- 1Department of Biology, Norwegian University of Science and Technology, Trondheim, Norway
- 2Department of Fisheries and New Biomarine Industry, SINTEF Ocean, Trondheim, Norway
- 3Institute of Marine Ecosystem and Fishery Science, University of Hamburg, Hamburg, Germany
Introduction: Focusing on circular bioeconomy and finding solutions for managing finite and scarce resources such as phosphorus are issues that need to be addressed across sectors to attain food security. Land-based aquaculture of Atlantic salmon (Salmo salar L.) smolt and post-smolt results in a large output of aquaculture sludge. These nutrient-rich side streams from aquaculture production constitute a valuable resource for cultivation of polychaetes Hediste diversicolor. The aim of this study was to evaluate the capacity of H. diversicolor to upcycle carbon (C), nitrogen (N), and phosphorus (P) contained in aquaculture sludge from Norwegian salmon production.
Material and methods: We investigated the effect of two diets, smolt sludge and post-smolt sludge, which were supplied at four different feed levels, on the gain of C, N, and P in H. diversicolor and established uptake rates for the upcycling of these nutrients. Additionally, we examined how different feed composition (type of diet) and supply (feel level) influenced C, N, and P content as well as the elemental ratios of H. diversicolor.
Results and discussion: Polychaetes fed with both types of sludge were shown to incorporate C, N, and P from their diets into biomass. Incorporation of C, N, and P in polychaete biomass was highest at the highest feed levels, following specific growth rates. Uptake of diet C, N, and P into polychaete biomass was similar for both diets and across feed levels. On average, C and N uptake rates amounted to 2–10% and 2–15%, respectively, while values for P uptake were lower at 0.5–1.5%. C, N, and P content of polychaetes was not affected by different diets or feed levels while the C:N ratio increased with increasing feed supply. Overall, we found that H. diversicolor can successfully take up nutrients contained in aquaculture sludge and thus can be beneficial for upcycling of side streams from Atlantic salmon production.
1 Introduction
According to the United Nations (2022), the global population is projected to reach 10 billion people within the next 50–100 years. This leads to an increasing demand for food, which will result in an estimated food gap of 56% in 2050 (Searchinger et al., 2019). In 2022, the Norwegian aquaculture sector produced 1.6 million tonnes of Atlantic salmon (Salmo salar L.) and Rainbow trout (Oncorhynchus mykiss Walbaum, 1792) (Fiskeridirektoratet, 2023a), making it a significant contributor to food security on a global scale (Olafsen et al., 2012). To produce this biomass, 1.9 million tonnes of feed were consumed by the salmon industry alone (Fiskeridirektoratet, 2023b). During production, significant fractions of the phosphorus (P) as well as carbon (C), and nitrogen (N) in the feed were released to the environment through feed wastage, excretion, respiration, and feces production, namely up to 76% of feed P, 62% of feed C and 57% of feed N (Wang et al., 2012, 2013). Based on a content of 49% C, 6% N and 1.5% P in feed (Olsen and Olsen, 2008), the theoretical nutrient dispersal from Norwegian salmon production in 2022 amounted to approximately 575,000 tonnes C, 65,000 tonnes N and 21,600 tonnes P (Fiskeridirektoratet, 2023b). Maximizing utilization nutrients contained in the salmon feed is of high importance for sustainable growth of the industry since 75–94% of greenhouse gas emissions from salmon production are related to feed (Pelletier et al., 2009; Johansen et al., 2022). Additionally, P scarcity is a significant global concern with implications for food security, environmental sustainability, and resource management. P is a critical component in food production, as it constitutes an essential nutrient for animal and plant growth (Cordell et al., 2009). Today, P is extracted through mining of rock phosphate which is unsustainable due to its high environmental impact (Elser and Bennett, 2011) and the rapid depletion of phosphate rock reserves which are estimated to be exhausted by the end of the century (Sverdrup and Ragnarsdottir, 2011; Li et al., 2016). Studies suggest that P recovery and recycling could be an effective strategy to address scarcity and reduce environmental pollution (Cordell et al., 2009; Bouwman et al., 2013).
The aquaculture industry is the single largest consumer of P in Norway, and depends on continuous supply of P. Feed used for cultivation of salmon and trout contains 1–1.5% P, giving an annual consumption up to 30,000 tonnes P in feed (EWOS, 2022a,b; Fiskeridirektoratet, 2023b). One strategy for increasing access to recycled C, N, and P would be to upcycle salmon aquaculture sludge by cultivation of extractive species. Previous studies showed that these waste streams have high nutritional value and constitute appropriate nutritional qualities for cultivation of the marine polychaete Hediste diversicolor (OF Müller, 1776) (Wang et al., 2019a,b; Malzahn et al., 2023). The species has been repeatedly proven to successfully upcycle and bioaccumulate globally scarce, high value compounds such as marine proteins, lipids and fatty acids contained in aquaculture sludge (Marques et al., 2018; Jerónimo et al., 2020; Anglade et al., 2023), however, the capacity of H. diversicolor to upcycle P from aquaculture sludge is yet to be established.
Feed requirements for salmon smolt and post-smolt are different and the feeds differ in contents of lipid, protein and carbohydrates (BioMar, 2022; EWOS, 2022a,b) as well as C, N, and P. Consequently, different availability of C, N, and P in smolt and post-smolt sludge can be expected. This study aimed to investigate the potential for C, N, and P recovery from aquaculture sludge in Norway by cultivation of H. diversicolor. Further, the effect of different dietary quality (i.e., smolt- and post-smolt sludge) and varying feed levels on C, N, and P recovery from aquaculture sludge was assessed. Hereby, the hypotheses were that (1) H. diversicolor can successfully upcycle C, N, and P from aquaculture sludge, (2) H. diversicolor will have different uptake rates for different nutrients (C, N, and P), (3) the source of C, N, and P (diet) will not affect nutrient removal and recovery, and (4) uptake of C, N and P will be affected by availability (feed level).
2 Materials and methods
Collection and preparation of H. diversicolor as well as the experimental setup were shared with Anglade et al. (2023). Therefore, descriptions in 2.1 and 2.2 are similar to Ch. 2 materials and methods in Anglade et al. (2023).
2.1 Collection and preparation of Hediste diversicolor
Specimens of H. diversicolor used in the experiment were collected from sediment at low tide from the intertidal zone of the Trondheimsfjord in Buvika (63°18′49.2”N 10°10′48.7″E). Collection took place before visible sexual maturation of individuals (Anglade et al., 2023). After retrieval, polychaetes were transferred to flow-through holding tanks (52 × 36 × 18 cm L × W × H) with sufficient water flow, containing a 15 cm thick layer of sand, and acclimated in the tanks for 4 weeks at 16 L:8D and 16°C and fed commercial fish feed (GEMMA DIAMOND 1.0, Skretting AS, Norway).
2.2 Experimental setup
Hediste diversicolor was cultivated in two fully automated XR3 cultivation racks operated as flow-through systems (Aquatic habitats, Pentair plc, United States) where 16 of 20 cultivation tanks (16-L, 47 × 26 × 21 cm L × W × H) in each rack were used. Tanks had an 8 cm thick layer of sand and were stocked with 15 polychaetes each, yielding a density of 140 ind. m−2. At the start of the trial, unscathed polychaetes were retrieved from the holding tanks, cleaned from sediment, and placed in clean seawater for gut evacuation for ≥2 h before being transferred to the cultivation tanks. Additionally, 15 individuals were sampled, pooled, and stored for later analyses (“Initial polychaetes”). The initial weight of all polychaetes used in this study was registered at 250 ± 48 mg (WW) (balance: Science Education, SE622, VWR Italy). The water volume of each tank was 8 L, seawater temperature (16.3 ± 0.2°C) and salinity (34.3 ± 0.2 ppt) were regulated by the system, the flow rate was set to 8 L d−1 (100% water exchange daily) to ensure an oxygen saturation ≥95%, and the tanks were aerated through air stones placed on top of the sediment. The photoperiod was set to 16 L:8D. Dead individuals were removed from the tanks daily, and the overall survival rate ranged from 80 to 90% in all tanks (Anglade et al., 2023). Polychaetes H. diversicolor were fed two different types of sludge (diets); smolt sludge (from Lerøy AS, Belsvik) and post-smolt sludge (from LetSea AS, Dønna), at four different feed levels per diet with four replicate tanks each (2 × 4 × 4). Both types of sludge were centrifuged at 3,000 rpm for 10 min, the supernatant discarded, and the remaining sludge frozen at −20°C to be thawed a day prior to feeding. As described by Malzahn et al. (2023), feed levels were calculated based on estimated N content of diets and the established 95 mg N g−1 DW of polychaetes (Wang et al., 2019b). Accordingly, the different groups in this trial received feed doses equivalent to different percentages of the N content of polychaetes. Polychaetes fed smolt sludge (S) received 6% of polychaete N (S6), 12% N (S12), 24% N (S24), 45% N (S45), and polychaetes fed post-smolt (PS) sludge received 6% N (PS6), 13% N (PS13), 25% N (PS25), 47% N (PS47). The polychaetes were fed every second day for 30 days as described in Anglade et al. (2023).
At the end of the experiment, polychaetes were retrieved from the tanks and placed in clean seawater for ≥2 h to again allow for gut evacuation. They were rinsed and adherent water was removed by placing the worms on paper tissue; afterwards they were weighed, freeze-dried, homogenized, and stored in nitrogen at −80°C for later analyses.
2.3 Carbon, nitrogen, phosphorus gain
Following the specific growth rate (SGR) as described in Anglade et al. (2023), the Von Bertalanffy growth function (Von Bertalanffy, 1938) was used to describe the function of average C, N, and P gain of individual polychaetes in relation to the feed quantity received:
Where Gainmax (mg ind−1) is the mean value of the highest observed gain of the respective nutrients C, N, and P in our trial, k (−) is the growth coefficient, Nfed (%) is the percentage of nitrogen contained in polychaetes that was fed per day (feed level), and Gain0 (%) is the value of Nfed for which the gain is zero.
2.4 Chemical analyses and nutrient uptake
C and N in both polychaetes and the diets, smolt and post-smolt sludge, were analyzed by means of gas chromatography using an organic elemental analyzer (vario EL cube, Elementar Analysensysteme GmbH, Germany), with acetonitrile as standard.
P was oxidized with potassium peroxydisulfate (Koroleff, 1976) and analyzed photometrically as phosphate following NS-EN ISO 6878 using an autoanalyzer (Flow Solution IV, O.I Analytical).
Elemental ratios of C:N, C:P, and N:P were determined based on C, N, and P content (mg g−1 DW) and uptake of C, N, and P (%) was calculated using the formula:
Where Uptake (%) is the fraction of nutrients C, N, and P supplied that is retained by polychaetes, Amountcontained (before) is the amount of C, N, and P (mg ind−1) contained in polychaetes prior to the experiment, Amountcontained (after) is the amount of C, N and P (mg ind−1) after the trial, and Amountsupplied is the total amount of C, N, and P (mg ind−1) that was fed during the experiment.
2.5 Statistical analysis
All statistical analyses were conducted using Sigmaplot for Windows Version 14.0 (Systat Software, Inc., United States).
Shapiro–Wilk normality tests were used to assess normal distribution of data, Brown-Forsythe tests were used to check for homogeneity of variance. When comparing two groups (diets and corresponding feed levels of polychaetes fed different diets), a Welch’s t-test was used, while comparison of more than two groups was done using a one-way ANOVA with subsequent Pairwise Multiple Comparison (Holm-Sidak method). Non-normal distributed data was log-transformed or analyzed using a non-parametric Mann–Whitney Rank Sum Test when comparing two groups and Kruskal–Wallis one way Analysis of Variance on ranks for multiple comparison. One-sample t-tests were conducted to determine significant differences in C, N, and P gain as well as differences between initial and final C, N, and P content from zero. Linear regression followed by one-way ANOVA was done to evaluate the effect of C, N, and P supply on uptake of C, N, and P, of feed supply on C:N, C:P, and N:P ratio.
Statistical analysis was performed at the 95% (p < 0.05) confidence level.
3 Results
3.1 Carbon, nitrogen, and phosphorus gain in Hediste diversicolor
C, N, and P gain of the polychaetes (Figures 1–3) at the different feed levels followed the Von Bertalanffy growth function (Von Bertalanffy, 1938) with a mean maximum gain of 20.50 mg C ind−1, 3.50 mg N ind−1, and 0.39 mg P ind−1. The growth coefficient k for C, N and P gain was 0.08, 0.09, and 0.07, respectively. Gain0 for all nutrients was found at a feed level of 5.8% N fed per day.
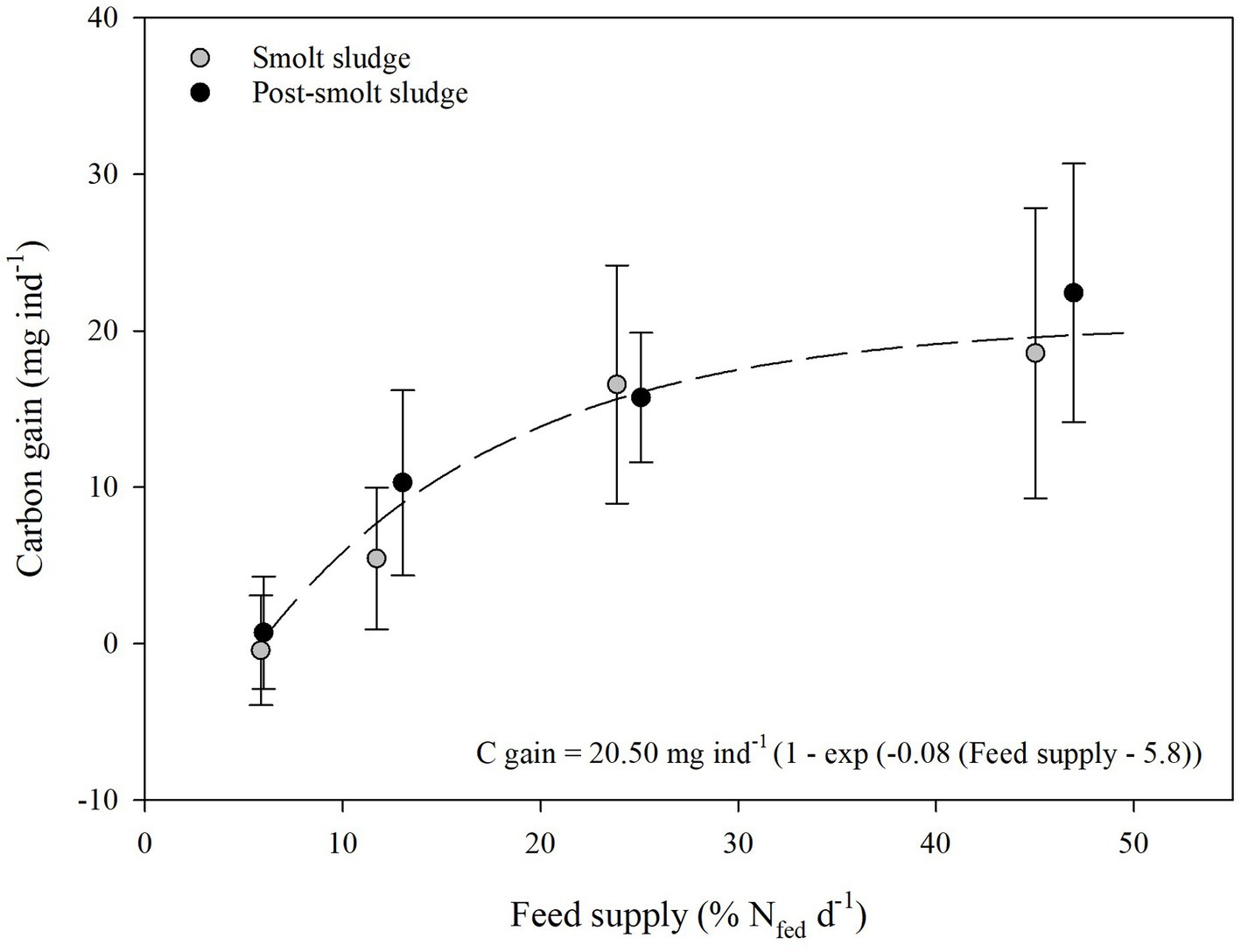
Figure 1. Carbon gain (mean ± SD, mg ind−1) of polychaetes Hediste diversicolor fed different levels of smolt sludge (S6-S24, n = 4; S45, n = 3) and post-smolt sludge (PS6-PS47, n = 4).
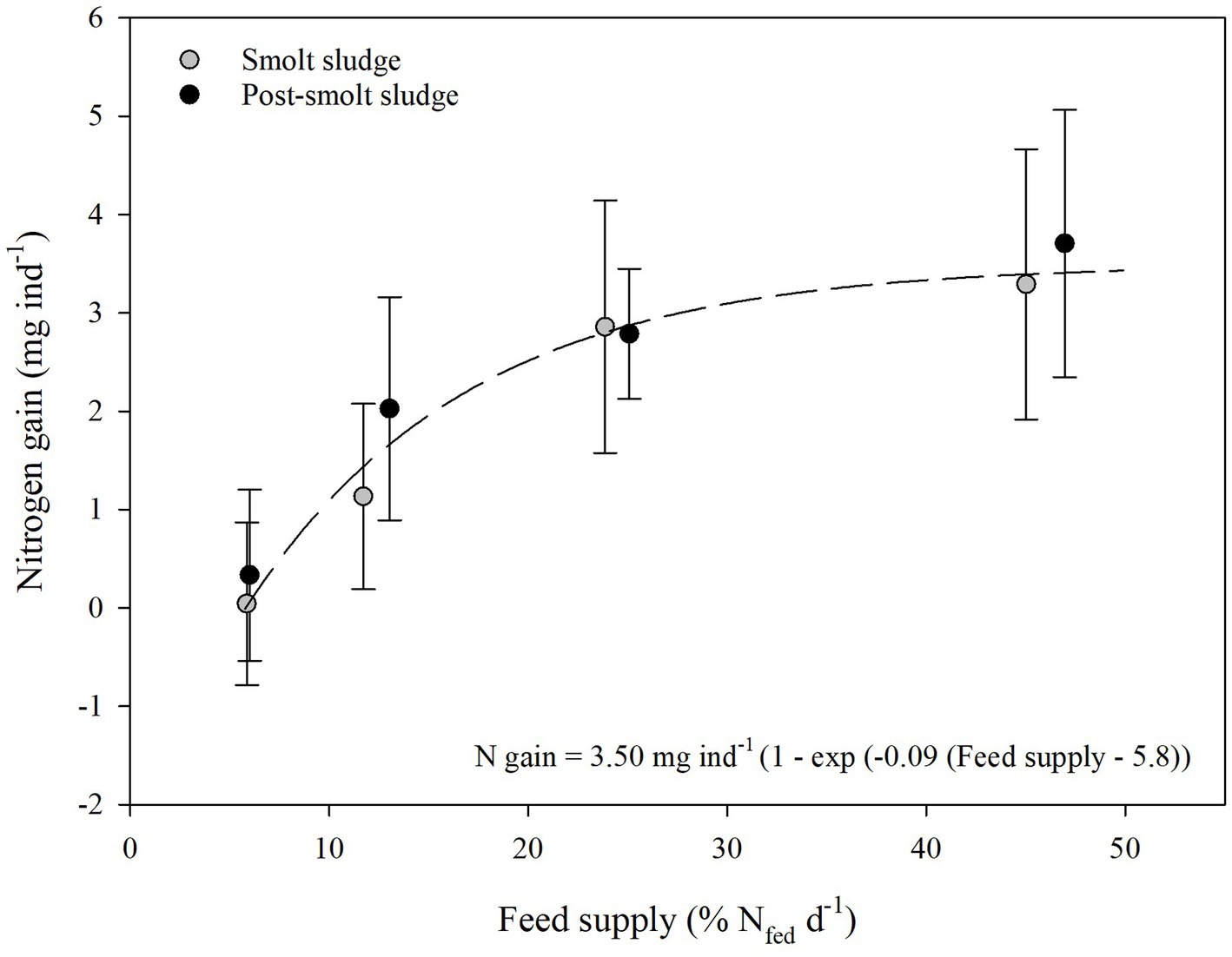
Figure 2. Nitrogen gain (mean ± SD, mg ind−1) of polychaetes H. diversicolor fed different levels of smolt sludge (S6-S24, n = 4; S45, n = 3) and post-smolt sludge (PS6-PS47, n = 4).
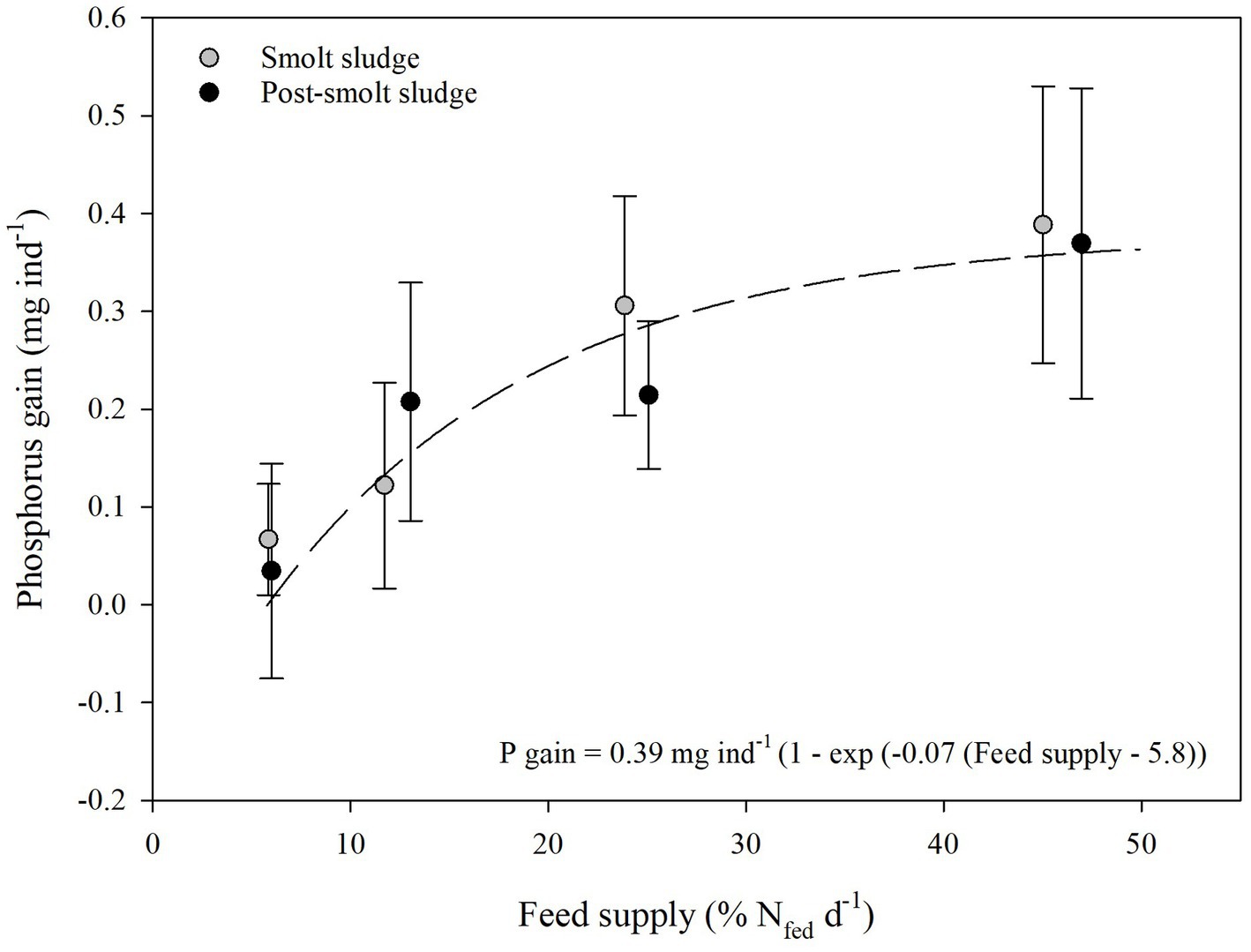
Figure 3. Phosphorus gain (mean ± SD, mg ind−1) polychaetes H. diversicolor fed different levels of smolt sludge (S6-S24, n = 4; S45, n = 3) and post-smolt sludge (PS6-PS47, n = 4).
The mean increase of C in individual polychaetes fed smolt sludge ranged from −0.4 ± 3.5 mg ind−1 (S6) to 18.6 ± 9.2 mg ind−1 (S45) (Figure 1). The C gain at the two highest feed levels S24 (16.6 ± 7.6 mg ind−1) and S45 (18.6 ± 9.2 mg ind−1) was significantly higher than at the lowest feed level S6 (−0.4 ± 3.5 mg ind−1) (one-way ANOVA, p < 0.05). C gain was significantly different from zero for treatments S24 and S45 (one-sample t-test, p < 0.05). Polychaetes fed post-smolt sludge showed a mean C gain between 0.7 ± 3.6 mg ind−1 (PS6) to 22.4 ± 8.3 mg ind−1 (PS47). C gain was significantly higher at the three highest feed levels PS13 (10.3 ± 5.9 mg ind−1), PS25 (15.7 ± 4.1 mg ind−1), and PS47 (22.4 ± 8.3 mg ind−1) than the lowest feed level PS6 (0.7 ± 3.6 mg ind−1) (one-way ANOVA, p < 0.05). Polychaetes at all feed levels except PS6 showed a C gain that was significantly different from zero (one-sample t-test, p < 0.05). There was no difference in C gain between corresponding feed levels (S6 vs. PS6, S12 vs. PS13, S24 vs. PS25, and S45 vs. PS47) of the two different diets smolt sludge and post-smolt sludge (Welch’s t-test, p ≥ 0.05).
An average increase of N between 0.04 ± 0.8 mg ind−1 (S6) and 3.3 ± 1.4 mg ind−1 (S45) was registered for polychaetes fed smolt sludge (Figure 2). Individuals at the two highest feed levels S24 and S45 had a significantly higher N gain than those at the lowest feed level S6 (one-way ANOVA, p < 0.05). For feed levels S24 and S45, the N gain was significantly different from zero (one-sample t-test, p < 0.05). Individuals fed post-smolt sludge had a mean N gain from 0.3 ± 0.9 mg ind−1 (PS6) to 3.7 ± 1.4 mg ind−1 (PS47). The N gain was higher at the two highest feed levels PS25 and PS47 than at the lowest feed level PS6. Further, the N gain of individual polychaetes was significantly different from zero at feed levels PS25 and PS47 (one-sample t-test, p < 0.05). There was no difference in N gain between corresponding feed levels of the different diets (Welch’s t-test, p ≥ 0.05).
Individual polychaetes fed smolt sludge had a mean increase in P from 0.1 ± 0.1 mg ind−1 (S6) to 0.4 ± 0.1 mg ind−1 (S45) (Figure 3). This P gain was significantly higher for polychaetes at the two highest feed levels S24 and S45 compared with the lowest feed levels S6 and S12 (one-way ANOVA, p < 0.05). Additionally, the P gain of individuals was significantly different from zero at the two highest feed levels S24 and S45 (one-sample t-test, p < 0.05). P gain for polychaetes fed post-smolt sludge ranged from 0.03 ± 0.1 mg ind−1 (PS6) to 0.4 ± 0.2 mg ind−1 (PS47). For polychaetes at the highest feed level PS47, a significantly higher P gain was recorded compared to the lowest feed level PS6. Individuals at all feed levels, except for PS6, showed a P gain that was significantly different from zero (one-sample t-test, p < 0.05). There was no difference in P gain between corresponding feed levels of the two diets (Welch’s t-test, p ≥ 0.05).
3.2 Carbon, nitrogen, and phosphorus content in diets and polychaetes
Smolt sludge had a significantly higher C content and C:N ratio than post-smolt sludge (Welch’s t-test, p < 0.05) (Table 1). The N and P content as well as the C:P and N:P ratio was not significantly different between the two diets (Welch’s t-test, p ≥ 0.05).
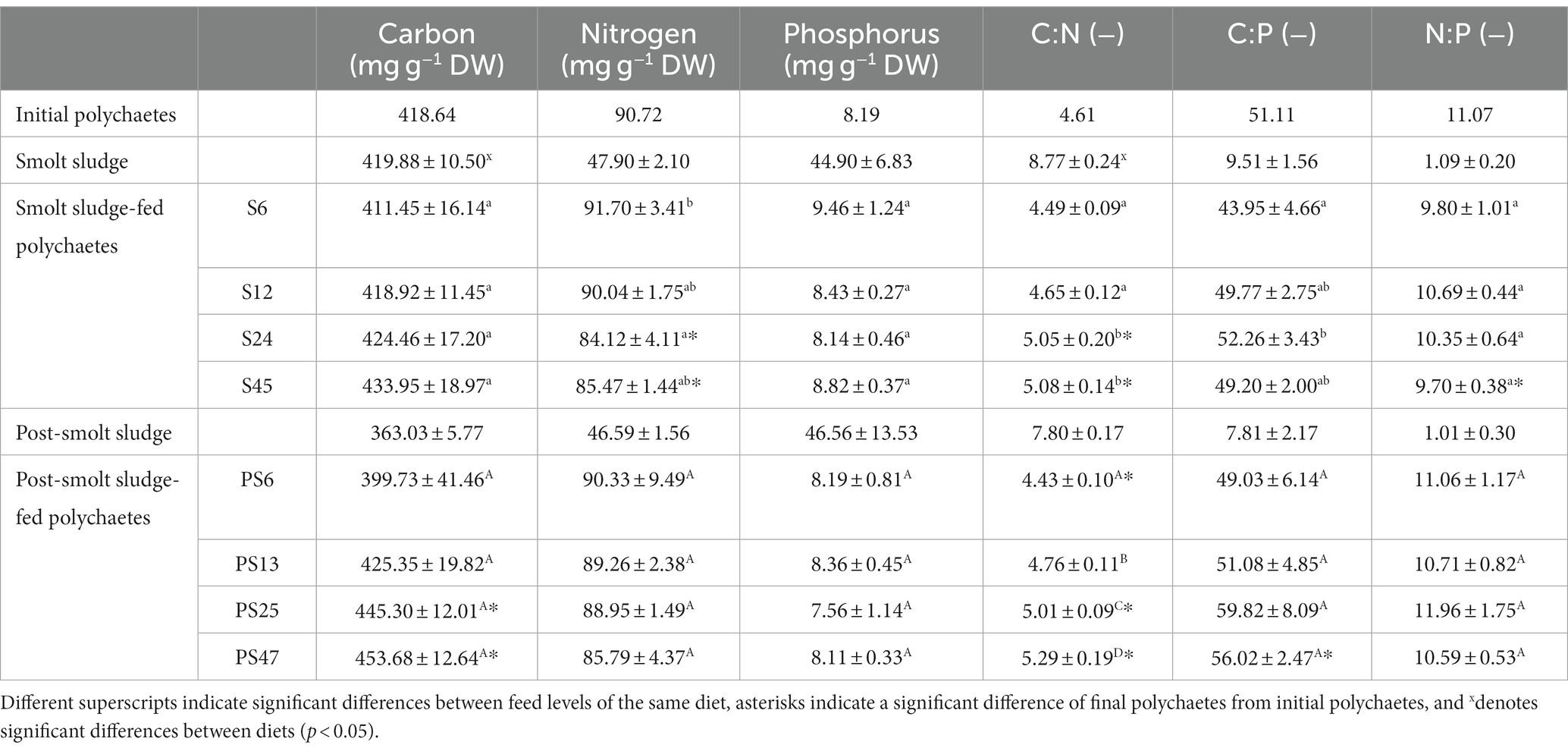
Table 1. Carbon, nitrogen, and phosphorus content (mg g−1 DW), and C:N, C:P, and N:P ratios (−) of initial polychaetes Hediste diversicolor (n = 1), diets smolt sludge (n = 3) and post-smolt sludge (n = 3), and final polychaetes Hediste diversicolor fed different levels of smolt sludge (S6-S24, n = 4; S45, n = 3) and post-smolt sludge (PS6-PS47, n = 4).
Polychaetes fed smolt sludge showed an increasing C content with increased feed supply, differences between feed levels were however non-significant (one-way ANOVA, p ≥ 0.05) (Table 1). The C content of polychaetes at all feed levels was not significantly different from initial polychaetes (one-sample t-test, p ≥ 0.05). The N content was similar for all feed levels except between feed level S6 and feed level S24 (one-way ANOVA, p < 0.05). Polychaetes fed at feed levels S24 and S45 showed a significantly lower N content than initial polychaetes (one-sample t-test, p < 0.05). P content of polychaetes fed smolt sludge was similar across feed levels (one-way ANOVA, p ≥ 0.05). The C:N ratio was significantly lower for polychaetes at the two lowest feed levels S6 and S12 compared to the two highest feed levels S24 and S45 (one-way ANOVA, p < 0.05). Additionally, the C:N ratio at the two highest feed levels was significantly higher than that of initial polychaetes (one-sample t-test, p < 0.05). Apart from a significantly different C:P ratio between feed levels S6 and S24, the C:P ratio was similar for polychaetes fed smolt sludge (one-way ANOVA, p ≥ 0.05) and not significantly different from the C:P ratio of the initial polychaetes (one-sample t-test, p ≥ 0.05). The N:P ratio was similar at all feed levels (one-way ANOVA, p ≥ 0.05). Polychaetes at feed level S45 had a significantly higher N:P ratio than initial polychaetes (one-sample t-test, p < 0.05).
The C content of polychaetes fed post-smolt sludge increased with increased feed supply, however, there was no significant difference between feed levels (one-way ANOVA, p ≥ 0.05). Polychaetes at feed level PS25 and PS47 had a significantly higher C content than initial polychaetes (one-sample t-test, p < 0.05). A slight decrease in N content with increasing feed supply was recorded, but the difference between feed levels was not significant (one-way ANOVA, p ≥ 0.05). Further, the N content of polychaetes at all feed levels was not significantly different from that of the initial polychaetes (one-sample t-test, p ≥ 0.05). P content of polychaetes fed post-smolt sludge was similar at all feed levels (one-way ANOVA) and not significantly different from P content of initial polychaetes (one-sample t-test, p ≥ 0.05). The C:N ratio of polychaetes significantly increased with each feed level (one-way ANOVA, p < 0.05). Further, we found a significantly lower C:N ratio for polychaetes at feed level PS6 than initial polychaetes, while those at feed levels PS25 and PS47 had a significantly higher C:N ratio compared with initial polychaetes (one-sample t-test, p < 0.05). The C:P ratio was similar across feed levels (one-way ANOVA, p ≥ 0.05) while the C:P ratio of polychaetes at feed level PS47 was significantly higher than that of initial polychaetes (one-sample t-test, p < 0.05). The N:P ratio of polychaetes fed post-smolt sludge was not different between feed levels (one-way ANOVA) and similar to that of initial polychaetes (one-sample t-test, p ≥ 0.05).
There was no difference between C, N, and P content, nor C:N, C:P, and N:P ratio between corresponding feed levels of the two different diets (Welch’s t-test, p ≥ 0.05).
Linear regression analysis revealed a strong positive correlation (R2 ≥ 0.65) between feed supply and C:N ratio (Figure 4). No correlation (R2 < 0.2) was found between feed supply and C:P ratio (Figure 5) or N:P ratio (Figure 6).
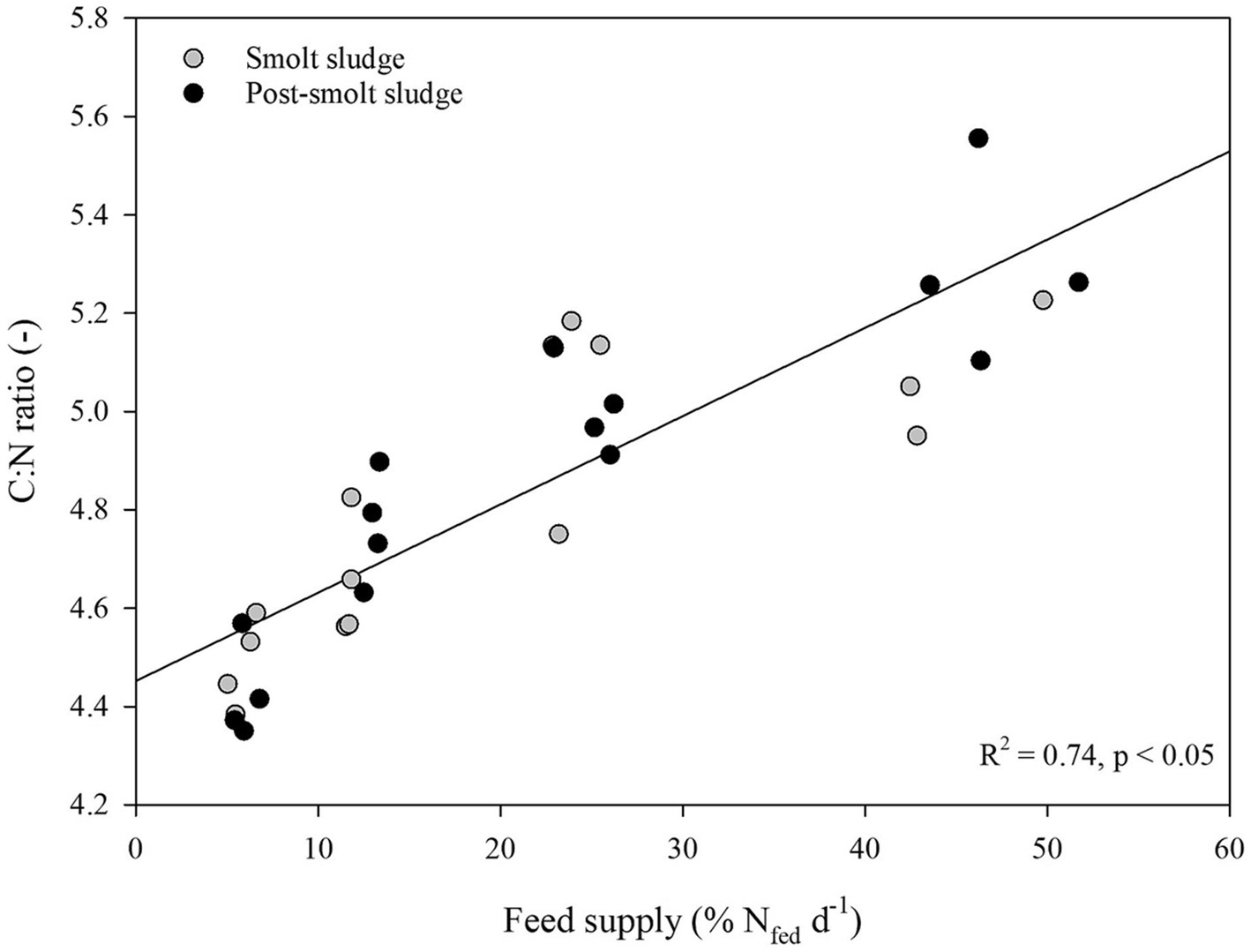
Figure 4. Linear regression analysis of C:N ratio of polychaetes H. diversicolor fed increasing levels of smolt sludge (S6-S24, n = 4; S45, n = 3) and post-smolt sludge (PS6-PS47, n = 4).
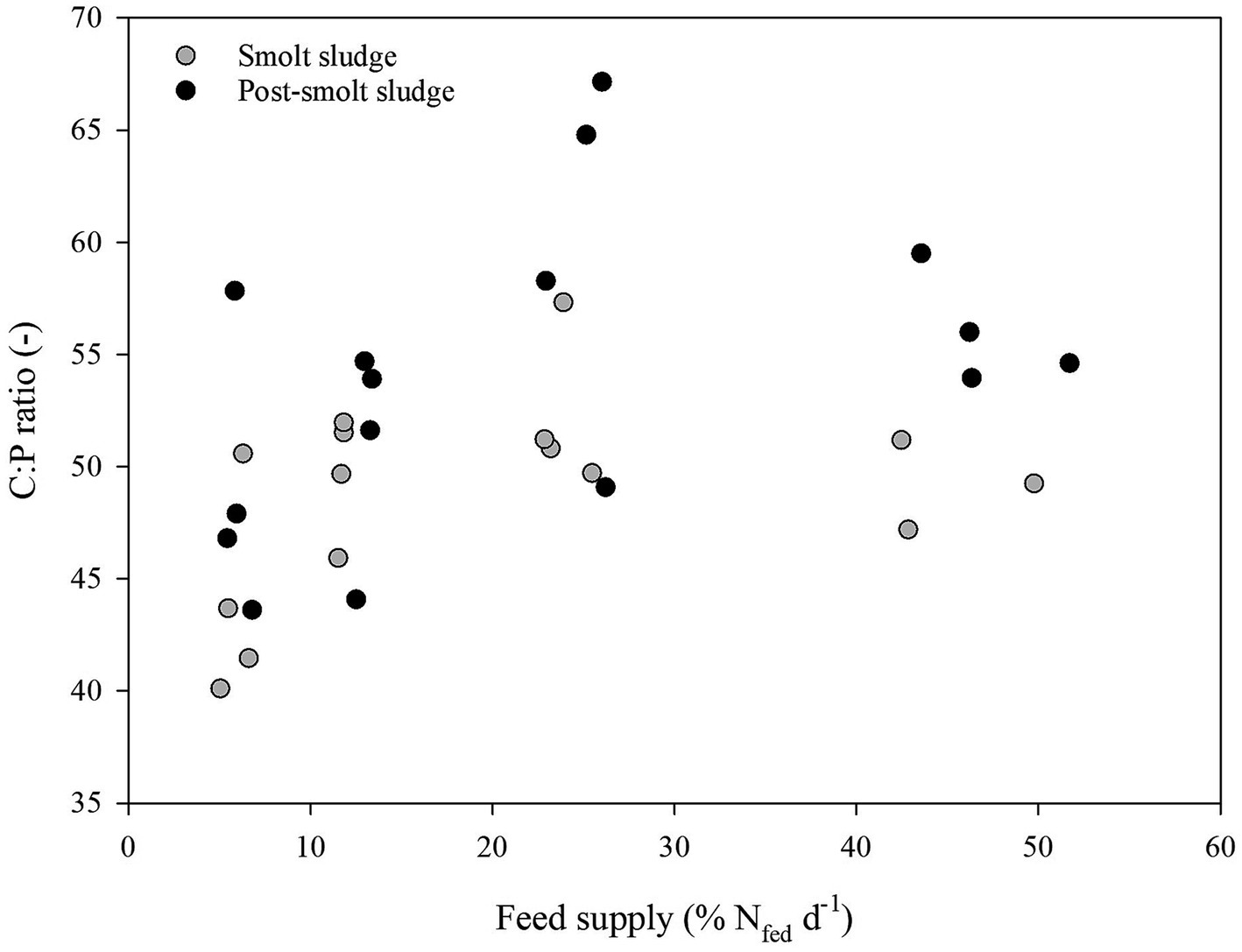
Figure 5. C:P ratio of polychaetes H. diversicolor fed increasing levels of smolt sludge (S6-S24, n = 4; S45, n = 3) and post-smolt sludge (PS6-PS47, n = 4).
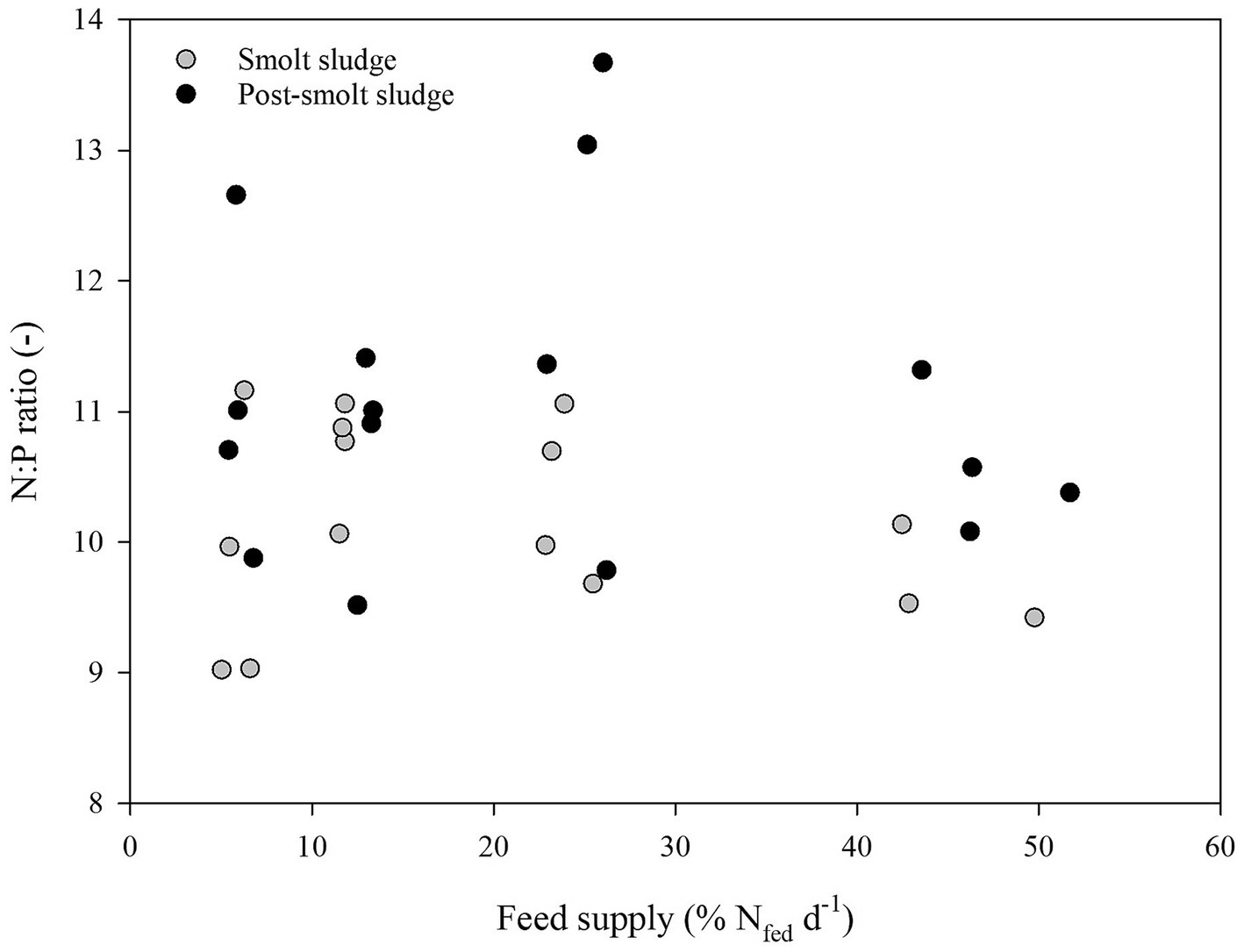
Figure 6. N:P ratio of polychaetes H. diversicolor fed increasing levels of smolt sludge (S6-S24, n = 4; S45, n = 3) and post-smolt sludge (PS6-PS47, n = 4).
3.3 Uptake of carbon, nitrogen, and phosphorus by Hediste diversicolor
For polychaetes fed smolt sludge, the C uptake rate ranged from 0.04 ± 3.5% (S6) to 5.8 ± 2.6% (S24), the N uptake rate was between 1.7 ± 7.5% (S6) and 8.8 ± 4.0% (S24), and P uptake ranged from 0.9 ± 0.8% (S6) and 1.0 ± 0.3% (S24) (Figure 7). At the lowest feed level S6, there was no difference in uptake rate of C, N, and P (one-way ANOVA, p ≥ 0.05). At feed level S12, C uptake was significantly higher than P uptake (one-way ANOVA, p < 0.05). At the highest feed levels S24 and S45, both C and N uptake was significantly higher than P uptake (one-way ANOVA, p < 0.05). No difference between C and N uptake was found for polychaetes fed smolt sludge within any of the feed levels (one way ANOVA, p ≥ 0.05). When comparing uptake of C, N, and P between feed levels, no significant difference was found with increased feed supply (one-way ANOVA, p ≥ 0.05).
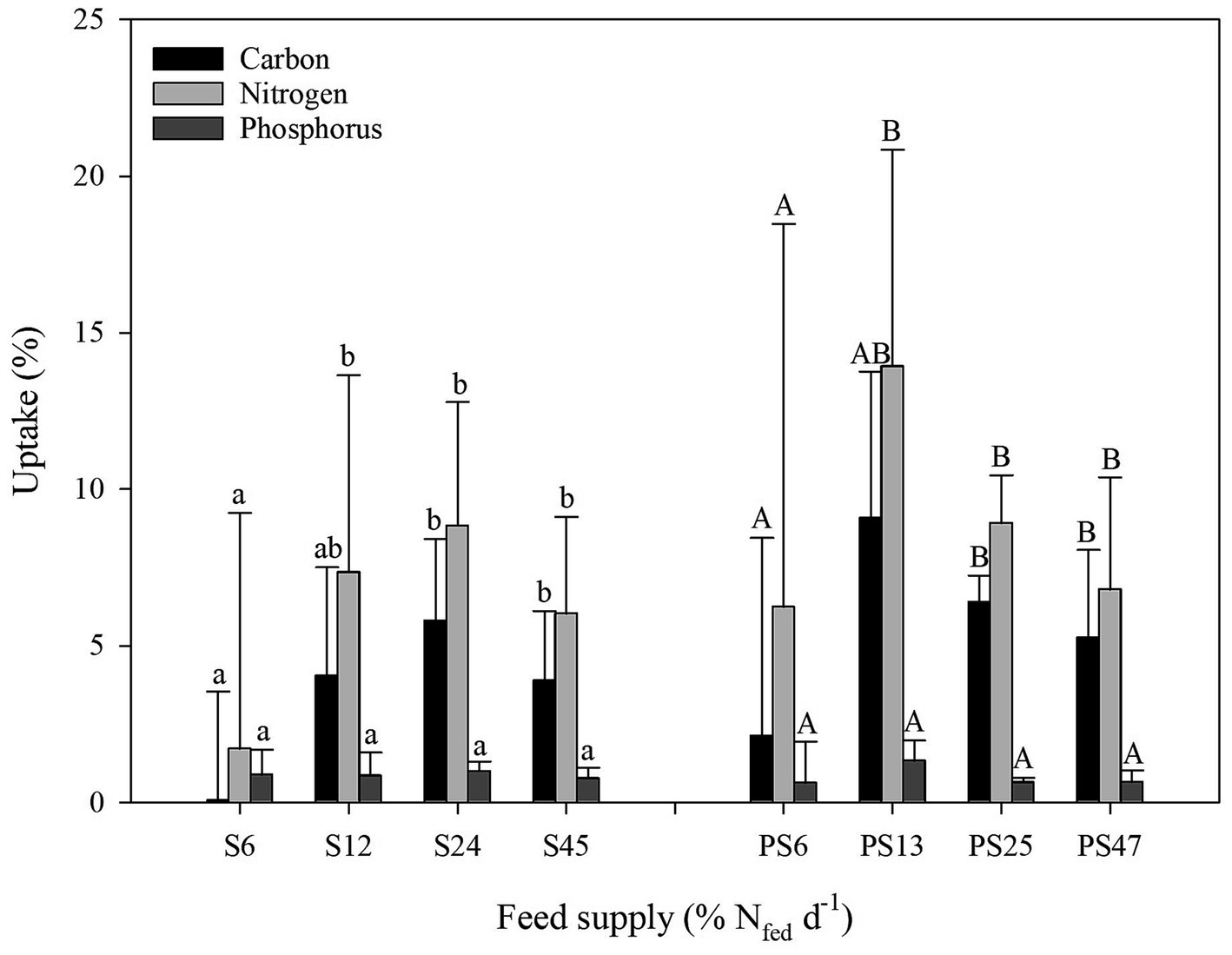
Figure 7. Uptake of carbon, nitrogen, and phosphorus (mean ± SD, %) by polychaetes H. diversicolor fed different levels of smolt sludge (S6-S24, n = 4; S45, n = 3) and post-smolt sludge (PS6-PS47, n = 4). Different lowercase superscripts indicate significant differences of C, N, and P uptake (%) within feed levels of smolt sludge-fed polychaetes, different uppercase superscripts indicate significant differences C, N, and P uptake (%) within feed levels of post-smolt sludge-fed polychaetes (p < 0.05).
The C uptake rate by polychaetes fed post-smolt sludge was between 2.1 ± 6.3% (PS6) and 9.1 ± 4.7% (PS13), N uptake ranged from 6.2 ± 12.2% (PS6) to 13.9 ± 6.9% (PS13), and the P uptake rate was between 0.6 ± 1.3% (PS6) and 1.3 ± 0.6% (PS13) (Figure 7). Uptake of C, N, and P at the lowest feed level PS6 was similar with no significant differences between the three (one-way ANOVA, p ≥ 0.05). At feed level PS13, uptake of C by polychaetes fed post-smolt sludge was significantly higher than P uptake (one-way ANOVA, p < 0.05). Both C and N uptake was higher than P uptake at the two highest feed levels PS25 and PS47 (one-way ANOVA, p < 0.05). Uptake of C and N by polychaetes fed post-smolt sludge was similar within all feed levels (one-way ANOVA, p ≥ 0.05). When comparing uptake of C, N, and P of polychaetes fed different feed levels of post-smolt sludge, no significant difference was found (one-way ANOVA, p ≥ 0.05).
Further, no significant difference was found when comparing uptake of C, N, and P of corresponding feed levels of the two different diets (Welch’s t-test, p ≥ 0.05) (Figure 7).
Linear regression analysis found no correlation (R2 < 0.2) between Carbon supplied (mg mg−1 C DW) and Carbon uptake (%) (Figure 8), Nitrogen supplied (mg mg−1 N DW) and Nitrogen uptake (%) (Figure 9), and Phosphorus supplied (mg mg−1 P DW) and Phosphorus uptake (%) (Figure 10).
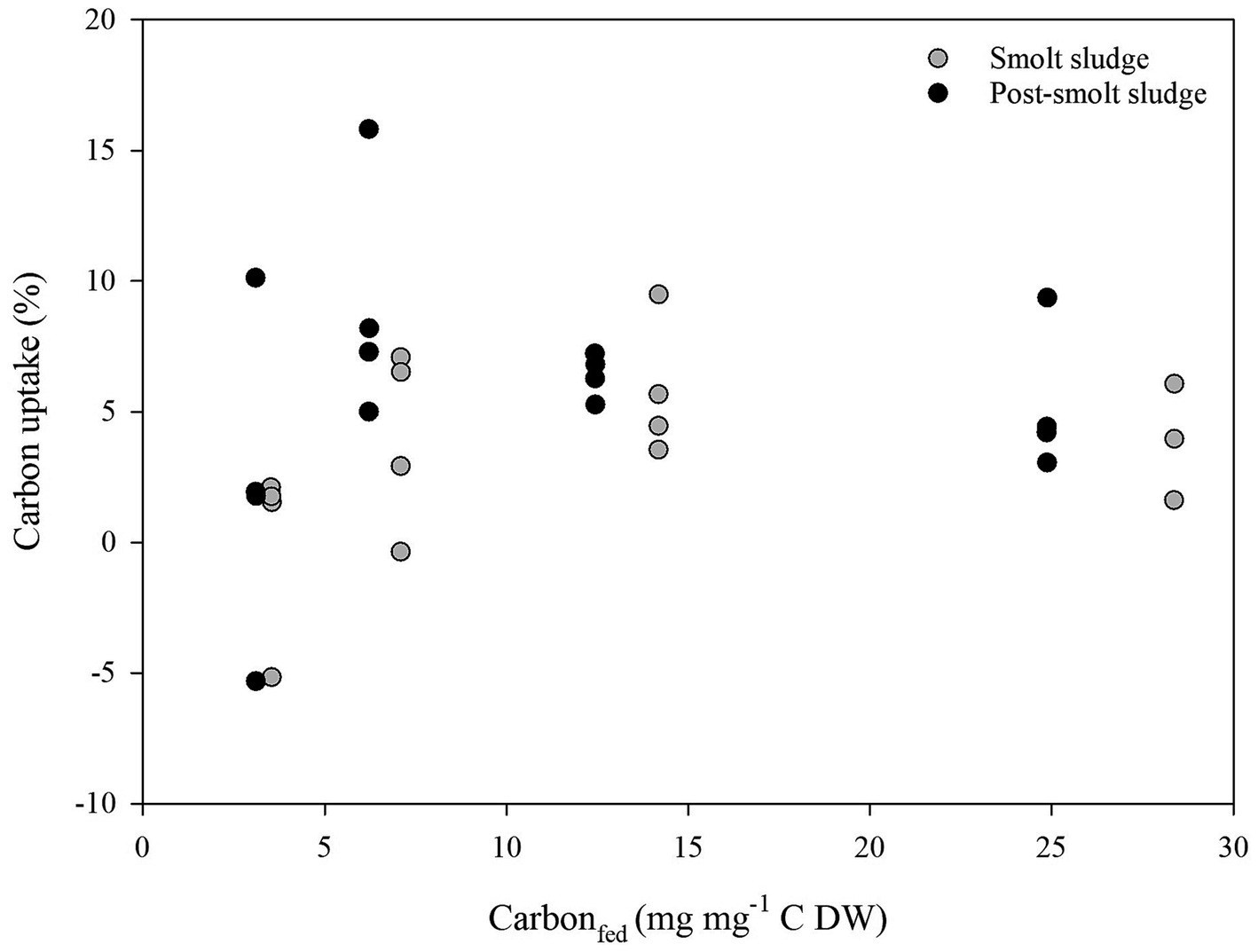
Figure 8. Scatterplot of Carbon supplied (mg mg−1 C DW) and Carbon uptake (%) by polychaetes H. diversicolor fed different levels of smolt sludge (S6-S24, n = 4; S45, n = 3) and post-smolt sludge (PS6-PS47, n = 4).
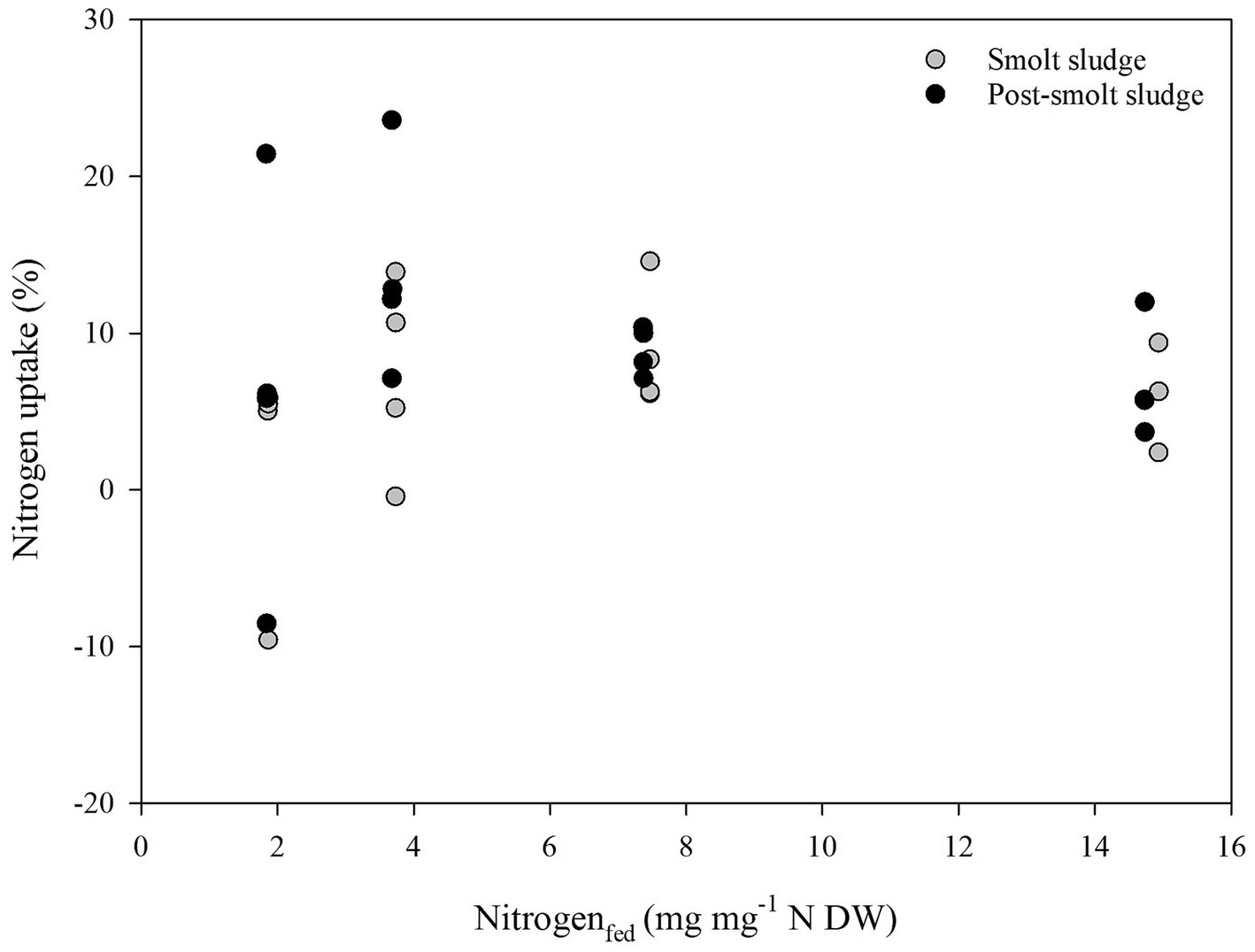
Figure 9. Scatterplot of Nitrogen supplied (mg mg−1 N DW) and Nitrogen uptake (%) by polychaetes H. diversicolor fed different levels of smolt sludge (S6-S24, n = 4; S45, n = 3) and post-smolt sludge (PS6-PS47, n = 4).
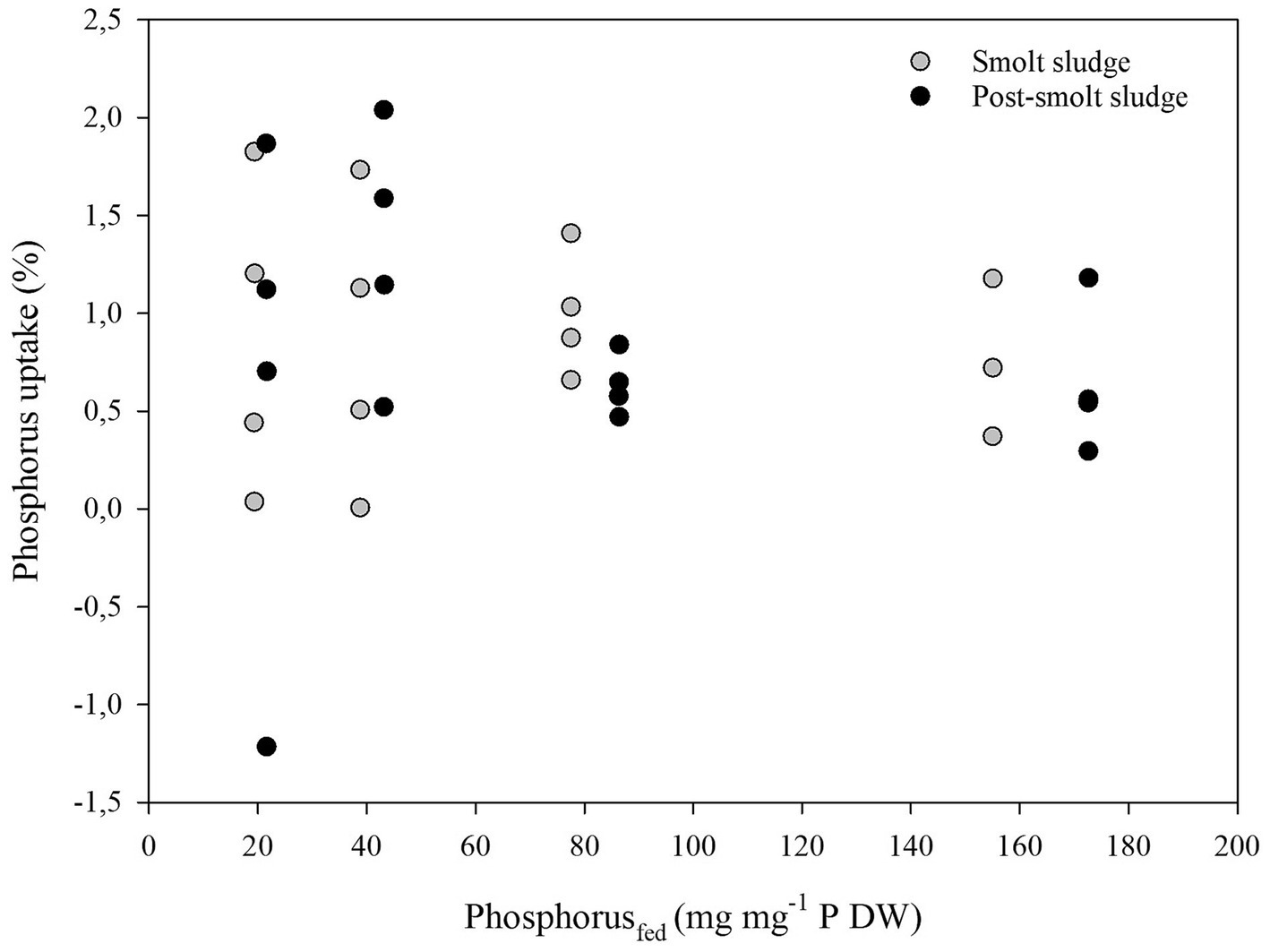
Figure 10. Scatterplot of Phosphorus supplied (mg mg−1 P DW) and Phosphorus uptake (%) by polychaetes H. diversicolor fed different levels of smolt sludge (S6-S24, n = 4; S45, n = 3) and post-smolt sludge (PS6-PS47, n = 4).
4 Discussion
Our results show that juveniles and adults of H. diversicolor could successfully be cultivated with both smolt sludge and post-smolt sludge as a sole feed source. Generally, the nutrient content and correlated elemental ratios of organisms, such as H. diversicolor, provide a good indication of their dietary nutrient requirements (Sterner and Schulz, 1998; Wagner et al., 2013). C content of polychaetes ranged at 400–450 mg g−1 DW, N content was approximately 90 mg g−1 DW, and P content was around 8 mg g−1 DW which is comparable to values found by other studies (Sterner and Elser, 2003; Palmer et al., 2014; Wang et al., 2019b). Accordingly, the C, N, and P gain we found in our study, with C having the highest gain, followed by N, and P having the lowest gain, reflect the nutritional requirements of polychaetes that were established by Nederlof et al. (2020). Differences in gain between polychaetes fed at the different feed levels, S6-S45 and PS6-PS47 followed the Bertanlanffy growth function (Von Bertalanffy, 1938) and can be attributed to differences in SGRs of the specimens. C, N, and P gain as well as the SGR had similar growth coefficients, thus the functions to describe them had a similar shape. Increases in SGRs of the polychaetes are reflected in increases in C, N, and P gain of H. diversicolor in both diet groups, with no significant growth and gain at the lowest feed levels S6 and PS6 and a levelling off of growth as well as C, N, and P gain of polychaetes in both diet groups at the highest feed levels, S24/PS25 and S45/PS47 (Anglade et al., 2023). A saturation threshold was reached at which the growth of individuals and the gain of C, N, and P showed no further positive correlation with increased feed supply. This is due to limitations tied to the organisms’ behavioral patterns and physiological attributes, including time spent on feeding, nutrient uptake, and metabolic rate (Brown et al., 2004).
Accordingly, differences in content of C, N, and P between the different feed levels and when comparing to initial polychaetes were not as pronounced as differences in net gain, which suggests that quantities of feed supply had only a limited effect on the C, N, and P content of H. diversicolor. Moreover, a significantly higher C content of smolt sludge compared to post-smolt sludge did not have a significant effect on the C content of polychaetes as there was no difference between corresponding feed levels of the two diets. Even though content of C, N, and P was similar in all polychaetes, we did register a significant effect on C:N ratio caused by the slight increase in C content and the simultaneous decrease in N content. The C:N ratio was strongly correlated with feed supply and increased significantly between the two lowest feed levels S6/S12 and the two highest feed levels S24/S45 for polychaetes fed smolt sludge and increased significantly with each feed level for polychaetes fed post-smolt sludge, even when SGRs levelled off. Since higher content of total lipids and a constant protein content in tissues correspond to a higher C:N ratio, as lipids are C-rich molecules with low N content (Barnes et al., 2007), this increase in C:N ratio in the polychaetes can be attributed to a relative increase in lipids as found by Anglade et al. (2023). Lipids provide an efficient way to store energy and when N is not limiting, which results in a low C:N ratio in the diet, marine organisms have sufficient N for essential processes such as growth and reproduction which allows them to divert resources towards lipid synthesis and storage (Luis and Passos, 1995; García-Alonso et al., 2008). The C:N ratio of smolt sludge was significantly higher than that of post-smolt sludge which may have resulted in a higher lipid storage of polychaetes fed with post-smolt sludge and, as a result, more pronounced differences of C:N ratio between each feed level for polychaetes fed post-smolt sludge (Anglade et al., 2023). Additionally, polychaetes fed post-smolt sludge at the highest feed levels, had a significantly higher C content when compared with initial polychaetes, which further supports this argumentation. On the other hand, research shows that organisms that experience periods of limited food availability or exhaust energy storages for search for feed or survival in more challenging N-limited conditions where the C:N ratio is higher, tend to prioritize N allocation for essential functions such as protein synthesis. As a result, lipid storage may be reduced, and the C:N ratio may be lower (Nielsen, 1997) which we observed for polychaetes fed at the lowest feed levels in this study.
Although previous studies have given promising results with regards to removal and upcycling of C, N, and P from aquaculture effluent using polychaetes (Pajand et al., 2017; Yousefi-Garakouei et al., 2018), uptake rates of C, N, and P in the present study showed that only small fractions of the supplied nutrients were converted into polychaete biomass. We found different uptake rates when comparing the three different nutrients, with H. diversicolor converting C and N to a similar degree regardless of feed level and the uptake of P being significantly lower than that of C and N at the highest feed levels S24/PS25 and S45/PS47, highlighting the mismatch between the requirement for C, N and P and the proportions in which these were available in the sludges. Linear regression analysis found no correlation between C, N, and P supply and the respective uptake rate and there was no significant difference between feed levels in either of the diet groups. The slightly different composition of smolt sludge and post-smolt sludge did not affect uptake rates as there was no difference between the corresponding feed levels. Contrary to our expectations, that low resource availability at the lowest feed levels S6 and PS6 would result in a higher fraction of the nutrients being taken up, uptake was not significantly higher than at higher feed levels with more feed availability. A potential explanation could be that energy was spent on searching for feed and that any feed consumed was exhausted for basic metabolic processes rather than growth as described by Anglade et al. (2023), and subsequently, no significant levels of C, N, or P were incorporated in polychaetes biomass. On the other end of the spectrum, we expected a decrease of uptake rate in all nutrients at the highest feed level due to an oversupply of C, N, and P, however, this was not registered. With regards to C, this could partly be attributed to continuous storage of lipids, even when growth levelled off. Generally, there is some uncertainty in our data due to a low number of replicates and the correlated high variability in calculated uptake rates which makes it difficult to detect significant differences. Moreover, it is important to note that the registered uptake rates exclusively quantify the percentage of C, N, and P was taken up and allocated for growth, and thus registered in polychaete biomass. However, prior studies have documented that a considerable fraction of ingested nutrients by different polychaete species are utilized for metabolic processes and have further shown that the proportion allocated towards growth can vary substantially depending on individual variation, feeding quantities, temperature, and physiological state of the organism (Fang et al., 2016; Nederlof et al., 2020). Additionally, there is a lack of knowledge on the quantity of the supplied feed that was ingested and how much was left uneaten, as well as the quantity and quality of feces produced by polychaetes in our study. Assessing these factors would give a better understanding of the actual utilization of nutrients and hence the capability of H. diversicolor to upcycle nutrients from aquaculture sludge. While the actual utilization rates of C, N, and P are likely to be significantly higher than the uptake rates that we registered in the polychaetes (Nederlof et al., 2020), they still give an indication of the approximate upcycling potential. Both the stable contents of C, N, and P in polychaetes as well as C:P and N:P ratios, with only minimal changes with increased feed supply, and the uptake rates that seemed independent of feed level, suggest that while H. diversicolor converts the nutrients contained in aquaculture sludge successfully, there is no selective enrichment apart from lipids (Wang et al., 2019a; Anglade et al., 2023).
Smolt sludge and post-smolt sludge have been shown to be suited for cultivation of H. diversicolor, however, according to our results, nutrient upcycling seems fairly inefficient due to suboptimal composition of the sludge. The P content of aquaculture sludge which is impacted by vegetable ingredients in the salmon feed diet constitutes a major challenge. While the composition of salmon feed can vary depending on factors such as the life stage of the fish and correlated nutritional requirements, as well as the feed manufacturer’s formulation strategy, typical salmon feed in Norway contains around 40% vegetable protein sources and 20% vegetable oils (Aas et al., 2022). Vegetables, grains, and legumes used in salmon feed formulation contain P in the form of phytic acid and its salt phytate, which is not bioavailable to monogastric animals such as salmonids (Eeckhout and De Paepe, 1994; Hardy, 2010; Kumar et al., 2012). Additionally, phytate is an anti-nutritional factor that can chelate with dietary minerals to form complex insoluble salts which adversely affects the absorption and digestion of these minerals as well as others (Papatryphon et al., 1999; Carter and Sajjadi, 2011). Since salmon has limited endogenous production of phytase to break down phytate, P present in vegetable ingredients is poorly digested and utilized by the species (Sajjadi and Carter, 2004; Denstadli et al., 2006). Efforts of phytase inclusion in salmon feed have been successful to a small extent (Carter and Sajjadi, 2011; Lemos and Tacon, 2017) but as our analysis shows, aquaculture sludge from both smolt and post-smolt production still contains high levels of P, presumably in the form of phytate. H. diversicolor, as salmon and most marine organisms, can be assumed to have a low digestive capacity of phytate contained in aquaculture sludge. However, the digestive system of polychaetes often harbors a range of bacteria such as Bacillus cereus, Bacillus subtilis, and Bacillus pumilus (Priscilla et al., 2022) which can produce phytase that breaks down phytate and releases phosphorus which could then be potentially absorbed by the specimens (Powar and Jagannathan, 1982; Hill et al., 2007; Sanni et al., 2023). Phytate digestion by H. diversicolor is however not well-studied, and further research is needed to explore the role of microorganisms in phytate digestion and the overall nutrient utilization in these organisms.
Regardless of the potential capability of polychaetes to break down phytate, the composition of smolt and post-smolt sludge is not ideal for H. diversicolor as discussed previously. An approach to create a more balanced diet that is better suited for H. diversicolor in terms of elemental ratios could be the mixing of aquaculture sludge with waste streams from other industries that are rich in C and N. As an example, spent grains in brewery waste consist to a large degree of cellulose and protein and thus have a high C content and N content (Verni et al., 2020), while spent yeast from beer production has a high N content (Jaeger et al., 2020). Traditionally, these waste products are used as terrestrial animal feed, for biogas production or as substrate for cultivation of microorganisms (Xiros and Christakopoulos, 2012; Čater et al., 2015; Jaeger et al., 2020) and could in combination with aquaculture sludge, and with appropriate pre-treatment, potentially serve as resource for cultivation of H. diversicolor which would result in a polychaete diet with a more balanced nutritional composition than smolt sludge and post-smolt sludge alone.
5 Conclusion
Our results confirmed the hypotheses that H. diversicolor could successfully upcycle nutrients C, N, and P contained in aquaculture sludge. Hereby, we showed that the species has different requirements for C, N, and P which was established by the differences in both gain and uptake of these nutrients. Uptake of C and N was significantly higher than that of P at the highest feed levels. The source of C, N, and P, meaning smolt sludge and post-smolt sludge, was not found to have an effect on the uptake, as uptake was similar for polychaetes fed different diets, even though these diets had a slightly different composition. Finally, we could not confirm that uptake of C, N, and P by H. diversicolor was affected by different feed supply within the scope and limits of our study, as there was no significant difference between feed levels.
Data availability statement
The raw data supporting the conclusions of this article will be made available by the authors, without undue reservation.
Author contributions
IA: Conceptualization, Formal analysis, Investigation, Writing – original draft, Writing – review & editing, Data curation, Visualization. BK: Formal analysis, Investigation, Writing – review & editing. TD: Formal analysis, Investigation, Writing – review & editing. AH: Conceptualization, Investigation, Writing – review & editing, Funding acquisition. AM: Conceptualization, Funding acquisition, Investigation, Project administration, Supervision, Writing – review & editing. KR: Conceptualization, Funding acquisition, Investigation, Project administration, Supervision, Writing – review & editing.
Funding
The author(s) declare financial support was received for the research, authorship, and/or publication of this article. This work was funded by the RCN projects POLYCHAETE (#280836), MIND-P (#268338), and the H2020 ERA-NET BlueBio COFUND Project “SIDESTREAM – secondary bio-production of low trophic organisms utilizing side streams from the Blue and Green sectors to produce novel feed ingredients for European aquaculture” (grant ID 68), co-funded through national funds provided by the Norwegian Research Council (#311701). The project was carried out in the framework of the national research infrastructure “Norwegian Center for Plankton Technology” (Norwegian Research Council #245937/F50) hosted by SINTEF and NTNU.
Conflict of interest
The authors declare that the research was conducted in the absence of any commercial or financial relationships that could be construed as a potential conflict of interest.
Publisher’s note
All claims expressed in this article are solely those of the authors and do not necessarily represent those of their affiliated organizations, or those of the publisher, the editors and the reviewers. Any product that may be evaluated in this article, or claim that may be made by its manufacturer, is not guaranteed or endorsed by the publisher.
References
Aas, T. S., Åsgård, T., and Ytrestøyl, T. (2022). Utilization of feed resources in the production of Atlantic salmon (Salmo salar) in Norway: an update for 2020. Aquacult. Rep. 26:101316. doi: 10.1016/j.aqrep.2022.101316
Anglade, I., Dahl, T. H., Kristensen, B. S., Hagemann, A., Malzahn, A. M., and Reitan, K. I. (2023). Biochemical composition of Hediste diversicolor (OF Müller, 1776) (Annelida: Nereidae) reared on different types of aquaculture sludge. Front. Mar. Sci. 10:899. doi: 10.3389/fmars.2023.1197052
Barnes, C., Sweeting, C., Jennings, S., Barry, J., and Polunin, N. (2007). Effect of temperature and ration size on carbon and nitrogen stable isotope trophic fractionation. Funct. Ecol. 21, 356–362. doi: 10.1111/j.1365-2435.2006.01224.x
Bouwman, L., Goldewijk, K. K., Van Der Hoek, K. W., Beusen, A. H. W., Van Vuuren, D. P., Willems, J., et al. (2013). Exploring global changes in nitrogen and phosphorus cycles in agriculture induced by livestock production over the 1900–2050 period. Proc. Natl. Acad. Sci. U. S. A. 110, 20882–20887. doi: 10.1073/pnas.1012878108
Brown, J. H., Gillooly, J. F., Allen, A. P., Savage, V. M., and West, G. B. (2004). Toward a metabolic theory of ecology. Ecology 85, 1771–1789. doi: 10.1890/03-9000
Carter, C. G., and Sajjadi, M. (2011). Low fishmeal diets for Atlantic salmon, Salmo salar L., using soy protein concentrate treated with graded levels of phytase. Aquac. Int. 19, 431–444. doi: 10.1007/s10499-010-9358-z
Čater, M., Fanedl, L., Malovrh, Š., and Marinšek Logar, R. (2015). Biogas production from brewery spent grain enhanced by bioaugmentation with hydrolytic anaerobic bacteria. Bioresour. Technol. 186, 261–269. doi: 10.1016/j.biortech.2015.03.029
Cordell, D., Drangert, J.-O., and White, S. (2009). The story of phosphorus: global food security and food for thought. Glob. Environ. Change 19, 292–305. doi: 10.1016/j.gloenvcha.2008.10.009
Denstadli, V., Skrede, A., Krogdahl, Å., Sahlstrøm, S., and Storebakken, T. (2006). Feed intake, growth, feed conversion, digestibility, enzyme activities and intestinal structure in Atlantic salmon (Salmo salar L.) fed graded levels of phytic acid. Aquaculture 256, 365–376. doi: 10.1016/j.aquaculture.2006.02.021
Eeckhout, W., and De Paepe, M. (1994). Total phosphorus, phytate-phosphorus and phytase activity in plant feedstuffs. Anim. Feed Sci. Technol. 47, 19–29. doi: 10.1016/0377-8401(94)90156-2
Elser, J., and Bennett, E. (2011). A broken biogeochemical cycle. Nature 478, 29–31. doi: 10.1038/478029a
EWOS (2022a). EWOS CLEAR 200 Extruded Feed for Salmon and Trout in Fresh Water. Bergen, Norway: EWOS AS.
EWOS (2022b). EWOS CLEAR 4–100 Ekstrudert Fullfôr til Laks og Ørret i Ferskvann. Bergen, Norway: EWOS AS.
Fang, J., Zhang, J., Jiang, Z., Du, M., Liu, Y., Mao, Y., et al. (2016). Environmental remediation potential of Perinereis aibuhitensis (Polychaeta) based on the effects of temperature and feed types on its carbon and nitrogen budgets. Mar. Biol. Res. 12, 583–594. doi: 10.1080/17451000.2016.1177653
Fiskeridirektoratet. (2023a). Uttak av Slaktet Fisk Fordelt på art 2017-2023 (Produksjonsområde). Available at: https://www.fiskeridir.no/Akvakultur/Tall-og-analyse/Biomassestatistikk/Biomassestatistikk-etter-produksjonsomraade (Accessed May 12, 2023).
Fiskeridirektoratet. (2023b). Forbruk av fôr Fordelt på art 2017-2022 (Produksjonsområde). Available at: https://www.fiskeridir.no/Akvakultur/Tall-og-analyse/Biomassestatistikk/Biomassestatistikk-etter-produksjonsomraade (Accessed June 27, 2023).
García-Alonso, J., Müller, C. T., and Hardege, J. (2008). Influence of food regimes and seasonality on fatty acid composition in the ragworm. Aquat. Biol. 4, 7–13. doi: 10.3354/ab00090
Hardy, R. W. (2010). Utilization of plant proteins in fish diets: effects of global demand and supplies of fishmeal. Aquac. Res. 41, 770–776. doi: 10.1111/j.1365-2109.2009.02349.x
Hill, J. E., Kysela, D., and Elimelech, M. (2007). Isolation and assessment of phytate-hydrolysing bacteria from the DelMarVa peninsula. Environ. Microbiol. 9, 3100–3107. doi: 10.1111/j.1462-2920.2007.01420.x
Jaeger, A., Arendt, E. K., Zannini, E., and Sahin, A. W. (2020). Brewer’s spent yeast (BSY), an underutilized brewing by-product. Fermentation 6:123. doi: 10.3390/fermentation6040123
Jerónimo, D., Lillebø, A. I., Santos, A., Cremades, J., and Calado, R. (2020). Performance of polychaete assisted sand filters under contrasting nutrient loads in an integrated multi-trophic aquaculture (IMTA) system. Sci. Rep. 10, 20871–20810. doi: 10.1038/s41598-020-77764-x
Johansen, U., Nistad, A. A., Ziegler, F., Mehta, S., Wocken, Y., and Hognes, E. S. (2022). Greenhouse gas emissions of Norwegian salmon products. SINTEF Rapportserie 2022, 1–92.
Koroleff, F. (1976). “Determination of nutrients,” in Methods of seawater analysis. vol. 10. New York: Verlag Chemie Weinhein, pp. 117–181.
Kumar, V., Sinha, A. K., Makkar, H. P. S., De Boeck, G., and Becker, K. (2012). Phytate and phytase in fish nutrition. J. Anim. Physiol. Anim. Nutr. 96, 335–364. doi: 10.1111/j.1439-0396.2011.01169.x
Lemos, D., and Tacon, A. G. J. (2017). Use of phytases in fish and shrimp feeds: a review. Rev. Aquac. 9, 266–282. doi: 10.1111/raq.12138
Li, B., Boiarkina, I., Young, B., Yu, W., and Singhal, N. (2016). Prediction of future phosphate rock: a demand based model. J. Environ. Inform. 31, 41–53. doi: 10.3808/jei.201700364
Luis, O. J., and Passos, A. M. (1995). Seasonal changes in lipid content and composition of the polychaete Nereis (Hediste) diversicolor. Comp. Biochem. Physiol. B Biochem. Mol. Biol. 111, 579–586. doi: 10.1016/0305-0491(95)00029-8
Malzahn, A. M., Villena-Rodríguez, A., Monroig, Ó., Johansen, Å., Castro, L. F. C., Navarro, J. C., et al. (2023). Diet rather than temperature determines the biochemical composition of the ragworm Hediste diversicolor (OF Müller, 1776) (Annelida: Nereidae). Aquaculture 569:739368. doi: 10.1016/j.aquaculture.2023.739368
Marques, B., Lillebø, A., Ricardo, F., Nunes, C., Coimbra, M., and Calado, R. (2018). Adding value to ragworms (Hediste diversicolor) through the bioremediation of a super-intensive marine fish farm. Aquac. Environ. Interact. 10, 79–88. doi: 10.3354/aei00255
Nederlof, M., Fang, J., Dahlgren, T., Rastrick, S., Smaal, A., Strand, Ø., et al. (2020). Application of polychaetes in (de)coupled integrated aquaculture: an approach for fish waste bioremediation. Aquac. Environ. Interact. 12, 385–399. doi: 10.3354/aei00371
Nielsen, K. S. (1997). Animal Physiology: Adaptation and Environment, Cambridge, UK: Cambridge University Press.
Olsen, Y., and Olsen, L. (2008). “Environmental impact of aquaculture on coastal planktonic ecosystems,” in Fisheries for global welfare and environment. Memorial book of the 5th World Fisheries Congress 2008. Tokyo, Japan: Yokohama, TERRAPUB.
Olafsen, T., Winther, U., Olsen, Y., and Skjermo, J. (2012). Value Created From Productive Oceans in 2050. Report for the Royal Norwegian Society of Sciences and Letters (DKNVS) and Norwegian Academy of Technological Sciences (NTVA). Trondheim, Norway: DKNVS/NTVA.
Pajand, Z. O., Soltani, M., Bahmani, M., and Kamali, A. (2017). The role of polychaete Nereis diversicolor in bioremediation of wastewater and its growth performance and fatty acid composition in an integrated culture system with Huso huso (Linnaeus, 1758). Aquac. Res. 48, 5271–5279. doi: 10.1111/are.13340
Palmer, P. J., Wang, S., Houlihan, A., and Brock, I. (2014). Nutritional status of a nereidid polychaete cultured in sand filters of mariculture wastewater. Aquac. Nutr. 20, 675–691. doi: 10.1111/anu.12129
Papatryphon, E., Howell, R. A., and Soares, J. H. (1999). Growth and mineral absorption by striped bass Morone saxatilis fed a plant feedstuff based diet supplemented with Phytase. J. World Aquac. Soc. 30, 161–173. doi: 10.1111/j.1749-7345.1999.tb00863.x
Pelletier, N., Tyedmers, P., Sonesson, U., Scholz, A., Ziegler, F., Flysjo, A., et al. (2009). Not all salmon are created equal: life cycle assessment (LCA) of global salmon farming systems. Environ. Sci. Technol. 43, 8730–8736. doi: 10.1021/es9010114
Powar, V. K., and Jagannathan, V. (1982). Purification and properties of phytate-specific phosphatase from Bacillus subtilis. J. Bacteriol. 151, 1102–1108. doi: 10.1128/jb.151.3.1102-1108.1982
Priscilla, L., Rajeev, M., Pandian, S. K., and Malathi, E. (2022). Gut associated culturable bacterial community in intertidal polychaete worms (Annelida: Polychaeta), their characterization and implications in captive shrimp aquaculture. Reg. Stud. Mar. Sci. 52:102274. doi: 10.1016/j.rsma.2022.102274
Sajjadi, M., and Carter, C. G. (2004). Dietary phytase supplementation and the utilisation of phosphorus by Atlantic salmon (Salmo salar L.) fed a canola-meal-based diet. Aquaculture 240, 417–431. doi: 10.1016/j.aquaculture.2004.07.003
Sanni, D. M., Jimoh, M. B., Lawal, O. T., and Bamidele, S. O. (2023). Purification and biochemical characterization of phytase from Bacillus cereus isolated from gastrointestinal tract of African giant snail (Achatina fulica). Int. Microbiol. 26, 961–972. doi: 10.1007/s10123-023-00350-4
Searchinger, T., Waite, R., Hanson, C., Ranganathan, J., Dumas, P., Matthews, E., et al. (2019). Creating a Sustainable Food Future: A Menu of Solutions to Feed Nearly 10 Billion People by 2050. Washington, D.C., USA: WRI.
Sterner, R. W., and Elser, J. J. (2003). Ecological Stoichiometry: The Biology of Elements from Molecules to the Biosphere, Princeton, New Jersey, USA: Princeton University Press.
Sterner, R. W., and Schulz, K. L. (1998). Zooplankton nutrition: recent progress and a reality check. Aquatic Ecol. 32, 261–279. doi: 10.1023/a:1009949400573
Sverdrup, H. U., and Ragnarsdottir, K. V. (2011). Challenging the planetary boundaries II: assessing the sustainable global population and phosphate supply, using a systems dynamics assessment model. Appl. Geochem. 26, S307–S310. doi: 10.1016/j.apgeochem.2011.03.089
United Nations (2022). World Population Prospects 2022: Summary of Results. UN DESA/POP/2022/TR/NO. 3. New York City, USA: Department of Economic and Social Affairs, Population Division
Verni, M., Pontonio, E., Krona, A., Jacob, S., Pinto, D., Rinaldi, F., et al. (2020). Bioprocessing of brewers’ spent grain enhances its antioxidant activity: characterization of phenolic compounds and bioactive peptides. Front. Microbiol. 11:1831. doi: 10.3389/fmicb.2020.01831
Von Bertalanffy, L. (1938). A quantitative theory of organic growth (inquiries on growth laws. II). Hum. Biol. 10, 181–213.
Wagner, N. D., Hillebrand, H., Wacker, A., and Frost, P. C. (2013). Nutritional indicators and their uses in ecology. Ecol. Lett. 16, 535–544. doi: 10.1111/ele.12067
Wang, X., Andresen, K., Handå, A., Jensen, B., Reitan, K., and Olsen, Y. (2013). Chemical composition and release rate of waste discharge from an Atlantic salmon farm with an evaluation of IMTA feasibility. Aquac. Environ. Interact. 4, 147–162. doi: 10.3354/aei00079
Wang, H., Hagemann, A., Reitan, K., Ejlertsson, J., Wollan, H., Handå, A., et al. (2019a). Potential of the polychaete Hediste diversicolor fed on aquaculture and biogas side streams as an aquaculture food source. Aquac. Environ. Interact. 11, 551–562. doi: 10.3354/aei00331
Wang, X., Olsen, L., Reitan, K., and Olsen, Y. (2012). Discharge of nutrient wastes from salmon farms: environmental effects, and potential for integrated multi-trophic aquaculture. Aquac. Environ. Interact. 2, 267–283. doi: 10.3354/aei00044
Wang, H., Seekamp, I., Malzahn, A. M., Hagemann, A., Carvajal, A. K., Slizyte, R., et al. (2019b). Growth and nutritional composition of the polychaete Hediste diversicolor (OF Müller, 1776) cultivated on waste from land-based salmon smolt aquaculture. Aquaculture 502, 232–241. doi: 10.1016/j.aquaculture.2018.12.047
Xiros, C., and Christakopoulos, P. (2012). Biotechnological potential of brewers spent grain and its recent applications. Waste Biomass Valorization 3, 213–232. doi: 10.1007/s12649-012-9108-8
Yousefi-Garakouei, M., Kamali, A., and Soltani, M. (2018). Effects of rearing density on growth, fatty acid profile and bioremediation ability of polychaete Nereis diversicolor in an integrated aquaculture system with rainbow trout (Oncorhynchus mykiss). Aquac. Res. 50, 725–735. doi: 10.1111/are.13918
Keywords: Hediste diversicolor , nutrient upcycling, polychaeta, salmon aquaculture, aquaculture sludge, nutrient uptake, phosphorus
Citation: Anglade I, Kristensen BSB, Dahl TH, Hagemann A, Malzahn AM and Reitan KI (2023) Upcycling of carbon, nitrogen, and phosphorus from aquaculture sludge using the polychaete Hediste diversicolor (OF Müller, 1776) (Annelida: Nereididae). Front. Sustain. Food Syst. 7:1278586. doi: 10.3389/fsufs.2023.1278586
Edited by:
José Mogollón, Leiden University, NetherlandsReviewed by:
Helena Fernandes, University of Vigo, SpainRobert Nesta Kagali, Nagasaki University, Japan
Copyright © 2023 Anglade, Kristensen, Dahl, Hagemann, Malzahn and Reitan. This is an open-access article distributed under the terms of the Creative Commons Attribution License (CC BY). The use, distribution or reproduction in other forums is permitted, provided the original author(s) and the copyright owner(s) are credited and that the original publication in this journal is cited, in accordance with accepted academic practice. No use, distribution or reproduction is permitted which does not comply with these terms.
*Correspondence: Inka Anglade, aW5rYS5zZWVrYW1wQG50bnUubm8=