- 1Department of Soil, Crop and Climate Sciences, University of the Free State, Bloemfontein, South Africa
- 2Department of Agronomy, McCain Foods SA, Delmas, South Africa
- 3Department of Plant and Soil Sciences, University of Pretoria, Hatfield, South Africa
- 4Department of Agronomy, Stellenbosch University, Stellenbosch, South Africa
Precise water management is imperative for sustainable crop production under irrigation in semi-arid regions with increasingly variable rainfall. Water use efficiencies achieved by farmers often differ widely, even within regions with homogenous agro-ecological conditions. To better understand the drivers of this variability and derive management recommendations, detailed measurements of water and associated nutrient dynamics were conducted on 20 potato farms in two regions of South Africa. Crop characteristics and typical growing conditions in South Africa make potato cultivation sensitive to water and nutrient losses. Water and nutrient inputs and losses through drainage and plant uptake were measured in potato and the follow-up crop, along with yield and weather variables. Seasonal mean irrigation and evapotranspiration were lower in winter crops (240 mm irrigation, 236 mm evapotranspiration) than in summer crops (598 mm irrigation, 608 mm evapotranspiration). Seasonal field water balances were often close to zero, indicating that estimations of evapotranspiration and drainage were representative for the greater field. Lysimeter observations however appeared more representative in sandy unstructured soils than in heavier structured soils. Rainfall during cropping reduced demand for irrigation and increased water use efficiency based on irrigation water, but caused substantial drainage. Leaching of N and K was considerable (on average 30 kg N, 55 kg K ha−1, much higher in some fields), while P leaching was negligible, with the exception of one field (51 kg P ha−1). Variation in water and nutrients use efficiencies was the result of management decisions and environmental factors, such as rainfall and soil type. Without substantial rainfall, application of irrigation scheduling tools can keep drainage and nutrient losses to a minimum, as was observed on almost half the fields, even on sandy soils with a low water holding capacity. Potato yields greatly varied among farmers (9.1–24.7 t dry matter tuber ha−1). Efficient farmers included those who used water sparsely and had minimal drainage, but also farmers who used inputs judiciously and obtained excellent productivity. The role of follow-up crops as users of surplus nutrients and water left behind by the potato crop was not always evident.
1. Introduction
Irrigation is often regarded as a key tool to enhance productivity, and reduce production risks and food insecurity in Africa, which is experiencing an increasingly variable climate. Nevertheless, the development and adoption of irrigation practices have remained low in most of Africa (Mutambara et al., 2016; Nakawuka et al., 2018). An exception to this is South Africa, with 1.3 M ha of cultivated land under irrigation managed by mostly large-scale farmers. This represents only 9.6% of the total cultivated area (Backeberg, 2018), but takes up a large share of the total plant production in the country and is therefore essential for national and regional food security. As in other water-scarce regions of Africa, the availability of irrigation water is a key limitation to expanding the area under irrigation. Climate change and socio-economic developments will likely further constrain the availability and quality of water for agricultural use in future. In South Africa, irrigation for agricultural production is responsible for 62% of the total water consumption (Harding, 2015). Historical rainfall data for Southern Africa have shown a general trend of increasing variability and incidence of extreme events (Kruger and Nxumalo, 2017; McBride et al., 2022), and this trend is likely to continue in future as climate change intensifies, resulting in reduced capture and storage efficiency of rainfall. Moreover, with an increasing human population, economic development and continuing urbanization, water demand in urban areas is likely to increase in future at the expense of the share available to agriculture.
Potato (Solanum tuberosum) is the main vegetable crop of South Africa with over 80% of the potato area under irrigation (PSA, 2017). Potato is generally seen as a water efficient crop, however, it is drought sensitive and has a shallow root system (Haverkort, 2018). The dependence of potato production on irrigation water from surface water or boreholes makes farmers vulnerable to changes in water availability, due to climate change or the unsustainable use of water resources (Steyn et al., 2016). Potato production in southern Africa often takes place in warm climates on sandy, freely draining soils with a low water holding capacity, implying that frequent and precise irrigation scheduling is required to ensure a crop free of water stress, while avoiding drainage below the root zone of the crop. However, not all farmers use decision support tools for irrigation scheduling. Uncertainty in rainfall forecasts, with rainfall typically being of a convective nature, further complicates adjusting irrigation scheduling to weather forecasts.
Water and nutrient dynamics in irrigated potato are closely linked. Potato may have a poor recovery of nutrients due to its shallow root system (Milroy et al., 2019b; Gondwe et al., 2020). On freely draining soils, mobile nutrients such as N and K easily accumulate below the rooted zone and gradually leach to deeper sub-soil layers and eventually enter sub-surface aquifers (Alva et al., 2010; Zotarelli et al., 2015), which are in contact with surface waters. Phosphorus is relatively immobile in soils, and P losses by surface erosion and run-off represent the main non-point source of pollution (Nyiraneza et al., 2021). Elevated nutrient concentrations in surface waters cause eutrophication which has become a serious problem in many catchments, with 60% of South African rivers under threat from eutrophication, and 25% being critically endangered (Harding, 2015). Potato is typically followed by one or two other crops, whereafter the land is left fallow for 4–7 years and extensively grazed by livestock (Franke et al., 2011). This wide rotation helps to curb pests and diseases in potato production systems and is enabled by the abundance of land. The type of follow-up crops and their management are expected to impact the fate of water and nutrients left behind in the soil after the potato harvest (Liang et al., 2019).
For irrigated crop production in dry areas to be sustainable, production practices should move toward optimization of water and nutrient usage, minimizing leaching risk and maximizing water and nutrient use efficiencies (Ierna et al., 2011; Hendricks et al., 2014). A survey among potato growers in South Africa highlighted large differences between farmers in water and nutrient use efficiencies of potato over multiple years, even within relatively homogenous agro-ecological zones (Steyn et al., 2016). This highlights the importance of crop management in determining the ecological sustainability and economic performance of potato growers. To explain and learn from differences in water and nutrient use efficiencies between farms, actual water and nutrient input rates, and losses from potato-based systems need to be quantified through detailed in-field measurements.
This study was designed to investigate three objectives aimed at improving water and nutrient management in irrigated potato-based production systems in a semi-arid climate: firstly, to quantify water and nutrient inputs, outputs, and use efficiencies of irrigated potato; secondly, to quantify deep drainage and nutrient losses through leaching; and thirdly, to assess the role of follow-up crops in reducing nutrient losses.
2. Methodology
2.1. Site description
Twenty potato fields under irrigation were intensively monitored on commercial farms in two potato production regions of South Africa: (1) the interior of South Africa covering North West Province and the very western part of the Free State Province, which is also referred to as the western summer cropping area, and (2) the Sandveld region in the Western Cape Province (Table 1; Figure 1). Fields were located in the vicinity of the villages of Christiana (the western Free State, two fields), Vryburg (North West, six fields) and Piketberg (the Sandveld, 12 fields). The climate of the interior is characterized by warm to hot, humid summers and dry, cold winters when regular frost inhibits potato cultivation (Köppen-Geiger classification: BSk). Potato is typically planted between late August and early February in this region. Mean annual rainfall is 507 mm in Christiana and 445 mm in Vryburg, occurring mostly between October and April. The sites are 1,200–1,360 meters above sea level (masl). In the western Free State, the Vaal River is the prime source of irrigation water, while in the Vryburg area, farmers rely on sub-surface water from boreholes. The Sandveld, a coastal region north of Cape Town, has a Mediterranean climate with hot dry summers and mild humid winters (Köppen-Geiger classification: Csb). Temperatures allow year-round production of potato in this largely frost-free region where mean annual rainfall ranges from 150 to 300 mm. Farmers have two broad main planting seasons: March–May (referred to as a winter crop in this article) and June–November (referred to as a summer crop). Farmers in this region use sub-surface water fed from an inland catchment area (the Cederberg Mountain range) for irrigation. The sites were located at 8–220 masl.
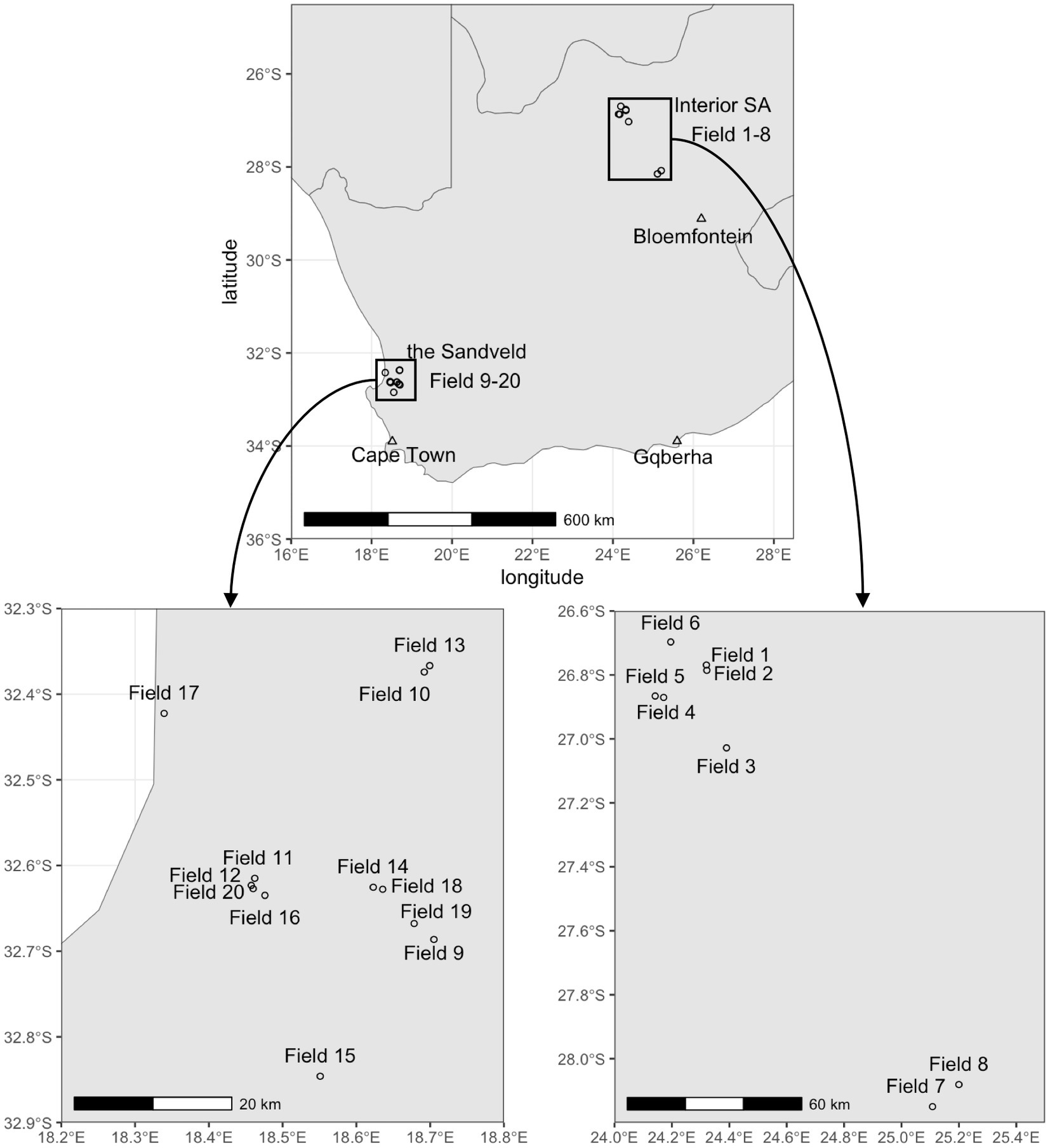
Figure 1. Map of the interior and western parts of South Africa with the location of the trial sites indicated.
2.2. Crop rotations and management
The research team did not prescribe a particular form of management or crop choices and farmers were free to manage their crops in their usual ways. All farmers cultivated potatoes using center-pivot irrigation systems. Potato varieties Mondial, Sifra, Lanorma and FL2108 were planted (Table 1). Pasture grass (Anthephora pubescens), maize (Zea mays), groundnuts (Arachis hypogaea), onions (Allium cepa), and paprika pepper (Capsicum annuum) were planted after potato in the interior (Table 1). In the Sandveld, a cover crop like oats is typically planted after potato harvest with minimal inputs to curb wind erosion. Thereafter the field is typically left uncropped until the next potato crop, as irrigation water in this region is too scarce to grow annual crops other than potato. No data were collected from the cover crops in the Sandveld.
Nutrient inputs in potato primarily came from inorganic fertilizers applied through broadcasting, band placement, fertigation and spraying from a tractor. Nutrients naturally present in irrigation water also represented a considerable source in some situations. Most follow-up crops received nutrients through fertilizer application and irrigation water as well.
In these regions potatoes are typically planted in tram rows on beds, but the configuration varied slightly between farms. The two rows on a bed were spaced 60–75 cm apart and the distance between the centers of beds was 1.8–2.0 m. Seed tuber size ranged between 80 and 120 g each and were spaced ±25 cm apart within the row, giving a plant population of about 44,000 plants ha−1. Seed beds were 20–30 cm in height and tuber planting depth was 15–20 cm.
2.3. Field measurements
2.3.1. Soil
Composite soil samples (composed of 12 sub-samples per depth per field) were taken at 0–0.3 m, 0.3–0.6 m, and 0.6–0.9 m depth before planting and analyzed for chemical and physical characteristics. Soil samples were analyzed by the Agricultural Research Council—Small Grain Institute, Bethlehem, South Africa (samples from the interior) and by SGS Woodmead, South Africa (samples from the Sandveld) to determine pH (KCl), extractable P (Bray-1), exchangeable cations (ammonium acetate extraction), soil texture (bouyoucous hydrometer) and organic carbon (dry combustion using an elemental analyzer). Soil texture was analyzed in the Sandveld for one field only.
2.3.2. Precipitation and irrigation
Irrigation scheduling practices were not prescribed to farmers. They therefore managed irrigation according to their existing practices, including use of soil capacitance probes, evapotranspiration data (ETo) or from experience. The actual water supply to crops (rain and irrigation) was measured using tipping bucket rain gages installed within fields above the crop canopy. In addition, irrigation amounts were recorded using volumetric flow meters or a pressure transducer and logger connected to the central irrigation pipe. The pressure transducer measured the time the irrigation pivot was running. This, combined with a once-off measurement of the flow rate using a transit time ultrasonic flow meter, allowed continuous monitoring of irrigation rates (Rajita and Mandal, 2016).
2.3.3. Water drainage and nutrient leaching
Drainage was measured through drain gage lysimeters (DG G3 - Decagon Devices, United States) installed in potato fields around the time of crop emergence when soil operations were completed. Lysimeters stayed in the field until potato, or the follow-up crop was harvested. The drain gauges were filled with an undisturbed soil core and represented drainage below 1 m depth. The drained water was assumed inaccessible to the potato crop, as potato typically does not produce roots below 1 m depth. Drainage water was extracted from the gauges every 2–3 weeks. The volume of the extracted leachate was measured and then compared to readings recorded by a depth sensor, which monitored drainage continuously. The leachate was analyzed for nitrogen, phosphorus and potassium concentrations. Irrigation water was also sampled once during the season and analyzed for the same nutrients. No lysimeter was installed in Field 5, as a hard chalk layer at 1 m depth prohibited its installation. It was unlikely any substantial deep drainage occurred in this field due to this impenetrable layer. No lysimeters were also installed in Fields 4, 9, 15, and 17 due to a shortage of equipment (Table 1).
2.3.4. Crop parameters
Tuber and aboveground biomass were measured through destructive sampling around final harvest. In the Sandveld and North West, two rows of 10 m of crop were harvested in the vicinity of the lysimeter. In the western Free State, four plots of 1.8 × 3.0 m were harvested per field. The dry matter content of the roots, stems, leaves and tubers was determined from representative sub-samples that were oven-dried at 70°C to constant weight. Dried sub-samples were also analyzed for nutrient content using the ICP perchloric acid digestion method. Plant nutrient uptake was determined from the nutrient concentration of the analyzed sub-samples.
2.3.5. Weather data
Weather data were recorded using automatic weather stations installed in or near the fields. The weather stations recorded air temperature, relative humidity, solar radiation, and wind speed. To represent the Western Free State, data were obtained from a weather station at Jan Kempdorp (27o 57′ 27.36”S 24o 50′ 23.64″E), managed by the Agricultural Research Council. In North West, automatic weather stations were installed in the vicinity of Fields 1 and 2. In the Sandveld, automatic weather stations were installed nearby Fields 10, 15, 18, and 17.
2.4. Data handling and analyses
Nutrient use efficiencies were expressed as the amount of yield produced for a certain amount of N, P, or K applied:
is the nutrient (N, P, or K) use efficiency (kg kg−1); the dry matter tuber yield (kg ha−1); and the total amount of nutrient (N, P, or K) applied (kg ha−1).
Nutrient uptake efficiency was calculated as the ratio of the total amount of nutrient (N, P, or K) taken up by the plant to the amount of the nutrient applied;
is the nutrient (N, P, or K) uptake efficiency; and the nutrient (N, P, or K) plant uptake (kg ha−1).
Total nutrient inputs in potato were obtained by summing up the nutrients supplied through fertilizers and via irrigation water. The indigenous nutrient supply by the soil was not quantified. Nutrient removal was calculated by totaling the nutrients removed in harvested plant components (potato tubers, paprika pods, onion bulbs, groundnuts, and maize grain) and those lost through deep drainage. Partial nutrient balances in the potato crops were calculated as the difference between nutrient inputs and the losses of nutrients through harvest of tubers (excluding aboveground plant parts) and leaching.
Nutrients in the non-harvested potato parts remaining in the field were assumed to be available for uptake by the follow-up crop. Thus, the total nutrient input in the follow-up crop was calculated as the sum of the nutrients in the potato residues and those applied to the follow-up crop through fertilizer and irrigation water. Nutrient losses in the follow-up crop included nutrients leached and those removed through harvested plant parts. Nutrient leaching rates were calculated from the volume and nutrient concentration of leachate extracted from the drain gauge.
Weather data were used to estimate crop evapotranspiration (ETC) in potato from ETO using the Penman Monteith equation (Allen et al., 1998), assuming a KC value of 0.3 at emergence, linearly increasing to 1.1 at full soil cover, and thereafter linearly decreasing to 0.9 at crop end (Kadam et al., 2021; Machakaire et al., 2021; Francisco Gonzalez et al., 2023). Full soil cover was assumed to be reached when 650 degree days with a base temperature of 2°C had passed from emergence (Haverkort et al., 2015). Daily and seasonal water dynamics (irrigation, precipitation, drainage, and evapotranspiration) in potato were analyzed from emergence. Irrigation (measured from the central irrigation pipe) was assumed to be applied with an efficiency of 83% (Machakaire et al., 2021). Fields typically receive around 150 mm of irrigation water between first soil cultivation and emergence, and this was not included in the water balances, as measurements started from emergence. Water use efficiencies (kg ha−1 mm−1) were calculated as the dry matter tuber yield (kg ha−1) divided by the amount of water applied (mm) as irrigation water only (WUEirri) or as irrigation water and rainfall (WUEirri + rain). Water use efficiencies represented efficiencies over the entire crop cultivation period, including the assumption that 150 mm of irrigation water was applied pre-emergence in all fields.
A correlation matrix was constructed using non-parametric Spearman correlation to determine the relationships between input variables, use efficiencies and potato tuber yield. To facilitate data presentation, the sites were categorized into three distinct potato cropping systems: crops in the interior (8 sites, all growing in summer), Sandveld—summer crops (6 fields) and Sandveld—winter crops (6 fields). Differences in crop parameters between the three systems were analyzed using a linear model, and differences between means were assessed using Tukey’s range test. Letters were used in tables and in Figure 2 to indicate significantly different means using a confidence level of 5%. Yields of maize grain is presented at 13% moisture content, while yields of all other crops are presented as dry yield. R Studio (Version 2023.03.0) was used for data analyses. Also Figure 1 was constructed with the help of R Studio (ggspatial package).
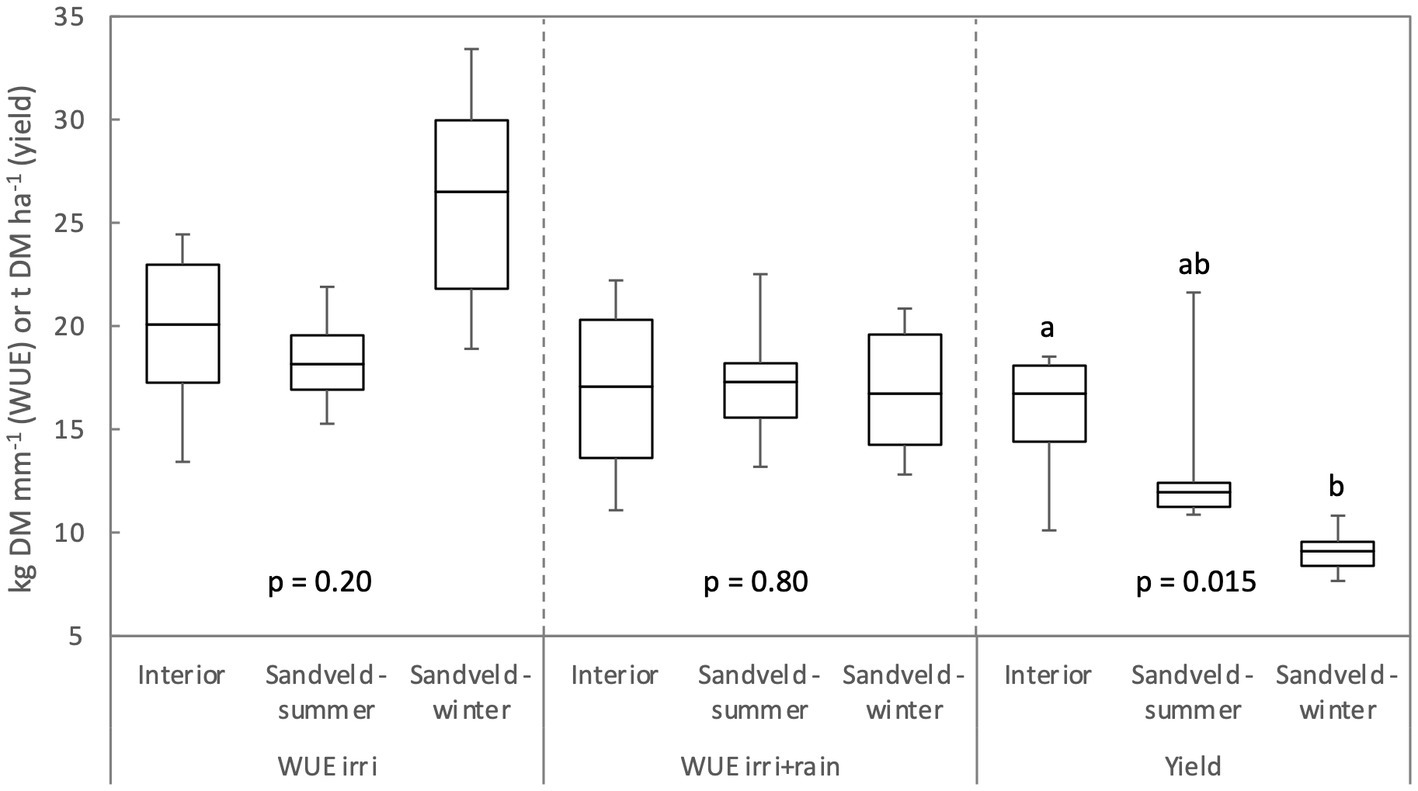
Figure 2. Box plots of water use efficiencies (WUE) based on irrigation water only and on irrigation and rainfall (kg tuber DM mm−1 water), and of dry matter (DM) tuber yields (t DM ha−1) per system. The whiskers indicate the 5th and 95th percentile.
3. Results
3.1. Soil and weather characteristics
Soils at the monitored sites were generally sandy with a very low organic carbon (C) content, making them vulnerable to nutrient leaching (Table 2). Although soil texture was not assessed for all fields in the Sandveld, soils in this region tend to be very sandy with a silt and clay content below 5%. The pH of soils in the interior was neutral, while the Sandveld had slightly acidic soils. A large variability in soil available P between fields within regions (data not shown) was likely caused by differences in historical P fertilizer applications.
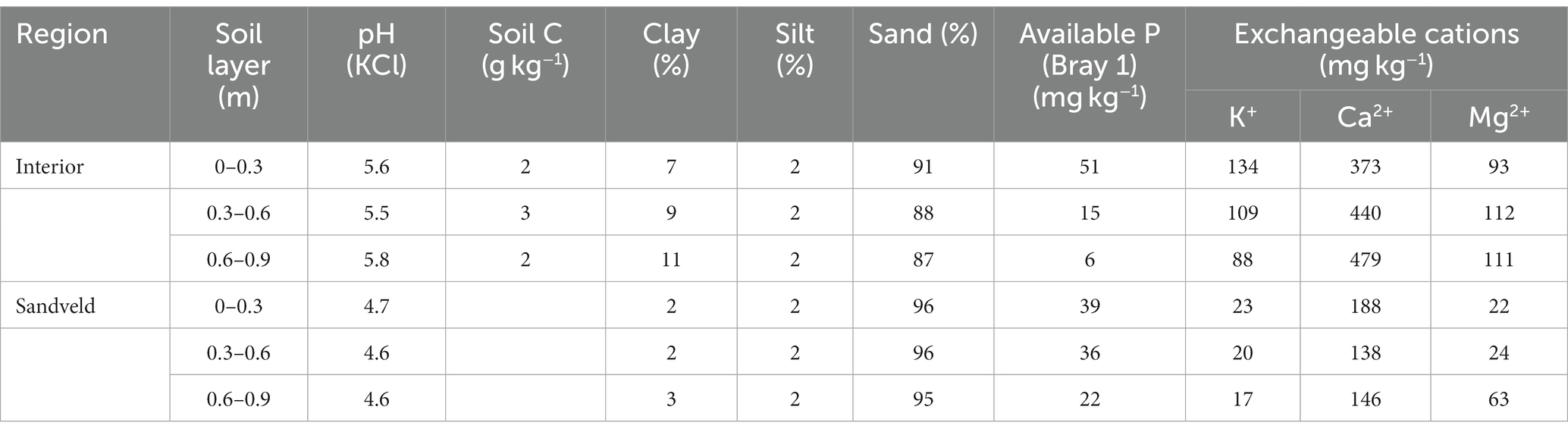
Table 2. Soil physical and chemical properties at the monitored sites: pH, soil C, fraction clay, silt and sand, available P and exchangeable cations (means per region).
Temperatures were warmer and radiation was more intense during potato cultivation in the interior, compared to the summer crops in the Sandveld (Table 3). This is related to the moderating impact of the ocean on temperatures in the coastal region of the Sandveld, as well as the wide planting window of potatoes in the Sandveld, with some crops categorized as summer crops not growing in the middle of the summer. Temperatures and solar radiation were considerably lower in winter in the Sandveld, as expected.
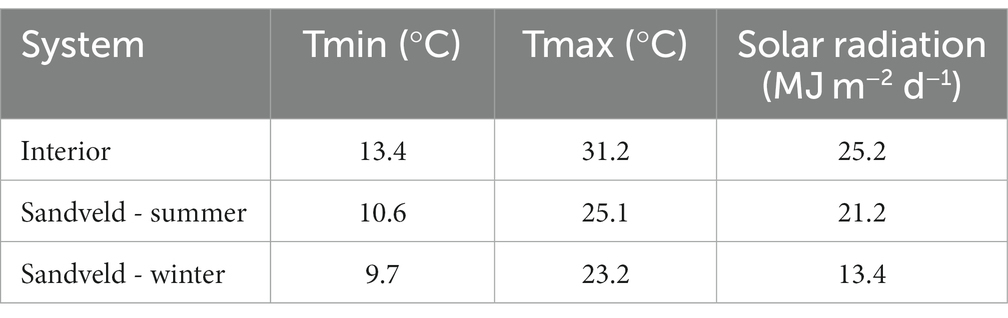
Table 3. Mean minimum and maximum day temperature and total solar radiation from potato emergence to harvest (means per region).
3.2. Seasonal water dynamics
Potato crops in the interior received the highest water inputs (800 mm on average), mostly as irrigation water, as they were predominantly planted in spring (Aug-Sep), growing into the hot summer with little rainfall experienced in spring and early summer in the relevant seasons (Tables 3, 4). Only Fields 7 and 8, planted in November and harvested in February, experienced substantial rainfall. The summer crops of the Sandveld received more irrigation water (526 mm) and less rainfall (93 mm) than the winter crops (240 mm irrigation and 180 mm rain) (Tables 3, 4). Evapotranspiration was greatest in the interior (723 mm) due to hot temperatures during the growing season, while the summer crop of the Sandveld transpired significantly more (493 mm) than the winter crop (236 mm), as expected (Tables 3, 4).

Table 4. Accumulated water inputs into the soil through irrigation (I) and rainfall (R), losses through evapotranspiration (ET) and deep drainage (D), and the soil water balance in potato crops from emergence to harvest (means per region and range in brackets) (mm).
In four fields (of the 15 fields with drain gauges), all located in the interior, no drainage was observed during potato cropping (Table 4). Three fields showed minimal leaching (<20 mm over the cropping period), while substantial leaching (>70 mm) was recorded in the other fields. Drainage rates were generally higher in the Sandveld than in the interior due to the very sandy nature and low water holding capacity of soils in the Sandveld. Soil water balances in the Sandveld tended to be close to zero, indicating that estimated inputs of water into the soil from irrigation and rainfall matched the estimated losses through evapotranspiration and drainage, with the exception of Field 13 having a positive water balance of 96 mm (Table 4). While the mean soil water balances of fields in the interior were also close to zero, the water balance of individual fields varied considerably.
The water use efficiency of potato grown in the Sandveld in winter based on irrigation amounts only (on average 24.0 kg mm−1) was higher than in the other systems, as substantial rainfall supplemented the irrigation inputs. Water demand in the Sandveld in winter was lower than in the other systems, due to low evapotranspiration, but yields (on average 9.1 t DM tuber ha−1) were generally also lower in winter (Figure 2). The mean water use efficiency based on both irrigation water and rainfall did not significantly differ between the systems, but a large variability in water use efficiency could be observed between fields in the same system, with the most efficient fields having a water use efficiency that was approximately double that of the least efficient fields (Figure 2). The performance of the individual fields is discussed in more detail in Section 3.5.
3.3. Seasonal nutrient dynamics and use efficiencies
While N application rates to potato were high (typically around 300 kg N ha−1), uptake rates were also high, leading to uptake efficiencies often close to 1 and occasionally above 1 (Table 5). In the Sandveld, N use efficiencies and uptake efficiencies were lower than in the interior. Uptake efficiencies of K were often above 1, indicating that the crop took up more K than supplied through fertilizer and irrigation water, with the difference presumably supplied by the soil. In the Sandveld, K use and uptake efficiencies were lower than in the interior. The very sandy nature of soils and the high application rates of N and K in the Sandveld were likely responsible for the low use and uptake efficiencies of N and K. Nitrogen and K leaching was variable (Table 5) with 6 out of 14 fields having no or low leaching rates (<10 kg ha−1), with four fields showing high leaching rates (>100 kg N or K ha−1). Nitrogen and K leaching rates were closely associated with each other (r = 0.89, p < 0.001). As N and K move through the soil profile dissolved in water, the amount of drainage explained a great deal of the variation in N and K leaching between fields (r = 0.85 and 0.90 for N and K, p < 0.001). The field balances of N and K were often negative in the interior, indicating that more N and K were removed through harvested tubers and drainage than applied through fertilizer and irrigation water during potato cultivation, with the difference in N and K presumably supplied by the soil. In the Sandveld, N and K balances were more often positive, despite higher leaching rates.
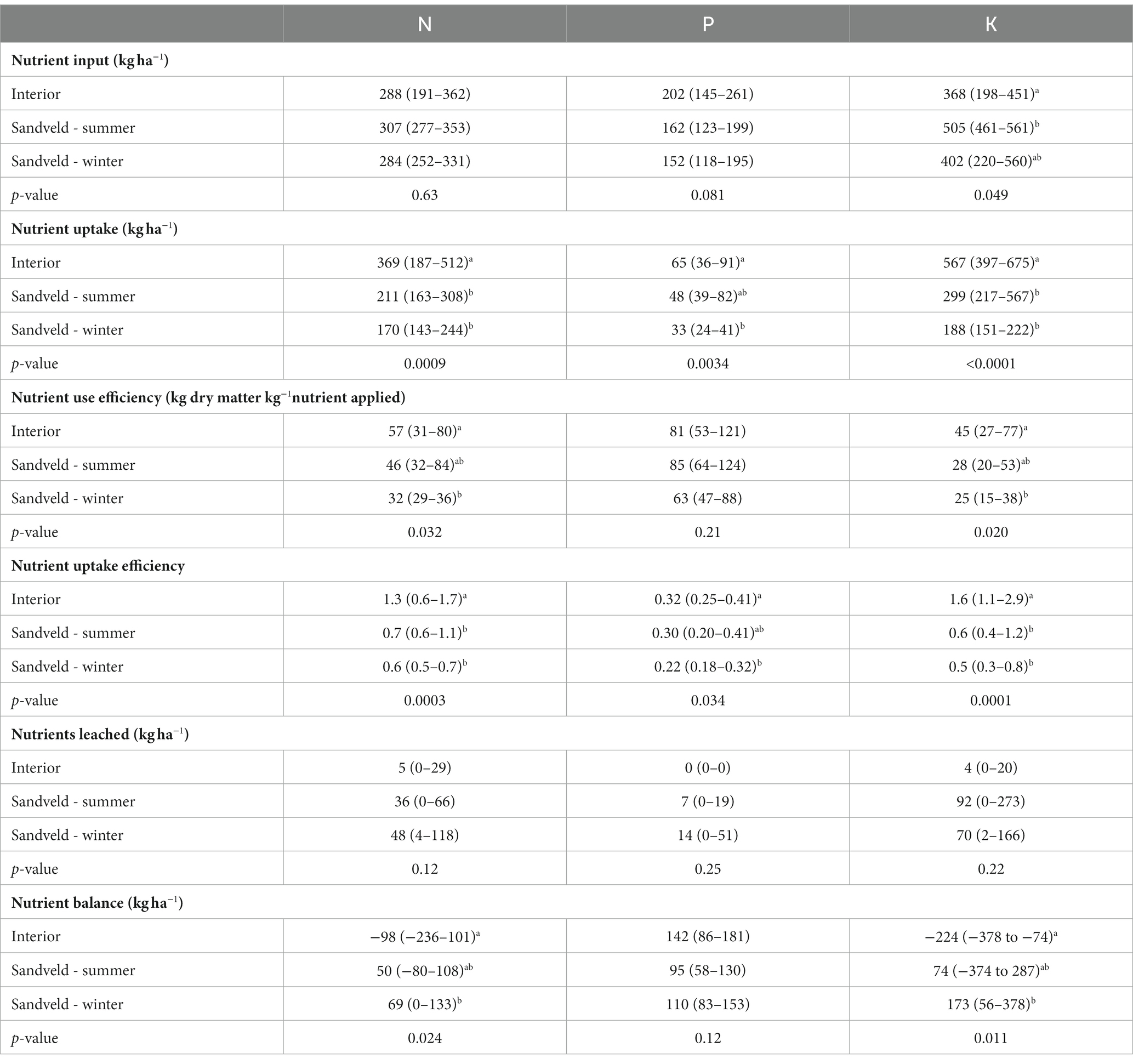
Table 5. The input, uptake, use efficiency, uptake efficiency, leaching, and nutrient balance (inputs—removal through harvest and leaching) of N, P, and K in potato in three systems (means and range in brackets).
Phosphate application rates were high (145–261 kg P ha−1) (Table 5), despite high soil P reserves (Table 2). Phosphorus uptake was generally well below the inputs, as may be expected given the low plant availability of fertilizer P, with the uptake efficiencies varying between 0.18 and 0.41. Phosphorus leaching was minimal in the interior, while substantial P leaching of 19 and 51 kg ha−1 was recorded for Fields 20 and 11, respectively, in the Sandveld. The P balance was positive in all fields, implying more P was added to the fields than removed through harvest and leaching.
3.4. Potato yield
The yield of potato crops growing in winter in the Sandveld was relatively low (Figure 2) due to reduced solar radiation (on average 13.4 MJ m−2 d−1) and perhaps cool temperatures hindering crop development during the growing season (Table 3). A modeling study also indicated that the potential yield in the Sandveld in a winter crop is considerably lower than in a summer crop (Franke et al., 2011). While reduced fertilizer rates in a winter crop (Table 5) in theory may have further contributed to lower yields of a winter crop, it is likely that farmers adjusted fertilizer rates to the lower yield potential in this season. The relatively low nutrient use efficiencies and positive nutrient balances achieved in winter crops in the Sandveld (Table 5) also suggest that nutrient supply was unlikely to limit yield. One field in the Sandveld (Field 19, summer crop) gave an exceptionally high yield of 24.7 t tuber DM ha−1, on a small pivot though (2.3 ha). Mean yields in the interior were somewhat higher than in the Sandveld, which may be related to more intense solar radiation received by crops in the interior (on average 25.2 MJ m−2 d−1) (Table 3).
A strong positive correlation was found between yield and total radiation received during the growing season (r = 0.73, p = 0.0003). This reflects the fact that yields of crops growing in summer tended to be considerably higher than that of winter crops if farmers can avoid the worst heat stress. Although P application rates may seem rather high (Table 5), P input rate was positively correlated with yield (r = 0.51, p = 0.02) suggesting that high P application rates relieved growth limitations, whereas no associations were observed between N and K application rates and yield. Yield did correlate with soil exchangeable K (r = 0.65, p = 0.0038) but not with other soil characteristics. Since soil characteristics and weather conditions were confounded, it is difficult to draw general conclusions from this.
Strong positive correlations were observed between nutrient use/uptake efficiencies and yield (r > 0.64; p < 0.002) and a weaker correlation between water use efficiency and yield (r = 0.47, p = 0.04). Use efficiencies were also strongly, positively correlated among each other. Variation in use and uptake efficiencies was presumably driven by yield. High yielding crops tended to be the most efficient crops and crops that were efficient with one resource tended to be efficient with other resources as well. Only in case of K, its application rate was negatively correlated with the K use efficiency (r = −0.71, p = 0.0005) and K uptake efficiency (r = −0.74; p = 0.0002), indicating that high application rates were associated with low use efficiencies. In case of N and P, no relation was found between input rates and use/uptake efficiencies.
3.5. Daily water dynamics
High drainage observed in Field 1 (486 mm over the season) was strongly driven by one specific event on 23 November 2018 when the irrigation system had a breakdown due to a power failure and got stuck in the vicinity of the lysimeter, resulting in 123 mm of drainage recorded in the next 2 days (Figure 3A). The soil in this field consisted of coarse unstructured sand with a low water holding capacity, resulting in substantial drainage during the rest of the growing season as well, especially toward the end of the crop cycle when the gap between water inputs and evapotranspiration widened. Nutrient leaching was nevertheless limited in this crop, probably because the farmer applied modest amounts of N and K on a weekly basis (21 kg N and 28 kg K ha−1 2–7 weeks after planting, 28 kg N and 38 kg K ha−1 8–9 weeks after planting) through fertigation, apparently balancing nutrient supply with crop demand.
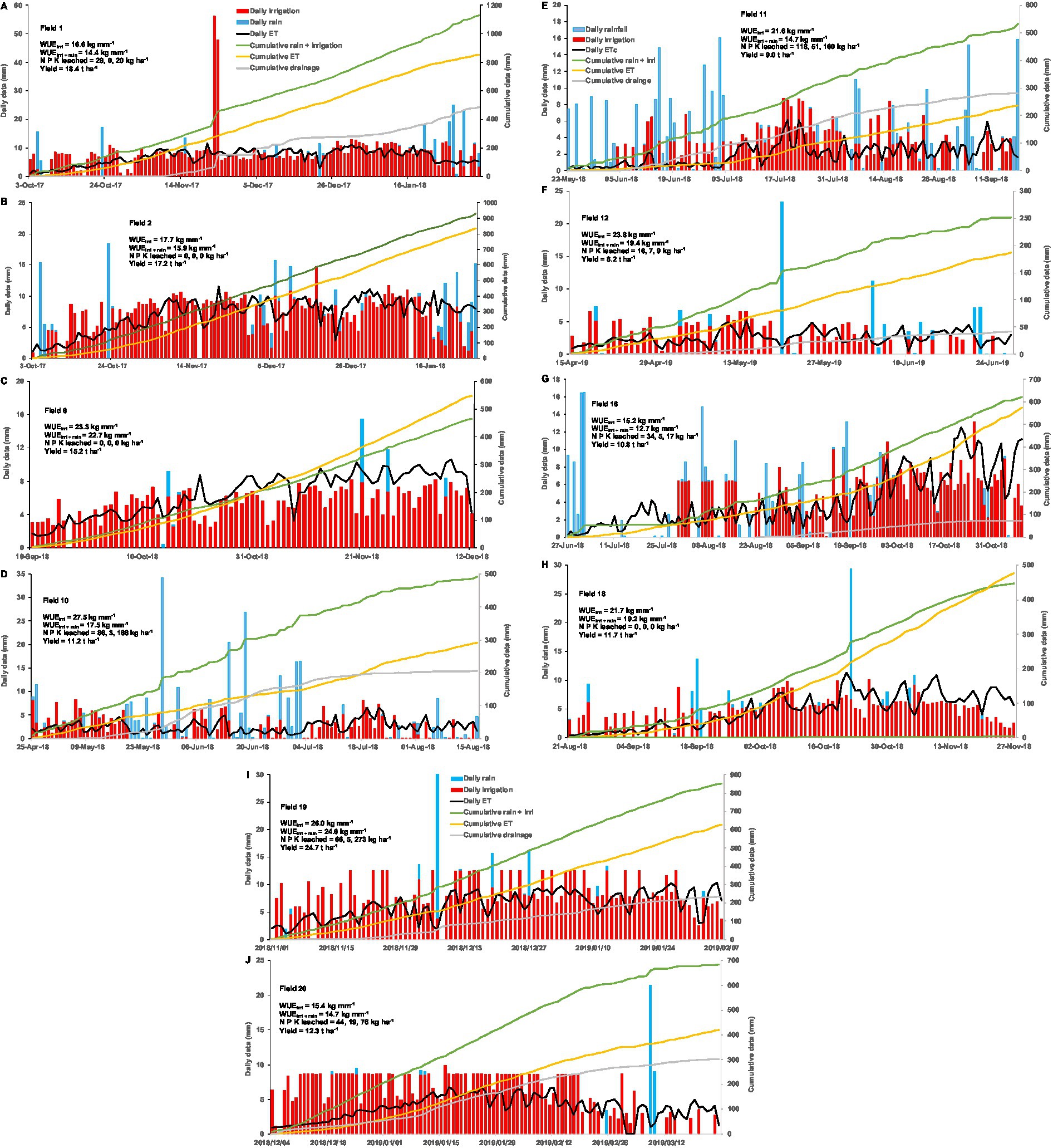
Figure 3. Daily rain, irrigation and evapotranspiration (ET), and cumulative rain and irrigation, evapotranspiration and drainage in potato from crop emergence until crop end for (A) Field 1, (B) Field 2, (C) Field 6, (D) Field 10, (E) Field 11, (F) Field 12, (G) Field 16, (H) Field 18, (I) Field 19, and (J) Field 20.
Fields 2 (Figure 3B) and 3 (daily data not given) both had a crumbly chalk layer at 0.8–1.0 m depth, impeding but not blocking water flows. This explains the lack of drainage during potato cultivation, despite water inputs being greater (by 98 and 103 mm in Field 2 and 3 respectively) than losses through evapotranspiration. In the 8-month period between potato harvest and planting of the follow-up crop in Field 2, 226 mm of drainage was recorded due to substantial rainfall in this period.
In Fields 4 and 5, no lysimeters were installed. In Field 4, a hard chalk layer at 1 m depth inhibited the installation of a lysimeter, and probably also blocked all drainage. In both Fields 4 and 5, total water inputs (721 and 835 mm) over the season were well balanced with the estimated evapotranspiration (744 and 852 mm). In Field 6, water inputs into the soil from irrigation and minimal rainfall were slightly below the estimated evapotranspiration, especially later in the growing season (Figure 3C). As a result, no drainage was observed in this freely draining, sandy soil profile. In line with this, it was observed that the crop suffered from mild drought stress toward the end of the growing season. The crop nevertheless provided a good yield, resulting in a high water use efficiency for this field (Figure 3C).
Fields 7 and 8 (daily data not given) were both located in the western Free State and had 105 mm and 99 mm greater water inputs as rainfall and irrigation than losses through evapotranspiration over the season. Drainage over the season was however minimal (16 mm and 0 mm in Fields 7 and 8, respectively). Soils in Fields 7 and 8 were more prone to crusting due to a slightly higher silt content and substantial run-off was observed after heavy rain showers in both fields. Run-off was, however, not formally recorded in this study. As a result, soil water balances did not match well for these two fields.
Fields 9, 15, and 17 in the Sandveld had no lysimeters installed (Table 1). Potato in Field 10 (winter crop in the Sandveld) only received 258 mm of irrigation over the season (Figure 3D). However due to substantial rainfall (233 mm) between 19 May and 2 July 2018, water inputs exceeded the low evapotranspiration rates in winter, and substantial drainage (205 mm) and associated leaching of N and K were recorded for this field (Figure 3D). Field 10 achieved a high water use efficiency based on irrigation only, but an average water use efficiency based on both irrigation and rainfall. In Field 11, a pattern similar to Field 10 could be observed where precipitation and some over-irrigation in the middle of the season, combined with low evapotranspiration rates in winter, resulted in substantial drainage (Figure 3E). Also in this field, substantial leaching of N and K was observed. This field also had a high P leaching rate (51 kg P ha−1), perhaps due to P fertilizer added through fertigation. As the rate of irrigation often exceeded that of evapotranspiration in Field 11, the water use efficiency based on both irrigation water and rainfall was relatively low in this field (Figure 3E).
Less rainfall occurred in the 2019 winter season in the Sandveld and therefore in Field 12, water inputs were more in balance with evapotranspiration (Figure 3F). Drainage in this field was limited to 44 mm over the season. Although yield was relatively low in this field harboring a winter crop, water use efficiencies were high due to the sparse use of irrigation water (Figure 3F). In Field 13, soil water input through irrigation and rainfall (303 mm over the season) exceeded the estimated evapotranspiration (196 mm) by 107 mm (daily data not shown). Drainage in this field was only 9 mm. This apparent discrepancy is related to dry soil conditions after emergence due to a breakdown of the irrigation system and a substantial amount of water had to be added to the soil to replenish the soil profile.
In Fields 16 and 18 (crops growing into summer), irrigation rates were well aligned with the crop’s evapotranspiration rates, with some rainfall in Field 16 and almost no rainfall in Field 18 (Figures 3G,H). Some drainage was recorded in Field 16, while almost no drainage occurred in Field 18. In Field 18, the subsoil was dry at planting and the soil profile only gradually refilled after some rain during the season. In Field 19 and 20 on the other hand, irrigation water was applied at rates well above the evapotranspiration rates of the crop and substantial drainage of 233 and 302 mm was observed (Figures 3I,J). The farmers managing Field 19 and 20 applied fixed daily irrigation rates. Especially in Field 20 irrigation was carried out with little consideration of soil moisture conditions. Field 20 was located close to the ocean and due to strong winds, regular irrigations were applied to limit sand blasting of plants. Moreover, the actual application rate of the irrigation system was higher than assumed by the farmer. The rates of nutrients leached over the season were high in both fields. The yield achieved in Field 19 was greater than the yield in Field 20 (Figures 3I,J). As a result, Field 19 achieved high water use efficiencies (despite irrigation rates that were often well above evapotranspiration rates), whereas Field 20 did not.
3.6. Follow-up crops
3.6.1. Nutrient input and uptake
In Field 1, bottlebrush grass was planted as a pasture crop in autumn, 2 months after the potato harvest (Table 6). The pasture was exclusively rainfed and did not receive any nutrient applications until the end of the measurements 14 months after planting (Table 7). The pasture was grazed a few months after seeding in the dry winter. The grass only recovered from grazing and managed to cover the soil in the following summer. As a result of the grazing, nutrient removal in this crop could not be determined. Substantial drainage occurred in the following summer (Table 8), though with little leaching of nutrients.

Table 6. Characteristics of follow-up crops: fallow period refers to the period between potato harvest and planting of the follow up crop.
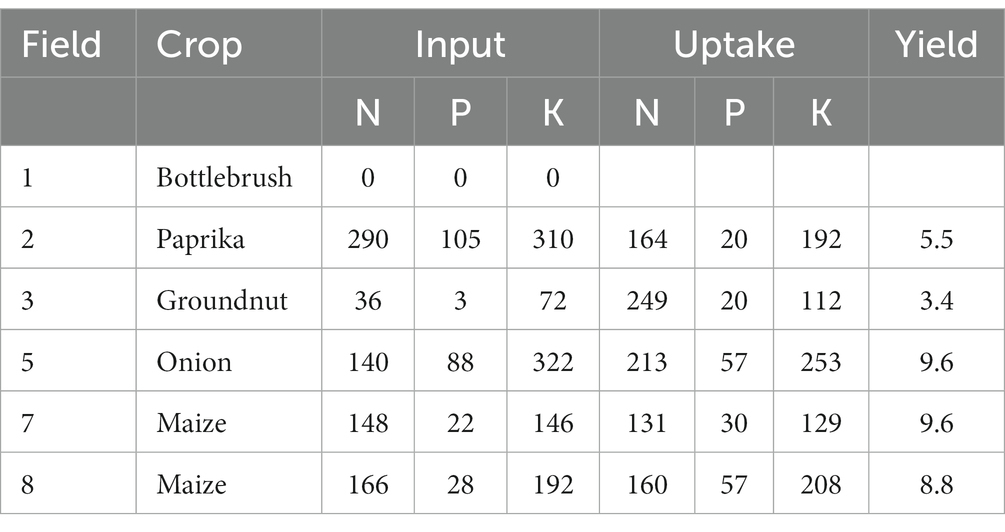
Table 7. Nutrient input application (kg ha−1), uptake (kg ha−1), and harvestable yield (t DM ha−1) in follow-up crops.
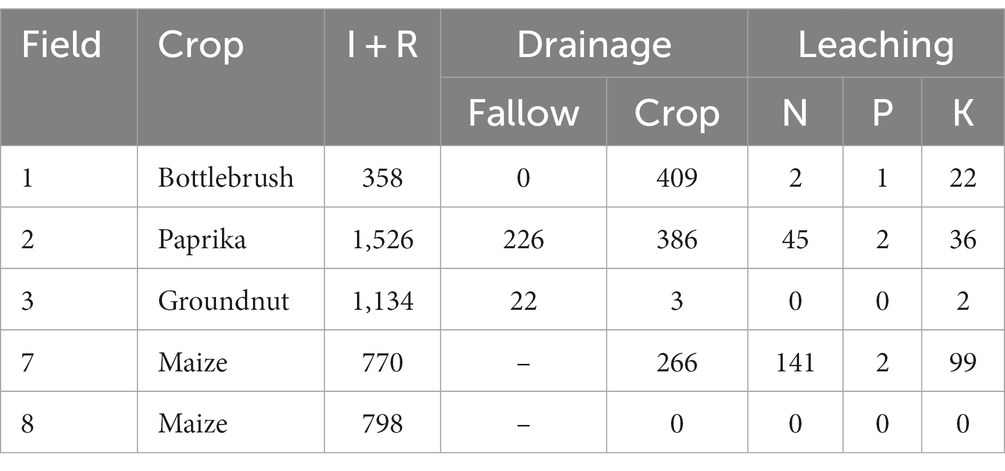
Table 8. Cumulative irrigation (I) and rainfall (R) (mm), drainage (mm) in the fallow period between potato harvest and planting of the next crop and in the follow-up crop, and N, P, K leaching rates in follow up crops (kg ha−1).
In Field 2, substantial drainage (226 mm) occurred when the field was left fallow after the potato harvest due to heavy rains. Paprika and onion on Fields 2 and 5 respectively, are both high-value crops and received substantial nutrient inputs through fertilizer (Table 7). While the uptake of nutrients by paprika was considerably less than what was supplied, nutrient losses through leaching during paprika crop growth were limited (Tables 7, 8). The input and uptake of nutrients in onion in Field 5 were reasonably balanced (Table 7).
The groundnut crop in Field 3 was not fertilized and took up more nutrients than supplied as inputs (Table 7). Apart from the nitrogen fixed by this legume crop, groundnut presumably largely relied on nutrients left behind in the soil by the previous potato crop. As drainage was minimal in this field (Table 8), nutrient losses through leaching were also minimal.
The irrigated maize crops following potato in Fields 7 and 8 were modestly fertilized and the uptake of N and K was well in line with the supply, while more P was taken up than applied (Table 7). Field 7 recorded substantial drainage and nutrient leaching (Table 8) toward crop end from 3 to 16 February 2017. This was partly due to 72 mm of rainfall received in the period 4–15 February. While maize with its deep rooting system has a good potential to act as a crop utilizing the nutrients left behind by the shallowly rooting, well fertilized potato crop, the monitored maize crops did not evidently fulfill this role.
4. Discussion
Given the technical challenges to measure directly deep drainage in cropped fields under commercial management, this study provided unique and detailed insights into soil water and nutrient dynamics of irrigated fields. As it requires major efforts to monitor water and nutrient dynamics throughout a season, the study is inevitably somewhat of an anecdotal nature. However, sufficient fields were monitored to elucidate larger trends.
The daily data indicated that in most fields, drainage followed the difference between water inputs from rain and irrigation and water losses through evapotranspiration, though often with a delay in time. At crop harvest, the balances between soil water inputs and water losses through evapotranspiration and drainage were close to zero in most cases, with the difference presumably accounted for by changes in moisture in the soil profile. These results indicate that evapotranspiration estimated from ETo and a crop coefficient dependent on crop stage served as a good indicator of the actual evapotranspiration by the crop (Nyawade et al., 2021). Most commercial farmers have access to daily weather data from nearby weather stations that allow for the estimation of ETo. The method of estimating evapotranspiration applied in this study can be easily used by farmers to estimate crop water demand and irrigation needs to complement or replace information from soil moisture probes. This approach may be further refined in future by using a crop coefficient informed by Normalised Difference Vegetation Index (NDVI) values obtained from satellite imagery (French et al., 2020).
In the interior, where soils tended to be higher in clay content and more structured, the discrepancies between water inputs and outputs over the season were often greater than in the Sandveld. Seasonal water balances close to zero observed in the Sandveld suggest that drainage measurements through the type of portable lysimeters used in this study were likely representative for the greater field in these unstructured sandy soils. Also Yost et al. (2019) observed little spatial variation in drainage for potato grown on a sandy soil. The more structured soils of the interior may lead to preferential flow paths, reducing the representativeness of the lysimeter measurements. Moreover, the occurrence of run-off in Fields 7 and 8 (not formally assessed) added to the discrepancies between soil water inputs and outputs in the interior.
Drainage occurred following rainfall or irrigation rates exceeding crop evapotranspiration rates, especially in very sandy soils (the Sandveld) with low water holding capacity. In situations where little rainfall occurred and irrigation rates were in balance with evapotranspiration rates, hardly any drainage was observed. No drainage was recorded on almost half the fields, indicating that many farmers manage their irrigation well in accordance with the crop’s needs. Appropriate irrigation scheduling tools to balance irrigation rates with crop demands are available to farmers, and these tools are increasingly refined by integrating remotely sensed data into irrigation recommendations. Forecasts of convective rainfall are however inherently uncertain and therefore of limited value to adapt irrigation rates. The low water holding capacity of sandy soils leaves little room for error and catch up if anticipated rainfall is not realized. However, planting potato with a drier sub-soil which may then be refilled by excess rain as the season progresses in an option to reduce drainage, as was observed in Field 13. In the interior, field measurements took place in a relatively dry cycle of growing seasons. In seasons with more rainfall, more drainage may occur. In the Sandveld, cropping in the dry summer offers better possibilities to avoid rainfall and associated drainage than in winter. Observed drainage rates however were not significantly different between summer and winter crops due to over-irrigation in summer and water use efficiencies based on irrigation water were higher in the winter crops. It is concluded that optimizing productivity per ha and minimizing drainage and nutrient losses are more feasible in a summer season receiving minimal rainfall and high radiation, while for optimizing the use efficiency of scarce irrigation water, a growing season with rain is preferable (Franke et al., 2011). Some of the highest water and nutrient use efficiencies were observed in Field 19, despite substantial over-irrigation and drainage, due to a very high yield achieved in this field. This points to yield being an important factor driving resource use efficiency, besides water management. Water and nutrient efficient farmers may be those who use water sparsely (e.g., Field 6), or farmers who use inputs judiciously and obtain excellent productivity (e.g., Field 19).
While there is a common perception that commercial potato farmers over-apply nutrients, the observed N and K uptake efficiencies in the interior were often above 1, indicating that more N and K were taken up by the crop than supplied. Given that land is usually left fallow and undisturbed in dry climates for several years before potato is grown on it again, extensive soil cultivations prior to potato planting along with irrigation likely led to a burst of microbial activity, and the associated breakdown of organic carbon and mineralization of nutrients (Shakoor et al., 2022). This was less likely the case in the Sandveld, where sandy soils with very low carbon contents and nutrient holding capacity have a poor inherent ability to supply nutrients. Nutrient use and uptake efficiencies in the Sandveld were lower than in the interior, while N and K application rates were higher in the Sandveld summer crops than in the interior. The nutrient use efficiencies in potato observed in this study were well below the values observed in Zimbabwe (97–162 g potato g−1 N) (Svubure et al., 2015), presumably due to lower input rates used in Zimbabwe and a stronger reliance on soil nutrient supply. Nutrient input use and uptake observed in this study were comparable with that of high-input farmers in other parts of the world (Vos, 1996; Alva et al., 2010; Milroy et al., 2019a).
Although drainage and leaching rates of N and K were closely associated with each other and substantial leaching rates of N and K were recorded in some fields, there are options to reduce the susceptibility of applied nutrients to leaching. Frequent irrigation in potato offers the possibility to fertigate the crop with regular small doses of mobile nutrients such as N and K in line with crop demand, reducing the amount of nutrients available for leaching (Ahmad and Sharma, 2023; da Silva et al., 2023). Field 1 provided an example of ‘spoon feeding’ a crop, leading to low nutrient leaching despite high drainages rates. Other options to reduce nutrient leaching on sandy soils that has not been widely tested yet in South Africa include the use of slow-release fertilizers and of super adsorbent polymers. Further research is still required to evaluate products to ensure that nutrient release closely matches plant growth requirements in different environments, and assess the cost-effectiveness of products (Duan et al., 2023; Pan et al., 2023; Zheng et al., 2023). Although P leaching rates were generally low, the observed leaching of 51 kg P ha−1 (Field 11) does indicate a risk of P leaching in case P is applied through fertigation. The results suggest that N leaching from irrigated cropped fields may contribute considerably to eutrophication of surface waters in the area, while the impacts from P leaching may be limited (Mararakanye et al., 2022).
The role of follow-up crops as users of surplus nutrients and water left behind by the potato crop was less obvious than anticipated, although only six follow-up crops were monitored, and the evidence therefore remained anecdotal. Only groundnut in Field 3 took up substantially more nutrients than supplied, presumably relying on nutrients remaining from the potato crop. In the other crops, nutrient supply to the crop exceeded in most cases nutrient uptake. Moreover, during the fallow period between potato harvest and the planting of a follow-up crop, rainfall can easily lead to drainage and the loss of nutrients (e.g., in Field 2). From that perspective, planting a cover crop or a pasture grass such as bottlebrush grass soon after potato harvest may be a good approach to minimize drainage and nutrient leaching and provide soil coverage (Neumann et al., 2012).
5. Conclusion
• Passive drainage lysimeters were successfully applied to estimate drainage and nutrient leaching in cropped fields, but their point observations were more representative for cropped fields in sandy unstructured soils than in heavier structured soils.
• While rainfall during the cropping season reduced the demand for irrigation water and therefore increased the water use efficiency of potato production based on irrigation water only, rainfall is a major cause of drainage and nitrogen and potassium leaching on sandy soils. The low water holding capacity of the soil and unpredictable nature of convective rainfall make it difficult for farmers to anticipate rainfall from forecasts by reducing irrigation rates.
• The observed N and K leaching in deep drainage water was substantial. It is likely that N leaching from irrigated cropped fields forms a considerable contribution to N pollution of sub-surface and surface waters. Substantial leaching of P was observed in one field (51 kg P ha−1), but in all other fields P leaching was minimal and unlikely to provide any major contribution to eutrophication of surface waters.
• Variation in use efficiencies of water and nutrients in potato cultivation was the result of a combination of management factors and environmental factors such as rainfall and soil type that are out of the farmers’ control. Without substantial rainfall, the use of irrigation scheduling tools can keep drainage and nutrient losses to a minimum, even on very sandy soils with a low water holding capacity. With irrigation water becoming increasingly scarce in future due to climate change and socio-economic developments, the development and use of state-of-the-art irrigation scheduling tools are essential for the sustainability of irrigated crop production.
Data availability statement
The raw data supporting the conclusions of this article will be made available by the authors, without undue reservation.
Author contributions
AF and JS were responsible for the conceptualization and original design of the study. AF, ATBM, AM, MK, PS, and JS contributed to the implementation of field work, data analyses, and writing up of results. All authors contributed to the article and approved the submitted version.
Funding
This research was financially supported by Potatoes South Africa, PepsiCo, Yara Fertilizer International, Yara Africa Fertilizer (Pty) Ltd. and the Water Research Commission of South Africa (WRC Project No. K5/2501//4). We gratefully acknowledge the assistance and support of the farmers on whose farms this research was performed.
Conflict of interest
ATBM was employed by McCain Foods South Africa.
The remaining authors declare that the research was conducted in the absence of any commercial or financial relationships that could be construed as a potential conflict of interest.
Publisher’s note
All claims expressed in this article are solely those of the authors and do not necessarily represent those of their affiliated organizations, or those of the publisher, the editors and the reviewers. Any product that may be evaluated in this article, or claim that may be made by its manufacturer, is not guaranteed or endorsed by the publisher.
References
Ahmad, U., and Sharma, L. (2023). A review of best management practices for potato crop using precision agricultural technologies. Smart Agricult. Technol. 4:100220. doi: 10.1016/j.atech.2023.100220
Allen, R. G., Pereira, L. S., Raes, D., and Smith, M.. (1998). Crop evaporation. FAO paper no. 56, FAO, Rome.
Alva, A. K., Marcos, J., Stockle, C., Reddy, V. R., and Timlin, D. (2010). A crop simulation model for predicting yield and fate of nitrogen in irrigated potato rotation cropping system. J. Crop Improv. 24, 142–152. doi: 10.1080/15427520903581239
Backeberg, G. (2018). Estimating irrigated area and water use from remote sensing data. Pretoria, South Africa: Grain SA.
da Silva, A. L. B. R., Zotarelli, L., Dukes, M. D., van Santen, E., and Asseng, S. (2023). Nitrogen fertilizer rate and timing of application for potato under different irrigation methods. Agric. Water Manag. 283:108312. doi: 10.1016/j.agwat.2023.108312
Duan, Q., Jiang, S., Chen, F., Li, Z., Ma, L., Song, Y., et al. (2023). Fabrication, evaluation methodologies and models of slow-release fertilizers: a review. Ind. Crop. Prod. 192:116075. doi: 10.1016/j.indcrop.2022.116075
Francisco Gonzalez, T., Pavek, M. J., Holden, Z. J., and Garza, R. (2023). Evaluating potato evapotranspiration and crop coefficients in the Columbia Basin of Washington state. Agric. Water Manag. 286:108371. doi: 10.1016/j.agwat.2023.108371
Franke, A. C., Steyn, J. M., Ranger, K. S., and Haverkort, A. J. (2011). Developing environmental principles, criteria, indicators and norms for potato production in South Africa through field surveys and modelling. Agric. Syst. 104, 297–306. doi: 10.1016/j.agsy.2010.12.001
French, A. N., Hunsaker, D. J., Sanchez, C. A., Saber, M., Gonzalez, J. R., and Anderson, R. (2020). Satellite-based NDVI crop coefficients and evapotranspiration with eddy covariance validation for multiple durum wheat fields in the US southwest. Agric. Water Manag. 239:106266. doi: 10.1016/j.agwat.2020.106266
Gondwe, R. L., Kinosihita, R., Suminoe, T., Aiuchi, D., Palta, J. P., and Tani, M. (2020). Available soil nutrients and NPK application impacts on yield, quality and nutrient composition of potatoes growing during the main season in Japan. Am. J. Potato Res. 97, 234–245. doi: 10.1007/s12230-020-09776-2
Harding, W. R. (2015). Living with eutrophication in South Africa: a review of realities and challenges. Trans. R. Soc. South Africa 70, 155–171. doi: 10.1080/0035919X.2015.1014878
Haverkort, A. J. (2018). Potato handbook-crop of the future. Den Haag, The Netherlands: Potato World.
Haverkort, A. J., Franke, A. C., Steyn, J. M., Pronk, A. A., Caldiz, D. O., and Kooman, P. L. (2015). A robust potato model: LINTUL-POTATO-DSS. Potato Res. 58, 313–327. doi: 10.1007/s11540-015-9303-7
Hendricks, G. S., Shukla, S., Obreza, T. A., and Harris, W. G. (2014). Measurement and modelling of phosphorous transport in shallow groundwater environments. J. Contam. Hydrol. 164, 125–137. doi: 10.1016/j.jconhyd.2014.05.003
Ierna, A., Pandino, G., Lombardo, S., and Mauromicale, G. (2011). Tuber yield, water and fertilizer productivity in early potato as affected by a combination of irrigation and fertilization. Agric. Water Manag. 101, 35–41. doi: 10.1016/j.agwat.2011.08.024
Kadam, S. A., Gorantiwar, S. D., Mandre, N. P., and Tale, D. P. (2021). Crop coefficient for potato crop evapotranspiration estimation by field water balance method in semi-arid region, Maharashtra, India. Potato Res. 64, 421–433. doi: 10.1007/s11540-020-09484-8
Kruger, A. C., and Nxumalo, M. P. (2017). Historical rainfall trends in South Africa: 1921-2015. Water SA 43, 285–297. doi: 10.4314/wsa.v43i2.12
Liang, K., Jiang, Y., Nyiraneza, J., Fuller, K., Murnaghan, D., and Meng, F.-R. (2019). Nitrogen dynamics and leaching potential under conventional and alternative potato rotations in Atlantic Cananda. Field Crop Res. 242:107603. doi: 10.1016/j.fcr.2019.107603
Machakaire, A. T. B., Steyn, J. M., and Franke, A. C. (2021). Assessing evapotranspiration and crop coefficients of potato in a semi-arid climate using Eddy covariance techniques. Agric. Water Manag. 255:107029. doi: 10.1016/j.agwat.2021.107029
Mararakanye, N., Le Roux, J. J., and Franke, A. C. (2022). Long-term water quality assessments under changing land use in a large semi-arid catchment in South Africa. Sci. Total Environ. 818:151670. doi: 10.1016/j.scitotenv.2021.151670
McBride, C. M., Kruger, A. C., and Dyson, L. (2022). Changes in extreme daily rainfall characteristics in South Africa: 1921-2020. Weather Climate Extremes 38:100517. doi: 10.1016/j.wace.2022.100517
Milroy, S. P., Wang, P., and Sadras, V. O. (2019a). Defining upper limits of nitrogen uptake and nitrogen use efficiency of potato in response to crop N supply. Field Crop Res. 239, 38–46. doi: 10.1016/j.fcr.2019.05.011
Milroy, S. P., Wang, P., Sadras, V. O., Nurmanov, Y. T., Chernenok, V. G., Kuzdanova, R. S., et al. (2019b). Potato in response to nitrogen nutrition regime and nitrogen fertilization. Field Crop Res. 231, 115–121. doi: 10.1016/j.fcr.2018.11.014
Mutambara, S., Darkoh, M. B. K., and Atlhopheng, J. R. (2016). A comparative review of water management sustainability challenges in smallholder irrigation schemes in Africa and Asia. Agric. Water Manag. 171, 63–72. doi: 10.1016/j.agwat.2016.03.010
Nakawuka, P., Langan, S., Schmitter, P., and Barron, J. (2018). A review of trends, constraints and opportunities of smallholder irrigation in East Africa. Glob. Food Sec. 17, 196–212. doi: 10.1016/j.gfs.2017.10.003
Neumann, A., Torstensson, G., and Aronsson, H. (2012). Nitrogen and phosphorus losses from potatoes with different harvest times and following crops. Field Crop Res. 133, 130–138. doi: 10.1016/j.fcr.2012.03.011
Nyawade, S., Gitari, H. I., Karanja, N. N., Gachene, C. K. K., Schulte-Geldermann, E., and Parker, M. L. (2021). Yield and evapotranspiration characteristics of potat-legume intercropping simulated using a dual coefficient approach in a tropical highland. Field Crop Res. 274:108327. doi: 10.1016/j.fcr.2021.108327
Nyiraneza, J., Gilles, B., Fuller, K., and Hann, S. (2021). Critical phosphorus dilution curve and the phosphorus-nitrogen relationship in potato. Eur. J. Agron. 123:126205. doi: 10.1016/j.eja.2020.126205
Pan, Z., Fan, D., Jiang, R., Abbasi, N., Song, D., Zou, G., et al. (2023). Improving potato productivity and mitigating nitrogen losses using enhanced-efficiency fertilizers: a global meta-analysis. Agric. Ecosyst. Environ. 348:108416. doi: 10.1016/j.agee.2023.108416
PSA (2017). The south African potato industry in perspective. Potatoes South Africa, Pretoria, pp. 1–18.
Rajita, G., and Mandal, N. (2016). Review on Transit time Ultrasonic Flowmeter. 2016 2nd International Conference on Control, Instrumentation, Energy & Communication (CIEC), Kolkata, India.
Shakoor, A., Bosch-Serra, A. D., Lidon, A., Ginestar, D., and Boixadera, J. (2022). Soil nitrogen dynamics in fallow periods in a rainfed semiarid Mediterranean system. Pedosphere 33, 622–637. doi: 10.1016/j.pedsph.2022.06.051
Steyn, J. M., Franke, A. C., van der Waals, J. E., and Haverkort, A. J. (2016). Resource use efficiencies as indicators of ecological sustainability in potato production: a south African case study. Field Crop Res. 199, 136–149. doi: 10.1016/j.fcr.2016.09.020
Svubure, O., Struik, P. C., Haverkort, A. J., and Steyn, J. M. (2015). Yield gap analysis and resource footprints of Irish potato production systems in Zimbabwe. Field Crop Res. 178, 77–90. doi: 10.1016/j.fcr.2015.04.002
Vos, J. (1996). Input and offtake of nitrogen, phosphorus and potassium in cropping systems with potato as a main crop and sugar beet and spring wheat as subsidiary crops. Eur. J. Agron. 5, 105–114. doi: 10.1016/S1161-0301(96)02013-8
Yost, J. L., Huang, J., and Hartemink, A. E. (2019). Spatial-temporal analysis of soil water storage and deep drainage under irrigated potatoes in the Central Sands of Wisconsin, USA. Agric. Water Manag. 217, 226–235. doi: 10.1016/j.agwat.2019.02.045
Zheng, H., Mei, P., Wang, W., Yin, Y., Li, H., Zheng, M., et al. (2023). Effects of super adsorbent polymer on crop yield, water productivity and soil properties: a global meta-analysis. Agric. Water Manag. 282:108290. doi: 10.1016/j.agwat.2023.108290
Keywords: crop rotation, drainage, evapotranspiration, irrigation, lysimeter, resource use efficiency, South Africa
Citation: Franke AC, Machakaire ATB, Mukiibi A, Kayes MJ, Swanepoel PA and Steyn JM (2023) In-field assessment of the variability in water and nutrient use efficiency among potato farmers in a semi-arid climate. Front. Sustain. Food Syst. 7:1222870. doi: 10.3389/fsufs.2023.1222870
Edited by:
Patricia Amankwaa-Yeboah, CSIR Crops Research Institute, GhanaReviewed by:
Koffi Djaman, New Mexico State University, United StatesZhang Yucui, Chinese Academy of Sciences (CAS), China
Copyright © 2023 Franke, Machakaire, Mukiibi, Kayes, Swanepoel and Steyn. This is an open-access article distributed under the terms of the Creative Commons Attribution License (CC BY). The use, distribution or reproduction in other forums is permitted, provided the original author(s) and the copyright owner(s) are credited and that the original publication in this journal is cited, in accordance with accepted academic practice. No use, distribution or reproduction is permitted which does not comply with these terms.
*Correspondence: A. C. Franke, ZnJhbmtlYWNAdWZzLmFjLnph