- 1College of Biology and Food Engineering, Hubei University for Nationalities, Enshi, China
- 2College of Life Sciences, Jilin Agricultural University, Changchun, China
- 3College of Forestry and Horticulture, Hubei University for Nationalities, Enshi, China
- 4College of Biological Engineering, Beijing Polytechnic, Beijing, China
Idesia polycarpa is a good source of high-quality vegetable oil and can potentially alleviate pressure on the supply of edible oil. Exploring Idesia polycarpa could enrich the world’s food and oil resources and make up for the shortage of vegetable oil types and production. Here, 15 varieties of wild Idesia polycarpa from major wild Idesia polycarpa resource belts in the middle and upper reaches of the Yangtze River in China were investigated to elucidate the quality and antioxidant activity of wild Idesia polycarpa fruits to tap the potential exploitation capacity of Idesia polycarpa. In this study, wild Idesia polycarpa varieties with high oil quality and antioxidant activity were screened by the comprehensive evaluation of 42 indexes including oil content, dry basis white grain weight, fatty acids, tocopherols, total flavonoids, and minerals, as well as comparisons of free radical scavenging of 2,2′-azino-bis (3-ethylbenzothiazoline-6-sulfonic acid (ABTS)) and 1,1-diphenyl-2-picrylhydrazyl (DPPH). The result showed the quality characteristics of 15 wild Idesia polycarpa: oil (MN:29.21/100 g), dry basis white grain weight (MN:17.32 g), total flavonoids (MN:27.27 RE/kg), total polyphenols (MN:159.63 GAE/kg), tocopherols [MN:356.10 mg/kg (α-tocopherol (MN:284.96 mg/kg), γ-tocopherol (MN:50. 20 mg/kg), β-tocopherol (MN:9.54 mg/kg), and δ-tocopherol (MN:11.40 mg/kg)], total mineral content (MN:25653.44 mg/kg), and fatty acids [MN:20.89/100 g (70.42% polyunsaturated fatty acids (MN:14.63/100 g), 20.29% saturated fatty acids (MN:4.25/100 g), and 9.29% monounsaturated fatty acids (MN:2.01/100 g)]. Correlation analysis revealed that flavonoids, C16:0, C18:1n9c, C18:2n6c, polyphenols, α-tocopherol, δ-tocopherol, and Ca were significantly correlated with DPPH and ABTS. Cluster analysis and principal component analysis classified the 15 wild samples into three clusters, with the first cluster represented by H8, H6, and H9 being the optimal sample as a woody oilseed crop, the second cluster represented by H10, H 12, and H11 being the optimal sample for functional oil development, and the rest could be selected with emphasis when conducting certain characteristic product development. The excellent lipids and rich nutritional and antioxidant properties suggest that Idesia polycarpa will be a new direction for the development of edible oil resources in the future.
1. Introduction
Idesia polycarpa is a deciduous tree of the genus Idesia. Idesia polycarpa fruit called oil grape and is known as a “tree oil bank” with ornamental and edible functions. Most of the research on Idesia polycarpa is focused on biodiesel, gardening, seed variation, nursery, and planting technology (Yang et al., 2009; Zhu et al., 2010; Gong et al., 2012; Jia et al., 2014). Idesia polycarpa was included in the general food management system in 2020, and its research mainly focuses on the accumulation of basic nutrients during the growth period (Wen et al., 2022), oil extraction and refinement (Song, 2021), and oil aroma analysis (Chen et al., 2022). Idesia polycarpa fruits are rich in unsaturated fatty acids, tocopherols, glycosides, alkaloids, squalene, phytosterols, flavonoids, polyphenols, and other nutrients and bioactive substances (Yang, 2008).
Idesia polycarpa is not only an excellent resource for developing new wood-based edible oils but also has good development value in many fields. The fresh fruit of Idesia polycarpa can be used as raw material to make edible sauces (Li, 2018). Cellular experiments have shown that Idesia polycarpa defatted extract can improve non-alcoholic fatty liver disease by activating antioxidant signaling pathways and regulating lipid metabolism (Li et al., 2020), and it was confirmed that it can delay or prevent skeletal muscle atrophy by inhibiting apoptosis (Jung et al., 2010).
The methanolic extract and isolated components of Idesia polycarpa in 3 T3-L1 cells can improve non-alcoholic fatty liver disease by inhibiting adipogenesis and upregulation of oxidase proliferator-activated receptor type γ (PPARγ) expression for the treatment of obesity and diabetes (Lee et al., 2013). Idesia polycarpa fruit extract isolated a new compound, Idesolide, with a novel spiro structure of tetrahydrobenzodioxole (Kim et al., 2005) determined by single crystal X-ray experimental NMR, and MS spectroscopy significantly reduced lipopolysaccharide-induced free radical nitric oxide production in BV2 microglia. The production of nitric oxide, which is involved in a variety of biological functions including pathogenic inflammation and cell proliferation and differentiation (Jung et al., 2010), was demonstrated by Xiang et al. (2023), who performed in vitro lipid digestion of Idesia polycarpa and confirmed that it is highly lipolytic, second only to olive oil, as a nutritious and easily digestible vegetable oil. These studies have uncovered the potential applications of Idesia polycarpa fruits as food, oil, and natural active products.
Many studies (Kim et al., 2005; Yang, 2008; Jung et al., 2010; Lee et al., 2013; Li, 2018; Li et al., 2020; Song, 2021; Chen et al., 2022; Wen et al., 2022; Xiang et al., 2023) reported the chemical and functional analyses of Idesia polycarpa fruit, but there is limited research on wild samples collected from different geographical regions. Furthermore, the antioxidant property analysis and correlation between all components still require attention. This study aimed to systematically investigate the fruit quality characteristics and correlation between all components of wild Idesia polycarpa fruit from different germplasm resources. Here, 15 varieties of wild Idesia polycarpa from major wild Idesia polycarpa resource belts in the middle and upper reaches of the Yangtze River in China were investigated to elucidate the quality and antioxidant activity of wild Idesia polycarpa fruits to tap the potential exploitation capacity of Idesia polycarpa. A comprehensive study and variety classification of different germplasm resources of Idesia polycarpa through correlation analysis, principal component, and cluster analysis were conducted to provide basic support for the selection and breeding of Idesia polycarpa varieties and comprehensive fruit development.
2. Materials and methods
2.1. Idesia polycarpa sample collection
Fresh fruit samples of 15 wild Idesia polycarpa were collected from nine cities and counties in Hubei Province, Yunnan Province, and Sichuan Province Municipality in October 2022. Fresh wild Idesia polycarpa fruits were kept in polythene bags in the refrigerator at about 4°C before testing and sent to the laboratory immediately after harvest. The germplasm resources (Table 1) of wild Idesia polycarpa samples were preserved at Hubei Minzu University, Enshi Tujia, and Miao Autonomous Prefecture, China. The 15 varieties of wild Idesia polycarpa fruit photos are presented in Appendix Figure B1.
2.2. Chemicals and reagents
Ethanol, n-Hexane, nitric acid, petroleum ether (boiling range 40–60°C), sodium carbonate, sodium hydroxide (NaOH), sodium nitrite (NaNO2), aluminum nitrate [Al(NO3)3], folin-phenol, ferrozine, rutin (purity≥95%), pyrogallic acid (purity≥98%), gallic acid (purity≥95%), 1,1-diphenyl-2-picrylhydrazyl (DPPH; purity≥97%), 2,2′-azino-bis(3-ethylbenzothiazoline-6-sulfonic acid) (ABTS; purity≥98%), potassium persulfate, ferrous sulfate (FeSO4·7H2O), methanol (HPLC grade), potassium persulfate (K2O8S2), and 37 fatty acid methyl ester mixed standards (purity≥99%) were purchased from Shanghai Anpel Scientific Instrument Co., Ltd. (Shanghai, China). A standard solution containing 13 analytical elements [Na, Mg, K, Ca, Fe, Zn, Mn, Li, B, Al, P, V, and Co (1,000 mg/L)] and four forms of tocopherols [α-, β-, γ-, and δ-tocopherols (purity≥98%)], reference standards were purchased from Sigma-Aldrich Co. (Shanghai, China). All chemicals were of analytical grade unless specially mentioned.
2.3. Fruit physical measurements
The harvested Idesia polycarpa fruits were kept in separate polythene bags in the refrigerator at about 4°C before testing. Transverse diameter and longitudinal diameter were precisely measured with a digital caliper. The fruit shape index was calculated using Equation (1).
2.4. Moisture content and dry basis 100 grain weight determination
The moisture content of Idesia polycarpa was stipulated by weighing 5.0 g of fresh fruit (National Standards of the People’s Republic of China, 2017). The sample was placed in an oven dryer at 105°C for 24 h until all samples were dried thoroughly. The sample was then cooled in a desiccator. Eq. (2) was used to determine the initial moisture content. The dry basis 100-grain weight of Idesia polycarpa was repeated as in the steps mentioned above; the difference is that 100 fruits have to be dried (Wen et al., 2022).
2.5. Oil content determination
Idesia polycarpa fruits were washed before being dried in the heat pump oven at 60°C for 48 h. The dried fruits were ground into powder. Idesia polycarpa oil was extracted from the fruit powder (5.0 g) using the soxhlet method at 55°C for 8 h with petroleum ether (200 mL) as a solvent (Li et al., 2019). The oil content was expressed as Eq. (3).
2.6. Sample preparation for phenolic extracts and antioxidant properties analysis
Idesia polycarpa samples were extracted by following the procedure by Li et al. (2020), with slight modifications. In brief, 1.0 g dry powder of each sample of the 15 Idesia polycarpa was transferred into 20 mL centrifugal tubes, and 10 mL of extraction solvent ethanol/water (60:40) was then added to each tube. All the tubes were mixed well using a vortex mixer, after which they were placed in an ultrasound bath for 15 min at 30°C and centrifuged at 4,500 r/min for 10 min, and then all the supernatants were transferred into another new batch of 20 mL tubes. Another 10 mL of extraction solution was added to the same 1.0 g sample tubes again by repeating the steps mentioned above. These two extracts were combined in 20 mL tubes. The extracts were stored at 4°C for further analysis.
2.7. Determination of total flavonoids contents
Xu and Chang (2007) defined a colorimetric method that was used to determine the TFC in Idesia polycarpa. In brief, 0.2 mL of sample extract was mixed with 0.8 mL of distilled water, then 0.4 mL of 5% NaNO2 was added into each tube and mixed properly with the vortex mixture. After 6 min, 0.4 mL of 10% Al (NO3)3 solution was added and stood for 6 min before 4 mL of 1 mol/L Na OH solution was added into each tube. The solution was mixed properly, and then distilled water was added to dilute it to 50 mL. It was left at room temperature for 15 min and again was mixed well. The absorbance was measured by a UV–visible spectrophotometer at 510 nm. The TFC was expressed as rutin equivalents (mg RE/g sample) of the external standard calibration curve in a linearity range from 10 to 70 μg/mL (R2 = 0.9997).
2.8. Determination of total phenolics contents
Total phenolic content was determined using the colorimetric method described by Xu and Chang (2007). In brief, 0.1 mL sample solution was mixed with 2.5 mL Folin-phenol reagent, 7.5 mL of 7% Na2CO3 was then added, and distilled water was added to dilute it to 50 mL. It was placed in the water bath at 45°C for 15 min, then the absorbance was measured at 765 nm against a blank by using the UV–visible spectrophotometer. The TPC was expressed as gallic acid equivalents (mg GAE/g sample) in accordance with the external standard calibration curve of the gallic acid in a linearity range of 2–30 μg/mL (R2 = 0.9979).
2.9. Determination of mineral elements by inductively coupled plasma-mass spectrometry
Mineral elements were determined according to the method by Canizo et al. (2018). Idesia polycarpa fruits were washed with ultrapure water before being thoroughly dried and finally pulverized with a mill. Samples were mineralized following the next procedure: 0.2 g were weighed and added with 5 mL of concentrated HNO3 and 2 mL of concentrated H2O2. This mixture was left at room temperature for 12 h. Afterward, the following heat treatment was applied using a heating plate: 60 min at 50°C, 90 min at 100°C, and finally 90 min at 150°C. The digested samples were left to cool until room temperature was reached and then transferred into a volumetric flask and taken to a final volume of 25 mL with ultrapure water. These solutions were finally analyzed by ICP-MS.
The instrumental conditions were as follows: auto lens mode on, peak hopping measure mode, dwell time of 50 m s, 10 sweeps per reading, one reading per replicate, and each analysis consisted of three replicates. After optimization of the instrument, the following conditions were chosen for all determinations: 1,500 W RF power, cooling gas flow of 14 L/min, sample introduction flow of 0.8 mL/min, nebulizer gas flow rate of 1.0 L/min, helium flow of 4.9 mL/min, peristaltic pump speed 40 r/min, atomization chamber temperature 2.6°C, and sampling depth of 5 mm. The standard calibration curve and linearity range of mineral elements are presented in Appendix Table A1.
2.10. Determination of tocopherols content by high-performance liquid chromatography
The tocopherol content of Idesia polycarpa was determined using the HPLC method described by GB 5009.82-2016 (National Standards of the People’s Republic of China, 2023) with slight modifications. In brief, 2.00 g of Idesia polycarpa powder was transferred into a 250-mL conical flask, then 10 mL of VC solution, 90 mL of ethanol, and 25 mL of 50% potassium hydroxide solution were added to the conical flask containing the sample. The conical flask was heated at 80°C for 30 min. After cooling down, the mixture was filtered, and the filtrated solution was transferred to the separatory funnel. The solution was extracted using 50 mL petroleum ether three times successively. The organic layer was washed with 100 mL of deionized water and the organic layer was transferred into a 250-mL round-bottom flask using a rotary evaporator to evaporate organic solvent. The residue was redissolved with 10 mL of absolute methanol. The solution was filtered through a 0.22-μm nylon syringe filter and transferred into 2 mL of 9 mm screw thread vials. The sample (10 μL) was injected into the HPLC system (Agilent System, China) with a diode array detector (DAD) and fluorescence detector (FLD; Agilent 1260 Series, China), and the compounds were separated using C18 column (C18, 4.6 mm × 150 mm, 5 μm; Agilent Technologies, China) with a flow rate of 1.0 mL/min. Methanol and water (98:2) were used as the mobile phase. Determination and quantification of α-, β-, γ-, and δ-tocopherol were performed at 20°C, and excitation and emission wavelengths of the fluorescence detector were 294 and 328 nm, respectively. Tocopherol content in Idesia polycarpa fruits was expressed as mg/kg in accordance with the external standard calibration curve of tocopherol. The standard calibration curve and linearity range of four tocopherols are presented in Appendix Table A2 and the separation chromatography of α-, β-, γ-, and δ-tocopherol are presented in Appendix Figure B2.
2.11. Determination of fatty acids content by gas chromatography
Idesia polycarpa fruits were dried and crushed into powder, and seed oil was extracted using an ultrasonic-assisted extraction method. Fatty acid methyl esters (FAMEs) were prepared according to the method by Zhao et al. (2020) with some modifications. In brief, a 20 mg sample was mixed with n-hexane and potassium hydroxide-methanol solution, 100 mg of pyrogallic acid as an antioxidant, followed by shaking for 30 min at 85°C to obtain fatty acid methyl esters. The fatty acid methyl esters were quantified using gas chromatography on an HP-88 capillary column (100 m, 0.25 mm, and 0.20 μm) by Agilent 7890A with a flame ionization detector (FID). The temperature of the injector and detector was set at 250°C. The initial temperature was held at 130°C for 5 min, ramped to 240°C at 4°C/min, and maintained for 30 min. N2 and air were used as carrier gas at the rate of 0.5 mL/min. A volume of 1 μL was injected. The standard calibration curve and linearity range of fatty acids are presented in Appendix Table A3 and the separation chromatography of fatty acids is presented in Appendix Figure B3.
2.12. Determination of DPPH free radical scavenging ability
1,1-diphenyl-2-picrylhydrazyl was determined using the colorimetric method described by Pan et al. (2019). In brief, 200 μL of sample extract was diluted in 2 mL of 60% ethanol and then mixed with 2.0 mL of 0.1 mmol/L DPPH solution. All tubes were mixed evenly with the vortex mixture and stood at room temperature in the dark for 30 min. The UV–visible spectrophotometer was used to measure the absorbance at 517 nm against a blank. Eq. (4) was used to determine the DPPH free radical scavenging activities.
2.13. Determination of ABTS radical scavenging ability
2,2′-azino-bis (3-ethylbenzothiazoline-6-sulfonic acid) was determined using the colorimetric method described by Pan et al. (2019). ABTS stock solution was prepared accordingly, 3.6 g (7 mmol/L) ABTS and 0.6623 g (2.45 mmol/L) potassium persulfate were dissolved in distilled water in an amber bottle and incubated in the dark at room temperature for about 16 h to generate ABTS radical cation. This stock solution was taken to dilute with ethanol, and the absorbance of 0.70 ± 0.02 at 734 nm was obtained to use as a working solution. Afterward, 20 μL of sample extract was diluted in 200 μL of 60% ethanol and then was mixed with 4 mL ABTS working solution and incubated for 6 min at 30°C in the dark.
2.14. Statistical analysis
The results were reported as mean ± standard deviation (SD). The differences among the Idesia polycarpa fruits were evaluated using one-way ANOVA with Tukey’s test and statistically significant differences were at p < 0.05. A PCA was performed on the complete dataset for each Idesia polycarpa using Origin (version 2023). A correlation matrix across all the variables of Idesia polycarpa was used to check whether the variables were highly correlated with any other variables before performing the PCA. Plotting was performed with Excel 2016, while the data were analyzed and plotted with Origin (version 2023), SPSS (version 26), and Cytoscape (version 3.9.1).
3. Results and discussion
3.1. Physical characteristics
3.1.1. Morphological diversity of wild Idesia polycarpa
Fruit morphological characteristics of 15 wild Idesia polycarpa samples were presented in Table 2. The results showed that there were significant differences (p < 0.05) in the value indicators between samples. Among these 15 wild Idesia polycarpa samples, the largest horizontal and longitudinal diameters were recorded in samples H10, H2, and H11, which were greater than 11.00 and 10.00 mm, respectively. The smallest horizontal and longitudinal diameters recorded in H1 and H8 were 7.10 and 7.20 mm, respectively, smaller than wild Idesia polycarpa samples. The coefficients of variation for horizontal and longitudinal diameters of wild Idesia polycarpa were 16.20 and 16.15%, respectively. Although wild Idesia polycarpa showed high variability in fruit size, it had an average fruit shape index of 0.92 and a coefficient of variation of 5.13%. This means that the shape of wild Idesia polycarpa was highly stable, being oval and round regardless of size.
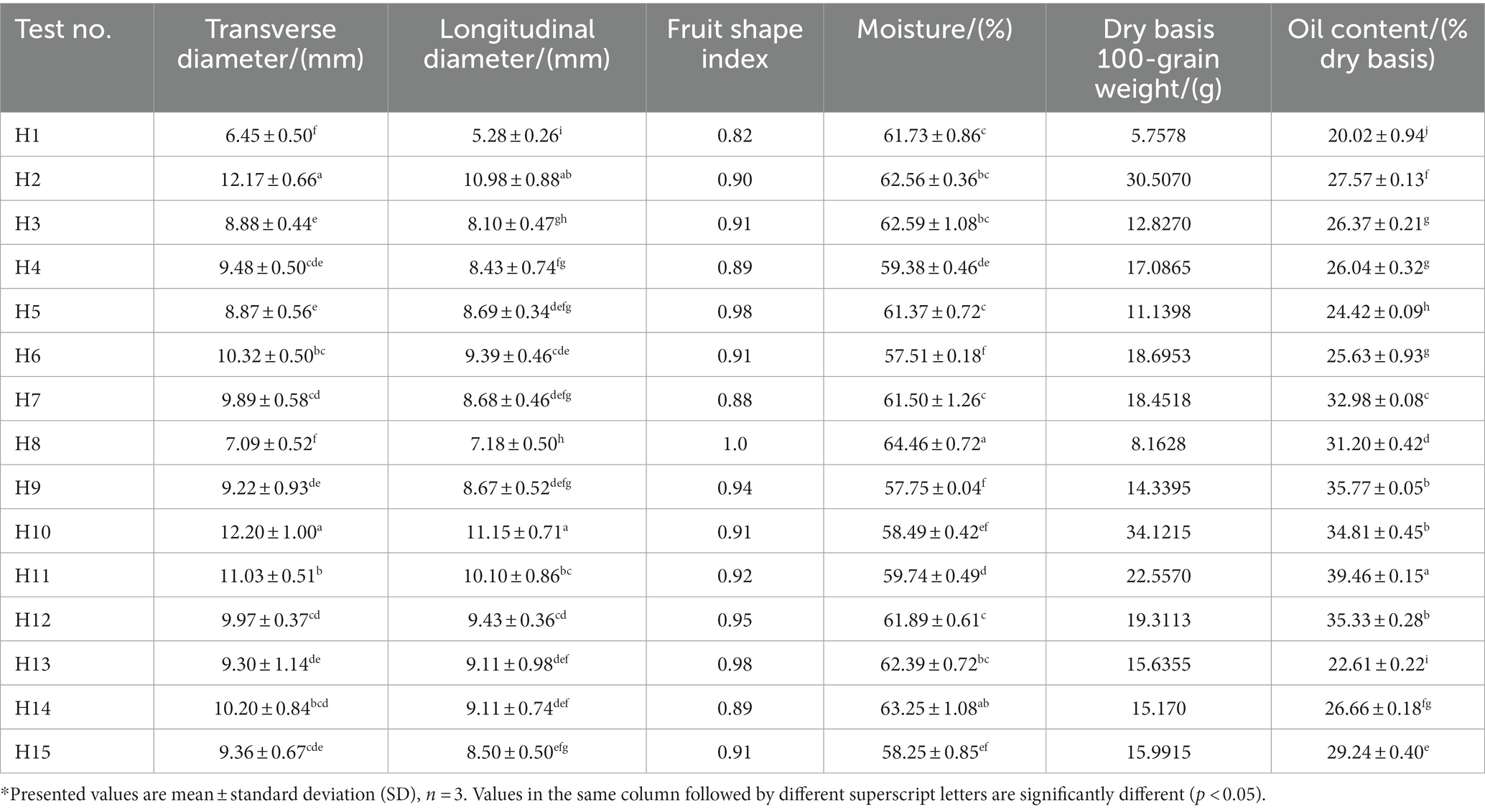
Table 2. Fruit size (transverse diameter and longitudinal diameter), fruit shape index, oil content, moisture content, and dry basis 100-grain weight of wild Idesia polycarpa.
3.1.2. Moisture content of wild Idesia polycarpa
The moisture contents of the 15 fresh wild Idesia polycarpa varieties are presented in Table 2. All the varieties showed a considerable amount of moisture content between 57.51 and 64.46% and a coefficient of variation of 3.45%, which showed a higher amount of moisture content in all fresh wild Idesia polycarpa. The stable moisture content of fresh wild Idesia polycarpa will reduce the burden of sorting and facilitate uniform drying after harvest.
3.1.3. Fruit dry base 100-grain weight of wild Idesia polycarpa
Fruit dry base 100-grain weight of 15 wild Idesia polycarpa varieties are presented in Table 2. The results showed that among these 15 wild Idesia polycarpa samples, the heaviest fruit dry base 100-grain weight was recorded in samples H10 and H2, which was higher than 30 g, while the lightest fruit dry base 100-grain weight recorded in H1 was below 6 g of wild Idesia polycarpa samples. The average value of the remaining wild Idesia polycarpa samples was recorded as 15.79 g, with a coefficient of variation of 43.24%. Fruit dry base 100-grain weight represents the dry biomass, and as an oilseed crop, the dry basis 100-grain weight of wild Idesia polycarpa is important for oil and fat-soluble nutrients.
3.2. Oil content of wild Idesia polycarpa
Oil was the major component of wild Idesia polycarpa. The total oil contents of 15 wild Idesia polycarpa varieties are presented in Table 2. The results showed that the maximum amount of oil content was recorded at 39.46/100 g in H11, while the lowest was recorded at 20.02/100 g in the H1 wild Idesia polycarpa sample. The average total oil content of all wild Idesia polycarpa samples was recorded as 29.12/100 g, with a coefficient of variation of 18.89%, and the high variability in oil content implies a more favorable selection of oil extraction varieties.
3.3. TFC and TPC of wild Idesia polycarpa
Flavonoids are natural antioxidants that scavenge free radicals and inhibit lipid peroxidation (Nimse and Pal, 2015), which are composed of a 15-carbon skeleton that includes two phenyl rings, benzo-γ-pyrone generally (Cook and Samman, 1996; ChandKumar et al., 2023). Flavonoids are signaling molecules that act as developmental regulators in plants and humans (Tattini et al., 2013), and are involved in significant roles to alter anti-allergic, anti-inflammatory, antioxidant, anti-mutagenic, and anti-carcinogenic activities (Kumar and Pandey, 2013; Ullah et al., 2020). Polyphenols are the vital responsible compounds in food products for antioxidant activity (Feng et al., 2022). The TPC was correlated with antioxidant activity (Abudayeh et al., 2019). Presti et al. (2017) applied Pearson correlation analysis to demonstrate that the phenolic content of extra virgin olive oil was positively correlated with the antioxidant activity that protects cells from oxidative stress. Polyphenol components help protect other minor components from oxidation, such as tocopherols, through their own oxidation or degradation (Presti et al., 2017; Lozano-Castellón et al., 2022).
TFC and TPC of 15 wild Idesia polycarpa varieties are presented in Figure 1. The results showed that among these 15 wild Idesia polycarpa samples, the highest TFC was recorded in samples H12 and H11, which was higher than 30 mg RE/g, while the highest TPC was recorded in samples H12 and H6, which was higher than 170 mg GAE/g. H12 was the sample with the highest TFC (32.14 mg RE/kg) and TPC (174.77 mg GAE/kg), while H13 was the sample with the lowest. The results in the current study showed that all the wild Idesia polycarpa varieties contained flavonoids and polyphenols, and the contents of total flavonoids and total polyphenols were not synchronized except for two samples which had relatively synchronized.
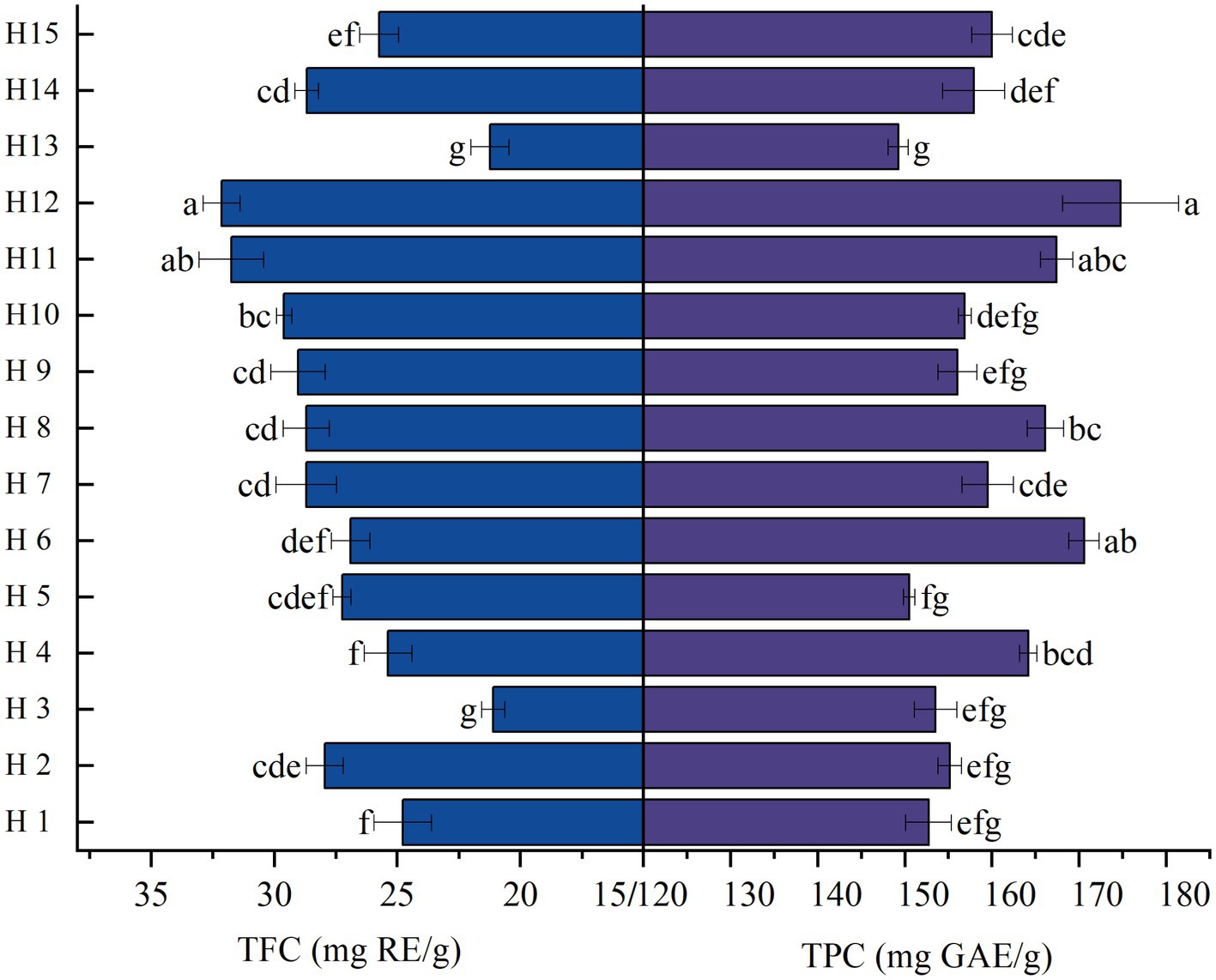
Figure 1. Significant differences in the contents of TFC and TPC in the fruits of wild Idesia polycarpa. TFC, Total flavonoid contents; TPC, Total phenolic contents. Values with different letters a–g are significantly different (p < 0.05).
3.4. Mineral element contents of Idesia polycarpa
Mineral elements play an important role in the synthesis of secondary plant metabolites and nutrient accumulation (Jia et al., 2016). B, Cu, Fe, Mn, Zn, and other elements are important components (Rengel et al., 2022) for activating decarboxylase, dehydrogenase, and oxidase, affecting plant cell division, cell development, photosynthesis, carbon cycle, etc.
Mineral element contents in 15 wild Idesia polycarpa are presented in Table 3. All Idesia polycarpa contain Na, Mg, K, Ca, Fe, Zn, Mn, Li, B, Al, P, V, and Co, and there was a significant difference (p < 0.05) in the mineral element contents. The results showed that K (15873.83 mg/kg), Ca (4084.37 mg/kg), P (2419.62 mg/kg), and Mg (2092.14 mg/kg) were the main mineral elements with the highest contents. The highest K content was found in H1, H3, and H11. The highest Ca content was found in H2, H8, and H10. The highest P content was found in H8, H6, and H4. The highest Mg content was found in H8, H9, and H7. Among them, K and Ca together account for 77.80% of the total mineral elements and the highest were recorded in H2, followed by H1 and H11. In addition, three mineral elements were also detected at lower levels, namely, Li, V, and Co, with the total content lower than 0.01% of the total mineral elements. The highest coefficient of variation was 55.55% in Mn, while the lowest was 10.98% in K. The average coefficient of variation for the remaining 11 elements was 35.67%. The wild Idesia polycarpa from different sub-regions owned unique mineral element content; it was also observed in peanuts (Zhao and Yang, 2022), soybeans (Enengl et al., 2022), and red pepper (Hur et al., 2023). The large variation in elemental content among individuals indicates that there are large differences in carbon skeleton formation, nutrient accumulation, and physiological metabolism (Martino et al., 2018; Rashid et al., 2021; Wu et al., 2023) of wild Idesia polycarpa plant cells, and this variation underlies the differences in protein, cellulose, fatty acids, tocopherols, sterols, flavonoids, and polyphenols contents among wild Idesia polycarpa species.
3.5. Tocopherols content of wild Idesia polycarpa
Tocopherols can prevent the peroxidation of unsaturated lipids through chain-breaking reactions and are recognized as effective lipophilic antioxidant compounds (Ma et al., 2023). Tocopherol contents in 15 wild Idesia polycarpa are presented in Figure 2. It is clear that α-tocopherol (MN 284.96 mg/kg, CV 35.25%) was the most tocopherol found in the Idesia polycarpa, followed by γ-tocopherol (MN 50.20 mg/kg, CV 36.55%), β-tocopherol (MN 39.54 mg/kg, CV 37.13%), and δ-tocopherol (MN 11.40 mg/kg, CV 54.73%). The results showed that the wild Idesia polycarpa contains δ-tocopherol, γ-tocopherol, β-tocopherol, and α-tocopherol. The highest δ-tocopherol content was recorded in samples H12 and H8, which was higher than 20 mg/kg, γ-tocopherol was recorded in samples H5 and H8, which was higher than 80 mg/kg, β-tocopherol was recorded in samples H10, which was higher than 60 mg/kg, and α-tocopherol was recorded in samples H5 and H1, which was higher than 470 mg/kg, while the lowest δ-tocopherol content recorded in H13 was below 4 mg/kg, γ-tocopherol content recorded in H2 was below 30 mg/kg, β-tocopherol content recorded in H5 was below 15 mg/kg, and α-tocopherol content recorded in H12 was below 170 mg/kg of wild Idesia polycarpa sample.
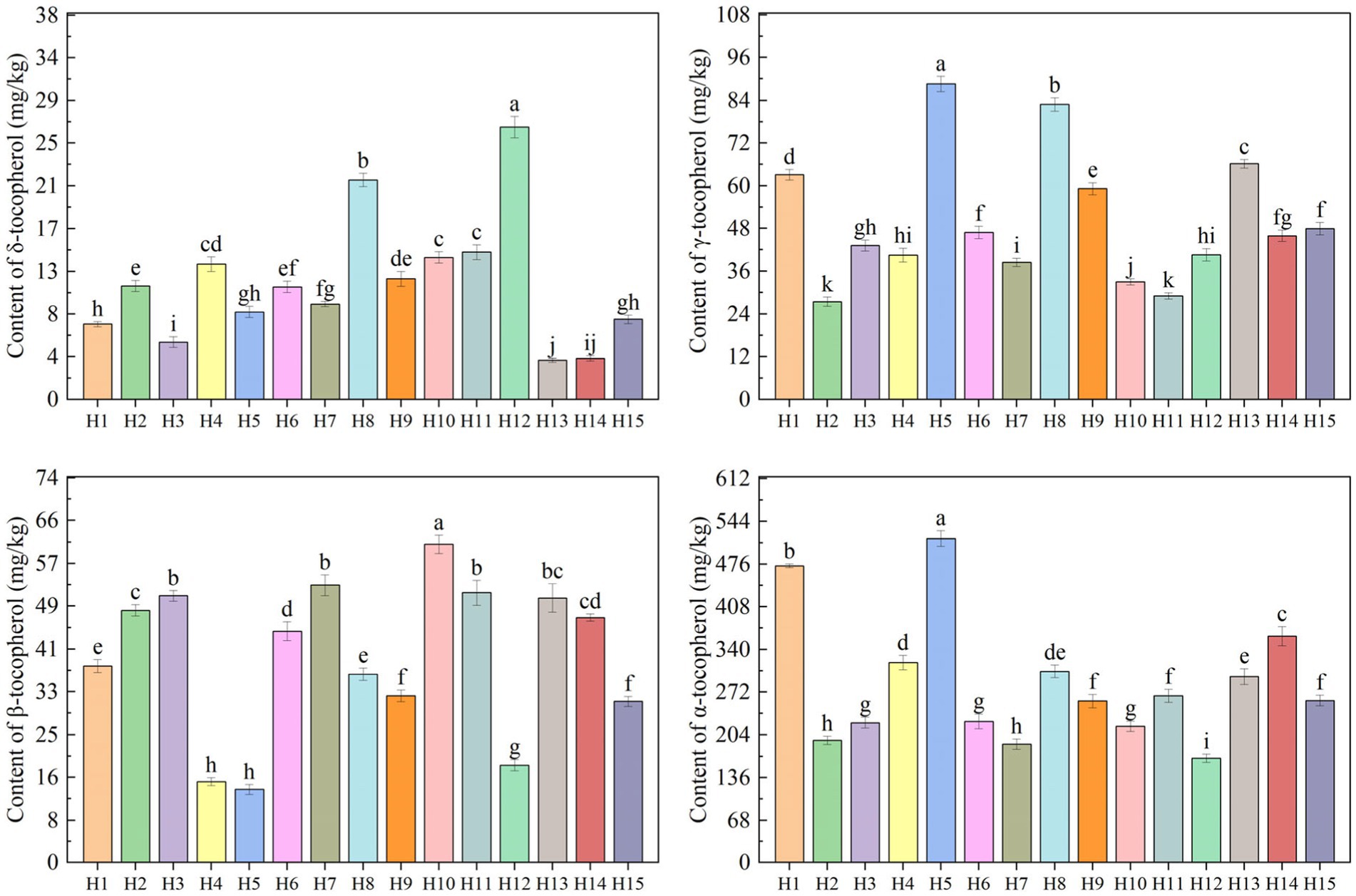
Figure 2. The contents of four tocopherols in the fruits of 15 wild Idesia polycarpa. Values with different letters a–k are significantly different (p < 0.05).
Among them, δ-tocopherol had the highest coefficient of variation, and γ-tocopherol had the highest significant difference. The α-tocopherol was the predominant tocopherol, accounting for 73.80% of the total tocopherols, while the differences were not significant for all samples except H5 and H1, which indicated that the content of α-tocopherol was high and stable. The most characterized samples were H5 (627.94 mg/kg) and H1 (581.56 mg/kg), which had the highest total tocopherol contents. It has been demonstrated that tocopherols play a vital role in human health because of their antioxidant effect (Chu et al., 2023). And optimal cellular function could be achieved by maintaining a healthy balance between tocopherols and polyunsaturated fatty acids (PUFA; Dong et al., 2018).
3.6. Fatty acid contents of wild Idesia polycarpa
Fatty acids are essential nutrients, building blocks of the body, and have many important functions in organisms, such as storing energy, participating in the composition of biofilms, and regulating various activities of organisms through cellular signaling pathways (Das, 2019; Liu et al., 2023).
Fatty acid contents in 15 wild Idesia polycarpa are presented in Figure 3. The results showed that wild Idesia polycarpa consists of 70.42% polyunsaturated fatty acids (PUFA; MN 14.63/100 g, CV 22.48%), 20.29% saturated fatty acids (SFA; MN 4.25/100 g, CV 27.95%), and 9.29% monounsaturated fatty acids (MUFA; MN 2.01/100 g, CV 42.37%), in the ratio of 1.48:1:4.86 (H12) to 2.91:1:10.24 (H13). The ratio of fatty acids in wild Idesia polycarpa was similar to that of iron walnut oil (PUFA 71.2, MUFA 20.8, and SFA 7.99%) and sacha inchi oil (PUFA 85.7, MUFA 7.72, and SFA 6.64%), while differs greatly from that of prinsepia utilis royle oil (PUFA 37.7, MUFA 38.6, and SFA 23.7%), olive oil (PUFA 6.63, MUFA 78.1, and SFA 15.2%), and avocado oil (PUFA 18.7, MUFA 52.3, and SFA 29%; Zhang et al., 2021). Wild Idesia polycarpa exhibits an excellent fatty acid profile compared to other woody oilseeds and was comparable to iron walnut and sacha inchi in terms of fatty acid ratio.
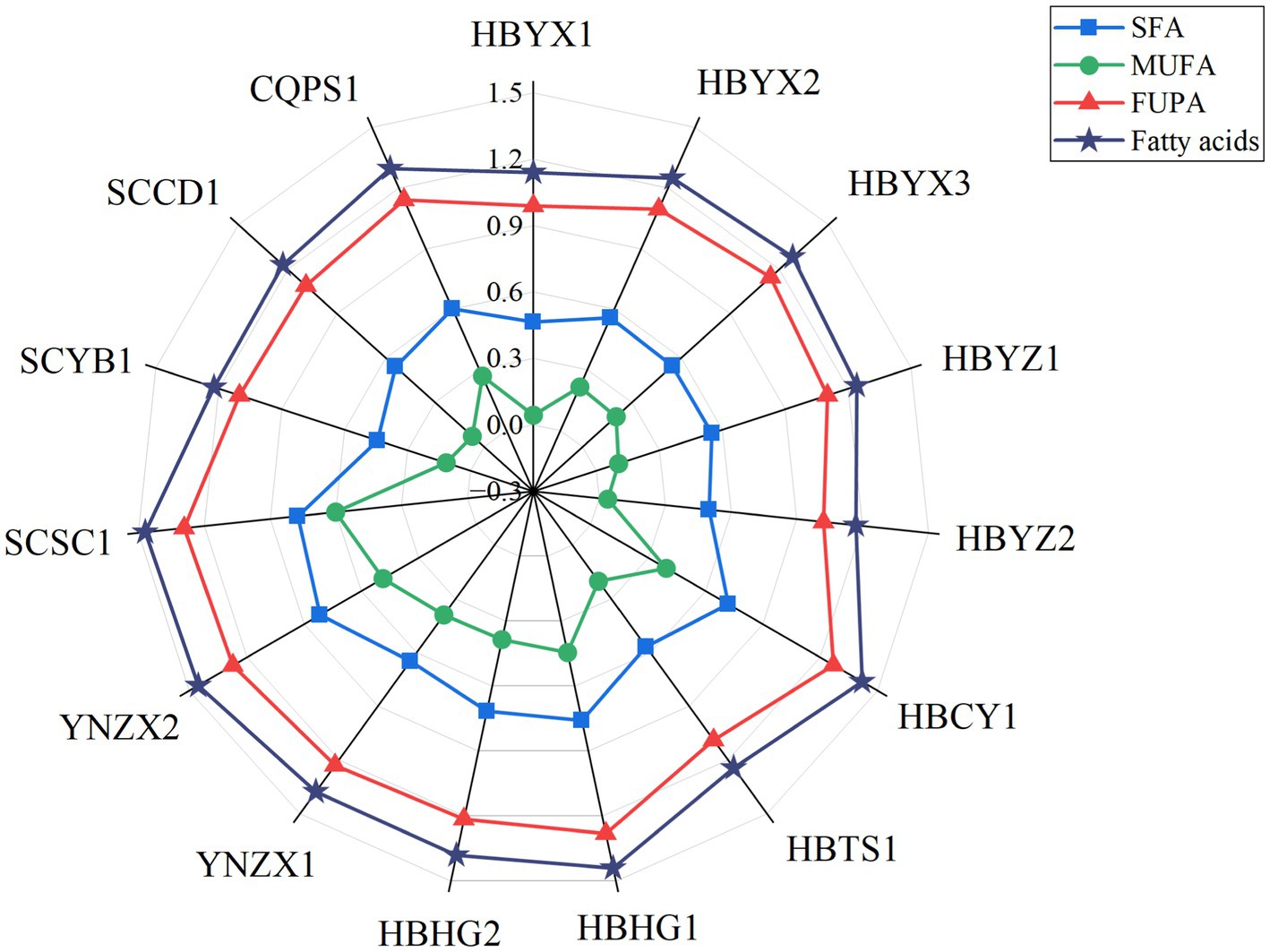
Figure 3. Radar map of SFA, MUFA, FUPA fatty acid content, and proportion in wild Idesia polycarpa. PUFA, Polyunsaturated fatty acids; SFA, Saturated fatty acids; and MUFA, Monounsaturated fatty acids.
The detailed types of fatty acids are presented in Table 4. The highest average percentage of C18:2n6c in PUFA was 98.46% with a coefficient of variation of 0.29% and the difference was not significant in the 15 wild Idesia polycarpa. The highest content of C18:2n6c was recorded in H12, H8, and H11, which was higher than 18.50/100 g. The average content of C18:3n3 was 0.20/100g, and the average proportion of C18:3n3 in PUFA was 1.42%. The highest C18:3n3 contents were found in H8, H6, and H5, which was higher than 0.02/100 g.C22:6n3 C20:2 was the remaining of PUFA which was less than 0.03/100 g. The contents and proportions of C16:0 (MN 3.64/100 g, 85.01%) and C18:0 (MN 0.45/100 g, 11.02%) were highest in SFA, while C14:0, C15:0, C17:0, C20:0, C22:0, C23:0, and C24:0 were the seven least abundant fatty acids among 9 SFA, with less than 0.05/100 g. The contents of C16:1 (MN 0.75/100 g, CV 60.93%) and C18:1n9c (MN 1.23/100 g, 37.46%) were the highest in MUFA with the largest coefficient of variation among the 17 fatty acids, while C20:1 and C22:1n9 were the least abundant fatty acids, with less than 0.03/100 g.
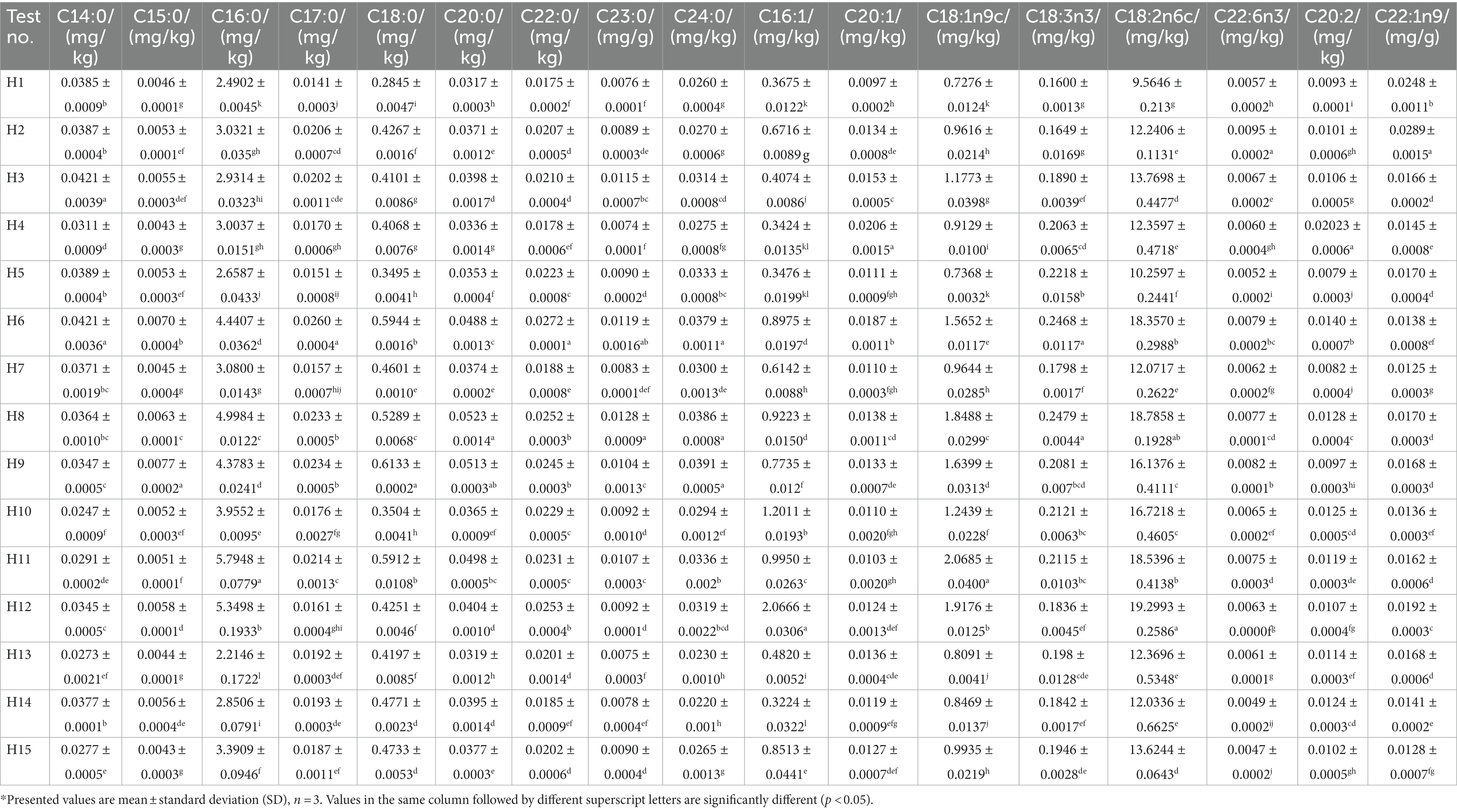
Table 4. Contents of 17 kinds of fatty acids and their relative proportions of 15 wild Idesia polycarpa.
The average percentage of n3 plus n6 in PUFA was 99.92% with a coefficient of variation of 0.03%, and the difference was not significant in the 15 wild Idesia polycarpa. This indicated a very stable proportion of beneficial fatty acids in the Idesia polycarpa. The fatty acids of n-6 instead of SFAs may prevent cardiovascular disease by reducing LDL cholesterol concentrations and may also reduce postprandial dyslipidemia in insulin-sensitive patients (Jean-Philippe et al., 2018). Xu et al. (2020) found that C18:2n6c promotes testosterone synthesis via the GPR120, ERK pathway, and consequently repairs toxic testicular reproductive damage induced by bisphenol A by feeding linoleic acid to mice. Mulligan et al. (2012) found that dietary high linoleic safflower oil preserved cardiolipin and maintained mitochondrial and cardiac contractile functions by feeding SHHF spontaneously hypertensive heart failure rats with high linoleic safflower oil, indicating the great potential of dietary intervention with dietary linoleic acid in the adjuvant treatment of heart failure associated with hypertensive heart disease. Idesia polycarpa contains large amounts of n-6 fatty acids in addition to certain amounts of α-linolenic acid and DHA, and studies have demonstrated that α-linolenic acid can improve neuroinflammation, memory impairment, and neuronal loss in rats with Alzheimer’s disease by altering the expression of α7 nAChR, NR2A, and NR2B genes in the hippocampal region of Wistar rats (Tofighi et al., 2021). DHA can inhibit lipopolysaccharide (LPS) signaling and the secretion of IL-6, an important pro-inflammatory factor, and exert anti-inflammatory effects on macrophages through regulation of the sphingolipid pathway (Jin et al., 2018), which not only affects metabolic pathways intracellularly but also acts as an extracellular signaling molecule at cell membrane receptors, thereby regulating insulin and neurological functions and promoting the accumulation of phosphatidylserine in neuronal membranes to inhibit neuronal apoptosis (Briscoe et al., 2003). The high linoleic acid content, high unsaturated fatty acids, and abundant fatty acid species suggest that Idesia polycarpa could be a potential functional vegetable oil and it was also an ideal raw material for the preparation of linoleic acid, conjugated linoleic acid, and high-quality edible vegetable oil. Therefore, Idesia polycarpa has the potential to benefit consumers as a nutritional source with great potential in the edible oil market.
3.7. Free radical scavenging ability of Idesia polycarpa
3.7.1. DPPH free radical scavenging ability of wild Idesia polycarpa
The ability of wild Idesia polycarpa antioxidants to scavenge the DPPH free radicals was measured, and the results are presented in Figure 4. The results showed that samples H12, H11, and H8 have the highest free radicals scavenging activity of 29.63, 28.42, and 27.68%, respectively, while H1 and H13 have the lowest free radical scavenging activity (14.86 and 17.49%). Among the 15 wild Idesia polycarpa, the average DPPH free radical scavenging rate was recorded as 25% at 0.1875 mg/mL level. The DPPH radical scavenging rate of wild Idesia polycarpa was 6.78–89.66% measured in the range of 0.05–2 mg/mL.
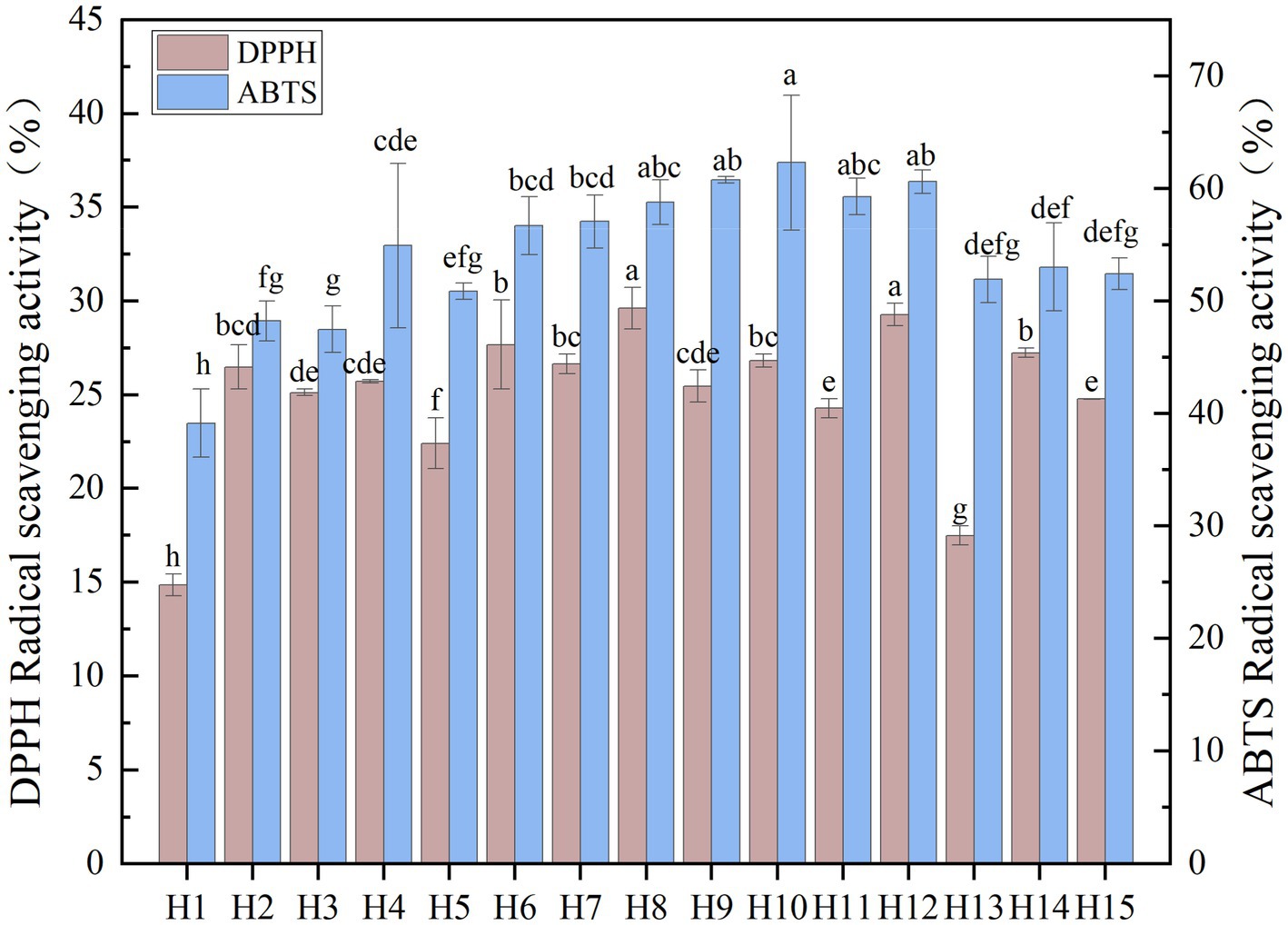
Figure 4. The oxidation resistance of 15 wild Idesia polycarpa. DPPH, 1,1-diphenyl-2-picrylhydrazyl; ABTS, 2,2′-azino-bis (3-ethylbenzothiazoline-6-sulfonic acid). Values with different letters a–h are significantly different (p < 0.05).
3.7.2. ABTS free radicals scavenging capacity of wild Idesia polycarpa
The antioxidant ABTS radicals scavenging activities of 15 wild Idesia polycarpa are presented in Figure 4. The results showed that samples H10, H9, and H12 have the highest free radical scavenging activity of 62.32, 60.77, and 60.62%, while H1, H3, and H2 have the lowest free radicals scavenging activity (39.16, 47.49, and 48.23%). The average radical scavenging activity of the remaining nine samples was recorded as 55.00% at 0.075 mg/mL level. The ABTS radical scavenging rate of wild Idesia polycarpa was 27.21–90.49% measured in the range of 0.02–0.4 mg/mL.
3.8. Correlation analysis
Oil content is key for wild Idesia polycarpa as a woody oil crop, which showed extremely significant correlations with flavonoid, C16:0, C16:1, C18:1n9c, C182n6c, and oil content, while showing significant correlations with α-tocopherol, δ-tocopherol, Ca, Mn, Zn, C18:0, and C20:0. C18:2n6c is the predominant fatty acid of wild Idesia polycarpa, which showed extremely significant correlations for oil content, TPC, C22:0, C23:0, C24:0, C16:1, C18:1n9c, C18:3n3, fatty acids, and δ-tocopherol, while showing significant correlations for TFC, C16:0, C15:0, C17:0, C18:0, C20:0, α-tocopherol, K, Ca, Mn, P, and Co (Figure 5).
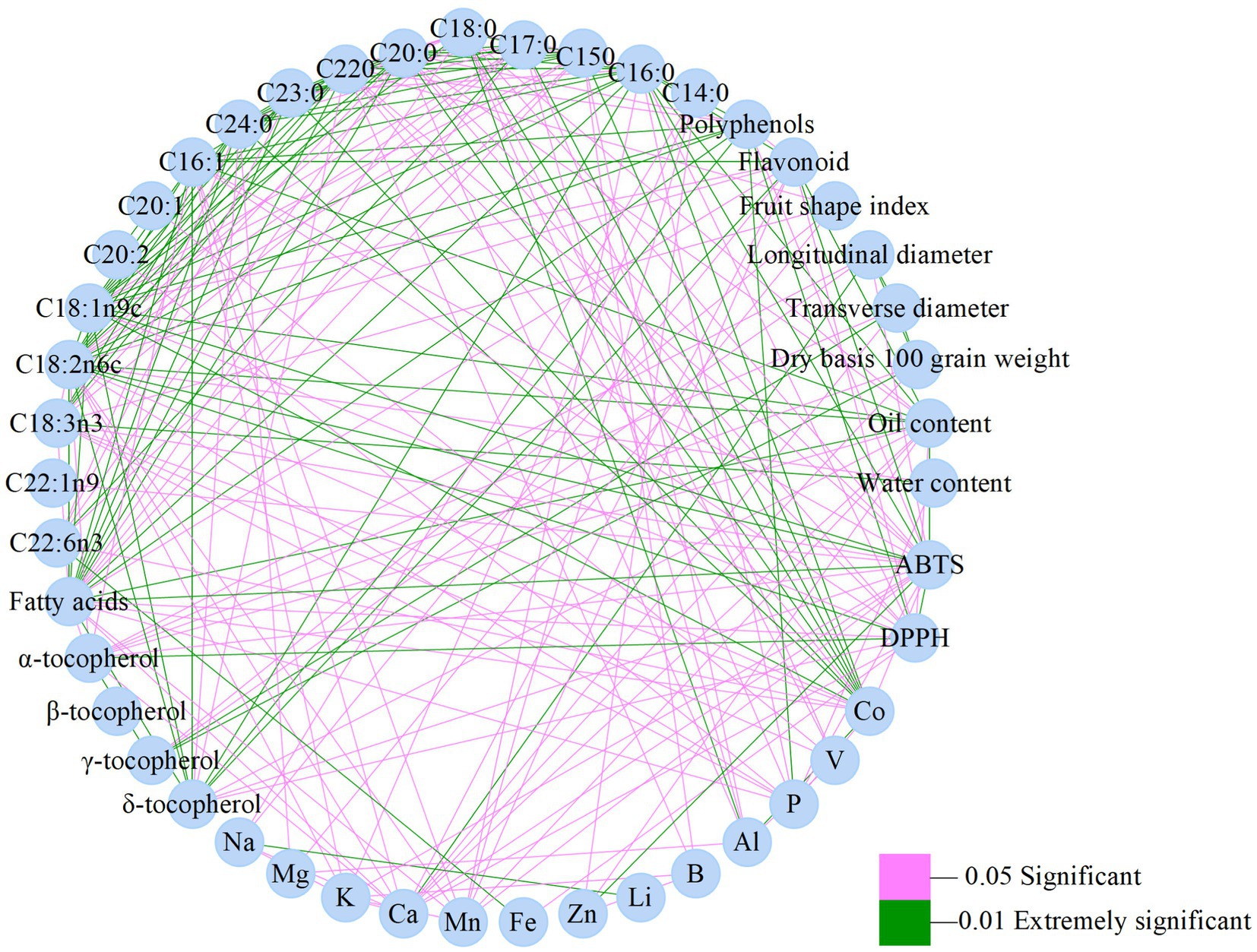
Figure 5. Visualization of the correlation among 46 detection index parameters in wild Idesia polycarpa.
From the correlation between the detection indexes, it can be obtained that the transverse diameter and the longitudinal diameter of wild Idesia polycarpa are extremely significant and positively correlated, which combined with its fruit shape traits indicates that the fruit shape of wild Idesia polycarpa is fuller; C16:0, C18:1n9c, and C18:2n6c are SFA, MUFA, and PUFA, respectively, which are extremely significant and positively correlated with the fatty acid content, indicating that the fatty acid content of wild Idesia polycarpa is mainly determined by these three fatty acids. The correlation coefficients among C16:0, C18:1n9c, and C18:2n6c were all greater than 0.9, indicating that the contents among C16:0, C18:1n9c, and C18:2n6c of wild Idesia polycarpa were more synchronized. The samples of wild Idesia polycarpa with high C16:0 content also had relatively high contents of C18:1n9c, C18:2n6c, and fatty acids. It has been reported that the IpDGAT2 was positively correlated with the rate of increase in lipid content at various growth periods of Idesia polycarpa (Fan et al., 2021), and the content of IpFAD2 was positively correlated with the concentration of unsaturated fatty acids at different growth stages of Idesia polycarpa. IpDGAT2 catalyzes the synthesis of lipids in plants, especially catalyzing the accumulation of mainly unsaturated fatty acids such as C18:2, C18:3, and C20:1. IpFAD2 catalyzes the conversion of OA to LA and plays a significant role in linoleic synthesis and accumulation and regulation of the PUFAs components at Idesia polycarpa (Fan et al., 2019), both of which were confirmed by heterologous expression in Arabidopsis thaliana.
The dry basis 100-grain weight was not related to oil content and bioactive substances, indicating that fruit quality rather than grain size and dry weight should be considered when extracting oil and bioactive substances. Li et al. (2019) evaluated the quality of Idesia polycarpa based on its fatty acid and fruit morphology, showing that the grain size of Idesia polycarpa did not affect linoleic acid content, which was consistent with the results of this study. By correlation analysis, samples with high linoleic acid content also had high polyphenols, flavonoids, and δ-tocopherol. For Idesia polycarpa, fruit size can be ignored with oil as the final product to reduce the burden of sorting and acquisition. H11 and H12 were the most typical species among the 15 samples through the correlation analysis among the indicators, combined with the characteristics of the sample’s fatty acids, tocopherols, mineral elements, and other detection indicators.
3.9. Network interaction antioxidant correlation
Antioxidant performance is an important indicator for evaluating oilseed crops and can be used to comprehensively assess quality differences. DPPH and ABTS were the most commonly used analyses for in vitro antioxidant compound activity of food. The DPPH method determines the ability of molecules to scavenge free radicals through hydrogen transfer or single electron transfer capability, in this analysis, the antioxidant reacts with DPPH by reduction, and the coloration of the sample changes from purple to yellow, while the ABTS method evaluates the compound’s antioxidant activity by capturing the ABTS radical cation and characterizing a green color in the sample (Mota et al., 2023).
Pearson’s correlation analysis is shown in Figure 6. The DPPH showed extremely significant correlations with polyphenols, C18:2n6c, C16:0, and α-tocopherol, while it was significantly correlated with crude fat content, flavonoids, C20:0, C22:0, C16:1, C18:1n9c, fatty acid content, δ tocopherol, Ca, and Fe. The ABTS showed extremely significant correlations with Crude fat content, flavonoids, C16:0, C18:1n9c, C18:2n6c, fatty acid content, and Zn, while it was significantly correlated with longitudinal diameter, polyphenols, C18:0, C20:0, C22:0, C16:1, C18:3n3, C22:1n9, α tocopherol, δ tocopherol, K, Ca, Mn, P, and Co. Among them, flavonoids, C16:0, C18:1n9c, C18:2n6c, polyphenols, C20:0, C22:0, C16:1, α-tocopherol, δ-tocopherol, and Ca were significantly correlated with both DPPH and ABTS. Fatty acids, tocopherols, polyphenols, and other beneficial trace substances are the main components of oilseed crops, which are closely related to vegetable oil quality and directly affect functionality and oxidative stability. It has been reported that in vivo antioxidant activity was correlated to polyphenol, squalene, MUFA, and PUFA, while in vitro radical scavenging capacity was significantly correlated to polyphenol, tocopherol, and squalene in 22 edible oils (Feng et al., 2022).
3.10. Cluster heat map analysis
Clustering and heat map analysis of wild Idesia polycarpa are performed by Euclidean distance method and mean distance between groups. The 45 indicators were divided into three clusters: Cluster I was the indicator represented by mineral elements such as Mn, Zn, and K; Cluster II was the indicator represented by dry basis 100-grain weight, grain size, etc.; Cluster III was the indicator represented by fatty acids and antioxidant properties. The 15 wild Idesiapolycarpa samples were divided into three clusters: cluster I, H12, H11, and H10 with high oil content and high antioxidant activity, cluster II, H9, H8, and H6 with high fatty acid content and high oil content, and Cluster III was the rest of the samples (Figure 7).
3.11. Principal component analysis
The PCA was performed on different chemical compounds and the distributions of wild Idesia polycarpa samples were presented in Figure 8. A total of 46 different components from 15 wild Idesia polycarpa samples were analyzed. When performing multivariate large sample analysis with certain correlations among variables, principal component analysis converts the measured variables into several uncorrelated but integrated indicators containing information from the original data for multivariate statistical analysis by dimensionality reduction (Naik, 2018).
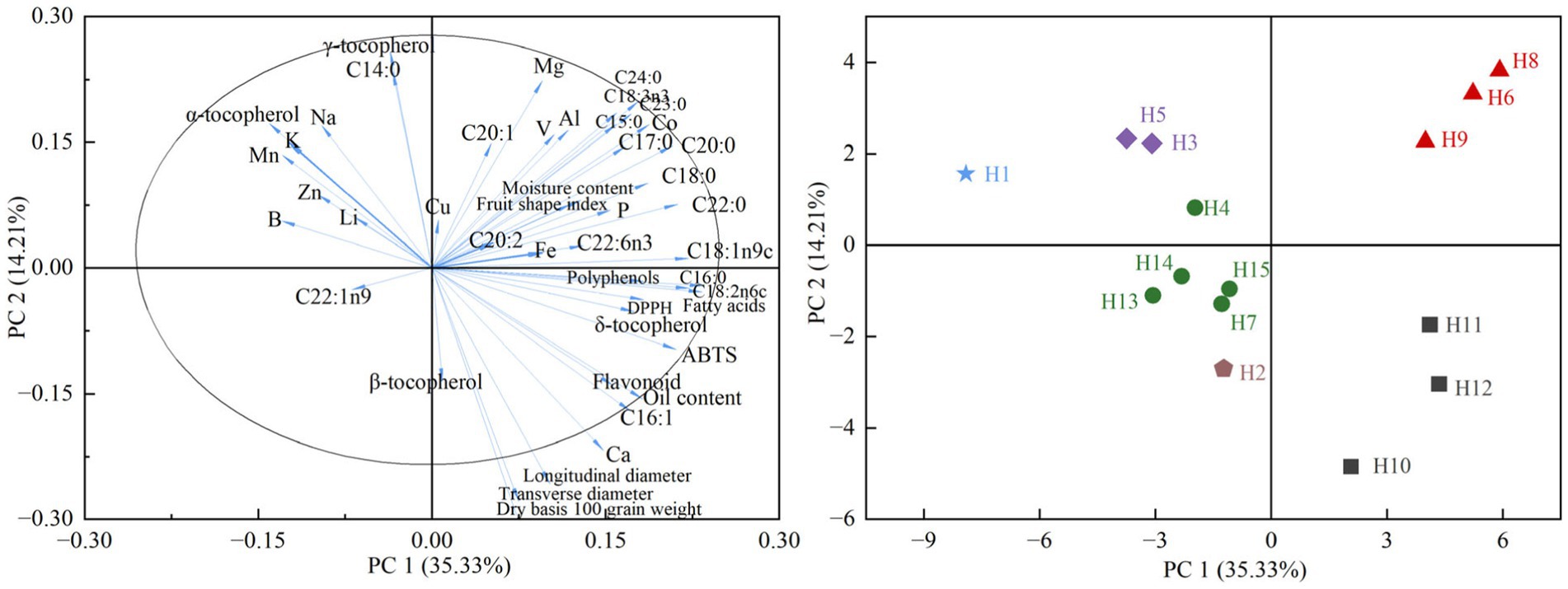
Figure 8. Principal component analysis of different material components and 15 germplasm resources of wild Idesia polycarpa. PC1, Principal component 1; PC2, Principal component 2.
The 46 indicators were concentrated and dispersed in quadrants I, II, and IV. Principal component (PC) 1 was summarized as the fatty acid richness and antioxidant activity of wild Idesia polycarpa, which was positively correlated with oil content, fatty acids, flavonoids, and ABTS, which were the most characteristic indicators of Idesia polycarpa; PC 2 was the shape characteristics and mineral element characteristics of Idesia polycarpa fruits, which were highly correlated with grain size, dry basis 100-grain weight, tocopherols, and mineral elements, which are important reference indicators for Idesia polycarpa of multipurpose development.
H8, H6, and H9 were in the first quadrant of the PCA diagram with a high positive correlation with PC1 and PC2, being the optimal sample for Idesia polycarpa as a woody oilseed crop; H10, H12, and H11 were in the fourth quadrant with negative correlation with PC1 and PC2, being the optimal samples for functional oil development; and the rest of the samples were located in the first and third quadrants which were less correlated with PC1 and PC2, while the samples with certain characteristics are the ones that can be selected with emphasis when conducting a certain characteristic product development.
4. Conclusion
In this study, the physical characteristics, nutritional values, and antioxidant properties of 15 wild Idesia polycarpa samples collected from different geographical regions in China were investigated. The present study showed that Idesia polycarpa was a good source of high-quality vegetable oil with superior nutritional value and a comparatively high content of unsaturated fatty acids and linoleic acids. In conclusion, the various chemical compositions of nutritional value were found in these 15 wild Idesia polycarpa varieties, which were rich sources of fatty acids [MN:20.89/100 g; 70.42% polyunsaturated fatty acids (MN:14.63/100 g), 20.29% saturated fatty acids (MN:4.25/100 g), and 9.29% monounsaturated fatty acids (MN:2.01/100 g)], tocopherols [MN:356.10 mg/kg (α-tocopherol (MN:284.96 mg/kg), γ-tocopherol (MN:50.20 mg/kg), β-tocopherol (MN:9.54 mg/kg), δ-tocopherol (MN:11.40 mg/kg)], mineral elements (MN:25653.44 mg/kg), flavonoids (MN:27.27 RE/kg), phenolic (MN:159.63 GAE/kg) antioxidant activities, and bioactive compounds. Among them, flavonoids, C16:0, C18:1n9c, C18:2n6c, polyphenols, C20:0, C22:0, C16:1, α-tocopherol, δ-tocopherol, and Ca were significantly correlated with both DPPH and ABTS. Furthermore, the PCA model indicated that the varieties of this sample could be characterized by clustered heat maps and it was possible to discriminate the different uses of species according to PC1 and PC2. This study will facilitate the analysis of germplasm resources of wild Idesia polycarpa and its multiple exploitation.
Data availability statement
The original contributions presented in the study are included in the article/Supplementary material, further inquiries can be directed to the corresponding authors.
Author contributions
XG: Conceptualization, Data curation, Methodology, Software, Visualization, Writing – original draft. QZ: Conceptualization, Formal analysis, Methodology, Writing – original draft. YC: Resources, Supervision, Writing – review & editing. XH: Formal analysis, Investigation, Supervision, Writing – review & editing. WY: Software, Validation, Visualization, Writing – review & editing. SoL: Investigation, Supervision, Validation, Writing – review & editing. ShL: Funding acquisition, Supervision, Writing – review & editing. KL: Formal analysis, Funding acquisition, Project administration, Writing – review & editing, Conceptualization, Resources. XX: Formal analysis, Funding acquisition, Project administration, Writing – review & editing.
Funding
The author(s) declare financial support was received for the research, authorship, and/or publication of this article. This research was funded by the Natural Science Foundation of Hubei Province (2023AFD080), the Support Plan for the Construction of High-level Teachers in Beijing Municipal Colleges and Universities (CIT&TCD 201904047), the R&D Program of Beijing Municipal Education Commission (KM202310858002), the Research on National Reference Material and Development of Natural Products in Beijing Polytechnic (SG030801), and the Key topics in science and technology of Beijing Polytechnic (2019Z002-032-KXZ).
Conflict of interest
The authors declare that the research was conducted in the absence of any commercial or financial relationships that could be construed as a potential conflict of interest.
Publisher’s note
All claims expressed in this article are solely those of the authors and do not necessarily represent those of their affiliated organizations, or those of the publisher, the editors and the reviewers. Any product that may be evaluated in this article, or claim that may be made by its manufacturer, is not guaranteed or endorsed by the publisher.
Supplementary material
The Supplementary material for this article can be found online at: https://www.frontiersin.org/articles/10.3389/fsufs.2023.1292746/full#supplementary-material
References
Abudayeh, Z. H., al Khalifa, I. I., Mohammed, S. M., and Ahmad, A. A. (2019). Phytochemical content and antioxidant activities of pomelo peel extract. Pharm. Res. 11, 244–247. doi: 10.4103/pr.pr_180_18
Briscoe, C. P., Tadayyon, M., Andrews, J. L., Benson, W. G., Chambers, J. K., Eilert, M. M., et al. (2003). The orphan G protein-coupled receptor GPR40 is activated by medium and Long chain fatty acids*. J. Biol. Chem. 278, 11303–11311. doi: 10.1074/jbc.M211495200
Canizo, B. V., Escudero, L. B., Pérez, M. B., Pellerano, R. G., and Wuilloud, R. G. (2018). Intra-regional classification of grape seeds produced in Mendoza province (Argentina) by multi-elemental analysis and chemometrics tools. Food Chem. 242, 272–278. doi: 10.1016/j.foodchem.2017.09.062
ChandKumar, P., Jain, H. R., Jain, A., and Jain, V. (2023). Flavonoids as omnipotent candidates for cancer management. S. Afr. J. Bot. 158, 334–346. doi: 10.1016/j.sajb.2023.05.025
Chen, Y. B., Li, M. D., Xia, L. X., Huang, X. F., and Luo, K. (2022). Comparison and analysis of aroma components of Idesia polycarpa maxim. (butter grape) from different varieties by solid-phase microextraction combined with GC-MS. Chin. J. Cereals Oils Assoc. 37, 163–169. doi: 10.3969/j.issn.1003-0174.2022.03.025
Chu, D. N., Zhang, Z. F., Hu, Y., Chao, F., Xu, X. D., Yuan, J., et al. (2023). Genome-wide scan for oil quality reveals a coregulation mechanism of tocopherols and fatty acids in soybean seeds. Plant Commun. 4:100598. doi: 10.1016/j.xplc.2023.100598
Cook, N. C., and Samman, S. (1996). Flavonoids—chemistry, metabolism, cardioprotective effects, and dietary sources. J. Nutr. Biochem. 7, 66–76. doi: 10.1016/S0955-2863(95)00168-9
Das, U. N. (2019). Saturated fatty acids, MUFAs and PUFAs regulate Ferroptosis. Cell Chem. Biol. 26, 309–311. doi: 10.1016/j.chembiol.2019.03.001
Dong, S. Q., Huang, X. C., Zhen, J., Van Halm-Lutterodt, N., Wang, J., Zhou, C., et al. (2018). Dietary vitamin E status dictates oxidative stress outcomes by modulating effects of fish oil supplementation in Alzheimer disease model APPswe/PS1dE9 mice. Mol. Neurobiol. 55, 9204–9219. doi: 10.1007/s12035-018-1060-6
Enengl, J., Liftinger, G., Ecklmayr, E., and Irmengard, S. (2022). Heavy metals and trace elements in soybeans cultivated in different regions of Austria, a comparison between the sampling sites and an overview of typical element contents in the soybeans. J. Trace Elem. Med. Biol. 72:126986. doi: 10.1016/j.jtemb.2022.126986
Fan, R. S., Cai, G., Zhou, X. Y., Qiao, Y. X., Wang, J. B., Zhong, H. M., et al. (2021). Characterization of diacylglycerol acyltransferase 2 from Idesia polycarpa and function analysis. Chem. Phys. Lipids 234:105023. doi: 10.1016/j.chemphyslip.2020.105023
Fan, R. S., Li, L., Cai, G., Ye, J., Liu, M. H., Wang, S. H., et al. (2019). Molecular cloning and function analysis of FAD2 gene in Idesia polycarpa. Phytochemistry 168:112114. doi: 10.1016/j.phytochem.2019.112114
Feng, S. L., Xu, X. Y., Tao, S. Y., Chen, T., Zhou, L. J., Huang, Y., et al. (2022). Comprehensive evaluation of chemical composition and health-promoting effects with chemometrics analysis of plant derived edible oils. Food Chem. X. 14:100341. doi: 10.1016/j.fochx.2022.100341
Gong, P. C., Li, D. W., Jiang, X. B., Wu, K. Y., Bai, J. J., and Peng, J. L. (2012). Variation analysis of fruit oil content of Idesia polycarpa maxim. From different populations. Acta Bot. Bras. 32, 1680–1685. doi: 10.3969/j.issn.1000-4025.2012.08.026
Hur, S. H., Kim, H. Y., Kim, Y. K., An, J. M., Lee, J. H., Kim, S., et al. (2023). Discrimination of the geographical origin of dry red pepper using inorganic elements: a multielement fingerprinting analysis. J. Food Compos. Anal. 116:105076. doi: 10.1016/j.jfca.2022.105076
Jean-Philippe, D. C., André, J. T., Marie-Claud, L., Valéry, L., Benoît, L., and Patrick, C. (2018). Substitution of dietary ω-6 polyunsaturated fatty acids for saturated fatty acids decreases LDL apolipoprotein B-100 production rate in men with dyslipidemia associated with insulin resistance: a randomized controlled trial1. Am. J. Clin. Nutr. 107, 26–34. doi: 10.1093/ajcn/nqx013
Jia, R. R., Jia, X. S., Dong, N., Tang, L., and Chen, F. (2014). Influence of diffeent mature degrees on seeds germination and quality of fruit oil of Idesia polycarpa var. vestita diels. Seed 33, 38–50. doi: 10.16590/j.cnki.1001-4705.2014.09.070
Jia, S. S., Wang, Y., Hu, J. H., Ding, Z. T., Liang, Q., Zhang, Y. F., et al. (2016). Mineral and metabolic profiles in tea leaves and flowers during flower development. Plant Physiol. Biochem. 106, 316–326. doi: 10.1016/j.plaphy.2016.06.013
Jin, J., Lu, Z. Y., Li, Y., Cowart, L. A., Lopes-Virella, M. F., and Huang, Y. (2018). Docosahexaenoic acid antagonizes the boosting effect of palmitic acid on LPS inflammatory signaling by inhibiting gene transcription and ceramide synthesis. PLoS One 13:e0193343. doi: 10.1371/journal.pone.0193343
Jung, M. H., YOO, J. M., Kang, Y. J., Lee, H. W., Kim, S. H., Sung, S. H., et al. (2010). Idesolide, an isolate of Idesia polycarpa, inhibits apoptosis through induction of intracellular heat shock protein 70 in C2C12 muscle cells. Biol. Pharm. Bull. 33, 1063–1066. doi: 10.1248/bpb.33.1063
Kim, S. H., Sung, S. H., Chio, S. Y., Chung, Y. K., Kim, J., and Kim, Y. C. (2005). Idesolide: a new spiro compound from Idesia polycarpa. Org. Lett. 7, 3275–3277. doi: 10.1021/ol051105f
Kumar, S., and Pandey, A. K. (2013). Chemistry and biological activities of flavonoids: an overview. Sci. World J. 2013:162750. doi: 10.1155/2013/162750
Lee, M., Lee, H. H., Lee, J. K., Ye, S. K., Kim, S. H., and Sung, S. H. (2013). Anti-adipogenic activity of compounds isolated from Idesia polycarpa on 3T3-L1 cells. Bioorg. Med. Chem. Lett. 23, 3170–3174. doi: 10.1016/j.bmcl.2013.04.011
Li, X.F. (2018). A kind of Idesia polycarpa sauce and its preparation method. CN107865398A. Accessed April 03, 2018.
Li, Y., Peng, T., Huang, L., Zhang, S. Y., He, Y. Y., and Tang, L. (2019). The evaluation of lipids raw material resources with the fatty acid profile and morphological characteristics of Idesia polycarpa Maxim. var. vestita Diels fruit in harvesting. Ind. Crop. Prod. 129, 114–122. doi: 10.1016/j.indcrop.2018.11.071
Li, N., Sun, Y. R., He, L. B., Huang, L., Li, T. T., Wang, T. Y., et al. (2020). Amelioration by Idesia polycarpa Maxim. Var. vestita Diels. Of oleic acid-induced nonalcoholic fatty liver in HepG2 cells through antioxidant and modulation of lipid metabolism. Oxidative Med. Cell. Longev., 2020:1208726. doi: 10.1155/2020/1208726
Liu, Y., Shen, N., Xin, H. W., Yu, L. L., Xu, Q., and Cui, Y. L. (2023). Unsaturated fatty acids in natural edible resources, a systematic review of classification, resources, biosynthesis, biological activities and application. Food Biosci. 53:102790. doi: 10.1016/j.fbio.2023.102790
Lozano-Castellón, J., Rinaldi de Alvarenga, J. F., Vallverdú-Queralt, A., and Lamuela-Raventós, R. M. (2022). Cooking with extra-virgin olive oil: a mixture of food components to prevent oxidation and degradation. Trends Food Sci. Technol. 123, 28–36. doi: 10.1016/j.tifs.2022.02.022
Ma, G. Q., Wang, Y. Y., Li, Y. F., Zhang, L. Y., Gao, Y., Li, Q., et al. (2023). Antioxidant properties of lipid concomitants in edible oils: a review. Food Chem. 422:136219. doi: 10.1016/j.foodchem.2023.136219
Martino, C., Palumbo, G., Vitullo, D., Santo, P., and Fuggi, A. (2018). Regulation of mycorrhiza development in durum wheat by P fertilization: effect on plant nitrogen metabolism. J. Plant Nutr. Soil Sci. 181, 429–440. doi: 10.1002/jpln.201700110
Mota, J. C., Almeida, P. P., Freitas, M. Q., Stockler-Pinto, M. S., and Guimarães, J. T. (2023). Far from being a simple question: the complexity between in vitro and in vivo responses from nutrients and bioactive compounds with antioxidant potential. Food Chem. 402:134351. doi: 10.1016/j.foodchem.2022.134351
Mulligan, C. M., Sparagna, G. G., le, C. H., de Mooy, A. B., Routh, M. A., Holmes, M., et al. (2012). Dietary linoleate preserves cardiolipin and attenuates mitochondrial dysfunction in the failing rat heart. Cardiovasc. Res. 94, 460–468. doi: 10.1093/cvr/cvs118
Naik, G.R. (2018). Advances in principal component analysis research and development. Intech Open [Preprint]. doi: 10.5772/intechopen.97992
National Standards of the People’s Republic of China (2017). GB 5009.3–2016, National food safety standard—Determination of moisture in food.
National Standards of the People’s Republic of China (2023). GB 5009.82–2016, National Food Safety Standard-Determination of vitamin A, D, E in food.
Nimse, S. B., and Pal, D. (2015). Free radicals, natural antioxidants, and their reaction mechanisms. RSC Adv. 5, 27986–28006. doi: 10.1039/c4ra13315c
Pan, G., Liu, R. J., Jin, Q. Z., and Wang, X. G. (2019). Comparative study of chemical compositions and antioxidant capacities of oils obtained from two species of walnut: Juglans regia and Juglans sigillata. Food Chem. 279, 279–287. doi: 10.1016/j.foodchem.2018.12.016
Presti, G., Guarrasi, V., Gulotta, E., Provenzano, F., Provenzano, A., Giuliano, S., et al. (2017). Bioactive compounds from extra virgin olive oils: correlation between phenolic content and oxidative stress cell protection. Biophys. Chem. 230, 109–116. doi: 10.1016/j.bpc.2017.09.002
Rashid, Z., Ahngar, T. A., Bashir, S., Sabina, N., Khuroo, N. S., Shabeena, M., et al. (2021). Influence of plant growth regulators and micronutrients on growth, yield and quality of Sorghum under temperate conditions. J. Exp. Agric. Int. 43, 18–23. doi: 10.9734/jeai/2021/v43i930732
Rengel, Z., Cakmak, I., and White, J. P. (2022). Marschner's mineral nutrition of plants. 4th Edn. New York, USA: Academic Press, 85–133.
Song, F.M. (2021). Extraction refining process optimization and antioxidant study of Idesia polycarpa oil. Master's degree thesis. Guizhou University, Guizhou.
Tattini, M., Pollastri, S., Fini, A., Brunetti, C., and Ferdinando, M. D. (2013). Flavonoids as antioxidants and developmental regulators: relative significance in plants and humans. Int. J. Mol. Sci. 14, 3540–3555. doi: 10.3390/ijms14023540
Tofighi, N., Asle-Rousta, M., Rahnema, M., and Amini, R. (2021). Protective effect of alpha-linoleic acid on Abeta-induced oxidative stress, neuroinflammation, and memory impairment by alteration of alpha7 nAChR and NMDAR gene expression in the hippocampus of rats. Neurotoxicology 85, 245–253. doi: 10.1016/j.neuro.2021.06.002
Ullah, A., Munir, S., Badshah, S. L., Khan, N., Ghani, L., Poulson, B. G., et al. (2020). Important flavonoids and their role as a therapeutic agent. Molecules 25:5243. doi: 10.3390/molecules25225243
Wen, L. Y., Mao, J. M., An, X. F., Yang, B., Wang, Z. R., and Kan, J. Q. (2022). Dynamic analysis and evaluation of the chemical constituents and fatty acids of Idesia polycarpa var. vestita diels fruit. Food Sci. 43, 198–207. doi: 10.7506/spkx1002-6630-20201229-330
Wu, S. W., Shi, Z. Y., Huang, M., Li, Y. J., and Gao, J. K. (2023). Effects of arbuscular mycorrhizal Fungi on leaf N: P: K stoichiometry in agroecosystem. Agronomy 13:358. doi: 10.3390/agronomy13020358
Xiang, X. W., Wen, L. Y., Wang, Z. R., Yang, G., Mao, J. M., An, X. F., et al. (2023). A comprehensive study on physicochemical properties, bioactive compounds, and emulsified lipid digestion characteristics of Idesia polycarpa var. Vestita Diels fruits oil. Food Chem. 404:134634. doi: 10.1016/j.foodchem.2022.134634
Xu, B. J., and Chang, S. K. (2007). A comparative study on phenolic profiles and antioxidant activities of legumes as affected by extraction solvents. Food Sci. 72, 159–166. doi: 10.1111/j.1750-3841.2006.00260.x
Xu, A., Li, X., Li, K., Zhang, J., Li, Y. Y., Gong, D., et al. (2020). Linoleic acid promotes testosterone production by activating Leydig cell GPR120/ERK pathway and restores BPA-impaired testicular toxicity. Steroids 163:108677. doi: 10.1016/j.steroids.2020.108677
Yang, C.F. (2008). Chemical composition and their antifungal activity from fruits of Idesia polycarpa var. vestita diels. Master's degree thesis. Northwest A&F University, Shanxi.
Yang, F. X., Su, Y. Q., Li, X. H., Zhang, Q., and Sun, R. C. (2009). Preparation of biodiesel from Idesia polycarpa var. vestita fruit oil. Ind. Crop. Prod. 29, 622–628. doi: 10.1016/j.indcrop.2008.12.004
Zhang, J. G., Wan, W. F., Yang, X. F., Li, Y., and Dong, S. D. (2021). Comparative study on fatty acid composition and squalene content of nine kinds of special woody oils in Yunnan province. Chin. J. Cereals Oils Assoc. 11, 1–14. doi: 10.3969/j.issn.1003-0174.2021.11.015
Zhao, D. Q., Li, T. T., Li, Z. Y., Sun, J., and Tao, J. (2020). Characteristics of Paeonia ostii seed oil body and OLE17.5 determining oil body morphology. Food Chem. 319:126548. doi: 10.1016/j.foodchem.2020.126548
Zhao, H. Y., and Yang, Q. L. (2022). Study on influence factors and sources of mineral elements in peanut kernels for authenticity. Food Chem. 382:132385. doi: 10.1016/j.foodchem.2022.132385
Keywords: Idesia polycarpa , edible oil, fruit characteristics, fatty acids, tocopherols, linoleic acid, antioxidant properties, principal component analysis
Citation: Guo X, Zhang Q, Chen Y, Huang X, Yang W, Li S, Li S, Luo K and Xin X (2023) A systematic study on composition and antioxidant of 15 varieties of wild Idesia polycarpa fruits in China. Front. Sustain. Food Syst. 7:1292746. doi: 10.3389/fsufs.2023.1292746
Edited by:
Liyou Zheng, Anhui Polytechnic University, ChinaReviewed by:
Sameh A. Korma, Zagazig University, EgyptJingjing Kang, Guizhou Medical University, China
Bulgariu Laura, Gheorghe Asachi Technical University of Iași, Romania
Jun Li, Henan University of Technology, China
Copyright © 2023 Guo, Zhang, Chen, Huang, Yang, Li, Li, Luo and Xin. This is an open-access article distributed under the terms of the Creative Commons Attribution License (CC BY). The use, distribution or reproduction in other forums is permitted, provided the original author(s) and the copyright owner(s) are credited and that the original publication in this journal is cited, in accordance with accepted academic practice. No use, distribution or reproduction is permitted which does not comply with these terms.
*Correspondence: Kai Luo, luokai_79@163.com; Xiulan Xin, xiulanxin@163.com
†These authors have contributed equally to this work and share first authorship