- 1Institute of Crop Protection, Guizhou University, Guiyang, China
- 2Research Center for Engineering Technology of Kiwifruit, Guizhou University, Guiyang, China
To find a precise, efficient, and safe method to control rice sheath blight disease (caused by Rhizoctonia solani), this study used the natural product magnolol as the fungicide. Based on the characteristics of cell wall-degrading enzymes (CWDEs) released by R. solani during the early stages of infection, a Mag@CMCS@CPSG (magnolol@carboxymethyl chitosan@carboxymethyl cellulose, pectin, sodium alginate, and glycerol) film was constructed to control the release of magnolol in response to CWDEs. The Mag@CMCS@CPSG film increased magnolol toxicity against R. solani, decreased EC50 from 7.33 μg/mL to 4.37 μg/mL, and effectively blocked the invasion of mycelia. Under the stimulation of CWDEs released during R. solani infection, the cumulative release of magnolol by the Mag@CMCS@CPSG film reached 75.62% after 96 h, and the disease index of rice treated with Mag@CMCS@CPSG film significantly decreased. The maximum field control effect of Mag@CMCS@CPSG film on rice sheath blight disease was 72.47%, which was not significantly different from that of the commercial preparation jinggangmycin; however, the holding period was better. The Mag@CMCS@CPSG film reduced the loss of rice yield and quality caused by infection with R. solani and did not cause phytotoxicity or residue. In conclusion, the Mag@CMCS@CPSG film can achieve accurate and green control of rice sheath blight.
1. Introduction
Rice is the most widely grown food crop globally and is a staple food for more than half of the world's population. However, rice sheath blight is one of the most destructive and economically harmful diseases affecting rice production worldwide, it can reduce rice yields by 2.5–50%, and rice quality can be reduced (Groth, 2008; Chen et al., 2023; Shen et al., 2023). Rice sheath blight is a fungal disease caused by Rhizoctonia solani. By secreting cell wall-degrading enzymes (CWDEs), R. solani decomposes the rice cell wall and destroys its mechanical defense function, thus completing the infection (Lakshman et al., 2016; Rao et al., 2020). Currently, synthetic chemical fungicides are the primary method used to control rice sheath blight. However, owing to the long-term and unscientific use of chemical pesticides, problems of pathogen resistance and pesticide residues have increased the risk to food security (Garefalaki et al., 2022; Wang et al., 2023; Yousefi et al., 2023).
To reduce the reliance on synthetic pesticides, we explored plant extracts that effectively inhibited R. solani. We found that natural magnolol (Mag), a natural compound derived from the Chinese herb Magnolia officinalis (Wang et al., 2020), destroyed the plasma membrane and caused the death of R. solani (Mo et al., 2021). However, Mag is insoluble in water, and its hydroxyl structure is easily oxidized, limiting its utilization (Han et al., 2021; Usach et al., 2021). To achieve the accurate and efficient control of plant diseases, Mag was loaded with carboxymethyl chitosan (CMCS) to form water-soluble Mag@CMCS particles in our previous studies, and carboxymethyl cellulose (CMC) and pectin (PT) were used to simulate plant cell wall components. Using sodium alginate (SA) and glycerol (Gl), an enzyme response-controlled release film, CPSG, was constructed. After coating with the Mag@CMCS particles, a Mag@CMCS@CPSG film was formed. The Mag@CMCS@CPSG film exhibited excellent flexibility, light transmittance, air permeability, and adhesion (Mo et al., 2023). However, the performance of the Mag@CMCS@CPSG films in practical applications is unclear.
This study used the rice sheath blight as the model disease to clarify the disease control effect and safety of the Mag@CMCS@CPSG film. The inhibition effect of Mag@CMCS@CPSG film on R. solani, the release of magnolol performance in response to R. solani infection, the field control effect on rice sheath blight disease, and the effect on rice quality were investigated using toxicity assay, in vivo inoculation, and a field experiment. The experimental results confirmed the application performance of the Mag@CMCS@CPSG film and provided a new method for efficiently utilizing plant extracts with poor stability.
2. Materials and methods
2.1. Materials
Rhizoctonia solani AG 1-IA was provided by the Crop Protection Institute of Guizhou University, cultured in potato dextrose agar (PDA) medium, and stored at 4 °C. Magnolol (Mag) ≥98% purchased from Aladdin Biochemical Technology Co., Ltd. (Shanghai, China). Carboxymethyl chitosan (CMCS), cellulase, and pectinase were purchased from Solaibao Technology Co., Ltd. (Beijing, China). Carboxymethyl cellulose (CMC), pectin (PT), sodium alginate (SA), and glycerol (Gl) were purchased from Macklin Biochemical Co., Ltd. (Shanghai, China). Surfactant OP-15 was purchased from Haian Petrochemical Plant (Nantong, China).
2.2. Preparation of Mag@CMCS@CPSG film
Following the method reported by Mo et al. (2023), 2 g of CMCS was added to 80 ml of water and stirred until completely dissolved. Two grams of magnolol were dissolved in 20 ml of 40% methanol solution and added to the CMCS solution. A multi-tube vortex oscillator (Ruicheng Instrument Co., Ltd., Hangzhou, China) was oscillated for 6 h at 1,500 rpm and then freeze-dried to form Mag@CMCS particles. Subsequently, 0.875 g CMC, 0.125 g PT, 0.625 g SA, and 0.375 g Gl were added to a beaker, and water was added to 60 ml and stirred until dissolution to form the CPSG solution. Mag@CMCS particles were dissolved in 40 ml of water and added to the CPSG membrane solution with 10 ml of surfactant OP-15. After magnetic stirring for 2 h, the solution was allowed to stand for 1 h until the bubbles completely disappeared. A 20 ml film solution was poured on a 15 × 15 cm acrylic plate, dried at room temperature (25 ± 2°C) for 4 h, and gently torn off, that is, Mag@CMCS@CPSG film. Using the same preparation method but without the addition of Mag, the resulting CMCS@CPSG film was obtained.
2.3. Toxicity test of Mag@CMCS@CPSG film against R. solani
The toxicity of Mag, CMCS@CPSG film, and Mag@CMCS@CPSG film to R. solani was determined using the mycelium growth rate method (Zhao et al., 2021), and 28% Jinggangmycin-soluble powder (SP) (Qianjiang Biochemical, Haining, China), a commercial preparation, was used as a control agent. Mag was dissolved in a small amount of methanol and diluted with sterile water. CMCS@CPSG film, Mag@CMCS@CPSG film, and 28% Jinggangmycin SP were diluted with sterile water. Five different drug concentrations were evenly mixed with PDA to prepare drug-containing media. R. solani colonies with a diameter of 5 mm were inoculated into the drug-containing medium, each concentration was treated six times and incubated at 28°C for 48 h under alternating light and dark conditions, and then, the colony diameter was measured. IBM SPSS Statistics 20.0 (Armonk, NY, United States) was used to calculate the effective medium concentration (EC50) of different drugs. CMCS@CPSG and Mag@CMCS@CPSG films were cut into 2 × 2 cm pieces and placed on a PDA medium. A 5 mm R. solani colony was placed on the film to observe mycelia growth.
2.4. Determination of magnolol release ability of Mag@CMCS@CPSG film stimulated by CWDEs
The Mag@CMCS@CPSG film was cut into 3 × 3 cm slices and placed in a dialysis bag with a molecular weight of 1,000. Mixed solutions of cellulase and pectinase at different concentrations were added simultaneously to the dialysis bag. Then, the dialysis bag was sealed and placed in 300 ml of distilled water at 35°C. The dialysis bag was taken out 96 h later and placed into methanol solution for an ultrasound for 30 min, and 10 ml solution was taken to determine the residual amount of Mag using high-performance liquid chromatography (HPLC). The detection methods were described by Tang et al. (2019) and Zhou et al. (2020). HPLC was performed using a ZORBAX 300SB-C18 column (Agilent Technologies Co., Ltd., Santa Clara, United States), with the measuring wavelength at 294 nm, mobile phase consisting of a methanol:water ratio of 78:22, a sample size of 5 μl, a flow rate of 1 ml/min, and column temperature of 30°C. The sum of the remaining Mag in the dialysis bag and magnolol in the aqueous solution outside the dialysis bag after 96 h was the total amount of Mag in the Mag@CMCS@CPSG film. The release rate of Mag was defined as the ratio of the Mag content in the solution outside the dialysis bag to the total amount of Mag in the film. Each treatment was repeated four times.
2.5. Determination of magnolol release ability of Mag@CMCS@CPSG film stimulated by R. solani infection
The leaf sheath was taken as the center, cut into 15 cm pieces of rice stem tissue with a similar diameter, wrapped at the end near the root with absorbent cotton moistened with sterile water, and 1 g Mag@CMCS@CPSG film was added to 500 ml water, sprayed on the surface of the rice stem tissue, and allowed to stand at room temperature for 4 h until the film dried. R. solani colonies with a diameter of 5 mm were inoculated on the surface of leaf sheaths (another treatment method was to inoculate R. solani on the surface of leaf sheaths for 48 h and then spray them with Mag@CMCS@CPSG film), with only R. solani treatment as the control. At 6, 12, 24, 48, 72, and 96 h after treatment, stem tissues (3 cm in length) were clipped with the leaf sheath as the center and ground into powder in liquid nitrogen for later use. Rice tissue powder (0.1 g) was extracted using a cellulase and pectinase extraction kit, and cellulase and pectinase activities were measured using a multifunctional enzyme marker. Each treatment was repeated four times. Simultaneously, the disease index of the rice tissue was investigated, and 18 rice plants were investigated each time. Disease grade value and investigation methods were described by Begum et al. (2010) and Jiang et al. (2018), as follows: Grade 0: no disease spots, Grade 1: spot diameter R ≤ 0.5 cm, Grade 3: spot diameter 0.5 cm < R ≤ 1.0 cm, Grade 5: spot diameter 1.0 cm < R ≤ 3.0 cm, Grade 7: spot diameter 3.0 cm < R ≤ 5.0 cm, and Grade 9: spot diameter R > 5.0 cm.
Using the same treatment method as described above, after 6, 12, 24, 48, 72, and 96 h, rice stem tissues were rinsed with 20 ml methanol for 20 s and then ground into powder in liquid nitrogen. One gram was added to a 10 ml centrifuge tube containing 5 mL methanol, and the ultrasonic scrubber was ultrasonicated for 30 min at 60 kHz. After centrifugation at 4°C for 10 min at 8,000 rpm, the supernatant was collected, and the magnolol content was determined using HPLC. Each treatment was repeated four times.
2.6. Determination of field control effect of Mag@CMCS@CPSG film on rice sheath blight disease
For the field experiment, the rice variety used was Guang-8-You 198 on flat land, and rice sheath blight disease occurred for 3 consecutive years. The trial comprised five treatments, 2, 1.25, and 0.83 g/L Mag@CMCS@CPSG film solution, 2 g/L Mag, 0.67 g/L 28% Jinggangmycin SP (the manufacturer recommends the dosage), and the water treatment was the blank control. Each treatment was repeated four times and each replicate consisted of one plot (20 m2). A total of 28 plots were constructed using a random block design. The field experiment used two different application methods as follows: one was applied at the booting stage (rice sheath blight did not occur), and the other was applied at the full heading stage (rice sheath blight early). The incidence at 7, 14, 21, and 28 days after the application was investigated, and the control effect was calculated. Disease grade values and investigation methods were described by Zheng et al. (2021) as follows: Grade 0: no disease in the whole plant; Grade 1: the incidence of the fourth leaf and the following leaf sheaths and leaves (with sword leaf as the first leaf); Grade 3: the incidence of the third leaf and the following leaf sheaths and leaves; Grade 5: disease of the second leaf and the following leaf sheaths and leaves; Grade 7: sword leaf blade and the following leaf sheath and leaf disease; and Grade 9: whole plant disease, early death. The disease index was calculated using Formula (1), and the control effect was calculated as follows:
In Formula (2), CK1 refers to the disease index after application to the blank control area, and PT1 refers to the disease index after application to the treatment area.
Simultaneously, chlorophyll content (SPAD value), intercellular carbon dioxide concentration, and photosynthetic rate of rice were measured by 3051D photosynthetic apparatus (Zhejiang TOP Cloud-agri Technology Co., Ltd, Hangzhou, China), to determine the phytotoxicity of Mag@CMCS@CPSG film to rice.
2.7. Determination of the effect of Mag@CMCS@CPSG film on rice quality
In the test in Section 2.6, the rice was treated with 2 g/L Mag@CMCS@CPSG film solution, 2 g/L Mag, and 0.67 g/L 28% Jinggangmycin SP and CK (52 days after application at the booting stage and 30 days after application at the full head stage). The number of rice holes in each plot was counted during harvesting, and the total number of rice holes per 667 m2 was calculated. Three rice holes were randomly selected from each cell to count the panicle number, and 10 panicles were randomly selected according to a five-point sampling method, to count the total grain per panicle, setting rate, and 1,000-grain weight of rice and to calculate the theoretical yield (Ravshanov et al., 2023).
After harvesting, the rice was dried in the oven at 50°C for 4 days, and the brown rice rate and head yield were measured (Tan et al., 2023). The grain chalkiness percentage and degree were determined using a TPMZ-A rice appearance quality tester (Topu Yunnong Technology Co., Ltd., Hangzhou, China). The amylose content was determined as follows: the milled rice sample was ground into a powder, and the rice flour sample was picked up through a sieve with a bore diameter of 0.25 mm. The rice flour sample was placed in a 100 mL volumetric bottle, and 1 mL of 95% ethanol was added. The volumetric bottle was gently shaken to moisten and disperse the sample, and then, 9.0 mL 1N NaOH solution was added to rotate the volumetric bottle to rinse the sample adhering to the bottle wall with the lye solution.
The rice was dried and peeled to obtain milled rice, which was ground into a powder using a multifunctional grinder (Mofei Household Appliances Co., Ltd., Jinhua, China). Rice powder (1 g) was added to 10 mL of methanol solution. After stirring, the rice powder was ultrasonicated for 5 min, centrifuged at 5,000 rpm at 4°C for 5 min using an ST 16R refrigerated centrifuge (Thermo Fisher Scientific, Waltham, United States), and the supernatant was collected. The Mag content in the rice powder was determined using HPLC, and each treatment was repeated four times.
2.8. Statistical analysis
All data were tested for normality and variance equality before statistical analysis, and the results were expressed as mean ±SE (standard error). Significance analysis was conducted using a one-way analysis of variance (ANOVA) and Duncan's new complex range method (P < 0.05). All statistical analyses and charting were performed using IBM SPSS Statistics 20.0 and Origin 2021 (Origin Lab, Northampton, MA, United States), respectively.
3. Results
3.1. Toxicity of the Mag@CMCS@CPSG film against R. solani
As shown in Figure 1, the EC50 of the CMCS@CPSG film against R. solani was 5,604.58 μg/mL, indicating that the CMCS and CPSG films to R. solani were less toxic and had no evident inhibitory effect at low concentrations. The EC50 value of the Mag@CMCS@CPSG film was only 4.37 μg/ml, which was significantly lower than that of Mag (7.33 μg/ml), indicating that Mag improved toxicity after processing into films. This may be because the film fixed the issue of water solubility and improved the dispersibility of Mag. Therefore, it was beneficial for the antifungal effect of Mag. Jinggangmycin is a commercial fungicide commonly used to control rice sheath blight, so it was used as the control preparation in this study (Jiang et al., 2012). The commercial preparation of 28% Jinggangmycin SP showed low in vitro toxicity of R. solani (EC50 was 56.65 μg/ml) because the control mechanism of Jinggangmycin was to weaken the pathogenicity of pathogenic fungi (Li et al., 2018).
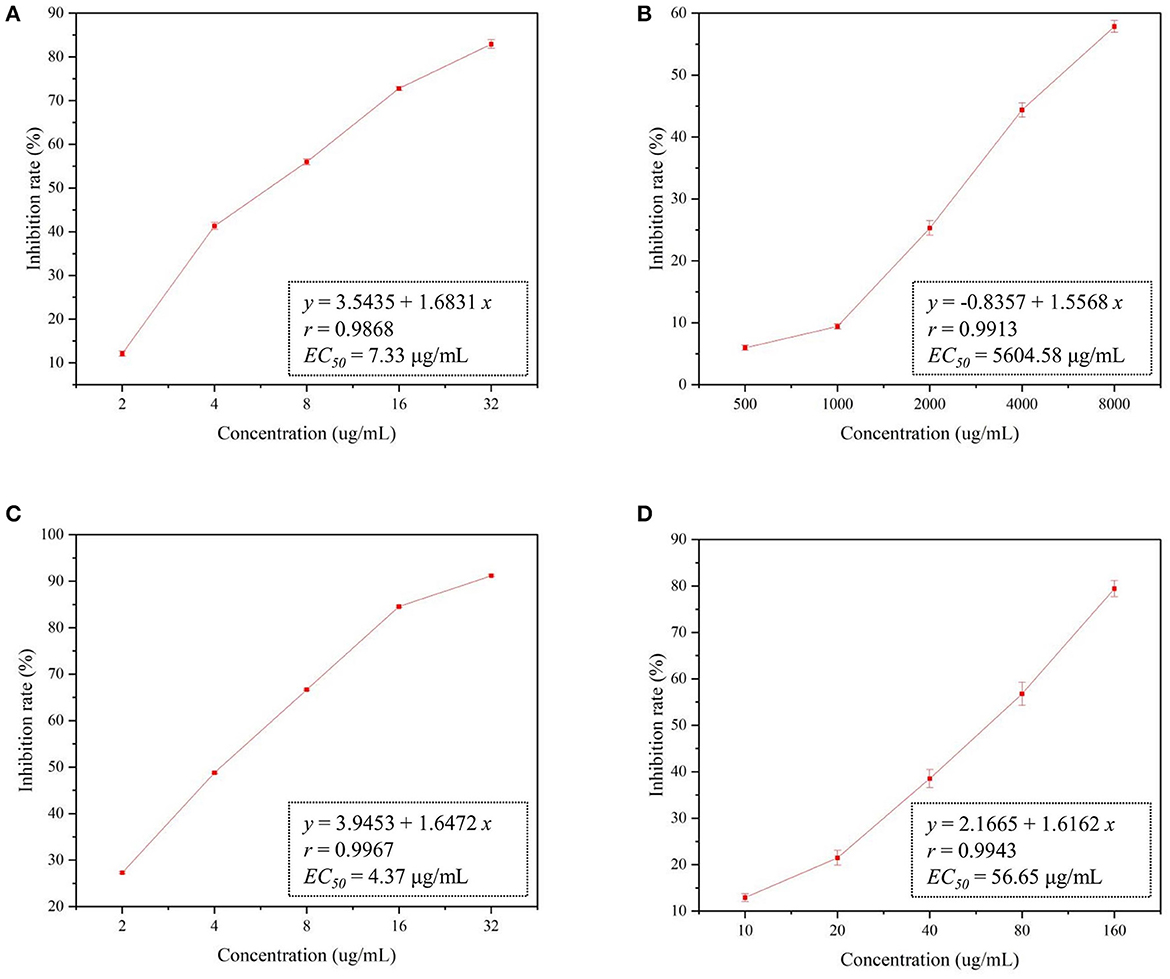
Figure 1. In vitro toxicity of R. solani by different drugs. (A): Mag, (B): CMCS@CPSG film, (C): Mag@CMCS@CPSG film, (D): Jinggangmycin.
As shown in Figure 2, R. solani could not grow on a PDA medium containing Mag, indicating that Mag significantly inhibited the hyphal growth of R. solani. When the CMCS@CPSG film was used to block R. solani from the PDA medium, the mycelia of R. solani could penetrate the CMCS@CPSG film and obtain nutrients from the medium to help the mycelia grow rapidly. This may have been due to the release of CWDEs by R. solani upon contact with the CPSG film, which destroyed the integrity of the CPSG film. When the Mag@CMCS@CPSG film was used to block R. solani from the medium, the mycelia could not penetrate the film to obtain nutrition. The Mag@CMCS@CPSG film effectively blocked the invasion of R. solani mycelia, possibly owing to the release of CWDEs after contact with the Mag@CMCS@CPSG film, and the magnolol in the film was released, thus inhibiting mycelia growth.
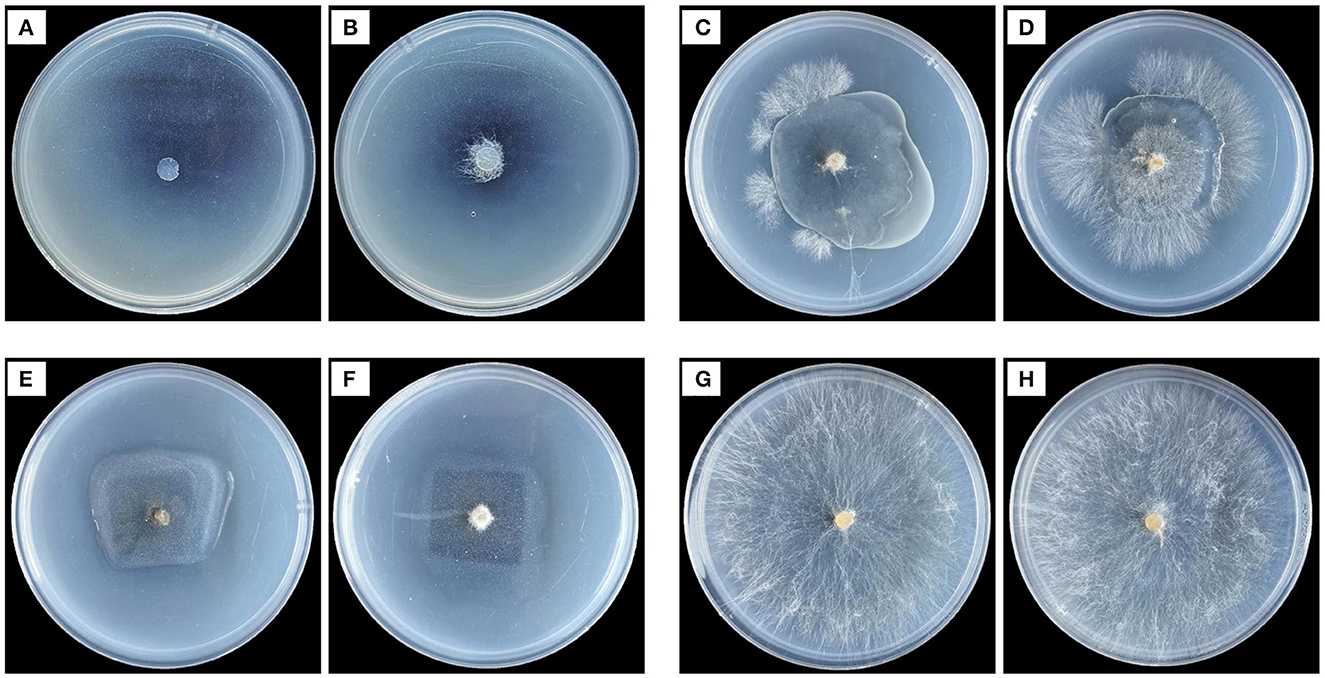
Figure 2. Effects of different treatments on hyphal growth of R. solani. (A, B) are Mag 10 μg/ml treatment, (C, D) are CMCS@CPSG film, (E, F) are MagCMCS@CPSG film, (G, H) are CK.
3.2. Mag@CMCS@CPSG film release Mag ability in response to CWDEs
Cellulases and pectinases are the two main types of CWDEs (Sun et al., 2023). As shown in Figure 3, the release of Mag from the Mag@CMCS@CPSG film increased with increasing cellulase and pectinase concentrations. However, the amount of Mag released by the Mag@CMCS@CPSG film treated with cellulase or pectinase alone at different concentrations was significantly lower than the synergistic action of the two enzymes after 96 h, and the cumulative release of Mag reached 76.09% after 96 h of treatment with 200 U/ml of cellulase and 200 U/ml of pectinase. In conclusion, the release of Mag was accelerated by stimulation with cellulase and pectinase, indicating that the Mag@CMCS@CPSG film could accelerate the release of Mag in response to CWDEs.
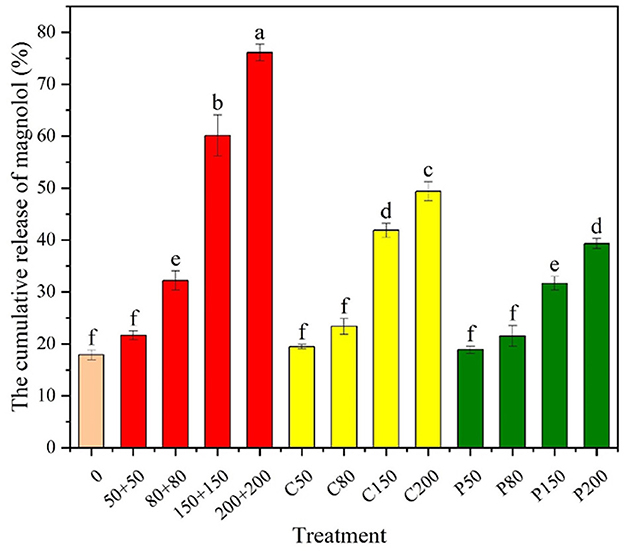
Figure 3. Magnolol release from the Mag@CMCS@CPSG film after CWDE stimulation for 96 h (n = 4). 0 represents the concentration of the enzyme 0, “50+50” refers to cellulase 50 U/ml+ pectinase 50 U/ml, C50 refers to cellulase 50 U/ml, and P50 refers to pectinase 50 U/ml. All data were expressed as mean ± SE, the lowercase letters in the figure represent significant differences (P < 0.05).
3.3. Mag@CMCS@CPSG film release Mag ability in response to the infection of R. solani
We determined whether the Mag@CMCS@CPSG film could form a protective layer on the surface of rice plants and release Mag in response to the infection of R. solani to exert an antifungal effect. As shown in Figure 4A, after spraying the Mag@CMCS@CPSG film for 4 h and inoculating R. solani, the cellulase and pectinase activities on the rice surface began to rise sharply after 12 h, and the cellulase activities increased to 193.68 U/g after 48 h and reached a peak of 195.24 U/g after 72 h. Pectinase activity also peaked at 166.90 U/g after 48 h. However, there was no significant change in the activities of cellulase and pectinase in the treatment with only the Mag@CMCS@CPSG film, which indicated that the cellulase and pectinase released by R. solani were responsible for the increase in the activities of the two enzymes on the surface of the rice plants. As shown in Figure 4C, the content of Mag on the surface of rice plants treated with the Mag@CMCS@CPSG film and inoculated with R. solani began to decrease rapidly after 12 h and decreased to 1.17 μg/kg after 96 h, with a release of 75.62%, whereas the release of Mag was only 21.98% when treated without R. solani.
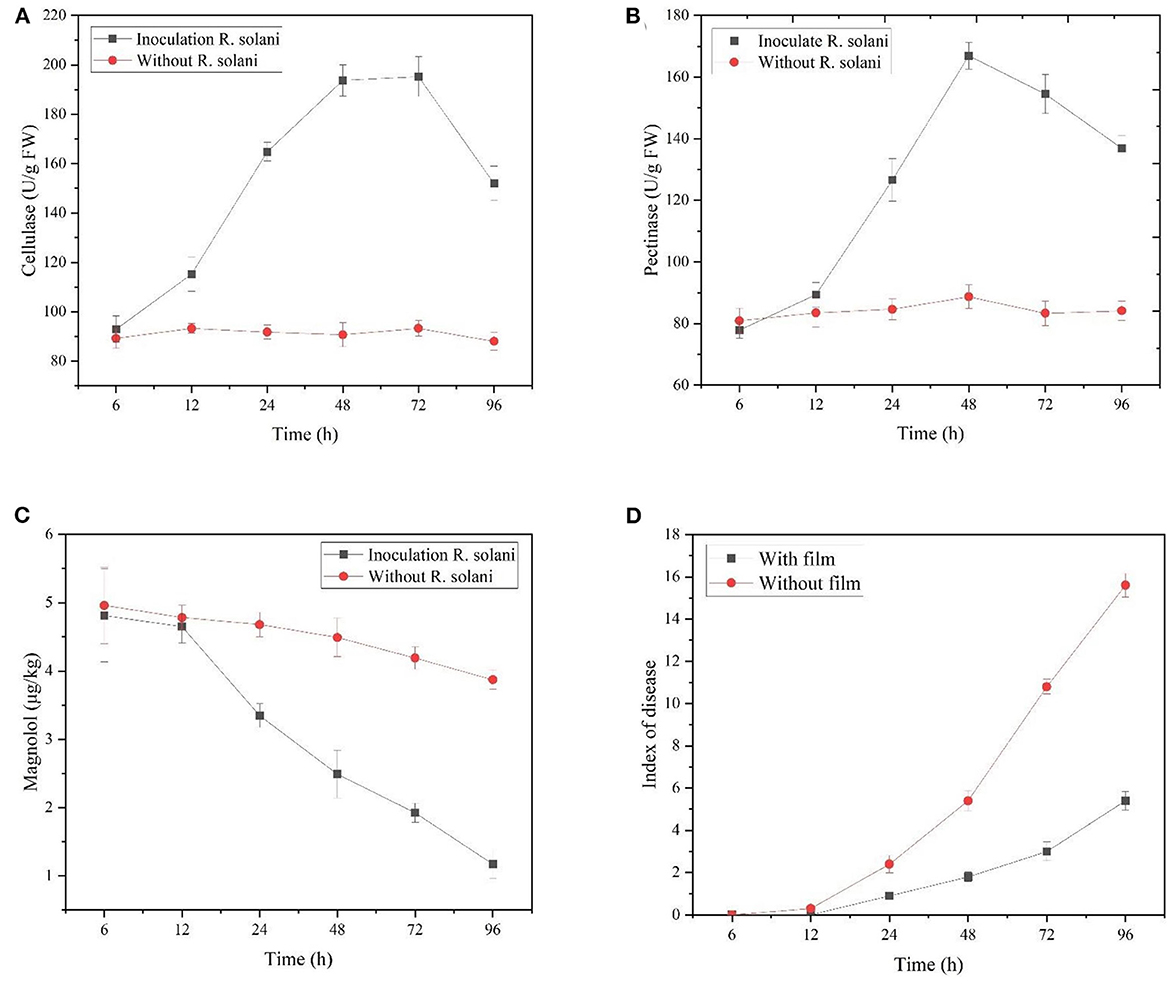
Figure 4. After spraying the Mag@CMCS@CPSG film for 4 h, the rice surface cellulase activities (A), pectinase activities (B), magnolol content (C), and rice sheath blight disease index (D) by inoculating with R. solani.
The disease indices of rice sheath blight disease caused by R. solani inoculated after spraying and without the Mag@CMCS@CPSG film were significantly different after 24 h (Figure 4D). After 96 h, the rice sheath blight disease index reached 15.6 after inoculation with R. solani without the Mag@CMCS@CPSG film, which was significantly higher than 5.4 after inoculation with the film. In addition, the barrier effect of the Mag@CMCS@CPSG film significantly reduced the pathogenic characteristics of rice (Figure 5). These results indicate that the Mag@CMCS@CPSG film can respond to the infection of R. solani, accelerate the release of Mag, inhibit the growth and spread of the pathogen, and reduce the occurrence of disease.
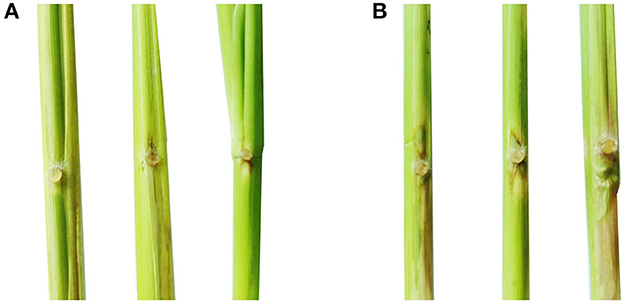
Figure 5. Disease spots on rice stem after 72 h. (A) Inoculated R. solani after spraying the Mag@CMCS@CPSG film for 4 h; (B) Inoculated R. solani without the Mag@CMCS@CPSG film.
By spraying the Mag@CMCS@CPSG film after inoculation with R. solani for 48 h, the Mag content decreased rapidly from 6 to 24 h and decreased slowly after 24 h (Figure 6A). After 96 h, the cumulative release of Mag was 57.44%, and the disease index was 12.3. This may be because the cellulase and pectinase activities decreased after infection with R. solani, decreasing magnolol release. The results showed that application of the Mag@CMCS@CPSG film after the occurrence of rice sheath blight disease decreased the ability of the Mag@CMCS@CPSG film to release Mag in response to the infection of R. solani decrease.
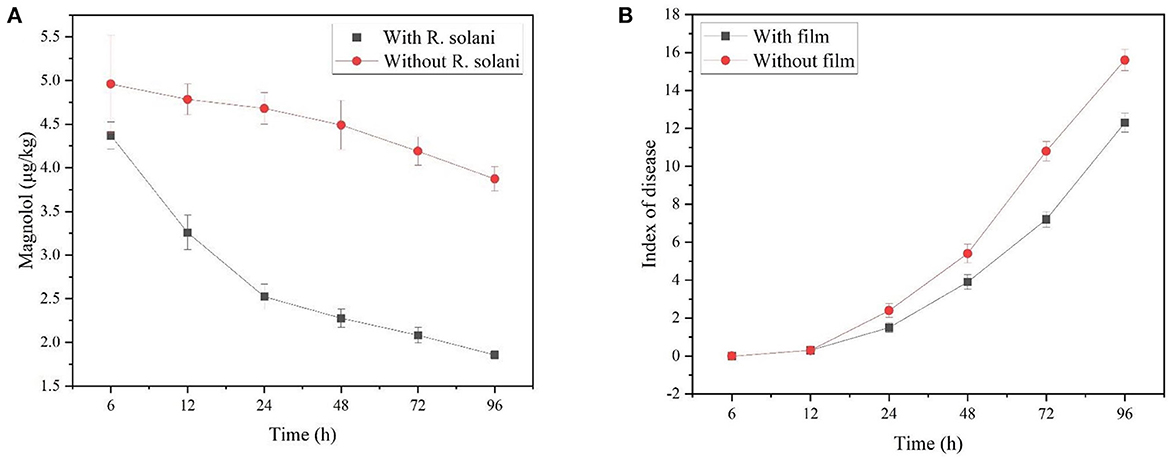
Figure 6. After spraying Mag@CMCS@CPSG film for 4 h, the rice surface magnolol content (A) and rice sheath blight disease index (B) by inoculating with R. solani.
3.4. Field control effect of Mag@CMCS@CPSG film on rice sheath blight disease
We applied the film at the booting and full head stages to determine the field-control effect of the Mag@CMCS@CPSG film before and after the occurrence of rice sheath blight disease. The control effect of the Mag@CMCS@CPSG film at the booting stage on rice sheath blight disease is shown in Table 1. In total, 7 days after the application of Mag@CMCS@CPSG film at 2 g/L, the control effect on rice sheath blight disease was 72.47%, which was not significantly different from that of the jinggangmycin treatment (69.92%) but significantly higher than that of the Mag treatment (18.31%). The control effect of the 2 g/L Mag@CMCS@CPSG film on rice sheath blight disease was 48.86% 28 days after application, which was significantly higher than that of jinggangmycin (35.43%). The results indicated that the Mag@CMCS@CPSG film had a good defensive effect before the occurrence of rice sheath blight disease, and its duration was significantly longer than that of the commercial preparation of 28% Jinggangmycin SP.
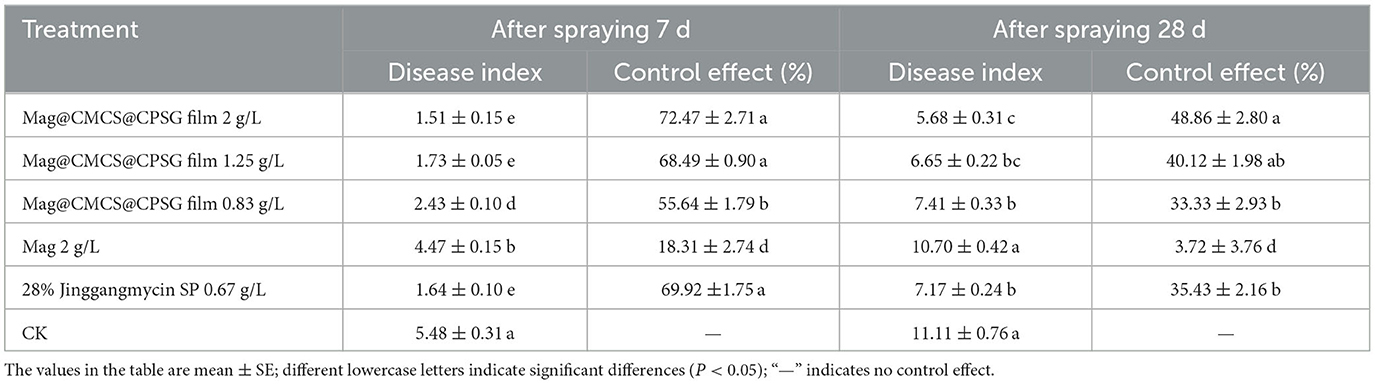
Table 1. Control effect of Mag@CMCS@CPSG film against rice sheath blight disease at the booting stage.
Rice sheath blight disease begins to occur at the full-head stage, and disease spots are observed on the surface of the rice plants. As shown in Table 2, 7 days after application, the control effects of 2, 1.25, and 0.83 g/L Mag@CMCS@CPSG film on rice sheath blight disease were 42.26, 39.80, and 22.61%, respectively, which were significantly lower than 48.68% of those treated with jinggangmycin. In total, 14 days after application, the control effect of the film treatments on rice sheath blight disease was not >35%, which was significantly lower than that of jinggangmycin (43.36%). The results showed that the control effect of the Mag@CMCS@CPSG film decreased after the occurrence of rice sheath blight disease.
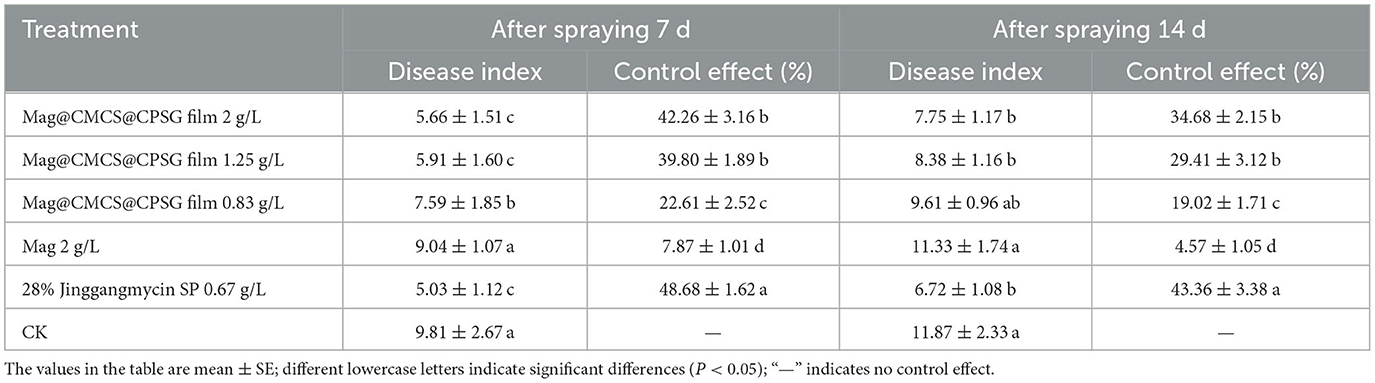
Table 2. Control effect of Mag@CMCS@CPSG film against rice sheath blight disease at the full head stage.
Table 3 shows that there were no significant differences in chlorophyll content, intercellular carbon dioxide concentration, and photosynthetic rate of rice after 7 days of application of 2, 1.25, 0.83, and 2 g/L magnolol, indicating that Mag@CMCS@CPSG film and Mag would not affect the physiological function of rice and were harmless to rice. Therefore, the Mag@CMCS@CPSG film can effectively control rice sheath blight without causing phytotoxicity in rice.
3.5. Effects of Mag@CMCS@CPSG film on rice quality
To further confirm the safety of the Mag@CMCS@CPSG film, the effects of film application at the booting stage on the rice yield and quality were determined. The effects of the Mag@CMCS@CPSG film on rice yield are shown in Table 4. The total panicle of each treatment ranged from 1.96 to 2.21 million/hm2, and there was no significant difference in the total grains and 1,000-grain weight among treatments. However, the seed setting rate of Mag@CMCS@CPSG film and jinggangmycin treatments was significantly higher than other treatments, which were 81.69 and 84.71%, respectively. As a result, the rice yield of these two treatments was also significantly higher than that of other treatments, reaching 9,743.85 and 9,801.30 kg/hm2, respectively. In contrast, compared with the Mag@CMCS@CPSG film treatment, the rice yield of the Mag and CK treatments decreased significantly, and the yield of CK decreased by 34.14%.
The percentages of brown rice, polished rice, chalky grains, chalkiness, and amylose are important indices for evaluating rice quality. The higher the percentage of brown and polished rice, the lower the percentage of chalky grains and chalkiness, and the better the quality. The lower the amylose content, between 10 and 20%, the better the taste (Ding et al., 2023). The effects of different agents applied at the booting stage on rice quality are shown in Table 5. The percentages of brown rice and polished rice of Mag@CMCS@CPSG film and jinggangmycin were significantly higher than those of other agents (78.67% and 79.16%, respectively). In contrast, the percentages of chalky grain, chalkiness, and amylose content were significantly lower than those of other agents (25.41 and 34.22%, 6.69 and 6.52%, 17.26 and 17.12%, respectively). This indicates that the quality of rice treated with Mag@CMCS @CPSG film and jinggangmycin was better than that of rice treated with the others.
To further evaluate the safety of the Mag@CMCS@CPSG film, the Mag content in rice treated with the 2 g/L Mag@CMCS @CPSG film and 2 g/L Mag was determined. The results are presented in Table 6. Mag was not detected in all rice samples and was subjected to different treatments. This indicates that the Mag@CMCS@CPSG film does not form a residue and exists in rice, demonstrating its high safety.
4. Discussion
Plant extracts with antimicrobial activity are considered a class of safe, efficient, and sustainable natural substances and ideal materials to replace chemical pesticides. Extensive studies on plant protection have been demonstrated (Jiang et al., 2021; Khaleil et al., 2021; Uwineza et al., 2022). However, plant extracts are generally difficult to dissolve in water and have poor stability. Therefore, many studies have attempted to solve these two problems by controlling their release (Charles et al., 2022; Yi et al., 2022; Ghosh et al., 2023). The natural Mag used in this study exhibited superior antifungal activity. By preparing and forming the Mag@CMCS@CPSG film, the problems of water insolubility and poor stability of magnolol were solved, and the controlled release of Mag was achieved in response to CWDEs. Compared with previous methods to improve the stability and water solubility of magnolol by preparing drug-carrying particles or synthetic derivatives (Chen et al., 2020; Liu et al., 2022; Qu et al., 2023), the Mag@CMCS@CPSG film achieves accurate delivery of magnolol.
The main components of CWDEs are cellulases and pectinases (Sharafaddin et al., 2019). Therefore, this study used CMC and PT to simulate plant cell wall components to construct a Mag@CMCS@CPSG film in response to CWDEs' control of Mag release. The film was sprayed onto the plant's surface to form a protective layer. When pathogenic fungi are infected, they would first contact the film and mistake the cell wall, inducing the secretion of CWDEs, destroying the integrity of the film, and releasing Mag to control the disease. Although, in nature, many pathogenic fungi complete the infection by releasing cell wall-degrading enzymes, such as Fusarium oxysporum and Alternaria alternata (Liu et al., 2021; Luo et al., 2023), Mag@CMCS in Mag@CMCS@CPSG film can be replaced with corresponding pesticides according to actual diseases to achieve precise control of plant diseases.
Magnolol is the main antifungal component in Mag@CMCS@CPSG film. Currently, it has been reported that it has antimicrobial activity against a variety of pathogenic microorganisms, and some researchers have found that magnolol achieves an antimicrobial effect by destroying plasma membranes (Sun et al., 2015a; Chen et al., 2019). In addition, the film-forming substances are polysaccharide compounds, which can induce plant disease resistance and may have a synergistic effect with magnolol (Borba et al., 2021; Dong et al., 2023). Field experiments proved that the Mag@CMCS@CPSG film had a good controlling effect on rice sheath blight disease. However, the Mag@CMCS@CPSG film prevented R. solani infection by forming a protective layer and could not be transmitted in plants. Therefore, the preventive effect of the Mag@CMCS@CPSG film was better than its therapeutic effect. Jinggangmycin has good endoscopic ability and can be used in rice (Ge et al., 2020); thus, its control effect was better than that of the Mag@CMCS@CPSG film when used after rice sheath blight disease.
Mag is a common medicine used for oral or topical treatment of various diseases, and its safety in humans is high (Sun et al., 2015b; Lin et al., 2021). In addition, CMCS, CMC, PT, SA, and Gl used in the Mag@CMCS@CPSG films are natural products that are often used in food processing (Riahi et al., 2022; Li et al., 2023). This indicates that the Mag@CMCS@CPSG film is a non-toxic or low-toxic agent and an ideal material for the green control of plant diseases.
5. Conclusion
The Mag@CMCS@CPSG film reduced the EC50 value of Mag against R. solani from 7.33 μg/ml to 4.37 μg/ml and effectively increased the toxicity of Mag. The Mag@CMCS@CPSG film released Mag in response to CWDEs and infection by R. solani, with the released amount exceeding 75% after 96 h. Before the occurrence of rice sheath blight disease, the field control effect of the Mag@CMCS@CPSG film on rice sheath blight disease was 72.47% after 7 days, which was not significantly different from that of jinggangmycin; however, the control effect of the Mag@CMCS@CPSG film treatment was 48.86% after 28 days, which was significantly higher than that of the jinggangmycin treatment. These results indicate that the Mag@CMCS@CPSG film had good persistence. The Mag@CMCS@CPSG film reduced the loss of rice yield and quality caused by R. solani infection without any phytotoxicity or residue. Although the safety of the Mag@CMCS@CPSG membrane against other non-target organisms was not determined in this study, its components were all compounds with high safety, indicating that the Mag@CMCS@CPSG film is a precise, efficient, and safe green control agent.
Data availability statement
The data analyzed in this study is subject to the following licenses/restrictions: If original data is required, corresponding author can be solicited via email. Requests to access these datasets should be directed to RL, ryli@gzu.edu.cn.
Author contributions
FM designed the study, participated in all the research, and wrote the initial manuscript. YL provided the field test site and guided the field test. ML directed the design of the enzyme response membrane. YD was involved in the toxicity tests. SW participated in the field test. RL directed the design of the entire study and the writing of the initial manuscript, and provided financial support. All authors contributed to the article and approved the submitted version.
Funding
This study was supported by demonstration base for transfer and transformation of scientific and technological achievements in colleges and universities to serve rural revitalization, the National Natural Science Foundation of China (Nos. 32160657 and 32160658), the Guizhou Provincial Department of Education (No. [2022]060), and the Cultivation Project of Guizhou University (No. [2019]43).
Conflict of interest
The authors declare that the research was conducted in the absence of any commercial or financial relationships that could be construed as a potential conflict of interest.
Publisher's note
All claims expressed in this article are solely those of the authors and do not necessarily represent those of their affiliated organizations, or those of the publisher, the editors and the reviewers. Any product that may be evaluated in this article, or claim that may be made by its manufacturer, is not guaranteed or endorsed by the publisher.
References
Begum, M. F., Rahman, M. A., and Alam, M. F. (2010). Biological control of alternaria fruit rot of chili by trichoderma species under field conditions. Mycobiology 38, 113–117. doi: 10.4489/MYCO.2010.38.2.113
Borba, M. C. D., Velho, A. C., Maia, G. A., Baltenweck, R., Magnin, R. M., Randoux, B., et al. (2021). The algal polysaccharide ulvan induces resistance in wheat against Zymoseptoria tritici without major alteration of leaf metabolome. Front. Plant Sci., 12, 703712. doi: 10.3389/fpls.2021.703712
Charles, A. P. R., Mu, R., Jin, T. Z., Li, D., Pan, Z., Rakshit, S., et al. (2022). Application of yellow mustard mucilage and starch in nanoencapsulation of thymol and carvacrol by emulsion electrospray. Carbohyd. Polym. 298, 120148. doi: 10.1016/j.carbpol.2022.120148
Chen, C. Y., Fang, J. Y., Chen, C. C., Chuang, W. Y., Leu, Y. L., Ueng, S. H., et al. (2020). H. 2-O-Methylmagnolol, a magnolol derivative, suppresses hepatocellular carcinoma progression via inhibiting class I histone deacetylase expression. Front. Oncol. 10,1319. doi: 10.3389/fonc.2020.01319
Chen, J., Xuan, Y., Yi, J., Xiao, G., Yuan, D. P., and Li, D. (2023). Progress in rice sheath blight resistance research. Front. Plant Sci. 14, 1141697. doi: 10.3389/fpls.2023.1141697
Chen, Y. H., Lu, M. H., Guo, D. S., Zhai, Y. Y., Miao, D., Yue, J. Y., et al. (2019). Antifungal effect of magnolol and honokiol from magnolia officinalis on Alternaria alternata causing tobacco brown spot. Molecules 24, 2140. doi: 10.3390/molecules24112140
Ding, C., Xu, C., Lu, B., Zhu, X., Luo, X., He, B., et al. (2023). Comprehensive evaluation of rice qualities under different nitrogen levels in South China. Foods 12, 697. doi: 10.3390/foods12040697
Dong, Y., Liu, J., Chen, Y., Zhu, T., Li, Y., Zhang, C., et al. (2023). Photothermal and natural activity-based synergistic antibacterial effects of Ti3C2Tx MXene-loaded chitosan hydrogel against methicillin-resistant Staphylococcus aureus. Int. J. Biol. Macromol. 240, 124482. doi: 10.1016/j.ijbiomac.2023.124482
Garefalaki, V., Manco, G., and Porzio, E. (2022). Use of biosensors for rapid and sensitive detection of pesticides in food samples for food safety chemical risk assessment. EFSA J. 20, e200922. doi: 10.2903/j.efsa.2022.e200922
Ge, L., Zhou, Z., Sun, K., Huang, B., Stanley, D., and Song, Q. S. (2020). The antibiotic jinggangmycin increases brown planthopper (BPH) fecundity by enhancing rice plant sugar concentrations and BPH insulin-like signaling. Chemosphere 249, 126463. doi: 10.1016/j.chemosphere.2020.126463
Ghosh, V., Ranjha, R., and Gupta, A. K. (2023). Polymeric encapsulation of anti-larval essential oil nanoemulsion for controlled release of bioactive compounds. Inorg. Chem. Commun. 150, 110507. doi: 10.1016/j.inoche.2023.110507
Groth, D. E. (2008). Effects of cultivar resistance and single fungicide application on rice sheath blight, yield, and quality. Crop Prot. 27, 1125–1130. doi: 10.1016/j.cropro.2008.01.010
Han, J., Li, L., Su, M., Heng, W., Wei, Y., Gao, Y., et al. (2021). Deaggregation and crystallization inhibition by small amount of polymer addition for a co-amorphous curcumin-magnolol system. Pharmaceutics 13, 1725. doi: 10.3390/pharmaceutics13101725
Jiang, L. B., Zhao, K. F., Wang, D. J., and Wu, J. C. (2012). Effects of different treatment methods of the fungicide jinggangmycin on reproduction and vitellogenin gene (Nlvg) expression in the brown planthopper Nilaparvata lugens Stål (Hemiptera: Delphacidae). Pestic. Biochem. Phys. 102, 51–55. doi: 10.1016/j.pestbp.2011.10.009
Jiang, S., Zhang, J., Yang, Q., Sun, D., Pu, X., Shen, H., et al. (2021). Antimicrobial activity of natural plant compound carvacrol against soft rot disease agent Dickeya zeae. Curr. Microbiol. 78, 1–11. doi: 10.1007/s00284-021-02609-3
Jiang, X., Lin, H., Lin, M., Chen, Y., Wang, H., Lin, Y., et al. (2018). A novel chitosan formulation treatment induces disease resistance of harvested litchi fruit to Peronophythora litchii in association with ROS metabolism. Food Chem. 266, 299–308. doi: 10.1016/j.foodchem.2018.06.010
Khaleil, M. M., Alnoman, M. M., Elrazik, E. S. A., Zagloul, H., and Khalil, A. M. A. (2021). Essential oil of Foeniculum vulgare Mill. as a green fungicide and defense-inducing agent against fusarium root rot disease in Vicia faba L. Biology 10, 696. doi: 10.3390/biology10080696
Lakshman, D. K., Roberts, D. P., Garrett, W. M., Natarajan, S. S., Darwish, O., Alkharouf, N., et al. (2016). Proteomic investigation of rhizoctonia solani AG 4 identifies secretome and mycelial proteins with roles in plant cell wall degradation and virulence. J. Agric. Food Chem. 64, 3101–3110. doi: 10.1021/acs.jafc.5b05735
Li, J., Duan, Y., Bian, C., Pan, X., Yao, C., Wang, J., et al. (2018). Effects of validamycin in controlling Fusarium head blight caused by Fusarium graminearum: inhibition of DON biosynthesis and induction of host resistance. Pestic. Biochem. Phys. 153, 152–160. doi: 10.1016/j.pestbp.2018.11.012
Li, Y., Hu, Z., Huo, R., and Cui, Z. (2023). Preparation of an indicator film based on pectin, sodium alginate, and xanthan gum containing blueberry anthocyanin extract and its application in blueberry freshness monitoring. Heliyon 9, e14421. doi: 10.1016/j.heliyon.2023.e14421
Lin, Y., Li, Y., Zeng, Y., Tian, B, Qu, X., Yuan, Q., et al. (2021). Pharmacology, toxicity, bioavailability, and formulation of magnolol: an update. Front. Pharmacol. 12, 632767. doi: 10.3389/fphar.2021.632767
Liu, L., Chen, F., Chen, S., Fang, W., Liu, Y., and Guan, Z. (2021). Dual species dynamic transcripts reveal the interaction mechanisms between Chrysanthemum morifolium and Alternaria alternata. BMC Genomics 22, 523. doi: 10.1186/s12864-021-07709-9
Liu, Y., Zhu, T., Li, J., Bao, Y., Cheng, B., Chen, S., et al. (2022). Magnolol hybrid nanofibrous mat with antibacterial, anti-inflammatory, and microvascularized properties for wound treatment. Biomacromolecules. 23, 1124–1137. doi: 10.1021/acs.biomac.1c01430
Luo, J., Zhang, A., Tan, K., Yang, S., Ma, X., Bai, X., et al. (2023). Study on the interaction mechanism between Crocus sativus and Fusarium oxysporum based on dual RNA-seq. Plant Cell Rep. 42, 91–106. doi: 10.1007/s00299-022-02938-y
Mo, F., Hu, X., Ding, Y., Li, R., Long, Y., and Li, M. (2023). Using carboxymethyl cellulose and pectin to construct an enzyme-responsive magnolol-release film against plant pathogenic fungi. Ind. Crop. Prod. 197, 116553. doi: 10.1016/j.indcrop.2023.116553
Mo, F., Hu, X., Ding, Y., Li, R., Long, Y., Wu, X., et al. (2021). Naturally produced magnolol can significantly damage the plasma membrane of Rhizoctonia solani. Pestic. Biochem. Phys. 178, 104942. doi: 10.1016/j.pestbp.2021.104942
Qu, S., Liu, J., Wu, Z., Li, J., Li, P., Wang, G., et al. (2023). Nanocomplexation is a promising strategy to enhance the solubility and anti-Ichthyophthirius multifiliis activity of magnolol. Aquaculture 565, 739105. doi: 10.1016/j.aquaculture.2022.739105
Rao, T. B., Chopperla, R., Prathi, N. B., Balakrishnan, M., Prakasam, V., Laha, G. S., et al. (2020). A comprehensive gene expression profile of pectin degradation enzymes reveals the molecular events during cell wall degradation and pathogenesis of rice sheath blight pathogen Rhizoctonia solani AG1-IA. J. Fungi 6, 71. doi: 10.3390/jof6020071
Ravshanov, B., Namozov, F., Kurbonov, A., Abdalova, G., Karimov, A., Khaitov, B., et al. (2023). Integrative effect of nitrogen fertilization and biotreatments on rice growth and yield potential under open-field agriculture. J. Plant Nutr. 46, 1701–1711. doi: 10.1080/01904167.2022.2097920
Riahi, Z., Rhim, J. W., Bagheri, R., Pircheraghi, G., and Lotfali, E. (2022). Carboxymethyl cellulose-based functional film integrated with chitosan-based carbon quantum dots for active food packaging applications. Prog. Org. Coat. 166, 106794. doi: 10.1016/j.porgcoat.2022.106794
Sharafaddin, A. H., Hamad, Y. K., El_Komy, M. H., Ibrahim, Y. E., Widyawan, A., Molan, Y. Y., et al. (2019). Cell wall degrading enzymes and their impact on Fusarium proliferatum pathogenicity. Eur. J. Plant Pathol. 155, 871–880. doi: 10.1007/s10658-019-01818-8
Shen, M., Cai, C., Song, L., Qiu, J., Ma, C., Wang, D., et al. (2023). Elevated CO2 and temperature under future climate change increase severity of rice sheath blight. Front. Plant Sci. 14, 1115614. doi: 10.3389/fpls.2023.1115614
Sun, J., Zhang, X., Zheng, J., Liu, G., and Chen, L. (2023). Importance of cell wall permeability and cell wall degrading enzymes during infection of Botrytis cinerea in Hazelnut. Forests 14, 565. doi: 10.3390/f14030565
Sun, L., Liao, K., and Wang, D. (2015b). Effects of magnolol and honokiol on adhesion, yeast-hyphal transition, and formation of biofilm by Candida albicans. PloS One 10, e0117695. doi: 10.1371/journal.pone.0117695
Sun, L. M., Liao, K., Liang, S., Yu, P. H., and Wang, D. Y. (2015a). Synergistic activity of magnolol with azoles and its possible antifungal mechanism against Candida albicans. J. Appl. Microbiol. 118, 826–838. doi: 10.1111/jam.12737
Tan, W., Miao, J., Xu, B., Zhou, C., Wang, Y., Gu, X., et al. (2023). Rapid production of novel beneficial alleles for improving rice appearance quality by targeting a regulatory element of SLG7. Plant Biotechnol. J. 14041. doi: 10.1111/pbi.14041
Tang, L., Zhu, Z., Xie, M., Cao, L., Yu, X. L., Zhang, R., et al. (2019). Effects of β-cyclodextrin and hydroxypropyl-β-cyclodextrin inclusions on the degradation of magnolol by intestinal bacteria. AAPS PharmSciTech 20, 244. doi: 10.1208/s12249-019-1397-9
Usach, I., Alaimo, A., Fernández, J., Ambrosini, A., Mocini, S., Ochiuz, L., et al. (2021). Magnolol and honokiol: two natural compounds with similar chemical structure but different physicochemical and stability properties. Pharmaceutics 13, 224. doi: 10.3390/pharmaceutics13020224
Uwineza, P. A., Urbaniak, M., Bryła, M., Stepie,ń, Ł, Modrzewska, M., and Waśkiewicz, A. (2022). In Vitro Effects of lemon balm extracts in reducing the growth and mycotoxins biosynthesis of Fusarium culmorum and F. proliferatum. Toxins 14, 355. doi: 10.3390/toxins14050355
Wang, L., Wang, D., Yuan, S., Feng, X., and Wang, M. (2020). Transcriptomic insights into the antifungal effects of magnolol on the growth and mycotoxin production of Alternaria alternata. Toxins 12, 665. doi: 10.3390/toxins12100665
Wang, Y., Qin, J., Lu, Q., Tian, J., Ke, T., Guo, M., et al. (2023). Residue detection and correlation analysis of multiple neonicotinoid insecticides and their metabolites in edible herbs. Food Chem. X 17,100603. doi: 10.1016/j.fochx.2023.100603
Yi, F., Liu, Y., Su, C., and Xue, Z. (2022). Research progress on the encapsulation and sustained controlled-release of essential oils. J. Food Process. Pres. 46, 17241. doi: 10.1111/jfpp.17241
Yousefi, M. H., Abbasi, E., Hadidi, M., Hashemi, S., Ghadimi, A. H., Yousefinejad, S., et al. (2023). Simultaneous analysis of mycotoxins, potentially toxic elements, and pesticides in rice: a health risk assessment study. Toxins 15, 102. doi: 10.3390/toxins15020102
Zhao, Y., Wang, Q., Wu, X., Jiang, M., Jin, H., Tao, K., et al. (2021). Unraveling the polypharmacology of a natural antifungal product, eugenol, against Rhizoctonia solani. Pest Manag. Sci. 77, 3469–3483. doi: 10.1002/ps.6400
Zheng, T., Liu, L., Nie, Q., Hsiang, T., Sun, Z., and Zhou, Y. (2021). Isolation, identification and biocontrol mechanisms of endophytic bacterium D61-A from Fraxinus hupehensis against Rhizoctonia solani. Biol. Control 158, 104621. doi: 10.1016/j.biocontrol.2021.104621
Zhou, J., Hu, Y., Chen, P., and Zhang, H. (2020). Preparation of restricted access monolithic tip via unidirectional freezing and atom transfer radical polymerization for directly extracting magnolol and honokiol from rat plasma followed by liquid chromatography analysis. J. Chromatogr. A 1625, 461238. doi: 10.1016/j.chroma.2020.461238
Keywords: magnolol, Rhizoctonia solani, cell wall degrading enzymes, controlled release, field control effect
Citation: Mo F, Long Y, Li M, Ding Y, Wu S and Li R (2023) Effect of enzyme-response Mag@CMCS@CPSG film on rice sheath blight disease (caused by Rhizoctonia solani) control and rice quality. Front. Sustain. Food Syst. 7:1204698. doi: 10.3389/fsufs.2023.1204698
Received: 12 April 2023; Accepted: 18 May 2023;
Published: 13 June 2023.
Edited by:
Yishan Yang, Agricultural Research Service (USDA), United StatesReviewed by:
Yong Liu, Hunan Academy of Agricultural Sciences (CAAS), ChinaZhiqiang Kong, Chinese Academy of Agricultural Sciences (CAAS), China
Copyright © 2023 Mo, Long, Li, Ding, Wu and Li. This is an open-access article distributed under the terms of the Creative Commons Attribution License (CC BY). The use, distribution or reproduction in other forums is permitted, provided the original author(s) and the copyright owner(s) are credited and that the original publication in this journal is cited, in accordance with accepted academic practice. No use, distribution or reproduction is permitted which does not comply with these terms.
*Correspondence: Rongyu Li, ryli@gzu.edu.cn