- 1Department of Agronomy, Bidhan Chandra Krishi Viswavidyalaya, Haringhata, West Bengal, India
- 2School of Agriculture and Allied Sciences, The Neotia University, Sarisha, West Bengal, India
- 3Department of Agricultural Meteorology and Physics, Bidhan Chandra Krishi Viswavidyalaya, Haringhata, India
- 4Department of Crop Science, Central Research Institute for Dryland Agriculture, Hyderabad, India
- 5Department of Agronomy and Agroforestry, Centurion University of Technology and Management, Paralekhamundi, India
- 6Department of Biology, College of Science, Taif University, Taif, Saudi Arabia
- 7Department of Physical Sport Science, College of Education, Princess Nourah bint Abdulrahman University, Riyadh, Saudi Arabia
- 8Division of Soil Science, Bangladesh Wheat and Maize Research Institute, Dinajpur, Bangladesh
Conventional tillage methods and indiscriminate use of chemical fertilizers are causes of edaphic problems like soil degradation and loss of soil fertility which reduces crop yield. Puddling now-a-days, has become a major challenge for farmers due to breaking the soil structure, deficit water regimes, and depletion of soil health. Keeping in view, the absolute need to fulfill food security as well as sustainability, an experiment was conducted for 2 years on a rice-lentil cropping system during 2018–2019 and 2019–2020 in sandy clay loam soil of a new alluvial zone of eastern India to evaluate effects of minimum tillage and integrated nutrient management on yield and soil health. Conventional tillage (CT) direct seeded rice-lentil, Minimum tillage(MT) direct seeded rice-lentil, MT transplanted rice-lentil and MT direct seeded rice-lentil were kept as main plot treatments and control (without any fertilizer), 100% RDF, 75% nitrogen + FYM, 75% nitrogen + FYM + Azospirillum and 75% Nitrogen + FYM + Azospirillum sp. + Zinc Sulphate were kept as subplot treatments during the study. Though conventional tillage treatments recorded higher LAI, CGR and yield in the first year of study in the case of rice; during the second year, minimum tillage treatments showed significantly (p ≤ 0.05) better results in the aforesaid aspects with MTDSR-L having a maximum yield of 5.17 t ha−1. In the case of lentil minimum tillage treatments had better results for both years, with MTDSR-L treatment having the highest yield of 8.084 t ha−1. Among the nutrient schedules, the crops had better LAI, CGR and yield during the first year in the case of 100% RDF treatment, but in the second year, 75% Nitrogen + FYM + Azospirillum sp. + Zinc Sulphate had the highest respective values. Soil organic carbon was marginally improved by both tillage and nutrient treatments, while soil microbial biomass carbon, dehydrogenase activity and microbial count were significantly influenced. The combination of MTDSR-L and 75% Nitrogen + FYM + Azospirillum sp. + Zinc Sulphate (N5) treatment showed the maximum values for all soil biological parameters leading to improve soil health. The results of the experiment concluded that the practice of minimum tillage and integrated nutrient management may be recommended to enhance the yield and stability of rice-lentil cropping systems in sandy clay loam soil of a new alluvial zone of eastern India by acting as an alternative for the decline of soil health.
Introduction
The conventional farming system, which previously increased food productivity, has now stagnated at the expense of the environment and natural resources. The population growth, which is anticipated to reach 9.7 billion people in 2050, makes the existing situation worse (FAO, 2019). Injudicious use of chemical fertilizers and different types of farm machinery for the aforesaid reasons has jeopardized the subtle balance of agro-ecosystems causing various ecological stresses and exploitation of natural resources (Sharma et al., 2019; Bisht and Chauhan, 2020). Similar to fertilizers, conventional tillage is also reported to have a huge impact on soil physical, chemical and biological properties which further are closely related to crop yield. Conventional tillage methods have given rise to edaphic problems like soil degradation, soil erosion and loss of soil fertility (Wani et al., 2023). Furthermore, the present issues with climate change, which cause abrupt floods, droughts, increases in temperature, and other changes, have been a huge burden for agriculture, because it is heavily dependent on the weather. So, developing a more resilient, climate-smart alternative to traditional agriculture is crucial for ensuring food security in a way that is economical and long-term so that all farm species and natural resources are protected (Pretty, 2008; Venugopalan et al., 2022).
Continuous use of conventional tillage has been found to degrade soil causing soil erosion and loss of soil fertility. The main objective of conservation tillage is to arrest such soil degradation by retaining crop residues in soil which in turn improves soil organic carbon. Conservation tillage maintains proper pore distribution and stability of the soil, unlike the conventional tillage practices that destructs the soil aggregates causing a hard pan (Das et al., 2020). Minimum tillage is one such conservation tillage practice where tillage is restricted to the minimum level necessary for a good seedbed preparation ensuring satisfactory crop stand and favorable growing conditions. Better nutrient cycling, energy transfers, and soil enzymatic activity have been reported in long-term conservation tillage systems and enzymatic activity and soil organic carbon are said to have a close relationship with soil health (Dick, 1994). Accumulation of crop residues increases the soil microbial biomass, carbon, dehydrogenase activity, and respiration activity of microbes gradually improve soil fertility in the long run (Hungria et al., 2009). Though soil has the innate ability to provide plants with the necessary nutrients, external application of nutrients in the form of organic or inorganic nutrient sources is necessary to supplement the soil sink for more crop production. A balanced approach can promote soil health in harmony with the agro-ecosystem, along with improving the production of high-quality crops without depleting natural resources (Sarkar et al., 2020). Integrated nutrient management refers to the maintenance of soil fertility and plant nutrient supply at an optimum level for sustaining the desired productivity through optimization of the benefits from all possible sources of organic, inorganic and biological components in an integrated manner. Implementing ecological principles like conservation practices and integrated nutrient management can improve species diversity in soil which further makes agro-ecosystem more sustainable (Lal, 2023).
India has the largest area of 45.07 Mha, in the world under rice cultivation of which West Bengal is the highest rice-producing state having production of 16.65 million tonnes (Directorate of Economics and Statistics, 2021; Moulick et al., 2021). Puddling is a common practice for rice cultivation. With the scarcity of water looming in the future due to uneven rainfall patterns, cultivating rice in puddled conditions is becoming a challenge. Minimum tillage in this case can act as an alternative since it reduces the time and water required for land preparation of the succeeding crop as well as reducing the use of tillage implements and thereby saving soil’s physical structure from deterioration. Considering the nutritional security and soil fertility, the cereal-cereal cropping system does not fulfill the sustainability aspect of the agroecosystem. Incorporation of a pulse crop in a cropping sequence is essential in terms of crop diversification, soil health enhancement, nitrogen economy and carry-over effects (Praharaj et al., 2021). The introduction of legumes in the cropping system highly improves soil aggregation due to the presence of its intricate roots and the organic root exudates. This results in the formation of newer soil aggregates. Hence a proper crop rotation helps in maintaining soil aggregation dynamics providing a healthy resilient soil environment (Hillel, 2004). After Chickpea, lentil is the second most important rabi pulse crop in India (Ahmed et al., 2018), having a production and productivity of 1.45 million tonnes and 1,001 kg ha−1, respectively, (Directorate of Economics and Statistics, 2021). West Bengal is the third-highest lentil-producing state in India. It is the most widely grown rabi pulse crop after Aman (June–November) rice in West Bengal having a productivity of 855 kg ha−1 (Directorate of Economics and Statistics, 2021). Growing profitable lentil on residual soil moisture and nutrients from preceding rice cultivation is an important resource conservation technology. With this background, a study was initiated to understand the impact of different conservation tillage practices and integrated nutrient management schedules on growth, yield and soil microbes.
Materials and methods
Experimental site
The field experiment was conducted during the kharif-rabi seasons of 2018–2019 and 2019–2020 at the Instructional Farm of Bidhan Chandra Krishi Viswavidyalaya, Jaguli, Nadia, West Bengal, India. The farm is located at 22° 93′ N latitude, 88° 53′ E longitude and 9.75 m above mean sea level (MSL). The experimental study site is medium land with good irrigation and drainage facilities. The soil is typical new alluvial, Entisol and sandy clay-loam in texture with moderate water holding capacity. The soil was having 26.19% silt (0.002–0.02 mm), 32.45% clay (<0.002 mm), 36.17% fine sand (0.02–0.2 mm) and 5.19% coarse sand (0.2–2 mm) with pH 6.59. The soil of the experimental field had low organic carbon (0.51%) (Walkley and Black method), medium available nitrogen (alkaline permanganate-oxidizable) (243 kg ha−1), high available P2O5(Olsen’P) (28.5 kg ha−1) and K2O (NH4OAC-extractable) (253.63 kg ha−1).
Climatic condition
The place under study lies in the sub-humid and subtropical zone since it’s situated near the Tropic of Cancer. It falls in the alluvial Indo-Gangetic agro-ecological zone. The average rainfall annually in this region is 1,440 mm. The mean monthly temperature ranges from 37.4°C (maximum) to 9.7°C (minimum). Generally, the average temperature ranges from 37.6°C to 25.4°C during summer months and 23.7 to 8.5°C during winter months. May is the hottest month in this region whereas winter here is quite mild and short. The temperature gradually increases from March and reaches its highest by the end of May. Generally, the second week of June is the onset of monsoon and it extends till the last of September to the middle of October. Pre-monsoon rain is quite common from March to May due to Nor’wester showers. The rainfall is erratic and irregular. During the winter months, rainfall was sufficient during both years of experiments due to the ‘depression’ occurring in the Bay of Bengal. For both the years of the experiment, maximum rainfall was received during the monsoon season. Maximum humidity was observed in July 2018 (89.18%) and in August 2019 (88.94%) while the minimum was recorded during the winter months of both years. The monthly mean meteorological data for the 2 years of the experiment are given in Supplementary Figure S1.
Experimental design and treatments
The design used in the experiment was a split-plot design, having four main plots, five subplots and three replications. The main plots included: i) Conventional tillage (CT) direct seeded rice –lentil ii) Minimum tillage(MT) direct seeded rice-lentil iii) conventional tillage (CT) transplanted rice-lentil iv) Minimum tillage(MT) transplanted rice-lentil whereas subplots included i) Control (no application of nutrients) – lentil, ii) 100% RDF (80:40:40) (N: P2O5:K2O) kg ha−1 (As per government of West Bengal recommendations) – lentil, iii) 75% Nitrogen + FYM (Blanket dose of 10 t ha−1) – lentil, iv) 75% Nitrogen +FYM (Blanket dose of 10 t ha-1) + Azospirillum sp. (2 kg ha−1) – lentil, v) 75% Nitrogen + FYM (Blanket dose of 10 t ha−1) + Azospirillum sp.(2 kg ha−1) + Zinc Sulphate Heptahydrate (ZnSO4,7H2O) @ 20 kg ha−1) – lentil. The treatment description and abbreviations used are shown in Table 1.
Crop management practices
Each experimental plot was having a size of 6 × 4 m2. In CT, two ploughings by tractor-drawn disc-harrow were followed by two ploughings by a harrow with the help of a rotovator and one planking for uniform land leveling. The CT practices were the same for both direct seeded and transplanted rice. In MT, one plowing was followed by one harrowing. MT practices were the same for both the direct seeded and transplanted rice. No further land preparation was done for sowing lentil. Fertilizer P2O5 and K2O were applied at the same rate, i.e., @ 40 kg ha−1 each in all subplots as basal dose except the control plot before sowing of rice. Nitrogen was applied @ 0/80/60 kg ha−1 as per treatment requirement. No fertilizers have been added for lentil cultivation. The experiment used the rice variety Satabdi (IET-4786) and the lentil variety HUL-57.
Growth and yield parameters
For studying various growth characteristics of plants (rice and lentil), ten random plants were selected from each treatment from one-meter row length leaving two rows from all sides of the border, in each phenotypical stage (vegetative, flowering and maturity stage) as plant samples. For calculation of LAI and CGR, samples were dried under the sun for one to two days before drying them in the oven at a regulated temperature of 65° C ± 1°CThree random plants per treatment were measured to determine the leaf area and the following equation (Eq. 1) was used to determine the LAI:
The crop growth rate is the rate of increase in dry matter accumulation per unit of time per unit of ground area. It is expressed in g m−2 day−1 and calculated by the formula:
Where, W2 is the dry weight of plant (g m−2) at time t2 and W1 is the dry weight of plant (g m−2) at time t1, t2−t1 is the time interval in days.
Both the yield of rice and lentil were recorded when crops achieved physiological maturity. After cutting from the ground, they were dried under the sun for 3–5 days on the threshing floor. Then the grains from the panicle were threshed and grain yield and straw yield from individual plots were taken and expressed as t ha−1. In the case of lentil the pods were threshed, and the seeds were collected and weighed for each treatment plot. The seed yield and stalk yield were further converted on a hectare basis.
Soil biological parameters
Soil upto 0–15 cm depth was collected with the help of an auger from each experimental plot prior to sowing and after harvesting of each crop for both years of experiment. Thereafter the soil samples were sealed in plastic packets and stored at 4°C in the fridge for different soil biological parameters analysis. The analysis was done within 1 month of soil sample collection. Organic carbon content in the soils was estimated by the modified Walkely and Black method as described by Jackson (1973). Soil microbial biomass carbon was calculated by the following equation as described by Jenkinson (1966):
Where, “B” is the soil “microbial biomass carbon, “X” is the amount of CO2-C which evolved from fumigated soil and “Y” is the amount of CO2-C evolved from non-fumigated control soil. “k” is the fraction of the killed biomass-C that decomposed to CO2 during the 10 days after fumigation. It was expressed in μg of CO2 evolved per g of soil.
For dehydrogenase activity fresh soil sample of 5 gm was treated with TTC (2,3,5-Triphenyltetrazolium chloride) and incubated with or without electron donating substrate for 96 h in the presence of glucose at 27°C (Klein et al., 1971). The dehydrogenase activity was measured in the form of formazan which was expressed in μg per gm of oven-dried soil.
The microbial population occurring in the soil samples were determined by serial dilution technique and pour plate method (Prammer and Schmidt) using agar plates having appropriate medium. Thornton’s agar medium (Thorton, 1922), Martin’s rose Bengal streptomycin agar medium (Martin, 1950) and Jensen’s agar medium (Jensen et al., 2012) were used for counting the total number of viable bacteria, fungi and actinomycetes, respectively.
Statistical analysis
Statistical analysis of all data was done by ANOVA technique for split-plot design and the least significant values were tested by Duncan’s Multiple Range Test (DMRT) (p ≤ 0.05) among various tillage and nutrient treatments to find out the trend and variations of different parameters. Pearson correlations were presented in a correlogram with significant levels (p ≤ 0.05, p ≤ 0.001, p ≤ 0.01) and were deduced with the help of ‘psych’ package in R to evaluate relationships between various soil microbial parameters with rice and lentil yield. Multiple linear regression (p ≤ 0.05, p ≤ 0.001, p ≤ 0.01 and p ≤ 0.1) was also conducted to identify the relationship between the microbial count data with organic carbon under different tillage systems.
Results
Impact of tillage and nutrients on LAI and CGR of rice and lentil
LAI and CGR are considered to be important growth parameters of any crop. In both the years of experiment, they varied significantly across various tillage treatments and nutrients. LAI and CGR increased with the advancement of age for both crops being maximum during the peak period of vegetative growth, i.e., 60 DAS. They showed a decreasing trend as the crops neared their maturity due to the effect of senescence. It was observed that the first year of transplanted rice grown in CT plots (Figures 1A, 2A) attained the highest LAI and CGR, followed by MTTPR-L (Figures 1B, 2B) at all the dates of observations. Rice grown in CTTPR-L plots recorded (6.361) 9.13% higher LAI and 10.74% more CGR (7.95 g m−2 day−1) compared to CTDSR-L plots which recorded the lowest LAI (5.829) and CGR (7.27 g m−2 day−1) among all tillage levels at maturity stage. Total rainfall recorded during the rice growing season in the first year was found to be lesser (641.1 mm) (Supplementary Figure S1) as compared to the second year. In the next year of experimentation, MTDSR plots had the highest LAI at all stages, having 4.68% more LAI (6.563) and 3.42% more CGR (7.80 g m−2 day−1) compared to rice grown in CTTPR-L plots.
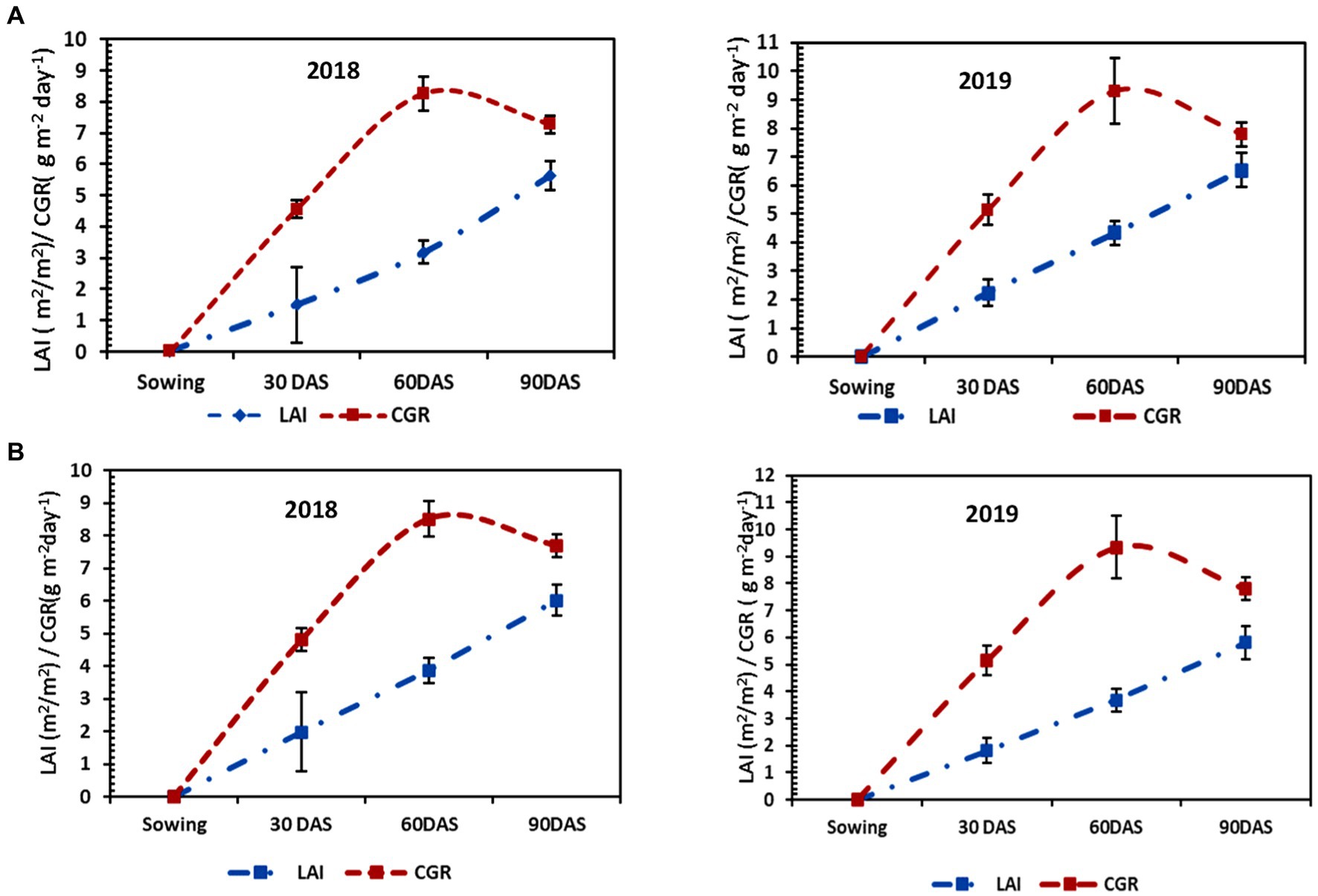
Figure 1. Growth parameters [i.e., leaf area index (LAI) and Crop Growth Rate (CGR)] of rice under (A) CTDSR-L and (B) MTDSR-L systems in both years (2018 & 2019). The error bar represents the standard deviation.
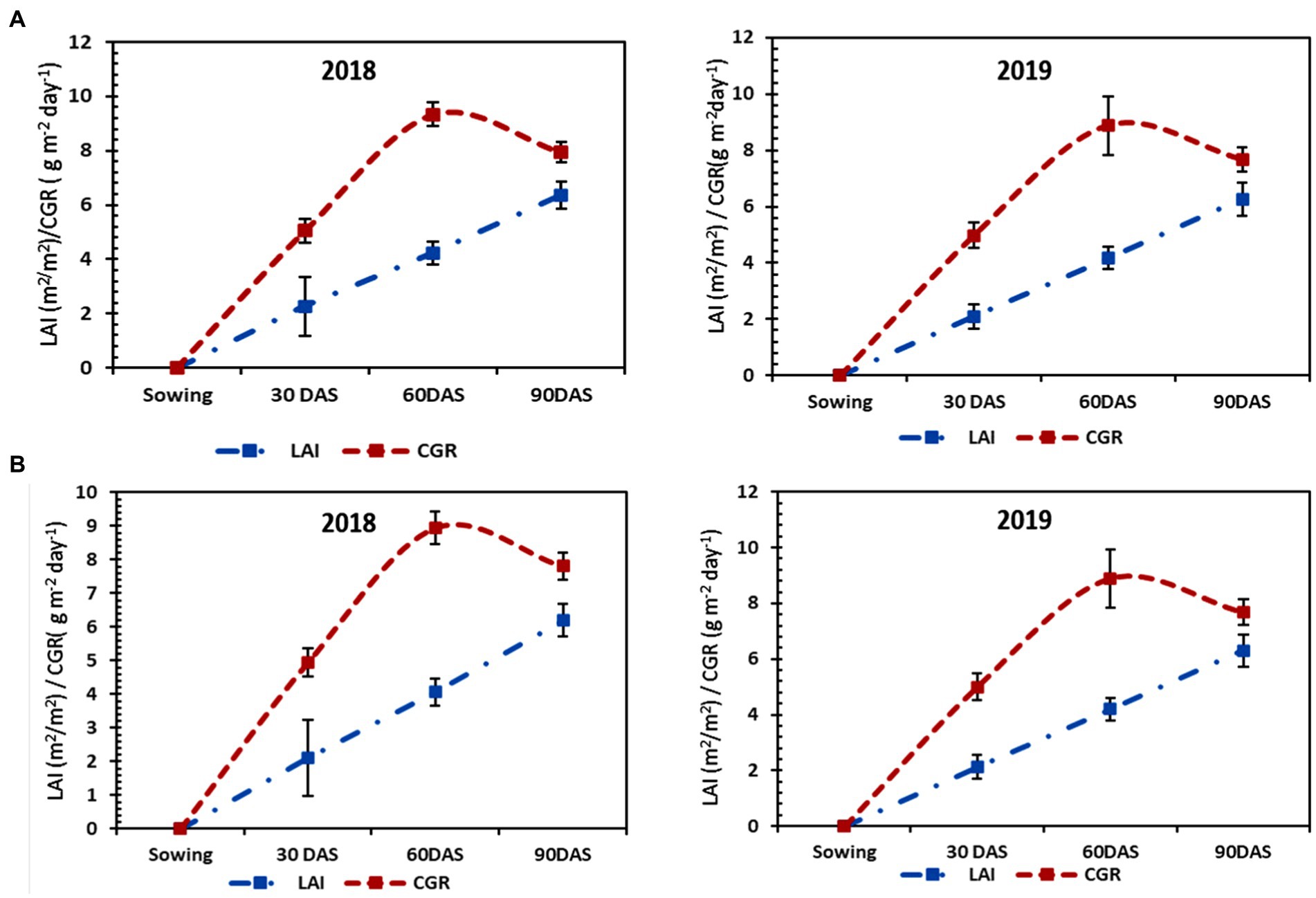
Figure 2. Growth parameters [i.e., leaf area index (LAI) and Crop Growth Rate (CGR)] of rice under (A) CTTPR-L and (B) MTTPR-L systems in both years (2018 & 2019). The error bar represents the standard deviation.
LAI and CGR of residual lentil grown on MTDSR-L plots (Figure 3B) were found to be maximum, which was statistically similar to MTTPR-L plots (Figure 4B), for all the dates of observation during the first year of study. At the maturity stage treatment of MTDSR-L obtained 1.878 as the LAI value which was 1.10% higher as compared to CTDSR-L treatment (Figures 3A,B), which recorded the lowest LAI for all stages of observation. During the flowering to maturity stage of the crop, CGR for MTDSR-L plots was 1.274 g m−2 day−1. In the next year of study, MTDSR-L treatment maintained its superiority over other treatments for both LAI & CGR values. At the maturity stage, in MTDSR-L plots the CGR value was 1.393 g m−2 day−1 which was 9.85 and 15.69% more than CTDSR-L plots (Figure 3A) and CTTPR-L plots (Figure 4A), respectively. At the maturity stage, MTDSR-L plots recorded a maximum LAI of 2.115. N4 treatment recorded the highest LAI values of 2.101 and 2.138 at the maturity stage, respectively, for both years. During the flowering to maturity stage, N4 plots recorded 1.492 g m−2 day−1 and 1.463 g m−2 day−1 as CGR, respectively, in the first and second year of study.
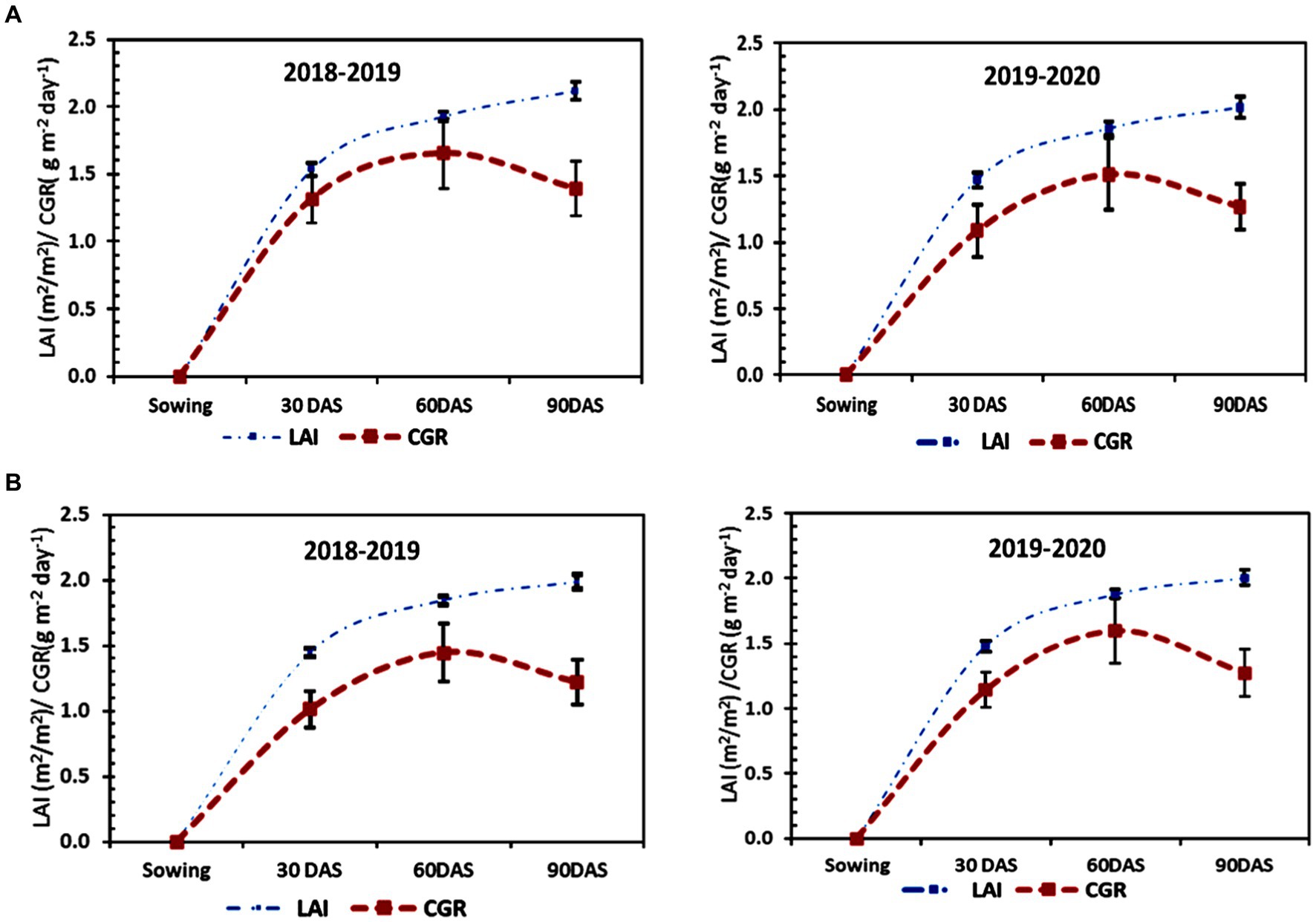
Figure 3. Growth parameters [i.e., leaf area index (LAI) and Crop Growth Rate (CGR)] of lentil under (A) CTDSR-L and (B) MTDSR-L systems in both years (2018–2019 & 2019–2020). The error bar represents the standard deviation.
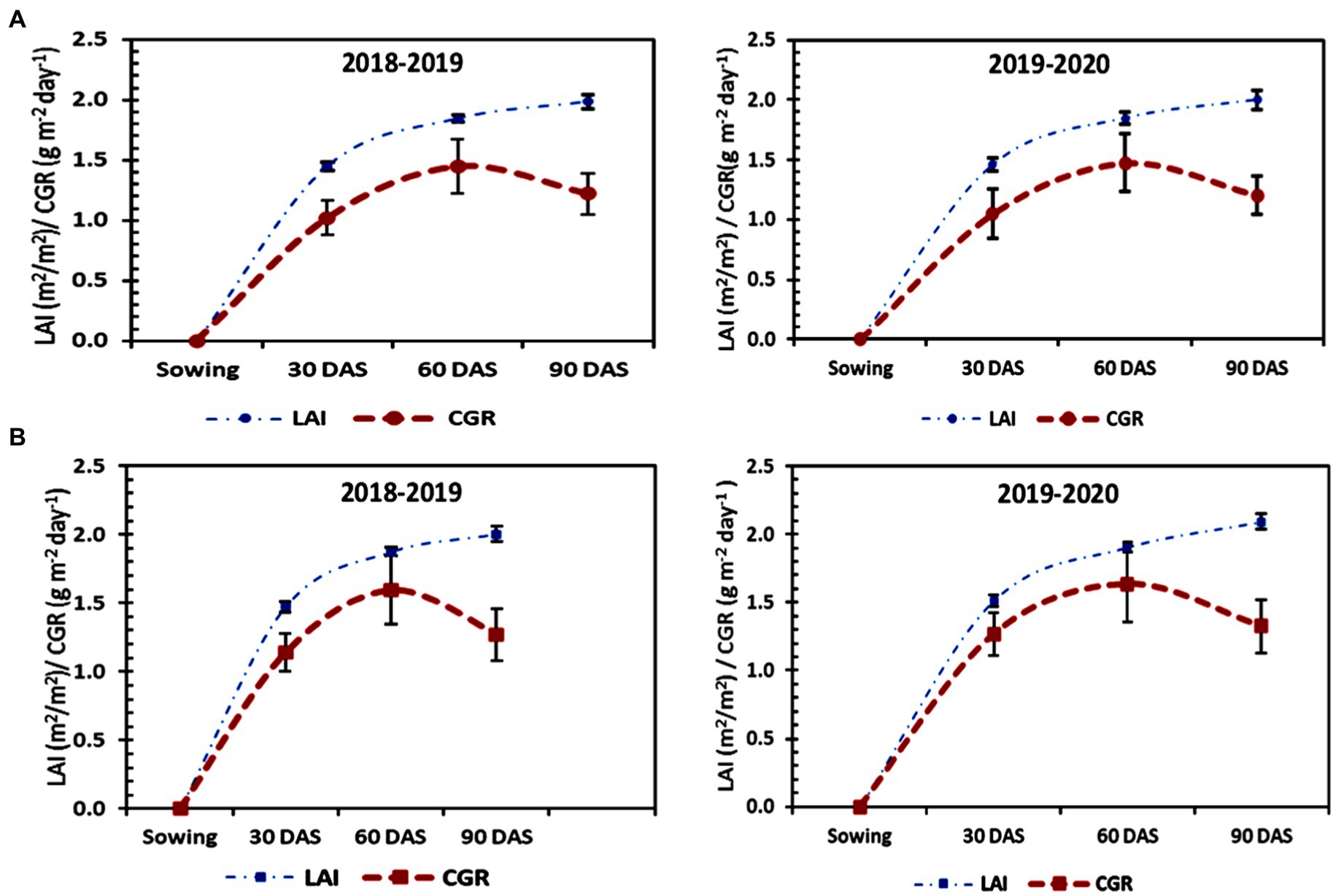
Figure 4. Growth parameters [i.e., leaf area index (LAI) and Crop Growth Rate (CGR)] of lentil under (A) CTTPR-L and (B) MTTPR-L systems in both years (2018–2019 & 2019–2020). The error bar represents the standard deviation.
There were significant changes across the various nutrient levels in terms of LAI and CGR for both rice and lentil. In the first year, the highest LAI and CGR for rice were observed in N1 plots (Figures 5A–D) throughout their entire growth period. At the maturity stage of rice, N1 plots recorded 2.47% more LAI (6.51) than N3 plots (6.353) and it maintained statistical superiority over other treatments at all stages where it recorded CGR (9.51 g m−2 day−1) during the late tillering stage to reproductive stage, indicating highest crop growth rate throughout the entire season. In the second year, N2, N3 and N4 treatments recorded statistically higher LAI and CGR than Nc and N1 treatments throughout all growth stages. N4 plots gave the highest LAI values compared to all treatments. It recorded 2.65, 4.70 & 6.995 LAI values during tillering, flowering and grain formation stages and maximum crop growth rates at all stages having 9.96 g m−2 day−1 during the late tillering stage to reproductive stage.
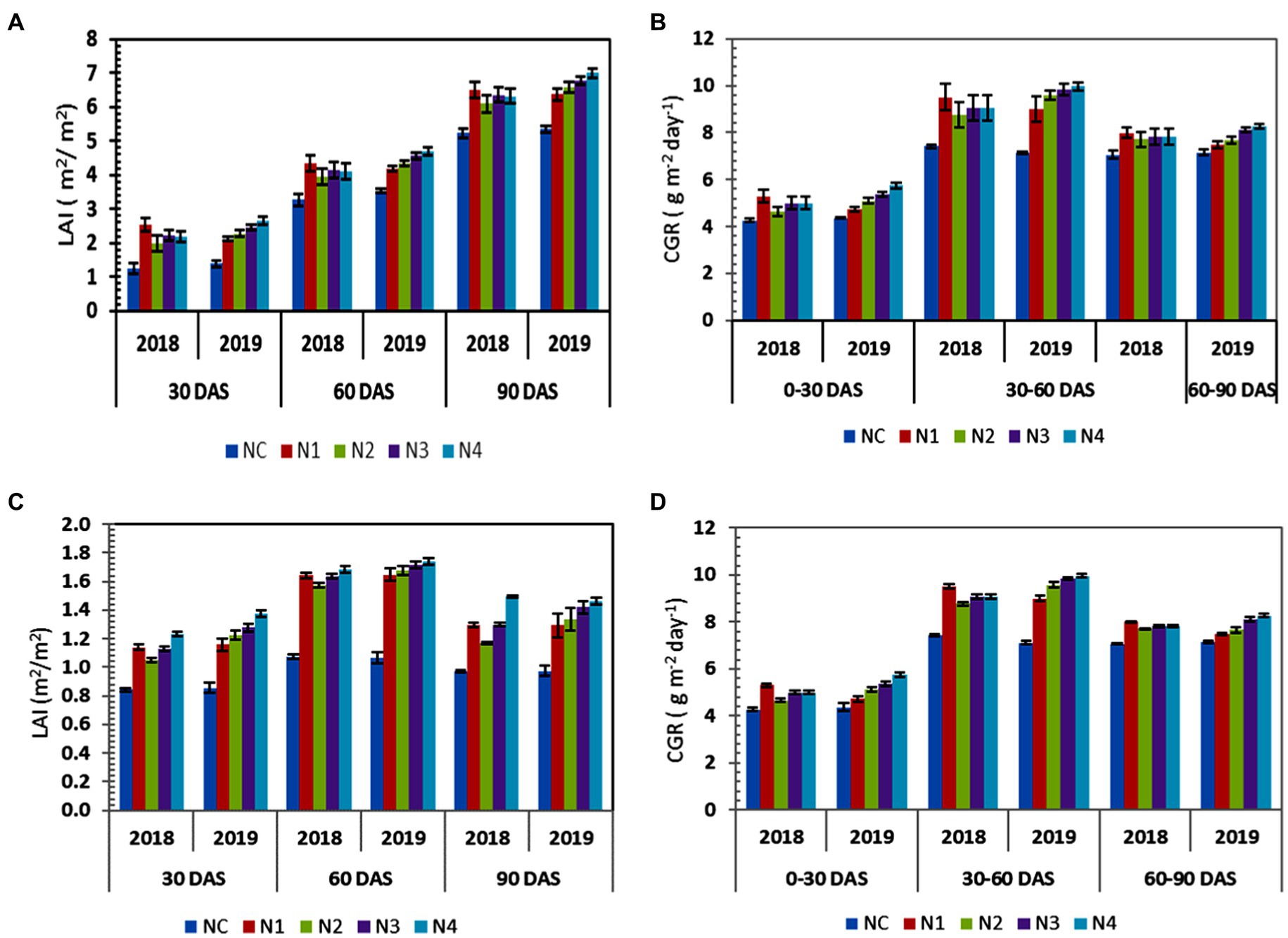
Figure 5. (A) LAI of rice under different nutrient treatments (2018 & 2019 crop seasons), (B) CGR of rice under different nutrient treatments (2018 & 2019 crop seasons), (C) LAI of lentil under different nutrient treatments (2018–2019 & 2019–2020 crop seasons), and (D) CGR of lentil under different nutrient treatments (2018–2019 & 2019–2020 crop seasons). The error bar represents the standard deviation.
Impact of tillage and nutrients on yield of rice and lentil
The yield of rice was significantly affected by different tillage treatments for the entire period of the experiment. In the first year, grain and straw yields were found to be the highest in CTTPR plots, resulting in 4.84 t ha−1 (Table 2) and 6.46 t ha−1, respectively.
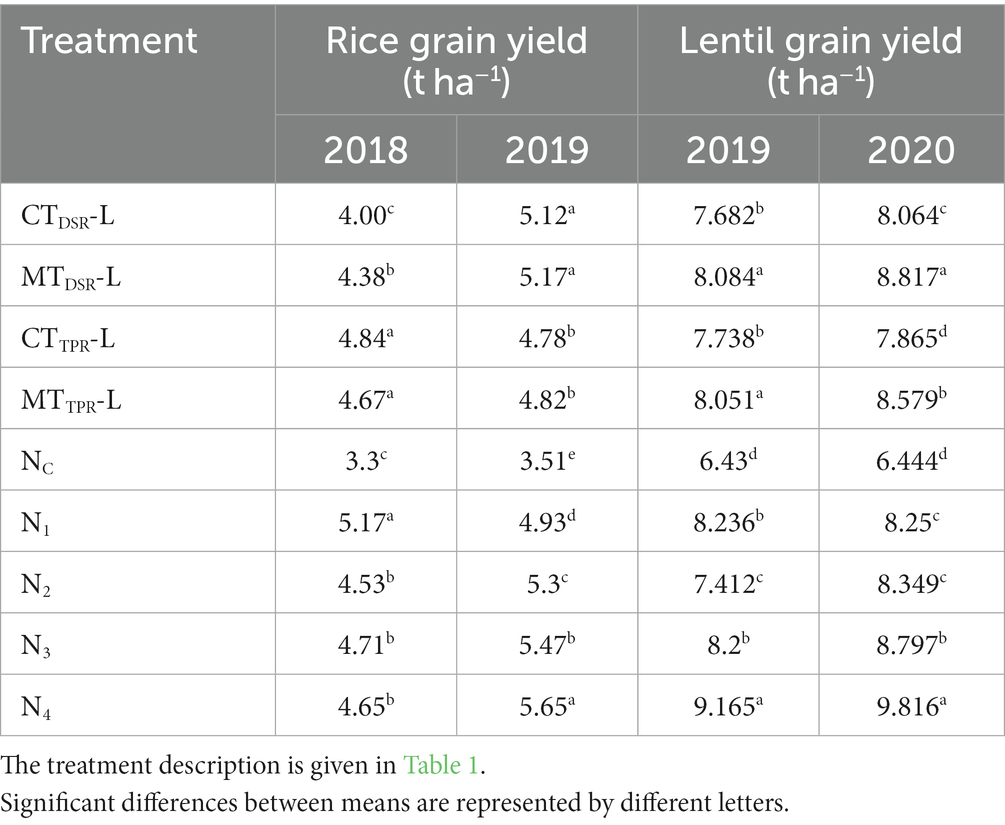
Table 2. Data of 2 years for rice and lentil yield as influenced by different levels of tillage and nutrients.
Rice grown in MTTPR plots recorded 3.64% less grain yield than CTTPR (4.84 t ha−1) plots. In the second year, the trend was found to be different, maximum grain yield and straw yield of 5.17 t ha−1 and 6.62 t ha−1, respectively, were observed in MTDSR plots, which was 8.15 and 5.58% higher than rice yield in CTTPR plots, respectively. Different nutrient schedules had a significant impact on rice yield for the entire period of the experiment. Rice grain (5.17 t ha−1) and straw yield (6.56 t ha−1) were maximum in N1 plots during the first year of the study. But in the second year, N4 treatment recorded the maximum rice grain and straw yield (5.65 t ha−1 and 7.17 t ha−1) respectively, which was 10.13% superior to N1 plots.
Residual lentil grown on MTDSR plots had the highest seed yield of 8.084 q ha−1 (Table 2) and stalk yield of 17.846 q ha−1 during the first year. In the second year, a similar trend was observed, with residual lentil yielding 12.10 and 10.33% higher seed yield (8.817 q ha−1) and stalk yield (18.852 q ha−1) respectively in MTDSR plots than CTTPR plots. Grain (9.165 & 9.816 q ha−1) and stalk yield (20.081 & 20.074 q ha−1) of residual lentil were found to be maximum in treatment of N4 treatment for both the years of study, respectively. N4, N3 and N2 performed better than N1 plots and N0 plots, during the second year which indicated that FYM-treated plots showed an improving trend of yield-attributing characters. The results concurred with the findings of Das et al. (2019). Several researchers found that organic manure is capable of providing a residual impact on two or more crop seasons in terms of nutrient supply (Dey et al., 2019).
Impact of tillage and nutrients on organic carbon and microbial biomass carbon
Different tillage levels and nutrient levels had a significant effect on the organic carbon content of soil, at the end of each year’s rice-lentil cropping system. In, 2019 highest carbon content of 0.5236% (Table 3) in soil was observed in the treatment of MTDSR-L, though it was statistically similar to MTTPR-L treatment.
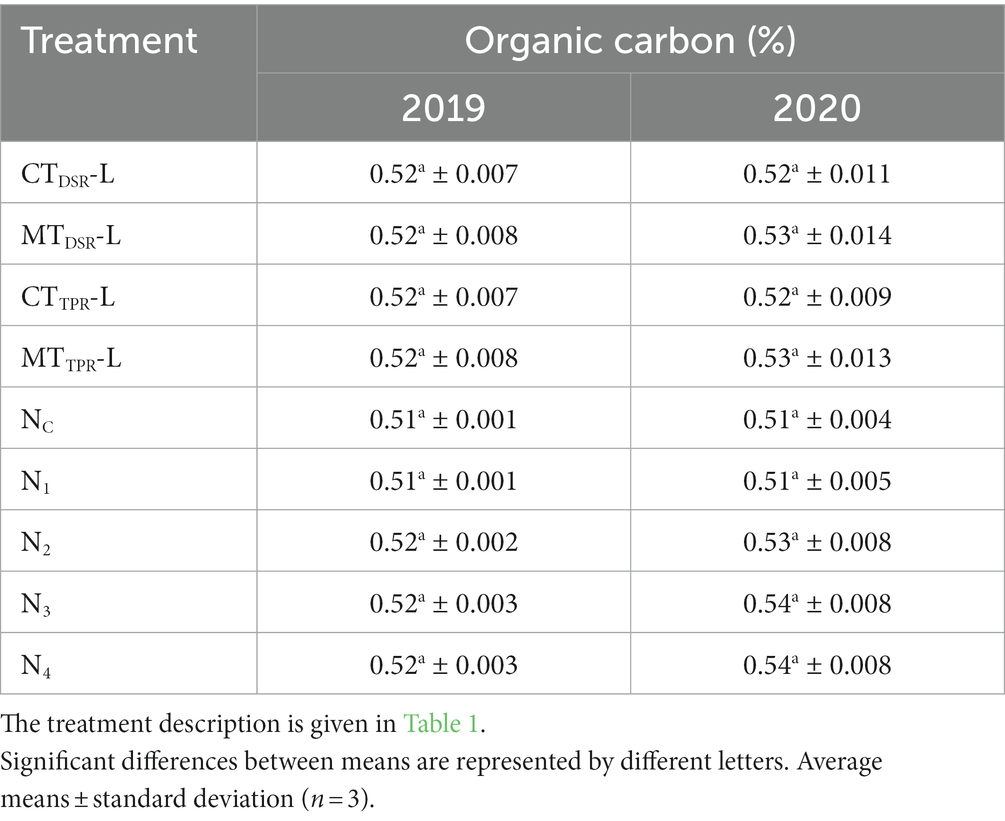
Table 3. Data of 2 years for organic carbon (%) as influenced by different levels of tillage and nutrients.
These were followed by conventionally tilled treatments, recording much lesser carbon values in soil. In 2020, the maximum carbon value in soil was observed with the treatment of MTDSR-L (0.5395%) (Table 3) followed by MTTPR-L treatment. In 2019 and 2020, the highest carbon content of 0.5294 and 0.5422% were observed in N3 which was statistically at par with N4. Though all the FYM treatments have close soil carbon values, but they were pronouncedly superior statistically over treatments of N1 and N0 for both years.
Microbial biomass carbon followed a similar trend as that of organic carbon in soil throughout the experiment. The values improved from the first to the second year. The highest microbial biomass carbon (316.66 μg C g −1 oven dry soil) was found in MTDSR plots (Figure 6A) which were 1.93 and 6.90% more than CTTPR plots, respectively for both the years of study. However, MTTPR plots were found to be statistically at par with MTDSR plots in the first year and were closely following MTDSR plots in the second year.
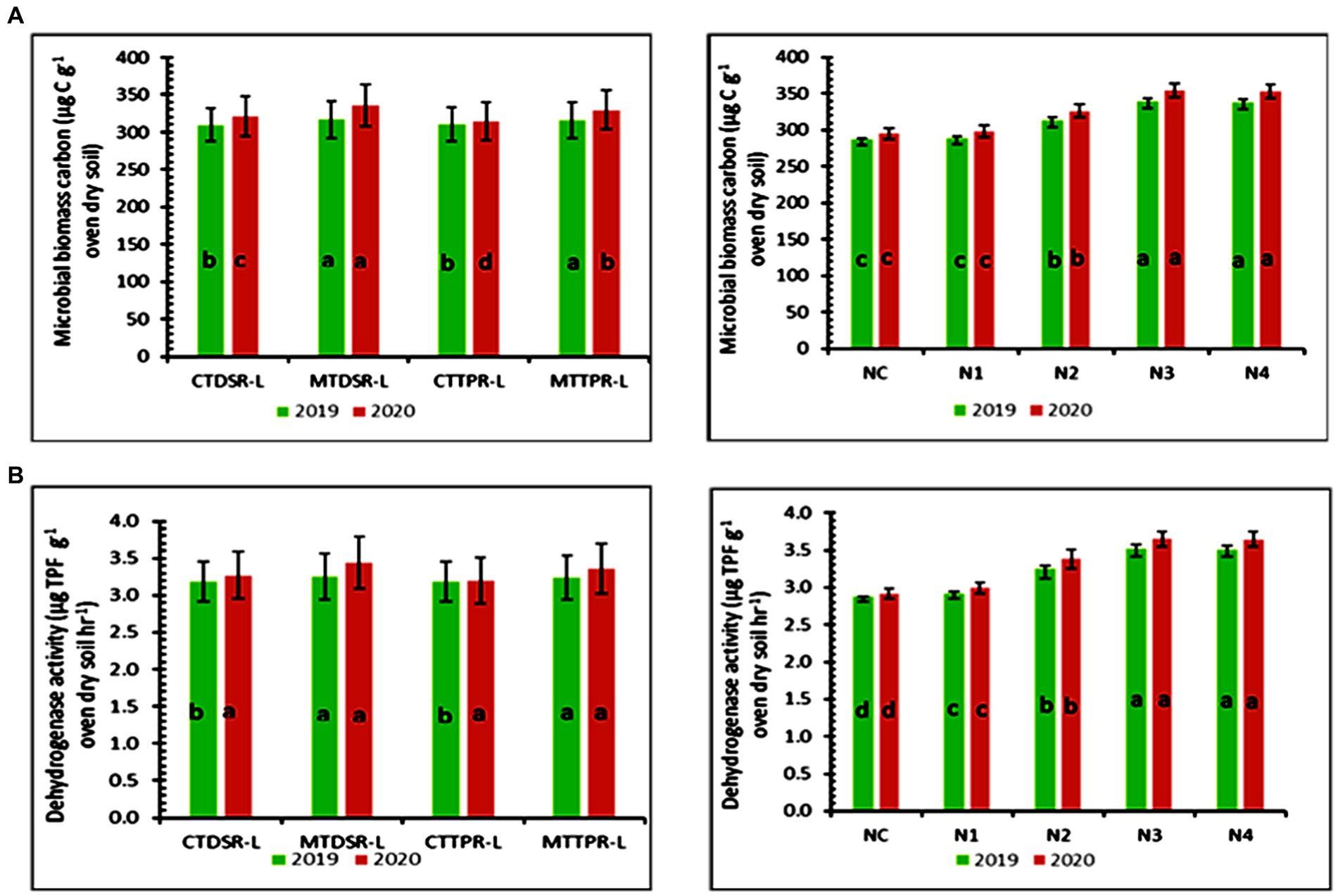
Figure 6. (A) Microbial biomass carbon as affected by different levels of tillage and nutrients in both years (Error bars represent standard deviation). (B) Dehydrogenase activity as affected by different levels of tillage and nutrients in both years. Treatment details are given in Table 1.
At the end of each year’s rice-lentil cropping system, microbial biomass carbon in soil was significantly affected by different nutrient treatments. Maximum soil microbial biomass carbon of 339.07 μg C g−1 oven-dry soil (Figure 6A) and 354.22 μg C g−1 oven-dry soil, respectively were attained by N3 which was statistically at par with N4. All FYM-treated plots recorded more soil microbial biomass carbon than N0 and N1 plots, which was due to the presence of higher soil organic carbon content in all FYM-treated plots.
Impact of tillage and nutrients on dehydrogenase activity
Dehydrogenase activity in soil was significantly affected by different tillage treatments at the end of each year’s rice-lentil cropping system. For the first year of study, MTDSR-L attained the highest value of dehydrogenase activity (3.251 μg TPF g−1 oven dry soil hr.−1) (Figure 6B) which was 2.07% more than CTDSR-L and statistically at par with MTTPR-L. In the second year, MTDSR-L outperformed all other treatments recording dehydrogenase activity of 3.439 μg TPF g−1 oven-dry soil hr.−1 which was 5.16 and 7.46% more than CTDSR-L and CTTPR-L, respectively, and was closely followed by MTTPR-L.
Maximum dehydrogenase activity of 3.521 μg TPF g−1 oven dry soil hr.−1 & 3.649 μg TPF g−1 oven dry soil hr.−1 was obtained in N3 (Figure 6B) respectively in both the years, though it was statistically at par with N4. Dehydrogenase activity was 20.14 and 21.78% higher in N3 compared to N1 in respective years.
Impact of tillage and nutrients on microbial population
Highest bacterial, fungal and actinomycetes population (74.47 × 105 CFU g−1 soil and 87.60 × 105 CFU g−1 soil; 32.47 × 103 CFU g−1 & 39.67 × 103 CFU g−1; 50.67 × 103 CFU g−1 soil and 61 × 103 CFU) (Figures 7A–C) was found in the treatment of MTDSR-L, respectively, for both the years, which was statistically at par with MTTPR-L. The bacterial population was found to be 21.21% higher in MTDSR-L compared to CTTPR-L in the final year of the study (Figure 7A). CTTPR-L recorded 22.27 and 22.81% less total fungal and actinomycetes population compared to MTDSR-L treatment in the last year of the experiment (Figures 7B,C). Results from this study reveal that bacterial, fungal and actinomycetes count increased from the first year to the second year in minimum tillage treatments. Total bacterial, fungal and actinomycetes count was significantly affected by different nutrient treatments, after each year’s rice-lentil cropping system. Maximum bacterial, fungal and actinomycetes population of 78.58 × 10 5 CFU g−1 soil & 88.50 × 10 5 CFU g−1 soil, 35.67 × 103 CFU g−1 & 40.58 × 103 CFU g−1 and 53.42 × 103 CFU g−1 & 62.25 × 103 CFU g−1 were found in N3, respectively, for both the years (Figures 7A–C and SupplementaryTables S1, S2, S3). FYM-treated treatments had higher values which are attributed to higher C input. All the FYM-treated plots had closer values for microbial population, while N1 and N0 had pronouncedly inferior statistical values.
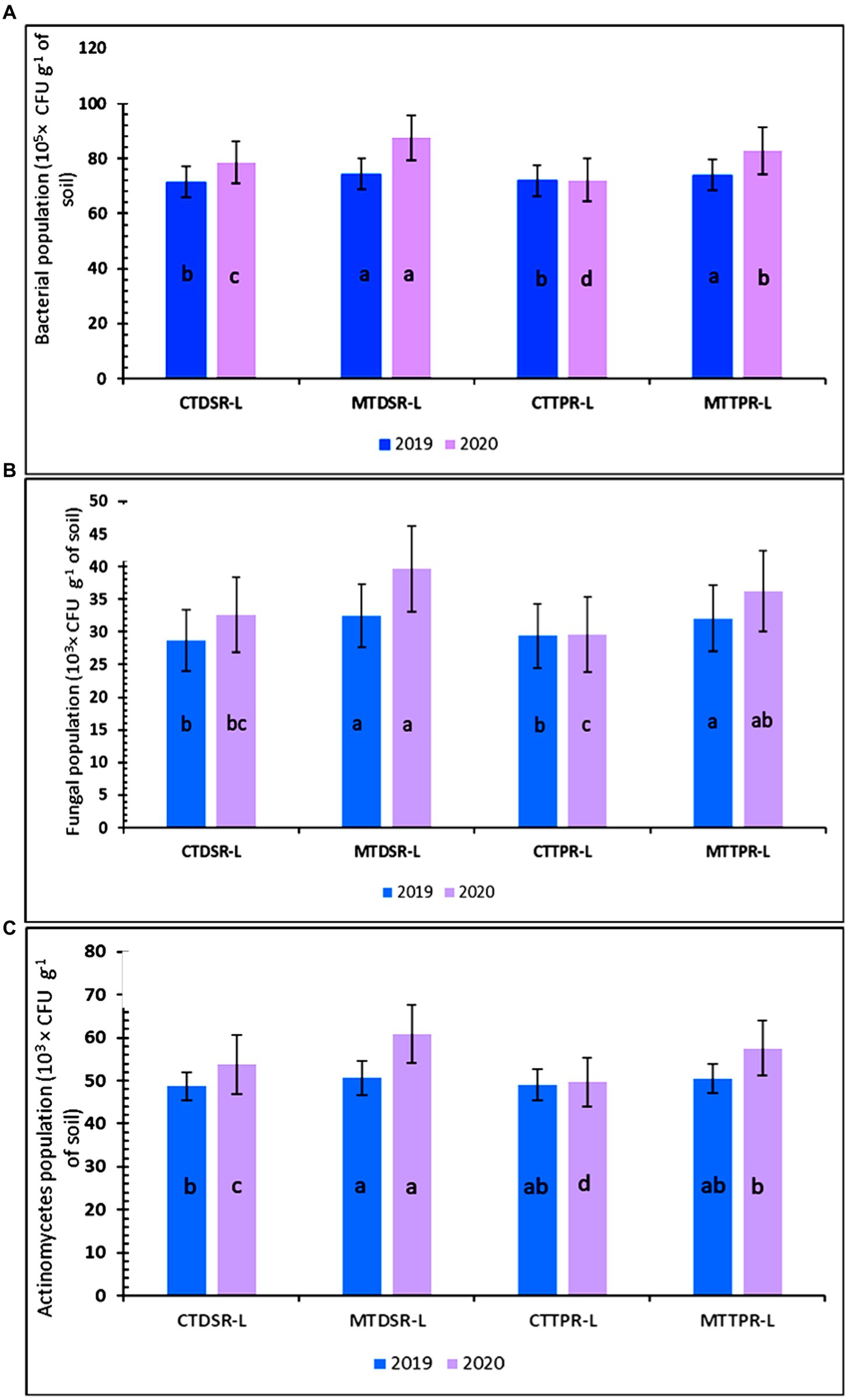
Figure 7. (A) The total bacterial, (B) fungal and (C) actinomycetes population as affected by different levels of tillage in both years (error bars represent standard deviation). Treatment details are given in Table 3.
Relation between different soil microbial parameters with rice and lentil yield
Different correlograms have been constructed according to different tillage practices for 2 years of experiment. In each correlogram, significant positive relationships have been observed between different soil microbial parameters with rice and lentil yield along with LAI values of 60 DAS of rice and lentil (Figure 8).
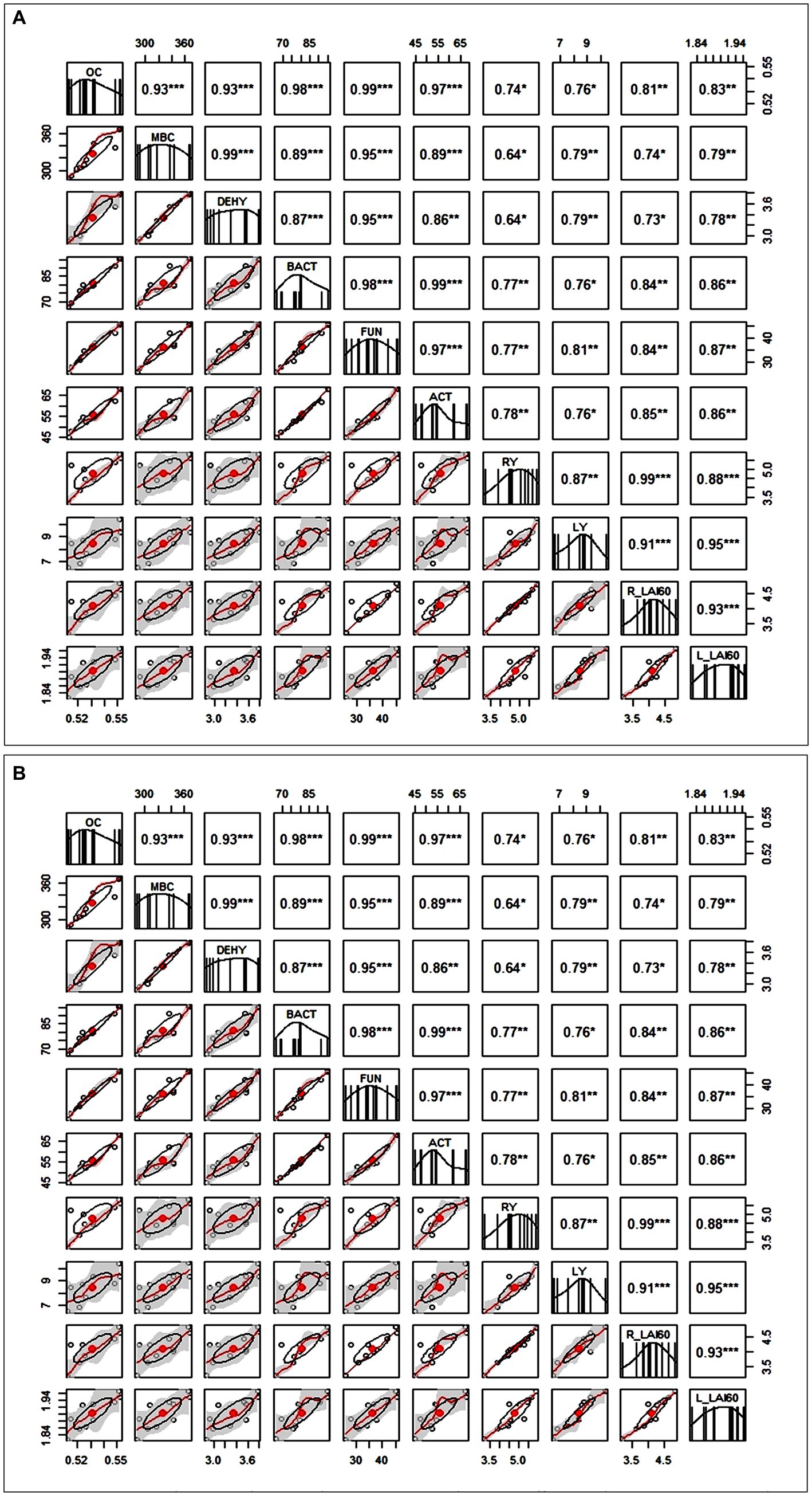
Figure 8. Correlogram showing the Pearson correlation coefficients between organic carbon (%OC), microbial biomass carbon (MBC), dehydrogenase (DEHY), bacterial population (BACT), fungal population (FUN), Actinomycetes population (ACT), rice (RY) & lentil (LY) yields, 60 DAS LAI of rice (R LAI60) and lentil (L LAI60) under (A) CTDSR-L system and (B) MTDSR-L system; *, Correlation coefficient significant at 0.05 level of probability, **, Correlation coefficient significant at 0.01 level of probability and ***, Correlation coefficient significant at 0.001 level of probability.
Rice yield and lentil yield were significantly correlated with organic carbon present in the soil. Under all tillage systems, a strong positive correlation (R > 0.6) was observed between all parameters. In Figure 8A, CTDSR-L system, the correlation was found to be strongest among MBC and DEHY, OC and BACT, OC and ACT, BACT and ACT, RY and RLAI60, LY and L LAI60. It implies that the interdependence of microbial populations is determined by the presence of organic carbon in the soil.
Whereas correlation coefficients for all the parameters with respect to direct seeded rice yield under conventional tillage were significant except for dehydrogenase activity (0.62). The lentil yield and 60 DAS LAI value were found to be significantly positively correlated with the microbial parameters. This indicates that conventional tillage results in lower activity of microbial population which is quite obvious due to the disturbance occurring through the tillage operations. From Figure 8B, depicting the MTDSR-L system, it was clear that the microbial characteristics in the soil were highly correlated with each other and they were found to be highly significant.
In the minimum tillage practiced in direct-seeded rice, the dehydrogenase activity was significantly correlated with the rice (R = 0.64) and lentil yield (R = 0.79) which clearly is the indicator of the microbial oxidation activity. The lentil yield and 60 DAS LAI value were significantly positively correlated with the microbial parameters. The strongest relationship was observed between MBC and DEHY, BACT and OC, FUN and OC, BACT and ACT, and RY and RLAI60. In the CTTPR-L system (Figure 9A) high positive correlation was observed in rice yield with other parameters except for MBC which indicates that the heavy tillage practiced in transplanted rice does not significantly contribute to proper microbial growth which might be the reason for later compaction of soil in this system resulting in lower MBC value. Interestingly in the MTTPR-L system (Figure 9B), rice yield was found to be only correlated with bacterial (0.65*) and fungal population (0.68*) which indicates that the system is not that suitable for soil health. The minimum tillage practiced in the puddled condition might have hindered the oxidation of organic matter leading to poor microbial growth affecting rice yield whereas in the case of lentil yield all the other soil biological parameters were found to be highly interdependent giving rise to better yield performance. LAI values at 60 DAS for both rice and lentil had higher significant coefficient values (R from 0.62 to 0.97) with most of the soil microbial parameters, for all tillage systems.
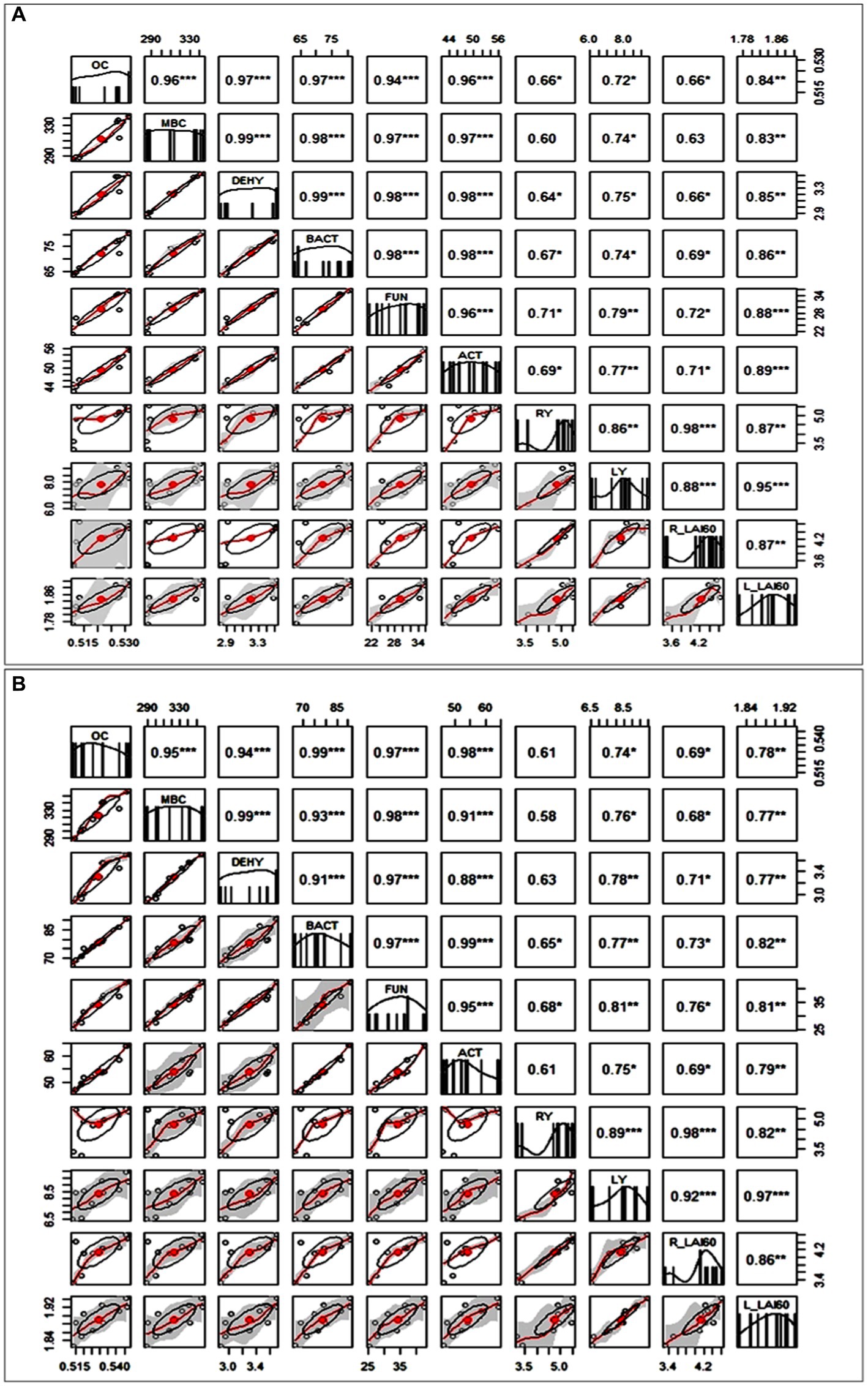
Figure 9. Different correlograms show the Pearson correlation coefficients between organic carbon (% OC), microbial biomass carbon (MBC), dehydrogenase (DEHY), bacterial population (BACT), fungal population (FUN), Actinomycetes population (ACT), rice (RY) & lentil (LY) yields, 60 DAS LAI of rice (R LAI60) and lentil (L LAI60) under (A) CTDSR-L system and (B) MTTPR-L system. *, Correlation coefficient significant at 0.05 level of probability; **, Correlation coefficient significant at 0.01 level of probability and ***, Correlation coefficient significant at 0.001 level of probability.
Relationship between the organic carbon (OC %) in soil with microbial population count under various tillage systems
The relationship between the OC (%) in soil with microbial population count under various tillage systems was deduced in Table 4. The relationship established between the organic carbon of the second year and the microbial population in both years can provide an in-depth idea about the intricate relationship between soil OC with various microbial populations. According to experiment results, soil organic carbon being the key indicator of soil quality, had significant relation with both years of bacteria, fungi and actinomycetes population as a whole, while individual microbial count did not have any significant relation with organic carbon.
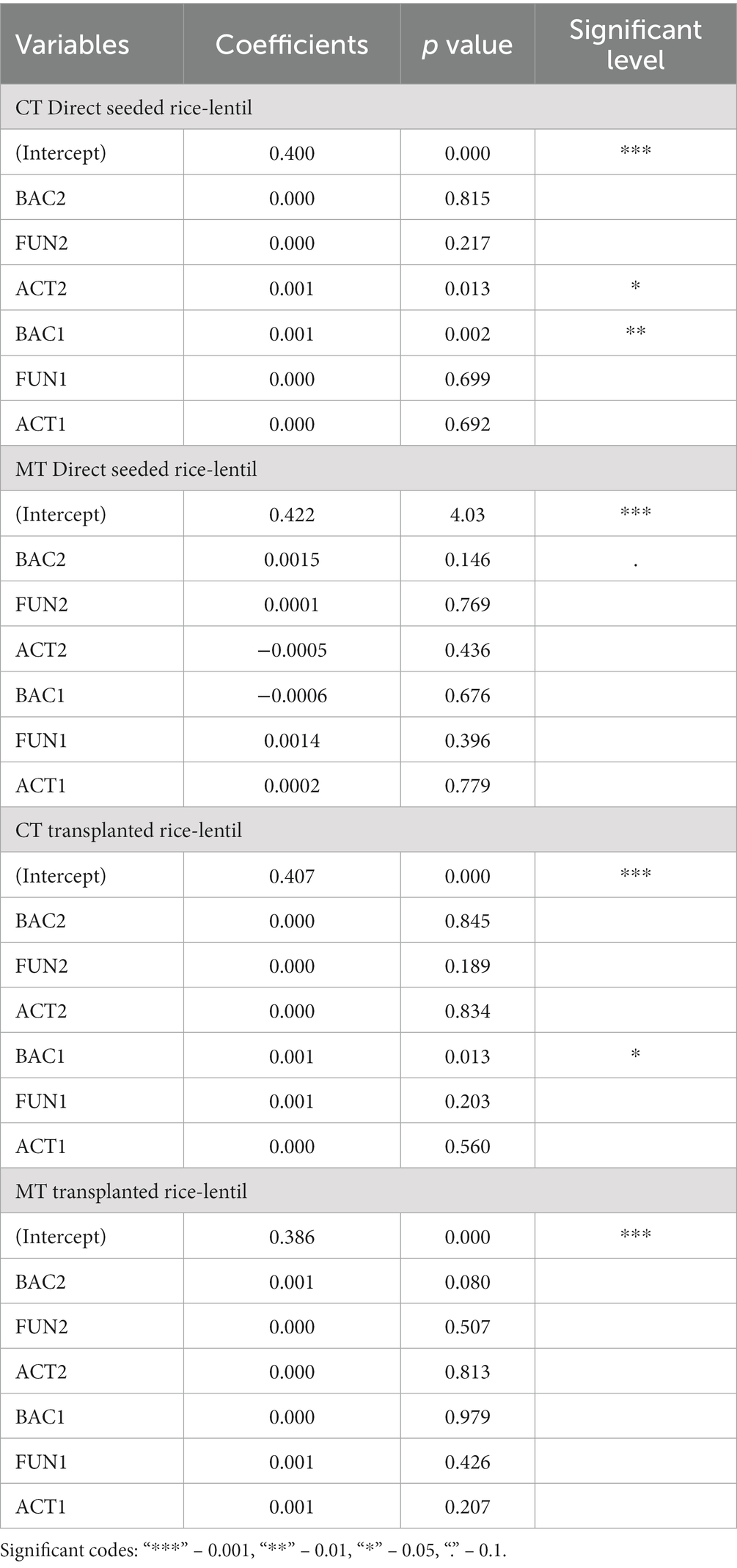
Table 4. Multiple linear regression with organic carbon (OC %) in soil with microbial population count under various tillage system.
The individual relation between microbial count and OC might be more pronounced if the experiment is furthered more which is indicated in the regression table. The microbial population is high in the minimum tillage system but the OC had a relatively significant relation under the conventional tillage system. The organic matter is oxidized faster in conventional tillage systems and thus exhausts the soil capacity for microbial activity in the long run. This may affect the yield in the long term reducing soil productivity and making it barren and unsuitable for rice-based cropping systems.
Discussion
LAI and CGR of rice-lentil as influenced by minimum tillage and integrated nutrient management
Direct-seeded plots did not perform well in the first year because of moisture stress owing to poor rainfall as there was poor germination of seeds, while it was quite a different scenario in the second year. Such a trend might be due to the overall effect of better climatic conditions (Supplementary Figure S1) during the second year and minimum tillage which was gradually getting pronounced with each passing year. Minimum soil disturbance results in the retention of soil organic matter and better soil chemical, physical and biological characteristics. More soil enzymatic activity, and microbial biomass, lead to higher nutrient availability and consequently higher uptake by the crop giving rise to higher leaf area per plant and better canopy cover hence improving the LAI. Higher LAI and better light interception have produced more dry matter causing higher CGR values in the case of minimum tillage. Qamar et al. (2013) in his study found that zero tillage treatment had resulted in higher LAI and CGR values in the case of wheat, which was due to higher moisture and availability of nutrients near the crop root zone that accelerated the growth. Das et al. (2020) concluded that the availability of nutrients was more in conservation tillage treatments compared to conventional tillage treatments. Zero tillage outperformed conventional tillage in respect of various growth attributes of crops as observed by Parihar et al. (2016), which he assigned to better soil water regimes, aeration and better root growth resulting from lesser compaction of soil. Azospirillum sp. is said to promote the secretion of amino acids, cytokinins, gibberellins, indole acetic acid etc. which directly facilitates better root growth in plants, increasing nutrient as well as water uptake by plants (Zeffa et al., 2019). Hafeez et al. (2013) reported that zinc activates numerous plant enzymes for carbohydrate metabolism. This might be a valid reason for N4 treatment to record the highest LAI and CGR values. Treatments receiving FYM showed better results in terms of LAI and CGR in the second year since it has a longer turnover period as it decomposes slowly due to its high C:N ratio to release nutrients. Imade et al. (2017) reported the highest LAI values in rice along with other growth attributes with the application of FYM. Singh et al. (2018) got similar results due to the application of FYM which improved growth attributes of rice due to lowering of nitrogen loss because of fixation of NH4+ ions in humus present in FYM and its slow release over time. Aerobic soil condition of the direct seeded rice establishment systems was responsible for better soil structure leading to better root growing conditions, which might result in higher nutrient uptake for lentil grown afterwards. Higher soil organic carbon content under minimum tillage plots due to the slow decomposition of organic residues on the soil surface as well as higher soil moisture conditions in minimally tilled plots might be a valid reason for residual lentil to have higher LAI and CGR values. The positive influence of minimum tillage practiced during the rice cultivation in improving growth attributes of the crop was quite visible in lentil, with minimally tilled plots recording comparatively more LAI and CGR than conventionally tilled plots. Bandyopadhyay et al. (2018) in his study observed higher LAI and CGR values in lentil in no-tillage and minimum tillage treatments which were due to better moisture content in the root zone which further led to higher relative water content and chlorophyll concentration. Higher relative water content is an important index for cell division whereas chlorophyll concentration determines the photosynthetic capacity. Both of these factors are important for the growth indices of any crop. Results tend to comply with the findings of Singh et al. (2016). This along with the application of farmyard manure might have resulted in better physiological characteristics of lentil which in turn improved LAI and CGR values.
The yield of rice-lentil as influenced by minimum tillage and integrated nutrient management
Various previous reports confirmed that during dry season or cases of low rainfall puddling has aided in enhancing the rice yield in general, owing to lowering of percolation loss of water and taming weeds which is due to less rainfall and deep water table (Yadav et al., 2017). This might be the reason behind the higher yield of transplanted rice in the first year, in both conventional and minimum tillage systems. While better climatic conditions favoring higher LAI and CGR values resulted in higher yield in the case of direct-seeded rice in the second year. Many researchers before have confirmed proper agronomic management like weed and water management and favorable climatic conditions have resulted in direct-seeded rice performing better than transplanted rice (Tao et al., 2006). Liu et al. (2015) in his study pertained higher yield of direct seeded rice because of more number of panicles per m2 than transplanted rice. Košutić et al. (2005) and Salahin et al. (2017) reported that minimum tillage with time improves the yield of crops. Less disturbance of soil in conservation practices like minimum tillage which leaves at least 30% residues on soil, stimulates the microbial biomass and increases its metabolic rate causing higher nutrient transformation of carbon, nitrogen, and phosphorus (Hungria et al., 2009). Nutrient availability is closely linked with robust dry matter accumulation, resulting in higher yield in the case of MTDSR-L. N4 recorded the highest yield due to release of phytohormones and nitrogen fixation by Azospirillum sp. along with the beneficial effect of zinc sulphate on nitrogen metabolism which might have led to maximum yield. Fukami et al. (2018) found out Azospirillum has a direct effect on root growth, resulting in higher nutrient and moisture uptake by plants. It also solubilizes phosphorus which further improves the yield attributes of crops. Integrated application of organic, and inorganic sources of nutrients along with biofertilizers improves soil organic carbon and soil structure (Das et al., 2015). Singh et al. (2009) reported similar instances of higher rice grain yield on the combined application of chemical fertilizers and Azospirillum owing to higher tillers m−2, panicles m−2 and test weight. Integrated application of chemical fertilizers, organic manures and bioinoculants of Azospirillum was found to give 7.20% more rice grain yield than treatments not receiving Azospirillum, as it secreted growth-promoting substance which thereby gave rise to better root structure, enhancing mineral absorption by plants (Sravan and Singh, 2019). Minimum tillage and direct seeded rice establishment result in better seedbed than conventionally tilled transplanted systems because of a reduction in soil crusting and higher infiltration of water (Gangwar et al., 2006). Bandyopadhyay et al. (2018) pointed out that crop residue in minimum tillage plots lowered the solar radiation reaching the soil surface hence lowering energy for evaporation of residual soil moisture, after the rice harvest. Several researchers confirmed that no or minimum tillage lowers the change in soil moisture storage. Any kind of organic residue in soil improves the water-stable aggregate build-up because of polysaccharides and other organic compounds released after its decomposition, that act as a cementing material for soil particles. This enhanced aggregation of soil particles results in the development of low-density materials that are rich in mineral fractions (Bhanwaria et al., 2022). Mineralization of nutrients highly varies according to soil type, moisture, temperature and microbial activities of soil etc., (Moorhead et al., 1996). Higher moisture content, proper nutrient cycling and better biological parameters of minimum tillage system might have resulted in a greater number of pods per plant, seeds per pod and test weight of lentil which in turn led to its better seed yield as compared to conventional tillage system. Das et al. (2019) found similar results in a two-year experiment when lentil grown under no-tillage conditions in minimally tilled rice plots after its harvest recorded the maximum number of seeds per pod (1.29 & 1.37) and the number of pods per plant (22.7 & 23.1) respectively for both the years of study. Robust plant growth, in minimally tilled plots led to better biomass accumulation which was the reason for better stalk yield. Farmyard manure increases available nutrients such as nitrogen along with other micronutrients which further favors phosphorus and potassium use efficiency that results in better root structure, reduced leaching and higher nutrient uptake capacity of the crop (Zhang et al., 2016). The beneficial carryover effect of Azospirillum, FYM and zinc sulphate was clearly observed in the performance N4 treatment, where Azospirillum acted as plant growth promoting rhizobacteria alongside slow nutrient-releasing bulky organic manure of FYM and zinc sulphate which perhaps facilitated nitrogen uptake by lentil, leading to better seed yield in the process. Application of organic manure improves the formation of stable metal–organic complexes, in the case of applied and natural micro-nutrient (Zn, Fe and Mn) in soil, which reduces their adsorption or precipitation in soil and enhances their uptake in crops (Swarup, 1984). This might be another reason for the N4 treatment to perform better than the rest.
Organic carbon and microbial biomass carbon
Plant litter, root additions, and external application of organic manures improve the organic matter in soil, which transforms into sources of humus carbon. It has been found that minimum tillage and zero tillage have considerably improved soil microbial biodiversity, their activity and biomass, since crop stubbles left on the surface act as their food source (Lupwayi et al., 2001) There was a circumspect improvement in carbon values from the first year to the second year. Minimally tilled plots had higher organic carbon content in soil than conventionally tilled plots throughout the entire experiment mainly because of limited manipulation of soil; oxidation of organic matter was reduced, hence conserving carbon within the soil. Various researchers (Beare et al., 1994; Six et al., 2000), stated that the presence of crop residues, in case of conservation tillage practices like minimum tillage, no-tillage etc., hinders the exposure of soil macroaggregates for microbial decomposition by increasing turnover rate of such soil macroaggregates by physically lowering the soil to residue contact, conserving soil organic carbon It takes several years for the organic carbon to increase to a substantial amount. Results from this experiment support this finding. After 11 years of experiment, Campbell et al. (1999), found that soil organic carbon improved to 0–3 Mg ha−1 under zero tillage within 0–15 cm. The introduction of legumes in the cropping system along with conservation tillage is another reason for the increase in the carbon values of soil (Yadav et al., 2017). Favorable crop rotations are associated with higher soil organic content as, root additions in conservation tillage systems, have a close relationship with the organic carbon stock of soil (dos Santos et al., 2011; Page et al., 2020). Khorami et al. (2018), from an experiment of 2 years, reported that soil organic carbon was highest in the case of reduced tillage (34%) followed by no-tillage (13%) as compared to conventional tillage Though some literatures suggest that leaving crop residues in soil or conservation tillage practices does not necessarily improve the soil organic content unless there is favorable soil temperature and humidity to act upon the decomposition rate of soil organic matter. Combined application of Azospirillum and farmyard manure accelerated robust root growth leading to higher root additions in soil, which might be a valid reason for N3 treatment to record the highest organic carbon values with respect to other nutrient treatments by the end of second year of experiment. The results complied with findings of Zhao et al. (2020) where after 5 years of experiment organic carbon increased by 41.15% due to combined application of compost and inorganic sources of nutrients which was five times more than the increase because of the application of only inorganic fertilizers (Zhao et al., 2020). Again long-term experiments at various locations in India on the combined application of 50% NPK and 50% N through FYM showed similar results (Nayak et al., 2012). Different cereal-legume cropping systems were evaluated with respect to integrated nutrient management, Venkatesh et al. (2013) found that soil organic carbon content significantly increased by 26% in the treatment of farmyard manures + biofertilizers when compared to control treatments. Conventionally tilled treatments recorded lesser microbial biomass carbon as it is highly affected by soil management practices, which alters soil microbial habitat and microclimate. It is used as an important index of soil health and quality, which tends to increase in agricultural practices that maximize organic carbon content in soil (Malobane et al., 2020). Results from this experiment reveal that minimum tillage practices leave behind crop residues on the soil surface which acts as the energy source for various soil microorganisms, leading to greater soil microbial biomass carbon. Soil aggregate formation is always found to be higher in conservation tillage practices (Fuentes et al., 2009; Poonam et al., 2017; Upadhyay et al., 2018). The pore space within these aggregates acts as an essential habitat for soil microbial biomass, which is disrupted by conventional tillage practices. The results of this experiment are in accordance with Salinas-Garcıa et al. (2002) who concluded that in the upper 0–5 cm layer of soil, microbial biomass carbon was 25 to 50% higher in ZT and MT treatments than that CT treatments due to the presence of organic substrates in the case of both ZT and MT, which led to the assimilation of nutrients that further improved microbial biomass and their activity. Various researchers have found higher soil microbial biomass carbon in conservation tillage systems than in conventional systems (Hungria et al., 2009; Das et al., 2020). The addition of organic sources of nutrients, increased the carbon content of the soil for both the years of the experiment, while control plots and treatment receiving only chemical sources of nutrients did not have a higher carbon content in the soil. Various literature suggests (Vineela et al., 2008; Venkatesh et al., 2013; Francioli et al., 2016; Kumar et al., 2019) that integrated application of nutrients improves soil microbial biomass carbon. From a five-year experiment in the maize-mustard cropping system, continuous application of inorganic fertilizers along with FYM, biofertilizer and lime had the highest microbial biomass carbon (373.02 mg kg−1) (Saha et al., 2010). Venkatesh et al. (2013) detected that the inclusion of pulses into any cropping system improves soil health through biological nitrogen fixation and their deep root system, hence increasing the below soil biomass. The results from this experiment confirm that the application of plant growth-promoting rhizobacteria like Azospirillum along with FYM resulted in higher root biomass and its exudates, providing carbon and energy for higher microbial proliferation in the soil, resulting in higher microbial biomass carbon.
Dehydrogenase activity
Minimally tilled systems recorded higher dehydrogenase values compared to conventionally tilled systems. Soil dehydrogenase enzymes are said to be one of the important enzymes taking part in biochemical routes to maintain balanced biogeochemical cycles. It’s used as an important index for microbial activity in soil. Previous literature suggested that the more organic matter in the soil more will be more dehydrogenase activity which actually oxidizes the organic matter to release nutrients (Madejón et al., 2009; Das et al., 2020). Under permanent bed treatments dehydrogenase activity was 62% higher than conventionally tilled treatments in long-term maize-wheat cropping systems (Singh et al., 2009). Intensive tillage destroys soil aggregation and accelerates organic matter decomposition. Soil enzymatic activities are highly related to microbial biomass which is found higher in conservation tillage systems (Madejón et al., 2009). Minimally tilled plots had higher organic content that acted as an energy source for soil microbial multiplication which led to higher dehydrogenase activity. Higher dehydrogenase activity was confirmed under various conservation tillage systems like zero tillage, reduced tillage, etc., by other researchers (Nisha et al., 2016; Singh et al., 2016; Kumar et al., 2017; Hatti et al., 2018). Dehydrogenase activity (6.53 μg g−1) at 0-15 cm soil layer was found to be more in CsT treatments than CT treatments as CsT methods improved the soil microbial activity and enzyme production under crop residue cover in the rice-rapeseed system (Das et al., 2020). Higher organic carbon content and microbial biomass carbon in FYM-applied treatments resulted in higher dehydrogenase activity Parewa et al. (2014) observed that in treatment where FYM along with bio inoculants were added accelerated the dehydrogenase activity due to the breakdown of organic substances present in the manure. The highest dehydrogenase activity was recorded by Brar et al. (2017) in their long-term experiment on maize when FYM, non-edible oilcake and biofertilizers were applied.
Microbial population
Soil enzymes are positively correlated with soil health and microbial activity (Dick, 1994). Results complied with Li et al. (2020), who suggested that conservation tillage practices have impacts on the total bacterial population. Higher dehydrogenase activity recorded in MTDSR-L can be an indicator of a higher bacterial population (Singh et al., 2009). Higher total and bioavailable carbon content enhance soil bacterial count (Navarro-Noya et al., 2013). Several other researchers have found a higher bacterial population under zero tillage and minimum tillage practices (Helgason et al., 2010). Diversified rotations of crops are another vital reason for the improvement in bacterial count in MT systems (Yang et al., 2013). During biological nitrogen fixation in legumes, hydrogen gas is produced in the soil rhizosphere which triggers the bacterial population of the soil (Gogoi et al., 2018). Fungal communities are found to similarly proliferate more in conservation tillage systems viz. minimum tillage, zero tillage etc. because of maintaining hyphal nets which otherwise get disturbed due to continuous tillage operations in CT (Hungria et al., 2009). Its abundance at the soil surface in conservation tillage systems has been reported by several researchers before (Zhang et al., 2012; Spurgeon et al., 2013). The fungal population increased in both soybean and wheat under zero tillage systems Singh et al. (2018). Nisha et al. (2016) found similar kind of results in his 3 years experiment where they reported 56.63% more fungal population in the rice-wheat-mungbean cropping system under zero tillage than that of CT. The results from this study conclude that the accumulation of organic residues in minimum tillage systems acted as carbon and nitrogen sources for higher fungal and bacterial proliferation and these were in support of the findings of Parewa et al. (2014), where they concluded treatment of 100% NPK + FYM+ plant growth promoting rhizobacteria + vesicular-arbuscular mycorrhiza increased fungal as well as bacterial population due to higher root exudates acting as a source of carbon and energy. The results were similar to Brar et al. (2017) who reported the highest actinomycetes population (76.17 × 104 cfu g−1 soil) in treatment receiving 50% N as FYM + Biofertilizers. Conventionally tilled plots throughout the entire study recorded a lesser total actinomycetes population when compared to minimum tillage plots. From the results, it is evident that cropping intensification as well as minimum tillage creates crop residues and exudates causing micro-habitats for diversified microbial communities. In a paddy-maize-soybean cropping system grown under conservation tillage actinomycetes population increased by 8.51, 2.3 and 3.4% at the harvest stage of rice, maize, and soybean, respectively, when compared to conventional tillage systems (Dongre et al., 2017). The results were consistent with the findings of Hatti et al. (2018) and Nisha et al. (2016) who reported a higher actinomycetes population under zero and minimum tillage systems. Application of organic sources of nutrients along with biofertilizers and chemical sources of nutrients have been said to promote soil microbial communities, which significantly influences soil biological fertility ensuring better nutrient cycling and nutrient dynamics in the soil in the longer run (Suhaibani et al., 2020).
Relation between different soil microbial parameters with rice and lentil yield
It is quite evident from this study that a favorable soil environment provided by minimum tillage is responsible for organic matter accumulation and higher enzymatic activity indicating microbial multiplication, which clearly has a significant beneficial effect on the yield of successive crops. Higher correlation values between various soil biological parameters with a respective yield of rice and lentil were observed among minimum tillage systems after 2 years of experiment implying limited tillage operations resulted in higher microbial activity and higher decomposition of organic matter leading to more availability of nutrients which might be taken up by plants (Somenahally et al., 2018) giving rise to higher leaf area which is successively contributing to higher accumulation of photosynthates and better yield in case of both rice and lentil. Soil health and fertility improve over time under minimum tillage along with the inclusion of pulse crops which is a valid reason behind the yield increase (Kaye and Quemada, 2017).
Conclusion
The findings of the study show that though minimum tillage did not have any effect on the growth and yield of rice in the first year, it had a synergistic effect on rice in the following year. In the second year of the experiment, all FYM-treated plots outperformed treatments without FYM. The residual effects of tillage and nutrients on lentil planted after rice were highly significant. Minimum tillage with integrated nutrient combinations resulted in higher LAI and CGR values contributing to higher crop yield. Though minimum tillage did not have a substantial effect on organic carbon but had influenced the microbial biomass carbon, dehydrogenase activity and microbial populations which are indicators of soil health. Organic carbon was found to be closely related to all the soil biological parameters, especially in the case of minimum tillage systems. The improvement of soil health clearly increased crop yields due to higher nutrient availability compared to conventional tillage systems where the organic matter was oxidized. It is clear that to gain long-term stability and sustainability of agro-ecosystem this kind of experiment needs to be furthered. However, to reverse soil degradation caused by conventional tillage practices and mono-cropping farmers need to have locally adjusted conservation tillage practices along with the inclusion of pulse crops in the cropping system. Farmers can get profitable yield in the long run under the rice-lentil cropping system if the minimum tillage is practiced along with the addition of FYM, Azospirillum and zinc sulphate.
Data availability statement
The original contributions presented in the study are included in the article/Supplementary material, further inquiries can be directed to the corresponding authors.
Author contributions
UB, MN, VV, SS, and PB led the research work, planned, supervised, conducted field experiments, read, and edited the manuscript. UB, MN, and SS collected soil/plant samples and performed chemical analysis, also wrote the initial draft of the manuscript, prepared Figures, and Tables. PB, AG, AA, AH, and SM, project supervision, reviewed, read, and edited the manuscript with significant contributions. AG, AA, AH, and SM, performed the statistical analysis. All authors contributed to the article and approved the submitted version.
Funding
This research was funded by the Department of Agronomy, Bidhan Chandra Krishi Viswavidyalaya, Haringhata, West Bengal, India and partially funded by Princess Nourah bint Abdulrahman University Researchers Supporting Project number (PNURSP2023R65), Princess Nourah bint Abdulrahman University, Riyadh, Saudi Arabia.
Acknowledgments
The authors of this study are very much thankful to the Department of Agronomy, Bidhan Chandra Krishi Viswavidyalaya, Haringhata, West Bengal, India and Princess Nourah bint Abdulrahman University Researchers Supporting Project number (PNURSP2023R65), Princess Nourah bint Abdulrahman University, Riyadh, Saudi Arabia for supporting the current research.
Conflict of interest
The authors declare that the research was conducted in the absence of any commercial or financial relationships that could be construed as a potential conflict of interest.
Publisher’s note
All claims expressed in this article are solely those of the authors and do not necessarily represent those of their affiliated organizations, or those of the publisher, the editors and the reviewers. Any product that may be evaluated in this article, or claim that may be made by its manufacturer, is not guaranteed or endorsed by the publisher.
Supplementary material
The Supplementary material for this article can be found online at: https://www.frontiersin.org/articles/10.3389/fsufs.2023.1225986/full#supplementary-material
References
Ahmed, N., Sinha, D. K., and Singh, K. M. (2018). Economic analysis of production and instability of lentil in major lentil growing states of India. Int. J. Pur. App. Biosci. 6, 593–598. doi: 10.18782/2320-7051.6213
Bandyopadhyay, P. K., Singh, C. K., and Nandi, R. (2018). Response of lentil (Lensculinaries) to post-rice residual soil moisture under contrasting tillage practices. Agric. Res. 7, 463–479. doi: 10.1007/s40003-018-0337-3
Beare, M. H., Hendrix, P. F., Cabrera, M. L., and Coleman, D. C. (1994). Aggregate-protected and unprotected organic matter pools in conventional-and no-tillage soils. Soil Sci. Soc. Am. J. 58, 787–795. doi: 10.2136/sssaj1994.03615995005800030021x
Bhanwaria, R., Singh, B., and Musarella, C. M. (2022). Effect of organic manure and moisture regimes on soil physiochemical properties, microbial biomass C(mic):N(mic):P(mic) turnover and yield of mustard grains in arid climate. Plan. Theory 11:722. doi: 10.3390/plants11060722
Bisht, N., and Chauhan, P. S. (2020). “Excessive and disproportionate use of chemicals cause soil contamination and nutritional stress” in Soil Contamination-Threats and Sustainable solutions. eds. M. L. Larramendy and S. Soloneski (London: IntechOpen).
Brar, A., Gosal, S. K., and Walia, S. S. (2017). Effect of biofertilizer and farmyard manure on microbial dynamics and soil health in maize (Zea Mays L.) rhizosphere. Chem. Sci. Rev. Lett. 6, 1524–1529.
Campbell, C. A., Biederbeck, V. O., McConkey, B. G., Curtin, D., and Zenter, R. P. (1999). Soil quality-effect of tillage and fallow frequency. Soil Biol. Biochem. 31, 1–7. doi: 10.1016/S0038-0717(97)00212-5
Das, A., Layek, J., Idapuganti, R. G., Basavaraj, S., Lal, R., Rangappa, K., et al. (2020). Conservation tillage and residue management improves soil properties under a upland rice–rapeseed system in the subtropical eastern Himalayas. Land Degrad. Dev. 31, 1775–1791. doi: 10.1002/ldr.3568
Das, A., Layek, J., Ramkrushna, G. I., Rangappa, K., Lal, R., Ghosh, P. K., et al. (2019). Effects of tillage and rice residue management practices on lentil root architecture, productivity and soil properties in India’s lower Himalayas. Soil Tillage Res. 194:104313. doi: 10.1016/j.still.2019.104313
Das, A., Ramkrushna, G. I., Yadav, G. S., Layek, J., Debnath, C., Choudhury, B. U., et al. (2015). Capturing traditional practices of rice based farming systems and identifying interventions for resource conservation and food security in Tripura, India. Appl. Ecol. Environ. Sci 3, 100–107. doi: 10.12691/aees-3-4-2
Dey, A., Srivastava, P. C., Pachauri, S. P., and Shukla, A. K. (2019). Time-dependent release of some plant nutrients from different organic amendments in a laboratory study. Int. J. Recycl. Org. Waste Agricult. 8, 173–188. doi: 10.1007/s40093-019-0287-1
Dick, R. P. (1994). “Soil enzymatic activities as indicators of soil quality” in Defining Soil Quality for a Sustainable Environment. eds. J. W. Doran, D. C. Coleman, D. F. Bezdicek, and B. A. Stewart (Hoboken, NJ: Wiley-Blackwell), 107–124.
Directorate of Economics and Statistics (2021). Agricultural statistics at a glance 2021. Government of India Ministry of Agriculture and farmers welfare Department of Agriculture, cooperation and farmers welfare Directorate of Economics and Statistics. Available at: http://~eands.dacnet.nic.in
Dongre, K., Sachidanand, B., and Porte, S. S. (2017). Assessment of different microbial population in the rhizosphere of Main kharif crop under conventional and conservation agriculture system. Int. J. Curr. Microbiol. App.Sci 6, 813–819. doi: 10.20546/ijcmas.2017.609.100
dos Santos, N. Z., Dieckow, J., Bayer, C., Molin, R., Favaretto, N., Pauletti, V., et al. (2011). Forages, cover crops and related shoot and root additions in notill rotations to C sequestration in a subtropical Ferralsol. Soil Till. Res. 111, 208–218. doi: 10.1016/j.still.2010.10.006
FAO. (2019). The State of food And Agriculture. Moving Forward on Food Loss and Waste Reduction. Food and agriculture Organization of United Nations. Rome. FAO.
Francioli, D., Schulz, E., Lentendu, G., Wubet, T., Buscot, F., and Reitz, T. (2016). Mineral vs organic amendments: microbial community structure, activity and abundance of agriculturally relevant microbes are driven by long-term fertilization strategies. Front. Microbiol. 7:1446. doi: 10.3389/fmicb.2016.0144
Fuentes, M., Govaerts, B., De León, F., Hidalgo, C., Dendooven, L., Sayre, K. D., et al. (2009). Fourteen years of applying zero and conventional tillage, crop rotation and residue management systems and its effect on physical and chemical soil quality. Eur. J. Agron. 30, 228–237. doi: 10.1016/j.eja.2008.10.005
Fukami, J., Cerezini, P., and Hungria, M. (2018). Azospirillum: benefits that go far beyond biological nitrogen fixation. Amb. Express 8: 73. doi: 10.1186/s13568-018-0608-1
Gangwar, K. S., Singh, K. K., Sharma, S. K., and Tomar, O. K. (2006). Alternative tillage and crop residue management in wheat after rice in sandy loam soils of Indo-Gangatic plains. Soil Tillage Res. 88, 242–252. doi: 10.1016/j.still.2005.06.015
Gogoi, N., Baruah, K. K., and Meena, R. S. (2018). Grain legumes: impact on soil health and agroecosystem. R. Meena, A. Das, G. Yadav, and R. Lal (eds) Legumes for Soil Health and Sustainable Management. Springer, Singapore.
Hafeez, B. M. K. Y., Khanif, Y. M., and Saleem, M. (2013). Role of zinc in plant nutrition-a review. Am. J. Exp. Agric. 3, 374–391. doi: 10.9734/AJEA/2013/2746
Hatti, V., Ramachandrappa, B. K., Sathishand, A., and Thimmegowda, M. N. (2018). Soil properties and productivity of rainfed finger millet under conservation tillage and nutrient management in Eastern dry zone of Karnataka. J. Environ. Biol. 39, 612–624. doi: 10.22438/jeb/39/5/MRN-724
Helgason, B. L., Walley, F. L., and Germida, J. J. (2010). Long-term no-till management affects microbial biomass but not community composition in Canadian prairie agroecosystems. Soil Biol. Biochem. 42, 2192–2202. doi: 10.1016/j.soilbio.2010.08.015
Hungria, M., Franchini, J. C., Junior, O. B., Kaschuk, G., and Souza, R. A. (2009). Soil microbial activity and crop sustainability in a long-term experiment with three soil-tillage and two crop-rotation systems. Appl. Soil Eco. 42, 288–296. doi: 10.1016/j.apsoil.2009.05.005
Imade, S. R., Thanki, J. D., Phajage, S. K., and Nandapure, S. P. (2017). Effect of integrated nutrient management on growth, yield and quality of rice. Bull. Env. Pharmacol. Life Sci. 6, 352–355.
Jenkinson, D. S. (1966). Studies on the decomposition of plant material in soil II-partial sterilization of soil and the soil biomass. J. Soil Sci. 17, 280–302. doi: 10.1111/j.1365-2389.1966.tb01474.x
Jensen, E. S., Peoples, M. B., Boddey, R. M., Gresshoff, P. M., Hauggaard-Nielsen, H., Alves, B. J. R., et al. (2012). Legumes for mitigation of climate change and the provision of feedstock for biofuels and biorefineries-a review. Agron. Sustain. Dev. 32, 329–364. doi: 10.1007/s13593-011-0056-7
Kaye, J. P., and Quemada, M. (2017). Using cover crops to mitigate and adapt to climate change. A review. Agron. Sustain. Dev. 37:4. doi: 10.1007/s13593-016-0410-x
Khorami, S. S., Kazemeini, S. A., Afzalinia, A., and Gathala, M. K. (2018). Changes in soil properties and productivity under different tillage practices and wheat genotypes: a short-term study in Iran. Sustainability 10:3273. doi: 10.3390/su10093273
Klein, D. A., Loh, T. C., and Goulding, R. L. (1971). A rapid procedure to evaluate the dehydrogenase activity of soils low in organic matter. Soil Biol. Biochem. 3, 385–387. doi: 10.1016/0038-0717(71)90049-6
Košutić, S., Filipović, D., Gospodarić, Z., Husnjak, S., Kovačev, I., and Čopec, K. (2005). Effects of different soil tillage systems on yield of maize, winter wheat and soybean on albic luvisol in north-West Slavonia. J. Cent. Eur. Agric. 6, 241–248. doi: 10.5513/jcea.v6i3.295
Kumar, A., Panda, A., Srivastava, L. K., and Mishra, V. N. (2017). Effect of conservation tillage on biological activity in soil and crop productivity under rainfed Vertisols of Central India. Int. J. Chem. Stud. 5, 1939–1946.
Kumar, V., Saikia, J., Barik, N., and Das, T. (2019). Effect of integrated nutrient management on soil enzymes, microbial biomass carbon and microbial population under okra cultivation. Int. J. Biochem. Res. Rev. 20, 1–7. doi: 10.9734/IJBCRR/2017/38868
Lal, R. (2023). Farming systems to return land for nature: It's all about soil health and re-carbonization of the terrestrial biosphere. Farming Syst. 1:100002. doi: 10.1016/j.farsys.2023.100002
Liu, H., Hussain, S., Zheng, M., Peng, S., Huang, J., Cui, K., et al. (2015). Dry direct-seeded rice as an alternative to transplanted-flooded rice in Central China Agron. Sustain. Dev. 35, 285–294. doi: 10.1007/s13593-014-0239-0
Li, Y., Zhang, Q., Cai, Y., Yang, Q., and Chang, S. X. (2020). Minimum tillage and residue retention increase soil microbial population size and diversity: implications for conservation tillage. Sci. Total Environ. 716:137164. doi: 10.1016/j.scitotenv.2020.137164
Lupwayi, N. Z., Arshad, M. A., Rice, W. A., and Clayton, G. W. (2001). Bacterial diversityin water-stable aggregates of soils under conventional and zero tillage management. Appl. Soil Eco. 16, 251–261. doi: 10.1016/S0929-1393(00)00123-2
Madejón, E., Murillo, J. M., Moreno, F., López, M. V., Arrue, J. L., Alvaro Fuentes, J., et al. (2009). Effect of long-term conservation tillage on soil biochemical properties in Mediterranean Spanish areas. Soil Tillage Res. 105, 55–62. doi: 10.1016/j.still.2009.05.007
Malobane, M. E., Nciizah, A. D., Nyambo, P., Mudau, F. N., and Wakindiki, I. I. C.. (2020). Microbial biomass carbon and enzyme activities as influenced by tillage, crop rotation and residue management in a sweet sorghum cropping system in marginal soils of South Africa. Heliyon ; 6:e05513. doi: 10.1016/j.heliyon.2020.e05513, PMCID: PMC7683310
Martin, J. P. (1950). Use of acid, rose Bengal and streptomycin in the plate method f prestimating soil fungi. Soil Sci. 69, 215–232. doi: 10.1097/00010694-195003000-00006
Moorhead, D. L., Sinsabaugh, R. L., Linkins, A. E., and Reynolds, J. F. (1996). Decomposition processes: modeling approaches and applications. Sci. Total Environ. 183, 137–149. doi: 10.1016/0048-9697(95)04974-6
Moulick, D., Samanta, S., Sarkar, S., Mukherjee, A., Pattnaik, B. K., Saha, S., et al. (2021). Arsenic contamination, impact and mitigation strategies in rice agro-environment: an inclusive insight. Sci. Total Environ. 800:149477. doi: 10.1016/j.scitotenv.2021.149477
Navarro-Noya, Y. E., Gómez-Acata, S., Montoya-Ciriaco, N., Rojas-Valdez, A., Suárez-Arriaga, M. C., Valenzuela-Encinas, C., et al. (2013). Relative impacts of tillage, residue management and crop-rotation on soil bacterial communities in a semi-arid agroecosystem. Soil Biol. Biochem. 65, 86–95. doi: 10.1016/j.soilbio.2013.05.009
Nayak, A. K., Gangwar, B., Shukla, A. K., Mazumdar, S. P., Kumar, A., Raja, R., et al. (2012). Long-term effect of different integrated nutrient management on soil organic carbon and its fractions and sustainability of rice–wheat system in Indo Gangetic Plains of India. Field Crops Res. 127, 129–139. doi: 10.1016/j.fcr.2011.11.011
Nisha, S., Kewat, M. L., and Sharma, A. R. (2016). Impact of conservation agriculture and weed control measures on soil physical and biological properties under rice – wheat-mungbean cropping system in vertisols. Int. J. Agric. Sci. 8, 2691–2695.
Page, K. L., Dang, Y. P., and Dalal, R. C. (2020). The ability of conservation agriculture to conserve soil organic carbon and the subsequent impact on soil physical, chemical, and biological properties and yield. Front. Sustain. Food Syst. 4:31. doi: 10.3389/fsufs.2020.00031
Parewa, H. P., Yadav, J., and Rakshit, A. (2014). Effect of fertilizer levels, FYM and bioinoculants on soil properties in inceptisol of Varanasi, Uttar Pradesh, India. Int. J. Agric. Environ. Biotechnol. 7, 517–525. doi: 10.5958/2230-732X.2014.01356.4
Parihar, C. M., Yadav, M. R., Jat, S. L., Singh, A. K., Kumar, B., Pooniya, V., et al. (2016). Long term conservation agriculture and intensified cropping systems: effect on growth, yield, water and energy-use efficiency of maize in North-Western India. Pedosphere 28, 952–963. doi: 10.1016/S1002-0160(17)60468-5
Poonam,, Srivastava, S., Pathare, V., and Suprasanna, P. (2017). Physiological and molecular insights into rice-arbuscular mycorrhizal interactions under arsenic stress. Plant Gene. 11, 232–237. doi: 10.1016/j.plgene.2017.03.004
Praharaj, C. S., Ali, M., Kumar, N., Dutta, A., Singh, U., and Singh, R. (2021). Pulses for crop intensification and sustainable livelihood. Indian J. Agron. 66, 60–72.
Pretty, J. (2008). Agricultural sustainability: concepts, principles and evidence. Philos. Trans. R. Soc. Lond., B, Biol. Sci. Philos T R Soc B 363, 447–465. doi: 10.1098/rstb.2007.2163
Qamar, R., Ehsanullah Rehman, A., Ali, A., Ghaffar, A., Mahmood, A., Javeed, H. M. R., et al. (2013). Growth and economic assessment of wheat under tillage and nitrogen levels in rice-wheat system. Am. J. Plant Sci. 4, 2083–2091. doi: 10.4236/ajps.2013.411260
Saha, R., Mishra, V. K., Majumdar, B., Laxminarayana, K., and Ghosh, P. K. (2010). Effect of integrated nutrient management on soil physical properties and crop productivity under a maize (Zea mays)–mustard (Brassica campestris) cropping sequence in acidic soils of Northeast India. Soil Sci. Plant Anal. 41, 2187–2200. doi: 10.1080/00103624.2010.504799
Salahin, N., Alam, K., Mondol, A., Islam, M. S., Rashid, M. H., and Hoque, M. A. (2017). Effect of tillage and residue retention on soil Propertiesand crop yields in wheat-Mungbean-Rice crop rotation under subtropical humid climate. Open J. Soil Sci. 7, 1–17. doi: 10.4236/ojss.2017.71001
Salinas-Garcıa, J. R., de Velázquez-Garcı́a, J., Gallardo-Valdez, M., Dıaz-Mederos, P., Caballero-Hernández, F., Tapia-Vargas, L. M., et al. (2002). Tillage effects on microbial biomass and nutrient distribution in soils under rain-fed corn production in Central-Western Mexico. Soil Till Res. 66, 143–152. doi: 10.1016/S0167-1987(02)00022-3
Sarkar, D., Kar, S. K., Chattopadhyay, A., Shikha, R. A., Tripathi, V. K., Dubey, P. K., et al. (2020). Low input sustainable agriculture: a viable climate-smart option for boosting food production in a warming world. Ecol. Indic. 115:106412. doi: 10.1016/j.ecolind.2020.106412
Sharma, A., Kumar, V., Shahzad, B., Tanveer, M., Sidhu, G. P. S., Handa, N., et al. (2019). Worldwide pesticide usage and its impacts on ecosystem. SN Appl. Sci. 1:1446. doi: 10.1007/s42452-019-1485-1
Singh, G., Marwaha, T. S., and Kumar, D. (2009). Effect of resource-conserving techniques on soil microbiological parameters under long-term maize (Zea mays) – wheat (Triticum aestivum) crop rotation. Indian J. Agri. Sci. 79, 94–100.
Singh, N. P., Singh, M. K., Tyagi, S., and Singh, S. S. (2018). Effect of integrated nutrient management on growth and yield of Rice (Oryza sativa L.). Int. J. Curr. Microbiol. App. Sci. 7, 3671–3681.
Singh, N., Singh, G., and Khanna, V. (2016). Growth of lentil (Lens culinaris Medikus) as influenced by phosphorus, rhizobium and plantgrowth promoting rhizobacteria. Indian J. Agric. Res. 50, 567–572. doi: 10.18805/ijare.v0iOF.4573
Six, J., Elliott, E. T., and Paustian, K. (2000). Soil microaggregate turnover and microaggregate formation: a mechanism for C sequestration under no-tillage agriculture. Soil Bio. Biochem. 32, 2099–2103. doi: 10.1016/S0038-0717(00)00179-6
Somenahally, A., DuPont, J. I., Brady, J., McLawrence, J., Northup, B., and Gowda, P. (2018). Microbial communities in soil profile are more responsive to legacy effects of wheat-cover crop rotations than tillage systems. Soil Biol. Biochem. 123, 126–135. doi: 10.1016/j.soilbio.2018.04.025
Spurgeon, D. J., Keith, A. M., Schmidt, O., Lammertsma, D. R., and Faber, J. H. (2013). Land-use and land-management change: relationships with earthworm and fungi communities and soil structural properties. BMC Ecol. 13:46. doi: 10.1186/1472-6785-13-46
Sravan, U. S., and Singh, S. P. (2019). Effect of integrated nutrient management on yield and Qualityof basmati Rice varieties. J. Agric. Sci. 11:93. doi: 10.5539/jas.v11n5p93
Suhaibani, N., Selim, M., Alderfasi, A., and Hendawy, S. (2020). Comparative performance of integrated nutrient management between composted agricultural wastes, chemical fertilizers, and biofertilizers in improving soil quantitative and qualitative properties and crop yields under arid conditions. Agronomy 10:1503. doi: 10.3390/agronomy10101503
Swarup, A. (1984). Effect of micronutrients and farmyard manure on the yield and micronutrient content of rice and wheat grown on a sodic soil. J. Indian Soc. Soil Sci. 32, 397–399.
Tao, H., Brueck, H., Dittert, K., Kreye, C., Lin, S., and Sattelmacher, B. (2006). Growth and yield formation of rice (Oryza sativa L.) in the water-saving ground cover rice production system (GCRPS). Field Crops Res. 95, 1–12. doi: 10.1016/j.fcr.2005.01.019
Thorton, H. G. (1922). On the development of standardized agar medium for counting soil bacteria with special regard to the repression of spreading colonies. Ann. Appl. Biol. 9, 241–274. doi: 10.1111/j.1744-7348.1922.tb05958.x
Upadhyay, M. K., Yadav, P., Shukla, A., and Srivastava, S. (2018). Utilizing the potential of microorganisms for managing arsenic contamination: a feasible and sustainable approach. Front. Environ. Sci. 6:24. doi: 10.3389/fenvs.2018.00024
Venkatesh, M. S., Hazra, K. K., Praharaj, C. S., and Kumar, N. (2013). Long-term effect of pulses and nutrient management on soil carbon sequestration in Indo-Gangetic plains of India. Can. J. Soil Sci. 93, 127–136. doi: 10.4141/cjss2012-072
Venugopalan, V. K., Nath, R., Sengupta, K., Pal, A. K., Banerjee, S., Banerjee, P., et al. (2022). Foliar spray of micronutrients alleviates heat and moisture stress in lentil (Lens culinaris Medik) grown under rainfed field conditions. Front. Plant Sci. 13:847743. doi: 10.3389/fpls.2022.847743
Vineela, C., Wani, S. P., Srinivasarao, C., Padmaja, B., and Vittal, K. P. R. (2008). Microbial properties of soils as affected by cropping and nutrient management practices in several long-term manurial experiments in the semi-arid tropics of India. Appl. Soil Ecol. 40, 165–173. doi: 10.1016/j.apsoil.2008.04.001
Wani, O. A., Sharma, V., Kumar, S. S., Babu, S., Sharma, K. R., Rathore, S. S., et al. (2023). Climate plays a dominant role over land management in governing soil carbon dynamics in North Western Himalayas. J. Environ. Manag. 338:117740. doi: 10.1016/j.jenvman.2023.117740
Yadav, G. S., Datta, R., Imran Pathan, S., Lal, R., Meena, R. S., Babu, S., et al. (2017). Effects of conservation tillage and nutrient management practices on soil fertility and productivity of rice (Oryza sativa L.)–rice system in north eastern region of India. Sustainability 9:1816. doi: 10.3390/su9101816
Yang, X., Drury, C. F., and Wander, M. M. (2013). A wide view of no tillage practices and soil organic carbon sequestration. ACTA. 63, 523–530. doi: 10.1080/09064710.2013.816363
Zeffa, D. M., Perini, L. J., Silva, M. B., de Sousa, N. V., Scapim, C. A., Oleivera, A. L., et al. (2019). Azospirillium brasiliense promotes increases in growth and nitrogen use efficiency of maize genotypes. PLoS One 14:e0215332. doi: 10.1371/journal.pone.0215332
Zhang, B., He, H., Ding, X., Zhang, X., Zhang, X., Yang, X., et al. (2012). Soil microbial community dynamics over a maize (Zea mays L.) growing season under conventional-and no-tillage practices in a rainfed agroecosystem. Soil Tillage Res. 124, 153–160. doi: 10.1016/j.still.2012.05.011
Zhang, Y., Li, C., Wang, Y., Hu, Y., Christie, P., Zhang, J., et al. (2016). Maize yield and soil fertility with combined use of compost and inorganic fertilizers on a calcareous soil on the North China plain. Soil Till. Res. 155, 85–94. doi: 10.1016/j.still.2015.08.006
Keywords: agro-ecosystem stability, integrated nutrient management, minimum tillage, soil health, yield
Citation: Bhattacharya U, Naskar MK, Venugopalan VK, Sarkar S, Bandopadhyay P, Maitra S, Gaber A, Alsuhaibani AM and Hossain A (2023) Implications of minimum tillage and integrated nutrient management on yield and soil health of rice-lentil cropping system – being a resource conservation technology. Front. Sustain. Food Syst. 7:1225986. doi: 10.3389/fsufs.2023.1225986
Edited by:
Arnab Majumdar, Jadavpur University, IndiaReviewed by:
Poonam Yadav, Banaras Hindu University, IndiaPradeep Kumar Dubey, Banaras Hindu University, India
Copyright © 2023 Bhattacharya, Naskar, Venugopalan, Sarkar, Bandopadhyay, Maitra, Gaber, Alsuhaibani and Hossain. This is an open-access article distributed under the terms of the Creative Commons Attribution License (CC BY). The use, distribution or reproduction in other forums is permitted, provided the original author(s) and the copyright owner(s) are credited and that the original publication in this journal is cited, in accordance with accepted academic practice. No use, distribution or reproduction is permitted which does not comply with these terms.
*Correspondence: Visha Kumari Venugopalan, visha.venugopal@gmail.com; Akbar Hossain, akbarhossainwrc@gmail.com