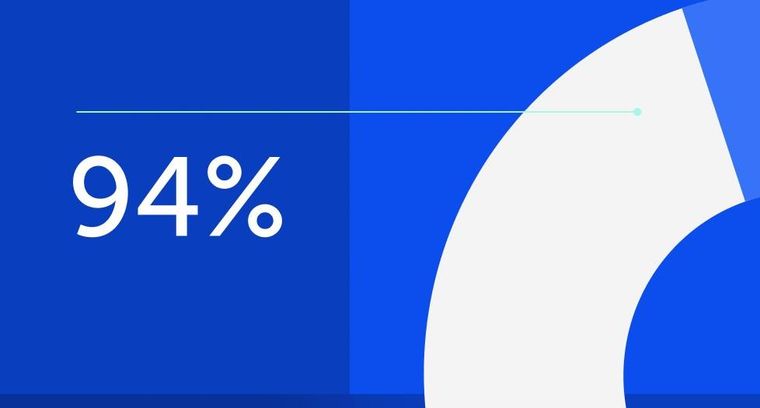
94% of researchers rate our articles as excellent or good
Learn more about the work of our research integrity team to safeguard the quality of each article we publish.
Find out more
REVIEW article
Front. Sustain. Food Syst., 19 October 2023
Sec. Sustainable Food Processing
Volume 7 - 2023 | https://doi.org/10.3389/fsufs.2023.1249199
This article is part of the Research TopicOmics Technology in Food and Agricultural NutritionView all 4 articles
Agricultural products may deteriorate due to various reasons during storage and transportation, resulting in serious losses. However, the mechanism of deterioration is complex, and many mechanisms are currently unclear. In recent years, the rapid development of omics technologies, such as genomics, proteomics, transcriptomics, and metabolomics, has led to breakthroughs in exploring the mechanism of product quality changes during the storage and transportation of agricultural products, and also help to understand molecular mechanisms. By using omics technology to gain a deeper understanding of the changes in agricultural products during storage and transportation, more efficient, environmentally friendly, and resource saving storage and transportation methods can be developed, providing a research foundation for the sustainability of China’s agricultural food system. In addition, research on omics technology has provided theoretical support for screening more suitable storage methods and transportation conditions. This review presents the potential application omics technologies in agricultural product storage and transportation. The application examples of omics technology in the storage and transportation process of agricultural products were discussed. Finally, the prospects indicate that omics technology has unlimited potential.
Agricultural products may undergo adverse changes during storage and transportation for various reasons, resulting in a decline in quality. The sale of these deteriorated products potentially endangers the health of consumers. But if these products cannot be sold, it will cause serious losses. According to data, the overall production of agricultural products in China for 2019 amounted to 1.98 billion tons. Notably, the production of fresh agricultural products exceeded 1.1 billion tons. The loss of fresh agricultural products can reach 20–30% due to improper transportation and storage (Comnews.cn, 2020). According to the Ministry of Agriculture experts, the postpartum loss rates of grain, potatoes, fruits, and vegetables in China are 7–11%, 15–20%, 15–20%, and 20–25%, respectively. The economic loss exceeds 300 billion yuan, equivalent to the waste of over 70 thousand hectares of farmland input and output (Hua and Lou, 2012). The economic waste resulting from these losses is significant. Therefore, how to reduce agricultural product loss and quality decline during storage and transportation is worth considering.
Strengthening the monitoring of changes in the quality of agricultural products during storage and transportation can effectively minimize losses in the storage and transportation process. The traditional methods of monitoring the quality changes of agricultural products during storage and transportation mainly include sensory testing, chemical testing, and biological testing. The sensory inspection of agricultural products involves an examination of sensory properties. This process can effectively detect product quality issues, allowing for early problem detection and resolution. However, a sensory inspection limits to evaluating the appearance and quality of agricultural products and is dependent on personal experience, making quantitative analysis challenging. This research should only be used for preliminary screening and processing of agricultural products experiencing quality changes, as the results are speculative. The primary purpose of chemical inspection is to determine and monitor changes in the nutritional components of agricultural products, such as carbohydrates, lipids and proteins, during storage and transportation. However, chemical inspection generally has a slow reaction speed and cannot conduct large-scale analysis, which requires a long time to achieve the expected experimental results. Biological testing mainly involves microbial analysis of agricultural products. Traditional microbial analysis can only detect the number of microorganisms present, and identification of microorganisms limited to the genus level (Jiang et al., 2022). Moreover, the traditional microbiological testing methods are complex and time-consuming. They also require a controlled experimental environment to study the transcription of all genes at the global level. These technologies have some shortcomings to some extent. Future monitoring methods will require higher sensitivity, faster detection, and greater precision to address these shortcomings. Therefore, the development of new monitoring technologies is of great significance.
Biochip technology is one of the emerging monitoring technologies, in the monitoring of the storage and transportation process of agricultural products, spoilage microorganisms are quickly detected through their cells, proteins, genes, and other biological components, and prevention measures are taken. With the rapid development of detection and analysis technology in the field of life sciences, biochip technology has shown broad application prospects in microbial detection due to its high throughput, high sensitivity, and high specificity (Ma et al., 2018). In addition, the use of infrared spectroscopy (IRS) technology to detect changes in the quality of agricultural products has gradually become a popular research direction. Infrared spectroscopy analysis has a wide range of applications and can be used for quality analysis of dairy products, composition analysis of tea and cocoa, as well as quality changes in meat, fruits, and vegetables. The application of shortwave infrared reflectance spectroscopy to online detect the content of fat, water, and protein in beef or pork has the characteristics of convenience, speed, and accuracy, making it the preferred method for meat quality inspection at present. Currently, near infrared spectroscopy (NIRS) technology is also commonly used to monitor the changes in the composition of agricultural products during storage and transportation. Near infrared (NIR) spectroscopy technology can provide fast and non-destructive methods to estimate the freshness of various foods based on changes in NIR region characteristic spectra (Lee et al., 2017). In the process of storage and transportation, near-infrared spectroscopy technology can quickly analyze the moisture and nutritional components of agricultural products, but the scope of analysis is limited. In the field of food and agricultural products in China, there is still significant space for improvement in the application research of this technology, and there is still a certain distance from entering the stage of comprehensive promotion and use.
The emergence of omics technology provides new ideas for monitoring quality changes during the storage and transportation of agricultural products. Omics technology refers to a collection of high-throughput methods for measuring different molecular components in biological samples, which are divided into genomics technology, transcriptomics technology, proteomics technology, and metabolomics technology according to the different measured molecular components. The study of omics technology has led to multi-omics integration and new omics technology development for studying specific substances. Their relationship is shown in Figure 1. Omics technology is a popular research topic due to its high throughput, short cycle, high sensitivity, high accuracy, and real-time dynamic monitoring capabilities.
Each omics has unique advantages and plays crucial role in studying changes in quality during the storage and transportation of agricultural products. For example, using proteomics to qualitatively and quantitatively analyze the protein of post-harvest fruits and vegetables, identify the important proteins and their functions in the process of fruit and vegetable maturation and senescence, and conduct in-depth research on the regulation mechanism of exogenous treatment of fruit maturation and senescence, stress resistance, and more, which has significant implications for guiding post-harvest preservation of fruits and vegetables during storage and transportation. Combining multiple omics technologies is a popular topic in research. For example, Guo et al. (2022) conducted a study on the quality of litchi pulp at stored room temperature utilizing transcriptomics, proteomics, and metabolomics. Changes in sugar and acid content and composition resulted in starch loss during storage and transportation. In addition, the degradation of polysaccharides softens the texture and reduces the nutritional value. This study aimed to investigate the physiological changes occurring in litchi fruit pulp when stored at room temperature serving as a basis for litchi fruit preservation and as a reference for future study of carbohydrate metabolism of litchi fruit. Cigliano et al. (2022) studied a long-lasting tomato variety by examining protein-coding genes and microRNA expression, DNA methylation patterns, and histone modifications in distinct post-harvest phases. Multi-omics data integration helped to reveal the molecular mechanisms responsible for prolonging shelf-life. The findings indicated that the down-regulation of the ripening regulatory factor MADS-RIN and genes associated with ethylene reaction and cell wall degradation were consistent with the delayed softening of the fruit. Large-scale epigenome reprogramming after fruit harvest may lead to delayed fruit senescence through multi-omics technology. In short, omics technology is a valuable tool for researching the storage and transportation of agricultural products. The unique advantage of omics technology compared to other technologies is that it can be used to explore the mechanism of quality changes, thereby preventing the quality decline of agricultural products during storage and transportation and identifying more suitable storage and transportation methods.
Firstly, previous research reviews on the application of omics technology in the field of agricultural products have mainly focused on the safety of agricultural products. However, little attention has been paid to the application of omics technology in the storage and transportation process of agricultural products. This review comprehensively summarizes the applications of various omics technologies in the storage and transportation process of agricultural products, with a focus on the discussion of applications. This article provides a hierarchical introduction to the application of various learning techniques in the storage and transportation process of agricultural products based on the connections between each group of learning, and provides corresponding research publication with examples. Finally, it provides prospects for future development. Therefore, this will help to understand the application of omics technology for quality analysis in agricultural product storage and transportation. Additionally, it may trigger a new revolution in understanding the mechanisms behind changes in agricultural product quality.
Genomics is the original omics. Other genomics, such as transcriptomics and proteomics, belong to post-genomics. The primary use of genomics technology is to detect the target genome through gene sequencing technology and conduct follow-up research. One of the more common methods is whole-genome sequencing. The basic steps of this method are shown in Figure 2. The application of genomics in the storage and transportation of agricultural products has achieved mature development. In recent years, there have been relatively few studies on genomics applications in the storage and transportation of agricultural products. There are two main ideas for the application of genomics technology. The first is to use gene sequencing technology to measure the genomes of bacteria and fungi and to deeply understand these microorganisms that affect agricultural product storage and transportation from the genetic level, and also the quality changes of agricultural products during the storage and transportation process. Researchers need to find effective strategies to deal with microorganisms, prevent agricultural products from deteriorating during storage and transportation, and ensure the quality and safety of these products. Ramirez et al. used genomics and transcriptomics methods to study ostreatus stored at 4°C for 7 days after various treatments and analyzed the collection of expressed sequence tags (EST) obtained from cutting Pleurotus ostreatus. A detailed picture of the life characteristics of ostreatus is provided. These findings contribute to our understanding of post-harvest mushroom decay and provide theoretical support for future storage and transportation (Ramirez et al., 2011). Aflatoxin is a strong carcinogen that can easily pollute grains. Yu et al. launched the aflatoxin genomics project intending to devise methods to manage aflatoxin pollution in pre-harvest crops and post-harvest grain storage. They identified the genes involved in aflatoxin biosynthesis, regulation and pathogenicity, enabling a better understanding of the mechanism of aflatoxin formation at the genetic level and preventing the contamination of agricultural products during storage (Yu et al., 2008).
Through gene regulation, genomic technology is also used to improve agricultural product varieties and extend storage life. Wu et al. identified gene markers based on quantitative trait loci (QTLs) and developed a genomic-assisted prediction (GAP) model for apple flesh firmness and brittleness retention. The research found the key genes that reduce flesh firmness and firmness, and provided insights into regulating flesh firmness and firmness retention to improve storage performance. The GAP model can help improve the quality of apples (Wu et al., 2021a). Anderson et al. used modern genomic methods to identify key genetic characteristics associated with resistance to yield-limiting factors such as cassava mosaic disease (CMD), cassava bacterial blight (CBB), drought, and acid soil to cultivate high-quality cassava that can overcome these challenges. Approximately 23,000 ESTs have been currently developed from various cassava tissues and genotypes. A deeper understanding of genes will also help to reduce the loss caused by deterioration during storage (Anderson et al., 2004).
Genomics technology is also of great significance in the storage and transportation of fermented products. Clostridium tyrobutyricum has been recognized as the primary cause of late-blowing defects in cheese, resulting in massive economic losses in the dairy industry. Podrzaj conducted the first study on the comparative genomics of Bacillus casei associated with LBD. Importantly, this study highlights the extensive genetic diversity of Tyrobacillus casei, which may help us understand the diversity of the putrefaction potential of T. casei in cheese and provide clues for further exploration of the genetic module underlying the putrefaction ability of this species. Thus, it also helps to improve the storage performance of cheese (Podrzaj et al., 2022). Lactic acid bacteria (LAB) is a common bacterial group that inhabits fermented vegetables and can produce beneficial organic acids. Okoye et al. performed whole-genome sequencing and comparative genomics on five LAB species. The results revealed the main LBA carbohydrate-active enzymes, putative operons, and unique mobile genetic elements. The metabolic pathway of organic acid biosynthesis identified the key genes encoding specific enzymes required for organic acid metabolism. The research provides theoretical support for using this pathway in processing and storage (Okoye et al., 2022). Genome sequence analysis provides fascinating insights into the evolution of microorganisms that lead to the decline of agricultural product quality during storage, as well as the mechanisms that lead to the growth of pathogenic bacteria. It is very likely to be used to predict the possibility of new microorganisms in the storage and transportation process in the future, and it also provides a direction for the genetic modification of agricultural products.
Transcriptomics refers to the study of gene transcription and the overall regulation of transcription in cells, which mainly focuses on RNA level. It is an essential means to study cell phenotype and function. The detection technology used in transcriptomics is similar to genomics, except that the detection object has changed from DNA to RNA. Transcriptomics technology can explore the mechanism of fruit deterioration in agricultural products during storage and transportation, considering different harvesting methods and conditions. In particular, the effect of a chilling injury on fruit can reveal potential mechanisms for preventing it through transcriptome technology. Banana fruit is prone to chilling injury (CI) during cold storage, resulting in quality decline and commodity loss. The mechanism behind chilling injury reduction through hot water treatment (HWT) is currently unclear. Si et al. analyzed the transcriptome of banana peel, revealing the mechanism of using hot water to alleviate chilling injury of bananas during cold storage by using transcriptome, which enriched the theoretical basis of using hot water to reduce chilling injury of fruit during storage and transportation (Si et al., 2022). Cold storage can prolong the storage time of blueberries to more than 60 days, but it will cause chilling injury. Zhang et al. used transcriptome analysis to fully reveal the chilling injury mechanism of blueberries when subjected to cold stress. They identified forty-five cold-induced transcription factor (TF) families containing 1,023 TFs. The study revealed the mechanism of the low-temperature chilling injury, which is necessary for preventing such injury during storage (Zhang F. et al., 2020). The omics technology also helps in understanding the changes in nutrient levels in fruits under different storage conditions, allowing for the selection of a more suitable storage method. Wang et al. used transcriptomics to analyze nectarine fruits stored at different temperatures and observed that the differentially expressed genes (DEGs) accumulated very high in starch and sucrose metabolism and the biosynthesis of terpene skeleton. This study explained the molecular mechanism underlying changes in sugar and carotenoid levels in nectarine fruit during storage at varying temperatures. The findings provide theoretical support for the quality maintenance of nectarine during storage (Wang et al., 2022). Ultraviolet-C (UV-C) radiation can be used as an effective way to prolong the shelf life of fruit. Zhou et al. studied the changes of UV-C-treated peaches with transcriptome and proteomics methods to better understand the role of UV-C treatment in regulating the metabolic pathway of peach fruit during cold storage. Proteomics and transcriptomics found peach genes and enzymes involved in acid and sugar changes and the general molecular mechanism of sugar, acid, and phenolic substances in response to UV-C changes (Zhou et al., 2020).
Furthermore, in terms of agricultural product storage and transportation, transcriptome technology is often used to clarify the mechanism of microbial infection to better prevent microbial pollution of agricultural products during storage and transportation. The characteristics of genes and metabolites in peach fruit following post-harvest by Monilinia fructicola are currently unclear. Cheng et al. used transcriptomics and metabonomics to explore the gene expression profile and different metabolites of peach infected by M. fructicola at different stages; and also proposed the molecular framework of peach systemic resistance, indicating the complex defense response of peach to M. fructicola infection (Cheng et al., 2023). To reveal the gene and adaptive pathway of S. enterica serovar Typhimurium in low water activity foods, Crucello et al. inoculated the bacteria on four kinds of low Aw foods, stored at25°C for 24 h and 72 h and studied the transcriptome of S. enterica serovar Typhimurium with RNA-Seq. The results revealed the genes that play a key role in the dehydration reaction of Salmonella and the pathway they participated in, showing that the gene regulation in the first 24 h was mainly affected by the food substrate itself and the following adaptation to low water activity was the same in different samples (Crucello et al., 2019). Penicillium digitatum is one of the important pathogens that cause the loss of citrus during storage and transportation after harvest. Yang et al. explored the infection mechanism of P. digitatum by conducting transcriptome sequencing and bioinformatics analysis, and found genes associated with pathogenic factors. In this study, a simple blueprint of the infection mechanism was displayed (Yang et al., 2019).
Table 1 is a publication review on the application of genomics and transcriptomics technology in the storage and transportation of agricultural products. As seen in Table 1, agricultural products such as fruits, meat, herbs, vegetables, fermented products, and more use genomics and transcriptomics technology during storage and transportation. Genomics and transcriptomics can be used to explore the mechanism of microbial deterioration. The quality change of agricultural products and the interaction mechanism between agricultural products and microorganisms are very complex topics. The use of transcriptome technology or genomics technology alone cannot effectively explore these mechanisms. It is necessary to combine various omics technologies. Utilization of genomics and transcriptome technologies for exploring microorganisms at the DNA and RNA levels can provide a more comprehensive understanding of the deterioration mechanism of agricultural products caused by microbial action during storage and transportation. Thus, this can help develop preventive measures and reduce agricultural product loss during storage and transportation. Genomics and transcriptome can also be used to study the chemical composition changes caused by different storage conditions during the storage and transportation of agricultural products and screen the most appropriate storage and transportation conditions. Transcriptome technology is often used with other omics technologies, such as metabonomics and proteomics (as indicated in Table 1), to enable agricultural product storage and transportation examination at both the gene and molecular levels. Genomic sequence analysis provides useful insights into the evolution of microorganisms that result in a decline in the quality of agricultural products during storage, as well as the mechanisms that lead to the evolution of pathogenic bacteria. Moreover, it is highly likely to be used to predict the possibility of new microorganisms appearing during storage and transportation in the future and also provides direction for gene transformation of agricultural products. There is much related literature about the application of transcriptome in the storage and transportation of agricultural products, but their application in the storage and transportation of agricultural products is still in the development stage.
Table 1. Some publications on the application of genomics and transcriptomics technology in the storage and transportation of agricultural products.
Proteomics technology considers proteomics crucial because it plays a significant role in analyzing and processing large-scale proteins (Dutta et al., 2022). The in-depth study of proteome analysis has resulted in significant improvements in protein analysis methods. The new protein analysis technology will provide a comprehensive food protein composition database, including some minor components that traditional protein analysis methods may overlook. Proteomics technology has significant advantages over some traditional protein analysis techniques. For example, the Kjeldahl method has complex pre-treatment and severe reagent consumption. The Biuret method has low sensitivity and complex operation, making it difficult to use for automated instrument analysis. Although the UV absorption method is simple to operate, it is susceptible to interference. If nucleic acids and other substances that absorb ultraviolet radiation appear in the sample, the results will be greatly disturbed and not very accurate. Proteomics technology does not require complete hydrolysis of proteins, has simple pre-treatment, low reagent consumption, and automated analysis. It can analyze a large number of samples at once. In addition, proteomics technology can be qualitative and quantitative, with little external interference, and accurate analysis results. Proteomics is used to monitor agricultural products during storage and transportation, which can help us better understand the changes in the quality of agricultural products. During food transportation and storage, further non-enzymatic post-translational protein modification (nePTM), such as the Maillard reaction, often occurs, increasing the complexity of the food proteome. Proteomics will fundamentally accelerate the acquisition of new knowledge in food and nutrition science while also assisting in understanding the complex interactions between various components in food storage and transportation. Therefore, this provides a technical and methodological prerequisite for the wide application of proteomics in food science (Pischetsrieder and Baeuerlein, 2009).
Proteomics can be considered the subject of large-scale analysis of proteins in specific biological systems. The main idea of using proteomics technology to analyze the quality changes in food during storage and transportation is to monitor any changes in specific proteins that may occur during these processes. Proteomics based on mass spectrometry (MS) has been recognized as an indispensable tool for accurately identifying and quantifying thousands of proteins from complex protein samples, and has been used in most proteomic studies (Carrera, 2021). Proteomics recognition and analysis of proteins and peptides involves an easy and simple experimental procedure. The first step is to isolate proteins from the samples to be tested. The second step is to identify and quantify proteins with significant differences in expression. The third step is to select the protein for validation on this basis and then conduct the bioinformatics analysis. There are 3 kinds of strategies: bottom-up, top-down, and middle-down. The bottom-up approach analyzes proteolytic peptides. The top-down method measures the intact proteins. The middle-down strategy analyzes larger peptides resulting from limited digestion or more selective proteases. Before MS analysis and database searching, one or more protein or peptide fractionation techniques can be applied (Zhang et al., 2013).
Protein is a crucial nutrient of animal food. Changes in protein during animal food storage and transportation are necessary when examining quality change. The meat quality of animals will change during transportation. By utilizing proteomics technology, it is possible to investigate and determine suitable transportation methods, including assessing the necessity of providing food and drink during transportation, and evaluating the impact of both long and short-distance transportation on product quality. Deters et al. divided 36 cattle into three groups to study the impact of cattle transport on beef quality. The CON group: no transit and ad libitum access to feed and water. DEPR group: no transit but deprived of feed and water for 18 h. TRANS group: trans 1790 km and no access to feed or water for 18 h. Proteomics and metabolomics analysis were performed on the treated tissue samples immediately, allowing for the selection of the most suitable method of beef transportation (Deters and Hansen, 2022). The stress responses of animals will affect the quality of animal products. Proteomics can provide theoretical references for changes in meat quality during transportation and provide insight into the mechanism of adverse effects caused by stress reactions. Luca et al. used LC–MS to identify proteins that indicate molecular processes underpinning transport stress. They analyzed the proteomic response of pigs of the same genetic line to transport stress (short circuit or long route transport), and identified 1,464 proteins. Among these, 66 proteins were discovered to distinguish between short-circuit and long-route transport pigs. This study explored the molecular process of pig adaptation to transport stress through proteomics technology (Di Luca et al., 2022). Ramell et al. divided dairy cows into three groups, and raised them under good, semi-good, and tough conditions. The analysis of cow serum is proteomic in different environments showed that living in a challenging environment with limited access to food and human care may cause oxidative stress in cows. This stress could potentially lead to cow mastitis and negatively affect milk production. An oxidative stress response is the primary physiological adaptation in cows under different production systems, as determined by proteomic analysis (Marco-Ramell et al., 2012). Lou et al. used 4D label-free quantitative (4D-LFQ) proteomics to study the proteomic changes in the longissimus dorsi muscle of Qinchuan beef during different storage periods. Additionally, high-performance liquid chromatography was used to detect changes in energy substances and to explore the energy metabolism mechanism of Qinchuan beef during cold storage and its impact on meat quality (Luo et al., 2021). Thus, this shows the potential of proteomics as a theoretical reference for studying the mechanism of meat quality changes during storage. Li et al. used proteomics to study the effect of cold stress on the composition, structure, and physical properties of the gel of muscle fibrillar protein (MP) of Procambarus clarkii, providing a theoretical reference for the quality change of crayfish during cold chain transportation (Li et al., 2022a). Xu et al. performed a proteomic analysis and comparison between normal meat and pale, soft, exudative (PSE) meat, and found proteins that may be related to PSE (Zequan et al., 2021).
Proteomics provides detailed information on the molecular mechanism of fruit quality changes. Some studies have shown that the resistance inducer based on chitosan can cause the defense reaction of strawberry fruit and prevent its oxidation during storage. The molecular mechanism of the resistance inducer of chitosan is still unclear. Dynamic proteomics provides a better understanding of the interaction mechanism between the strawberry resistance inducer and chitosan and is also a potential candidate for the fruit preservation and storage inducer (Ban et al., 2018). Hypoxia is a common occurrence in fruit post-harvest logistics, and it can significantly affect the storage performance of fruit. Here, Li et al. studied the dynamic changes of mitochondrial morphology in living citrus fruit cells, and revealed that hypoxia induced by wax treatment strongly triggered mitochondrial fission and fragmentation. The study characterized the proteomic changes of mitochondria after waxing and identified 167 key proteins related to the hypoxia response. These proteins were primarily involved in the metabolism of fatty acids, amino acids, and organic acids. It provides theoretical support for the post-harvest quality degradation of citrus fruit caused by hypoxia (Li et al., 2022b).
Metabolomics is a method used to detect changes in metabolites within (molecular weight < 1,500 Da) biological systems. Metabolomics is crucial component of systems biology as an extension of other ‘omics’ (Ferrocino and Cocolin, 2017). Agricultural product components interact during storage and transportation, and are susceptible to external factors. All compounds in agricultural products interact physically and chemically, changing their acceptability, safety, and shelf life. Therefore, a deeper understanding of the interaction and change mechanisms of agricultural product components enables improved control over the storage and transportation of agricultural products. Metabolomics is the study of systematic chemical components, which provides a systematic and comprehensive method for determining, quantifying, and identifying final product characteristics (Kushalappa et al., 2008). The mechanism of the application of metabolomics in the storage and transportation of agricultural products is based on the composition and changes of metabolites during the storage and transportation process of agricultural products, to study their metabolic pathways and mechanisms of action, and then understand the principle of deterioration. Alternatively, differential metabolites that can represent the occurrence of deterioration can be screened out through the differences in metabolites, and preventive measures can be taken in advance to predict the deterioration of agricultural products through the detection of these metabolites. Metabolomics can be divided into non-targeted and targeted metabolomics based on the research objectives. Their most common detection methods are LC–MS, GC–MS, and NMR, each with advantages and disadvantages. They are often combined to discover differential metabolites and in-depth research and analysis of subsequent metabolic sub-biomarkers.
Metabolomics has been widely recognized as a powerful, efficient, and sensitive analytical tool in food science (Utpott et al., 2022). In recent years, some applications of metabolomics technology in the storage and transportation of agricultural products are shown in Table 2. Metabolomics is commonly used to understand the mechanism of quality changes in agricultural products during storage and transportation, and to study the factors influencing these changes. Jujube fruit is prone to mechanical damage during storage and transportation, which degrades its quality. Zhang et al. studied morphology and endogenous metabolism of jujube at three developmental stages after partial compression simulating mechanical injury. The results showed that the pH of Jujube fruit increased after partial extrusion, and the phenol content decreased, which was proved by further metabonomic analysis. According to the study, metabonomics analysis can provide in-depth insights into the impact of mechanical damage on fruit nutrition and health benefits during storage and transportation (Zhang et al., 2023). Yokota et al. studied the factors affecting the quality of agricultural products by storing harvested tomatoes under different conditions, such as normal oxygen, hypoxia, and improved carbon dioxide and oxygen concentration. The tomatoes were stored at 25°C for 8 days. During the storage period, the metabolites of tomatoes were determined by metabolomics. The metabolites were affected by oxygen and carbon dioxide, indicating that metabolomics could effectively reveal the relationship between fruit metabolic status and different storage conditions to select an effective storage method for transportation (Yokota et al., 2019). Zhu et al. used non-targeted metabolomics to study the effect of freeze-drying on the metabolites of raw milk. The metabolomics of milk powder stored at three temperatures (room temperature, 4°C, −20°C) were analyzed using NMR and MS-based techniques. The research shows that freeze-drying is an effective drying method for extending the shelf life of milk. In terms of storage, the metabolites of milk powder remained stable at 4°C. However, while stored at room temperature, significant changes in metabolites occur. The results provide a basis for further research on milk metabolomics and offer a theoretical analysis for storing milk powder at different temperatures (Zhu et al., 2020). Besides, Han et al. developed a low-temperature sausage model and coated the surface with natural casing. After 12 days of storage at 20°C, the metabolomics analysis of sausages was conducted. After 4 days, the most common bacteria causing the deterioration of sausages was Beauveria bassiana. The main nonvolatile metabolites were also obtained. These results provide useful information for optimizing sausage storage conditions (Han et al., 2021).
Table 2. Some publications on the application of metabolomics technology in the storage and transportation of agricultural products.
It is difficult to analyze the regulatory mechanism of physiological processes systematically and comprehensively using a single data set due to biological processes of complexity and holistic nature. The joint analysis of multiple groups can jointly explore the potential regulatory network mechanism in the organism, providing more evidence for the mechanism of the organism. Transcriptomics can predict the change in gene expression. Metabolites are the end product of gene transcription in organisms affected by internal and external factors and can directly reflect physiological phenomena in organisms. Metabolomics can study the functional changes of these genes. The combination of transcriptomics and metabolomics can further understand the mechanism of cell physiological activities at the molecular and biochemical levels. The combination of transcriptomics and metabolomics can be used to screen more suitable varieties of agricultural products for storage and transportation. Ewas et al. compared the metabolome and transcriptome of SIMX1 transgenic tomato and wild tomato to evaluate how genetic engineering can improve the metabolome of tomato fruit. The results showed that the character improvement obtained by the SIMX1 genome has the potential for use in molecular breeding programs, resulting in changes to fruit size and yield, inducing secondary metabolites that promote health, improve fungal resistance, and ultimately extend the shelf life of fruit (Ewas et al., 2022). Apple fruit softening depends on the maturity at harvest and a series of orchard and environmental factors. Favre et al. investigated the effect of seasons on the softening of Royal Gala apples. The apples harvested four times, very early (H1), early (H2), commercial (H3), and late (H4), were stored at 0.5°C for 100 days and then analyzed by metabolomics and transcriptomics. The data showed that the pre-harvest pressure significantly influenced post-harvest softening, and 10 harvest biomarkers were identified that could be used to predict the long-term storage performance of fruit batches (Favre et al., 2023). On the other hand, the combination of transcriptomics and metabolomics is used to explore the quality change mechanism of agricultural products and provide theoretical support for optimizing storage conditions. Sugarbeet, a crucial raw material in sucrose production, often needs to be stored before sucrose can be produced. During the storage period, the yield and quality of sugar may decrease. Obtaining post-harvest metabolic information through omics technology helps to improve the storage quality. Gippert et al. used metabonomics and transcriptomics technology to study the primary and secondary metabolites of sugarbeet with varying storage quality (good, medium, and poor) during the storage process to explore the potential mechanism of quality change during storage (Gippert et al., 2022). In short, metabolomics has unlimited development potential and is a useful tool for studying the internal mechanism of biology.
In addition to the above four major omics, other omics technologies play a significant role in the agricultural storage and transportation of products. These include lipidomics, flavoromics, glycomics, and more. Lipidomics, flavoromics, glycomics from metabolomics, and lipids, sugars, and amino acids are all metabolites. The relationship between these omics techniques and metabolomics is shown in Figure 3.
Lipidomicis a systematic study of all lipids in cells, tissues, or organisms developed from metabolomics. It mainly studies the type, distribution, function, and interaction with other biomolecules of thousands of lipids, and their dynamic changes in physiological metabolism and pathological state; its analytical technology is similar to metabolomics. Lipidomic can be used as a new method to evaluate the freshness of agricultural products during storage and transportation. Lipids have a wide variety of species in plants and play a crucial role in the biological metabolism of plants; therefore, lipids characteristics may indicate the freshness of harvested vegetables. Zainal et al. clarified the changes in the lipid group of stored cabbage and identified the fresh lipid molecules. They stored cabbage at 5°C, 10°C, and 20°C, respectively, and analyzed the lipid content of its leaves using HPLC–MS. The cumulative respiratory CO2 production was measured too. Four lipid molecules were screened as potential freshness markers. The results of this study show that lipidomic analysis can be used as an effective way to evaluate the freshness of the whole cabbage during storage and transportation (Zainal et al., 2022). In addition, lipidomic is significant in revealing the quality change mechanism of agricultural products during storage and transportation. Low-temperature storage is the main method to maintain the quality and commercial value of fruits after harvest. However, pitting may occur during cold storage, especially during the shelf life. Wang et al. analyzed the potential relationship between pitting and membrane lipid metabolism of blueberries during cold storage within the shelf life using membrane lipidomic. Based on the results of the other characteristics and metabolites determination, the changes observed in blueberry phospholipids during storage are due to cold stress. This study shows that lipidomic can help in revealing the deterioration mechanism (Wang et al., 2019). Lipidomics relies on the advanced analysis platform of modern instruments such as MS, which has high sensitivity and selectivity, and lipids are also an essential component of food. Lipidomic methods offer unparalleled advantages in food safety and quality assurance revealing the interaction between diet and lipid metabolism, tracing food sources, and analyzing food ingredients. Additionally, these methods can distinguish quality changes and detect food adulteration during storage and transportation (Wu et al., 2021b).
Flavoromics is a group technology to analyze the basis of flavor substances and is a crucial part of systematic flavor research. It originates from metabolomics but is different from metabolomics. It is based on metabolomics to conduct targeted and comprehensive analysis of all flavor-related metabolites. The integrated metabolomics - flavoromics method is applicable for describing seasonal and ripening changes in fruits. Aung et al. evaluated the micromolecules of two mango varieties in two seasons by metabolomics and flavor omics. The results show that there are different biomarkers at different ripening stages, and the biomarker compounds at different changing stages are also identified, providing new insights into the different ripening stages of the two mango varieties in two seasons and the subsequent preservation measures (Lin Aung et al., 2021). Flavoromics has high accuracy, wide application range, high efficiency, and rapid analysis, and it can automatically establish models, creating full automation of data processing very convenient. For example, using flavor omics technology to predict the changes in food quality during storage and transportation, Gelinas and others used non-targeted LC–MS flavor omics chemical analysis to analyze the flavor instability of Rebaudioside A (Reb A) in acidified beverages stored at 35°C for 6 weeks. A high-quality orthogonal partial least squares analysis model with good prediction ability was developed based on the chemical data and the d-value from the quadrant sensory panel test, and the significant flavor change of aging beverages was tested. The results showed that degradation products directly affect the flavor stability of Reb A in acidified beverages (Gelinas et al., 2022). Changes in flavor are also crucial indicator of food quality. Although flavor genomics research has just started, its advantages cannot be underestimated.
Glycome is also a crucial nutrient in food. Glycomics is a discipline that studies the composition and function of glycoprotein chains. Glycomics, similar to proteomics, is significant in food science but still in its infancy. The number of relevant publications on glycomics in the field of food is small, but there are new publications every year, which indicates that glycomics has great and expected growth potential in food science (Tang et al., 2022). There are few articles on glycomics technology in food storage and transportation. Due to the high throughput and specificity of glycomics technology, it can efficiently monitor the changes in carbohydrate substances in animals during transportation, thereby reflecting the animal transportation status, and allowing for the selection of a more suitable animal transportation mode.
Lipidomics has a clear goal of understanding metabolic mechanisms through systems biology, identifying specific biomarkers that affect the quality of agricultural products during storage and transportation, and providing an effective and standardized measurement. Its processing process is stable, sensitive to changes, and efficient in data analysis (Zhu et al., 2020). Flavomics establishes the relationship between the chemical analysis of flavor compounds and sensory science. This method is well suitable for processing large data sets involved in chemical analysis methods, advanced chemometrics, and data mining programs due to the development of modern high-resolution analysis technology and new data analysis capability. Therefore, it can be used for rapid and large batch determination and is suitable for various foods (pork, beef, mutton, fish, seafood, and their products) and drinks (wine, tea, honey, dairy products, beverages, and more). A variety of sample types, such as cigarettes, essence, and spices, are potentially new ways to monitor changes in the quality of agricultural products during storage and transportation. For glycomics, glycomics of a cell reflects various events occurring at the molecular level in the cell. It is possible to develop highly specific recognition methods that can provide detailed information by utilizing the vast diversity of glycans. On the one hand, it can explore the molecular mechanisms of changes in agricultural products during storage and transportation at the cell molecular level while quickly identifying characteristic molecules that affect agricultural product storage and transportation during the detection process and taking preventive measures in advance. As agricultural products are a source of carbohydrates, glycomics has a wide range of applications. In summary, the development of new omics technologies has made omics analysis more targeted and can help individuals obtain the necessary information more effectively. These technologies can be combined and used to gain a more comprehensive perspective, and their applications in the storage and transportation of agricultural products have enormous potential.
Each omics technology plays an important role in the research of agricultural product storage and transportation, they have both connections and unique advantages. Genomics delves into the microorganisms that cause deterioration of agricultural products, explores the mechanisms and growth patterns of spoilage bacteria that cause deterioration of agricultural products, predicts potential new microorganisms, and takes preventive measures in advance to prevent deterioration of agricultural products during storage and transportation. In addition, research on genomes has provided direction for genetically modified agricultural products, improving them through gene regulation to obtain longer shelf life agricultural products. Genomics technology has a fast sequencing speed and a large coverage, which can comprehensively study the changes that occur during the storage and transportation of agricultural products. However, sequencing all genomes in agricultural products can obtain a huge amount of data, followed by tedious data analysis work such as gene annotation, which is relatively complex to process. Transcriptomics studies the sum of mRNA at a certain point in time. During the storage and transportation of agricultural products, transcriptome research can reveal the changes in nutritional composition under different storage and transportation conditions, select suitable storage and transportation conditions. However, only studying transcriptome to reveal the changes in agricultural products during storage and transportation is not comprehensive enough. It is still necessary to use it in conjunction with metabolomics and proteomics to more comprehensively reveal the mechanism of agricultural product deterioration. Proteomics focuses on all proteins, revealing how do changes in proteins occur during the storage and transportation of agricultural products, which helps to understand the mechanism of quality changes in agricultural products during storage and transportation. The high complexity and dynamism of proteomics determine that proteomics research faces more difficulties and challenges than other omics. For example, the study of proteomics is more complex than nucleic acid in terms of protein extraction, purification, and result analysis. Besides, in terms of current technology, the study of proteomics cannot achieve the high throughput and completeness as genomics research. The metabolites analyzed by metabolomics are a mixture of large and small molecules. Metabolomics studies the results that have already occurred, infers the mechanism of quality changes in agricultural products during storage and transportation through differences in metabolites, and selects appropriate storage and transportation methods to extend the shelf life of agricultural products. However, metabolomics technology requires a large amount of data, that means it will require a lot of time and effort to process, and requires high proficiency and experience from technical personnel. Besides, the repeatability and stability of metabolomics data need to be further improved. Glycomics, Lipidomicis and Flavoromics techniques are extensions of metabolomics techniques, which are more targeted than metabolomics.
Omics technology is widely used in the food field. Due to its high sensitivity and throughput, this technology is often used for food safety detection. Omics technology can effectively detect harmful substances in food and can also be used as an identification technology. The omics technology can help researchers better understand the mechanism that affects the quality of agricultural products and help individuals truly understand what is happening in the food-based system. Thus, this also determines its significant role in storing and transporting agricultural products. In recent years, the research on the application of omics technology in the storage and transportation of agricultural products has increased significantly annually showing the great scientific significance of omics technology in the storage and transportation of agricultural products. The loss of agricultural products during storage and transportation has become an important obstacle to food security and sustainable environmental development in developing countries. Omics technology, a new technology emerging in modern times, compared to other analytical methods, it is more efficient and fast. It can analyze a large number of samples at once and match the corresponding database, saving time and reagents, and has sustainable value. Omics technology studies the quality changes that occur during the storage and transportation of agricultural products, offering new ideas for selecting more suitable storage and transportation methods. Proper food storage and transportation methods can reduce the loss in the process of storage and transportation, reduce the deterioration of quality in the process, and make consumers more comfortable with consumption. For example, proteomics has been applied in the field of farm animal science to monitor the internal performance of livestock during storage and transportation, while also further understanding the molecular processes of meat substrate changes. Reduced waste caused by spoilage during storage and transportation (D'Alessandro and Zolla, 2013). Sun et al. revealed the biological mechanisms related to cow milk production through comprehensive metabolomics, metagenomics, and transcriptomics analysis, providing a new understanding and potential improvement strategies for ensuring the quality of milk and reducing waste during storage and transportation in the future storage and transportation (Sun et al., 2020). Paradiso et al. proposed a omics method to evaluate the role of oxidation and secondary compounds in food lipids. The omics method of oxidation product analysis provides new insights into the oxidation process, which may help deepen insight into unresolved oxidation issues and select more targeted transportation methods (Paradiso et al., 2018). In addition, they can also reduce transportation costs and prevent the psychological burden on consumers caused by over-packaging to keep products fresh. For example, in the post harvest supply chain, physiological disorders, fungal decay, and their combination can reduce the quality of fresh carrots. The use of omics methods can reveal the optimal combination of sustainable treatment methods to prevent carrot spoilage during storage and transportation (Papoutsis and Edelenbos, 2021). Appropriate pre-treatment for storage and transportation reduces transportation costs and eliminates the need for excessive packaging in subsequent sales to reduce losses. It can be said that omics technology has contributed to the sustainable development of China’s agricultural and food systems. At present, the main idea of many applications is to use omics technology as an effective means to explore the quality change mechanism in the storage and transportation process, to improve the storage performance of agricultural products. However, the quality change in the storage and transportation process is complex, involving various fields. Some require exploration at the genetic level, while some analysis of metabolite changes. A single omics technology cannot fully explain some internal mechanisms. Future attention should be placed on research on the application of multiple group combinations. However, due to differences in the representation of different omics datasets, especially for three or more omics data types, it is still challenging to integrate different results from multiple omics studies, establish correlations between results, and provide reasonable explanations for causal relationships. Due to the rich variety of agricultural products, the lack of bioinformatics and omics databases for various types of agricultural products limits the interpretation of specific phenomena and inhibits the understanding of certain biological processes in the storage and transportation of agricultural products. Therefore, there is an urgent need for more comprehensive bioinformatics resources for comprehensive analysis and visualization of different omics datasets. This is also a crucial direction for the future development of the agricultural products field.
LB studied, gathered the literature and compiled the entire manuscript. ZZ helped in revising and editing the manuscript. YZ supported for writing the original draft. KL provided guidance and suggestions, as well as funding acquisition, resources and writing-review. XP and ZG provided guidance during the process of revising the manuscript. All authors contributed to the article and approved the submitted version.
Hangzhou Science and Technology Development Plan Project (202203A05). The fundamental research funds for central public welfare research institutes (14231022).
ZG was employed by Hang zhou Nutritome Biotech Co., Ltd.
The remaining authors declare that the research was conducted in the absence of any commercial or financial relationships that could be construed as a potential conflict of interest.
All claims expressed in this article are solely those of the authors and do not necessarily represent those of their affiliated organizations, or those of the publisher, the editors and the reviewers. Any product that may be evaluated in this article, or claim that may be made by its manufacturer, is not guaranteed or endorsed by the publisher.
Anderson, J. V., Delseny, M., Fregene, M. A., Jorge, V., Mba, C., Lopez, C., et al. (2004). An EST resource for cassava and other species of Euphorbiaceae. Plant Mol. Biol. 56, 527–539. doi: 10.1007/s11103-004-5046-6
Ban, Z., Yan, J., Wang, Y., Zhang, J., Yuan, Q., and Li, L. (2018). Effects of postharvest application of chitosan-based layer-by-layer assemblies on regulation of ribosomal and defense proteins in strawberry fruit (Fragaria × Ananassa). Sci. Hortic. 240, 293–302. doi: 10.1016/j.scienta.2018.06.035
Carrera, M. (2021). Proteomics and food analysis: principles, techniques, and applications. Foods 10:2538. doi: 10.3390/foods10112538
Chen, J. H., Mei, S. A., Zheng, P., Guo, J. M., Zeng, Z. X., HuaZhong, L., et al. (2023). A multi-omics view of the preservation effect on Camellia sinensis leaves during low temperature postharvest transportation. LWT Food Sci. Technol. 178:114614. doi: 10.1016/j.lwt.2023.114614
Chen, S., Zhang, C., Xiong, Y., Tian, X., Liu, C., Jeevithan, E., et al. (2015). A GC-MS-based metabolomics investigation on scallop (Chlamys farreri) during semi-anhydrous living-preservation. Innovative Food Sci. Emerg. Technol. 31, 185–195. doi: 10.1016/j.ifset.2015.07.003
Cheng, C., Yan, C.-Y., Qi, C.-T., Zhao, X.-L., Liu, L.-X., Guo, Y.-Y., et al. (2023). Metabolome and transcriptome analysis of postharvest peach fruit in response to fungal pathogen Monilinia fructicola infection. LWT Food Sci. Technol. 173:114301. doi: 10.1016/j.lwt.2022.114301
Cigliano, R. A., Aversano, R., Matteo, A., Palombieri, S., Termolino, P., Angelini, C., et al. (2022). Multi-omics data integration provides insights into the post-harvest biology of a long shelf-life tomato landrace. Hortic Res 9:uhab042. doi: 10.1093/hr/uhab042
Comnews.cn . (2020). Agricultural Product Supply Chain Welcomes Digital Transformation. [EB/OL]. Available at: https://baijiahao.baidu.com/s?id=1671172538795622323&wfr=spider&for=pc,2020-07-03/2023-08-25 (Accessed October 04, 2023).
Crucello, A., Furtado, M. M., Chaves, M. D. R., and Sant'Ana, A. S. (2019). Transcriptome sequencing reveals genes and adaptation pathways in Salmonella typhimurium inoculated in four low water activity foods. Food Microbiol. 82, 426–435. doi: 10.1016/j.fm.2019.03.016
D'Alessandro, A., and Zolla, L. (2013). Meat science: from proteomics to integrated omics towards system biology. J. Proteome 78, 558–577. doi: 10.1016/j.jprot.2012.10.023
Deters, E. L., and Hansen, S. L. (2022). Long-distance transit alters liver and skeletal muscle physiology of beef cattle. Animal 16:100555. doi: 10.1016/j.animal.2022.100555
Di Luca, A., Ianni, A., Henry, M., Martino, C., Meleady, P., and Martino, G. (2022). Label-free quantitative proteomics and stress responses in pigs-the case of short or long road transportation. PLoS One 17:e0277950. doi: 10.1371/journal.pone.0277950
Dutta, B., Lahiri, D., Nag, M., Abukhader, R., Sarkar, T., Pati, S., et al. (2022). Multi-omics approach in amelioration of food products. Front. Microbiol. 13:955683. doi: 10.3389/fmicb.2022.955683
Ewas, M., Harlina, P. W., Shahzad, R., Khames, E., Ali, F., Nishawy, E., et al. (2022). Constitutive expression of SlMX1 gene improves fruit yield and quality, health-promoting compounds, fungal resistance and delays ripening in transgenic tomato plants. J. Plant Interact. 17, 517–536. doi: 10.1080/17429145.2022.2066730
Favre, L., Hunter, D. A., Erridge, Z. A., Napier, N. J., Punter, M., Carr, B., et al. (2023). Seasonal differences in softening of early-harvested? Royal Gala? Apple fruit are correlated with at-harvest biomarkers indicative of abiotic stress responses. Postharvest Biol. Technol. 195:112131. doi: 10.1016/j.postharvbio.2022.112131
Ferrocino, I., and Cocolin, L. (2017). Current perspectives in food-based studies exploiting multi-omics approaches. Curr. Opin. Food Sci. 13, 10–15. doi: 10.1016/j.cofs.2017.01.002
Gelinas, B. S., Liu, Y., Tello, E., and Peterson, D. G. (2022). Untargeted LC-MS based identification of Rebaudioside a degradation products impacting flavor perception during storage. Food Chem. 373:131457. doi: 10.1016/j.foodchem.2021.131457
Gippert, A.-L., Madritsch, S., Woryna, P., Otte, S., Mayrhofer, M., Eigner, H., et al. (2022). Unraveling metabolic patterns and molecular mechanisms underlying storability in sugar beet. BMC Plant Biol. 22:430. doi: 10.1186/s12870-022-03784-6
Guo, X., Luo, T., Han, D., Zhu, D., Li, Z., Zeyi, W., et al. (2022). Multi-omics analysis revealed room temperature storage affected the quality of litchi by altering carbohydrate metabolism. Sci. Hortic. 293:110663. doi: 10.1016/j.scienta.2021.110663
Han, H., Li, M., Peng, Y., Zhang, Z., Yue, X., and Zheng, Y. (2021). Microbial diversity and non-volatile metabolites profile of low-temperature sausage stored at room temperature. Front. Microbiol. 12:711963. doi: 10.3389/fmicb.2021.711963
Hua, Y., and Luo, Y. (2012). Ministry of Agriculture: 50 billion kilograms of grain are lost annually due to improper storage. [EB/OL].
Jiang, N., Rina, W., Chen, W., Wang, R., Junrui, W., and Shi, H. (2022). Multi-omics approaches to elucidate the role of interactions between microbial communities in cheese flavor and quality. Food Rev. Intl. 39, 1–15. doi: 10.1080/87559129.2022.2132261
Kayode, R. M. O., and Afolayan, A. J. (2014). Microbiological and chemical evaluation of decomposed open pollinated tomato (Lycopersicon esculentum) fruits in storage. J. Pure Appl. Microbiol. 8, 4441–4449.
Kereakede, E., Abdulsalami, M. S., Egbe, N. E., Yilwa, V. M., Oaikhena, E. E., Oladapo, E. O., et al. (2021). Molecular characterization of bacteria strians isolated from "JIKO": a herbal preparation consumed in some parts of Kaduna metropolis, Nigeria. Afr. J. Biotechnol. 20, 159–163. doi: 10.5897/AJB2020.17260
Kushalappa, A. C., Vikram, A., and Raghavan, G. S. V. (2008). Metabolomics of headspace gas for diagnosing diseases of fruits and vegetables after harvest. Stewart Postharvest Review 4, 1–7. doi: 10.2212/spr.2008.2.12
Lee, Seoho, Noh, Tae Gyoon, Choi, Jun Hoe, Han, Jeongsu, Ha, Joo Young, Lee, Ji Young, et al. (2017). "NIR Spectroscopic Sensing for Point-of-Need Freshness Assessment of Meat, Fish, Vegetables and Fruits." In Conference on Sensing for Agriculture and Food Quality and Safety IX. Anaheim, CA.
Li, X., Li, S., Shi, G., Xiong, G., Shi, L., Kang, J., et al. (2022a). Quantitative proteomics insights into gel properties changes of myofibrillar protein from Procambarus clarkii under cold stress. Food Chem. 372:130935. doi: 10.1016/j.foodchem.2021.130935
Li, X., Tian, Z., Chai, Y., Yang, H., Zhang, M., Yang, C., et al. (2022b). Cytological and proteomic evidence reveals the involvement of mitochondria in hypoxia-induced quality degradation in postharvest citrus fruit. Food Chem. 375:131833. doi: 10.1016/j.foodchem.2021.131833
Li, Z. W., Wang, S. L., Liu, B. Y., JianFeng, D., and Wang, Y. Z. (2023). Population identification and pathogenicity analysis of peach brown rot pathogens in Shandong Province. J. Fruit Sci. 40, 327–339.
Lin Aung, Y. Y., Lorjaroenphon, P. R., Sae-Tan, S., and Jom, K. N. (2021). Comparative investigation of combined metabolomics – flavoromics during the ripening of mango (Mangifera indica L.) cv. Nam Dok Mai Si thong and Nam Dok Mai no. 4. Plants (Basel) 10:2198. doi: 10.3390/plants10102198
Luo, H., He, Y. W., Zhang, X. Y., Ruan, Z. T., Luo, R. M., and Li, Y. L. (2021). Changes in postmortem energy metabolism of Qinchuan cattle meat during chilled storage and its effects on meat quality. Food Sci 42, 201–209. doi: 10.7506/spkx1002-6630-20201013-112
Ma, X., Wenzhong, H., Feng, K., and Si, Q. (2018). Application of biochip in microbial detection. Food Ferment. Ind. 44, 273–277.
Marco-Ramell, A., Arroyo, L., Saco, Y., Garcia-Heredia, A., Camps, J., Fina, M., et al. (2012). Proteomic analysis reveals oxidative stress response as the main adaptative physiological mechanism in cows under different production systems. J. Proteome 75, 4399–4411. doi: 10.1016/j.jprot.2012.04.002
Okoye, C. O., Dong, K., Wang, Y., Gao, L., Li, X., Wu, Y., et al. (2022). Comparative genomics reveals the organic acid biosynthesis metabolic pathways among five lactic acid bacterial species isolated from fermented vegetables. New Biotechnol. 70, 73–83. doi: 10.1016/j.nbt.2022.05.001
Papoutsis, K., and Edelenbos, M. (2021). Postharvest environmentally and human-friendly pre-treatments to minimize carrot waste in the supply chain caused by physiological disorders and fungi. Trends Food Sci. Technol. 112, 88–98. doi: 10.1016/j.tifs.2021.03.038
Paradiso, V. M., Pasqualone, A., Summo, C., and Caponio, F. (2018). An "omics" approach for lipid oxidation in foods: the case of free fatty acids in bulk purified olive oil. Eur. J. Lipid Sci. Technol. 120:1800102. doi: 10.1002/ejlt.201800102
Pischetsrieder, M., and Baeuerlein, R. (2009). Proteome research in food science. Chem. Soc. Rev. 38, 2600–2608. doi: 10.1039/b817898b
Podrzaj, L., Burtscher, J., and Domig, K. J. (2022). Comparative genomics provides insights into genetic diversity of Clostridium tyrobutyricum and potential implications for late blowing defects in cheese. Front. Microbiol. 13:889551. doi: 10.3389/fmicb.2022.889551
Ramirez, L., Oguiza, J. A., Perez, G., Lavin, J. L., Omarini, A., Santoyo, F., et al. (2011). Genomics and transcriptomics characterization of genes expressed during postharvest at 4 degrees C by the edible basidiomycete Pleurotus ostreatus. Int. Microbiol. 14, 111–120. doi: 10.2436/20.1501.01.141
Si, J., Fan, Y. Y., Liu, Z. L., Wei, W., Xiao, X. M., Yang, Y. Y., et al. (2022). Comparative transcriptomic analysis reveals the potential mechanism of hot water treatment alleviated-chilling injury in banana fruit. Food Res. Int. 157:111296. doi: 10.1016/j.foodres.2022.111296
Sun, H., Li, Q., Mao, L.-Z., Qiao-Ling Yuan, Y., Huang, M. C., Can-Fang, F., et al. (2021). Investigating the molecular mechanisms of pepper fruit tolerance to storage via transcriptomics and metabolomics. Horticulturae 7:242. doi: 10.3390/horticulturae7080242
Sun, H.-Z., Mi Zhou, O., Wang, Y. C., Liu, J.-X., and Guan, L. L. (2020). Multi-omics reveals functional genomic and metabolic mechanisms of milk production and quality in dairy cows. Bioinformatics 36, 2530–2537. doi: 10.1093/bioinformatics/btz951
Tang, W., Liu, D., and Nie, S.-P. (2022). Food glycomics in food science: recent advances and future perspectives. Curr. Opin. Food Sci. 46:100850. doi: 10.1016/j.cofs.2022.100850
Utpott, M., Rodrigues, E., Rios, A. O., Mercali, G. D., and Flores, S. H. (2022). Metabolomics: an analytical technique for food processing evaluation. Food Chem. 366:130685. doi: 10.1016/j.foodchem.2021.130685
Wang, Y., Ji, S., Dai, H., Kong, X., Hao, J., Wang, S., et al. (2019). Changes in membrane lipid metabolism accompany pitting in blueberry during refrigeration and subsequent storage at room temperature. Front. Plant Sci. 10:829. doi: 10.3389/fpls.2019.00829
Wang, Y., Sun, Y., Zhou, D., Zhang, Q., Pan, L., and Kang, T. (2022). Transcriptomics analysis provides insights into metabolisms of sugars and carotenoids of nectarine fruit subjected to different temperature storage. Sci. Hortic. 304:111262. doi: 10.1016/j.scienta.2022.111262
Worarad, K., Suzuki, T., Norii, H., Mochizuki, Y., Ishii, T., Shinohara, K., et al. (2021). Transcriptome profiling for pericarp browning during long-term storage of intact lotus root (Nelumbo nucifera). Plant Growth Regul. 95, 207–221. doi: 10.1007/s10725-021-00736-2
Wu, B., Shen, F., Wang, X., Zheng, W. Y., Xiao, C., Deng, Y., et al. (2021a). Role of MdERF3 and MdERF118 natural variations in apple flesh firmness/crispness retainability and development of QTL-based genomics-assisted prediction. Plant Biotechnol. J. 19, 1022–1037. doi: 10.1111/pbi.13527
Wu, B., Wei, F., Shuling, X., Xie, Y., Lv, X., Chen, H., et al. (2021b). Mass spectrometry-based lipidomics as a powerful platform in foodomics research. Trends Food Sci. Technol. 107, 358–376. doi: 10.1016/j.tifs.2020.10.045
Xu, F., and Li, G. (2023). Metabolomics reveals the effect of hypobaric treatment on energy metabolism in vibration-injured? Huangguan? Pears. Food Chem. 400:134057. doi: 10.1016/j.foodchem.2022.134057
Xu, D., Zheng, X., Li, C., Jiaxin, W., Sun, L., Qin, X., et al. (2022). Insights into the response mechanism of Litopenaeus vannamei exposed to cold stress during live transport combining untargeted metabolomics and biochemical assays. J. Therm. Biol. 104:103200. doi: 10.1016/j.jtherbio.2022.103200
Yang, Q., Qian, X., Dhanasekaran, S., Boateng, N. A. S., Yan, X., Zhu, H., et al. (2019). Study on the infection mechanism of Penicillium digitatum on postharvest citrus (Citrus reticulata blanco) based on transcriptomics. Microorganisms 7:672. doi: 10.3390/microorganisms7120672
Yokota, Y., Akihiro, T., Boerzhijin, S., Yamada, T., and Makino, Y. (2019). Effect of the storage atmosphere on metabolomics of harvested tomatoes (Solanum lycopersicum L.). Food Sci. Nutr. 7, 773–778. doi: 10.1002/fsn3.923
Yu, J., Payne, G. A., Nierman, W. C., Machida, M., Bennett, J. W., Campbell, B. C., et al. (2008). Aspergillus flavus genomics as a tool for studying the mechanism of aflatoxin formation. Food Addit. Contam. Part A Chem. Anal. Control Expo. Risk Assess. 25, 1152–1157. doi: 10.1080/02652030802213375
Yun, Z., Jin, S., Ding, Y., Wang, Z., Gao, H., Pan, Z., et al. (2012). Comparative transcriptomics and proteomics analysis of citrus fruit, to improve understanding of the effect of low temperature on maintaining fruit quality during lengthy post-harvest storage. J. Exp. Bot. 63, 2873–2893. doi: 10.1093/jxb/err390
Zainal, P. W., Syukri, D., Fahmy, K., Imaizumi, T., Thammawong, M., Tsuta, M., et al. (2022). Lipidomic profiling to assess the freshness of stored cabbage. Food Anal. Methods 16, 304–317. doi: 10.1007/s12161-022-02422-z
Zequan, X., Yonggang, S., Guangjuan, L., Shijun, X., Li, Z., Mingrui, Z., et al. (2021). Proteomics analysis as an approach to understand the formation of pale, soft, and exudative (PSE) pork. Meat Sci. 177:108353. doi: 10.1016/j.meatsci.2020.108353
Zhang, Y., Fonslow, B. R., Shan, B., Baek, M. C., and Yates, J. R. 3rd. (2013). Protein analysis by shotgun/bottom-up proteomics. Chem. Rev. 113, 2343–2394. doi: 10.1021/cr3003533
Zhang, F., Ji, S., Wei, B., Cheng, S., Wang, Y., Hao, J., et al. (2020). Transcriptome analysis of postharvest blueberries (Vaccinium corymbosum 'Duke') in response to cold stress. BMC Plant Biol. 20:80. doi: 10.1186/s12870-020-2281-1
Zhang, S., Wu, Z., Liu, L., Wang, L., Li, X., Ban, Z., et al. (2023). Partial compression increases acidity, but decreases phenolics in jujube fruit: evidence from targeted metabolomics. Food Res. Int. 164:112388. doi: 10.1016/j.foodres.2022.112388
Zhang, L., Zhu, B., Zeng, Y., Shen, H., Zhang, J., and Wang, X. (2020). Clinical lipidomics in understanding of lung cancer: opportunity and challenge. Cancer Lett. 470, 75–83. doi: 10.1016/j.canlet.2019.08.014
Zhao, H., Tang, X., Mingli, W., Li, Q., Yi, X., Liu, S., et al. (2021). Transcriptome characterization of short distance transport stress in beef cattle blood. Front. Genet. 12:616388. doi: 10.3389/fgene.2021.616388
Zhou, D., Zhang, Q., Li, P., Pan, L., and Tu, K. (2020). Combined transcriptomics and proteomics analysis provides insight into metabolisms of sugars, organic acids and phenols in UV-C treated peaches during storage. Plant Physiol. Biochem. 157, 148–159. doi: 10.1016/j.plaphy.2020.10.022
Keywords: omics, storage, transportation, agricultural product, application
Citation: Bai L, Zhang Z, Peng X, Gao Z, Zhao Y and Liang K (2023) Application of omics technology in the storage and transportation of agricultural products. Front. Sustain. Food Syst. 7:1249199. doi: 10.3389/fsufs.2023.1249199
Received: 28 June 2023; Accepted: 29 September 2023;
Published: 19 October 2023.
Edited by:
Raul Avila-Sosa, Benemérita Universidad Autónoma de Puebla, MexicoCopyright © 2023 Bai, Zhang, Peng, Gao, Zhao and Liang. This is an open-access article distributed under the terms of the Creative Commons Attribution License (CC BY). The use, distribution or reproduction in other forums is permitted, provided the original author(s) and the copyright owner(s) are credited and that the original publication in this journal is cited, in accordance with accepted academic practice. No use, distribution or reproduction is permitted which does not comply with these terms.
*Correspondence: Yan Zhao, emhhb3lhbjAxQGNhYXMuY24=; Kehong Liang, bGlhbmdrZWhvbmdAY2Fhcy5jbg==
Disclaimer: All claims expressed in this article are solely those of the authors and do not necessarily represent those of their affiliated organizations, or those of the publisher, the editors and the reviewers. Any product that may be evaluated in this article or claim that may be made by its manufacturer is not guaranteed or endorsed by the publisher.
Research integrity at Frontiers
Learn more about the work of our research integrity team to safeguard the quality of each article we publish.