- 1FiBL Research Institute of Organic Agriculture, Frick, Switzerland
- 2BETA Technological Center, Vic, Spain
- 3Ecotop Foundation, La Paz, Bolivia
- 4Department of Geosciences and Natural Resource Management, University of Copenhagen, Copenhagen, Denmark
Ageing plantations, poor genetic material, soil degradation, pests and diseases are, among other factors, limiting cacao production. To meet the increasing demand for cacao in the absence of productivity gains, forests are cleared and the use of external inputs is generalised, with severe negative impacts on biodiversity and GHG emissions. The use of improved plant genetic material should support a sustainable increase of production. In this study, we evaluate and compare the yield performance of four locally-selected clones with those of four widely-used international clones in South America and four full-sib families (crosses of the same international clones). The research was conducted in a long-term trial in Bolivia with different production systems, including monocultures and agroforestry systems under organic and conventional farming and a successional agroforestry system without external inputs. Their cacao yields and the factors determining productivity (pod index, flowering intensity, pod load, pod losses, aboveground biomass, harvesting period) were assessed during 5 years. The cacao trees grown in the two monocultures had higher yields than those in the agroforestry systems. This was the result of higher aboveground biomass, flowering intensity and pod load, and similar pod losses due to cherelle wilt and fungal diseases in the former when compared with the latter. No differences between conventional and organic management were observed. We did not identify any genotypes performing better in a specific production system. On average, the local clones had twofold and five times higher yields than the international ones and the full-sib families, respectively. This was related to their higher total pod load, bigger pods and higher yield efficiency, i.e., higher yield per unit of tree biomass. However, the local clones had less flowering intensity, more cherelle wilt and similar losses due to fungal diseases to those of the international clones. This study clearly shows the need to invest in selection and breeding programmes using locally-selected genetic material to increase cacao production and support renovation/rehabilitation plans. Breeding genetic material that is adapted to low light intensities is crucial to close the yield gap between monocultures and agroforestry systems, and to further promote the adoption of the latter.
1 Introduction
The cacao tree (Theobroma cacao L.) is an ancient crop native to the lower Amazonian rainforest. Nowadays, cacao is an important cash crop for over 5 million smallholder farmers in many developing countries of Latin America, West Africa, and Southeast Asia (World Cocoa Foundation, 2017). Globally, the popularity of cocoa consumption is increasing rapidly, and the global market of cacao beans is expected to grow at an annual rate of 7.3% until 2025 (Grand View Research, 2019). In the meantime, the productivity of cacao trees is declining due to old plantations, poor genetic material, deficient management, soil degradation, and pests and diseases (Blaser et al., 2018; Effendy Pratama et al., 2019). In the past decades, vast areas of pristine rain forests have been cleared and converted into cacao farms to meet the growing demand (Rice and Greenberg, 2000; Vieira et al., 2008; Vaast and Somarriba, 2014; Orozco-Aguilar et al., 2021). Moreover, the traditional cultivation of cacao in shaded agroforestry systems has gradually shifted towards full-sun production for the purpose of increasing cacao yields in the short term (Franzen and Mulder, 2007; Vaast and Somarriba, 2014; Armengot et al., 2016).
Although the productivity of cacao trees can increase significantly when grown intensively in full-sun monocultures, these systems require more inputs (fertilisers, pest and disease management, irrigation, etc.) than agroforestry systems to maintain their productivity stable over longer periods (Ahenkorah et al., 1987). Together with deforestation, conventionally-managed monocultures are a major cause for the loss of biodiversity and ecosystem services (Klein et al., 2002; Milestad and Darnhofer, 2003; Foley et al., 2005; Morris, 2010). On the contrary, cacao agroforestry systems are more biodiverse than monocultures and can increase food security among farmers, contribute to climate change mitigation and adaptation, and increase energy efficiency (Perfecto et al., 2005; Mbow et al., 2014; Pérez-Neira et al., 2020, 2023).
Given the growing demand for cocoa and the need for massive renovation/rehabilitation plans (Somarriba et al., 2021) the use of improved planting material adapted to specific environmental conditions, with high-yielding profiles and low disease susceptibility, is overall a promising practice to improve productivity sustainably. In comparison with other tropical crops, such as rubber (Hevea brasiliensis), the genetic improvement of cacao through breeding has been rather slow due to several reasons, including long selection cycles, misidentification of parents, and lack of financial resources (Gutiérrez et al., 2016; Bekele and Phillips-Mora, 2019). Even though large collections maintain over 30,000 different genotypes only in Ecuador and Brazil (Bekele and Phillips-Mora, 2019), most breeding and selection programmes are based on less than 80 genotypes (Lopes et al., 2011; CacaoNet et al., 2012) and do not fully use the cacao diversity in the respective countries (Ceccarelli et al., 2022). It is estimated that more than 75% of cacao famers are currently using poor genetic material from unknown provenance, which is one of the major factors behind the low yields and high losses caused by fungal diseases (End et al., 2018). Improving the genetic material for agroforestry systems, where cacao yields are usually lower, is even more relevant. However, most breeding and selection of cacao genotypes is nowadays adapted to full-sun production. Breeding in agroforestry systems adds a source of variation mostly unwanted by breeders, and, in addition, the potential for increasing yields is higher in full-sun monocultures compared to agroforestry systems.
The biggest improvement in cacao breeding is represented by the discovery of heterosis around the 60s (Lopes et al., 2011). This led to the development of full-sib families, which are the result of controlled crosses between two selected clones. Compared with local selections, the progenies of hybrid crosses produce higher yields, and present higher resistance to biotic and abiotic stresses (Lopes et al., 2011). However, more recently, in America and Southeast Asia, the recommendation has shifted towards the use of grafted clones, which are highly resistant to specific fungal diseases, and high-quality cacao beans (End et al., 2018). An example of this recent shift towards grafted clones is found in the Alto Beni region of Bolivia, where the production of cacao has been improved through a selection programme carried out by the Bolivian cacao farmers’ cooperative El Ceibo.
In this study, we evaluate and compare the yield performance of four local clones from the El Ceibo selection with four international clones widely used in South America and four full-sib families (obtained from crosses of the same international clones) in various cacao monocultures and agroforestry systems in a long-term trial in Bolivia. Through this research, we aim to answer the question of whether cacao trees under full-sun monocultures and conventional management are more productive than under shaded agroforests and organic management, and whether locally-selected clones perform better than international clones and full-sib families. Furthermore, by directly comparing the different production systems on the same site, this study allows us to search for possible interactions between cacao genotypes and production systems, and to identify promising genotypes specifically adapted to shaded low-input systems.
To better understand the behaviour of the different genotypes and production systems, we also evaluate various crucial factors determining cacao productivity, such as the flowering intensity, pod load, pod losses due to cherelle wilt (the premature abortion of young pods) and fungal diseases, and vegetative growth of the cacao trees. Despite the economic relevance of cacao cultivation for millions of smallholders and for the large cocoa value chain, little information is available on the long-term potential of different cacao production systems [but see Somarriba and Beer (2011) and Ramírez-Argueta et al. (2022) for studies in agroforestry systems] and even less on their interaction with different genotypes. This study provides solid data about different production methods and helps understand which factors contribute the most to increasing productivity.
2 Materials and methods
2.1 Study site
The study site is located in Sara Ana, Alto Beni, Bolivia (15° 27′ 36.60” S and 67° 28′ 20.65” W), at an altitude of 380 metres above sea level, where the Research Institute of Organic Agriculture (FiBL, Switzerland) and its Bolivian partners, i.e., Ecotop Foundation, Instituto de Ecologia UMSA and PIAF- El Ceibo, established an experimental long-term trial in 2008/09 to compare conventional and organic cacao production in monoculture (full-sun) and agroforestry (shaded) systems, as well as different cacao genotypes. The region is characterised as tropical humid with dry winters. Between 1964 and 2019, the average annual precipitation was 1,540 mm, the average annual temperature 26.6°C, and the average annual relative humidity 83% at the nearby Sapecho weather station (410 m a.s.l., 15° 33′ 56” S and 67° 19′ 30” W). The soils are characterised as Luvisols and Lixisols.
2.2 Cacao genetic material
In total, 12 different cacao genotypes from three different genetic groups were compared, i.e., four local clones, four international clones, and four international full-sib families. The local genotypes, i.e., IIa-22, IIa-58, III-6, and III-13, were originally selected by the El Ceibo cooperative in areas close to the study site (the numbers refer to the area where they were selected). El Ceibo initially evaluated a total of 340 trees, of which 113 were selected. Thirty of them were prioritised for their high productivity [Trujillo, 2007; Institutio Nacional de Innovación Agropecuaria y Forestal (INIAF), 2016]. Eighty local selections from El Ceibo, including the ones selected for this study, were widely distributed to the farmers (Somarriba and Trujillo, 2005). All four genotypes were chosen because of their high yielding profile and tolerance to the fungal diseases black pod and witches’ broom, which caused severe yield losses until the 1990s [Institutio Nacional de Innovación Agropecuaria y Forestal (INIAF), 2016]. An early harvest period was also among the selection criteria, as a potential mechanism to escape from the peak of the black pod infection. In Bolivia, propagation through grafting started already in the 1990s.
The international clones selected were ICS-1, ICS-6, ICS-95, from the Imperial College Selection, and TSH-565, from the Trinidad Selection Hybrids. The choice was based on the yield evaluations of these clones made by Trujillo (2007) in the study region, more specifically, at the research station of the IBTA (Instituto Boliviano de Tecnología Agropecuaria), between the 1960s and the 1980s. ICS and TSH clones are widely used globally, as well as by farmers in the region because of their high production potential and moderate-to-high tolerance to fungal diseases (Phillips-Mora et al., 2005; Trujillo, 2007; Johnson et al., 2009). Except for TSH-565, all clones are self-compatible, i.e., they can be pollinated by the pollen of the same tree.
The full-sib families (henceforth, FS-families) were generated by using the four international clones as female parent and pollinating them with the clone from the Iquitos Mixed Collection IMC-67 (male parent). Hence, the resulting families ICS-1 × IMC-67, ICS-6 × IMC-67, ICS-95 × IMC-67, and TSH-565 × IMC-67. The IMC-67 clone is frequently used in crossings and as rootstock for grafting because of its tolerance to the fungal disease commonly known as mal de machete (Ceratocystis cacaofunesta) and it is high yielding (Maharaj et al., 2011). Moreover, it is reported to be a good pollen donor due to its high sexual compatibility (Trujillo, 2007; Phillips-Mora et al., 2012).
2.3 Production systems
The 12 genotypes were tested in five different production systems: two monocultures and two agroforestry systems under organic and conventional management, and one successional agroforestry system without external inputs (see Supplementary material 1). The cacao trees were planted at a spacing of 4 m × 4 m (625 trees ha−1) in all production systems, which is common practice in the study area (Quenta et al., 2005) and in other Latin American countries (Cerda et al., 2014). However, planting density in other regions and countries can increase up to 1,600 cacao trees ha−1 (Niether et al., 2020). The plantations of the conventional and organic monocultures consisted of cacao trees only. In the two agroforestry systems (under organic and conventional management), banana trees and different shade and timber trees were planted in the inter-row space between the cacao trees. The banana trees were planted at a spacing of 4 m × 4 m. At a lower density, shade trees were planted at 8 m × 8 m, and other fruit and timber species at 16 m × 8 m. The total shade tree density was about 304 trees ha−1. In the successional agroforestry system (SA), the same trees and crops as in the agroforestry systems were planted at the same density, but additional timber and shade trees, as well as understorey crops such as coffee, ginger and curcuma, were included. The total shade tree density was about 1,180 trees ha−1. The average canopy cover (measured monthly in 2018 and 2019 with a GRS densimeter in 84 points in each net plot) was 48, 34 and 37% in the SA, OA and CA, respectively. It changed over the year, with maximum values before the main shade tree pruning (SA: 60%, OA: 41%, OC: 45%) and minimum values just after the pruning (SA: 29%, OA: 22%, OC: 18%). Further details and the complete list of species planted in the trial can be found in Niether et al. (2018).
The herbaceous stratum in the conventional monoculture and agroforestry system plots was controlled with brush cutters and chemical herbicides, which were applied four to five times per year. The cacao trees in the conventional plots received two applications (March and December) of mineral fertiliser per year (Blaukorn BASF, Germany, 12–8–16-3 N-P2O5-K2O-MgO) from 2010 onwards. The monocultures received 112 kg ha−1 year−1, and the agroforestry systems received half of the dose, 56 kg ha−1 year−1. In the organic system plots, weeds were managed with a leguminous perennial (Neotonia wigthii) cover crop and, occasionally, with brush cutters. The organic plots were fertilised with self-made compost from 2010 onwards, 8 t ha−1 year−1 in the monocultures and 4 t ha−1 year−1 in the agroforestry systems. From 2017 onwards, the organic agroforestry system did not receive any compost. Half doses were applied to the agroforestry systems because the potential of added nutrients can only be fully exploited at high light intensities (Evans and Murray, 1953).
In all production systems, pests and diseases were fought exclusively by implementing cultural management practices, without the application of chemical pesticides or biological control agents. The practices consisted in harvesting and removing diseased pods every 2 weeks, regularly pruning to maintain canopy density and adjust the shade, and removing branches with visual symptoms of witches’ broom.
The field trial was established as a split-plot design with four blocks, five plots (corresponding to the five production systems), and 12 subplots (corresponding to the 12 genotypes; Supplementary material 1). In each block, each of the five production systems was randomly distributed among the plots. Each plot measured 48 m × 48 m, and comprised a net plot for data collection of 24 m × 32 m. The net plots of all production systems contained a total of 48 cacao trees. The genotypes were randomly distributed, but the distribution within each block was the same for all five production systems. In total, 960 trees were planted in the net plots, 80 of each genotype.
The cacao seedlings and grafted clones were grown in the nurseries of Ecotop and El Ceibo. The seeds of the rootstocks and the seeds of the FS-families were planted at the same time, between May and June 2008. The locally selected and the international clones were grafted onto 4- to 6-month-old seedlings that originated from the two clones IMC-67 and ICS-6 by open pollination. In November 2008, all seedlings of the FS-families and the grafted clones were ready to be transplanted into the field. The planting was completed within 7 weeks. The transplanted trees that died during their first year of growth in 2009 were replaced with the surplus trees of the same age that had been kept in the nurseries. Trees that died in the following years (24 trees) were replaced at the end of each cropping season (between November and March) with surplus young trees (6 to 8 months of age) from the nursery.
2.4 Data collection
The collection of data was performed at tree level over a period of 5 years, from 2015 to 2019. The mature pods were harvested approximately every 15 days all year round, even if the main production period starts in June and ends in September. At each harvest event, the number of healthy pods of each tree was registered and the total fresh bean weight of each tree was measured.
Fungal diseases of pods were recorded 1 day before each harvest event during a phytosanitary inspection. They included frosty pod rot (Moniliophthora roreri), black pod rot (Phytophthora spp.), and witches’ broom (Cripinellis perniciosa) and were identified in the field by recognising their visible infection symptoms. The number of pods affected by each disease was recorded and the pods were removed and left on the floor close to the tree trunk. In addition, if additional diseased pods were observed during the harvest event, the same procedure was applied. Pods damaged by insects and animals such as birds or small mammals were not taken into consideration, due to their very low incidence (only 0.36% were damaged) and because these losses are not expected to be related with the cacao genotype.
Furthermore, all pods lost due to cherelle wilt (a thinning mechanism leading to a premature abortion of the small fruits) were recorded following the same procedure as the one used for the diseased pods, i.e., the number of pods affected by cherelle wilt was counted during the phytosanitary inspection (and during the harvest, if any) and they were cut to avoid double counting. In 2017, 2018, and 2019, the number of unripe pods that were lost during the regular maintenance pruning, which usually occurs in early September, shortly after the main harvest period, was also recorded. Across all years, approximately 2% of the overall pod production was lost due to maintenance pruning. In 2015, the diameter of each cacao tree in the net plots was recorded to estimate the aboveground biomass. The diameter was measured 30 cm above ground. In case of ramifications below 30 cm, the diameters of all branches were measured at the height of 30 cm.
The flowering intensity was assessed every 15 days, also during the phytosanitary inspection. For every observation, the number of flowers on each tree was visually estimated and assigned a flowering intensity score ranging from 0 to 4, with 0 assigned if no flowers were present and 4 assigned if almost all branches were covered with a large amount of flowers (Esche et al., 2023). To guarantee an objective and homogeneous ranking, reference photos of the different genotypes representing the respective score were used and the assessments were always performed by the same observers.
2.5 Analysed variables
A detailed summary of all the variables analysed is included in the Supplementary material 2. The number of total pods produced annually was calculated by adding the healthy and the diseased pods of every sampling date. The fresh weight was converted into dry cacao bean weight by applying a factor of 0.33, which is the conversion factor used in previous studies conducted in the same field trial (Armengot et al., 2016, 2020). The cacao pod index, i.e., the number of pods needed to produce 1 kg of dry cacao beans, was calculated for each year by dividing the dry cacao bean weight by the number of healthy pods produced at tree level.
An assessment of the annual distribution of the cacao harvest was performed to identify the length and the starting, peak, and final day of the harvest period. For the sake of simplicity, the “harvest period” was defined as the estimated number of days required to produce 80% of the total annual production. The “first day” defines the estimated calendar day in which the harvest period starts; the “peak day,” the day when the harvest is peaking; and the “last day,” the day in which the harvest period is completed. The first day, the last day, and the production period of each tree were found by using the macro facility tool of the SAS software (SAS Institute Inc, 2015). The FS-families were excluded from these analyses because of their low production throughout the cropping year (see result section 3.1.1), which made it difficult to define the main production period with a start, an end, and a peak day.
Furthermore, all yield-determining factors were analysed, such as flowering intensity, cherelle wilt, pod load, incidence of fungal diseases, and aboveground biomass production. The flowering intensity scores, ranging from 0 to 4, were transformed into percentages, and the annual mean values of each tree were used for the analysis. The total pod load was calculated for every tree and year by adding the total number of pods lost due to cherelle wilt to the total number of healthy pods and the diseased pods. The percentages of healthy pods, wilted cherelles, and diseased pods were calculated in relation to the total pod load. The aboveground biomass was estimated through the allometric equation of Andrade et al. (2008), based on the trunk diameter measured at 30 cm (see details of the equation in Supplementary material 2). This equation was developed mainly for seed-based cacao plants, which have a distinct architecture than grafted plants. In our study, local and international clones were grafted and FS-families were seed-based. To our best knowledge, an allometric equation for cacao grafted trees has not been developed. For this reason, we used the same equation for the three genetic groups. The estimation of the biomass with this equation may not be fully accurate, but we decided to use it because the biomass values obtained corresponded very well to the observations in the field, i.e., FS-families were the biggest trees and the local selections the smallest trees (see results section). Allometric formulas estimate more accurately woody biomass, which represents almost 90% of the aboveground biomass (Bastide et al., 2006). Furthermore, the aboveground biomass was used to estimate the yield biomass ratio (YBR), i.e., the relationship between cacao yield and vegetative vigour. The YBR was a proxy for the tree yield efficiency (e.g., Daymond et al., 2002; Padi et al., 2012), calculated using the existing allometric equation for seed-based cacao plants.
2.6 Data analyses
The effect of the production system, the genotypes and their interaction across the 5 years of data was tested for all the variables analysed (except for the incidence of fungal diseases and frosty pod rot) using a model for split-plot designs with the MIXED procedure of the SAS 9.2 software and applying the Kenward-Roger approximation (SAS Institute Inc, 2015), according to the following model:
(1).
Where Yijklm is the trait in question, bi is the random effect of block i Sj is the fixed effect of production system j, bSij is the random interaction between block i and production system, Ck is the fixed of cultivar/full-sib family k, CSjk is the fixed interaction between production system j and cultivar/family k, bSCijk is the interaction between block i, production system j and cultivar k, yl is the random effect of year l; ybil is the random interaction between year l and block i; ySjl is the random interaction between yl and production system j; yCkl is the random interaction between yl and genotype k; yearijklm is the linear covariate of the planting year nested within year; and eijklm is the residual. A first-order autoregressive structure between years, with individual trees as subjects, was added in the MIXED procedure of the SAS 9.2 software (SAS Institute Inc, 2015).
Data were square root- or logarithmic-transformed in case of lack of variance homogeneity, examined by plots of studentised residuals as function of predicted values, or in case of skewed distributions of studentised residuals. A generalised linear mixed model for the total incidence of fungal diseases and frosty pod rot was applied due to highly skewed distributions. The procedure GLIMMIX of the SAS software was used for the generalised linear modelling, assuming a binomial distribution and using a logit link (SAS Institute Inc, 2015). Least square means were estimated and inverse logit-transformed values were calculated to obtain frequency estimates.
The plots did not show any outlier. In addition to the across-years analyses (2015–2019), a similar model was repeated for each year to verify the consistency of the results over the 5 years. Least square means were calculated for genotypes and production systems to estimate the correlation between years as a scale-free estimate of their repeatability. High correlations between the least square means of the genotypes between years indicate low interaction between genotype and year. Similarly, high correlations between the least square means of the production systems between years indicate low interaction between production and year, and vice versa. For most variables analysed, the correlations between years were high and significant, showing high consistency in rank for both genotypes and production systems (data not shown). However, for the variables “incidence of fungal diseases,” the correlations between years were low and non-significant, suggesting high production system × year and genotype × year interactions.
For all analyses, only trees planted between 2008 and 2012 were used. Generally, breeding programmes start the evaluation of cacao progenies after 5 years of planting (Dos Santos Días and Kageyama, 1998). Trees planted after 2012 were considered too young to provide consistent data throughout the data collection period. To adjust for the effect of different planting years, the planting year was included in the model as a covariate (see above) if it proved to have a significant effect at α = 0.05. Trees that died or stopped producing pods during the period of data collection were also excluded (because of their unknown cause of death). All progenies of the FS-families were grafted in 2018 with new genetic material because of their low and unstable yield performance (see the Results section). Therefore, all data collected in 2019 from these trees were not considered.
3 Results
3.1 Cacao production
3.1.1 Dry bean yield, number of pods and pod index
The overall cacao dry bean yield and the number of harvested pods were on average 1.24 kg tree−1 year−1 and 30.7 per tree−1 year−1, respectively.
We found significant differences in the dry bean yield between production systems: the trees under conventional and organic monocultures were the highest yielding trees across all 5 years, followed by the cacao trees in the agroforestry systems, and the ones in the SA (Figure 1A; Table 1; Supplementary material 3). On the other hand, in the SA system, the cacao trees produced the biggest pods with the highest dry bean yield per pod, as indicated by its significantly lower pod index (19.7) compared with the other production systems (the overall average pod index of the other production systems was 23; Table 1; Supplementary material 3). The two monocultures had the highest pod index (conventional: 25.9; organic: 23.4), and therefore produced the smallest pods. No significant differences were found between organic and conventional management in the monocultures or in the agroforestry systems for the variables dry cacao bean yield, number of pods, and pod index (Figure 1A; Supplementary material 3).
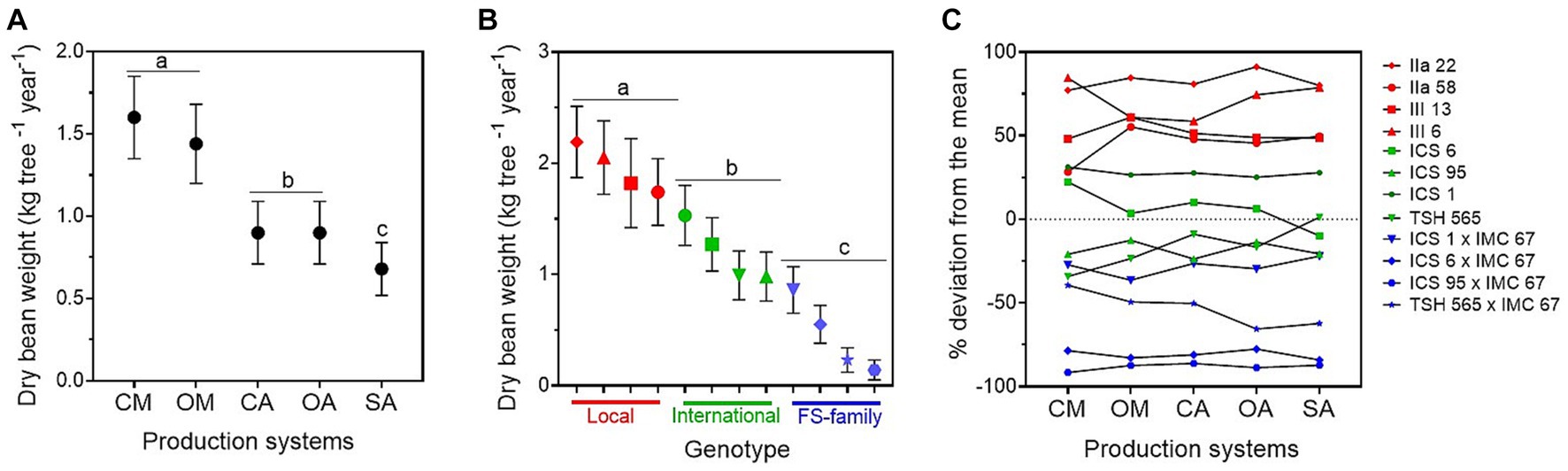
Figure 1. Annual mean dry bean yield (kg tree−1) ± standard error (average for the years 2015–2019) estimated for (A) the five cacao production systems, i.e., conventional monoculture (CM), organic monoculture (OM), conventional agroforestry (CA), organic agroforestry (OA), and successional agroforestry (SA); and for (B) the genetic groups, i.e., local clones in red, international clones in green, and full-sib families in blue. In (C), deviation of the cocoa yield of the different genotypes (in percentage) from the mean. It was estimated as the least square mean for the genotype plus the least square mean for the production system × genotype interaction over the overall mean of the least square mean estimates.
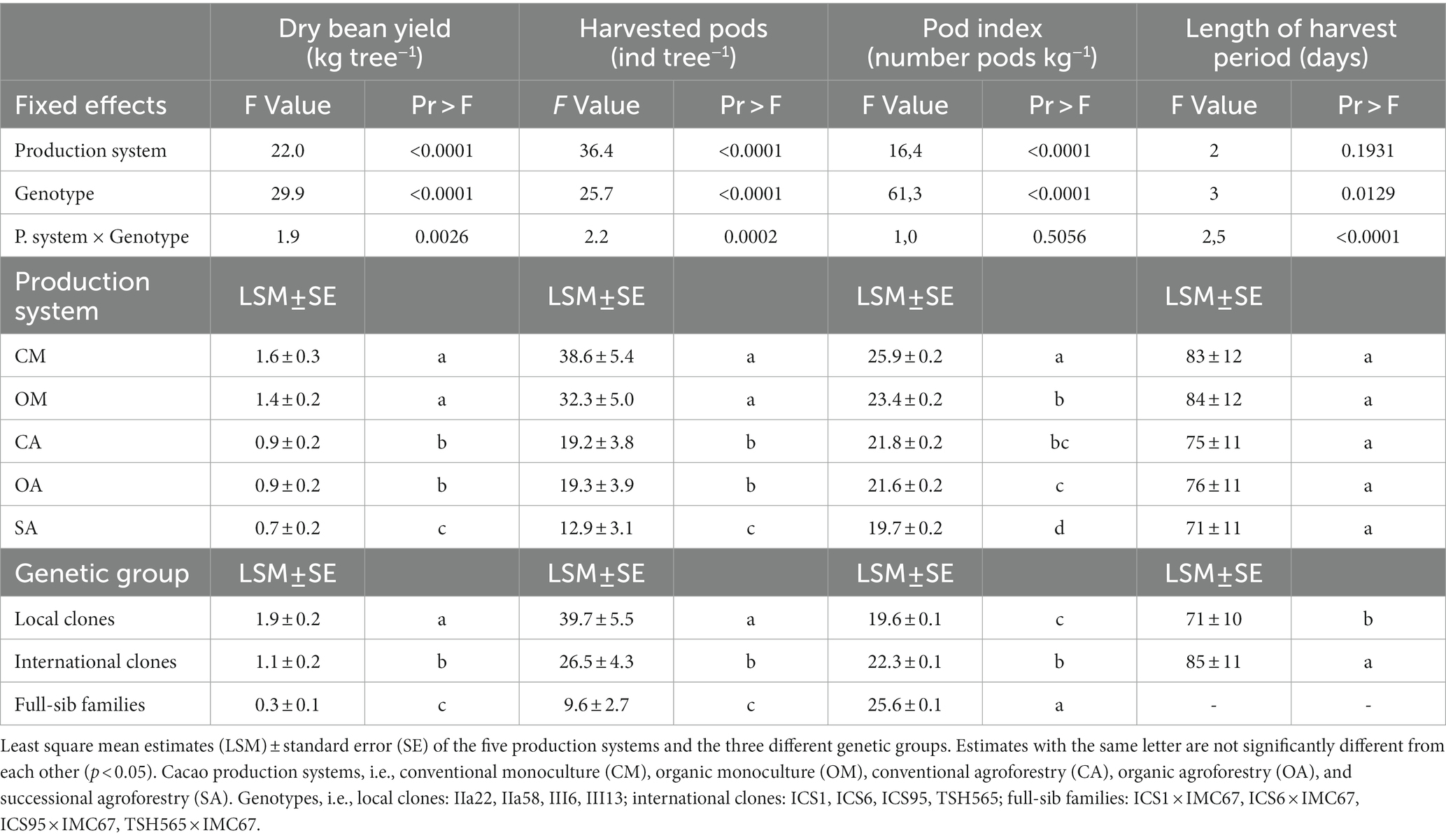
Table 1. Anova results testing the effect of the production system, genotype and their interaction on the dry bean weight, number of harvested pods, pod index, and harvest period from 2015 to 2019.
Across production systems, the local clones produced the highest yield and number of pods, and differed significantly from the international clones and FS-families (Table 1; Figure 1B). Overall, IIa-22 and III-6 were the highest yielding clones, but there was no significant difference between IIa-58 and III-13 and the best international clone, ICS-1 (Figure 1B; Supplementary material 3). Over the whole period analysed, the FS-families presented not only the lowest yield but also the highest heterogeneity in yield production. For instance, in 2018, the number of pods per tree produced by the FS-families ranged from 4 to 26 pods, and 5 to 40% of their progenies did not produce any pods at all. In addition, the local clones produced bigger pods than the international ones and the FS-families, as indicated by their lower pod indexes (Table 1). In the 5 years analysed, on average, the local clones needed 19.6 pods to produce 1 kg of dry cacao, the international ones 22.3, and the FS-families 25.6.
The interaction between production systems and genotypes was significant for the dry bean yield (Table 1). However, the rank of best-performing genotypes hardly changed, i.e., the best genotypes had good performances in all five systems and the worst ones performed badly in all of them too (Figure 1C).
3.1.2 Harvest period
On average, the main harvest period lasted approximately 80 days, starting on calendar day 161 (around June 10), peaking on day 198 (around July 17), and ending on day 248 (around September 5). Across years, no significant differences were found between production systems concerning the first and the last days, the peak day, and the length of the harvest period. However, a non-significant trend towards shorter length was observed in the agroforestry systems, especially the SA, in comparison with the monocultures (Table 1; Supplementary material 4).
In contrast, we found significant differences between genotypes for all the above-mentioned variables (Table 1; Supplementary material 4). On average, the local clones started their main harvest period 24 days earlier than the international clones, and had a shorter production period by 13 days (Table 1; Figure 2). Moreover, the local clones reached the peak day only 34 days after the harvest period started, 32 days earlier than the international ones, and completed their harvest 39 days before the international clones.
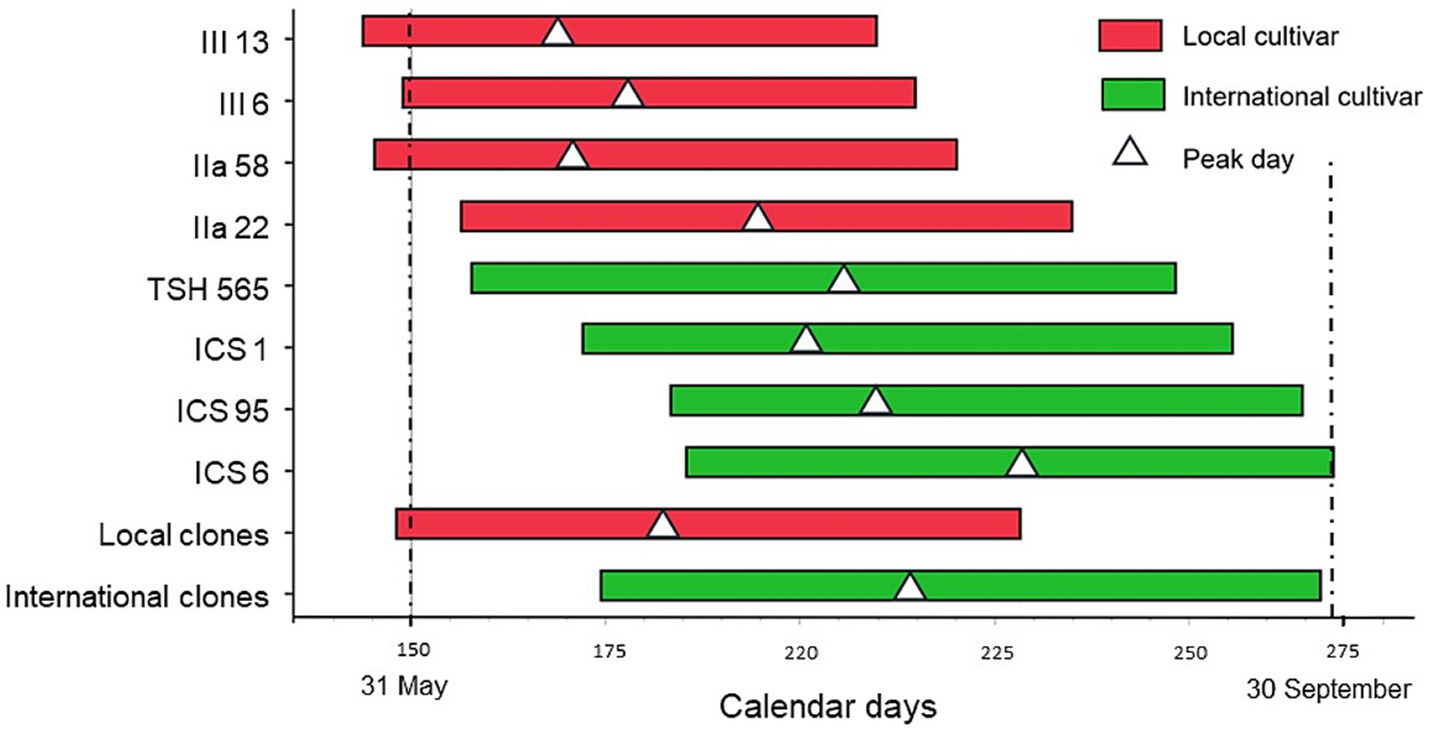
Figure 2. Estimated harvest period and peak day (white triangle) for the genetic groups, with local clones in red and international clones in (green).
3.2 Factors determining cacao production
3.2.1 Flowering intensity
The average flowering intensity across the years was 32%, with significantly higher values in the monocultures than in the agroforestry systems (Figure 3; Table 2; Supplementary material 5). There was no significant difference between conventional and organic management, neither in the monocultures nor in the agroforestry systems. The lowest flowering intensity was recorded in the SA.
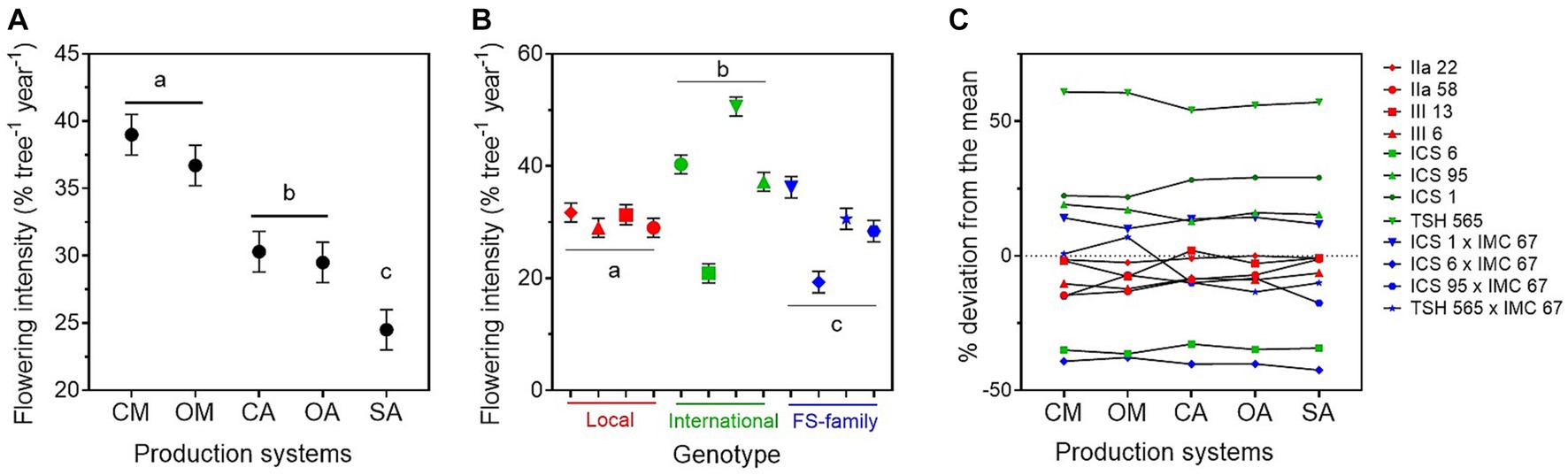
Figure 3. Annual flowering intensity ± standard error (average for the years 2015–2019) estimated for (A) the five cacao production systems, i.e., conventional monoculture (CM), organic monoculture (OM), conventional agroforestry (CA), organic agroforestry (OA), and successional agroforestry (SA); and for (B) the varieties of the three genetic groups, i.e., local clones in red, international clones in green, and full-sib families in blue. In (C), deviation of the flowering of the different genotypes (in percentage) from the mean. It was estimated as the least square mean for the genotype plus the least square mean for the production system × genotype interaction over the overall mean of the least square mean estimates.
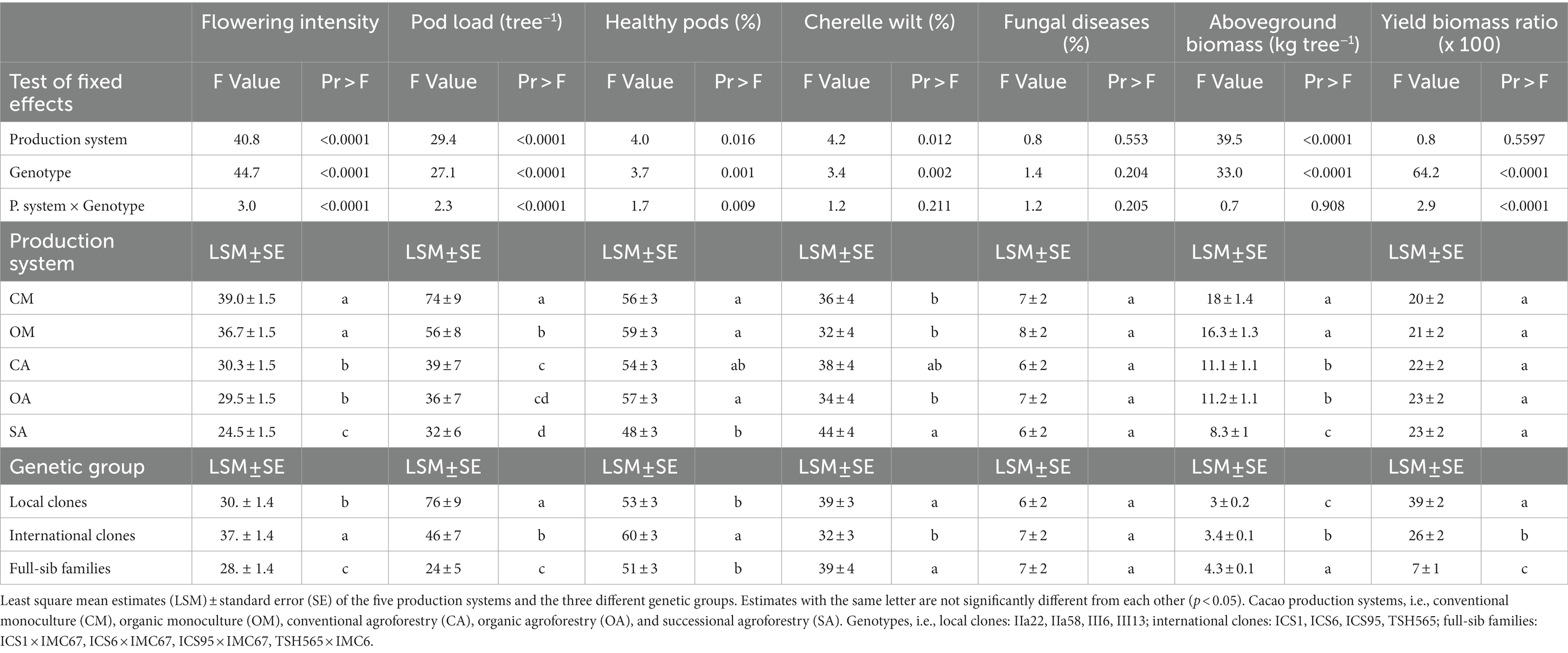
Table 2. Anova results testing the effect of the production system, cacao genotype and their interaction on flowering intensity, poad lod, percentage of healthy pods, cherelle wilt and pod affected by fungal diseases, aboveground biomass and yield biomass ratio from 2015 to 2019.
We found relevant differences in flowering intensity between genotypes. The international clones had higher flowering intensity than the local clones and the FS-families (Table 2; Figure 3B; Supplementary material 5). TSH-565 was by far the genotype with the highest flowering intensity (51% across years), and ICS6 the one with the lowest. The interaction between genotypes and production systems was significant (Table 2). For instance, we found that the FS-family TSH-565 × IMC-67 had a low flowering intensity in the shaded systems and a much higher one in the monocultures (Figure 3C). Nevertheless, the interaction was small compared to differences between genotypes (Figure 3C).
3.2.2 Pod load: cherelle wilt, diseased and healthy pods
Overall, the trees produced an average pod load of 49 pods tree−1 year−1 across all years, including harvested pods and pods lost due to cherelle wilt and diseases. On average, 55% of the pod load developed into mature healthy pods, 37% was lost due to cherelle wilt and 7% due to fungal diseases.
Pod load differed between production systems. Conventional monoculture produced the highest pod load, followed by the organic monoculture; in both systems, the pod load was significantly higher than in all agroforestry systems (Table 2). The percentage of losses due to both cherelle wilt and fungal diseases did not significantly differ between production systems, except for the SA, which had more premature abortions (Table 2). This resulted in a higher absolute number of healthy pods in the systems with higher pod load, i.e., monocultures (Table 1).
The group of local clones produced by far the highest pod load, followed by the international clones and the FS-families (Table 2; Supplementary material 5). All genotypes produced higher pod load in the two monocultures, especially in the conventional one, except for TSH-565, which was the only genotype producing the same amount in all five production systems (Supplementary Figure S5; material 5).
The international clones showed significantly lower incidence of cherelle wilt (31.7%) than the local ones (39.3%) and the FS-families (39%). In contrast, losses due to diseased pods were overall very low (4.2% on average) and did not differ significantly between genotypes (Table 2; Supplementary material 5). Excluding the pods lost due to cherelle wilt, the average incidence of fungal diseases was overall 14% across all years. Frosty pod rot had the highest incidence (9%), followed by black pod rot (3%) and witches’ broom (1%). As a result, the percentage of healthy pods was highest among the international clones. However, the absolute number of healthy pods harvested was higher for the local clones due to the higher pod load produced.
3.2.3 Tree aboveground biomass
Tree aboveground biomass, estimated through the allometric equation of Andrade et al. (2008), was highest in the monocultures, followed by the two agroforestry systems and, finally, the SA, and no differences were found between organic and conventional management (Figure 4; Table 2). In contrast, the estimation of the dry bean yield produced per unit of biomass, i.e., the yield biomass ratio (YBR), was similar in all production systems (Figure 4; Table 2).
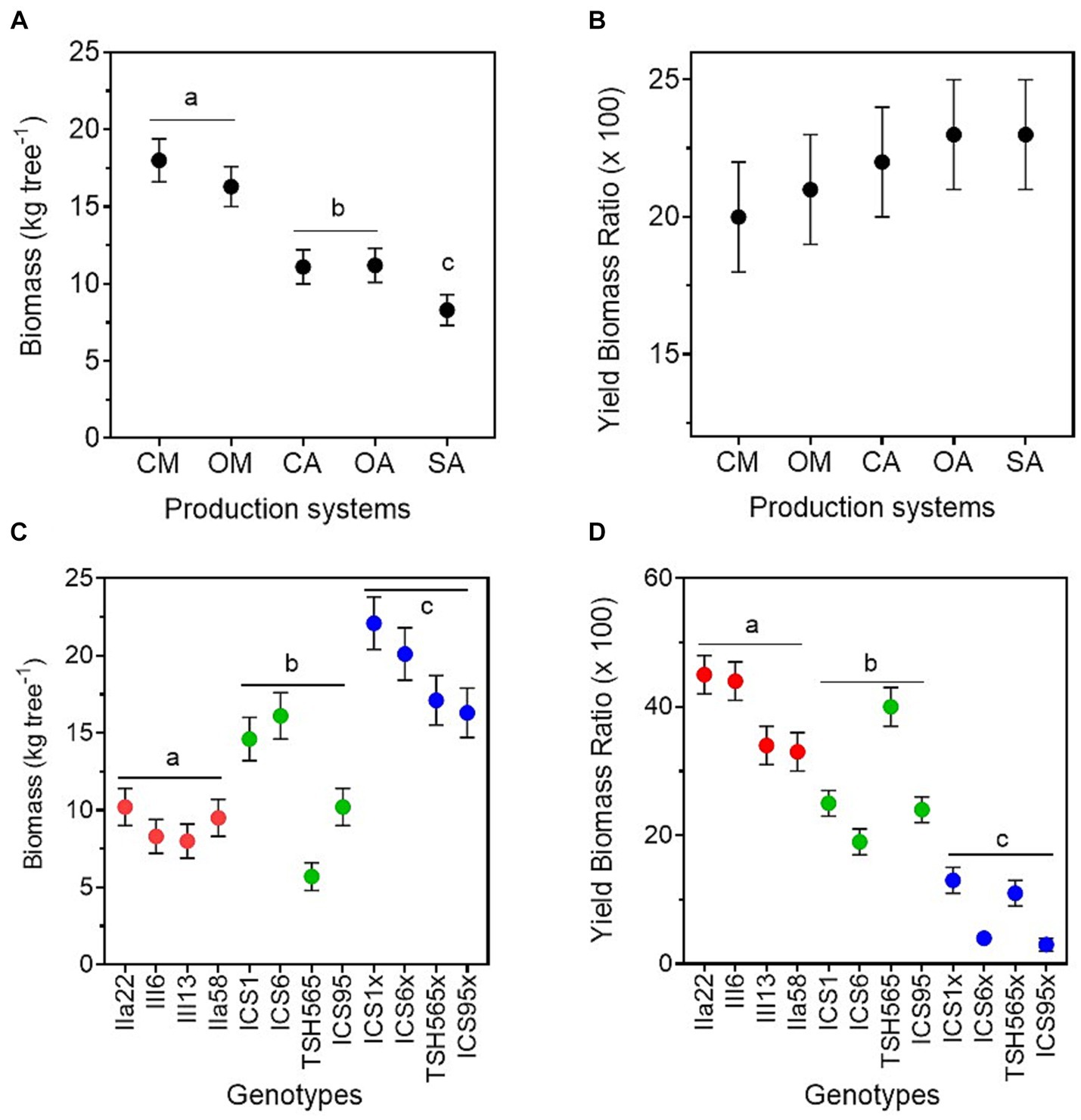
Figure 4. Aboveground tree biomass and dry bean weight relation with aboveground tree biomass for the five cacao production systems (A,B), i.e., conventional monoculture (CM), organic monoculture (OM), conventional agroforestry (CA), organic agroforestry (OA), and successional agroforestry (SA), and for the three genetic groups (C,D), i.e., local clones in red, international clones in green, and full-sib families in blue.
The tree aboveground biomass also differed between genetic groups, being highest among the FS-families and lowest among the local clones (Supplementary Figure S6; Table 2; Supplementary material 6). However, the lowest value was found for the international clone TSH-565. Contrary to what happened in the production systems, there were differences in the YBR between genotypes. The local genotypes had the highest YBR, followed by the international clones and the FS-families. In the same line, we found a highly significant negative correlation between individual tree biomass and pod load (−0.85, p = 0.0009). In other words, the genotypes with higher aboveground biomass per tree produced lower pod load and lower yield than the genotypes with lower aboveground biomass. Interestingly, the international clone THS-565 increased its YBR notably in the agroforestry systems and had the highest YBR in the SA (Supplementary material 6), but with low yield and biomass per tree, as mentioned above.
4 Discussion
4.1 Production systems
The cacao trees grown in the two monocultures showed a higher number of pods and a higher yield than the agroforestry systems. This was the result of their higher flowering intensity, higher total number of pods produced (pod load), similar pod losses due to cherelle wilt and fungal diseases, and higher aboveground biomass compared with the cacao trees in the agroforestry systems.
Even though cacao is a shade-adapted species and is traditionally grown in forest/agroforestry systems, its productivity can significantly increase in full-sun systems (Ahenkorah et al., 1987; de Almeida and Valle, 2007; Blaser et al., 2018). For instance, below certain light intensities, cacao trees suppress their flower production (Asomaning et al., 1971), which causes a significant reduction in pod loading. Furthermore, competition for nutrients and water between cacao trees and agroforestry trees may also limit cacao yields in agroforestry systems (De Almeida and Valle, 2007). In our study, cacao trees in the agroforestry systems received half of the dose of the monocultures, in view of the lower efficiency in the uptake of nutrients at lower light intensities (see “Material and methods”). However some studies have reported increased cacao productivity in the presence of legum trees, even at short distances from the cacao trees (Nygren et al., 2013; Notaro et al., 2021). In addition, a complementary use of water between cacao trees (which have a shallow root system) and agroforestry trees (usually with deeper rooting systems) has been observed (Niether et al., 2017). However, shade in agroforestry systems reduces the transpiration rates of cacao trees (Saavedra et al., 2020; Blaser-Hart et al., 2021), which may lead to a lower soil nutrient uptake and limit the yield. This may also explain the smaller trees found in the agroforestry systems compared with the monocultures, which had an influence on the final yield. Interestingly, the yield produced per unit of aboveground biomass (YBR) was similar in both systems, which resulted in lower yields produced by smaller trees, i.e., cacao trees in the agroforestry systems. The YBR also indicated that the trees allocated similar resources to reproduction per unit of biomass. It is expected that the cacao trees in the agroforestry systems will eventually reach a similar size as the ones in the monocultures, since they grow at a slower pace than those in monocultures (Schneider et al., 2016). This can contribute to increasing the yield and may reduce the yield gap between shaded and full-sun production systems. The methodology for assessing the aboveground biomass using allometric equations estimates more accurately the woody biomass than leaf biomass, since they are based on the trunk dimensions. The relationship between the aboveground biomass and yield found in this study is therefore more directly related to the woody biomass rather than to the leaves, which only account for about 10% of the total biomass (Bastide et al., 2006).
With regard to diseases, the incidences were overall rather low when compared with those found in other studies (Krauss and Soberanis, 2001; Phillips-Mora and Wilkinson, 2007). This could be due to the good implementation of cultural management practices, which consist in regularly pruning and harvesting diseased pods throughout the year, and removing branches with visual infection symptoms (Armengot et al., 2020). This contrasts with some local recommendations to cut shade trees in order to prevent fungal diseases (personal communication, El Ceibo). However, contrasting results concerning the role of agroforestry systems on pests and diseases are also found in the literature (Niether et al., 2020).
Interestingly, when comparing organic and conventional management, we did not find any differences in cacao yield between the monocultures and the agroforestry systems, which shows the potential for organic cacao production. This is not the case for most of the crops: an overall yield gap of about 20% is usually reported (De Ponti et al., 2012), but this seems to be very crop-specific. Lower cacao yields were produced in the organic monoculture, compared with the conventional one, during the first years of the trial (Armengot et al., 2016). This shows the importance of analysing long-term data. In addition to the well-known benefits of organic production for the environment, including diversity conservation and reduced environmental impact, among others (Marconi and Armengot, 2020; Armengot et al., 2021; Pérez-Neira et al., 2023), organic management has lower costs (Armengot et al., 2016; Pérez-Neira, 2016) and is more economically efficient (Caicedo-Vargas et al., 2022) than the conventional production. The potential difference in resource availability between conventional and organic agroforestry systems (the conventional system received mineral fertilisers consistently throughout the period, whereas no compost was applied in the organic systems from 2017 onwards) did not translate into a difference in production or in any of the other parameters analysed (e.g., flowering or pod load). Consequently, the limitation of light (see “Material and methods”) could be the reason why the available extra resources were not exploited (Evans and Murray, 1953; Ahenkorah et al., 1987). For the same reason, even though we cannot discount the influence of the reduced fertiliser dose in the lower yields of the trees under agroforestry compared to the monocultures, the limitation of light in the agroforestry systems might have reduced the uptake of nutrients by the trees in the scenario of equal dosage. In the case of the monocultures, we observed that the lower number of pods produced in the organic system was compensated by a higher weight per pod. This explains that, even though the organic monoculture produced less pods than the conventional one, the final yield harvested was similar under both managements. The pod index can be determined by both the number of beans per pod and the bean weight (Lachenaud, 1995). A higher pollination intensity in the organic systems could have increased the dry bean weight per pod. But it could also be related to a competition for resources between the number of pods and the number of seeds per pod (Lachenaud, 1995). This compensation was also observed in relation to the agroforestry systems, i.e., the dry seed weight per pod was higher in the agroforestry systems compared with the monocultures. However, this mechanism was not enough to reach similar values in dry bean yield between monocultures and agroforestry systems. It is important to mention that the dry seed weight is relevant for cacao buyers, i.e., a high seed weight is a positive component of seed quality.
4.2 Genotypes
This study demonstrates the importance of selecting and using local genetic material for cacao production, since the locally-selected genotypes performed better than the recommended international clones and the FS-families in all the parameters assessed.
4.2.1 Yield and productivity
On average, the local clones produced 50% more pods than the international ones and four times as many as the FS-families. Other elite tree-selection programmes in various cacao-producing countries in Latin America and West Africa have reported positive results as well (Lachenaoud et al., 2007; Ahnert and Eskes, 2018; Feumba de Tchoua et al., 2021). The yield data presented here do not reflect the yield potential of the cacao trees, since the trees were not yet fully grown (data collection included 6 to 10 years after planting).
The yield data for the international genotypes were lower in this study than in the literature (e.g., Johnson et al., 2004). It is also worth mentioning that we expected lower competitive interactions between cacao trees in this study (625 cacao trees ha−1) compared to others with higher cacao planting densities. At higher cacao densities, the competition starts quite early in the production phase (Trebissou et al., 2021). International clones are usually managed at high densities, even twice the density in this trial. Genetic selection of trees based on the performance of individual genotypes does not usually consider the competitive interactions between cacao trees (and even less with other trees in the system) that develop over time (Trebissou et al., 2021), which can be substantial in highly competitive and highly-yielding individuals. Therefore, the performance of the local selections should be evaluated also at higher densities to consider this effect before recommending their use at a different planting scheme.
Overall, the grafted clones, i.e., the local and international clones, performed significantly better than the FS-families, which showed considerably lower yields and high heterogeneity throughout the studied years. The poor production performance of the FS-Families may be partly attributed to a poor breeding value of the common father IMC-67 in terms of reproduction at the study site. Phillips-Mora et al. (2012) reported very low levels of inter-compatibility of IMC-67 as mother and father, which contradicts the widespread belief that this clone acts as a universal pollen donor in the plantations, and it have been found to be incompatible with some clones (Cadavid-Vélez, 2006). Our results cannot definitively exclude the possibility of using seed-propagated seedlings. Actually, until the late 1990s such crosses between Upper Amazon clones (IMC) and Trinitarios (ICS and TSH) were the ones usually recommended to increase yield potential and disease tolerance (CacaoNet et al., 2012). Nevertheless, our study demonstrates that utilising tested clones can lead to earlier, stable, and high productivity. In general, seed-planted trees need more time to reach the reproductive stage than clones, which are grafted with physiologically older material. In our case, even if the FS-families significantly increased their productivity in the following years, they would hardly be able to compensate for the low production of their long juvenile period. Actually, the main motivation for the grafting programme started in the Alto Beni region of Bolivia in the early 1990s was the low performance of the FS-families that were planted during the 1960s and 1970s (Quenta et al., 2005). Initially, international clones were widely distributed and used by farmers in the region, but they did not produce as much as it was expected. The local elite tree-selection programme was then initiated by the El Ceibo cooperative to improve the cacao yields (Somarriba and Trujillo, 2005).
Not only did the local clones produce the highest number of pods, but they also had the lowest pod index (i.e., bigger pods). A low pod index is not only an important breeding and selection criterion (bigger pods with more and bigger beans; Cilias et al., 2010), but it is also of particular interest to farmers, since, with the same yield, a lower pod index reduces the amount of labour needed for harvesting and opening the pods. For instance, the clones IIa-58 and ICS-95 produced a similar number of pods, but the international ICS-95 needed almost twice as many pods to produce the same amount of dry cacao beans as IIa-58 (Supplementary material 3).
The highest pod indexes were found among the FS-families, indicating that they produced not only fewer pods, but considerably smaller ones with a lower dry bean weight. This is in contrast to the results obtained from the production systems, where the trees in the less productive systems (e.g., SA) compensated to some extent their low pod number with bigger pods.
4.2.2 Harvest period
The local clones started and finished their main harvest period significantly earlier than the international ones, and they also had the earliest harvest peak and the shortest harvest period. These parameters are in fact important escape mechanisms against fungal diseases (Maddison et al., 1995). An early harvest period was among the selection criteria of the cacao tree-selection programme of El Ceibo (Trujillo, 2007); the aim was to avoid pod infection by black pod disease, which was the main disease at that time in the study region. However, our results do not show any clear differences in the incidence of fungal diseases.
In addition to the potential advantages of disease avoidance, a shorter harvest period increases labour efficiency by reducing the number of days required for harvesting. It is important to highlight that a shorter harvest period did not indicate a lower pod production in this study; actually, the locally-selected clones produced higher yields. Moreover, a shorter and more compact harvest period facilitated the management of agronomic practices. For instance, we found a high and significant correlation between the last harvest day and the percentage of unripe pods cut during regular maintenance pruning (an average of 1.6% of the total number of pods produced, data not shown).
4.2.3 Yield potential and yield-determining factors
When looking at the individual genotypes, we found that flowering intensity was not related to pod load and yields, contrary to the results obtained for the production systems. For instance, the local clones, which were the highest-yielding ones, showed a significantly lower flowering intensity compared with the international clones. In addition, the highest flowering intensity was registered for TSH-565, the clone with the lowest pod load and yield. Since we did not manipulate pollination in this study, the lack of correlation between pod load (and pod production) and flower intensity indicates that other factors, such as sexual incompatibility for some genotypes and plant resources limitation/allocation, may have been important drivers determining the final pod production. Among the international clones in this study, only TSH-565 is known to be highly self-incompatible (Lopes et al., 2015), for it depends 100% on pollen from other genotypes to achieve successful fertilisation. This might explain why TSH-565 produced the lowest pod load among the tested clones despite its high flowering intensity.
Valle et al. (1990) showed that with an increasing pod production, the number of flowers gradually decreases because they compete for assimilates with the maturing pods. This could explain the significantly higher flowering intensity of the international genotypes TSH-565, ICS-1, and ICS-95, which may have not produced enough pods to suppress the resource allocation that induces flowering.
Vegetative growth is another important sink that competes with the production of flowers and pods (Valle et al., 1990). A strong vegetative growth could potentially reduce pod load, independently of the amount of fertilised flowers. We found that the local clones were more efficient in allocating assimilates to reproduction than the other genotypes, since they showed the highest yield biomass ratio, i.e., a proxy for the tree yield efficiency calculated using an allometric equation for seed-based cacao trees. Several authors consider yield efficiency to be an important trait of the improvement of cacao productivity (Daymond et al., 2002; Padi et al., 2012). Additionally, breeding smaller trees with higher productivity could increase the productivity per cultivated area (Larsen et al., 1992; Lachenaud, 1995) and reduce the time devoted to management tasks, for example, pruning activities. We should consider that the allometric equation used to estimate the cacao tree biomass was developed for seed-based cacao trees but it was applied to both seed-based (FS-families) and grafted (local and internal clones) cacao plants (see “Materials and methods”). This might have overestimated the biomass of the grafted trees. But even in this case, our results showed lower tree biomass and higher yield efficiency of the local clones compared to the international ones and the FS-families.
4.2.4 Pod losses due to cherelle wilt and fungal diseases
The final number of pods reaching maturity is mainly conditioned by the physiological phenomenon known as cherelle wilt, and further limited by pest and diseases.
Losses due to cherelle wilt are usually high, reaching up to 75% (Bos et al., 2007). In this study, the average incidence of cherelle wilt was 37%. This is explained by the fact that the pollinated flowers dropping soon after pollination could not be registered, only the ones where the wilted pod remained attached to the trunk. However, the genotypes tested showed high variability in the percentage of cherelle wilt, and this was not consistently related to the three genetic groups. For instance, the highest percentage of cherelle wilt was observed in the FS-family ICS-95 × IMC-67 and the local clone III-13, which were the genotypes with, respectively, the lowest and highest pod load. The number of aborted pods could be linked to vegetative growth and vegetative events such as leaf flushing (Valle et al., 1990). This would explain the high losses in the low-producing FS-families, which overall had the highest tree biomass. But cherelle wilt is also the physiological mechanism through which trees regulate the number of pods according to nutrient availability (Valle et al., 1990). Therefore, the higher cherelle wilt percentage of the local genotypes compared with the international ones could be explained by the higher pod load of the former.
The total incidence of fungal diseases was quite low considering the high potential for yield losses (Ten Hoopen et al., 2012). Overall, we did not find any differences in the incidence of fungal diseases among the genetic groups. With higher disease pressure we might have observed a clearer pattern in susceptibility and resistance, as described for some of the genotypes tested: for instance, the inherited resistance of ICS-95 to frosty pod rot (Phillips-Mora et al., 2005) but high susceptibility of ICS-1 and TSH-565, which was nevertheless described as resistant in another study (Lopes et al., 2015). The active limitation of spore dispersal through fortnightly removal of infected pods may have been more important in suppressing disease outbreaks than genotype characteristics.
5 Conclusion
The highest yield of the local selections compared to the international clones (ICS-1, ICS-6, ICS-95, and TSH 565) and full sib-families (ICS-1 × IMC-67, ICS-6 × IMC-67, ICS-95 × IMC-67, and TSH-565 × IMC-67) clearly show the need to invest in local selection and breeding programmes using locally-selected genetic material to increase cacao production. To validate the performance and recommend the further propagation of the local selections evaluated in this study, additional evaluations need to be done at farmers’ fields. This would allow to explore the capacity and adaptability of these clones to sustain their performance in different planting contexts, e.g., pollen genetic diversity (different neighbouring cacao trees) or nutrients availability. Farmers’ organisations should be empowered to select and evaluate their own best-performing local material, which might result in better yield improvements than those of international or national governmental programmes. Farmers should have direct access to the improved genetic material.
We did not identify any genotypes performing better in a specific production system, i.e., the best-performing genotypes (mainly the local IIa-22 and III-6) were so in all production systems. But our results highlight the potential of organically-managed cacao production systems, since they reach similar yields to those of conventional systems. In addition, the lower cacao yields recorded in the agroforestry systems compared with the full-sun monocultures indicate the need to select and breed genetic material that is better adapted to low light intensities. This might help increase cacao production in agroforestry systems and favour a broader adoption of these more sustainable systems, compared to full-sun systems.
Data availability statement
The raw data supporting the conclusions of this article will be made available by the authors, without undue reservation.
Author contributions
LA, MS, and JM contributed to conception and design of the study. MP and LA organised the database and wrote the first draft of the manuscript. MP and JH performed the statistical analysis. All authors contributed to the article and approved the submitted version.
Funding
This study is part of the SysCom Bolivia project (https://systems-comparison.fibl.org/project-sites/bolivia.html), financed by the Swiss Agency for Development and Cooperation (SDC), the Liechtenstein Development Service (LED), the Biovision Foundation for Ecological Development, and the Coop Sustainability Fund. LA was supported by the Ramón y Cajal RYC2021-032601-I fellowship, funded by MCIN/ AEI/10.13039/501100011033 and UE “NextGeneration EU”/PRTR.
Acknowledgments
The authors thank all the staff at Sara Ana Research Station for their support in data collection.
Conflict of interest
JM has been employed by ECOTOP SRL and ECOTOP Suisse GmbH.
The remaining authors declare that the research was conducted in the absence of any commercial or financial relationships that could be construed as a potential conflict of interest.
Publisher’s note
All claims expressed in this article are solely those of the authors and do not necessarily represent those of their affiliated organizations, or those of the publisher, the editors and the reviewers. Any product that may be evaluated in this article, or claim that may be made by its manufacturer, is not guaranteed or endorsed by the publisher.
Supplementary material
The Supplementary material for this article can be found online at: https://www.frontiersin.org/articles/10.3389/fsufs.2023.1253063/full#supplementary-material
References
Ahenkorah, Y., Halm, B. J., Appiah, M. R., Akrofi, G. S., and Yirenkyi, J. E. K. (1987). Twenty years’ results from a shade and fertilizer trial on Amazon cocoa (Theobroma cacao) in Ghana. Exp. Agric. 23, 31–39. doi: 10.1017/S0014479700001101
Ahnert, D., and Eskes, A. B. (2018). “Developments in cacao breeding programmes in Africa and the Americas” in Achieving sustainable cultivation of cocoa. ed. P. Umaharan (Cambridge: Burleigh Dodds Science Publishing), 1–40.
Andrade, H. J., Segura, M., Somarriba, E., and Villalobos, M. (2008). Valoración biofísica y financiera de la fijación de carbono por uso del suelo en fincas cacaoteras indígenas de Talamanca, Costa Rica. Turrialba (Costa Rica). Centro Agronómico Tropical de Investigación y Enseñanza.
Armengot, L., Barbieri, P., Andres, C., Milz, J., and Schneider, M. (2016). Cacao agroforestry systems have higher return on labor compared to full-sun monocultures. Agron. Sustain. Dev. 36:70. doi: 10.1007/s13593-016-0406-6
Armengot, L., Beltrán, M. J., Schneider, M., Simón, X., and Pérez-Neira, D. (2021). Food-energy-water nexus of different cacao production systems from a LCA approach. J. Clean. Prod. 304:126941. doi: 10.1016/j.jclepro.2021.126941
Armengot, L., Ferrari, L., Milz, J., Velásquez, F., Hohmann, P., and Schneider, M. (2020). Cacao agroforestry systems do not increase pest and disease incidence compared with monocultures under good cultural management practices. Crop Prot. 130:105047. doi: 10.1016/j.cropro.2019.105047
Asomaning, E. J. A., Kwakwa, R. S., and Hutcheon, W. V. (1971). Physiological studies on an Amazon shade and fertilizer trial at the cocoa research institute, Ghana. Ghana J. Agricul. Sci. (Ghana) 4, 47–64.
Bastide, P., Aguilar, P., Lachenaud, Ph, Paulin, D., Jimmy, I., and Bouletare, G. (2006). Yield variation and biomass measurements on mature cocoa trees in Vanuatu. In Proceedings of the 15th International Cocoa Research Conference, San José, Costa Rica. Ghana, Nigeria: Cocoa Producers’ Alliance (COPAL)/CATIE.
Bekele, F., and Phillips-Mora, W. (2019). “Cacao (Theobroma cacao L.) breeding” in Advances in plant breeding strategies: Industrial and food crops. Eds. J. Al-Khayri, S. Jain, and Johnson (Cham: Springer), 409–487.
Blaser, W. J., Oppong, J., Hart, S. P., Landolt, J., Yeboah, E., and Six, J. (2018). Climate-smart sustainable agriculture in low-to-intermediate shade agroforests. Nature Sustain. 1, 234–239. doi: 10.1038/s41893-018-0062-8
Blaser-Hart, W. J., Hart, S. P., Oppong, J., Kyereh, D., Yeboah, E., and Six, J. (2021). The effectiveness of cocoa agroforests depends on shade-tree canopy height, agriculture. Ecosyst. Environ. 322:107676. doi: 10.1016/j.agee.2021.107676
Bos, M. M., Steffan-Dewenter, I., and Tscharntke, T. (2007). Shade tree management affects fruit abortion, insect pests and pathogens of cacao. Agric. Ecosyst. Environ. 120, 201–205. doi: 10.1016/j.agee.2006.09.004
CacaoNetBioversity InternationalLberté, B. (comp.). (2012). A global strategy for the conservation and use of cacao genetic resources, as the foundation for a sustainable cocoa economy. 186 p.
Cadavid-Vélez, S. (2006). Características de compatibilidad sexual de algunos clones de cacao y su aplicación en siembras comerciales. Compañía Nacional de Chocolates, Colombia.
Caicedo-Vargas, C., Pérez-Neira, D., Abad-González, J., and Gallar, D. (2022). Assessment of the environmental ipact and economic performance of cacao agroforestry systems in the Ecuadorian Amazon region: an LCA approach. Sci. Total Environ. 849:157795. doi: 10.1016/j.scitotenv.2022.157795
Ceccarelli, V., Lastra, S., Loor Solórzano, R. G., Chacón, W. W., Nolasco, M., Sotomayor Cantos, I. A., et al. (2022). Conservation and use of genetic resources of cacao (Theobroma cacao L.) by gene banks and nurseries in six Latin American countries. Genet. Resour. Crop. Evol. 69, 1283–1302. doi: 10.1007/s10722-021-01304-3
Cerda, R., Deheuvels, O., Calvache, D., Niehaus, L., Saenz, Y., Kent, J., et al. (2014). Contribution of cocoa agroforestry systems to family income and domestic consumption: looking toward intensification. Agrofor. Syst. 88, 957–981. doi: 10.1007/s10457-014-9691-8
Cilias, C., Rebouças Machado, R. C., and Motamayor, J. C. (2010). Relations between several traits linked to sexual plant reproduction in Theobroma cacao L.: number of ovules per ovary, number of seed per pod, and seed weight. Tree Genet. Genomes 6, 219–226. doi: 10.1007/s11295-009-0242-9
Daymond, A. J., Hadley, P., Machado, R. C., and Ng, E. (2002). Genetic variability in partitioning to the yield component of cacao (Theobroma cacao L.). HortScience 37, 799–801. doi: 10.21273/HORTSCI.37.5.799
De Almeida, A.-A., and Valle, R. R. (2007). Ecophysiology of the cacao tree. Braz. J. Plant Physiol. 19, 425–448. doi: 10.1590/S1677-04202007000400011
De Ponti, T., Rijk, B., and Van Ittersum, M. K. (2012). The crop yield gap between organic and conventional agriculture. Agric. Syst. 108, 1–9. doi: 10.1016/j.agsy.2011.12.004
Dos Santos Días, L. A., and Kageyama, P. Y. (1998). Repeatability and minimum harvest period of cacao (Theobroma cacao L.) in southern Bahia. Euphytica 102, 29–35. doi: 10.1023/A:1018373211196
Effendy Pratama, M. F., Rauf, R. A., Antara, M., and Basir-Cyio, M. (2019). Factors influencing the efficiency of cocoa farms: a study to increase income in rural Indonesia. PLoS One 14:e0214569. doi: 10.1371/journal.pone.0214569
End, M., Laliberté, B., Sena Gomes, R., Guiltinan, M., and Maximova, S. (2018). “Cocoa plant propagation techniques to supply farmers with improved planting materials” in Achieving sustainable cultivation of cocoa. ed. P. Umaharan (Cambridge: Burleigh Dodds Science Publishing), 1–40.
Esche, L., Schneider, M., Milz, J., and Armengot, L. (2023). The role of shade tree pruning in cocoa agroforestry systems: agronomic and economic benefits. Agrofor. Syst. 97, 175–185. doi: 10.1007/s10457-022-00796-x
Evans, H., and Murray, D. (1953). A shade and fertilizer experiment on young cocoa. Rep. Cacao Res. 51, 67–76.
Feumba de Tchoua, L., Sounigo, O., Bourgoing, R., Efombagn, M. I. B., Abolo, D., Ambang, Z., et al. (2021). Evaluation of adaptation and yield stability of cocoa progenies in marginal conditions: results from an on farm cocoa trial set up in a forest–savannah transition area in Cameroon. Crops 1, 20–31. doi: 10.3390/crops1010004
Foley, J. A., DeFries, R., Asner, G. P., Barford, C., Bonan, G., Carpenter, S. R., et al. (2005). Global consequences of land use. Science 309, 570–574. doi: 10.1126/science.1111772
Franzen, M., and Mulder, M. B. (2007). Ecological, economic and social perspectives on cocoa production worldwide. Biodivers. Conserv. 16, 3835–3849. doi: 10.1007/s10531-007-9183-5
Grand View Research. (2019). Cocoa beans market size, share & trends analysis report by application (cosmetics, confectionery, pharmaceuticals), by product (butter, powder, liquor), by distribution channel (online, offline), and segment forecasts, 2019–2025. Retrieved from https://www.grandviewresearch.com/industry-analysis/cocoa-beans-market
Gutiérrez, O. A., Campbell, A. S., and Phillips-Mora, W. (2016). “Breeding for disease resistance in cacao” in Cacao diseases: a history of old enemies and new encounters. Eds. B. A. Bailey and L. W. Meinhardt (Cham: Springer), 567–609.
Institutio Nacional de Innovación Agropecuaria y Forestal (INIAF). (2016). Genotipos de cacao en el Alto Beni – Bolivia. Bolivia: Catálogo de selecciones locales de cacao.
Johnson, E. S., Bekele, F. L., Brown, S. J., Song, Q., Zhang, D., Meinhardt, L. W., et al. (2009). Population structure and genetic diversity of the Trinitario cacao (Theobroma cacao L.) from Trinidad and Tobago. Crop Sci. 49, 564–572. doi: 10.2135/cropsci2008.03.0128
Johnson, E., Bekele, F, and Schnell, R. (2004). Field guide to the ICS clones of Trinidad. Tropical Agricultural Research and Higher Education Center. Manual técnico No. 54. CATIE. Turrialba, Costa Rica. 31.
Klein, A. M., Steffan-Dewenter, I., Buchori, D., and Tscharntke, T. (2002). Effects of land-use intensity in tropical agroforestry systems on coffee flower-visiting and trap-nesting bees and wasps. Conserv. Biol. 16, 1003–1014. doi: 10.1046/j.1523-1739.2002.00499.x
Krauss, U., and Soberanis, W. (2001). Rehabilitation of diseased cacao fields in Peru through shade regulation and timing of biocontrol measures. Agrofor. Syst. 53, 179–184. doi: 10.1023/A:1013376504268
Lachenaoud, P. H., Paulin, D., Ducamp, M., and Thevenin, J. M. (2007). Twenty years of agronomic evaluation of wild cocoa trees (Theobroma cacao L.) from French Guiana. Sci. Hortic. 113, 313–321. doi: 10.1016/j.scienta.2007.05.016
Lachenaud, P. H. (1995). Variations in the number of beans per pod in Theobroma cacao L. in the Ivory Coast. II. Pollen germination, pod loadting and ovule development. J. Hort. Sci. 70, 1–6. doi: 10.1080/14620316.1995.11515266
Larsen, F. E., Higgins, S. S., and Dolph, C. A. (1992). Rootstock influence over 25 years on yield, yield efficiency and tree growth of cultivars ‘delicious’ and ‘Golden delicious’ apple (Malus domestica Borkh.). Sci. Hortic. 49, 63–70. doi: 10.1016/0304-4238(92)90143-Z
Lopes, U. V., Dos Santos, R. P., Yamada, M. M., Ramos, M., and Da Silva, W. R. M. (2015). Origin of the incompatibility allele carried by cacao clone TSH-1188. Agrotrópica (Brazil) 27, 129–132. doi: 10.21757/0103-3816.2015v27n2p129-132
Lopes, U. V., Monteiro, W. R., Pires, J. L., Clement, D., Yamada, M. M., and Gramacho, K. P. (2011). Cacao breeding in Bahia, Brazil: strategies and results. Crop Breeding App. Biotechnol. 11, 73–81. doi: 10.1590/S1984-70332011000500011
Maddison, A. C., Macias, G., Moreira, C., Arias, R., and Neira, R. (1995). Cocoa production in Ecuador in relation to dry-season escape from pod rot caused by Crinipellis perniciosa and Moniliophthora roreri. Plant Pathol. 44, 982–998. doi: 10.1111/j.1365-3059.1995.tb02657.x
Maharaj, K., Maharaj, P., Bekele, F. L., Ramnath, D., Bidaisee, G. G., Bekele, I., et al. (2011). Trinidad selected hybrids: an investigation of the phenotypic and agro-economic traits of 20 selected cacao cultivars. Trop. Agric. 88:4.
Marconi, L., and Armengot, L. (2020). Complex agroforestry systems against biotic homogenization: the case of plants in the herbaceous stratum of cocoa production systems. Agric. Ecosyst. Environ. 287:106664. doi: 10.1016/j.agee.2019.106664
Mbow, C., Van Noordwijk, M., Luedeling, E., Neufeldt, H., Minang, P. A., and Kowero, G. (2014). Agroforestry solutions to address food security and climate change challenges in Africa. Curr. Opin. Environ. Sustain. 6, 61–67. doi: 10.1016/j.cosust.2013.10.014
Milestad, R., and Darnhofer, I. (2003). Building farm resilience: the prospects and challenges of organic farming. J. Sustain. Agric. 22, 81–97. doi: 10.1300/J064v22n03_09
Morris, R. J. (2010). Anthropogenic impacts on tropical forest biodiversity: a network structure and ecosystem functioning perspective. Philosop. Trans. Royal Society B: Biolog. Sci. 365, 3709–3718. doi: 10.1098/rstb.2010.0273
Niether, W., Armengot, L., Andres, C., Schneider, M., and Gerold, G. (2018). Shade trees and tree pruning alter throughfall and microclimate in cocoa (Theobroma cacao L.) production systems. Ann. For. Sci. 75:38. doi: 10.1007/s13595-018-0723-9
Niether, W., Jacobi, J., Blaser, W. J., Andres, C., and Armengot, L. (2020). Cocoa agroforestry systems versus monocultures: a multi-dimensional meta-analysis. Environ. Res. Lett. 15:104085. doi: 10.1088/1748-9326/abb053
Niether, W., Smit, I., Armengot, L., Schneider, M., Gerold, G., and Pawelzik, E. (2017). Environmental growing conditions in five production systems induce stress response and affect chemical composition of cocoa (Theobroma cacao L.) beans. J. Agric. Food Chem. 65, 10165–10173. doi: 10.1021/acs.jafc.7b04490
Notaro, M., Collado, C., Depas, J. K., Dumovil, D., Denis, A. J., Deheuvels, O., et al. (2021). The spatial distribution and height of associated crops influence cocoa tree productivity in complex agroforestry systems. Agron. Sustain. Dev. 41:60. doi: 10.1007/s13593-021-00716-w
Nygren, P., Leblanc, H. A., Lu, M., and Gómez Luciano, C. A. (2013). Distribution of coarse and fine roots of Theobroma cacao and shade tree Inga edulis in a cocoa plantation. Ann. For. Sci. 70, 229–239. doi: 10.1007/s13595-012-0250-z
Orozco-Aguilar, L., and López-Sampson, A., Leandro-Muñoz, ME., Robiglio, V., Reyes, M., Bordeaux, M., Sepúlveda, N., and Somarriba, E. (2021) Elucidating pathways and discourses linking cocoa cultivation to deforestation, reforestation, and tree cover change in Nicaragua and Peru. Front. Sustain Food Syst. 5:635779. doi: 10.3389/fsufs.2021.635779
Padi, F. K., Opoku, S. Y., Adomako, B., and Adu-Ampomah, Y. (2012). Effectiveness of juvenile tree growth rate as an index for selecting high yielding cocoa families. Sci. Hortic. 139, 14–20. doi: 10.1016/j.scienta.2012.02.039
Pérez-Neira, D. (2016). Energy efficiency of cacao agroforestry under traditional and organic management. Agron. Sustain. Dev. 36:49. doi: 10.1007/s13593-016-0386-6
Pérez-Neira, D., Schneider, L., and Armengot, L. (2020). Crop-diversification and organic management increase the energy efficiency of cacao plantations. Agric. Syst. 177:102711. doi: 10.1016/j.agsy.2019.102711
Pérez-Neira, D., Schneider, M., Esche, L., and Armengot, L. (2023). Sustainability of food security in different production systems: a labour, energy and food quality nexus approach. Resour. Conserv. Recycl. 190:106874. doi: 10.1016/j.resconrec.2023.106874
Perfecto, I., Vandermeer, J., Mas, A., and Pinto, L. S. (2005). Biodiversity, yield, and shade coffee certification. Ecol. Econ. 54, 435–446. doi: 10.1016/j.ecolecon.2004.10.009
Phillips-Mora, W., Arciniegas-Leal, A., Mata-Quirós, A., and Motamayor-Arias, J.C. (2012) Catálogo de clones de cacao seleccionados por CATIE para siembras comerciales. Série técnica. Manual técnico / CATIE: Bolivia. 5.
Phillips-Mora, W., Castillo, J., Krauss, U., Rodríguez, E., and Wilkinson, M. J. (2005). Evaluation of cacao (Theobroma cacao) clones against seven Colombian isolates of Moniliophthora roreri from four pathogen genetic groups. Plant Pathol. 54, 483–490. doi: 10.1111/j.1365-3059.2005.01210.x
Phillips-Mora, W., and Wilkinson, M. J. (2007). Frosty pod of cacao: a disease with a limited geographic range but unlimited potential for damage. Phytopathology 97, 1644–1647. doi: 10.1094/PHYTO-97-12-1644
Quenta, W.L., Bentes-Gama, M., Somarriba, E., and Pastrana, A. (2005). Adopción prospectiva de las innovaciones tecnológicas para la producción orgánica de cacao en el Alto Beni, Bolivia, Agroforesteria en als Américas, 43–44. Available at: https://ainfo.cnptia.embrapa.br/digital/bitstream/item/48999/1/michelliny2005.pdf
Ramírez-Argueta, O., Orozco-Aguilar, L., Dubón, A. D., Díaz, F. J., Sánchez, J., and Casanoves, F. (2022). Timber growth, cacao yields, and financial revenues in a long-term experiment of cacao agroforestry systems in northern Honduras. Front. Sustain. Food Syst. 6:941743. doi: 10.3389/fsufs.2022.941743
Rice, R. A., and Greenberg, R. (2000). Cacao cultivation and the conservation of biological diversity. AMBIO: J. Human Environ. 29, 167–173. doi: 10.1579/0044-7447-29.3.167
Saavedra, F., Jordan Peña, E., Schneider, M., and Naoki, K. (2020). Effects of environmental variables and foliar traits on the transpiration rate of cocoa (Theobroma cacao L.) under different cultivation systems. Agrofor. Syst. 94, 2021–2031. doi: 10.1007/s10457-020-00522-5
Schneider, M., Andres, C., Trujillo, G., Alcon, F., Amurrio, P., Perez, E., et al. (2016). Cocoa and total system yields of organic and conventional agroforestry vs monoculture systems in a long-term field trial in Bolivia. Exp. Agric. 53, 351–374. doi: 10.1017/S0014479716000417
Somarriba, E., and Beer, J. (2011). Productivity of Theobroma cacao agroforestry systems with timber or legume service shade trees. Agrofor. Syst. 81, 109–121. doi: 10.1007/s10457-010-9364-1
Somarriba, E., Peguero, F., Cerda, R., Orozco-Aguilar, L., López-Sampson, A., Leandro-Muñoz, M. E., et al. (2021). Rehabilitation and renovation of cocoa (Theobroma cacao L.) agroforestry systems. A review. Agron. Sustain. Dev. 41:64. doi: 10.1007/s13593-021-00717-9
Somarriba, E., and Trujillo, L. (2005). El Proyecto ‘Modernización de la cacaocultura orgánica del Alto Beni, Bolivia. Agroforesteria en las Américas 6, 43–44.
Ten Hoopen, G. M., Deberdt, P., Mbenoun, M., and Cilas, C. (2012). Modelling cacao pod growth: implications for disease control. Ann. Appl. Biol. 160, 260–272. doi: 10.1111/j.1744-7348.2012.00539.x
Trebissou, C. I., Tahi, M. G., Munoz, F., Sanchez, L., N’Guetta, S. A., Cilas, C., et al. (2021). Cocoa breeding must take into account the competitive value of cocoa trees. Eur. J. Agron. 128:126288. doi: 10.1016/j.eja.2021.126288
Trujillo, G. (2007). Estudio de evaluación de clones foráneos, selección y características de plantas superiores de cacao. Bolivia: Topaz.
Vaast, P., and Somarriba, E. (2014). Trade-offs between crop intensification and ecosystem services: the role of agroforestry in cocoa cultivation. Agrofor. Syst. 88, 947–956. doi: 10.1007/s10457-014-9762-x
Valle, R. R., De Almeida, A. A., and De Leite, R. M. (1990). Energy costs of flowering, fruiting, and cherelle wilt in cacao. Tree Physiol. 6, 329–336. doi: 10.1093/treephys/6.3.329
Vieira, I. C. G., Toledo, P. D., Silva, J. D., and Higuchi, H. (2008). Deforestation and threats to the biodiversity of Amazonia. Braz. J. Biol. 68, 949–956. doi: 10.1590/S1519-69842008000500004
World Cocoa Foundation. (2017). Cutting deforestation out of the cocoa supply chain. Retrieved from https://www.worldcocoafoundation.org/blog/cutting-deforestation-out-of-the-cocoa-supply-chain/
Keywords: agroforestry, monocultures, full-sib families, international clones, flowering intensity, yield–biomass ratio, cherelle wilt, diseases
Citation: Armengot L, Picucci M, Milz J, Hansen JK and Schneider M (2023) Locally-selected cacao clones for improved yield: a case study in different production systems in a long-term trial. Front. Sustain. Food Syst. 7:1253063. doi: 10.3389/fsufs.2023.1253063
Edited by:
Pedro Talhinhas, University of Lisbon, PortugalReviewed by:
Bruno Rapidel, Institut National de la Recherche Agronomique (INRA), FrancePablo Siles, International Center for Tropical Agriculture (CIAT), Colombia
Luis Alberto Orozco Aguilar, Centro Agronomico Tropical De Investigacion Y Ensenanza Catie, Costa Rica
Copyright © 2023 Armengot, Picucci, Milz, Hansen and Schneider. This is an open-access article distributed under the terms of the Creative Commons Attribution License (CC BY). The use, distribution or reproduction in other forums is permitted, provided the original author(s) and the copyright owner(s) are credited and that the original publication in this journal is cited, in accordance with accepted academic practice. No use, distribution or reproduction is permitted which does not comply with these terms.
*Correspondence: Laura Armengot, bGF1cmEuYXJtZW5nb3RAdXZpYy5jYXQ=
†Present address: Marco Picucci, Agropalma, Tailândia, (PA), Brazil
‡These authors share first authorship