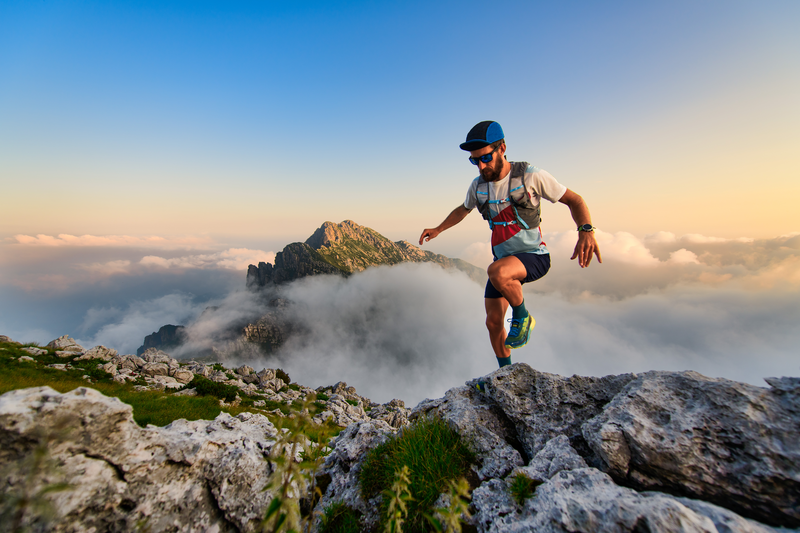
95% of researchers rate our articles as excellent or good
Learn more about the work of our research integrity team to safeguard the quality of each article we publish.
Find out more
ORIGINAL RESEARCH article
Front. Sustain. Food Syst. , 10 October 2023
Sec. Sustainable Food Processing
Volume 7 - 2023 | https://doi.org/10.3389/fsufs.2023.1267075
This article is part of the Research Topic Green Technologies for the Extraction of Bioactive Compounds, its Use for the Production of Nanomaterials, and Their Application in the Food Industry View all 5 articles
Introduction: Curcumin has highly antimicrobial and antioxidant activities but has poor bioavailability and low solubility in water. The current study aimed to assess curcumin nanoparticles (Curcumin-NPs) antibacterial and antioxidant efficacy against some pathogens in chicken fingers at 4 °C/27 days.
Methods: Curcumin-NPs with particle sizes of 80 ± 2 nm were synthesized using a planetary ball-mill and chitosan-gelatin nanoparticle (CS-G-NPs) solution and then placed into chicken fingers at three concentrations, (2, 5, and 10 μg g−1). The physicochemical properties, antioxidant activity, and antibacterial capacity were evaluated.
Results and discussion: Curcumin-NPs showed high phenolic content (67.48 mg GAE g−1) and antioxidant activity (22.47 μg ml−1) at 10 μg g−1 compared to other concentrations and curcumin bulk. Curcumin-NPs showed noticeably greater antibacterial ability (in vitro) against S. aureus (18 mm), E. coli (15 mm), and B. cereus (13 mm). In challenge studies, Curcumin-NPs effectively inhibited the three inoculated pathogens ~3–4 log CFU g−1; in vivo; in chicken fingers kept for up to 27 days, compared to the control. In curcumin-NPs chicken samples, the contents of thiobarbituric acid reactive substances (TBARS) and total volatile base nitrogen (TVB-N) compared to the control were substantially lower (27 days). TEM analysis provided an estimate of the antibacterial mechanism of Curcumin-NPs. The findings demonstrated that Curcumin-NPs at 10 μg g−1 were more successful in reducing microbial load in chicken fingers as well as improving quality parameters, enhancing shelf life, and reducing lipid oxidation in poultry meat products.
Unsafe food is a serious global problem affecting trade and health (Garridogamarro et al., 2023). Food-borne pathogens are a major threat to public health worldwide (Crotta et al., 2022). An estimated 76 million food-borne infections occur annually in the USA, along with 5,000 deaths and 325,000 hospitalizations (Faizy et al., 2022). According to estimates from the WHO, 30% of adults in affluent countries experience food-borne illnesses each year, although food-borne diseases cause the deaths of 2 million people annually in developing nations (Bajpai et al., 2022). Major pathogens liable for more than 90% of food-linked deaths are L. monocytogenes, E. coli O157:H7, Campylobacter, S. aureus, and Salmonella spp. (Owusu-Apenten and Vieira, 2022). Although Egyptian food products have been identified as the source of recent outbreaks, Egypt's annual foodborne illness rate and outbreak rate are currently unknown (EFSA, 2013). Egypt has recently detected a number of cases of foodborne illness involving raw meat and its products (Farag et al., 2022).
Therefore, preventing foodborne illnesses requires controlling the growth of pathogens in food. In this context, various chemical preservatives and antimicrobials are typically used in the food industry to prevent food spoilage and pathogens (Tropea, 2022). However, these synthetic preservatives, such as sodium nitrite and sodium benzoate (Bensid et al., 2022), are harmful to human health. Currently, natural and safe biochemicals, including isolated bioactive compounds and plant extracts, effectively stop foodborne pathogens and food spoilage (Zang et al., 2022; Alqahtani et al., 2023).
Curcumin [(E, E)-1,7-bis(4-hydroxy-3-methoxy-phenyl)-1,6- heptadiene-3,5-ione] is a well-known bioactive compound with an orange-yellow color that was isolated from turmeric (Curcuma longa L.) and is approved (E-100) used in food processing as a color, spice, flavor, and preservative (Jyotirmayee and Mahalik, 2022; Singh et al., 2023). It exhibits excellent antibacterial and antioxidant properties and is non-toxic. Curcumin has long been a staple in daily diets, primarily in Asian countries and occasionally in African nations. It has been extensively used for anti-inflammatory, antioxidant, anticancer, and antimicrobial properties in pharmaceuticals (Urošević et al., 2022). The mechanism of curcumin's antimicrobial activity includes (1) the disruption of the membrane walls of specific bacteria, (2) the damaging effect on bacterial DNA, and (3) membrane leakage of the bacterium, producing a lot of reactive oxygen species, either as singlet oxygen or as hydroxyl radicals (Hewlings and Kalman, 2017; Zheng et al., 2020). Additionally, the structure-based polyphenolic group of curcumin gives it its antioxidant properties (Tylewicz et al., 2018). Curcumin has a hydrophobic phenol group that is rapidly metabolized but has little to no solubility in water (He et al., 2015).
Although curcumin serves several useful purposes, its applications are limited due to its low water solubility, rapid intestine metabolism, slow dissolution rate, and low bioavailability (Maleki Dizaj et al., 2022). Therefore, the bioavailability and antioxidant concentration of curcumin in foods will determine their potency. Consequently, an alternative processing technology that is capable of producing new ingredients with optimized techno-functional and nutritional attributes is required (Wu et al., 2022). Numerous studies have reported a variety of approaches for overcoming this challenge of the low water solubility of curcumin and increasing its bioavailability, particularly nanotechnology (Chen et al., 2022). In this regard, curcumin is a prospective candidate for creating novel natural materials, such as microparticles and nanoparticles to increase their durability against the aforementioned conditions and to take advantage of biological features (Maleki Dizaj et al., 2022).
Nanotechnology is an important cutting-edge technology that has the potential to improve food quality (Malik et al., 2023). There is a wide variety of nanotechniques, such as nanoparticles or nanoemulsions, that have been used to enhance food safety via their strong antioxidant and antibacterial activities (Morsy et al., 2014; Awan et al., 2022), especially in minced beef (Morsy et al., 2018), beef patties (Zhao et al., 2022), and chicken fillets (Niaz et al., 2022). Nanoparticles are perceived to kill bacteria through disruption of DNA replication and essential cellular processes by binding to sulfhydryl or disulfide functional groups on the surfaces of membrane proteins and inducing oxidative stress through the catalysis of reactive oxygen species (Duncan, 2011). Numerous studies have reported that nanomaterials were used as antioxidants, such as garlic nanoparticles (Abdelli et al., 2022) and date seed nanoparticles (Mostafa et al., 2022). There are few reports on chicken finger processing (Barutçu Mazi, 2009; Bozzato et al., 2021), and there is almost no information on chicken fingers containing nano-curcumin. Previous research has shown that, using natural preservatives, such as essential oil (Morsy et al., 2014) and oleaster leaf extract (Yaghoubi et al., 2023), in meat and chicken products improves microbial quality and shelf life. Curcumin nanoparticles are more effective against pathogens, i.e., S. aureus, B. subtilis, E. coli, Pseudomonas aeruginosa, and Penicillium notatum (Bhawana et al., 2011; Chopra et al., 2021). Thus, this study aimed to (i) assess the antimicrobial and antioxidant abilities of Curcumin-NPs against foodborne pathogens and (ii) enhance the quality attributes and safety markers of the chicken finger model stored at 4°C.
Curcumin powder (Cod No. C7727), polyethylene glycol, chitosan (Cod No. 448877), gelatin, 2,2-diphenyl-1-picrylhydrazyl (DPPH), Folin–Ciocalteu, 95% ethanol, casein, sodium carbonate, sodium nitrite, sodium hydroxide, acetic acid, gallic acid, methanol, and acetonitrile were bought from Sigma–Aldrich (St. Louis, MO, USA). The raw chicken filet was supplied from a supermarket in Cairo, Egypt and then moved to the laboratory within 15 min, minced, and kept in the refrigerator. Wheat flour, oat flour, and fat were obtained from a local market in Cairo, Egypt.
Three bacterial strains, E. coli (ATCC 25922), S. aureus (ATCC 6538), and B. cereus (ATCC 6633), were supplied from the Animal Health Research Institute, Dokki, Giza, Egypt. The bacteria were activated in tryptic soy broth (TSB; Biolife, Italy) and cultivated in the same media at 37 ± 1°C for 24 h. The bacterial population of ~6 log10 CFU ml−1 (colony-forming unit) confirmed the count by enumeration on tryptic soy agar.
Then, 200 g of curcumin was minimized, according to the study of Khataee et al. (2017). Briefly, the curcumin powder was crushed by a grinder (Model MC300, Moulinex, France) and then milled by a planetary ball mill (PM 2400, Iran) at 310 rpm/2 h. Under ambient conditions, the ball-milling procedure was used with the following ball mass-to-powder ratio of 10:1. After that, the curcumin was transformed into nanoparticles through a solid dispersion technique. Then, 100 mg of curcumin was dissolved in 5 mL of a polyethylene glycol (PEG) solution (80% w/v). Under constant magnetic stirring (~2 h), the curcumin-PEG complex was added drop-wise into chitosan (3%)-gelatin (1%) nanoparticle (CS-G-NPs; 1:1; v/v) solutions (polysaccharide mixture was 50 ml). The chitosan-gelatin nanoparticle (CS-GNPs) was used as a carrier and improved the solubility of curcumin. Subsequently, NPs were centrifuged at 10,000 rpm for 10 min (Farnia et al., 2016). The size of curcumin nanoparticles (Curcumin-NPs) was measured by a Zetasizer (NS300, UK). The sample was placed in a glass cuvette (1 ml) to avoid bubbles and then placed in a Zetasizer at a temperature of 10–90°C. Curcumin-NPs were kept at −80°C and applied in the experiments.
Total phenolic (TP) was measured based on the study of Hajimahmoodi et al. (2010). TP was expressed as mg of gallic acid equivalent (GAE) per g of curcumin. The total flavonoid (TF) content of curcumin was measured colorimetrically at 510 nm (Formagio et al., 2014). TF was computed as mg rutin equivalents (RE) per gram of curcumin. The analysis was performed in triplicate. The antioxidant ability of curcumin was estimated using DPPH radical scavenging at 517 nm (Liu et al., 2007). The results were expressed as IC50 (μg ml−1) (Ebrahimzadeh et al., 2010).
HPLC-Agilent Technologies (Santa Clara, CA, USA) was used to analyze the phenolic and flavonoid fractions (Ruslay et al., 2007). One gram of curcumin-NPs was added to 50 ml of methanol (70%), sonicated for 30 min, and then cooled. The curcumin extract was filtrated (Whatman paper No. 1) and concentrated to dryness by a rotary evaporator (IKA-WERKE; Germany) at the following conditions (speed 250 rpm, temperature 40°C, under vacuum). The sample was reconstituted with 100 ml of the mobile phase and filtered through an acrodisc syringe filter (0.45 μm; Gelman Laboratory, Ann Arbor, MI, USA), and then, 10 μl injected into the HPLC system. A detector (DAD) with a C18 guard column, the HPLC column was an Agilent Eclipse XDB-C18 with an ID of 4.6 mm, a length of 150 mm, and a particle size of 5 μm (4.6 m × 5 μm). Two solvents formed the mobile phase: the first solvent was acetonitrile, and the second was acetic acid in acetonitrile (0.5%: 99.5%; v/v). Gradient elution was used to elute the solution, beginning with 100% solvent (1) and ending with 100% solvent (2). A DAD detector frequency of 425 nm was utilized. The flow rate was set at 0.8 mL/min−1, and the run time was ~65 min. Consistent retention times and UV spectra were used to identify peaks and compare standards.
The antimicrobial ability of curcumin-NPs was determined by the disc diffusion method (Khezerlou et al., 2018). Briefly, tryptic soy agar plates were poured with 10 ml of semi-soft tryptic agar seeded with 100 ml of E. coli O157:H7 or S. aureus and/or B. cereus (~6 log10 CFU ml−1). The CFU was confirmed using the following equation:
Concentration (start) × Volume (start) = Concentration (final) × Volume (final)
The discs, which were placed over the plates, included curcumin-NPs at 2, 5, and 10 μg ml-1 (equivalent to 2, 5, and 10 ppm). The plates were examined for inhibition zones after 48 h at 37 ± 1°C. The inhibition zones were expressed as mm.
The morphology of curcumin-NPs and their mode of action against E. coli, S. aureus, and B. cereus were estimated. Briefly, 2 ml of sterilized TSB, 1 ml of bacterial, and 1 ml of curcumin-NPs were added in a falcon tube. The tubes were placed at 37 ± 1°C for 24 h. The pellets were recovered by centrifuging at 2,500 rpm min−1 for 10 min. The pellets were placed on a carbon-coated copper grid, spread, and then dried under a lamp before being examined using transmission electron microscopy (TEM; JEOL JEM 1400, USA) at a voltage of 200 Kv with negative staining of phosphor tungstic acid (PTA 1%) (He et al., 2016). A Malvern Zetasizer (ZS90, Malvern Instruments, Worcester, UK) was used to measure the size and distribution of the nanoparticles.
The chicken finger was made using the technique described by Sharma et al. (2015) with slight modifications. Briefly, chicken filet (72%; w/w), wheat flour (7%; w/w), oat flour (8%; w/w), casein (2.5%; w/w), and fat (7.5%; w/w) were mixed and shaped. The chicken fingers were treated with UV-C (254 nm) for 15 min (Mcleod et al., 2018) to reduce background bacteria (Morsy et al., 2018). The bacterial cultures (~6 log CFU cm−2) were spread on the surface of the chicken finger.
After inoculation, samples were placed at room temperature for 10 min to allow cell attachment. The best concentration of antimicrobials in the challenge study was chosen according to Section 2.6, Antimicrobial Activity Assay (2, 5, and 10 μg g−1). Curcumin-NPs at a level of 10 μg g−1 were placed on the chicken finger surface and spread consistently. The control sample was chicken fingers without curcumin addition. Every sample was put into a sterile bag separately and tightly closed. For 27 days, the packed samples were placed at 4 ± 1°C. On days 0, 3, 6, 9, 12, 15, 18, 21, 24, and 27, the samples were assessed for any remaining microbes.
After being opened aseptically, we placed 10 g of each chicken sample in 90 ml of purified peptone water (PPW; Biolife; 0.1%) and shaken for 1 min. After preparing 10-fold serial dilutions, 1 ml of the supernatant was spread on Eosin methylene blue (EMB; Biolife) for E. coli and Paired Parker agar (Biolife) for S. aureus, and B. cereus agar base (Biolife). After 24 h of incubation at 37 ± 1°C, the colonies were counted (log10 CFU g−1) (Mangalassary et al., 2007).
A digital pH meter (Consort, Belgium) was used to measure the pH value. Total volatile base nitrogen (TVB-N; N 100 g−1 of the sample) was estimated according to the approach of AOAC (2005). Thiobarbituric acid-reactive substances (TBARS; MDA kg−1) were determined using spectrophotometry (AOAC, 2005). The results were expressed in terms of optical density at a wavelength of 538 nm, measured using a digital spectrophotometer (CE 599 Universal, USA).
Statistical evaluation was conducted using a one-way ANOVA to assess physicochemical properties and bacterial populations in the experiments (P ≤ 0.05) using SPSS 22 (IBM's SPSS Statistics Software). The data were analyzed using a completely random design (Armonk, New York, USA). For each treatment, each challenge experiment was conducted in triplicate. The LSD and Tukey's tests were utilized for multiple mean comparisons (Steel and Torrie, 1980).
The characteristics of curcumin NPs were assessed. TEM was used to confirm the curcumin's morphology and droplet size (Figure 1). The results showed that curcumin-NPs were spherical, smooth, and had a particle size of 80 ± 2 nm. The polydispersity index (PDI) was 0.14, and the zeta potential was 4.5 mV. In addition, it was observed that curcumin-NPs had a higher size distribution compared with nanoparticles produced by Bhawana et al. (2011). The curcumin-NP powder had high physical stability and chemical reliability and was easily dispersible in water. No curcumin residues remained after dissolution and uniform dispersion. The larger surface area of nano-sized curcumin particles, which encourages dissolution, may be the reason for their improved aqueous solubility (Stefan and Monchaud, 2019). Similar outcomes have been noted in previous studies, where active ingredients' efficacy, solubility, and bioavailability were enhanced by reducing their particle size to nanoparticles (Nishimoto-Sauceda et al., 2022).
Figure 1. Characterization of curcumin-NPs using an SEM image (a), curcumin-NPs solution (b), and size distribution (c) (n = 3).
The antagonistic effects of curcumin-NPs at concentrations of 2, 5, and 10 μg g−1 were evaluated against E. coli, S. aureus, and B. cereus, as reported in Table 1. It has been shown that curcumin-NPs inhibit all foodborne pathogens. The inhibition zone diameters gradually increased when the concentration of curcumin-NPs increased. Curcumin-NPs at 10 μg g−1 were found to be more active against S. aureus than E. coli and B. cereus. Additionally, the antimicrobial ability of Curcumin-NPs was examined in the literature (Zorofchian Moghadamtousi et al., 2014). It has been established that free curcumin has antimicrobial properties against a variety of bacteria, including the Gram-positive bacteria, i.e., B. cereus, B. subtilis, and S. aureus, as well as the Gram-negative bacteria, i.e., E. coli, P. aeruginosa, and Yersinia entero (Mukhtar and Ghori, 2012; Rai et al., 2020; Dai et al., 2022). One study by Sankhwar et al. (2021) demonstrated that nano-curcumin is more effective against Gram-positive bacteria than Gram-negative bacteria. Pandit et al. (2015) found nano-curcumin (in vitro) antibacterial activity against E. coli and S. aureus with diameters of 12 and 15 mm, respectively.
Table 1. The antimicrobial capacity of curcumin-NPs against food-borne pathogens using the disc diffusion method (in vitro) (mean ± SD, n = 3).
Polyphenols and flavonoids exhibit functional properties such as antimicrobial and/or antioxidant activities. Table 2 presents the phenolic profiles of curcumin and curcumin-NPs. A total of 19 phenolics were discovered, and the main compounds were salicylic acid, e-vanillic, ellagic, rutin, caffeine, benzoic acid, p-coumaric, and pyrogallol. However, eight flavonoid compounds were identified: apigenin, hispertin, luteolin, rosmarinic, kaempferol, rutin, quercetin, and quercetrin. It was observed that the curcumin-NPs contain higher levels of phenolic and flavonoid compounds compared to bulk curcumin. The results demonstrated that curcumin-NPs contain higher levels of salicylic acid (305.58 mg 100 g−1), e-vanillic acid (244.76 mg 100 g−1), and ellagic acid (107.35 mg 100 g−1) compared to bulk curcumin. The phenolic compounds are of considerable interest in dietary antioxidant supplementation. In contrast, the major flavonoid compound is apegnin (239.03 mg/100 g−1). These results are consistent with those mentioned by Shalaby et al. (2016), who reported that curcumin-NPs are rich in phenolic compounds, especially salicylic acid, e-vanillic acid, ellagic, rutin, caffeine, benzoic acid, p-coumaric, and pyrogallol.
Table 2. Polyphenolic and flavonoid profiles of curcumin (5 μm) and curcumin-NPs (80 nm) extracts (at 10 μg g−1).
In Table 3, the curcumin-NPs had a higher total phenolic content (67.48 mg g−1 as gallic acid equivalent) than curcumin (58.33 mg g−1 as gallic acid equivalent). Curcumin-NPs also had a higher total flavonoid content than curcumin. Faid (2021) found phenolic and flavonoid contents of 55.35 and 40.12 mg g−1gallic acid in the ethanol extract of curcumin. However, Shalaby et al. (2016) reported that phenolic contents ranged from 18.4 to 20 mg g−1gallic acid, and flavonoid content ranged from 36.07 to 40.48 mg g−1 rutin in water and ethanol extracts of turmeric. These results are consistent with those reported by Hettiarachchi et al. (2022). The presence of polyphenols and flavonoids illustrates the antimicrobial and antioxidant activities of curcumin nanoparticles. When in nanoform, curcumin dissolves in water and is just as effective as regular curcumin (with increased phenolic and flavonoid levels). Circumin-NPs's greater efficacy compared to curcumin is attributed to its smaller particle size. It is worth remembering that, as curcumin becomes nanoparticles, its size decreases to 80 nm, which is significantly smaller than the size of curcumin particles, which allows for improved cell penetration and uptake.
Furthermore, Table 3 shows the antioxidant activity of curcumin-NPs (scavenging DPPH free radicals). The curcumin-NPs had a powerful ability to eliminate free radicals (IC50; 22.47 μg ml−1) compared to α-tocopherol and BHT (IC50; 15.58 and 5.16 μg ml−1, respectively), considering that the antioxidant capacity and the IC50 amount have a negative correlation. IC50 denotes the concentration of the sample required to scavenge 50% of DPPH radicals. Curcumin nanoparticles showed better IC50 than curcumin, possibly due to particle size, speed solubility, and distribution. The study by Ak and Gülçin (2008) reported that curcumin extract has a high free radical-scavenging capacity compared to trolox, and the EC50 for curcumin was 34.86 g ml−1. Faid (2021) found that 50 μg ml−1 of nano-curcumin extract had the highest scavenging capacity in ethanol extract compared to its chloroform and aqueous counterparts. Moreover, the IC50 value was 22.35 μg ml−1.
The mechanism of curcumin-NPs against food-borne pathogens appears necessary. Figure 2 depicts the TEM images of cells of E.coli, S. aureus, and B.cereus. It was observed that, during the growth of the pathogens in the presence of curcumin-NPs, these compounds appeared as dark, electron-dense spheres anchored to the bacterial cell wall. They disrupted the peptidoglycan layer and penetrated the cell, leading to cell lysis and resulting in cell death and the disruption of organelles.Curcumin-NPs were highly effective against both Gram-positive (G+) and Gram-positive (G–) bacteria. This is illustrated in Figure 3. The NPs alter the shape of the bacteria's cells and interact with their membrane, such as (a) the disruption of the membrane walls of specific bacteria, (b) the damaging effect on bacterial DNA, and (c) membrane leakage of the bacterium, producing a lot of reactive oxygen species, either as singlet oxygen or hydroxyl radicals. Our findings are in line with earlier research that demonstrated the mobilization of nanoparticles made of various materials within the bacterial cell. Previous research on B. subtilis 168 has shown that curcumin's ability to inhibit microbes involves interfering with the GTPase activity of FtsZ protofilaments, which are essential for bacterial cytokinesis (Jain et al., 2009). This dysfunction kills bacteria and prevents bacterial cell division by inhibiting the cluster dynamics of FtsZ in the Z ring. In another study by Bhawana et al. (2011), it was found that curcumin extract prevents cell adhesion to fibronectin and positively inhibits the bacterial surface protein sortase A, thereby demonstrating its antibacterial activity against S. aureus.
Figure 2. TEM images of curcumin-NPs against foodborne pathogens, curcumin-NPs against E. coli (a), curcumin-NPs against S. aureus (b), and curcumin-NPs against B. cereus (c).
Figure 3. The proposed mechanisms underlie the antibacterial efficacy of Curcumin-NPs against bacteria.
Another study reported that the antimicrobial ability of curcumin-NPs could be due to (i) the formation of transmembrane cell pores, which results in the leakage of essential metabolites, and (ii) the disruption of the structure of the bacterial cell wall, such as ergosterol, which is responsible for the synthesis and maintenance of cell wall rigidity (Rai et al., 2020). In the current study, curcumin-NPs are believed to exhibit an antibacterial effect by adhering to the bacterial cell wall, rupturing it, entering the cell, and interfering with the organization of cell organelles.
As is known, the most important bacteria related to chicken are Salmonella and Campylobacter. However, in recent years, the literature in Egypt and around the world has isolated E. coli, S. aureus, and B. cereus from chickens. Our study also aimed to evaluate the antibacterial effect of nano-curcumin against different foodborne pathogens, a representative type of bacteria from each of Gram +ve (S.aureus), Gram –ve (E. coli), and spore-forming (B. cereus). The antimicrobial effectiveness and modes of action of curcumin-NPs at a concentration of 10 μg g−1 against E. coli, S. aureus, and B. cereus in chicken fingers stored under refrigeration for up to 27 days. Figure 3 demonstrates the antimicrobial effectiveness of curcumin-NPs against pathogens found on chicken fingers. The bacterial community developed persistently during the 27 days of refrigerated storage in the control. Conversely, treated samples with Curcumin-NPs revealed a decrease of nearly 2 log units in populations of E. coli and S. aureus after 3 days, while a 1 log decrease in B. cereus after 6 days stayed fixed for the rest of the challenge study. E. coli and S. aureus were sensitive to the Curcumin-NPs, as evidenced by the decline in population (~3 to 4 log10 CFU g−1). However, compared to control, B. cereus (~2 to 3 log10 CFU g−1) was found on chicken fingers after 27 days of storage (Figure 4). These findings demonstrate that curcumin nanoparticles applied to processed chicken can restrain foodborne microorganisms for up to 27 days of refrigerated stockpiling. The outcomes concur with those detailed by Rai et al. (2020), who found that curcumin-stacked nanoparticles are more successful against foodborne microbes such as E. coli and S. aureus.
Figure 4. Impact of curcumin-NPs against foodborne pathogens, i.e., E. coli (A), S. aureus (B), and B. cereus (C) of the refrigerated chicken fingers during storage.
The pH, TVB-N, and TBARS contents are important freshness indices of meat quality. Figure 5A shows the progression of the pH value of chicken fingers during refrigerated storage. There were no huge contrasts (P ≥ 0.05) in pH values between the treated chicken samples and the control at time zero. However, a significant difference (P ≤ 0.05) was observed during storage at 4°C. After 9 days, the control sample's pH value quickly increased between 6.6 and 6.7. However, samples treated with curcumin-NPs at 10 μg g−1 demonstrated only a slight increase in pH during storage. The pH score of chicken samples incorporating curcumin-NPs ranged from 6.33 to 6.44 after 21 days. The findings reveal that nitrogenous compounds are broken down by endogenous or microbial enzymes, thereby increasing the pH (Fijałkowska et al., 2015). Untreated chicken fingers began to deteriorate and were rejected on day 12 of cold storage as a result of this pH increase.
Figure 5. Changes in pH value (A), TVB-N (mg N2 g−1) (B), and TBARS (mg MDA Kg −1) (C) in chicken fingers incorporating curcumin-NPs (10 μg g−1) during storage at (4°C).
Figure 5B shows the changes in the TVB-N content of chicken fingers, including curcumin-NPs, during storage at 4°C for 27 days. Curcumin-NP addition and storage period significantly affected protein quality (P ≤ 0.05). In the control sample, the TVB-N level rapidly increased from 2.86 to 21.59 mg N2100 g−1 on the 9 day of storage due to the presence of various bacteria. The chicken fingers that included curcumin-NPs had the lowest TVB-N score, indicating that curcumin-NPs inhibit microbial growth, particularly proteolytic bacteria, which break down proteins into volatile nitrogen compounds. The increase in TVB-N during the storage of chicken fingers (control) might be attributed to a breakdown of nitrogenous mixtures by microbial action (El-Nashi et al., 2015).
Figure 5C, at time zero, the TBARS scores in various chicken finger samples ranged from 0.08 to 0.11 mg MDA kg−1. However, during storage time, a rapid increase in the MDA level was recorded in the control samples (with different bacteria), ranging from 0.88 to 1.09 mg MDA kg−1, after 9 days. These outcomes are consistent with previous data on chicken meat (Khajeh Bami et al., 2020; Panahi et al., 2022). In general, the storage of the chicken finger samples prompted a remarkable increase in the TBARS scores in most treatments, but it could be observed that there was a higher increase in TBARS in the control samples than in the treated samples. The samples included Curcumin-NPs with the lowest TBARS value due to their antioxidant activity. The antioxidant effect of the addition of curcumin to meat products was studied, such as rabbit burgers (Mancini et al., 2015), beef meatballs (Milon et al., 2016), lamb sausage (De Carvalho et al., 2020), and chicken mince (Sharma et al., 2012). Mughal (2019) has shown that turmeric's antioxidant properties prevent peroxide formation in food. Curcumin, which is found in turmeric, has a unique structure that enables it to act as an antioxidant and break the chains by trapping oxygen-free radicals (Urošević et al., 2022).
In conclusion, curcumin-NPs have higher phenolic content and antioxidant activity at 10 μg g−1 than curcumin bulk. Curcumin-NPs exhibited a higher antimicrobial capacity (in vitro) against S. aureus than E. coli and B. cereus. In the challenge studies, curcumin-NPs effectively inhibited pathogens in chicken fingers during storage for up to 27 days. In curcumin-NPs-treated chicken samples, the contents of TBARS and TVB-N were lower than the control (over 27 days of storage). The experimental findings from the bioactive compound, antimicrobial activity, antioxidant capacity, and chemical markers confirm the efficacy of nano-curcumin at a level of 10 μg g−1. Generally, nanotechnology enhances the efficacy of materials and overcomes the challenges of using natural additives in the meat industry, which may otherwise alter the sensory attributes of meat products. Therefore, curcumin-NPs have shown a promising improvement in microbial quality, enhancing shelf life and reducing lipid oxidation in poultry meat products.
The raw data supporting the conclusions of this article will be made available by the authors, without undue reservation.
MM: Conceptualization, Formal analysis, Investigation, Methodology, Visualization, Writing—original draft, Writing—review and editing. SA-D: Investigation, Methodology, Writing—review and editing. MH: Investigation, Methodology, Writing—review and editing. MD: Investigation, Methodology, Writing—review and editing. EA-E: Investigation, Visualization, Writing—review and editing. AA: Data curation, Software, Supervision, Writing—review and editing. SI: Funding acquisition, Resources, Supervision, Writing—original draft. MS: Software, Validation, Writing—review and editing. IB-D: Formal analysis, Resources, Writing—review and editing. LF: Funding acquisition, Project administration, Writing—original draft. HG: Resources, Software, Writing—review and editing. AE-S: Formal analysis, Validation, Writing—review and editing. MA: Investigation, Methodology, Writing—original draft. NK: Resources, Validation, Writing—review and editing. RE: Formal analysis, Investigation, Methodology, Writing—original draft.
The author(s) declare financial support was received for the research, authorship, and/or publication of this article. This study was supported by the Deanship of Scientific Research at King Khalid University through a large group research project under grant number RGP2/435/44; the Princess Nourah bint Abdulrahman University Researchers Supporting Project number (PNURSP2023R127), Princess Nourah bint Abdulrahman University, Riyadh, Saudi Arabia; and the project 6PFE of the University of Life Sciences King Mihai I from Timisoara and the Research Institute for Biosecurity and Bioengineering from Timisoara.
The authors extend their appreciation to the Deanship of Scientific Research at King Khalid University for funding this work through a large group research project under grant number RGP2/435/44. Additionally, the appreciation is extended to the Princess Nourah bint Abdulrahman University Researchers Supporting Project number (PNURSP2023R127), Princess Nourah bint Abdulrahman University, Riyadh, Saudi Arabia.
The authors declare that the research was conducted in the absence of any commercial or financial relationships that could be construed as a potential conflict of interest.
All claims expressed in this article are solely those of the authors and do not necessarily represent those of their affiliated organizations, or those of the publisher, the editors and the reviewers. Any product that may be evaluated in this article, or claim that may be made by its manufacturer, is not guaranteed or endorsed by the publisher.
Abdelli, N., Mekawi, E., Ebrahim Abdel-Alim, M., Salim, N., El-Nagar, M., Al-Dalain, S., et al. (2022). QTRAP LC/MS/MS of garlic nanoparticles and improving sunflower oil stabilization during accelerated shelf life storage. Foods 2022, 113962. doi: 10.3390/foods11243962
Ak, T., and Gülçin, I. (2008). Antioxidant and radical scavenging properties of curcumin. Chem. Biol. Interact. 174, 27–37. doi: 10.1016/j.cbi.2008.05.003
Alqahtani, N. K., Alnemr, T. M., Al-Asmari, F., and Ali, S. A. (2023). Quality attributes of cold-stored khalal barhi dates treated with guava leaf extract and/or lactic acid as natural preservatives. Foods 12, 2115. doi: 10.3390/foods12112115
AOAC (2005). Official Methods of Analysis. 18th Edn. Washington DC: Association of Official Analytical, Chemists.
Awan, A. M., Farid, A., Shah, S. U., Khan, D., Ur Rehman, F., Dar, M. J., et al. (2022). Nanocrytals-mediated oral drug delivery: enhanced bioavailability of amiodarone. Pharmaceutics 14, 1300. doi: 10.3390/pharmaceutics14061300
Bajpai, V. K., Bahuguna, A., Kumar, V., Khan, I., Alrokayan, S. H., Khan, H. A., et al. (2022). Cellular antioxidant potential and inhibition of foodborne pathogens by a sesquiterpene ilimaquinone in cold storaged ground chicken and under temperature-abuse condition. Food Chem. 373, 131392. doi: 10.1016/j.foodchem.2021.131392
Barutçu Mazi, I. (2009). Effects of Different Batter Formulations on Physical and Chemical Properties of Microwave and Conventionally Fried Chicken Fingers. Roma: FAO of the UN.
Bensid, A., El Abed, N., Houicher, A., Regenstein, J. M., and Özogul, F. (2022). Antioxidant and antimicrobial preservatives: properties, mechanism of action and applications in food–a review. Crit. Rev. Food Sci. Nutr. 62, 2985–3001. doi: 10.1080/10408398.2020.1862046
Bhawana Basniwal, R. K., Buttar, H. S., Jain, V., and Jain, N. (2011). Curcumin nanoparticles: preparation, characterization, and antimicrobial study. J. Agric. Food Chem. 59, 2056–2061. doi: 10.1021/jf104402t
Bozzato, A., Pippia, E., Tiberi, E., and Manzocco, L. (2021). Air impingement to reduce thawing time of chicken fingers for food service. J. Food Process. Preserv. 45, e15962. doi: 10.1111/jfpp.15962
Chen, X., Zhang, T.-Y., Wu, Y.-C., Gong, P.-X., and Li, H.-J. (2022). Foxtail millet prolamin as an effective encapsulant deliver curcumin by fabricating caseinate stabilized composite nanoparticles. Food Chem. 367, 130764. doi: 10.1016/j.foodchem.2021.130764
Chopra, H., Dey, P. S., Das, D., Bhattacharya, T., Shah, M., Mubin, S., et al. (2021). Curcumin nanoparticles as promising therapeutic agents for drug targets. Molecules 26, 4998. doi: 10.3390/molecules26164998
Crotta, M., Prakashbabu, B. C., Holt, H., Swift, B., Pedada, V. C., Shaik, T. B., et al. (2022). Microbiological risk ranking of foodborne pathogens and food products in scarce-data settings. Food Control 141, 109152. doi: 10.1016/j.foodcont.2022.109152
Dai, C., Lin, J., Li, H., Shen, Z., Wang, Y., Velkov, T., et al. (2022). The natural product curcumin as an antibacterial agent: current achievements and problems. Antioxidants 11, 459. doi: 10.3390/antiox11030459
De Carvalho, F. A. L., Munekata, P. E., de Oliveira, A. L., Pateiro, M., Domínguez, R., Trindade, M. A., et al. (2020). Turmeric (Curcuma longa L.) extract on oxidative stability, physicochemical and sensory properties of fresh lamb sausage with fat replacement by tiger nut (Cyperus esculentus L.) oil. Food Res. Int. 136, 109487. doi: 10.1016/j.foodres.2020.109487
Duncan, T. V. (2011). Applications of nanotechnology in food packaging and food safety: barrier materials, antimicrobials and sensors. J. Colloid Interface Sci. 363, 1–24. doi: 10.1016/j.jcis.2011.07.017
Ebrahimzadeh, M. A., Nabavi, S. M., Nabavi, S. F., Bahramian, F., and Bekhradnia, A. R. (2010). Antioxidant and free radical scavenging activity of H. officinalis L. var. angustifolius, V. odorata, B. hyrcana and C. speciosum. Pak. J. Pharm. Sci. 23, 29–34. doi: 10.3109/13880209.2012.655859
EFSA (2013). Tracing Seeds, in Particular Fenugreek (Trigonella Foenum-Graecum) Seeds, in Relation to the Shiga Toxin-Producing E. coli (STEC) O104: H4 2011 Outbreaks in Germany and France. Technical report of EFSA. Available online at: http://www.efsa.europa.eu/en/supporting/doc/176e.pdf (accessed February 1, 2023).
El-Nashi, H. B., Fattah, A. F., Rahman, N. R. A., and Abd El-Razik, M. (2015). Quality characteristics of beef sausage containing pomegranate peels during refrigerated storage. Ann. Agric. Sci. 60, 403–412. doi: 10.1016/j.aoas.2015.10.002
Faid, S. M. (2021). Curcumin (Curcuma longa) and quercetin nanoparticles as antimicrobial and anticancer agents. Afr. J. Biol. Sci. 17, 205–219. doi: 10.21608/ajbs.2021.192445
Faizy, H., Azimi, A., Alam, S., Safari, Z. S., and Atif, A. (2022). Food safety status on poultry meat and egg in Afghanistan. AgroTech Food Sci. Technol. Environ. 1, 57–60. doi: 10.53797/agrotech.v1i1.8.2022
Farag, M. A., Zain, A. E., Hariri, M. L., El Aaasar, R., Khalifa, I., and Elmetwally, F. (2022). Potential food safety hazards in fermented and salted fish in Egypt (Feseekh, Renga, Moloha) as case studies and controlling their manufacture using HACCP system. J. Food Saf. 42, e12973. doi: 10.1111/jfs.12973
Farnia, P., Mollaei, S., Bahrami, A., Ghassempour, A., Velayati, A. A., and Ghanavi, J. (2016). Improvement of curcumin solubility by polyethylene glycol/chitosan-gelatin nanoparticles (CUR-PEG/CS-G-nps). Biomed. Res. 27, 659–665.
Fijałkowska, M., Pysera, B., Lipiński, K., and Strusińska, D. (2015). Changes of nitrogen compounds during ensiling of high protein herbages–a review. Ann. Anim. Sci. 15, 289–305. doi: 10.1515/aoas-2015-0008
Formagio, A. S. N., Volobuff, C. R. F., Santiago, M., Cardoso, C. a,.L, Vieira, M. D. C., et al. (2014). Evaluation of antioxidant activity, total flavonoids, tannins and phenolic compounds in Psychotria leaf extracts. Antioxidants 3, 745–757. doi: 10.3390/antiox3040745
Garridogamarro, E., Svanevik, C. S., Lundebye, A.-K., Sanden, M., D'agostino, E., Kjellevold, M., et al. (2023). Challenges in the implementation of food safety and quality assurance systems in small-scale fisheries. Food Qual. Saf. 7, 1–9. doi: 10.1093/fqsafe/fyad007
Hajimahmoodi, M., Faramarzi, M. A., Mohammadi, N., Soltani, N., Oveisi, M. R., and Nafissi-Varcheh, N. (2010). Evaluation of antioxidant properties and total phenolic contents of some strains of microalgae. J. Appl. Phycol. 22, 43–50. doi: 10.1007/s10811-009-9424-y
He, Y., Ingudam, S., Reed, S., Gehring, A., Strobaugh, T. P., and Irwin, P. (2016). Study on the mechanism of antibacterial action of magnesium oxide nanoparticles against foodborne pathogens. J. Nanobiotechnol. 14, 1–9. doi: 10.1186/s12951-016-0202-0
He, Y., Yue, Y., Zheng, X., Zhang, K., Chen, S., and Du, Z. (2015). Curcumin, inflammation, and chronic diseases: how are they linked? Molecules 20, 9183–9213. doi: 10.3390/molecules20059183
Hettiarachchi, S. S., Perera, Y., Dunuweera, S. P., Dunuweera, A. N., Rajapakse, S., and Rajapakse, R. M. G. (2022). Comparison of antibacterial activity of nanocurcumin with bulk curcumin. ACS Omega 7, 46494–46500. doi: 10.1021/acsomega.2c05293
Hewlings, S. J., and Kalman, D. S. (2017). Curcumin: a review of its effects on human health. Foods 6, 92. doi: 10.3390/foods6100092
Jain, J., Arora, S., Rajwade, J. M., Omray, P., Khandelwal, S., and Paknikar, K. M. (2009). Silver nanoparticles in therapeutics: development of an antimicrobial gel formulation for topical use. Mol. Pharm. 6, 1388–1401. doi: 10.1021/mp900056g
Jyotirmayee, B., and Mahalik, G. (2022). A review on selected pharmacological activities of Curcuma longa L. Int. J. Food Prop. 25, 1377–1398. doi: 10.1080/10942912.2022.2082464
Khajeh Bami, M., Afsharmanesh, M., and Ebrahimnejad, H. (2020). Effect of dietary Bacillus coagulans and different forms of zinc on performance, intestinal microbiota, carcass and meat quality of broiler chickens. Probiot. Antimicrob. Proteins 12, 461–472. doi: 10.1007/s12602-019-09558-1
Khataee, A., Fathinia, S., and Fathinia, M. (2017). Production of pyrite nanoparticles using high energy planetary ball milling for sonocatalytic degradation of sulfasalazine. Ultrason. Sonochem. 34, 904–915. doi: 10.1016/j.ultsonch.2016.07.028
Khezerlou, A., Alizadeh-Sani, M., Azizi-Lalabadi, M., and Ehsani, A. (2018). Nanoparticles and their antimicrobial properties against pathogens including bacteria, fungi, parasites and viruses. Microb. Pathog. 123, 505–526. doi: 10.1016/j.micpath.2018.08.008
Liu, X., Ardo, S., Bunning, M., Parry, J., Zhou, K., Stushnoff, C., et al. (2007). Total phenolic content and DPPH radical scavenging activity of lettuce (Lactuca sativa L.) grown in Colorado. LWT Food Sci. Technol. 40, 552–557. doi: 10.1016/j.lwt.2005.09.007
Maleki Dizaj, S., Alipour, M., Dalir Abdolahinia, E., Ahmadian, E., Eftekhari, A., Forouhandeh, H., et al. (2022). Curcumin nanoformulations: beneficial nanomedicine against cancer. Phytother. Res. 36, 1156–1181. doi: 10.1002/ptr.7389
Malik, S., Muhammad, K., and Waheed, Y. (2023). Nanotechnology: a revolution in modern industry. Molecules 28, 661. doi: 10.3390/molecules28020661
Mancini, S., Preziuso, G., Dal Bosco, A., Roscini, V., Szendrő, Z., Fratini, F., et al. (2015). Effect of turmeric powder (Curcuma longa L.) and ascorbic acid on physical characteristics and oxidative status of fresh and stored rabbit burgers. Meat Sci. 110, 93–100. doi: 10.1016/j.meatsci.2015.07.005
Mangalassary, S., Han, I., Rieck, J., Acton, J., Jiang, X., Sheldon, B., et al. (2007). Effect of combining nisin and/or lysozyme with in-package pasteurization on thermal inactivation of Listeria monocytogenes in ready-to-eat turkey bologna. J. Food Prot. 70, 2503–2511. doi: 10.4315/0362-028X-70.11.2503
Mcleod, A., Hovde Liland, K., Haugen, J. E., Sørheim, O., Myhrer, K. S., and Holck, A. L. (2018). Chicken fillets subjected to UV-C and pulsed UV light: reduction of pathogenic and spoilage bacteria, and changes in sensory quality. J. Food Saf. 38, e12421. doi: 10.1111/jfs.12421
Milon, M., Kabir, M., Hossain, M., Rahman, M., Azad, M., and Hashem, M. (2016). Value added beef meatballs using turmeric (Curcuma longa) powder as a source of natural antioxidant. Int. J. Nat. Soc. Sci. 3, 52–61.
Morsy, M. K., Elsabagh, R., and Trinetta, V. (2018). Evaluation of novel synergistic antimicrobial activity of nisin, lysozyme, EDTA nanoparticles, and/or ZnO nanoparticles to control foodborne pathogens on minced beef. Food Control 92, 249–254. doi: 10.1016/j.foodcont.2018.04.061
Morsy, M. K., Khalaf, H. H., Sharoba, A. M., El-Tanahi, H. H., and Cutter, C. N. (2014). Incorporation of essential oils and nanoparticles in pullulan films to control foodborne pathogens on meat and poultry products. J. Food Sci. 79, M675–M684. doi: 10.1111/1750-3841.12400
Mostafa, H., Airouyuwa, J. O., and Maqsood, S. (2022). A novel strategy for producing nanoparticles from date seeds and enhancing their phenolic content and antioxidant properties using ultrasound-assisted extraction: a multivariate based optimization study. Ultrason. Sonochem. 87, 106017. doi: 10.1016/j.ultsonch.2022.106017
Mughal, M. H. (2019). Turmeric polyphenols: A comprehensive review. Integrative Food, Nutrition and Metabolism, Volume 6, 1–6. Available online at: https://pdfs.~semanticscholar.org/497b/30547906fe211facca4c7d01e3f95457f12a.pdf (accessed June 15, 2023).
Mukhtar, S., and Ghori, I. (2012). Antibacterial activity of aqueous and ethanolic extracts of garlic, cinnamon and turmeric against Escherichia coli ATCC 25922 and Bacillus subtilis DSM 3256. Int. J. Appl. Biol. Pharm. Technol. 3, 131–136. doi: 10.30574/gscbps.2022.19.1.0094
Niaz, T., Shabbir, S., Noor, T., and Imran, M. (2022). Active composite packaging reinforced with nisin-loaded nano-vesicles for extended shelf life of chicken breast filets and cheese slices. Food Bioprocess Technol. 15, 1284–1298. doi: 10.1007/s11947-022-02815-2
Nishimoto-Sauceda, D., Romero-Robles, L. E., and Antunes-Ricardo, M. (2022). Biopolymer nanoparticles: a strategy to enhance stability, bioavailability, and biological effects of phenolic compounds as functional ingredients. J. Sci. Food Agric. 102, 41–52. doi: 10.1002/jsfa.11512
Owusu-Apenten, R., and Vieira, E. (2022). “Microbial foodborne disease outbreaks,” in Elementary Food Science (Springer International Publishing), 171–196.
Panahi, Z., Khoshbakht, R., Javadi, B., Firoozi, E., and Shahbazi, N. (2022). The effect of sodium alginate coating containing citrus (Citrus aurantium) and Lemon (Citrus lemon) extracts on quality properties of chicken meat. J. Food Qual. 2022, 1–9. doi: 10.1155/2022/6036113
Pandit, R. S., Gaikwad, S. C., Agarkar, G. A., Gade, A. K., and Rai, M. (2015). Curcumin nanoparticles: physico-chemical fabrication and its in vitro efficacy against human pathogens. 3 Biotech 5, 991–997. doi: 10.1007/s13205-015-0302-9
Rai, M., Ingle, A. P., Pandit, R., Paralikar, P., Anasane, N., Santos, C., et al. (2020). Curcumin and curcumin-loaded nanoparticles: antipathogenic and antiparasitic activities. Expert Rev. Anti Infect. Ther. 18, 367–379. doi: 10.1080/14787210.2020.1730815
Ruslay, S., Abas, F., Shaari, K., Zainal, Z., Sirat, H., Israf, D. A., et al. (2007). Characterization of the components present in the active fractions of health gingers (Curcuma xanthorrhiza and Zingiber zerumbet) by HPLC–DAD–ESIMS. Food Chem. 104, 1183–1191. doi: 10.1016/j.foodchem.2007.01.067
Sankhwar, R., Yadav, S., Kumar, A., and Gupta, R. K. (2021). Application of nano-curcumin as a natural antimicrobial agent against gram-positive pathogens. J. Appl. Nat. Sci. 13, 110–126. doi: 10.31018/jans.v13i1.2482
Shalaby, M., Ghoniem, G. A., Domma, M., and Elias, A. K. (2016). Protective effect of turmeric and garlic powder on hypercholesterlemia level in rats. J. Food Dairy Sci. 7, 355–362. doi: 10.21608/jfds.2016.46022
Sharma, H., Talukder, S., Sharma, B., Goswami, M., and Giriprasad, R. (2015). Development and quality assessment of value added chicken fingers. Indian J. Poult. Sci. 50, 66–71.
Sharma, J., Ponnusamy Pazhaniandi, P., Tanwar, V. K., Das, S. K., and Goswami, M. (2012). Antioxidant effect of turmeric powder, nitrite and ascorbic acid on stored chicken mince. Int. J. Food Sci. Technol. 47, 61–66. doi: 10.1111/j.1365-2621.2011.02807.x
Singh, B. P., Manju, K., Sharma, R., Bhushan, B., Ghosh, S., and Goel, G. (2023). Nano-conjugated food-derived antimicrobial peptides as natural biopreservatives: a review of technology and applications. Antibiotics 12, 244. doi: 10.3390/antibiotics12020244
Steel, R. G. D., and Torrie, J. H. (1980). Principles and Procedures of Statistics, a Biometrical Approach. New York, NY: McGraw-Hill Kogakusha, Ltd.
Stefan, L., and Monchaud, D. (2019). Applications of guanine quartets in nanotechnology and chemical biology. Nat. Rev. Chem. 3, 650–668. doi: 10.1038/s41570-019-0132-0
Tropea, A. (2022). Microbial contamination and public health: an overview. Int. J. Environ. Res. Public Health 19, 7441. doi: 10.3390/ijerph19127441
Tylewicz, U., Nowacka, M., Martín-García, B., Wiktor, A., and Caravaca, A. M. G. (2018). “Target sources of polyphenols in different food products and their processing by-products,” in Polyphenols: Properties, Recovery, and Applications (Elsevier; Woodhead Publishing), 135–175.
Urošević, M., Nikolić, L., Gajić, I., Nikolić, V., Dinić, A., and Miljković, V. (2022). Curcumin: Biological activities and modern pharmaceutical forms. Antibiotics 11, 135. doi: 10.3390/antibiotics11020135
Wu, Y., Wang, K., Liu, Q., Liu, X., Mou, B., Lai, O.-M., et al. (2022). Selective antibacterial activities and storage stability of curcumin-loaded nanoliposomes prepared from bovine milk phospholipid and cholesterol. Food Chem. 367, 130700. doi: 10.1016/j.foodchem.2021.130700
Yaghoubi, M., Alirezalu, K., Hesari, J., Peighambardoust, S. H., Marcinkowska-Lesiak, M., Barzegar, Y., et al. (2023). Application of oleaster leaves (Elaeagnus angustifolia L.) essential oil and natural nanoparticle preservatives in frankfurter-type sausages: An assessment of quality attributes and stability during refrigerated storage. Meat Sci. 198, 1–12. doi: 10.1016/j.meatsci.2023.109097
Zang, E., Jiang, L., Cui, H., Li, X., Yan, Y., Liu, Q., et al. (2022). Only plant-based food additives: an overview on application, safety, and key challenges in the food industry. Food Rev. Int. 39, 5132–5163. doi: 10.1080/87559129.2022.2062764
Zhao, J., Qian, J., Luo, J., Huang, M., Yan, W., and Zhang, J. (2022). Application of Ag@ SiO2 nanoparticles within PVA to reduce growth of E. coli and S. aureus in beef patties. J. Food Sci. 87, 4569–4579. doi: 10.1111/1750-3841.16292
Zheng, D., Huang, C., Huang, H., Zhao, Y., Khan, M. R. U., Zhao, H., et al. (2020). Antibacterial mechanism of curcumin: a review. Chem. Biodivers. 17, e2000171. doi: 10.1002/cbdv.202000171
Keywords: curcumin, nanoparticles, chicken fingers, antimicrobial, antioxidant, foodborne pathogens
Citation: Morsy MK, Al-Dalain SY, Haddad MA, Diab M, Abd-Elaaty EM, Abdeen A, Ibrahim SF, Shukry M, Banatean-Dunea I, Fericean L, Ghamry HI, El-Sayed A, Abdelaziz M, Kadhim N and Elsabagh R (2023) Curcumin nanoparticles as a natural antioxidant and antimicrobial preservative against foodborne pathogens in processed chicken fingers. Front. Sustain. Food Syst. 7:1267075. doi: 10.3389/fsufs.2023.1267075
Received: 25 July 2023; Accepted: 12 September 2023;
Published: 10 October 2023.
Edited by:
Tomás Jesús Madera-Santana, National Council of Science and Technology (CONACYT), MexicoReviewed by:
Mohammad Nejatian, Baqiyatallah University of Medical Sciences, IranCopyright © 2023 Morsy, Al-Dalain, Haddad, Diab, Abd-Elaaty, Abdeen, Ibrahim, Shukry, Banatean-Dunea, Fericean, Ghamry, El-Sayed, Abdelaziz, Kadhim and Elsabagh. This is an open-access article distributed under the terms of the Creative Commons Attribution License (CC BY). The use, distribution or reproduction in other forums is permitted, provided the original author(s) and the copyright owner(s) are credited and that the original publication in this journal is cited, in accordance with accepted academic practice. No use, distribution or reproduction is permitted which does not comply with these terms.
*Correspondence: Mohamed K. Morsy, bW9oYW1lZC5hYmRlbGhhZmV6QGZhZ3IuYnUuZWR1LmVn; Ahmed Abdeen, YWhtZWQuYWJkZWVuQGZ2dG0uYnUuZWR1LmVn; Liana Fericean, bWloYWVsYWZlcmljZWFuQHVzYWItdG0ucm8=
Disclaimer: All claims expressed in this article are solely those of the authors and do not necessarily represent those of their affiliated organizations, or those of the publisher, the editors and the reviewers. Any product that may be evaluated in this article or claim that may be made by its manufacturer is not guaranteed or endorsed by the publisher.
Research integrity at Frontiers
Learn more about the work of our research integrity team to safeguard the quality of each article we publish.