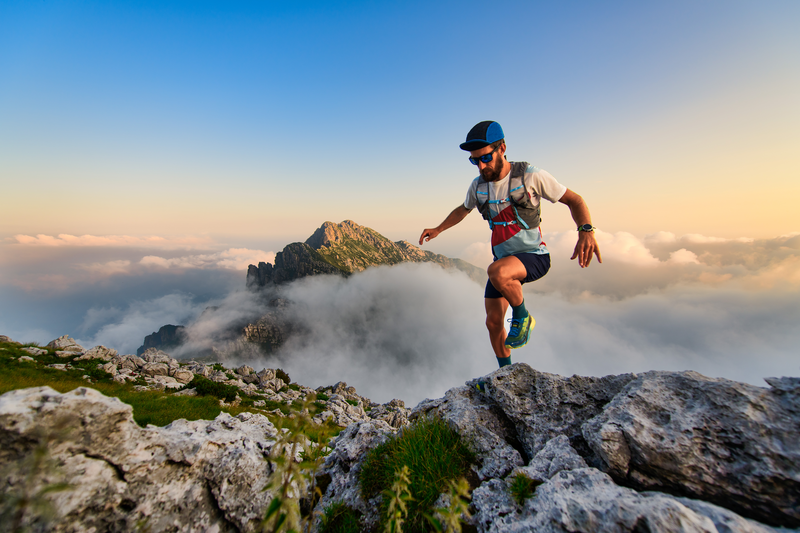
94% of researchers rate our articles as excellent or good
Learn more about the work of our research integrity team to safeguard the quality of each article we publish.
Find out more
ORIGINAL RESEARCH article
Front. Sustain. Food Syst. , 16 November 2023
Sec. Sustainable Food Processing
Volume 7 - 2023 | https://doi.org/10.3389/fsufs.2023.1255941
This article is part of the Research Topic High Value Utilization of Waste in Food Processing View all 7 articles
At present in Peru, a substantial quantity of avocado by-products (peel and seed) is produced due to avocado processing. It is essential to revalue these products. The meticulous selection of parameters in solid–liquid extraction through maceration, which is the industrial preference, is crucial to obtain a greater recovery of phenolic compounds from avocado Hass peels. Therefore, the aim of this study was to assess the impact of process variables (ethanol concentration, temperature and solvent:feed (S/F) ratio) on the global yield of extraction (GY), total phenolic content (TPC), total flavonoid content (TFC), and antioxidant capacity (DPPH) of avocado Hass peel extract at two different stages of maturity. Furthermore, the quantification of phenolic compounds was using High Performance Liquid Chromatography with Diode Array Detection (HPLC-DAD) and antioxidant capacity using ABTS and FRAP assays were determined in higher yielding extracts. The dry matter content (dm) was determined in the pulp of unripe avocados (UA, 22.57% dm) and ripe avocados (RA, 27.30% dm). The results showed that, for all treatments, ethanol concentration, temperature, S/F ratio had a significant influence (p < 0.05) on GY, TPC, TFC and antioxidant capacity, and the UA peel extracts obtained with 40% ethanol, 49.3°C, S/F ratio (14.3 mL/g) and 60 min showed the highest values of TPC (44.24 mg GAE/g peel dw), TFC (786.08 mg QE/g peel dw) and antioxidant capacity against DPPH (564.82 μmTE/g peel dw), ABTS (804.40 μmTE/g peel dw) and FRAP (1006.21 μmTE/g peel dw). Meanwhile, for the HPLC-DAD analysis, vanillic acid and 4-hydroxyphenylacetic acid are the main phenolic compounds present in avocado peel extracts. The results of this research indicate that avocado peels are a source of natural phenolic components, with potential application in the food industry as a viable alternative to synthetic antioxidants, thus reducing their use.
One of the major current global problems is the generation of by-products from global the agro-industrial sector on a global scale. By 2010, 140 billion tons were already being produced annually, and in recent years approximately 600 million tons of fruit by-products have been generated worldwide. The by-products produced, which are considered to possesses low commercial value, exhibit a diverse variety, including peels, seeds, leaves, roots, stems, bark, bagasse, pulp, pomace, among others. One of the main concerns about these by-products is their disposal into the environment without any form of treatment, thereby posing an environmental hazard due to their high biodegradability. The biological degradation of plant by-products is the third anthropogenic contribution to atmospheric methane emissions (Banerjee et al., 2017; Mazzutti et al., 2021; Šelo et al., 2021). This process not only has environmental implications but it also has economic consequences, with estimated annual losses of approximately 940,000 million dollars (Martínez-Inda et al., 2023). In certain cases, these by-products are used for animal feed or as an ingredient in the production of animal diets (Mazzutti et al., 2021). Faced with this scenario, decisive changes in the food industry management system must be implemented within the context of the circular economy to prevent, reuse or recover the by-products by this sector (Del Rio Osorio et al., 2021; Martínez-Inda et al., 2023). In this context, the avocado processing industry is no stranger to this reality.
Avocado is one of the most produced and consumed fruits globally, and its demand has grown significantly in recent years (Rodríguez-Martínez et al., 2021; Bangar et al., 2022). Peru is the third largest avocado producer worldwide and annually yields 672,232 thousand tons (FAOSTAT, 2020). The processed avocado market will rise from US$1.70 billion in 2018 to approximately US$2.70 billion by 2024 (Ramos-Aguilar et al., 2021; Nyakangi et al., 2023). During avocado processing, the pulp is used for oil extraction, paste production, and other products. Approximately 2.42 million tons of by-products such as peel and seed are generated, which are typically discarded into the environment (Nyakangi et al., 2023). Hass variety peel represents 11–17% of the weight of the fruit (Wang et al., 2010; Rodríguez-Carpena et al., 2011; Calderón-Oliver et al., 2016; Araújo et al., 2021) and several studies indicate that it contains bioactive compounds such as organic acids (citric acid and quinic acid), phenolic acids and phenolic alcoholic derivatives (gallic acid, 5-O-caffeoylquinic acid, 3,4-dihydroxybenzoic acid, 4-hydroxybenzoic acid, syringic acid, O-caffeoylquinic acid, 5-O-caffeoyoylquinic acid, tyrosol-glucosyl-pentoside and 4-O-caffeoylquinic acid), flavonoids (rutin, quercetin-diglucoside, luteolin 7-O-(2″-O-pentosyl) hexoside, quercetin-O-arabinosyl-glucoside, quercetin glucuronide, quercetin, multinoside A, naringenin, quercetin-xylosyl-rhamnoside, kaempferol-O-glucosyl-rhamnoside, quercetin-3-β-glucoside, kaempferol and quercetin-3-O-arabinoside), catechins and procyanidins [(+)-catechin, (−)-epicatechin, procyanidin A and procyanidin B], also including β-sitosterol, α-tocopherol, cyanidin-3-glucoside, perseitol, chlorophyll a and b, lutein and volemitol (Wang et al., 2010; Rodríguez-Carpena et al., 2011; Kosińska et al., 2012; López-Cobo et al., 2016; Figueroa et al., 2018; Melgar et al., 2018; Tremocoldi et al., 2018; Araújo et al., 2021; Figueroa et al., 2021; Ramos-Aguilar et al., 2021; Rodríguez-Martínez et al., 2021; Trujillo-Mayol et al., 2021; Rojas-García et al., 2022; Martínez-Gutiérrez, 2023). Furthermore, several studies have demonstrated that the extract derived from the avocado peel antioxidant and antimicrobial properties (Raymond Chia and Dykes, 2010; Kosińska et al., 2012; Melgar et al., 2018; Tremocoldi et al., 2018; Figueroa et al., 2021; Ferreira and Santos, 2022). Additionally, it exhibits neuroprotective effects (Ortega-Arellano et al., 2019), which makes this by-product another alternative for obtaining phenolic compounds that are of interest to the food, pharmaceutical and cosmetic industries. Particularly, the food industry can use it as a nutraceutical or functional food ingredient, while promoting the utilization of avocado waste, a latent concern of the circular economy (Nyakangi et al., 2023).
The extraction of phenolic compounds from avocado Hass peel can be obtained using non-conventional technologies such as microwave-assisted extraction, ultrasound-assisted extraction, pressurized liquid extraction, and two-phase aqueous system (Del Castillo-Llamosas et al., 2021). These technologies have advantages (green, economical, processes can be completed quickly with high reproducibility, lower solvent consumption that minimizes environmental impact, higher extract purity and lower energy consumption) (Chemat et al., 2019), however, these technologies are expensive and require specialized equipment, therefore conventional methods such as maceration are still preferred in industry (Martínez-Gutiérrez, 2023), due to the combination of simplicity (easy manual handling), basal pressure (ambient conditions) and possibly mild temperature (Gil-Martín et al., 2022). Regarding the obtaining of phenolic compounds from Hass variety avocado peel by maceration, studies were conducted using fixed extraction parameters and organic solvents such as 80% methanol (Kosińska et al., 2012; López-Cobo et al., 2016; Figueroa et al., 2018, 2021), 80% acetone (Widsten et al., 2014; Figueroa et al., 2018, 2021), 70% acetone (Saavedra et al., 2017), acetone/water/acetic acid (70:29.7:0.3, v/v/v/v) (Wang et al., 2010), ethyl acetate, acetone/water (70:30 v/v) or methanol/water (70:30 v/v) (Rodríguez-Carpena et al., 2011), methanol with 0.10% trifluoroacetic acid (Ramos-Aguilar et al., 2021) and also using generally recognized as safe (GRAS) such as absolute ethanol (Raymond Chia and Dykes, 2010; Ferreira and Santos, 2022), 95% ethanol (Bowen et al., 2018), 80% ethanol (Melgar et al., 2018; Tremocoldi et al., 2018; Figueroa et al., 2021; Trujillo-Mayol et al., 2021), 60% ethanol (Rojas-García et al., 2022), 20% ethanol (Figueroa et al., 2021), boiled water (Calderón-Oliver et al., 2016), in order to characterize the individual and total compounds, and also to evaluate the biological activity of the obtained extracts.
A study carried out by Melgar et al. (2018) demonstrated a high recovery yield of phenolic compounds from Hass variety avocado peel (227.9 mg/g extract) was obtained by conventional methods using 80% ethanol as solvent. However, extraction parameters were not evaluated and the effect of ethanol concentration, temperature and solvent/solid ratio on the maximum recovery of phenolic compounds is unknown. Hence, it was hypothesized that the correct application of ethanol concentration, temperature and solvent/solid ratio would increase the total phenolic and flavonoids contents in Hass avocado peel extracts over a 60-min extraction period by maceration and, consequently, the antioxidant capacity would also increase. In this particular solid–liquid extraction method the most important parameters that impact the extraction efficiency and the minimization of loss of these compounds are the solvent concentration, the solvent:feed (S/F) ratio and the temperature (Spigno et al., 2007; Bucić-Kojić et al., 2009; Chuen et al., 2015; Drosou et al., 2015; Papoutsis et al., 2016). Within this context, the aim of this study was to assess the effect of process variables (ethanol concentration, temperature and S/F ratio) on the global yield of extraction (GY), total phenolic content (TPC), total flavonoid content (TFC), and antioxidant capacity (DPPH) of avocado Hass peel extract at two stages of maturity. Furthermore, the quantification of phenolic compounds was conducted using HPLC-DAD and the determination of antioxidant capacity (ABTS and FRAP) was performed on extracts with higher yields at both stages of maturity.
Gallic acid, Folin–Ciocalteu’s phenol reagent, calcium carbonate, quercetin, sodium nitrite, aluminum chloride, sodium hydroxide, trolox (6-hydroxy-2,5,7,8-tetramethylchroman-2-carboxylic acid), potassium persulfate, sodium acetate trihydrate, hydrochloric acid, glacial acetic acid, ferric chloride hexahydrate, DPPH (2,2-diphenyl-1-picrylhydrazyl), ABTS (diammonium salt of 2,2-azinobis (3-acid) ethylbenothiazoline-6-sulfonic acid), TPTZ (2,4,6tripyridyl-s-triazine) were purchased from Sigma-Aldrich (St. Louis, MO, United States). Other regents such as ethanol and methanol were analytical grade and were manufactured from Spectrum Chemicals (New Brunswick, NJ, United States).
The avocado fruits of the Hass, known for their export quality, were harvested from La Calera Agricola farm (Alto Laran, Chincha, Peru). The fresh fruits were rinsed followed by disinfection with Kuma Nat solution (5 mL/L) for 3 min. Subsequently the fruits were left to drain. A portion of the treated fruits was stored in a ripening chamber (CLIMACELL EVO, Germany) at 28°C for a period of 3 days, while another portion was stored for 6 days. In order to separate the peel from the pulp and seed, the two distinct stages of maturation were individually subjected to manual processing. The dry matter content of the pulp was subsequently determined using the methodology proposed by Salameh et al. (2022). The present study investigated the dry matter (dm) content of avocado pulp following storage for 3 and 6 days. Results indicated that unripe avocados (UA) had a dm content of 22.57%, while ripe avocados (RA) had a significantly higher DM content of 27.30%. To remove any pulp residues, the peels were washed and then chopped into small fragments and conditioned in a dehydrator (Excalibur 4526T220FW-60, United States) and dried at 45°C for 24 h. The dehydrated peels were crushed (Bosch MMR08R2, Gerlingen, DEU) followed by sifting (Bertel, Caieiras, BR), the average particle diameter was determined in triplicate in accordance with the ASAE (1997), and was found to be 0.50 ± 0.01. The dried crushed peels were subsequently stored in a frost-free freezer (Indurama RI-279D, China) at −18°C until further use.
The experimental design was conducted with three independent variables and their respective ranges: ethanol concentration (EtOH, X1: 40, 60, and 80%, v/v), temperature (T, X2, 40°C, 50°C, and 60°C), and S/F ratio (X3, 5, 10, and 15 mL/g, v/m). The duration of the extraction process was set at 60 min. All these parameters were considered in accordance with the outcomes of preliminary experiments. The experimental design used in this study was the Box-Behmken design (BBD), which consisted of 12 independent variable runs (runs 1–12) and three replicates at the central point (designated as runs 13–15). The BBD used three factors and three level as observed in Table 1. The relationship between the independent variables and the answers was modeled using a second-order polynomial equation (Eq. 1):
where Y is the response variable (GY, TPC, TFC, DPPH), X’s are the extraction parameters, and β’s are the coefficients. To fit the experimental data to the model equation, DESIGN-EXPERT 11.0 software (Trial version, Stat-Ease Inc., Minneapolis, MN, United States) was used.
Table 1. Matrix of Box–Behnken design and responses of global yield of extraction, total phenolic content, total flavonoid content, and antioxidant capacity (DPPH) of the extracts from unripe and ripe avocado peels.
For each extraction, a volume of 9 mL of ethanol solution with varying concentrations (EtOH, %, v/v) was used for the S/F ratio according to the experimental design (Table 1). The dried crushed peel of either UA or RA was added to a tube according to the S/F ratio. Subsequently, EtOH was added to the same tube and each extraction temperature (Table 1) was controlled using a water tank (Biobase, WT- 42, Shandong, China) for 60 min. Then, the extract was obtained via filtration using Whatman No. 4 filter paper, and the solvent of the extract was evaporated under vacuum condition (KANKUN, R-1010, Shanghai, China). The recovered extract was weighed and stored in a frost-free freezer (Indurama RI-279D, China) at −18°C until further analysis.
The GY was calculated by the ratio of the dry extract mass to dried crushed UA or RA peel mass on dry basis, according to Eq. 2.
The analysis of the total phenolic content was performed according to the methodology described by Cornelio-Santiago et al. (2019) with the following modifications. Test tubes were filled with 500 μL of each diluted extract, 250 μL of Folin–Ciocalteu 1 N, and left to stand for 5 min in the dark. Next, each tube received 1,250 μL of 7.5% Na2CO3, and the tubes were allowed to stand for 120 min in the dark. The absorbance of the samples and gallic acid (0–60 mg/L) were measured using a spectrophotometer at 760 nm (Genesys 50 UV–VIS Thermo Fisher, United States) and results were represented as mg of gallic acid equivalents (GAE)/g peel dry weight (dw).
For the analysis of total flavonoid content, some adjustments were made to the method described by Zhishen et al. (1999) as follows. In test tubes, 250 μL of each diluted extract was added, followed by 1,250 μL of distilled water, then 75 μL of 5% NaNO2 was added, was left to stand in the dark for 6 minutes. Each tube received 150 μL of 10% AlCl3.6H2O, and then left 5 min in the dark. Next, 500 μL of 1 M NaOH and distilled water were added to each tube to a final volume of 2,500 μL. Finally, using a spectrophotometer (Genesys 50 UV–VIS Thermo Fisher, United States), the absorbances of the samples and quercetin (0.2–1.2 mg/mL) were measured at 510 nm and results were represented as mg of quercetin equivalent (QE)/ g peel dry weight (dw).
The following adjustments were made based on the methodology proposed by Brand-Williams et al. (1995) for DPPH analysis. To create a stock solution, 2 mg of DPPH was dissolved in 100 mL of reagent grade methanol. Subsequently, this solution was diluted with methanol until an absorbance range of 0.9 ± 0.02 at 515 nm, thereby yielding a solution suitable for practical use. Test tubes were filled with 150 μL of each diluted extract, 2,850 μL of working solution, and allowed to stand for 30 min in the dark. The methodology used to assess the antioxidant capacity using ABTS was proposed by Re et al. (1999). The ABTS reagent was dissolved in water at a concentration of 7 mM and combined with 2.45 mM potassium persulfate in a 1:1 ratio. The mixture was kept in the dark at room temperature for 16 h allowing the formation of the radical cation ABTS (ABTS +). Methanol was used to dilute the solution of ABTS + to an absorbance of 0.70 ± 0.02 at 734 nm. Test tubes were filled with 150 μL of each diluted extract, 2,850 μL of diluted ABTS•+ solution and allowed to stand in the dark for 30 min. The FRAP method described by Benzie and Strain (1996) was prepared by combining 5 mL of a 10 mM TPTZ solution with 5 mL of a 10 mM TPTZ solution. FeCl3.6H2O 20 mM and 50 mL of 0.3 mM acetate buffer (pH 3.6). Test tubes were filled with 150 μL of each diluted extract, 2,850 μL of FRAP reagent, then left to stand in the dark for 30 min. Finally, the absorbances were measured at 515, 734 and 593 for DPPH, ABTS and FRAP, respectively, in a spectrophotometer (Genesys 50 UV–VIS Thermo Fisher, United States) and the results were calculated from a standard curve of Trolox (0.1–0.4 μM) and expressed as μmol of Trolox Equivalent (TE)/g peel dry weight (dw).
The HPLC-DAD was used to determine phenolic compounds in accordance with the methodology proposed by Ramos-Escudero et al. (2021). The analysis was carried out with a High-Performance Liquid Chromatograph Chromaster with a diode array detector (Hitachi High-Technologies, Tokyo, JP). The separation of the analytes was performed using a LiChrospher® 100 RP-18 end-capped, 5 μm, 4.6 × 250 mm cartridge column (Millipore, Darmstadt, Germany). The injection volume was 20 μL, the flow was 1 mL/min, and the column was kept at a constant temperature of 30°C. The solvent elution used a mixture of methanol:acetonitrile (50:50 v/v) as mobile phase B and 0.5% orthophosphoric acid in water as mobile phase A. The following gradient system was used to elute the analytes (solvent B percentages are shown; the difference in the mobile phase corresponds to the mobile phase A): Starting with 5% (B), the elution progressed to 30% (B) after 25 min, 38% (B) after 35 min, 38% (B) after 40 min, 45% (B) after 45 min, 52.2% (B) after 50 min, and 100% (B) after 55 min. It was then kept at 100% (B) for 5 min to clean the column. The execution of the chromatogram lasted 60 min. Chromatograms were obtained at a wavelength of 280 nm. The identification of phenolic compounds was carried out by comparing the retention times of the extracts and the standard of phenolic compounds. The quantification of phenolic compounds in the extracts was calculated through the corresponding peak areas by external standardization, and the results were expressed in mg per Kg peel dw.
The statistical analysis of the outcomes obtained from the Box-Behmken design (BBD) was statistically analyzed using DESIGN-EXPERT 11.0 (trial version, Stat-Ease Inc., Minneapolis, MN, United States). To assess the efficacy of the model, various statistical measures were employed, including value of p, F-value, test for lack of fit, R2, and Adj.R2 were used by ANOVA. Furthermore, Student’s t-test using SPSS software (ver. 24.0; SPSS Inc., Chicago, IL, United States) was used to evaluate the antioxidant capacity, phenolic compound profile, and validity of predicted and experimental data of extracts with higher extraction yield in unripe and ripe avocado. For each evaluation, three replicates of the study results were used to calculate the mean and standard deviation, and a 95% confidence level was used in all analyses.
The effects of varying concentration of ethanol were investigated (X1, 40, 60, and 80% (v/v)) on several variables (GY, TPC, TFC, and DPPH). A significant effect (p < 0.05) of ethanol concentration was observed in GY and TFC in UA (Table 2), while TPC, TFC and DPPH were significant (p < 0.05) in RA (Table 3). Different concentrations of ethanol have impact on extraction yields due to the reduction of the solvent dielectric constant, causing an increase in both the solubility and diffusivity of the solute. This phenomenon was also observed by Park et al. (2012) and Garcia-Castello et al. (2015). Conversely, the highest extraction outcomes of TFC, TFC and DPPH were observed at an ethanol concentration of 40%, as shown in Table 4. Close values were found by Araújo et al. (2021)) who reported that a solution of approximately 42.58% ethanol is suitable for the extraction of phenolic compounds in avocado Hass. In contrast to the utilization of a pure solvent, it is possible to achieve superior extraction yields with excellent antioxidant capacity using a dissolvent mixed with water (Rodríguez-Carpena et al. (2011). Nevertheless, high ethanol concentrations may cause denaturation, dehydration and collapse of plant cells, which would likely be reflected in extraction yields (Garcia-Castello et al., 2015).
Table 2. Regression coefficients, value of p and analysis of variance (ANOVA) for GY, TPC, TFC, and antioxidant capacity (DPPH) of the extract from unripe avocado peel.
Table 3. Regression coefficients, value of p and analysis of variance (ANOVA) for GY, TPC, TFC and antioxidant capacity (DPPH) of the extract from in ripe avocado peel.
Table 4. Experimental and predicted values of total phenolic content, total flavonoid content and antioxidant capacity (DPPH) of unripe and ripe avocado peel extracts obtained under the highest extraction yield conditions.
The extraction effectiveness and the quality of the target chemicals compounds are both significantly affected by temperature. This study also evaluated the effects of temperature (X2, °C) on GY, TPC, TFC and DPPH. It was observed that UA had a significant influence (p < 0.05) on both TPC and TFC (Table 2). Conversely, RA only presented TFC effect (Table 3). According to Mustafa and Turner (2011), the use of thermal energy enhances the efficiency of extraction by modifying cellular structures, due to a reduction in the surface tension of the solvent and an increase in its viscosity (Vergara-Salinas et al., 2012; Jovanović et al., 2017), which would be reflected in an increase in the permeability of the cell membrane, rupture of the interactions between secondary metabolic products and the matrix; augmentation of solubility and mass transfer. Increase temperature during the extraction process may cause bioactive compounds degradation, resulting in a loss of their biological activity, as reported by Cengiz et al. (2021) and Yusoff et al. (2022). In the present investigation it was observed that at a temperature of 50°C the best outcomes were obtained in terms of TPC, TFC and DPPH. However, as the temperature was raised to 60°C the level of these compounds decreased (Table 1).
The S/F ratio is a significant parameter that effects on the extraction efficiency of bioactive compounds (Guiné et al., 2019). In this research, the effects of S/F (X3, mL/g) on GY, TPC, TFC and DPPH were evaluated. There were significant differences (p < 0.05) on GY, TPC and DPPH in UA, while in RA it had effect on GY, TPC and TFC, the results can be seen in Tables 2, 3. The higher the S/F, the greater the concentration gradient difference between the outside and the inside of the plant cell, facilitating the solvent to penetrate into the center of the plant cells and dissolve the phenolic compounds to be extracted (Nguyen and Phan, 2023). This was observed in the Figures 1, 2 indicate that an increase in the S/F from to 5 to 15 mL/g. This is the first research on avocado Hass peels that evaluates the effect of S/F ratio on extraction efficiency. It examined the highest values in TPC, TFC and DPPH in UA were achieved with the S/F ratio of 15:1 (Table 1). On the other hand, the S/F ratio has been studied in custard apple (Annona squamosa L.) peel (Nguyen and Phan, 2023), as well as in ground berries by Cacace and Mazza (2003) and seeds by Al-Farsi and Lee (2008).
Figure 1. Surface response in unripe avocado peels for global yield of extraction (GY, A1–A3), total phenolic content (TPC; B1–B3), total flavonoid content (TFC; C1–C3) and antioxidant capacity (DPPH; D1–D3) influenced by ethanol concentration (EtOH, X1; 40, 60, and 80%), temperature (T, X2; 40, 50, and 60°C) and S/F ratio (S/F, X3;5, 10, and 15 mL/g).
Figure 2. Surface response in ripe avocado peels for global yield of extraction (GY, A1–A3), total phenolic content (TPC; B1–B3), total flavonoid content (TFC; C1–C3) and antioxidant capacity (DPPH; D1–D3) influenced by ethanol concentration (EtOH, X1; 40, 60, and 80%), temperature (T, X2; 40, 50, and 60°C) and S/F ratio (S/F, X3;5, 10, and 15 mL/g).
Extraction parameters, namely ethanol concentration (X1, %), temperature (X2, °C) and S/F ratio (X3, mL/g) were evaluated by BBD for the GY, TPC, TFC and DPPH for UA and RA. The extraction yields obtained are presented in Table 1, while Tables 2, 3 provide the results of the analysis of variance (ANOVA) of the regression model coefficients.
The regression models for GY demonstrate a good fit to the experimental data, as evidenced by the low value of ps (p < 0.05), the lack of fit is found to be non-significant (p > 0.05), and excellent coefficient of determination (R2) values (R2 > 0.98). The use of response surface plots facilitated the visualization of the significance of individual extraction variables on GY in UA (Figures 1A1–A3) and RA (Figures 2A1–A3). Positive linear term coefficients (X1 and X3) and negative quadratic term coefficients (X12 and X32) in Tables 2, 3 indicated that yields increased with the increase of ethanol concentration and S/F ratio, reaching a maximum. However, it is noteworthy that this relationship reaches a peak, further increments in ethanol concentration and S/F ratio did not result in additional yield enhancements. On the other hand, interaction terms (X1X3, X2X3) significant (p < 0.05), imply that there was a strong interaction between these two characteristics in the GY.
Upon evaluation of the TPC, it was observed that the second order model in UA and RA were significant (p < 0.05), indicating that the TPC variation is well described by the model used. This assertion is supported by the high R2 values (R2 ≥ 0.92), the absence of a significant lack of fit (p > 0.05) and the low coefficient of variation (CV < 2.11%). Detailed information can be observed in Tables 2, 3. The response surface plots also facilitate the visualization of individual variables in the total phenolic content (TPC) for both UA (Figures 1B1–B3) and RA (Figures 2B1–B3). The linear terms (X2 and X3) exhibited positive coefficients in UA, indicating a direct correlation with TPC, while the negative coefficients of the quadratic terms (X22) indicated an inverse relationship with TPC. Conversely, interaction terms (X1X3,) were significantly affected by the TFC of each other. In the context of RA, the positive linear terms coefficients (X1 and X3) and the negative quadratic terms (X12 and X32) exhibited a similar pattern as the UA. On the other hand, interaction terms (X2X3) show that the TPC was considerably impacted by these two variables in relation to one another.
Figure 1 displays the regression coefficients and RSM plots for the extraction variables c1, c2, and c3 for UA. Similarly, Figure 2 presents the regression coefficients and RSM plots for the extraction variables C1–C3 for RA. The visual representations aid in the comprehension and analysis of each extraction variable. The data presented in Table 2 indicates that for UA, the coefficients of the following terms were negative: (X1), (X22 and X32) and (X1X3, and X2X3,) having an indirect relationship with the TFC. Furthermore, the change in the temperature (X2) positively affects TFC. In contrast, with respect to RA, the negative coefficients (X1, X2 and X3 and X12) decrease the extraction of TFC, on the contrary (X22, X1X2, and X2X3) positively influence the extraction of TFC.
The statistical analysis of DPPH revealed that the second order model in UA and RA exhibited significant results (p < 0.05). This suggests that the DPPH variation is well described by the model employed. Similarly, the results demonstrate a strong correlation between the variables, as evidenced by the high values of R2 (R2 ≥ 0.92) and low coefficient of variation (CV < 2.11%), indicating a good fit to the proposed model. This can be that observed in the UA graphs (Figures 1D1–D3) and RA graphs (Figures 2D1–D3). When analyzing the UA, the positive coefficients (X3) positively influence the extraction of DPPH. On the other hand, the negative quadratic terms (X22 and X32) have a negative effect on the DPPH. In contrast, upon analyzing, the regression coefficients of the RA, it is observed that they are negative (X1, X12, X22, X32), thereby suggesting that they have a negative influence on the DPPH. When evaluating the coefficients of the interaction (X2X3), it is evident that the interaction between temperature and the S/F ratio have a positive influence on DPPH. The subsequent equations, namely Eq 3 and Eq 4, can be used to calculate the condition with the highest extraction yield for TPC and DPPH in ripe avocado peels.
where the test variables (ethanol concentration, temperature, and S/F ratio, respectively) are X1, X2, and X3, respectively.
Validation experiments were performed with the levels obtained for the subsequent extraction of individual responses. The experimental values are presented in Table 4. It should be noted that the experimental values in UA (TFC, TPC, and DPPH) and RA (DPPH) were higher than predicted, while in RA (TFC and TPC) they were slightly lower. These results could be associated with the fact that the raw material used for validation presented different initial values of these bioactive compounds, which was also observed by Bengardino et al. (2019). Despite these differences between the values, it should be noted that the values found experimentally reaffirm the usefulness of this tool (BBD) together with the desirability function (d) that presented a value d = 0, 92 in UA and a value of d = 1 in RA, considered as “satisfactory” by Lazic (2006), which could be verified because it maximized TFC, TPC and DPPH in UA; as well as DPPH in RA. The values obtained under the conditions suggested in the extraction are the best solution considering the responses obtained. In fact, this tool (BBD) has been used in other studies that observed similar effects (Chen et al., 2018; Kushwaha et al., 2018; Bengardino et al., 2019; Alam et al., 2021).
In this study, three antioxidant capacity techniques, based on free radical scavenging capacity (DPPH, ABTS) and reducing capacity (FRAP), were used to evaluate the antioxidant properties of unripe and ripe avocado extracts obtained with the highest extraction parameter combinations as shown in Table 4. The findings of this research demonstrate that the values of DPPH, FRAP and ABTS exhibit a statistically higher (p < 0.05) in unripe avocado in comparison to ripe avocado. Likewise, the present values of UA as RA exhibit higher values than those previously published by Tremocoldi et al. (2018), who reported 310 μmolTE/g to DPPH and 791,5 μmol TE/g to ABTS in ethanol extracts of avocado Hass peels. However, the present values are lower than those reported by Araújo et al. (2021), who obtained 233.85 mg TE/g dry extract for DPPH and 949 mg TE/g dry extract for ABTS. In addition, Morais et al. (2015) observed that avocado peels exhibited a greater antioxidant capacity, as determined by the FRAP assay, when compared to peels from various other tropical fruits such as pineapple, papaya, passion fruit, banana, melon, and watermelon.
Table 5 presents different values depending on the technique used to assess the antioxidant capacity, with ABTS exhibiting the highest value (1006.21 μmol TE/g peel dw). Upon conducting a comparison of antioxidant activity methods, it was observed that the FRAP method exhibited a 1.78-fold increase in activity as compared to the DPPH method. This discrepancy since the reduction procedures are divergent for each method being the main cause of the disparity between them. For instance, the DPPH method bases on the content of phenolic compounds by measuring the reduction of molybdenum/tungsten salts by phenolic groups. The FRAP methodology bases on the reduction of the antioxidant de Fe3+ to Fe2+. The method ABTS + bases on the quantification of the discoloration of the radical ABTS +, resulting from its interaction with hydrogen or electron donating species. These findings suggest that the radical structure, reaction processes, and types of phenols may influence the antioxidant efficacy of avocado extracts. This study provides new information on the high antioxidant capacity of ethanol extracts from avocado Hass peels at two distinct stages of maturity. Since the ethanolic extract of UA presents high antioxidant properties (ABTS, FRAP, DPPH) which makes this attribute interesting to the food and pharmaceutical industry.
Table 5. Antioxidant capacity (DPPH, FRAP and ABTS) of extracts with the highest yield from unripe and ripe avocado peel.
Figure 3 shows the profile of phenolic compounds found in UA and RA peels, and Table 6 lists the peak properties (phenolic compound type, molecular formula and mass spectral data) of the nine compounds that were discovered by comparison of their retention time (RT). All phenolic substances showed statistically significant differences (p < 0.05) between UA and RA. Three acids were detected; peak 1 gallic acid (RT = 5.66 min), peak 4 chlorogenic acid (RT = 15.77 min) and peak 6 syringic acid (RT = 17.70 min). Chlorogenic acid has also been identified by Martínez-Gutiérrez (2023) in ethanol extracts of avocado peels with a value of 7.2 ± 0.3 mg/100 g d.m, the aforementioned value is lower than that found in the present investigation for AU with 8.37 mg/100 g.d.m. Additionally, three hydroxybenzoic acids were also found, peak 2 with RT = 9.15 was characterized as 3,4-dihydroxybenzoic acid, peak 5 vanillic acid (RT = 16.147 min). Moreover, vanillin, identified as a monohydroxybenzoic acid, was found in peak 7 with a retention time of 18.85 min. The presence of vanillin was also reported by De la Torre-Carbot et al. (2005) in avocado oil. Two hydroxycinnamic acids were detected in peak 8 ferulic acid (RT = 23.69 min) and in peak 9, 2-hydroxycinnamic acid (RT = 28.30 min). These last two phenolic acids have not been previously described in ethanol extracts of Hass avocado peels.
Figure 3. Chromatogram of phenolic compounds present in avocado peel extracts obtained at the highest extraction yield conditions; (A) Unripe avocado; (B) Ripe avocado.
Table 6. Phenolic compounds present in the highest yielding extract from unripe and ripe avocado peels.
In peak 3 (Table 6; Figure 3) was founded 4-hydroxyphenylacetic acid (RT = 13.20 min); it is a monocarboxylic acetic acid in which one of the methyl hydrogens is substituted by a 4-hydroxyphenyl group. This acid is the major phenolic compound present in both UA and RA with 3,257,86 and 2624.39 mg/Kg, respectively. The 4-hydroxyphenylacetic acid is used to treat lung diseases, and it is used as an inhibitor to alleviate hypoxia and hypertonicity (Ng et al., 2003; Liu et al., 2014), due to the high antioxidant capacity that can decrease free radicals and the high concentration of hydroxyl groups it produces (Kosińska et al., 2012; Zhou et al., 2018). It can also be used as an intermediate for the synthesis of a β-receptor blocking agent (Thakur et al., 2018), as a natural antioxidant and a potential substitute to replace some synthetic antioxidant food additives.
The phenolic chemical profile (chlorogenic acid, gallic acid, 3,4-dihydroxybenzoic acid, syringic acid, vanillin, vanillic acid and ferulic acid) discovered in this study is consistent to other studies that have covered the phenolic profile in avocado peels (De La Torre-Carbot et al., 2005; Figueroa et al., 2018; Martínez-Gutiérrez, 2023). It is noteworthy to acknowledge that a number of compounds, such as 2-hydroxycinnamic acid and 4-hydroxyphenylacetic acid, have been identified for the first time in this research within the ethanolic extract derived from avocado Hass peels. Furthermore, it is crucial to remember that the cultivar., climate conditions, growing region, stage of fruit maturity, precipitation pattern, and fruit genetics collectively have an impact on the composition of phytochemical components found in avocado peels (Araújo et al., 2021).
The results showed that, for all tests, ethanol concentration, temperature and S/F ratio significantly (p < 0.05) influenced the GY, TPC, TFC and antioxidant capacity (DPPH) of the extracts obtained from unripe and ripe Hass avocado peel and the unripe avocado peel extracts obtained using 40% ethanol, 49.3°C, S/F ratio (14.3 mL/g) and 60 min exhibited the highest values of TPC (44.24 mg GAE/g peel dw), TFC (786.08 mg QE/g peel dw) and antioxidant capacity against DPPH (564.82 μmTE/g peel dw), ABTS (804.40 μmTE/g peel dw) and FRAP (1006.21 μmTE/g peel dw). Through HPLC-DAD analysis, vanillic acid and 4-hydroxyphenylacetic acid are the main phenolic compounds found in avocado peel extracts. Unripe avocado extract could be a viable candidate to validate its functionality and use in the pharmaceutical, cosmetic industries, as well as to substitute synthetic antioxidants in the food industry due to their significant amounts of TPC, TFC, and antioxidant capacity.
The original contributions presented in the study are included in the article/supplementary materials, further inquiries can be directed to the corresponding author.
FG-R: Conceptualization, Data curation, Formal analysis, Investigation, Software, Supervision, Validation, Writing – original draft, Writing – review & editing. MM-R: Conceptualization, Funding acquisition, Supervision, Writing – original draft, Writing – review & editing. JR-M: Conceptualization, Formal analysis, Funding acquisition, Writing – review & editing. JV-M: Data curation, Formal analysis, Investigation, Methodology, Software, Writing – original draft. LN-V: Supervision, Writing – review & editing. HC-S: Conceptualization, Data curation, Supervision, Validation, Visualization, Writing – original draft, Writing – review & editing. AS-M: Investigation, Supervision, Writing – original draft.
The author(s) declare financial support was received for the research, authorship, and/or publication of this article. This research has been possible thanks to the framework and contribution of Project No. 077–2021 of the “DESAFÍO 2021” competition, funded by the National Program for Applied Science (PROCIENCIA), a non-profit organization whose mission is to promote the development of research in Perú.
FG-R, MM-R, JV-M, and JR-M are employees of the Bio Natural Solutions (BNS).
The remaining authors declare that the research was conducted in the absence of any commercial or financial relationships that could be construed as a potential conflict of interest.
All claims expressed in this article are solely those of the authors and do not necessarily represent those of their affiliated organizations, or those of the publisher, the editors and the reviewers. Any product that may be evaluated in this article, or claim that may be made by its manufacturer, is not guaranteed or endorsed by the publisher.
Alam, P., Siddiqui, N. A., Rehman, M. T., Hussain, A., Akhtar, A., Mir, S. R., et al. (2021). Box–Behnken design (BBD)-based optimization of microwave-assisted extraction of Parthenolide from the stems of Tarconanthus camphoratus and cytotoxic analysis. Molecules 26:1876. doi: 10.3390/MOLECULES26071876
Al-Farsi, M. A., and Lee, C. Y. (2008). Optimization of phenolics and dietary fibre extraction from date seeds. Food Chem. 108, 977–985. doi: 10.1016/J.FOODCHEM.2007.12.009
Araújo, R. G., Rodríguez-Jasso, R. M., Ruíz, H. A., Govea-Salas, M., Pintado, M., and Aguilar, C. N. (2021). Recovery of bioactive components from avocado peels using microwave-assisted extraction. Food Bioprod. Process. 127, 152–161. doi: 10.1016/J.FBP.2021.02.015
ASAE (1997). Standards. Method of determining and expressing fineness of feed materials by Sievieng. ASAE, 547.
Banerjee, J., Singh, R., Vijayaraghavan, R., MacFarlane, D., Patti, A. F., and Arora, A. (2017). Bioactives from fruit processing wastes: green approaches to valuable chemicals. Food Chem 225, 10–22. doi: 10.1016/J.FOODCHEM.2016.12.093
Bangar, S. P., Dunno, K., Dhull, S. B., Kumar Siroha, A., Changan, S., Maqsood, S., et al. (2022). Avocado seed discoveries: chemical composition, biological properties, and industrial food applications. Food Chem X 16:100507. doi: 10.1016/J.FOCHX.2022.100507
Bengardino, M. B., Fernandez, M. V., Nutter, J., Jagus, R. J., and Agüero, M. V. (2019). Recovery of bioactive compounds from beet leaves through simultaneous extraction: modelling and process optimization. Food Bioprod. Process. 118, 227–236. doi: 10.1016/J.FBP.2019.09.013
Benzie, I. F. F., and Strain, J. J. (1996). The ferric reducing ability of plasma (FRAP) as a measure of “‘antioxidant power’”: the FRAP assay. Anal. Biochem. 239, 70–76. doi: 10.1006/abio.1996.0292
Bowen, J., Billing, D., Connolly, P., Smith, W., Cooney, J., and Burdon, J. (2018). Maturity, storage and ripening effects on anti-fungal compounds in the skin of ‘Hass’ avocado fruit. Postharvest Biol. Technol. 146, 43–50. doi: 10.1016/J.POSTHARVBIO.2018.08.005
Brand-Williams, W., Cuvelier, M. E., and Berset, C. (1995). Use of a free radical method to evaluate antioxidant activity. LWT-Food Sci.Technol. 28, 25–30. doi: 10.1016/S0023-6438(95)80008-5
Bucić-Kojić, A., Planinić, M., Tomas, S., Jakobek, L., and Šeruga, M. (2009). Influence of solvent and temperature on extraction of phenolic compounds from grape seed, antioxidant activity and colour of extract. Int. J. Food Sci. Technol. 44, 2394–2401. doi: 10.1111/J.1365-2621.2008.01876.X
Cacace, J. E., and Mazza, G. (2003). Mass transfer process during extraction of phenolic compounds from milled berries. J. Food Eng. 59, 379–389. doi: 10.1016/S0260-8774(02)00497-1
Calderón-Oliver, M., Escalona-Buendía, H. B., Medina-Campos, O. N., Pedraza-Chaverri, J., Pedroza-Islas, R., and Ponce-Alquicira, E. (2016). Optimization of the antioxidant and antimicrobial response of the combined effect of nisin and avocado byproducts. LWT-Food Sci.Technol. 65, 46–52. doi: 10.1016/J.LWT.2015.07.048
Cengiz, M. F., Babacan, U., Akinci, E., and Tuncer Kesci, S., and Kaba, A. (2021). Extraction of phenolic acids from ancient wheat bran samples by ultrasound application. J. Chem. Technol. Biotechnol. 96, 134–141. doi: 10.1002/jctb.6519
Chemat, F., Abert-Vian, M., Fabiano-Tixier, A. S., Strube, J., Uhlenbrock, L., Gunjevic, V., et al. (2019). Green extraction of natural products. Origins, current status, and future challenges. TrAC Trends Anal. Chem. 118, 248–263. doi: 10.1016/J.TRAC.2019.05.037
Chen, S., Zeng, Z., Hu, N., Bai, B., Wang, H., and Suo, Y. (2018). Simultaneous optimization of the ultrasound-assisted extraction for phenolic compounds content and antioxidant activity of Lycium ruthenicum Murr. Fruit using response surface methodology. Food Chem. 242, 1–8. doi: 10.1016/J.FOODCHEM.2017.08.105
Chuen, T. L. K., Vuong, Q. V., Hirun, S., Bowyer, M. C., Goldsmith, C. D., and Scarlett, C. J. (2015). Optimum aqueous extraction conditions for preparation of a phenolic-enriched Davidson’s plum (Davidsonia pruriens F. Muell) extract. Int. J. Food Sci. Technol. 50, 2475–2482. doi: 10.1111/IJFS.12915
Cornelio-Santiago, H. P., Mazalli, M. R., Rodrigues, C. E. C., and de Oliveira, A. L. (2019). Extraction of Brazil nut kernel oil using green solvents: effects of the process variables in the oil yield and composition. J. Food Process Eng. 42:e13271. doi: 10.1111/JFPE.13271
De La Torre-Carbot, K., Jauregui, O., Gimeno, E., Castellote, A. I., Lamuela-Raventós, R. M., and López-Sabater, M. C. (2005). Characterization and quantification of phenolic compounds in olive oils by solid-phase extraction, HPLC-DAD, and HPLC-MS/MS. J. Agric. Food Chem. 53, 4331–4340. doi: 10.1021/JF0501948/SUPPL_FILE/JF0501948SI20050317_051623.PDF
Del Castillo-Llamosas, A., del Río, P. G., Pérez-Pérez, A., Yáñez, R., Garrote, G., and Gullón, B. (2021). Recent advances to recover value-added compounds from avocado by-products following a biorefinery approach. Curr Opin Green Sustain Chem 28:100433. doi: 10.1016/J.COGSC.2020.100433
Del Rio Osorio, L. L., Flórez-López, E., and Grande-Tovar, C. D. (2021). The potential of selected Agri-food loss and waste to contribute to a circular economy: applications in the food, cosmetic and pharmaceutical industries. Molecules 26:515. doi: 10.3390/MOLECULES26020515
Drosou, C., Kyriakopoulou, K., Bimpilas, A., Tsimogiannis, D., and Krokida, M. (2015). A comparative study on different extraction techniques to recover red grape pomace polyphenols from vinification byproducts. Ind. Crop. Prod. 75, 141–149. doi: 10.1016/J.INDCROP.2015.05.063
FAOSTAT. (2020). https://www.fao.org/faostat/en/#data/QCL.
Ferreira, S. M., and Santos, L. (2022). From by-product to functional ingredient: incorporation of avocado peel extract as an antioxidant and antibacterial agent. Innovative Food Sci. Emerg. Technol. 80:103116. doi: 10.1016/J.IFSET.2022.103116
Figueroa, J. G., Borrás-Linares, I., Del Pino-García, R., Curiel, J. A., Lozano-Sánchez, J., and Segura-Carretero, A. (2021). Functional ingredient from avocado peel: microwave-assisted extraction, characterization and potential applications for the food industry. Food Chem. 352:129300. doi: 10.1016/J.FOODCHEM.2021.129300
Figueroa, J. G., Borrás-Linares, I., Lozano-Sánchez, J., and Segura-Carretero, A. (2018). Comprehensive characterization of phenolic and other polar compounds in the seed and seed coat of avocado by HPLC-DAD-ESI-QTOF-MS. Food Res. Int. 105, 752–763. doi: 10.1016/J.FOODRES.2017.11.082
Garcia-Castello, E. M., Rodriguez-Lopez, A. D., Mayor, L., Ballesteros, R., Conidi, C., and Cassano, A. (2015). Optimization of conventional and ultrasound assisted extraction of flavonoids from grapefruit (Citrus paradisi L.) solid wastes. LWT Food Sci. Technol. 64, 1114–1122. doi: 10.1016/J.LWT.2015.07.024
Gil-Martín, E., Forbes-Hernández, T., Romero, A., Cianciosi, D., Giampieri, F., and Battino, M. (2022). Influence of the extraction method on the recovery of bioactive phenolic compounds from food industry by-products. Food Chem. 378:131918. doi: 10.1016/J.FOODCHEM.2021.131918
Guiné, R. P. F., Mendes, M., and Gonçalves, F. (2019). Optimization of bioactive compound’s extraction conditions from beetroot by means of artificial neural networks (ANN). Agric. Eng. Int. CIGR J. 21, 216–223.
Jovanović, A. A., Đorđević, V. B., Zdunić, G. M., Pljevljakušić, D. S., Šavikin, K. P., Gođevac, D. M., et al. (2017). Optimization of the extraction process of polyphenols from Thymus serpyllum L. herb using maceration, heat- and ultrasound-assisted techniques. Sep. Purif. Technol. 179, 369–380. doi: 10.1016/J.SEPPUR.2017.01.055
Kosińska, A., Karamać, M., Estrella, I., Hernández, T., Bartolomé, B., and Dykes, G. A. (2012). Phenolic compound profiles and antioxidant capacity of persea americana mill. Peels and seeds of two varieties. J. Agric. Food Chem. 60, 4613–4619. doi: 10.1021/JF300090P/ASSET/IMAGES/LARGE/JF-2012-00090P_0002.JPEG
Kushwaha, R., Kumar, V., Vyas, G., and Kaur, J. (2018). Optimization of different variable for eco-friendly extraction of Betalains and phytochemicals from beetroot pomace. Waste Biomass Valorizat. 9, 1485–1494. doi: 10.1007/S12649-017-9953-6/FIGURES/4
Lazic, Z. R. (2006). Design of experiments in chemical engineering: a practical guide. John Wiley & Sons, 176–177.
Liu, Z., Xi, R., Zhang, Z., Li, W., Liu, Y., Jin, F., et al. (2014). 4-Hydroxyphenylacetic acid attenuated inflammation and edema via suppressing HIF-1α in seawater aspiration-induced lung injury in rats. Int. J. Mol. Sci. 15, 12861–12884. doi: 10.3390/IJMS150712861
López-Cobo, A., Gómez-Caravaca, A. M., Pasini, F., Caboni, M. F., Segura-Carretero, A., and Fernández-Gutiérrez, A. (2016). HPLC-DAD-ESI-QTOF-MS and HPLC-FLD-MS as valuable tools for the determination of phenolic and other polar compounds in the edible part and by-products of avocado. LWT 73, 505–513. doi: 10.1016/J.LWT.2016.06.049
Martínez-Gutiérrez, E. (2023). Study of influence of extraction method on the recovery bioactive compounds from peel avocado. Molecules 28:2557. doi: 10.3390/molecules28062557
Martínez-Inda, B., Esparza, I., Moler, J. A., Jiménez-Moreno, N., and Ancín-Azpilicueta, C. (2023). Valorization of Agri-food waste through the extraction of bioactive molecules. Prediction of their sunscreen action. J. Environ. Manag. 325:116460. doi: 10.1016/J.JENVMAN.2022.116460
Mazzutti, S., Pedrosa, R. C., and Ferreira, S. R. S. (2021). Green processes in Foodomics. in Supercritical fluid extraction of bioactives. Comprehensive Foodomics. Reference Module in Food Science, Ed. S. R. Salvador Ferreira (Cambridge: Elsevier), 1– 19.
Melgar, B., Dias, M. I., Ciric, A., Sokovic, M., Garcia-Castello, E. M., Rodriguez-Lopez, A. D., et al. (2018). Bioactive characterization of Persea americana mill. By-products: a rich source of inherent antioxidants. Ind. Crop. Prod. 111, 212–218. doi: 10.1016/J.INDCROP.2017.10.024
Morais, D. R., Rotta, E. M., Sargi, S. C., Schmidt, E. M., Bonafe, E. G., Eberlin, M. N., et al. (2015). Antioxidant activity, phenolics and UPLC–ESI(−)–MS of extracts from different tropical fruits parts and processed peels. Food Res. Int. 77, 392–399. doi: 10.1016/J.FOODRES.2015.08.036
Mustafa, A., and Turner, C. (2011). Pressurized liquid extraction as a green approach in food and herbal plants extraction: a review. Anal. Chim. Acta 703, 8–18. doi: 10.1016/J.ACA.2011.07.018
Ng, T. B., Liu, F., Lu, Y., Cheng, C. H. K., and Wang, Z. (2003). Antioxidant activity of compounds from the medicinal herb Aster tataricus. Comp. Biochem. Physiol. Part C 136, 109–115. doi: 10.1016/S1532-0456(03)00170-4
Nguyen, T. T., and Phan, T. H. (2023). Stirred maceration extraction of custard apple (Annona squamosa L.) peel. IOP Conf. Ser. Earth. Environ. Sci. 1155:012016. doi: 10.1088/1755-1315/1155/1/012016
Nyakangi, C. O., Ebere, R., Marete, E., and Arimi, J. M. (2023). Avocado production in Kenya in relation to the world, avocado by-products (seeds and peels) functionality and utilization in food products. Appl. Food Res. 3:100275. doi: 10.1016/J.AFRES.2023.100275
Ortega-Arellano, H. F., Jimenez-Del-Rio, M., and Velez-Pardo, C. (2019). Neuroprotective effects of Methanolic extract of avocado Persea americana (var. Colinred) Peel on Paraquat-induced locomotor impairment, lipid peroxidation and shortage of life span in transgenic knockdown Parkin Drosophila melanogaster. Neurochem. Res. 44, 1986–1998. doi: 10.1007/S11064-019-02835-Z/FIGURES/6
Papoutsis, K., Pristijono, P., Golding, J. B., Stathopoulos, C. E., Scarlett, C. J., Bowyer, M. C., et al. (2016). Impact of different solvents on the recovery of bioactive compounds and antioxidant properties from lemon (Citrus limon L.) pomace waste. Food Sci. Biotechnol. 25, 971–977. doi: 10.1007/S10068-016-0158-8/METRICS
Park, H. Y., Park, Y., Lee, Y., Noh, S. K., Sung, E. G., and Choi, I. (2012). Effect of oral administration of water-soluble extract from citrus peel (Citrus unshiu) on suppressing alcohol-induced fatty liver in rats. Food Chem. 130, 598–604. doi: 10.1016/J.FOODCHEM.2011.07.081
Ramos-Aguilar, A. L., Ornelas-Paz, J., Tapia-Vargas, L. M., Gardea-Béjar, A. A., Yahia, E. M., Ornelas-Paz, J. D. J., et al. (2021). Effect of cultivar on the content of selected phytochemicals in avocado peels. Food Res. Int. 140:110024. doi: 10.1016/J.FOODRES.2020.110024
Ramos-Escudero, F., Morales, M. T., Ramos Escudero, M., Muñoz, A. M., Cancino Chavez, K., and Asuero, A. G. (2021). Assessment of phenolic and volatile compounds of commercial Sacha inchi oils and sensory evaluation. Food Res. Int. 140:110022. doi: 10.1016/J.FOODRES.2020.110022
Raymond Chia, T. W., and Dykes, G. A. (2010). Antimicrobial activity of crude epicarp and seed extracts from mature avocado fruit (Persea americana) of three cultivars. Pharm. Biol. 48, 753–756. doi: 10.3109/13880200903273922
Re, R., Pellegrini, N., Proteggente, A., Pannala, A., Yang, M., and Rice-Evans, C. (1999). Antioxidant activity applying an improved ABTS radical cation decolorization assay. Free Radic. Biol. Med. 26, 1231–1237. doi: 10.1016/S0891-5849(98)00315-3
Rodríguez-Carpena, J. G., Morcuende, D., Andrade, M. J., Kylli, P., and Estevez, M. (2011). Avocado (Persea americana mill.) phenolics, in vitro antioxidant and antimicrobial activities, and inhibition of lipid and protein oxidation in porcine patties. J. Agric. Food Chem. 59, 5625–5635. doi: 10.1021/JF1048832/ASSET/IMAGES/LARGE/JF-2010-048832_0001.JPEG
Rodríguez-Martínez, B., Ferreira-Santos, P., Gullón, B., Teixeira, J. A., Botelho, C. M., and Yáñez, R. (2021). Exploiting the potential of bioactive molecules extracted by ultrasounds from avocado peels—food and nutraceutical applications. Antioxidants 10:1475. doi: 10.3390/ANTIOX10091475
Rojas-García, A., Fuentes, E., Cádiz-Gurrea, M. D. L. L., Rodriguez, L., Villegas-Aguilar, M. D. C., Palomo, I., et al. (2022). Biological evaluation of avocado residues as a potential source of bioactive compounds. Antioxidants 11:1049. doi: 10.3390/ANTIOX11061049/S1
Saavedra, J., Córdova, A., Navarro, R., Díaz-Calderón, P., Fuentealba, C., Astudillo-Castro, C., et al. (2017). Industrial avocado waste: functional compounds preservation by convective drying process. J. Food Eng. 198, 81–90. doi: 10.1016/J.JFOODENG.2016.11.018
Salameh, M., Nacouzi, D., Lahoud, G., Riachy, I., and El Kayal, W. (2022). Evaluation of postharvest maturity indices of commercial avocado varieties grown at various elevations along Lebanon’s coast. Front. Plant Sci. 13:895964. doi: 10.3389/FPLS.2022.895964/BIBTEX
Šelo, G., Planinić, M., Tišma, M., Tomas, S., Koceva Komlenić, D., and Bucić-Kojić, A. (2021). A comprehensive review on valorization of agro-food industrial residues by solid-state fermentation. Foods 10:927. doi: 10.3390/FOODS10050927
Spigno, G., Tramelli, L., and De Faveri, D. M. (2007). Effects of extraction time, temperature and solvent on concentration and antioxidant activity of grape marc phenolics. J. Food Eng. 81, 200–208. doi: 10.1016/J.JFOODENG.2006.10.021
Thakur, N., Kumar, V., Thakur, S., Sharma, N. S., and Bhalla, T. C. (2018). Biotransformation of 4-hydroxyphenylacetonitrile to 4-hydroxyphenylacetic acid using whole cell arylacetonitrilase of Alcaligenes faecalis MTCC 12629. Process Biochem. 73, 117–123. doi: 10.1016/J.PROCBIO.2018.07.012
Tremocoldi, M. A., Rosalen, P. L., Franchin, M., Massarioli, A. P., Denny, C., Daiuto, É. R., et al. (2018). Exploration of avocado by-products as natural sources of bioactive compounds. PLoS One 13:e0192577. doi: 10.1371/JOURNAL.PONE.0192577
Trujillo-Mayol, I., Casas-Forero, N., Pastene-Navarrete, E., Silva, F. L., and Alarcón-Enos, J. (2021). Fractionation and Hydrolyzation of avocado Peel extract: improvement of antibacterial activity. Antibiotics 10, 1–22. doi: 10.3390/ANTIBIOTICS10010023
Vergara-Salinas, J. R., Pérez-Jiménez, J., Torres, J. L., Agosin, E., and Pérez-Correa, J. R. (2012). Effects of temperature and time on polyphenolic content and antioxidant activity in the pressurized hot water extraction of deodorized thyme (Thymus vulgaris). J. Agric. Food Chem. 60, 10920–10929. doi: 10.1021/JF3027759/SUPPL_FILE/JF3027759_SI_001.PDF
Wang, W., Bostic, T. R., and Gu, L. (2010). Antioxidant capacities, procyanidins and pigments in avocados of different strains and cultivars. Food Chem. 122, 1193–1198. doi: 10.1016/J.FOODCHEM.2010.03.114
Widsten, P., Cruz, C. D., Fletcher, G. C., Pajak, M. A., and McGhie, T. K. (2014). Tannins and extracts of fruit byproducts: antibacterial activity against foodborne bacteria and antioxidant capacity. J. Agric. Food Chem. 62, 11146–11156. doi: 10.1021/JF503819T/ASSET/IMAGES/LARGE/JF-2014-03819T_0002.JPEG
Yusoff, I. M., Taher, Z. M., and Rahmat, Z., and Chua, L. S. (2022). A review of ultrasound-assisted extraction for plant bioactive compounds: Phenolics, flavonoids, thymols, saponins and proteins. Food Res. Int. 157:111268. doi: 10.1016/j.foodres.2022.111268
Zhishen, J., Mengcheng, T., and Jianming, W. (1999). The determination of flavonoid contents in mulberry and their scavenging effects on superoxide radicals. Food Chem. 64, 555–559. doi: 10.1016/S0308-8146(98)00102-2
Keywords: avocado Hass peel, S/F ratio, bioactive compounds, phenolic compounds profile, circular economy, sustainability
Citation: García-Ramón F, Malnati-Ramos M, Rios-Mendoza J, Vivar-Méndez J, Nieva-Villegas LM, Cornelio-Santiago HP and Sotelo-Méndez A (2023) Avocado Hass peel from industrial by-product: effect of extraction process variables on yield, phenolic compounds and antioxidant capacity. Front. Sustain. Food Syst. 7:1255941. doi: 10.3389/fsufs.2023.1255941
Received: 10 July 2023; Accepted: 27 October 2023;
Published: 16 November 2023.
Edited by:
Zhen Chen, Xinyang Normal University, ChinaReviewed by:
Pedro Ferreira Santos, School of Engineering, University of Minho, PortugalCopyright © 2023 García-Ramón, Malnati-Ramos, Rios-Mendoza, Vivar-Méndez, Nieva-Villegas, Cornelio-Santiago and Sotelo-Méndez. This is an open-access article distributed under the terms of the Creative Commons Attribution License (CC BY). The use, distribution or reproduction in other forums is permitted, provided the original author(s) and the copyright owner(s) are credited and that the original publication in this journal is cited, in accordance with accepted academic practice. No use, distribution or reproduction is permitted which does not comply with these terms.
*Correspondence: Fernando García-Ramón, ZmVybmFuZG9fZ2FyY2lhQHVzcC5icg==; Heber Peleg Cornelio-Santiago, aGViZXJjb3JzYW5AZ21haWwuY29t
Disclaimer: All claims expressed in this article are solely those of the authors and do not necessarily represent those of their affiliated organizations, or those of the publisher, the editors and the reviewers. Any product that may be evaluated in this article or claim that may be made by its manufacturer is not guaranteed or endorsed by the publisher.
Research integrity at Frontiers
Learn more about the work of our research integrity team to safeguard the quality of each article we publish.