- 1Department of Animal Science, University of Connecticut, Storrs, CT, United States
- 2Department of Nutritional Sciences, University of Connecticut, Storrs, CT, United States
Listeria monocytogenes is a major foodborne pathogen in the United States that is capable of forming sanitizer-tolerant biofilms on diverse food contact surfaces and under varying temperature conditions. A plethora of research in the last decade has explored the potential of phytochemicals as antibiofilm agents. However, the low solubility of phytochemicals is a significant challenge that needs to be addressed to develop plant-based disinfectants that can be applied in the industry for controlling L. monocytogenes biofilms and improving food safety. This study investigated the efficacy of eugenol nanoemulsion (EGNE) in inhibiting biofilm formation in two strains of L. monocytogenes (Scott A and AT19115) on stainless steel surfaces at two temperatures (25 or 10°C). In addition, the effect of EGNE on pathogen motility, extracellular polymeric substances (EPS) production, eDNA production, and quorum sensing activity during biofilm formation was studied using standard bioassays. Moreover, the efficacy of EGNE in killing mature L. monocytogenes biofilm was also investigated against both the strains and temperature combinations. All experiments had a completely randomized design with duplicate samples and were repeated at least three times. EGNE had a particle size of ~75 nm, a polydispersity index of 0.25, and a high negative surface charge. EGNE 700 mg/L inhibited L. monocytogenes biofilm formation significantly by ~1.89 log in 72 h at 25°C and ~1.25 log on day 16 at 10°C, when compared to control (p < 0.05). EGNE at 2,750 mg/L concentration completely inactivated (~7 log CFU/coupon reduction as compared to control) L. monocytogenes biofilm cells developed at 25 or 10°C as early as 1 min of treatment time (p < 0.05). In addition, EGNE was able to significantly reduce the motility, EPS, eDNA production, and quorum sensing activity which plays a major role in biofilm formation. Both L. monocytogenes Scott A and AT19115 strains exhibited similar sensitivity to EGNE treatments. The results suggest that EGNE could potentially be used as a natural sanitizer to effectively control L. monocytogenes biofilms in food processing environments.
1. Introduction
Listeria monocytogenes is a gram-positive, psychrotolerant bacteria that causes serious foodborne infection in humans (Scallan et al., 2011). Even though the incidence of listeriosis is low, the infection has a high case fatality rate of ~20% and often leads to meningitis, abortion, and death in susceptible individuals (Radoshevich and Cossart, 2018; WHO, 2022). Due to the low infectious dose and high case fatality rates associated with Listeriosis, the United States Department of Agriculture Food Safety Inspection Service (USDA-FSIS) has a zero-tolerance policy for L. monocytogenes in poultry and ready-to-eat (RTE) products (FSIS, 2012). Contaminated fresh produce, soft cheese, and RTE meat products are frequently implicated in L. monocytogenes outbreaks, and product contamination is often traced back to a contaminated processing environment (Leong et al., 2014; Malley et al., 2015; Fagerlund et al., 2022). As L. monocytogenes is ubiquitous in natural environments such as soil, irrigation water, and animal reservoirs (Haase et al., 2014; WHO, 2018), it is very difficult to prevent its entry into processing areas. In such environments, L. monocytogenes has been reported to persist for several years leading to repeated contamination of food products (Ferreira et al., 2014; Stoller et al., 2019; Cherifi et al., 2020; Gray et al., 2021). While the exact mechanism of survival is still an area of active research, high-stress tolerance and the capability to form sanitizer-tolerant biofilms are two factors that facilitate high persistence rates. Bacterial biofilms are communities of bacteria (mono-specie or multi-specie) found attached to a biotic or abiotic surface and embedded in a self-generated matrix of extracellular polymeric substances (EPSs). The EPSs are primarily composed of polysaccharides, proteins, and extracellular DNA and serve as a site for chemical signaling and nutrient assimilation (Costa et al., 2018). In addition, EPS facilitates surface attachment, confers cohesion, and protects against the action of various antimicrobials (Murugan et al., 2016; Zhao et al., 2022). Similarly, quorum sensing plays a major role in biofilm formation in L. monocytogenes by facilitating cell-to-cell communication in response to the changing external environments and bacterial populations (Castillo et al., 2014). Autoinducer 2 (AI-2) is a signaling molecule produced by the luxS gene that mediates quorum sensing in L. monocytogenes (Sela et al., 2006; Garmyn et al., 2009; Melian et al., 2019). The ability of L. monocytogenes to form biofilm enhances the survival of the pathogen against daily cleaning and disinfection routine (Lunden et al., 2002; Pan et al., 2006; Skowron et al., 2019) as cells inside a biofilm are less sensitive to disinfectants compared to their planktonic counterparts, especially when the biofilm is mature and well-developed (Ceri et al., 2001; Wagner and Iglewski, 2008; Korany et al., 2018). In some instances, the sanitizer tolerance of microbes present in the biofilm can be as high as 32-fold, as compared to planktonic cells (Malik et al., 2017). According to the National Institute of Health and the Centre for Disease Control, 65% of foodborne outbreaks are due to contact of food products with biofilms and hence its presence in food processing areas poses a significant safety concern (Galié et al., 2018).
Regular cleaning and disinfection are the primary tools to control L. monocytogenes in the food processing industry (Tompkin, 2002; FSIS, 2016). However, FDA-approved disinfectants such as quaternary ammonium compounds and hypochlorite are not effective in controlling L. monocytogenes biofilms completely in the processing environment (Soumet et al., 2005; Pan et al., 2006; Clayton et al., 2021). There is also a concern about using the abovementioned chemical compounds as a sanitizer due to the production of secondary metabolites which are of high health risk to handlers (Zhang and Farber, 1996; Parish et al., 2003; Donato and Zani, 2010). Moreover, the abovementioned compounds are tested against planktonic cells, and their efficacy against complex bacterial communities present in the industry setting is not completely known (Coughlan et al., 2016). Therefore, there is a need to develop an alternative biofilm control strategy that is safe, efficient, and simple to implement in the food processing industry. In the past decade, the use of plant compounds as natural antimicrobial agents to control pathogenic microorganisms has gained significant interest. Eugenol (4-allyl-2-ethoxyphenol), obtained from clove (Eugenia caryophyllus), is a phytochemical with Generally Recognized as Safe (GRAS) status according to the Code of Federal Regulations 21 Part 172. Several studies have demonstrated the antibiofilm capability of eugenol against pathogens such as Vibrio parahaemolyticus (Ashrafudoulla et al., 2020), Porphyromonas gingivalis (Zhang et al., 2017), methicillin-resistant Staphylococcus aureus (Al-Shabib et al., 2017), and L. monocytogenes (Upadhyay et al., 2013). However, eugenol oil has low solubility in water (2,460 mg/L at 25°C), which limits its application as a water-soluble disinfectant in the food processing industry (Yalkowsky and Banerjee, 1992). To overcome this challenge, we used GRAS status emulsifiers (gum Arabic and lecithin) to prepare eugenol nanoemulsion and thereby improve the dispersion of eugenol in water.
The goal of this study was to investigate the efficacy of eugenol nanoemulsion (EGNE) prepared with a combination of gum Arabic and lecithin in inhibiting and inactivating L. monocytogenes biofilms on stainless steel surfaces at 25 or 10°C. In addition, the effects of EGNE on EPS synthesis, extracellular DNA release, pathogen motility, and quorum sensing activity were tested.
2. Materials and methods
2.1. Bacterial strains and culture conditions
This study included two strains of L. monocytogenes, namely, Scott A and AT19115. The organisms were inoculated onto Oxford agar plates (Oxoid Ltd., United Kingdom) from the glycerol stock and thereafter incubated at a temperature of 37°C for a duration of 48 h. Individual colonies were selected and cultured in 10 ml of tryptic soy broth (TSB, Fisher Scientific Co LLC, Hanover Park, IL) at 37°C for 24 h. For inoculum preparation, the individual overnight culture was centrifuged at 5,500 g for 15 min at 25°C. The bacterial pellet was washed three times and resuspended in 10 ml of sterile 1X PBS (pH 7.0). The average inoculum for the inhibition and inactivation assay was ∼6 log CFU/ml. The confirmation of the bacterial population’s concentration in the initial culture was achieved through plating on Oxford agar, followed by incubation at a temperature of 37°C for a duration of 24–48 h.
2.2. Preparation of eugenol nanoemulsion
The preparation of eugenol nanoemulsion was conducted according to the method by Hu et al. (2016). A 4% stock solution of gum Arabic or lecithin was separately made in water (w/v). These solutions were then combined in equal quantities to create a mixture of gum Arabic and lecithin. Eugenol in ethanol was gradually added into a solution comprising gum Arabic and lecithin. The addition was carried out drop-by-drop until a final concentration of 1.25% was achieved. The formulation, with a total volume of 120 ml, underwent sonication utilizing the high-speed homogenizer Q 700 (QSonica L.L.C, Newtown, CT, USA) for 10 min. The sonication process involved a cycle of 10 s of operation followed by 5 s of rest, with an amplitude of 60 Watts. According to Balasubramanian et al. (2022), the nanoemulsion’s composition consisted of 1.25% eugenol, 0.5% gum Arabic, 0.5% lecithin, and 16.66% ethanol.
2.3. Characterization of eugenol nanoemulsion stability during storage at 25 or 10°C for 4 months
The eugenol nanoemulsion was stored at 25 or 4°C for 4 months. The stability of the nanoemulsion was measured by characterizing the size, polydispersity index (PDI), and zeta potential on months 0, 2, and 4 using Nano Zetasizer ZS (Malvern Instruments, Ltd., Worcestershire, UK). By determining the minimum bactericidal concentration, the effectiveness of the antibacterial efficacy was determined (CLSI, 2019).
2.4. Determination of sub-inhibitory and minimum bactericidal concentration of EG or EGNE against L. monocytogenes
The sub-inhibitory (SIC) and minimum bactericidal concentration (MBC) of EG or EGNE were determined as previously described (Johny et al., 2010). Sterile 24-well polystyrene tissue culture plates (Costar, Corning Incorporated, Corning, NY) containing TSB (1 ml/well) were inoculated separately with ∼6.0 log CFU/ml of L. monocytogenes, followed by the addition of EG or EGNE at various concentrations (Sigma Aldrich). The plates were incubated at 37°C for 24 h, and bacterial growth was determined by culturing on duplicate tryptic soy agar (TSA) and Oxford agar plates. The two highest concentrations of EG or EGNE below its minimum inhibitory concentration (MIC) that did not inhibit bacterial growth after 24 h of incubation as compared to control samples were selected as the SICs for this study, whereas the lowest concentration of EG or EGNE that reduced L. monocytogenes population in TSB by ∼5.0 log CFU/ml after incubation at 37°C for 24 h was taken as the MBC.
2.5. Preparation of stainless steel coupons
Stainless steel coupons (type 304; diameter 1 cm) with no. 4 finish were prepared, as described by Djordjevic et al. (2002). Coupons were cleaned with 100% ethanol and rinsed in distilled water. Finally, the coupons were sterilized in an autoclave for 15 min at 121°C.
2.6. Effect of sub-inhibitory concentration of EGNE in inhibiting L. monocytogenes biofilm formation, motility, EPS, quorum sensing activity, and eDNA production
2.6.1. Biofilm formation assay on stainless steel surface
The inhibition of L. monocytogenes biofilm formation by EGNE on stainless steel coupons was determined as previously described (Kim et al., 2008). In brief, sterile stainless steel coupons were individually placed in each well of a 24-well cell culture plate containing 1 ml of TSB with or without (control) SIC of EG or EGNE. Each L. monocytogenes strain (~6.0 log CFU/ml; 1 ml in TSB) was added to the wells and incubated at 25°C for 96 h or 10°C for 24 days. At regular intervals (24, 48, 72, or 96 h at 25°C and 8th, 16th, or 24th day at 10°C), coupons were washed with 1 ml of sterile deionized water (DI) with gentle agitation for 5 s and transferred to a sterile centrifuge tube containing 1 ml of Dey-Engley neutralizing (DE) broth with sterile glass beads. The tubes were vortexed for 1 min to detach the L. monocytogenes from the coupons. The DE broth suspension was serially diluted and surface-plated (0.1 ml, in duplicate) on TSA and Oxford agar plates and incubated at 37°C for 48 h for pathogen enumeration (Upadhyay et al., 2013).
2.6.2. Bacterial motility assay
The motility of L. monocytogenes in the presence or absence (control) of a sub-inhibitory concentration of EGNE was assessed by measuring the zone of motility in 0.3% semi-solid agar after incubating at room temperature for 6 days (Niu and Gilbert, 2004). Separate Luria Bertani (LB) agar (0.3%) plates containing the respective SICs of EG or EGNE were prepared. Luria Bertani agar plates not supplemented with EG or EGNE were served as controls. An overnight culture of L. monocytogenes was centrifuged at 5,500 g for 15 min at 25°C and washed three times with sterile 1X PBS. Then, 5 μl of resuspended culture (~6 log CFU) was spot inoculated at the center of agar plates followed by incubation at 25°C for 6 days. The zone of motility (distance traveled by the bacteria from the center spot to the agar edge) was measured in cm.
2.6.3. Extracellular polymeric substances production assay
The effect of SIC of EGNE on exopolysaccharide production during biofilm formation was determined using a ruthenium red staining assay. Sterile 96-well polystyrene plates were inoculated with 200 μl of the culture suspension (~6.0 log CFU), followed by the addition of SICs of EG or EGNE. The culture plates were incubated at 25°C for 96 h for biofilm development. After the incubation, the biofilms were washed with sterile 1X PBS three times followed by the addition of 0.01% ruthenium red (Sigma Aldrich) to each well. For blank, the wells without biofilm were added with 200 μl of stain. The plates were incubated at room temperature for 60 min. After the incubation period, the residual stain was transferred to a fresh plate and optical density was measured at 450 nm using a synergy HT multi-mode microplate reader (BioTek). The amount of dye bound to the biofilm was determined by the formula ODBF = ODB−ODS, where ODB refers to an optical density (450 nm) measured for blanks and ODS refers to the optical density (450 nm) obtained from residual stain collected from sample wells (Zameer et al., 2010).
2.6.4. Quorum sensing inhibition assay
The effect of a sub-inhibitory concentration of EGNE on autoinducer-2 (AI-2) levels of L. monocytogenes was determined by Vibrio harveyi bioluminescence assay as per the published protocol with slight modifications (Melian et al., 2019). In brief, L. monocytogenes was cultured for 12 h in the presence or absence of SIC of EGNE at 25°C followed by centrifugation at 5500 g for 15 min. The supernatant was filtered using a 0.2-μm syringe filter to obtain cell-free supernatant (CFS). AI-2 levels in the supernatant were detected by the V. harveyi BB170 reporter strain. The V. harveyi BB170 was cultured aerobically in Luria Bertani broth for 24 h at 30°C. The culture was then diluted in an autoinducer assay medium in the ratio of 1:5,000 (~3 log CFU/ml). V. harveyi BB152 strain was used as a positive control in this assay. Ninety microliters of reporter strain (BB170) were added to the 96-well plate followed by the addition of CFS of L. monocytogenes cultured in the presence or absence of EGNE or CFS of V. harveyi BB152 (positive control) or autoinducer assay medium (negative control). Bioluminescence of the mixture was measured for 8 h at 30°C using Cytation 5 multi-mode reader (BioTek), and self-induction of bioluminescence in BB170 was deducted from positive control and treatments before the data analysis.
2.6.5. eDNA visualization using confocal microscopy
To study the effect of EGNE on eDNA production, L. monocytogenes was allowed to develop biofilm in the presence or absence of SIC of EGNE on Lab-Tech four-chamber no. 1 borosilicate glass coverslip (Lab-tek, Nalge Nunc International, Rochester, NY) at 25°C for 96 h as described in section (2.6.1). The biofilms were washed with sterile PBS three times followed by staining with 0.0025 mM SYTO, 0.005 mM propidium iodide, and 300 nM DAPI. In situ, confocal laser scanning microscopy was performed using a Leica true confocal scanner SP2 microscope with a water immersion lens. Fluorochromes were excited using a krypton-argon mixed-gas laser with a PMT2 filter. The laser was excited at 488 nm, and the emission was observed at 528 nm for SYTO and 645 nm for propidium iodide. For DAPI, excitation and emission of 358 and 461 nm were used. For each treatment, four randomly selected fields were analyzed (Upadhyay et al., 2013).
2.7. Effect of minimum bactericidal concentration of EGNE in inactivating L. monocytogenes mature biofilm on stainless steel surface
2.7.1. Biofilm inactivation assay
To investigate the bactericidal effect of EGNE on fully formed, mature L. monocytogenes biofilms, the sterile coupons were placed separately in 1 ml of TSB containing ~6 log CFU/ml of L. monocytogenes and incubated for 96 h at 25 or 10°C for 24 days to facilitate biofilm formation. After the development of mature biofilm, at regular intervals (24, 48, 72, or 96 h at 25°C and 8th, 16th, or 24th day at 10°C), the coupons were rinsed in 1 ml of sterile DI water three times with gentle agitation for 5 s, transferred to a sterile petri dish containing the MBC and a concentration greater than the MBC of EG or EGNE, and then incubated at 25°C for 1, 5, 10, 15, or 30 min. After treatment, the coupons were transferred to a centrifuge tube containing 1 ml of DE broth with sterile glass beads. The tubes were vortexed for 1 min to detach the L. monocytogenes from the coupons. The DE broth suspension was serially diluted and surface-plated (0.1 ml, in duplicate) on TSA and Oxford agar plates and incubated at 37°C for 24–48 h (Upadhyay et al., 2013).
2.7.2. Visualization of live-dead L. monocytogenes cells in biofilm using confocal microscopy
To study the effect of MBC of EGNE on L. monocytogenes biofilm, the pathogen was allowed to form biofilm at 25°C for 96 h in TSB using a Lab-Tech four-chamber no. 1 borosilicate glass coverslip system (Lab-tek) followed by EGNE treatment as described in section (2.7.1). Live and dead bacteria in the biofilm were imaged after staining with 0.0025 mM SYTO (Molecular Probes, Oregon) and 0.005 mM propidium iodide (PI; Molecular probes) for 20 min.
2.8. Statistical analysis
The entire study was designed in a completely randomized model with duplicate/triplicate samples, and the study was repeated at least three times (n = 9). For each treatment and control, the data from independent replicate trials were pooled and analyzed using two-way ANOVA in R version 4.0.1. A p-value ≤ 0.05 was considered statistically significant.
3. Results
3.1. Average particle size, PDI, and zeta potential of eugenol nanoemulsion
The average particle size, PDI, and zeta potential of EGNE are presented in Table 1. The average particle size, PDI, and zeta potential of freshly prepared EGNE on day 0 were ~75.25 ± 0.25 nm, 0.25 ± 0.01, and −34.75 ± 0.25 mV, respectively. The prepared nanoemulsion was homogenous in nature with a high surface negative charge. EGNE stored at 4°C maintained its particle size of ~77.50 ± 0.50 nm, PDI of ~0.28 ± 0.01, and zeta potential of ~ −31.75 ± 0.75 mV till 2 months of storage (p > 0.05). However, a slight increase in size (~4.00 nm) and PDI (~0.04) was observed at 4 months (p < 0.05). No significant changes were observed in zeta potential after storage for 4 months (p < 0.05).
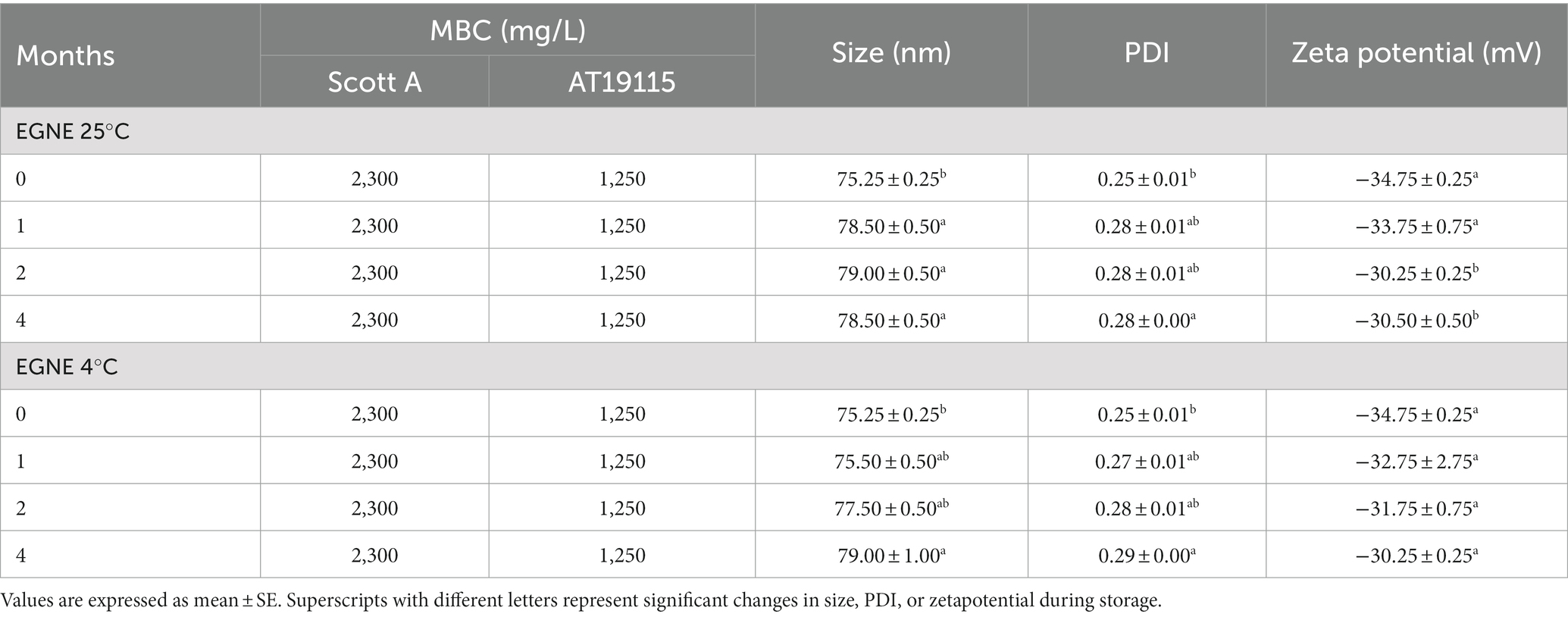
Table 1. Minimum bactericidal concentration, average particle size, PDI, and zeta potential of EGNE during storage at 25 or 4°C for 4 months.
When EGNE was stored at 25°C, a slight increase in size (~3.50 nm) was observed after 1 month. Changes in PDI (~0.28 ± 0.00) and zeta potential (~−30.25 ± 0.25 mV) were observed after 4 and 2 months, respectively (p < 0.05). Even though we observed significant changes, all values remained within the acceptable criteria for a good-quality nanoemulsion.
3.2. Sub-inhibitory and minimum bactericidal concentration of EG or EGNE against L. monocytogenes
The SIC of EG or EGNE against L. monocytogenes Scott A and AT19115 was 700 and 600 mg/L, respectively. The SIC and one concentration below SIC were chosen for the biofilm inhibition assay.
The MBC of EG or EGNE against L. monocytogenes Scott A was 2,300 mg/L. The MBC of EG or EGNE against L. monocytogenes AT19115 was 1,250 mg/L, respectively. The MBC and two concentrations above MBC were chosen for the biofilm inactivation assay. No change in the MBC of EG or EGNE was observed upon storage at 25 or 4°C for 4 months (p > 0.05; Table 1).
3.3. Effect of sub-inhibitory concentration of EGNE in inhibiting L. monocytogenes biofilm formation on the stainless steel surface
3.3.1. Biofilm formation assay at 25 or 10°C
To investigate the effect of EGNE in inhibiting biofilm formation, L. monocytogenes strains were allowed to develop biofilms in the presence or absence of EGNE for 96 h at 25°C (Table 2A) or 24 d at 10°C (Table 2B).
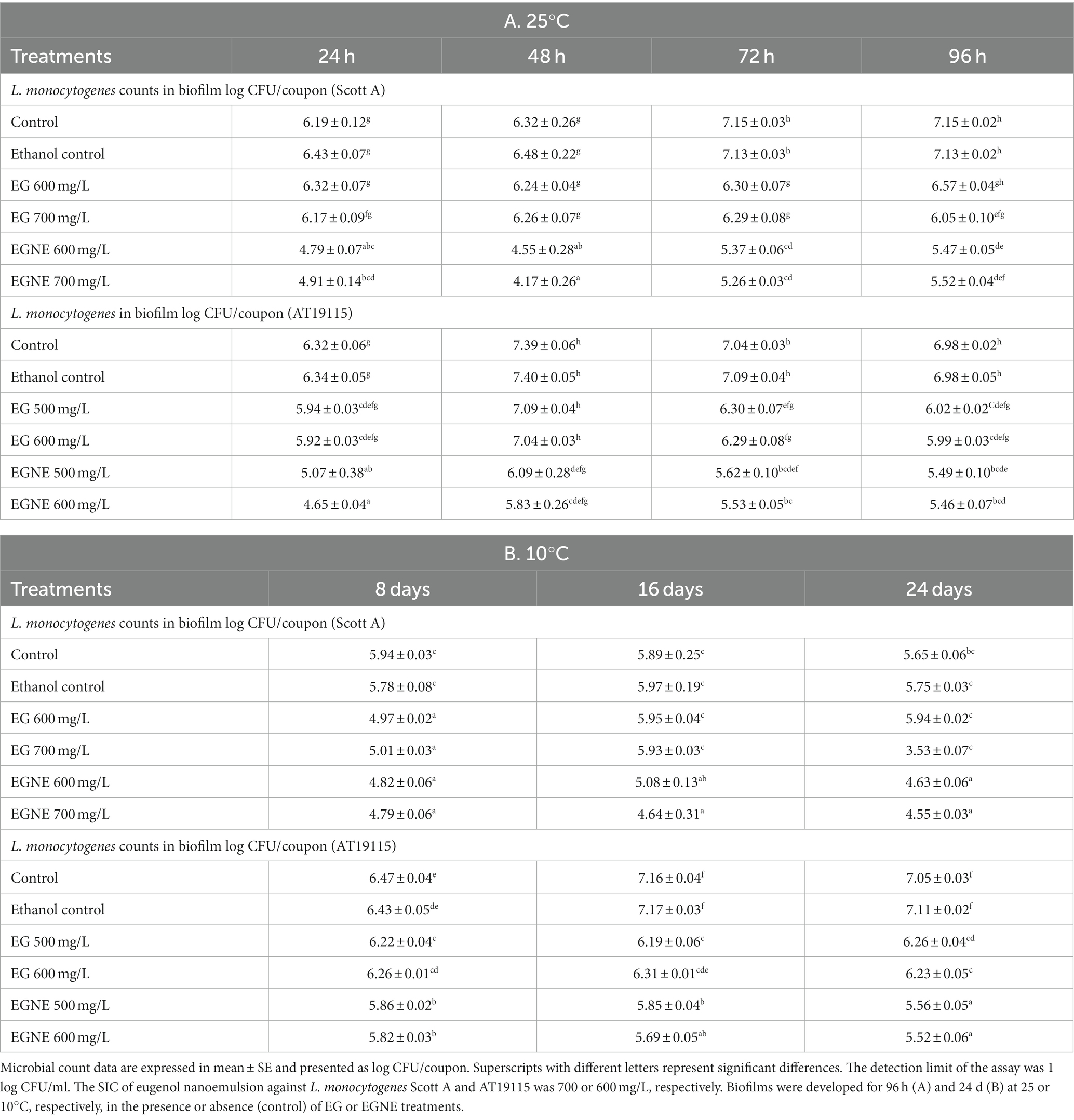
Table 2. Effect of sub-inhibitory concentration of EGNE on L. monocytogenes (Scott A, AT19115) biofilm formation on stainless steel surface at 25 or 10°C.
At 25°C, a gradual increase in the L. monocytogenes population in the developing biofilm was observed during the incubation period. L. monocytogenes Scott A population increased from ~6 log CFU/coupon to 7.15 log CFU/coupon in 72 h during biofilm formation (p < 0.05). In the case of AT19115, the bacterial population increased from ~6.32 log CFU/coupon at 24 h to ~7.4 at 48 h (p < 0.05). When the biofilm was developed at 10°C, a pathogen population of ~5.94 log CFU/coupon was observed for Scott A strain in the biofilm after 8 days of incubation. The pathogen population did not significantly increase during further incubation for 24 days. A strain difference was observed with AT19115 wherein the pathogen population in the biofilm gradually increased from d 8 (~6.4 log CFU/coupon) to d 16 (~7.16 log CFU/coupon) of incubation (p < 0.05). No further increase in pathogen load in the biofilm was observed from d 16 to 24. Since EGNE nanoemulsion was prepared with ethanol as a co-surfactant, the effect of ethanol on biofilm formation was tested. The presence of ethanol did not significantly modulate biofilm formation as compared to the control at 25 or 10°C (p > 0.05). The presence of EGNE at its SIC inhibited biofilm formation (as indicated by lower pathogen load in biofilm) in both strains of L. monocytogenes as early as 24 h (at 25°C) or d 8 (at 10°C) of incubation (Tables 2A,B). At 25°C, EGNE reduced pathogen load (both strains) in the biofilm by ~1–1.5 log after 24 h of incubation (p < 0.05). While a gradual increase in pathogen load was observed in the control biofilm upon incubation, the presence of SIC of EGNE exerted an inhibitory effect. At 96 h of incubation, the pathogen load in control biofilm and biofilm developed in the presence of SIC of EGNE differed by ~1.5 log CFU/coupon in both strains (p < 0.05). In the case of EG, no reduction in pathogen load was observed until 72 h of incubation at 25°C. Thereafter, a minor reduction of ~0.5–0.9 log was observed as compared to control. EGNE treatments were more effective than corresponding EG controls in reducing pathogen load (both strains) at 24 and 48 h of incubation. At 72 h of incubation, all EGNE treatments (except EGNE 500 mg/L against AT19115) were more effective in reducing L. monocytogenes in the biofilm as compared to the corresponding EG control. At 96 h of incubation, EG and EGNE had similar efficacy in reducing L. monocytogenes counts in biofilm as compared to the control (Table 2A). At 10°C, EGNE treatments were consistently more effective than corresponding EG treatments at d 16 and d 24 and reduced L. monocytogenes (both strains) in the biofilm by ~1–1.5 log/coupon, as compared to the control (p < 0.05; Table 2B).
3.3.2. Effect of sub-inhibitory concentration of EGNE on L. monocytogenes motility
The effect of EGNE on L. monocytogenes motility is presented in Figures 1A,B. EG and EGNE reduced the motility of both strains of L. monocytogenes, as indicated by a reduced zone of motility, compared to the control (p < 0.05). EGNE was more effective than EG in reducing L. monocytogenes motility (p < 0.05).
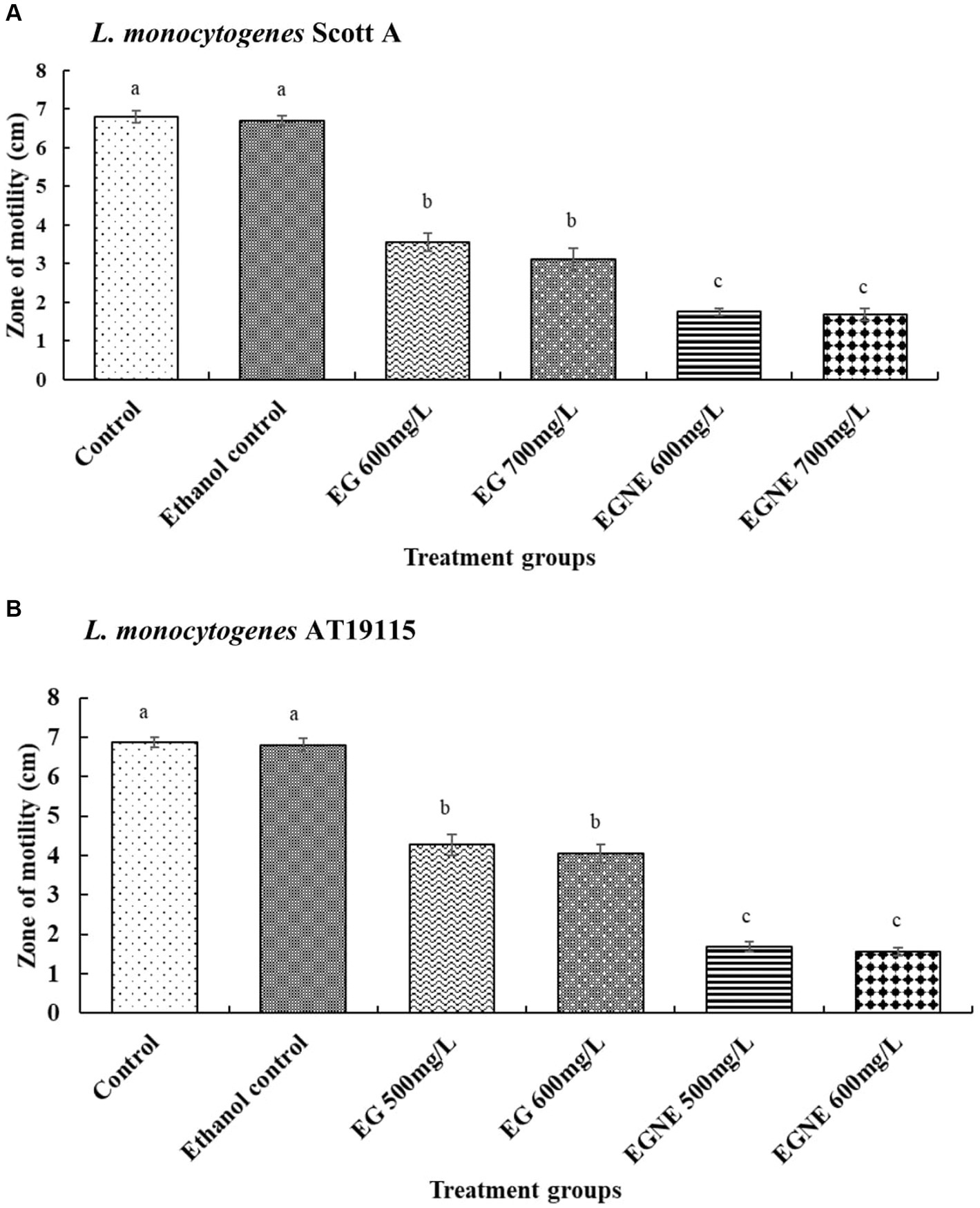
Figure 1. Effect of sub-inhibitory concentration of EGNE on the motility of L monocytogenes. (A) L. monocytogenes Scott A. (B) L. monocytogenes AT19115. The error bar represents mean ± SE. Superscripts with different letters represent significant differences between treatments.
3.3.3. Effect of sub-inhibitory concentration of EGNE on L. monocytogenes extracellular polymeric substances production
Biofilm developed in the presence of SIC of EGNE exhibited a significant reduction in EPS production, as compared to control and EG (Figures 2A,B; p < 0.05) in both L. monocytogenes Scott A and AT19115 strains. EG had no effect in reducing the EPS production during biofilm formation, as compared to the control (p > 0.05).
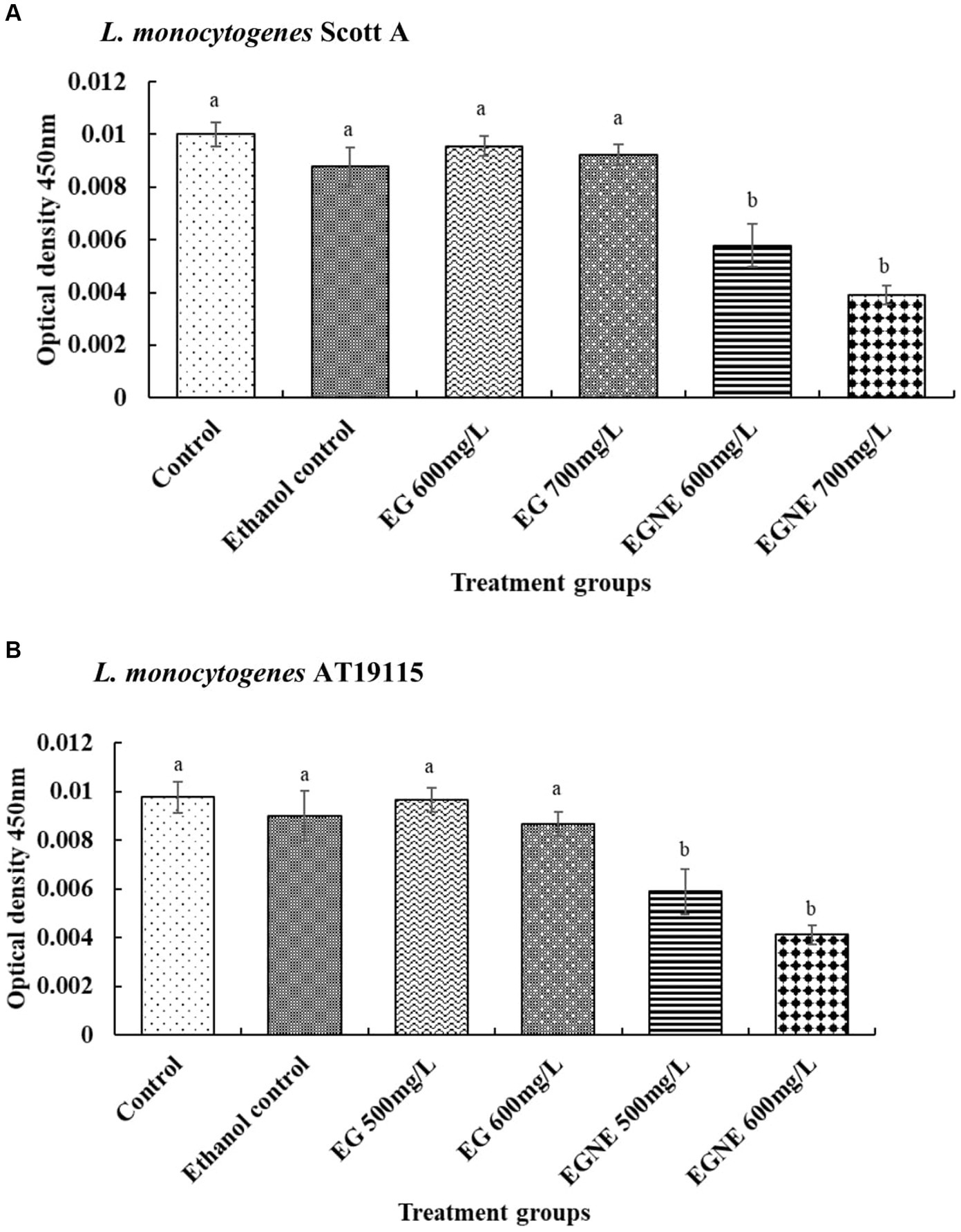
Figure 2. Effect of sub-inhibitory concentration of EGNE on the EPS production of L. monocytogenes biofilm. (A) L. monocytogenes Scott A. (B) L. monocytogenes AT19115. The error bar represents mean ± SE. Superscripts with different letters represent significant differences between treatments.
3.3.4. Effect of sub-inhibitory concentration of EGNE on quorum sensing activity of L. monocytogenes
The effect of EGNE on quorum sensing activity is presented in Figures 3A,B. The AI-2 levels in the CFS of untreated L. monocytogenes determined by luminescence measurement were ~1,438 and 4,109 relative light units (RLU) for Scott A and AT19115, respectively. The presence of EG and EGNE reduced the AI-2 levels in both strains of L. monocytogenes (p < 0.05). No significant difference between the efficacy of EG and EGNE was observed. The presence of ethanol did not influence quorum sensing activity, as compared to control (p > 0.05).
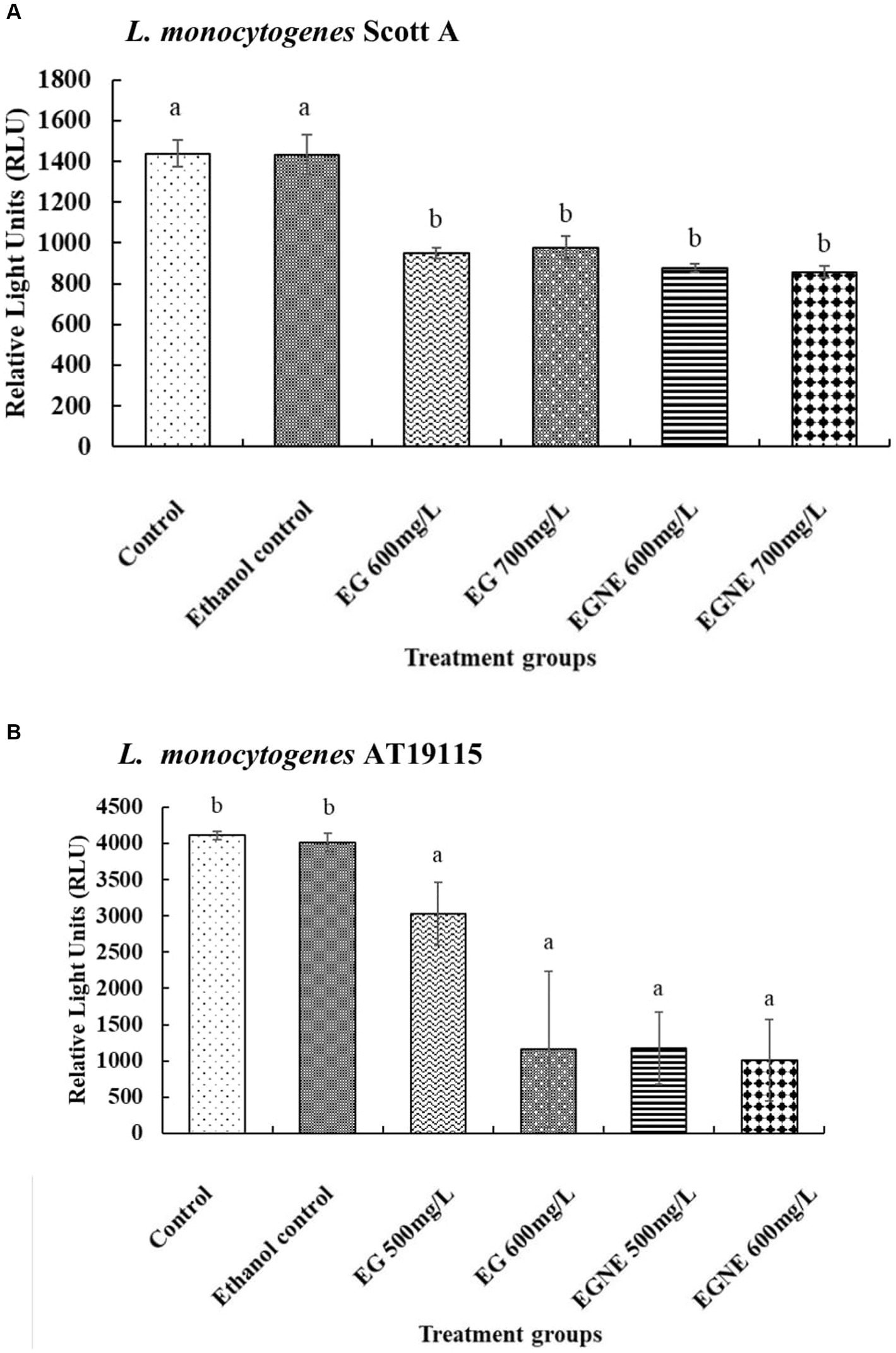
Figure 3. Effect of sub-inhibitory concentration of EGNE on quorum sensing inhibition in L. monocytogenes biofilm. (A) L. monocytogenes Scott A. (B) L. monocytogenes AT19115. The error bar represents mean ± SE. Superscripts with different letters represent significant differences between treatments.
3.3.5. eDNA visualization using confocal microscopy
The effect of SIC of EGNE on L. monocytogenes eDNA is presented in Figure 4A-C. The biofilm developed in the presence of SIC of EGNE appeared to have reduced eDNA (stained blue by DAPI) in the biofilm. Moreover, there was a reduction in the number of bacteria present in the biofilm architecture as observed by a reduction in SYTO and PI-stained cells.
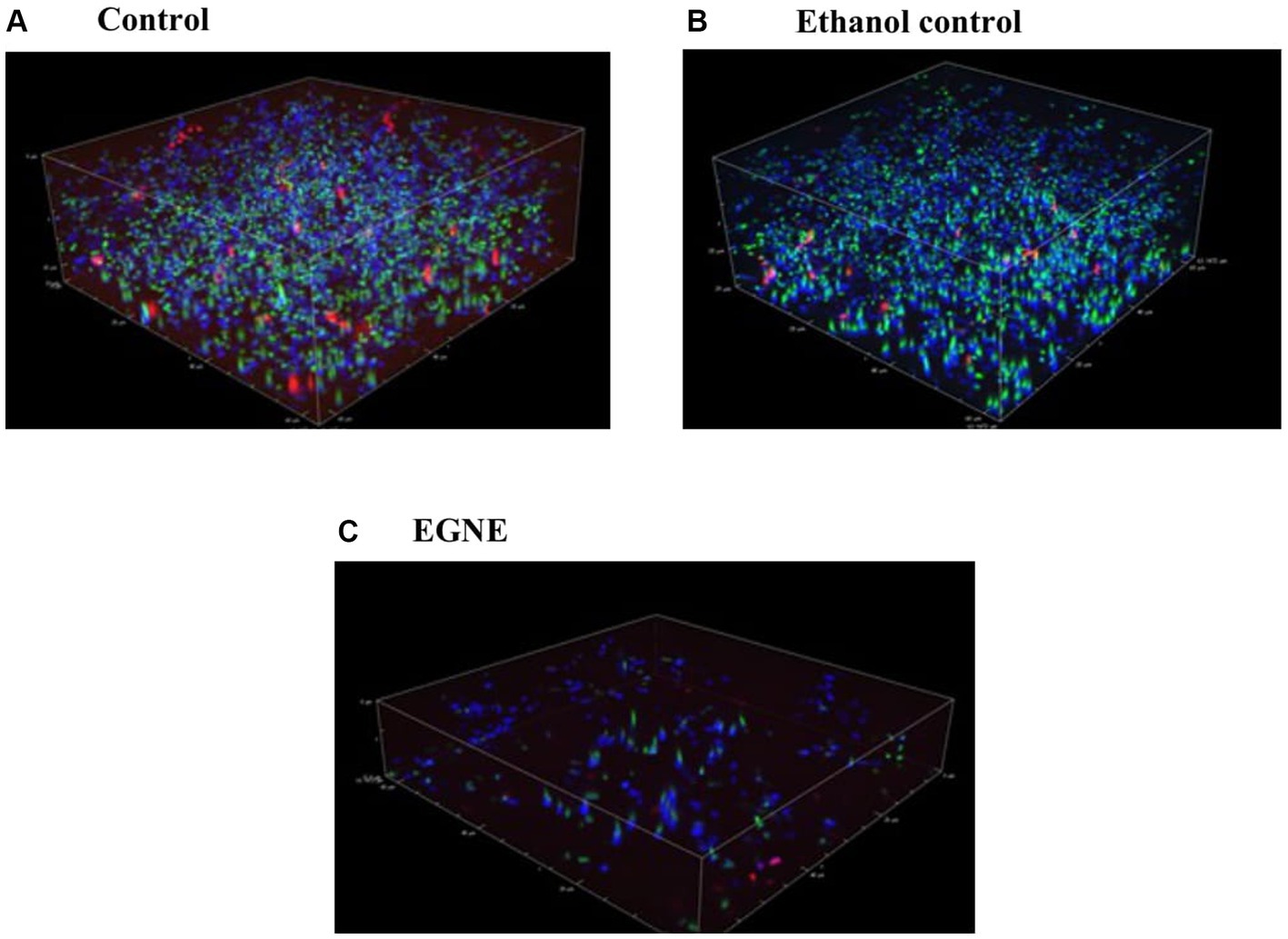
Figure 4. Effect of sub-inhibitory concentration of EGNE on eDNA present in L. monocytogenes Scott A biofilm developed at 25°C. (A) Control. (B) Ethanol control. (C) EGNE. Green and red color indicates live or dead bacterial cells respectively, in the biofilm. Blue color indicates presents of eDNA in the biofilm matrix.
3.4. Effect of minimum bactericidal concentration of EG or EGNE in inactivating L. monocytogenes mature biofilm on stainless steel surface
The efficacy of EG and EGNE in inactivating the mature biofilm on stainless steel coupons at 25 or 10°C is presented in Tables 3, 4, respectively. The average population of L. monocytogenes recovered in control coupons after incubation at 25 for 96 h or 10°C for 24 days was ~6–7 log CFU/coupon. No increase in pathogen load in the biofilm was observed from d 16 to 24 at 10°C, and thus we conducted inactivation assay on d 16. Washing coupons with sterile DI water did not reduce the L. monocytogenes population in mature biofilm at 25 or 10°C (p > 0.05). Increasing the washing time with DI water had no significant effect in reducing the pathogen population in the biofilm (p > 0.05). Similarly, exposure to ethanol did not reduce the pathogen population in the mature biofilm even after 30 min of treatment time, as compared to control (p < 0.05). All doses of EG (2,300, 2,750, and 3,500 mg/L) significantly reduced L. monocytogenes Scott A population in the mature biofilm by at least ~2.65 log as early as 1 min of treatment time at 25°C. Time and dose-dependent effect was observed in EG treatments wherein increasing the treatment time and concentration resulted in better reduction (p < 0.05). The highest dose of EG, by 30 min of treatment time, reduced the L. monocytogenes Scott A population in the biofilm by ~4 log/coupon (p < 0.05). However, EGNE 2,300 mg/L reduced the biofilm cells below the detection limit as early as 30 min of treatment time at 25°C (p < 0.05). Concentrations above MBC, EGNE 2,750, or 3,500 resulted in below detection limit as early as 1 min of treatment time in L. monocytogenes Scott A biofilm on a stainless steel surface (p < 0.05). A similar trend was observed in L. monocytogenes AT19115, where MBC of EGNE 1,250 mg/L exhibited a significant effect in reducing the biofilm cells below the detection limit as early as 30 min of treatment time (p < 0.05). EGNE 2,300 or 2,750 mg/L completely inactivated the mature biofilm to below the detection limit as early as 1 min of treatment time at 25°C (p < 0.05; Table 3). The treatments with below detection limit were enriched in Listeria enrichment broth and found to be enrichment negative. Similar results were observed at 10°C for both L. monocytogenes Scott A and AT19115 strains (Table 4). Being eugenol as an antimicrobial agent, it showed reduced efficacy compared to its nanoemulsion form suggesting that solubility and uniform distribution play a major role in its antibiofilm activity.
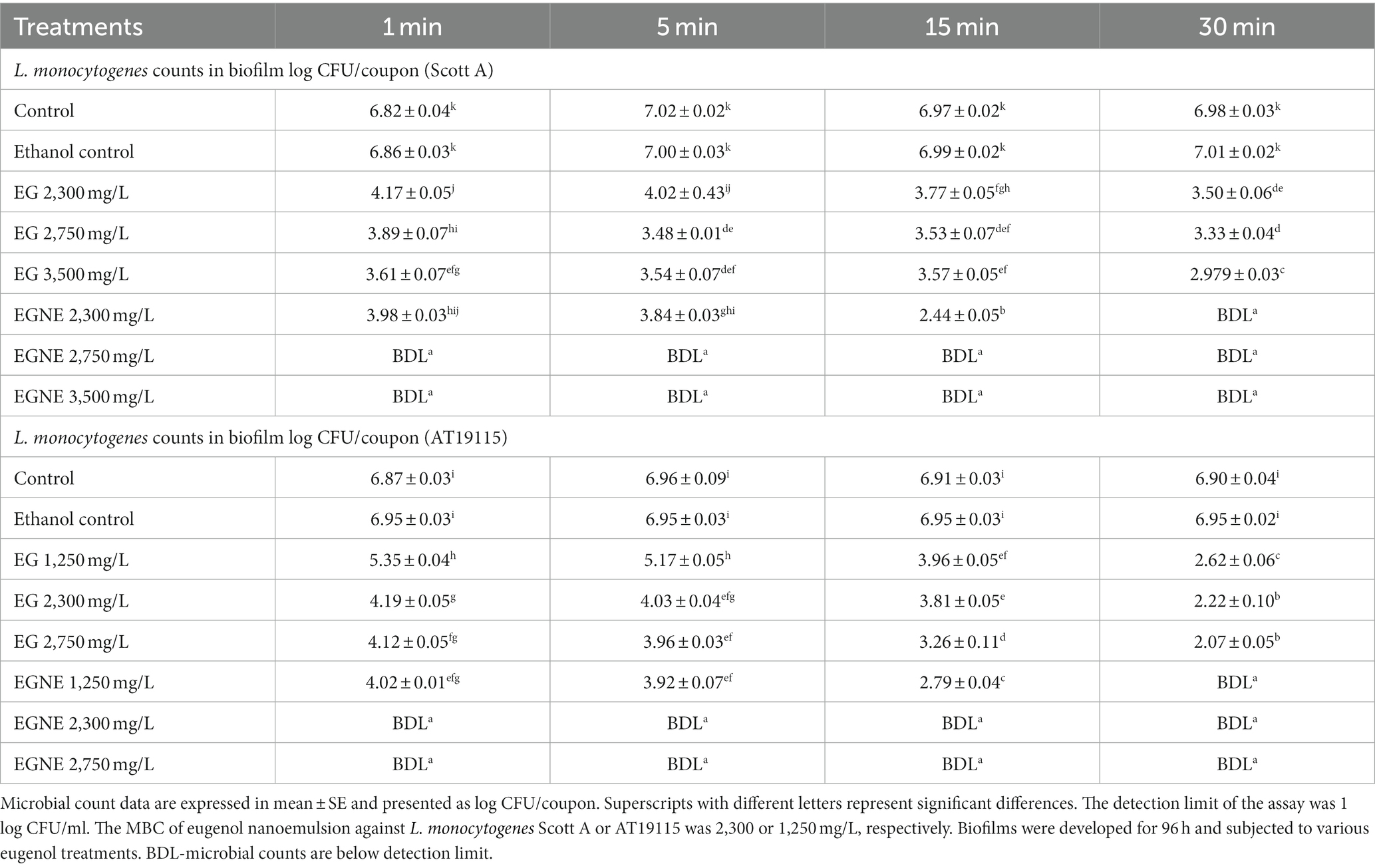
Table 3. Effect of minimum bactericidal concentration of EGNE in inactivating L. monocytogenes biofilms developed at 25°C on stainless steel surface.
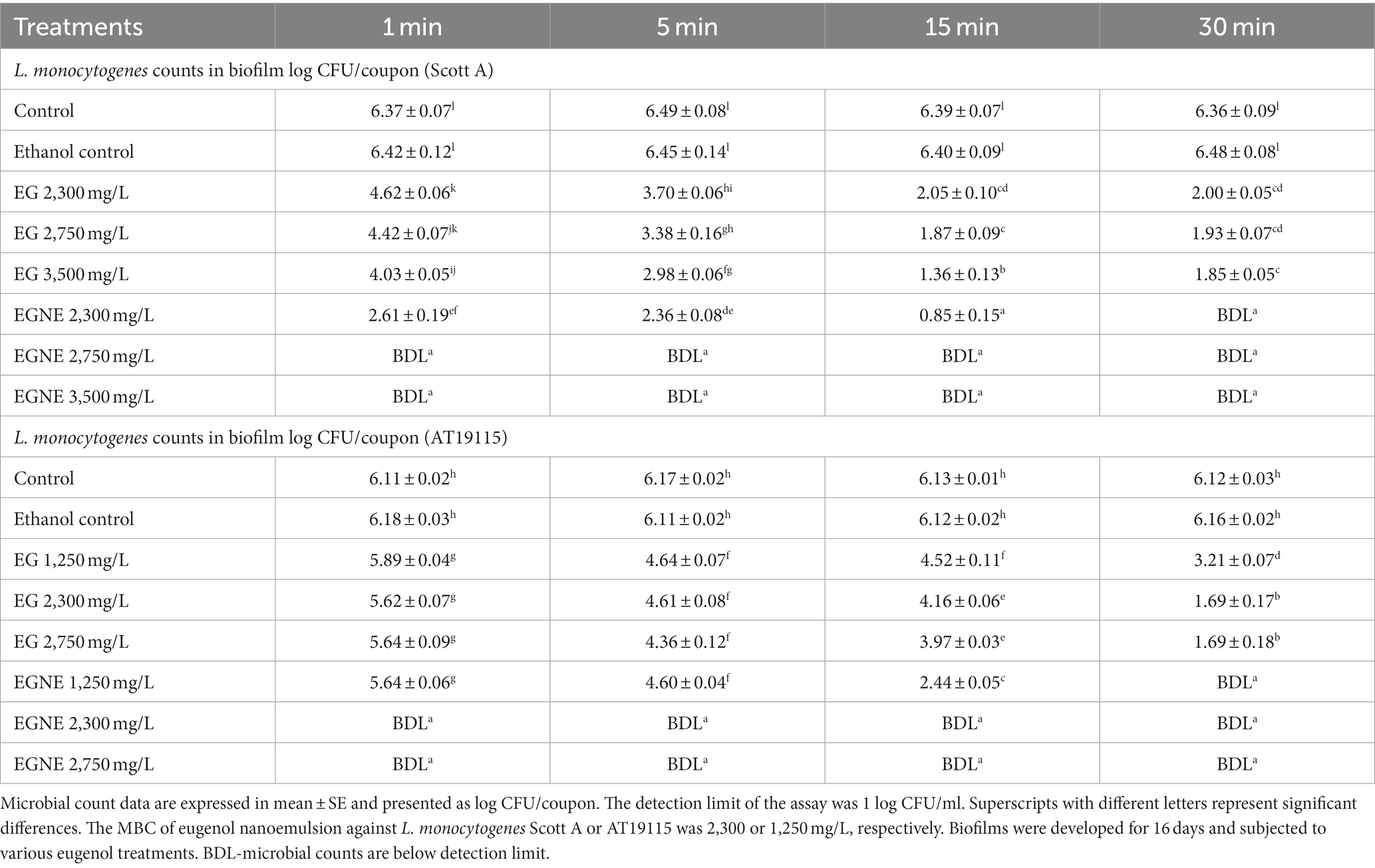
Table 4. Effect of minimum bactericidal concentration of EGNE in inactivating L. monocytogenes biofilms developed at 10°C on stainless steel surface.
3.4.1. Visualization of live-dead L. monocytogenes cells in biofilm using confocal microscopy
Qualitative confocal microscopy revealed that the control biofilm (not exposed to EG or EGNE treatments) consists of a dense and uniform layer of live cells stained green by SYTO dye. Biofilm treated with EGNE showed the presence of dead cells stained red by propidium iodide dye (Figure 5A-C).
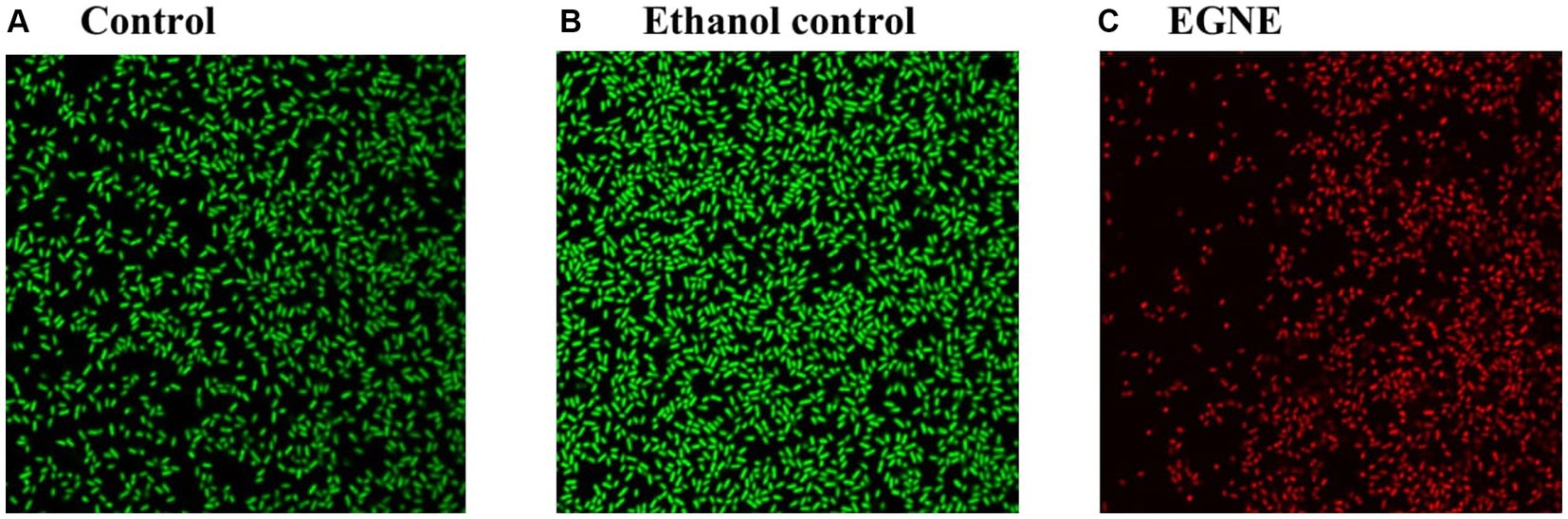
Figure 5. Effect of minimum bactericidal concentration of EGNE on L. monocytogenes Scott A biofilms developed at 25°C by scanning confocal microscopy. (A) Control. (B) Ethanol control. (C) EGNE. Green and red color indicates live or dead bacterial cells respectively.
4. Discussion
The presence of L. monocytogenes biofilms in food processing facilities increases the risk of product contamination and subsequent human infections (Chmielewski and Frank, 2006; Mazaheri et al., 2021). Therefore, developing efficient biofilm control strategies is critical for safeguarding human health. Numerous studies conducted in the past decade have examined the effectiveness of phytochemicals as a viable strategy for controlling biofilms (Borges et al., 2016; Unlu et al., 2018; Wagle et al., 2019; Gonçalves et al., 2023). However, the low solubility of phytochemicals is a significant disadvantage for uniform application, scale-up operations, and cost. To overcome this challenge, we developed a nanoemulsion of eugenol using food-grade emulsifiers and assessed its effectiveness in controlling L. monocytogenes biofilms. Encapsulation of phytochemicals using emulsifiers enhances their water dispersibility, resistance to environmental conditions, and antimicrobial potency (Ray et al., 2016; Ferreira and Nunes, 2019). Several researchers (Moghimi et al., 2016; Rinaldi et al., 2021) including our lab (Balasubramanian et al., 2022; Allen et al., 2023) have reported that antibacterial potency of volatile essential oils is augmented in nanoemulsion form as compared to its corresponding oil form against various food borne pathogens. In this study, we used gum Arabic and lecithin which are food-grade emulsifiers and are widely employed in the food industry (Patel and Goyal, 2015; Sethuraman and Rajendran, 2019). Eugenol nanoemulsion was prepared by high-energy sonication which uses a minimal concentration of emulsifiers with high efficiency in generating small particle sizes (Kaltsa et al., 2013; Espinosa-Sandoval et al., 2021). Prepared nanoemulsion exhibited stability for a minimum of 4 months at 25 or 4°C (Table 1). This could be attributed to the hydrophilic portion of gum Arabic that provides stability against droplet aggregation through steric and electrostatic repulsion (Anarjan and Tan, 2013).
Both strains of L. monocytogenes (Scott A, 19115) exhibited the ability to attach and form biofilm on stainless steel coupons, regardless of the temperature (25 or 10°C). Biofilm counts recovered from the stainless steel coupons were ~7 log CFU/coupon after 96 h of incubation at 25°C. Similar results were reported by Upadhyay et al. (2013), where ~7.5 log CFU/coupon of L. monocytogenes counts in biofilm formed on a stainless steel surface were recovered at 25°C. L. monocytogenes adaptation to low temperatures is one of the crucial factors for its survival and persistence in the food processing areas (Santos et al., 2019). We observed that both strains of L. monocytogenes formed biofilms at 10°C. The pathogen load reached 6–7 log CFU/coupon by 8 days of incubation. Similar to our study, Melian et al. (2022) reported that various strains of L. monocytogenes including Scott A were able to form biofilm at 10°C.
Generally, biofilm formation by bacterial pathogens occurs in five stages. These include (1) initial/reversible attachment to a surface, (2) colonization/irreversible attachment to a surface, (3) proliferation/micro-colony formation, (4) maturation, and (5) dispersion (Srinivasan et al., 2021). During the initial/reversible attachment phase, the free-swimming planktonic cells attach to the surface through weak Van der Wall or electrostatic forces. During this stage, it is relatively easier to prevent biofilm formation. In the subsequent stage, the bacteria attach irreversibly via collagen-binding proteins, flagella, or pili. During the proliferation and maturation phases, significant EPS production provides a 3D structure to the biofilm and protects the underlying bacterial population from various environmental stressors (Cheng et al., 2007). Therefore, to eradicate a mature biofilm, a significantly higher concentration of disinfectants is needed. In our study, we tested the efficacy of EGNE in inhibiting biofilm formation and their efficacy in eradicating a fully formed biofilm. We observed that EGNE was more effective in inhibiting the biofilm formation compared to its oil form. Previous research has shown the antibiofilm efficacy of EG (Upadhyay et al., 2013; Liu et al., 2021). The improved efficacy of EGNE could be due to better distribution of the active compound in the medium via nanoemulsion. In L. monocytogenes, cellular motility is a major factor in attachment and colonization during the initial stages of biofilm formation (Lemon et al., 2008; Bonsaglia et al., 2014). One of the possible mechanisms of action of EGNE could be due to the reduction of bacterial motility. In our study, L. monocytogenes in the presence of SIC of EGNE reduced the motility of L. monocytogenes by ~80% when compared to control suggesting that the EGNE had a direct effect on bacterial motility. In another study, Kim et al. (2016) reported that the antibiofilm activity of eugenol was due to suppression of fimbriae production which is one of the critical factors for biofilm formation. In addition to motility, many other factors such as temperature, surface hydrophobicity, virulence, quorum sensing, eDNA, and extracellular polymeric substance are involved in biofilm formation (Ibáñez de Aldecoa et al., 2017; Lou et al., 2019; Xie et al., 2019; Fan et al., 2020). EPS plays a critical role in biofilm survival by maintaining the structure and protecting the biofilm cells from the action of disinfectants. Mu et al. (2021) reported that Staphylococcus epidermidis exposed to quercetin, a plant-derived compound, reduced its biofilm-forming ability by reducing EPS production. In other studies, Wang et al. (2018) reported that D-limonene nanoemulsion inhibited the E. coli biofilm formation by reducing the EPS and swarming motility without inhibiting the cell growth. In agreement with the above findings, we observed that EGNE reduced the EPS production during L. monocytogenes biofilm formation at 25 and 10°C (Figure 2). Previous investigations have demonstrated that the extracellular biofilm matrix is composed of EPS and eDNA (Karygianni et al., 2020; Nwaiwu et al., 2021). The study by Peng et al. (2020) reported that the interconnection of biofilm 3D structures is facilitated by the presence of eDNA and EPS in the surrounding environment in Bacillus subtilis. Moreover, the results of this study contribute to a deeper understanding of the interplay between eDNA and EPS in influencing the three-dimensional structure of biofilms. Similarly, in L. monocytogenes, eDNA plays a major role in biofilm formation and stabilization of the three-dimensional biofilm structure in L. monocytogenes (Nguyen and Burrows, 2014; Kim et al., 2018; Boháčová et al., 2019; Zetzmann et al., 2019).
Anti-QS or quorum quenching is an effective tool in controlling the virulence of pathogens, including biofilm formation (Grandclémen et al., 2016; Paluch et al., 2020). We selected 12 h as the time point for the quorum sensing assay since previous studies have shown that the compound mediates cell-to-cell communication during the mid to late log phase (Lu et al., 2017). In our study, reduced AI-2 levels were observed when L. monocytogenes were cultured in the presence of EG or EGNE. Our results agree with previous findings where eugenol (Zhou et al., 2013) and other essential oils have shown an inhibitory effect on quorum sensing in various bacteria including Salmonella Enteritidis, S. typhimurium (Guillín et al., 2021), and Campylobacter jejuni (Li et al., 2022). While the quorum sensing assay cannot differentiate between reduced production of AI-2 or binding of the compound with EG/EGNE, previous research has shown that eugenol reduces the expression of genes involved in quorum sensing in L. monocytogenes (Upadhyay et al., 2013; Liu et al., 2021). Therefore, the reduced biofilm formation could be due to reduced AI-2 production and impaired cell-to-cell communication within the population of L. monocytogenes involved in biofilm formation. Confocal imaging of L. monocytogenes biofilm developed in the presence of EGNE revealed a reduction in eDNA content in the biofilm along with reduced bacterial load. However, since confocal imaging is qualitative in nature, the development of a quantitative assay is recommended to further investigate the impact of phytochemicals (or their nanoemulsions) on eDNA production in bacteria. Our experiments investigating the effect of higher doses (2,750 and 3,500 mg/L) of EGNE on mature L. monocytogenes biofilm showed rapid inactivation of the biofilm formed at 25 or 10°C. These results are in agreement with previous findings with L. monocytogenes (Upadhyay et al., 2013; Liu et al., 2021), Pseudomonas aeruginosa (Kim and Park, 2013), E. coli O157:H7 (Hu et al., 2020), and C. jejuni (Wagle et al., 2019), where they reported that the natural antimicrobial compounds were able to reduce the biofilm formation by various pathogen by modulating the motility, quorum sensing, and virulence factors. In particular, Liu et al. (2021) reported that sub-MIC of eugenol inhibited L. monocytogenes biofilms by suppressing the QS system rather than by inhibiting flagella formation. These results corroborate with Upadhyay et al. (2013), who demonstrated that eugenol reduced the biofilm formation in L. monocytogenes by regulating the motility and genes involved in the quorum sensing and virulence.
In conclusion, EGNE was effective in inhibiting biofilm formation and inactivating mature biofilms of L. monocytogenes on stainless steel coupons at 25 or 10°C. In addition, EGNE reduced pathogen motility, ability to produce EPS, eDNA abundance, and quorum sensing activity which plays a major role in biofilm formation. Both Scott A and AT19115 strains of L. monocytogenes exhibited similar sensitivity to EGNE treatments. Conducting a follow-up study to examine the correlation between reduced biofilm formation and a corresponding decrease in pathogen load on food products could provide valuable insights into the efficacy of phytochemical nanoemulsions as a potential disinfectant for the food industry.
Data availability statement
The original contributions presented in the study are included in the article/supplementary material, further inquiries can be directed to the corresponding author.
Author contributions
BB: Conceptualization, Formal analysis, Investigation, Methodology, Software, Validation, Writing – original draft, Writing – review & editing. JX: Methodology, Writing – review & editing. YL: Supervision, Writing – review & editing. AU: Conceptualization, Formal analysis, Funding acquisition, Investigation, Project administration, Resources, Validation, Visualization, Writing – review & editing.
Funding
The author(s) declare financial support was received for the research, authorship, and/or publication of this article. This research was supported by a Small and Medium Sized Farms program grant (#2020-69006-31684; Accession #1022281) from the USDA National Institute of Food and Agriculture.
Conflict of interest
The authors declare that the research was conducted in the absence of any commercial or financial relationships that could be construed as a potential conflict of interest.
Publisher’s note
All claims expressed in this article are solely those of the authors and do not necessarily represent those of their affiliated organizations, or those of the publisher, the editors and the reviewers. Any product that may be evaluated in this article, or claim that may be made by its manufacturer, is not guaranteed or endorsed by the publisher.
References
Allen, J., Balasubramanian, B., Rankin, K., Shah, T., Donoghue, A. M., Upadhyaya, I., et al. (2023). Trans-cinnamaldehyde nanoemulsion wash inactivates Salmonella Enteritidis on shelled eggs without affecting egg color. Poult. Sci. 102:102523. doi: 10.1016/j.psj.2023.102523
Al-Shabib, N. A., Husain, F. M., Ahmad, I., and Baig, M. H. (2017). Eugenol inhibits quorum sensing and biofilm of toxigenic MRSA strains isolated from food handlers employed in Saudi Arabia. Biotechnol. Biotechnol. Equip. 31, 387–396. doi: 10.1080/13102818.2017.1281761
Anarjan, N., and Tan, C. (2013). Physico-chemical stability of astaxanthin nanodispersions prepared with polysaccharides as stabilizing agents. Int. J. Food Sci. Technol. 64, 744–748. doi: 10.3109/09637486.2013.783001
Ashrafudoulla, M., Mizan, M. F. R., Ha, A. J., Park, S. H., and Ha, S. D. (2020). Antibacterial and antibiofilm mechanism of eugenol against antibiotic resistance Vibrio parahaemolyticus. Food Microbiol. 91:103500. doi: 10.1016/j.fm.2020.103500
Balasubramanian, B., Shah, T., Allen, J., Rankin, K., Xue, J., Luo, Y., et al. (2022). Eugenol nanoemulsion inactivates L. monocytogenes, Salmonella enteritidis, and Escherichia coli O157:H7 on cantaloupes without affecting rind color. Front. Sustain. Food Syst. 6:984391. doi: 10.3389/fsufs.2022.984391
Boháčová, M., Pazlarová, J., Fuchsová, V., Švehláková, T., and Demnerová, K. (2019). Quantitative evaluation of biofilm extracellular DNA by fluorescence-based techniques. Folia Microbiol. 64, 567–577. doi: 10.1007/s12223-019-00681-8
Bonsaglia, E. C. R., Silva, N. C. C., Fernades Júnior, A., Araújo Júnior, J. P., Tsunemi, M. H., and Rall, V. L. M. (2014). Production of biofilm by Listeria monocytogenes in different materials and temperatures. Food Control 35, 386–391. doi: 10.1016/j.foodcont.2013.07.023
Borges, A., Abreu, A. C., Dias, C., Saavedra, M. J., Borges, F., and Simões, M. (2016). New perspectives on the use of phytochemicals as an emergent strategy to control bacterial infections including biofilms. Molecules 21:877. doi: 10.3390/molecules21070877
Castillo, S., Heredia, N., Arechiga-Carvajal, E., and García, S. (2014). Citrus extracts as inhibitors of quorum sensing, biofilm formation and motility of Campylobacter jejuni. Food Biotechnol. 28, 106–122. doi: 10.1080/08905436.2014.895947
Ceri, H., Olson, M., Morck, D., Storey, D., Read, R., Buret, A., et al. (2001). The MBEC assay system: multiple equivalent biofilms for antibiotic and biocide susceptibility testing. Methods Enzymol. 337, 377–385. doi: 10.1016/s0076-6879(01)37026-x
Cheng, C., Zhang, Z., Chen, S., Bryersand, J. D., and Jiang, S. (2007). Inhibition of bacterial adhesion and biofilm formation on zwitterionic surfaces. Biomaterials 28, 4192–4199. doi: 10.1016/j.biomaterials.2007.05.041
Cherifi, T., Arsenault, J., Pagotto, F., Quessy, S., Côté, J. C., Neira, K., et al. (2020). Distribution, diversity and persistence of Listeria monocytogenes in swine slaughterhouses and their association with food and human listeriosis strains. PLoS One 15:e0236807. doi: 10.1371/journal.pone.0236807
Chmielewski, R. A. N., and Frank, J. F. (2006). Biofilm formation and control in food processing facilities. Compr. Rev. Food Sci. Food Saf. 2, 22–32. doi: 10.1111/j.1541-4337.2003.tb00012.x
Clayton, G. E., Thorn, R. M. S., and Reynolds, D. M. (2021). The efficacy of chlorine-based disinfectants against planktonic and biofilm bacteria for decentralised point-of-use drinking water. NPJ Clean. Water 4, 1–8. doi: 10.1038/s41545-021-00139-w
CLSI (2019). Performance standards for antimicrobial susceptibility testing. 29th edition Wayne, PA: Clinical and Laboratory Standards Institute.
Costa, O. Y. A., Raaijmakers, J. M., and Kuramae, E. E. (2018). Microbial extracellular polymeric substances: ecological function and impact on soil aggregation. Front. Microbiol. 9:1636. doi: 10.3389/fmicb.2018.01636
Coughlan, L. M., Cotter, P. D., Hill, C., and Alvarez-Ordóñez, A. (2016). New weapons to fight old enemies: novel strategies for the (bio)control of bacterial biofilms in the food industry. Front. Microbiol. 7:1641. doi: 10.3389/fmicb.2016.01641
Djordjevic, D., Wiedmann, M., and McLandsborough, L. A. (2002). Microtiter plate assay for assessment of Listeria monocytogenes biofilm formation. Appl. Environ. Microbiol. 68, 2950–2958. doi: 10.1128/AEM.68.6.2950-2958.2002
Donato, F., and Zani, C. (2010). Chronic exposure to organochlorine compounds and health effects in adults: cancer, non-Hodgkin lymphoma. Review of literature. Annali di Igiene: Medicina Preventiva e di Comunita 22, 357–367.
Espinosa-Sandoval, L., Ochoa-Martínez, C., Ayala-Aponte, A., Pastrana, L., Gonçalves, C., and Cerqueira, M. A. (2021). Polysaccharide-based multilayer nano-emulsions loaded with oregano oil: production, characterization, and in vitro digestion assessment. Nano 11:878. doi: 10.3390/nano11040878
Fagerlund, A., Wagner, E., Møretrø, T., Heir, E., Moen, B., Rychli, K., et al. (2022). Pervasive Listeria monocytogenes is common in the Norwegian food system and is associated with increased prevalence of stress survival and resistance determinants. Appl. Environ. Microbiol. 88:e0086122. doi: 10.1128/aem.00861-22
Fan, Y., Qiao, J., Lu, Z., Fen, Z., Tao, Y., Lv, F., et al. (2020). Influence of different factors on biofilm formation of Listeria monocytogenes and the regulation of cheY gene. Food Res. Int. 137:109405. doi: 10.1016/j.foodres.2020.109405
Ferreira, C. D., and Nunes, I. L. (2019). Oil nanoencapsulation: development, application, and incorporation into the food market. Nanoscale Res. Lett. 14:9. doi: 10.1186/s11671-018-2829-2
Ferreira, V., Wiedmann, M., Teixeira, P., and Stasiewicz, M. J. (2014). L. monocytogenes persistence in food-associated environments: epidemiology, strain characteristics, and implications for public health. J. Food Prot. 77, 150–170. doi: 10.4315/0362-028X.JFP-13-150
FSIS (2012). Available at: https://www.fsis.usda.gov/shared/PDF/Controlling_LM_RTE_guideline_0912.pdf [Accessed May 26, 2023].
FSIS (2016). Available at: https://www.fsis.usda.gov/inspection/compliance-guidance/sanitation-performance-standards-compliance-guide [Accessed May 26, 2023].
Galié, S., García-Gutiérrez, C., Miguélez, E. M., Villar, C. J., and Lombó, F. (2018). Biofilms in the food industry: health aspects and control methods. Front. Microbiol. 9:898. doi: 10.3389/fmicb.2018.00898
Garmyn, D., Gal, L., Lemaitre, J. P., Hartmann, A., and Piveteau, P. (2009). Communication and autoinduction in the species Listeria monocytogenes: a central role for the agr system. Commun. Integr. Biol. 2, 371–374. doi: 10.4161/cib.2.4.8610
Gonçalves, A. S., Leitão, M. M., Simões, M., and Borges, A. (2023). The action of phytochemicals in biofilm control. Nat. Prod. Rep. 40, 595–627. doi: 10.1039/D2NP00053A
Grandclémen, C., Tannieres, M., Moréra, S., Dessaux, Y., and Faure, D. (2016). Quorum quenching: role in nature and applied developments. FEMS Microbiol. Rev. 40, 86–116. doi: 10.1093/femsre/fuv038
Gray, J., Chandry, P. S., Kaur, M., Kocharunchitt, C., Fanning, S., Bowman, J. P., et al. (2021). Colonisation dynamics of Listeria monocytogenes strains isolated from food production environments. Sci. Rep. 11:12195. doi: 10.1038/s41598-021-91503-w
Guillín, Y., Cáceres, M., Torres, R., Stashenko, E., and Ortiz, C. (2021). Effect of essential oils on the inhibition of biofilm and quorum sensing in salmonella enteritidis 13076 and salmonella typhimurium 14028. Antibiotics (Basel) 10:1191. doi: 10.3390/antibiotics10101191
Haase, J. K., Didelot, X., Lecuit, M., and Korkeala, H. (2014). L. monocytogenes MLST study group; Achtman M. The ubiquitous nature of Listeria monocytogenes clones: a large-scale multilocus sequence typing study. Environ. Microbiol. 16, 405–416. doi: 10.1111/1462-2920.12342
Hu, Q., Hannah, G., Upadhyaya, I., Venkitanarayanan, K., and Luo, Y. (2016). Antimicrobial eugenol nanoemulsion prepared by gum arabic and lecithin and evaluation of drying technologies. Int. J. Biol. Macromol. 87, 130–140. doi: 10.1016/j.ijbiomac.2016.02.051
Hu, W. S., Min Nam, D., Kim, J. S., and Koo, O. K. (2020). Synergistic anti-biofilm effects of Brassicaceae plant extracts in combination with proteinase K against Escherichia coli O157:H7. Sci. Rep. 10:21090. doi: 10.1038/s41598-020-77868-4
Ibáñez de Aldecoa, A. L., Zafra, O., and González-Pastor, J. E. (2017). Mechanisms and regulation of extracellular DNA release and its biological roles in microbial communities. Front. Microbiol. 8:1390. doi: 10.3389/fmicb.2017.01390
Johny, A. K., Darre, M. J., Donoghue, A. M., Donoghue, D. J., and Venkitanarayanan, K. (2010). Antibacterial effect of trans-cinnamaldehyde, eugenol, carvacrol, and thymol on Salmonella enteritidis and Campylobacter jejuni in chicken cecal contents in vitro. J. Appl. Poult. Res. 19, 237–244. doi: 10.3382/japr.2010-00181
Kaltsa, O., Michon, C., Yanniotis, S., and Mandala, I. (2013). Ultrasonic energy input influence on the production of sub-micron o/w emulsions containing whey protein and common stabilizers. Ultrason. Sonochem. 20, 881–891. doi: 10.1016/j.ultsonch.2012.11.011
Karygianni, L., Ren, Z., Koo, H., and Thurnheer, T. (2020). Biofilm matrixome: extracellular components in structured microbial communities. Trends Microbiol. 28, 668–681. doi: 10.1016/j.tim.2020.03.016
Kim, H., Bang, J., Beuchat, L. R., and Ryu, J. H. (2008). Fate of Enterobacter sakazakii attached to or in biofilms on stainless steel upon exposure to various temperatures or relative humidities. J. Food Prot. 71, 940–945. doi: 10.4315/0362-028x-71.5.940
Kim, M., Jeon, J., and Kim, J. (2018). Streptococcus mutans extracellular dna levels depend on the number of bacteria in a biofilm. Sci. Rep. 8:13313. doi: 10.1038/s41598-018-31275-y
Kim, Y. G., Lee, J. H., Gwon, G., Kim, S. I., Park, J. G., and Lee, J. (2016). Essential oils and eugenols inhibit biofilm formation and the virulence of Escherichia coli O157:H7. Sci. Rep. 6:36377. doi: 10.1038/srep36377
Kim, H. S., and Park, H. D. (2013). Ginger extract inhibits biofilm formation by Pseudomonas aeruginosa PA14. PLoS One 8:e76106. doi: 10.1371/journal.pone.0076106
Korany, A. M., Hua, Z., Green, T., Hanrahan, I., El-Shinawy, S. H., El-Kholy, A., et al. (2018). Efficacy of ozonated water, chlorine, chlorine dioxide, quaternary ammonium compounds and peroxyacetic acid against L. monocytogenes biofilm on polystyrene surfaces. Front. Microbiol. 9:2296. doi: 10.3389/fmicb.2018.02296
Lemon, K. P., Earl, A. M., Vlamakis, H. C., Aguilar, C., and Kolter, R. (2008). Biofilm development with an emphasis on Bacillus subtilis. Curr. Top. Microbiol. Immunol. 322, 1–16. doi: 10.1007/978-3-540-75418-
Leong, D., Alvarez-Ordóñez, A., and Jordan, K. (2014). Monitoring occurrence and persistence of L. monocytogenes in foods and food processing environments in the Republic of Ireland. Front. Microbiol. 5:436. doi: 10.3389/fmicb.2014.00436
Li, S., Chan, K. K., Hua, M. Z., Gölz, G., and Lu, X. (2022). Inhibition of AI-2 quorum sensing and biofilm formation in Campylobacter jejuni by decanoic and lauric acids. Front. Microbiol. 12:811506. doi: 10.3389/fmicb.2021.811506
Liu, Y., Wu, L., Han, J., Dong, P., Luo, X., Zhang, Y., et al. (2021). Inhibition of biofilm formation and related gene expression of Listeria monocytogenes in response to four natural antimicrobial compounds and sodium hypochlorite. Front. Microbiol. 11:617473. doi: 10.3389/fmicb.2020.617473
Lou, Z., Letsididi, K. S., Yu, F., Pei, Z., Wang, H., and Letsididi, R. (2019). Inhibitive effect of eugenol and its Nanoemulsion on quorum sensing–mediated virulence factors and biofilm formation by Pseudomonas aeruginosa. J. Food Prot. 82, 379–389. doi: 10.4315/0362-028X.JFP-18-196
Lu, S. Y., Zhao, Z., Avillan, J. J., Liu, J., and Call, D. R. (2017). Autoinducer-2 quorum sensing contributes to regulation of Microcin PDI in Escherichia coli. Front. Microbiol. 8:2570. doi: 10.3389/fmicb.2017.02570
Lunden, J. M., Autio, T. J., and Korkeala, H. J. (2002). Transfer of persistent Listeria monocytogenes contamination between food-processing plants associated with a dicing machine. J. Food Prot. 65, 1129–1133. doi: 10.4315/0362-028x-65.7.1129
Malik, H., Rajagunalan, S., Kumar, M. S., Kataria, J. L., Anjay, P., Sachan, S., et al. (2017). Assessment of antibiotics effect on planktonic and biofilm forms of campylobacter isolates. Isr. J. Vet. Med. 72:4.
Malley, T. J., Butts, J., and Wiedmann, M. (2015). Seek and destroy process: Listeria monocytogenes process controls in the ready-to-eat meat and poultry industry. J. Food Prot. 78, 436–445. doi: 10.4315/0362-028X.JFP-13-507
Mazaheri, T., Cervantes-Huamán, B. R. H., Bermúdez-Capdevila, M., Ripolles-Avila, C., and Rodríguez-Jerez, J. J. (2021). Listeria monocytogenes biofilms in the food industry: is the current hygiene program sufficient to combat the persistence of the pathogen? Microorganisms 9:181. doi: 10.3390/microorganisms9010181
Melian, C., Bentencourt, E., Castellano, P., Ploper, D., Vignolo, G., and Mendoza, L. M. (2022). Biofilm genes expression of Listeria monocytogenes exposed to Latilactobacillus curvatus bacteriocins at 10 °C. Int. J. Food Microbiol. 370:109648. doi: 10.1016/j.ijfoodmicro.2022.109648
Melian, C., Segli, F., Gonzalez, R., Vignolo, G., and Castellano, P. (2019). Lactocin AL705 as quorum sensing inhibitor to control Listeria monocytogenes biofilm formation. J. Appl. Microbiol. 127, 911–920. doi: 10.1111/jam.14348
Moghimi, R., Aliahmadi, A., McClements, D. J., and Rafati, H. (2016). Investigations of the effectiveness of nanoemulsions from sage oil as antibacterial agents on some food borne pathogens. Food Sci. Technol. 71, 69–76. doi: 10.1016/j.lwt.2016.03.018
Mu, Y., Zeng, H., and Chen, W. (2021). Quercetin inhibits biofilm formation by decreasing the production of EPS and altering the composition of EPS in Staphylococcus epidermidis. Front. Microbiol. 12:631058. doi: 10.3389/fmicb.2021.631058
Murugan, K., Selvanayaki, K., and Al-Sohaibani, S. (2016). Urinary catheter indwelling clinical pathogen biofilm formation, exopolysaccharide characterization and their growth influencing parameters. Saudi J. Biol. Sci. 23, 150–159. doi: 10.1016/j.sjbs.2015.04.016
Nguyen, U. T., and Burrows, L. L. (2014). DNase I and proteinase K impair Listeria monocytogenes biofilm formation and induce dispersal of pre-existing biofilms. Int. J. Food Microbiol. 187, 26–32. doi: 10.1016/j.ijfoodmicro.2014.06.025
Niu, C., and Gilbert, E. S. (2004). Colorimetric method for identifying plant essential oil components that affect biofilm formation and structure. Appl. Environ. Microbiol. 70, 6951–6956. doi: 10.1128/AEM.70.12.6951-6956.2004
Nwaiwu, O., Wong, L., Lad, M., Foster, T., MacNaughtan, W., and Rees, C. (2021). Properties of the extracellular polymeric substance layer from minimally grown planktonic cells of Listeria monocytogenes. Biomol. Ther. 11:331. doi: 10.3390/biom11020331
Paluch, E., Rewak-Soroczyńska, J., Jędrusik, I., Mazurkiewicz, E., and Jermakow, K. (2020). Prevention of biofilm formation by quorum quenching. Appl. Microbiol. Biotechnol. 104, 1871–1881. doi: 10.1007/s00253-020-10349-w
Pan, Y. K., Breidt, Jr., and Kathariou, S. (2006). Resistance of L. monocytogenes biofilms to sanitizing agent in a simulated food-processing environment. Appl. Environ. Microbiol. 72, 7711–7717. doi: 10.1128/AEM.01065-06
Parish, M. E., Beuchat, L. R., Suslow, T. V., Harris, L. J., Garrett, G. H., Farber, J. N., et al. (2003). Methods to reduce/eliminate pathogens from fresh and fresh-cut produce. Compr. Rev. Food Sci. Food Saf. 2, 161–173. doi: 10.1111/j.1541-4337.2003.tb00033.x
Patel, S., and Goyal, A. (2015). Applications of natural polymer gum Arabic: a review. Int. J. Food Prop. 18, 986–998. doi: 10.1080/10942912.2013.809541
Peng, N., Cai, P., Mortimer, M., Wu, Y., Gao, C., and Huang, Q. (2020). The exopolysaccharide–eDNA interaction modulates 3D architecture of Bacillus subtilis biofilm. BMC Microbiol. 20:115. doi: 10.1186/s12866-020-01789-5
Radoshevich, L., and Cossart, P. (2018). Listeria monocytogenes: towards a complete picture of its physiology and pathogenesis. Nat. Rev. Microbiol. 16, 32–46. doi: 10.1038/nrmicro.2017.126
Ray, S., Raychaudhuri, U., and Chakraborty, R. (2016). An overview of encapsulation of active compounds used in food products by drying technology. Food Biosci. 13, 76–83. doi: 10.1016/j.fbio.2015.12.009
Rinaldi, F., Maurizi, L., Conte, A. L., Marazzato, M., Maccelli, A., Crestoni, M. E., et al. (2021). Nanoemulsions of Satureja montana essential oil: antimicrobial and antibiofilm activity against avian Escherichia coli strains. Pharmaceutics 13:134. doi: 10.3390/pharmaceutics13020134
Santos, T., Viala, D., Chambon, C., Esbelin, J., and Hébraud, M. (2019). Listeria monocytogenes biofilm adaptation to different temperatures seen through shotgun proteomics. Front. Nutr. 6:89. doi: 10.3389/fnut.2019.00089
Scallan, E., Hoekstra, R. M., Angulo, F. J., Tauxe, R. V., Widdowson, M. A., Roy, S. L., et al. (2011). Foodborne illness acquired in the United States--major pathogens. Emerg. Infect. Dis. 17, 7–15. doi: 10.3201/eid1701.p11101
Sela, S., Frank, S., Belausov, E., and Pinto, R. (2006). A mutation in the luxS gene influences Listeria monocytogenes biofilm formation. Appl. Environ. Microbiol. 72, 5653–5658. doi: 10.1128/AEM.00048-06
Sethuraman, S., and Rajendran, K. (2019). Is gum Arabic a good emulsifier due to CH…π interactions? How urea effectively destabilizes the hydrophobic CH…π interactions in the proteins of gum Arabic than amides and GuHCl? ACS Omega 4, 16418–16428. doi: 10.1021/acsomega.9b01980
Skowron, K., Wałecka-Zacharska, E., Grudlewska, K., Gajewski, P., Wiktorczyk, N., Wietlicka-Piszcz, M., et al. (2019). Disinfectant susceptibility of biofilm formed by Listeria monocytogenes under selected environmental conditions. Microorganisms 7:280. doi: 10.3390/microorganisms7090280
Soumet, C., Ragimbeau, C., and Maris, P. (2005). Screening of benzalkonium chloride resistance in Listeria monocytogenes strains isolated during cold smoked fish production. Lett. Appl. Microbiol. 41, 291–296. doi: 10.1111/j.1472-765X.2005.01763.x
Srinivasan, R., Santhakumari, S., Poonguzhali, P., Geetha, M., Dyavaiah, M., and Xiangmin, L. (2021). Bacterial biofilm inhibition: a focused review on recent therapeutic strategies for combating the biofilm mediated infections. Front. Microbiol. 12:676458. doi: 10.3389/fmicb.2021.676458
Stoller, A., Stevens, M. J. A., Stephan, R., and Guldimann, C. (2019). Characteristics of Listeria monocytogenes strains persisting in a meat processing facility over a 4-year period. Pathogens 8:32. doi: 10.3390/pathogens8010032
Tompkin, R. B. (2002). Control of L. monocytogenes in the food processing environment. J. Food Prot. 65, 709–725. doi: 10.4315/0362-028x-65.4.709
Unlu, A., Sar, T., Seker, G., Erman, A. G., Kalpar, E., and Akbas, M. Y. (2018). Biofilm formation by Staphylococcus aureus strains and their control by selected phytochemicals. Int. J. Dairy Technol. 71, 637–646. doi: 10.1111/1471-0307.12520
Upadhyay, A., Upadhyaya, I., Kollanoor-Johny, A., and Venkitanarayanan, K. (2013). Antibiofilm effect of plant derived antimicrobials on L. monocytogenes. Food Microbiol. 36:79. doi: 10.1016/j.fm.2013.04.010
Wagle, B. R., Upadhyay, A., Upadhyaya, I., Shrestha, S., Arsi, K., Liyanage, R., et al. (2019). Trans-cinnamaldehyde, eugenol and carvacrol reduce Campylobacter jejuni biofilms and modulate expression of select genes and proteins. Front. Microbiol. 10:1837. doi: 10.3389/fmicb.2019.01837
Wagner, V. E., and Iglewski, B. H. (2008). P. aeruginosa biofilms in CF infection. Clin. Rev. Allergy Immunol. 35, 124–134. doi: 10.1007/s12016-008-8079-9
Wang, R., Vega, P., Xu, Y., Chen, C. Y., and Irudayaraj, J. (2018). Exploring the anti-quorum sensing activity of a d-limonene nanoemulsion for Escherichia coli O157:H7. J. Biomed. Mater. Res. A 106, 1979–1986. doi: 10.1002/jbm.a.36404
WHO (2018). Available at: https://www.who.int/news-room/fact-sheets/detail/listeriosis#:~:text=Listeria%20monocytogenes%20are%20widely%20distributed,by%20the%20bacterium%20Listeria%20monocytogenes [Accessed February 16, 2023].
WHO (2022). Available at: https://www.who.int/news-room/fact-sheets/detail/food-safety [Accessed February 14, 2023].
Xie, T. T., Zeng, H., Ren, X. P., Wang, N., Chen, Z. J., Zhang, Y., et al. (2019). Antibiofilm activity of three Actinomycete strains against Staphylococcus epidermidis. Lett. Appl. Microbiol. 68, 73–80. doi: 10.1111/lam.13087
Yalkowsky, S. H., and Banerjee, S. (1992). “Aqueous solubility” in Methods of estimation for organic compounds (New York, NY: Marcel Dekker).
Zameer, F., Gopal, S., Krohne, G., and Kreft, J. (2010). Development of a biofilm model for Listeria monocytogenes EGD-e. World J. Microbiol. Biotechnol. 26, 1143–1147. doi: 10.1007/S11274-009-0271-4
Zetzmann, M., Bucur, F. I., Crauwels, P., Borda, D., Nicolau, A. I., Grigore-Gurgu, L., et al. (2019). Characterization of the biofilm phenotype of a L. monocytogenes mutant deficient in agr peptide sensing. Microbiol. Open 8:e00826. doi: 10.1002/mbo3.826
Zhang, S., and Farber, J. M. (1996). The effects of various disinfectants against L. monocytogenes on fresh-cut vegetables. Food Microbiol. 13, 311–321. doi: 10.1006/fmic.1996.0037
Zhang, Y., Wang, Y., Zhu, X., Cao, P., Wei, S., and Lu, Y. (2017). Antibacterial and antibiofilm activities of eugenol from essential oil of Syzygium aromaticum (L.) Merr. & L. M. Perry (clove) leaf against periodontal pathogen Porphyromonas gingivalis. Microb. Pathog. 113, 396–402. doi: 10.1016/j.micpath.2017.10.054
Zhao, W., You, J., Yin, S., Yang, H., He, S., Feng, L., et al. (2022). Extracellular polymeric substances-antibiotics interaction in activated sludge: a review. Environ. Sci. Ecotechnol. 13:100212. doi: 10.1016/j.ese.2022.100212
Keywords: Listeria monocytogenes, stainless steel, biofilm, eugenol, nanoemulsion, quorum sensing inhibitor
Citation: Balasubramanian B, Xue J, Luo Y and Upadhyay A (2023) Eugenol nanoemulsion reduces Listeria monocytogenes biofilm by modulating motility, quorum sensing, and biofilm architecture. Front. Sustain. Food Syst. 7:1272373. doi: 10.3389/fsufs.2023.1272373
Edited by:
Yishan Yang, Agricultural Research Service (USDA), United StatesReviewed by:
Xinyi Pang, Nanjing University of Finance and Economics, ChinaXiangwu Nou, United States Department of Agriculture (USDA), United States
Copyright © 2023 Balasubramanian, Xue, Luo and Upadhyay. This is an open-access article distributed under the terms of the Creative Commons Attribution License (CC BY). The use, distribution or reproduction in other forums is permitted, provided the original author(s) and the copyright owner(s) are credited and that the original publication in this journal is cited, in accordance with accepted academic practice. No use, distribution or reproduction is permitted which does not comply with these terms.
*Correspondence: Abhinav Upadhyay, YWJoaW5hdi51cGFkaHlheUB1Y29ubi5lZHU=