- 1Department of Economics, University of Life Science, Prague, Czechia
- 2Department of Trade and Finance, University of Life Sciences, Prague, Czechia
Introduction: The paper aims to examine the prospects of algae production as a source of protein in the European market. As well as highlighting the promising developments in the algae food industry in Europe. By 2027, it is expected that the algae protein market will be worth approximately USD 300 million.
Methods: We conducted thematic analysis and synthesis of scientific literature and conceptual documents at the European level.
Results: The studies reviewed show that the nutritional value of food products can be increased by using algae. The production of algae for food should be encouraged in Europe because it is a viable alternative protein source. To fully utilize algae as a source of protein, however, a number of technological, regulatory, and market-related obstacles must be resolved despite the indicative advantages. Developing cost-effective and efficient methods for algae cultivation, harvesting, and processing are also necessary. Uniform and consistent regulations are needed to guarantee the safety and quality of products that contain algae, as well as consumer awareness campaigns and education about the advantages of algae protein.
Conclusion: In addition to providing evidence of the viability of algae production as a source of food, this study demonstrates that algae land needs are negligible compared to other protein sources—animal-based like pork, chicken and beef production but also plant-based alternatives such as nuts, pulses, grains and peas. Furthermore, results in this study may inspire a more targeted focus on algae production as a source of nutrition and inspire more organizations around the world to move ahead with the alternative protein source production from algae.
1 Introduction
Algae offers many benefits for both natural ecosystems and human applications such as biofuel production, cosmetics or pharmaceuticals (Sáez et al., 2022; Zrimec et al., 2023). Also, algae are an important component of global food production. According to Ścieszka and Klewicka (2018), algae has been used in many industrial areas due to its varied chemical makeup and high bioactive component concentration. Agar, alginate, and carrageenan are a few examples of algae derivatives used due to their gelling, thickening, and stabilizing abilities. Algae are used in the food sector as functional foods and dietary supplements. They are also used to enhance the quality of meat products like patties, steaks, frankfurters, and sausages in addition to fish, fish products and oils (Ścieszka and Klewicka, 2018). More people are consuming macroalgae and microalgae meals hence driving the growing global demand for them for nutrition and health reasons.
In recent years, algae have gained popularity as a substitute for animal protein due to their nutrient-dense nature, providing protein, vitamins, and minerals (Bygora, 2021). According to Chronakis and Madsen (2011), algae proteins are valued for their excellent nutritional profile, which includes high levels of essential amino acids. Almost every ecosystem contains algae due to their role as a component of the food chain. Additionally, their functional properties, like coagulation, water absorption and fat, emulsification and foaming, are similar to those of land-based plants (Leandro et al., 2020). This makes algae-based foods versatile ingredients for use in a variety of food products.
This review contributes to the scientific literature on algae as a sustainable food source. Previous papers include research articles (Stengel and Connan, 2015; Kadam et al., 2017; Liao et al., 2021) and systematic literature reviews (Vilkhu et al., 2008; Ścieszka and Klewicka, 2018; Lee et al., 2020) focused on the algae production industry. Likewise, Araujo et al. (2021) provide an overview of countries in Europe producing microalgae and macroalgae. Algae as a source of nutrients, specifically fibers, proteins, omega 3 and 6 unsaturated fatty acids, vitamins, and minerals are reviewed in Leandro et al. (2020). Specific regions for seaweed production are also studied (e.g., Peru) (Avila-Peltroche and Padilla-Vallejos 2020). Risks related to algae capacity to biosorption were discussed in Sá Monteiro et al. (2019) or Filippini et al. (2021). However, there is little literature on the linkages between algae, protein content and environmental sustainability (Caporgno and Mathys, 2018; Diaz et al., 2023). We conducted thematic analysis and synthesis of scientific literature and conceptual documents at the European level. The paper aims to examine the prospects of algae production as a source of protein in the European market. More specifically, we established the following objectives: (1) to analyse the algae varieties as a source of protein; (2) to access algae food and protein production in Europe; (3) to identify technological, environmental and economic challenges and barriers to algae production in Europe.
2 Classification and cultivation of algae
Algae can be classified into nine large taxonomic groups called divisions. These are Chlorophyta, Xanthophyta, Bacillariophyta, Phaeophycophyta, Rhodophyta, Chrysophycophyta, Euglena Phycophyta, Cryptophycophyta, and Pyrrophycophyta (Ashwathi, 2021). Conversely, some studies show three classes of algae: Chlorophyceae, Phaeophyceae, and Rhodophyceae (Ulvskov et al., 2013). Algae are photoautotrophic prokaryotic (cyanobacteria) or eukaryotic organisms that can use light, CO2, and water to transform nitrogen and phosphorus from the environment into biomass. Algae can exist as mixotrophs or facultative heterotrophs, or they can use sunlight for photosynthesis (Li-Beisson et al., 2019). Some of the former developed into obligate heterotrophic parasites like Plasmodium and Toxoplasma (Plasmodium malarie, Plasmodium knowlesi, Plasmodium falciparum, Plasmodium vivax, and Plasmodium ovale) after losing the capacity to photosynthesize (Brodie et al., 2017). Green (Chlorophyta), blue-green (Cyanobacteria), yellow-green (Ochrophyta xanthophyta), and golden (Ochrophyta chrysophyta) algae, as well as diatoms (Bacillaryophyta), are examples of microalgae (Lee et al., 2020). Microalgae are also simple, one-celled, photosynthetic as well as occasionally heterotrophic microorganisms that are highly bioavailable. Microalgae are one of the oldest organisms on Earth, but their importance lies in the fact that they produce about 60 percent of the oxygen on Earth. Microalgae can be a source for the production of food, food supplements and further for industrial use (Piccolo, 2012).
Macroalgae include red, green, and brown algae (Ochrophyta). In Europe, the production of macroalgae concentrates mostly on brown algae such as Ascophyllim nodosum, Laminaria digitata, and Laminaria hyperborea, and is complemented by the production of other species on a small scale. Other most cultivated brown algae varieties include Saccharina japonica (Japanese kelp or kombu) and Undaria pinnatifida (Japanese wakame). While the least cultivated include red algae varieties like Eucheuma sp., Gracilaria spp., Kappaphycus spp., and Porphyra spp. (Japanese nori), and green algae varieties like Monostroma spp. and Enteromorpha spp.
Microalgae are common photosynthetic organisms, but they must be grown individually under ideal conditions to produce commercial quantities of biomass. They are generally grown in open ponds, stagnant water bodies, 20 to 30 cm deep (Arora et al., 2021). Similar to terrestrial plants, microalgae’s maximum performance is 0.5% PCE (photo-conversion efficiency) (Sun et al., 2018). Interestingly, their PCE can rise to 2% when CO2 and appropriate mixing are both present. The use of heterotrophic culture to produce industrial goods on a large scale has proven to be the most effective and does so by making carbon a suitable medium for light harvesting, and energy conversion (Sun et al., 2018). In contrast to photoautotrophic systems, these offer relatively higher volume growing conditions and better biomass productivity. Higher cell densities are provided via fed-batch cultures and membrane recycling systems, which also help to lower the extraction process costs. They use the respiratory metabolism process to generate energy from carbon substrates like acetate, glucose, and glycerol. This article further addresses an affordable carbon option for the use of wastewater and agricultural residues as a growth medium, such as starch hydrolysates, waste molasses, and rice straws (Morales-Sánchez et al., 2017; Xu et al., 2020).
3 Algae as a source of protein
Algae are a source of vital nutrients for humans. Algae are rich in iron and calcium because of their ability to absorb minerals and trace elements from the surrounding environment (Adluri et al., 2010; Wells et al., 2017). A lot of algae contain high levels of beta-carotene and are rich in vitamins like vitamin C and B12 (García et al., 2017; Galasso et al., 2019). They contain little fat but a lot of protein. The abundant dietary fibre in algae are called β-glucans (Galasso et al., 2019). β-glucans have been shown to provide a remarkable range of health benefits, and promote healthy cholesterol and blood glucose levels and enhance immune system functions. They are especially important against the two most common conventional causes of death in industrialized countries, i.e., cardiovascular diseases and cancer (Barsanti et al., 2011). Moreover, compared to plant sources like beans and grains, some algae species have been found to have a significantly higher protein content. While some also produce plenty of polyunsaturated fatty acids, like omega-3 (McHugh and Sinrod, 2019).
The commonly consumed algae are the green ones: Caulerpa racemosa, Codium, and Ulva, as well as the red ones: Porphyra (nori, kim, laver), Asparagopsis taxiformis (limu), Gracilaria, Chondrus crispus (Irish moss), Palmaria palmata (dulse), the Kelps laminaria (kombu), Undaria (wakame), and Macrocystis and these are usually eaten fresh, dried, or pickled (Adluri et al., 2010; Xu et al., 2020). Agar-agar and carrageenan, the two macroalgae with a high hydrocolloid content, are frequently used as stabilizers, thickeners, and gelling agents in the food industry (Wells et al., 2017; Liao et al., 2021). Even though 68% of macroalgae-producing units rely on wild stock harvesting, macroalgae aquaculture (in fresh or salt water) is growing in several European nations and accounted in 2005 for 32% of the macroalgae-producing units (Spolaore et al., 2006).
In addition to being a source of bioactive substances like amino acids, polysaturated fatty acids, vitamins, and minerals for the enrichment of traditional foods, algae offer an intriguing possibility for meeting the rising demand for protein (Borowitzka, 1998; Matos et al., 2022). A growing number of people are using microalgae as food ingredients, whether to add flavour, texture or as a nutrient source. Arthrospira platensis (Spirulina) and Chlorella vulgaris are the most popular microalgae species, and they also serve as vegan protein sources because of their high protein content (50%–60% of dry weight), along with corresponding levels of essential amino acids and vitamin B12 content (Khan et al., 2005; Chronakis and Madsen, 2011; Chia et al., 2019).
Algae are being pursued as a functional ingredient due to the rapidly expanding healthy and plant-based food sector (Khan et al., 2005). Algae’s credibility as a potential source for novel food development is elevated by the numerous examples of new and sophisticated foods enriched with algae biomass, such as plant-based fish, meat, and dairy equivalents, as well as other innovative components (Ścieszka and Klewicka, 2018; Matos et al., 2022). Nestlé is one company that has collaborated with ingredient supplier Corbion to commercialize microalgae in plant-based products. While in July 2020, Unilever and the biotech start-up Algenuity announced a new partnership in which they are using microalgae Chlorella vulgaris in producing plant-based products (Castim, 2022). These microalgae are ideal because of their quick growth ability, and provision of nutritional benefits ranging from healthy lipids and antioxidants to a variety of vitamins and minerals and have a low environmental footprint (Castim, 2022).
Conventionally, algae proteins are extracted using aqueous, acidic, and alkaline procedures. This is followed by many centrifugation cycles and recovery methods, including ultrafiltration, precipitation, or chromatography (Kadam et al., 2017). Ascophyllum nodosum, Ulva species, and Laminaria digitata proteins have been successfully extracted using chemical extraction techniques, such as two-phase acid and alkali treatments, as shown in Table 1. However, the availability of the protein molecules, which can be significantly hampered by high viscosity and anionic cell-wall polysaccharides, such as alginates in brown seaweed and carrageenan in red seaweed, can have a major impact on the success of algae protein extraction (Fleurence et al., 1995).
Algae protein extraction can also be done using the following methods: (1) ultrasound-assisted extraction (UAE) which can be applied to food sources for a number of different purposes. These include increasing the bioactivity of phenolics and carotenoids through targeted hydroxylation, increasing the bioavailability of plant micronutrients, simultaneous extraction and encapsulation, quenching radical sonochemistry to prevent bioactive degradation, and improving the bioavailability of plant micronutrients (Fleurence et al., 1995; Barbarino and Lourenço, 2005); (2) pulsed electric field (PEF) where high electric currents are used to cause reversible or irreversible electroporation by piercing a cell wall or cell membrane (Vilkhu et al., 2008); (3) microwave-assisted extraction (MAE) which involves heating a material to remove moisture, which produces bubbles under high pressure that may burst and disrupt the contents of cells (Vanthoor-Koopmans et al., 2013; Barba et al., 2014).
As mentioned before, algae proteins have good nutritional value in terms of their protein content, amino acid purity, and nutritional acceptability (Chronakis and Madsen, 2011). Algae share similar functional properties with terrestrial plants, such as the capacity to gel, absorb water and fat, emulsify, and foam. The protein-rich microalgae Nostoc, Arthrospira (Spirulina), and Aphanizomenon have been staples of the human diet for thousands of years (Spolaore et al., 2006). Table 2 summarizes the protein content of algae per 100 mg. Both Arthrospira and Chlorella have high-quality proteins with well-balanced amino acid profiles that meet the WHO/FAO/UNU standards for the amount of essential amino acids that humans need (Kumar et al., 2021). While protein content per 100 mg is highest in Spirulina (Table 2).
Additionally, red algae varieties like Porphyra, Asparagopsis taxiformis, Gracilaria, Irish moss, and dulse; kombu, wakame, and Macrocystis; and green algae varieties Caulerpa racemosa, Codium, and Ulva have been consumed for a long time by people all over the world. These can be gathered from farms or outdoors (Conte and Payri, 2006; Sá Monteiro et al., 2019; Lozano Muñoz and Díaz, 2020; Kumar et al., 2021) and can be eaten fresh, dried, or pickled (Borowitzka, 1998). Hydrocolloids, like agar-agar and carrageenan, that are used as stabilizers, thickeners, and gelling agents in food products are also derived from many macroalgae (Häder, 2021).
Algae can also be used in a variety of ways in the food processing industry. It is used as a gelling agent, raw food, cooked ingredient, texture enhancer, and vegan egg substitute. Furthermore, it is added to products to improve their nutritional content (Ścieszka and Klewicka, 2018) and because they contain a significant amount of superior-quality protein, algae contribute important nutrients to the human diet. Algae proteins also have a good nutritional value in terms of protein amount, amino acid purity, and nutritional acceptability (Chronakis and Madsen 2011). Protein-rich microalgae Nostoc, Arthrospira (Spirulina), and Aphanizomenon have been staples of the human diet over the course of human history (Conte and Payri, 2006; Vanthoor-Koopmans et al., 2013).
As a source of bulk proteins, microalgae are a relatively new idea. Generally, microalgae-based proteins have several advantages over currently used protein sources, that could significantly contribute to meeting Europe’s population protein requirements. Moreover, microalgae-based proteins require less land than animal-based proteins. For instance, they require approximately 2.5 m2 of land per kilogram of protein as opposed to 47–64 m2, 42–52 m2, and 144–258 m2 for the production of pork, chicken and beef, respectively, (Caporgno and Mathys, 2018). Compared to plant-based alternatives seems to be also favorable for algae, whereas nuts nees 7.9 m2, pulses 7.3 m2, grains 4.6m2 and peas 3.4m2 (Poore and Nemecek, 2018).
Arthrospira and Chlorella both boast high-quality proteins and well-balanced amino acid profiles which meet the WHO/FAO/UNU standards that humans need (Barba et al., 2014). In actuality, both these species of microalgae have amino acid profiles that are comparable to those of other conventional protein sources like eggs and soybeans. However, plants and microalgae typically lack the sulfur-rich amino acids methionine and cysteine (Bikker et al., 2016). Additional studies show that some microalgae supplements are deficient in other amino acids (Becker, 2007).
Only a small percentage of microalgae and microbes are currently consumed as food. However, because of their rapid growth and independence from arable agricultural land, algae can offer an intriguing substitute for traditional crops (Becker, 2007). Due to their abundance in vitamins, beta-carotene, polyunsaturated fatty acids, and other nutrients, single-cell organisms like Dunaliella, Spirulina, and Chlorella, their potential for use in the food and feed industries is enormous (Ritala et al., 2017).
To measure the concentration of protein and amino acids in algae, nitrogen-to-protein conversion is often used. The total nitrogen-to-protein (NTP) conversion factors 6.25 and 5 are frequently used to estimate the protein concentration in algae based on the assumption that the majority of the nitrogen in them is present as protein. However, it was found that the most appropriate NTP conversion factor is 5, as the NTP conversion factor of 6.25 has been found to overestimate protein by 43% (Lourenco et al., 2002; Angell et al., 2015; Wells et al., 2017). For instance, in one study, the conversion factor for Chlorella vulgaris (walled) crude biomass was determined to be 6.35, yet it was 5.96 based on direct protein extracts (Lourenco et al., 2002). Similar studies on 19 tropical marine algae produced even lower average factors, 4.59 for red algae, 5.13 for green algae, and 5.38 for brown algae (Angell et al., 2015). This may be because tropical surface waters receive less nitrogen at specific times of the year. The fact that these ratios change with the seasons based on the amino acid composition, highlights the need for protein and amino acid studies to determine the seasonal optimum for harvesting among algae foods (Zhou et al., 2012).
4 Algae food production in Europe
Many industries, including the agri-horticultural industry, the food and beverage industry, pharmaceuticals, nutraceuticals, and cosmeceuticals use biomass derived from microalgae and macroalgae as a source of chemical constituents. The extraction of valuable ingredients for nutraceuticals and dietary supplements is one of the frequent uses of algae biomass in Europe. The distribution of businesses involved in the utilization of biomass for food production is shown in Table 3 which is based on data from Araujo et al. (2021). Although there was only a small number of companies producing algae for food in Europe in 2020, there is a significant potential for growth.
According to the number of companies in Table 3, microalgae is the preferred type of algae for food production in Europe. Spirulina producers are also primarily found in France, Italy, Germany and Spain, as shown by the figure. The number of macroalgae food production units is highest in France, Norway, Denmark, Ireland, and Spain, while the number of microalgae food production units is highest in France, Germany, the Netherlands, Spain, and Italy. It is also critical to assess the proportion of businesses in the European algae sector that are engaged in the production of food from algae (Figure 1).
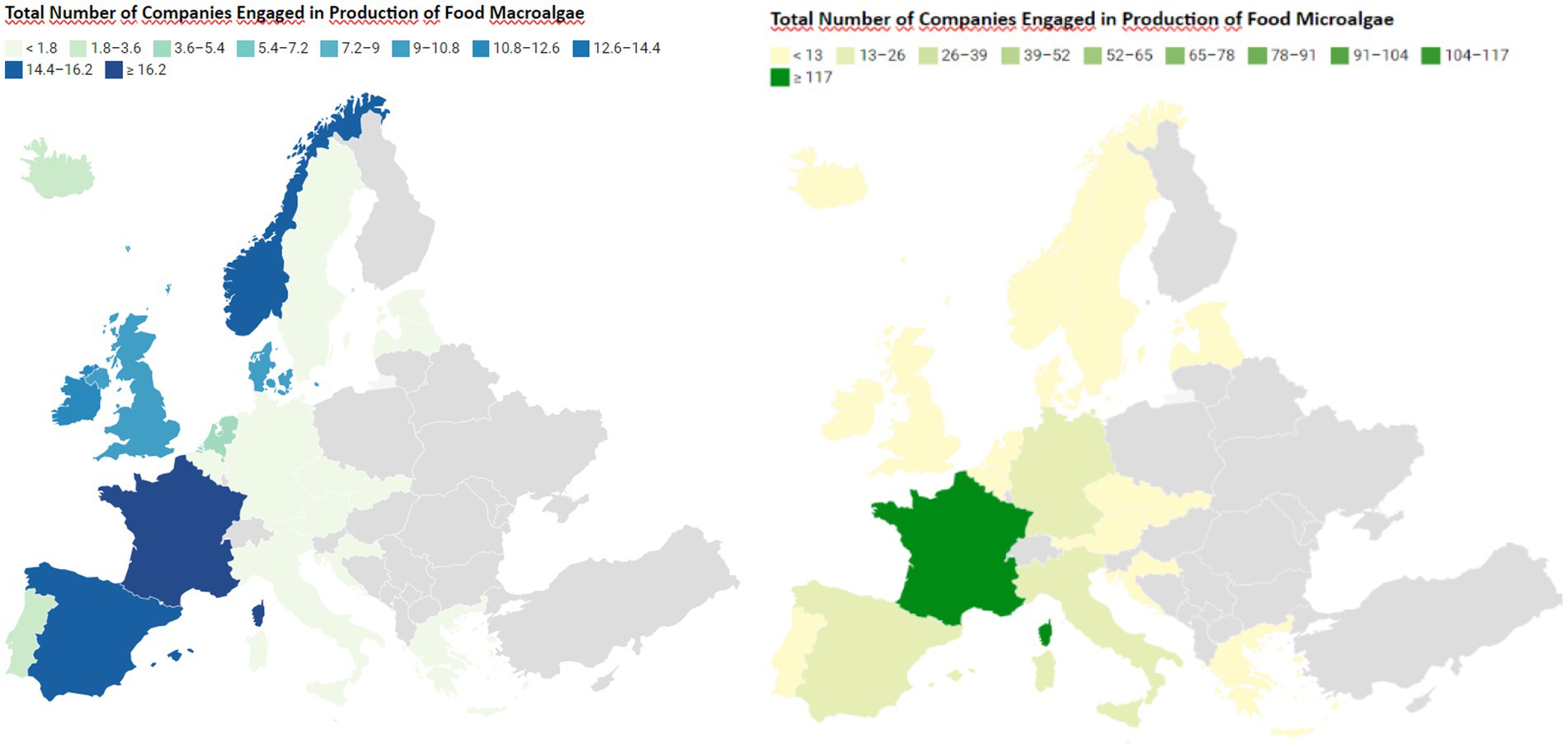
Figure 1. Total number of companies engaged in production of food macro/micro algae. Source of data: Araujo et al. (2021) own processing.
Based on information from Araujo et al. (2021), Figure 2 demonstrates that Spirulina has the highest proportion of food-producing businesses out of all the businesses in the algae sector. This demonstrates that Spirulina is preferred in the European algae market. The share of businesses that produce food is typically under 20% in the macroalgae sector. This highlights the possibility of raising food production in Europe. According to the Figure 2, microalgae are the least preferred type of biomass food production.
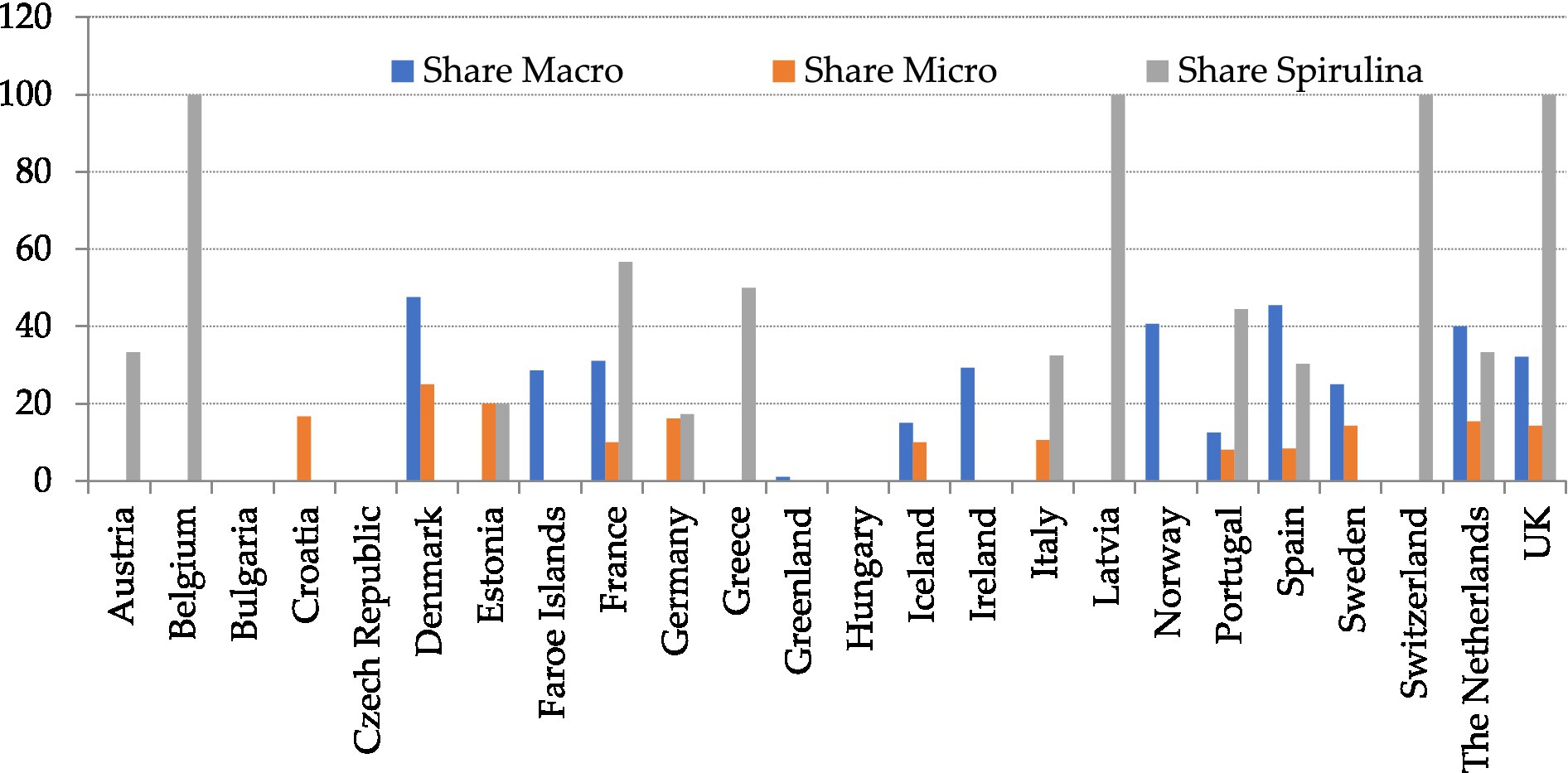
Figure 2. Share of firms in food production on total number of firms in the algae sector—various types of algae (Europe, 2020). Source of data: Araujo et al. (2021).
Although there are few businesses in the algae food production, the market for protein in Europe as a whole was worth about USD 193.271 million as at 2022. The predictions shown in Figure 3 were made using a trendline chart and historical data.
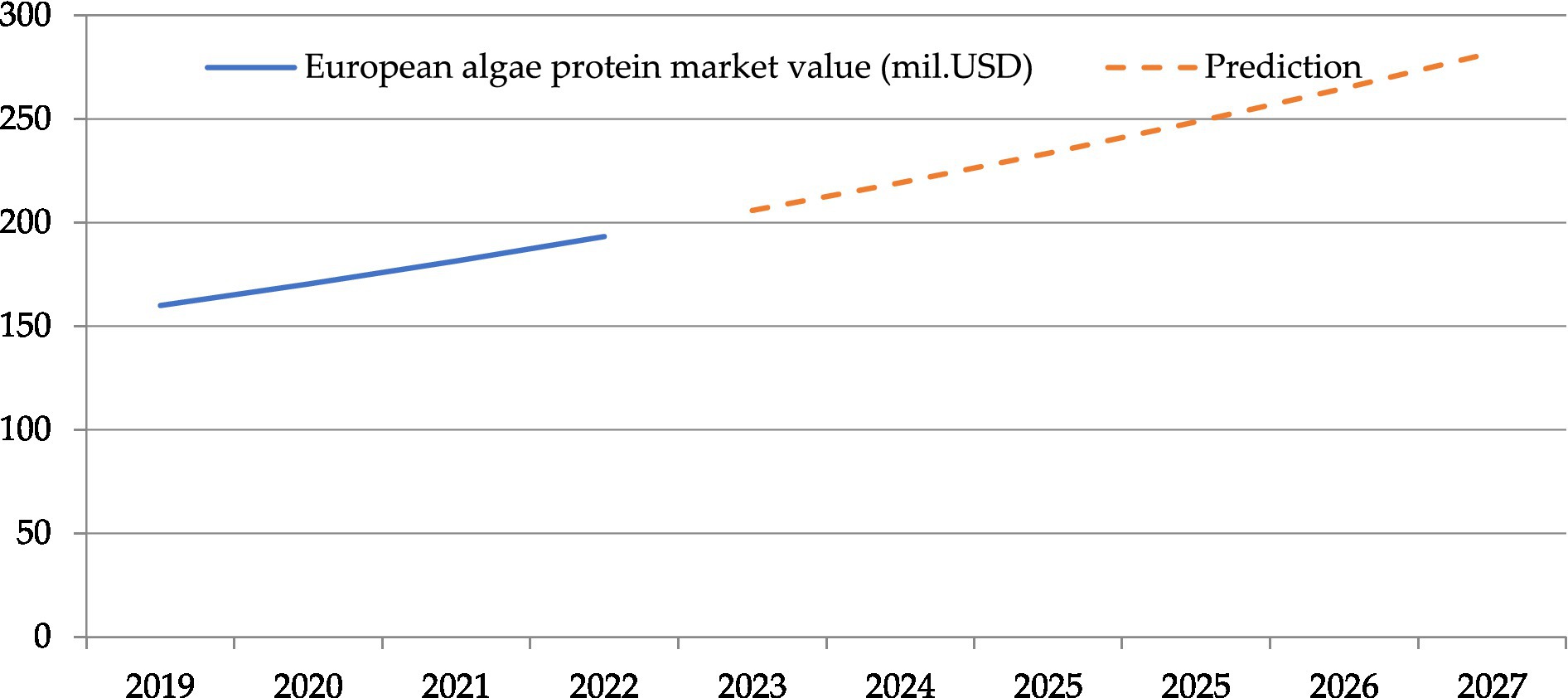
Figure 3. Europe algae protein market and its forecast from 2020–2026. Source of data: European Commission (2022).
The size of the European algae protein market exceeded USD 193 million in 2022 and is projected to increase, with a potential to reach almost USD 300 million by 2027. While the data for turnover specifically for the food industry is not available, the total turnover of firms in the algae industry in Europe is presented in Figure 4 (European Commission, 2022).
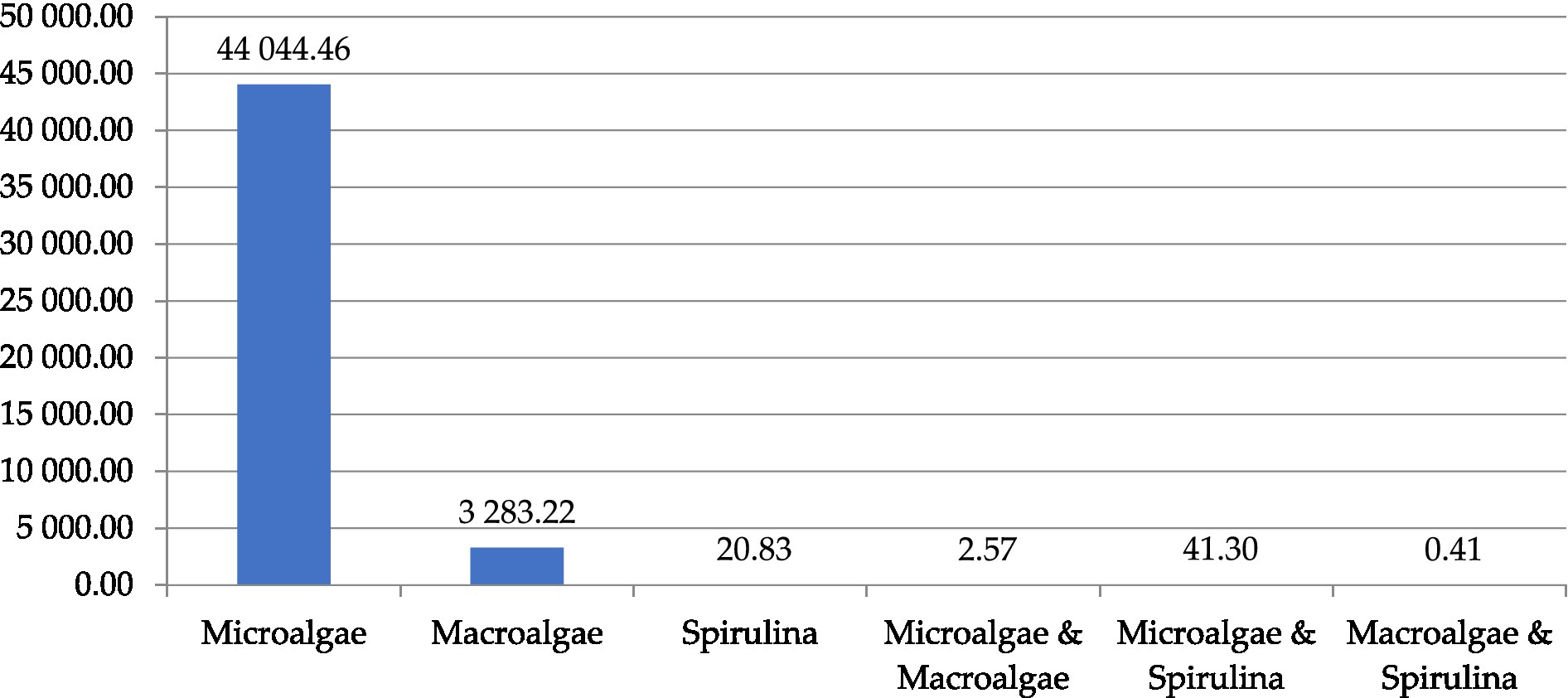
Figure 4. Algae production turnover firms in Europe (2016–2021) in million US$. Source of data: Araujo et al. (2021).
Spirulina in some countries is used almost exclusively for food production (on average approximately 40%), and the turnover of firms in the food industry is approximately US$ 8 million (European Commission, 2022). Biomass from microalgae and macroalgae is globally recognized as a source of chemical constituents with different applications, such as in the agri-horticultural sector, as human food and food ingredients, as nutraceuticals, cosmeceuticals, and pharmaceuticals (Smetana et al., 2017). In Europe, algae biomass is generally used for applications involving food and food-related goods, such as the extraction of valuable ingredients for nutraceuticals and dietary supplements (Spolaore et al., 2006).
Furthermore, the European Commission began an online consultation period on its roadmap in 2020 with the title “Blue Economy: Towards a Strong and Sustainable Algae Sector,” to enhance the EU’s algae sustainable production, safe consumption, and algae innovation. The main goal of the initiative was to assist The EU in achieving the European Green Deal, which included a post-CO2 recovery and the creation of a green, circular, and carbon-neutral EU (Zhou et al., 2012).
According to reports, there is a rising market for edible marine algae and seaweeds in Europe. Seaweeds have a variety of qualities that can satisfy the needs of European consumers. Rising demand for alternative proteins is driving growth in the edible seaweed market in Europe. However, many technological, regulatory, and market-related obstacles continue to restrict the production of algae in Europe. Additionally, the problems with society and the environment are still not sufficiently addressed (Becker, 2007).
5 Challenges of algae production in Europe
Accelerating microalgae growth and product synthesis, dehydrating algae cultures to produce biomass, pretreating biomass, and improving the fermentation process in the case of algae bioethanol production are the most common and significant challenges associated with algae production (Ritala et al., 2017). Being a relatively new field, protein extraction from algae like seaweed and microalgae is currently not feasible on a large scale (Harnedy and Fitzgerald, 2011; Bleakley and Hayes, 2017).
Algae production in Europe is also hampered by stringent regulations. Europe has strict legal restrictions for the safest possible exposure to heavy metals in foods intended for human consumption, such as mercury, arsenic, lead, and cadmium. Additionally, because algae supplements are not governed by any regulations, there are significant variations between batches even from the same producer (Görs et al., 2009). Moreover, issues around quality and contamination during the production and processing phases are continually being brought up (GENIALG, 2021).
Large-scale cultivation of microalgae and cyanobacteria frequently results in serious problems with biological contamination because the contaminants can easily enter the environment through water or air (Zhu et al., 2020; Cai et al., 2021).
There are still many challenges faced by the sector that can be summarized according to García et al. (2017) and represented in Table 3. The challenges are divided into environmental, economic, policy-related and technological.
Potential solutions to environmental challenges are presented in the Table 4. When considering how food algae production in Europe will develop in the future, it is important to take them into account.
Using renewable energy sources for algae cultivation can reduce the carbon footprint of the process.
To encourage the use of algae for production and nutrition throughout Europe, a European algae stakeholder platform (EU4 Algae), was established in 2021, it is a 3 years project. The project prompted a collaboration of the European Commission, sustainability consultants, the European Climate, Infrastructure, as well as other algae organizations including the European Union’s umbrella organizations like the European Union for the production of yeast, algae, and insects. The platform also aims to urge EU consumers and businesses to use algae for nutrition and other purposes and speed up the development of a European algae sector (European Commission, 2021). The collaborative roadmap is focused on four key pillars: opening up regulatory opportunities, easing access to green investments, assisting with Research and Development initiatives, and creating joint promotion and communication activities (Araujo et al., 2021).
Noteworthy, the European Commission has funded several projects under its Horizon 2020 programme that focuses on advancing algae production technologies, improving algae biomass quality and developing new algae products and applications. Some examples of these projects are Algae for a Billion (ALGAE4A-B), Sustainable Algae Biorefinery for Agriculture and Aquaculture (SABANA) and Valuable Products from Algae Using New Magnetic Cultivation and Extraction Techniques (Araujo et al., 2021).
In developing standards and guidelines for algae production, the European Committee for Standardization (CEN) established a technical committee (CEN/TC454) that is responsible for developing standards and guidelines for algae cultivation, harvesting, processing and quality control. These standards and guidelines will help ensure the safety, quality and sustainability of algae products in Europe (European Commission, 2021).
6 Conclusion and discussion
The studies reviewed show that the nutritional value of food products can be increased by using algae. The production of algae for food should be encouraged in Europe because it is a viable alternative protein source (Chronakis and Madsen, 2011; Lucas et al., 2018; Chia et al., 2019). Contrary to these studies, Araujo et al. (2021) did not demonstrate a linkage between algae and protein content. However, these findings were explained by the high variability of the amount of algae added during testing.
Beyond previous systematic literature reviews (Ścieszka and Klewicka, 2018; Lee et al., 2020), the authors identified relevant contexts between algae and environmental policy. The production and consumption of algae can support the goals of the European Commission’s Green Deal in the blue economy, biodiversity protection and climate neutrality. The public sector and governments should recognize algae farming as a development priority and use mechanisms to reward the environmental benefits of algae farming and processing. FAO is one of the supporters of sustainable development and works to educate and develop algae cultivation. Among other things, he is working on a technical platform that will facilitate capacity building, technology transfer and knowledge sharing in this area, as well as market development for the use of algae as human food.
As has been advocated in several research papers, algae can be grown in a variety of environments while requiring fewer resources than other protein sources like beef, pork and chicken (Häder, 2021).
The results indicate that Europe has considerable market growth potential to achieve. The growing popularity of algae is due to the demand for healthy, plant-based and vegan foods. The two main types of algae that can be used as protein sources in Europe are microalgae (especially Spirulina) and macroalgae, which are discussed in detail in this article. The largest number of producers is in the large countries of the European Union (France, Italy, Germany, and Spain). The use of algae has the potential to be profitable and sustainable. These baseline observations are consistent with published literature (García et al., 2017; Smetana et al., 2017; Boukid and Castellari, 2021).
In the Czech Republic, although this data is missing in Araujo et al. (2021), microscopic algae are grown for human nutrition in Třebon at the Algatech company. This workplace participated in the development of medicinal preparations from microalgae and was also involved in the Interkosmos program, when in the last century it tested the Czech Republic. Cosmonaut Vladimír Remek growing chlorella in space. Today, research focuses on the use of algae for nutrition and as food supplements (BusinessInfo.cz, 2019).
The present research confirmed technological factors that limit the algae sector (Bleakley and Hayes, 2017; Ritala et al., 2017; Galasso et al., 2019; Cai et al., 2021). There is a need for the development of new methods for processing and preserving algae for use in food as well as the creation of more efficient and cost-effective techniques for algae cultivation and harvesting. The proportions of protein and amino acids in algae vary according to geographical areas, seasons, and methods of harvesting, storage and processing. Of course, growing algae also has some environmental limitations. According to Cai et al. (2021), these are, for example, the spread of non-native species, the devastation of natural coastlines and, in the case of seaweed, the high consumption of drinking water for washing them. However, according to our study, the environmental benefits of algae cultivation prevail.
Despite the mentioned obstacles, the algae sector constitutes an opportunity to diversify food activities, fulfil consumer needs, and achieve current environmental sustainability goals.
This review has revealed several limitations and directions for future research. Future studies could focus in more detail on the environmental aspects of algae production. It would be interesting to investigate individual consumer needs and behavior across European countries. Finally, further research should consider legislative regulation and quality management of algae production and processing in the European Union and individual countries.
Data availability statement
The original contributions presented in the study are included in the article/supplementary material, further inquiries can be directed to the corresponding author.
Author contributions
JC: Conceptualization, Writing – original draft, Formal analysis, Investigation. PP: Formal analysis, Investigation, Methodology, Supervision, Writing – original draft. JA: Conceptualization, Writing – original draft, Writing – review & editing, Methodology. JS: Data curation, Formal analysis, Conceptualization, Writing – original draft. CO: Conceptualization, Formal analysis, Investigation, Writing – original draft. KM: Writing – original draft, Formal analysis, Investigation. LS: Methodology, Supervision, Writing – original draft, PS: Writing – original draft, Conceptualization, Formal analysis.
Funding
The author(s) declare financial support was received for the research, authorship, and/or publication of this article. This research was supported by the Czech University of Life Sciences Faculty of Economics and Management (2022B0005).
Conflict of interest
The authors declare that the research was conducted in the absence of any commercial or financial relationships that could be construed as a potential conflict of interest.
The author(s) declared that they were an editorial board member of Frontiers, at the time of submission. This had no impact on the peer review process and the final decision.
Publisher’s note
All claims expressed in this article are solely those of the authors and do not necessarily represent those of their affiliated organizations, or those of the publisher, the editors and the reviewers. Any product that may be evaluated in this article, or claim that may be made by its manufacturer, is not guaranteed or endorsed by the publisher.
References
Adluri, R. S., Zhan, L., Bagchi, M., Maulik, N., and Maulik, G. (2010). The effects of a novel plant-based calcium supplement compared to two common calcium salts on human osteoblast cell proliferation and mineralization. Mol. Cell. Biol. 340, 73–80. doi: 10.1007/s11010-010-0402-0
Angell, A. R., Mata, L., de Nys, R., and Paul, N. A. (2015). The protein content of seaweeds: a universal nitrogen-to-protein conversion factor of five. J. Appl. Phycol. 28, 511–524. doi: 10.1007/s10811-015-0650-1
Araujo, R., Vázquez Calderón, F., Sánchez López, J., Azevedo, I. C., Bruhn, A., Fluch, S., et al. (2021). The current state of the European algae production industry: an emerging sector of the blue bioeconomy. Front. Mar. Sci. 7:626389. doi: 10.3389/fmars.2020.626389
Arora, K., Kumar, P., Bose, D., Li, X., and Kulshrestha, S. (2021). Potential algae applications in biochemical and bioenergy sectors. 3 Biotech 11:296. doi: 10.1007/s13205-021-02825-5
Ashwathi, P. . (2021). Classification of algae Available at: https://www.biologydiscussion.com/algae/classification-algae/classification-of-algae/49912. (Accessed April 30, 2023).
Avila-Peltroche, J., and Padilla-Vallejos, J. (2020). The seaweed resources of Peru. Bot. Mar. 63, 381–394. doi: 10.1515/bot-2020-0026
Barba, F. J., Grimi, N., and Vorobiev, E. (2014). New approaches for the use of non-conventional cell disruption technologies to extract potential food additives and nutraceuticals from microalgae. Food Eng. Rev. 7, 45–62. doi: 10.1007/s12393-014-9095-6
Barbarino, E., and Lourenço, S. O. (2005). An evaluation of methods for extraction and quantification of protein from marine macro-and microalgae. J. Appl. Phycol. 17, 447–460. doi: 10.1007/s10811-005-1641-4
Barsanti, L., Passarelli, V., Evangelista, V., Frassanito, A. M., and Gualtieri, P. (2011). Chemistry, physico-chemistry and applications linked to biological activities of β-glucans. Nat. Prod. Rep. 28, 457–466. doi: 10.1039/c0np00018c
Becker, E. W. (2007). Micro-algae as a source of protein. Biotechnol. Adv. 25, 207–210. doi: 10.1016/j.biotechadv.2006.11.002
Bikker, P., van Krimpen, M. M., van Wikselaar, P., Houweling-Tan, B., Scaccia, N., van Hal, J. W., et al. (2016). Biorefinery of the green seaweed Ulva Lactuca to produce animal feed, chemicals and biofuels. J. Appl. Phycol. 28, 3511–3525. doi: 10.1007/s10811-016-0842-3
Bleakley, S., and Hayes, M. (2017). Algal proteins: extraction, application, and challenges concerning production. Foods 6, 1–34. doi: 10.3390/foods6050033
Borowitzka, M. A. (1998). “Algae as food” in Microbiology of fermented foods. ed. B. J. B. Wood (Boston: Springer), 585–602.
Boukid, F., and Castellari, M. (2021). Food and beverages containing algae and derived ingredients launched in the market from 2015 to 2019: a front-of-pack labeling perspective with a special focus on Spain. Foods 10, 1–14. doi: 10.3390/foods10010173
Brodie, J., Chan, C. X., Clerck, O. D., Cock, J. M., Coelho, S. M., Gachon, C., et al. (2017). The algal uprising. Trends Plant Sci. 22, 726–738. doi: 10.1016/j.tplants.2017.05.005
BusinessInfo.cz , (2019) Available at: https://www.businessinfo.cz/clanky/algatech-predstavi-na-expo-rasy-jako-tovarny-na-dulezite-latky/
Bygora (2021). Algae for food, Available at: https://bygora.com/2021/10/algae-for-food. (Accessed March 30, 2023).
Cai, J., Lovatelli, A., Aguilar-Manjarrez, J., Cornish, L., Dabbadie, L., Desrochers, A., et al. (2021). Seaweeds and microalgae: an overview for unlocking their potential in global aquaculture development. FAO fisheries and aquaculture circular No. 1229. Rome, FAO.
Caporgno, M. P., and Mathys, A. (2018). Trends in microalgae incorporation into innovative food products with potential health benefits. Front. Nutr. 5:58. doi: 10.3389/fnut.2018.00058
Castim, D. . (2022). Nestlé and Corbion to explore microalgae ingredients for plant-based products. Available at: https://worldbiomarketinsights.com/nestle-and-corbion-to-explore-microalgae-ingredients-for-plant-based-products/ (Accessed June 7, 2023)
Chia, S. R., Chew, K. W., Zaid, H. F. M., Chu, D. T., Tao, Y., and Show, P. L. (2019). Microalgal protein extraction from Chlorella vulgaris FSP-E using triphasic partitioning technique with sonication. Front. Bioeng. Biotechnol. 7:396. doi: 10.3389/fbioe.2019.00396
Chronakis, I. S., and Madsen, M. (2011). “Algal proteins” in Handbook of food proteins. eds. G. O. Phillips and P. A. Williams (Sawston, United Kingdom: Woodhead Publishing Limited), 353–394.
Conte, E., and Payri, C. (2006). Present day consumption of edible algae in French Polynesia: a study of the survival of pre-European practices. J. Polynes. Soc. 115, 77–93.
Diaz, C. J., Douglas, K. J., Kang, K., Kolarik, A. L., Malinovski, R., Torres-Tiji, Y., et al. (2023). Developing algae as a sustainable food source. Front. Nutr. 9:3147. doi: 10.3389/fnut.2022.102984
European Commission (2021). Algae production industry in Europe. Available at: https://knowledge4policy.ec.europa.eu/bioeconomy/algae-production-industry-europe_en (Accessed June 30, 2023).
European Commission (2022). An overview of the algae industry in Europe–producers, production systems, species, biomass uses, other steps in the value chain and socio-economic data. Publications Office of the European Union. Available at: https://data.europa.eu/doi/10.2760/813113
Filippini, M., Baldisserotto, A., Menotta, S., Fedrizzi, G., Rubini, S., Gigliotti, D., et al. (2021). Heavy metals and potential risks in edible seaweed on the market in Italy. Chemosphere 263:127983. doi: 10.1016/j.chemosphere.2020.127983
Fleurence, J., Le Coeur, C., Mabeau, S., Maurice, M., and Landrein, A. (1995). Comparison of different extractive procedures for proteins from the edible seaweeds Ulva rigida and Ulva rotundata. J. Appl. Phycol. 7, 577–582. doi: 10.1007/bf00003945
Galasso, C., Gentile, A., Orefice, I., Ianora, A., Bruno, A., Noonan, D. M., et al. (2019). Microalgal derivatives as potential nutraceutical and food supplements for human health: a focus on cancer prevention and interception. Nutrients 11, 1–22. doi: 10.3390/nu11061226
García, J. L., de Vicente, M., and Galán, B. (2017). Microalgae, old sustainable food and fashion nutraceuticals. Microb. Biotechnol. 10, 1017–1024. doi: 10.1111/1751-7915.12800
GENIALG (2021). European Commission seeks views on sustainable algae production, consumption and innovative uses. Available at: https://genialgproject.eu/2021/01/07/european-commission-seeks-views-on-sustainable-algae-productioSn-consumption-and-innovative-uses (Accessed April 25, 2023).
Görs, M., Schumann, R., Hepperle, D., and Karsten, U. (2009). Quality analysis of commercial Chlorella products used as dietary supplement in human nutrition. J. Appl. Phycol. 22, 265–276. doi: 10.1007/s10811-009-9455-4
Häder, P. (2021). Phycocolloids from macroalgae, Natural bioactive compounds. Cambridge, Massachusetts, USA: Academic Press. 187–201.
Harnedy, P. A., and Fitzgerald, R. J. (2011). Bioactive proteins, peptides, and amino acids from macroalgae 1. J. Phycol. 47, 218–232. doi: 10.1111/j.1529-8817.2011.00969.x
Kadam, S. U., Álvarez, C., Tiwari, B. K., and O’Donnell, C. P. (2017). Extraction and characterization of protein from Irish Brown seaweed Ascophyllum nodosum. Food Res. Int. 99, 1021–1027. doi: 10.1016/j.foodres.2016.07.018
Khan, Z., Bhadouria, P., and Bisen, P. (2005). Nutritional and therapeutic potential of Spirulina. Curr. Pharm. Biotechnol. 6, 373–379. doi: 10.2174/138920105774370607
Kumar, Y., Tarafdar, A., and Badgujar, P. C. (2021). Seaweed as a source of natural antioxidants: therapeutic activity and food applications. J. Food Qual. 2021, 1–17. doi: 10.1155/2021/5753391
Leandro, A., Pacheco, D., Cotas, J., Marques, J. C., Pereira, L., and Gonçalves, A. M. (2020). Seaweed’s bioactive candidate compounds to food industry and global food security. Life 10:140. doi: 10.3390/life10080140
Lee, X. J., Ong, H. C., Gan, Y. Y., Chen, W.-H., and Mahlia, T. M. I. (2020). State-of-the-art review on conventional and advanced pyrolysis of macroalgae and microalgae for biochar, bio-oil, and bio-syngas production. Energy Convers. Manag. 210:112707. doi: 10.1016/j.enconman.2020.112707
Liao, Y.-C., Chang, C.-C., Nagarajan, D., Chen, C.-Y., and Chang, J.-S. (2021). Algae-derived hydrocolloids in foods: applications and health-related issues. Bioengineered 12, 3787–3801. doi: 10.1080/21655979.2021.1946359
Li-Beisson, Y., Thelen, J. J., Fedosejevs, E., and Harwood, J. L. (2019). Eukaryotic algae lipid biochemistry. Prog. Lipid Res. 74, 31–68. doi: 10.1016/j.plipres.2019.01.003
Lourenco, S. O., Barbarino, E., De-Paula, J. C., Pereira, L. O., and Marquez, U. M. L. (2002). Amino acid composition, protein content and calculation of nitrogen-to-protein conversion factors for 19 tropical seaweeds. Phycol. Res. 50, 233–241. doi: 10.1111/j.1440-1835.2002.tb00156.x
Lozano Muñoz, I., and Díaz, N. F. (2020). Minerals in edible seaweed: health benefits and food safety issues. Crit. Rev. Food Sci. Nutr. 62, 1592–1607. doi: 10.1080/10408398.2020.1844637
Lucas, B. F., de Morais, M. G., Santos, T. D., and Costa, J. A. V. (2018). Spirulina for snack enrichment: nutritional, physical and sensory evaluations. LWT 90, 270–276. doi: 10.1016/j.lwt.2017.12.032
Matos, Â. P., Novelli, E., and Tribuzi, G. (2022). Use of algae as food ingredient: sensory acceptance and commercial products. Front. Food Sci. Technol. 2:989801. doi: 10.3389/frfst.2022.989801
Morales-Sánchez, D., Martinez-Rodriguez, O. A., and Martinez, A. (2017). Heterotrophic cultivation of microalgae: production of metabolites of commercial interest. J. Chem. Technol. Biotechnol. 92, 925–936. doi: 10.1002/jctb.5115
Piccolo, A. , (2012), Spirulina a livelihood and a business venture, Available at: www.fao.org/3/a-az386e.pdf.
Poore, J., and Nemecek, T. (2018). Reducing food’s environmental impacts through producers and consumers. Science 360, 987–992. doi: 10.1126/science.aaq0216
Ritala, A., Häkkinen, S. T., Toivari, M., and Wiebe, M. G. (2017). Single cell protein—state-of-the-art, industrial landscape and patents 2001–2016. Front. Microbiol. 8:2009. doi: 10.3389/fmicb.2017.02009
Sá Monteiro, M., Sloth, J., Holdt, S., and Hansen, M. (2019). Analysis and risk assessment of seaweed. EFSA J. 17:e170915. doi: 10.2903/j.efsa.2019.e170915
Sáez, M. I., Galafat, A., Vizcaíno, A. J., Chaves-Pozo, E., Ayala, M. D., Arizcun, M., et al. (2022). Evaluation of Nannochloropsis gaditana raw and hydrolysed biomass at low inclusion level as dietary functional additive for gilthead seabream (Sparus aurata) juveniles. Aquaculture 556:738288. doi: 10.1016/j.aquaculture.2022.738288
Ścieszka, S., and Klewicka, E. (2018). Algae in food: a general review. Crit. Rev. Food Sci. Nutr. 59, 3538–3547. doi: 10.1080/10408398.2018.1496319
Smetana, S., Sandmann, M., Rohn, S., Pleissner, D., and Heinz, V. (2017). Autotrophic and heterotrophic microalgae and Cyanobacteria cultivation for food and feed: life cycle assessment. Bioresour. Technol. 245, 162–170. doi: 10.1016/j.biortech.2017.08.113
Spolaore, P., Joannis-Cassan, C., Duran, E., and Isambert, A. (2006). Commercial applications of microalgae. J. Biosci. Bioeng. 101, 87–96. doi: 10.1263/jbb.101.87
Stengel, D. B., and Connan, S. (2015). “Marine algae: a source of biomass for biotechnological applications” in Natural products from marine algae. methods in molecular biology (New York, NY: Humana Press), 1–37.
Sun, H., Zhao, W., Mao, X., Li, Y., Wu, T., and Chen, F. (2018). High-value biomass from microalgae production platforms: carbon metabolism and energy conversion strategies and progress. Biotechnol. Biofuels 11, 227–223. doi: 10.1186/s13068-018-1225-6
Ulvskov, P., Paiva, D. S., Domozych, D., and Harholt, J. (2013). Classification, naming and evolutionary history of glycosyltransferases from sequenced green and red algal genomes. PLoS One 8:e76511. doi: 10.1137/journal.pone.0076511
Vanthoor-Koopmans, M., Wijffels, R. H., Barbosa, M. J., and Eppink, M. H. M. (2013). Biorefinery of microalgae for food and fuel. Bioresour. Technol. 135, 142–149. doi: 10.1016/j.biortech.2012.10.135
Vilkhu, K., Mawson, R., Simons, L., and Bates, D. (2008). Applications and opportunities for ultrasound assisted extraction in the food industry—a review. Innovative Food Sci. Emerg. Technol. 9, 161–169. doi: 10.1016/j.ifset.2007.04.014
Wells, M. L., Potin, P., Craigie, J. S., Raven, J. A., Merchant, S. S., Helliwell, K. E., et al. (2017). Algae as nutritional and functional food sources: revisiting our understanding. J. Appl. Phycol. 29, 949–982. doi: 10.1007/s10811-016-0974-5
Xu, Y., Ye, J., Zhou, D., and Su, L. (2020). Research progress on applications of calcium derived from marine organisms. Sci. Rep. 10:18425. doi: 10.1038/s41598-020-75575-8
Zhou, C., Yu, X., Zhang, Y., He, R., and Ma, H. (2012). Ultrasonic degradation, purification and analysis of structure and antioxidant activity of polysaccharide from Porphyra yezoensis Udea. Carbohydr. Polym. 87, 2046–2051. doi: 10.1016/j.carbpol.2011.10.026
Zhu, Z., Jiang, J., and Fa, Y. (2020). Overcoming the biological contamination in microalgae and Cyanobacteria mass cultivations for photosynthetic biofuel production. Molecules 25, 1–13. doi: 10.3390/molecules25225220
Keywords: algae, macro-algae, micro-algae, Spirulina, protein, nutrition
Citation: Procházka P, Abrham J, Cerveny J, Soukupová J, Ouma CN, Mullen KJ, Sanova P and Smutka L (2023) Algae as a source of protein in the sustainable food and gastronomy industry. Front. Sustain. Food Syst. 7:1256473. doi: 10.3389/fsufs.2023.1256473
Edited by:
Monica Trif, Centre for Innovative Process Engineering, GermanyReviewed by:
João Cotas, University of Coimbra, PortugalVeronika Zidova, University of Hradec Králové, Czechia
Andrea Cajkova, University of St. Cyril and Methodius, Slovakia
Copyright © 2023 Procházka, Abrham, Cerveny, Soukupová, Ouma, Mullen, Sanova and Smutka. This is an open-access article distributed under the terms of the Creative Commons Attribution License (CC BY). The use, distribution or reproduction in other forums is permitted, provided the original author(s) and the copyright owner(s) are credited and that the original publication in this journal is cited, in accordance with accepted academic practice. No use, distribution or reproduction is permitted which does not comply with these terms.
*Correspondence: Josef Abrham, abrhamj@pef.czu.cz