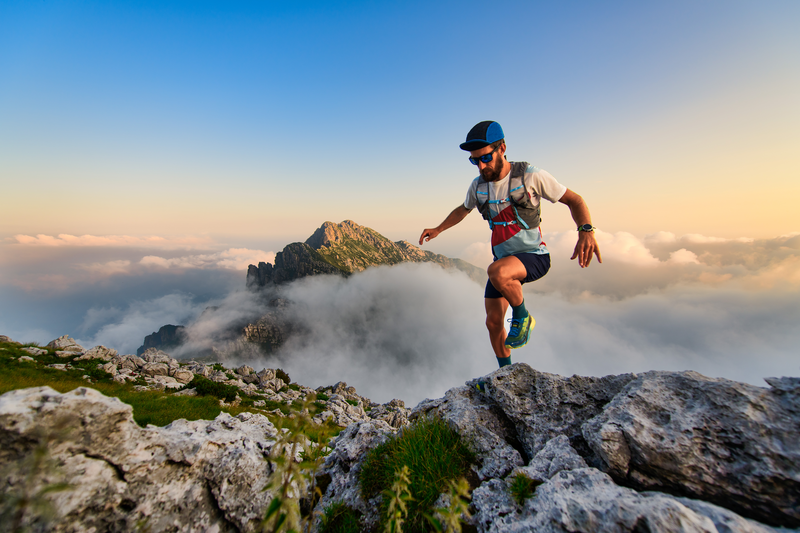
94% of researchers rate our articles as excellent or good
Learn more about the work of our research integrity team to safeguard the quality of each article we publish.
Find out more
ORIGINAL RESEARCH article
Front. Sustain. Food Syst. , 12 September 2023
Sec. Crop Biology and Sustainability
Volume 7 - 2023 | https://doi.org/10.3389/fsufs.2023.1242806
This article is part of the Research Topic ‘Save Soil’ by Managing Soil Nutrient Losses, Agronomic Practices and Crop-Microbial Interaction: World Soil Day 2022 View all 12 articles
Introduction: Soil organic matter (SOM) plays a vital role in enhancing soil characteristics and promoting sustainable crop production. The active and passive components of SOM tend to be more effective indicators of soil changes than total SOM content.
Methods: This study aimed to examine the impact of integrated nutrient management (INM) on the active and passive segments of SOM in maize during the kharif seasons of 2019 and 2020 at the Instructional Farm of Rajasthan College of Agriculture, Udaipur. A total of 11 treatments comprising of control (no application of manures/fertilizers/biofertilizers), different INM combinations, and application of inorganic fertilizers were laid in a randomized block design (RBD) with three replications tested in this study.
Results: The use of INM through enriched phosphorus compost (EPC), biofertilizers, and chemical fertilizers significantly boosted both the active and passive constituents of the organic matter of the soil. In this study, a combination of 75% NPK fertilizers via soil test response (STR), EPC @ 5 t ha−1, an Azotobacter consortium, phosphorus solubilizing bacteria (PSB), and a foliar spray of 0.5% Zn considerably increased the active fraction of SOM than other treatments as indicated by microbial biomass carbon (251 mg kg−1), microbial biomass nitrogen (36.8 mg kg−1), microbial biomass phosphorus (6.82 mg kg−1), water-soluble organic carbon (73.9 mg kg−1), water-soluble carbohydrates (43.8 mg kg−1), presence of dehydrogenase in soil (6.82 μg TPF g−1 soil 24 h−1), and carbon mineralization (43.8 mg CO2C kg−1 soil 24 h−1). This treatment was also found to increase the passive fraction as shown by the presence of humic acid (0.332%), fulvic acid (0.210%), hymatomelanic acid (0.052%), brown humic acid (0.252%), and humin (0.604%).
Discussion: From this study, it can be concluded that the application of 75% NPK fertilizers as per STR + EPC + Azotobacter + PSB + 0.5% foliar Zn spray can improve soil health in maize-based cropping systems.
Soil organic matter (SOM) encompasses all organic materials present in the soil, excluding living roots and animals. Constituting 58% of total SOM, carbon is a prominent indicator of soil health and fertility. High levels of soil organic carbon (SOC) are critical for soil biogeochemical processes (Doetterl et al., 2015; Laik et al., 2021a; Pramanick et al., 2023). The rates of decomposition of stable SOC and newly added carbon are regulated by immobilization and mineralization processes. The soil carbon storage capacity defines the rate of increase in SOC stock after the adoption of improved management practices such as the addition of organic matter in the soil. The amount of mineralization and equilibrium levels of carbon are crucial indicators of potential soil productivity (Riffaldi et al., 1996).
The primary constituents of SOM are humic substances (Stevenson, 1994a). These substances, particularly humic and fulvic compounds, directly boost plant growth through physiological and nutritional means (Vaughan and Malcolm, 1985; Chen and Aviad, 1990; Nardi et al., 2002). Some can act as natural plant hormones, accelerating seed germination, root formation and development (Piccolo et al., 1993), and uptake of nutrients (Dell'Agnola and Nardi, 1987; Piccolo et al., 1993), and serve as sources of nitrogen (N), phosphorus (P), and sulfur (S) (Arslan and Pehlivan, 2008). These substances indirectly impact plant growth by modifying soil's physical (Johnston, 2007), chemical, and biological properties, enhancing soil water holding capacity and cation exchange capacity and improving tilth and aeration (Stevenson, 1994b; Johnston, 2007; Murphy, 2015). SOM comprises active and passive fractions. The passive fraction, encompassing of durable stock of materials that can remain for a longer period of hundred decades in soil, is a decomposition product. It accounts for 58–82% of most SOM, and its quality changes slowly. Passive fractions enhance the soil's cation interchanging ability, water retention potential, and colloidal properties (Smith and Paul, 1990).
In sustainable agriculture, organic matter not only contributes to soil fertility but also stimulates and moderates soil formation, biology, physio-chemical properties, and hydro-thermal characteristics (Katyal, 2000; Pramanick et al., 2018, 2022; Naik et al., 2022). The nature, content, composition, and transformations of SOM play a crucial role in crop productivity in varying climate conditions. However, the costs associated with the procurement, transportation, and application of organic manures in large quantities are significant for farmers in developing countries such as India. Thus, a combination of inorganic fertilizers and organic manure could offer a solution to maintain crop yield (Sher et al., 2022) and soil health (Huang et al., 2010; Song et al., 2015; Lakshmi et al., 2021).
Maize-based cropping system in India is known as a chemical fertilizer-intensive cropping system as the nutrient requirement of maize is higher than other cereals (Singh et al., 2017; Pramanick et al., 2022). Continuous maize cultivation with the application of chemical fertilizers may deplete soil health in the long run. Hence, organic amendment as well as the application of biofertilizers are important to make the soil sustainable for production. In this study, the soil of a maize-based cropping system was selected to know whether the INM practices can improve the SOM pools or not.
Given these factors, the present experimental trial was directed to assess the operation of impacts of integrated utilization of biological, organic, and inorganic nutrient cause of sources on the SOM pools of maize soils and to identify the optimal nutrient sources and combinations for maintaining soil health.
The study was carried out at the College Farm of the Rajasthan Agricultural College in Udaipur, Rajasthan, across two crop seasons: Kharif 2019 and Kharif 2020. The experimental plot range is positioned at 24°34′ N latitude and longitudinal reference of 73°42′ E, at an elevation of 579.5 m more than the average sea level (Figure 1). The site falls under the CA'w Climatic classification as per Thornthwaite's system, signifying a humid subtropical climate with warm, wet summers and dry winters that receive sparse rainfall. The location typically receives an average rainfall of 637 mm, with over 70% of this precipitation occurring during the southwest monsoon season (July to September). The relative humidity during these monsoon months is relatively high, often exceeding 65%.
The soil at the experimental site is classified as clay loam, falling under the Vertisols category—typified by fine texture, iso-hyperthermic temperature regime, montmorillonitic mineralogical class, and Typic Haplustept taxonomic class. The soil has a slightly saline pH level of 8.29 and contains 0.62% organic carbon.
The experiment consisted of 11 treatments replicated thrice in a randomized block design (RBD). The treatment imposition details are given in Table 1.
The control treatment (T1) was conducted without the application of any fertilizers. The soil test-based recommendation (STR) was used to calculate the appropriate amounts of urea, diammonium phosphate, and muriate of potash for each treatment. These were subsequently applied at the recommended stages of crop growth. Enriched phosphorus compost (EPC) was locally prepared using maize stover, rock phosphate, and waste mica. To decrease the C:N ratio of the maize stover, we applied a urea solution at a rate of 0.25 kg N per 100 kg of stover and fresh cow dung at 10 kg per 100 kg of stover. These were used as natural inoculants along with phosphate solubilizing microorganisms (Aspergillus awamori, Pseudomonas striata, and Bacillus polymixa) at 50 g per 100 kg for treatments T6 to T11. The fully decomposed EPC contained 0.85% N, 1.21% P, 1.15% K, and 115 ppm of Zinc (Table 2). The EPC was applied to the field as dictated by the treatments and was thoroughly mixed into the soil a week before sowing. To administer treatments T4 and T5, we treated the seeds with a consortium of Azotobacter and phosphorus-solubilizing bacteria, i.e., PSB (Bacillus megatherium var. phosphaticum). For seed treatment, 200 g of each inoculant of Azotobacter and PSB was mixed in 500 ml water and a slurry was prepared with 50 g of jaggery. Afterward, the slurry was mixed evenly with the seeds before sowing. Nutrient management was applied as per the treatment details. For maize cultivation, two irrigations were given at silking and tasseling stage. Weed management was performed using the preemergence application of Atrazine at 1,000 g ha−1 applied 2 days after sowing (DAS) followed by one manual weeding at 30 DAS. The cost of cultivation is included in Supplementary Table S1.
Table 2. Characteristics of enriched phosphorus compost (EPC) and in-organic fertilizers used in the experimental field study.
The soil microbial biomass carbon content was determined using the chloroform fumigation-extraction method as described by Vance et al. (1987). The soil microbial biomass nitrogen was analyzed using a similar method by Brookes et al. (1985). Soil microbial biomass phosphorus was assessed using the standard procedure proposed by Brookes et al. (1982). Water-soluble carbon was determined using the acid extraction method outlined by Meloon and Sommers (1996), and water-soluble carbohydrates were quantified using the hydrolytic extraction with the H2SO4 method by Cheshire and Mundie (1966). The Anthrone extraction procedure, as explained by Casida et al. (1964), was utilized for analyzing soil dehydrogenase activity. Carbon mineralization was calculated using the rubber cork method (Kukreja et al., 1991). For the analysis of humic substances, various methods were employed. Humic acid (HA) and fulvic acid were determined using a 0.5 N NaOH solution method. Hymatomelanic acid (HyMA) was analyzed using Soxhlet's apparatus method. Brown humic acid (BHA) was measured using a 5% NaOH solution method. Gray humic acid (GHA) was quantified using the formula GHA = (weight of HA before extracting HyMA—weight of HA after extracting HyMA)—BHA. Humin was determined using a 1-N HCl method. All these methods were based on the procedures provided by Stevenson (1965).
Data were analyzed using the standard techniques of analysis of variance (ANOVA) for randomized block design (RBD) with the help of statistical software, SAS (version 9.2). Bartlett's χ2 test was performed to analyze the homogeneity of the error variance. Then, pooled analysis was performed as per Cocharan and Cox (1957) as the error variance was homogeneous. Differences were tested for Fisher's F-test at a 5% level of significance. When the F-test was found significant at a 5% level of significance, the least significant difference (LSD) was used to test the significance of differences between the two treatment means (Gomez and Gomez, 1976).
The dynamics of SOM are crucial for enhancing soil quality and maintaining crop productivity under intensive farming. In the following sections, we will discuss the results and interpretations of the active pools of SOM.
The integration of organic manure with fertilizer and biofertilizers got a positive impact on soil microbial biomass carbon (Table 3). Compared to non-compost treatments (T1-T5), the microbial biomass carbon was higher in compost-based INM treatments (T5-T11). The lowest microbial carbon (180 mg kg−1) was found in control (T1), whereas treatment with 75% NPK via STR + EPC+ Azotobacter + PSB + Zn (T8) recorded the highest value (251 mg kg−1). Compared to the unfertilized plot, treatment T8 had 1.4 folds of higher soil microbial biomass carbon. Biofertilizers application also poses a significant and pragmatic influence on soil microbial biomass carbon. The application of Zn as foliar spraying did not induce significant changes as evident from comparisons made between T7 and T8 and T10 and T11. In addition to EPC and biofertilizers (BF), i.e., Azotobacter and PSB, increasing levels of NPK bring significant improvement in microbial biomass carbon to control.
Microbial biomass is closely tied to SOC, and its levels rise with the addition of a carbon substrate and fall when the carbon content is consumed (Manna and Ganguly, 2000; Zhao et al., 2009). The increase in soil microbial biomass carbon in treatments with EPC could also be due to a decrease in soil pH and electrical conductivity resulting from the addition of organic matter. This improvement in soil microbial biomass carbon with compost application aligns with findings from Kallenbach and Grandy (2011) and Meena and Biswas (2015). Similarly, numerous studies have found that organic amendments significantly impact microbial biomass carbon levels due to the range of macro- and micronutrients added via these amendments (Hu et al., 2011; Chinnadurai et al., 2014; Tamilselvi et al., 2014; Obalum et al., 2017; Rajput et al., 2019; Ogumba et al., 2020; Padbhushan et al., 2021).
Significant differences in the microbial biomass of soil nitrogen were recorded in response to various nutrient sources and their combinations (Table 3). Like microbial biomass of soil carbon, the highest (36.8 mg kg−1) and lowest values (26.4 mg kg−1) of microbial biomass of soil nitrogen were observed in 75% NPK via STR + EPC+ Azotobacter + PSB + Zn (T8) and control (T1), respectively. The biomass nitrogen content of the soil microbial population is significantly influenced by the levels of NPK. Compared to the control, 17.25% and 6.5% higher biomass nitrogen were obtained in 125% NPK and 100% NPK + Zn, respectively. In addition to EPC and inorganic fertilizers, the co-application of Zn and biofertilizers also brought significant improvement in microbial biomass nitrogen as evident from comparing T9 and T11 and T6 and T8.
The enhancement in the microbial biomass of soil carbon is perhaps ascribed to the balanced nutrient provision to soil microbes through an INM approach (Rajput et al., 2019). The higher levels of SOC and improved nitrogen availability mediated by EPC can be an additional factor contributing to the increased soil microbial biomass nitrogen observed in EPC-based plots. Significantly higher soil microbial biomass nitrogen levels were observed in 100% and 125% NPK treatments compared to the control. This aligns with findings by Verma and Mathur (2009) and Padbhushan et al. (2021), who noted that microbial biomass nitrogen increased with rising nitrogen levels.
Differences in phosphorus content of microbial biomass between the non-compost treatments (T1-T5) and the compost-based treatments (T6-T11) are significant except for T2 and T9 which were non-significantly different (Table 3). The maximum microbial biomass phosphorus values were found in T8, followed by T7, T6, T11, T10, and T9 treatments, respectively. The treatments T1 to T4 recorded the least value of microbial biomass phosphorus. The addition of biofertilizers and Zn did not improve the microbial biomass of soil phosphorus significantly. The microbial biomass of soil phosphorus was higher under integrated nutrient application than sole use of NPK fertilizers. However, compared to the control (T1) and 100% NPK + Zn (T3), significantly higher soil microbial biomass phosphorus was observed in 125% NPK (T2). Balanced NPK application in conjunction with EPC enhanced the soil microbial biomass phosphorus more than the rest of all treatments. Integrated nutrient management provides substrates needed for microbes' growth and activity, thus enhancing microbial biomass phosphorus (Verma and Mathur, 2009; Kumar et al., 2017). Lack of nutrient addition in the soil might have resulted in low microbial biomass phosphorus in control plots. Additionally, the optimal level of P fertilizer might have promoted microbial metabolism processes that in turn raised the level of microbial biomass phosphorus (Verma and Mathur, 2009).
Integration of organic manure with fertilizer and biofertilizers recorded a positive impact on the water-soluble organic carbon (WSC). Its value in soil varied from 46.2 to 73.9 mg kg−1 under different treatments (Table 4). The maximum value of WSC (73.86 mg kg−1) in soil was recorded under T8 (75% NPK via STR + EPC+ Azotobacter + PSB + Zn), and this treatment was found at par with T7 (75% NPK via STR + EPC+ Azotobacter + PSB). The lowest value of WSC (46.2 mg kg−1) in soil was recorded in the control treatment (T1). The WSC is the most mobile as well as most labile C pool, and it serves as an immediate C source for soil microbes (Verma and Mathur, 2009). Verma and Mathur (2009) also reported that the highest WSC was recorded in INM treatments. In the INM, organic manures, inorganic fertilizers, and biofertilizers all are applied in combination to the soil and this kind of management practice increases the SOC as well as the activities of the soil beneficial microbes which ultimately attributed to higher WSC in soil (Laik et al., 2021b). In contrast to the above, the beneficial effects of organic manures on WSC content were reported by Dutta et al. (2018). The addition of organic manures increased WSC irrespective of inorganic fertilizers usage.
Table 4. Effect of integrated nutrient management practices on water-soluble organic carbon and water-soluble carbohydrates, dehydrogenase activity, and carbon mineralization in the soil after harvest of maize.
The integrated nutrient management practices significantly enhanced the water-soluble organic carbohydrates of soil (Table 4). The water-soluble organic carbohydrates in soil varied from 26.45 to 43.80 mg kg−1 under various treatments. The minimum value (26.45 mg kg−1) of water-soluble organic carbohydrates was recorded in control (T1) whereas the maximum value (43.80 mg kg−1) was recorded in T8 (75% NPK via STR + EPC+ Azotobacter + PSB + Zn) which was statistically at par with T7 (75% NPK via STR + EPC+ Azotobacter + PSB). Water-soluble carbohydrates function as both sources and sink for mineral nutrients and organic substrates in the short term. Over a longer period, they act as catalysts for plant nutrient conversion, therefore, influencing crop productivity and nutrient cycling (Kumari et al., 2011). Water-soluble carbohydrates are the most readily accessible energy source for microbes, and they play a substantial role in stabilizing soil structure (Verma and Mathur, 2009). Studies conducted by Padbhushan et al. (2014) and Verma and Mathur (2009) have also reported that the addition of organic manure to soil increases the total SOM which ultimately increases the carbohydrate content in the soil.
The activity of soil dehydrogenase is known as the index of microbial activities happening inside the soil (Dutta et al., 2018); therefore, it is a good indicator of soil biological activities. The effect of different treatments on dehydrogenase activity in soils was found significant (Table 4). The highest value of dehydrogenase activity in soil (6.82 μg TPF g−1 soil 24 hr−1) was estimated under T8 (75% NPK via STR + EPC+ Azotobacter + PSB + Zn) and this treatment was found statistically at par with T7 (75% NPK via STR + EPC+ Azotobacter + PSB). Soil dehydrogenase activity was greatly stimulated by organic, inorganic, and integrated management practices (Gao et al., 2020). Application of EPC augmented dehydrogenase activity in the soil. The extended activity of dehydrogenase in EPC-amended plots might be due to increased microbial activity under a higher organic manure supply. Earlier, Dutta et al. (2018) also reported similar results while working on various nutrient management practices.
Carbon mineralization plays a critical role in the dynamics of SOM as it determines the amount of carbon that accumulates in the soil (Zhao et al., 2008). The combined use of organic manures, fertilizers, and biofertilizers significantly influenced carbon mineralization in soil (Table 4). The value of carbon mineralization in soil ranges from 26.45 to 43.80 mg CO2C kg−1 soil 24 hr−1 under various treatments. The maximum value of carbon mineralization (43.80 mg CO2-C kg−1 soil 24 hr−1) in soil observed in T8 (75% NPK via STR + EPC+ Azotobacter + PSB + Zn), and this treatment was found statistically at par with T7 (75% NPK via STR + EPC+ Azotobacter + PSB), whereas the lowest value (26.45 mg CO2-C kg−1 soil 24 hr−1) was observed in control treatment (T1) (Table 4). Balanced nutrient supply and adequate carbonaceous substrate availability and a favorable environment under INM may enhance microbial growth that leads to an increased soil carbon mineralization (Mandal et al., 2020). Depletion of nutrients in unfertilized control plots can be the reason for the least carbon mineralization. This study echoes the findings of Mandal et al. (2020), who observed similar effects of INM on carbon mineralization. The increase in carbon mineralization observed in plots treated with both NPK fertilizer and organic amendments can be attributed to the ample supply of labile carbon substrates, as pointed out by Majumder et al. (2008). This finding is corroborated by studies from Kaur et al. (2008) and Mohanty et al. (2013). Zhao et al. (2009) further proclaimed that long-duration employed usage of both intensive fertilizers and organic manures not only provides a supportive domain for microbial growth and activity but also supplies the necessary substrates for the course of carbon mineralization.
Humic acid, as the second most significant component of SOM, plays a crucial role in enhancing nutrient recovery and transformation in soil, thereby contributing to higher yield production. This study discovered that INM significantly increased the humic acid levels in the soil post-cutting of the maize (as shown in Table 5). The content of humic acid in the soil ranged from 0.230% to 0.332% under different treatment conditions. The lowest value of humic acid (0.230%) in soil was observed under control. The treatment T8 (75% NPK via STR + EPC+ Azotobacter + PSB + Zn) showed a significantly higher amount of humic acid (0.332%) status in soil and this treatment was closely followed by T7 (75% NPK via STR + EPC+ Azotobacter + PSB) which can be due to enhanced soil physical parameters and directed favorable conditions for the evolution of humic acid (Santhy et al., 2001). The findings are in line with those reported by Deshmukh et al. (2015). Similarly, studies by Prasad et al. (1991), Banik and Sanyal (2006), Gathala et al. (2007), Bhoye et al. (2011), Meshram et al. (2016), and Meshram et al. (2018) all found that the greatest amount of humic acid was present when organic manure/residue was integrated with inorganic fertilizers. This might be attributed to the enhancement of SOM through the application of organic manures coupled with the biofertilizers that created a suitable condition for humus evolution (Kurbah and Dixit, 2020).
Table 5. Effect of integrated nutrient management practices on humic, fulvic, and hymatomelanic acid in soil.
It is the uttermost dominant pool of SOM which was also significantly affected by various nutrient management practices (Table 5).
Fulvic acid, a component of the passive pool of SOM, remains soluble in alkaline solutions and stays in the solution even after humic acid has precipitated with acid. Fulvic acid components assist in binding soil particles into skeletal portions known as aggregates, which are crucial in mobilizing and transporting sesquioxides in the soil complex. The amalgamation of diverse nutrient sources noticeably elevated the levels of fulvic acid in the soil following the maize harvest. It ranges from 0.136 to 0.210% under various treatments. Similar to humic acid, fulvic acid (0.210%) also recorded the highest in T8 (75% NPK via STR + EPC+ Azotobacter + PSB + Zn), which was found statistically at par with T7 (75% NPK via STR + EPC+ Azotobacter + PSB). Kumari et al. (2011) noted that the repeated usage of organic manures, either alone or in tandem with intensive fertilizers, had a significant impact on the fulvic acid fraction in the soil. This might be due to the increase in SOM and beneficial microorganisms in the soil. These results align with the findings of Gathala et al. (2007) and Deshmukh et al. (2015), who documented increased fulvic acid levels following the combined application of NPK and farmyard manure.
Integrated nutrient management significantly enhanced hymatomelanic acid (HyMA) in the soil after cutting the maize crop in both years and in the combined analysis (Table 5). The maximum value of HyMA (0.052%) in soil was obtained in T8 (75% NPK via STR + EPC+ Azotobacter + PSB + Zn), and this treatment was found statistically at par with T7 (75% NPK via STR + EPC+ Azotobacter + PSB). The lowest value of HyMA (0.033%) in soil was obtained under control (T1). These outcomes are in tune with the salient findings of Kumari et al. (2011). The content of HyMA was low because it consists of more than 90% aliphatic structure and is poorer in amino acid fragments and twice as rich in hydrocarbons as humic acid (Ranjana et al., 1984).
Application of compost with NPK fertilizer and biofertilizers enhanced the brown humic acid (BHA). The brown humic acid in the soil varied from 0.175 to 0.252% under varied treatments (Table 6). The maximum value of BHA (0.252%) in soil was obtained in T8 (75% NPK via STR + EPC+ Azotobacter + PSB + Zn), and this treatment was statistically at par with treatment T7 (75% NPK via STR + EPC+ Azotobacter + PSB). It might be due to added N through inorganic N and EPC and these fractions of SOM, especially BHA, showed stronger signals for N compounds (Saizet et al., 1979). These outcomes are perfectly synced with the findings of Kumari et al. (2011).
Table 6. Effect of integrated nutrient management practices on brown humic acid, gray humic acid, and humin in soil.
This is the smallest passive SOM pool in the study region. Despite the various nutrient management strategies applied, no significant improvement was noted in the content of gray humic acid. The values varied between 0.023 and 0.034% under different treatment conditions (Table 6). The lowest value of gray humic acid (0.023%) in soil was recorded in the control (T1). Combined use of EPC, 50% NPK fertilizers, foliar application of Zn, and biofertilizer (T11) exhibited the maximum value of gray humic acid (0.034%) in soil followed by the treatment T8 (75% NPK via STR + EPC+ Azotobacter + PSB + Zn). It might be due to the added N through inorganic nitrogen and EPC and a gray humic acid fraction of SOM showed stronger signals for N compounds (Saizet et al., 1979). These outcomes are fine-tuned with the evident findings of Kumari et al. (2011). Moreover, the potential explanation for the lack of significant improvement in gray humic acid content may be due to already optimized soil physical parameters and a conducive environment for this fraction.
The extremely impervious and resistant fraction of SOM is humin and its distribution or extent of share is the largest among the passive fraction of SOM. Among various passive pools of SOM, humin is the biggest soil pool followed by humic acid and fulvic acid fractions. The INM practices in maize significantly increased humin content in the soil after the harvest of the crop (Table 6). The humin in soil varied from 0.383% to 0.604% under various treatments. Unfertilized plots had the lowest humin (0.383%) whereas integrated use of 75% NPK via STR + EPC @ 5 t ha−1 + Azotobacter + PSB + Foliar application of Zn @ 0.5% (T8) exhibited the largest humin pool, and this treatment was closely followed by T7 (75% NPK via STR + EPC+ Azotobacter + PSB). The increase in humin fraction could be related to increased mineralization activity due to higher surface soil temperatures in tropical regimes by Santhy et al. (2001). When organic manure is added, it can serve as a source of humus. Compounds, such as cellulosic, xylose, mono and polysaccharides, glucosides, fatty acids, and amino acids, that are utilized by microorganisms can be indirectly converted into humic substances within microbial cells. These transformed substances can then be involved in the setup of humic substances evolution (Kononova, 1966). Previous studies by Prasad et al. (1991), Banik and Sanyal (2006), Gathala et al. (2007), Bhoye et al. (2011), Meshram et al. (2016), and Meshram et al. (2018) have all shown that applying 100% NPK via STR together with organic manures outstandingly enriched humus values in soil. This could be attributed to the stabilized employment of intensive fertilizers in conjunction with organic manure or matter, which led to more humus contributions in the soil, eventually resulting in greater nutrient accessibility for plants. Meena (2017) also reported that the highest humin fraction was obtained under the application of organic manure, compared to other treatments comprising chemical fertilizers.
Based on the findings of this research study, it was found that the combined application of organic and inorganic nutrient sources coupled with the application of biofertilizers led to a significant improvement in the active and passive fractions of SOM in the maize-based cropping system. From this study, it was observed that the application of 75% NPK via soil test response (STR) + enriched phosphorus compost (EPC) at 5 t ha−1 + Azotobacter + PSB + Zn foliar spray at 0.5% during 35 DAS resulted in the maximum attainments of microbial biomass carbon (251 mg kg−1), microbial biomass nitrogen (36.8 mg kg−1), microbial biomass phosphorus (6.82 mg kg−1), water-soluble organic carbon (73.9 mg kg−1), water-soluble carbohydrates (43.8 mg kg−1), presence of dehydrogenase in soil (6.82 μg TPF g−1 soil 24 hr−1), and carbon mineralization (43.8 mg CO2C kg−1 soil 24 hr−1). This treatment was also found to increase the passive fraction of SOM in the maize-based cropping system. This INM practice was also found to be closely followed by the application of 75% NPK via STR + EPC + Azotobacter + PSB. Hence, from this 2-year-long study, it can be concluded that the active and passive pools of SOM in a maize-based cropping system can be increased through the application of 75% NPK via STR + EPC @ 5 t ha−1 + Azatobacter + PSB + 0.5% Zn foliar spray at 35 DAS.
The original contributions presented in the study are included in the article/Supplementary material, further inquiries can be directed to the corresponding authors.
JB, HP, BN, AH, AA, AG, and BP performed the statistical analysis, project supervision, reviewed, read, and edited the manuscript with significant contributions. JB, HP, BN, BP, ShB, SuB, KD, SS, and SM collected soil/plant samples and performed chemical analysis, also wrote the initial draft of the manuscript, prepared figures and tables, led the research work, planned, supervised, and conducted field experiments, and read and edited the manuscript. All authors contributed to the article and approved the submitted version.
This research was funded by the Department of Soil Science, College of Agriculture, Agriculture University, Jodhpur, Rajasthan, Pali−306902, India, and also partially funded by Princess Nourah bint Abdulrahman University researchers supporting Project Number (PNURSP2023R65), Princess Nourah bint Abdulrahman University, Riyadh, Saudi Arabia.
The authors are very grateful to the Princess Nourah bint Abdulrahman University researchers supporting Project Number (PNURSP2023R65), Princess Nourah bint Abdulrahman University, Riyadh, Saudi Arabia, for supporting the current research.
The authors declare that the research was conducted in the absence of any commercial or financial relationships that could be construed as a potential conflict of interest.
All claims expressed in this article are solely those of the authors and do not necessarily represent those of their affiliated organizations, or those of the publisher, the editors and the reviewers. Any product that may be evaluated in this article, or claim that may be made by its manufacturer, is not guaranteed or endorsed by the publisher.
The Supplementary Material for this article can be found online at: https://www.frontiersin.org/articles/10.3389/fsufs.2023.1242806/full#supplementary-material
Arslan, G., and Pehlivan, E. (2008). Uptake of Cr3+ from aqueous solution by lignite-based humic acids. Bioresour Technol. 99, 7597–7605. doi: 10.1016/j.biortech.2008.02.007
Banik, G. C., and Sanyal, S. K. (2006). A study on chromium-humic complexation: Part 1. Characterization of humic substances. J. Indian Soc. Soil. Sci. 54, 163–169.
Bhoye, K. P., Badole, W. P., Shete, P. G., and Baviskar, V. S. (2011). Influence of partially and fully decomposed FYM on humic, fulvic acid, microbial count in soil and yield of sorghum. Green Farming. 2, 309–311.
Brookes, P. C., Landman, A., Pruden, G., and Jenkinson, D. S. (1985). Chloroform fumigation and release of soil “a rapid direct extraction method to measure microbial biomass N in soil”. Soil Biol. Biochem. 17, 837–842. doi: 10.1016/0038-0717(85)90144-0
Brookes, P. C., Powlson, D. S., and Jenkinson, D. S. (1982). Measurement of microbial biomass phosphorus in soil. Soil Biol Biochem. 14, 319–329. doi: 10.1016/0038-0717(82)90001-3
Casida, L. E., Klein, D., and Santoro, E. (1964). Soil dehydrogenase activity. Soil Sci. 98, 371–376. doi: 10.1097/00010694-196412000-00004
Chen, Y., and Aviad, T. (1990). Effects of humic substances on plant growth. In: MacCarthy P, Malcolm RL, Clapp CE, Bloom PR, editors. Humic Substances in Soil and Crop Science: Selected Readings. Madison: American Society of Agronomy and Soil Science Society of America. p. 161–187.
Cheshire, M. V., and Mundie, C. M. (1966). The hydrolytic extraction of carbohydrates from soil by H2SO4. Eur. J. Soil Sci. 17, 372–381. doi: 10.1111/j.1365-2389.1966.tb01480.x
Chinnadurai, C., Gopalaswamy, G., and Balachandar, D. (2014). Impact of long term organic and inorganic nutrient managements on the biological properties and eubacterial community diversity of the indian semi-arid alfisol. Arch. Agron. Soil Sci. 60, 531–548. doi: 10.1080/03650340.2013.803072
Cocharan, W. G., and Cox, G. M. (1957). Experimental Designs. 2nd ed. New York, NY: John Wiley and Sons. p. 611
Dell'Agnola, G., and Nardi, S. (1987). Hormone-like effect and enhanced nitrate uptake induced by depolycondensed humic fractions obtained from Allobophorarosea and A. caliginosa faeces. Biol Fertil Soils. 4, 115–118. doi: 10.1007/BF00256983
Deshmukh, P. W., Shinde, R., Jadhao, S. D., Kharche, V. K., and Mali, D. V. (2015). Effect of integrated plant nutrient system on passive and active pools of organic carbon in soybean-chickpea sequence. Agropedology. 25, 133–139.
Doetterl, S., Stevens, A., and Six, J. (2015). Soil carbon storage controlled by interactions between geochemistry and climate. Nat. Geosci. 8, 780–783. doi: 10.1038/ngeo2516
Dutta, D., Singh, D. K., Subash, N., Kumar, V., Meena, A. L., Mishra, R. P., et al. (2018). Effect of long-term use of organic, inorganic and integrated management practices on carbon sequestration and soil carbon pools in different cropping systems in Tarai region of Kumayun hills. Indian J. Agri. Sci. 88, 523–552. doi: 10.56093/ijas.v88i4.79066
Gao, C., El-Sawah, A. M., Ali, D. F. I., Hamoud, Y. A., Shaghaleh, H., and Sheteiwy, M. S. (2020). The integration of bio and organic fertilizers improve plant growth, grain yield, quality and metabolism of hybrid maize (Zeamays L.). Agronomy. 10, 319. doi: 10.3390/agronomy10030319
Gathala, M. K., kanthalia, P., Verma, C., and Chahar, M.S. (2007). Effect of integrated nutrient management on soil properties and humus fraction in the long term fertilizer experiments. J. Indian Soc. Soil Sci. 55, 360–363.
Gomez, K. A., and Gomez, A. A. (1976). Statistical Procedure for Agricultural Research. New York, NY: Wiley Inter-Science Publication. p. 680.
Hu, J.;, Lin, X., Wang, J., Dai, J., Chen, R., and Zhang, J. (2011). Microbial functional diversity, metabolic quotient, and invertase activity of a sandy loam soil as affected by long-term application of organic amendment and mineral fertilizer. J. Soils Sediments. 11, 271–280. doi: 10.1007/s11368-010-0308-1
Huang, S., Zhang, W., Yu, X., and Huang, Q. (2010). Effects of long-term fertilization on corn productivity and its sustainability in an Ultisol of southern China. Agric. Ecosyst. Environ. 138, 44–50. doi: 10.1016/j.agee.2010.03.015
Johnston, A. E. (2007). Soil organic matter, effects on soil and crop. Soil Use Manag. 2, 97–105. doi: 10.1111/j.1475-2743.1986.tb00690.x
Kallenbach, C., and Grandy, A. S. (2011). Controls over soil microbial biomass responses to carbon amendments in agricultural systems: a meta-analysis. Agric Ecosyst Environ. 144, 241–252. doi: 10.1016/j.agee.2011.08.020
Katyal, J. C. (2000). Organic matter maintenance, main stay of soil quality. J Indian Soc Soil Sci. 48, 704–716.
Kaur, T., Brar, B. S., and Dhillon, N. S. (2008). Soil organic matter dynamics as affected by long-term use of organic and inorganic fertilizers under maize-wheat cropping system. Nutr. Cycl. Agroecosyst. 81, 59–69. doi: 10.1007/s10705-007-9152-0
Kononova, M. M. (1966). Soil Organic Matter: Its Nature, Its Role in Formation and Soil Fertility. Moscow: Pub.Izd. AkadNewk, USSR.
Kukreja, K., Mishra, M., Dhankar, S. S., Kapoor, K. K., and Gupta, A. P. (1991). Effect of long-term manurial application on microbial biomass. J Indian Soc Soil Sci. 39, 685–688.
Kumar, V., Saikia, J., Barik, N., and Das, T. (2017). Effect of integrated nutrient management on soil enzymes, microbial biomass carbon and microbial population under okra cultivation. IJBCRR 20, 1–7. doi: 10.9734/IJBCRR/2017/38868
Kumari, G., Mishra, B., Kumar, R., Agarwal, B. K., and Sing, B. P. (2011). Long term effect of manure, fertilizer and lime application on active and passive pools of soil organic carbon under maize-wheat cropping system in an alfisols. J. Indian Soc. Soil. Sci. 59, 245–250.
Kurbah, I., and Dixit, S. P. (2020). Effect of fertilizer application based on soil test crop response approach on active and passive pools of soil organic matter in maize–wheat cropping system. Arch. Agron. Soil. Sci. 66, 858–871. doi: 10.1080/03650340.2019.1642468
Laik, R., Kumara, B. H., Pramanick, B., Singh, S. K., Nidhi, A.lhomrani, M., Gaber, A., et al. (2021a). Labile soil organic matter pools are influenced by 45 years of applied farmyard manure and mineral nitrogen in the wheat—pearl millet cropping system in the sub-tropical condition. Agronomy 11, 2190. doi: 10.3390/agronomy11112190
Laik, R., Singh, S. K., Pramanick, B., Kumari, V., Nath, D., Dessoky, E. S., et al. (2021b). Improved method of boron fertilization in rice (Oryza sativa L.)–mustard (Brassica juncea L.) cropping system in upland calcareous soils. Sustainability 13, 5037. doi: 10.3390/su13095037
Lakshmi, P. V., Singh, S. K., Pramanick, B., Kumar, M., Laik, R., Kumari, A., et al. (2021). Long term zinc fertilization in calcareous soils improves wheat (Triticum aestivum L.) productivity and soil zinc status in the rice-wheat cropping system. Agronomy 11, 1306. doi: 10.3390/agronomy11071306
Majumder, B., Mandal, B., and Bandyopadhyay, P. K. (2008). Organic amendments influence soil organic carbon pools and rice-wheat productivity. Sci. Soc. Am. J. 72, 775–785. doi: 10.2136/sssaj2006.0378
Mandal, A., Toor, A. S., and Dhaliwal, S. S. (2020). Assessment of sequestered organic carbon and its pools under different agricultural land-uses in semi-arid soils of south-western Punjab, India. J. Soil Sci. Plant. Nutr. 20, 259–273. doi: 10.1007/s42729-019-00137-5
Manna, M. C., and Ganguly, T. K. (2000). Rock phosphate and pyrite in compost technology. Fert News. 45, 41–45.
Meena, M. D., and Biswas, D. R. (2015). Effect of rock phosphate enriched compost and chemical fertilizers on microbial biomass phosphorus and phosphorus fraction. Afr. J. Microbiol. Res. 9, 1519–1526. doi: 10.5897/AJMR2014.6957
Meena, S. K. (2017). Effect of Long Term Application of Fertilizer and Manure on Soil Organic Carbon Fractions under Maize-Wheat Cropping Sequence in Haplustepts. Ph.D Thesis. Udaipur: MaharanaPratap University of Agriculture and Technology.
Meloon, S. J., and Sommers, R. S. (1996). Acid extraction method (water soluble carbon) in soil. Soil Biol. Biochem. 39, 511–513.
Meshram, N. A., Syed, I., and Patil, V. D. (2016). Long-term effect of organic manuring and inorganic fertilization on humus fractionation, microbial community and enzymes assay in vertisol. J. Pure. Appl. Microbiol. 10, 139–150.
Meshram, N. A., Syed, I., and Rathod, P. K. (2018). Effect of FYM and inorganic fertilization on soil organic matter fractions under long term fertilizer experiment in vertisol. J. Agric. Res. Technol. 43, 459–464.
Mohanty, S., Nayak, A. K., and Kumar, A. (2013). Carbon and nitrogen mineralization kinetics in soil of rice-rice system under long-term application of chemical fertilizers and farmyard manure. Eur. J. Soil. Biol. 58, 113–121. doi: 10.1016/j.ejsobi.2013.07.004
Murphy, B. W. (2015). Impact of soil organic matter on soil properties- a review with emphasis on Australian soils. Soil Res. 53, 605–635. doi: 10.1071/SR14246
Naik, B. S. S. S., Sharma, S. K., Pramanick, B., Chaudhary, R., Yadav, S. K, Tirunagari, R., Gaber, A., and Hossain, A. (2022). Silicon in combination with farmyard manure improves the productivity, quality and nitrogen use efficiency of sweet corn in an organic farming system. Silicon 14, 5733–5743. doi: 10.1007/s12633-022-01818-0
Nardi, S., Pizzeghello, D., Muscolo, A., and Vianello, A. (2002). Physiological effects of humic substances on higher plants. Soil Biol. Biochem. 34, 1527–1536. doi: 10.1016/S0038-0717(02)00174-8
Obalum, S. E., Chibuike, G. U., Peth, S., and Ouyang, Y. (2017). Soil organic matter as sole indicator of soil degradation. Environ. Monit. Assess 189, 176. doi: 10.1007/s10661-017-5881-y
Ogumba, P. O., Obalum, S. E., and Uzoh, I. M. (2020). Soil biochemical and microbial properties of sandy loam Ultisols as affected by some tillage and nutrient management practices. Int. J. Agric. Rural Develop. 23, 5184–5195.
Padbhushan, R., Sharma, S., Kumar, U., Rana, D., Kohli, A., Kaviraj, M., et al. (2021). Meta-analysis approach to measure the effect of integrated nutrient management on crop performance, microbial activity, and carbon stocks in Indian soils. Front. Environ. Sci. 9, 724702. doi: 10.3389/fenvs.2021.724702
Padbhushan, R., Rakshit, R., Das, A., and Sharma, R. P. (2014). Effects of various organic amendments on organic carbon pools and water stable aggregates under a scented rice-potato-onion cropping system. Paddy Water Environ. 14, 481–489. doi: 10.1007/s10333-015-0517-8
Piccolo, A., Celano, G., and Pietramellara, G. (1993). Effects of fractions of coal-derived humic substances on seed germination and growth of seedlings (Lactuga sativa and Lycopersicumesculentum). Biol. Fertil. Soil. 16, 11–15. doi: 10.1007/BF00336508
Pramanick, B., Brahmachari, K., Ghosh, D., and Bera, P. S. (2018). Influence of foliar application seaweed (Kappaphycus and Gracilaria) saps in rice (Oryza sativa)–potato (Solanum tuberosum)–blackgram (Vigna mungo) sequence. Indian J. Agron. 63, 7–12.
Pramanick, B., Kumar, M., Naik, B. M., Kumar, M., Singh, S. K., Maitra, S., et al. (2022). Long-term conservation tillage and precision nutrient management in maize–wheat cropping system: effect on soil properties, crop production, and economics. Agronomy 12, 2766. doi: 10.3390/agronomy12112766
Pramanick, B., Mahapatra, B. S., Datta, D., Dey, P., Singh, S. P., Kumar, A., et al. (2023). An innovative approach to improve oil production and quality of mustard (Brassica juncea L.) with multi-nutrient-rich polyhalite. Heliyon 9, e13997. doi: 10.1016/j.heliyon.2023.e13997
Prasad, A., Totey, N. G., Khatri, P. K., and Bhowmik, A. K. (1991). Effect of added tree leaves on the composition of humus and availability of nutrient in soil. J Indian Soc Soil Sci. 39, 429–434.
Rajput, R., Pokhriya, P., Panwar, P., Arunachalam, A., and Arunachalam, K. (2019). Soil Nutrients, Microbial Biomass, and Crop Response to Organic Amendments in rice Cropping System in theShiwaliks of Indian Himalayas. Int. J. Recycl Org. Waste Agricult. 8, 73–85. doi: 10.1007/s40093-018-0230-x
Ranjana, A., Gupta, B. R., and Bajpai, P. P. (1984). Studies on composition of humus in different soil groups of Uttar Pradesh. J Indian Soc Soil Sci. 32, 26–31.
Riffaldi, R., Saviozzi, A., and Levi-Minzi, R. (1996). Carbon mineralization kinetics as influenced by soil properties. Biol. Fertil. Soils. 22, 293–298. doi: 10.1007/BF00334572
Saizet, J. C., Haider, K., and Meuzelaar, H. L. C. (1979). Comparisons of soil organic matter and its fractions by pyrolysis mass-spectometry. Geoderma. 32, 25–37. doi: 10.1016/0016-7061(79)90037-5
Santhy P. Muthuvel and, P. Selvi D. (2001). Humus and impact of organic matter fractions on yield, uptake and available nutrients in a long-term fertilizer experiment. J Indian Soc Soil Sci. 49, 281–285.
Sher, A., Adnan, M., Sattar, A., Ul-Allah, S., Ijaz, M., Hassan, M. U., et al. (2022). Combined application of organic and inorganic amendments improved the yield and nutritional quality of forage sorghum. Agronomy. 12, 896. doi: 10.3390/agronomy12040896
Singh, R., Pramanick, B., Singh, A. P., Neelam, K.umar, S., Kumar, A., and Singh, G. (2017). Bio-efficacy of Fenoxaprop-P-Ethyl for grassy weed control in onion and its residual effect on succeeding maize crop. Indian J. Weed Sci. 49, 63–66.
Smith, J. L., and Paul, E. A. (1990). The Significance of Soil Microbial Biomass Estimations. Soil Biology. Vol. 6, Bollag, J. M. and Stotzuy, G. Eds. New York, NY: Marcel Dekker. p. 357.
Song, Z., Gao, H., Zhu, P., Peng, C., Deng, A., Zheng, C., et al. (2015). Organic amendments increase corn yield by enhancing soil resilience to climate change. Crop. J. 3, 110–117. doi: 10.1016/j.cj.2015.01.004
Stevenson, F. J. (1994a). Humus Chemistry: Genesis, Composition, Reactions, 2nd Edition. New York, NY: John Wiley, pp. 512.
Stevenson, F. J. (1994b). Organic forms of soil nitrogen. In: John, W., editor. Humic Chemistry: Genesis, Composition, Reaction. New York, NY: John Wiley. p. 59–95.
Stevenson, F. J. (1965). “Gross chemical fractionation of organic matter,” in Methods of Soil Analysis: Part 2 Chemical and Microbiological Properties, ed A. G. Norman (American Society of Agronomy, Inc.). doi: 10.2134/agronmonogr9.2.c43
Tamilselvi, S. M., Chinnadurai, C., Ilamurugu, K., Arulmozhiselvan, K., and Balachandar, D. (2014). Effect of long-term nutrient managements on biological and biochemical properties of semi-arid tropical alfisol during maize crop development stages. Ecol. Indic. 48, 76–87. doi: 10.1016/j.ecolind.2014.08.001
Vance, E. D., Brookers, P. C., and Jenkinson, D. S. (1987). An extraction method for measuring soil microbial biomass carbon. Soil Biol Biochem. 19, 703–707. doi: 10.1016/0038-0717(87)90052-6
Vaughan, D., and Malcolm, R. E. (1985). Influence of humic substances on growth and physiological processes. In: Vaughan D, Malcolm RE, editors. Soil Organic Matter and Biological Activity. Dordrecht: MartinusNijhoff/Junk W. p. 37–76.
Verma, G., and Mathur, A. K. (2009). Effect of integrated nutrient management on active pools of soil organic matter under maize-wheat system of a typichaplustept. J. Indian Soc. Soil. Sci. 57, 317–322.
Zhao, M., Zhou, J., and Kalbitz, K. (2008). Carbon mineralization and properties of water-extractable organic carbon in soils of the south Loess Plateau in China. Eur. J. Soil. Biol. 44, 158–165. doi: 10.1016/j.ejsobi.2007.09.007
Keywords: integrated nutrient management, active fractions, passive fractions, soil organic matter, humic acid
Citation: Bamboriya JS, Purohit HS, Naik BSSS, Pramanick B, Bamboriya SD, Bamboriya SD, Doodhawal K, Sunda SL, Medida SK, Gaber A, Alsuhaibani AM and Hossain A (2023) Monitoring the effect of integrated nutrient management practices on soil health in maize-based cropping system. Front. Sustain. Food Syst. 7:1242806. doi: 10.3389/fsufs.2023.1242806
Received: 19 June 2023; Accepted: 10 August 2023;
Published: 12 September 2023.
Edited by:
Sudhakar Srivastava, Banaras Hindu University, IndiaReviewed by:
Sunday Ewele Obalum, University of Nigeria, Nsukka, NigeriaCopyright © 2023 Bamboriya, Purohit, Naik, Pramanick, Bamboriya, Bamboriya, Doodhawal, Sunda, Medida, Gaber, Alsuhaibani and Hossain. This is an open-access article distributed under the terms of the Creative Commons Attribution License (CC BY). The use, distribution or reproduction in other forums is permitted, provided the original author(s) and the copyright owner(s) are credited and that the original publication in this journal is cited, in accordance with accepted academic practice. No use, distribution or reproduction is permitted which does not comply with these terms.
*Correspondence: Biswajit Pramanick, Ymlzd2FqaXRAcnBjYXUuYWMuaW4=; Akbar Hossain, YWtiYXJob3NzYWlud3JjQGdtYWlsLmNvbQ==
Disclaimer: All claims expressed in this article are solely those of the authors and do not necessarily represent those of their affiliated organizations, or those of the publisher, the editors and the reviewers. Any product that may be evaluated in this article or claim that may be made by its manufacturer is not guaranteed or endorsed by the publisher.
Research integrity at Frontiers
Learn more about the work of our research integrity team to safeguard the quality of each article we publish.