- 1Department of Water Resources and Reclamation, Kazakh National Agrarian Research University, Almaty, Kazakhstan
- 2Faculty of Resources and Environmental Science, Hubei University, Wuhan, China
- 3Kazakh Scientific Research Institute of Water Economy LLP, Almaty, Kazakhstan
- 4Institute of Natural Sciences and Geography, Abai Kazakh National Pedagogical University, Almaty, Kazakhstan
- 5Faculty of Economics and Business, John von Neumann University, Kecskemét, Hungary
- 6Institute of Rural Development and Sustainable Economy, Hungarian University of Agriculture and Life Sciences, Gödöllő, Hungary
The escalating demand for water and the impact of climate change are posing significant challenges to global water scarcity and agricultural policies. The aim of this research is to investigate the factors influencing water losses in irrigation systems and provide recommendations to minimize such losses. The research methodology includes conducting studies to identify the factors causing water losses through evaporation and seepage from irrigation canals in the Kazakh part of the Ile River basin. The research results are theoretically substantiated, and a series of field studies are carried out at the inter-farm canal experimental site in Almaty province. Based on the factors affecting evaporation and seepage losses in irrigation canals, a new design of irrigation canal made from geocomposite material has been developed and introduced. This design helps to prevent water losses in inter-farm and on-farm canals of reclamation systems. The geocomposite polymer troughs have improved the technical level of irrigation systems and have enabled additional irrigation areas by increasing the efficiency of irrigation water use in land reclamation systems of Kazakhstan, thereby enhancing the efficiency factor of the irrigation network. Water seepage losses are influenced by several factors, including soil type, canal length, working flow, canal operation mode, canal condition, operation season, and wind impact. Under uniform canal operation mode, water losses are relatively small. However, intermittent modes and incomplete filling of the canal result in increased costs. The research findings indicate that for soils with a flow velocity between 0.10 and 0.30 M/s, water losses due to leakage and evaporation in constant-impact and intermittent-impact channels increase linearly. In intermittent-impact channels, irrigation water losses range from 33.7 to 40.1%. The introduction of geocomposite polymer troughs has the potential to enhance the technical level of irrigation systems by improving the efficiency of the irrigation network and enabling the addition of more irrigation plots. This research aims to reduce water losses in the irrigation canals of reclamation systems, optimize the management of water distribution in irrigation systems, enhance equipment measures, and introduce digital irrigation water metering technologies. Future studies propose the application of digital technologies to enhance irrigation water design and accounting as part of measures to minimize water losses in irrigation canals.
1. Introduction
Due to global climate change and the high water consumption of the agricultural sector, water scarcity has become an increasingly pressing issue worldwide (Abuzeid, 2021; Elkamhawy et al., 2021). Currently, the issue of water resources is at the forefront of global politics, with water supply predicted to be as vital as hydrocarbons in the near future (Aghvami et al., 2013). Non-flow losses and evaporation losses are considered serious causes of water losses in irrigation canals (Waller and Yitayew, 2015). Various factors, such as soil base permeability, depth of channel water, length of wetted perimeter, water table, and velocity of flowing water, contribute to losses in irrigation networks (Abd-Elhamid et al., 2021; El-Molla and El-Molla, 2021). While evaporation losses are relatively small, irrigation water outflow from surface canals is a major cause of water loss (Christian and Trivedi, 2018; Garg, 2020). Materials such as geomembrane, bentonite, and concrete can be used to reduce these losses under earthen channels (Stark and Hynes, 2009). Geomembranes have been found to significantly reduce water consumption, with priming canals with geomembrane leading to almost a 90% reduction in water utilization (Blanco et al., 2012).
Open screens made of sheet butyl rubber were first used in US canals in 1948, with the condition of the coating allowing for the prediction of a service life of up to 40 years after 15 years of operation. In the 1960s, the USA saw an increase in the water utilization factor from 0.51–0.53 to 0.65, with the efficiency factor reaching 0.99 in the 1990s. When installing impervious blankets, films based on polychlorovinyl, polyethylene, or butyl rubber with a thickness of at least 0.2 mm and reinforcement were used (Kosichenko et al., 2014). Polyvinyl chloride films have also been used successfully in the USA since 1955 to prevent seepage through the bottom and slopes of canals located in permeable soil (Kosichenko and Baev, 2014). Sheet polymer materials with a thickness of more than 0.5 mm and high reliability indicators compared to similar polymer films were also widely used in hydraulic engineering (Arifjanov et al., 2022). These materials were later referred to as “geomembrane” (Cazzuffi, 1987) and were made from synthetic polymers, plasticizers, elastomers, and light stabilizers. Nowadays, geomembrane coatings in channels are left open to maximize the channel cross-section and improve the low hydraulic roughness of the material. Open geomembrane coatings are also less susceptible to fouling and more easily repaired, as any damages are quickly detected and repaired. In the late 1960s, geomembranes became popular in Europe during the reconstruction of dams (Bocharnikov and Borovikov, 2021). Geomembranes have since been used worldwide to reconstruct 97 large dams of various types, either covering the entire dam surface or damaged elements. By 2005, over 250 watertight geomembrane dams were in use worldwide, serving as unfiltered screens and diaphragms, and in the repair of damaged pressure surfaces of arch, gravity, and support dams (Scuero and Vaschetti, 2019).
In accordance with the research topic, Balgabaev (2022) distributes water losses due to seepage in the irrigation network as follows: 30–35% in main canals, their branches and inter-farm distributors; 50–55% in on-farm irrigation networks; up to 10% in temporary irrigation sprinklers. Consequently, only 24% of the water withdrawn is effectively used for crop irrigation. It should be noted that over the last 30–40 years, the share of water losses in main canals has decreased by 10–15%, while losses in irrigation systems and fields have increased. This can be explained by natural precipitation settling and improvement of the design of main canals and their main distributors, as well as degradation of irrigation systems and technologies on agricultural lands.
Currently, the irrigation system of Kazakhstan has about 3,500 km of main and inter-farm canals, 55% of which are in unsatisfactory condition. Table 1 shows the technical condition of irrigation canals in Kazakhstan of different forms of ownership.
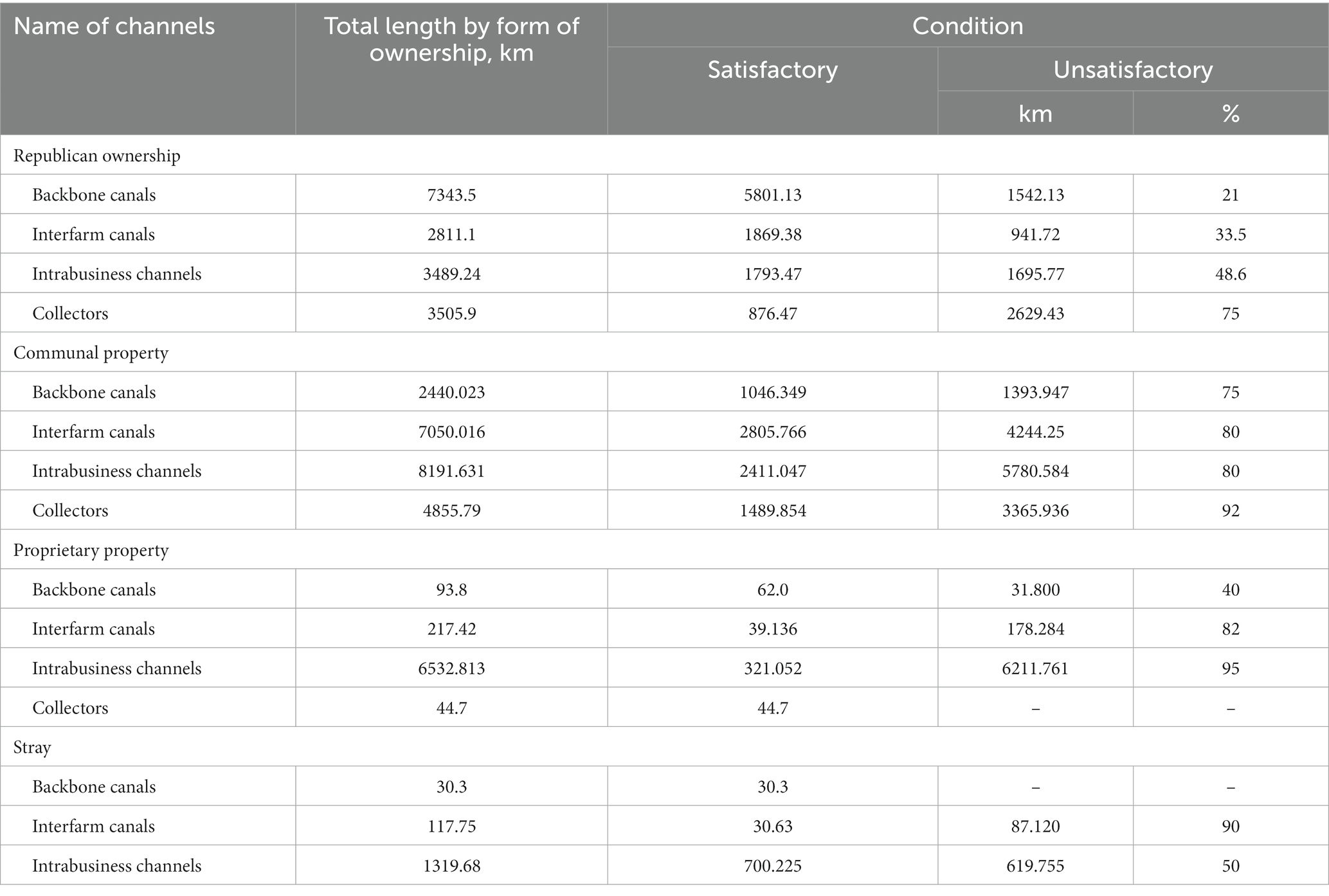
Table 1. Technical condition of irrigation canals in Kazakhstan (Kenshimov, 2019).
In Kazakhstan, physical deterioration of irrigation canals and insufficient technical equipment lead to significant losses of irrigation water. Table 2 shows that irrigation water yield is 6–8 times lower than in foreign countries, and irrigation water consumption per unit yield is 4–8 times higher (Kenshimov, 2019).
Analysis of information and analytical materials on the Republic of Kazakhstan has shown that unproductive losses on open irrigation systems make up 50–65% of water withdrawal from water sources, including seepage losses in canals from 45 to 55% (Kosichenko, 2012). At the same time, seepage losses from poorly equipped canals exceed the permissible level by 5–10 times, which leads to waterlogging of adjacent territories and deterioration of ecological and ameliorative condition of saline irrigated lands (Kosichenko et al., 2014).
An important direction of research and development for solving these problems is scientific substantiation and promotion of new methodologies for planning and implementation of water use processes in irrigation systems. These techniques should take into account the current level of development of the construction industry in order to develop new impermeable materials and structures that reduce seepage and evaporation losses in canals while ensuring good ameliorative condition of irrigated lands and normal ecological situation in agrolandscapes (Alimov, 2008; Solsky et al., 2015).
At present, a new generation of water-repellent packing of irrigation canals has been developed using geosynthetic materials such as geomembranes, geotextiles, geocomposites, geogrids, geobags, bentomats and gabions, which create watertight structures and laying methods, completely eliminate seepage costs and provide an increase in the efficiency of irrigation canals up to 0.97–0.98 (Chuprin and Cherednichenko, 1970; Radchenko and Semenkov, 1992).
The choice of impervious construction should ensure the necessary permeability of the canal soil, sufficient strength of the structure, its resistance to temperature fluctuations and destructive effects of weeds. In addition, construction works should be mechanised and the choice of impermeable cover should be based on technical and economic comparison of options.
The analysis has shown that in the world practice, a number of measures are taken to reduce water losses in canals. There are two ways to control water infiltration from irrigation canals: reduction of soil permeability and construction of impervious blankets. Diverse natural and economic conditions of irrigation areas have determined the use of different designs of impervious blankets on irrigation canals.
Reduction of water permeability of channel soil can be achieved by mechanical compaction and colmatage – filling of soil pores with small particles, for example, for sandy soils colmatage with clay and silty soils can be applied (Anakhaev et al., 2006). A special way of reducing water permeability is the method of adding special materials to the channel soil. This may include artificial salinisation of soil, artificial ogling, and oiling, etc., but such methods lead to pollution of water flow (Kosichenko and Baev, 2019).
President K. Tokayev (Message of Head of the State K.K. Tokayev to the people of Kazakhstan, 2022) and recommendations of scientists (Giroud et al., 2004; Olgarenko, 2013; Prabakaran et al., 2019; Baev et al., 2022), taking into account the problems we have identified, in this paper, based on the study of factors affecting water losses in irrigation systems, we aimed to identify the main directions of their reduction and propose specific measures to reduce water losses in irrigation canals of reclamation systems. The conducted research will make it possible to further improve, implement measures that reduce water loss in irrigation canals of reclamation systems with the help of innovative technologies, effectively manage water distribution processes in irrigation systems, improve structures and implement digital technologies of irrigation water accounting.
2. Materials and methods
Currently, the excessive loss of irrigation water during the transportation and supply process to irrigated areas of farms in Almaty, Zhambyl, Turkestan, and Kyzylorda provinces reaches 3 million m3. As a result, farmers receive lower yields, worth approximately 400 billion tenge, even at average levels of agrotechnics and irrigation technology, while the operating organizations incur unreasonable operating costs of approximately 2–2.5 billion tenge per year (Balgabaev, 2022).
An increase in excessive water losses is one of the signs of decreasing operational reliability of irrigation systems. The permeability of soils depends on several factors (Rau et al., 2023), including mineral and granulometric composition, porosity, stratification, composition of filtered liquid, temperature, hydrostatic head, and periodicity of canal operation. The majority of interfarm and on-farm canals in the studied area are earthen channels, which lead to low efficiency values due to significant filtration losses. This degradation not only reduces the hydraulic efficiency of the canal but also affects the surrounding territory.
To address this problem, a pilot site was selected 0.8 km south of Saimasai village in Enbekshikazakh district, Almaty region of Kazakhstan, with geographical coordinates of 43.431669 N, 77.330309 E (Figure 1). Seepage control studies were conducted on interfarm and on-farm canals at this site.
At the experimental site, a study was conducted to evaluate water evaporation losses from canals using a well-established methodology. Glass vessels in the form of measuring cups filled with water from the canal were utilized for this purpose. These vessels were mounted on a float on 1 m2 polychlorovinyl boards with five holes, with five pieces in each. Each vessel was attached to two sections of the canal, with one stationary vessel installed on a section of the canal enclosed on both sides by forests and fully shaded by tree crowns during the daytime, while the other was installed on an open, non-flooded section of the canal. The vessels were filled with a medical pipette from the canal whenever water evaporated. To monitor the process, the control duration was from 6.00 to 20.00 h, with measurements taken every 2 hours (hours 6.00, 8.00, 10.00, 12.00, 14.00, 16.00, 18.00, and 20.00 h) synchronously at both stations. During the identical hours at both stations, air temperature and humidity above the water were measured using an aspiration psychrometer, water temperature in the canal at a depth of 5 cm was measured using a water thermometer, illumination above the canal water was measured using a light meter, and speed of movement was measured using an anemometer.
During the research, we utilized GR-21 M and ISVP-GR-21 M microcirculators to measure water flow velocity. The mechanical composition and water percolation coefficient of soil samples were determined in a certified laboratory at the Institute of Hydrogeology and Geoecology named after Akhmedsafin, using a device for determining the seepage coefficient. Additionally, at the pilot site, we conducted studies to assess water evaporation losses from canals, utilizing an aspiration psychrometer, an air and water thermometer for determining temperature in the canal, a luxometer for measuring illumination over the water surface of the canal, and an anemometer for determining wind speed.
To determine dependence of water losses from canals on such factors as seepage properties, geological structure of soils along the route, depth of occurrence of natural groundwater table and draining soil layers or groundwater table, length of canal perimeter and its operation mode, presence of impervious devices and others, soil samples were taken from pits along inter-farm canals in Saimasai settlement (SP 100.13330.2016. SNiP 2.06.03–85 Ameliorative systems and structures, 2023) (Figure 2).
The results of the analysis on mechanical composition, specific gravity, density of soils comprising the Saimasai in-farm canal bed in Almaty region are given in Table 3. Analysis of the data in Table 3 shows that on the surface 0–20 cm layer the content of fines less than 0.1 mm are in the bottom of the on-farm channel (0.93%), while on the bottom of the inter-farm channel 1.09% is sand and on the surface layer in the ditch 15.78% is sandy loam soil (Figure 3).

Table 3. Results of soil particle size distribution and density (bottom of the on-farm canal at Saimasai).
The solid soil density is the mass of a unit volume of absolutely dry soil taken in its natural state, expressed in g/cm3. It is one of the most important properties that de-termines the ability of soil to permeate and retain moisture. According to the results of the analysis, the soil on the surface 0–20 cm layer is normal, on the 20–40 cm layer the soil is compacted, on the 40–60 cm layer the soil is heavily compacted and beyond.
The technical condition of the ducts is primarily characterized by the efficiency factor. The higher the efficiency the better the technical condition of the ducts.
The movement of water in channels takes place in different rocks and materials, which have different porosity, fracturing and permeability.
To quantify seepage losses from an irrigation canal, the flow rates are determined at two sections of the canal and the difference between the upper and lower sections is used to calculate the seepage losses.
The methodology for calculating water losses in irrigation canals was based on the empirical formula of A.N. Kostyakov, which determines the percentage of flow losses per 1 km of canal length:
For medium permeable soils, the A = 1.9 and m = 0.4, while for highly permeable soils, A = 3.4 and m = 0.5 (SP 100.13330.2016. SNiP 2.06.03–85 Ameliorative Systems and Structures, 2023).
For this purpose, water discharges were determined at two sections of the canal, and water losses were calculated from the difference in discharges at the upper and lower sections.
Flow rates in the canal were measured using Chippoletti weirs: at the head – #1, B = 0.75 m, at the end – #2 B = 0.75 m (Figure 4).
Water losses in irrigation canals vary depending on several factors, including hydraulic parameters of the canal, type of soil that makes up the canal bed, and discharge. The amount of water lost per 1 km of canal length ranges from 16 L/s to 37.5 L/s, while the daily water losses range from 1728 m3/(per day 2) to 3,240 m3/(per day 2).
The obtained experimental values correlate with A.N. Kostyakov’s formula for determining the amount of seepage from channels:
Due to diverse natural and economic conditions of irrigation areas, different designs of impervious blankets are used on irrigation canals. The most common are monolithic concrete, prefabricated reinforced concrete, concrete-foam, and soil-foam covers, as well as flume canals. Canals constructed on subsidence and scour and landslide resistant soils may not require lining. Water seepage can be prevented by reducing the permeability of the channel bottom through mechanical compaction and colmatisation, whereby the soil pores are filled with fine particles. For example, sandy soils can be colmatised with clay and silty soils with silt. In addition, special materials can be added to the canal soil to reduce water permeability. However, techniques such as artificial desalination, artificial gleying and oiling can lead to pollution of watercourses.
Losses from irrigation canals are the main types of loss that significantly impact the coefficient of performance of the canal and the entire irrigation system.
The research program consisted of both theoretical and field studies on water use efficiency, technical conditions, and equipment of irrigation systems. Five sites were designated for field studies, where various canal bed protection variants were installed: (a) a geomembrane pavement with geotextile at the base and a concrete filler covered with geogrid, (b) a geomembrane pavement with a gravel filler and geotextile at the base, (c) a geomembrane pavement with geotextile in the base, (d) a bentonite mat, and (e) a geocomposite (plastic) flume-model of an irrigation canal. At the selected sites, the water flow velocity was measured using a microturbine, after which the water flow rate was determined and the value of the water flow rate between the groats was established. Actual water losses were compared with theoretical ones, and the mechanical composition and leakage coefficient of soil samples were determined in a certified laboratory.
At the experimental site, evaporation losses from canals were evaluated using the aspiration psychrometer, thermometer, luxometer for illumination on the water surface of the canal, and anemometer for wind speed. The determination of water losses in an unshielded irrigation canal involved several stages of work outlined in the flowchart (Figure 5).
3. Results and discussion
As a result, the magnitude of seepage losses depends on several factors, including soil type, canal length, and canal velocity. Seepage losses depend on the canal operation mode, canal condition, season of use and other minor factors such as wind and other environmental influences. Under uniform canal operation mode, water losses are relatively small. On the contrary, intermittent operation and incomplete filling of the canal lead to increased costs.
The analysis of the technical condition of irrigation canals in the Republic of Kazakhstan has shown that irrigation systems mainly operate periodically during irrigation periods, and during inter-irrigation periods they “rest,” i.e., are without water. These periods alternate every 5–15 days during the irrigation season, and on average there are from 2 to 8 irrigation periods and from 1 to 7 inter-irrigation periods per season.
During inter-irrigation periods, canal beds dry up, which leads to increased seepage losses. Such canals include mainly lower-order irrigation canals, such as former on-farm canals. On the contrary, main canals and their first-order distributaries operate continuously during the irrigation period, which leads to low water losses determined by the formula.
To evaluate the influence of operation periodicity on the amount of seepage through the canal bottom, a coefficient is introduced into formula (3). The coefficient takes into account water absorption into the ground for the first unit of time (Sharov, 1959):
Taking this into account, calculation of water losses from batch canals for seepage (Qsp., m3/s-km) can be carried out according to the formula of N. Pavlovskiy (Sharov, 1959), by introducing the absorption coefficient Kac into the formula (4.10):
To better account for all water losses from canals, the evapotranspiration of the irrigation system (Qel, m3/s-km) can be determined using the formula:
The necessity and size of the anti-permeability cover for a canal depend on several factors, including the soil type, slope of the route, and design parameters of the canal. Additionally, the irrigation system’s operation mode, such as the level of observance of planned round-the-clock water use, plays a role. Table 4 shows the results of calculations on determination of water losses for seepage and evaporation for irrigation canals of “continuous operation” and “periodic operation” taking into account soil filtration characteristics and normal operation and maintenance of the irrigation network (Bogushevsky et al., 1981).
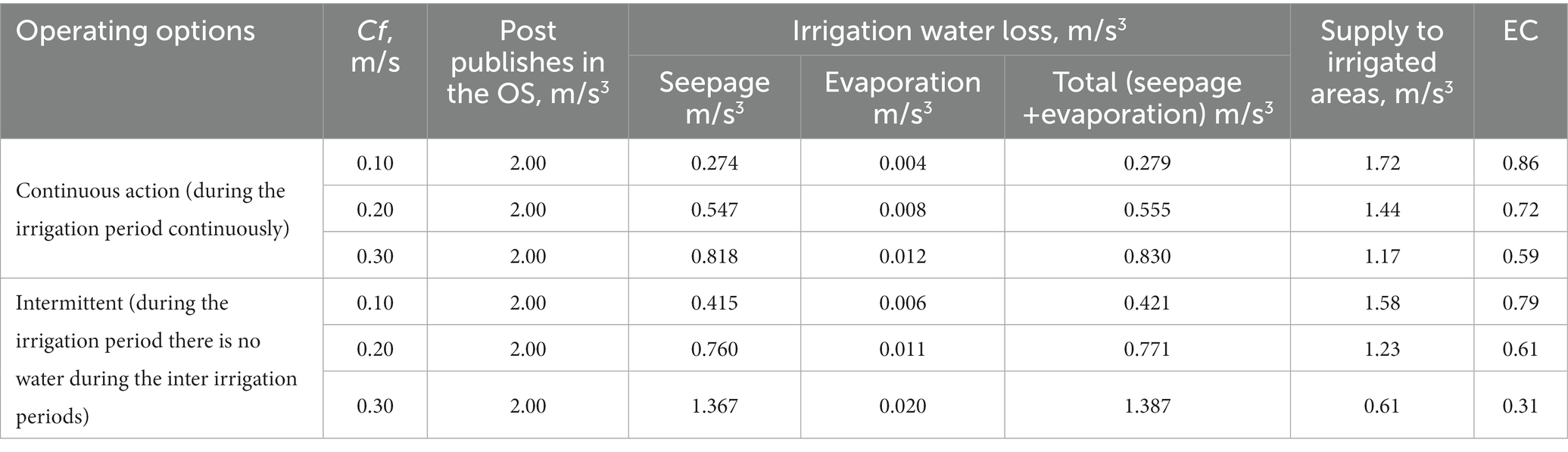
Table 4. Required minimum share of irrigation system canal lining depending on soil seepage properties and operation mode (design area 2000 ha, length of irrigation canals −40 km).
The criterion for assessing and selecting impervious measures is based on the layer of water lost from the canal through the wetted perimeter in a day. A water layer of more than 30 cm lost from the canal indicates that an impervious measure is needed, while a loss of 5–10 cm indicates that the canal is working satisfactorily.
A careful analysis of Graphs 1 and 2 presented in Figure 6 reveals that water leakage and evaporation losses in channels with flow velocities ranging from 0.10 to 0.30 m/s increase linearly. However, in channels with periodic influence, irrigation water losses occur more intensively (from 33.7 to 40.1%) than in channels operating continuously throughout the entire irrigation period.
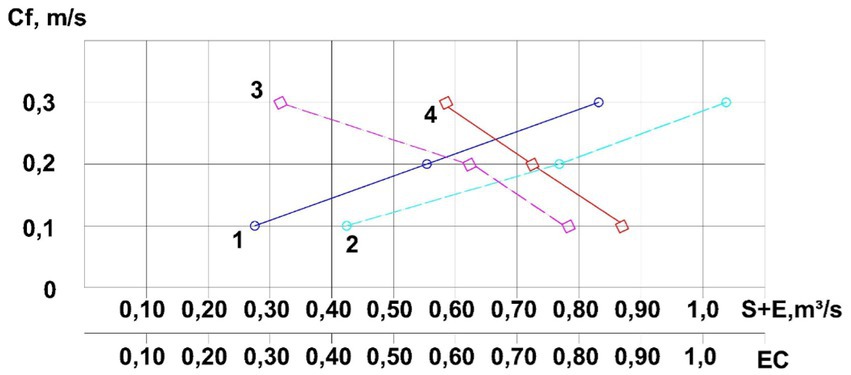
Figure 6. Water losses for infiltration and evaporation from irrigation canals “continuous operation” and “periodic operation” taking into account soil seepage characteristics under normal operation and maintenance of the irrigation network.
Furthermore, an examination of the graphs depicting the relationship between channel efficiency and leakage coefficient (curves 3 and 4) confirms an inversely proportional dependence for channels with constant and periodic influence. In channels with water-repellent soils, the efficiency increases from 0.07 to 0.28.
Table 4 indicates that water losses increase by 30–60% under the canal operation regime with intermittent interirrigation periods, i.e., periods without water. The total interirrigation period accounts for up to 50% of the irrigation period, which varies between 80–140 days depending on natural, agrotechnological, and organizational conditions.
Based on the data in Table 4 and Figure 6, it is advisable to prioritize measures to prevent water losses due to filtration on intermittent channels, especially those passing through light and medium soils. For canals constructed on heavy soils, such as heavy loam and clay, linings can be avoided or made for a maximum of 50% of the length.
Table 5 presents the results of calculations for determining the optimal percentage of linings for the total length of irrigation canals.
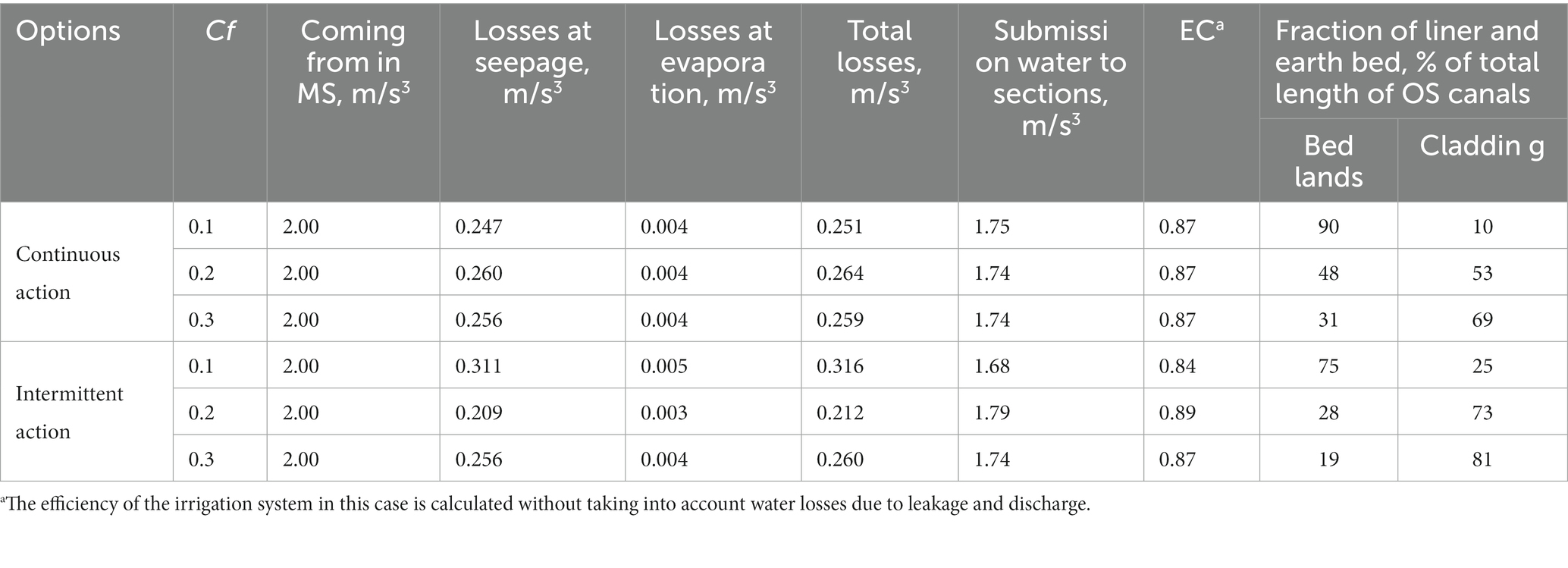
Table 5. Results of calculations carried out to establish the rational share of linings from the total length of irrigation canals (40 km in total).
Based on Table 5, it is noteworthy that the need for impervious covers for permanent canals on heavy soils decreases. Therefore, it is advisable to make final decisions based on a feasibility study.
For intermittent irrigation systems, impervious covers should be a mandatory retrofit measure. At least 35% coverage is required for heavy soils for the entire length of irrigation system canals, while at least 70% coverage is necessary for medium soils and at least 80% coverage for light soils, including light soils (Shtepa et al., 1990).
This study aims to propose measures for reducing irrigation water losses in land reclamation system canals. By analyzing various contemporary impervious techniques, we have developed a design for an artificial irrigation canal in the form of a composite plastic flume.
The design of a flume for a flow rate of up to 300 L/s was developed, manufactured, and implemented at a pilot site: the interfarm canal site located within the experimental-production training farm of the Kazakh National Agrarian Research University in Saimasay village, Enbekshikazakhskiy district, Almaty region. The flume, which serves as a model irrigation canal, is constructed of geocomposite polymer material to prevent water seepage losses (referred to as a geocomposite flume). The design was granted a utility model patent under the number 7345 on August 12, 2022, titled “Irrigation canal for reclamation systems” (Useful model patent KZ, 2022). This utility model pertains to hydraulic engineering construction, specifically the construction of impermeable covers for irrigation canals constructed from polymeric materials placed on a ground base.
The objective of this utility model is to prevent water losses due to seepage in irrigation canals of land reclamation systems. The technical solution provides execution of irrigation canal of land reclamation system in the form of a trapezoidal tray made of plastic, including transverse stiffeners on the forming walls along the entire length of the tray, except for the ends, longitudinal stiffeners on the edges of the walls along the entire length of the tray and transverse strips on the upper part of the tray. The trays are butt jointed on one side and tongue and groove jointed on the other side. In this technical solution, the desired result of preventing water losses during seepage is achieved by making an irrigation channel in the form of a trapezoidal plastic tray, including transverse and longitudinal stiffening ribs and transverse strips in the upper part of the tray, and connecting the trays by butt joints on one side and tongue-and-groove joints on the other.
Figure 7 depicts the general view and cross-section of a trapezoidal irrigation canal made of geocomposite plastic material, which was manufactured according to the developed working drawings. The irrigation canal for the reclamation system features a trapezoidal-shaped tray (1), transverse stiffening ribs (2), longitudinal stiffening ribs (3), and transverse slats (4). The troughs are equipped with a groove (5) on one side and a tongue and groove joint (6) on the other. The process of laying the irrigation canal in the form of a trapezoidal tray begins by digging a trapezoidal canal (7) that corresponds to the dimensions of the installed trays (1) and the design documentation. The trays (1) are then placed into the canal (7), with each subsequent tray section placed with the groove (5) and tongue (6) of the previous tray on the ground. The installation is then performed as usual, with trays with turning used if necessary. After installation, the flumes are backfilled. Figure 8 shows the working points of the laying process and investigation of the geocomposite flume in the pilot section of the interfarm irrigation canal located in Saimasai village, Almaty region.
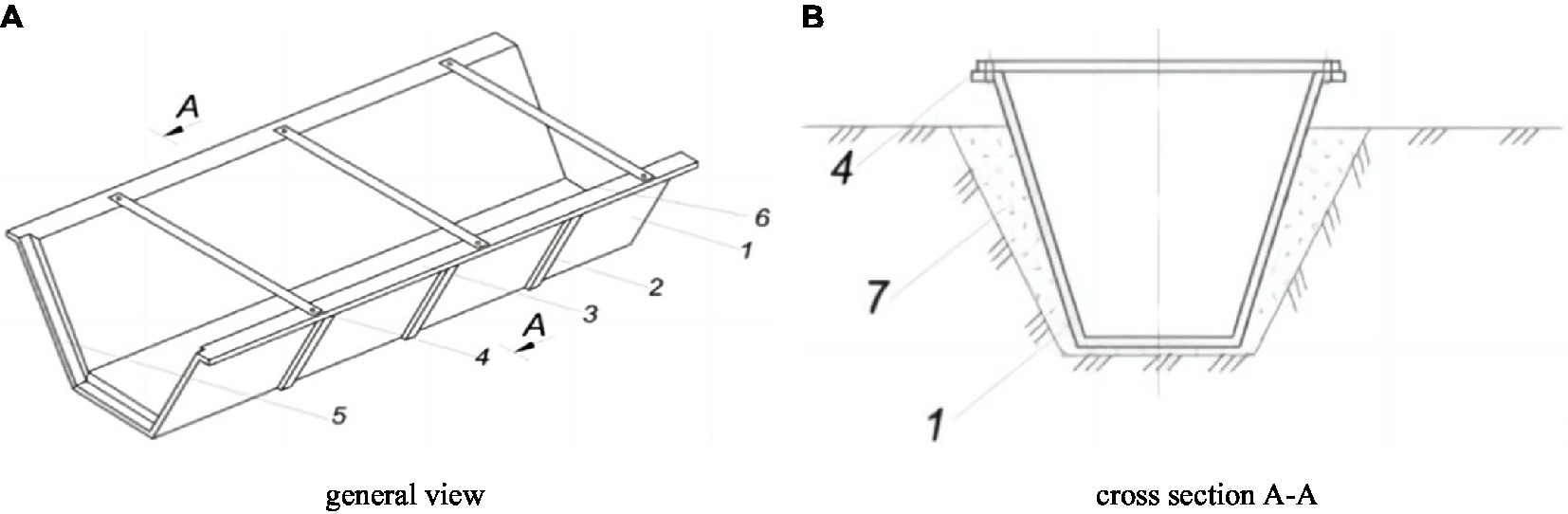
Figure 7. Trapezoidal geocomposite tray, (A) general view; (B) cross section A-A. 1- tray of trapezoidal shape, 2- transverse stiffeners, 3- longitudinal stiffeners, 4- transversal strips, 5- tray groove, 6- tongue and groove, and 7- trapezoidal earth channel.
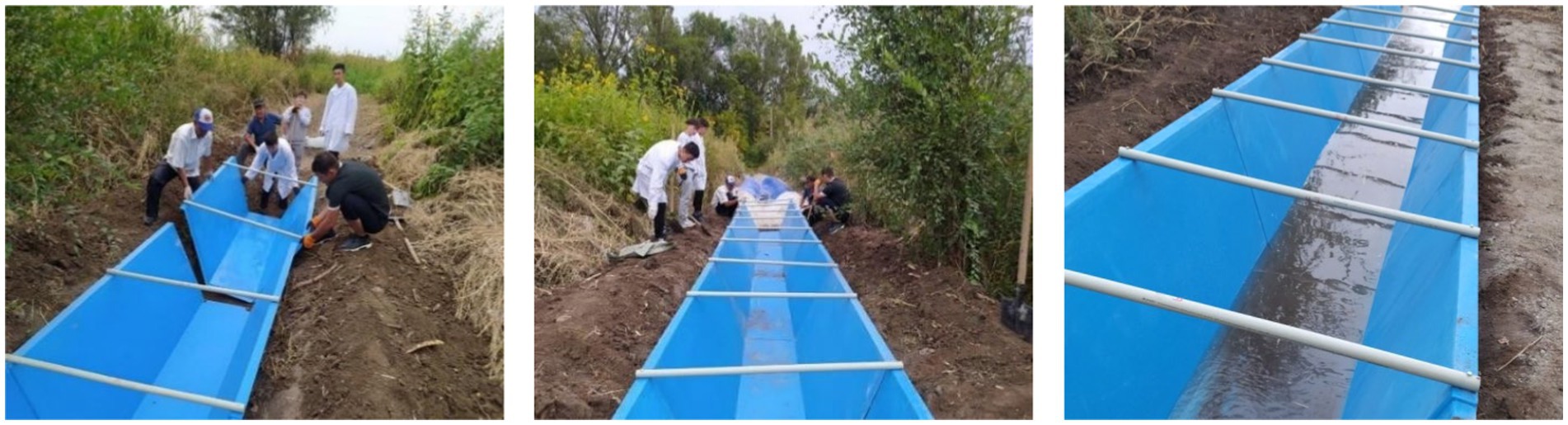
Figure 8. Laying of a geocomposite tray on a pilot section of an interfarm irrigation canal in Saimasai village, Almaty Region.
The tray parameters are as follows: the cross-sectional shape is trapezoidal, with dimensions of 50 cm for the bottom width, 70 cm for the height, a slope setting of m = 1.5, and 120 cm for the top width. The tray length depends on the size, with options of 2 m, 4 m, and 6 m, but in our case, it is 2 m. The tray wall thickness is 8 mm. The trays are joined together using butt joints, with a groove on one side and a tongue on the other. The tray used in the pilot section is designed to handle a capacity of up to 300 L/s (Figure 8).
The proposed canal design offers a significant advantage in that the irrigation flumes can be manufactured in individual sizes to meet size and configuration requirements as much as possible. Moreover, they can be used on channels made in a half excavation-half embankment, as well as in a flume network on supports. When renovating existing irrigation systems or constructing new ones, polymer trough design is indispensable. No crane is required to install the trays, and several people can handle the task. Transportation is easy, as the trays do not take up much space and can be stacked on top of each other. The prefabricated and collapsible options of trays and connections allow for periodic changes in water supply routes to adapt to changing needs. The irrigation tray system is easy to install, modify, and relocate. Additional elements, such as stiffening ribs, increase strength and allow the irrigation trays to withstand any loads. The design of the channels can also take into account various installation variants (aboveground or underground), water-carrying capacity, and connection methods. The plastic material used in the design is given a proper shape before polymerization, followed by a process of glass fiber reinforcement. It has high strength, resistance to biologically active substances, and moisture resistance. GRP, which has gone through a complete production cycle, does not contain volatile compounds, making it safe for humans and the environment. Its durability is comparable to that of concrete, and studies have shown high reliability, practicality, and acceptability for practical application as an irrigation canal of a reclamation system.
It should be noted that the proposed irrigation canal design can also be used as a measure to control evaporation from the water surface of canals on reclamation systems. For this purpose, it is proposed to install protective meshes made of polyethylene materials with different sizes of cells stretched on stiffening ribs and stretched along the entire length of the canal (Figure 9). The proposed devices are inexpensive, readily available, easy to manufacture, and easy to install (mounting and dismounting).
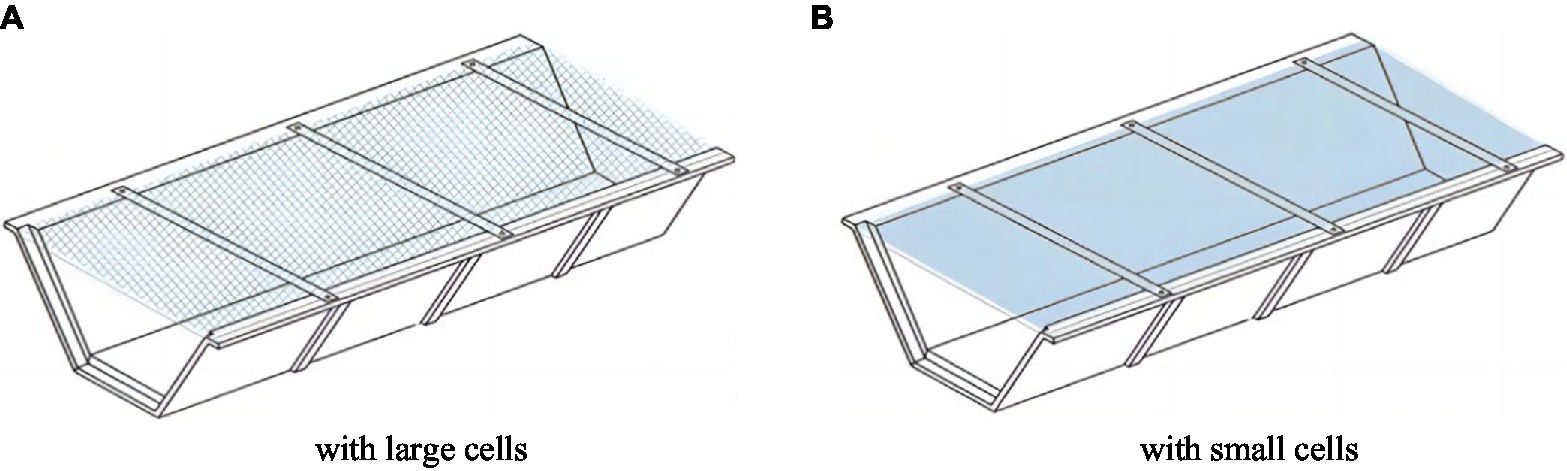
Figure 9. Protective screens to reduce evaporation losses at irrigation canals, (A) with large cells; (B) with small cells.
In addition to its main task of preventing seepage and reducing water losses by reducing its heating, the installation of protective nets significantly reduces water evaporation losses. These nets also prevent debris from entering the canal, which is a cause of reduced canal capacity and is especially relevant for Kazakhstan. In addition, they protect the canals from various small animals such as hedgehogs and turtles.
It is recommended to install an improved design of impervious cover of the irrigation canal made of composite material on inter-farm and on-farm canals of reclamation systems. This allows to reduce water losses not only during transportation, but also in the general irrigation system and promotes introduction of new irrigation areas.
However, Water losses from irrigation canals are directly influenced by soil permeability, which is affected by several factors, including mineral composition, granulometric composition, porosity, stratification, temperature, and hydrostatic pressure (Sokolskaya, 1969; Nauman and Sergeyev, 1981; Chahar, 2007; Nam et al., 2016; Abd-Elaty et al., 2022).
• Mineral composition. Dispersibility depends on the mineral composition – the degree of refinement of solid particles. Their influence on water permeability is the most important.
• Granulometric composition. Coarse-grained soils and sands have high water permeability. Reducing the diameter of sand particles leads to a decrease in the index.
• Porosity. The rate of passage of liquid directly depends on their shape and size of soil particles.
• Layering. Many soils have a layered structure. Their particles are directed in a certain direction. The water permeability of the material depends on this. For example, in banded clays, water flows freely in a horizontal direction.
• Temperature. As the temperature rises by 0–90°, the water permeability of the soil increases. This process occurs most intensively at 65–75°C, which is associated with a decrease in the viscosity of water in the pores, the breakdown of colloidal bonds between liquid and solid components of the soil, the transition of bound water into free water, an increase in the number of soil aggregates (grains stuck together), and an increase in porosity.
• Hydrostatic head. Hydrostatic pressure of water on the ground occurs under the action of gravity. It is also created by artificial irrigation with a strong pressure jet.
The frequency of irrigation canal operation is one of the factors that affect water losses. Irrigation systems usually operate intermittently during the irrigation period and without water at all other times except during the irrigation period. These periods alternate during the 5–15 day irrigation period (Alimov, 2008, 2011). During summer, intense evaporation occurs from the surface of reservoirs and irrigation canals. Many factors influence evaporation, but the most crucial ones are the water surface temperature and air humidity at the water surface (Ghazaw, 2002; Alimov, 2012; Kolganov et al., 2022; Mukhamedjanovich et al., 2022). Both of these factors are affected by a number of other factors. For example, water temperature is influenced by the ambient air temperature, and heat transfer from air to water and back occurs through direct heat transfer without mixing and convection. As the temperature increases, the average velocity of the molecules also increases, which causes more high-velocity molecules to fly away from the surface. On the other hand, air humidity at the water surface is affected by the intensity of water evaporation, surface area, wind, or other types of convection in the air. When the evaporation rate increases, the surface temperature of the liquid decreases, which results in an increase in heat transfer between the surface and the water column, as well as an increase in the heat transfer between the water surface and the air, and the convection flow over the water.
It is recommended to install the improved design of an impervious cover for irrigation canals made of geocomposite material in inter-farm and on-farm canals of reclamation systems. This will not only reduce water losses during transport but also in the overall irrigation system. Additionally, it will contribute to the introduction of new irrigation areas.
In terms of the advantages and disadvantages of the geocomposite flume, it has been found that it can effectively reduce water leakage. However, the negative aspect is its price instability. The cost of one block of geocomposite flume with a length of 2 meters is approximately 110 euros. However, in the case of mass production, the cost may be lower. Studies have demonstrated that the flume has high reliability, durability, and practical use as an irrigation channel for land reclamation systems.
4. Conclusion
In conclusion, this paper delineates the fundamental principles and techniques for controlling irrigation water losses in reclamation systems in Kazakhstan, while also highlighting outstanding issues. The study has revealed the need to transition towards more robust, fail-safe, durable, and cost-effective modern methods for reducing leakage costs. In the field of water distribution management in inter-farm and internal canals of irrigation systems, there still exist several challenges that necessitate a systematic approach based on technical means to cover canals with impermeable coatings.
The study also demonstrated that when calculating seepage water from irrigation canals, losses from absorption and evaporation of water in permanent and intermittent canals increase linearly for soils with leakage coefficients ranging from 0.10 M/s to 0.30 M/s. However, losses in intermittent canals range from 0.142 M3/s to 0.557 M3/s or 33.7 to 40.1%, respectively. For soils with shrinkage coefficients up to M/s, the efficiency factors increase linearly from 0.07 to 0.28. As a mandatory modernization measure for intermittent irrigation systems, there should be a waterproof covering occupying at least 35% of the total canal length on heavy soils, at least 70% on medium soils, and at least 80% on light soils (including low-power soils). The use of an improved design of watertight covering of irrigation canals from composite polymer material, proposed for the arrangement of reclamation systems on inter-farm and on-farm canals, has enabled the reduction of water consumption from canals and the irrigation system as a whole, as well as the introduction of new irrigation plots.
The conducted studies have demonstrated that the geocomposite plastic flume is the most suitable option for the impermeable cover on inter-farm and on-farm canals of land reclamation systems. This is due to its strength, wear resistance, low weight, ease of installation and dismantling, economy, and long service life. These features have made fibreglass plastic trays highly demanded in modern conditions. Additionally, an important advantage of irrigation trays is that they can be customized to meet individual dimensions and configuration requirements to the maximum extent possible.
5. Recommendations
The deployment of advanced waterproof canal coverings, constructed from composite polymer materials, is proposed for application in both inter-farm and on-farm irrigation canals within reclamation systems. This strategy serves to mitigate water losses across the irrigation system, facilitating the activation of new irrigation sectors. The findings from this study lay the groundwork for comprehensive design, maintenance, and enhancement of existing irrigation infrastructures.
Integrating geocomposite polymer flumes can augment the efficacy of water use within the reclamation system, elevate the technical standard of irrigation networks, and enable the addition of further irrigation areas by boosting the efficiency quotient of the irrigation grid. Seepage costs are contingent on various factors including soil composition, canal length, operational frequency, and operational expenses of the canal. Often, these costs are influenced by the canal’s operational mode, its current state, and the seasonal usage, among other subordinated factors such as wind impact. Accordingly, future research endeavors should focus on refining the technical attributes of the polymer materials used in constructing irrigation flumes, enhancing their robustness and longevity. Moreover, effective management of water partitioning processes within irrigation systems and the incorporation of digital technologies are also suggested avenues of research.
Data availability statement
The original contributions presented in the study are included in the article/supplementary material, further inquiries can be directed to the corresponding authors.
Author contributions
YK, BN, ZK, and KZ: conceptualization. IS, TI, YI, and AY: methodology. IS and GI: software. YK, BN, YI, and IS: validation. YK, ZK, and TI: formal analysis. YK, GI, IS, and ZK: investigation. YK, TI, KZ, and LD: resources. YK, BN, YI, and KZ: data curation. YK: writing—original draft preparation. AY and KZ: writing—review and editing. YK, YI, KZ, and LD: visualization. YK, KZ, and LD: supervision, project administration, and funding acquisition. All authors contributed to the article and approved the submitted version.
Funding
The research was carried out within the framework of the BR10764920 project entitled Irrigation Technologies and Techniques During Putting New Irrigation Lands, Reconstruction and Modernization of Existing Irrigation Systems funded by the Ministry of Agriculture of the Republic of Kazakhstan and is based on the results of the Scientific technical project for 2021–2023.
Acknowledgments
This research was supported by the Hungarian University of Agriculture and Life Sciences and the Doctoral School of Economic and Regional Sciences (MATE), Hungary.
Conflict of interest
The authors declare that the research was conducted in the absence of any commercial or financial relationships that could be construed as a potential conflict of interest.
Publisher’s note
All claims expressed in this article are solely those of the authors and do not necessarily represent those of their affiliated organizations, or those of the publisher, the editors and the reviewers. Any product that may be evaluated in this article, or claim that may be made by its manufacturer, is not guaranteed or endorsed by the publisher.
References
Abd-Elaty, I., Pugliese, L., Bali, K. M., Grismer, M. E., and Eltarabily, M. G. (2022). Modelling the impact of lining and covering irrigation canals on underlying groundwater stores in the Nile Delta, Egypt. Hydrol. Process. 36:e14466. doi: 10.1002/hyp.14466
Abd-Elhamid, H. F., Said, A. M., Abdelaal, G. M., and Abd-Elaty, I. (2021). Impact of polluted open-drain geometry on groundwater contaminant in unconfined aquifers. Arab. J. Geosci. 14, 1–12. doi: 10.1007/s12517-021-06491-y
Abuzeid, T. S. (2021). Conveyance losses estimation for open channels in middle Egypt case study: Almanna main canal, and its branches. JES. J. Eng. Sci. 49, 64–84. doi: 10.21608/jesaun.2021.57454.1027
Aghvami, E., Abbaspour, A., Ghorbani, M. A., and Salmasi, F. (2013). Estimation of channels seepage using seep/w and evolutionary polynomial regression (EPR) modelling (case study: Qazvin and Isfahan channels). J. Civ. Eng. Urban. 3, 211–215. Available at: http://www.ojceu.ir/main/attachments/article/26/J.%20Civil%20Eng.%20Urban.,34-211-215.pdf
Alimov, A. G. (2008). Antifiltration protection of canals and reservoirs. Hydrotech. Constr., 36–41. Available at: https://elibrary.ru/item.asp?ysclid=lhau3g7z9j720195093&id=10437263
Alimov, A. G. (2011). Modern methods and methodology for studying the water permeability of impervious structures of hydraulic structures. Innov. Technol. Land Reclam., 482–491. Available at: https://elibrary.ru/item.asp?id=29125502&ysclid=lk1xt4a53u978060316
Alimov, A. G. (2012). Refinement of theoretical bases, methods, and methodologies for investigation of anti-filtration effectiveness of protective linings in canals and water bodies. Power Technol. Eng. 46, 276–282. doi: 10.1007/s10749-012-0345-6
Anakhaev, K. N., Amshokov, B. X., and Ishchenko, A. V. (2006). On filtration calculation of earthen dams with a core. Hydrotech. Constr. 5, 26–34. Available at: https://elibrary.ru/item.asp?id=9186123&ysclid=lk1y3ccmxo53235301
Arifjanov, A. M., Juraev, S. S., Maqsud Yusufovich, O., Kasimov Takhirjon, O., and Akhatov Davron, N. (2022). Conditions for the effective use of anti-filtration coating types in uncoated channels. Middle Eur. Sci. Bull. 29, 80–86. Available at: http://eprints.umsida.ac.id/id/eprint/10829
Baev, O. A., Kosichenko, Y. M., and Silchenko, V. F. (2022). Effect of subsoil moisture on filtration through a screen defect. Mag. Civ. Eng. 111:11109. doi: 10.34910/MCE.111.9
Blanco, M., Castillo, F., Soriano, J., Noval, A. M., Touze-Foltz, N., Pargada, L., et al. (2012). Comparative study of three different kinds of geomembranes (PVC-P, HDPE, EPDM) used in the waterproofing of reservoirs. Eurogeo 5:46. Available at: https://hal.science/hal-00763745/
Bocharnikov, V. S., and Borovikov, A. A. (2021). Efficiency of anti-filtration sand sapropel curtain on the example of the gateway-regulator of reclamation systems. Proceedings of nizhnevolzskiy agrouniversity complex: science and higher vocational education, 4, 463–471.
Bogushevsky, A. A., Golovanov, A. I., and Kutergin, V. A. (1981). Agricultural hydrotechnical meliorations. Kolos, Moscow, Russia. Available at: (https://search.rsl.ru/ru/record/01001052149?ysclid=lhaxptyu5a691948119).
Cazzuffi, D. (1987). The use of geomembranes in Italian dams. Int. Water Power Dam Constr. 24, 17–21. doi: 10.1016/0148-9062%2887%2990390-1
Chahar, B. R. (2007). Analysis of seepage from polygon channels. J. Hydraul. Eng. ASCE 133, 451–460. doi: 10.1061/(ASCE)0733-9429(2007)133:4(451)
Christian, S. S., and Trivedi, N. M. (2018). Seepage through canals-a review. Int. J. Res. Appl. Sci. Eng. Technol. 6, 865–867. doi: 10.22214/ijraset.2018.4146
Chuprin, I. A., and Cherednichenko, N. F. (1970). Bor'ba s poteriami orositel'noi vody na sistemakh. Moskva, Rossel’khozizdat.
Elkamhawy, E., Zelenakova, M., and Abd-Elaty, I. (2021). Numerical canal seepage loss evaluation for different lining and crack techniques in arid and semi-arid regions: a case study of the river Nile, Egypt. Water 13:3135. doi: 10.3390/w13213135
El-Molla, D. A., and El-Molla, M. A. (2021). Seepage losses from trapezoidal earth canals with an impervious layer under the bed. Water Pract. Technol. 16, 530–540. doi: 10.2166/wpt.2021.010
Garg, S. K. (2020). Irrigation engineering and hydraulic structures: water resources engineering, vol. II. Delhi, India: Khanna Publisher.
Ghazaw, Y. M. (2002). Design and analysis of a canal section for minimum water loss. Alex. Eng. J. 50, 337–344. doi: 10.1016/j.aej.2011.12.002
Giroud, J. P., Thiel, R. S., and Kavazanjian, E. (2004). Hydrated area of a bentonite layer encapsulated between two geomembranes. Geosynth. Int. 11, 330–354. doi: 10.1680/gein.2004.11.4.330
Kenshimov, A. (2019). Consulting services for the development of the industry qualifications framework and professional standards in the direction of the agro industrial complex. Project report 12. Available at: https://kazaral.org/wp-content/uploads/2020/01/%D0%9E%D0%A0%D0%9A-%D0%92%D0%A5-26.08.19%D0%B3.pdf
Kolganov, A. V., Kosichenko, Y. M., and Sklyarenko, E. O. (2022). Anti-filtration lining of channels using geosynthetic materials. Melioration Hydraul. Eng. 12, 210–226. doi: 10.31774/2712-9357-2022-12-3-210-226
Kosichenko, Y. M. (2012). Investigations of filtration control and operational reliability for earth hydraulic structures. Sci. J. Russ. Res. Inst. Land Reclam. Probl. 2, 86–94.
Kosichenko, Y. M., and Baev, O. A. (2014). Antifiltration coatings from geosynthetic materials. IP Belousov a. Yu., Novocherkassk, Russia, 239. Available at: https://elibrary.ru/item.asp?id=22259799&ysclid=lk1wvo3n3y928103979
Kosichenko, Y. M., and Baev, O. A. (2019). Multilayer anti-seepage liners based on bentonite mats: comparison of their effectiveness. Power Technol. Eng. 53, 288–293. doi: 10.1007/s10749-019-01073-1
Kosichenko, U. M., Baev, O. A., and Ischenko, A. V. (2014). Modern methods of controlling filtration in irrigation systems. Eng. Vestnik Don 3. Available at: https://elibrary.ru/item.asp?id=22843936&ysclid=lk1wttu1ga563049760
Message of Head of the State K.K. Tokayev to the people of Kazakhstan. (2022). Fair State. A united nation. Prosperous society. Available at: https://www.akorda.kz/ru/poslanie-glavy-gosudarstva-kasym-zhomarta-tokaeva-narodu-kazahstana-181130
Mukhamedjanovich, A. A., Sharipovich, J. S., Otakhanov, M. Y., Olimjanovich, K. T., and Nurmakhamatovich, A. D. (2022). Conditions for the effective use of anti-filtration coating types in uncoated channels. Middle Eur. Sci. Bull. 29, 80–86. Available at: https://cejsr.academicjournal.io/index.php/journal/article/view/1548
Nam, W., Hong, E., and Choi, J. (2016). Assessment of water delivery efficiency in irrigation canals using performance indicators. Irrig. Sci. 34, 129–143. doi: 10.1007/s00271-016-0488-6
Nauman, K. G., and Sergeyev, S. D. (1981). Experience of application of synthetic films for impervious lining of irrigation canals in Iraq. Land reclamation abroad. Moscow, Central Bureau of Scientific and Technical Information of Water Ministry of the USSR, 6.
Olgarenko, I. V. (2013). Information technologies of water use planning and operational management of water distribution in irrigation systems D. thesis for the degree of Doctor of Technical Sciences, 448 Available at: https://new-disser.ru/_avtoreferats/01007210128.pdf?ysclid=lk1ye4tvv6603931023
Prabakaran, P. A., Sathyamoorty, G. L., and Adhimayan, M. (2019). An experimental and comparative study on canal lining exploitation geo synthetic material, cement mortar and material lining. Int. J. Recent Technol. Eng. 7, 264–266.
Radchenko, V. G., and Semenkov, V. M. (1992). Use of geosynthetic materials in dam construction. Hydrotech. Constr. 26, 685–691. doi: 10.1007/BF01544774
Rau, A., Koibakova, Y., Nurlan, B., Nabiollina, M., Kurmanbek, Z., Issakov, Y., et al. (2023). Increase in productivity of chestnut soils on irrigated lands of northern and Central Kazakhstan. Land 12:672. doi: 10.3390/land12030672
Scuero, A., and Vaschetti, G. (2019). “Rehabilitation of canals with watertight geomembranes, in the dry and underwater” in Advances in geosynthetics engineering. Geo MEast 2018. Sustainable civil infrastructures. eds. M. Meguid, E. Guler, and J. Giroud (Cham: Springer)
Shtepa, B., Nosenko, V., and Vinnikova, N. (1990). Mechanization of irrigation. Handbook Agropromizdat, Moscow, Russia, 336. Available at: https://c.twirpx.one/file/1751286/?ysclid=lhaxua8lq7979932629
Sokolskaya, V. V. (1969). Durability of polymer film impervious screens. Hydraul. Eng. Constr. 11, 18–21. Available at: https://ozlib.com/982260/tehnika/spisok_ispolzovannyh_istochnikov?ysclid=lhax3rmgyh848818218
Solsky, S. V., Lopatina, M. G., and Orlova, N. L. (2015). Laboratory studies of geosynthetic materials for justification of their application in the structures of ground hydraulic structures. Proceedings of the all-Russian research Institute of Hydraulic Engineering, 276, 84–89. Available at: (https://elibrary.ru/item.asp?id=23754799&ysclid=lhaukd4kuh424899866).
SP 100.13330.2016. SNiP 2.06.03-85 Ameliorative systems and structures. (2023). Available at: https://docs.cntd.ru/document/456050590?ysclid=lhaxnxyhuo435870342
Stark, T. D., and Hynes, J. M. (2009). Geomembranes for canal lining. In Proceedings of geosynthetics, 54–64.
Useful model patent KZ. (2022). (ISO 3166-1)№ 7346 from 12. 08.2022. Available at: https://qazpatent.kz/ru/content/poleznaya-model-12082022
Keywords: permeability of soils, seepage, water loss, irrigation canals, impermeability activities, geocomposite irrigation flume
Citation: Kalybekova Y, Zhu K, Nurlan B, Seytassanov I, Ishangaliyev T, Yermek A, Ismailova G, Kurmanbek Z, Issakov Y and Dávid LD (2023) Minimizing seepage in irrigation canals in land reclamation systems via an innovative technology. Front. Sustain. Food Syst. 7:1223645. doi: 10.3389/fsufs.2023.1223645
Edited by:
Abdelazim Negm, Zagazig University, EgyptReviewed by:
Asaad Mater Armanuos, Tanta University, EgyptIsmail Abd-Elaty, Zagazig University, Egypt
Copyright © 2023 Kalybekova, Zhu, Nurlan, Seytassanov, Ishangaliyev, Yermek, Ismailova, Kurmanbek, Issakov and Dávid. This is an open-access article distributed under the terms of the Creative Commons Attribution License (CC BY). The use, distribution or reproduction in other forums is permitted, provided the original author(s) and the copyright owner(s) are credited and that the original publication in this journal is cited, in accordance with accepted academic practice. No use, distribution or reproduction is permitted which does not comply with these terms.
*Correspondence: Kai Zhu, aGl6aHVrYWlAMTYzLmNvbQ==; Lóránt Dénes Dávid, ZGF2aWQubG9yYW50LmRlbmVzQHVuaS1tYXRlLmh1
†These authors have contributed equally to this work