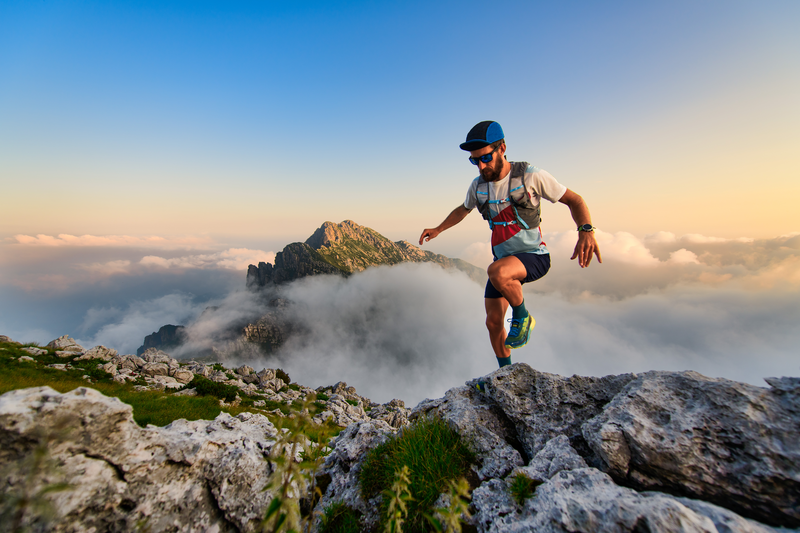
95% of researchers rate our articles as excellent or good
Learn more about the work of our research integrity team to safeguard the quality of each article we publish.
Find out more
ORIGINAL RESEARCH article
Front. Sustain. Food Syst. , 23 August 2023
Sec. Agro-Food Safety
Volume 7 - 2023 | https://doi.org/10.3389/fsufs.2023.1216273
Introduction: Chlorine dioxide (ClO2) is a promising antimicrobial with various food applications, one of those being inclusion in packaging. The purpose of this study was to evaluate a novel ClO2-based antimicrobial packaging system (InvisiShield™) for its efficacy against human norovirus (hNoV) and hepatitis A virus (HAV) in refrigerated fresh produce.
Methods: Grape tomatoes or blueberries were placed in polypropylene trays and selectively inoculated with 6.0 log10 hNoV Genome Equivalent Copies (GEC; 20% stool suspension) or 6.2 log10 HAV GEC (cell culture lysate). Trays were heat sealed with a three-phase polymer film consisting of a base, channeling agent, and the ClO2 active (treatment); or control (no active) film and stored at 7°C for 24, 48 h, and 7 days. At each timepoint, the product was collected and processed for virus concentration using the sequential steps of elution and polyethylene glycol precipitation. Viruses in extracts were quantified using RNase-RT-qPCR.
Results and discussion: Log10 reductions (LR) in hNoV GEC for tomatoes were 2.2 ± 1.3, 2.9 ± 0.7, and 3.6 ± 0.3, after 24, 48 h and 7 days, respectively. For blueberries, hNoV LR were 1.4 ± 0.7, 1.7 ± 0.5, and 2.7 ± 0.2 GEC, respectively. Hepatitis A virus GEC LR were 0.4 ± 0.2, 1.0 ± 0.1, and 2.1 ± 0.7 for tomatoes, and 0.1 ± 0.2, 1.2 ± 0.4, and 3.2 ± 0.2 for blueberries, after 24, 48 h and 7 days, respectively. Position of the fruit in the tray did not affect inactivation (p > 0.05). Sensory analysis on the treated tomato products revealed no statistically significant difference in appearance, flavor and texture attributes compared to the control. This novel ClO2-based antimicrobial packaging system effectively reduced concentrations of hNoV and HAV, as evaluated using reduction in GEC as proxy for infectivity, on grape tomatoes and blueberries after one day, with efficacy improving over 7 days of refrigerated storage. This technology shows promise as an antiviral treatment as applied to refrigerated fresh produce items.
Foodborne illness is a major cause of illness worldwide. In the United States alone, there are an estimated 9.4 million cases occurring every year (Scallan et al., 2011). A considerable number of these are attributed to outbreaks involving contaminated fresh produce (Lynch et al., 2008; Bennett et al., 2018; Chatziprodromidou et al., 2018; Carstens et al., 2019). From 1998 to 2013, a total of 972 outbreaks involving fresh produce were reported through U.S. Centers for Disease Control and Prevention’s Foodborne Disease Outbreak Surveillance System, resulting in a total of 34,674 illnesses, 2,315 hospitalizations, and 72 deaths (Bennett et al., 2018).
Pathogens commonly associated with fresh produce outbreaks include bacteria such as Listeria monocytogenes, Escherichia coli and Salmonella enterica (Carstens et al., 2019), as well as viral pathogens such as human norovirus (hNoV) and hepatitis A virus (HAV) (Chatziprodromidou et al., 2018). Human noroviruses are the leading cause of foodborne disease outbreaks, with over 5 million food-associated cases estimated per year (Scallan et al., 2011). Leafy vegetables, fruits and nuts are the general categories of produce items associated with hNoV outbreaks (Hall et al., 2012). Although often the source of contamination in hNoV-produce outbreaks is not definitively established, infected food handlers at the preparation phase are a commonly implicated route (Hall et al., 2012). Hepatitis A virus, while a less common cause of produce-associated outbreaks, causes a more severe disease and has been associated with fresh produce including, green onions (Centers for Disease Control and Prevention, 2003) and lettuce (Rosenblum et al., 2011). As described for hNoV, the most common source of contamination is the hands of infected food handlers who have not practiced adequate personal hygiene.
Of the produce items most often implicated in virus outbreaks, berries stand out. In fact, virus-contaminated raspberries, strawberries, and blueberries have been the cause of notable hNoV and HAV outbreaks, some very large and high profile (Bozkurt et al., 2021). For instance, the largest recorded foodborne outbreak in German history, with nearly 11,000 cases, was caused by hNoV contamination of frozen strawberries (Bernard et al., 2014). Similarly, a large and prolonged foodborne outbreak in Italy was caused by HAV contamination of frozen berries, resulting in greater than 1,800 cases spanning over 12 months (Scavia et al., 2017). Virus outbreaks associated with tomatoes, while occurring less frequently, have also been reported (Donnan et al., 2012; Shieh et al., 2014), including a multistate Australian HAV outbreak associated with semi-dried tomatoes and resulting in over 500 cases (Donnan et al., 2012).
Human norovirus and HAV are environmentally stable and are quite recalcitrant to many processes and sanitizers/disinfectants used routinely in food processing and preservation, including pH and water activity manipulation, high pressure, alcohols and quaternary ammonium compounds (Li et al., 2012; Bosch et al., 2018). Chlorine and chlorine dioxide (ClO2) have shown relatively broad efficacy against various microorganisms (Park et al., 2018), including hNoV and HAV (and their surrogates) (Li et al., 2004; Lim et al., 2010; Kingsley et al., 2014; Song et al., 2022). In fact, CDC recommends high concentrations of hypochlorite (1,000-5,000 ppm) when cleaning surfaces suspected of being contaminated with hNoV. Chlorine dioxide gas has demonstrated efficacy for decontamination and preservation of candidate food products such as fruits, vegetables and raw meats (Gómez-López et al., 2009; Singh et al., 2021). Unfortunately, ClO2 gas presents unique challenges including difficulty of use in the supply chain, elevated cost, and effects on food product quality, all of which can limit its current commercial use (Ridenour and Ingols, 1947; Singh et al., 2021). Organoleptic issues can include the potential to promote product bleaching and/or browning (Bunzel and Ralph, 2006; Goméz-Lopez et al., 2008). Nonetheless, ClO2 has its advantages, including its rapid dissipation and reduced corrosivity when compared with other forms of chlorine (Singh et al., 2021).
InvisiShield™ technology (hereby referred to as “the product”) is a novel ClO2 gas delivery system for active antimicrobial packaging. The product material contains a base polymer, a channeling agent, and the active ingredient, ClO2. The release of the ClO2 gas is triggered by the relative humidity in the package, which then causes the ClO2 to migrate through the channeling agent into the package in a controlled manner. InvisiShield™ is Generally Recognized as Safe (GRAS) by an expert panel and has regulatory allowance on all fresh fruits and vegetables in the U.S. and Canada. For the EU, data on the technology has been submitted to the European Food Safety Authority’s Active and Intelligence body for review. However, the efficacy of this product against microbial pathogens on foods is yet to be reported in the literature. The purpose of this study was to evaluate this novel ClO2-based antimicrobial packaging system for its efficacy against hNoV and HAV in refrigerated fresh produce.
Human norovirus GII.4 Sydney, obtained as a deidentified human stool specimen from an outbreak (courtesy of Dr. Shermalyn Greene, North Carolina State Laboratory of Public Health, Raleigh, NC), was suspended 20% in phosphate buffered saline (PBS) with clarification by centrifugation (3,100 × g for 5 min at 4°C; Eppendorf 5810R, Eppendorf, Hamburg, Germany). The initial titer of the prepared stock suspension was 6.0 log10 genome equivalent copies (GEC) per ml.
Hepatitis A virus, cultivable strain HM-175, which is routinely used in our lab, was propagated on the rhesus monkey kidney cell line FRhK-4 (CRL-1688, American Type Culture Collection, Manassas, VA) as previously described (Provost and Hilleman, 1979). Infected cells showing CPE were subjected to multiple freeze–thaw cycles and further clarified by centrifugation at 2,800 × g for 20 min (Papafragkou et al., 2008). The initial titer of the HAV cell culture lysate, which was used as inoculum, was 6.2 log10 GEC per ml.
Blueberries and unsliced grape tomatoes were purchased from a local grocery store no more than 3 h prior to inoculation. They were washed in a 200 ppm household bleach solution (diluted in sterile distilled water) for 2 min, rinsed twice with sterile deionized water, and allowed to dry. Twelve tomatoes or twelve blueberries were placed in FreshWell™ food packaging trays (Mid-America Packaging & Distribution, St Louis, MO) and two tomatoes or two blueberries per tray were spot inoculated by adding a 10 μL volume of the challenge viral suspension per fruit item. One of the inoculated samples was placed in the middle of the tray, and the second at the outer edge of the tray. A total of two trays of samples was prepared for each replicate (total of 3–4 experimental replicates) for each sampling time point, and uninoculated controls as well as no treatment controls were included for each replicate/time point. Produce was packaged per manufacturer’s instructions using InvisiShield™ lidding film (the product) or negative control lidding film (Figure 1). Packages were held at 7°C for 0, 24 and 48 h, and 7 days. Treatment and control trays were stored in separate locations to prevent confounding by potential ClO2 outgassing.
Figure 1. Diagram of InvisiShield™ technology, a novel ClO2 gas delivery system, for modified atmosphere packaging.
Treatment trays, and associated controls, were removed from refrigerated storage at the designated timepoints and processed for recovery and enumeration of residual virus. The two inoculated tomatoes or blueberries per package were processed separately. To each fruit was added 0.1 M Tris-base glycine (Fisher Scientific, Waltham, MA) beef extract (Acumedia, Lansing, MI; 3% in sterile distilled water, pH 9.5), which served as eluant and neutralizer, equal to three times the weight of the sample. The viruses were eluted by shaking (SHLD0415DG Orbital Shaker, OHAUS, Parsippany, NJ) at 400 rpm for 20 min at room temperature in filter stomacher bags (Whirl-Pak, Nasco, Atlanta, GA). Eluate solutions were recovered and centrifuged (Eppendorf 5810R) at 10,000 × g for 30 min at 4°C to sediment debris, followed by polyethylene glycol precipitation (12% PEG; Fisher Scientific) overnight at 4°C. Virus-laden precipitates were collected by centrifugation at 10,000 × g for 30 min at 4°C and resuspended in 1 mL of PBS. Sample concentrates were pretreated with RNase (RNase One, Promega, Madison, WI) prior to RNA extraction using the automated EasyMag system (bioMerieux, Durham, NC) as per manufacturer instructions and previously described (Escudero-Abarca et al., 2022). RNA was amplified by RT-qPCR (CFX96, BioRad, Hercules, CA) targeting the conserved ORF1-ORF2 junction of hNoV (Jothikumar et al., 2005) and the highly conserved 5′ noncoding region of HAV (Costafreda et al., 2006). For quantification, the resulting cycle threshold (CT) values were compared to a standard curve produced by serial dilutions of viral RNA obtained from the inoculum. Reduction in GEC as a function of treatment was calculated by subtracting the remaining virus log10 GEC for each treatment from that obtained for the no treatment control.
Tomatoes were purchased commercially from a local wholesaler and used within 3 h of purchase. The tomatoes were washed in a 200 ppm commercial bleach solution for two min and were then rinsed with tap water before slicing. Slicing was done using a Tomato Sabor® 943-D slicer (Prince Castle Carol Stream, IL) that was pre-sterilized with 200 ppm bleach and rinsed with tap water. Tomatoes were sliced with the calyx facing down.
After slicing, the blossom and calyx ends of the tomatoes were discarded, and 42 slices were packed into each tray, some of which contained the antimicrobial, and some of which served as control trays that lacked the antimicrobial. Trays were stored at 4°C for 3 days, based on the assumption that this would be the earliest possible point along the supply chain at which the product would be opened and consumed. Single slices from the control or treatment trays were equilibrated to room temperature and used for the sensory analysis.
The sensory evaluation was conducted using a triangle test to determine if a detectable difference existed between the samples stored for 3 days at 4°C. Panelists were asked to sign consent forms and excluded if they had food allergies or other issues. In addition, panelists were given an orientation to familiarize them with the particulars of the test procedures and characteristics of the product. Panelists were presented three samples in which two were the same and one different, and asked to indicate which sample was different from the other two. They were also instructed to ‘guess’ if they felt there was no difference. The samples were presented in 8-oz plastic cups, with each being labelled with a random 3-digit number. In addition, every combination and order were prepared and counterbalanced across the evaluators- that is ABB, BBA, AAB, BAB, ABA and BAA, where A are samples stored without the antimicrobial and B those stored with the antimicrobial. A total of 42 untrained panelists were recruited from staff, students and faculty at the University of Georgia, Department of Food Science & Technology. Each panelist selected differences based on appearance, flavor and/or texture.
For the triplicate virological tests, results were expressed as mean ± standard deviation of log10 GEC reduction for each produce product at each exposure time. This was used as a proxy to measure degree of virus inactivation. Data were compared statistically using ANOVA and the Tukey–Kramer test (Minitab Statistical Software, State College, PA). Statistical significance was established at a level of p < 0.05. For analysis of sensory data, a Triangle Test of Difference was performed (Poste et al., 1991) to determine if detectable differences existed between treated/control samples for appearance, flavor and texture. Statistical analysis was conducted using tables assuming a binomial distribution. This test is a one-tailed test with the null hypothesis that the probability of making a correct selection by chance alone is one in three. The data were analyzed by tabulating the number of “correct” responses and compared to values in tables for the minimum number of correct responses needed to conclude that a perceptible difference existed at α = 0.001, 0.01, 0.05 or 0.1%.
Position of the fruit in the tray did not affect virus inactivation, as evaluated using reduction of GEC as a proxy for infectivity (p > 0.05), so results for each piece of fruit were combined for each experimental replicate. Log10 reductions (LR) in HAV GEC for tomatoes were 0.4 ± 0.2, 1.0 ± 0.1, and 2.1 ± 0.7 after 24, 48 h and 7 days, respectively (Figure 2A). Efficacy continued to improve at each timepoint but never reached the assay limit of detection (LOD, 3.8 log10 GEC reduction). There was significantly greater reduction in log10 HAV GEC after 7 days compared to 24 and 48 h (p < 0.05). Hepatitis A virus GEC LR for blueberries was 0.1 ± 0.2, 1.2 ± 0.4, and 3.2 ± 0.2 after 24, 48 h and 7 days, respectively and efficacy reached the LOD (3.1 log10 GEC reduction) at 7 days (Figure 2B). For blueberries, there was significantly greater reduction in log10 HAV GEC after 7 days compared to 48 h (p < 0.05), and at 48 h compared to 24 h (p < 0.05).
Figure 2. Inactivation of hepatitis A virus (HAV) on grape tomatoes (A) (n = 4) and blueberries (B) (n = 3) by InvisiShield Technology™, expressed as reduction in log10 HAV genome equivalent copy (GEC). Different letters indicate statistically significant differences in log10 virus GEC reduction on fruits when comparing exposure times (p < 0.05).
Similar to the HAV results, position of the fruit in the tray did not affect virus inactivation, based on GEC reduction (p > 0.05), so results for each piece of fruit were combined for each experimental replicate. Log10 reductions (LR) in hNoV GEC for tomatoes were 2.2 ± 1.3, 2.9 ± 0.7, and 3.6 ± 0.3 after 24, 48 h and 7 days, respectively (Figure 3A). For blueberries, LR in hNoV GEC were 1.4 ± 0.7, 1.7 ± 0.5, and 2.7 ± 0.2 after 24, 48 h and 7 days, respectively (Figure 3B). For both produce types, efficacy against hNoV continued to improve at each time point and reached the LOD at 7 days (3.7 log10 and 2.7 log10 hNoV GEC reduction for tomatoes and blueberries, respectively). However, there were no statistically significant differences in log10 hNoV GEC reduction on tomatoes when comparing exposure times (p > 0.05). For blueberries, there was significantly greater reduction in log10 hNoV GEC reduction after 7 days compared to 24 and 48 h (p < 0.05).
Figure 3. Inactivation of human norovirus (hNoV) on grape tomatoes (A) (n = 3) and blueberries (B) (n = 3) by InvisiShield Technology™, expressed as reduction in log10 hNoV genome equivalent copy (GEC). Different letters indicate statistically significant differences in log10 virus GEC reduction on fruits when comparing exposure times (p < 0.05).
As noted above, the sensory data were analyzed by tabulating the number of correct responses needed to conclude that a perceptible difference existed. For n = 42 panelists, the minimum number needed to conclude a perceptible difference was 22 (α = 0.01) (Meilgaard et al., 2014). Based on this approach, treated tomatoes were rated as not significantly different in appearance, flavor or texture attributes compared to control samples.
Chlorine dioxide gas, when delivered using InvisiShield™ Technology (the product), showed efficacy against both hNoV and HAV on the fresh produce items included in this study (grape tomatoes and blueberries) when stored under refrigeration conditions (7°C). Using log10 GEC reduction as proxy for infectivity, hNoV was inactivated such that reduction approached or reached the limit of detection (LOD; 2.7-3.7 log10 hNoV GEC reduction) on both produce items after 7 days of refrigerated storage. Hepatitis A virus was more difficult to inactivate, but a 2.1 log10 GEC reduction was reached on tomatoes and a 3.1 log10 HAV GEC reduction was observed for blueberries within 7 days. Only the latter was reflective of the assay LOD.
While antimicrobial active packaging is not a new concept, advancements in nanotechnology and developments in packaging technologies has allowed for growth in this field. The most common gaseous compounds used in antimicrobial active packaging include ethanol, carbon dioxide and ClO2 (Chen et al., 2019; Firouz et al., 2021). As reviewed by Firouz et al. (2021), the release of ethanol into a package headspace has been shown to inhibit the growth of yeasts, bacteria and molds on mango, sliced bread and bayberry. Active packaging containing carbon dioxide gas has also been shown to extend the shelf-life of many food products, including meat, fish and fruits (Firouz et al., 2021). The release of ClO2 from a modified film was also been shown to reduce counts of Salmonella spp. and E.coli O157:H7 on tomatoes (Chen et al., 2019). While the efficacy of ClO2 and ClO2 gas against viruses has been described, as detailed below, to our knowledge, this is the first study to evaluate its efficacy against viral pathogens under active packaging conditions.
In general, chlorine and ClO2 gas have been shown to be efficacious against viral pathogens such as HAV and hNoV on fresh produce (Li et al., 2004; Lim et al., 2010; Kingsley et al., 2014; Song et al., 2022), but oftentimes at the sacrifice of organoleptic properties. The novel ClO2 delivery system utilized by the product evaluated in this study produced no significant differences in appearance, flavor or texture attributes of sliced tomatoes as compared to an untreated control sample after a 3-day refrigerated hold time. This demonstrates the potential for this technology as an effective antiviral treatment for refrigerated tomatoes, while maintaining product sensory qualities. Due to funding restrictions, the organoleptic properties of blueberries following treatment were not evaluated in this study, and it is unknown if these results can be extrapolated to this commodity. Treatments with ClO2 gas have been reported to have differing effects on a variety of fruit and vegetables, and these have primarily been related to appearance. One such defect has been the development of browning. This is likely related to oxidation of mono- and di-phenols into quinones that condense to form brown polymeric pigments (Gómez-López et al., 2009). Typically, this is mediated by the presence of oxygen and the enzyme polyphenol oxidase (PPO) and is most prevalent when fruit or vegetables have been sliced or ground. However, some evidence suggests that ClO2 can directly oxidize these compounds and cause browning even without PPO.
Browning has been observed in some commodities treated with ClO2 gas, but not others. For instance, shredded lettuce exposed to ClO2 showed evidence of browning whereas shredded cabbage and carrots did not (Gómez-López et al., 2008). Apples and peaches also showed signs of browning when treated with ClO2 gas (Sy et al., 2005). Whether or not a food item would brown depends on the type of commodity, levels of PPO enzymes, whether the product has been cut (thus releasing enzymes), and the level and total treatment time with ClO2 gas. As observed in this study, the absence of organoleptic changes to treated tomatoes, even when cut, may be suggestive that tomatoes are less susceptible to enzymatic browning, at least as compared to commodities such as apples or lettuce. In addition, it may be that the controlled-release of ClO2 gas by the InvisiShield™ system better protects the fruit.
Gaseous chlorine dioxide treatment has also been known to cause bleaching. In fact, it is used in the treatment of paper pulp for just that purpose. One theory is that it causes oxidation of oligosaccharides such as cellulose or hemicellulose, but it is unclear how this promotes lightening or translucency (Gómez-López et al., 2008). In addition, for leafy plants the oxidation of chlorophyll can cause the loss of green color. Thus, bleaching has been seen in iceberg lettuce (Mahmoud and Linton, 2008) as well as julienne carrots and strawberries (Sy et al., 2005). However, blueberries and raspberries were not affected by ClO2 treatment nor were tomato and onions (Sy et al., 2005). In contrast, strawberries did not undergo color change when treated with 5 mg/L ClO2 for 10 min (Mahmoud and Linton, 2008). Again, this highlights the importance of optimizing concentration and length of treatment time. Future studies should include evaluation of bleaching as a potential sensory detriment occurring as a consequence of ClO2 treatment, especially since this phenomenon is likely to be product-specific. Collectively, further studies investigating the effects of gaseous ClO2 on organoleptic properties of other fruits and vegetables are warranted.
This work is particularly significant given that enteric viruses are recalcitrant to most non-thermal food technology control measures when applied under conditions allowable by law (Seymour and Appleton, 2001), with the added benefit of maintaining product organoleptic properties. Chlorine solutions are one of the more commonly used antimicrobial agents in food processing, however these solutions pose issues for equipment, as they are highly corrosive to stainless-steel surfaces and can cause nose, throat, and eye irritation (Singh et al., 2021). In addition, when chlorine dioxide reacts with dissolved organic compounds in water, it forms disinfection by-products, such as chlorite and chlorate ions. Chlorine dioxide therefore breaks down into chlorate, chlorite, and ultimately chloride. This reaction is rapid and results in the development of chloride salts, very similar to table salt (Gómez-López et al., 2009). InvisiShield™ has been evaluated by third parties using an IC column to ensure the intermediate products, chlorate, and chlorite, are at safe levels for all humans, including children. These studies showed no detectable chlorate ions were present at an LOD of 0.799 mg/kg (data not shown). Further evaluation of the technology using methods such as LC–MS/MS which have lower limits of detection are ongoing in order to ensure the chlorates and perchlorate levels are lower than 0.05 mg/kg as permitted by the European Commission (2020).
Other chemical treatments, such as organic acid washes, have also been utilized in the processing of fruits and vegetables grown organically (Seymour and Appleton, 2001). However, the ability of viruses that initiate infection through the gastrointestinal tract, including hNoV and HAV, to withstand conditions of extreme pH is well documented and such washes are unlikely to have significant effect on these viruses (Cromeans et al., 2014). Ozone inactivation of enteric viruses on produce has also been studied, with results showing food-matrix associated variability. Specifically, Hieisen et al. (2016) demonstrated that ozone treatment of 6.25 ppm for 10 min resulted in a 3 log10 reduction in feline calicivirus (FCV) and murine norovirus (MNV), two cultivable hNoV surrogates, on lettuce, and close to 4 log10 reduction of MNV on green onions. However, only an approximate 2 log10 reduction of FCV was observed in green onions. In short, the efficacy of chemical treatments to inactivate viruses on foods appears to be quite variable between viruses and also between different food matrices.
Non-chemical approaches to controlling pathogens on fresh produce include ionizing radiation, pulsed light, and high-pressure processing (HPP). DiCaprio et al. (2016) reported that application of ionizing radiation at a dose greater than the FDA regulated limit, to fresh strawberries inoculated with hNoV and its cultivable surrogate, Tulane virus, did not effectively inactivate the viruses, resulting in less than one log10 reduction. Similarly, in part due to varying surface characteristics for berries, pulsed light was found to not be an effective means by which to reduce the concentrations of HAV and MNV on blackberries, strawberries and raspberries, with only 1–2 log10 reductions (Jubinville et al., 2022). Pulsed light also caused a reduction in surface luminosity (browning) for certain produce items, including blueberries and lettuce (Jubinville et al., 2022). High pressure processing has been shown to effectively inactivate the MNV cultivable surrogate, up to 5 log10 on lettuce berries and in fruit purees, however the impact on product quality was variable, leading to the opinion that HPP may be better suited for products such as purees and sauces (Lou et al., 2011). As with chemical treatments, the performance of non-chemical approaches to the control of viruses in foods is quite variable.
A major barrier in studying the efficacy of antimicrobials against human enteric viruses is the absence of methods by which wild-type strains can be cultivated in the laboratory. While a cell culture-adapted strain of HAV is commercially available, its growth is slow and its cultivation expensive. As a proof-of-concept study, sufficient funds were not available to commit to the use of HAV infectivity assays in our study. Recent hNoV cultivation methods have met with some success (Ettayebi et al., 2016; Dycke et al., 2019) but they are in the early phases of adoption, and importantly, cannot provide quantitative data on log10 inactivation. As described in many of the studies cited above, cultivable surrogates such as MNV, FCV and Tulane virus are often used as proxies, however they have been shown to respond differently, usually being more sensitive to inactivation strategies than are hNoVs (Cromeans et al., 2014). For these reasons, we chose to evaluate the efficacy of the product against both HAV and hNoV using the RNase-RT-qPCR method. While technically not an infectivity assay, this approach remains widely used and its efficacy in direct comparison to hNoV surrogates has been demonstrated in several studies (Knight et al., 2012). In a companion piece, Knight et al. (2016) concluded that the persistence of RT-qPCR signals for hNoV strains exposed to sodium hypochlorite was equal to or greater than that which was observed for the cultivable surrogates. This was the case regardless of inclusion of an RNase pre-treatment and may suggest that using RT-qPCR to evaluate chemical treatments such as ClO2 may actually produce data that underestimates, rather than overestimates, the efficacy of the technology. Clearly, there is precedence in using RNase-RT-qPCR in enteric virus inactivation studies, and the data produced may be conservative.
Further study of InvisiShield™ Technology is warranted. Future research could focus on investigating this technology relative to inactivation of other foodborne pathogens, particularly those that are non-cultivable; exploring the efficacy of the product against viral pathogens via infectivity assay or on other produce items; alternative storage conditions (such as frozen or room temperature environments); and the sensory analysis of other commodities following treatment with InvisiShield™. Nonetheless, this study is a good first step toward proof-of-concept that active ClO2-based packaging has antiviral efficacy against hNoV and HAV on blueberries and tomatoes, while showing little effect on sensory characteristics of tomatoes. This makes the technology a good candidate for future study.
The original contributions presented in the study are included in the article/supplementary material, further inquiries can be directed to the corresponding author.
RG, JF, WK, AR, MJ, and L-AJ contributed to the experimental design. JF performed the microbial bench top work. RG and L-AJ performed data analysis for microbial work. WK performed the sensory analysis experiments, data analysis, and wrote sections of the manuscript related to sensory work. RG drafted the first full version of the manuscript. All authors contributed to the article and approved the submitted version.
This work was made possible by funding from Aptar CSP Technologies.
AR and MJ are employees of Aptar CSP Technologies, who produce the product tested in this study.
The remaining authors declare that the research was conducted in the absence of any commercial or financial relationships that could be construed as a potential conflict of interest.
All claims expressed in this article are solely those of the authors and do not necessarily represent those of their affiliated organizations, or those of the publisher, the editors and the reviewers. Any product that may be evaluated in this article, or claim that may be made by its manufacturer, is not guaranteed or endorsed by the publisher.
Bennett, S. D., Sodha, S. V., Ayers, T. L., Lynch, M. F., Gould, L. H., and Tauxe, R. V. (2018). Produce-associated foodborne disease outbreaks, USA, 1998–2013. Epidemiol. Infect. 146, 1397–1406. doi: 10.1017/s0950268818001620
Bernard, H., Faber, M., Wilking, H., Haller, S., Höhle, M., Schielke, A., et al. (2014). Large multistate outbreak of norovirus gastroenteritis associated with frozen strawberries, Germany, 2012. Eur. Secur. 19:20719. doi: 10.2807/1560-7917.es2014.19.8.20719
Bosch, A., Gkogka, E., Guyader, F. S. L., Loisy-Hamon, F., Lee, A., Van, Lieshout, et al. (2018). Foodborne viruses: detection, risk assessment, and control options in food processing. Int. J. Food Microbiol. 285, 110–128. doi: 10.1016/j.ijfoodmicro.2018.06.001
Bozkurt, H., Phan-Thien, K.-Y., Ogtrop, F.Van, Bell, T., and McConchie, R. (2021). Outbreaks, occurrence, and control of norovirus and hepatitis a virus contamination in berries: a review. Crit. Rev. Food. Sci. 61, 116–138. doi: 10.1080/10408398.2020.1719383
Bunzel, M., and Ralph, J. (2006). NMR characterization of Lignins isolated from fruit and vegetable insoluble dietary Fiber. J. Agr. Food Chem. 54, 8352–8361. doi: 10.1021/jf061525z
Carstens, C. K., Salazar, J. K., and Darkoh, C. (2019). Multistate outbreaks of foodborne illness in the United States associated with fresh produce from 2010 to 2017. Front. Microbiol. 10:2667. doi: 10.3389/fmicb.2019.02667
Centers for Disease Control and Prevention (2003). Hepatitis A Outbreak associated with green onions at a restaurant—Monaca, Pennsylvania, 2003. Morb. Mortal. Wkly Rep. 290:3187. doi: 10.1001/jama.290.24.3187
Chatziprodromidou, I. P., Bellou, M., Vantarakis, G., and Vantarakis, A. (2018). Viral outbreaks linked to fresh produce consumption: a systematic review. J. Appl. Microbiol. 124, 932–942. doi: 10.1111/jam.13747
Chen, X., Chen, M., Xu, C., and Yam, K. L. (2019). Critical review of controlled release packaging to improve food safety and quality. Crit. Rev. Food Sci. 59, 2386–2399. doi: 10.1080/10408398.2018.1453778
European Commission (2020). COMMISSION REGULATION (EU) 2020/685 of 20 may 2020 amending Regulation (EC) no 1881/2006 as regards maximum levels of perchlorate in certain foods. Available at: https://eur-lex.europa.eu/legal-content/EN/TXT/PDF/?uri=CELEX:32020R0685&;amp;qid=1591624227155&from=EN (Accessed July 27, 2023).
Costafreda, M. I., Bosch, A., and Pintó, R. M. (2006). Development, evaluation, and standardization of a real-time TaqMan reverse transcription-PCR assay for quantification of hepatitis a virus in clinical and shellfish samples. Appl. Environ. Microb. 72, 3846–3855. doi: 10.1128/aem.02660-05
Cromeans, T., Park, G. W., Costantini, V., Lee, D., Wang, Q., Farkas, T., et al. (2014). Comprehensive comparison of cultivable norovirus surrogates in response to different inactivation and disinfection treatments. Appl. Environ. Microb. 80, 5743–5751. doi: 10.1128/aem.01532-14
DiCaprio, E., Phantkankum, N., Culbertson, D., Ma, Y., Hughes, J. H., Kingsley, D., et al. (2016). Inactivation of human norovirus and Tulane virus in simple media and fresh whole strawberries by ionizing radiation. Int. J. Food Microbiol. 232, 43–51. doi: 10.1016/j.ijfoodmicro.2016.05.013
Donnan, E. J., Fielding, J. E., Gregory, J. E., Lalor, K., Rowe, S., Goldsmith, P., et al. (2012). A multistate Outbreak of hepatitis a associated with semidried tomatoes in Australia, 2009. Clin. Infect. Dis. 54, 775–781. doi: 10.1093/cid/cir949
Dycke, J. V., Ny, A., Conceição-Neto, N., Maes, J., Hosmillo, M., Cuvry, A., et al. (2019). A robust human norovirus replication model in zebrafish larvae. PLoS Pathog. 15:e1008009. doi: 10.1371/journal.ppat.1008009
Escudero-Abarca, B. I., Goulter, R. M., Bradshaw, J., Faircloth, J., Leslie, R. A., Manuel, C. S., et al. (2022). Efficacy of an alcohol-based surface disinfectant formulation against human norovirus. J. Appl. Microbiol. 132, 3590–3600. doi: 10.1111/jam.15479
Ettayebi, K., Crawford, S. E., Murakami, K., Broughman, J. R., Karandikar, U., Tenge, V. R., et al. (2016). Replication of human noroviruses in stem cell–derived human enteroids. Science 353, 1387–1393. doi: 10.1126/science.aaf5211
Firouz, M. S., Mohi-Alden, K., and Omid, M. (2021). A critical review on intelligent and active packaging in the food industry: research and development. Food Res. Int. 141:110113. doi: 10.1016/j.foodres.2021.110113
Goméz-Lopez, V. M., Devlieghere, F., Ragaert, P., Chen, L., Ryckeboer, J., and Debevere, J. (2008). Reduction of microbial load and sensory evaluation of minimally processed vegetables treated with chlorine dioxide and electrolysed water. Ital. J. Food Sci. 109, 187–197. doi: 10.1016/j.ijfoodmicro.2005.12.013
Gómez-López, V. M., Rajkovic, A., Ragaert, P., Smigic, N., and Devlieghere, F. (2009). Chlorine dioxide for minimally processed produce preservation: a review. Trends. Food Sci. Tech. 20, 17–26. doi: 10.1016/j.tifs.2008.09.005
Hall, A. J., Eisenbart, V. G., Etingüe, A. L., Gould, L. H., Lopman, B. A., Parashar, U. D., et al. (2012). Epidemiology of foodborne norovirus outbreaks, United States, 2001–2008 - volume 18, number 10—October 2012 - emerging infectious diseases journal - CDC. Emerg. Infect. Dis. 18, 1566–1573. doi: 10.3201/eid1810.120833
Hieisen, K. A., Markland, S. M., and Kniel, K. E. (2016). Ozone inactivation of norovirus surrogates on fresh produce. J. Food Protect. 74, 836–839. doi: 10.4315/0362-028x.jfp-10-438
Jothikumar, N., Lowther, J. A., Henshilwood, K., Lees, D. N., Hill, V. R., and Vinjé, J. (2005). Rapid and sensitive detection of noroviruses by using TaqMan-based one-step reverse transcription-PCR assays and application to naturally contaminated shellfish samples. Appl. Environ. Microb. 71, 1870–1875. doi: 10.1128/aem.71.4.1870-1875.2005
Jubinville, E., Trudel-Ferland, M., Amyot, J., and Jean, J. (2022). Inactivation of hepatitis a virus and norovirus on berries by broad-spectrum pulsed light. Int. J. Food Microbiol. 364:109529. doi: 10.1016/j.ijfoodmicro.2021.109529
Kingsley, D. H., Vincent, E. M., Meade, G. K., Watson, C. L., and Fan, X. (2014). Inactivation of human norovirus using chemical sanitizers. Int. J. Food Microbiol. 171, 94–99. doi: 10.1016/j.ijfoodmicro.2013.11.018
Knight, A., Haines, J., Stals, A., Li, D., Uyttendaele, M., Knight, A., et al. (2016). A systematic review of human norovirus survival reveals a greater persistence of human norovirus RT-qPCR signals compared to those of cultivable surrogate viruses. Int. J. Food Microbiol. 216, 40–49. doi: 10.1016/j.ijfoodmicro.2015.08.015
Knight, A., Li, D., Uyttendaele, M., and Jaykus, L.-A. (2012). A critical review of methods for detecting human noroviruses and predicting their infectivity. Crit. Rev. Microbiol. 39, 295–309. doi: 10.3109/1040841x.2012.709820
Li, J., Predmore, A., Divers, E., and Lou, F. (2012). New interventions against human norovirus: Progress, opportunities, and challenges. Annu Rev. Food Sci. T. 3, 331–352. doi: 10.1146/annurev-food-022811-101234
Li, J. W., Xin, Z. T., Wang, X. W., Zheng, J. L., and Chao, F. H. (2004). Mechanisms of inactivation of hepatitis a virus in water by chlorine dioxide. Water Res. 38, 1514–1519. doi: 10.1016/j.watres.2003.12.021
Lim, M. Y., Kim, J.-M., and Ko, G. (2010). Disinfection kinetics of murine norovirus using chlorine and chlorine dioxide. Water Res. 44, 3243–3251. doi: 10.1016/j.watres.2010.03.003
Lou, F., Neetoo, H., Chen, H., and Li, J. (2011). Inactivation of a human norovirus surrogate by high-pressure processing: effectiveness, mechanism, and potential application in the fresh produce industry. Appl. Environ. Microb. 77, 1862–1871. doi: 10.1128/aem.01918-10
Lynch, M. F., Tauxe, R. V., and Hedberg, C. W. (2008). The growing burden of foodborne outbreaks due to contaminated fresh produce: risks and opportunities. Epidemiol. Infect. 137, 307–315. doi: 10.1017/s0950268808001969
Mahmoud, B. S. M., and Linton, R. H. (2008). TInactivation kinetics of inoculated Escherichia coli O157:H7 and Salmonella enterica on lettuce by chlorine dioxide gas. Food Microbiol. 25, 244–252. doi: 10.1016/j.fm.2007.10.015
Meilgaard, M. C., Carr, B. T., and Carr, B. T. (2014). Sensory evaluation techniques. Boca Raton, FL: CRC Press.
Papafragkou, E., Plante, M., Mattison, K., Bidawid, S., Karthikeyan, K., Farber, J. M., et al. (2008). Rapid and sensitive detection of hepatitis a virus in representative food matrices. J. Virol. Methods 147, 177–187. doi: 10.1016/j.jviromet.2007.08.024
Park, S. H., Kim, W. J., and Kang, D. H. (2018). Effect of relative humidity on inactivation of foodborne pathogens using chlorine dioxide gas and its residues on tomatoes. Lett. Appl. Microbiol. 67, 154–160. doi: 10.1111/lam.13002
Poste, L. M., Mackie, D. A., Butler, G., and Larmond, E. (1991) in Laboratory methods for sensory analysis of food. ed. J. T. Buckley Ottowa (Canada: Canada Communication Group Publishing Centre)
Provost, P. J., and Hilleman, M. R. (1979). Propagation of human hepatitis a virus in cell culture in vitro (40422) Provost, P. J. and Hilleman M. R. (1979). Proceedings of the Society for Experimental Biology and Medicine, 160, 213. Proceedings of the Society for Experimental Biology and medicine, 213–221.
Ridenour, G. M., and Ingols, R. S. (1947). Bactericidal properties of chlorine dioxide. J. Am. Water Works Assoc. 39, 561–567. doi: 10.1002/j.1551-8833.1947.tb17703.x
Rosenblum, L. S., Mirkin, I. R., Allen, D. T., Safford, S., and Hadler, S. C. (2011). A multifocal outbreak of hepatitis a traced to commercially distributed lettuce. Am. J. Public Health 80, 1075–1079. doi: 10.2105/ajph.80.9.1075
Scallan, E., Hoekstra, R. M., Angulo, F. J., Tauxe, R. V., Widdowson, M.-A., Roy, S. L., et al. (2011). Foodborne illness acquired in the United States—major pathogens - volume 17, number 1—January 2011 - emerging infectious diseases journal - CDC. Emerg. Infect. Dis. 17, 7–15. doi: 10.3201/eid1701.p11101
Scavia, G., Alfonsi, V., Taffon, S., Escher, M., Bruni, R., Medici, D. D., et al. (2017). A large prolonged outbreak of hepatitis a associated with consumption of frozen berries, Italy, 2013–14. J. Med. Microbiol. 66, 342–349. doi: 10.1099/jmm.0.000433
Seymour, I. J., and Appleton, H. (2001). Foodborne viruses and fresh produce. J. Appl. Microbiol. 91, 759–773. doi: 10.1046/j.1365-2672.2001.01427.x
Shieh, Y. C., Tortorello, M. L., Fleischman, G. J., Li, D., and Schaffner, D. W. (2014). Tracking and modeling norovirus transmission during mechanical slicing of globe tomatoes. Int. J. Food Microbiol. 180, 13–18. doi: 10.1016/j.ijfoodmicro.2014.04.002
Singh, S., Maji, P. K., Lee, Y. S., and Gaikwad, K. K. (2021). Applications of gaseous chlorine dioxide for antimicrobial food packaging: a review. Environ. Chem. Lett. 19, 253–270. doi: 10.1007/s10311-020-01085-8
Song, M., Hossain, M. I., Jung, S., Yeo, D., Wang, Z., Min, A., et al. (2022). Comparison of virucidal efficacy of sodium hypochlorite, chlorine dioxide, peracetic acid, and ethanol against hepatitis a virus by carrier and suspension tests. Int. J. Food Microbiol. 363:109506. doi: 10.1016/j.ijfoodmicro.2021.109506
Sy, K. V., Murray, M. B., Harrison, M. D., and Beuchat, L. R. (2005). Evaluation of gaseous chlorine dioxide as a sanitizer for killing Salmonella, escherichia coli O157:H7, Listeria monocytogenes, and yeasts and molds on fresh and fresh-cut produce. J. Food Prot. 68, 1176–1187. doi: 10.4315/0362-028x-68.6.1176
Keywords: human norovirus, hepatitis a virus, food safety, packaging, active packaging, fresh produce
Citation: Goulter RM, Frye JW, Kerr WL, Richard A, Johnston M and Jaykus L-A (2023) Evaluation of a novel chlorine dioxide-based packaging technology to reduce human enteric virus contamination on refrigerated tomatoes and blueberries. Front. Sustain. Food Syst. 7:1216273. doi: 10.3389/fsufs.2023.1216273
Received: 03 May 2023; Accepted: 04 August 2023;
Published: 23 August 2023.
Edited by:
María Belén Pisano, Universidad Nacional de Córdoba, ArgentinaReviewed by:
Trevor Phister, PepsiCo, United KingdomCopyright © 2023 Goulter, Frye, Kerr, Richard, Johnston and Jaykus. This is an open-access article distributed under the terms of the Creative Commons Attribution License (CC BY). The use, distribution or reproduction in other forums is permitted, provided the original author(s) and the copyright owner(s) are credited and that the original publication in this journal is cited, in accordance with accepted academic practice. No use, distribution or reproduction is permitted which does not comply with these terms.
*Correspondence: Rebecca M. Goulter, cmViZWNjYV9nb3VsdGVyQG5jc3UuZWR1
Disclaimer: All claims expressed in this article are solely those of the authors and do not necessarily represent those of their affiliated organizations, or those of the publisher, the editors and the reviewers. Any product that may be evaluated in this article or claim that may be made by its manufacturer is not guaranteed or endorsed by the publisher.
Research integrity at Frontiers
Learn more about the work of our research integrity team to safeguard the quality of each article we publish.