- 1Department of Livestock and Pasture Science, University of Fort Hare, Alice, South Africa
- 2Department of Agronomy, University of Fort Hare, Alice, South Africa
- 3Department of Animal Production, Agribusiness and Economics, University of Namibia, Neudamm Campus, Windhoek, Namibia
Smallholder livestock production in the Eastern Cape Province of South Africa has been hampered by a lack of high-quality feed. As such, improving ruminant nutrition using optimized production of herbaceous forage legumes has been suggested. However, the production of forage legumes for livestock feed is poor in communal areas in the province due to the necessity to use high levels of chemical fertilizers to provide high-quality feed that meets animal nutrient demands. The current study aimed at investigating the effects of arbuscular mycorrhizal fungi (AMF) and Rhizobium inoculation on the chemical composition of three herbaceous forage legumes, namely, Vigna unguiculata (cowpea), Lablab purpureus (lablab), and Mucuna pruriens (mucuna). The field trials were established over two growing seasons (2017/2018 and 2018/2019) at the University of Fort Hare Research Farm (South Africa) and were carried out as a 3 x 2 x 2 factorial experiment arranged in a randomized complete block design (RCBD) with 12 treatments that were replicated 4 times. The 12 treatments included three legume species (cowpea, lablab, and mucuna), two AMF (with and without AMF inoculation), and two Rhizobia inoculations (with or without). The results showed that the dual inoculation of AMF and Rhizobia bacteria significantly (p < 0.05) increased the contents of crude protein, neutral detergent fiber and acid detergent fiber, hemicellulose, ash, magnesium (Mg), phosphorus (P), calcium (Ca), zinc (Zn), and iron (Fe) of three herbaceous forage legumes. Furthermore, solo inoculation with AMF or Rhizobia bacteria resulted in substantially higher potassium (K), Ca, and Mg cation ions, and Ca/P, Zn, manganese (Mn), and Fe concentrations in forage legumes than in other treatments. In conclusion, the dual inoculation produced optimal nutrient accumulation in both growing seasons when compared to single inoculation and uninoculated forages. Therefore, this could assist in alleviating livestock malnutrition in smallholder farming.
1. Introduction
Agriculture contributes significantly to the quality of life among rural communities in South Africa since livestock and crop production are their main sources of income (Mabapa et al., 2017). Farmers raise livestock and rely mostly on natural grazing land due to their lack of ability to purchase feed supplements or cultivate fodder crops under intensive management methods where water and fertilizer inputs are prohibitively expensive (Mabapa et al., 2017). Nevertheless, climate change poses an imminent threat to the availability of food and feed, with some smallholder farmers in South Africa losing livestock due to a lack of fodder (Rankoana, 2016).
Smallholder livestock production in the Eastern Cape Province of South Africa has been significantly hampered by a lack of high-quality feed (Dziba et al., 2003). During the prolonged dry season, rangeland grasses have low crude protein (CP) (<7%) and a high crude fiber content, which increases greenhouse gas emissions (Lamidi et al., 2005). Livestock experience malnutrition on an ongoing basis, and this leads to low productivity, susceptibility to diseases (to some extent, mortality), and subsequently economic losses for farmers (Amole et al., 2013). As a standard, good quality fodder and feed contain high digestibility, high non-structural carbohydrates, high CP, moderate tannins, high palatability, high sulfur amino acids, suitable minerals, and a low anti-nutritional factor (Krämer-Schmid et al., 2016; Wilkins, 2018; Chand et al., 2022).
Several studies have been conducted to improve ruminant nutrition using optimized production of herbaceous forage legumes. Herbaceous forage legumes such as Lablab purpureus (lablab), Vigna unguiculata L. Walp. (cowpea), and Mucuna pruriens (mucuna) are underutilized, and these legumes have a high fodder yield as well as a high CP content (18–38%) (Lamidi et al., 2005). However, production of these forage legumes for livestock feed is poor in communal areas in the Eastern Cape Province (Dziba et al., 2003). This is because the production of these forages necessitates the application of a high level of chemical fertilizers to provide high-quality feed that meets animal nutrient demands (Halder et al., 2015; Duan et al., 2016). Farmers have often and widely used chemical fertilizers to enhance crop productivity due to low intrinsic fertility (Bhattacharyya et al., 2020). However, the excessive usage of these chemical fertilizers, on the other hand, has been linked to groundwater pollution, high soil acidity, and degradation of the land (Umesha et al., 2018; Bisht and Chauhan, 2020; Mokabel et al., 2022; Nacoon et al., 2023). The adverse effects of long-term usage of synthetic fertilizers, such as weed infestation, opportunistic diseases, and declining yield as well as quality, have been reported on the production of many crops. Furthermore, their exorbitant costs (Sharma and Singhvi, 2017) warrant the urgent use of low-cost, environmentally friendly, and safe natural phytonutrient alternatives.
Farmers in the smallholder system are currently under pressure to enhance crop productivity and quality with fewer agricultural inputs while at the same time reducing greenhouse gas emissions and safeguarding the environment (Benaffari et al., 2022). Indeed, innovative alternatives are urgently needed to increase crop productivity and minimize overdependency on chemical fertilizers (Meddich et al., 2018). Among these alternatives, the use of biostimulants such as arbuscular mycorrhizal fungi (AMF) and Rhizobia has been recognized as a potential approach in farming practices for improving soil fertility, crop production, and crop tolerance to harsh environmental circumstances (Anli et al., 2020; Bartucca et al., 2022). AMF have an obligate symbiotic relationship with most plant species (Ingraffia et al., 2020; Andersone-Ozola et al., 2021). Rhizobia, on the other hand, are root-nodulating bacteria that fix nitrogen (N) and interact mostly with legumes (Amole et al., 2013; Schwab et al., in press). This symbiosis begins when legume roots and compatible Rhizobia exchange signals in the soil, resulting in rhizobia being enclosed by plant cells to create a root nodule (Sachs et al., 2018). In exchange for photosynthate, microorganisms within the nodule fix atmospheric nitrogen for the plant (White et al., 2007).
The dual inoculation with both AMF and Rhizobia bacteria results in a tripartite mutualistic symbiosis and stimulates nodulation as well as plant development (Chalk et al., 2006; Halder et al., 2015). This tripartite symbiosis of legumes, AMF, and Rhizobia bacteria has been reported to benefit legumes with phosphorus (P) and N uptake (Kawaguchi and Minamisawa, 2010; Haruna and Usman, 2013; Püschel et al., 2017). The dual inoculation of AMF and Rhizobium has the potential to significantly reduce the number of synthetic fertilizers required while also enhancing crop growth by improving soil aeration and root accessibility to water and soil nutrients (Berruti et al., 2016; Konvalinková et al., 2017; Anli et al., 2020). For example, several studies demonstrated that the dual inoculation of AMF and Rhizobia improved the growth and productivity of legumes such as Phaseolus vulgaris (Mortimer et al., 2012), Vicia faba (Abd-Alla et al., 2014), Medicago sativa (alfalfa) (Ben-Laouane et al., 2019), and dry beans (Moila et al., 2020). Dual inoculation also enhanced the nodule number and mycorrhizal infection under normal and stressed conditions (Ben-Laouane et al., 2019). In the semi-arid region of Morocco where the issue of land degradation reduced crop production, Ben-Laouane et al. (2021) demonstrated that combining AMF and Rhizobium inoculation had the highest positive effect on dry matter production, the number of leaves and nodules of alfalfa, and the rate of AMF infection. In contrast, positive effects of the AMF and/or Rhizobium inoculation on growth and productivity were also reported on non-legume plants such as tomatoes (Ait Rahou et al., 2020; Soussani et al., 2023), Arizona cypress (Aalipour et al., 2020), rice (Mbodj et al., 2018), and wheat (Varinderpal-Singh et al., 2019).
In addition to these advantages, dual inoculation has been shown to increase the chemical composition of chickpea and cowpea leaves, including CP, calcium (Ca), P, and magnesium (Mg) (Farzaneh et al., 2009; Yaseen et al., 2011). These combinations also improved stomatal conductance, photosystem II efficiency, photosynthetic pigments (chlorophyll and carotenoids), protein and sugar contents, and nutrient uptake [e.g., P, N, potassium (K), and Ca] by alfalfa. On the other hand, some studies showed that single inoculation of Rhizobium species enhanced the growth and yield parameters of legumes such as mung bean (Zahir et al., 2010; Qureshi et al., 2023), common bean (Korir et al., 2017), white clover (Trifolium repens) (Dumsane et al., 2020), and chickpea (Hemissi et al., 2011; Benjelloun et al., 2021) when compared to the uninoculated control.
The open-field experiments carried out in the current study were the same as those published in Mpongwana et al. (2023). In brief, it was shown that the dual inoculation of AMF and Rhizobia bacteria improved plant growth parameters, biomass, and yield components of three herbaceous forage legumes (lablab, cowpea, and mucuna). It was observed that dual inoculation of AMF and Rhizobia in both seasons significantly improved the nodulation characteristics (nodulation numbers, nodulation rate, and active nodule number) of three forage legumes used in both seasons. Similar results of the effect of AMF and Rhizobia inoculation were observed on the nodule numbers of various legumes such as cowpea (Yaseen et al., 2011) and groundnut (Ramakrishnan and Lenin, 2010). The variation in nodule number per plant can be caused by several factors, including species genotypes and their ability to establish symbiosis between legume species and Rhizobium (Rajendran et al., 2008).
However, little is known about the effect of AMF and Rhizobia on the chemical composition of these forage legumes. This is because forage nutritional quality features have been overlooked and are not regarded as an economic determinant factor in the same way that forage biomass is (Battenfield et al., 2016; Chand et al., 2022). Therefore, the current study investigated the effects of inoculating AMF and Rhizobia bacteria on the chemical and nutritional composition of three herbaceous forage legumes (lablab, cowpea, and mucuna). It is hypothesized that the chemical composition of these three forage legumes could vary due to dual inoculation with AMF and Rhizobium.
2. Materials and methods
2.1. Study site
The research was carried out at the University of Fort Hare Research Farm (Eastern Cape Province, South Africa) during the growing summer seasons of 2017/18 and 2018/2019. The farm is located at latitude 32°46′ S and longitude 26°50′ E at an elevation of approximately 535 m above sea level (m.a.s.l.). It has an average annual rainfall of about 575 mm, most of which falls during the summer (November–March). The highest and minimum temperatures are 24.6 and 11.1°C, respectively, with an average temperature of 17.8°C (Mpongwana et al., 2023). The land was fallow for 4 years before planting in 2017 after having previously been used for forage maize production. According to the International Union of Soil Sciences (IUSS) Working Group World Reference Base (2022), the soil at the farm is alluvial in origin and is classified as Eutric Cambisols (ochric). Further details on the soil physicochemical properties of the experimental site before the commencement of a field trial are presented in a study by Mpongwana et al. (2023).
2.2. Research design and treatments
The study was carried out as a 3 x 2 x 2 factorial experiment arranged in a randomized complete block design (RCBD) with 12 treatments that were replicated four times. The field area was 59 x 22 m, and measurements were gathered from 48 plots, each measuring 4 × 4 m. The plots and blocks were separated by 1 and 2 m, respectively. The treatments comprised three forage legumes [cowpea (black-eyed pea cultivar), lablab (Rongai), and mucuna (Utilis)]; two AMF (with and without inoculation); and two Rhizobium (with or without inoculation).
Before planting, forage legume seeds were treated with MycorootTM products comprising a combination of arbuscular mycorrhizal isolates such as Rhizophagus clarus, Gigaspora gigantean, Funneliformis mosseae, Claroideoglomus etunicatum, and Paraglomus occulum. It was applied on the planting holes when sowing forage legume seeds. The product comprised 10 spores per gram and was subjected to regular quality tests at the South African Rhodes University production facility (Dames and Ridsdale, 2012). The rates for inoculating seeds with MycorootTM products were calculated using the optimal recommended rate of 45 kg P ha−1 (Moila et al., 2020). A night before sowing, the seeds were also treated with a stain powder mixture of Rhizobium inoculum (Bradyrhizobium japonicum strain), which was obtained from Soygro (Pty) Ltd. (Potchefstroom, South Africa).
The plots were fertilized with a single superphosphate rate of 40 kg P ha−1 with 10.5% phosphate (0:46:0%; N: P2O5:K2O). The forage legumes were planted at a depth of 4–6 cm with an inter- and intra-row spacing of 0.9 x 0.3 m and a seeding rate of 50 kg.ha−1, making a plant density of 37 037 plants ha−1. The experimental field was cleared in preparation for the second planting season, and the residues were removed following harvesting. This was followed by plowing the land, which was then left fallow for a while to allow weeds to develop. The fields were plowed with a ripper and disked 2 weeks before the initiation of the second trial (see Mpongwana et al., 2023).
2.3. Forage chemical composition analysis
The harvested forage (at 120 days) was measured from each plot, and fresh weights were oven-dried at 60°C for 48 h to obtain dry mass. The samples were then ground using a Wiley mill to pass through a 1-mm sieve screen for chemical analysis and kept in plastic bags at room temperature before laboratory analysis (Matizha et al., 2001). The dry matter content (DMC) was determined by oven drying at 105°C for 24 h [Association of Official Analytical Chemists (AOAC), 2005]. The samples were analyzed for CP (N% x 6.25) and ash content [Association of Official Analytical Chemists (AOAC), 2005]. Ether extract (EE) was determined by extraction with anhydrous ether using a Soxhlet apparatus [Association of Official Analytical Chemists (AOAC), 2005]. Neutral detergent fiber (NDF) and acid detergent fiber (ADF) were determined using fiber bags and fiber analyzer equipment (Fiber Analyzer, Ankom Technology, Macedon, NY, USA) following an adaptation procedure described by Van Soest et al. (1991). The NDIN was determined by measuring the N in NDF residues, as described by Van Soest et al. (1991). The acid detergent insoluble N (ADIN) was also measured in accordance with Van Soest et al. (1991). The non-structural carbohydrates (NSCs) were calculated using the following equation: [100 - (%NDF + %CP + %Fat + Ash)] [Association of Official Analytical Chemists (AOAC), 2005].
Hemicellulose was calculated using the following equation: hemicellulose = NDF - ADF; while cellulose was determined through this equation: cellulose = ADL - ADF. Nevertheless, the samples for macro- and microelements were digested by nitric and chloric acid mixtures (ratio = 4.1 v/v). The concentrations of minerals such as Ca, K, P, Mg, zinc (Zn), sodium (Na), copper (Cu), iron (Fe), and manganese (Mn) in the samples were determined by an atomic absorption spectrophotometer procedure using the methods described by Van Soest et al. (1991).
2.4. Statistical analysis
Data were analyzed using analysis of variance (ANOVA) by the general linear model procedure of SAS (SAS., 2012) version 9.4. Means were separated using the least significant difference (LSD) at a 5% probability level. The following statistical model was used: Yijkl = μ + Bh + Li + Mj + Rk + (LM)ij + (LR)ik + (MR)jk + (LMR)ijk + eijkl, where: Yijkl = is the dependent variable (e.g., chemical nutrient composition); μ = overall mean; Bh = hth block effect (h = 1, 2, 3, 4); Li = ith effect of legume species (i = 1, 2, 3); Mj = jth effect of AMF (j = 1, 2); P = effect of Rhizobium inoculum (k = 1, 2); LMij = ijth interaction between legume species and AMF; LRik = ikth interaction between legume species and Rhizobium inoculum; MRjk = jkth interaction between AMF and Rhizobium inoculum; LMRikj = ijkth interaction effect of legume species, AMF, and Rhizobium inoculum; eijkl = residual error.
3. Results
3.1. Effect of treatment factors on dry matter, ash, crude protein, NDIN ADIN, and EE contents of three herbaceous legumes
The results showed that there was no significant effect (P > 0.05) of treatment interaction factors on the DMC of forage in both seasons (Table 1). However, single inoculations of legume species and AMF had a significant effect on the DMC at P ≤ 0.001 and P ≤ 0.05 levels, respectively, in the first season. Only legume species showed a significant effect (P ≤ 0.001) in the second season on the measured parameter. The treatment factors showed a significant two-way interaction effect on forage ash content in both seasons (Table 1). In both seasons, ash content was significantly affected (P ≤ 0.001) by sole legume species, AMF, and Rhizobia bacteria and interactions between legume species and AMF, and legume species and Rhizobia bacteria. The highest ash content was observed on lablab control (LC) in the first season, followed by the interaction of lablab with AMF, and the lowest ash content was observed under the treatment of cowpea + AMF + Rhizobia bacteria (CAR). However, there was no significant three-way interaction (P > 0.05) on legume, AMF, or Rhizobia bacteria. The CP exhibited a three-way interaction (P ≤ 0.01) as affected by legume species, AMF, and Rhizobia bacteria and their interactions in both seasons. The cowpea and its combination treatments produced the highest CP in both seasons when compared to other legume types (Table 1).
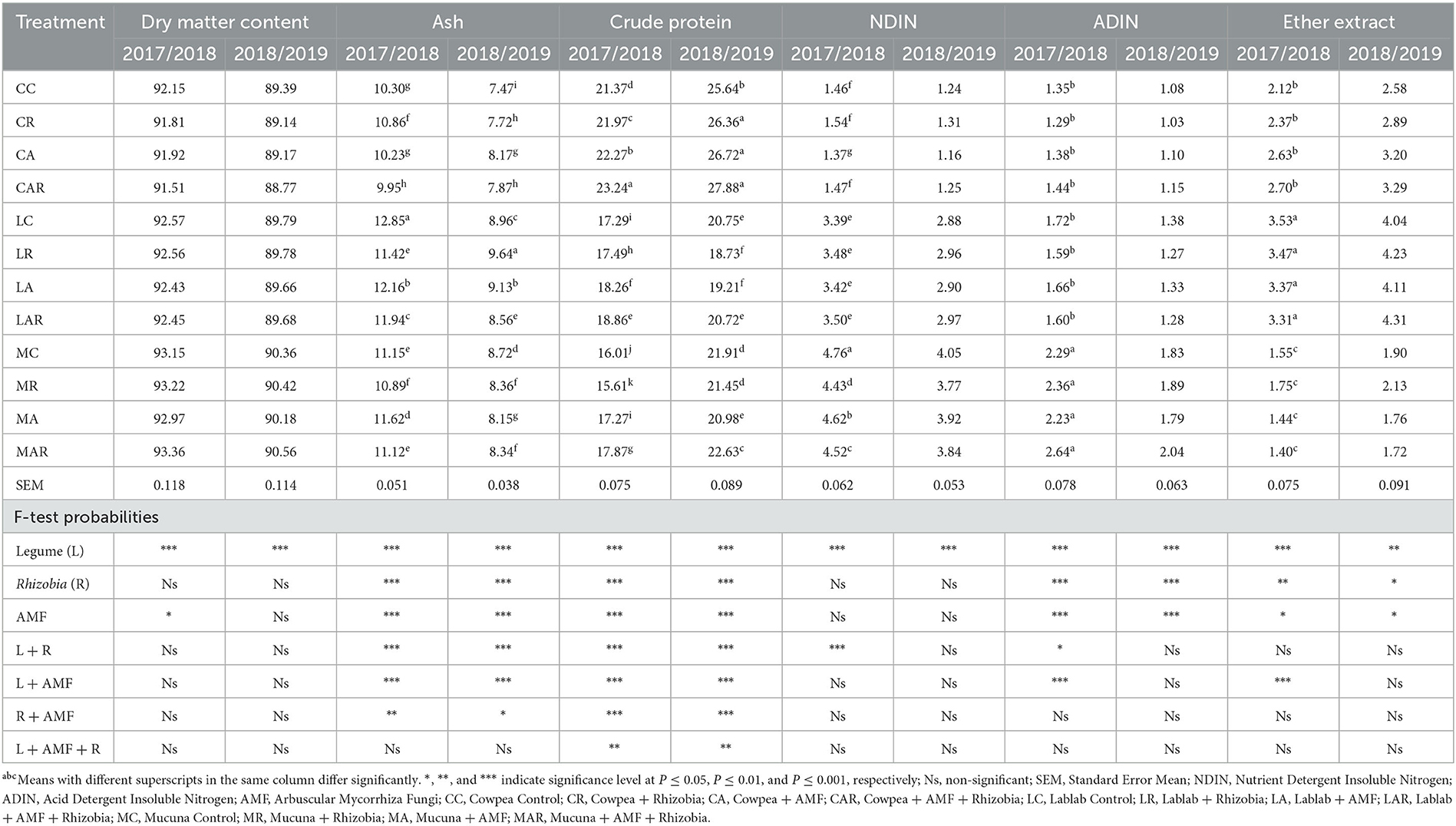
Table 1. The effects of AMF and Rhizobium on dry matter, ash, crude protein, NDIN, ADIN, and ether extract of three herbaceous forage legumes (% DM).
There was a two-way interaction in the first season for treatment factors such as legume species, Rhizobia bacteria, AMF, and the interaction of legume and Rhizobia bacteria, as well as legume species and AMF for the ADIN content (Table 2). Mucuna control (MC) and its interaction with AMF and Rhizobia bacteria produced higher ADIN content than other treatments in both seasons; however, significant differences between treatments were only observed in the first season. The NDIN content was influenced by legume species and the interaction of legume and Rhizobia bacteria in a two-way interaction (P ≤ 0.001) in the first season, with mucuna forage having the highest values as compared to other treatments. However, there was no significant effect (P > 0.05) of treatment factors on NDIN content in the second season.
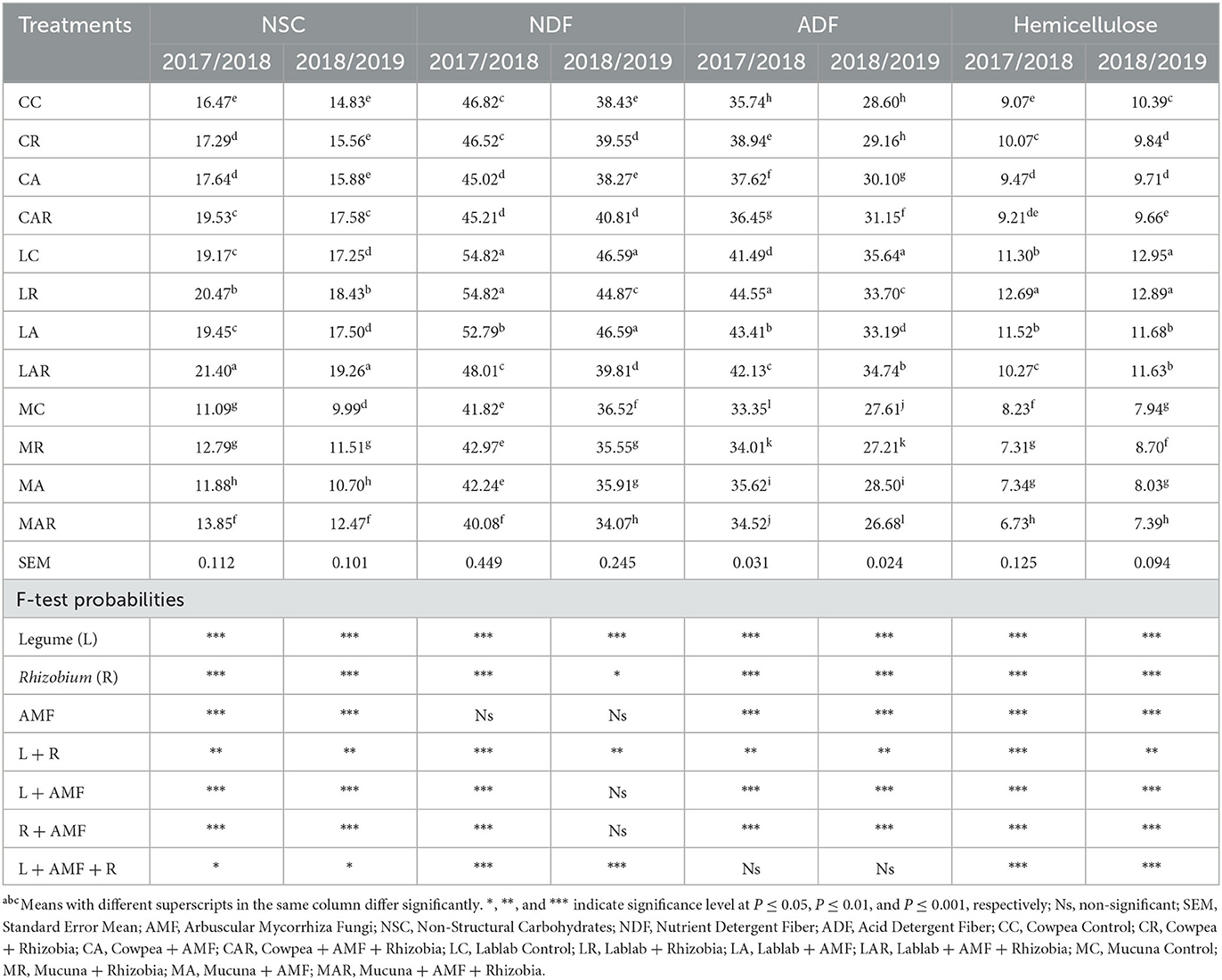
Table 2. The effects of legume variety, AMF, and Rhizobium on acid detergent insoluble nitrogen, nutrient detergent insoluble nitrogen, non-structural carbohydrates, nutrient detergent fiber, acid detergent fiber contents, and hemicellulose of three herbaceous legumes (% DM).
The results presented in Table 1 further show that the EE content was noticeably influenced (P ≤ 0.05) by sole legume species, AMF, and Rhizobia bacteria. The differences in legume species had a remarkable influence over AMF or Rhizobia bacteria and interaction in the first season for the EE. All interactions did not significantly differ in both seasons, except for legume species and AMF interactions in the first season. The lablab treatments and their interactions with AMF and Rhizobia bacteria produced significantly higher EE than other treatments in the first season. Although there was no significant difference (P > 0.05) observed among treatments for EE in the second season, there was a slightly higher EE found on LC and its interaction treatments.
3.2. Effect of treatments on NSC, NDF, ADF, and hemicellulose contents of three legumes
Non-structural carbohydrates (NSCs), NDF, and hemicellulose content were significantly (P ≤ 0.05) affected by the legume species, Rhizobia bacteria, and AMF and their three-way interaction in both seasons. The highest values of NSC, NDF, and hemicellulose were observed on all treatments of lablab in both seasons, whereas the lowest values were recorded on all treatments comprising mucuna forage. On the other hand, ADF content was significantly affected by legume species, AMF, and Rhizobia bacteria, and two-way interactions between legume species and Rhizobia bacteria; legume species and AMF; as well as Rhizobia bacteria and AMF in both seasons. The highest ADF content was recorded on LC and its combination treatments in both seasons (Table 2).
3.3. Correlation matrix of chemical composition in two seasons for three herbaceous legumes
In the first season, DMC was negatively correlated (P ≤ 0.001) with CP, EE, and NSC, while positively correlated (P ≤ 0.001) with NDIN, NDF, ADF, and hemicellulose (Table 3). The ash content was positively correlated (P ≤ 0.001) with EE, NDIN, and NSC, while negatively correlated to hemicellulose content. The EE content was negatively (P ≤ 0.001) correlated with NDIN, NDF, ADF, and hemicellulose, and positively correlated with ADIN and NSC in both seasons. In the second season, significant positive correlations (P ≤ 0.01) were observed between DMC and NDF, ADF, hemicellulose, and NDIN contents, whereas DMC was negatively correlated to CP, EE, and NSC contents. Ash and CP contents had a positive correlation (P ≤ 0.01) with EE, NDIN, and NSC, while ash and CP were negatively correlated with ADF and hemicellulose. There was a positive correlation between ADF and hemicellulose as well as NDIN and a negative correlation between NSC and ADF. A similar trend was also observed for NDF (Table 3).
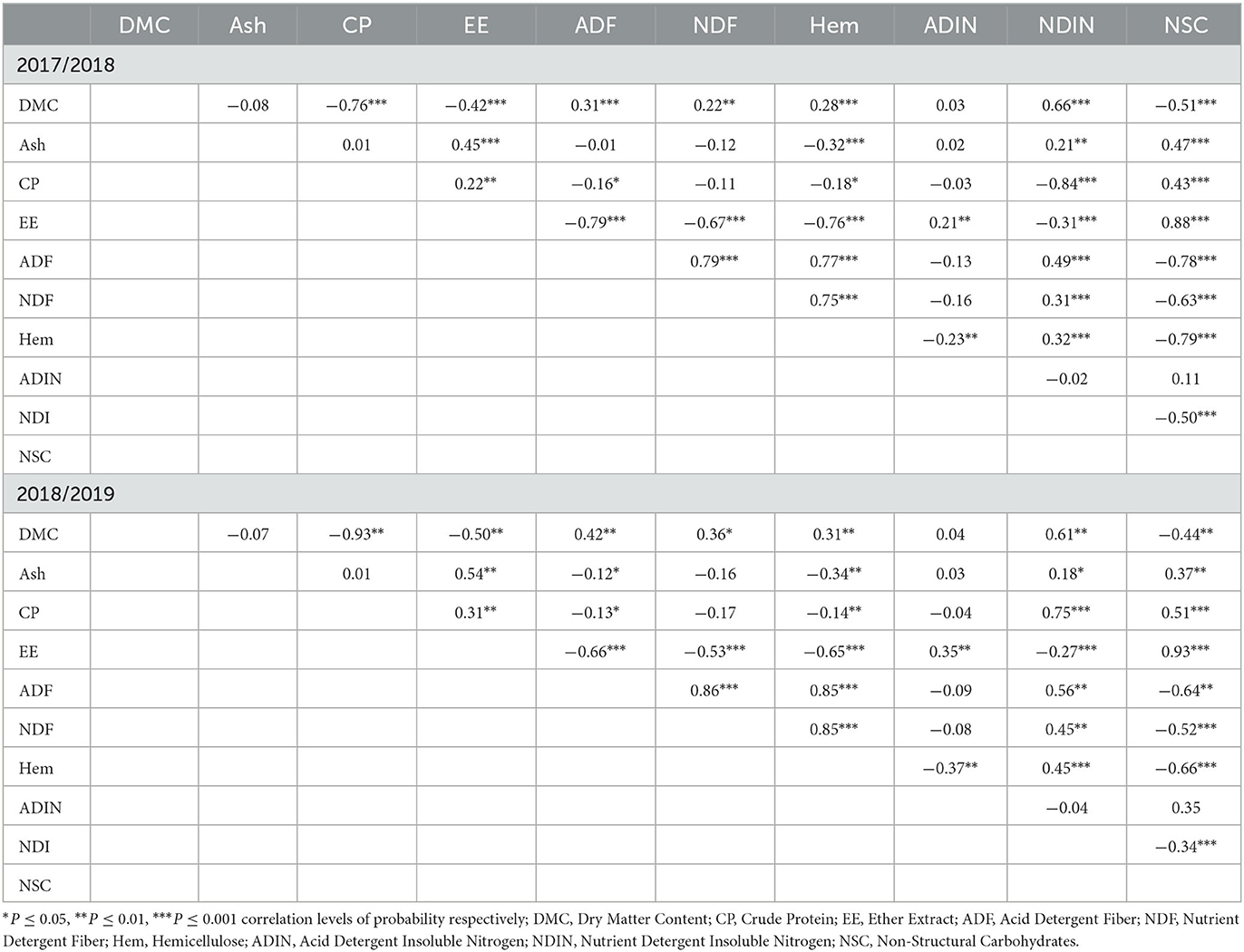
Table 3. The mean correlation coefficients analysis among chemical composition constituents in the 2017/2018 and 2018/2019 seasons.
3.4. Effect of treatment factors on macronutrient contents of three herbaceous legumes
There were significant differences (P ≤ 0.01) in individual treatment factors such as legume species, AMF, Rhizobia bacteria, and the two-way interaction of legume species and AMF on Ca and P contents in both seasons (Table 4). Sole inoculation with AMF significantly (P ≤ 0.001) increased the Ca content of forage legumes, whereas sole inoculations of AMF and Rhizobia bacteria improved the P content of legumes. The treatments of lablab + AMF (LA) and lablab + AMF + Rhizobium (LAR) produced higher contents of Ca and P than other treatments in both seasons, while low contents of both mineral nutrients were observed on MC and mucuna + Rhizobia (MR) treatments. In both seasons, however, there were no significant three-way interactions (P > 0.05) between legumes, AMF, and Rhizobia and either Ca or P content. In general, the second season had a higher Ca or P content compared with the first season.
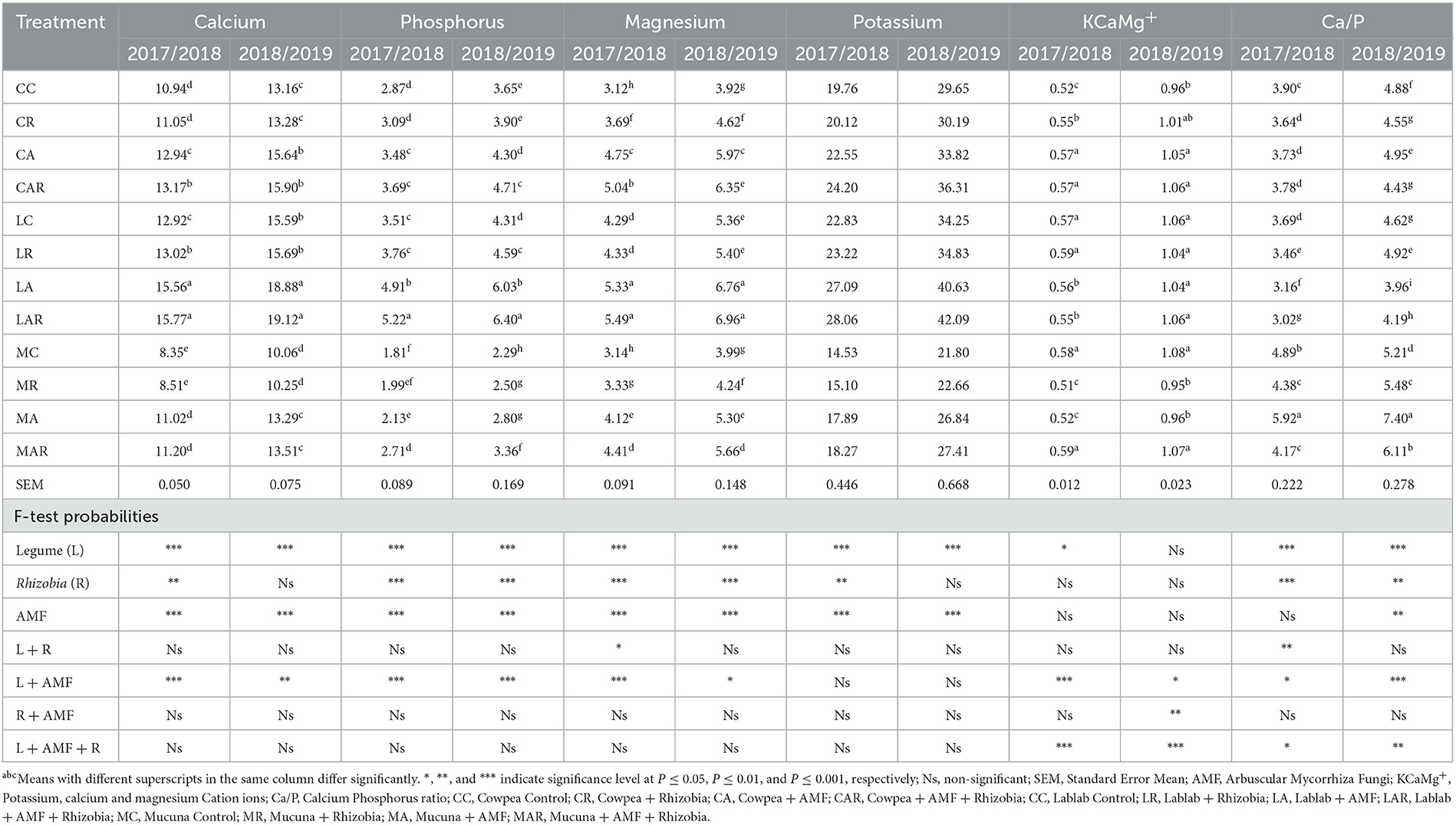
Table 4. The effects of legume variety, AMF, and Rhizobium macronutrient of three herbaceous legumes (% DM).
Magnesium content was significantly (P ≤ 0.001) affected in a two-way interaction by legume species, AMF, and Rhizobia bacteria in the first season. Dual inoculation with AMF and Rhizobia as well as sole inoculation with AMF were mostly effective in increasing the Mg content in forages in both seasons. The optimal Mg content was recorded on LAR and LA treatments in both seasons. On the other hand, different treatment factors and their interactions showed no significant (P > 0.05) difference in affecting the P content in both seasons (Table 4). However, in the first season, legume species, AMF, and Rhizobia as sole treatments significantly affected the P content. In the second season, only the treatment factors of legume species and AMF significantly affected the P content. Although the treatments did not significantly affect P content, slightly higher values of P were observed on lablab forage.
3.5. Effect of treatment factors on KCaMg+ ions and Ca/P ratio of three herbaceous legumes
The results in Table 4 reveal that K, Ca, and Mg cation (KCaMg+) ions were significantly influenced by legume species (P ≤ 0.05) and the interactions (P ≤ 0.001) with legume species, AMF, and legume species, as well as AMF and Rhizobia bacteria in the first season. Sole inoculation showed no significant influence on cation ion content, while dual inoculation with AMF and Rhizobia bacteria significantly improved cation ion content. Cation ion content was higher under mucuna + AMF + Rhizobia (MAR) and lablab + Rhizobia (LR) treatments, which were similar (P > 0.05) to cowpea + AMF (CA), CAR, and LC. In the second season, a significant three-way interaction effect on KCaMg+ ions was also observed between legumes and AMF (P ≤ 0.05); AMF and Rhizobia (P ≤ 0.01); and legume species, AMF, and Rhizobia bacteria (P ≤ 0.001).
The results show that in the first season, Ca/P ratio content was significantly influenced by legume species and Rhizobia bacteria (P ≤ 0.001) and the interactions of legume species and Rhizobia bacteria (P ≤ 0.01); legume species and AMF (P ≤ 0.05); and legume species, AMF, and Rhizobia bacteria (P ≤ 0.05). In the second season, legume species, AMF, and Rhizobia bacteria and their interactions showed a significant (P ≤ 0.01) effect on Ca/P ratio content. Compared to other legume treatments, the highest Ca/P ratio was recorded on MC and its interaction treatments in both seasons (Table 4).
3.6. Effect of treatment factors on the composition of micronutrients of three herbaceous legumes
Table 5 shows that there was no significant (P > 0.05) difference observed for the treatment factors and their interactions with the Na content of the forages in both seasons. Although there were no significant differences between treatments, the highest values of Na were recorded on MC and its interactive treatments. A significant two-way interaction effect (P ≤ 0.05) of legume species and their interactions with AMF and Rhizobia bacteria was observed on Zn content in both seasons. The MC as well as its interaction treatments produced higher Zn content than all other treatments in both seasons, while the lowest values were observed on cowpea treatments.
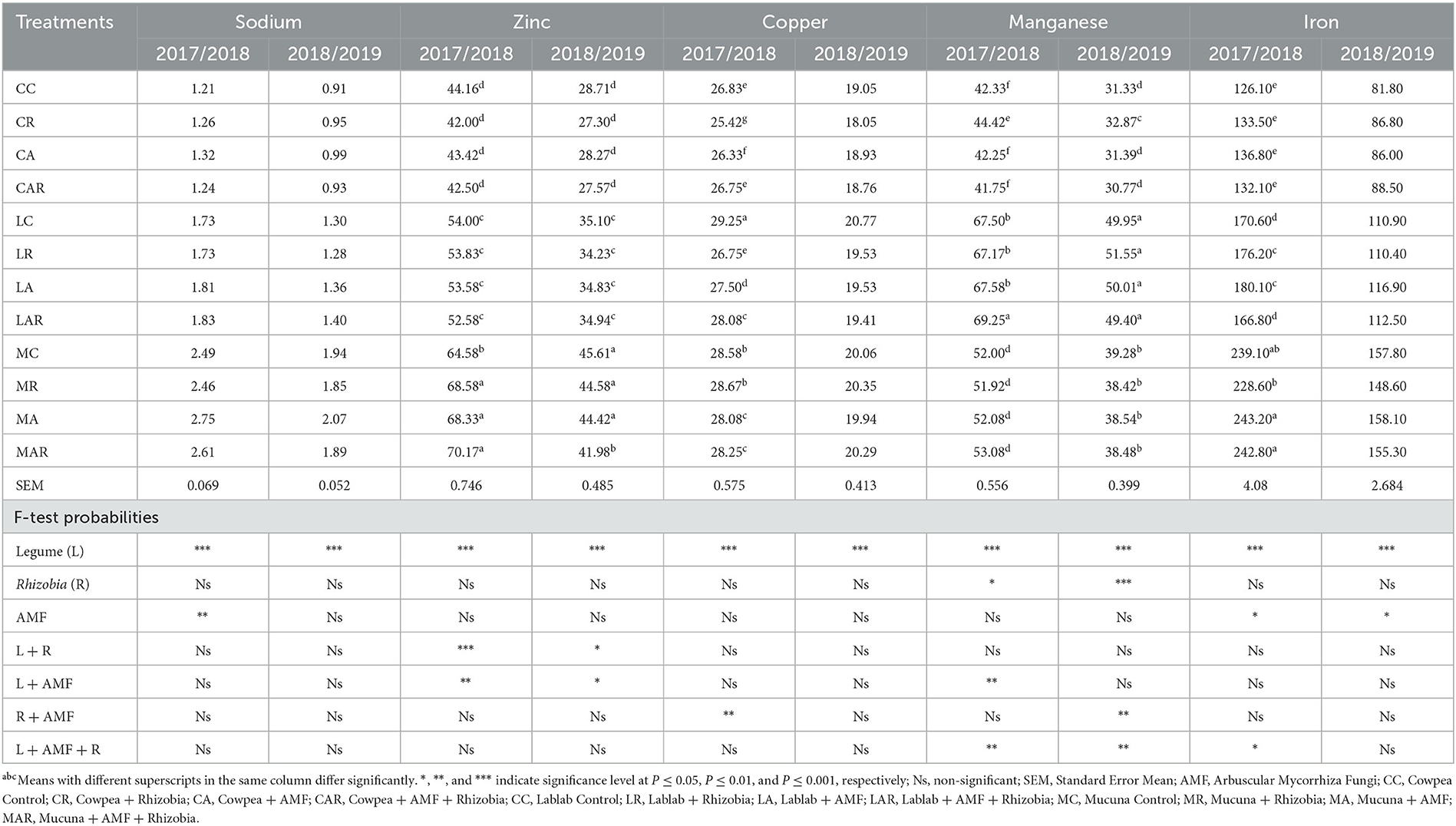
Table 5. The effects of legume variety, AMF, and Rhizobium on micronutrients of three herbaceous legumes (% DM).
The Cu content was greatly influenced (P ≤ 0.001) by legume species in both seasons. However, the Cu content was not influenced (P > 0.05) by single inoculations or interactions in both seasons, except for the interaction of AMF and Rhizobia bacteria in the first season (Table 5). In both seasons, there was a significant (P ≤ 0.05) three-way interaction observed for treatment factors such as legume species and Rhizobia bacteria as well as legume species, AMF, and Rhizobia bacteria on Mn content. Furthermore, there were significant two-way interactions (P ≤ 0.01) between legume species and AMF in the first season, while significant interactions between AMF and Rhizobia bacteria (P ≤ 0.01) were observed in the second season (Table 5). Sole inoculation with Rhizobia bacteria and dual inoculation with AMF and Rhizobia bacteria enhanced the Mn content in forages. The highest values of Mn were recorded in LC and its interactions with AMF and Rhizobia bacteria in both seasons as compared to other legume treatments.
On the other hand, the results in Table 5 further indicate that the Fe content was considerably influenced by sole legume species and AMF in both seasons. However, there was a three-way interaction (P ≤ 0.05) between legume species, AMF, and Rhizobia bacteria only in the first season. In both seasons, MC and its treatment interactions had a higher Fe content than all other legume treatments. In addition, the mucuna + AMF (MA) treatment significantly (P ≤ 0.05) had the greatest Fe content, although this was not statistically different from other mucuna treatments. However, the lowest values of Fe were recorded on cowpea-related treatments.
3.7. Correlation matrix of macro- and micronutrient composition in two growing seasons
A correlation matrix analysis was made to test the relationship between macro- and micronutrient composition in both seasons (Table 6). In the first season, Ca and P were positively correlated (P ≤ 0.001) with Mg (R2 = 0.65, 0.58), K (R2 = 0.75, 0.68), and Mn (R2 = 0.18, 0.18), and negatively correlated (P ≤ 0.001) with Na (R2 = 0.08, 0.11), Ca/P (R2 = 0.25, 0.43), Zn (R2 = 0.13, 0.15), and Fe (R2 = 0.09, 0.13), respectively. A significant positive correlation (P ≤ 0.001) was observed between Mg and K (R2 = 0.70), and Mn (R2 = 0.07), and negatively correlated with Ca/P (R2 = 0.16, P ≤ 0.001) and Zn (R2 = 0.05, P ≤ 0.05). The negative correlation was also detected between K and Na (R2 = 0.05, P ≤ 0.01), Ca/P (R2 = 0.25, P ≤ 0.001), and Zn (R2 = 0.17, P ≤ 0.001), as well as Fe (R2 = 0.0.9, P ≤ 0.001), and positively correlated with Mn (R2 = 0.15, P ≤ 0.001). However, there was a positive correlation between Na and Ca/P, Zn, Cu, Mn, and Fe, while KCaMg+ was only positively correlated (P ≤ 0.05) with Mn. In addition, Zn, Cu, Mn, and Fe were significantly correlated with each other, while Mn was only negatively correlated with Ca/P.
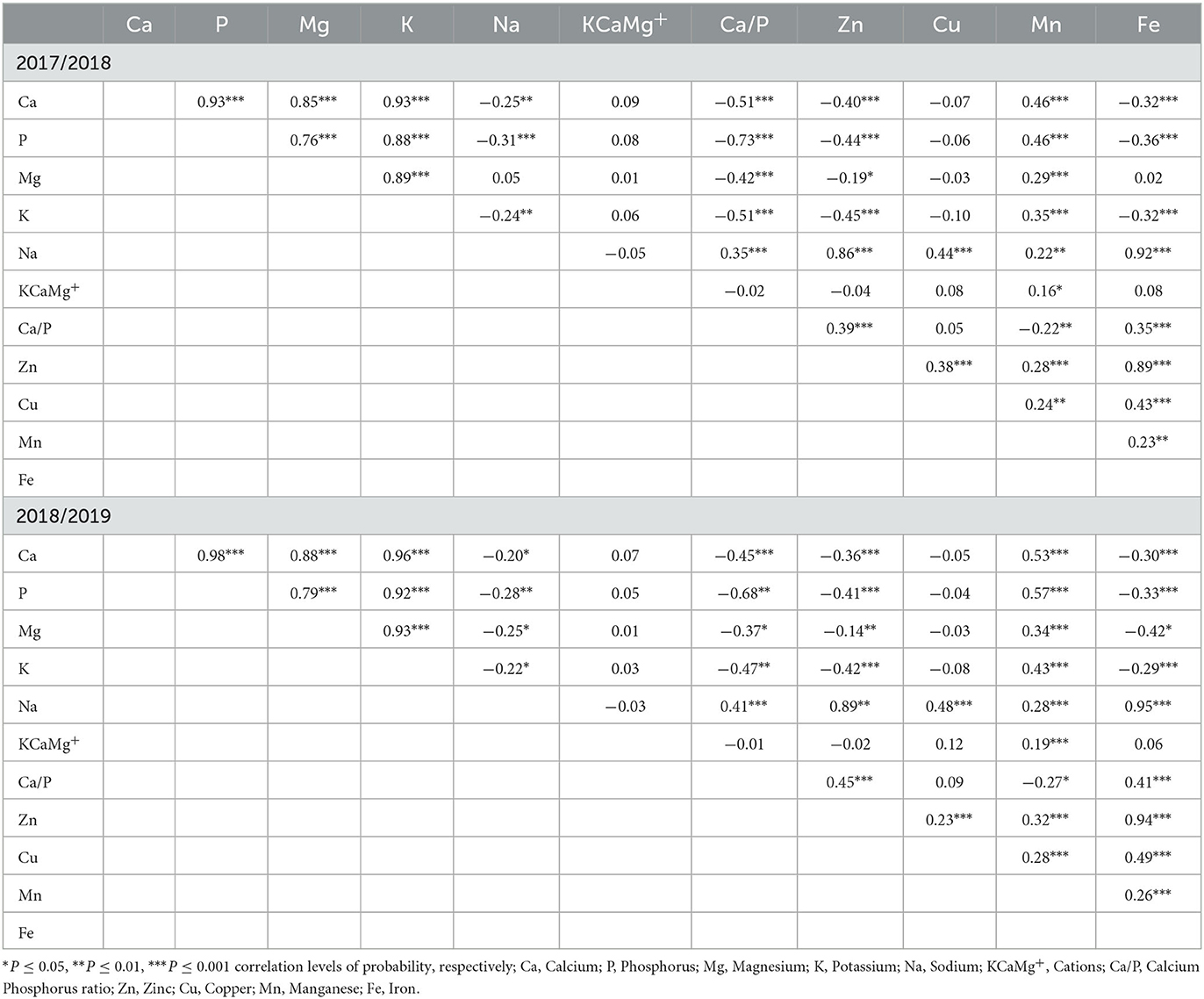
Table 6. The mean correlation analysis among nutrient composition constituents in the 2017/2018 and 2018/2019 growing seasons.
In the second season, Ca, P, Mg, and K were positively correlated with each other. Meanwhile, Ca, P, Mg, and K were negatively correlated with Na, Ca/P, Zn, and Fe. Although they were negatively correlated with various nutrient elements, these components were positively correlated with Mn. On the other hand, Na was positively correlated with Ca/P (R2 = 0.15, P ≤ 0.001), Zn (R2 = 0.61, P ≤ 0.001), Cu (R2 = 0.21, P ≤ 0.001), Mn (R2 = 0.08, P ≤ 0.001), and Fe (R2 = 0.77, P ≤ 0.001). The KCaMg+ was only positively correlated (P ≤ 0.05) with Mn (R2 = 0.09). Finally, Zn, Cu, Mn, and Fe were positively correlated with each other.
4. Discussion
4.1. Effect of treatment factors on dry matter, ash, CP, NDIN, ADIN, and EE contents of forage legumes
Forage legumes have a successful synergic association with both AMF and Rhizobia bacteria, which eventually improves their chemical composition. However, the presence of these microbial symbionts in roots is not always beneficial for plant growth. The current study showed that inoculation with AMF or Rhizobia as the sole treatment and their interaction with forage legume species had no significant influence on DMC in both growing seasons. Similarly, the results are in line with the observation by Xavier and Germida (2003), who reported that different forages had similar DMC regardless of the treatment applied. The lack of difference in the DMC of forage legumes as affected by dual inoculation could be due to the harvesting stage at 120 days as forages were about to become lignified. According to Crowder and Chheda (1982), the increasing lignification and proportion of the leaves at maturity are the main causes of a decline in forage dry matter content. As forages mature, the accumulation of dry matter content decreases despite rising forage dry matter yields (Tjelele, 2006).
Ash contents are components of minerals in forages that reduce feed intake, palatability, digestibility, and nutrient composition in forages when they are in abundance (Halder et al., 2015). The present study demonstrated that sole inoculation with AMF and Rhizobia bacteria as well as their interaction significantly affected the concentration of ash in herbaceous forage legumes over two seasons. Even though dual inoculation had a great impact on the ash content of legumes, the three-way interaction of forage legume species with AMF and Rhizobia showed no significant differences in both seasons. According to Yaseen et al. (2011), dual inoculation reduces the ash content and increases the N and CP contents in forages. The current results could be due to differences in genotype, climatic conditions, stage of maturity, and harvesting (Samanhudi et al., 2014). For instance, the increase in ash content in the first season might be attributed to low rainfall and high temperatures. Moreover, under high temperatures and low rainfall in the first season, the ash content of forages was significantly high and low under LC and CAR, respectively. In the second season, the ash content was low with cowpea control (CC) and high with LR treatment. The present results demonstrated that dual inoculation could improve the ash content of forage legumes as compared to uninoculated control. This indicates that lablab forage has fewer nutrients compared to other forages (cowpea and mucuna). Nworgu and Ajayi (2005) reported that high ash content reduces forage quality, as some minerals such as Ca, Mg, K, and P are reduced at high ash content. Yoseph and Worku (2014) further reported that high ash content might be attributed to contamination from dirt or soil during harvesting time. However, feeds with high ash content are considered poor-quality feed since this mineral is only required in small quantities (Nworgu and Ajayi, 2005; Yoseph and Worku, 2014).
The current study showed that the dual and sole inoculations with AMF and Rhizobia bacteria significantly improved the CP content of forage legumes in both seasons. The CAR treatment significantly had the highest CP content. This could be justified by the fact that AMFs can improve inorganic and organic soil N mobilization (Halder et al., 2015). In addition, the increase in the CP content of forage legumes could be attributed to the increase in nutrient uptake of N to extra-radical mycorrhizae hyphae beyond the root hair and nutrient depletion zones (Ayasan et al., 2020). The present results are in line with findings by Ashrafi et al. (2014) and Ben-Laouane et al. (2021), which indicated that the dual inoculation of AMF and Rhizobia bacteria had a positive effect on the CP content of alfalfa forage legume. In particular, the range of CP content of forage legumes was within the range reported by Singh et al. (2004), Yaseen et al. (2011), and Halder et al. (2015), and was comparable to the feed requirements of ruminants (NRC, 2004). As such, it is sufficient to be used as a supplement for poor-quality pastures during the dry seasons to increase productivity in ruminant livestock.
NDIN and ADIN are components of N that are bound to the NDF and ADF fractions of the cell wall, respectively. The treatment combinations of legumes, AMF, and Rhizobia bacteria showed no significant differences in the content of NDIN and ADIN in both growing seasons. Since there was no significant interaction between soil microbes and the root system for the components; this simply showed that the two organisms were highly effective and had a strong synergistic effect on each other to produce more digestible protein and less non-digestible protein (Haruna and Usman, 2013). Furthermore, it is evident that biofertilization with AMF and Rhizobia bacteria is effective in reducing the non-bounded structural cell wall protein in forages. In support of this, the current study showed that biofertilization increased root nodule development and soluble N digestible while suppressing the NDIN and ADIN contents. This can be attributed to the increase in NDIN and ADIN contents because of the increase in NDF and ADF contents, respectively (Konvalinková et al., 2017).
The results indicated that sole inoculation with AMF or Rhizobia bacteria had an impact on the EE content in both seasons. However, the dual inoculation showed insignificant effects on EE content, except for the interaction of legumes and AMF in the first season. The lablab forage contained more EE content than cowpea and mucuna in both seasons. The increase in EE content was likely due to the increase in mycorrhizal infection resulting from mycorrhizal and/or Rhizobium inoculation, especially sole inoculation. The results are in line with the findings by Konvalinková et al. (2017), which reported that sole inoculation of AMF increased EE content. This may be because certain forages thrive under suitable growing conditions without the support of soil microbes; however, several authors have shown that dual inoculation enhances growth and plant components more in saline or toxic acidic soils (Yaseen et al., 2011).
4.2. Effect of treatments on NSC, NDF, ADF, and hemicellulose contents of three legumes
The results showed that there were significant interactions among treatment combinations on NSC content, with lablab forage containing more NSC than cowpea and mucuna forages in both seasons. The most noticeable effect of the dual inoculation was observed with the LAR treatment. The increase in NSC content in the forage could be due to the interaction of legume species, stage of harvesting, and climatic and environmental conditions (Okeleye and Okelama, 2000; Artursson et al., 2006; Mpongwana et al., 2023). In addition, its significant increase might be due to time and the method of cutting the forages. For example, cutting forages in the morning can result in low NSC compared to forages harvested during the day (Haruna and Usman, 2013).
It was evident that higher NDF, ADF, and hemicellulose contents were significantly affected by legume species, AMF, and Rhizobia bacteria and their interactions. Lablab forage produced a higher amount of NDF, ADF, and hemicellulose than other forages. The higher crude fiber components over two seasons could be related to genetic differences among forage species, and climatic and environmental factors. The present study showed that regardless of the effect of single or dual inoculation with AMF and Rhizobia bacteria, forages with low CP produced high crude fibers. This could be explained by the fact that CP is negatively related to NDF and ADF, meaning if CP increases, NDF and ADF will decrease. Artursson et al. (2006) mentioned that the decrease in the content of NDF was caused by an increase in lignin in plants. The other factor might be the strong interaction of dual inoculation with host plants in prolonging the vegetation components, which resulted in delaying the aging of the forages and making them more lignified at the stage of harvest (Yoseph and Worku, 2014). Moreover, other authors suggested that the low crude fiber components on dual-inoculated forages could be because forages with dual or single inoculation could increase stomatal conductance, photosynthetic activity, and transpiration, which eventually contribute to green leaf production (Konvalinková et al., 2017).
4.3. The effects of treatment factors on macro- and micronutrients of three forage legumes
The current study indicated that sole inoculation with AMF or Rhizobia bacteria as well as reciprocal interactions between legume species and AMF significantly enhanced forage Ca and P contents over two growing seasons. The highest Ca and P contents were associated with LA and LAR treatments in both seasons. The current results of dual inoculation positively affecting Ca and P contents were contradicted by the findings of others (Yaseen et al., 2011). This is because dual inoculation does not always improve the nutrient composition of forages as compared with sole inoculation. In the present study, this has been advocated by the dual inoculation of plants with AMF and Rhizobia, which promoted root development. AMF and Rhizobium inoculation are known to increase soil chemical and nutritional quality through various mechanisms such as symbiotic nitrogen fixation, siderophores and exopolysaccharide synthesis, and phosphate and potassium solubilization (Naseem et al., 2018; Primo et al., 2020). Therefore, this results in plant hosts enhancing nutrient uptake of P, Ca, and other mineral nutrients (Kawaguchi and Minamisawa, 2010; Mpongwana et al., 2023). The symbiosis relationship of forage legumes with AMF provides a greater absorptive surface through the hyphal network, thus improving the uptake of relatively immobile ions from soil (Aziz and Khan, 2001; Farzaneh et al., 2009).
Sole inoculation with either AMF or Rhizobia bacteria and their interaction with legume species significantly increased Mg, KCaMg+ ions, Ca/P, Zn, Mn, and Fe contents when compared with control treatments. However, sole and dual inoculation showed no significant differences in the K, Na, and Cu contents of forages in both seasons. Correspondingly, Ashrafi et al. (2014) reported that dual inoculation with AMF and Rhizobia bacteria had a positive effect on forage Mg, K, Mn, Na, Cu, Zn, and Fe contents in alfalfa plants. Moreover, Aziz and Khan (2001) reported that biofertilization with AMF and Rhizobia bacteria enhanced these mineral contents of Ceriops tagal. The improved uptake of nutrients such as Mg, Zn, and Fe in the current study could be attributed to AMF creating extra-radical mycelium, which can be dispersed in the rhizosphere and increase intake by the absorbing surface of roots (Farzaneh et al., 2009).
The present study demonstrated that a single application of AMF and Rhizobia bacteria or dual inoculation had no effect on K, Na, Cu, and Fe contents for both growing seasons. In contrast, Baslam et al. (2013) demonstrated an increase in these mineral contents on various crops due to inoculation with AMF. According to Clark and Zeto (2000), AMF application encourages biological N fixation through P and other immobile nutrients acquired in legumes to increase the accessibility of Cu and Zn contents. The different results reported in the current study could be due to the lack of response of the original AMF and Rhizobia bacteria strains to interact with non-native applications of AMF and Rhizobia bacteria to form an association with the host plant. As a result, the response to single or dual inoculation on the nutrient composition of forage legumes can be greatly enhanced by ensuring that soil conditions and environmental conditions are suitable for plant hosts to make associations with inoculants.
4.4. Correlation matrix of chemical composition in two seasons for three herbaceous legumes
The correlation analysis for two growing seasons showed that chemical nutrients were correlated with each other. The DMC was negatively correlated with CP, EE, and NSC contents while positively correlated with NDIN, NDF, ADF, and hemicellulose contents. Similarly, Yaseen et al. (2011) and Konvalinková et al. (2017) reported a significant positive correlation between DMC and NDF as well as ADF and lignin in mug beans and chickpeas, respectively. A positive correlation was also observed between Ca and nutrient constituents such as P, Mg, K, and Mn, while Ca was negatively correlated with Na, Ca/P, Zn, and Fe. This simply showed that there was a positional interaction between AMF and Rhizobia bacteria with the plant host to increase macronutrients and other chemical constituents. The dual inoculation plays a vital role in achieving a positive correlation among chemical constituents for improved quality of legume foliage (Tufenkci et al., 2006; Karaman et al., 2013).
5. Conclusion
The present study demonstrated that the use of AMF and Rhizobia bacteria has a positive effect on the chemical and nutritional composition of herbaceous legumes, namely cowpea, lablab, and mucuna. It demonstrated that the NDF, ADF, hemicellulose, CP, ash, Mg, P, Ca, Zn, and Fe of three herbaceous forage legumes were greatly improved by the dual inoculation with AMF and Rhizobia bacteria. Furthermore, solo inoculation with AMF or Rhizobia bacteria and their interaction with legume species resulted in substantially higher KCaMg+ ions, Ca/P, Zn, Mn, and Fe concentrations than control treatments. When compared to single inoculation and uninoculated forages, dual inoculation produced optimal nutrient accumulation in both growing seasons. Given that dual inoculation of AMF and Rhizobia improved growth parameters and biomass production in our previous study (Mpongwana et al., 2023), dual inoculation could be suitable in efforts to lower the dependency on chemical fertilizers while maintaining the integrity of the chemical composition of these three herbaceous forage legumes in semi-arid regions. Overall, the results showed that the improved nutritional quality of forages due to the application of AMF and Rhizobia bacteria could be sufficient to be used as a supplement for poor-quality pastures during the dry seasons to reduce malnutrition and improve livestock nutrition. However, it is important to point out that the efficiency of AMF utilization and Rhizobia in enhancing nutrient uptake and biological nitrogen fixation, as well as the increasing foliar nutritional composition of legumes, is influenced by AMF species, plant genotype, availability of soil nutrients to plants, environment, and stress factors.
Data availability statement
The original contributions presented in the study are included in the article/supplementary material, further inquiries can be directed to the corresponding author.
Author contributions
SM: Conceptualization, Data curation, Formal analysis, Investigation, Methodology, Visualization, Writing—original draft. AM: Conceptualization, Funding acquisition, Methodology, Project administration, Resources, Supervision, Validation, Writing—review & editing. JM: Conceptualization, Funding acquisition, Methodology, Project administration, Resources, Supervision, Validation, Writing—review & editing. CTM: Conceptualization, Funding acquisition, Methodology, Project administration, Resources, Supervision, Validation, Writing—review & editing. CVM: Validation, Writing—original draft, Writing—review & editing.
Funding
The author(s) declare financial support was received for the research, authorship, and/or publication of this article. The study was funded by the National Research Foundation (T353) of South Africa and the Govan Mbeki Research and Development Centre (C203) (South Africa).
Conflict of interest
The authors declare that the research was conducted in the absence of any commercial or financial relationships that could be construed as a potential conflict of interest.
Publisher's note
All claims expressed in this article are solely those of the authors and do not necessarily represent those of their affiliated organizations, or those of the publisher, the editors and the reviewers. Any product that may be evaluated in this article, or claim that may be made by its manufacturer, is not guaranteed or endorsed by the publisher.
References
Aalipour, H., Nikbakht, A., Etemadi, N., Rejali, F., and Soleimani, M. (2020). Biochemical response and interactions between arbuscular mycorrhizal fungi and plant growth promoting rhizobacteria during establishment and stimulating growth of Arizona cypress (Cupressus arizonica G.) under drought stress. Sci. Hortic. 261:108923. doi: 10.1016/j.scienta.2019.108923
Abd-Alla, M. H., El-Enany, A. -W. E., Nafady, N. A., Khalaf, D. M., and Morsy, F. M. (2014). Synergistic interaction of Rhizobium leguminosarum bv. viciae and arbuscular mycorrhizal fungi as a plant growth promoting biofertilizers for faba bean (Vicia faba L.) in alkaline soil. Microbiol. Res. 169, 49–58. doi: 10.1016/j.micres.2013.07.007
Ait Rahou, Y., Ait-El-Mokhtar, M., Anli, M., Boutasknit, A., Ben-Laouane, R., Douira, A., et al. (2020). Use of mycorrhizal fungi and compost for improving the growth and yield of tomato and its resistance to Verticillium dahliae. Arch. Phytopathol. Plant Prot. 54, 665–690. doi: 10.1080/03235408.2020.1854938
Amole, T. A., Oduguwa, B. O., Shittu, O., Famakinde, A., Okwelum, N., Ojo, V. O. A., et al. (2013). Herbage yield and quality of Lablab purpureus during the late dry season in Western Nigeria. Slovak J. Anim. Sci. 46, 22–30.
Andersone-Ozola, U., Jekabsone, A., Purmale, L., Romanovs, M., and Ievinsh, G. (2021). Abiotic stress tolerance of coastal accessions of a promising forage species, Trifolium fragiferum. Plants 10:1552. doi: 10.3390/plants10081552
Anli, M., Kaoua, M. E., ait-el-Mokhtar, M., Boutasknit, A., ben-Laouane, R., Toubali, S., et al. (2020). Seaweed extract application and arbuscular mycorrhizal fungal inoculation: a tool for promoting growth and development of date palm (Phoenix dactylifera L.) cv ≪Boufgous≫. S. Afr. J. Bot. 132, 15–21. doi: 10.1016/j.sajb.2020.04.004
Artursson, V., Finlay, R. D., and Jansson, J. K. (2006). Interactions between arbuscular mycorrhizal fungi and bacteria and their potential for stimulating plant growth. Environ. Microbiol. 8, 1–10. doi: 10.1111/j.1462-2920.2005.00942.x
Ashrafi, E., Zahedi, M., and Razmjoo, J. (2014). Co-inoculations of arbuscular mycorrhizal fungi and Rhizobia under salinity in alfalfa. Soil Sci. Plant Nutr. 60, 619–629. doi: 10.1080/00380768.2014.936037
Association of Official Analytical Chemists (AOAC) (2005). Official Methods of Analysis of the AOAC International, 18th edn. Gaitherburg, MD: AOAC International.
Ayasan, T., Cabi, E., Esen, S., Esen, V. K., and Eseceli, H. (2020). Effect of arbuscular mycorrhizal inoculation on the quality and in vitro gas production of einkorn wheat straw. S. Afr. J. Anim. Sci. 50, 415–420. doi: 10.4314/sajas.v50i3.8
Aziz, I., and Khan, M. A. (2001). Experimental assessment of salinity tolerance of Ceriops tagal seedlings and saplings from the Indus delta, Pakistan. Aquat. Bot. 70, 259–268. doi: 10.1016/S0304-3770(01)00160-7
Bartucca, M. L., Cerri, M., Del Buono, D., and Forni, C. (2022). Use of biostimulants as a new approach for the improvement of phytoremediation performance: a review. Plants 11:1946. doi: 10.3390/plants11151946
Baslam, M., Antolin, M. C., Gogotcena, Y., and Munuz, F. (2013). Changes in alfalfa forage quality and stem carbohydrates induced by arbuscular mycorrhizal fungi and elevated atmospheric CO2. Ann. Appl. Biol. 164, 190–199. doi: 10.1111/aab.12092
Battenfield, S. D., Guzmán, C., Gaynor, R. C., Singh, R. P., Peña, R. J., Dreisigacker, S., et al. (2016). Genomic selection for processing and end-use quality traits in the CIMMYT spring bread wheat breeding program. Plant Genome 9, 1–12. doi: 10.3835/plantgenome2016.01.0005
Benaffari, W., Boutasknit, A., Anli, M., Ait-El-Mokhtar, M., Ait-Rahou, Y., Ben-Laouane, R., et al. (2022). The native arbuscular mycorrhizal fungi and vermicompost-based organic amendments enhance soil fertility, growth performance, and the drought stress tolerance of quinoa. Plants 11:393. doi: 10.3390/plants11030393
Benjelloun, I., Thami Alami, I., El Khadir, M., Douira, A., and Udupa, S. M. (2021). Co-inoculation of mesorhizobium ciceri with either Bacillus sp. or enterobacter aerogenes on chickpea improves growth and productivity in phosphate-deficient soils in dry areas of a mediterranean region. Plants 10:571. doi: 10.3390/plants10030571
Ben-Laouane, R., Ait-El-Mokhtar, M., Anli, M., Boutasknit, A., Ait Rahou, Y., Raklami, A., et al. (2021). Green compost combined with mycorrhizae and Rhizobia: a strategy for improving alfalfa growth and yield under field conditions, Gesunde Pflanz 73, 193–207. doi: 10.1007/s10343-020-00537-z
Ben-Laouane, R., Meddich, A., Bechtaoui, N., Oufdou, K., and Wahbi, S. (2019). Effects of arbuscular mycorrhizal fungi and Rhizobia symbiosis on the tolerance of Medicago sativa to salt stress. Gesunde Pflanz 71, 135–146. doi: 10.1007/s10343-019-00461-x
Berruti, A., Lumini, E., and Bianciotto, V. (2016). Arbuscular mycorrhizal fungi as natural biofertilizers: let's benefit from past successes. Front. Microbiol. 6:1559. doi: 10.3389/fmicb.2015.01559
Bhattacharyya, C., Roy, R., Tribedi, P., Ghosh, A., and Ghosh, A. (2020). “Biofertilizers as substitute to commercial agrochemicals,” in Agrochemicals Detection, Treatment And Remediation: Chapter 11 (Oxford: Butterworth-Heinemann), 263–290. doi: 10.1016/B978-0-08-103017-2.00011-8
Bisht, N., and Chauhan, P. S. (2020). “Excessive and disproportionate use of chemicals cause soil contamination and nutritional stress,” in Soil Contamination-Threats and Sustainable Solutions (Lucknow: IntechOpen).
Chalk, P. M. R., de Souza, F., Urquiaga, S., Alves, B. J. R., and Boddey, R. M. (2006). The role of arbuscular mycorrhiza in legume symbiotic performance. Soil Biol. Biochem. 38, 2944–2951. doi: 10.1016/j.soilbio.2006.05.005
Chand, S., Indu Singhal, R. K., and Govindasamy, P. (2022). Agronomical and breeding approaches to improve the nutritional status of forage crops for better livestock productivity. Grass Forage Sci. 77, 11–32. doi: 10.1111/gfs.12557
Clark, R. B., and Zeto, S. K. (2000). Mineral acquisition by arbuscular mycorrhizal plants. Plant Nutr. 23, 867–902. doi: 10.1080/01904160009382068
Crowder, L. V., and Chheda, H. R. (1982). Tropical Grassland Husbandry. London and New York, NY: Longman.
Dames, J., and Ridsdale, C. (2012). What we know about arbuscular mycorrhizal fungi and associated soil bacteria. Afr. J. Biotechnol. 11, 13753–13760. doi: 10.5897/AJBX11.053
Duan, Y., Xu, M., Gao, S., Liu, H., Huang, S., and Wang, B. (2016). Long-term incorporation of manure with chemical fertilizers reduced total nitrogen loss in rain-fed cropping systems. Sci. Rep. 6:33611. doi: 10.1038/srep33611
Dumsane, M., Cheng-Hua, H., Yuh-Ming, H., and Ming-Yi, Y. (2020). Effects of co-inoculation of Rhizobium with plant growth promoting rhizobacteria on the nitrogen fixation and nutrient uptake of Trifolium repens in low phosphorus soil. J. Plant Nutr. 43, 739–752. doi: 10.1080/01904167.2019.1702205
Dziba, L. E., Scogings, P. F., Gordon, I. J., and Raats, J. G. (2003). Effects of season and breed on browse species intake rates and diet selection by goats in the false thornveld of the Eastern Cape, South Africa. Small Rumin. Res. 47, 17–30. doi: 10.1016/S0921-4488(02)00235-3
Farzaneh, M., Wichman, S., Vierheilig, H., and Kaul, H. P. (2009). The effects of arbuscular mycorrhizal and nitrogen nutrition on growth of chickpea and barley. Pflanzenbawissenschaften 13, 15–22.
Halder, M., Mujib, A. S. M., Khan, M. S., Joardar, J. C., Akhater, S., and Dhar, P. P. (2015). Effect of arbuscular mycorrhizal fungi inoculation on growth and uptake of mineral nutrition in Ipomoea aquatica. Curr. World Environ. 10, 67–75. doi: 10.12944/CWE.10.1.08
Haruna, I. M., and Usman, A. (2013). Agronomic efficiency of cowpea varieties (Vigna unguiculata (L.) Walp) under varying phosphorus rates in Lafia, Nassarawa state, Nigeria. Asian J. Crop Sci. 5, 209–215. doi: 10.3923/ajcs.2013.209.215
Hemissi, I., Mabrouk, Y., Abdi, N., Bouraoui, M., Saidi, M., and Sifi, B. (2011). Effects of some Rhizobium strains on chickpea growth and biological control of Rhizoctonia solani. Afr. J. Microbiol. Res. 5, 4080–4090. doi: 10.5897/AJMR10.903
Ingraffia, R., Amato, G., Sosa-Hernández, M. A., Frenda, A. S., Rillig, M. C., and Giambalvo, D. (2020). Nitrogen type and availability drive mycorrhizal effects on wheat performance, nitrogen uptake and recovery, and production sustainability. Front. Plant Sci. 11:760. doi: 10.3389/fpls.0.2020.00760
International Union of Soil Sciences (IUSS) Working Group World Reference Base (2022). World Reference Base for Soil Resources, International Soil Classification System for Naming Soils and Creating Legends for Soil Maps, 4th edn. Vienna: International Union of Soil Sciences (IUSS), 234.
Karaman, M. R., Sahin, S., Duzdemir, O., and Kandemir, N. (2013). Selection of chickpea cultivars with agronomic phosphorus (P) utilization characters as influenced by Rhizobium inoculation. Sci. Res. Essays 8, 676–681. doi: 10.5897/sre12.619
Kawaguchi, M., and Minamisawa, K. (2010). Plant-microbe communications for symbiosis. Plant Cell Physiol. 51, 1377–1380. doi: 10.1093/pcp/pcq125
Konvalinková, T., Püschel, D., and Øezáèová, V. (2017). Carbon flow from plant to arbuscular mycorrhizal fungi is reduced under phosphorus fertilization. Plant Soil 419, 319–333. doi: 10.1007/s11104-017-3350-6
Korir, H., Mungai, N. W., Thuita, M., Hamba, Y., and Masso, C. (2017). Co-inoculation effect of Rhizobia and plant growth promoting rhizobacteria on common bean growth in a low phosphorus soil. Front. Plant Sci. 8:141. doi: 10.3389/fpls.2017.00141
Krämer-Schmid, M., Lund, P., and Weisbjerg, M. R. (2016). Importance of NDF digestibility of whole crop maize silage for dry matter intake and milk production in dairy cows. Anim. Feed Sci. Technol. 219, 68–76. doi: 10.1016/j.anifeedsci.2016.06.007
Lamidi, O. S., Jibril, A., and Adeyinka, I. A. (2005). Preference studies and short-term intake of Browse mixtures by cattle. ASSET Series A 5, 45–52.
Mabapa, M. P., Ayisi, K. K., and Mariga, I. K. (2017). Effect of plant density and harvest interval on leaf yield and quality of moringa (Moringa oleifera) under diverse agro-ecological conditions of Northern South Africa. Int. J. Agron. 9:2941432. doi: 10.1155/2017/2941432
Matizha, W., Ngongoni, N. T., Topps, J. H., and Sibanda, S. (2001). Chemical composition of three herbaceous tropical forage legumes grown successfully in Zimbabwe. J. Appl. Sci. South. Afr. 7, 71–83.
Mbodj, D., Effa-Effa, B., Kane, A., Manneh, B., Gantet, P., Laplaze, L., et al. (2018). Arbuscular mycorrhizal symbiosis in rice: establishment, environmental control and impact on plant growth and resistance to abiotic stresses. Rhizosphere 8, 12–26. doi: 10.1016/j.rhisph.2018.08.003
Meddich, A., Ait El Mokhtar, M., Bourzik, W., Mitsui, T., Baslam, M., and Hafidi, M. (2018). “Optimizing growth and tolerance of date palm (Phoenix dactylifera L.) to drought, salinity, and vascular fusarium-induced wilt (Fusarium oxysporum) by application of Arbuscular Mycorrhizal Fungi,” in Root Biology. Soil Biology, Vol. 52, eds B. Giri, R. Prasad, and A. Varma (Berlin; Heidelberg: Springer), 239–250. doi: 10.1007/978-3-319-75910-4_9
Moila, M. M., Manyevere, A., Mthimkhulu, S., and Mariga, I. (2020). Short-term impact of arbuscular mycorrhizal fungi and different phosphorus levels on selected soil properties and growth components of two dry bean cultivars in phosphorus-deficient soils. S. Afr. J. Plant Soil 37, 321–325. doi: 10.1080/02571862.2020.1757768
Mokabel, S., Olama, Z., Ali, S., and El-Dakak, R. (2022). The role of plant growth promoting Rhizosphere microbiome as alternative biofertilizer in boosting Solanum melongena L. adaptation to salinity stress. Plants 11:659. doi: 10.3390/plants11050659
Mortimer, P. E., Pérez-Fernández, M. A., and Valentine, A. J. (2012). Arbuscular mycorrhiza maintains nodule function during external NH 4+supply in Phaseolus vulgaris (L.). Mycorrhiza 22, 237–245. doi: 10.1007/s00572-011-0396-9
Mpongwana, S., Manyevere, A., Mupangwa, J., Mpendulo, C. T., and Mashamaite, C. V. (2023). Optimizing biomass yield of three herbaceous forage legumes through dual inoculation of arbuscular mycorrhizal fungi and Rhizobia. S. Afr. J. Bot. 159, 61–71. doi: 10.1016/j.sajb.2023.06.006
Nacoon, S., Seemakram, W., Ekprasert, J., Theerakulpisut, P., Sanitchon, J., Kuyper, T. W., et al. (2023). Arbuscular Mycorrhizal Fungi enhance growth and increase concentrations of anthocyanin, phenolic compounds, and antioxidant activity of black rice (Oryza sativa L.). Soil Syst. 7:44. doi: 10.3390/soilsystems7020044
Naseem, H., Ahsan, M., Shahid, M. A., and Khan, N. (2018). Exopolysaccharides producing rhizobacteria and their role in plant growth and drought tolerance. J. Basic. Microbiol. 58, 1009–1022. doi: 10.1002/jobm.201800309
NRC (2004). Nutrient Requirements of Dairy Cattle. 7th Revision Edn. Washington DC: National Research Council, The National Academy Press.
Nworgu, F. C., and Ajayi, F. T. (2005). Biomass, dry matter yield, proximate and mineral composition of forage legumes grown as early dry season feeds. Livest. Res. Rural. Dev. 17, 121.
Okeleye, K. A., and Okelama, M. A. O. (2000). Effect of phosphorus fertilizer on nodulation, growth, and yield of cowpea (Vigna unguiculata) varieties. Indian J. Agric. Sci. 67, 10–12.
Primo, E. D., Cossovich, S., Nievas, F., Bogino, P., Humm, E. A., Hirsch, A. M., et al. (2020). Exopolysaccharide production in Ensifer meliloti laboratory and native strains and their effects on alfalfa inoculation. Arch. Microbiol. 202, 391–398. doi: 10.1007/s00203-019-01756-3
Püschel, D., Janoušková, M., Voríšková, A., Gryndlerová, H., Vosátka, M., and Jansa, J. (2017). Arbuscular Mycorrhiza stimulates biological nitrogen fixation in two Medicago spp. through improved phosphorus acquisition. Front. Plant Sci. 8:390. doi: 10.3389/fpls.2017.00390
Qureshi, M. A., Ali, A., Ali, M. A., Ehsan, S., Javed, H., Rafique, M., et al. (2023). Co-inoculation of Rhizobium sp and Rhizobacteria for growth promotion of mungbean (Vigna radiata L.). J. Pure Appl. Agric. 8, 16–23.
Rajendran, G., Sing, F., Desai, A. J., and Archana, A. (2008). Enhanced growth and nodulation of pigeon pea by co-inoculation of Bacillus strains with Rhizobium spp. Bioresour. Technol. 99, 4544–4550. doi: 10.1016/j.biortech.2007.06.057
Ramakrishnan, K., and Lenin, M. (2010). Effect of Glomus intaradices and Rhizobium on the growth of groundnut (Arachis hypogaea L.). J. Ecobiotechnol. 2, 1–8.
Rankoana, S. A. (2016). Perceptions of climate change and the potential for adaptation in a rural community in Limpopo Province, South Africa. Sustainability 8:672. doi: 10.3390/su8080672
Sachs, J. L., Quides, K. W., and Wendlandt, C. E. (2018). Legumes versus Rhizobia: a model for ongoing conflict in symbiosis. New Phytol. 219, 1199–1206. doi: 10.1111/nph.15222
Samanhudi, A., Yunus, B., Pujiasmanto, B., and Rahayu, M. (2014). Application of organic manure and mycorrhizal for improving plant growth and yield of Temulawak (Curcuma xanthorrhiza Roxb.). Sci. Resour. J. 2, 2201–2796.
Schwab, S. T., Quides, K. W., Wendlandt, C. E., Trinh, J., Sung, M., and Cardenas, P. (in press). Effective Rhizobia enhance legume growth during subsequent drought despite water costs associated with nitrogen fixation. Plant Soil doi: 10.1007/s11104-023-06164-7.
Sharma, N., and Singhvi, R. (2017). Effects of chemical fertilizers and pesticides on human health and environment: a review. Int. J. Agric. Environ. Biotechnol. 10, 675–679. doi: 10.5958/2230-732X.2017.00083.3
Singh, A. L., Basu, M. S., and Singh, N. B. (2004). Mineral Disorders of Groundnut. JunagadhP: National Research Centre for Groundnut (ICAR), 85.
Soussani, F. E., Boutasknit, A., Ben-Laouane, R., Benkirane, R., Baslam, M., and Meddich, A. (2023). Arbuscular Mycorrhizal Fungi and compost-based biostimulants enhance fitness, physiological responses, yield, and quality traits of drought-stressed tomato plants. Plants 12:1856. doi: 10.3390/plants12091856
Tjelele, T. J. (2006). Dry matter production, intake and nutritive value of certain indigofera species (MSc Thesis). University of Pretoria, South Africa.
Tufenkci, S., Somemez, F., and Sensory, R. I. G. (2006). Effects of Arbuscular Mycorrhizal Fungus inoculation and phosphorus and nitrogen fertilizations on some plant growth parameters and nutrient content of soybean. Pak. J. Biol. Sci. 9, 1121–1127. doi: 10.3923/pjbs.2006.1121.1127
Umesha, S., Manukumar, H. M. G., and Chandrasekhar, B. (2018). “Sustainable agriculture and food security,” in Biotechnology for Sustainable Agriculture, eds R. L. S. Singh (Gurgaon: Elsevier Inc.), 67–92. doi: 10.1016/B978-0-12-812160-3.00003-9
Van Soest, P. J., Robertson, J. B., and Lewis, B. A. (1991). Methods for dietary fiber, neutral detergent fiber, and nonstarch polysaccharides in relation to animal nutrition. J. Dairy Sci. 74, 3583–3597. doi: 10.3168/jds.S0022-0302(91)78551-2
Varinderpal-Singh Sharma, S., Kunal, S., Gosal, K., Choudhary, R., Singh, R., et al. (2019). Synergistic use of plant growth-promoting rhizobacteria, arbuscular mycorrhizal fungi, and spectral properties for improving nutrient use efficiencies in wheat (Triticum aestivum L.). Commun. Soil Sci. Plant Anal. 51, 14–27. doi: 10.1080/00103624.2019.1689259
White, J., Prell, J., James, E. K., and Poole, P. (2007). Nutrient sharing between symbionts. Plant Physiol. 144, 604–614. doi: 10.1104/pp.107.097741
Wilkins, R. J. (2018). “The nutritive value of silages,” in Nutrition Conference for Feed Manufacturers, eds H. Swan and D. Lewis (Elsevier Inc. University of Nottingham), 167–200.
Xavier, L. J., and Germida, J. J. (2003). Selective interactions between arbuscular mycorrhizal and Rhizobium leguminosarum bv. viceae enhance pea yield and nutrition. Biol. Fertil. Soils 37, 261–267. doi: 10.1007/s00374-003-0605-6
Yaseen, T., Burni, T., and Hussain, F. (2011). Effect of arbuscular mycorrhizal inoculation on nutrient intake, growth and productivity of cowpea (Vigna unguiculata) varieties. Afr. J. Biotechnol. 10, 8593–8598. doi: 10.5897/AJB10.1494
Yoseph, T., and Worku, W. (2014). Effect of NP fertilizer rate and bradyrhizobium inoculation on nodulation, N-uptake and crude protein content of soybean [Glycine max (L.) Merrill], at Jinka, Southern Ethiopia. J. Biol. Agric. Healthcare 4, 49–54.
Keywords: arbuscular mycorrhizal fungi, chemical constituents, dual inoculation, forage nutrient optimization, livestock malnutrition, Rhizobium, sustainable production
Citation: Mpongwana S, Manyevere A, Mupangwa J, Mpendulo CT and Mashamaite CV (2023) Foliar nutrient content responses to bio-inoculation of arbuscular mycorrhizal fungi and Rhizobium on three herbaceous forage legumes. Front. Sustain. Food Syst. 7:1256717. doi: 10.3389/fsufs.2023.1256717
Received: 11 July 2023; Accepted: 29 September 2023;
Published: 25 October 2023.
Edited by:
Som Dutt, Central Potato Research Institute (ICAR), IndiaReviewed by:
Raja Ben-Laouane, Cadi Ayyad University, MoroccoMarika Pellegrini, University of L'Aquila, Italy
Copyright © 2023 Mpongwana, Manyevere, Mupangwa, Mpendulo and Mashamaite. This is an open-access article distributed under the terms of the Creative Commons Attribution License (CC BY). The use, distribution or reproduction in other forums is permitted, provided the original author(s) and the copyright owner(s) are credited and that the original publication in this journal is cited, in accordance with accepted academic practice. No use, distribution or reproduction is permitted which does not comply with these terms.
*Correspondence: Alen Manyevere, amanyevere@ufh.ac.za