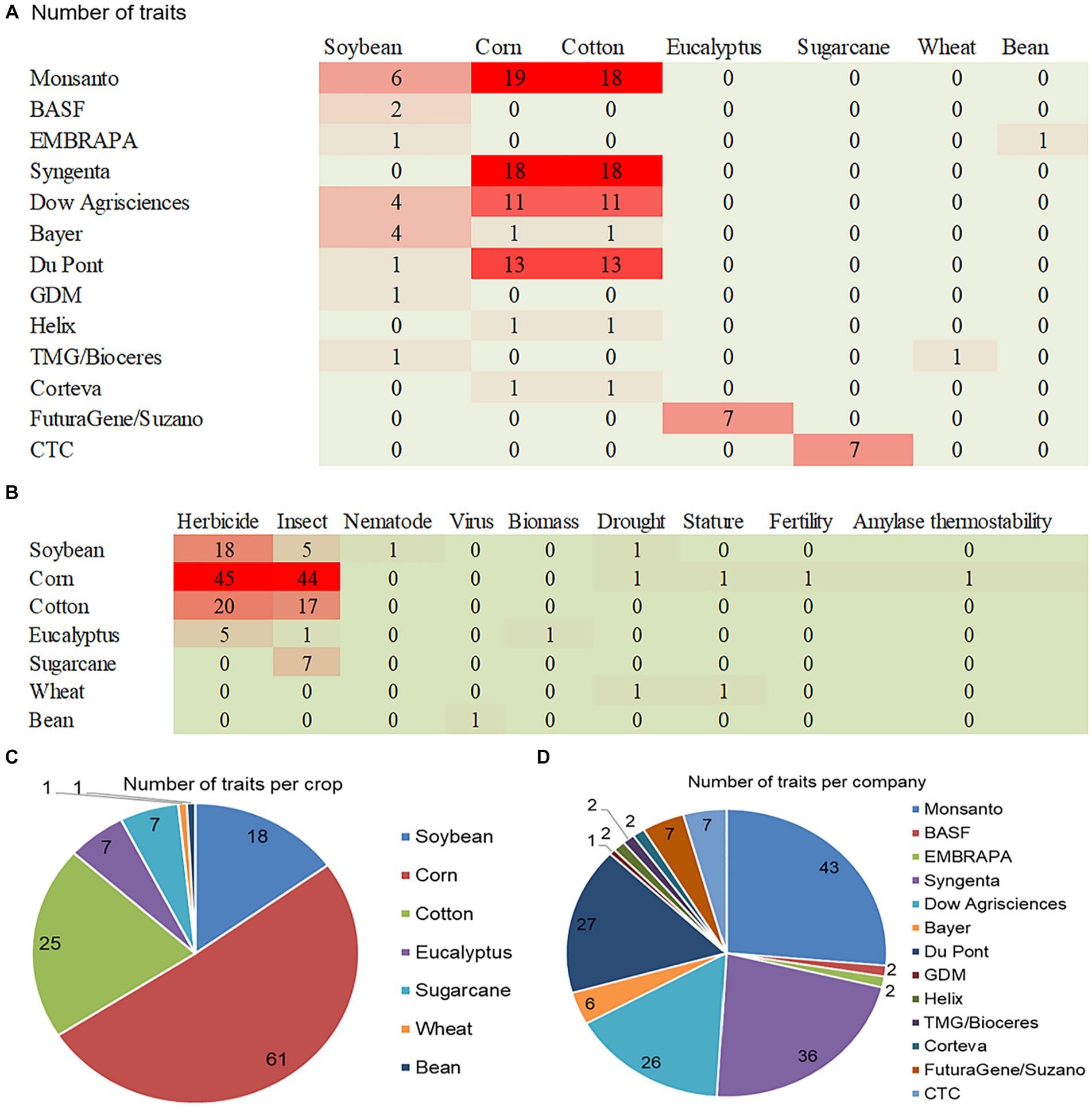
Figure 2. Number of genetically modified plants and agronomic traits approved by the National Technical Biosafety Commission (CTNBio; Brazil) for commercial use in Brazil. (A) Number of transgenic traits per crop and per company. Some transgenic traits are owned by more than one company cited. (B) Number of traits per crop. (C) Total number of traits per crop. (D) Total number of traits per company. Data source: CTNBio; Brazil. Reference: November 2023 (Supplementary File S1). CTC, Centro de Tecnologia Canavieira; TMG, Tropical Melhoramento & Genética. Dow AgroSciences and DuPont merged to originate Corteva. Bayer merged with Monsanto. TMG and Bioceres Crop Solutions are independent companies that own the HB4 technology in soybean and wheat in Brazil. FuturaGene has been a wholly-owned subsidiary of Suzano of Brazil.
In addition to technologies using transgenics, genome editing has gained prominence in the development of new traits of agronomic interest through gene knockdown, not only in soybeans and sugarcane in Brazil. Although there are no commercially released cultivars generated by this genome editing technology, some applications for evaluation and approval are already being processed at CTNBio. Among the possibilities of CRISPR/Cas9 genome editing technology, classified by CTNBio as SDN1 to SDN3 (Site-Directed Nucleases), from turning off target genes to modifying entire genetic regions, SDN1 in particular makes it possible to obtain a trait or cultivar with a gene turned off without containing a transgene (Basso et al., 2021). In this way, the traits generated by the SDN1 strategy allow greater regulatory flexibility when compared to SDN2 and SDN3, as they are similar to a classical transgenic. Similarly to the regulation of transgenic plants, CTNBio has the competence to assess, on a case-by-case basis, plants generated by genome editing, through Normative Resolution number 16 of 15 January 2018 (RN16). As mentioned above, the genome editing technology using the SDN1 strategy has its advantage in regulation but has minor limitations in the generation potential of other traits that do not require gene knockdown. In addition, given this advantage of SDN1 and the scientific information available in the literature to date, several genes are known whose knockout results in improved traits and are potential targets for genome editing in important crops. Thus, alongside classical plant breeding, transgenics and genome editing remain important biotechnological tools for developing new traits in crops worldwide.
On the other hand, the topical application of RNAi is also considered a disruptive technology for Brazilian agriculture with a large market potential (Távora et al., 2022). Although no RNAi-based products have yet been commercialized for agricultural use in Brazil, several initiatives and research are underway by public and private companies to control weeds, insect pests, and diseases in the main agricultural crops. The current main bottlenecks of topical RNAi technology in Brazil are the identification of powerful target genes (lethality genes) for regulation in the target organism (either in weeds, pathogens, or insect pests), the high cost of producing intact RNAi molecules, and the stability of these molecules when applied under field conditions. By overcoming these three major bottlenecks, RNAi technology for topical application is expected to make a significant contribution to national agriculture, particularly by reducing reliance on synthetic chemicals that are not very selective. To date, significant progress has been made with the generation of genomic and transcriptomic data from target organisms and laboratory tests to validate genes with lethality by RNAi in planta or microinjection. Similarly, by reducing the cost of RNAi production, it is expected that the dose–response rate and thus the efficacy will increase. The use of carrier nanoparticles and RNAi-stabilizing agents has shown significant advances (Vasquez et al., 2023). Furthermore, the regulation of this topical RNAi technology in Brazil is still being reformulated and discussed by CTNBio.
Similar to topical RNAi technology, several genomic, taxonomy, interactomic, and microbiological studies have allowed the identification of different macro-, micro-organisms, and semiochemical and biochemical molecules with beneficial effects for plants or with inhibitory effects on undesirable organisms (Rocha et al., 2017). To date, 616 biological products have been registered for commercial use in Brazil, with an emphasis on products based on microorganisms which reaches 65% (Figure 3A). Furthermore, it is worth mentioning that the use of these biological products in the main crops already reaches up to 42% of the cultivated area per crop (Figure 3B). In particular, the largest number of biopesticides registered in Brazil target nematodes, Dalbulus maidis, caterpillars, and Euschistus heros indicating the high diversity of products currently used (Figure 3C). Biological products containing transgenics or with pyramiding of insecticidal genes have not yet been commercially approved by CTNBio for biological control in Brazil.
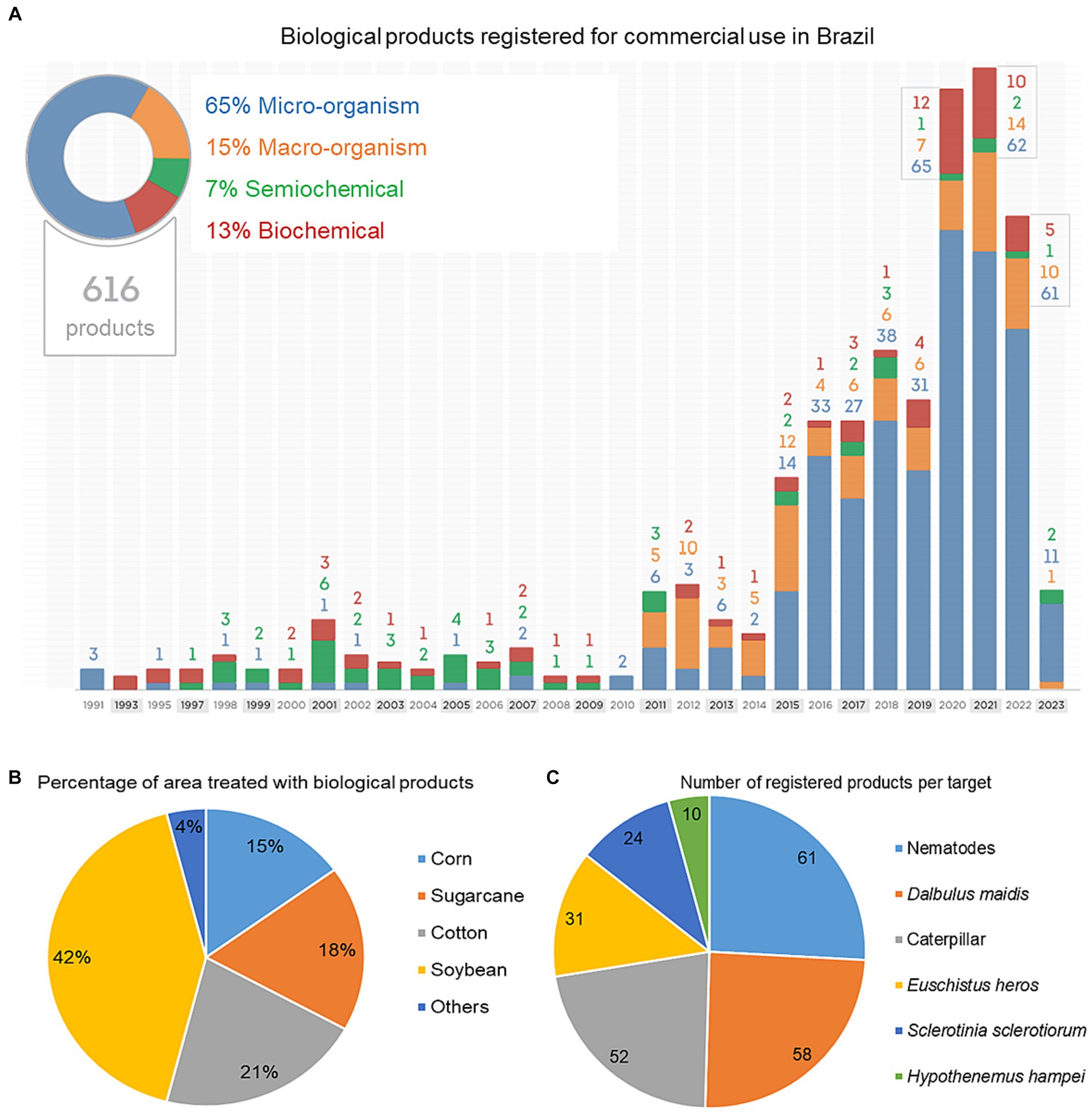
Figure 3. Market for biological products in Brazil. (A) Number of biological products registered in Brazil over the years. (B) Agricultural areas treated with biodefensives. (C) Number of products registered by target organism. Data source: Ministry of Agriculture, Livestock and Food Supply (MAPA; Brazil) and CropLife Brazil, November 2023.
In this context, basic research in molecular sciences has outstanding importance in the national scenario, supporting these breeding programs and the genetic engineering of plants, as well as RNAi technologies and biological products (Reis et al., 2023). The transfer of technology generated by this useful basic research to the productive sector is still the main bottleneck in Brazil. However, it is expected that the consolidation of new biotechnology companies and start-ups will tend to contribute to improving the transfer of knowledge into products in the agribusiness market.
6 The way forward: growing sustainably to meet high demand
It is also important to highlight that global climate change is becoming more evident and intense, with negative impacts on agriculture (Howden et al., 2007). In this context, the balance and sustainability of ecosystems have become increasingly important worldwide (Tillman et al., 2011). The recent upsurge of the ESG (Environmental, Social, and Corporate Governance) concept has organized a new path based on environmental sustainability to be followed by agriculture, as well as agribusiness worldwide (Pinheiro, 2023). These good agricultural and commercial practices are already directly reflected in the increased use of bio-inputs (natural and biological products) in agriculture and the reduction of dependence on synthetic agrochemicals. In this regard, the greater use of symbiotic microorganisms that promote better plant growth and yields by increasing the availability of nutrients, such as nitrogen and phosphorus, and improving the rhizosphere has shown important results (Gomes et al., 2018; Silva et al., 2021a; Thiebaut et al., 2022). In addition, the use of antagonistic microorganisms or those that provide biological control of pathogens and insect pests are some examples of this new direction of sustainable agriculture (Costa et al., 2019; Ribichich et al., 2020; Moreira-Pinto et al., 2021; Kumar et al., 2022; Nakei et al., 2022). This set of measures and technologies already reflects on the best use of the world’s agricultural system, and puts any country dependent on agriculture or agribusiness on alert for environmental sustainability (Martha Junior and Lopes, 2023). In this context, it should be noted that Brazil is an example of environmental sustainability in the agribusiness sector. Currently, Brazil uses only 32.6% of its territory for agriculture (24% native and cultivated pasture, 2.5% soybean, 1.5% maize, 1.1% forestry, 0.7% sugarcane, 0.3% coffee, and 2.5% other crops), 64% for protected and conserved vegetation (native vegetation, indigenous lands, integral conservation units, and conservation of rural vegetation), and 3.4% for other uses such as infrastructure (Figure 4A). Apart from the fact that more than 45% of Brazil’s energy comes from renewable sources and almost 90% of the electricity generated is used in the country. Also, it is also worth noting that Brazil has been a pioneer in biofuels research and is now one of the world’s top two producers, focusing on bioethanol from sugarcane and biodiesel and HVO from processed seeds (Aizarani, 2023). In addition, Brazil has adopted the Low Carbon Emission Agriculture Program (ABC Program), which integrates actions by the government, the productive sector, and civil society to reduce carbon gas emissions from agricultural and livestock activities (MAPA, 2023). For example, the Low Carbon Soybean Program (PSBC), led by Embrapa Soybean, is developing a protocol to certify soybean production areas with low carbon gas emissions, which will allow the recognition of rural properties with sustainable production. Brazil has also adopted a strong and restrictive environmental code for farmers and other agribusiness sectors and has one of the lowest per capita carbon emissions in the world among the most industrialized countries.
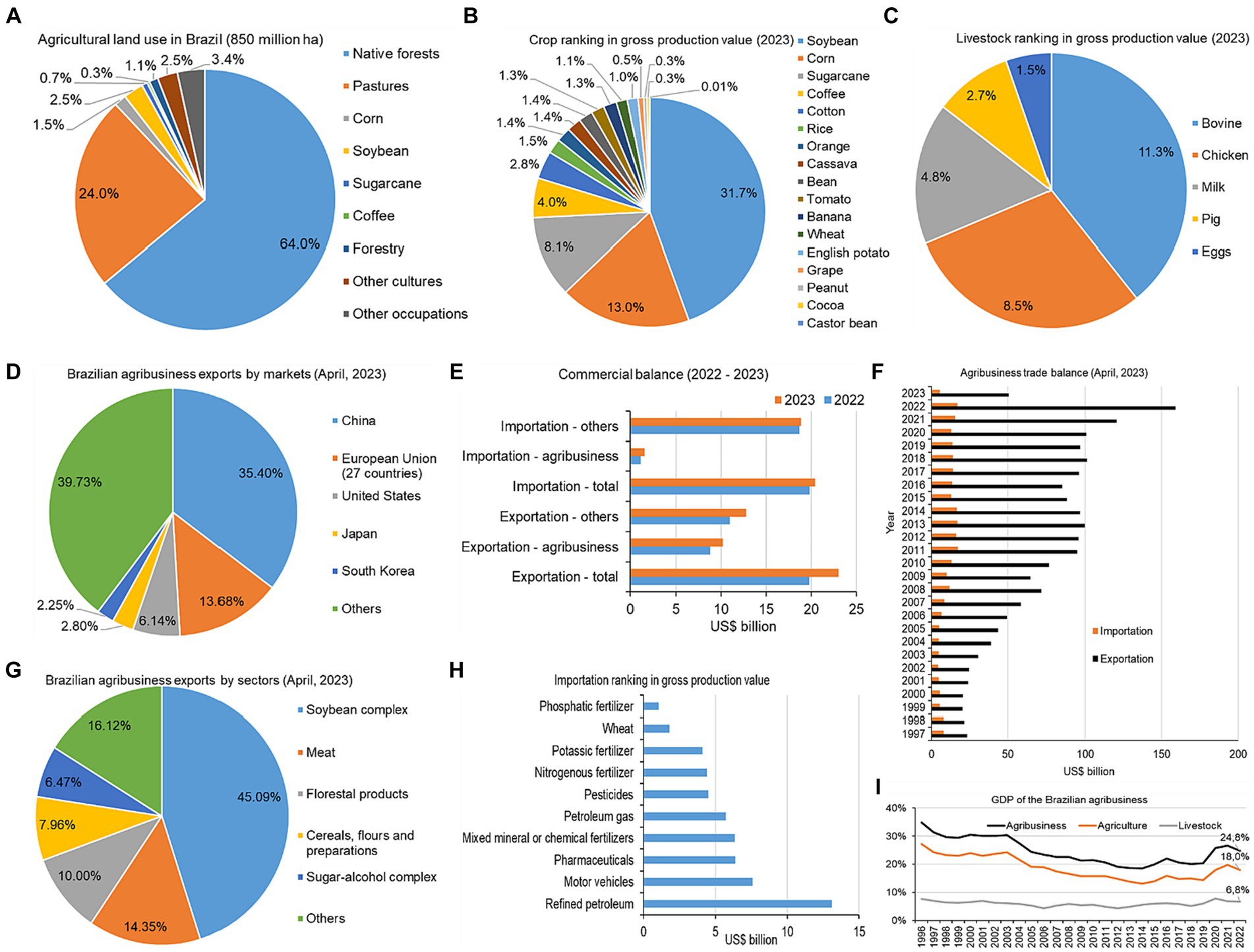
Figure 4. Importance of Brazilian agribusiness, agriculture, and livestock to the domestic and external foreign economies. (A) Agricultural land use in Brazil. Data source: Brazilian Institute of Geography and Statistics (IBGE, 2023) and MAPA. (B) Ranking of crops in terms of gross production value in 2023. Data source: IBGE (2023) and MAPA. (C) Livestock ranking in terms of gross production value in 2023. Data source: IBGE (2023) and MAPA. (D) Brazilian agribusiness exports by market. Data source: IBGE (2023) and MAPA. (E) Trade balance between imports and exports and the agribusiness contributions for the years 2022 and 2023. Data source: Comex Stat/SECINT elaborated by the Institute for Applied Economic Research (IPEA; Brazil). (F) Agribusiness trade balance between imports and exports from 1997 to 2023. Data source: IBGE (2023) and MAPA. (G) Brazilian agribusiness exports by sector. Data source: Observatory of Economic Complexity (OEC). (H) Ranking of imports by gross production value. Data source: OEC. (I) Gross Domestic Product (GDP) of the Brazilian agribusiness, agriculture, and livestock from 1996 to 2022 (CEPA, 2023).
Another important example of the initiative to support national agribusiness is the National Institutes of Science and Technology (INCTs), funded by the Brazilian Federal Government. The INCTs have been fundamental in the articulation, aggregation, and promotion, at national and international levels, of the best scientific and technological research groups in cutting-edge science and strategic areas, in order to contribute to the sustainable development of the country. In the same context, 2023 will mark the 50th anniversary of Embrapa (Brazilian Agricultural Research Corporation), recognized for its decisive role in the development of Brazilian agribusiness through scientific research and the development of solutions.
This set of actions of public and private sector measures to support Brazilian agribusiness has contributed significantly to the development of the national and international economy. In particular, the soybean, maize, and sugarcane (Figure 4B; Table 1) and cattle poultry, dairy, and pig (Figure 4C; Table 2) stand out in terms of the gross production value of Brazilian agriculture and livestock. Furthermore, Brazilian agribusiness is not only important domestically but is also extremely relevant in supplying food and agribusiness products to other countries, as an outstanding example for Europe and Asia. Brazil’s main export markets are China, the European Union, the United States, and Japan (Figure 4D). The trade balance data show that Brazilian agribusiness is extremely important in the export market compared to the import market (Figure 4E), highlighting the year 2022 as an export record in terms of US$ values (Figure 4F). Soybeans and their derivatives, meat, forest products, cereals, flour and preparations, and sugar-alcohol complex are the main export sectors (Figure 4G). By the end of 2023, Brazil is expected to lead international trade in 9 agribusiness chains, according to USDA and FAO data. The leading one is orange juice, where the country accounts for almost 80% of world trade, followed by soybeans with about 55%, sugar with 45%, poultry meat with 33%, maize with 31%, coffee with about 28%, and beef meat with almost 25%. With smaller shares, but also leading in international trade, are tobacco and cellulose, and cotton, which has almost overtaken the United States to become the world’s largest supplier.
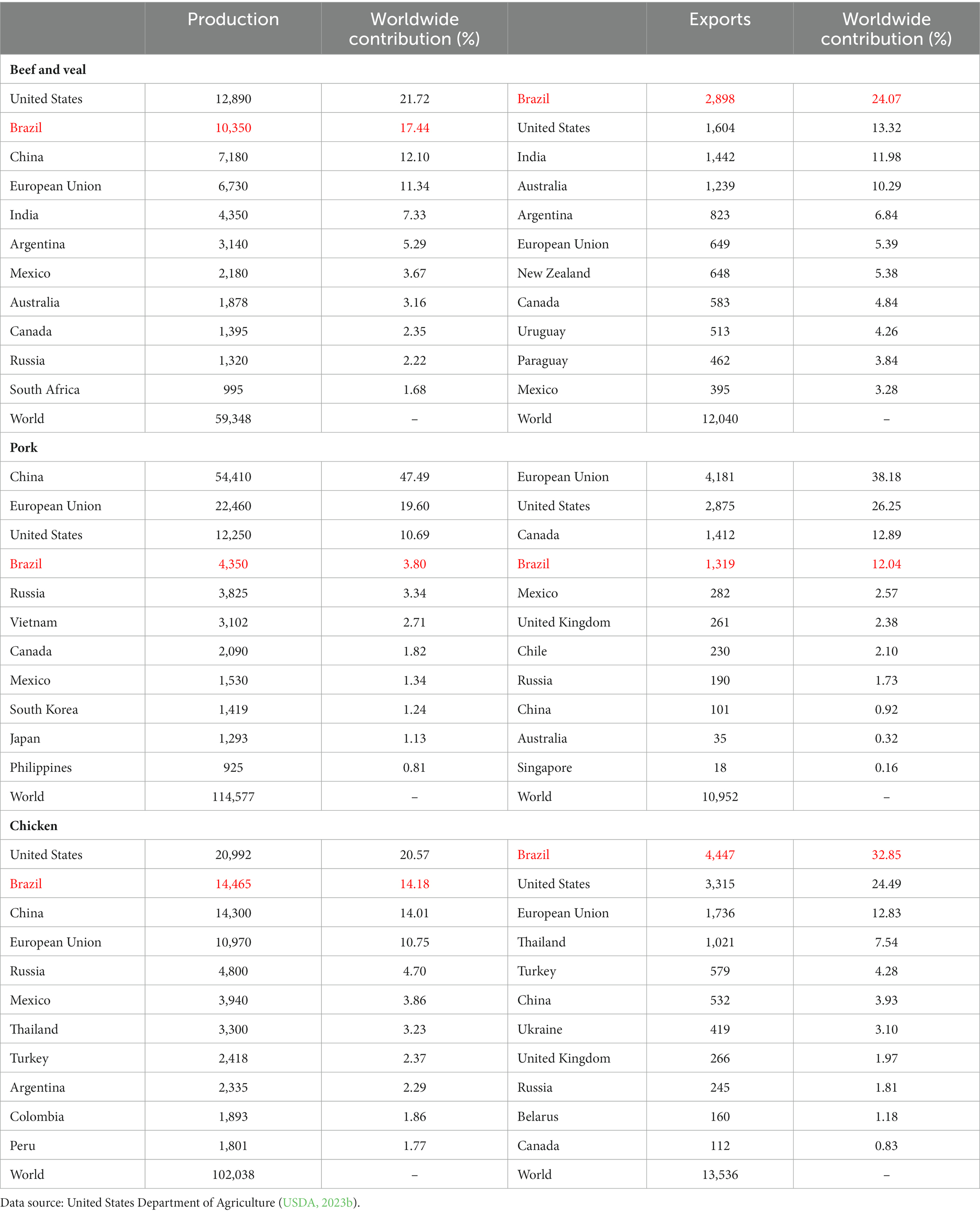
Table 2. World rankings of livestock and poultry (beef and veal, pork, and chicken meat) production and exports (in 1.000 tonnes) for the year 2022.
Meanwhile, fertilizers (isolated or mixed), pesticides, and wheat are the main products in demand for imports directly related to the Brazilian agricultural sector (Figure 4H). In this way, Brazilian agriculture, livestock, and agribusiness as a whole will contribute 18, 6.8, and 24.8%, respectively, to the country’s GDP in 2023 (Figure 4I). Over the past four years, Brazil has added nearly 15 million hectares of new cereal land, much of it converted from degraded pastures, and produced more than 60 million tonnes, helping to reduce hunger. If it were not for Brazil’s performance, the FAO’s World Food Price Index would be much worse.
Allied with these recent advances in sustainable agriculture, seed treatment using a combination of technologies has become increasingly important. Among these technologies are examples of mixing bio-inputs for the biocontrol of insect pests and pathogens, molecules that promote plant growth, macro and micronutrients, and elicitor molecules to boost the plant’s immune system (Cardarelli et al., 2022). Together, these technologies collectively have contributed to the emergence of a new generation of seed treatments. In addition to this universe of new-generation seeds, the use of nanotechnologies combined with RNAi technologies, through topical application spraying bioprotected RNAi molecules that are active only in the target organism, is revolutionizing a new area of agriculture worldwide (Ribeiro et al., 2022; Távora et al., 2022; Vasquez et al., 2023). However, regulatory issues for the commercial use of topical RNAi technologies still need to be addressed on a global level, especially in Brazil (Dietz-Pfeilstetter et al., 2021). Particularly, regarding nanotechnologies for agriculture, the development of nanoparticles or carrier nanostructures has shown their potential for use in the protection, better internalization, and greater efficacy of encapsulated biomolecules (DNA, RNA, proteins, and elicitors) (Vasquez et al., 2023). This new frontier of knowledge aims to develop alternative technologies to agrochemicals for the highly specific control of weeds, insect pests, and phytopathogens. For example, Brazilian agriculture records annual losses of more than 15% due to pathogens (e.g., nematodes; Lopes-Caitar et al., 2019), insect pests (e.g., CBW; Oliveira et al., 2012; IMEA, 2023), and weeds (e.g., Amaranthus palmeri; Cruz et al., 2020), which can be minimized by using these ecologically sustainable technologies.
All these new disruptive technologies and their evolution have opened the possibility for the emergence of new players in innovation, technology transfer, startups, and portfolios in agribusiness worldwide, by democratizing access to markets (Martha Junior and Lopes, 2023; Silva et al., 2023). The increased supply of innovative solutions, services, and technologies has contributed significantly to the increased implementation and use of these technological assets in agribusiness. Obviously, the valorization by the Brazilian agribusiness sector of the technologies and assets generated by the national public or private initiative will be of great importance for a greater financial return from agriculture and the strengthening of research and development in the country. Evidently, greater incentives for non-predatory basic and applied scientific research are extremely important for any country to be at the forefront of developing or following new technologies and implementing them in agriculture. Similarly, greater financial support and opportunities for the education system are extremely important for the good training of human and professional resources in all areas, with a particular focus on agribusiness. In addition to this essential support for the education, research, and development sector, other sectors related to agribusiness need initiatives to support their development. An example of this is the fertilizer sector, which has been affected by the war between Russia and Ukraine (and the impending tensions between China, Taiwan, and the United States, or the Middle East war) and their impact on foreign trade and public and private embargoes. This eminent instability has alerted Brazilian agribusiness to the high risk of dependence on imported fertilizer from countries in geopolitical conflict. In this context, government support for initiatives to increase local production and reduce Brazil’s dependence on imported fertilizers will be crucial for national agribusiness in the coming years. Currently, Brazilian agriculture ranks fourth in the world in terms of fertilizer dependence, behind China, India, and the United States (IFA, 2019). Brazilian self-sufficiency in fertilizers is expected to be one of the most important milestones for the stability of a country where agribusiness is the main activity driving the economy, a factor that could define the fifth Agricultural Revolution in Brazil. This Fifth Agricultural Revolution, as well as the Sixth to Eighth Agricultural Revolutions, cited here in sequence, are optimistic forecasts for the next 200 years of Brazilian agriculture, based on the demand and benefits for national agribusiness, which can have an impact on self-sufficient food production. Brazil is also highly dependent on the imports of active ingredients and chemicals, mainly from China and India.
Likewise, more intensive and effective efforts by public and private initiatives in terms of logistics for the transport and distribution of agricultural production, through the development, expansion, and improvement of the rail network and port infrastructure, are important to improve the dynamics and reduce the costs of Brazilian logistics. Among countries with a strong agricultural sector, Brazil has the lowest rail density (km of rail per km2 of surface area), compared to the three major rail powers, the United States, India, and Canada. Similarly, investments to improve the infrastructure for the storage of agricultural products, on farms, in cooperatives, traders, trading companies and other stakeholders are necessary and could bring more stability to the Brazilian agribusiness sector. The implementation of an extensive rail network linking all Brazilian states and ports, along with improvements in the storage infrastructure, will bring major changes to the agribusiness sector and could herald Brazil’s Sixth Agricultural Revolution. These improvements in the logistics of agricultural products could bring direct benefits to those involved in both exporters and importers. The main benefits of these outstanding initiatives are greater stability in the production chain up to the importer or exporter of the products. It is worth noting that not only Brazil but also several other countries have had their economies severely affected by recent global events. These include the new geopolitical reconfiguration and its impacts, trade wars (China, Russia, the United States, and the European Union), political conflicts between democracies and autocracies, unprecedented private boycotts of countries in conflict, and crises in international logistics (strikes, maritime accidents, disruptions, geopolitical conflicts, container shortages, and price increases). It is also important to mention that any instability in the countries that are trade hubs (top 4: United States, China, Japan, and Germany), which move more than 50% of the global trade flow, the resulting negative effects are spread or networked to other partner countries. Among the many consequences, it is important to highlight the wide fluctuations in prices in general (supply and demand imbalances, product shortages, inflation, and exchange rate volatility) also need to be highlighted. Furthermore, the impact of pandemics on human health (e.g., COVID-19, Monkeypox, Marburg virus, and Ebola virus diseases) and animal health (e.g., African swine fever in China, avian influenza, and bovine spongiform encephalopathy), extreme weather events (e.g., frequent droughts and severe frosts in unfavorable seasons, excessive rainfall, and El Niño and La Niña phenomena), and the intensification of insect pests and plant diseases (increasing production costs) reinforce the importance of immediate action to mitigate risks. In addition, another relevant issue to consider is the increase in labor costs and the shortage of skilled labor in the agribusiness sector. In a different way, but also of great importance, are the changing habits of consumers in general influenced by online and offline social media, influencers, e-commerce, marketplaces, censorship of free speech, fake news in various means of communication, and activism for various causes. To separate the good part of all this, these claimed actions generally aim to improve the quality of life in general, the production of healthy food, and the preservation of the environment. In this context of environmental sustainability, the long-term adoption of food production systems without agrochemicals, or at least without synthetic or non-selective pesticides, could lead to the seventh Agricultural Revolution in Brazil. However, it is important to note that current agricultural production worldwide depends on agrochemicals to provide the necessary food for the population. Brazilian agriculture ranks ninth in the world in terms of relative pesticide use (kg of active ingredient per hectare), led by Netherlands, Belgium, Italy, and Montenegro. On the positive side, the adoption of biological products, biotechnologies, and sustainable systems will help to reduce this dependence on chemicals in world agriculture.
All these disruptive technologies and advances in different segments of global agribusiness could encourage a return of part of the population from urban centers to the agricultural environment, leading to what could be Brazil’s Eighth Agricultural Revolution. It is evident that the world’s population is essentially concentrated in urban areas and increasingly dependent on a few rural producers for food production. So, this Eighth Revolution could therefore be characterized by the exodus from the cities, the appreciation of the small producers, the diversification of agriculture (polyculture), and the use of agriculture as a sustainable family business and provider of a healthier life (Ioris, 2018). Finally, the constant generation of scientific knowledge, technological innovation, technology transfer to the agricultural sector, dynamic and assertive communication, and the sustainability of ecosystems will be crucial for global agriculture and for improving the quality of life of people at all levels of society. In the same sense, the strengthening of a long-term thinking mentality, with more investments in priority areas and the export of industrialized products, with a higher added value, investing in improvements for the excellence of national products (quality, taste, safety), will be important for the socio-economic development of Brazil and other dependent countries on agribusiness. These short-, medium- and long-term government initiatives, without politicizing or damaging agribusiness, and with the harmony between the public and private sectors and between urban and rural populations, all working towards a common goal, will certainly make it possible to increase food production. In addition to this increase in food production, these initiatives will also allow for better use of cultivated land (producing more in a smaller area), with fewer resources invested (cost–benefit ratio), and will keep Brazilian agribusiness among the four major world powers in food production, as a major supplier of food to the world, and as a reference in environmental and social sustainability, contributing to reducing food costs and hunger, and consequently contributing to peace.
Brazil also plays an important role in biofuels and bioelectricity, and these markets have a promising future on the planet. The blending of biodiesel to diesel in the country is increasing and will reach 20%, the addition of ethanol to petrol is 27.5% and has a government plan to reach 30%, and also several investments in ethanol from corn, biogas, biomethane, and other renewable energy sources make the domestic market for bioenergy very promising. The international market for bioenergy is also very demanding, with most countries in the world adopting regulations to increase the proportion of renewable energy in the fossil fuels they use (Majeed et al., 2023). The most important examples are ethanol (growing in India, Thailand, France, South Africa, and several other countries), biodiesel (new targets coming in Indonesia, Europe, and others), renewable diesel from soybeans and other sources (now strongly demanded in the United States), jet fuel, which represents a new and large market, renewable fuel for shipping, and other needs that require developments in agriculture.
7 Conclusion remarks and management implications
It is important to emphasize the importance of agriculture and the agribusiness sector in increasing the production of healthy food with better nutritional quality for the entire world (Neves, 2020). Furthermore, it is undeniable that agricultural practices, as well as urban planning activities, need to mitigate any negative impact on the environment and, consequently, on human life (Smith et al., 2023). Technological advances on the different fronts of agriculture have been evident over the years, but global climate change is the main challenge currently facing this sector (Das et al., 2023). Overall, some linear climate changes can improve particular agronomic traits, while other non-linear climate changes are and could severely impact even more crop yield and system, food production, human life, and the ecosystem balance (Rezaei et al., 2023). Therefore, the adoption of eco-friendly technologies in global agriculture will be a necessary and irreversible process for sustainable food production (Neves et al., 2023). Fortunately, there is an estimate that these disruptives and eco-sustainable technologies will allow linear increased crop yields, despite adverse climate conditions (Munaweera et al., 2022). In addition to increasing food production, there is a booming market for bioenergy as part of the challenge to move to renewable energy (Majeed et al., 2023). In this context, renewable fuel blending programs in several parts of the world increasing markets for bioethanol, biodiesel, biohydrogen, renewable diesel, renewable jet fuel, bioplastics, biomass for power generation, and various other sources (Santos et al., 2023). In the same way, this growth in the agro-bioenergy sector must be accompanied by the need to consider and reduce the impact on the environment, under the imperative of sustainability (Nair et al., 2022).
In particular, the generation of scientific knowledge by public or private institutions and the transfer of these technological assets to the agribusiness productive sector will be of importance to achieve self-sufficiency in the production of healthy food (Reis et al., 2023). Therefore, encouraging innovation and the emergence of new technological players will allow these new disruptive technologies to reach the production sector with better conditions for their implementation (Silva et al., 2023). Also, it is undeniable that the innovations briefly described in this review study and not yet commercially applied in agriculture could bring major transformations in socioeconomic and environmental terms and for food production (Martha Junior and Lopes, 2023). On the other hand, it is also important that this sector is valued and strengthened by initiatives from the consumer citizens to politicians, in order to improve the quality and stability of life for everyone, from all social classes, linked or not to the agribusiness sector (Grossi-de-Sa and Basso, 2024). In particular, it is also necessary to provide support to family farming, smallholder producers, and subsistence agricultural practices (Ioris, 2018). Finally, it should be noted that Brazilian agribusiness is not only extremely important for the socioeconomic stability of the country but also the main supplier of food and raw materials to several other countries on different continents (Bolfe et al., 2023). In addition to consolidated technologies and sectors, it also has great potential for expanding the production of other crops (e.g., sesame, castor bean, sunflower, fruits, legumes, and vegetables), hardwoods, and freshwater fish (e.g., tilapia, curimbata, and tambaqui) (Greschuk et al., 2023). In terms of management implications, it is imperative that public and private policies and strategies focus on research and development to achieve innovations that will allow this growth in global demand to be addressed in a sustainable way, each time using fewer resources to produce more (Neves et al., 2023). Also, creative ideas should be developed to promote the integration of public and private initiatives to achieve this goal. Therefore, this review study brought to the discussion advances, bottlenecks, and opportunities in Brazilian and global agriculture, and highlights the contribution of technologies for food production and the need for eco-sustainable agricultural practices.
Author contributions
MB: Conceptualization, Writing – original draft. MN: Conceptualization, Writing – review & editing. MG-d-S: Conceptualization, Funding acquisition, Writing – review & editing.
Funding
The author(s) declare financial support was received for the research, authorship, and/or publication of this article. This work was supported by grants from CAPES, CNPq, FAP-DF, ABRAPA, and INCT Plant Stress Biotech.
Acknowledgments
MB is grateful to CNPq for a postdoctoral research fellowship (process number: 106655/2023-0).
Conflict of interest
The authors declare that the research was conducted in the absence of any commercial or financial relationship that could be construed as a potential conflict of interest.
Publisher’s note
All claims expressed in this article are solely those of the authors and do not necessarily represent those of their affiliated organizations, or those of the publisher, the editors and the reviewers. Any product that may be evaluated in this article, or claim that may be made by its manufacturer, is not guaranteed or endorsed by the publisher.
Supplementary material
The Supplementary material for this article can be found online at: https://www.frontiersin.org/articles/10.3389/fsufs.2023.1296337/full#supplementary-material
Footnotes
1. ^ http://ctnbio.mctic.gov.br/liberacao-comercial
2. ^ https://www.gov.br/agricultura/pt-br/assuntos/inovacao/bioinsumos
3. ^ https://croplifebrasil.org/produtos-biologicos/
4. ^ https://www.ibge.gov.br/estatisticas/economicas/agricultura-e-pecuaria
5. ^ https://www.gov.br/agricultura/pt-br/assuntos/sustentabilidade
6. ^ http://comexstat.mdic.gov.br/pt/home
7. ^ https://oec.world/en/profile/country/bra
8. ^ https://www.cepea.esalq.usp.br/en/brazilian-agribusiness-gdp.aspx
9. ^ https://apps.fas.usda.gov/psdonline/circulars/production.pdf
10. ^ https://unicadata.com.br/?&idioma=2
11. ^ https://apps.fas.usda.gov/psdonline/circulars/livestock_poultry.pdf
References
Ainsworth, E. A., and Long, S. P. (2005). What have we learned from 15 years of free-air CO2 enrichment (FACE)? A meta-analytic review of the responses of photosynthesis, canopy properties and plant production to rising CO2. New Phytol. 165, 351–372. doi: 10.1111/j.1469-8137.2004.01224.x
Aizarani, J. (2023) Ethanol fuel production in top countries 2022. Available at: https://www.statista.com/statistics/281606/ethanol-production-in-selected-countries/ (Accessed November 2023)
Akmal, F., Khan, M. A., Sharif, M., Aurangzeb, K., and Javed, M. Y. (2020) Advanced machine learning algorithm based system for crops leaf diseases recognition. Sixth Conference on Data Science and Machine Learning Applications (CDMA), IEEE Xplore, pp. 146–151.
Alves de Oliveira, B. F., Bottino, M. J., Nobre, P., and Nobre, C. A. (2021). Deforestation and climate change are projected to increase heat stress risk in the Brazilian Amazon. Commun. Earth Environ. 2:207. doi: 10.1038/s43247-021-00275-8
Aragão, F. J., Nogueira, E. O., Tinoco, M. L., and Faria, J. C. (2013). Molecular characterization of the first commercial transgenic common bean immune to the bean golden mosaic virus. J. Biotechnol. 166, 42–50. doi: 10.1016/j.jbiotec.2013.04.009
Basso, M. F., Arraes, F. B. M., Grossi-de-Sa, M., Moreira, V. J. V., Alves-Ferreira, M., and Grossi-de-Sa, M. F. (2020). Insights into genetic and molecular elements for transgenic crop development. Front. Plant Sci. 11:509. doi: 10.3389/fpls.2020.00509
Basso, M. F., Duarte, K. E., Santiago, T. R., de Souza, W. R., Garcia, B. O., da Cunha, B. D. B., et al. (2021). Efficient genome editing and gene knockout in Setaria viridis with CRISPR/Cas9 directed gene editing by the non-homologous end-joining pathway. Plant Biotechnol. 38, 227–238. doi: 10.5511/plantbiotechnology.21.0407a
Basso, M. F., Ferreira, P. C. G., Kobayashi, A. K., Harmon, F. G., Nepomuceno, A. L., Molinari, H. B. C., et al. (2019). MicroRNAs and new biotechnological tools for its modulation and improving stress tolerance in plants. Plant Biotechnol. J. 17, 1482–1500. doi: 10.1111/pbi.13116
Bharadiya, J. P., Tzenios, N., and Reddy, M. (2023). Forecasting of crop yield using remote sensing data, agrarian factors and machine learning approaches. J. Eng. Res. Rep. 24, 29–44. doi: 10.9734/jerr/2023/v24i12858
Bibi, F., and Rahman, A. (2023). An overview of climate change impacts on agriculture and their mitigation strategies. Agriculture 13:1508. doi: 10.3390/agriculture13081508
Bolfe, E. L., Barbedo, J. G. A., Massruhá, S. M. F. S., de Souza, K. X. S., and Assad, A. D. (2023). “Challenges, trends and opportunities in digital agriculture in Brazil” in Digital agriculture: research, development and innovation in production chains. eds. M. SMFS, L. MAA, O. SRM, A. J. Luchiari, and E. L. Bolfe (Brasília: Embrapa Agricultura Digital; Embrapa Unidades Centrais), 281–299.
Bourke, P. M. A. (1964). Emergence of potato blight, 1843-1846. Nature 203, 805–808. doi: 10.1038/203805a0
Cardarelli, M., Woo, S. L., Rouphael, Y., and Colla, G. (2022). Seed treatments with microorganisms can have a biostimulant effect by influencing germination and seedling growth of crops. Plan. Theory 11:259. doi: 10.3390/plants11030259
CEPEA (2023) Brazilian Agribusiness GDP. Available at: https://www.cepea.esalq.usp.br/en/brazilian-agribusiness-gdp.aspx (Accessed November 2023).
Chand, S., Power, S., Walsh, K., Holbrook, N., McInnes, K., Tory, K., et al. (2023). Climate processes and drivers in the Pacific and global warming: a review for informing Pacific planning agencies. Clim. Chang. 176:5. doi: 10.1007/s10584-022-03467-z
ClimateWatch (2023) Historical GHG emissions. Available at: https://www.climatewatchdata.org/ghg-emissions?end_year=2020&gases=all-ghg®ions=WORLD&start_year=1990 (Accessed December 2023)
Costa, V. H. D., Soares, M. A., Dimate, F. A. R., Mendes de Sá, V. G., Zanuncio, J. C., and Valicente, F. H. (2019). Genetic identification and biological characterization of baculovirus isolated from Helicoverpa armigeonra (Lepidoptera: Noctuidae) in Brazil. Fla. Entomol. 102, 59–64. doi: 10.1653/024.102.0109
CropLife Brasil (2023) Produtos biológicos. Available at: https://croplifebrasil.org/produtos-biologicos/ (Accessed November 2023)
Cruz, R. A., Oliveira, G. M., Carvalho, L. B., and da Silva, M. F. G. F. (2020). “Herbicide resistance in Brazil: status, impacts, and future challenges” in Pests, weeds and diseases in agricultural crop and animal husbandry production. eds. D. Kontogiannatos, A. Kourti, and K. F. Mendes (Rijeka: IntechOpen)
CTNBio (2023) Comissão Técnica Nacional de Biossegurança. Available at: http://ctnbio.mctic.gov.br/ and http://ctnbio.mctic.gov.br/documents/566529/1684467/Tabela+de+Plantas+Aprovadas+para+Comercializa%C3%A7%C3%A3o/e3087f9c-c719-476e-a9bd-bfe75def842f?version=1.21 (Accessed November 2023)
Das, S., Ray, M. K., Panday, D., and Mishra, P. K. (2023). Role of biotechnology in creating sustainable agriculture. PLOS Sustain. Transform. 2:e0000069. doi: 10.1371/journal.pstr.0000069
Diamond, J. (2002). Evolution, consequences and future of plant and animal domestication. Nature 418, 700–707. doi: 10.1038/nature01019
Dietz-Pfeilstetter, A., Mendelsohn, M., Gathmann, A., and Klinkenbuß, D. (2021). Considerations and regulatory approaches in the USA and in the EU for dsRNA-based externally applied pesticides for plant protection. Front. Plant Sci. 12:682387. doi: 10.3389/fpls.2021.682387
Elli, E. F., Ciampitti, I. A., Castellano, M. J., Purcell, L. C., Naeve, S., Grassini, P., et al. (2022). Climate change and management impacts on soybean N fixation, soil N mineralization, N2O emissions, and seed yield. Front. Plant Sci. 13:849896. doi: 10.3389/fpls.2022.849896
Falcon, W. P., Naylor, R. L., and Shankar, N. D. (2022). Rethinking global food demand for 2050. Popul. Dev. Rev. 48, 921–957. doi: 10.1111/padr.12508
Ferreira, P. C. G., Hemerly, A. S., and Carvalho, T. B. (2014) Method for promoting an exacerbated increase in vegetable biomass. Brazil Patent, WO2015127521A1.
Gao, H., Gadlage, M. J., Lafitte, H. R., Lenderts, B., Yang, M., Schroder, M., et al. (2020). Superior field performance of waxy corn engineered using CRISPR-Cas9. Nat. Biotechnol. 38, 579–581. doi: 10.1038/s41587-020-0444-0
Gomes, E. A., Lana, U. G. P., Quensen, J. F., Sousa, S. M., Oliveira, C. A., Guo, J., et al. (2018). Root-associated microbiome of maize genotypes with contrasting phosphorus use efficiency. Phytobiomes J. 2, 129–137. doi: 10.1094/PBIOMES-03-18-0012-R
Greschuk, L. T., Demattê, J. A. M., Silvero, N. E. Q., and Rosin, N. A. (2023). A soil productivity system reveals most Brazilian agricultural lands are below their maximum potential. Sci. Rep. 13:14103. doi: 10.1038/s41598-023-39981-y
Grossi-de-Sa, M. F., and Basso, M. F. (2024) Ciências agrárias e as revoluções na produção de alimentos: Do passado ao futuro. Capítulo 8, pág. 48–55. In. Hungria MH (2024) Segurança Alimentar e Nutricional: O Papel da Ciência Brasileira no Combate à Fome. Academia Brasileira de Ciências 170 pág.
He, L., and Rosa, L. (2023). Solutions to agricultural green water scarcity under climate change. PNAS Nexus 2:pgad117. doi: 10.1093/pnasnexus/pgad117
Hemerly, A. S., Ferreira, P. C. G., Gong, P., Nelissem, H., Inze, D., Grossi-de-Sa, M. F., et al. (2022) Method to increase plant biomass, plant yield and plant drought tolerance. Brazil Patent, WO2015127521 A1 and US11525143B2.
Howden, S. M., Soussana, J.-F., Tubiello, F. N., Chhetri, N., Dunlop, M., and Meinke, H. (2007). Adapting agriculture to climate change. PNAS 104, 19691–19696. doi: 10.1073/pnas.0701890104
Hu, H., Scheben, A., and Edwards, D. (2018). Advances in integrating genomics and bioinformatics in the plant breeding pipeline. Agriculture 8:75. doi: 10.3390/agriculture8060075
IBGE (2023) Censo Agropecuário. Available at: https://censoagro2017.ibge.gov.br/ and https://www.ibge.gov.br/estatisticas/economicas/agricultura-e-pecuaria/21814-2017-censo-agropecuario.html?utm_source=landing&utm_medium=explica&utm_campaign=producao_agropecuaria ().
IFA (2019) International Fertilizer Association. Executive Summary Fertilizer Outlook 2019-2023. Available at: https://api.ifastat.org/reports/download/12620 (Accessed November 2023).
IMEA (2023) Custo de Produção do Algodão, Safra 2022/23. Weekly Bulletin. Available at: https://www.imea.com.br/imea-site/relatoriosmercado (Accessed November 2023).
Ioris, A. A. R. (2018). The politics of agribusiness and the business of sustainability. Sustainability 10:1648. doi: 10.3390/su10051648
ISAAA (2023) GM crop events approved in Brazil. Available at: https://www.isaaa.org/gmapprovaldatabase/approvedeventsin/default.asp?CountryID=BR (Accessed April 2023).
Jinek, M., Chylinski, K., Fonfara, I., Hauer, M., Doudna, J. A., and Charpentier, E. (2012). A programmable dual-RNA-guided DNA endonuclease in adaptive bacterial immunity. Science 337, 816–821. doi: 10.1126/science.1225829
Kumar, S., Diksha, S. S. S., and Kumar, R. (2022). Biofertilizers: an ecofriendly technology for nutrient recycling and environmental sustainability. Curr. Res. Microb. Sci. 3:100094. doi: 10.1016/j.crmicr.2021.100094
Li, Y., Brando, P. M., Morton, D. C., Lawrence, D. M., Yang, H., and Randerson, J. T. (2022). Deforestation-induced climate change reduces carbon storage in remaining tropical forests. Nat. Commun. 13:1964. doi: 10.1038/s41467-022-29601-0
Lin, J., and Qian, T. (2019). Switch between El nino and La nina is caused by subsurface ocean waves likely driven by lunar tidal forcing. Sci. Rep. 9:13106. doi: 10.1038/s41598-019-49678-w
Liu, Y., Cai, W., Lin, X., Li, Z., and Zhang, Y. (2023). Nonlinear El Niño impacts on the global economy under climate change. Nat. Commun. 14:5887. doi: 10.1038/s41467-023-41551-9
Liu, Y., and Dai, L. (2020). Modelling the impacts of climate change and crop management measures on soybean phenology in China. J. Clean. Prod. 262:121271. doi: 10.1016/j.jclepro.2020.121271
Lopes-Caitar, V. S., Pinheiro, J. B., and Marcelino-Guimarães, F. C. (2019). Nematodes in horticulture: an overview. J. Hortic. Sci. Crop Res. 1:106. doi: 10.15744/2767-8709.1.105
Majeed, Y., Khan, M. U., Waseem, M., Zahid, U., Mahmood, F., Majeed, F., et al. (2023). Renewable energy as an alternative source for energy management in agriculture. Energy Rep. 10, 344–359. doi: 10.1016/j.egyr.2023.06.032
MAPA (2023) Ministério da Agricultura e Pecuária – Plano setorial de mitigação e de adaptação às mudanças climáticas para a consolidação de uma economia de baixa emissão de carbono na agricultura. Available at: https://www.gov.br/agricultura/pt-br/assuntos/sustentabilidade/plano-abc. Governo Federal, Brasília, MAPA/ACS, 173 p. (Accessed April 2023).
Marks, R. A., Hotaling, S., Frandsen, P. B., and VanBuren, R. (2021). Representation and participation across 20 years of plant genome sequencing. Nature Plants 7, 1571–1578. doi: 10.1038/s41477-021-01031-8
Martha Junior, G. B., and Lopes, M. A. (2023). Charting new sustainable agricultural innovation pathways in Brazil. Sci. Agric. 80:e20230067. doi: 10.1590/1678-992X-2023-0067
Miko, I. (2008). Gregor Mendel and the principles of inheritance. Nat. Educ. 1:134. Available at: https://www.nature.com/scitable/topicpage/gregor-mendel-and-the-principles-of-inheritance-593/
Moreira-Pinto, C. E., Coelho, R. R., Leite, A. G. B., Silveira, D. A., de Souza, D. A., Lopes, R. B., et al. (2021). Increasing Anthonomus grandis susceptibility to Metarhizium anisopliae through RNAi-induced AgraRelish knockdown: a perspective to combine biocontrol and biotechnology. Pest Manag. Sci. 77, 4054–4063. doi: 10.1002/ps.6430
Moura, M. M., Santos, A. R., Pezzopane, J. E. M., Alexandre, R. S., Silva, S. F., Pimentel, S. M., et al. (2019). Relation of El Niño and La Niña phenomena to precipitation, evapotranspiration and temperature in the Amazon basin. Sci. Total Environ. 651, 1639–1651. doi: 10.1016/j.scitotenv.2018.09.242
Munaweera, T. I. K., Jayawardana, N. U., Rajaratnam, R., and Dissanayake, N. (2022). Modern plant biotechnology as a strategy in addressing climate change and attaining food security. Agric. Food Secur. 11:26. doi: 10.1186/s40066-022-00369-2
Nair, L. G., Agrawal, K., and Verma, P. (2022). An overview of sustainable approaches for bioenergy production from agro-industrial wastes. Energy Nexus 6:100086. doi: 10.1016/j.nexus.2022.100086
Nakei, M. D., Venkataramana, P. B., and Ndakidemi, P. A. (2022). Soybean-nodulating rhizobia: ecology, characterization, diversity, and growth promoting functions. Front. Sustain. Food Syst. 6:824444. doi: 10.3389/fsufs.2022.824444
Neves, M. F. (2014). Agronegócio: Ameaças para as cadeias produtivas integradas. Agroanalysis (FGV) 34:21. Available at: http://www.agroanalysis.com.br/9/2014/mercado-negocios/agronegocio-ameacas-para-as-cadeias-produtivas-integradas
Neves, M. F. (2020). O Agronegócio nos tempos de coronavírus. Rev. Agron. Bras. 4, 1–7. doi: 10.29372/rab202018
Neves, M. F., Casagrande, B. P., Cambaúva, V., Teixeira, G. O., and Toledo, P. J. F. (2023). Agriculture 6.0: a new proposal for the future of agribusiness. Rev. Gest. Soc. Ambient. 17:e04004. doi: 10.24857/rgsa.v17n9-021
NOAA (2023) El niño/southern oscillation (ENSO) diagnostic discussion. Climate Prediction Center, National Weather Service, National Centers for Environmental Prediction Climate Prediction Center. Available at: https://www.cpc.ncep.noaa.gov/products/analysis_monitoring/enso_advisory/ensodisc.shtml (Accessed November 2023).
Oliveira, C. M., Auad, A. M., Mendes, S. M., and Frizzas, M. R. (2012). Economic impact of exotic insect pests in Brazilian agriculture. J. Appl. Entomol. 137, 1–15. doi: 10.1111/jen.12018
Osei, E., Jafri, S. H., Saleh, A., Gassman, P. W., and Gallego, O. (2023). Simulated climate change impacts on corn and soybean yields in Buchanan County, Iowa. Agriculture 13:268. doi: 10.3390/agriculture13020268
Pinheiro, C. (2023) Environmental, social, and governance (ESG) reporting and Brazilian agriculture: constraints and opportunities to sustainability. In: N. Søndergaard, C. D. Dias de Sá, and A. F. Barros-Platiau (eds) Sustainability challenges of Brazilian agriculture. Environment & policy, 64:249–269. Berlin: Springer International Publishing
Reis, P. A. B., Magalhaes, J. V., Miller, R. N. G., and Fontes, E. P. B. (2023). State-of-the-art molecular plant sciences in Brazil. Int. J. Mol. Sci. 24:8909. doi: 10.3390/ijms24108909
Rezaei, E. E., Webber, H., Asseng, S., Boote, K., Durand, J. L., Ewert, F., et al. (2023). Climate change impacts on crop yields. Nat. Rev. Earth Environ. 4, 831–846. doi: 10.1038/s43017-023-00491-0
Ribeiro, T. P., Arraes, F. B. M., Lourenço-Tessutti, I. T., Silva, M. S., Lisei-de-Sá, M. E., Lucena, W. A., et al. (2017). Transgenic cotton expressing Cry10Aa toxin confers high resistance to the cotton boll weevil. Plant Biotechnol. J. 15, 997–1009. doi: 10.1111/pbi.12694
Ribeiro, T. P., Basso, M. F., Carvalho, M. H., Macedo, L. L. P., Silva, D. M. L., Lourenço-Tessutti, I. T., et al. (2019). Stability and tissue-specific Cry10Aa overexpression improves cotton resistance to the cotton boll weevil. Biotechnol. Res. Innov. 3, 27–41. doi: 10.1016/j.biori.2019.12.003
Ribeiro, T. P., Vasquez, D. D. N., Macedo, L. L. P., Lourenço-Tessutti, I. T., Valença, D. C., Oliveira-Neto, O. B., et al. (2022). Stabilized double-stranded RNA strategy improves cotton resistance to CBW (Anthonomus grandis). Int. J. Mol. Sci. 23:13713. doi: 10.3390/ijms232213713
Ribichich, K. F., Chiozza, M., Ávalos-Britez, S., Cabello, J. V., Arce, A. L., Watson, G., et al. (2020). Successful field performance in warm and dry environments of soybean expressing the sunflower transcription factor HB4. J. Exp. Bot. 71, 3142–3156. doi: 10.1093/jxb/eraa064
Rocha, T. L., Soll, C. B., Boughton, B. A., Silva, T. S., Oldach, K., Firmino, A. A. P., et al. (2017). Prospection and identification of nematotoxic compounds from Canavalia ensiformis seeds effective in the control of the root-knot nematode Meloidogyne incognita. Biotechnol. Res. Innov. 1, 87–100. doi: 10.1016/j.biori.2017.10.003
Rolla, A. A. P., Carvalho, J. F. C., Fuganti-Pagliarini, R., Engels, C., Rio, A., Marin, S. R., et al. (2014). Phenotyping soybean plants transformed with rd29A:AtDREB1A for drought tolerance in the greenhouse and field. Transgenic Res. 23, 75–87. doi: 10.1007/s11248-013-9723-6
Salgotra, R. K., and Stewart, C. N. Jr. (2020). Functional markers for precision plant breeding. Int. J. Mol. Sci. 21:4792. doi: 10.3390/ijms21134792
Santos, D. C. L. P., Correa, C., Alves, Y. A., Souza, C. G., and Boloy, R. A. M. (2023). Brazil and the world market in the development of technologies for the production of second-generation ethanol. Alex. Eng. J. 67, 153–170. doi: 10.1016/j.aej.2022.09.004
Sharma, R. K., Kumar, S., Vatta, K., Dhillon, J., and Reddy, K. N. (2022). Impact of recent climate change on cotton and soybean yields in the southeastern United States. J. Agric. Food Res. 9:100348. doi: 10.1016/j.jafr.2022.100348
Silva, F. T., Baierle, I. C., Correa, R. G. F., Sellitto, M. A., Peres, F. A. P., and Kipper, L. M. (2023). Open innovation in agribusiness: barriers and challenges in the transition to agriculture 4.0. Sustainability 15. doi: 10.3390/su15118562
Silva, G. J., Berg, E. C., Calijuri, M. L., Santos, V. J., Lorentz, J. F., and Alves, S. C. (2021b). Aptitude of areas planned for sugarcane cultivation expansion in the state of São Paulo, Brazil: a study based on climate change effects. Agric. Ecosyst. Environ. 305:107164. doi: 10.1016/j.agee.2020.107164
Silva, U. C., Cuadros-Orellana, S., Silva, D. R. C., Freitas-Júnior, L. F., Fernandes, A. C., Leite, L. R., et al. (2021a). Genomic and phenotypic insights into the potential of rock phosphate solubilizing bacteria to promote millet growth in vivo. Front. Microbiol. 11:574550. doi: 10.3389/fmicb.2020.574550
Sinha, D., Maurya, A. K., Abdi, G., Majeed, M., Agarwal, R., Mukherjee, R., et al. (2023). Integrated genomic selection for accelerating breeding programs of climate-smart cereals. Genes 14:1484. doi: 10.3390/genes14071484
Smith, C., Baker, J. C. A., and Spracklen, D. V. (2023). Tropical deforestation causes large reductions in observed precipitation. Nature 615, 270–275. doi: 10.1038/s41586-022-05690-1
Souza, T. T., Antolin, L. A. S., VJM, B., RAA, P., EHFM, S., and Marin, F. R. (2019). Longer crop cycle lengths could offset the negative effects of climate change on Brazilian maize. Bragantia 78, 622–631. doi: 10.1590/1678-4499.20190085
Souza, B., and Haddad, E. (2022). Climate change in Brazil: dealing with uncertainty in agricultural productivity models and the implications for economy-wide impacts. Spat. Econ. Anal. 17, 83–100. doi: 10.1080/17421772.2021.1934524
Távora, F. T. P. K., Diniz, F. A. S., Rêgo-Machado, C. M., Freitas, N. C., Arraes, F. B. M., Andrade, E. C., et al. (2022). CRISPR/Cas- and topical RNAi-based technologies for crop management and improvement: reviewing the risk assessment and challenges towards a more sustainable agriculture. Front. Bioeng. Biotechnol. 10:913728. doi: 10.3389/fbioe.2022.913728
Thiebaut, F., Urquiaga, M. C. O., Rosman, A. C., da Silva, M. L., and Hemerly, A. S. (2022). The impact of non-nodulating diazotrophic bacteria in agriculture: understanding the molecular mechanisms that benefit crops. Int. J. Mol. Sci. 23:11301. doi: 10.3390/ijms231911301
Thrall, P. H., Bever, J. D., and Burdon, J. J. (2010). Evolutionary change in agriculture: the past, present and future. Evol. Appl. 3, 405–408. doi: 10.1111/j.1752-4571.2010.00155.x
Tillman, D., Balzer, C., Hill, J., and Belfort, B. (2011). Global food demand and the sustainable intensification of agriculture. PNAS 108, 20260–20264. doi: 10.1073/pnas.1116437108
UNICA (2023) Brazilian Sugarcane Industry Association. Available at: https://unicadata.com.br/?&idioma=2 (Accessed November 2023).
United Nations (2023) Peace, dignity and equality on a healthy planet. Available at: https://www.un.org/en/global-issues/population#:~:text=The%20world%20population%20is%20projected,surrounding%20these%20latest%20population%20projections (Accessed November 2023).
USDA (2023a) World agricultural production. Available at: https://apps.fas.usda.gov/psdonline/circulars/production.pdf (Accessed November 2023).
USDA (2023b) Livestock and poultry: world markets and trade. Available at: http://www.fas.usda.gov/psdonline/circulars/livestock_poultry.pdf (Accessed November 2023).
van Dijk, M., Morley, T., Rau, M. L., and Saghai, Y. (2021). A meta-analysis of projected global food demand and population at risk of hunger for the period 2010-2050. Nature Food 2, 494–501. doi: 10.1038/s43016-021-00322-9
Vasquez, D. D. N., Pinheiro, D. H., Teixeira, L. A., Moreira-Pinto, C. E., Macedo, L. L. P., Salles-Filho, A. L. O., et al. (2023). Simultaneous silencing of juvenile hormone metabolism genes through RNAi interrupts metamorphosis in the cotton boll weevil. Front. Mol. Biosci. 10:1073721. doi: 10.3389/fmolb.2023.1073721
Wake, B. (2023). Economics of adaptation. Nature. Climate Change 13:764. doi: 10.1038/s41558-023-01773-7
Wang, B., Sun, W., Jin, C., Luo, X., Yang, Y.-M., Li, T., et al. (2023). Understanding the recent increase in multiyear La Niñas. Nat. Clim. Chang. 13, 1075–1081. doi: 10.1038/s41558-023-01801-6
Wang, L., Xiao, Y., Wei, X., Pan, J., and Duanmu, D. (2021). Highly efficient CRISPR-mediated base editing in Sinorhizobium meliloti. Front. Microbiol. 12:686008. doi: 10.3389/fmicb.2021.686008
Wong, A. C. S., Massel, K., Lam, Y., Hintzsche, J., and Chauhan, B. S. (2022). Biotechnological road map for innovative weed management. Front. Plant Sci. 13:887723. doi: 10.3389/fpls.2022.887723
Woodward, J. (2014). Bi-allelic SNP genotyping using the TaqMan® assay. Methods Mol. Biol. 1145, 67–74. doi: 10.1007/978-1-4939-0446-4_6
Yi, Y., Li, Z., Song, C., and Kuipers, O. P. (2018). Exploring plant-microbe interactions of the rhizobacteria Bacillus subtilis and Bacillus mycoides by use of the CRISPR-Cas9 system. Environ. Microbiol. 20, 4245–4260. doi: 10.1111/1462-2920.14305
Yin, K., Gao, C., and Qiu, J.-L. (2017). Progress and prospects in plant genome editing. Nature Plants 3:17107. doi: 10.1038/nplants.2017.107
Keywords: biotechnology, climate changes, disruptive technologies, molecular sciences, scientific research, sustainable agriculture
Citation: Basso MF, Neves MF and Grossi-de-Sa MF (2024) Agriculture evolution, sustainability and trends, focusing on Brazilian agribusiness: a review. Front. Sustain. Food Syst. 7:1296337. doi: 10.3389/fsufs.2023.1296337
Edited by:
Josef Abrham, Metropolitan University Prague, CzechiaReviewed by:
Poonam Yadav, Banaras Hindu University, IndiaFrancesco Bozzo, University of Bari Aldo Moro, Italy
Copyright © 2024 Basso, Neves and Grossi-de-Sa. This is an open-access article distributed under the terms of the Creative Commons Attribution License (CC BY). The use, distribution or reproduction in other forums is permitted, provided the original author(s) and the copyright owner(s) are credited and that the original publication in this journal is cited, in accordance with accepted academic practice. No use, distribution or reproduction is permitted which does not comply with these terms.
*Correspondence: Maria Fatima Grossi-de-Sa, ZmF0aW1hLmdyb3NzaUBlbWJyYXBhLmJy; Marcos Fernando Basso, bWFyY29zYmlvdGVjQGdtYWlsLmNvbQ==
†This work was part of the TCC in the MBA in agribusiness USP/ESALQ ED221/2023