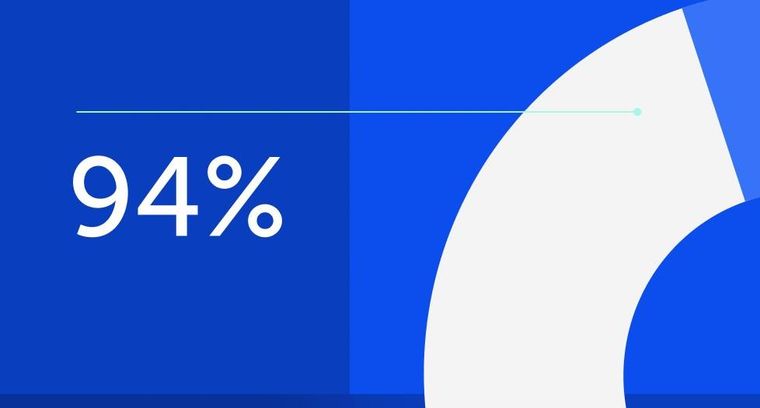
94% of researchers rate our articles as excellent or good
Learn more about the work of our research integrity team to safeguard the quality of each article we publish.
Find out more
ORIGINAL RESEARCH article
Front. Sustain. Food Syst., 14 July 2023
Sec. Crop Biology and Sustainability
Volume 7 - 2023 | https://doi.org/10.3389/fsufs.2023.1208319
This article is part of the Research TopicEvaluation of Preharvest and Postharvest Factors on Forage Crop Quality, Physiology, and Ensiling CharacteristicsView all 11 articles
Crop–livestock integration systems are efficient technologies for diversifying production and promoting agricultural sustainability. However, less is known about the triple intercropping of crops for silage production. The objective of this study was to evaluate the dry mass production, fermentation profile, and nutritive value of sorghum silage intercropped with Tamani guinea grass and Stylosanthes cv. Bela in integrated systems. We used an experimental design with randomized blocks with four replicates. The treatments consisted of silage of (1) sorghum in monocropped; (2) Tamani guinea grass in monocropped (Panicum maximum cv. BRS Tamani); (3) Stylosanthes cv. Bela in monocropped (Stylosanthes guianensis cv. BRS Bela); (4) sorghum intercropped with Tamani guinea grass; (5) sorghum intercropped with Stylosanthes cv. Bela; (6) Stylosanthes cv. Bela intercropped with Tamani guinea grass; and (7) sorghum intercropped with Tamani guinea grass and Stylosanthes cv. Bela, totaling 28 experimental silos. Our results demonstrated that intercropping sorghum with tropical forages can be utilized in integrated silage production systems. This practice led to an increase in silage mass production per unit area while also providing pasture forage after the crop harvest for silage production, ultimately enhancing land-use efficiency in a sustainable manner. Silage produced from sorghum intercropped with Tamani guinea grass and Stylosanthes cv. Bela exhibited improved fermentative characteristics, as well as higher ether extract and total digestible nutrient contents compared with silage from monocropped forages. Tropical forages contributed to an increase in the crude protein content of monocropped sorghum silage, which could potentially reduce costs associated with acquiring protein salts for ruminant feed supplementation. Consequently, we recommend the triple intercropping of sorghum, Tamani guinea grass, and Bela for silage production, as it offers advantages for the cultivation of annual and tropical forage crops.
The sustainability of agribusiness has become a prerequisite for maintaining a position in the global market, encompassing both socioenvironmental appeal and the necessity for integrated negotiation (Borsellino et al., 2016). In this regard, conservative production systems that integrate crops and livestock have emerged as an established strategy for intensifying food production while addressing environmental concerns (Simões et al., 2023). Integrated crop–livestock systems are widely recognized as one of the most sustainable and competitive technologies for advancing agribusiness (Dias et al., 2020).
The ecosystem services provided by integrated systems have been documented in numerous studies, including enhanced land use efficiency and increased grain production (Muniz et al., 2021); reduced soil compaction, improved water infiltration rates, and lower erosion risks (Linhares et al., 2020); maintenance of soil fertility through enhanced nutrient cycling (Dias et al., 2020); greater carbon sequestration, increased soil organic matter, and improved microclimatic conditions (Vincent-Caboud et al., 2019); pasture regeneration (Santos et al., 2020); and improved silage production during the dry season (Oliveira et al., 2020). However, due to the intricate interactions between annual and tropical forage crops, integrated systems have become dynamic and complex, requiring more sophisticated technologies to consolidate environmental and productive sustainability (Soussana and Lemaire, 2014).
Among the annual crops employed in integrated systems, Sorghum bicolor L. (sorghum) stands as a significant forage crop in numerous global regions owing to its adaptability across diverse environments (Perazzo et al., 2017), capability to flourish in low soil fertility conditions, potential for regrowth post-grain harvest, and high tolerance against water deficits (Buffara et al., 2018). These characteristics enable a wider range of sowing seasons and greater resilience to adverse environmental factors compared with maize (Mateus et al., 2016). Consequently, sorghum has been recognized as crucial in recent years for the production of preserved forage (Oliveira et al., 2020), offering high dry mass productivity, preservation of nutritional value, and favorable fermentation patterns (Cruz et al., 2020).
However, sorghum silage typically exhibits a lower crude protein content compared with tropical forages (Ribeiro et al., 2017), necessitating the adoption of cultivation strategies to enhance the crude protein content of sorghum silage. Notably, Oliveira et al. (2020) demonstrated the advantageous effects of intercropping annual and tropical forage crops in integrated systems for silage production. Intercropping not only enhances the quality of sorghum silage but also intensifies production systems, resulting in higher yields and improved silage nutritional value. Moreover, this approach mitigates the challenges associated with silage fermentation in exclusive grass and legume systems.
Among tropical forages, Tamani guinea grass possesses significant potential as a conserved forage resource due to its high mass yield, slender stems and leaves, and high tillering capacity (Dias et al., 2020). The exceptional quality and adaptability of this grass make it suitable for silage production (Paludo et al., 2020). Additionally, tropical legume silage has gained attention due to its superior nutritional value (Silva et al., 2023). Recently introduced Stylosanthes cv. Bela (Stylosanthes guianensis cv. BRS Bela) has shown positive results in ruminant production, owing to its elevated crude protein content (Braga et al., 2020) and ability to fix biological nitrogen. Given the escalating costs of mineral fertilizers and their significant contribution to greenhouse gas emissions, the integration of legumes into integrated systems emerges as a promising technology for increasing crop productivity and ensuring enhanced sustainability (Epifanio et al., 2019a,b). This approach allows for the partial or complete replacement of mineral (nitrogen) fertilizers, ensuring adequate plant nutrition, soil conservation, fertility maintenance, and carbon sequestration (Bourscheidt et al., 2023; Silva et al., 2023).
In this context, sorghum silage, tropical grasses, and legumes present a favorable combination for achieving a balanced nutritional value, improved qualitative characteristics in dry matter (DM), and higher nutrient production per unit area (Perazzo et al., 2017). Moreover, their versatility in use establishes them as significant alternative food sources during the off-season period (Oliveira et al., 2020).
However, limited knowledge exists regarding triple intercropping (sorghum + tropical grass + legumes) for silage production. Understanding the optimal intercropping approach can enhance both silage production and quality within integrated systems while also facilitating crop diversification to meet the demand for high-quality feed during periods of limited forage availability. Therefore, we hypothesized that double and triple intercropping of sorghum with forage crops would have a positive impact on the bromatological characteristics and yield of the ensiled mass, without compromising the fermentation process required for silage preparation. The objective of this study was to assess the production of dry mass, fermentation profile, and nutritive value of silage derived from sorghum intercropped with Tamani guinea grass and Stylosanthes cv. Bela in integrated systems.
This experiment was conducted under field conditions at Instituto Federal Goiano, Campus Rio Verde, located in the municipality of Rio Verde, State of Goiás, Brazil (17°48′ 22″S, 50°54′ 11″W; 832 m altitude). According to the Köppen–Geiger classification, the climate of the region is defined as tropical (Aw), with a dry season in winter.
Before the implementation of the experiment, soil samples were collected from the 0 to 20 cm layer for physicochemical characterization. The soil in the experimental area was characterized as Dystroferric Red Latosol (Santos et al., 2018). The soil characteristics were as follows: it has 364, 83, and 553 g kg−1 of clay, silt, and sand, respectively; pH in CaCl2: 5.4; Ca: 2.69 cmolc dm−3; Mg: 1.00 cmolc dm−3; Al: 0.01 cmolc dm−3; Al + H: 3.79 cmolc dm−3; K: 0.69 cmolc dm−3; cation exchange capacity: 8.6 cmolc dm−3; current base saturation of the soil (V1): 56%; P (Mehlich): 3.8 mg dm−3; S: 8.5 mg dm−3; Cu: 3.7 mg dm−3; Zn: 1.0 mg dm−3; Fe: 17.3 mg dm−3; organic matter (OM): 39.8 g dm−3. No irrigation system was installed during the experiment. Precipitation, maximum, average, and minimum temperatures were monitored throughout the duration of the study, as shown in Figure 1.
Figure 1. Monthly precipitation and minimum, average and maximum temperatures recorded from February to August 2022 in Rio Verde—GO, Brazil.
The experimental design consisted of randomized blocks with four replicates. The treatments consisted of the silage of (1) sorghum in monocropped; (2) Tamani guinea grass in monocropped (Panicum maximum cv. BRS Tamani); (3) Stylosanthes cv. Bela in monocropped (Stylosanthes guianensis cv. BRS Bela); (4) sorghum intercropped with Tamani guinea grass; (5) sorghum intercropped with Stylosanthes cv. Bela; (6) Stylosanthes cv. Bela intercropped with Tamani guinea grass; and (7) sorghum intercropped with Tamani guinea grass and Bela Stylosanthes, totaling 28 experimental silos. The sorghum used was grain sorghum (AG 1077) with an early cycle and high production potential.
For monocropped cultures, a spacing of 0.5 m between rows was used (Figures 2A–C). In the double intercropping of annual and forage crops, sorghum was sown at 0.5 m spacing and Tamani guinea grass and/or legume between rows was sown at a 0.25 m distance from the sorghum row (Figures 2D, E). In the double intercropping of forage plants, Tamani guinea grass was sown at 0.25 m of the Stylosanthes cv. Bela (Figure 2F). In the triple intercropping, sorghum was sown at 0.9 m spacing, with Tamani guinea grass and Stylosanthes cv. Bela sown between the rows at a 0.3 m distance from the sorghum row, as shown in Figure 2G. No herbicides were applied to suppress grass or legume growth during the intercropping.
Figure 2. Arrangement of crops: Sorghum in monocropped (A), Tamani guinea grass in monocropped (B), Stylosanthes cv. Bela in monocropped (C), Sorghum intercropped with Tamani guinea grass (D), Sorghum intercropped with Stylosanthes cv. Bela (E), Stylosanthes cv. Bela intercropped with Tamani guinea grass (F) and Sorghum intercropped with Tamani guinea grass and Stylosanthes cv. Bela (G).
The seeding of forage systems was carried out manually on 7 March 2022, and 120 kg ha−1 of P2O5 and 20 kg ha−1 of FTE BR 12 (9% Zn, 1.8% B, 0.8% Cu, 2% Mn, 3.5% Fe, and 0.1% Mo) were applied in the planting furrow using simple superphosphate and Frit sources, respectively. A total of 12 sorghum seeds were used per meter, and for grass and legumes, 3 kg of pure viable seeds were used per hectare.
When sorghum plants were at the stage of three and six fully developed leaves, two fertilizer applications of 80 and 60 kg ha−1 of N and K2O from urea and potassium chloride, respectively, were applied to the following systems: sorghum in monocropped, Tamani guinea grass in monocropped, and sorghum intercropped with Tamani guinea grass. For the systems intercropped with legumes (sorghum intercropped with Stylosanthes cv. Bela, Tamani guinea grass intercropped with Stylosanthes cv. Bela, and sorghum intercropped with Tamani guinea grass and Bela), 60 kg ha−1 of K2O and only half of the dose of nitrogen (i.e., 40 kg ha−1) were applied, aiming to utilize the nitrogen obtained through the biological fixation by legumes. For the Stylosanthes cv. Bela monocropped system, only 60 kg ha−1 K2O was applied.
To Spodoptera frugiperda and Dalbulus maidis, we applied the insecticides Klorpan (active ingredient Chlorpyrifos) and Connect (active ingredient Beta-cyfluthrin and Imidacloprid) at the rate of 0.4 and 0.1 L ha−1 of commercial product, respectively. Both applications were performed using knapsack sprayers.
Harvesting of cultures for silage was carried out 93 days after sowing the cultures. During this period, sorghum, Tamani guinea grass, and Stylosanthes cv. Bela were harvested at 340.66, 276.16, and 285.04 g kg−1 dry matter (DM), respectively. To evaluate the dry mass production and proportion of the ensiled material (Table 1), the material was collected, separated, and weighed to determine the proportions of sorghum, Tamani guinea grass, and Stylosanthes. Subsequently, the material was placed in an oven at 55°C until it reached a constant mass, and its dry weight was determined and expressed in kg ha−1.
Table 1. Proportion of material ensiled from sorghum intercropped with Tamani guinea grass and Stylosanthes cv. Bela.
To make the silage, the material was ground together in the treatments of the intercropping systems, in particles of ~10 mm size. The material was stored in experimental PVC silos measuring 10 cm in diameter and 40 cm in length. The material was compacted with an iron pendulum, closed with a PVC lid, and sealed with adhesive tape to prevent the entry of air. They were then stored at room temperature and protected from rain and sunlight.
In the material in natura (before ensiling), bromatological analyses were performed (Table 2) to determine the dry matter (DM), method 934.01; crude protein (CP), method 920.87; lignin, method 973.18; ether extract (EE) contents, method 920.85, and mineral matter (MM), method 924.05, according to the methodologies described by the AOAC (1990). Neutral detergent fiber (NDF) and acid detergent fiber (ADF) were measured as described by Mertens (2002). Total digestible nutrients (TDN) were calculated using the equation proposed by Chandler (1990). For the determination of in vitro dry matter digestibility (IVDM), the technique described by Tilley and Terry (1963) was used, adapted to the artificial rumen, developed by ANKON®, using the instrument “Daisy incubator” by Ankom Technology (in vitro true digestibility—IVTD). The ruminal collection was carried out in dairy cattle fed on pasture and receiving corn silage twice a day. The liquid was collected through a ruminal cannula, in the morning, 3 h after feeding the animal. The initial project was approved by the Research Ethics Committee (REC) of the Federal Goiano Institute, protocol 53752405-16.
Table 2. Chemical–bromatological composition (g kg−1) of sorghum, Tamani guinea grass, and Stylosanthes cv. Bela in monocropped and intercropped before ensiling.
After 50 days of fermentation, the silos were opened, and the upper and lower portions of each silo were discarded. The central portion of the silo was homogenized and placed in a plastic tray. Part of the in natura silage was separated for the analysis of fermentation parameters: buffering capacity, pH, and ammoniacal nitrogen in total nitrogen (N-NH3/NT), following the method described by Bolsen et al. (1992).
The pH and buffering capacity analyses were performed when the silos were opened to avoid changes in the expected values due to heat and humidity. To determine ammoniacal nitrogen, the silage was frozen to inactivate the activity of anaerobic bacteria, thus avoiding the volatilization of nitrogen, and the samples were later thawed for juice extraction (Bolsen et al., 1992). Total dry matter loss and effluent production were determined according to the methodology proposed by Jobim et al. (2007). Organic acids were determined using high-performance liquid chromatography (HPLC), according to the method described by Kung and Shaver (2001) for the determination of lactic, acetic, propionic, and butyric acids.
The other portion of the material (~0.5 kg) was weighed and dried in a forced ventilation oven at 55°C until it reached a constant mass. The samples were, then, ground in a knife mill with a 1 mm sieve and stored in plastic containers. Subsequently, the chemical–bromatological characteristics of the silage were analyzed following the methodology described above for the in natura material.
The variables were subjected to an analysis of variance using the R program version R-3.1.1 (R Core Team, 2014) and the ExpDes package (Ferreira et al., 2014). Means were compared using Tukey's test at 5% probability.
To understand the cause-and-effect relationship between the variables, Pearson's correlation analyses (low: r ≤ 0.30; moderate: 0.30 < r ≤ 0.70: and high r > 0.70) and trail analysis were performed, considering pH and IVDMD as dependent variables due to the importance of these variables for the fermentative profile and nutritional value of silages. To define the causal diagram, multiple linear regression analysis was performed using the “stepwise” procedure with the “backward” option (Coimbra et al., 2005; Charnet et al., 2008). Subsequently, multicollinearity was diagnosed based on the condition factor (ratio between the highest and lowest Eigenvalues), and the number of conditions (NC) < 100 was verified, indicating that multicollinearity is weak and does not constitute a problem for the analysis (Cruz et al., 2014).
The contributions of the direct and indirect effects of the variables were quantified as percentages. Contributions above 50% were considered high direct effect (Botelho et al., 2019; Ribeiro et al., 2019). For the statistical analysis, the “corrplot,” “lavaan,” and “semPlot” packages of the R development computational program were used.
The cropping systems had a significant impact (p < 0.05) on dry mass production for silage (Figure 3). The highest production was observed in the triple intercropping of sorghum + Tamani guinea grass + Bela, followed by the double intercropping of sorghum + Tamani guinea grass and sorghum + Bela. Monocropped forages exhibited lower silage mass production. The intercropping systems resulted in a 36.20% increase in silage mass in the triple intercrop and a 22.09% increase in the double intercrop compared with monocropped sorghum.
Figure 3. Dry mass production of silage material from sorghum, Tamani guinea grass, and Stylosanthes cv. Bela in monocropped and intercropping. Means followed by different letters, differ by Tukey's test (p < 0.05). The vertical bars represent the standard error of the mean.
Fermentation characteristics, including pH, buffering capacity, dry matter (DM), and N-NH3 content, were influenced by the different silages (Figure 4). Silages derived from Tamani guinea grass and Stylosanthes cv. Bela in both the monocropped and intercropping systems exhibited the highest pH values (4.42, 4.30, and 4.28, respectively). In the intercropping systems with sorghum, there was an average reduction of 10.5% in the pH values of the silage for sorghum + Tamani guinea grass + Bela, sorghum + Bela, and sorghum + Tamani guinea grass consortia compared with the Tamani guinea grass and Stylosanthes cv. Bela silages in monocropped systems. The sorghum silage displayed the lowest pH value (3.47). Similar results were observed for buffering capacity (Figure 4B), with a reduction of 13.9% for the triple and double intercrop silages with sorghum compared with the monocropped silages of the respective forages.
Figure 4. pH (A), buffering capacity (B), dry matter (C), and N-NH3 (D) of silage sorghum, Tamani guinea grass and Stylosanthes cv. Bela in monocropped and intercropping. Means followed by different letters differ by Tukey's test at 5% probability. Vertical bars represent standard error of mean of each point. SEM, standard error of mean.
Sorghum silage exhibited the highest dry matter (DM) content (341.50 g kg−1), followed by sorghum + Tamani guinea grass + Bela silage (326.91 g kg−1; Figure 4C). Silages of sorghum + Tamani guinea grass and sorghum + Bela showed similar results, averaging at 306.64 g kg−1 DM. Monocropped silages of the forages displayed the lowest DM contents (279.99 g kg−1 for Tamani guinea grass and 282.35 g kg−1 for Stylosanthes cv. Bela, respectively).
The monocropped silage of Stylosanthes cv. Bela exhibited the highest N-NH3 value (72.74 g kg−1 DM), followed by Tamani guinea grass silage in monocropped and Tamani guinea grass + Bela silage, with an average of 63.43 g kg−1 DM, which did not differ significantly from the silage of sorghum + Bela and sorghum + Tamani guinea grass + Bela consortia. The silages of sorghum in monocropped and sorghum + Tamani guinea grass had the lowest N-NH3 values (39.85 and 47.80 g kg−1 DM, respectively; Figure 4D).
The silages of Stylosanthes cv. Bela in monocropped and Tamani guinea grass + Bela exhibited the highest dry matter losses (26.47 and 27.15 g kg−1 DM, respectively), followed by the silages of Tamani guinea grass in monocropped, sorghum + Bela, and sorghum + Tamani guinea grass + Bela, which did not differ significantly from the silage of sorghum + Tamani guinea grass. Sorghum silage showed the lowest DM losses at 13.30 g kg−1 DM (Figure 5A).
Figure 5. Total dry matter losses (A), effluent production (B), lactic acid (C), and acetic acid (D) of silage sorghum, Tamani guinea grass, and Stylosanthes cv. Bela in monocropped and intercropping. Means followed by different letters differ by Tukey's test at 5% probability. Vertical bars represent standard error of mean of each point. SEM, standard error of mean.
The highest effluent productions were observed in the silages of Tamani guinea grass (17.89 kg t−1 FM), Stylosanthes cv. Bela (17.44 kg t−1 FM), and Stylosanthes cv. Bela + Tamani guinea grass (18.39 kg t−1 FM; Figure 5B). Intercropping sorghum with forage crops contributed to an average reduction of 17.16% in effluent production compared with the silages of Tamani guinea grass and Stylosanthes cv. Bela in monocropped. Sorghum silage exhibited the lowest effluent production (11.42 kg t−1 FM).
Monocropped sorghum silage exhibited the highest lactic acid content (44.43 g kg−1 DM) (Figure 5C). In contrast, silages of Tamani guinea grass, monocropped Stylosanthes, and Stylosanthes cv. Bela + Tamani guinea grass had the lowest values, with an average of 19.31 g kg−1 DM. The intercropped systems with sorghum silage showed a 39.36% increase in lactic acid content compared with the silages of grass and legumes in monocropped and the Bela + Tamani guinea grass combination.
Silage of Stylosanthes cv. Bela and Tamani guinea grass in monocropped and the Bela + Tamani guinea grass combination exhibited the highest values of acetic acid (Figure 5D), with an average of 8.93 g kg−1 DM. On the other hand, silages from intercropped systems containing sorghum in their composition showed a 13.78% reduction in acetic acid. Sorghum silage in monocropped exhibited the lowest values (5.94 g kg−1 DM) of acetic acid.
Bromatological characteristics such as NDF, ADF, lignin, mineral matter, crude protein, ether extract, IVDMD, and TDN were significantly influenced by the different silages (Figures 6, 7). Monocropped sorghum silage exhibited the lowest NDF (542.08 g kg−1 DM) and ADF (297.90 g kg−1 DM) contents (Figures 6A, B). Conversely, the highest NDF (609.12 g kg−1 DM) and ADF (336.20 g kg−1 DM) contents were observed in the monocropped Tamani guinea grass silage. Silages from intercropped systems (double and triple-cropped) showed intermediate values.
Figure 6. Neutral detergent fiber (A), acid detergent fiber (B), lignin (C), and mineral matter (D) contents of sorghum, Tamani guinea grass, and Stylosanthes cv. Bela silage in monocropped and intercropping. Means followed by different letters differ by Tukey's test at 5% probability. Vertical bars represent standard error of mean of each point. SEM, standard error of mean.
Figure 7. Crude protein (A), ether extract (B), IVDMD (C), and TDN (D) contents of sorghum, Tamani guinea grass, and Stylosanthes cv. Bela silage in monocropped and intercropping. Means followed by different letters differ by Tukey's test at 5% probability. Vertical bars represent standard error of mean of each point. SEM, standard error of mean.
The monocropped sorghum silage exhibited the highest lignin content (Figure 6C) at 43.02 g kg−1 DM. On the other hand, the lowest levels were observed in the silages of Stylosanthes cv. Bela (26.59 g kg−1 DM) and Tamani guinea grass (27.69 g kg−1 DM) in monocropped and double-cropping systems (28.50 g kg−1 DM). The intercropping systems effectively reduced lignin concentration, with reductions of 10.52, 17.43, and 23.01% observed in the sorghum + Tamani guinea grass + Bela, sorghum + Tamani guinea grass, and sorghum + Bela silages, respectively, compared with monocropped sorghum silage. Regarding mineral matter (Figure 6D), the highest values were observed in the Tamani guinea grass + Bela (66.55 g kg−1), Tamani guinea grass (65.91 g kg−1), and Stylosanthes cv. Bela (62.66 g kg−1) silages in monocropped. The double sorghum + Tamani guinea grass and sorghum + Bela, as well as the triple sorghum + Tamani guinea grass + Bela silages, showed a 16.2% reduction in mineral matter compared with the forage silages in monocropped.
Silage of Stylosanthes cv. Bela in the monocropped had the highest crude protein content (152.33 g kg−1), followed by Stylosanthes cv. Bela + Tamani guinea grass at 140.88 g kg−1 DM (Figure 7A). The silages of Tamani guinea grass in the monocropped, sorghum + Tamani guinea + Bela, and sorghum + Bela showed similar results, with an average of 126.43 g kg−1 DM. There was 35.32 and 41.88% increase in the crude protein content of the sorghum + Tamani guinea grass and sorghum + Bela double intercrop silages, respectively, and 43.54% for the triple intercrop silage when compared with the silage of sorghum in monocropped, which showed a crude protein content of 71.46 g kg−1 DM.
The sorghum silage had the highest ether extract content (40 g kg−1 DM; Figure 7B). There was 20.05, 32.4, and 32.4% reduction in the ether extract content for silages of sorghum + Tamani guinea grass + Bela silage, sorghum + Tamani guinea grass, and sorghum + Bela, respectively, compared with silage of sorghum in the monocropped. Silages of Stylosanthes cv. Bela + Tamani guinea grass and Tamani guinea grass and Stylosanthes cv. Bela in monocropped showed the lowest ether extract values (20.32, 19.89, and 19.81 g kg−1 DM, respectively). The content of IVDMD (Figure 7C) was similar among the silages, with an average of 612.36 g kg−1 DM.
Silage of sorghum in monocropped showed the highest value of TDN (61.90 g kg−1 DM), followed by silage of sorghum + Tamani guinea grass, sorghum + Bela, and sorghum + Tamani guinea grass + Bela, showing increases of 5.8, 3.41, and 4.27%, respectively, for the intercrop silages compared with the silages of the forages in monocropped (Figure 7D).
In the correlation analysis (Figure 8), IVDMD was the only variable that did not show a correlation with any analyzed variable. Moreover, the formation of two groups of variables was observed: Group 1 was composed of NDF, N-NH3, acetic acid, pH, CP, effluent production, buffering capacity, DM loss, and ADF, whereas Group 2 was composed of DM, lactic acid, EE, lignin, and TDN. The variables within the same group showed positive correlations and those between distinct groups showed negative correlations.
Figure 8. Pearson's correlation between the variables of the fermentation profile and nutritional value of silages of sorghum, Tamani guinea grass, and Stylosanthes cv. Bela in monocropped and intercropping systems.
Through the causal diagram obtained from the multiple regression analysis using the “stepwise” procedure with the “backward” option, it was found that the variables IVDMD, ADF, TDN, CP, buffering capacity, EE, and lactic acid were maintained in the model to explain the pH, with significance for the first four variables and a coefficient of determination of 0.96 (Table 3). The model to explain IVDMD showed a coefficient of determination of 0.72 and was composed of the variables, such as pH, buffering capacity, ADF, NDF, TDN, and CP, all of which were significant in the model.
Table 3. Direct and indirect effects, correlation, and coefficient of determination of the causal models.
In the causal diagram of pH (Figure 9), it was verified by the decomposition of correlation that CP, ADF, and IVDMD had direct effects with contributions above 50% of the correlation coefficient and positive values for the first two variables and negative values for IVDMD (Table 3). The other variables showed low direct effects and a high correlation with pH. For the causal diagram of IVDMD (Figure 10), there was a high contribution of direct effects to the correlation for pH, CP, NDF, and ADF, and the correlation was negative for all variables except ADF.
Figure 9. Causal diagram showing the correlations between the independent variables and the cause–effect relationships with pH.
Figure 10. Causal diagram showing the correlations between the independent variables and the cause–effect relationships with IVDMD.
Integrated systems promote diversification of production and food production without the need for clearing new areas, making them a sustainable approach (Costa et al., 2016) that contributes to global food security (Sekaran et al., 2021).
In this study, the triple intercropping system demonstrated the highest dry mass production due to the simultaneous cultivation of three crops, enabling better utilization of the available land and resulting in increased silage yield. These findings highlight the benefits of intercropping annual and tropical forage crops (grasses and legumes) for crop-livestock integration, as it enhances the yield of dry mass for silage compared with monocropped systems. Furthermore, after harvesting crops for silage production, the formed pasture can be utilized for low-cost animal grazing during the off-season when weather conditions are unfavorable for most crops (Santos et al., 2020). Therefore, this cultivation strategy is an effective means to improve land-use efficiency (Costa et al., 2016), particularly in tropical regions (Oliveira et al., 2020).
In addition to these advantages, legumes contribute to integrated systems by reducing the need for nitrogen application in grasses. Through biological nitrogen fixation, legumes increase the availability of this macronutrient in the soil, thereby enhancing the sustainability of cropping systems and reducing the reliance on mineral nitrogen fertilizers in the system (Bolson et al., 2022).
The findings of this study are highly significant in evaluating the fermentation characteristics and nutritional value of silage produced in integrated systems. Legumes and grasses possess a high buffering capacity, low soluble carbohydrate concentration, and low dry matter content, which makes it challenging to lower the pH during ensiling (Hawu et al., 2022). This explains the observed results for Tamani guinea grass and Stylosanthes cv. Bela silages in monocropped and combined (Bela + Tamani guinea grass) systems, where the pH stabilized above 4.2 (Figure 4A). Such pH values promote the growth of undesirable microorganisms during silage fermentation, thereby compromising the final quality (Kung et al., 2018).
On the other hand, the silages from intercropped systems with sorghum were effective in achieving pH reduction, displaying values within the recommended range of 3.7–4.2 for good-quality silage classification, as suggested by Mcdonald et al. (1991). Grasses such as sorghum and maize exhibit good stability due to their adequate levels of soluble carbohydrates and lactic acid, which contribute to pH reduction when ensiled with appropriate levels of dry matter (Rodrigues et al., 2020).
The higher buffering capacity of Stylosanthes cv. Bela and Tamani guinea grass silages in monocropped and combined systems (Stylosanthes cv. Bela + Tamani guinea grass; Figure 4B) can be attributed to the inherent buffering capacity of forage grasses and legumes compared with sorghum silage in monocropped systems (Oliveira et al., 2020). This increased buffering capacity makes them more susceptible to proteolysis during the fermentation process, impeding pH reduction and resulting in inadequate forage preservation (Gomes et al., 2021). The intercropping of sorghum with forage crops contributed to a reduction in buffering capacity due to the higher proportion of sorghum in the ensiled mass (Table 1). Similar findings were reported by Oliveira et al. (2020), who evaluated silage production of sorghum intercropped with Paiaguas palisadegrass and observed that a higher proportion of sorghum effectively reduced the buffering capacity of monocropped grass silage and contributed to pH reduction. Studies by Carvalho et al. (2016) and Aloba et al. (2022) also observed improvements in the fermentation characteristics of monocropped legume silage with the inclusion of sorghum.
The dry matter (DM) content of the ensiled material significantly impacts the final silage quality as it affects material compaction and the fermentation process (Teixeira et al., 2021). Recommended DM values for good-quality silage classification range from 300 to 350 g kg−1 DM (Mcdonald et al., 1991), indicating that the fermentation process of silages from intercropping systems with sorghum occurred adequately. However, the silages of monocropped forage crops exhibited DM contents below 300 g kg−1 (Figure 4C), leading to increased effluent production and higher activity of Clostridium bacteria, compromising silage quality (Muck and Shinners, 2001). Additionally, as previously mentioned, tropical forage silages in monocropped systems without additives can display inadequate fermentation characteristics (Oliveira et al., 2020; Hawu et al., 2022). These results underscore the importance of silage production in integrated systems, as intercropping can reduce inadequate fermentation characteristics and increase the DM concentration of grass and legume silages, ensuring proper preservation of the ensiled material.
N-NH3 is a parameter used to assess the quality of the silage fermentation process because an increase in its production can neutralize the acids necessary for the proper fermentation of the ensiled material (Veriato et al., 2018). Silages with adequate fermentation, as defined by Kung et al. (2018), should have values below 100 g kg−1 of N-NH3, similar to the results observed in this study. Silages from intercropping systems with sorghum were more effective in reducing N-NH3 compared with silages of grass and legumes in monocropped and combination systems. Sorghum contains a sufficient amount of soluble carbohydrates and represents the largest proportion in intercropped silages (Table 1), enabling proper fermentation and lower nutrient loss, which aligns with the findings of Ni et al. (2018) and Li et al. (2022).
Higher DM losses were observed in Stylosanthes cv. Bela in monocropped and Bela + Tamani guinea grass systems (Figure 4A) due to the higher moisture content and lower DM content of legumes and grasses at the time of cutting for ensiling compared with monocropped sorghum (Table 2). Tropical forages typically have higher moisture content than annual crops at harvest (Bernardes et al., 2018). Additionally, high water activity and low concentrations of soluble carbohydrates can contribute to DM losses during secondary fermentation (Borreani et al., 2018).
Silage production should be optimized to achieve favorable fermentation patterns and preserve the nutritive value of the ensiled material, ultimately providing high-quality feed. One key management strategy to attain this objective is to minimize forage losses (Köhler et al., 2019). In the current study, silages from intercropping systems with sorghum demonstrated the potential in reducing total DM losses in tropical forage silages due to the higher proportion of sorghum (Table 1) incorporated in these silages, along with an appropriate DM content (340.66 g kg−1) at harvest.
Regarding effluent production, monocropped Tamani guinea grass and Stylosanthes cv. Bela silages, as well as the intercropping of Bela + Tamani guinea grass, exhibited the highest effluent production (Figure 5B) due to the high moisture content typically present in grasses and legumes at harvest, as previously mentioned. High effluent production leads to nutrient losses through leaching, which can result in nutritional degradation of the feed and potential environmental contamination (Araújo et al., 2020).
The intercropping systems with sorghum exhibited a significant reduction in effluent production, which was directly influenced by the higher proportion of sorghum present in these silages (Table 1). The high DM content of sorghum efficiently absorbed excess moisture from Tamani guinea grass and Stylosanthes cv. Bela, highlighting the close relationship between effluent production and the DM content of the ensiled material (Paludo et al., 2020). These results underscore the importance of silage production in intercropping systems for enhancing the fermentation process of tropical forage silages in monocropped systems and ensuring proper conservation of the ensiled material.
Silage production is a microbial fermentation process in which the lactic acid bacterial community plays a crucial role. These bacteria quickly establish dominance by consuming nutrients in the silage, creating a low-pH environment that inhibits the growth of undesirable protease and other bacterial communities, thus facilitating an adequate fermentation process (Wang et al., 2021). In this context, silages from intercropping systems with sorghum were more effective in increasing lactic acid values compared with silages of tropical forages in monocropped and combination systems, as shown in Figure 5C. Similar findings were reported by Meng et al. (2022), who evaluated the effects of different proportions of soybean and maize in an intercropping system and observed that intercropping enhanced the microbial community, particularly Lactobacillus and Weissella (co-producers of lactic acid), thereby improving fermentation and silage quality.
The silages of monocropped forages and their combination exhibited higher acetic acid values (Figure 5D). The intercropping systems resulted in a 13.78% reduction in acetic acid compared with the silages from monocropped and combination forages. However, the observed acetic acid values in all produced silages did not exceed 20 g kg−1 DM, which is considered suitable for classifying silage quality and ensuring proper preservation of the ensiled material, as reported by Kung et al. (2018). Therefore, the production of acetic acid did not negatively affect the stability of the silages, and the predominant production of lactic acid ensured the adequate preservation of the ensiled material (Oliveira et al., 2020).
Regarding the fibrous fractions (Figure 6), the NDF content represents the hemicellulose, cellulose, and lignin fractions, while the ADF content represents the cellulose and lignin fractions. These two fractions are important indicators of forage quality, with lower levels indicating better quality forage (Umesh et al., 2022), as they are negatively correlated with ruminant intake and digestibility (Tang et al., 2018). NDF values above 600 g kg−1 DM can reduce dry matter consumption due to rumen filling (Paludo et al., 2020). For ADF, values above 400 g kg−1 DM reduce fiber digestibility due to the unavailability of degradable structural carbohydrates, and the lignin content in the material hinders the adherence of rumen microbiota and the subsequent enzymatic hydrolysis of components such as cellulose and hemicellulose (Van Soest, 1994). In the present study, the ADF and NDF contents of all silages were lower than those previously reported.
The silages from the intercropping systems demonstrated the potential to reduce the fibrous fractions of the silages due to the lower NDF and ADF levels in sorghum (Table 2), resulting in the production of high-quality feed. Supporting these results, Oliveira et al. (2020) also observed a dilution of NDF and ADF contents when sorghum was intercropped with guava grass and when a higher proportion of sorghum was included in the silage. However, monocropped sorghum silage had the highest lignin content (Figure 6C). This can be attributed to the higher proportion of stalks in the crop, which is responsible for higher lignin accumulation (Oliveira et al., 2021).
The silage of Tamani guinea grass and Stylosanthes cv. Bela in monocropped and combination showed the lowest lignin contents due to the higher leaf blade-to-stalk ratio of Tamani guinea grass (Muniz et al., 2022), which contributed to the low accumulation of lignin in the forage and allowed for better digestibility (Paludo et al., 2020). Additionally, legumes such as Stylosanthes cv. Bela, which have herbaceous growth habit characterized by soft stems and a high number of leaves, have reduced lignin content (Castro-Montoya and Dickhoefer, 2020).
Thus, in the present study, it was observed that the double consortia of sorghum + Bela and sorghum + Tamani guinea grass, as well as the triple intercropping of sorghum + Tamani guinea grass + Bela, was efficient in diluting the lignin content of monocropped sorghum silage when ensiled. This emphasizes the importance of intercropping in the production of high-quality silage.
Silage production using integrated production systems is of fundamental importance for improving the nutritional characteristics of traditional maize and sorghum silage. The intercropping of cereals, grasses, and/or legumes primarily aims to increase the crude protein content (Ligoski et al., 2020) of the ensiled mass (Oliveira et al., 2020), as well as to enhance nitrogen use efficiency through biological fixation and minimize the use of mineral fertilizers (N), thereby reducing potential environmental impacts (Zhang et al., 2022) and promoting greater sustainability in food production.
The higher mineral matter content observed in the silages of Tamani guinea grass and Bela in monocropped and mixed systems is attributed to their lower DM content, higher pH, buffering capacity, and N-NH3 levels (Table 2). These factors contribute to an inadequate fermentation process with DM losses during fermentation, resulting in increased mineral matter content (Oliveira et al., 2020). In contrast, the higher proportion of sorghum in the intercropped silages (Table 1) leads to a reduction in mineral matter, as cereals generally have a low mineral matter content (Paula et al., 2016).
In the present study, we observed that the double consortia of sorghum + Tamani guinea grass, sorghum + Bela, and Bela + Tamani guinea grass, as well as the triple intercropping (sorghum + Tamani guinea grass + Bela), were more effective in increasing the crude protein content of the silage compared with sorghum silage in monocropped systems. Intercrops that included legumes had higher crude protein content than those with Tamani guinea grass (Figure 7A). The inclusion of Tamani guinea grass and Stylosanthes cv. Bela in the silage resulted in an increase in crude protein levels, with respective values of 130.80 and 155.35 g kg−1 DM. Thus, silages from integrated systems serve as not only an efficient alternative to increase silage mass production per unit area (Souza et al., 2019) but also a cost-effective approach as they maximize nutrient production per unit area in a sustainable manner (Umesh et al., 2022).
In addition to the nutritional benefits for animals, intercropping systems of grasses and legumes offer agronomic advantages such as reducing insects and pests, producing biomass for no-till farming systems, and lowering fertilizer costs through nutrient cycling, particularly with the presence of legumes that can supply adequate amount of nutrients to the soil-plant system (Ligoski et al., 2020; Bourscheidt et al., 2023). These systems also contribute to pasture recovery (Santos et al., 2020), providing sufficient high-quality feed for animals during the dry season. After forage regrowth, the established pasture can be utilized by animals (Oliveira et al., 2020), facilitating the sustainable intensification of the production system (Herrera et al., 2023).
The higher ether extract content observed in monocropped sorghum silage is attributed to the higher fat content in sorghum grains (Oliveira et al., 2021). These findings further support the observations of Bueno et al. (2020), who reported that well-preserved silages exhibit similar ether extract levels to those found in the original ensiled material. The IVDMD contents were similar among the studied silages, with an average value of 612.36 g kg−1. IVDMD can be used to assess the nutritional value and animal feed intake (Xie et al., 2022) and is considered one of the main determinants of forage quality (Daniel et al., 2019).
Silages from the intercropping systems demonstrated efficiency in increasing ether extract and TDN contents compared with silages from monocropped and combined forages, primarily due to the higher proportion of sorghum present in the material (Table 1). The increase in TDN content associated with sorghum in the intercrop was also observed by Ribeiro et al. (2017). It is worth noting that TDN content plays a crucial role in ruminant production as it, along with protein, can be a limiting factor (Oliveira et al., 2020).
Positive correlations between variables within the same group indicate a direct relationship, meaning that an increase in one variable leads to an increase in another, either directly or indirectly through other variables. On the other hand, negative correlations between variables from different groups indicate an inverse relationship, where an increase in one variable causes a reduction in the other and vice versa. Therefore, these results contribute to a better understanding of the relationship between the fermentation profile and the nutritional value of silage (Htet et al., 2021).
The regression models obtained for pH and IVDMD showed high coefficients of determination (R2 > 0.70), indicating that the independent variables included in the models are significant in determining pH and IVDMD. It is recommended to use regression models for bromatological variables when R2 is higher than 0.70 (Paludo et al., 2020; Rodrigues et al., 2020). Previous studies in the literature have also reported trail analyses with R2 values below 0.7, providing support for conducting this analysis (Crevelari et al., 2020; Ligoski et al., 2020).
The causal diagram of pH revealed high direct effects on CP, ADF, and IVDMD contents, suggesting that these variables are less influenced indirectly by other variables in their correlations with pH. However, despite having the highest percentage of direct effects, IVDMD showed a non-significant correlation with pH. Therefore, the variables with the greatest impact on pH were CP and ADF contents. On the other hand, CP, EE, lactic acid, and TDN exhibited low direct effects but had high correlations with pH, indicating that their effects occur indirectly through other variables in the model. Consequently, their inclusion is of limited importance in determining the effects of independent variables on pH.
The high direct effects of pH, CP, NDF, and ADF, along with their low correlation with IVDMD, indicate that these independent variables can provide significant benefits for estimation purposes when used in analyses together with other independent variables. However, they should not be relied upon solely. On the other hand, CP and TDN exhibited weak direct effects and correlations with IVDMD, suggesting that these variables have limited utility in the causal model involving IVDMD. These findings imply that the bromatological variables studied have minimal interference with IVDMD. Future research should explore other bromatological variables or different crops to determine how IVDMD can be improved, as it is a crucial variable in animal production.
Thus, our study highlights the importance of integrated systems, particularly the triple intercropping of annual and tropical forage crops (grasses and legumes), as a promising technique for silage production and the restoration of degraded areas or establishment of new pastures (Santos et al., 2020). Furthermore, this system ensures sustainable food production (Simões et al., 2023), mitigates greenhouse gas emissions (Eugène et al., 2021), promotes greater soil carbon sequestration, diversifies production (Bourscheidt et al., 2023), and reduces costs, particularly those associated with mineral nitrogen fertilizer inputs in the system (Bolson et al., 2022).
In conclusion, our study supports the use of sorghum intercropped with tropical forages in integrated silage production systems. This approach enhances land-use efficiency by increasing the production of ensiled mass per area and providing pasture after crop harvest. The intercropping improves fermentation characteristics and increases EE and TDN contents in monocropped forage silages. Additionally, the inclusion of tropical forages in sorghum silage reduces the need for protein salts in ruminant feed, leading to cost savings. Overall, the triple intercropping of sorghum + Tamani guinea grass + Bela is recommended for the production of high-quality silage and annual and tropical forage crops.
The raw data supporting the conclusions of this article will be made available by the authors, without undue reservation.
LP, KC, and LS wrote the manuscript. LP, LS, JC, and JS collected data in the field and processed the data. KC, AC, EH, and ES conceived and designed the experiments. All authors contributed to the revision of the manuscript and approved the submitted version.
The authors would like to thank the Instituto Federal Goiano for supporting this study and the Coordination for the Improvement of Higher Education Personnel (CAPES) for granting the Ph.D. scholarship.
The authors declare that the research was conducted in the absence of any commercial or financial relationships that could be construed as a potential conflict of interest.
All claims expressed in this article are solely those of the authors and do not necessarily represent those of their affiliated organizations, or those of the publisher, the editors and the reviewers. Any product that may be evaluated in this article, or claim that may be made by its manufacturer, is not guaranteed or endorsed by the publisher.
Aloba, T. A., Corea, E. E., Mendoza, M., Dickhoefer, U., and Castro-Montoya, J. (2022). Effects of ensiling length and storage temperature on the nutritive value and fibre-bound protein of three tropical legumes ensiled alone or combined with sorghum. Anim. Feed Sci. Technol. 283, 115172. doi: 10.1016/j.anifeedsci.2021.115172
AOAC (1990). Official Methods of Analysis. 15th Edn. Vol. 1, Agricultural Chemical; Contaminants; Drugs. Arlington: – Association of Official Analytical Chemists Inc., 768. Available online at: https://law.resource.org/pub/us/cfr/ibr/002/aoac.methods.1.1990.pdf (accessed April 15, 2020).
Araújo, J. A. S., Almeida, J. C. C., Reis, R. A., Carvalho, C. A. B., and Barbero, R. P. (2020). Harvest period and baking industry residue inclusion on production efficiency and chemical composition of tropical grass silage. J. Clean. Prod. 266, 12195. doi: 10.1016/j.jclepro.2020.121953
Bernardes, T. F., Daniel, J. L. P., Adesogan, A. T., Mcallister, T. A., Drouin, P., Nussio, L. G., et al. (2018). Silage review: unique challenges of silages made in hot and cold regions. J. Dairy Sci. 101, 4001–4019. doi: 10.3168/jds.2017-13703
Bolsen, K. K., Lin, C., and Brent, B. E. (1992). Effect of silage additives on the microbial succession and fermentation process of alfalfa and corn silages. J. Dairy Sci. 75, 3066–3083. doi: 10.3168/jds.S0022-0302(92)78070-9
Bolson, D. C., Jacovaci, F. A., Gritti, V. C., Bueno, A. V. I., Daniel, J. L. P., Nussio, L. G., et al. (2022). Intercropped maize-soybean silage: effects on forage yield, fermentation pattern and nutritional composition. Grassland Sci. 68, 3–12. doi: 10.1111/grs.12323
Borreani, G., Tabacco, E., Schmidt, R. J., Holmes, B. J., and Muck, R. E. (2018). Silage review: factors affecting dry matter and quality losses in silages. J. Dairy Sci. 101, 3952–3979. doi: 10.3168/jds.2017-13837
Borsellino, V., Migliore, G., D'acquisto, M., Di Franco, C. P., Asciuto, A., and Schimmenti, E. (2016). Green'wine through a responsible and efficient production: a case study of a sustainable Sicilian wine producer. Agric. Agric. Sci. Proc. 8, 186–192. doi: 10.1016/j.aaspro.2016.02.092
Botelho, H. A., Lago, A. A., Costa, A. L., Costa, A. C., Reis Neto, R. V., Silva, Z., et al. (2019). Application of morphometric measures in estimation of body weight and discrimination of Astyanax lacustris and Astyanax fasciatus. Aquac. Res. 50, 2429–2436. doi: 10.1111/are.14196
Bourscheidt, M. L., Gomes, F. J., Pedreira, C. G., Boote, K. J., Hoogenboom, G., Pereira, D. H., et al. (2023). Highlighting the benefits of biological nitrogen fixation on agronomic, physiological, and nutritive value traits of Brachiaria grass. Eur. J. Agron. 143, 126730. doi: 10.1016/j.eja.2022.126730
Braga, G. J., Ramos, A. K. B., Carvalho, M. A., Fonseca, C. E. L., Fernandes, F. D., and Fernandes, C. D. (2020). Liveweight gain of beef cattle in Brachiaria brizantha pastures and mixtures with Stylosanthes guianensis in the Brazilian savannah. Grass Forage Sci. 75, 206–215. doi: 10.1111/gfs.12473
Bueno, A. V. I., Lazzari, G., Jobim, C. C., and Daniel, J. L. P. (2020). Ensiling total mixed ration for ruminants: a review. Agronomy 10, 879. doi: 10.3390/agronomy10060879
Buffara, M. A., Silva, A. G., Teixeira, I. R., Costa, K. A. P., Simon, G. A., and Goulart, M. M. P. (2018). Seeding system and density for winter Urochloa ruziziensis intercropped with sorghum between soybean crops. Comun. Sci. 9, 340–350. doi: 10.14295/cs.v9i3.2536
Carvalho, W. G., Costa, K. A. P., Epifanio, P. S., Perim, R. C., Teixeira, D. A. A., and Medeiros, L. T. (2016). Silage quality of corn and sorghum added with forage peanuts. Rev. Caatinga 29, 465–472. doi: 10.1590/1983-21252016v29n224rc
Castro-Montoya, J. M., and Dickhoefer, U. (2020). The nutritional value of tropical legume forages fed to ruminants as affected by their growth habit and fed form: a systematic review. Anim. Feed Sci. Technol. 269, 114641. doi: 10.1016/j.anifeedsci.2020.114641
Charnet, R., Luna, C. A. L., Charnet, E. M. R., and Bonvino, H. (2008). Análise de modelos de regressão linear com aplicações, 2nd Edn. Campinas: Unicamp.
Coimbra, J. L. M., Benin, G., Vieira, E. A., Oliveira, A. C. D., Carvalho, F. I. F., Guidolin, A. F., et al. (2005). Consequências da multicolinearidade sobre a análise de trilha em canola. Ciênc Rural 35, 347–352. doi: 10.1590/S0103-84782005000200015
Costa, N. R., Andreotti, M., Crusciol, C. A. C., Pariz, C. M., Lopes, K. S. M., Yokobatake, K. L. A., et al. (2016). Effect of intercropped tropical perennial grasses on the production of sorghum-based silage. Agronomy J. 108, 2379–2390 doi: 10.2134/agronj2016.07.0385
Crevelari, J. A., Durães, N. N. L., Gonçalves, G. M. B., Ferreira Junior, J. A., Goncalves, V. M. L., Santanna, C. Q. S. S., et al. (2020). Phenotypic correlations, and path analysis between morphoagronomic and bromatological traits in corn hybrids for silage production. Aust. J. Crop Sci. 14, 1905–1912. doi: 10.21475/ajcs.20.14.12.2721
Cruz, C. D., Carneiro, P. C. S., and Regazzi, A. J. (2014). Modelos biométricos aplicados ao melhoramento genético. Rev. E Ampl. Viçosa, 2, 688.
Cruz, S. S. D., Andreotti, M., Pascoaloto, I. M., Lima, G. C. D., and Soares, C. D. A. (2020). Production in forage sorghum intercropped with grasses and pigeon pea at crop cutting. Rev. Ciênc Agron. 51, e20165389. doi: 10.5935/1806-6690.20200031
Daniel, J. L. P., Bernardes, T. F., Jobim, C. C., Schmidt, P., and Nussio, L. G. (2019). Production and utilization of silages in tropical areas with focus on Brazil. Grass For. Sci. 74, 188–200. doi: 10.1111/gfs.12417
Dias, M. B. C., Costa, K. A. P., Severiano, E. C., Bilego, U., Furtini Neto, A. E., Almeida, D. P., et al. (2020). Brachiaria and Panicum maximum in an integrated crop-livestock system and second-crop corn system in succession with soybean. J. Agric. Sci. 158, 1–12. doi: 10.1017/S0021859620000532
Epifanio, P. S., Costa, K. A. P., Severiano, E. C., Souza, W. F., Teixeira, D. A. A., Silva, J. T., et al. (2019b). Productive and nutritional characteristics of Brachiaria brizantha cultivars intercropped with Stylosanthes cv. Campo Grande in different forage systems. Crop Past. Sci. 70, 718–729. doi: 10.1071/CP18447
Epifanio, P. S., Costa, K. A. P., Severiano, E. D. C., Simon, G. A., and Silva, V. R. (2019a). Nitrogen nutrition and changes in the chemical attributes of the soil for cultivars of Brachiaria brizantha intercropped with Stylosanthes in different forage systems. Arch. Agron. Soil Sci. 66, 1154–1169. doi: 10.1080/03650340.2019.1658867
Eugène, M., Klumpp, K., and Sauvant, D. (2021). Methane mitigating options with forages fed to ruminants. Grass For. Sci. 76, 196–204. doi: 10.1111/gfs.12540
Ferreira, E. B., Cavalcanti, P. P., and Nogueira, D. A. (2014). ExpDes: an R package for ANOVA and experimental designs. Appl. Math. 5, 2952–2958. doi: 10.4236/am.2014.519280
Gomes, A. L. M., Auerbach, H. U., Lazzari, G., Moraes, A., Nussio, L. G., Jobim, C. C., et al. (2021). Sodium nitrite-based additives improve the conservation and the nutritive value of guinea grass silage. Anim. Feed Sci. Technol. 279, 115033. doi: 10.1016/j.anifeedsci.2021.115033
Hawu, O., Ravhuhali, K. E., Mokoboki, H. K., Lebopa, C. K., and Sipango, N. (2022). Sustainable use of legume residues: effect on nutritive value and ensiling characteristics of maize straw silage. Sustainability 14, 6743. doi: 10.3390/su14116743
Herrera, D. M., Peixoto, W. M., Abreu, J. G., Reis, R. H. P., Sousa, F. G., Balbinot, E., et al. (2023). Is the integration between corn and grass under different sowing modalities a viable alternative for silage? Animals 13, 425. doi: 10.3390/ani13030425
Htet, M. N. S., Hai, J. B., Bo, P. T., Gong, X. W., Liu, C. J., Dang, K., et al. (2021). Evaluation of nutritive values through comparison of forage yield and silage quality of mono-cropped and intercropped maize-soybean harvested at two maturity stages. Agriculture 11, 452. doi: 10.3390/agriculture11050452
Jobim, C. C., Nussio, L. G., Reis, R. A., and Schmidt, P. (2007). Avanços metodológicos na avaliação da qualidade da forragem conservada. Rev. Bras. Zootec. 36, 01–119. doi: 10.1590/S1516-35982007001000013
Köhler, B., Taube, F., Ostertag, J., Thurner, S., Klub, C., and Spiekers, H. (2019). Dry-matter losses and changes in nutrient concentrations in grass and maize silages stored in bunker silos. Grass For. Sci. 74, 274–283. doi: 10.1111/gfs.12430
Kung, L. Jr., and Shaver, R. (2001). Interpretation and use of silage fermentation analyses reports. Focus For. 3, 1–5.
Kung, L. Jr., Shaver, R. D., Grant, R. J., and Schmidt, R. J. (2018). Silage review: interpretation of chemical, microbial, and organoleptic components of silages. J. Dairy Sci. 101, 4020–4033. doi: 10.3168/jds.2017-13909
Li, J., Wen, X., Yang, J., Yang, W., Xin, Y., Zhang, L., et al. (2022). effects of maize varieties on biomass yield and silage quality of maize–soybean intercropping in the Qinghai–Tibet Plateau. Fermentation 8, 542. doi: 10.3390/fermentation8100542
Ligoski, B., Gonçalves, L. F., Cláudio, F. L., Alves, E. M., Kruger, A. M., Bizzuti, B. E., et al. (2020). Silage of intercropping corn, palisade grass, and pigeon pea increases protein content and reduces in vitro methane production. Agronomy 10, 1784. doi: 10.3390/agronomy10111784
Linhares, A. J. S., Goncalves, W. G., Cabral, S. M., Brito, M. F, Brandstetter, E. V., Silva, J. F. G., et al. (2020). Soil compaction affects sunflower and Paiaguas palisadegrass forage productivity in the Brazilian savanna. Aust. J. Crop Sci. 14, 1131–1139. doi: 10.21475/ajcs.20.14.07.p2305
Mateus, G. P., Crusciol, C. A. C., Pariz, C. M., Borghi, E., Costa, C., Martello, J. M., et al. (2016). Sidedress nitrogen application rates to sorghum intercropped with tropical perennial grasses. Agron. J. 108, 433–447. doi: 10.2134/agronj2015.0236
Mcdonald, P. J., Henderson, A. R., and Heron, S. J. E. (1991). The Biochemistry of Silage. Edinburgh:Mallow Chalcombe publications.
Meng, H., Jiang, Y., Wang, L., Wang, S., Zhang, Z., Tong, X., et al. (2022). Effects of different soybean and maize mixed proportions in a strip intercropping system on silage fermentation quality. Fermentation 8, 696. doi: 10.3390/fermentation8120696
Mertens, D. R. (2002). Gravimetric determination of amylase-treated neutral detergent fiber in feeds with refluxing in beaker or crucibles: collaborative study. J. AOAC Int. 85, 1217–1240.
Muck, R. E., and Shinners, K. J. (2001). Conserved Forage (Silage an Hay): Progress an Priorities. International Grassland Congress. São Pedro; Piracicaba: FEALQ, 753.
Muniz, M. P., Costa, K. A. D. P., Severiano, E. C, Bilego, U. O., Vilela, L., Dias, M. B. C., et al. (2022). Production, forage quality and cattle performance in Paiaguas palisadegrass and Tamani grasses in different forms of animal supplementation in crop-livestock integration. Aust. J. Crop Sci. 16, 381–388. doi: 10.21475/ajcs.22.16.03.p3413
Muniz, M. P., Costa, K. A. P., Severiano, E. C., Bilego, U. O., Almeida, D. P., Furtini Neto, A. E., et al. (2021). Soybean yield in integrated crop–livestock system in comparison to soybean–maize succession system. J. Agric. Sci. 1, 1–11. doi: 10.1017/S0021859621000393
Ni, K., Zhao, J., Zhu, B., Su, R., Pan, Y., Ma, J., et al. (2018). Assessing the fermentation quality and microbial community of the mixed silage of forage soybean with crop corn or sorghum. Bioresour. Technol. 265, 563–567. doi: 10.1016/j.biortech.2018.05.097
Oliveira, N. C., Costa, K. A. P., Rodrigues, L. G., Silva, A. C. G., Costa, J. V. C. P., Silva, S. Á. A., et al. (2021). Fermentation characteristics and nutritive value of sweet sorghum silage with Paiaguas palisadegrass and Ipypora grass. Semina: Ciênc. Agrár. 42, 1923–1940. doi: 10.5433/1679-0359.2021v42n3Supl1p1923
Oliveira, S. S., Costa, K. A. P., Souza, W. F., Santos, C. B., Teixeira, D. A. A., and Silva, V. C. (2020). Production and quality of the silage of sorghum intercropped with Paiaguas palisadegrass in different forage systems and at different maturity stages. Anim. Prod. Sci. 60, 694–704. doi: 10.1071/AN17082
Paludo, F., Costa, K. A. P., Dias, M. B. C., Silva, F. A. S., Silva, A. C. G., Rodrigues, L. G., et al. (2020). Fermentative profile and nutritive value of corn silage with Tamani guinea grass. Semina Ciênc. Agrár. 41, 2733–2746. doi: 10.5433/1679-0359.2020v41n6p2733
Paula, F. L. M., Menezes, L. F. G., Paris, W., Ronsani, R., Hoppen, S. M., and Ciesca, J. (2016). Produção química de silagem e composição do consórcio de milho e capim-Tanzânia. Semina Ciênc. Agrár. 37, 1607–1616. doi: 10.5433/1679-0359.2016v37n3p1607
Perazzo, A. F., Carvalho, G. G., Santos, E. M., Bezerra, H. F., Silva, T. C., Pereira, G. A., et al. (2017). Agronomic evaluation of sorghum hybrids for silage production cultivated in semiarid conditions. Front. Plant Sci. 8, 088. doi: 10.3389/fpls.2017.01088
R Core Team (2014). R: A Language and Environment for Statistical Computing. Vienna: R Foundation for Statistical Computing (2014).
Ribeiro, F. M., Lima, M., Costa, P. A. T., Pereira, D. M., Carvalho, T. A., Souza, T. V., et al. (2019). Associations between morphometric variables and weight and yields carcass in Pirapitinga Piaractus brachypomus. Aquac. Res. 50, 2004–2011. doi: 10.1111/are.14099
Ribeiro, M. G., Costa, K. A. P., Souza, W. F., Cruvinel, W. S., Silva, J. T., and Santos Júnior, D. R. (2017). Silage quality of sorghum and Urochloa brizantha cultivars monocroppedped or intercropped in different planting systems. Acta Sci. Anim. Sci. 39, 243–250. doi: 10.4025/actascianimsci.v39i3.33455
Rodrigues, P. H. M., Pinedo, L. A., Meyer, P. M., Silva, T. H., and Guimarães, I. C. S. B. (2020). Sorghum silage quality as determined by chemical–nutritional factors. Grass For. Sci. 75, 462–473. doi: 10.1111/gfs.12495
Santos, C. B., Costa, K. A. P., Souza, W. F., Silva, A. G., Silva, V. C., Oliveira, I. P., et al. (2020). Intercropping of sorghum with Paiaguas palisadegrass in a crop-livestock integration system for pasture recovery. Aust. J. Crop Sci. 14, 1072–1080. doi: 10.21475/ajcs.20.14.07.p2216
Santos, H. G., Jacomine, P. K. T., Anjos, L. H. C., Oliveira, V. A., Lumbreras, J. F., Coelho, M. R., et al. (2018). Sistema Brasileiro de Classificação de Solos. Embrapa CNPS 5th Edn (Brasília: Embrapa), 356.
Sekaran, U., Lai, L., Ussiri, D. A., Kumar, S., and Clay, S. (2021). Role of integrated crop-livestock systems in improving agriculture production and addressing food security–A review. J. Agric. Food Res. 5, 100190. doi: 10.1016/j.jafr.2021.100190
Silva, L. M., Costa, K. A. P., Costa, A. C., Oliveira, K. J., Silva, J. A. G., Costa, J. V. C. P, et al. (2023). Fermentation dynamics and quality of maize silage with Pigeon pea. Semina Cienc. Agrár. 44, 567–584. doi: 10.5433/1679-0359.2023v44n2p567
Simões, V. J. L. P., Souza, E. S., Martins, A. P., Tiecher, T., Bremm, C., Ramos, J. S., et al. (2023). Structural soil quality and system fertilization efficiency in integrated crop-livestock system. Agric. Ecosyst. Environ. 349, 108453. doi: 10.1016/j.agee.2023.108453
Soussana, J. F., and Lemaire, G. (2014). Coupling carbon and nitrogen cycles for environmentally sustainable intensification of grasslands and crop-livestock systems. Agric. Ecosyst. Environ. 190, 9–17. doi: 10.1016/j.agee.2013.10.012
Souza, W. F., Costa, K. A. P., Guarnieri, A., Severiano, E. C., Silva, J. T., Teixeira, D. A. A., et al. (2019). Production, and quality of the silage of corn intercropped with Paiaguas palisadegrass in different forage systems and maturity stages. Rev. Bras. Zootec 48, e20180222. doi: 10.1590/rbz4820180222
Tang, C., Yang, X., Chen, X., Ameen, A., and Xie, G. (2018). Sorghum biomass and quality and soil nitrogen balance response to nitrogen rate on semiarid marginal land. Field Crops Res. 215, 12–22. doi: 10.1016/j.fcr.2017.09.031
Teixeira, D. A. A., Costa, K. A. P., Souza, W. F., Severiano, E. C., Guimaraes, K. C., Silva, J. T., et al. (2021). Fermentation profile and nutritive value of maize silage with 'Brachiaria' species. Aust. J. Crop Sci. 15, 695–702. doi: 10.21475/ajcs.21.15.05.p3004
Tilley, J. M. A., and Terry, R. A. (1963). A two-stage technique of the “in vitro” digestion of forage crop. J. Br. Grassl. Soc. 18, 04–111. doi: 10.1111/j.1365-2494.1963.tb00335.x
Umesh, M. R., Angadi, S., Begna, S., and Gowda, P. (2022). Planting density and geometry effect on canopy development, forage yield and nutritive value of sorghum and annual legumes intercropping. Sustainability 14, 4517. doi: 10.3390/su14084517
Van Soest, P. J. (1994). Nutritional Ecology of the Ruminant, 2nd Edn. Ithaca, NY: Cornell University Press.
Veriato, F. T., Pires, D. A. A., Tolentino, D. C., Alves, D. D., Jayme, D. G., and Moura, M. M. A. (2018). Fermentation characteristics and nutritive values of sorghum silages. Acta Sci. Anim. Sci. 40, e34458. doi: 10.4025/actascianimsci.v40i1.34458
Vincent-Caboud, L., Casagrande, M., David, C., Ryan, M. R., Silva, E. M., Peigne, J., et al. (2019). A review. Agron. Sustain. Dev. 39, 1–15. doi: 10.1007/s13593-019-0590-2
Wang, M., Gao, R., Franco, M., Hannaway, D. B., Ke, W., Ding, Z., et al. (2021). Effect of mixing alfalfa with whole-plant corn in different proportions on fermentation characteristics and bacterial community of silage. Agriculture 11, 174. doi: 10.3390/agriculture11020174
Xie, Y., Wang, L., Li, W., Xu, S., Bao, J., Deng, J., et al. (2022). Fermentation quality, in vitro digestibility, and aerobic stability of total mixed ration silage in response to varying proportion alfalfa silage. Animals 12, 1039. doi: 10.3390/ani12081039
Keywords: fiber fraction, Panicum maximum cv. BRS Tamani, crude protein, Sorghum bicolor, Stylosanthes guianensis cv. Bela, fermentation profile
Citation: Prado LG, Costa KAdP, da Silva LM, Costa AC, Severiano EdC, Costa JVCP, Habermann E and e Silva JAG (2023) Silages of sorghum, Tamani guinea grass, and Stylosanthes in an integrated system: production and quality. Front. Sustain. Food Syst. 7:1208319. doi: 10.3389/fsufs.2023.1208319
Received: 18 April 2023; Accepted: 20 June 2023;
Published: 14 July 2023.
Edited by:
Siran Wang, Nanjing Agricultural University, ChinaReviewed by:
Glayciane Costa Gois, Federal University of São Francisco Valley, BrazilCopyright © 2023 Prado, Costa, da Silva, Costa, Severiano, Costa, Habermann and e Silva. This is an open-access article distributed under the terms of the Creative Commons Attribution License (CC BY). The use, distribution or reproduction in other forums is permitted, provided the original author(s) and the copyright owner(s) are credited and that the original publication in this journal is cited, in accordance with accepted academic practice. No use, distribution or reproduction is permitted which does not comply with these terms.
*Correspondence: Kátia Aparecida de Pinho Costa, a2F0aWEuY29zdGFAaWZnb2lhbm8uZWR1LmJy
Disclaimer: All claims expressed in this article are solely those of the authors and do not necessarily represent those of their affiliated organizations, or those of the publisher, the editors and the reviewers. Any product that may be evaluated in this article or claim that may be made by its manufacturer is not guaranteed or endorsed by the publisher.
Research integrity at Frontiers
Learn more about the work of our research integrity team to safeguard the quality of each article we publish.