- 1Centre for Advanced Studies on Climate Change, Dr. Rajendra Prasad Central Agriculture University (RPCAU), Samastipur, Bihar, India
- 2Rajendra Prasad Central Agriculture University (RPCAU), Samastipur, Bihar, India
- 3KVK Birauli, RPCAU, Samastipur, Bihar, India
- 4KVK Saran, RPCAU, Samastipur, Bihar, India
- 5KVK Piprakothi, RPCAU, Samastipur, Bihar, India
- 6KVK Sukhet, RPCAU, Samastipur, Bihar, India
- 7KVK Begusarai, RPCAU, Samastipur, Bihar, India
- 8KVK Vaishali, RPCAU, Samastipur, Bihar, India
- 9KVK Siwan, RPCAU, Samastipur, Bihar, India
- 10KVK Turki, RPCAU, Samastipur, Bihar, India
- 11KVK Saraiya, RPCAU, Samastipur, Bihar, India
- 12KVK Jale, RPCAU, Samastipur, Bihar, India
- 13KVK Sheohar, RPCAU, Samastipur, Bihar, India
- 14KVK Madhopur, RPCAU, Samastipur, Bihar, India
- 15KVK Gopalganj, RPCAU, Samastipur, Bihar, India
- 16KVK Sitamadhi, RPCAU, Samastipur, Bihar, India
- 17KVK Lada, RPCAU, Samastipur, Bihar, India
- 18KVK Narkatiyaganj, RPCAU, Samastipur, Bihar, India
- 19CASCC, RPCAU, Samastipur, Bihar, India
- 20KVK Parsauni, RPCAU, Samastipur, Bihar, India
- 21KVK Sitamarhi, RPCAU, Samastipur, Bihar, India
- 22KVK West Champaran, RPCAU, Samastipur, Bihar, India
Rice followed by wheat is the dominant cropping system in the middle Indo-Gangetic plains (IGP). Lower productivity (4.8 t ha−1) of this cropping system in Bihar, compared to the national average (6.8 t ha−1) due to several climate- and production-related issues, is a matter of concern for the farmers and the policymakers. Keeping all these in view, an experiment with rice–wheat cropping system was carried out during 2020–21 and 2021–22 in 17 adopted villages of 13 districts of Bihar under the Project “Climate Resilient Agriculture Program (CRAP)” to evaluate the feasibility of early transplanting of rice in the month of June with the aim of achieving higher system productivity by early harvesting of rice and subsequent timely sowing of wheat before 15 November with the provision of assured irrigation. In this study, the concept of an innovative community irrigation approach and single-phase 3-hp submersible pump was employed. Long-duration rice variety (150 days) Rajendra Mahsuri-1 was sown during 20–25 May in the nursery and transplanted through puddling operation during 15–20 June in 17 locations. Under delayed conditions, the nursery sowing and transplanting window were 10–15 June and 10–15 July, respectively. Timely sown rice grown with the provision of a community irrigation system achieved a grain yield of 5.2 t ha−1 and 85.8% higher water productivity, compared to late-sown crops. Following the harvest of rice, the HD-2967 variety of wheat was planted in the first fortnight of November and harvested in the first week of April, yielding 4.9 t ha−1 with the application of 2–3 irrigations based on soil type and evaporative demand. Timely harvesting of wheat facilitated farmers of the region to take an additional crop of summer green gram. With an assured irrigation system and shifting planting dates and thereby managing climatic risks, the overall productivity of the rice–wheat cropping system was achieved to the tune of 10.1 t ha−1 with a cropping intensity of 300% for better adaptation and sustainable production.
1 Introduction
Rice–wheat cropping system (RWCS) is the most prevalent agricultural production system covering nearly 14 million hectares in the Indo-Gangetic Plains (IGP) of South Asia (Alam et al., 2016), of which 10 million hectares lies in India alone. This cropping system is predominantly followed in the states of Punjab, Haryana, Bihar, Uttar Pradesh, and Madhya Pradesh contributing around 75% of the national food grain production. However, the sustainability of RWCS in India is facing severe challenges mainly due to resource paucity (Jat et al., 2018; Mondal et al., 2020), water scarcity (Bhatt et al., 2016), and climatic variability (Jain et al., 2014). In the state of Bihar located in the middle IGP, the major food demand is met through RWCS, which contributes about 77.4% of the total food grain production from around 70% of the gross cropped area, but the productivity of both crops is very low due to the frequent occurrence of climatic stresses such as abnormal temperature, erratic rainfall, increased frequency and duration of dry spells, and early withdrawal and erratic nature of monsoon, which adversely affects the production potential and food security (Lal, 2019; Arunrat et al., 2020). The mean productivity of rice in Bihar stands at 2.5 t ha−1, that too, with a decreasing compound agricultural growth rate of (−) 3.61% in 2017–2018, as compared to 2014–2015 (Economic Survey of Bihar, 2019–2020).
In Bihar such as in all other states of eastern India, the major constraint in getting the potential yield of rice is late transplanting (Sattar et al., 2017) due to delays in obtaining water for puddling operations following the late onset of monsoon on many occasions and the lack of irrigation facility at an affordable rate. There should be ponded water in the initial stage of transplanting for the smooth recovery of plants. For ensuring timely sowing and transplanting of rice, the major source of irrigation is groundwater, which is extracted by a diesel pump set. The diesel pump sets have centrifugal pumps, and they are not able to extract groundwater during peak hours in summer when the water table goes down and there is a great rush for transplanting. Diesel pump sets have high diesel consumption with comparatively lesser water discharge and higher greenhouse gas emission. In view of this, the government recently started electrifying the irrigation system with the installation of three-phase 5/7.5 hp. diesel pumps. These pumps operated by the farmers do not perform well as it requires a constant supply of electricity in all three phases. Moreover, the electricity consumption is almost 5 times per hour, compared to a 3-hp single-phase tube well. Accordingly, it requires an investment of significant funds. Considering the cost of irrigation, it is around INR 6000.00 per hectare for a diesel pump set, INR 3000.00 per hectare for a three-phase electrified irrigation system, and INR 300.00 per hectare for a 3-hp single-phase pump set. Hence, the high cost of irrigation coupled with the late onset of monsoon is also a major factor that compels the farmers of the region for late transplanting of rice. Consequently, late transplanting tends to significantly impact the occurrence of critical phenological stages of the crop. Under delayed transplanting, the flowering of the crop coincides with the prevalence of low temperatures beyond September. Under such a situation, there is a greater possibility that a large percentage of chaffy grains would be produced. Not only this but also the delay in transplanting affects the sowing of succeeding wheat crops. In this region, when wheat is sown beyond November, the problem of terminal heat stress during the flowering to the milking stage of the crop arises in most of the years and consequently, it affects grain setting and wheat productivity significantly. About 80% of wheat sowing is delayed in the region beyond the period of 25 November, forcing the crop to encounter higher temperatures (terminal heat) during the growing season (end of February to March) (Sattar et al., 2023). Therefore, terminal heat stress associated with dry westerly wind is a serious climatic constraint for successful wheat cultivation in India, particularly when it occurs during the grain-filling stage (Sandhu et al., 2016; Sattar et al., 2020). In one study on wheat, Poudel et al. (2021) observed that the optimum temperature during the anthesis and grain-filling stage ranges from 12 to 22°C. As evident, among all the weather factors, temperature plays a crucial role in determining the sowing time and consequently the duration of different phenophases, which ultimately affect crop productivity. Moreover, when the farmers go for delayed sowing (beyond 25 November), there is always a greater chance that the crop will face the fury of a hailstorm during the ripening and harvesting stage in the month of April as the region experiences a greater probability (>70%) of hailstorms during this month.
Participatory rural appraisal (PRA) provides an opportunity to analyze the livelihood issues of the farmers and helps scientists to understand the problems. It is an important tool to identify the location-specific researchable issues and find out possible short- and long-term benefits. Kumari et al. (2019) used the PRA tool in some districts of Bihar to develop problem-solution tree of the issues faced by the farmers. The climatic issues affecting rice–wheat production in the region were reported by Srivastava et al. (2018). In the present study, the PRA tool was used to address the problems faced by the farmers of rice–wheat cropping system, and based on this, villages in different districts were selected for this study.
Considering the above facts, the present study was undertaken to evaluate the feasibility of early transplanting of rice through an assured irrigation system and its impact on phenology, yield, and yield attributes. Early transplanting of rice ensured its early harvesting and timely sowing of subsequent wheat crops. Accordingly, the yield potential of RWCS by advancing planting dates and water productivity of rice under timely and late transplanted conditions were evaluated by employing an innovative approach through the Climate Resilient Agriculture (CRA) Program for higher system productivity, better adaptation, and sustainability under changing climatic scenarios. The innovation in this case refers to the early transplanting of rice through assured community irrigation by a 3-hp single-phase tube well.
2 Materials and methods
2.1 Study area
Bihar is located in the middle Gangetic Plains of India. The study was conducted in 17 different adopted villages of different Farm Science Centres (Popularly known as Krishi Vigyan Kendras, KVKs) of Dr. Rajendra Prasad Central Agricultural University, Pusa, namely, (1) Sukhet (Madhubani), (2) Jale (Darbhanga), (3) Manjhi (Saran), (4) Bhagwanpur Hat (Siwan), (5) Sipaya (Gopalganj), (6) Sheohar, (7) Sitamarhi, (8) Madhopur (West Champaran), (9) Narkatiaganj (West Champaran), (10) Parsauni (East Champaran), (11) Piprakothi (East Champaran), (12) Lada (Samastipur), (13) Birauli (Samastipur), (14) Vaishali, (15) Turki (Muzaffarpur), (16) Saraiya (Muzaffarpur), and (17) Khodabanpur (Begusarai), under the Project “Climate Resilient Agriculture (CRA) Program” funded by the Government of Bihar, India, during kharif seasons of 2020 and 2021, rabi seasons of 2020–2021 and 2021–2022, and summer seasons of 2021 and 2022. The location of the study area is given in Figure 1. The depth of the water table in the study area varies from 2 to 5 m below ground level (Anon, 2022).
2.2 Climate, soil, and cropping system
The region has a sub-humid subtropical monsoon climate. About 85% of the annual rainfall occurs during the monsoon season. Considering the cropping season, it is known as kharif season, during which rice crop is grown. The region experiences four seasons, viz., Summer (March–May), Monsoon (June–September), Post-Monsoon (October–November), and Winter (December–February). The average annual rainfall of the region ranges from 1,230 to 1,400 mm. The month-wise rainfall distribution pattern is given in Figure 2. May is the warmest summer month of the year with a daily maximum temperature of 37–41°C, while the coldest winter month is January with a daily minimum temperature of 5–8°C in January (Bal et al., 2023). Although December, January, and February are the main winter months, temperature decreases significantly from November. The average temperature falls appreciably from 26.9°C in October to 21.9°C in November.
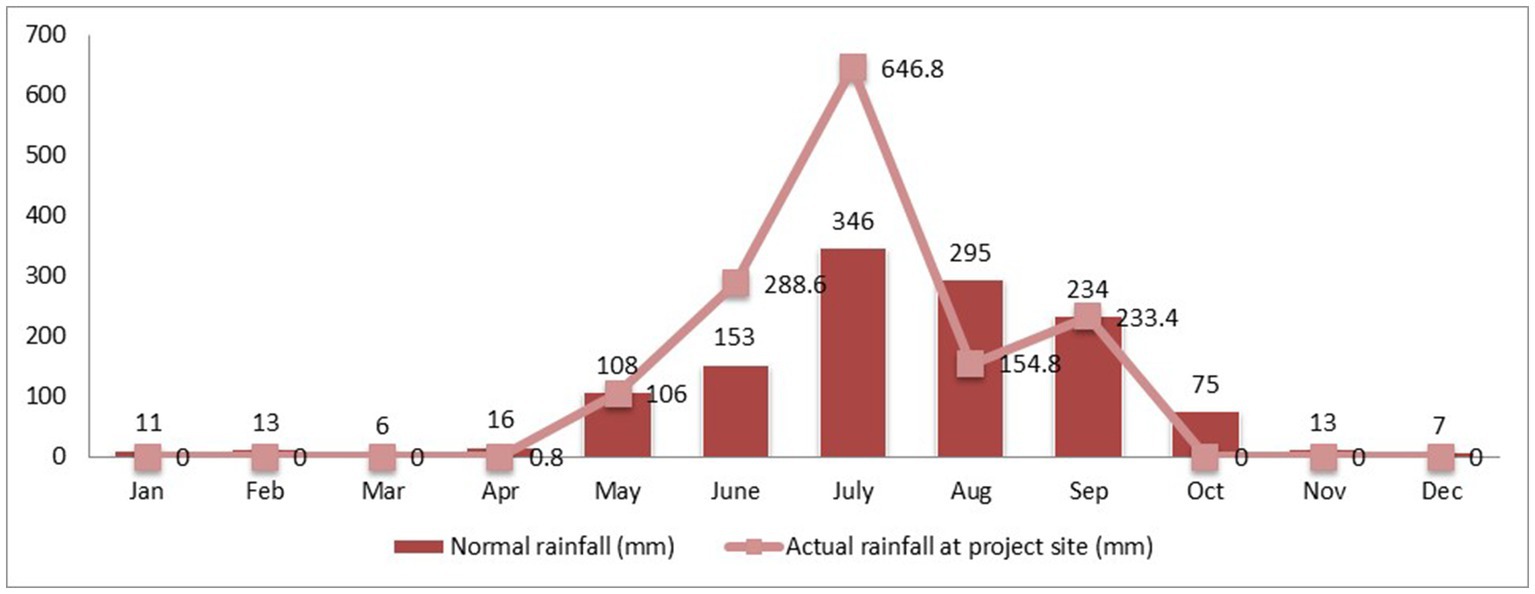
Figure 2. Comparative distribution pattern of normal rainfall (mm) and actual rainfall over the study area.
Soils of the study area are mainly young alluvium, calcareous, and predominantly sandy loam to loamy in texture. Soils are deep having calcium carbonate more than 10%. The water holding capacity varies from moderate to high. It has moderate drainage behavior. There is a wide variation in the nitrogen and available potassium status of these soils. Soil pH varies from 6.5 to 8.4. Rice–wheat is the major cropping system of the region and comprises about 60% of all crops and cropping sequences followed by the farmers (Kumari et al., 2022).
2.3 Methodology
2.3.1 Field criteria adopted for the study
The villages were selected through the Participatory Rural Appraisal Survey. In this region, late sowing and transplanting of rice are a usual practice due to the lack of affordable irrigation facilities and farmers’ dependency on monsoon rains both for transplanting and for meeting further irrigation requirements during its growing period (Najmuddin et al., 2018). While selecting the villages, it was kept in mind that wheat sowing gets delayed in the area due to late harvesting of paddy, thus forcing the crop to be affected by terminal heat stress. In addition, another criterion was that the successive green gram crop was either non-productive or non-remunerative due to the late harvesting of wheat in these villages. In case of late harvesting of wheat following late planting, subsequent summer green gram is affected on many occasions due to the initial burst of pre-monsoon showers and monsoon rains, leading to crop damage. An area of 15 acres was selected in each of 17 villages, and one 4-inch tube well fitted with a 3-hp single-phase submersible pump was installed with a discharge capacity of 7–9 lps and irrigation command capacity of 15–20 acres each costing around 1.6 lakhs. While comparing the cost with a 15-hp three-phase tube well, it comes to around 17 lakhs per tube well. Moreover, these pumps do not perform well due to voltage fluctuations. It has a higher discharge rate as compared to the water demand of crops, it causes losses in irrigation water, and consequently, low water use efficiency is observed when compared with a 3-hp single-phase tube well. A group of 10–20 farmers from each village was formed with an agreement that all participating farmers will have an equal right and equal opportunity to avail irrigation facility as and when needed by the crop. One rural youth of the same adopted village was given the task of scheduling irrigation with a nominal cost of INR 15 per hour. The amount, thus collected, was deposited in a revolving account for future management of the tube wells, operational cost, and honorarium for the youth facilitator.
Long-duration high-yielding rice variety (Rajendra Mahsuri-1) of 150-day duration was sown on 20–25 May in the nursery and transplanted on 15–20 June after puddling. In total, 2–3 irrigations were applied to the crop to maintain soil moisture above field capacity. Scheduling of irrigation was based on the disappearance of standing water from the crop field, and it was ensured that cracking in the rice field does not develop. The same variety of rice was sown during 10–15 June in the nursery and transplanted during 10–15 July, which is generally practiced by the farmers near the experimental plots. After the harvest of paddy, HD-2967 variety of wheat was sown on 7 November, and 2–3 irrigations were provided as per soil type and physiological demand of the crop. The same variety of wheat was sown during 25–30 November (regarded as delayed sowing) after the harvest of paddy crops during 15–20 November. The third crop of green gram was sown on 10 and 25 April, respectively, at different locations. All the agronomic parameters and yield attributing characters were studied, recorded, and analyzed.
Nitrogen, phosphorus, and potassium were applied to the rice crop in the ratio of 120:60:40. During the growing period of the crop, 50% of nitrogen and full doses of phosphorus and potassium were used as basal application. The remaining nitrogen was applied in two equal splits: one at tillering and another at the panicle initiation stage. For weed management, two hand weedings were done at 30 and 60 days after transplanting. In the case of wheat, nitrogen, phosphorus, and potassium at 120, 60, and 40 kg ha−1 were applied. All the phosphorus and potassium and half dose of nitrogen were applied at sowing as basal dose. The remaining half dose of nitrogen was top dressed in two equal splits at crown root initiation and boot stages.
2.3.2 Calculation of water productivity
Crop water productivity, irrigation water productivity, and effective rainfall were calculated using standard methodologies. Renfro equation as quoted by Chow (1964) has been employed in this study to work out effective rainfall as follows:
where Re is effective rainfall, Rg is growing season rainfall, A is average irrigation application, and E is the ratio of consumptive use of water (CU) to rainfall during the growing season.
The water productivity of rice was determined as the grain yield obtained per unit of rainfall plus irrigation water applied (Chahal et al., 2007).
2.3.3 Detection of change in rainfall pattern
As mentioned earlier, 17 locations were selected for the study. To detect the change in rainfall pattern over the study area, the daily rainfall of four stations (located in the study area) based on the availability of data for a period of 1990–2019 was analyzed. These stations are, viz., Pusa (25.98°N, 85.67°E; Samastipur district), Muzaffarpur (26.07°N, 85.24°E; Muzaffarpur district), Motihari (26.65°N, 84.91°E, East Champaran district), and Chhapra (25.78°N, 84.73°E, Saran district). Two sets of data, viz., 1990–2019 and 2010–2019, were considered to detect the changes in mean values of annual rainfall and rainy days, and number of dry days during monsoon. Trends and significance of these parameters were determined based on the Mann-Kendall Test (Mann, 1945; Kendall, 1975; Yadav et al., 2014), which is a function of the ranks of the observations rather than their actual values (Oguntunde et al., 2011). The test is non-parametric for identifying trends and is not affected by the actual distribution of the data and is less sensitive to outliers. Trends of maximum and minimum temperature during different months of the wheat growing season were also worked out.
3 Results and discussion
3.1 Effect of preponing sowing dates on yield attributes and yield of rice
The grain yield of rice sown during 20–25 May with assured irrigation was found to be 5.2 t ha−1, whereas the yield obtained for 10–15 June sown rice with farmers’ practice was 3.2 t ha−1, which was 62.5% higher over the later sown crop (Table 1). This quantum jump was only because of higher physiological maturity days of the timely sown crop and the availability of proper moisture regimes in the rice field, fed through assured irrigation. In the late-sown crops, the growth period was short that ultimately limited the leaf surface area, panicle length, and number of grains per panicle, probably due to limited moisture in the field during dry spells, as this crop was not scientifically managed by the farmers. Delayed sowing had a bearing on the seed-setting rate. Ahmed et al. (2011) while working on rice observed that the 1,000-grain weight and the seed-setting rate decreased beyond the temperature of 27.0°C. Considering the 1,000-grain weight, it was found to be 6.4% higher in the case of early sown crops (23.0 g) as compared to late-sown crops (21.6 g). Quite contrary to this, the number of unfilled tillers per m2 was found to be 5.8% higher in late-sown crops (24.3), compared to early sown crops (22.9). The critical temperature for inducing spikelet sterility in rice varied from 10 to 15°C (Tinarelli, 1989). Alvarado (2002) found that the average temperature under 20°C for 5 days during flowering stage increased the probability of obtaining spikelet sterility greater than 10–12%. The total biological yield (including both grains and straw) was found to be 52.3% higher in early sown crops (12.18 t ha−1) as compared to late-sown crops (8.0 t ha−1) with harvest index of 42.8 and 39.8%, respectively. Harvest index refers to quantify the grain yield versus total amount of biological yield that a crop produces, signifying the reproductive efficiency of the crop.
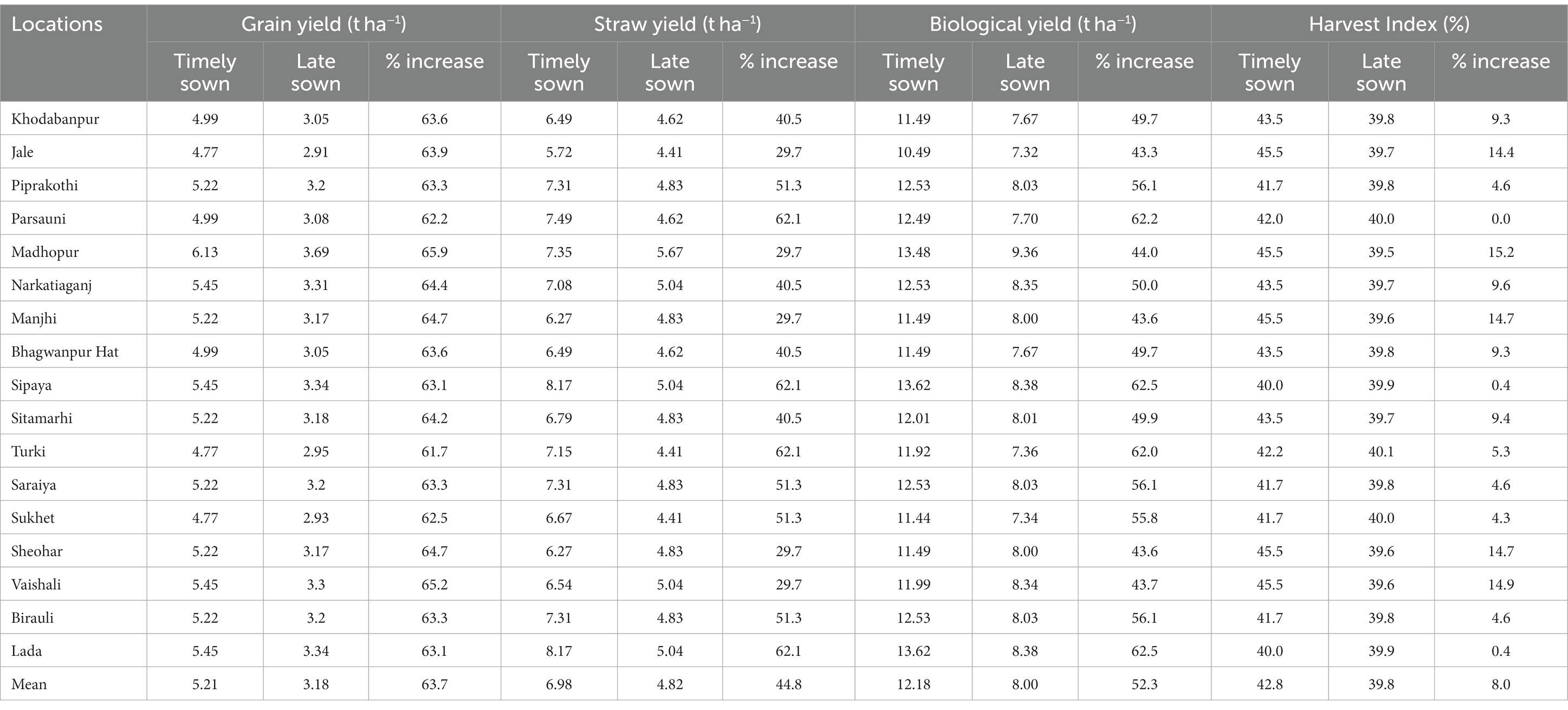
Table 1. Grain yield, total biological yield, and harvest index of rice under timely sown and late-sown conditions.
Early sown crops of rice recorded a 19.9% higher number of tillers (245.4 per m2) in comparison with the late-sown crops (204.8 per m2) (Table 2 and Figures 3, 4), which might be ascribed to the fact that younger age (21 days) of the seedlings with higher tillering capacity contributed to this increase. The old aged seedlings, of 30 days or more duration, as in the case of late transplanted rice might have produced a lesser number of tillers, probably some of its early vigor and tiller-bearing capacity got exhausted in the nursery itself because of its age. The aged seedlings as transplanted for late-sown crops might have experienced greater uprooting and transplanting shocks, prompting the crop to remain at an initial slow rate of growth. The number of panicles per m2 and number of grains per panicle in early sown rice were found to be 23.4 and 24.5% higher (222.5 and 142.2, respectively), compared to late transplanted crops (180.5 and 114.3, respectively). The number of empty spikelets increased with shading and low temperature, and a decrease in filled grain percentage was observed due to the lower solar radiation. In long-duration varieties, low light stress synchronizing with the vegetative lag phase resulted in considerable tiller mortality and fewer productive panicles per square meter (Murty et al., 1975). The reasons for higher yield, higher number of tillers per m2, higher number of panicles per m2, and number of grains per panicle might be due to congenial thermal and as well field moisture balance in the timely sown crop than the crops conventionally raised by the farmers. Moisture stress after 10 days of 50% flowering significantly reduced single panicle weight, test weight, fertile spikelets per panicle, and total spikelets per panicle and significantly increased sterile spikelets per panicle.
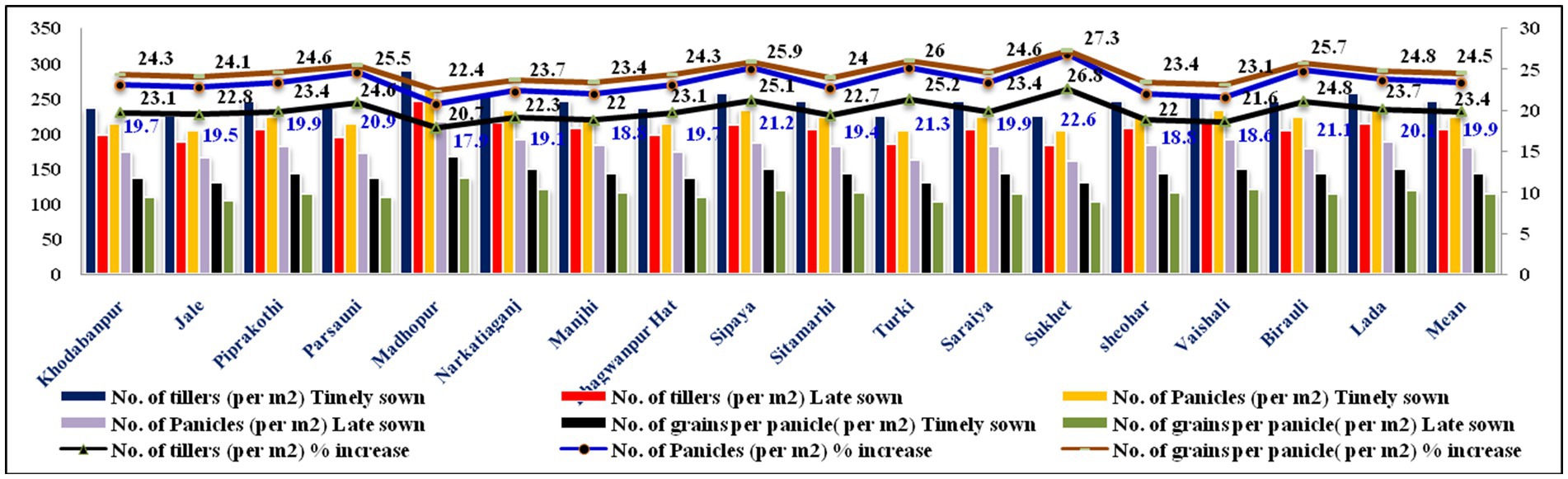
Figure 3. Yield attributing traits such as number of tillers per m2, number of panicles per m2, and number of grains per panicle under timely and late-sown rice in Bihar.
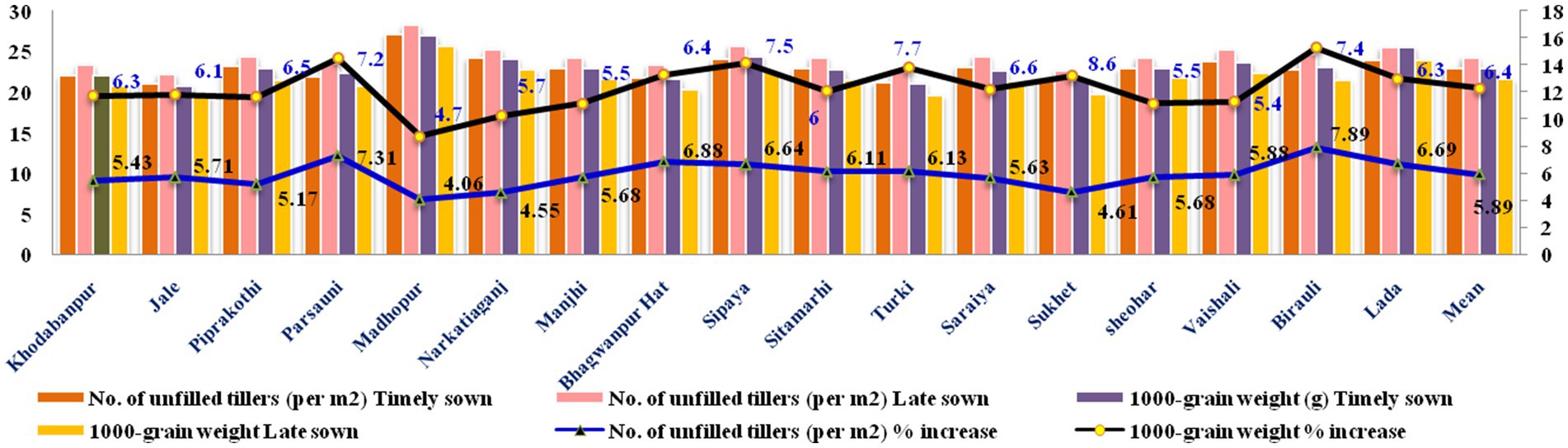
Figure 4. Yield attributing traits such as number of unfilled tillers per m2 and 1,000 grain weight under timely sown and late-sown rice in Bihar.
Weather data presented in Figure 2 revealed that there was hardly any variation in actual and normal rainfall during May. However, June and July experienced deficit rainfall. Under such a water-stressed environment, the resource-poor farmers and the farmers with limited or no provision of irrigation sowed rice during 10–15 June in the nursery and transplanted during 10–15 July in the main field. The experiment revealed that in the late transplanted rice as in the case of farmers’ practice, the days taken to attain 50% flowering decreased substantially as compared to early sown crops. The 15–20 June transplanted rice took 91 days to attain 50% flowering and 116 days to attain the dough stage, while the 10–15 July transplanted rice took 84 days to attain 50% flowering and 101 days to attain the dough stages. Thermal time exerts great influence on the growth and yield of crops. Sattar et al. (2017) observed that the rice crop sown on 15 July required 2 days more to attain the maximum tillering stage than that sown on 31 May. However, the duration required to attain 50% flowering on 31 May and 15 July sown crops was 109 and 96 days, respectively. The crop sown on 31 May attained maturity in 142 days, while the crop sown on 31 July reached maturity in 119 days. Kobayashi et al. (2010) concluded that higher air temperature and incident radiation tend to advance anthesis in rice. Thus, delayed transplanting forced the crop to pass through a relatively lower temperature regime during its reproductive phase (Bal et al., 2023). They also reported significant variation in rice yield in response to weather variabilities during different growth phases of the crop under the diverse ecosystems of India. Srivastava et al. (2018) observed that with delayed transplanting, the percentage of chaffy grains per panicle increased, which resulted in reduced grain yields. This is in conformity with the results of previous research, where the setting of lower temperature resulted in the irregular opening of flowers and inadequate filling of the panicles (Venkataraman and Krishnan, 1992). The maximum temperature required for proper germination of pollen should be 33–34°C (Grist, 1986). Moreover, late transplanting produced lower yields due to the higher percentage of chaffy grains in the panicle under a non-congenial temperature regime (Anon, 2016). The formation of chaffy grains coincided with the prevalence of lower air temperature (Tmax < 32.5°C and Tmin < 23.0°C) during the 50% flowering to dough stage of rice crop (Sattar et al., 2017).
3.2 Effect of preponing sowing dates of rice on yield and productivity of succeeding wheat crop
In the rice–wheat–green gram cropping system, the HD-2967 variety of wheat was sown on 7 November after the harvest of paddy on 30 October under a timely sown assured irrigation system, whereas in the farmers’ practice, wheat was sown during 25–30 November after the harvest of paddy crops during 15–20 November. Timely sowing of wheat in the cropping system in the experimental plots was made possible because of the timely harvesting of the previous rice crop. This resulted in a drastic change in yield and yield attributing characteristics of wheat. On average, a 61% increase in grain yield was observed for the crop sown at the appropriate sowing window, compared to late sowing (Table 3). In one study at New Delhi, a decrease in wheat yield by 70, 29, and 77 kg ha−1 per day due to delay in sowing beyond the first week of November was observed in varieties HD-2932, WR-544, and HD-2967, respectively (Dubey et al., 2019). In our study, the total biological yield (both grain and straw) was 51.3% higher in timely sown wheat (11.8 t ha−1), as compared to late-sown wheat (7.8 t ha−1) with a 6.6% increase in harvest index. The lower grain, straw, and biological yields in the late-sown wheat might be due to the negative impact of higher temperature regimes and terminal heat stress during its flowering and milking stages (Sattar et al., 2020; Sattar and Srivastava, 2021). They reported enhanced productivity of rice–wheat cropping system in Indo-Gangetic plains through manipulation of sowing windows of both crops. The number of shoots per m2, number of spikes per m2, and number of grains per spike in the timely sown wheat were 9.0, 9.0, and 24.8% higher, respectively, as compared to late-sown wheat (Table 4 and Figure 5). The number of fertile spikelets and 1,000-grain weight were also found to be, respectively, 8.9 and 6.3% higher in timely sown wheat as compared to late-sown wheat, whereas the number of unfilled spikes was 9.4% higher in late-sown wheat as compared to timely sown wheat (Figure 6). In case of grain yield, it was observed to be 60.9% higher in timely sown wheat (4.9 t ha−1) as compared to late-sown wheat (3.05 t ha−1). Both maximum temperature and minimum temperature played a decisive role in determining the grain yield of wheat. Optimum thresholds of maximum and minimum temperatures during different growth stages of wheat for achieving higher yields in the study area revealed that temperature above 30.2°C during the 50% flowering to the milking stage and temperature above 33.1°C during the 50% flowering to maturity stage reduced grain yield below 2.0 t ha−1. Similarly, a minimum temperature of 16.8°C during the 50% flowering to the milking stage and a minimum temperature of 18°C during the 50% flowering to maturity stage significantly affected the crop yield, and it produced a yield below 2.0 t ha−1. For achieving a yield target of 4.0 t ha−1 or more, Sattar et al. (2020) while working on crop weather relationships on wheat observed that the maximum and minimum temperatures from sowing to tiller formation should be 23.7 and 11.8°C, respectively. Similarly, the maximum and minimum temperatures from 50% flowering to milking should be 24.6 and 11.6°C, respectively, and from 50% flowering to maturity, it should be 29.2 and 14.4°C, respectively (Table 5).
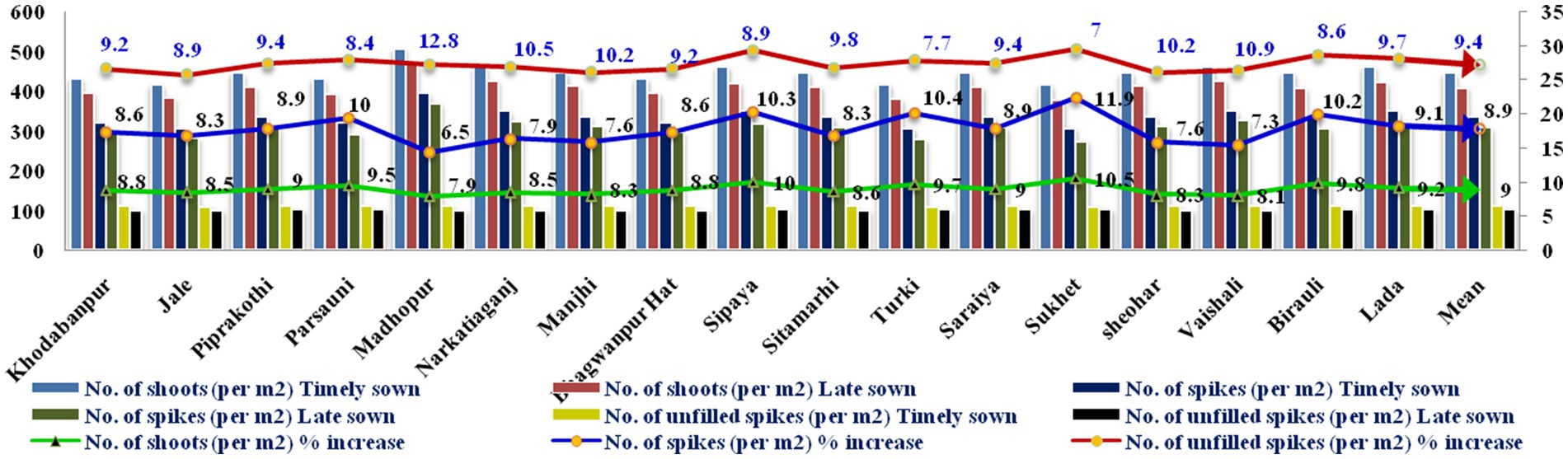
Figure 5. Yield attributing traits such as number of shoots per m2, number of spike per m2, and number of unfilled spike per m2 under timely sown and late-sown wheat crops.
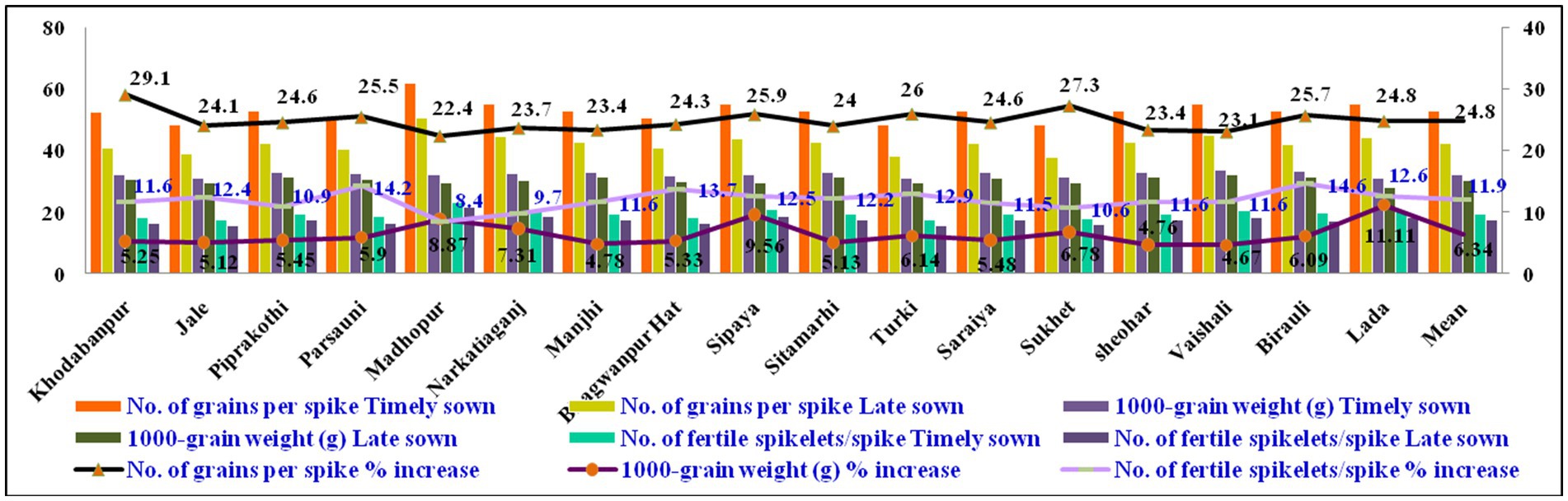
Figure 6. Yield attributing traits such as number of grains per spike, 1,000 grain weight, number of fertile spikes, and number of grains per spike under timely sown and late-sown wheat crops.
A comparative analysis of the ideal temperature (Table 5) suitable for wheat growth and actual temperature during the experimental years (Table 6) revealed that the wheat crop sown on 7 November experienced optimum temperature during flowering to maturity stages, while the late-sown crop encountered higher temperature, resulting in lesser number of panicles per m2, lesser number of grains per panicle, lesser 1,000-grain weight, and higher number of unfilled grains per m2. Moreover, there is a general trend of increasing minimum temperature and decreasing maximum temperature during the later growing period of wheat (Table 7), which tends to increase the rate of photorespiration and thereby reduces the net photosynthesis. Accordingly, the increase in minimum temperature in general tends to affect the process of anthesis to a great extent. It could be inferred from the results that the sowing of wheat around 7 November instead of 25–30 November could reduce the negative impact of increasing temperature during the fag end of the growing season on the growth and yield of wheat and consequently help the farmers to achieve higher yield.

Table 6. Average thermal time and maximum temperature during different phenological stages of wheat.

Table 7. Temperature trends during the wheat growing season at Pusa located in the study area (database 1990–2019).
3.3 Changing rainfall patterns in the study area, crop water productivity, and effective rainfall for timely and late transplanted rice
Since we are discussing about the importance of monsoon rainfall, climatic risks and assured rainfall on rice productivity, and its sustainability under timely and late conditions, it would be prudent to assess the trend and variability of rainfall in the study area in relation to the water use and water productivity of rice. In this context, we have evaluated the change in rainfall patterns using time series data. The result revealed that rainfall in the study area has decreased significantly, leading to drier conditions in the recent 10-year period (Table 8). The maximum number of dry days during the monsoon season over the last 30 years (1990–2019) was observed to be 70 days, which increased to 87 days during the recent 10-year period (2010–2019). The peak rate of increase in the number of dry days during monsoon is calculated as 1.5 days per year, signifying the decrease in annual rainy days in the region. Moreover, decreasing trends in annual rainfall were found to occur over the area. This poses a threat to the sustainability of rice crop production as more than 60% of rice is cultivated under rainfed conditions during monsoon season. Hence, erratic behavior of rainfall, decreasing number of rainy days, and increasing dry spell have enormous stakes in rice productivity. In this context, it is necessary to adopt climate-resilient interventions such as changing planting schedule, community irrigation approach, and provision of assured irrigation to sustain rice production in the region. A dry spell if coincided with critical growth stages of rice leads to reduced crop yield and sometimes crop failure. Sattar and Srivastava (2021) evaluated the performance of rice crops under different moisture regimes as induced by different planting dates and observed that shifting planting dates helped achieve higher water productivity and grain yield.
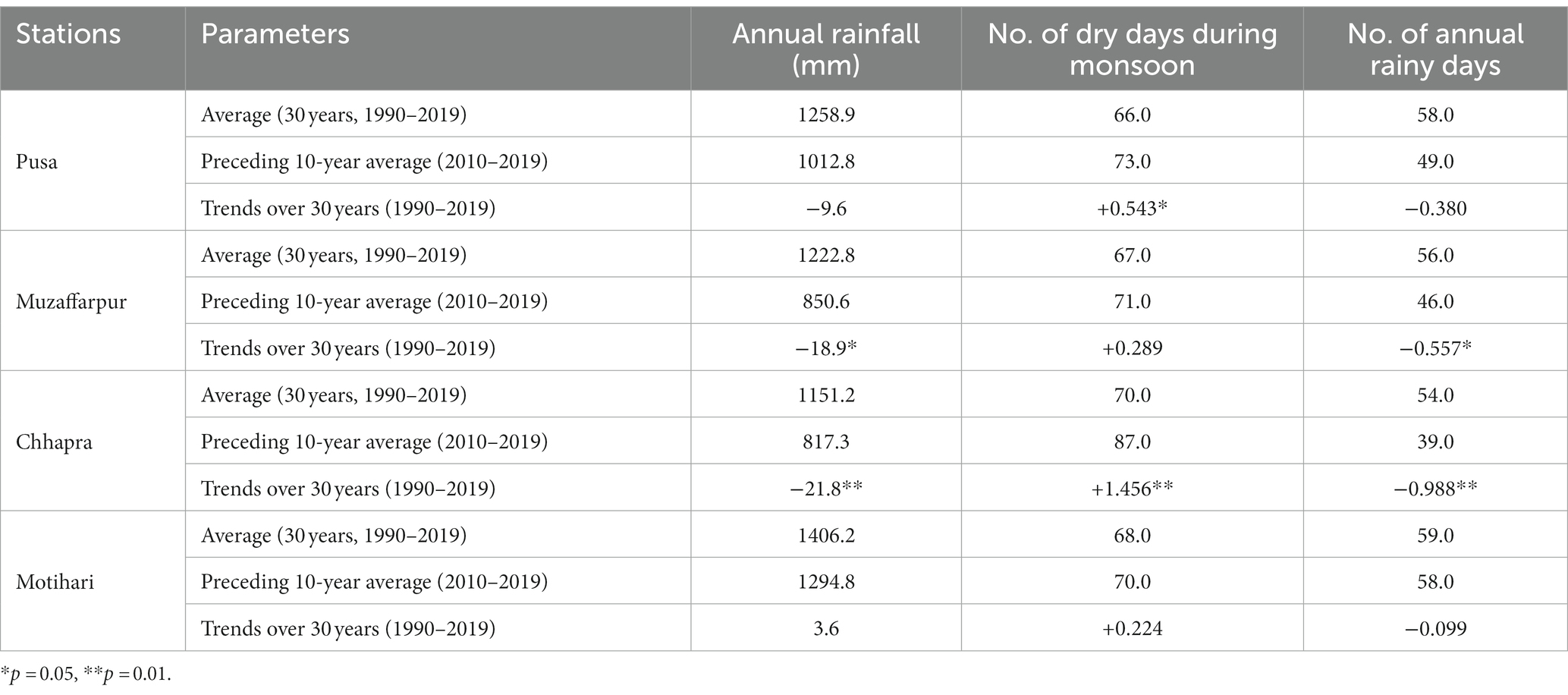
Table 8. Trend statistics of annual rainfall, number of dry days during monsoon, and annual rainy days at different locations over the study area.
The data on water productivity and effective rainfall of timely and late transplanted rice grown at 17 locations of the study area revealed that the water productivity of timely transplanted kharif rice ranged from 0.673 kg m−3 ha−1 at Khodabanpur to 1.052 kg m−3 ha−1 at Madhopur (Table 9 and Figure 7). On the other hand, it varied from 0.370 kg m−3 ha−1 at Bhagwanpur Hat to 0.560 kg m−3 ha−1 at Madhopur for late transplanted rice. The highest water productivity of rice for both timely and late transplanted conditions was recorded for Madhopur, while lower values were observed for Khodabanpur. It was observed that the average water productivity of timely transplanted rice was 85.8% higher than that of late transplanted one. Studies showed that the water productivity of rice can be increased by shifting transplanting dates (Jalota et al., 2009; Sattar and Srivastava, 2021). The higher water productivity of timely transplanted rice in comparison with late transplanted one may be attributed to higher yield, resulting from longer growing period and assured irrigation. The water productivity decreased with a decrease in crop duration when transplanting was delayed beyond 30 June (Sattar and Srivastava, 2021). While considering the irrigation water productivity for timely and late transplanted rice, it was found to vary from a low of 2.38 kg m−3 ha−1 to a high of 8.76 kg m−3 ha−1 and from 1.45 to 5.27 kg m−3 ha−1, respectively (Figure 8). The highest and the lowest values of irrigation water productivity under both conditions were associated with rice grown at Bhagwanpur Hat and Madhopur, respectively. The water productivity of rice in the present study under timely and late conditions varies with other studies conducted elsewhere, perhaps due to differential growing environments, growing period length, water use, and grain yield. While working on rice water productivity, Tuong et al. (2005) reported an average water productivity of 0.4 kg grain m−3 with respect to total water input (irrigation plus rainfall). Under water-stressed environment, an increase in water productivity to 0.8–1.0 kg grain m−3 was reported (Kato et al., 2009; de Vries et al., 2010). Zwart and Bastiaanssen (2004) estimated the water productivity and evapotranspiration requirement of rice as 0.6–1.6 kg m−3 and 400–800 mm, respectively.
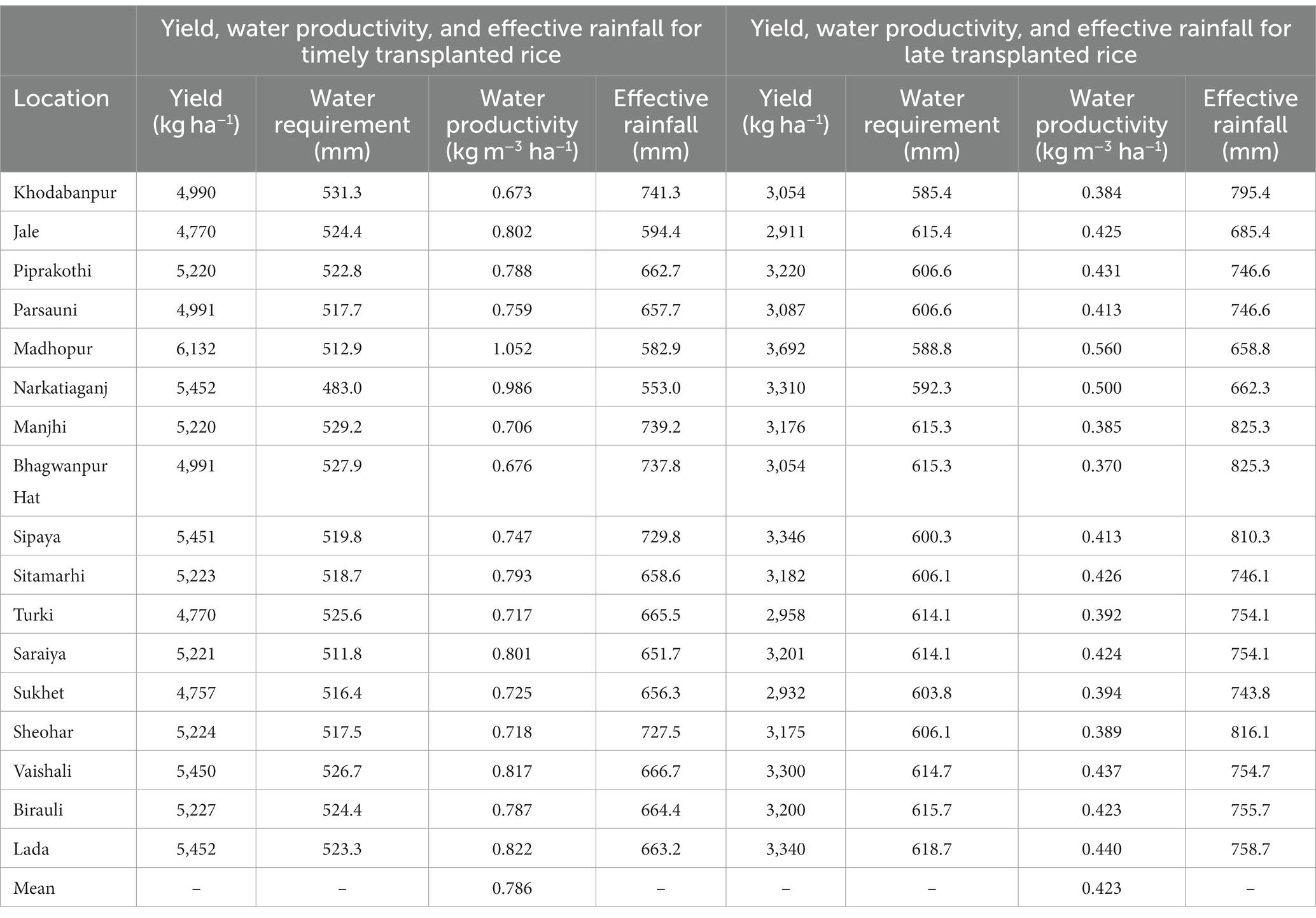
Table 9. Yield, water productivity, and effective rainfall for timely and late transplanted kharif rice at different locations over the study area.
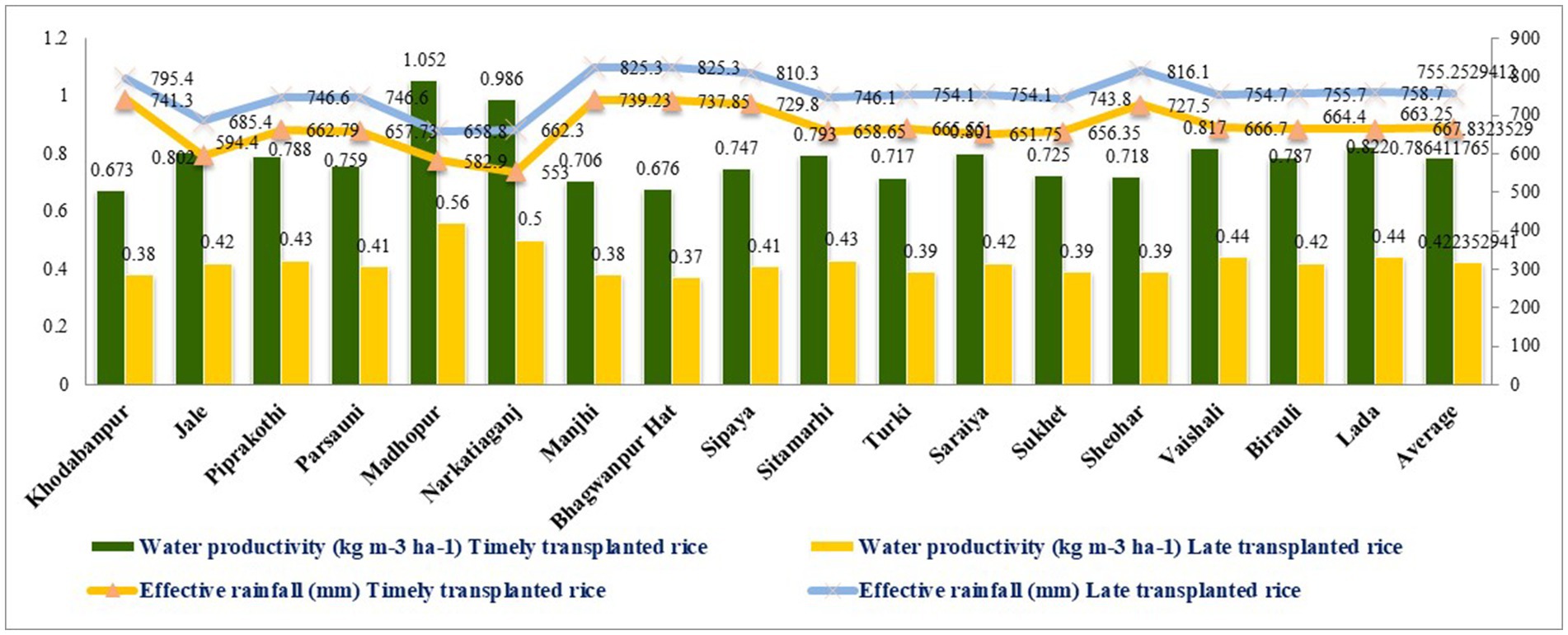
Figure 7. Water productivity and effective rainfall for timely and late transplanted kharif rice at different locations over the study area.
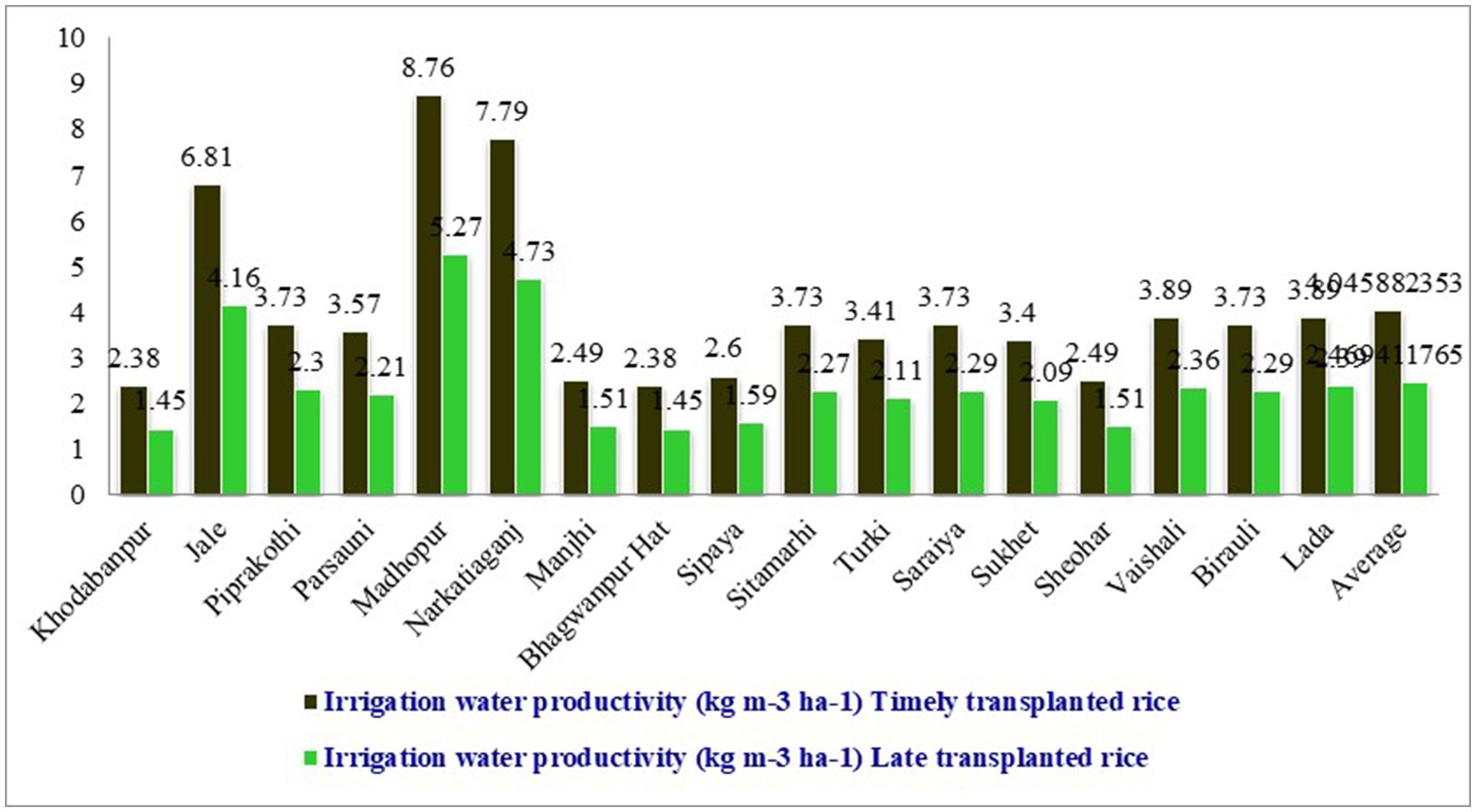
Figure 8. Irrigation water productivity of timely and late transplanted kharif rice at different locations over the study area.
The variations in effective rainfall during the growing season of early and late transplanted rice are presented in Table 9. In general, the effective rainfall was higher for the late transplanted rice. The effective rainfall for timely transplanted rice ranged from a low of 553.0 mm to a high of 741.3 mm across the study area. In the case of late transplanted rice, it was found to vary from 658.8 to 825.3 mm. Since the late transplanted crop in farmers’ field faced moisture stress of varying intensity, the efficiency of rainwater utilization was much higher leading to higher effective rainfall. A small amount of rainfall in dry soils could be more useful and effective, whereas the same amount of rainfall in wet soil may be insignificant. Chang (1963) observed that the effective rainfall increased with the storage capacity and drying condition of the soil. In the present study, lesser rainfall availed for late transplanted crops might have been more effective in meeting evapotranspiration requirements of the crop. On the other hand, for early transplanted crops, a higher fraction might have been ineffective due to seepage and percolation losses.
Considering water-saving methods, direct seeded rice (DSR) and System of Rice Intensification (SRI) planting methods of rice are gaining importance under water-stressed environments in the wake of climate change. At present, it is only about 2.5% of the total rice-growing area in Bihar. In the study area, the farmers tend to opt it for upland areas under conditions of erratic monsoon. On the other hand, the SRI method does not find any taker among the farmers of the region.
3.4 Effect of early wheat harvesting on the yield of subsequent green gram
The manipulation of sowing dates of both rice and wheat in the cropping system provided sufficient space and opportunity for the sowing of green gram at appropriate time with the provision of assured irrigation. Thus, the early sowing of rice–wheat helped us to achieve a grain yield of green gram to the tune of 7.21 t ha−1 (Table 10), whereas in the farmers’ practice, the yield was much lower (6.09 t ha−1). An obvious increase of 18.4% in the grain yield of green gram was recorded.
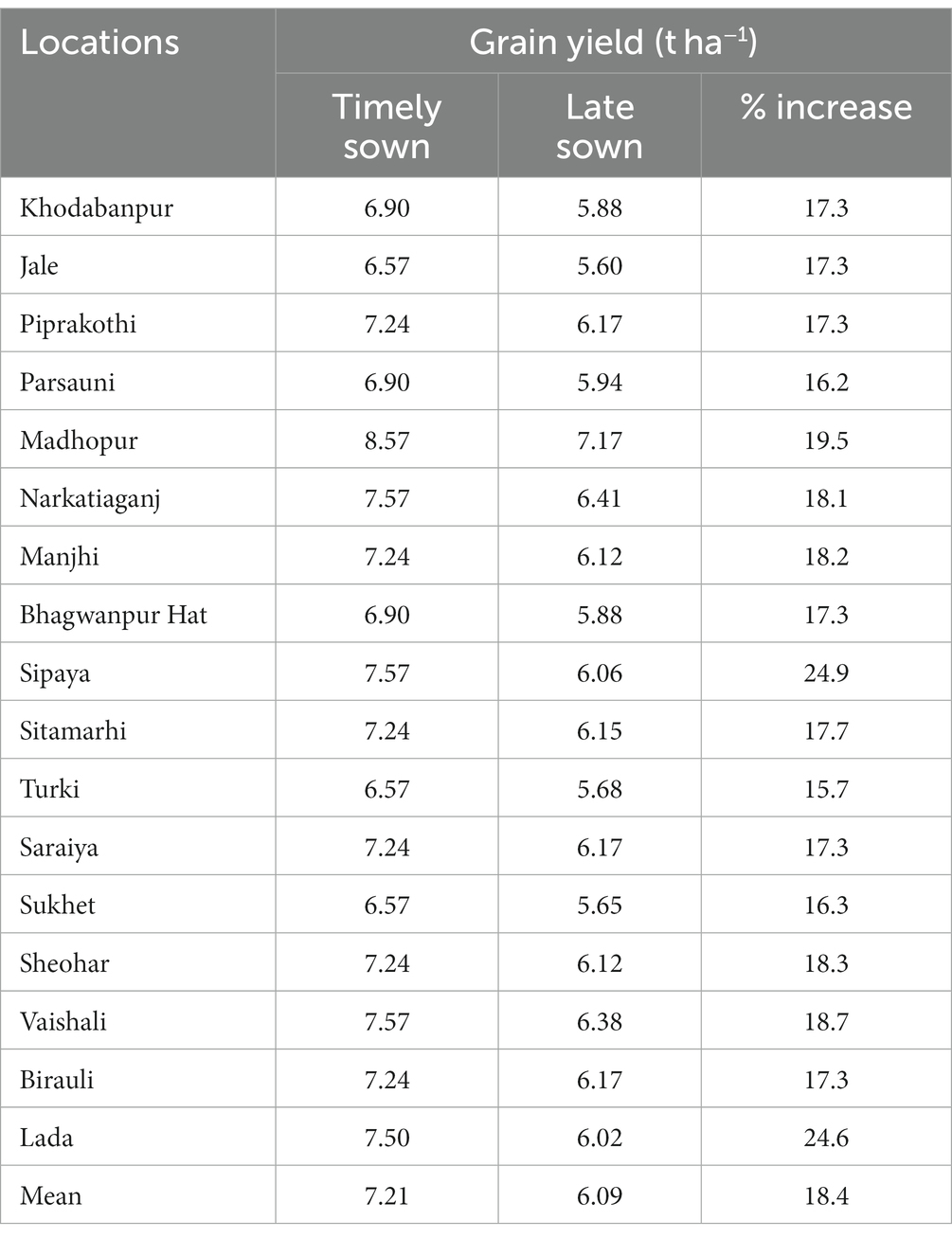
Table 10. Yield performance of green gram at different locations under timely sown and late-sown conditions.
3.5 Effect of optimization of the sowing window on the system productivity and economics of rice–wheat–green gram system
The cropping intensity of experimental plots and the farmers’ practice was found to be >300%. However, the rice equivalent yield (REY) of timely sown assured irrigation plots was found to be 13.25 t ha−1, whereas under farmers’ practice (delayed condition), REY was observed as 8.89 t ha−1. The production efficiency was found to be 35.3 kg ha−1 day−1 for timely sown assured irrigation plots. On the other hand, it was paltry (24.0 kg ha−1 day−1) under farmers’ practice. In the case of the land use efficiency, it was found to be 96.9% under timely sown assured irrigation plots, and under farmers’ practice, it was 93.1%. Net return from rice was found to be INR 76117.00 per hectare with a benefit: cost ratio of 2.15 in the timely sown crop as against the net return of INR 36803.00 with benefit: cost ratio of 1.04 under the farmers’ practice. Considering wheat from the same piece of land, the net return was INR 86890.00 with benefit: cost ratio of 2.44 in the treated plots as against INR 59315.00 and 1.72, respectively, from the farmers’ practice. The green gram provided a net return of INR 34972.00 with benefit: cost ratio of 1.54 in the treated plots as against the net return of INR 25160.00 with benefit: cost ratio of 1.05 for the crop grown under farmers’ practice.
4 Conclusion
By manipulating the sowing dates of rice and wheat, the adverse impacts of climate variability can be minimized to achieve higher system productivity. Moreover, the provision of an assured irrigation system can help ward off the negative impact of dry spells and unfavorable soil moisture regimes on rice production. The major source of irrigation is groundwater, which is extracted by diesel pump sets or by a three-phase 5/7.5 hp. electric pumping system. However, this requires high infrastructure, which is time taking, and voltage fluctuation is a major limiting factor. The high cost of irrigation coupled with the late onset of monsoon is also a major factor, which compels the farmers of the region to go for late sowing and late transplanting. Hence, the productivity of the crop remains low, and under such situation, the state is bound to grow rice in 3.2 million hectares to meet the food requirements. If infrastructure is created for less costly irrigation systems with 3-hp single-phase tube wells fitted with submersible pumps, the productivity can be increased by 50–60% and the food demand of the state can be supported with only 1.2–1.5 million hectares of land with a lower cost of cultivation and lesser investment. Accordingly, the remaining land can be diversified for other cash crops and agri-entrepreneurship development. Alternatively, if rice cultivation is still continued in 3.2 million hectares, the production can be almost doubled. The same is the case with wheat, where we can enhance the productivity from 2.9 to 5.0 t ha−1 with timely sowing and assured irrigation to escape the impacts of terminal heat. With this productivity of wheat, the food demand of the state can be achieved with just 60% of the wheat growing area, and the leftover land can be diversified for other enterprises. Green gram can be best suited in the rice–wheat system, if the provision of timely sowing and assured irrigation is made with the installation of 3-hp single-phase tube wells with submersible pumps.
The results of the study showed that with the assured community irrigation system and shifting planting dates, the productivity of rice–wheat cropping system can be achieved greater than 10.0 t ha−1 with a cropping intensity of 300% for better adaptation and sustainable production. Moreover, the water productivity of timely transplanted rice was observed to be 85.8% higher than that of late transplanted one. With the application of our simple innovative technology, the negative impacts of climatic challenges on crop production can be resolved to a large extent in the region for achieving higher productivity of rice–wheat cropping system. However, it is important to assess the impacts of the technology with regard to enhanced farm income, alleviation of rural poverty, and lesser energy utilization. On the other hand, the impact of the technology with respect to groundwater depletion would be a topic for further investigation for upscaling the benefits among the farmers at larger domain.
Data availability statement
The original contributions presented in the study are included in the article/supplementary material, further inquiries can be directed to the corresponding author.
Author contributions
RJ: Conceptualization, Investigation, Writing – original draft, Writing – review & editing, Funding acquisition, Methodology, Resources. AbS: Conceptualization, Writing – original draft, Writing – review & editing, Methodology. AnKS: Project administration, Resources, Supervision, Visualization, Writing – review & editing. MK: Investigation, Supervision, Writing – review & editing. RT: Writing – review & editing. AbKS: Writing – review & editing, Investigation, Project administration, Resources. ArKS: Writing – review & editing, Data curation, Investigation, Project administration, Resources. SudhD: Writing – review & editing, Investigation, Project administration, Resources. RP: Writing – review & editing, Investigation, Resources. SKus: Writing – review & editing, Investigation, Methodology, Project administration, Resources. AK: Writing – review & editing, Data curation, Investigation, Resources. MM: Writing – review & editing, Investigation, Methodology, Project administration, Resources. PS: Supervision, Visualization, Writing – review & editing. SG: Writing – review & editing, Investigation, Project administration, Resources. DS: Investigation, Resources, Writing – review & editing. SaR: Investigation, Methodology, Resources, Writing – review & editing. SKG: Writing – review & editing, Investigation, Methodology, Project administration, Resources. RR: Writing – review & editing, Investigation, Resources. RIP: Writing – review & editing, Investigation, Resources. APS: Writing – review & editing, Investigation, Resources. RPS: Writing – review & editing, Data curation, Investigation, Methodology. PKSi: Resources, Writing – review & editing, Investigation, Methodology. PKSr: Project administration, Resources, Writing – review & editing. BJ: Data curation, Supervision, Writing – review & editing. RSe: Data curation, Visualization, Writing – review & editing. SudeD: Data curation, Formal analysis, Writing – review & editing. SS: Data curation, Writing – review & editing. GS: Data curation, Investigation, Writing – original draft, Writing – review & editing. ShR: Data curation, Writing – review & editing. NiK: Data curation, Writing – review & editing. AR: Data curation, Investigation, Writing – review & editing. SaK: Data curation, Resources, Writing – review & editing. VKa: Data curation, Methodology, Resources, Writing – review & editing. SuK: Investigation, Resources, Validation, Writing – review & editing. KC: Data curation, Investigation, Methodology, Writing – review & editing. TK: Investigation, Methodology, Resources, Writing – review & editing. SaP: Investigation, Methodology, Writing – review & editing. AG: Data curation, Resources, Writing – review & editing. AN: Data curation, Investigation, Writing – review & editing. AP: Investigation, Resources, Writing – review & editing. RSi: Investigation, Resources, Writing – review & editing. CR: Data curation, Formal analysis, Investigation, Writing – review & editing. ShP: Data curation, Investigation, Visualization, Writing – review & editing. KR: Data curation, Formal analysis, Validation, Writing – review & editing. SSP: Data curation, Investigation, Writing – review & editing. VKu: Data curation, Methodology, Writing – review & editing. LC: Investigation, Resources, Writing – review & editing. BH: Investigation, Resources, Writing – review & editing. STK: Data curation, Investigation, Writing – review & editing. JS: Data curation, Investigation, Writing – review & editing. SC: Data curation, Investigation, Writing – review & editing. ST: Data curation, Investigation, Writing – review & editing. NaK: Investigation, Writing – review & editing. DT: Investigation, Data curation, Writing – review & editing.
Funding
The author(s) declare financial support was received for the research, authorship, and/or publication of this article. This study was funded by the Climate Resilient Agriculture Program (CRAP), Govt. of Bihar, India.
Acknowledgments
This study has been carried out under the Climate Resilient Agriculture (CRA) Program funded by the Government of Bihar, India. The authors thank the authority of Dr. Rajendra Prasad Central Agricultural University for providing the necessary facilities for carrying out the research under the project.
Conflict of interest
The authors declare that the research was conducted in the absence of any commercial or financial relationships that could be construed as a potential conflict of interest.
Publisher’s note
All claims expressed in this article are solely those of the authors and do not necessarily represent those of their affiliated organizations, or those of the publisher, the editors and the reviewers. Any product that may be evaluated in this article, or claim that may be made by its manufacturer, is not guaranteed or endorsed by the publisher.
References
Ahmed, S., Islam, M. R., Alam, M. M., Haque, M. M., and Karim, A. J. M. S. (2011). Rice production and profitability as influenced by integrated crop and resources management. Eco-Friendly Agril. J. 4, 720–725.
Alam, M. K., Biswas, W. K., and Bell, R. W. (2016). Greenhouse gas implications of novel and conventional rice production technologies in the eastern-Gangetic plains. J. Clean. Prod. 112, 3977–3987. doi: 10.1016/j.jclepro.2015.09.071
Alvarado, J. R. (2002). Influence of air temperature on rice population, length of period from sowing to flowering and spikelet sterility, proceedings of the second temperate rice conference, Held at IRRI, Philippines.
Anon (2016). Annual progress report, All India Coordinated Research Project on Agrometeorology, DRPCAU, Bihar.
Anon, (2022). Ground water year book. Bihar: Government of India, Ministry of Jal Shakti, Department of Water Resources, River Development and Ganga Rejuvenation, Central Ground Water Board.
Arunrat, N., Pumijumnong, N., Sereenonchai, S., Chareonwong, U., and Wang, C. (2020). Assessment of climate change impact on rice yield and water footprint of large-scale and individual farming in Thailand. Sci. Total Environ. 726:137864. doi: 10.1016/j.scitotenv.2020.137864
Bal, S. K., Sattar, A., Nidhi, C., Chandran, M. A. S., Subba Rao, A. V. M., Manikandan, N., et al. (2023). Critical weather limits for paddy rice under diverse ecosystems of India. Front. Plant Sci. 14:1226064. doi: 10.3389/fpls.2023.1226064
Bhatt, R., Kukal, S. S., Busari, M. A., Arora, S., and Yadav, M. (2016). Sustainability issues on rice–wheat cropping system. Int. Soil Water Conserv. Res 4, 64–74. doi: 10.1016/j.iswcr.2015.12.001
Chahal, G. B. S., Sood, A., Jalota, S. K., Choudhury, B. U., and Sharma, P. K. (2007). Yield, evapotranspiration and water productivity of rice (Oryza sativa L.)–wheat (Triticum aestivum L.) system in Punjab (India) as influenced by transplanting date of rice and weather parameters. Agric. Water Manag. 88, 14–22. doi: 10.1016/j.agwat.2006.08.007
Chang, J.-H. (1963). The role of climatology in the Hawaiian sugarcane industry: an example of applied agricultural climatology in the tropics. Pacific Sci. 17, 379–397.
Chow, V. T. (1964). Handbook of applied hydrology. New York, NY, McGraw Hill Book Co Department of Agriculture, Govt. of Bihar.
De Vries, M. E., Rodenburg, J., Bado, B. V., Sow, A., Leffelaar, P. A., and Giller, K. E. (2010). Rice production with less irrigation water is possible in a Sahelian environment. Field Crop Res. 116, 154–164. doi: 10.1016/j.fcr.2009.12.006
Dubey, R., Pathak, H., Singh, S., Chakraborty, B., Thakur, A. K., and Fagodia, R. K. (2019). Impact of sowing dates on terminal heat tolerance of different wheat (Triticum aestivum L.) cultivars. Natl. Acad. Sci. Lett. 42, 445–449. doi: 10.1007/s40009-019-0786-7
Jain, N., Dubey, R., and Dubey, D. S. (2014). Mitigation of greenhouse gas emission with system of rice intensification in the Indo-Gangetic Plains. Paddy Water Environ. 12, 355–363. doi: 10.1007/s10333-013-0390-2
Jalota, S. K., Singh, K. B., Chahal, G. B. S., Gupta, R. K., Chakraborty, S., Sood, A., et al. (2009). Integrated effect of transplanting date, cultivar and irrigation on yield, water saving and water productivity of rice (Oryza sativa L.) in Indian Punjab: field and simulation study. Agric. Water Manage. 96, 1096–1104. doi: 10.1016/j.agwat.2009.02.005
Jat, H. S., Datta, A., Sharma, P. C., Kumar, V., Yadav, A. K., Choudhary, M., et al. (2018). Assessing soil properties and nutrient availability under conservation agriculture practices in a reclaimed sodic soil in cereal-based systems of north-West India. Arch. Agron. Soil Sci. 64, 531–545. doi: 10.1080/03650340.2017.1359415
Kato, Y., Okami, M., and Katsura, K. (2009). Yield potential and water use efficiency of aerobic rice (Oryza sativa L.) in Japan. Field Crop Res. 113, 328–334. doi: 10.1016/j.fcr.2009.06.010
Kobayashi, K., Matsui, T., Yoshimoto, M., and Hasegawa, T. (2010). Effects of temperature, solar radiation and vaporpressure deficit on flower opening time in rice. Plant Prod. Sci. 13, 21–28. doi: 10.1626/pps.13.21
Kumari, S., Seth, T., Prajapat, K., Harsur, M. M., Reddy, B. R., and Kumar, D. (2019). Case study-participatory rural appraisal: an approach for identification of the problems and solution for the problems. Mutilogic Sci. 16, 118–126.
Kumari, S., Singh, A. K., and Lal, S. P. (2022). Rice varietal preference of farmers in rice bowl region of Bihar: a polychotomous logistic regression analysis. Indian J. Extens. Educ. 58, 48–53. doi: 10.5958/2454-552X.2022.00032.9
Lal, R. (2019). “Adaptation and mitigation of climate change by improving agriculture in India” in Climate change and agriculture in India: impact and adaptation. ed. S. Sheraz Mahdi (Cham: Springer International Publishing), 217–227.
Mann, H. B. (1945). Nonparametric tests against trend. Econometrica 13, 245–259. doi: 10.2307/1907187
Mondal, S., Poonia, S. P., Mishra, J. S., Bhatt, B. P., Karnena, K. R., and Saurabh, K. (2020). Short-term (5 years) impact of conservation agriculture on soil physical properties and organic carbon in a rice–wheat rotation in the Indo-Gangetic Plains of Bihar. Eur. J. Soil Sci. 71, 1076–1089. doi: 10.1111/ejss.12879
Murty, K. S., Nayak, S. K., and Sahu, G. (1975). “Effect of low light stress on rice crop” in Proceedings of the Symposium on Crop Plant Response of Environmental Stresses, Held at VPKAS (Almora: ICAR-Vivekananda Krishi Anusundhan Sansthan), 74–84.
Najmuddin, O., Rasul, G., Hussain, A., Molden, D., Wahid, S., and Debnath, B. (2018). Low water productivity for Rice in Bihar, India—a critical analysis. Water 10:1082. doi: 10.3390/w10081082
Oguntunde, P. G., Abiodun, B. J., Olukunle, O. J., and Olufayoa, A. A. (2011). Trends and variability in pan evaporation and other climatic variables at Ibadan, Nigeria, 1973–2008. Meteorol. Appl. 19, 464–472. doi: 10.1002/met.281
Poudel, P. B., Poudel, M. R., and Puri, R. R. (2021). Evaluation of heat stress tolerance in spring wheat (Triticum aestivum L.) genotypes using stress tolerance indices in western region of Nepal. J. Agric. Food Res. 5:100179. doi: 10.1016/j.jafr.2021.100179
Sandhu, S. S., Prabhjyot, K., Padmakar, T., Patel, S. R., Rajinder, P., Solanki, N. S., et al. (2016). Effect of intraseasonal temperature on wheat at different locations of India: a study using CERES-wheat model. J. Agrometeorol. 18, 222–233. doi: 10.54386/jam.v18i2.939
Sattar, A., Gulab, S., Singh Shruti, V., Mahesh, K., Vijaya Kumar, P., and Bal, S. K. (2020). Evaluating temperature thresholds and optimizing sowing dates of wheat in Bihar. J. Agrometeorol. 22, 158–164. doi: 10.54386/jam.v22i2.156
Sattar, A., Kumar, M., Vijaya Kumar, P., and Khan, S. A. (2017). Crop weather relation in kharif rice for north-west alluvial plain zone of Bihar. J. Agrometorol. 19, 71–74. doi: 10.54386/jam.v19i1.760
Sattar, A., Nanda, G., Singh, G., Jha, R. K., and Bal, S. K. (2023). Responses of phenology, yield attributes, and yield of wheat varieties under different sowing times in IndoGangetic Plains. Front. Plant Sci. 14:1224334. doi: 10.3389/fpls.2023.1224334
Sattar, A., and Srivastava, R. C. (2021). Modelling climate smart rice-wheat production system in the middle Gangetic Plains of India. Theor. Appl. Climatol. 144, 77–91. doi: 10.1007/s00704-020-03497-6
Srivastava, R. C., Singh, N. K., Nilanjaya,, Singh, S. K., Sattar, A., Kumar, V., et al. (2018) Enhancement of rice-wheat productivity through climate smart agriculture. Publication Division, RPCAU, Bihar.
Tuong, T. P., Bouman, B. A. M., and Mortimer, M. (2005). More rice, less water—integrated approaches for increasing water productivity in irrigated rice-based systems in Asia. Plant Prod. Sci. 8, 231–241. doi: 10.1626/pps.8.231
Venkataraman, S., and Krishnan, A. (1992). “Weather relations of crops” in Crops and weather (New Delhi: ICAR), 302–457.
Yadav, R., Tripathi, S. K., Pranuthi, G., and Dubey, S. K. (2014). Trend analysis by Mann-Kendall test for precipitation and temperature for thirteen districts of Uttarakhand. J. Agrometerol. 16, 164–171. doi: 10.54386/jam.v16i2.1507
Keywords: rice–wheat, climate resilience, assured irrigation, risk management, adaptation
Citation: Jha RK, Sattar A, Singh AK, Kundu MS, Tiwari RK, Singh AK, Singh AK, Das S, Pal R, Kushwah S, Kumari AR, Meena M, Singh P, Gupta SK, Shekhar D, Rai SK, Kumar Gangwar S, Rai RK, Prasad RI, Singh AP, Singh RP, Singh PK, Srivastawa PK, Jha BK, Senapati R, Das S, Suman SK, Singh G, Rajak SK, Kumari N, Rai A, Kumar S, Kashyap V, Kumari S, Chhetri KB, Kumar T, Prasad S, Gangwar A, Nalia A, Patra A, Singh R, Ramulu C, Praharaj S, Regar KL, Patel SS, Kumari V, Chauhan L, Harsh BR, Kapil ST, Soren J, Choudhury S, Tamta S, Kumar N and Tiwari DK (2023) Managing climatic risks in rice–wheat cropping system for enhanced productivity in middle Gangetic plains of India. Front. Sustain. Food Syst. 7:1259528. doi: 10.3389/fsufs.2023.1259528
Edited by:
Siemen Van Berkum, Wageningen University and Research, NetherlandsReviewed by:
Ganesh Malik, Palli-Siksha Bhavana Institute of Agriculture, IndiaPintoo Bandopdhyay, Bidhan Chandra Krishi Viswavidyalaya, India
Amnah Alsuhaibani, Princess Nourah Bint Abdulrahman University, Saudi Arabia
Copyright © 2023 Jha, Sattar, Singh, Kundu, Tiwari, Singh, Singh, Das, Pal, Kushwaha, Kumari, Meena, Singh, Gupta, Shekhar, Rai, Kumar Gangwar, Rai, Prasad, Singh, Singh, Singh, Srivastawa, Jha, Senapati, Das, Suman, Singh, Rajak, Kumari, Rai, Kumar, Kashyap, Kumari, Chhetri, Kumar, Prasad, Gangwar, Nalia, Patra, Singh, Ramulu, Praharaj, Regar, Patel, Kumari, Chauhan, R, Kapil, Soren, Choudhury, Tamta, Kumar and Tiwari. This is an open-access article distributed under the terms of the Creative Commons Attribution License (CC BY). The use, distribution or reproduction in other forums is permitted, provided the original author(s) and the copyright owner(s) are credited and that the original publication in this journal is cited, in accordance with accepted academic practice. No use, distribution or reproduction is permitted which does not comply with these terms.
*Correspondence: Abdus Sattar, asattar@rpcau.ac.in