- 1Innovative Agricultural Machinery and Post-harvest Technology Research Cluster, Mahasarakham University, Maha Sarakham, Thailand
- 2Drying Technology Research Unit, Faculty of Engineering, Mahasarakham University, Maha Sarakham, Thailand
- 3National Center for Genetic Engineering and Biotechnology (BIOTEC), National Science and Technology Development Agency, Pathum Thani, Thailand
- 4Department of Biology, Institut Teknologi Sepuluh Nopember, Surabaya, Indonesia
Holy basil (Ocimum Tenuiflorum L.) contains several bioactive compounds useful to the pharmaceutical and food industries. Microwave drying (MD) is a powerful technique for rapid drying of food or plant materials while preserving bioactive compounds during the process. However, little is known about the optimal combination of MD power with hot air drying (HAD) that can preserve the quality and yet only consume reasonable energy when drying holy basils. For that purpose, the effects of drying methods using MD combined with HAD at 45°C were examined to prevent losses in quality, antioxidant activities, and volatile flavor compounds in two holy basil cultivars (green and red cultivars). Holy basil leaves were dried at different MD powers of 200, 400, and 600 W combined with HAD and compared with a traditional tray drying (TD) at 45°C. Drying using MD at 600 W with HAD displayed significantly high levels of color retention, chlorophyll, and carotenoid content in both cultivars. The green cultivar showed a greater accumulation of total phenolic compounds (TPC), terpenoids, and DPPH free radical scavenging at 400 W with HAD. However, the red cultivar had the highest TPC, flavonoid, and terpenoid content at 600 W with HAD. The accumulation of major volatile organic compounds (VOCs) was also affected, and treatment at 600 W exhibited the highest methyl eugenol and β-caryophyllene content in both cultivars. The use of the highest power of MD (600 W) with HAD for leaf drying reduced the effective drying time and energy consumption among both cultivars. Taking into consideration the dried quality of antioxidant accumulation and energy consumed for drying, we recommend using MD at 400 or 600 W with HAD for the green cultivar and 600 W for the red.
Introduction
The genus Ocimum is a member of the family Lamiaceae, comprising approximately 68 species indigenous to tropical regions of Asia, Africa, and Central and South America (Singh and Chaudhuri, 2018). Holy basil, Ocimum sanctum Linn. synonym Ocimum tenuiflorum L., is the most important species of the genera due to its medicinal, perfumery, religious, ceremonial, cuisine, and essential oil uses (Nandkarni, 1982). This aromatic shrub is commonly identified as two cultivars, namely, the green holy basil with green leaves and red holy basil with purple leaves (Darrah, 1974; Vani et al., 2009). Holy basil is reported to exhibit antidiabetic, wound-healing, antioxidant, radiation protective, immunomodulatory, antifertility, anti-inflammatory, antimicrobial, antistress, and anticancer activities (Godhwani et al., 1988; Kelm et al., 2000; Vats et al., 2004; Yanpallewar et al., 2004; Mukherjee et al., 2005; Singh et al., 2005; Gupta et al., 2006; Salles Trevisan et al., 2006). The aroma compounds of holy basil essential oil (methyl eugenol chemotype, 56.18%) have been identified by solid phase microextraction (SPME)/GC–MS/flame ionization detection (FID) and olfactoric evaluations. The spicy-green-notes of essential oil are due to methyl eugenol, β-caryophyllene oxide, and germacrene D, while spicy-peppery-notes correspond to germacrene D (Jirovetz et al., 2003). Moreover, the major pharmacological activities of holy basil essential oil, such as mosquitocidal, antimicrobial, and anthelmintic, were found to be mediated by its marker constituent, eugenol (Kelm and Nair, 1998; Asha et al., 2001; Kumar et al., 2010).
Fresh leaves of plants are highly perishable and tend to lose quality immediately after harvest and thus require preservation against deterioration and spoilage. Water content in fresh Lamiaceae herbs is approximately 75–80% wet basis; however, to preserve its quality, water levels need to be reduced to less than 15% wet basis for preservation (Dixon and Paiva, 1995). Drying is one of the oldest and the most commonly used preservation techniques. Drying the herbs inhibits microbial growth and forestalls certain biochemical changes. Yet, it can cause alterations that affect herb quality, such as changes in structure and alterations in aroma caused by losses in volatiles or the formation of new volatiles as a result of oxidation or esterification reactions (Díaz-Maroto et al., 2002; OrphAnides et al., 2013). Certain compounds have been observed to increase in different herbs after drying—for example, eugenol in bay leaf (Díaz-Maroto et al., 2002; OrphAnides et al., 2013), thymol in thyme (Venskutonis, 1997), and some sesquiterpenes in different herbs (Baritaux et al., 1992; Yousif et al., 1999). Most studies have reported changes in the color and volatile compounds of aromatic herbs after drying (Díaz-Maroto et al., 2002; Di Cesare et al., 2003). The quality of the dried product is mostly influenced by drying conditions as well as the method adopted for drying. Currently, most dehydration techniques that are used to preserve fruits are fluidized bed drying, solar drying, hot air drying, microwave drying, osmotic dehydration, foam mat, spray drying, and freeze-drying (Barrozo et al., 2014).
Tray drying is the conventional method of drying that is widely used on a commercial scale because of its simplicity and low-cost equipment. However, tray drying involves a longer drying time due to the requirements of convective hot air. In addition to longer drying durations, the products are also exposed to undesirable effects such as reductions in bioactive compounds, shrinkage, slow rehydration, and loss of color (Dev et al., 2011; Workneh and Oke, 2012; Horuz and Maskan, 2015; Hihat et al., 2017; Kaveh et al., 2021; Zia and Alibas, 2021). Hence, the use of microwaves has emerged as an interesting alternative technique. In contrast to convective drying, microwaves can generate large amounts of heat inside rather than externally. Heat is generated by water molecules interacting with electromagnetic waves directly, leading to water vapor pressure differences between the interior and surface areas, leading to rapid external migration of moisture.
Microwave-assisted hot air has been widely used for agricultural drying with a positive effect on quality, antioxidant activity, and bioactive compounds (Arslan and Özcan, 2010; Dev et al., 2011; Workneh and Oke, 2012; Chaikham et al., 2013; Ozcan-Sinir et al., 2018; Poogungploy et al., 2018). Sensory evaluation of persimmon chips processed by microwave-assisted hot air microwave and freeze techniques showed higher sensory scores and nutritional values than traditional hot air drying (Jia et al., 2019). This indicated that dried samples using microwave techniques were positively affected by food products. In recent times, the application of microwave hot air rolling drying (MHRD) has developed to incorporate the advantages of microwave drying and hot air drying. The MHRD technique could improve the cooking efficiency of kidney beans, with a reduction of 2.5 times drying time at the same temperature when compared to without microwaves (Li et al., 2022). It has also been applied in ginger drying to determine the appropriate microwave power and drying temperature for the final product (Zeng et al., 2023). Similarly, microwave drying was applied for dewatered sludge, and it was found that the application of microwaves reduced the drying time by at least 37.5% compared to using hot air (Wulyapash et al., 2022). In addition to quality prospectus, concerns that producers are preoccupied with are energy usage that influences drying costs. These concerns may be reduced by microwave-assisted hot air drying (Poogungploy et al., 2018; Kaveh et al., 2021; Yue et al., 2021).
This study, therefore, aimed to evaluate the effect of alternative microwave drying processes under different conditions while protecting and maintaining dried leaf quality and bioactive compound accumulation in the red and green holy basil cultivars. We investigated the energy consumption at an industrial scale for food production and cosmetics industry use.
Materials and methods
Plant material
The fresh stems and leaves of two commercial holy basil cultivars (Ocimum tenuiflorum L.; green and red holy basil) were collected at the harvesting stage (49 days after transplantation) from a plant factory using artificial light (PFAL) at the National Center for Genetic Engineering and Technology Development Agency (BIOTEC), Thailand Science Park, Klong Lung, Pathum Thani, Thailand. Holy basil plants were grown under controlled environmental conditions with artificial light at 200 μmolm−2 s−1 of light intensity, 25 ± 1°C air temperature, 75 ± 3% relative humidity, and 1,000 ± 150 μmol mol−1 CO2 concentration (Thongtip et al., 2022; Chutimanukul et al., 2022b).
Drying methods and energy efficiency
The leaves of green and red holy basil were dried under four different conditions, each with four replications. The drying methods comprised commercial tray drying (TD) with hot air at 45°C and previously unevaluated regimes of microwave drying (MD) assisted hot air drying at 45°C under three different power strengths of 200, 400, and 600 W. The TD with hot air for industrial scale is presented in Figure 1A. We used 12 drying trays with width, length, and height of 53 × 72 × 3 cm. MD was designed and constructed by staff from the Faculty of Engineering, Mahasarakham University, Thailand. The apparatus for microwave-assisted hot air drying at the pilot scale is shown in Figure 1B. Four magnetrons at 1000 W were installed, generating microwaves at a frequency of 2,455 MHz. Under MD, the air was heated by a 9 kW electric heater and circulated by a 1.5 kW centrifugal fan with an electric motor. The drying chamber was 80 cm each in length, width, and height and featured eight drying trays with a diameter of 60 cm. Plant material samples for each run were 500 g placed on rotated trays, and fresh holy basil leaves were laid out in a single layer for drying with a thickness of less than 2 mm. Samples were dried until water activity (aw) was lower than 0.6 (i.e., the recommended safe level for storage). After drying, the weight of each sample was recorded to calculate water evaporation.
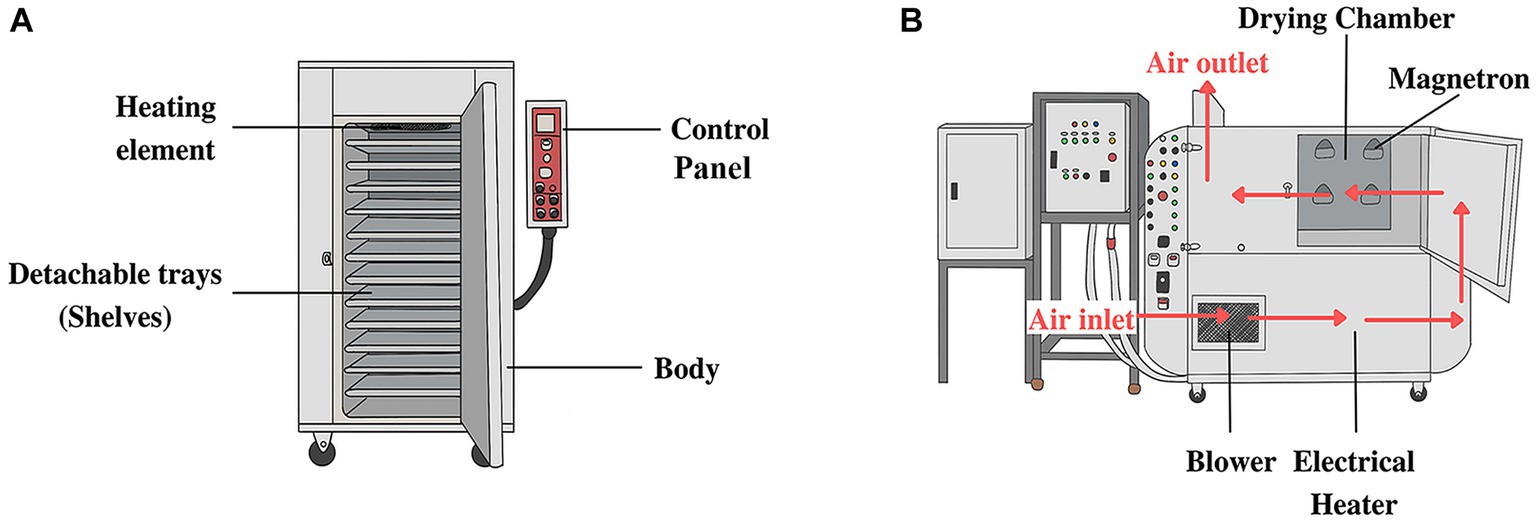
Figure 1. The pilot scale of the drying process of commercial tray drying with hot air (A) and microwave-assisted hot air dryer with the airflow direction (B).
Moisture content and aw determination
Fresh leaves were chopped into small pieces before determining moisture content. Approximately 5 g samples were placed in an aluminum can and put in the oven at 103°C for 24 h. Moisture content was calculated from the water evaporated divided by the fresh weight (AOAC, 1995). The dried samples were measured for aw using an AquaLab water activity meter (Aqua-Link 3.0, Pullman, WA). It was calibrated with distilled water to obtain aw in the range of 1.000 ± 0.003. Five dried leaves were used for each measurement.
Specific energy consumption
SEC is the total energy consumed during drying divided by the mass of water evaporation. Tray drying energy consumption was evaluated from the blower and heater apparatus. The energy consumption of the microwave-assisted hot air drying was determined from four components, namely, microwave, blower, motor, and heater apparatus. A clamp multimeter was used to measure the voltages and electrical currents of all apparatus. The electrical energy consumption of the magnetron and heater was calculated by
The electrical energy consumption of the blower and motor was calculated by
where is the energy consumption of each component (kWh), V is voltage (V), is electrical current (A), t1 is time (h) when the device is turned on during drying, and t2 is drying time (h) throughout the experiment.
The SEC of the microwave-assisted hot air dryer was reported in MJ/g of water evaporation using the following expression:
The SEC of the tray dryer was reported in MJ/g of water evaporation using the following expression:
where 3.6 is the electric energy conversion factor from kilowatt hour to megajoule, is initial mass and is the final mass (g).
Color measurements
Leaf samples of both green and red cultivars were ground to a fine powder before measuring color with a Hunter Lab Colorimeter (type Color Flex, United States). Color coordinate values, L*, a*, b *, for each sample were recorded. L* represented lightness, varying from 0 (dark) to 100 (light). Positive a* and b* values indicated redness and yellowness, while negative a* and b* values indicated greenness and blueness. Chroma (C) and hue angle (h) were calculated using the following equations (Pathare et al., 2013):
Pigments accumulation
All pigment contents such as chlorophyll a, chlorophyll b, total chlorophyll, and carotenoids in the holy basil were examined according to Wellburn (1994). The dry samples were ground to a fine powder in a mortar, from which 100 g was extracted with 1 mL of absolute methanol solvent. The mixture was then incubated at 15°C in darkness for 72 h and then centrifuged at 8,000 rpm, 15°C for 5 min. After centrifugation, the supernatant solution was separated completely. The absorbance of the extracted solution was recorded at wavelengths of 470, 646.8, and 663.2 nm using a spectrophotometer (Agilent 8,453 UV–vis Spectroscopy System, Country). The concentration of the pigments was calculated using the following formulae (Wellburn, 1994):
Pigment accumulation was presented as ug per gram dry weight (DW) of the sample.
Antioxidant activity evaluation
Sample extraction
Holy basil samples, after harvesting and drying by different methods, were ground to a fine powder and extracted following a modified method (Chutimanukul et al., 2022a) for analyzing the total content of phenolic compounds (TPC), total flavonoid compounds (TFC), and the scavenging activity of DPPH radicals. Ten microgram of the fine powder sample was thoroughly mixed with 5 mL absolute methanol containing 1% HCL, which was used as a solvent. After incubation at room temperature for 3 h, the mixture solution was centrifuged for 5 min at 12,000 rpm. The supernatant was transferred to another microtube and used for the determination of TPC, TFC, and DPPH radical scavenging activity.
Total phenolic content
The total phenolic content (TPC) was assayed following modified Folin-Ciocaltea colorimetric methods (Chutimanukul et al., 2022b). Two hundred microliter of extracted solution was mixed with 200 μL of 1 N Folin–Ciocalteu reagent and incubated at room temperature for 15 min. Then, the mixture was added to 600 μL of 7.5% sodium carbonate (Na2CO3) and incubated for 1 h before detecting the absorbance at 730 nm by spectrophotometer (MultiskanSky, Thermo Scientific). Gallic acid was used as a standard solution, and the results were presented as milligrams of gallic acid equivalent per gram dry weight of the sample (mg of GAE / g DW).
Total flavonoids content
The quantification of total flavonoid content in holy basil was evaluated based on the method of (Chutimanukul et al., 2022b) with minor modification of the colorimeter method. Three hundred and fifty microliter of the extracted solution was added to 75 μL of 5% sodium nitrite (NaNO2) and completely mixed using a vortex. The mixture was incubated for 5 min before adding 75 μL of 10% aluminum chloride (AlCl3·6H2O) and kept at room temperature for 5 min. Lastly, 1 M sodium hydroxide (NaOH) was pipetted to the mixture solution and left to stand for 15 min. The absorbance of the homogenate solution was determined at 515 nm by a spectroradiometer. The content of total flavonoids was calculated using rutin as the standard solution and showed as milligrams of rutin equivalent per gram dry weight of holy basil (mg of rutin/g DW).
Total terpenoids content
To investigate the content of total terpenoids in the holy basil after drying, 100 mg of fine powder of the dried sample from several drying treatments was extracted by mixing with 1 mL absolute methanol and incubating in the dark for 48 h. After incubation, the mixture was centrifuged at 6,500 rpm for 15 min. The determination of total terpenoids of the extracted solution was performed using the procedures of Ghorai et al. (2012) and Chutimanukul et al. (2022a). Absorbance was measured at a wavelength of 540 nm using a spectrophotometer with 99.9% methanol as a blank control. Linalool was used as the standard solution, and the content of total terpenoids was exhibited as milligrams of linalool per gram dry weight (mg of linalool /g DW).
DPPH radical scavenging activity
Antioxidant scavenging activity of 2,2-diphenyl-1-picrylhydrazyl (DPPH) free radicals was detected by following the method of Chutimanukul et al. (2022b). Hundred microliter of extracted solution was completely mixed with 900 μL of 0.1 mM DPPH and kept in the dark at 25°C for 30 min. The absorbance of the mixture was determined at 515 nm using a spectroradiometer. Trolox was used as a reference antioxidant. The percentage of DPPH scavenging as an antioxidant activity was calculated by the equation (Yen and Duh, 1994): Inhibition% = (Acontrol − A515)/(Acontrol) × 100. Where Acontrol and A515 were the absorbance of 0.1 mM DPPH without sample and absorbance of sample at wavelength 515 nm, respectively.
Volatile compound quantification
The analysis of volatile organic compound (VOCs) content was obtained by gas chromatography with mass spectrometry (Agilent; 7890B) combined with a quadruple time-of-flight mass spectrometer (GC/Q-TOF, Agilent, 7250) and PAL autosampler system (CTC Analytics AG, Switzerland). Extraction of the sample and analysis were carried out according to the method described by Chutimanukul et al. (2022a). Hundred milligram of the dried leaf powder was placed in 1 mL of absolute methanol with 10 μL of 2000 ppm gamma-hexalactone, and then uniformly mixed for 1 min. The samples were sonicated by ultrasonic bath for 30 min. To eliminate the insoluble material, the prepared extracts were centrifuged at 10,000 rpm for 5 min. The supernatant was collected and stored in a vial at −20°C for further analysis.
For the analysis of VOCs of dried leaves of holy bail in each MD treatment, 1 μL of the extract was injected into a multimode inlet (MMI). The inlet temperature was controlled at 250°C, and the samples were carried at a constant flow rate of 1.0 mL/min by high-purity helium (>99.999%). Chromatographic separation was conducted with a DB-FFAP column (30 m × 0.25 mm × 0.25 μm) (Agilent Technologies, United States). GC operational conditions were as follows: the temperature program was initiated at 60°C for 1 min, increased to 250°C by 10°C/min for 3 min; transfer line, 250°C; ion source (EI) temperature, 240°C; quadruple temperature, 150°C; EI energy 70 eV; and scanning mode from 20 to 350 m/z. Four target compounds, including eugenol, methyl eugenol, β-Caryophyllene, and Linalool, were investigated. The calibration curves were constructed with the relative internal response ratio as a function of the analyte concentration of the mixtures of the four target standard compounds with a range of 0.04–100 ppm.
For data processing, the target compounds, including eugenol, methyl eugenol, and α-Humulene, were determined by comparing the calibration curves against relative internal response ratios. Concentrations of target analytes were reported as μg per gram of DW.
Experimental plan and data analysis
The experiments were designed according to a completely randomized design (CRD) with four replications. Statistical data were analyzed by IBM SPSS (IBM Corporation; Armonk, NY, United States). One-way analysis of variance (ANOVA) was used for sample comparisons in each parameter. Mean separations were analyzed with Duncan’s Multiple Range Test (DMRT) at a 95% significance (p < 0.05). The data were presented as means ± S.E (standard error).
Results
Energy consumption
To investigate the energy consumption and specific energy consumption of the four different drying treatments on the two cultivars of holy basil, the initial moisture content, final moisture content, and water activity (aw) of leaves after drying were calculated. Table 1 indicates the effects of the four drying methods: microwave drying (MD) under three different powers of 200, 400, and 600 W combined with hot air drying (HAD) at 4°C and the tray drying (TD) method using 45°C of hot air affected drying time. The total drying times for TD were substantially longer in comparison to microwave oven drying of both cultivars. Among different powers for MD treatments, the highest MD power caused the lowest average drying time. For a load of 500 g fresh leaves at 200, 400, and 600 W of MD with HAD, green holy basil leaves were dried after 6, 3.5, and 3 h, respectively, while the red holy basil leaves took 5, 4, and 2.5 h, respectively. Moreover, across drying methods, the drying process caused reductions in moisture contents of fresh leaves, from between 87.5 and 88.6% at harvest to 8.0–9.9% in 2.5–7 h of drying. After drying, the observed aw value ranged from 0.30 to 0.45. The appearance of two holy basil cultivar leaves after drying treatments is shown in Figures 2, 3.
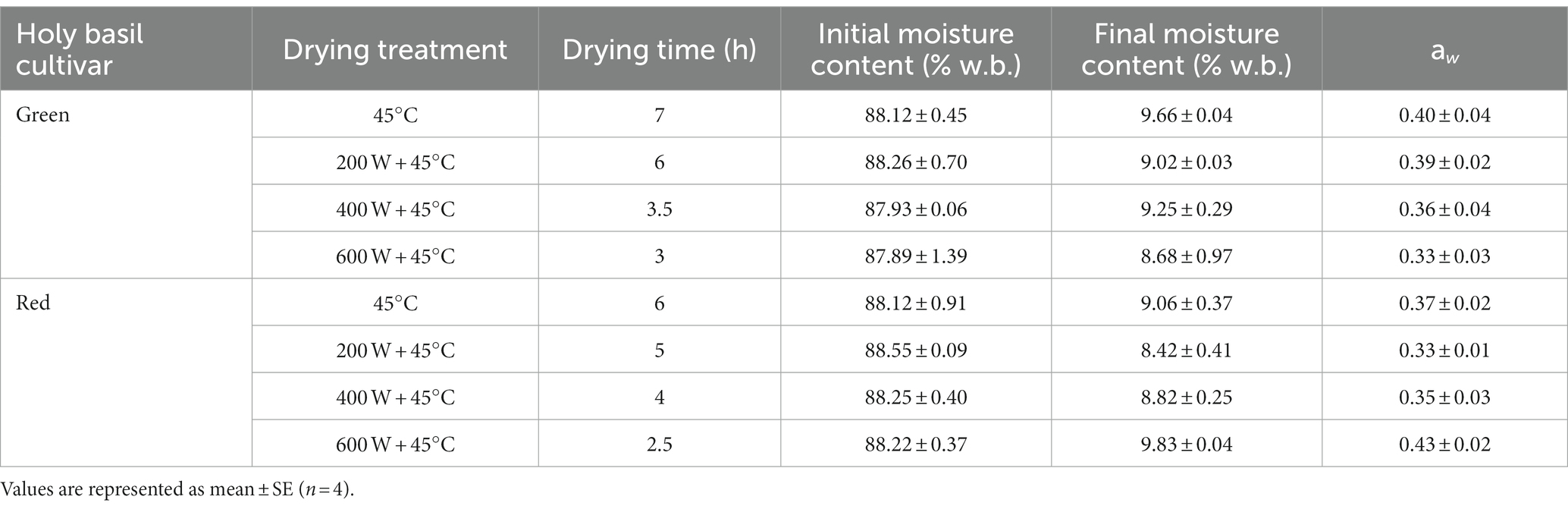
Table 1. Drying time, initial moisture content, final moisture content, and water activity (aw) of green and red holy basil leaves after drying by tray drying (TD, 45°C) and various microwave powers combined with hot air drying at 45°C (HAD) (200 W + 45°C, 400 W + 45°C and 600 W + 45°C).
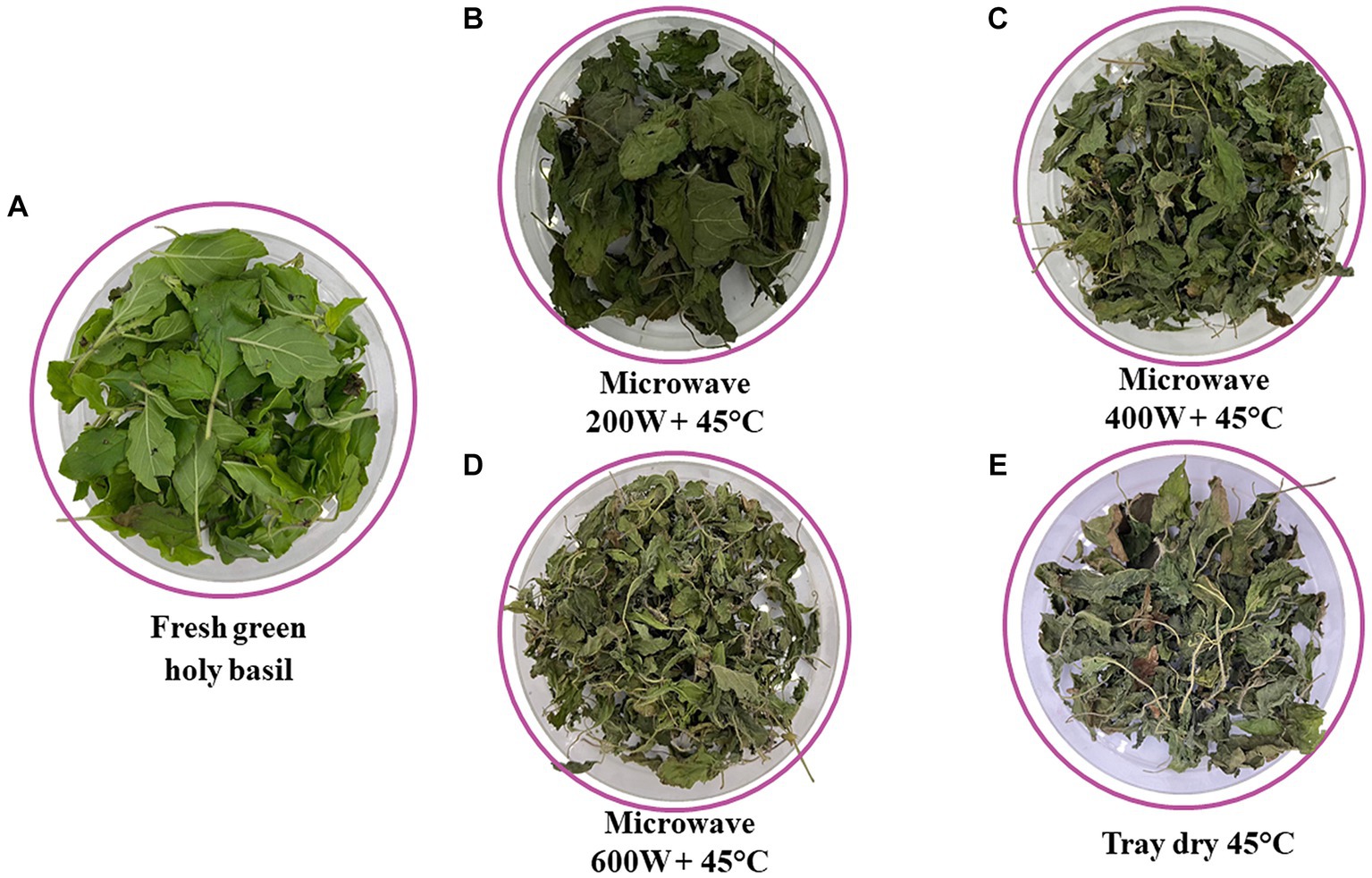
Figure 2. Quality retention of green holy basil leaves after drying by various microwave power combinations with hot air at 45°C (HAD), fresh holy basil (A), 200 W + 45°C (B), 400 W + 45°C (C), 600 W + 45°C (D) and tray drying (E).
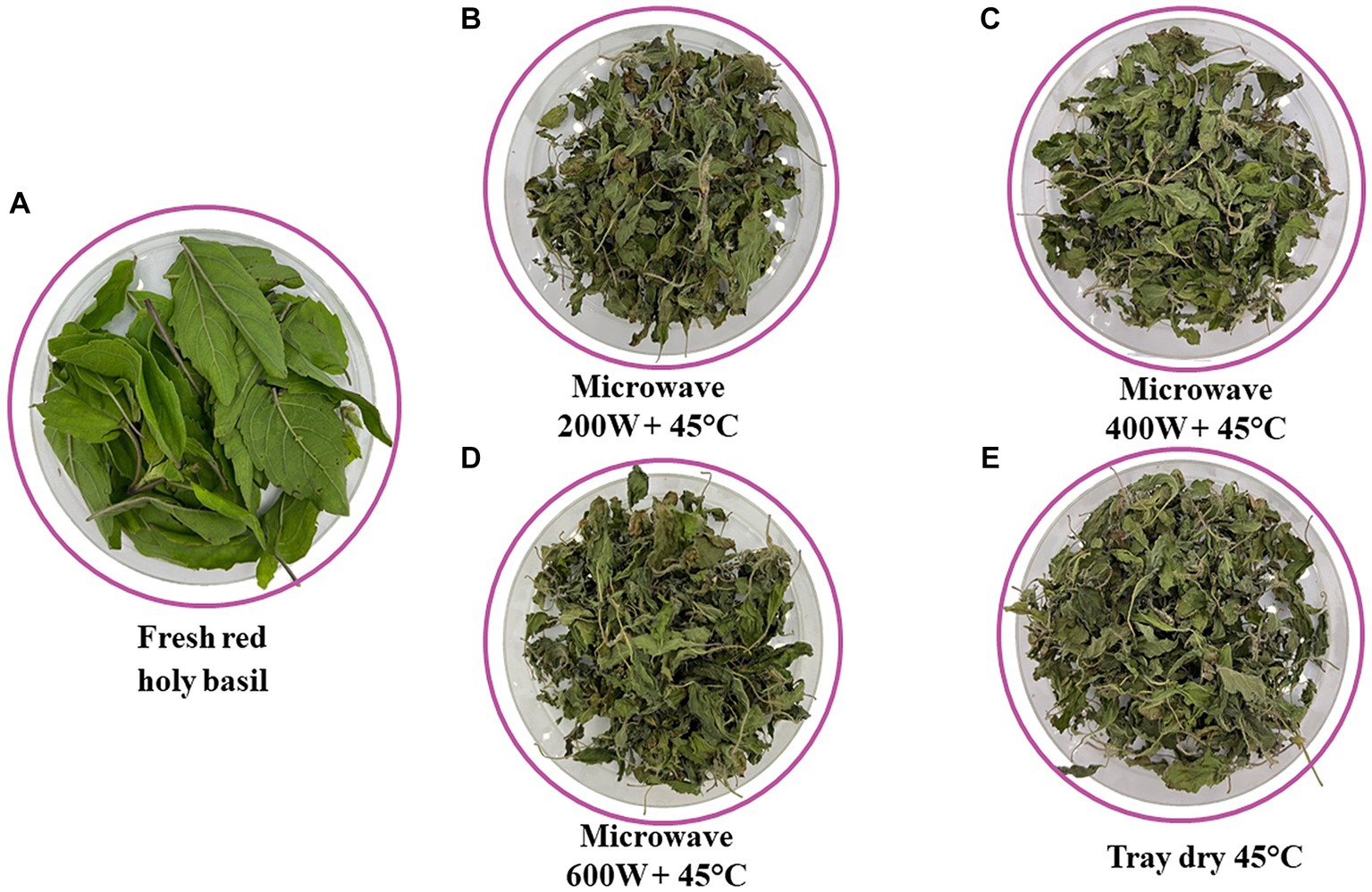
Figure 3. Quality retention of red holy basil leaves after drying by various microwave power combinations with hot air at 45°C (HAD), fresh holy basil (A), 200 W + 45°C (B), 400 W + 45°C (C), 600 W + 45°C (D) and tray drying (E).
Energy efficiency via evaluation of energy consumption and specific energy consumption (SEC) was assessed. Blower (Eblower), motor (Emotor), heater (Eheater), microwave (Emocrowave), total energy (Etotal), evaporated water, and SEC were considered. The details are presented in Table 2. After drying two cultivars of holy basil, we found that the MD treatments had the lowest Eblower, Eheater, Etotal, and SEC as the power of the microwave increased. On the other hand, TD had the highest value. Among different drying treatments, there were no differences in the values of evaporated water. Compared to the values obtained through the TD treatment, the SEC value at 600 W of MD was 3.5 times in green holy basil and 4 times in red holy basil.
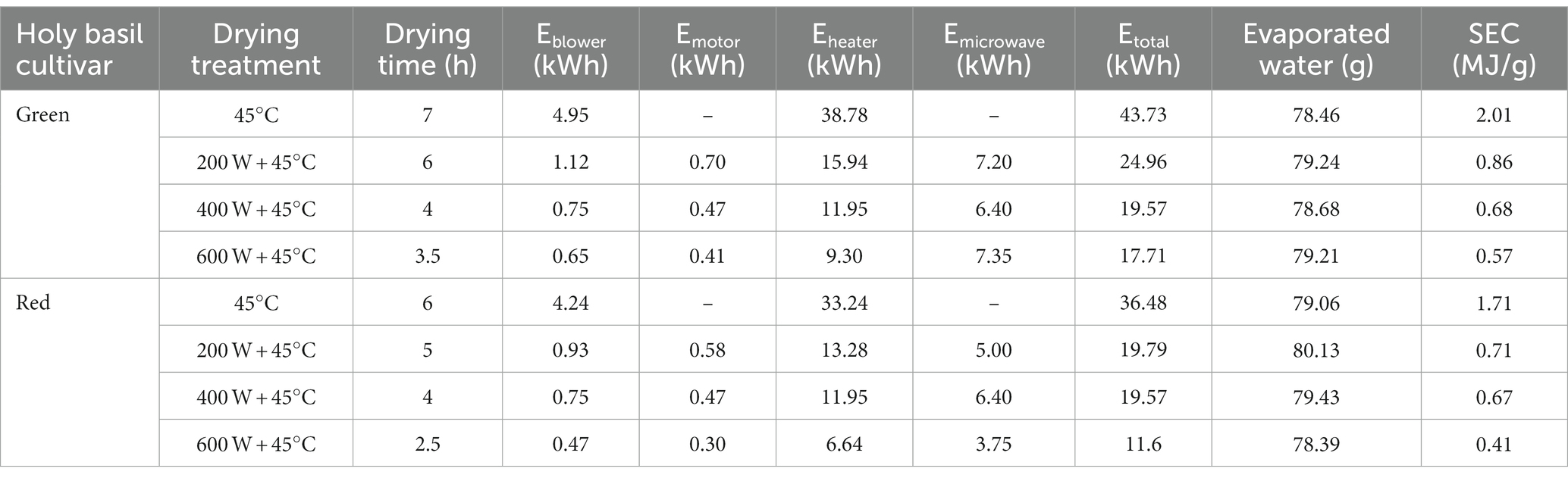
Table 2. Energy consumption values of green and red holy basil leaf samples with tray drying (TD, 45°C) and various microwave powers combined with hot air drying at 45°C (HAD) (200 W + 45°C, 400 W + 45°C, and 600 W + 45°C).
Qualitative properties
The color change of green and red holy basil dried leaf powder is shown in Table 3. Overall, lighter-colored leaf samples were dried with the highest MD power. For dried leaves of both green and red cultivars, the parameters of L* (Lightness) value of MD at 600 W were the highest when compared with other methods. When comparing the a* values of all dried leaves to those of fresh leaves, the lowest a* (redness: green to red) values were observed under MD at 600 W, while TD showed the highest value. Moreover, b* (yellowness) of both cultivars showed the lowest value under 600 W. C (chroma value: color saturation) and h (hue angle: color angle) increased with an increase in the power of MD, with leaf samples becoming clearer after drying. For MD at 200 and 400 W, dried leaf samples of each cultivar appeared lighter and greener than for TD treatment.
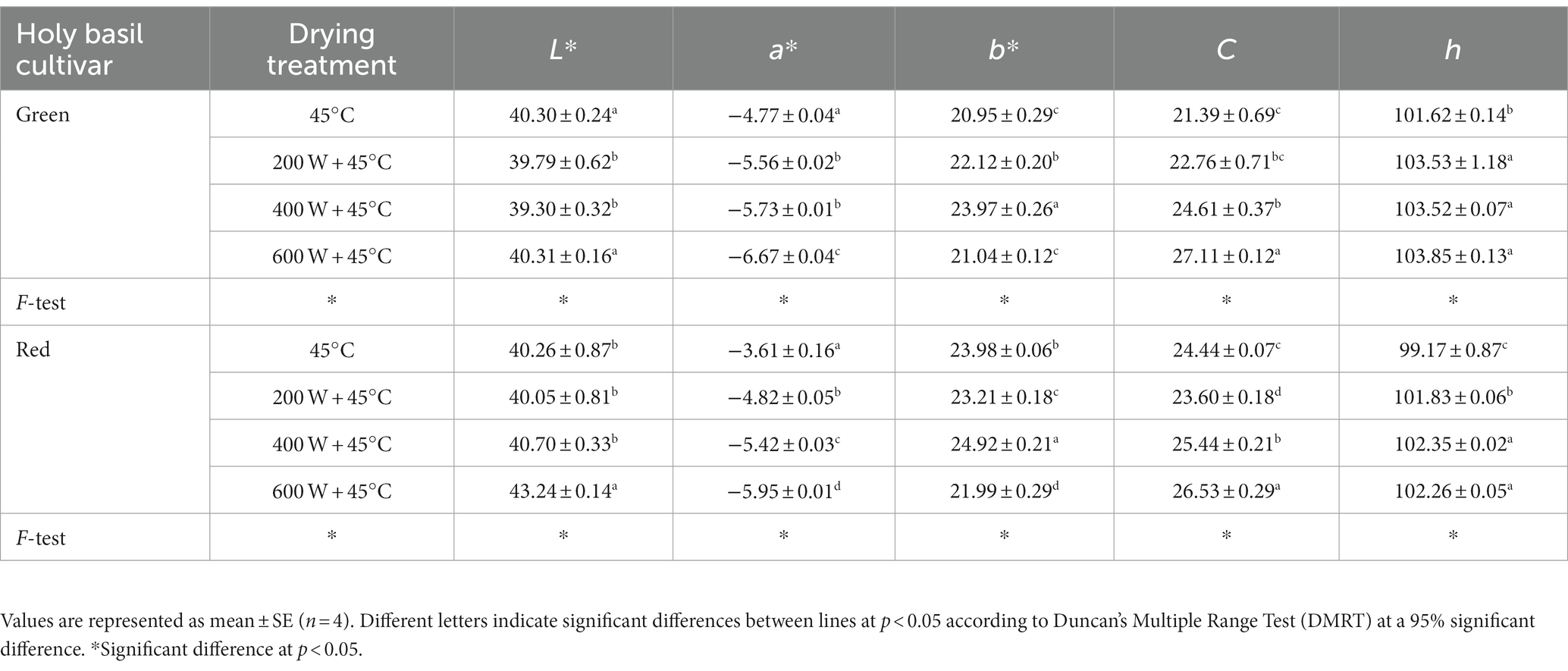
Table 3. The color parameters (L*, a*, b*, C, and h) of green and red holy basil leaves after drying using tray drying (TD, 45°C) and various microwave powers combined with hot air drying at 45°C (HAD) (200 W + 45°C, 400 W + 45°C, and 600 W + 45°C).
Pigment accumulation
The effect of different drying techniques on pigment content was investigated (see Figure 4 for details). Leaves of green and red holy basils were significantly influenced by drying treatment. A significant accumulation of total chlorophyll (TChl), chlorophyll a (Chl a), and chlorophyll b (Chl b) was identified in both cultivars. It was the highest at 600 W MD (Figures 4A–C). Additionally, MD at 600 W resulted in increased carotenoid content in dried leaves of green holy basil, while carotenoid content was lowest when leaves were dried under TD. However, the red cultivar did not show significant differences across drying treatments (Figure 4D).
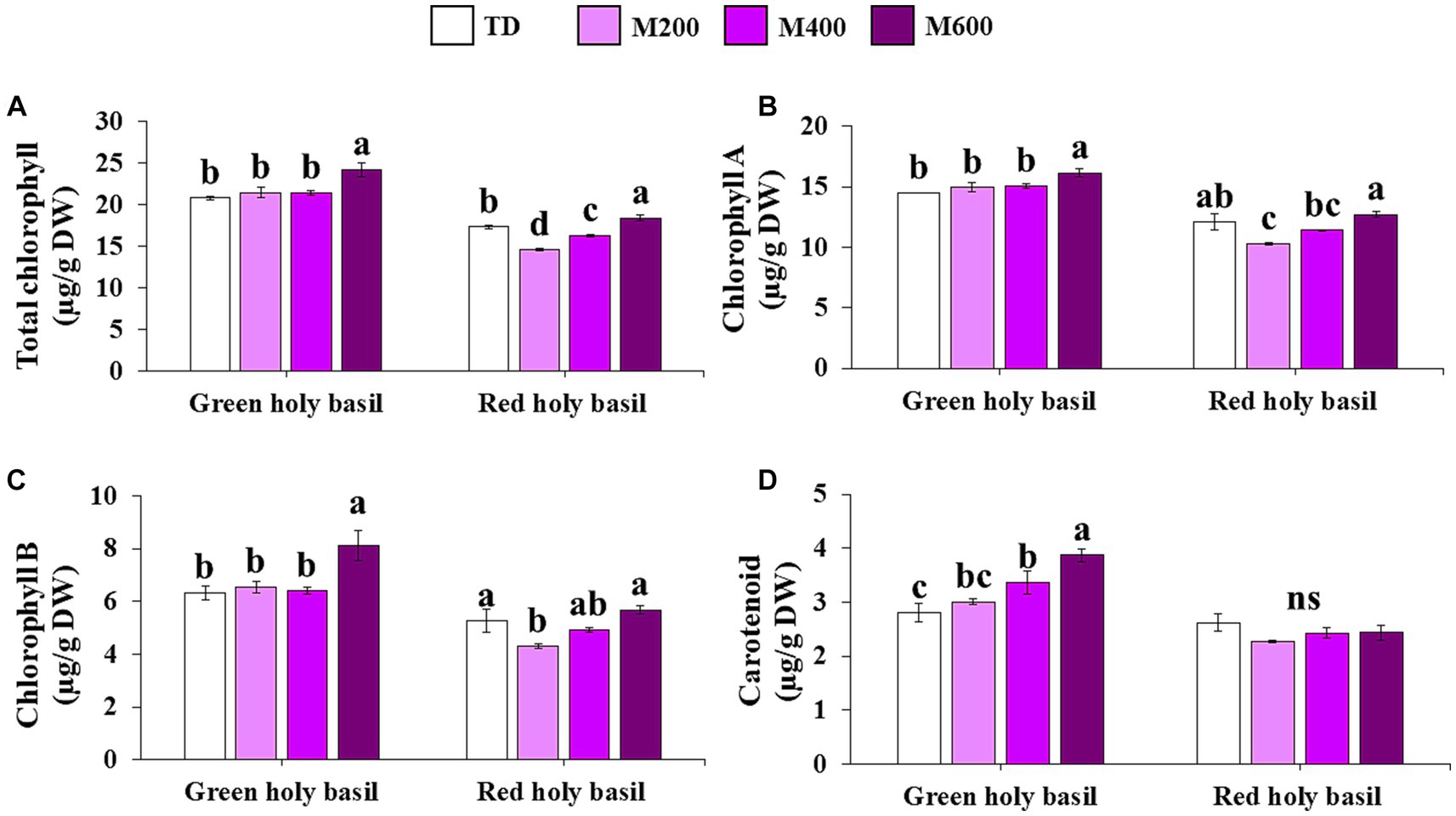
Figure 4. Pigment accumulation, total chlorophyll (A), Chl a (B), Chl b (C), and carotenoid (D) in green and red holy basil leaves after tray drying (TD, 45°C) and drying under various microwave power combinations with hot air at 45°C (HAD) (200, 400, and 600 W). ANOVA was performed, followed by mean comparisons with DMRT. The different letters above the bars show significant differences between treatments at p < 0.05. “ns” indicates no significant difference.
Bioactive compounds and antioxidant capacity
After drying, the content of total phenolic compounds (TPC), total flavonoid content (TFC), and total terpenoids were found to be affected by the different drying treatments (Figure 5). The TPC content of both green and red holy basil leaves showed significant differences. Green holy basil leaves showed the highest TPC levels at 400 W of MD, while red holy basil leaves had the highest TPC content at 600 W (Figure 5A). Across different drying treatments, there were no significant differences for TFC in green holy basil. However, different drying treatments affected TFC accumulation in red basil, with 600 W MD showing the highest accumulation (Figure 5B). Different drying treatments significantly influenced the total terpenoid content in both green and red holy basil leaves (Figure 5C). MD at 400 and 600 W revealed the highest values for green holy basil leaves, and the levels were highest at 600 W in red holy basil. The total terpenoid content was lowest in TD treatments for red holy basil. DPPH analysis among drying treatments showed that antioxidant activity, i.e., the scavenging rates of DPPH, in green holy basil leaves had the highest value at MD 200 W. However, it was not significantly different than MD 400 W. Further, MD at 600 W recorded the lowest reading. Drying treatment did not significantly affect the antioxidant capacity among red holy basil leaves (Figure 5D).
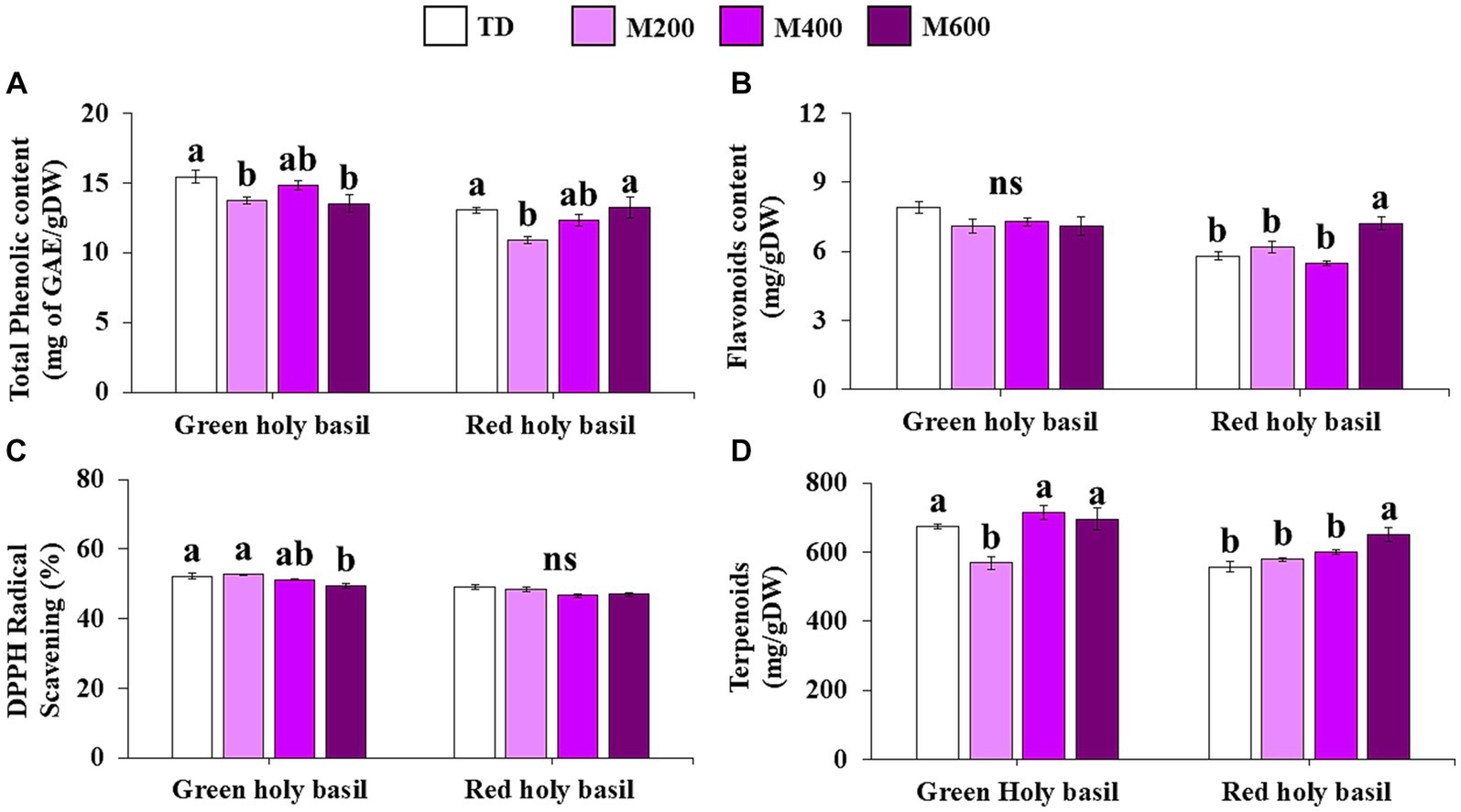
Figure 5. The content of total phenolic content (TPC) (A), total flavonoid content (TFC) (B), total terpenoids content (C), and DPPH free radical scavenging rate (D) in green and red holy basil leaves after tray drying (TD, 45°C) and various microwave power combinations with hot air at 45°C (HAD) (200, 400, and 600 W). ANOVA was performed, followed by mean comparison with DMRT. The different letters above the bars show significant difference between treatments at p < 0.05. “ns” indicates no significant difference.
Volatile content
Volatile organic compounds (VOCs) of the two holy basil cultivars were analyzed using gas chromatography coupled with mass spectrometry (GC/Q-TOF). Results were obtained for the following VOCs: eugenol, methyl eugenol, Linalool, and β-caryophyllene (Figure 6). The highest eugenol levels for green holy basil were observed in TD treatments, followed by 200, 400, and 600 W MD, respectively (Figure 6A). However, red holy basil leaves did not show significant differences among drying treatments. In contrast, a significantly greater amount of methyl eugenol was present at 400 and 600 W MD in green holy basil leaves, but it was not significantly affected in red holy basil leaves (Figure 6B). Linalool alone was significantly different in the green holy basil leaves (Figure 6C). Regarding β-caryophyllene, TD and 200 W MD recorded the highest values, and MD 400 and 600 W showed the lowest β-caryophyllene values, which were significantly different for both holy basil varieties (Figure 6D). MD 600 W displayed the highest content in green holy basil leaves but was not significantly different from the TD treatment. β-caryophyllene content was highest in MD 400 and 600 W.
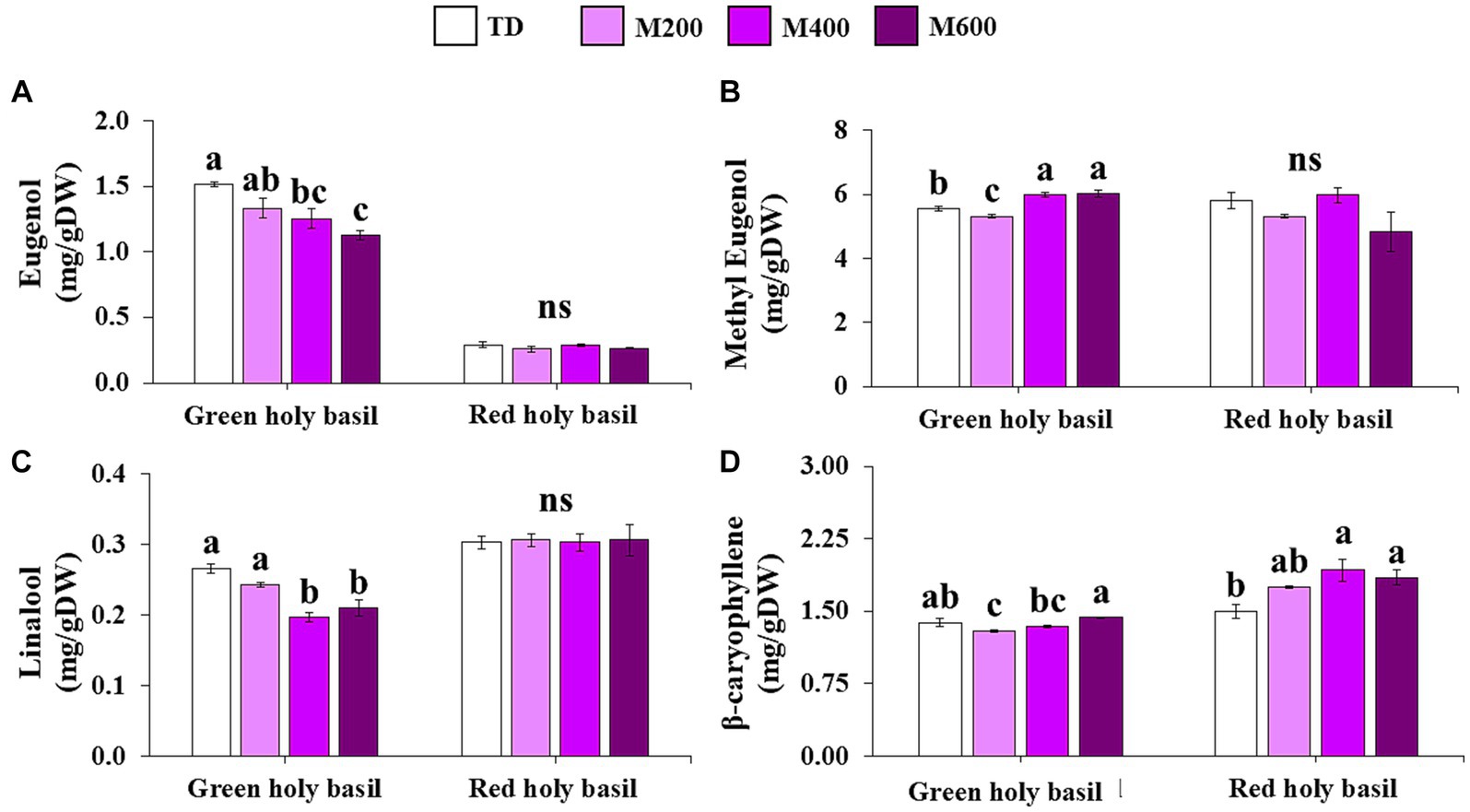
Figure 6. The accumulation of eugenol (A), methyl eugenol (B), Linalool (C), and β-caryophyllene (D) in green and red holy basil leaves after tray drying (TD, 45°C) and various microwave power combinations with hot air at 45°C (HAD) (200, 400, and 600 W). ANOVA was performed, followed by mean comparison with DMRT. The different letters above the bars show a significant difference between treatments at p < 0.05. “ns” indicates no significant difference.
Discussion
Our study indicates that variations in microwave drying treatments have significant impacts on the energy consumption of green and red holy basil leaves at an industrial scale. According to the industrial standards for dried herbal materials, the final moisture content is usually below 12% w.b., depending on the type of plant tissue (Preparations WECoSfP and World Health Organization, 1996). Such moisture content typically enhances shelf life during storage without affecting the quality of the plant products by reducing their susceptibility to post-harvest pathogens (Poós and Varju, 2017). Several studies have shown that a moisture content range of 7–12% is ideal for raw herbal materials in medicine preparations (Tarhan et al., 2011; Jayaraman and Gupta, 2020; Shalaby et al., 2020; Wiset et al., 2021). Consequently, the drying method is an important factor that has a considerable impact on the quality of resulting herbal materials. Our holy basil leaf samples from the two cultivars under plant factory cultivation showed 8.0–9.9% final moisture content after the various drying treatments (Table 1). We dried leaf samples until aw was lower than 0.6, which is safe for storage and prevents microbial growth (Rahman and Labuza, 2007).
Overall, the increased power of MD was associated with reduced drying times of the herbal products. Microwaves increase moisture evaporation rates from plant tissues by the rapid activation of energy absorption of water molecules. This leads to the generation of a temperature gradient between the sample and the air in the drying chamber that promotes the moisture in the samples to move outwards (Hemis et al., 2011; Doymaz et al., 2015; Hou et al., 2021; Mouhoubi et al., 2022). Previous reports of the Trabzon persimmon fruit (Alibas et al., 2019) and Kaffir lime leaf (Pradechboon et al., 2022) showed that increases in MD power induced a rapid increase in temperature due to the high energy transfer into samples. Our study indicates that MD at 600 W with HAD treatment ensured the shortest drying time for both green and red holy basil leaves of 3 and 2.5 h, respectively. In comparison, holy basil leaves dried by tray drying (TD) showed the longest drying time at 7 and 6 h for green and red holy basil leaves, respectively. This result was consistent with previous reports showing that an increase in MD power causes a dramatic increase in the average drying rates of various plants (Alibas et al., 2019; Liu et al., 2021; Pradechboon et al., 2022).
Energy consumption values among different drying methods of red and green holy basil leaves are shown in Table 2. MD methods had high energy-saving effects when compared with TD, which is widely used in chemical, pharmaceutical, and food industries to preserve herbal products for downstream processing and extended storage (Mujumdar, 2008; Chen and Mujumdar, 2009). Our study shows that all MD treatments have a lower and specific energy consumption (SEC) than TD. Further, microwave drying at 600 W with HAD provided around 2–3-fold energy consumption reduction over TD for green and red holy basil leaves, respectively. The increase in microwave power enhanced the movement of water molecules since microwaves can easily penetrate inside the sample, where the microwave energy is transformed into heat. When the molecules of water are rubbed together, they produce heat, which increases the water temperature. In the case of TD, the heat is transferred from the surface of the sample to inside it by heat conduction, causing a longer drying time (Chen et al., 2019). These results are in accordance with previous findings that energy consumption and SEC decrease as microwave power increases (Varith et al., 2007; Kaveh et al., 2021). This is largely due to the drying time being shorter. Moreover, these results suggest that the highest MD power (600 W with HAD) might increase evaporation rates during the drying process, leading to decreases in energy consumption.
Drying processes can significantly affect the qualitative features of herbal production. Color is a psychological property of food products that directly influences the perception of flavor and quality (García et al., 2009), and the drying process is one of the most important causes of color degradation or dehydration (Lozano and Ibarz, 1997; Demiray et al., 2017). This is likely due to the gradual rising of heat, leading to the decomposition of colored pigments (Orikasa et al., 2014). Studies in various plant leaves and fruits have shown that the temperature during the drying process results in color changes in spearmint leaf (Islam et al., 2019), holy basil leaf (Raksakantong et al., 2011), Kaffir lime leaf (Pradechboon et al., 2022), papaya (Islam et al., 2019), and kiwi (Izli et al., 2017; Taghinezhad et al., 2021).
Likewise, in the current study, we observed that the drying treatment significantly influenced color change in the dried leaf powders of green and red cultivars. Colorimetric parameters, such as L*, a*, b*, and C, are widely used to quantify the coloration of food and fruit products (Arias et al., 2000; Pathare et al., 2013). L* represents lightness, a* represents red (positive value) and green (negative value), b* represents yellow (positive value), and blue (negative value), and C represents color saturation. H represents relative amounts of redness and yellowness, with 90° for yellow, 180° for green, and 270° for blue (Francis, 1980; Voss, 1992; Ayala-Silva et al., 2005).
Our results showed that 600 W of MD with HAD caused the highest values of L*, a*, C, and h (hue) of green and red holy basils with b* at its lowest when compared with other MD treatments and conventional TD (Table 3). This is consistent with previous studies that found MD (increased power) with HAD caused low a* values (Yilmaz and Alibas, 2021; Pradechboon et al., 2022). However, there were no significant differences between the L* and b* values of green holy basil at 600 W of MD with HAD and under TD conditions. This may be explained by the drying time of TD, which, without a microwave, took longer time to dry than 600 W of MD with HAD. The TD condition had lower heat than microwave power and resulted in brightness and yellow shades that were similar to 600 W of MD with HAD treatment. Similarly, the leaf pigment contents of the dried samples of green and red holy basils showed the highest accumulation of total chlorophyll (TChl), chlorophyll a (Chl a), and chlorophyll b (Chl b) in MD 600 W (Figure 4). These findings indicate that MD 600 W with HAD treatment, with its shortest drying duration, is promising in terms of psychologically interpreted properties of herbal products by maintaining greener and lighter colors in dried holy basil leaves of both cultivars.
Secondary metabolites play an important role in plant defense response and are widely used to relieve several ailments in traditional medicine (Afzal et al., 2022; Aziz et al., 2022; Kainat et al., 2022; Khalid et al., 2022). Essential oils, terpenes, phenolics, phenolic acids, and flavonoid compounds are the main constituents of antioxidants in the holy basil plant (Kadian and Parle, 2012; Agarwal et al., 2017; Maqbool et al., 2023; Waliat et al., 2023). The drying process may also induce changes in the secondary metabolites, bioactive compounds, and volatile oils among herbal products. Several studies have demonstrated that high temperature seriously damages secondary plant metabolites such as total phenolic compounds (TPC), total flavonoid compounds (TFC), terpenoids, antioxidant properties, and volatile oils in various plant species (Sang et al., 2014; Hihat et al., 2017; Nguyen and Le, 2018). Phenolic compounds and flavonoids are the most important phytochemical components and have potential against diseases, presumably due to their antioxidant properties (Rice-Evans, 2001). In our study, after drying the green holy basil leaves, levels of TPC, DPPH, and terpenoids were highest at 400 W MD and similar to that of TD treatment. In contrast, red holy basil leaves showed the highest of these secondary metabolites and antioxidants at 600 W of MD with HAD. However, the highest power of MD caused degradation in secondary metabolites and antioxidants in green holy basil leaves, whereas red holy basil leaves were not significantly affected by the highest power of MD. This result is consistent with previous reports that found that phenolics and flavonoids decreased at higher microwave powers in herbal leaves and cereal grains (Xiao et al., 2008; Ragaee et al., 2014; Thiangma et al., 2022; Zia et al., 2022). This may be because high microwave power levels beyond a threshold limit cause oxidation and thermal degradation of aromatic compounds by enzyme inactivation, causing decreases in bioactive compound levels (Nüchter et al., 2004; Thiangma et al., 2022). Optimization of drying and operating conditions mainly depends on the characteristics of plant raw materials as well as processing methods. This necessitates that the drying conditions be chosen based on the type of herbal material. Our findings clearly indicate that dried leaves of green holy basil with the highest TPC, terpenoids, and antioxidant contents were found at 400 W of MD with HAD.
The major components among volatile organic compounds (VOCs) of holy basil are phenylpropanoids and terpenoids (Chutimanukul et al., 2022a,b). Several target compounds have shown beneficial impacts on humans, including antioxidant properties, antibiotic-resistant bacteria, and antifungal and anti-inflammatory activities (Burt, 2004; Pandey et al., 2017). The target compounds required by food, pharmaceutical, and cosmetic industries are methyl eugenol, eugenol, Linalool, and β-caryophyllene content, which are the primary VOC compounds produced in holy basil (Vyas et al., 2014; Chutimanukul et al., 2022b). Eugenol and methyl eugenol are widely used for flavoring foods and have been extensively used for their anti-inflammatory, antioxidant, antibacterial, and analgesic properties (De Vincenzi et al., 2000; Choi et al., 2010; Pramod et al., 2010; Nejad et al., 2017). Linalool is a monoterpene that occurs naturally in aromatic plants and is commonly used as a chemical ingredient for flavor and fragrance in cosmetics (Arctander, 1969; Villa et al., 2007; Kamatou and Viljoen, 2008). β-caryophyllene is the primary sesquiterpene used in medicinal applications due to its anticancer (Fidyt et al., 2016), diabetes (Basha and Sankaranarayanan, 2016), colitis (Dutra et al., 2011), anxiety, and depression properties (Bahi et al., 2014).
Our study showed that VOC content was influenced by drying variables, particularly in green holy basil (Figure 6). For green holy basil leaves, raising the power of MD decreased eugenol and linalool content (Figures 6A,C). However, raising the MD power from 200 to 400 W increased the content of methyl eugenol and β-caryophyllene, especially at the maximum power of MD 600 W (Figures 6B,D). For red holy basil, the drying treatment did not significantly affect the content of eugenol, methyl eugenol, and Linalool. Interestingly, increasing MD power caused higher β-caryophyllene content in red holy basil leaves (Figure 6). This was consistent with previous reports (Harborne et al., 1993) that examined the effect of different drying processes on the yield of volatile products in plants and vegetables. The physicochemical changes of volatile compounds during the drying process depend on several factors, such as the drying method, post-harvest practice, and the instability of essential properties (Venskutonis, 1997; Thamkaew et al., 2021). One of the most important chemical alterations of VOCs is oxidation and isomerization, which are deterioration processes of secondary products either enzymatically or chemically (Turek and Stintzing, 2013). Moreover, heat promotes the initial formation of free radicals, which catalyzes the autoxidation reaction (Lee et al., 2007). These results suggest the highest power at 600 W of MD with HAD condition could maintain the methyl eugenol and β-caryophyllene content in green holy basil leaves, while 400 and 600 W of MD could induce higher contents of β-caryophyllene in dried leaves of red holy basil. This finding should be of great value in fine-tuning the drying processes of holy basil in terms of food quality preservation and product development. Notably, the application of MD power with the HAD method could be used to produce high-value products while maintaining desired characteristics for the food and cosmetic industries.
Conclusion
Our study has established that the use of microwave drying (MD) combined with hot air drying at 45°C (HAD) can be successfully used to preserve the quality, bioactive compounds, and volatile organic compounds (VOCs) of the two cultivars of holy basil leaf for food and pharmaceutical industries. Our data clearly indicates that MD power at 600 W with HAD has the highest ability to maintain color change of leaf in both cultivars by decreasing chlorophyll and carotenoid content. While 400 W of MD with HAD can be proposed for stabilization of TPC and DPPH free radical scavenging activity in green holy bail leaves, these bioactive compounds in red cultivars had the highest accumulation at 600 W of MD treatment. Moreover, 600 W of MD with HAD also exhibited the highest VOC content comprising methyl eugenol and β-caryophyllene for both cultivars. Based on the findings of our study, using 600 W of MD proved to be highly efficient in terms of energy savings resulting from significantly shortened drying times. This alternative method of drying shows promise as a viable option for green and red holy basils and could potentially replace conventional drying methods. The adoption of these alternatives could lead to better product qualities and higher bioactive compound retention, as well as lower electrical energy consumption at industrial scales.
Data availability statement
The original contributions presented in the study are included in the article/supplementary material, further inquiries can be directed to the corresponding author.
Author contributions
PC, TT, and TS: study conception and design. LW, HJ, AT, and PC: data collection. LW, NP-a, KM, and PC: analysis and interpretation of results. LW and PC: writing the original draft. CD: revise the manuscript. PC and CD: writing review and editing. All authors reviewed the results and approved the final version of the manuscript.
Funding
This research was supported by the National Center for Genetic Engineering and Biotechnology (BIOTEC), Thailand (P1951788), and Mahasarakham University.
Acknowledgments
The authors would like to thank Patiphan Charat and Wannisa Harnchiew for their help in plant factory experiments and Jirada Rodsom for preparing all the figures for this manuscript.
Conflict of interest
The authors declare that the research was conducted in the absence of any commercial or financial relationships that could be construed as a potential conflict of interest.
Publisher’s note
All claims expressed in this article are solely those of the authors and do not necessarily represent those of their affiliated organizations, or those of the publisher, the editors and the reviewers. Any product that may be evaluated in this article, or claim that may be made by its manufacturer, is not guaranteed or endorsed by the publisher.
References
Afzal, M. F., Khalid, W., Akram, S., Khalid, M. A., Zubair, M., Kauser, S., et al. (2022). Bioactive profile and functional food applications of banana in food sectors and health: a review. Int. J. Food Prop. 25, 2286–2300. doi: 10.1080/10942912.2022.2130940
Agarwal, K., Singh, D. K., Jyotshna, J., Ahmad, A., Shanker, K., Tandon, S., et al. (2017). Antioxidative potential of two chemically characterized Ocimum (Tulsi) species extracts. Biomed. Res. Ther. 4, 1574–1590. doi: 10.15419/bmrat.v4i9.366
Alibas, I., Zia, M. P., and Yilmaz, A. (2019). The effect of drying methods on color and chlorophyll content of parsley leaves. Turk. J. Agric. Food Sci. Technol. 7, 919–926. doi: 10.24925/turjaf.v7i6.919-926.2548
AOAC (1995). Official methods of analysis 16th ed. Association of Official Analytical Chemists. Washington, DC.
Arctander, S. (1969). Perfume and flavor chemicals: (aroma chemicals) Allured Publishing Corporation. USA: The University of Michigan.
Arias, R., Lee, T.-C., Logendra, L., and Janes, H. (2000). Correlation of lycopene measured by HPLC with the L*, a*, b* color readings of a hydroponic tomato and the relationship of maturity with color and lycopene content. J. Agric. Food Chem. 48, 1697–1702. doi: 10.1021/jf990974e
Arslan, D., and Özcan, M. M. (2010). Study the effect of sun, oven and microwave drying on quality of onion slices. LWT Food Sci. Technol. 43, 1121–1127. doi: 10.1016/j.lwt.2010.02.019
Asha, M., Prashanth, D., Murali, B., Padmaja, R., and Amit, A. (2001). Anthelmintic activity of essential oil of Ocimum sanctum and eugenol. Fitoterapia 72, 669–670. doi: 10.1016/s0367-326x(01)00270-2
Ayala-Silva, T., Winterstein, R. J., Meerow, A. W., Winterstein, M., Cervantes, C., and Brown, J. S. (2005). Determination of color and fruit traits of half-sib families of mango (Mangifera indica L.). Proceed. Flor. State Horticult. Soc. 118, 253–257.
Aziz, A., Noreen, S., Khalid, W., Mubarik, F., Niazi, M. K., Koraqi, H., et al. (2022). Extraction of bioactive compounds from different vegetable sprouts and their potential role in the formulation of functional foods against various disorders: a literature-based review. Molecules 27:7320. doi: 10.3390/molecules27217320
Bahi, A., Al Mansouri, S., Al Memari, E., Al Ameri, M., Nurulain, S. M., and Ojha, S. (2014). β-Caryophyllene, a CB2 receptor agonist produces multiple behavioral changes relevant to anxiety and depression in mice. Physiol. Behav. 135, 119–124. doi: 10.1016/j.physbeh.2014.06.003
Baritaux, O., Richard, H., Touche, J., and Derbesy, M. (1992). Effects of drying and storage of herbs and spices on the essential oil. Part I. Basil, Ocimum basilicum L. Flavour Fragr. J. 7, 267–271. doi: 10.1002/ffj.2730070507
Barrozo, M., Mujumdar, A., and Freire, J. (2014). Air-drying of seeds: a review. Dry. Technol. 32, 1127–1141. doi: 10.1080/07373937.2014.915220
Basha, R. H., and Sankaranarayanan, C. (2016). β-Caryophyllene, a natural sesquiterpene lactone attenuates hyperglycemia mediated oxidative and inflammatory stress in experimental diabetic rats. Chem. Biol. Interact. 245, 50–58. doi: 10.1016/j.cbi.2015.12.019
Burt, S. (2004). Essential oils: their antibacterial properties and potential applications in foods—a review. Int. J. Food Microbiol. 94, 223–253. doi: 10.1016/j.ijfoodmicro.2004.03.022
Chaikham, P., Kreungngern, D., and Apichartsrangkoon, A. (2013). Combined microwave and hot air convective dehydration on physical and biochemical qualities of dried longan flesh. Int. Food Res. J. 20:2145.
Chen, X. D., and Mujumdar, A. S. (2009). Drying technologies in food processing John Wiley & Sons. Canada: Toronto, Ontario M4P 2Y3.
Chen, X., Zhan, L., Pu, Y., Huang, M., Chen, X., Guan, C., et al. (2019). Variation of voids and inter-layer shear strength of advanced polymer-matrix composites at different pressures with high-pressure microwave. J. Eng. Fib. Fab. 14:155892501986395. doi: 10.1177/1558925019863958
Choi, Y. K., Cho, G.-S., Hwang, S., Kim, B. W., Lim, J. H., Lee, J.-C., et al. (2010). Methyleugenol reduces cerebral ischemic injury by suppression of oxidative injury and inflammation. Free Radic. Res. 44, 925–935. doi: 10.3109/10715762.2010.490837
Chutimanukul, P., Jindamol, H., Thongtip, A., Korinsak, S., Romyanon, K., Toojinda, T., et al. (2022a). Physiological responses and variation in secondary metabolite content among Thai holy basil cultivars (Ocimum tenuiflorum L.) grown under controlled environmental conditions in a plant factory. Front. Plant Sci. 13:1008917. doi: 10.3389/fpls.2022.1008917
Chutimanukul, P., Wanichananan, P., Janta, S., Toojinda, T., Darwell, C. T., and Mosaleeyanon, K. (2022b). The influence of different light spectra on physiological responses, antioxidant capacity and chemical compositions in two holy basil cultivars. Sci. Rep. 12, 588–517. doi: 10.1038/s41598-021-04577-x
Darrah, H. H. (1974). Investigation of the cultivars of the basils (Ocimum). Econ Bot 28, 63–67. doi: 10.1007/BF02861381
De Vincenzi, M., Silano, M., Stacchini, P., and Scazzocchio, B. (2000). Constituents of aromatic plants: I. Methyleugenol. Fitoterapia 71, 216–221. doi: 10.1016/s0367-326x(99)00150-1
Demiray, E., Seker, A., and Tulek, Y. (2017). Drying kinetics of onion (Allium cepa L.) slices with convective and microwave drying. Heat Mass Transf. 53, 1817–1827. doi: 10.1007/s00231-016-1943-x
Dev, S., Geetha, P., Orsat, V., Gariépy, Y., and Raghavan, G. (2011). Effects of microwave-assisted hot air drying and conventional hot air drying on the drying kinetics, color, rehydration, and volatiles of Moringa oleifera. Dry. Technol. 29, 1452–1458. doi: 10.1080/07373937.2011.587926
Di Cesare, L. F., Forni, E., Viscardi, D., and Nani, R. C. (2003). Changes in the chemical composition of basil caused by different drying procedures. J. Agric. Food Chem. 51, 3575–3581. doi: 10.1021/jf021080o
Díaz-Maroto, M. C., Pérez-Coello, M. S., and Cabezudo, M. D. (2002). Effect of drying method on the volatiles in bay leaf (Laurus nobilis L.). J. Agric. Food Chem. 50, 4520–4524. doi: 10.1021/jf011573d
Dixon, R. A., and Paiva, N. L. (1995). Stress-induced phenylpropanoid metabolism. Plant Cell 7:1085. doi: 10.2307/3870059
Doymaz, I., KipÇak, A., and Piskin, S. (2015). Microwave drying of green bean slices: drying kinetics and physical quality. Czech J. Food Sci. 33, 367–376. doi: 10.17221/566/2014-CJFS
Dutra, R. C., Claudino, R. F., Bento, A. F., Marcon, R., Schmidt, E. C., Bouzon, Z. L., et al. (2011). Preventive and therapeutic euphol treatment attenuates experimental colitis in mice. PLoS One 6:e27122. doi: 10.1371/journal.pone.0027122
Fidyt, K., Fiedorowicz, A., Strządała, L., and Szumny, A. (2016). β-Caryophyllene and β-caryophyllene oxide—natural compounds of anticancer and analgesic properties. Cancer Med. 5, 3007–3017. doi: 10.1002/cam4.816
Francis, F. (1980). Color quality evaluation of horticultural crops. HortScience 15, 58–59. doi: 10.21273/HORTSCI.15.1.58
García, M. A., Pinotti, A., Martino, M. N., and Zaritzky, N. E. (2009). “Characterization of starch and composite edible films and coatings,” in Eds. Embuscado, M. E., and Huber, K. C. Edible films and coatings for food applications (New York, USA: Springer), 169–209.
Ghorai, N., Chakraborty, S., Gucchait, S., Saha, S. K., and Biswas, S. (2012). Estimation of total terpenoids concentration in plant tissues using a monoterpene, Linalool as standard reagent. Protocol. Exchange. 1–5. doi: 10.1038/protex.2012.055
Godhwani, S., Godhwani, J., and Was, D. (1988). Ocimum sanctum—a preliminary study evaluating its immunoregulatory profile in albino rats. J. Ethnopharmacol. 24, 193–198. doi: 10.1016/0378-8741(88)90151-1
Gupta, S., Mediratta, P. K., Singh, S., Sharma, K., and Shukla, R. (2006). Antidiabetic, antihypercholesterolaemic and antioxidant effect of Ocimum sanctum (Linn) seed oil. Indian J. Exp. Biol. 44, 300–304.
Harborne, J., Baxter, H., and Moss, G. (1993). A handbook of bioactive compounds from plants. London: Taylor and Francis.
Hemis, M., Singh, C., and Jayas, D. (2011). Microwave-assisted thin layer drying of wheat. Dry. Technol. 29, 1240–1247. doi: 10.1080/07373937.2011.584999
Hihat, S., Remini, H., and Madani, K. (2017). Effect of oven and microwave drying on phenolic compounds and antioxidant capacity of coriander leaves. Int. Food Res. J. 24, 503–509.
Horuz, E., and Maskan, M. (2015). Hot air and microwave drying of pomegranate (Punica granatum L.) arils. J. Food Sci. Technol. 52, 285–293. doi: 10.1007/s13197-013-1032-9
Hou, L., Li, R., Wang, S., and Datta, A. K. (2021). Numerical analysis of heat and mass transfers during intermittent microwave drying of Chinese jujube (Zizyphus jujuba miller). Food Bioprod. Process. 129, 10–23. doi: 10.1016/j.fbp.2021.06.005
Islam, M., Saha, T., Monalisa, K., and Hoque, M. (2019). Effect of starch edible coating on drying characteristics and antioxidant properties of papaya. J. Food Measur. Charact. 13, 2951–2960. doi: 10.1007/s11694-019-00215-3
Izli, N., Izli, G., and Taskin, O. (2017). Drying kinetics, colour, total phenolic content and antioxidant capacity properties of kiwi dried by different methods. J. Food Measur. Charact. 11, 64–74. doi: 10.1007/s11694-016-9372-6
Jayaraman, K., and Gupta, D. D. (2020). “Drying of fruits and vegetables,” in Mujumdar, A. S. Handbook of industrial drying (Boca Raton: CRC Press), 643–690.
Jia, Y., Khalifa, I., Hu, L., Zhu, W., Li, J., Li, K., et al. (2019). Influence of three different drying techniques on persimmon chips’ characteristics: a comparison study among hot-air, combined hot-air-microwave, and vacuum-freeze drying techniques. Food Bioprod. Process. 118, 67–76. doi: 10.1016/j.fbp.2019.08.018
Jirovetz, L., Buchbauer, G., Shafi, M. P., and Kaniampady, M. M. (2003). Chemotaxonomical analysis of the essential oil aroma compounds of four different Ocimum species from southern India. Eur. Food Res. Technol. 217, 120–124. doi: 10.1007/s00217-003-0708-1
Kadian, R., and Parle, M. (2012). Therapeutic potential and phytopharmacology of tulsi. Int. J. Pharm. Life Sci. 3, 1858–1867.
Kainat, S., Arshad, M. S., Khalid, W., Zubair Khalid, M., Koraqi, H., Afzal, M. F., et al. (2022). Sustainable novel extraction of bioactive compounds from fruits and vegetables waste for functional foods: a review. Int. J. Food Prop. 25, 2457–2476. doi: 10.1080/10942912.2022.2144884
Kamatou, G. P., and Viljoen, A. M. (2008). Linalool–a review of a biologically active compound of commercial importance. Nat. Prod. Commun. 3:1934578X0800300. doi: 10.1177/1934578X0800300727
Kaveh, M., Golpour, I., Gonçalves, J. C., Ghafouri, S., and Guiné, R. (2021). Determination of drying kinetics, specific energy consumption, shrinkage, and colour properties of pomegranate arils submitted to microwave and convective drying. Open Agric. 6, 230–242. doi: 10.1515/opag-2020-0209
Kelm, M. A., and Nair, M. G. (1998). Mosquitocidal compounds and a triglyceride, 1, 3-dilinoleneoyl-2-palmitin, from Ocimum sanctum. J. Agric. Food Chem. 46, 3092–3094. doi: 10.1021/jf980304t
Kelm, M., Nair, M., Strasburg, G., and Dewitt, D. (2000). Antioxidant and cyclooxygenase inhibitory phenolic compounds from Ocimum sanctum Linn. Phytomedicine 7, 7–13. doi: 10.1016/S0944-7113(00)80015-X
Khalid, W., Ali, A., Arshad, M. S., Afzal, F., Akram, R., Siddeeg, A., et al. (2022). Nutrients and bioactive compounds of Sorghum bicolor L. used to prepare functional foods: a review on the efficacy against different chronic disorders. Int. J. Food Prop. 25, 1045–1062. doi: 10.1080/10942912.2022.2071293
Kumar, A., Shukla, R., Singh, P., and Dubey, N. K. (2010). Chemical composition, antifungal and antiaflatoxigenic activities of Ocimum sanctum L. essential oil and its safety assessment as plant based antimicrobial. Food Chem. Toxicol. 48, 539–543. doi: 10.1016/j.fct.2009.11.028
Lee, J., Lee, Y., and Choe, E. (2007). Temperature dependence of the autoxidation and antioxidants of soybean, sunflower, and olive oil. Eur. Food Res. Technol. 226, 239–246. doi: 10.1007/s00217-006-0532-5
Li, M., Wang, B., Lv, W., Lin, R., and Zhao, D. (2022). Characterization of pre-gelatinized kidney bean (Phaseolus vulgaris L.) produced using microwave hot-air flow rolling drying technique. LWT 154:112673. doi: 10.1016/j.lwt.2021.112673
Liu, H., Liu, H., Liu, H., Zhang, X., Hong, Q., Chen, W., et al. (2021). Microwave drying characteristics and drying quality analysis of corn in China. PRO 9:1511. doi: 10.3390/pr9091511
Lozano, J., and Ibarz, A. (1997). Colour changes in concentrated fruit pulp during heating at high temperatures. J. Food Eng. 31, 365–373. doi: 10.1016/S0260-8774(96)00079-9
Maqbool, Z., Khalid, W., Atiq, H. T., Koraqi, H., Javaid, Z., Alhag, S. K., et al. (2023). Citrus waste as source of bioactive compounds: extraction and utilization in health and food industry. Molecules 28:1636. doi: 10.3390/molecules28041636
Mouhoubi, K., Boulekbache-Makhlouf, L., Mehaba, W., Himed-Idir, H., and Madani, K. (2022). Convective and microwave drying of coriander leaves: kinetics characteristics and modeling, phenolic contents, antioxidant activity, and principal component analysis. J. Food Process Eng. 45:e13932. doi: 10.1111/jfpe.13932
Mukherjee, R., Dash, P., and Ram, G. (2005). Immunotherapeutic potential of Ocimum sanctum (L) in bovine subclinical mastitis. Res. Vet. Sci. 79, 37–43. doi: 10.1016/j.rvsc.2004.11.001
Nandkarni, A. K. (1982). Indian materia medica popular prakashan Bombay I&II (reprinted). Published by Popular Prakashan Pvt Ltd, 2000.
Nejad, S. M., Özgüneş, H., and Başaran, N. (2017). Pharmacological and toxicological properties of eugenol. Turk. J. Pharm. Sci. 14, 201–206. doi: 10.4274/tjps.62207
Nguyen, V. T., and Le, M. D. (2018). Influence of various drying conditions on phytochemical compounds and antioxidant activity of carrot peel. Beverages 4:80. doi: 10.3390/beverages4040080
Nüchter, M., Ondruschka, B., Bonrath, W., and Gum, A. (2004). Microwave assisted synthesis–a critical technology overview. Green Chem. 6, 128–141. doi: 10.1039/B310502D
Orikasa, T., Koide, S., Okamoto, S., Imaizumi, T., Muramatsu, Y., Takeda, J.-I., et al. (2014). Impacts of hot air and vacuum drying on the quality attributes of kiwifruit slices. J. Food Eng. 125, 51–58. doi: 10.1016/j.jfoodeng.2013.10.027
Orphanides, A., Goulas, V., and Gekas, V. (2013). Effect of drying method on the phenolic content and antioxidant capacity of spearmint. Czech J. Food Sci. 31, 509–513. doi: 10.17221/526/2012-CJFS
Ozcan-Sinir, G., Ozkan-Karabacak, A., Tamer, C. E., and Copur, O. U. (2018). The effect of hot air, vacuum and microwave drying on drying characteristics, rehydration capacity, color, total phenolic content and antioxidant capacity of kumquat (Citrus japonica). Food Sci. Technol. 39, 475–484. doi: 10.1590/fst.34417
Pandey, A. K., Kumar, P., Singh, P., Tripathi, N. N., and Bajpai, V. K. (2017). Essential oils: sources of antimicrobials and food preservatives. Front. Microbiol. 7:2161. doi: 10.3389/fmicb.2016.02161
Pathare, P. B., Opara, U. L., and Al-Said, F. A.-J. (2013). Colour measurement and analysis in fresh and processed foods: a review. Food Bioprocess Technol. 6, 36–60. doi: 10.1007/s11947-012-0867-9
Poogungploy, P., Poomsa-Ad, N., and Wiset, L. (2018). Control of microwave assisted macadamia drying. J. Microw. Power Electromagn. Energy. 52, 60–72. doi: 10.1080/08327823.2017.1421872
Poós, T., and Varju, E. (2017). Drying characteristics of medicinal plants. Int. Rev. Appl. Sci. Eng. 8, 83–91. doi: 10.1556/1848.2017.8.1.12
Pradechboon, T., Dussadee, N., Unpaprom, Y., and Chindaraksa, S. (2022). Effect of rotary microwave drying on quality characteristics and physical properties of kaffir lime leaf (Citrus hystrix DC). Biomass Convers. Bioref., 1–10. doi: 10.1007/s13399-022-02722-8
Pramod, K., Ansari, S. H., and Ali, J. (2010). Eugenol: a natural compound with versatile pharmacological actions. Nat. Prod. Commun. 5:1934578X1000501. doi: 10.1177/1934578X1000501236
Preparations WECoSfP and World Health Organization (1996). WHO expert committee on specifications for pharmaceutical Preparations: thirty-fourth report. Geneva, Switzerland: World Health Organization.
Ragaee, S., Seetharaman, K., and Abdel-Aal, E.-S. M. (2014). The impact of milling and thermal processing on phenolic compounds in cereal grains. Crit. Rev. Food Sci. Nutr. 54, 837–849. doi: 10.1080/10408398.2011.610906
Rahman, M. S., and Labuza, T. P. (2007). “Water activity and food preservation,” in Ed. Rahman, M. S. Handbook of food preservation (CRC Press), 465–494.
Raksakantong, P., Siriamornpun, S., Ratseewo, J., and Meeso, N. (2011). Optimized drying of Kaprow leaves for industrial production of holy basil spice powder. Dry. Technol. 29, 974–983. doi: 10.1080/07373937.2011.558649
Rice-Evans, C. (2001). Flavonoid antioxidants. Curr. Med. Chem. 8, 797–807. doi: 10.2174/0929867013373011
Salles Trevisan, M. T., Vasconcelos Silva, M. G., Pfundstein, B., Spiegelhalder, B., and Owen, R. W. (2006). Characterization of the volatile pattern and antioxidant capacity of essential oils from different species of the genus Ocimum. J. Agric. Food Chem. 54, 4378–4382. doi: 10.1021/jf060181+
Sang, S. Y., Jamharee, F., Prasad, K. N., Azlan, A., and Maliki, N. (2014). Influence of drying treatments on antioxidant capacity of forage legume leaves. J. Food Sci. Technol. 51, 988–993. doi: 10.1007/s13197-011-0596-5
Shalaby, S., Darwesh, M., Ghoname, M., Salah, S. E., Nehela, Y., and Fetouh, M. (2020). The effect of drying sweet basil in an indirect solar dryer integrated with phase change material on essential oil valuable components. Energy Rep. 6, 43–50. doi: 10.1016/j.egyr.2020.10.035
Singh, D., and Chaudhuri, P. K. (2018). A review on phytochemical and pharmacological properties of holy basil (Ocimum sanctum L.). Ind. Crop. Prod. 118, 367–382. doi: 10.1016/j.indcrop.2018.03.048
Singh, S., Malhotra, M., and Majumdar, D. (2005). Antibacterial activity of Ocimum sanctum L. fixed oil. Indian J. Exp. Biol. 43, 835–837.
Taghinezhad, E., Kaveh, M., and Szumny, A. (2021). Thermodynamic and quality performance studies for drying kiwi in hybrid hot air-infrared drying with ultrasound pretreatment. Appl. Sci. 11:1297. doi: 10.3390/app11031297
Tarhan, S., Telci, I., Taner Tuncay, M., and Polatci, H. (2011). Peppermint drying performance of contact dryer in terms of product quality, energy consumption, and drying duration. Dry. Technol. 29, 642–651. doi: 10.1080/07373937.2010.520421
Thamkaew, G., Sjöholm, I., and Galindo, F. G. (2021). A review of drying methods for improving the quality of dried herbs. Crit. Rev. Food Sci. Nutr. 61, 1763–1786. doi: 10.1080/10408398.2020.1765309
Thiangma, P., Nakbanpote, W., Poomsa-Ad, N., and Wiset, L. (2022). Effect of drying condition on Shatavari (Asparagus racemosus Willd) root quality and energy consumption. Eng. Access 8, 330–335. doi: 10.14456/mijet.2022.41
Thongtip, A., Mosaleeyanon, K., Korinsak, S., Toojinda, T., Darwell, C. T., Chutimanukul, P., et al. (2022). Promotion of seed germination and early plant growth by KNO3 and light spectra in Ocimum tenuiflorum using a plant factory. Sci. Rep. 12, 6995–6913. doi: 10.1038/s41598-022-11001-5
Turek, C., and Stintzing, F. C. (2013). Stability of essential oils: a review. Compr. Rev. Food Sci. Food Saf. 12, 40–53. doi: 10.1111/1541-4337.12006
Vani, S. R., Cheng, S., and Chuah, C. (2009). Comparative study of volatile compounds from genus Ocimum. Am. J. Appl. Sci. 6, 523–528. doi: 10.3844/ajas.2009.523.528
Varith, J., Dijkanarukkul, P., Achariyaviriya, A., and Achariyaviriya, S. (2007). Combined microwave-hot air drying of peeled longan. J. Food Eng. 81, 459–468. doi: 10.1016/j.jfoodeng.2006.11.023
Vats, V., Yadav, S., and Grover, J. (2004). Ethanolic extract of Ocimum sanctum leaves partially attenuates streptozotocin-induced alterations in glycogen content and carbohydrate metabolism in rats. J. Ethnopharmacol. 90, 155–160. doi: 10.1016/j.jep.2003.09.034
Venskutonis, P. (1997). Effect of drying on the volatile constituents of thyme (Thymus vulgaris L.) and sage (Salvia officinalis L.). Food Chem. 59, 219–227. doi: 10.1016/S0308-8146(96)00242-7
Villa, C., Gambaro, R., Mariani, E., and Dorato, S. (2007). High-performance liquid chromatographic method for the simultaneous determination of 24 fragrance allergens to study scented products. J. Pharm. Biomed. Anal. 44, 755–762. doi: 10.1016/j.jpba.2007.03.020
Voss, D. H. (1992). Relating colorimeter measurement of plant color to the Royal Horticultural Society Colour Chart. HortScience 27, 1256–1260. doi: 10.21273/HORTSCI.27.12.1256
Vyas, P., Haque, I., Kumar, M., and Mukhopadhyay, K. (2014). Photocontrol of differential gene expression and alterations in foliar anthocyanin accumulation: a comparative study using red and green forma Ocimum tenuiflorum. Acta Physiol. Plant. 36, 2091–2102. doi: 10.1007/s11738-014-1586-9
Waliat, S., Arshad, M. S., Hanif, H., Ejaz, A., Khalid, W., Kauser, S., et al. (2023). A review on bioactive compounds in sprouts: extraction techniques, food application and health functionality. Int. J. Food Prop. 26, 647–665. doi: 10.1080/10942912.2023.2176001
Wellburn, A. R. (1994). The spectral determination of chlorophylls a and b, as well as total carotenoids, using various solvents with spectrophotometers of different resolution. J. Plant Physiol. 144, 307–313. doi: 10.1016/S0176-1617(11)81192-2
Wiset, L., Poomsa-Ad, N., and Onsaard, W. (2021). Drying characteristics and quality evaluation in microwave-assisted hot air drying of cherry tomato. Eng. Appl. Sci. Res. 48, 724–731. doi: 10.14456/easr.2021.74
Workneh, T. S., and Oke, M. O. (2012). The influence of the combined microwave power and hot air ventilation on the drying kinetics and color quality of tomato slices. Afr. J. Biotechnol. 11, 15353–15364. doi: 10.5897/AJB11.318
Wulyapash, W., Phongphiphat, A., and Towprayoon, S. (2022). Comparative study of hot air drying and microwave drying for dewatered sludge. Clean Techn. Environ. Policy, 24, 423–436. doi: 10.1007/s10098-021-02242-5
Xiao, W., Han, L., and Shi, B. (2008). Microwave-assisted extraction of flavonoids from Radix Astragali. Sep. Purif. Technol. 62, 614–618. doi: 10.1016/j.seppur.2008.03.025
Yanpallewar, S., Rai, S., Kumar, M., and Acharya, S. (2004). Evaluation of antioxidant and neuroprotective effect of Ocimum sanctum on transient cerebral ischemia and long-term cerebral hypoperfusion. Pharmacol. Biochem. Behav. 79, 155–164. doi: 10.1016/j.pbb.2004.07.008
Yen, G. C., and Duh, P. D. (1994). Scavenging effect of methanolic extracts of peanut hulls on free-radical and active-oxygen species. J. Agric. Food Chem. 42, 629–632. doi: 10.1021/jf00039a005
Yilmaz, A., and Alibas, I. (2021). The impact of drying methods on quality parameters of purple basil leaves. J. Food Proces. Preserv. 45:e15638. doi: 10.1111/jfpp.15638
Yousif, A. N., Scaman, C. H., Durance, T. D., and Girard, B. (1999). Flavor volatiles and physical properties of vacuum-microwave-and air-dried sweet basil (Ocimum basilicum L.). J. Agric. Food Chem. 47, 4777–4781. doi: 10.1021/jf990484m
Yue, T., Xing, Y., Xu, Q., Yang, S., Xu, L., Wang, X., et al. (2021). Physical and chemical properties of purple cabbage as affected by drying conditions. Int. J. Food Prop. 24, 997–1010. doi: 10.1080/10942912.2021.1953070
Zeng, S., Wang, B., Lv, W., and Wu, Y. (2023). Effects of microwave power and hot air temperature on the physicochemical properties of dried ginger (Zingiber officinale) using microwave hot-air rolling drying. Food Chem. 404:134741. doi: 10.1016/j.foodchem.2022.134741
Zia, M. P., and Alibas, I. (2021). Influence of the drying methods on color, vitamin C, anthocyanin, phenolic compounds, antioxidant activity, and in vitro bioaccessibility of blueberry fruits. Food Biosci. 42:101179. doi: 10.1016/j.fbio.2021.101179
Zia, S., Khan, M. R., Shabbir, M. A., Aslam Maan, A., Khan, M. K. I., Nadeem, M., et al. (2022). An inclusive overview of advanced thermal and nonthermal extraction techniques for bioactive compounds in food and food-related matrices. Food Rev. Intl. 38, 1166–1196. doi: 10.1080/87559129.2020.1772283
Keywords: Ocimum Tenuiflorum L., secondary metabolite, plant factory, bioactive compounds, drying methods, tray drying
Citation: Wiset L, Poomsa-ad N, Jindamol H, Thongtip A, Mosaleeyanon K, Toojinda T, Darwell CT, Saputro TB and Chutimanukul P (2023) Quality and bioactive compound accumulation in two holy basil cultivars as affected by microwave-assisted hot air drying at an industrial scale. Front. Sustain. Food Syst. 7:1219540. doi: 10.3389/fsufs.2023.1219540
Edited by:
Abid Hussain, Karakoram International University, PakistanReviewed by:
Waseem Khalid, Government College University Faisalabad, PakistanWeerachet Jittanit, Kasetsart University, Thailand
Mohan Naik, University of Horticultural Sciences, Bagalkot, India
Copyright © 2023 Wiset, Poomsa-ad, Jindamol, Thongtip, Mosaleeyanon, Toojinda, Darwell, Saputro and Chutimanukul. This is an open-access article distributed under the terms of the Creative Commons Attribution License (CC BY). The use, distribution or reproduction in other forums is permitted, provided the original author(s) and the copyright owner(s) are credited and that the original publication in this journal is cited, in accordance with accepted academic practice. No use, distribution or reproduction is permitted which does not comply with these terms.
*Correspondence: Panita Chutimanukul, cGFuaXRhLmNodUBiaW90ZWMub3IudGg=